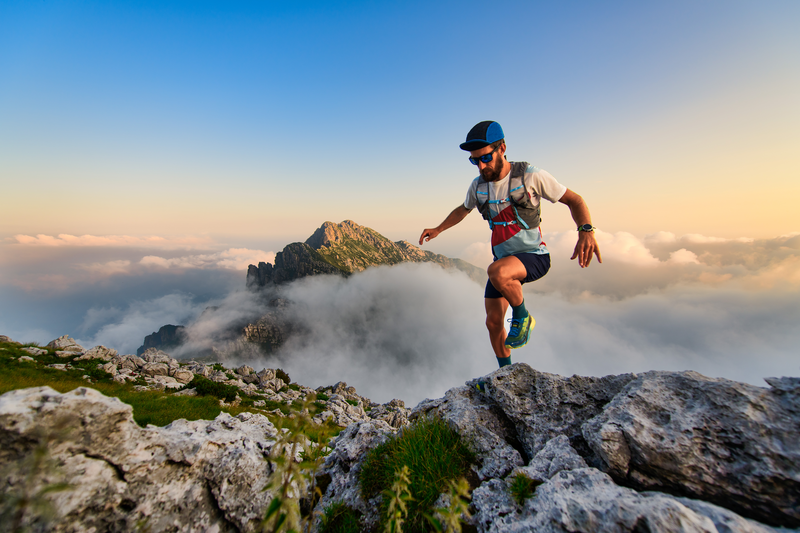
94% of researchers rate our articles as excellent or good
Learn more about the work of our research integrity team to safeguard the quality of each article we publish.
Find out more
ORIGINAL RESEARCH article
Front. Ecol. Evol. , 19 December 2023
Sec. Conservation and Restoration Ecology
Volume 11 - 2023 | https://doi.org/10.3389/fevo.2023.1280157
This article is part of the Research Topic Biodiversity Conservation and Sustainable Development of Protected Areas View all 12 articles
Shrub encroachment threatens grassland ecosystem services including herbaceous plant diversity, productivity, and overall grazing capacity. Hence, various shrub control methods including mechanical removal and herbicides are applied to restore herbaceous vegetation. However, the knowledge regarding the outcomes of integrated shrub management on herbaceous vegetation is limited. We investigated herbaceous vegetation responses after 7 years following the integration of shrub control and herbivory at Telperion Nature Reserve, Mpumalanga Province, South Africa. In a split-plot experiment using four enclosures and non-enclosures as main plots, we randomly assigned each of the following treatments in four 4 × 4-m subplots in each main plot: 1) foliar metsulfuron-methyl herbicide, 2) 50% mechanical shrub removal, 3) 100% removal, and 4) no removal (untreated controls). A one-off Seriphium plumosum removal and the application of species-specific herbicide were conducted in 2015. Shrub control by herbivory interaction was significant (p < 0.05) for herbaceous canopy cover, basal cover, density, and species richness. The effects of herbivory were significant (p < 0.05) in the 50% removal treatment, increasing herbaceous plant diversity, density, and richness to the same level as 100% removal and herbicide treatments. In enclosures, however, the 50% shrub removal treatment attained relatively low herbaceous plant cover, density, species diversity, and richness compared to the herbicide treatment. Overall, this study revealed that herbivory and shrub control interact to facilitate herbaceous vegetation restoration, with 50% shrub removal by herbivory combination showing potential for management of S. plumosum-encroached grasslands.
Shrub encroachment defined as the increase in shrub cover, density, and biomass disrupts ecosystem processes and services and changes the structure and functioning of grasslands (Zhou et al., 2019; Mochi et al., 2022; Wieczorkowski and Lehmann, 2022). This phenomenon is ascribed to complex interactions of overgrazing and inappropriate fire regimes together with global drivers including elevated atmospheric CO2 and variable rainfall regimes (Stevens et al., 2016). Overgrazing reduces herbaceous cover, abundance, and biomass, giving shrubs a competitive advantage and a recruitment pulse, especially when the rainfall is above average (Erfanzadeh et al., 2016; Weber-Grullon et al., 2020). Overgrazing not only reduces understory vegetation production and cover but also changes the composition from grass to unpalatable forb-dominated herbaceous communities (Erfanzadeh et al., 2016). Even in water-limited ecosystems, high atmospheric CO2 by increasing water use efficiency and fertilization facilitates an increase in shrub cover and biomass (Stevens et al., 2016; Mndela et al., 2022a). Shrub proliferation is more prevalent in infrequently burned rangelands with low browsing ungulate densities (Dreber et al., 2019), as this promotes an increase in shrub cover and root biomass, thereby intensifying shrub–grass competition for light and below-ground resources (Van Zyl and Avenant, 2018).
Shrub encroachment causes biodiversity loss and a decline in rangeland and livestock productivity, adversely affecting the local economy (Anadon et al., 2014; Wieczorkowski and Lehmann, 2022) and pastoralist’s livelihoods (Liao et al., 2018; Hare et al., 2021). In South Africa, a dwarf shrub, Seriphium plumosum (L.) Thunb. (Asteraceae) previously known as Stoebe vulgaris, has encroached vastly in grassland and savanna biomes (Urban et al., 2021). The encroachment of this shrub was first noticed early 1920s in Southern Africa, after which its abundance increased abruptly (Clark et al., 2020). An increase in S. plumosum encroachment characterizes degradation (Wepener, 2007) and is partly driven by the high production of wind-dispersed seeds together with high recruitment success in nutrient-poor soils (Snyman, 2012).
S. plumosum both competes with understory plants and limits germination and establishment via allelopathy (Snyman, 2010). Nonetheless, the legacy effects of the S. plumosum allelopathy following shrub control are transient, with its autotoxicity persisting only for 12 to 16 weeks in the soil (Van Zyl and Avenant, 2018). This presents a great opportunity for herbaceous vegetation restoration through shrub control. Various shrub control methods have been proposed including chemical, burning, and mechanical measures (Avenant, 2015; Pule et al., 2023). These control measures have different efficacies (Marquart et al., 2022) and impose differential effects on the herbaceous plant diversity, cover, and production (Nkosi et al., 2018; Clark et al., 2020; Graham et al., 2020). Thus, herbaceous vegetation responses following shrub control need to be ascertained from short- to long-term temporal scales.
A plethora of research has been conducted on S. plumosum control (Snyman, 2012; Clark et al., 2020; Marquart et al., 2022); however, none of these studies assessed long-term vegetation responses above 3 years. This limits our understanding of the long-term outcomes of shrub control on understory vegetation. Monitoring long-term vegetation responses to shrub control is important to devise appropriate vegetation restoration management (Mndela et al., 2022b). In most studies, e.g., Marquart et al. (2022), shrub control has never been integrated with herbivory to mimic the ideal scenarios where ungulates are an integral part of the ecosystem. This questions the reliability and practicality of the results obtained from such studies for biodiversity conservation and vegetation restoration. Ungulates through browsing and trampling open shrub canopies for herbaceous plant recruitment (O’Connor et al., 2020). Furthermore, ungulates play a crucial role in reducing shrub seedling recruitment (Hare et al., 2021) and resprouting of partially killed shrubs, thereby minimizing re-encroachment (Venter et al., 2017).
A comprehensive study that considers holistic responses of herbaceous vegetation to integrated management of S. plumosum is crucial (Nkosi et al., 2018). This study, therefore, investigates herbaceous plant responses after 7 years following the integration of chemical and mechanical shrub control with herbivory. The objective of the study was to assess the long-term impacts of shrub control on herbaceous vegetation and determine how wild ungulates modulate herbaceous vegetation responses to shrub control.
The study was conducted at Telperion Nature Reserve (TNR) close to the town of Bronkhorstspruit in the Mpumalanga Province of South Africa (Figure 1). The reserve is 11,000 hectares in size and is 1,350 m above sea level. The reserve is used mainly for the conservation of populations of wild ungulates including Plains zebra, Blue and black wildebeest, Red hartebeest, Eland, Springbok, Kudu, Gemsbok, Waterbuck, and Blesbok (Table 1; Roux, 2017). The stocking rate of the wild ungulates at TNR is 0.20 large stock unit/ha (MacFadyen, 2014). The mean annual rainfall at TNR ranges from 650 to 700 mm per year, with the highest rain received during the summer months around January (Graham et al., 2020). The average minimum and maximum temperatures are 7°C and 27°C, respectively. The reserve comprises grasslands, wetlands, mosaics of woodlands on the ridges, forest in the valleys, and the free-flowing Wilge River, which supports wild ungulates through forage provision, drinking, and insulation against heat. The main vegetation type at TNR is Rand Highveld Mesic Grassland, with the largest parts being classified as Eragrostis curvula–S. plumosum midslope plateau grassland (Brown et al., 2022). The common grass species are Themeda triandra, Elionurus muticus, and Tristachya leucothrix (Mucina et al., 2006). S. plumosum encroaches heavily with the highest density of 9,500 plants/ha (Graham et al., 2020) and a mean canopy cover of 50.0% ± 8.9% where ungulates are present to 83.8% ± 6.3% where ungulates are absent (Figure 2). The shrub cover was on average 80% across the plots before shrub control. In 2022, the shrub cover in 50% removal treatment was 25% and 68% in non-enclosures and enclosures, respectively, and these were 2- and 1.2-fold lower than shrub cover in no removal treatments (Figure 2; Supplementary Table 1). The soil types characterizing the reserve are sandy soils derived from Quartzite of the Witwatersrand Supergroup, Pretoria group, and the Selons River formation of the Rooiberg group (Mucina et al., 2006).
Four spatially distributed sites were located at TNR. At each site, a 50 × 25-m area was demarcated and divided into two paired 25 × 25-m plots, with one plot fenced to preclude wild ungulates (enclosure) and the other plot left open to wild ungulates (non-enclosure; Table 2). A 1.5-m-high wire mesh was erected to prevent access of medium- to large-sized ungulates to enclosures. Thus, the enclosures were only accessible to small mammals including rodents and lagomorphs. The wild ungulates kept in the reserve and their feeding regime (Mentis, 1981) and diet composition (Venter et al., 2017; Cerling et al., 2003) are presented in Table 1. Five ungulates are grazers, two are browsers, and four are intermediate feeders (Table 1). More than 80% of the diet of grazers is C4 grasses, whereas 82%–96% diet of browsers consists of woody plant twigs, pods, and leaves (Table 1).
Table 2 The study design indicating shrub control treatments within enclosures and non-enclosures across three experimental sites.
Following a split-plot design, each enclosure or non-enclosure was subdivided into sixteen 4 × 4-m subplots, for which each of the following shrub control treatments was assigned randomly in four subplots: foliar herbicide, 50% shrub removal, 100% removal, and no removal (untreated controls) (Nkosi et al., 2018). The subplots were interspaced by 1.5 m to avoid spillover effects of shrub control treatment to another. A 2-m wide buffer was maintained around the main plot to account for the edge effect. Collectively, enclosures and non-enclosures are loosely referred to as herbivory treatment in this study.
The mechanical treatment, which included 50% and 100% shrub removal, was conducted by cutting and grubbing the above-ground stems and rhizomes of S. plumosum stems (Nkosi et al., 2018). Since the shrub is rhizomatous, cutting the above-ground stem only would lead to resprouting, which, therefore, justifies pulling out rhizomes from the soil by grubbing in 50% and 100% removal treatments (Snyman, 2012). For 50% shrub removal, half of the S. plumosum individuals in each subplot were removed, whereas all S. plumosum plants were removed for the 100% removal treatment. For the herbicide treatment, metsulfuron-methyl herbicide was applied at a concentration of 4 g/L of water by spraying the photosynthetic foliage of S. plumosum using knapsack sprayers. Metsulfuron-methyl was selected owing to its known efficacy in shrub control (Marquart et al., 2022) and its high selectivity against non-target plants when applied to the foliage (Du Toit, 2012). This is a systematic herbicide that is translocated to plant parts below ground (Spencer, 2012), making it the most relevant to control rhizomatous shrub species. To avoid the pre-emergent effects of the herbicide on the soil-stored seeds, shrub control treatments were applied during the 2015 growing season long after seedling recruitment (Nkosi et al., 2018).
Vegetation was assessed in October at the end of the spring season of 2022 after 7 years of the establishment of shrub control and herbivory experiment. Three 1-m2 quadrats were sampled in two opposite corners and at the center of each subplot, giving a total of 384 quadrats. All plants encountered in each quadrat were identified to species level using the nomenclature by Van Oudtshoorn (1999) for grasses and Van der Walt (2009) for forbs, legumes, shrubs, and sedges. The minimum and maximum basal diameters were measured at the 3-cm stubble height of each plant encountered in each quadrat using a standard ruler. For creeping plants, each above-ground shoot was identified as an independent plant when the distance between shoots was >10 cm (Davies et al., 2012). All individuals encountered in each 1-m2 quadrat were used to estimate plant density, whereas the total number of different species per quadrat was used as the estimate of species richness. Plant density was divided into graminoids (grasses and sedges) and non-graminoids (forbs and rushes). The herbaceous cover was estimated in each subplot as a collective proportion of graminoids, forbs, and rushes. The plant cover was estimated visually in the whole subplot by two researchers, and the estimates were later averaged to obtain a single representative cover. The basal cover was calculated as the total ellipsoid area of all basal crowns of plants per quadrat and expressed as a proportion of the area of a quadrat. The ellipsoid area was calculated from the minimum and maximum basal diameters.
All analyses were conducted in JASP statistical software. Two-way analysis of variance (ANOVA) was performed in a split-plot design, with enclosures and/or non-enclosures (n = 2) and shrub control treatments (n = 4) included as the main plots and subplots, respectively. Generalized linear mixed-effects models (GLMEMs) were fitted to determine the fixed effects of herbivory, shrub control treatments, and their interactions on herbaceous cover, basal cover, species richness, diversity, and plant density. Experimental sites were added as random factors in the GLMEMs. For species richness and plant density, plant functional groups (graminoids and non-graminoids) were further included as the additional independent variable to assess three-way interactions between herbivory, shrub control, and plant functional groups. The species diversity was calculated using the Shannon–Wiener diversity index (H′) according to Magurran (2004), as follows (Equation 1):
where S is the number of species in each subplot and Pi is the relative abundance of species i.
When the interactions were significant at α = 0.05, a Tukey’s post-hoc test was used to conduct multiple mean comparisons.
Herbivory (F1, 128 = 31.24, p < 0.001), shrub control (F3, 128 = 21.11, p < 0.001), and herbivory by shrub control interaction (F3, 128 = 2.83, p = 0.050) had significant effects on herbaceous canopy cover (Table 3). Non-enclosures had 3-fold and 4-fold higher herbaceous canopy cover compared to enclosures for 50% removal (t = 4.86, p < 0.001) and no removal treatments (t = 3.62, p = 0.018), respectively (Figure 3A). In non-enclosures, the shrub control treatments had similar herbaceous canopy cover, with herbicide treatment exhibiting higher herbaceous cover (t = 3.20, p = 0.050) than no removal treatment (Figure 3A). In enclosures, 100% removal and herbicide treatments attained similar herbaceous canopy cover (t = 0.07, p = 1.000), which was 3 to 6-fold higher than in the 50% removal and no removal treatments (Figure 3A).
Table 3 The F-test and p-values of the herbivory treatment, shrub control, plant functional groups (PFG), and their interactions on herbaceous vegetation indicators.
Figure 3 Herbaceous canopy cover (A) and basal cover (B) across different shrub control treatments in enclosures and non-enclosures. Bold dotted and undotted lines inside the box denote the mean and median, respectively, and the lower and upper limits of the box denote 25th and 75th quartiles, respectively. The lower and upper whiskers are minimum and maximum values, respectively.
The herbivory (F1, 128 = 14.42, p < 0.001), shrub control (F3, 128 = 10.53, p < 0.001), and herbivory by shrub control interaction (F3, 128 = 4.49, p = 0.009) had a significant effect on basal cover (Table 3). Basal cover (BC) was 4-fold and 2-fold higher in non-enclosures relative to enclosures for 50% removal (t = 3.60, p = 0.019) and 100% removal treatments (t = 3.36, p = 0.034), respectively (Figure 3B). Herbicide treatment had a significant 3- to 5-fold higher basal cover compared to other shrub control treatments in enclosures (p < 0.001; Figure 3B).
Herbivory (F1, 128 = 18.51, p < 0.001) and shrub control (F3, 128 = 4.39, p = 0.009), but not their interaction (F3, 128 = 1.26, p = 0.301), had a significant effect on species diversity (Table 3). The 50% removal and no removal treatments attained a significantly higher diversity in non-enclosures than enclosures (Figure 4A). The 100% removal and herbicide treatments had comparable diversity in enclosures and non-enclosures (Figure 4A). Generally, shrub control treatments exhibited a similar diversity of 1.70 (±0.24) on average in non-enclosures, whereas herbicide treatment had a significantly higher diversity (p < 0.05) of 1.60 (±0.17) compared to 50% removal (0.76±0.17) and no removal treatments (0.63±0.17) in enclosures (Figure 4A).
Figure 4 Species diversity (A) and total species richness (B) across different shrub control treatments in enclosures and non-enclosures. Bold dotted and undotted lines inside the box denote the mean and median, respectively, and the lower and upper limits of the box denote 25th and 75th quartiles, respectively. The lower and upper whiskers are minimum and maximum values, respectively.
Species richness was significantly affected by herbivory (F1, 128 = 19.08, p < 0.001), shrub control (F3, 128 = 7.15, p < 0.001), and their interaction (F3, 128 = 3.29, p = 0.031; Table 3). Species richness was similar (p > 0.05) between non-enclosures and enclosures for all shrub control treatments except 50% removal, which had significant (t = 4.54, p = 0.001) 3-fold more species in non-enclosures than in enclosures (Figure 4B). On average, shrub control treatments had eight species in non-enclosures, which were 3-fold more than species recorded in 50% removal and no removal treatments in enclosures (Figure 4B).
A three-way interaction between herbivory, shrub control, and plant functional groups (PFGs) was not significant (Table 3). Shrub control by PFG (F3, 128 = 3.24, p = 0.028) and herbivory by PFG (F1, 128 = 13.34, p < 0.001) interactions were significant for species richness (Table 3). For all shrub control treatments, the species richness of graminoids was significantly higher than that of non-graminoids (p < 0.01; Figure 5A). Graminoid richness in 100% removal and herbicide treatments was similar but significantly higher compared to that in 50% removal and no removal treatments (Figure 5A). There were more graminoid species in non-enclosures than enclosures (p < 0.001) and even three times more than non-graminoids in both enclosures and non-enclosures (Figure 5B).
Figure 5 Species richness (A) and plant density (C) of different plant functional groups across shrub control treatments and between enclosures (B) and non-enclosures (D). The bars indicate the mean (), and the error bars indicate the standard error.
The herbivory (F1, 128 = 22.86, p < 0.001), shrub control (F3, 128 = 4.51, p = 0.008), and their interaction (F3, 128 = 3.59, p = 0.022) had a significant effect on the total plant density (Table 3). The plant density was significantly higher (t = 4.39, p = 0.002) in non-enclosures relative to enclosures for 50% removal (Figure 6). All shrub control treatments had similar plant densities in non-enclosures, but these densities were 4-fold higher than for 50% removal and no removal treatments in enclosures (Figure 6).
Figure 6 Plant density across different shrub control treatments in enclosures and non-enclosures. Bold dotted and undotted lines inside the box denote the mean and median, respectively, and the lower and upper limits of the box denote 25th and 75th quartiles, respectively. The lower and upper whiskers are minimum and maximum values, respectively.
A three-way interaction of the herbivory, shrub control, and PFGs was not significant for plant density (Table 3). Nonetheless, shrub control by PFG (F3, 128 = 2.85, p = 0.044) and herbivory by PFG interactions (F1, 128 = 14.16, p < 0.001) significantly affected plant density (Table 3). Graminoids had higher densities than non-graminoids in 100% removal (t = 2.95, p = 0.049) and herbicide treatment (t = 2.95, p < 0.001; Figure 5C). When graminoids were compared across shrub control treatments, herbicide treatment attained a 2-fold higher density than the 50% removal (t = −4.10, p = 0.003) and no removal treatment (t = 4.14, p = 0.002; Figure 5C). However, graminoid density was nearly 2-fold more in non-enclosures than enclosures and 7-fold more in non-graminoids in both enclosures and non-enclosures (Figure 5D).
Our results revealed that herbaceous canopy cover and basal cover responses depended largely on the interactive effects of herbivory and shrub control, indicating that herbivory had additive effects. These effects were more apparent in the 50% shrub removal and no removal treatments (Figure 3), largely due to ungulates, which reduced shrub cover further in non-enclosures (Figure 2; Supplementary Table 1). For 50% removal treatment particularly, wild ungulates reduced shrub cover to 25%, which is below a 40% encroachment threshold identified by Roques et al. (2001) for encroached rangelands of Southern Africa (Figure 2). Generally, if shrub cover increases above 40%, herbaceous cover and density decline due to competition for light and below-ground resources in the semi-arid savannas of Southern Africa (Roques et al., 2001). Thus, the shrub–grass competition is expected to be minimal in 50% removal treatment in non-enclosures, given that shrub cover was nearly 2-fold less than the threshold. These responses were due to wild ungulates in non-enclosures that opened shrub cover, thereby increasing herbaceous plant cover, basal cover, and plant density in the 50% removal to similar levels as the 100% removal and herbicide treatments. This result is attributable to browsing and trampling by wild ungulates, which break shrub twigs and open shrub cover further, subsequently enhancing herbaceous plant recruitment and establishment (O’Connor et al., 2020). This was affirmed by plant density response trends, which resembled herbaceous cover responses (Figure 6), with densities responding more positively in non-enclosures that received 50% removal relative to 100% removal and herbicide treatments. These results signified that the integration of wild ungulates and shrub control facilitated plant colonization in non-enclosures, probably by creating a microclimate favorable for plant regeneration and recruitment.
A low herbaceous plant cover in enclosures for 50% removal treatment was probably due to shrub cover recovery due to the absence of ungulates to control resprouting. S. plumosum exerts serious competitive effects (Snyman, 2012; Marquart et al., 2022), with shading from shrub canopy reducing leaf production, leading to low herbaceous plant cover (Mndela et al., 2022c). In contrast to previous studies (e.g., Stokely et al., 2020), we found higher plant cover in herbicide treatment regardless of the herbivory treatment. Our findings concur with Marquart et al. (2022), who recorded higher herbaceous plant cover in plots where S. plumosum was treated with metsulfuron-methyl. This herbicide is highly selective against non-target herbaceous plants (Du Toit, 2012) and generally achieves a 100% mortality of S. plumosum (Marquart et al., 2022), though a minor shrub recovery of 10% and 16% cover occurred in non-enclosures and enclosures, respectively, in this study (Figure 2; Supplementary Table 1). Since metsulfuron-methyl was applied during summer long after several rains, seedling recruitment and establishment were not interrupted; hence, plant density and cover were the highest in shrub-cleared treatments.
Herbaceous cover responses to herbicide and 100% removal treatment were similar (Figure 3), and this was not surprising, given that shrub cover recovery was minimal after 7 years in these treatments (Figure 2; Supplementary Table 1). Hence, graminoid densities were the highest in the herbicide and 100% removal treatments compared to 50% removal and no removal treatments (Figure 5). This finding indicates that graminoids, largely grasses, are more vulnerable to S. plumosum encroachment and that a reduction in shrub cover created habitat conditions favorable for grass colonization. Generally, a reduction in shrub cover triggers an increase in seed production by graminoids, contributing greatly to herbaceous plant recruitment (Bakker et al., 2014; Mndela et al., 2020). The increase in graminoid densities is a result of increased light availability to the understory layer and a release from shrub competition for soil nutrients and moisture (Haussman et al., 2016). This was further underpinned by the interaction between plant functional groups and herbivory treatment, with graminoid density responding more positively to wild ungulates in non-enclosures where shrub cover was low for all treatments. A reduction in shrub cover increases the decomposition and N positively of organic material due to increased temperatures and microbial activity (Ward et al., 2015). This, therefore, increases organic matter and nutrient availability, thereby creating safe sites for plant recruitment and establishment. Since the clearing of shrubs allows the dispersal of seeds from nearby areas into cleared sites, dispersed seeds together with those produced by local vegetation colonize vigorously in nutrient-rich patches following shrub control (Mndela et al., 2022b; Mndela et al., 2022c).
Furthermore, we found that species diversity responded similarly in enclosures and non-enclosures, more so for 100% removal and herbicide treatments (Figure 4), highlighting that herbivory had a marginal effect on species diversity in these treatments. As a result, for both 100% removal and herbicide treatments, diversity was not different from that of 50% removal in non-enclosures. Generally, habitat heterogeneity in 100% removal and herbicide treatments is low due to the removal of almost all shrubs (Nkosi et al., 2018); hence, higher species richness did not translate to higher diversity in these treatments than in the 50% removal treatment. This is ascribed to the dominance of a few species, which led to these few species homogenizing vegetation in the 100% removal and herbicide treatments. Certain herbaceous species adapted to high light conditions tend to monopolize space, exploit resources, and eliminate late colonizers following shrub control (Mndela et al., 2022a).
This study was designed to provide a mechanistic understanding of how integration of shrub control and herbivory affected herbaceous vegetation after 7 years of a one-off shrub management event. The results revealed that herbaceous vegetation responses with regard to plant cover, density, and richness depend on the interactions between herbivory and shrub control. Herbivory effects were more apparent in the 50% shrub removal treatment signifying that wild ungulates had additive effects on the herbaceous vegetation responses. This suggests that ungulates should be considered an integral part of the management plan for S. plumosum encroachment in mesic grasslands. Although the 50% removal treatment had lower graminoid richness than the 100% removal and herbicide treatments, species diversity together with herbaceous plant cover and density remained comparable between the former and latter two treatments. Overall, our results suggest that apart from herbicide treatment, the integration of 50% removal and herbivory holds great promise for the restoration of S. plumosum-encroached mesic grasslands. The use of 50% shrub removal combined with wild ungulates is not only ecologically significant but also economically viable relative to 100% shrub removal. The results of this study are a basis for the management and conservation of herbaceous vegetation diversity and productivity for sustainable wildlife production.
The raw data supporting the conclusions of this article will be made available by the authors, without undue reservation.
The manuscript presents research on animals that do not require ethical approval for their study.
MM: Conceptualization, Data curation, Formal analysis, Investigation, Methodology, Software, Visualization, Writing – original draft, Writing – review & editing. AB: Conceptualization, Writing – review & editing. LB: Conceptualization, Investigation, Resources, Supervision, Validation, Visualization, Writing – review & editing.
The author(s) declare that no financial support was received for the research, authorship, and/or publication of this article.
Mr. MK Sithole is appreciated for his assistance during field data collection. We further extend a hand of gratitude to the Telperion Nature Reserve owners and management for allowing us to conduct our research on their property. The Applied Behavioural Ecology and Ecosystem Research Unit (ABEERU) is also thanked for availing resources to accomplish data collection.
The authors declare that the research was conducted in the absence of any commercial or financial relationships that could be construed as a potential conflict of interest.
All claims expressed in this article are solely those of the authors and do not necessarily represent those of their affiliated organizations, or those of the publisher, the editors and the reviewers. Any product that may be evaluated in this article, or claim that may be made by its manufacturer, is not guaranteed or endorsed by the publisher.
The Supplementary Material for this article can be found online at: https://www.frontiersin.org/articles/10.3389/fevo.2023.1280157/full#supplementary-material
Anadon J. D., Sala O. E., Turner B. L., Bennett E. M. (2014). Effect of woody-plant encroachment on livestock production in North and South America. PNAS. 111, 12948–12953. doi: 10.1073/pnas.1320585111
Avenant P. (2015). Report on the national bankrupt bush (Seriphium plumosum) survey, (2010- 2012) (South Africa: Department: Agriculture, Forestry and Fisheries. Directorate Land Use and Soil Management Sub-Directorate Natural Resources Inventories and Assessments).
Bakker J. P., Hoffmann F., Ozinga W. A., Rosen E. (2014). Shading results in depletion of the soil seed bank. Nordic J. Bot. 32, 674–679. doi: 10.1111/j.17564561051.2013.00288.x
Brown L. R., Magagula I. P., Barret A. S. (2022). A vegetation classification and description of Telperion Nature Reserve, Mpumalanga, South Africa. Veg. Classif. Surv. 3, 199–219. doi: 10.3897/VCS.85209
Clark M. D., Wonkka C. L., Kreuter U. P., Rogers W. E. (2020). Interactive effects of prescribed fire and livestock grazing on Seriphium plumosum in South African sour bushveld. Afr J. Range Forage Sci. 37, 278–285. doi: 10.2989/10220119.2020.1751287
Cerling T. E., Harris J. M., Passey B. H. (2003). Diets of east African Bovidae based on stable isotope analysis. J. Mammal. 84, 456–470. doi: 10.1644/1545-1542
Davies K. W., Bates J. D., Nafus A. M. (2012). Comparing burned and mowed treatments in mountain Big Sagebrush Steppe. Environ. Manage. 50, 451–461. doi: 10.1007/s00267-012-9898-2
Dreber N., Van Rooyen S. E., Kellner K. (2019). One savanna, many shapes: How bush control affects the woody layer in the southern Kalahari. S. Afri. J. Bot. 125, 511–520. doi: 10.1016/j.sajb.2019.08.026
Du Toit J. C. O. (2012). Problems with using the herbicide Tebuthiuron for controlling Seriphium plumosum. Grootfontein Agric. 12, 31–40.
Erfanzadeh R., Kamali P., Ghelichnia H., Pétillon J. (2016). Effect of grazing removal on aboveground vegetation and soil seed bank composition in sub-alpine grasslands of northern Iran. Plant Ecol. Divers. 9, 309–320. doi: 10.1080/17550874.2016.1221479
Graham S. C., Barrett A. S., Brown L. R. (2020). Impact of Seriphium plumosum densification on Mesic Highveld Grassland biodiversity in South Africa. R. Soc. Open Sci. 7, 192025. doi: 10.1098/rsos.192025
Hare M. L., Xu X. W., Wang Y. D., Yuan Y., Gedda A. E. (2021). Do woody tree thinning and season have effect on grass species’ composition and biomass in a semi-arid savanna? the case of a semi-arid savanna, southern Ethiopia. Front. Environ. Sci. 9. doi: 10.3389/fenvs.2021.692239
Haussman N. S., Kalwij J. M., Bezuidenhout S. (2016). Some ecological side-effects of chemical and physical bush clearing in a southern African rangeland ecosystem. S. Afr J. Bot. 102, 234–239. doi: 10.1016/j.sajb.2015.07.012
Liao C., Clark P. E., DeGloria S. D. (2018). Bush encroachment dynamics and rangeland management implications in southern Ethiopia. Ecol. Evol. 8, 11694–11703. doi: 10.1002/ece3.4621
MacFadyen D. N. (2014). The dynamics of small mammal populations in Rocky Highveld Grassland, Telperion, South Africa. PhD thesis (South Africa: University of Pretoria).
Marquart A., Slooten E., Jordaan F. P., Vermeulen M., Kellner K. (2022). The control of the encroaching shrub Seriphium plumosum (L.) Thunb. (Asteraceae) and the response of the grassy layer in a South African semi-arid rangeland. Afr J. Range Forage Sci. 40, 316–321. doi: 10.2989/10220119.2022.2086620
Mentis N. M (1981). The animal as a factor in pasture and veld management. In: Veld and pasture management in South Africa, Tainton N. M (ed)., 289–311. Pietermaritzburg: Shuter and Shooter.
Mndela M., Madakadze I. C., Nherera-Chokuda F., Dube S. (2020). Is the soil seed bank a reliable source for passive restoration of bush-cleared semi-arid rangelands of South Africa? Ecol. Process. 9, 1–16. doi: 10.1186/s13717-019-0204-6
Mndela M., Madakadze I. C., Nherera-Chokuda F. V., Dube S., Ramoelo A., Mangwane M., et al. (2022b). Short-term response of herbaceous vegetation to bush clearing in semi-arid rangelands of South Africa. Past: Res. Pol. Prac. 12, 1–13. doi: 10.1186/s13570-022-00235-7
Mndela M., Madakadze I. C., Tjelele J. T., Mangwane M., Nherera-Chokuda F., Dube S., et al. (2022c). Responses of grass productivity traits to bush clearing in semi-arid rangelands in North-West Province of South Africa. Rangel J. 44, 33–45. doi: 10.1071/RJ21053
Mndela M., Tjelele J. T., Madakadze I. C., Mangwane M., Samuels I. M., Muller F., et al. (2022a). A global meta-analysis of woody plant responses to elevated CO2: implications on biomass, growth, leaf N content, photosynthesis, and water relations. Ecol. Process. 11, 1–21. doi: 10.1186/s13717-022-00397-7
Mochi L. S., Mazia N., Biganzoli F., Aguair M. R. (2022). Contrasting effects of grazing on the early stages of woody encroachment in a Neotropical savanna. Basic Appl. Ecol. 60, 554 13–554 24. doi: 10.1016/j.baae.2022.01.007
Mucina L., Rutherford M. C., Palmer A. R., Milton S. J., Scott L., Lloyd J. W., et al. (2006). “Nama-karoo biome,” in The vegetation of South Africa, Lesotho and swaziland. Eds. Mucina L., Rutherford M. C. (Pretoria, South Africa: SANBI), 324–347.
Nkosi E. S., Brown L. R., Barret A. S. (2018). A baseline study for controlling the indigenous encroacher 582 Stoebe vulgaris in the natural grasslands of Southern Africa. Agric. Ecosyst. Environ. 265, 209–216. doi: 10.1016/j.agee.2018.06.013
O’Connor R. C., Taylor J. H., Nippert J. B. (2020). Browsing and fire decreases dominance of a resprouting shrub in woody encroached grassland. Ecology. 101, e02935. doi: 10.1002/ecy.2935
Pule H. T., Tjelele J. T., Tedder M. J. (2023). Post-fire soil nutrient dynamics in Seriphium plumosum L. encroached semi-arid grassland of Gauteng Province, South Africa. Agriculture. 13, 1971. doi: 10.3390/agriculture13101971
Roques K. G., O’Connor T. G., Watkinson A. R. (2001). Dynamics of shrub encroachment in an African savanna: relative influences of fire, herbivory, rainfall, and density dependence. J. Appl. Ecol. 38, 268–280. doi: 10.1046/j.1365-2664.2001.00567.x
Roux R. (2017). Spatio-temporal ecology of the Rusty-spotted genet, Genetta maculata, in Telperion nature reserve (Mpumalanga, South Africa). MSc thesis (South Africa: University of South Africa, Johannesburg).
Snyman H. A. (2010). Allelopathic potential, seed ecology and germination of the encroacher shrub Seriphium plumosum. Afr J. Range Forage Sci. 27, 29–37. doi: 10.2989/10220111003718482
Snyman H. A. (2012). Habitat preferences of the encroacher shrub, Seriphium plumosum. S. Afr J. Bot. 81, 34–39. doi: 10.1080/02571862.2012.745905
Spencer H. J. (2012). “Metsulfuron-methyl impact on native vegetation in the Daintree lowlands, far-north Queensland, Australia,” in Eighteenth Australasian Weeds Conference (Melbourne, Victoria, Australia). 8-11
Stevens N., Erasmus B. F. N., Archibald S., Bond W. J. (2016). Woody encroachment over 70 years in South African savannahs: overgrazing, global change or extinction aftershock? Phil. Trans. R. Soc B 371, 20150437. doi: 10.1098/rstb.2015.0437
Stokely T. D., Kormann U. G., Betts M. G. (2020). Synergistic effects of wild ungulates and management intensification suppress native plants and promote exotics. For. Ecol. Manage 460. doi: 10.1016/j.foreco.2019.117772
Urban M., Schellenberg K., Morgenthal T., Dubois C., Hirner A., Gessner U., et al. (2021). Using Sentinel-1 and Sentinel-2 time series for Slangbos mapping in the Free State Province, South Africa. Remote Sens. 13, 3342. doi: 10.3390/rs13173342
Van der Walt R. (2009). Wildflowers of the limpopo valley including mapungubwe national park. 1st ed (Musina: Buisness Print), 11–335.
Van Oudtshoorn F. (1999). Guide to grasses of southern Africa (Pretoria, South Africa: Briza Publications).
Van Zyl E., Avenant P. (2018). Bankrupt bush: A serious threat to South Africa’s central grassland. FarmBiz. Pp, 40–43.
Venter Z. S., Hawkins J., Cramer M. D. (2017). Implications of historical interactions between herbivory and fire for rangeland management in African savannas. Ecosphere. 8, e01946. doi: 10.1002/ecs2.1946
Venter A. J., Kalule-Sabiti M. J. (2016). Diet composition of the large herbivores in Mkambati Nature Reserve, Eastern Cape, South Africa. Afr. J. Wildl. Res. 46, 49–56. doi: 10.3957/056.046.0049
Ward S. E., Orwin K. H., Ostle N. J., Briones M. J. I., Thomson B. C., Griffiths R. I., et al. (2015). Vegetation exerts a greater control on litter decomposition than climate warming in peatlands. Ecol. 96, 113–123. doi: 10.1890/14-0292.1
Weber-Grullon L., Gherardi L., Rutherford W. A., Archer S. R., Sala O. E. (2020). Woody-plant encroachment: Precipitation, herbivory, and grass-competition interact to affect shrub recruitment. Ecol. Appl., 32, e2536. doi: 10.1002/eap.2536
Wepener J. P. (2007). The control of Stoebe vulgaris encroachment in the Hartbeesfontein area of the North-West Province. MSc thesis (Potchefstroom, South Africa: North-West University).
Wieczorkowski J. D., Lehmann C. E. R. (2022). Encroachment diminishes herbaceous plant diversity in grassy ecosystems worldwide. Glob Change Biol. 28, 5532–5546. doi: 10.1111/gcb.16300
Keywords: enclosures, grassland ecosystem services, herbicide, herbivory, mechanical shrub removal, Seriphium plumosum
Citation: Mndela M, Barrett AS and Brown LR (2023) Wild ungulates and shrub control interact to restore herbaceous vegetation in shrub-encroached mesic grassland of South Africa. Front. Ecol. Evol. 11:1280157. doi: 10.3389/fevo.2023.1280157
Received: 19 August 2023; Accepted: 04 December 2023;
Published: 19 December 2023.
Edited by:
Wei Wang, Chinese Research Academy of Environmental Sciences, ChinaReviewed by:
Huang Wen jie, Beijing Union University, ChinaCopyright © 2023 Mndela, Barrett and Brown. This is an open-access article distributed under the terms of the Creative Commons Attribution License (CC BY). The use, distribution or reproduction in other forums is permitted, provided the original author(s) and the copyright owner(s) are credited and that the original publication in this journal is cited, in accordance with accepted academic practice. No use, distribution or reproduction is permitted which does not comply with these terms.
*Correspondence: Mthunzi Mndela, TW1uZGVsYUB1ZmguYWMuemE=
Disclaimer: All claims expressed in this article are solely those of the authors and do not necessarily represent those of their affiliated organizations, or those of the publisher, the editors and the reviewers. Any product that may be evaluated in this article or claim that may be made by its manufacturer is not guaranteed or endorsed by the publisher.
Research integrity at Frontiers
Learn more about the work of our research integrity team to safeguard the quality of each article we publish.