- 1Comparative Cognition Unit, Messerli Research Institute, Department for Interdisciplinary Life Sciences, University of Veterinary Medicine Vienna, Medical University Vienna, University of Vienna, Vienna, Austria
- 2Research Centre for Biosystematics & Evolution, National Research and Innovation Agency (BRIN), Cibinong, Indonesia
The study of animal behaviour and cognition would not be complete without investigations of wild, free-ranging individuals in their natural environment. However, direct observations of species living in dense habitats can be challenging, leading many studies to focus on attracting target species to pre-selected, monitored locations baited with food. So far, researchers have rarely applied this approach to studying wild psittacines – an avian group of high scientific interest due to their advanced cognitive skills and conservation threats. We developed hoistable feeding platforms and tested their suitability for reliably attracting groups of wild Tanimbar corellas (hereafter: Goffins), opportunistic feeding generalists well-known for their advanced cognitive skills. To this end, we recorded the visitation rates of free-ranging groups at feeding platforms temporarily baited with dry corn. Moreover, we investigated the effects of several weather-related measures, the temporal distribution of foraging activity, and the effect of conspecific contact calls (playback stimulus). The results indicate that Goffins accepted the feeding platforms and reliably aggregated within their natural daily foraging bouts. While group size during visitation bouts depended on rainfall and resource abundance, platforms were still regularly visited by fewer, possibly locally residing individuals. These results provide the first systematic investigation of the temporal distribution and social dynamics of foraging bouts in wild Goffins. This study presents a novel method for reliably attracting wild opportunistic feeding generalist psittacines to a standardised, monitored location. It provides a suitable approach for observing foraging aggregations and, in the future, testing the problem-solving abilities of free-ranging Goffins in their natural habitat.
1 Introduction
In order to gain a holistic understanding of animal behaviour and cognition, it is crucial to investigate the actions of wild, free-ranging individuals. Conducting such studies in the natural setting is relevant for several reasons: they generate ecologically valid information about how different mechanisms and environmental factors interact and integrate, provide context in which cognitive skills are employed during the daily lives of a species, and can highlight evolutionary benefits of traits that improve survival and reproduction (Thornton, 2014; Shaw et al., 2015; Bond and Diamond, 2019; Janmaat, 2019; Rosati et al., 2022). The most valuable data comes from video-recorded, unobscured, and continuous close-range observations (McGrew, 1992). However, wild individuals of species living in dense habitats can often remain inconspicuous during their daily activities, making direct behavioural observations challenging. Exploring this fieldwork frontier demands approaches beyond tracking focal individuals and conventional direct observations (Troscianko and Rutz, 2015).
Field experiments are considered essential elements of ethological research programs (Fischer, 2022). Participation of wild animals can be encouraged by linking empirical paradigms to a familiar context (Healy and Hurly, 1995). Foraging is fundamental to sustaining metabolism, providing energy, and supporting reproduction (Pyke, 2019). Therefore, providing feeding opportunities could present a reliable method for attracting otherwise elusive, free-ranging species to a specific location. The ability to predict the presence of target species (McGrew, 1992) in a pre-selected, monitored location and their activity times would significantly improve the efficiency of behavioural data collection and allow the possibility of conducting cognitive tests in the wild. However, intentionally feeding wildlife is a topic of ongoing debate (for reviews, see Fedigan, 2010; Hill, 2017), and empirical designs should be carefully considered to prevent lasting modifications of the natural behavioural repertoire within the target population (McGrew, 1992; Rutz, 2018).
Intentional wildlife feeding encompasses varied practices (often with overlapping definitions) that differ in their aims, durations, and potential effects on the wild populations. These practices are commonly used in conservation and wildlife management programs, including artificial (e.g., supplemental, emergency, or winter) feeding and baiting (for reviews, see Dunkley and Cattet, 2003; Sorensen et al., 2014). In research, two types of wildlife feeding can be generally distinguished: provisioning and baiting. During provisioning, researchers regularly provide natural or novel food items to target animals, either directly (by hand) or indirectly (at feeding stations; Dubois and Fraser, 2013; Hill, 2017). Historically, this method was used to facilitate the habituation of primates to human observers and allow close-range direct observations. During baiting, researchers temporarily place natural or novel food resources in the environment to attract wild animals to a specific area. This method typically allows observations, facilitates engagement with experimental tasks, or provides capture opportunities (Dunkley and Cattet, 2003; Fedigan, 2010; Sorensen et al., 2014). While provisioning has led to scientific breakthroughs on socio-ecology and technical intelligence in primates (e.g., Goodall, 1986), baiting studies have provided details of otherwise difficult-to-observe complex behaviours (e.g., Holzhaider et al., 2010).
Free-ranging individuals of various avian species can be attracted to visit specific feeding locations. Examples of investigated topics related to avian behaviour and cognition include diurnal foraging patterns of songbirds (Farine and Lang, 2013), the spread of novel foraging techniques in Great tits (Parus major; Aplin et al., 2015), reactions to dead conspecifics in American crows (Corvus brachyrhynchos) and Rock pigeons (Columba livia; Swift and Marzluff, 2015), responses to novelty and risk in Jackdaws (Corvus monedula; Greggor et al., 2016), or first insights into exploration and neophobia in Common buzzards (Buteo buteo; Mioduszewska et al., 2021). The approach of attracting wild individuals to a specific location (baited tables or sites) was perhaps most often used to study wild New Caledonian crows (Corvus moneduloides; e.g., Hunt, 2000; Hunt and Gray, 2004; Hunt et al., 2006; Hunt and Gray, 2007; Hunt et al., 2007; Holzhaider et al., 2010; St Clair et al., 2015), an avian species of high scientific interest due to their advanced cognitive skills as well as their proficiency in using and crafting tools (for a review, see Hunt et al., 2013). As these crows are sensitive to human presence and inhabit forested mountainous regions of the New Caledonian islands, visibility is limited, and closely following focal subjects is not feasible (Rutz et al., 2007). Supplemental feeding is also part of a soft-release procedure, during which animals are initially habituated to the release area and subsequently provided with post-release support (food and water) until they become gradually independent. Soft-release is preferred when reintroducing avian species from rehabilitation centres (Jones, 2004), which allows the study of released psittacines (e.g., problem-solving tasks in Blue-fronted amazons, Amazona aestiva; Godinho et al., 2020). However, only a handful of studies have used outdoor feeding stations to study the behaviour of wild, free-ranging psittacines (e.g., ontogeny of innovative abilities in Kakas, Nestor meridionalis; Loepelt et al., 2016).
Psittacines (parrots and cockatoos; order Psittaciformes) are of significant scientific interest due to their advanced cognitive abilities (for a review, see Auersperg and von Bayern, 2019) and conservation threats (Olah et al., 2016; Forshaw and Knight, 2017). However, similarly to New Caledonian crows and arboreal monkeys, the study of psittacines in the wild is known for its considerable challenges, as most species are highly mobile and often live in dense tropical forests (Struhsaker, 1975; Beissinger and Snyder, 1992; Collar, 1997; Gilardi and Munn, 1998; McGraw and Zuberbühler, 2007; Perry and Manson, 2008; Morand-Ferron et al., 2016). Therefore, most studies on the cognitive skills (other than vocalisations) of wild psittacines were conducted on relatively conspicuous species, either due to their large size (Hyacinth macaws, Anodorhynchus hyacinthinus; e.g., Schneider et al., 2006; Palm cockatoos, Probosciger aterrimus; e.g., Wood, 1984; Heinsohn et al., 2017), limited timidity around humans (Kea, Nestor notabilis; e.g., Diamond and Bond, 1999; Gajdon et al., 2004), or habituation to humans coupled with accessibility and frequent ground foraging in urban environments (Sulphur-crested cockatoos, Cacatua galerita; e.g., Aplin et al., 2021; Klump et al., 2021).
The Tanimbar corella (Cacatua goffiniana; alternative common name: Goffin’s cockatoo; indigenous Tanimbarese name: Manik tilgnoi; hereafter: Goffin) has emerged as an important model species in comparative cognition research, owing to its advanced cognitive abilities in both the technical and the social domain (for a review, see Auersperg and von Bayern, 2019). It also presents a valuable model to study potential ecological precursors for the emergence of domain-general intelligence and flexible problem-solving skills due to their socio-ecology and island habitat (O’Hara et al., 2018; Mioduszewska et al., 2022). Goffins are a medium-sized (30–32 cm) corella species of the cockatoo family (Eaton et al., 2021). They are endemic to the remote Tanimbar Islands in southeast Indonesia, where they can be mainly encountered in dense, seasonal tropical forests and on agriculture fields (Jepson et al., 2001; O’Hara et al., 2018; Mioduszewska et al., 2022). Introduced Goffins can also be found in several locations with a tropical climate, such as Singapore or Taiwan (for reviews, see (Calzada Preston and Pruett-Jones, 2021; Mioduszewska et al., 2023). The only published population estimates were conducted in 1993 and reported between 231,500 and 347,088 individuals (Cahyadin et al., 1994a; Cahyadin et al., 1994b; Jepson et al., 2001). While these numbers may seem sufficient for maintaining a stable population in the wild, these assessments could be considered uncertain due to the wide range of population estimates (VKM, 2020). Considering these large ranges and the datedness of these results, a reassessment is needed to establish the current population trends of this remarkable species.
In the native habitat of the Tanimbar Islands, Goffins are opportunistic feeding generalists, potentially engaging in two main foraging bouts in the early mornings and late afternoons (Cahyadin et al., 1994b; O’Hara et al., 2018). They consume various partially seasonal food sources in the forest, such as seeds and fruit. Depending on the foraging ecology of a species, agriculture fields can provide localised, rich food sources for birds (Noske, 1980). Goffins are known to take advantage of several (native and introduced) crops, which has rendered them unpopular among some local farmers (Mioduszewska et al., 2018). Particularly, corn (Zea mays), thought to have been introduced to the Tanimbar Islands around the 16th century (Pesireron et al., 2021), attracts large groups of Goffins that repeatedly congregate for several days on a field before moving to the next one (Cahyadin et al., 1994b; B.M. & M.O., personal observations). Local farmers intermittently protect their crops by chasing Goffins from cultivated areas or capturing them in leg-noose traps hidden in the corn plants (B.M. & M.O., personal observations). These practices likely associate agriculture fields with certain risks for the Goffins (such as harassment or injury; Hockings and McLennan, 2012). A similar attraction to cornfields was also observed in other psittacine species, such as Green parrots (Poicephalus senegalus; Weladji and Tchamba, 2003), Red-fronted macaws (Ara rubrogenys; Pitter and Christiansen, 1995), or Sulphur-crested cockatoo (Cacatua galerita; Styche, 2000).
The Goffins’ natural diet also includes food sources that require extraction (e.g., young coconuts or papayas; Mioduszewska et al., 2018; O’Hara et al., 2018; Mioduszewska et al., 2022) and even complex tools (a tool set; O’Hara et al., 2021). The first observation of tool use in wild Goffins to extract the embedded seed matter of a tropical fruit (Wawai, Cerbera manghas; O’Hara et al., 2021) was recorded in a field capture-release aviary, which enabled the collection of extensive and detailed behavioural data. An opportunistic observation in the dense tropical forest would have been either exceedingly improbable or potentially unattainable due to the small-scale, dynamic, and undercover characteristics (intraoral processing and manipulation of small wooden fragments) of this complex behaviour (O’Hara et al., 2018; Mioduszewska et al., 2022). Potentially complex behaviours of other psittacine species might likely face similar detection limitations. Therefore, establishing a setup that can reliably attract wild individuals to a specific, secure (beyond the reach of local villagers), and monitored location is crucial for future detailed investigation of behaviours under natural, free-ranging conditions.
Using playback calls of the target species could further enhance the visitation rate of a feeding station (e.g., Goldberg and Ewald, 1991). Contact calls function to coordinate movements and activities between individuals in a wide variety of social species (Kondo and Watanabe, 2009; Berg et al., 2011) and are often individually recognisable when visibility in the environment is low and repeated interactions occur between familiar individuals (Berg et al., 2011). Flocks of wild Goffins have also been observed to produce loud screeching calls that seem to attract conspecifics to a specific location (M.O., unpublished data). Most psittacine species are generally non-territorial and social (for a review, see Bradbury, 2003). Therefore, they are likely to respond to playback of conspecific calls as non-territorial animals living in fission-fusion groups (i.e., groups that flexibly change in size, typically due to changes in the availability of resources across space and time or varying needs/activities of group members) often produce loud contact calls to facilitate group formation (Bradbury and Vehrencamp, 2011) and which typically elicit a response from conspecifics (Bradbury, 2003). Therefore, loud contact calls present a promising stimulus for playback sessions (rebroadcasting signals to animals and observing their reactions; McGregor, 1992), aiming to facilitate visitation of feeding sites. Acoustic playbacks are frequently performed in ornithological field conditions, with diverse applications, such as investigating behavioural responses, population surveys, determining the presence of rare/elusive species, or attracting individuals to a location (for reviews, see Rosenthal, 2019; De Rosa et al., 2022).
Fieldwork projects depend on weather conditions, which also significantly impact avian biology (Elkins, 2010). Climate change, extreme weather conditions, and even minor-scale fluctuations can have an effect. Brief or localised temperature, rainfall, or wind changes can substantially influence individual behaviour, life history, and physiology, impacting the population and species levels (for a review, see Mainwaring et al., 2021). In terms of the effects of specific abiotic factors on foraging, increased environmental temperatures (>27°C) may elicit heat stress in birds and led to a reduction of foraging activity in Western Australian magpies (Edwards et al., 2015). In psittacines, some foraging behaviours are influenced by weather conditions (more clay licking by various species on sunny than rainy mornings; Brightsmith, 2004), whereas others (feeder visitations by Blue-fronted amazons; Amazona aestiva) are not interrupted by temperature, humidity, and luminosity (although morning visits were best explained by variable humidity and afternoon visits by temperature and luminosity; Fraga et al., 2023). Overall, higher daily maximum temperatures seem to reduce foraging activities and increase rest time (Carnaby’s cockatoos, Zanda latirostris; Riley et al., 2023).
We report implementing a novel feeding platform design to attract and study wild, free-ranging psittacine species in situ. It consisted of a hoistable feeding plate with attached perches, monitored by a motion-detection camera. We present the first systematic data on the foraging bouts of wild Goffins on the Tanimbar Islands and the environmental factors affecting their foraging activity. Based on previous behavioural observations, we predicted that visitations to the feeding platforms would occur mainly in the mornings and afternoons (Cahyadin et al., 1994b; O’Hara et al., 2018). While primarily driven by nutritional needs, daily recurring weather variation likely influences these foraging bouts. Based on previous literature, we hypothesised that increasing sunlight intensity and accompanying increase in temperature would impact Goffin activity and might account for limiting foraging bouts in open areas to relatively cooler times of the day. Furthermore, we discuss potential mechanisms that may lead to reduced visitation rates under adverse environmental factors, such as heavy rainfall and winds. Finally, we explored whether acoustic playback had an effect on attracting Goffins to a feeding location. Based on the literature (Rosenthal, 2019; De Rosa et al., 2022), we expected that contact calls at the platforms might increase the detectability of these novel feeding sites and visitation rates.
2 Material and methods
2.1 Study site
The Tanimbar Islands are a relatively small island group (5440 km2) in the Maluku Province of southeast Indonesia. The islands were formed from uplifted reef limestone during the Pleistocene (Monk et al., 1997). The climate on the Tanimbar Islands is tropical wet and dry, determined by seasonal monsoons (Beck et al., 2018). Two rain seasons occur in December–March (West Monsoon) and May–September (East Monsoon), whereas the main dry season occurs in September–December and a short dry season occurs during the shifting of wind directions in April (Laumonier and Nasi, 2018; O’Hara et al., 2018). Seasonal durations do not seem consistent across years, and interannual rainfall variability is high. Temperatures are stable (annual mean: 21°C, between 1977 and 2003), with the humidity typically remaining high (above 70%) throughout the year (Laumonier and Nasi, 2018).
Our study site is located on Yamdena (7°36’S 131°25’E), the largest island (approx. 3260 km2) in the Tanimbar archipelago. It is relatively flat, with only low (120m-high) hills running down its southeast side (Jepson et al., 2001). Its forest cover (approx. 70% of the total surface area) includes seasonal evergreen, dry deciduous, and moist deciduous forests (Jepson et al., 2001; Laumonier and Nasi, 2018). Local villagers engage in swidden agriculture (shifting cultivation or slash-and-burn farming), fishing, and hunting. Small to medium-size, patchy agriculture fields (or gardens) consist of a mixture of fruit tree plantations (e.g., papaya, bananas) and dryland farming (e.g., beans, corn, or cassava; Cahyadin et al., 1994a). Produce is used either for own consumption or selling at local markets. Coconut (Cocos nucifera) plantations stretch along the south-eastern seaboard (2–3 km inland), whereas agriculture fields are situated in the area between these plantations and the forest (Jepson et al., 2001) and interspersed (either near the forest edge or in the forest; approx. 5km from the coastline) along the main road traversing the island (B.M. & M.O., personal observations). Swidden agriculture, the primary subsistence farming practice in the tropics (for a review, see Li et al., 2014), involves the preparation of a new field by cutting and burning a patch in the forest. Such fields are typically used for three to six consecutive years until the soil is depleted (Cahyadin et al., 1994b). Afterwards, they are moved to a new location, and the abandoned field becomes overgrown (fallow phase) with either shrub or alang-alang (Imperata cylindrica) grasses. We established a research station on an old field bordering the forest (7°48′59.3′′S, 131°22′41.2′′E) with the permission and collaboration of local indigenous farmers from Lorulun village and the local government. Old logging routes and paths used by local hunters provide access to the primary and secondary forest, whereas the main road provides access to the station.
2.2 Feeding platforms
We piloted different feeding systems in our study area since 2018, optimised them, and gradually (December 2021–December 2022) established eight hoistable feeding platforms in the current design (Figure 1A). Psittacines prefer tall perching spots, as they provide all-round vision (Noske, 1980). Therefore, an elevated platform setup (instead of lower feeding tables) was selected to attract wild Goffins to new foraging locations. When raiding agriculture fields, cockatoos prefer locations away from roads and nearby trees (Noske, 1980; Bomford, 1992; O’Hara et al., 2018; B.M. & M.O., personal observations). Therefore, all platforms were positioned in clearings at the forest edge (bordering either active or abandoned agriculture fields) to ensure their conspicuousness and availability of nearby trees for perching and potential hiding opportunities. The spacing and locations of these feeding sites mimicked the natural distribution of feeding opportunities (O’Hara et al., 2018), as food is generally distributed in an environment in patches rather than uniformly (Pyke, 2019).
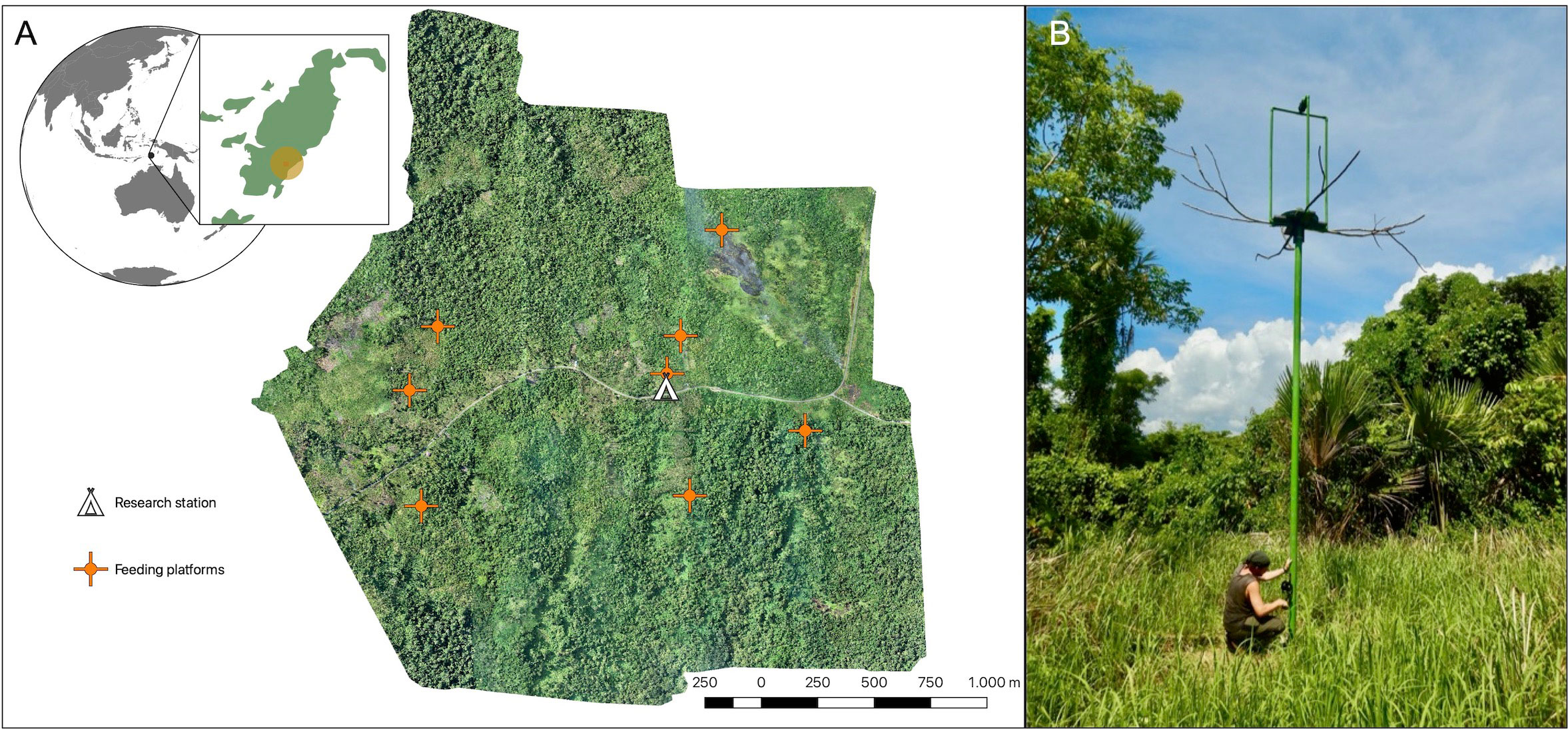
Figure 1 Feeding platforms at the field site. (A) Overview and orthographic map of the study site based on aerial imagery within a radius of approximately 2km around the research station (indicated by the house symbol; red crosses indicate established feeding platforms). (B) The hoistable feeding platform system with long side perches and a camera holder on top of the feeding platform.
The feeding platforms were designed and custom-built to resemble medium-high trees with dead branches, as Goffins often aggregate on trees with dead branches (B.M. & M.O., personal observations). Long metal pipes (6m; coloured green for a natural appearance; brown paint was not available) were erected by digging a small container filled with stones and concrete mixture into the ground (approx. 1m deep) and placing the pipe inside the container. This semi-permanent solution ensured the stability of the platform structure despite the shifting of the ground after heavy rains and strong winds. Additionally, the presence of the container allows for the potential relocation of platforms. The pipe visible above the ground measured 5m in height and held a platform constructed from a wooden cross (made of two connected boards, each 70x25cm), with an attached plastic feeding plate (Ø 60cm; green or brown colour for a natural appearance), that allowed efficient cleaning. The plastic plates were perforated with lateral holes to allow rainwater drainage. While corn kernels did not absorb standing water during the rain, they became wet and gradually dried once it stopped. The feeding plate and platform had a central hole (Ø 10cm) through which the platform could slide along the pipe. Underneath the wooden cross, four 35cm long, square (3x3cm) metal rods, containing two sets of bearing wheels each, were attached, clasping the pipe. These rods with wheels acted as rails, stabilising the platform and enabling smooth up–and–down movement. Platforms were suspended on a 5mm steel rope, which led from the top into the pipe (via a pulley) and exited through a hole in the pipe’s lower section (approx. 50cm above the ground). The rope’s end was affixed to a winch, which included a safety breaker and a locking system with a padlock. This mechanism enabled efficient platform operation and allowed comfortable rebaiting, modification, and securing of the platform in place (access was provided only to researchers; Figure 1B). Long branches (approx. 1m) were attached at each side of the wooden cross to provide natural perching locations. A scaffold (1.8m high) made from 1” PVC pipes (Ø 2.5cm) served as a holder for motion-detection cameras. It did not obscure the visibility of the food on the platform to passing Goffins.
2.3 Biosecurity
Providing concentrated food sources might unintentionally facilitate pathogen transmission (for a review, see Becker et al., 2018). Therefore, the materials used to construct the feeding platforms were selected to be reliable (withstanding the tropical climate) and hygienic (easy cleaning to ensure birds’ welfare). Goffins typically perched on the provided branches, the wooden cross, and the plate edges. Perching on the camera holder above the feeding platform occurred rarely and was always brief. Therefore, contaminating corn with faecal matter did not pose a health hazard to the foraging Goffins. Successfully applying clinical disinfection protocols during fieldwork is challenging as field conditions lack the controlled setting of a laboratory (such as quarantine barriers or clean rooms; Fogell, 2019). Ensuring the required contact times between disinfectants and surfaces in a hot and humid climate might also necessitate repeated re-application of chemicals. To the best of our knowledge, only one study has investigated the effectiveness of disinfection of supplemental feeding stations used in the conservation of wild Echo parakeets (Psittacula eques; Fogell, 2019). Results indicate that even intensified disinfection does not reduce the probability of infection with a Psittacine Beak and Feather Disease (PBFD) virus (common in wild psittacines; Thomas et al., 2007). Instead, it hinders reproductive success by negatively impacting egg hatching.
The feeding platforms were cleaned by removing food remnants before baiting them with fresh corn. In terms of disinfection, to avoid introducing potentially harmful chemicals (chlorine-based, Virkon S, or F10 SC disinfectants; Das, 2002; Hangartner and Laurila, 2012; F10 Products Limited, 2016) to the environment, we relied on the strong daily ultraviolet (UV) radiation from the sun, which reaches extreme levels on the Tanimbar Islands (UVI range: 12–14; levels above 11 are categorised as extreme; Rehfuess, 2002). The elevated, open design of the feeding platforms and their placement in clearings fully exposed them and corn to sunlight and UV radiation. UV radiation has been considered a clean disinfection process (not producing toxic compounds; Das, 2002) and the primary germicide in nature (Lytle and Sagripanti, 2005). Its efficiency was proven against bacteria (Coohill and Sagripanti, 2009) and viruses found on surfaces (Lytle and Sagripanti, 2005; Nicastro et al., 2021). Notably, the PBFD virus (present on the Tanimbar Islands within the Goffin population; M.O., unpublished data) was estimated to be susceptible to deactivation through UV after approximately 5 minutes of high-intensity sun exposure (Lytle and Sagripanti, 2005).
2.4 Data collection
A total of eight feeding platforms were constructed, assembled, and erected across the study area (Figure 1). The platforms were baited with 1kg of dry corn (sourced from local farmers and hand-sieved for quality control), which was uniformly spread on the feeding plate. The feeding period overlapped with the locally-occurring corn harvest times (naturalistic baiting schedule). Goffins are known for raiding cornfields in large flocks, and corn seems to be the preferred food source during the harvest season (Cahyadin et al., 1994a; Cahyadin et al., 1994b; Jepson et al., 2001). An average consumption speed was estimated to be approximately nine corn kernels per minute (or 2g/min), with single individuals potentially consuming as much as 60g of corn per foraging bout (observations were conducted for approx. 30 minutes; Cahyadin et al., 1994a). Considering a conservative average Goffin group size of 10 individuals, the daily corn consumption of a group could equate to up to 1.2kg. Therefore, the amount of corn provided on the feeding platforms was considerably less (1kg over 10 days) than what Goffins would typically consume. Once corn was placed on the feeding platforms (first baiting; Day 0), individuals could freely visit the platforms for 10 days. After this period, a second baiting was conducted with 1kg of corn, followed by a further 10 days of free access. All platforms were baited on different days to allow for potential maintenance (e.g., replacing damaged branches or feeding plates).
Research activities in the field, including the mere human presence, can influence the behaviour of study species (Fair et al., 2010). Therefore, data was collected by deploying motion-detection cameras (also known as camera traps; Bushnell Core NoGlow S 4K, DS & DS 4), which are commonly used for monitoring wildlife behaviour (for reviews, see O’Connell et al., 2011; Trolliet et al., 2014; Rovero and Zimmermann, 2016). One device was attached to the camera holder of each platform, providing an overhead view of the plastic feeding plate. Cameras were configured to take three images at medium image size and use a no-glow flash setting adjusted for fast motion illumination in low-light conditions. Intervals between image bouts were set to 3 minutes. Weather data were recorded every five minutes by a weather station (Sainlogic WS3500) installed at the research station. These data comprised temperature (°C), wind speed (km/h), luminosity (Lux), sunlight intensity (UVI), humidity (%) and rainfall (mm/min). The weather station provided detailed reports at 5-minute intervals in April 2022 and March–April 2023.
2.5 Playback sessions
We pseudo-randomly assigned half of our feeding platforms to receive a playback session in the morning of Day 1 after the first baiting and the other half to receive a playback on Day 1 after the second baiting. The playback setup included a camouflaged wireless speaker (JBL Charge 5) concealed on trees or shrubs within 10m proximity to a feeding platform and researchers (B.M. & M.O.) positioned in a camouflaged hide (approx. 50m from the speaker). Materials used to construct the hide included a paracord, cable binders, camouflage nets, and natural vegetation, which provided a flexible, breathable, and affordable system. The hide was constructed the day before the playback session, whereas the speaker was placed in a camouflage-patterned string bag and positioned in the early morning (1h before sunrise) on the day of the playback session. The playback stimulus consisted of a 19-second sample of loud calls (recorded from a close distance with a directional Sennheiser ME66 microphone and a Tascam DR-44WL handheld audio recorder) produced by a Goffin flock foraging on a cornfield while a second flock was approaching (for the audio file, see “Playback.wav” in SM). The sample included multiple loud calls from different individuals. Post-hoc measurements of sound pressure levels from the speaker (measured with a dBMeter phone application) revealed an average of 86.6dB and a maximum of 92.3dB at a 3m distance. Playbacks were semi-interactive, broadcasted once whenever a Goffin was spotted within approximately 100m of the platform or heard nearby unless another individual was already present within a 100m radius. In addition, the playback stimulus was broadcasted once every half hour between 06:00 and 08:00, except when individuals were already present in the feeding platform area. Playback broadcasts conducted at each feeding platform could not be heard at the locations of nearby platforms due to the distance and dense vegetation.
2.6 Data processing and scoring
Images from the motion-detection cameras were collected during each baiting session. A list of image names and creation date was derived with XnView software (ver. 1.4.3). Each image series was loaded as an image stack in Fiji software (ImageJ v. 2.14.0/1.54f; Schindelin et al., 2012) with reduced file size (30%). We created a z-composite image to centre the images and registered the stack using the ‘TurboReg’ plug-in (Thévenaz et al., 1998). Based on the registered stack, we created another z-composite image to create a mask showing only the feeding plate and applied this mask to the entire image stack. Finally, the number of individuals in each image (within the visible unmasked area of the feeding plate) was counted manually using the ‘Cell Counter’ plug-in.
2.7 Statistical analysis
As several weather measures were highly correlated (Supplementary Figure 1), we employed a Principal Component Analysis (PCA) to generate a composite score of recurring daily weather measures: temperature, humidity, light intensity, and UV index. The first principal component (PC1) of our PCA, based on a correlation matrix of the weather measures, explained 88.43% of the variance in the data. This component was strongly negatively correlated with humidity (-0.48) and positively related to temperature (0.51), UV index (0.51), and illumination (0.51).
To estimate the effects of playback, correlated recurring daily weather variation composed of temperature, luminosity and humidity (as PC1), wind (km/h), rain (mm/h), days since baiting (resource depletion), and assumed daily foraging patterns (all fixed effects) on visitation rates, we employed a hurdle model. This model treats zero- and non-zero outcomes as two separate categories (Brooks et al., 2017a). Fitting a truncated Poisson distribution to all cases with counts greater than zero examines the effects of predictors on the number of individuals visiting the platforms. Fitting a binomial distribution to non-zero outcomes (treated as ones) and zero count cases allows for the evaluation of general visitation of feeding platforms by one or more individuals. We modelled daily variation in visitation rates by turning time into radians by first dividing them by 24 and then multiplying with 4 × π (to simulate two foraging bouts: morning and afternoon) and adding –(π*6/6) to induce a horizontal phase shift with cosine-maxima at 06:00 and 18:00. Finally, we included the sine and cosine of the resulting variable into the model (Stolwijk et al., 1999).
As we conducted repeated observations at the same locations during morning and afternoon bouts of different days, we included random intercept effects for location and an explicit term for nesting bouts within days and days within locations. To keep the type I error (false-positive) rate at the nominal level of 0.05, we included random slopes (Schielzeth and Forstmeier, 2009; Barr et al., 2013) of days since baiting and the two terms representing season (sine and cosine of the radians of time) within a location and the nested term into the model. We then z-transformed continuous variables (wind, rain, and days since baiting) to a mean of zero and a standard deviation of one to ease the interpretation of the model coefficients and ease model convergence (Schielzeth, 2010). As an overall test of the effect of the fixed effects and to avoid cryptic multiple testing (Forstmeier and Schielzeth, 2011), we compared the full model as described above with a reduced model, including the sine and cosine of radians time to control for daily foraging variation and identical random structure, but lacking the fixed effects of weather-related data, playback, and days since last baiting. We estimated model stability by dropping the random factor levels one at a time from the data and comparing the estimates derived for models fitted to these subsets with those obtained for the entire data set (for mixed effects models, exclusion of levels of random effects is preferred over excluding individual cases; Nieuwenhuis, 2012). This method revealed a good stability for the conditional (truncated Poisson) model and moderate stability for the zero-inflated (binomial) model (for more details, see SM).
In order to check for collinearity among the predictors, we determined the Variance Inflation Factor (VIF) based on a standard linear model, lacking random effects (Field, 2005). As expected, the analysis revealed moderate collinearity of daily variation in Goffin visits and the composite intrinsic daily weather variation (PC1; maximum VIF: 5.416). However, all further predictors showed little signs of collinearity (maximum VIF: 1.661). The dispersion parameter was calculated according to the suggestions in the ‘glmmTMB’-vignette and tested with the ‘check_overdispersion’ function of the ‘performance’ package. With a dispersion parameter of 1.002, the response was not over-dispersed.
All statistical analyses were conducted in R software (v. 4.3.1; R Core Team, 2023). The model was fitted using the function ‘glmer’ of the package ‘glmmTMB’ (Brooks et al., 2017b). VIF was determined using the function ‘vif’ of the package ‘car’ (Fox and Weisberg, 2019). The results were visually represented using the package ‘ggplot’ (Wickham, 2016). The data analysed for this model comprised a total of 7850 observations at eight feeding platforms, with two daily foraging bouts over a period of 20 days.
2.8 Ethical note
Goffins have a protected status in Indonesian Government Regulation No. 7 of 1999 Presiden Republik Indonesia, 1999a; Presiden Republik Indonesia, 1999b. International trade was banned within Appendix I of the 1994 Convention on International Trade in Endangered Species (CITES; 55). Our research plans were reviewed and approved (Ref No.: 047/KE.02/SK/9/2022) by the Animal Research Ethics Committee of the Indonesian National Research and Innovation Agency (NRIA), and all our research activities were following the Indonesian Ministry of Environment and Forestry Regulation No. 92 of 2018 (dan Kehutanan, 2018).
3 Results
Overall, the full model was significant as compared to the reduced model (likelihood ratio test: χ2 = 193.16, df = 18, P < 0.001). We found significant effects of foraging bouts, with most visitations occurring in the early morning and late afternoon, which is likely associated with the significant effect of daily recurring weather variation (temperature, sunlight, and humidity). However, no other measures seemed to have a strong effect on the general visitation of one or more Goffins to platforms (Table 1, zero-inflated model).
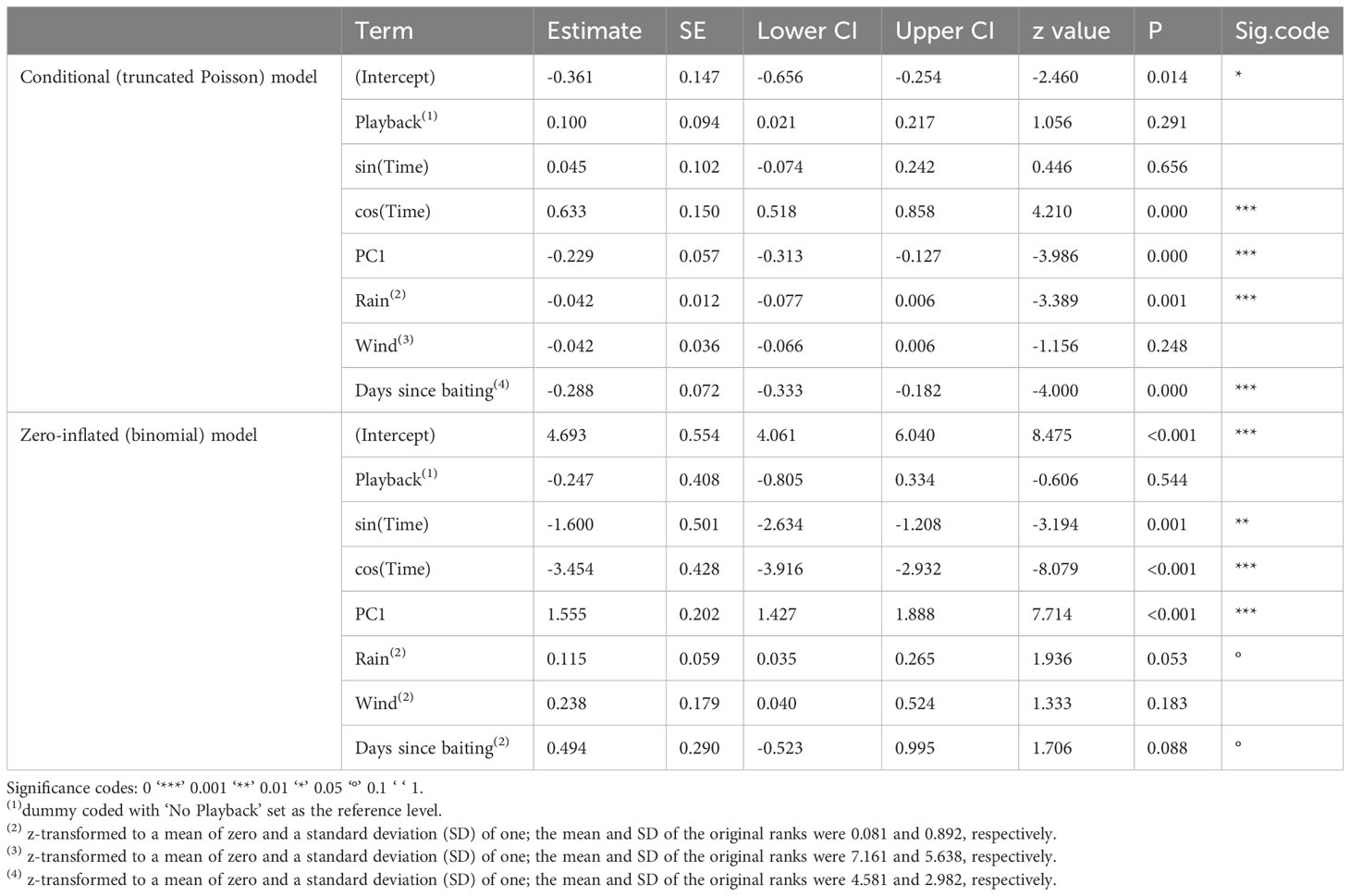
Table 1 Results of the employed hurdle model with the conditional (truncated Poisson) and zero-inflated (binomial) parts; estimates are provided with standard errors, confidence intervals, z values and significance codes.
The two daily foraging bouts (shown descriptively in Figure 2) had peaks at 06:00 and 17:00, as indicated by the number of camera images triggered by visitations and false positive triggers caused by either moving vegetation in the background or shadows. These bouts were confirmed in the binomial part of the hurdle model by the highly significant effect of our modelled temporal variation (as the sin and cosine in radians time) in visitations (Table 1). The principal component variable representing recurring weather variation (PC1) had a highly significant negative effect on visitations. At higher values of PC1 (corresponding to higher levels of solar intensity, higher temperature, and decreased humidity), significantly fewer visitations were recorded (Figure 3).
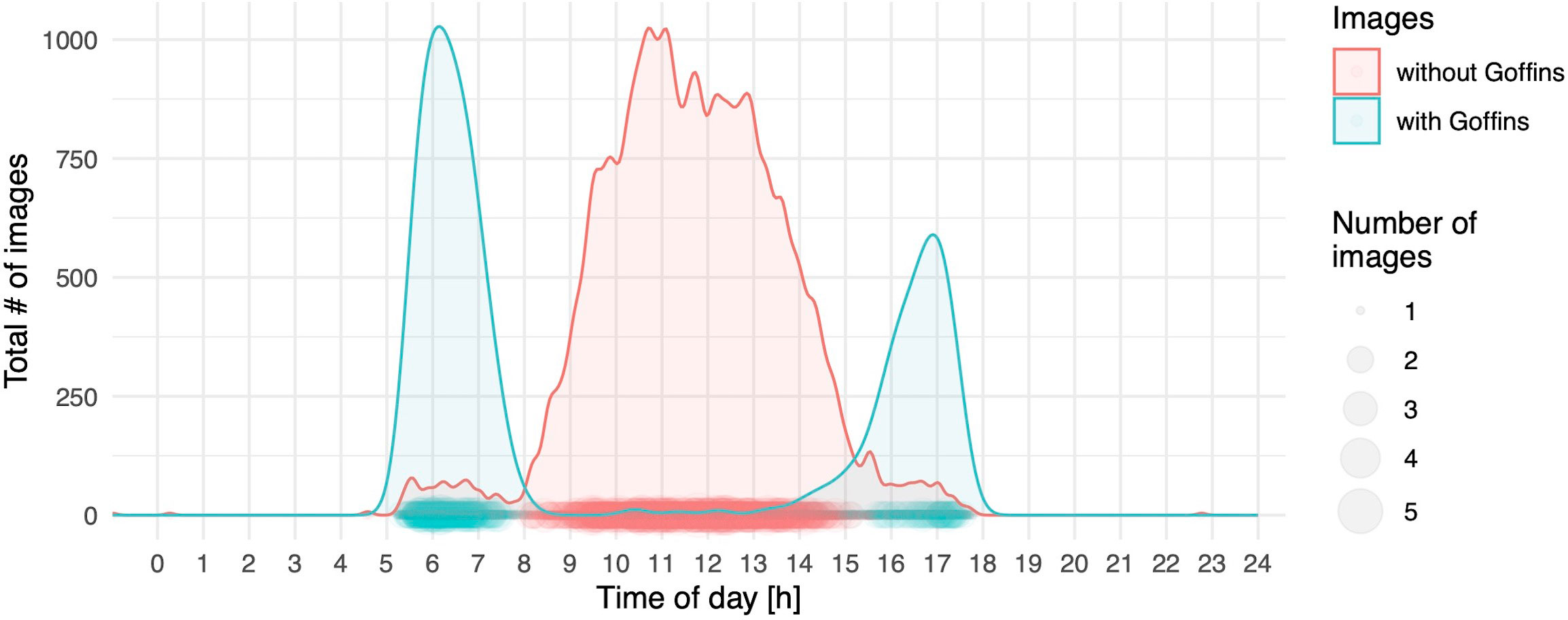
Figure 2 Number of all images taken by motion-detection cameras pooled over the course of one day as density plots; the size of dots indicates the number of images available per minute of the day; blue indicates images with at least one (or more) individuals present in the image, whereas red represents images without any Goffins.
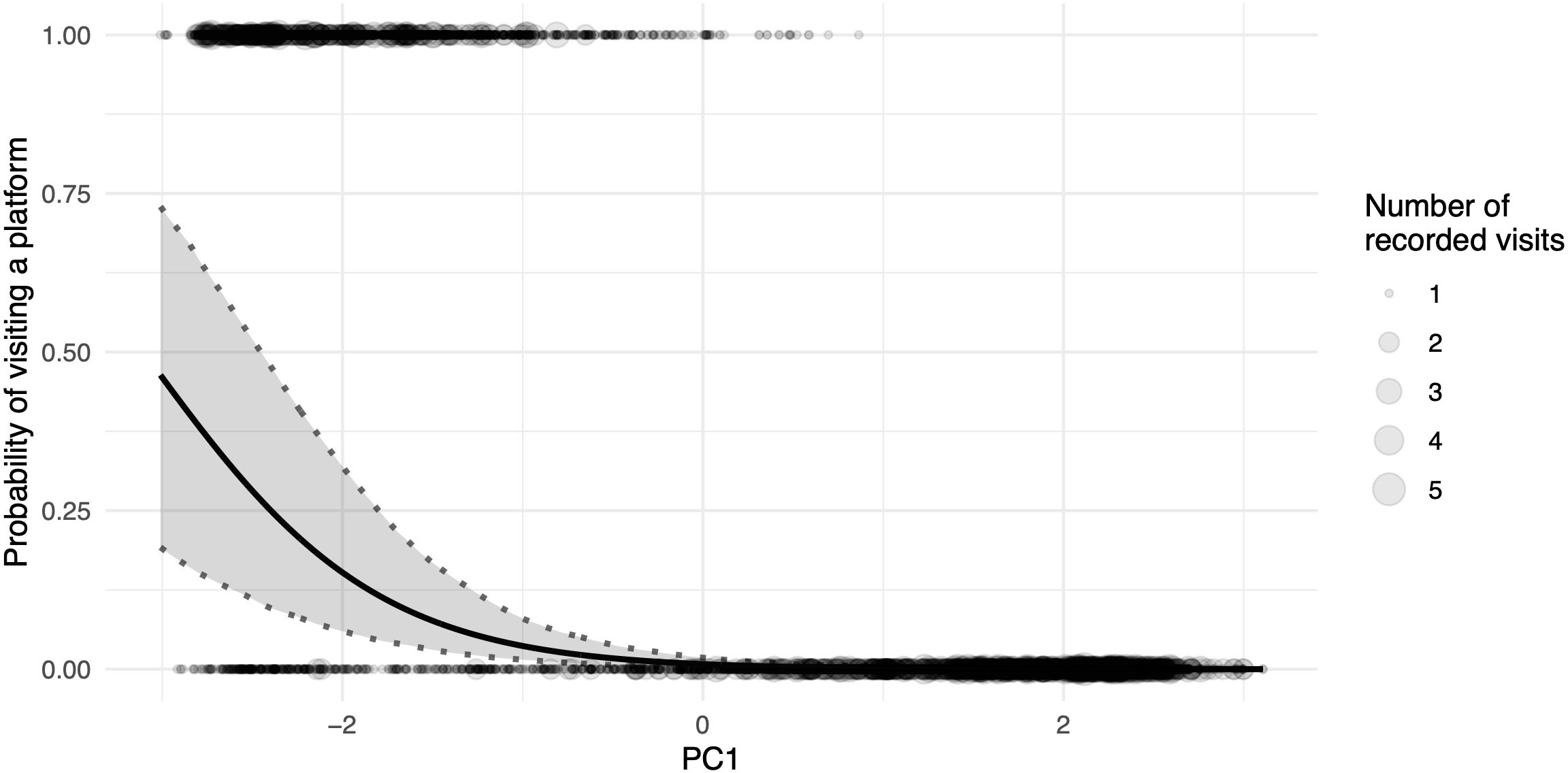
Figure 3 Proportion of general visits to platforms as a function of the principal component for daily weather variation; higher values of PC1 correlate to a higher temperature and light intensity, whereas they simultaneously correlate with lower humidity; the black line depicts the fitted model, and shaded areas represent 95% confidence intervals of model estimates based on 1000 bootstraps; the size of the dots indicates the number of images recorded at the same specific value for PC1.
While no other predictors seemed to affect general visitation rates, the number of individuals present during visitations (conditional/Poisson model) was significantly affected by the amount of rainfall and days since baiting, in addition to the compound predictor for recurring weather variation (PC1) and two temporally spaced foraging bouts (Table 1). With the increasing value of PC1 (corresponding to higher levels of solar intensity, higher temperature, and decreased humidity), the number of individuals decreased (Figure 4A). To illustrate the effects of different environmental measures (PC1: temperature, sunlight, and humidity), we also provide a composite graph for these measures in relation to the number of individuals present at the feeding platforms throughout a 24-hour period (Figure 4B). Note that, as all of the measures are correlated with each other and load strongly on PC1, it is not possible to pinpoint one single factor (sunlight intensity, temperature, or humidity) as driving or limiting the number of individuals visiting the platforms.
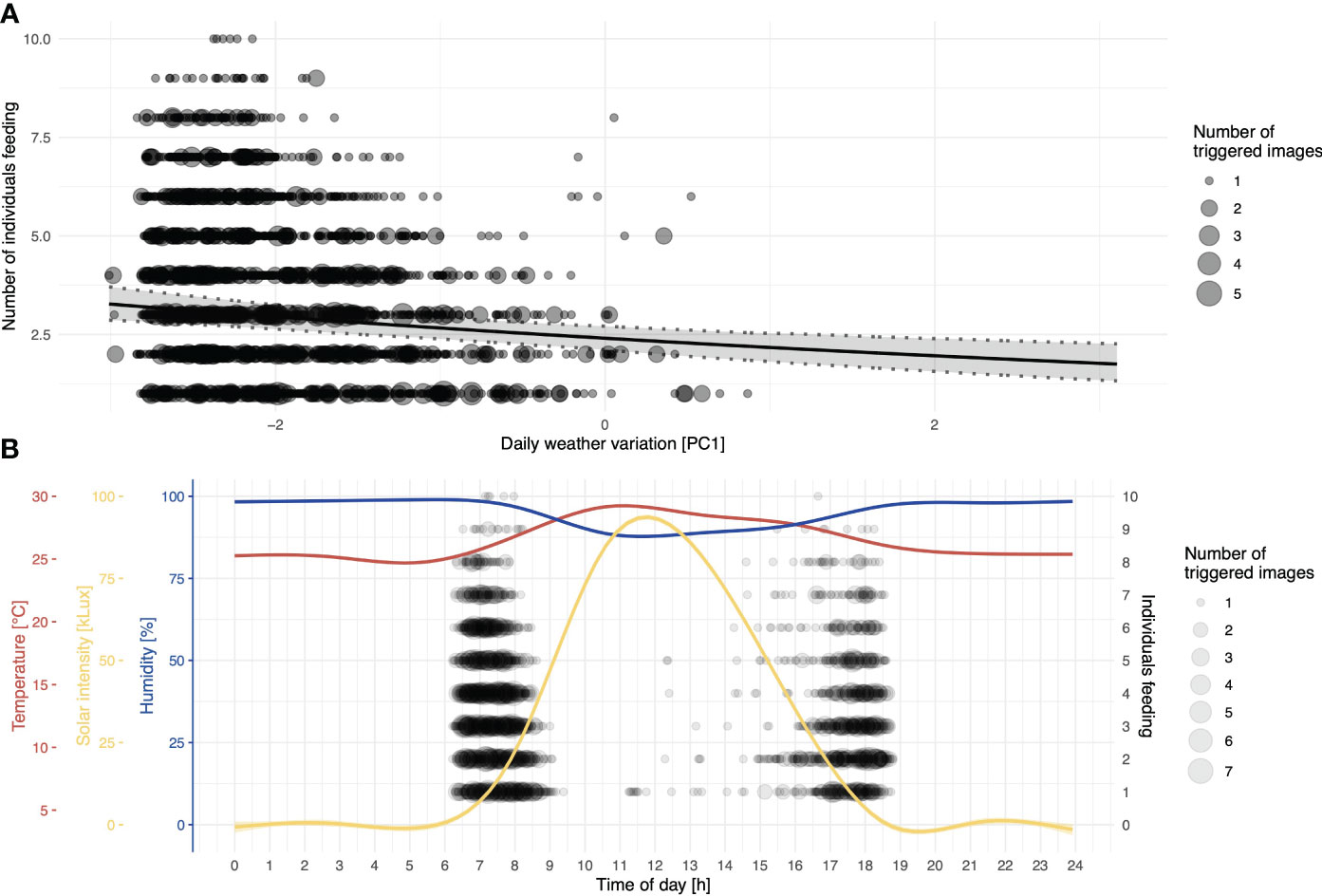
Figure 4 Numbers of individuals feeding per day in relation to daily weather variation. (A) The black line depicts the fitted model, and shaded areas represent 95% confidence intervals of model estimates based on 1000 bootstraps; the size of the dots indicates the number of images recorded at the same specific value for PC1. (B) Descriptive representation of the distribution of the number of individuals feeding at platforms as a function of time of day in hours; to show the contribution of daily weather variation, smoothed lines represent different recorded weather measures; the corresponding axes are colour-coded.
With increasing rainfall, the number of individuals visiting the platforms (triggering motion-detection cameras) significantly decreased (Figure 5A). Nevertheless, dry and wet corn seemed to be consumed at similar rates. Similarly, fewer individuals visited the platforms as the number of days since baiting increased (Figure 5B). Notably, neither wind speed nor playback affected general visitations or the number of individuals during the visitation events. Random effects of location and foraging bouts on different days showed relatively large variation compared to fixed effects, especially for the general visitations (binomial model; Table 2). Hence, visitation rates differed substantially between different sites and days (Supplementary Figure 2A), but only little variation in the maximum number of foraging individuals was found between different locations (Supplementary Figure 2B).
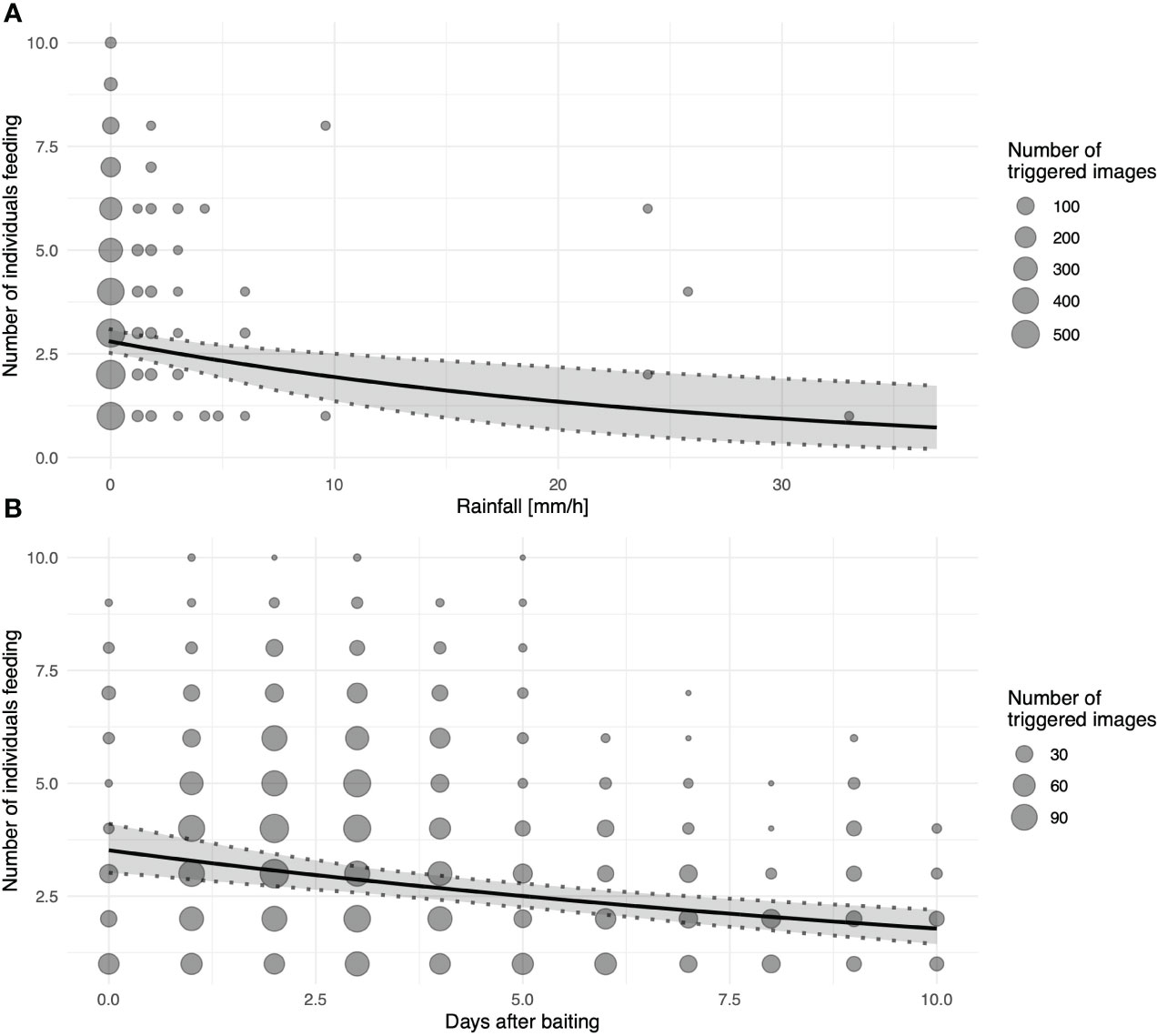
Figure 5 Number of individuals visiting platforms as a function of (A) rainfall measured as mm/h and (B) days since last baiting as an approximation of resource depletion; for both graphs, the black line depicts the fitted model, and shaded areas represent 95% confidence intervals of model estimates based on 1000 bootstraps; size of the dots indicates number of images recorded at the same specific value for PC1.
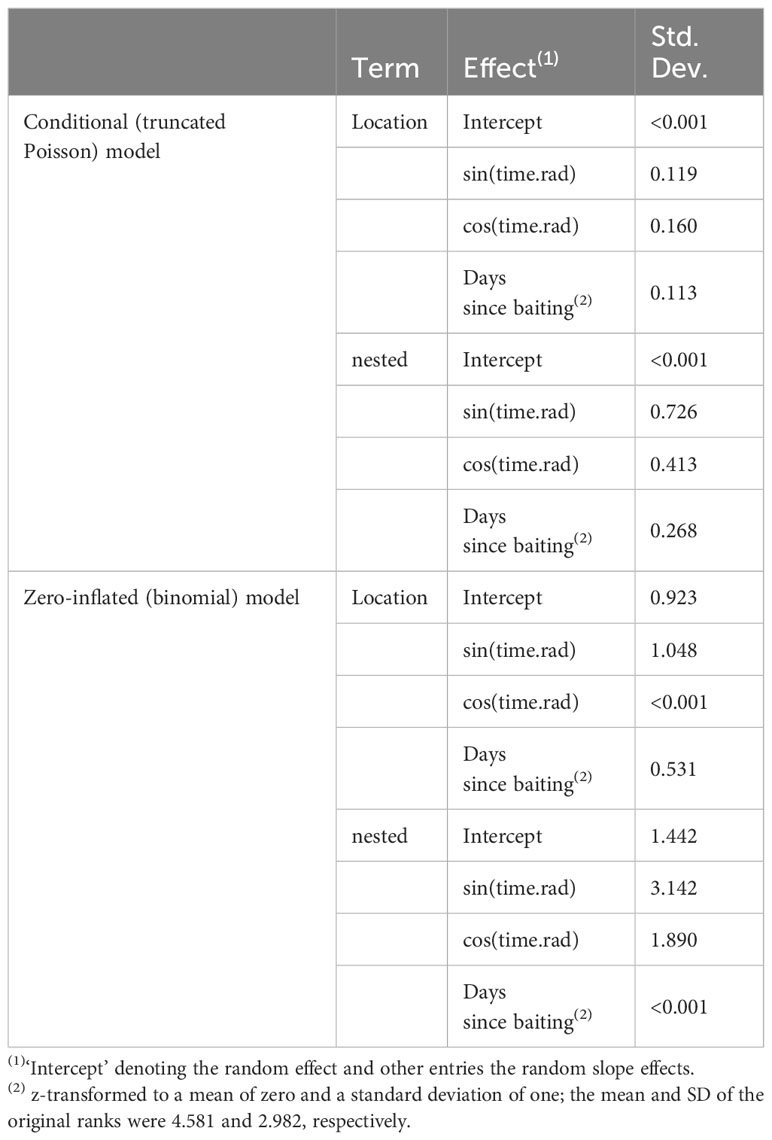
Table 2 Estimated standard deviations for the contribution of the random effects and residual standard deviation from the employed hurdle model.
4 Discussion
4.1 General discussion
The novel custom-designed feeding platforms located at forest edges successfully and reliably attracted wild, free-ranging Goffins. Importantly, as the naturalistic corn baiting schedule overlapped with the locally occurring harvest times, our approach did not disturb the Goffins’ seasonal foraging patterns or interfere with their social/family relationships. Therefore, observations collected from the feeding platforms can be considered generally indicative of wild Goffins’ behaviour on the Tanimbar Islands. Based on the obtained data, we could confirm the previously observed diurnal activity patterns consisting of two main foraging bouts in the mornings and afternoons (O’Hara et al., 2018). Psittacines generally have two main foraging bouts, in the mornings and the afternoons/evenings (for a review, see Koutsos et al., 2001), separated by a roosting period (Riley et al., 2023). Focusing foraging activities in the cooler parts of the day reduces metabolic heat production when heat dissipation is most difficult (Riley et al., 2023). For example, a similar temporal distribution of feeding patterns has been reported in Galahs (Eolophus roseicapilla; Noske, 1980) and Little corellas (Cacatua sanguinea; Hubregtse, 2020). Additionally, we observed higher visitation rates during the morning than the afternoon bouts, which resembles the foraging strategy observed in songbirds (Farine and Lang, 2013). Due to the high correlation between time of day, temperature, sunlight intensity, and humidity, it is not possible to pinpoint a single environmental factor responsible for influencing the Goffins’ behaviour.
Intriguingly, not all factors affecting group size showed the same effect on visitations in general. While increasing rainfall seemed to deter larger groups, it did not affect visitation on the feeding platforms in general, as shown by a significant effect of rainfall on the conditional, but not the zero-inflated, model. As Goffins readily consume wet corn (B.M. & M.O., personal observations), the condition of the bait itself did not contribute to the decreased visitations. Instead, we speculate that larger groups were deterred by heavy rainfall, while smaller groups (single individuals, pairs, or potentially family groups) could have been composed of individuals residing locally in the areas surrounding the feeding platforms. Similarly, a study on Echo parakeets (Psittacula echo; Tollington et al., 2019) has shown that individuals residing near feeding stations utilise them more. Therefore, it is likely that in heavy rainfall, the foraging range might be limited, leading to only resident Goffins visiting the feeding platforms. However, as we currently lack individual recognition (limited individual distinction relied on visible body differences, such as a missing toe or eye; B.M. & M.O. personal observations) and movement data on this species, this hypothesis remains to be tested. Furthermore, it is important to note that the study period coincided with the dry season and that larger variation in rainfall could lead to different foraging activity patterns.
Similarly, the days since baiting (food resource depletion) affected the number of individuals foraging at the feeding platforms but not visitations generally. The largest number of Goffins (10 individuals simultaneously on the feeding plate) was attracted shortly after baiting when most corn was available. Fresh patches provide food quickly, but the intake rate declines as foraging individuals deplete the source (Pyke, 2019). Nevertheless, even after several days and corresponding decreasing corn availability, the platforms were frequently visited by a few individuals (even after the fifth day after baiting; M = 2, min = 0, max = 7). Upon locating a reliable food source, such as sunflower crops, some cockatoo species (Galahs and White cockatoos) continually revisit that location to forage (Noske, 1980). Similarly, flocks of Goffins were observed to raid cornfields for 4–5 days on the Tanimbar Islands (Cahyadin et al., 1994a; Jepson et al., 2001). Group foraging might vary within species and individuals over time, and flocking was suggested to correlate with food patchiness, even in a dense forest habitat (Terborgh and Diamond, 1970). Forest-dwelling prey animals rely on inconspicuousness to avoid predators (for a review, see 61). However, foraging might involve trade-offs when locations with abundant/high-quality food carry higher predation risk (e.g., due to increased visibility when foraging on open agriculture fields; 10). However, in these circumstances, group foraging provides multiple anti-predatory benefits to individuals: dilution of predation risk, faster detection and early warning of predator presence, and group defence (Giraldeau and Pyke, 2019), such as mobbing (Curio, 1978; B.M. & M.O., personal observation).
Playback sessions did not have an effect on attracting Goffins to the feeding platforms. We interpreted the recorded vocalisations as contact calls because of the context in which they occurred: multiple individuals were calling during group foraging on a cornfield as further individuals were arriving. Psittacines seem to be attracted by vocalisations of foraging conspecifics, as indicated by a study on Brown-throated conures (Aratinga pertinax xanthogenesis; Buhrman-Deever et al., 2008), in which overflying flocks were more likely to arrive at a location with a foraging group calling to the approaching individuals. Loud contact calls were also proposed to coordinate fission-fusion dynamics of multiple groups during foraging instead of being simple greetings exchanged (Saunders, 1983; Balsby and Bradbury, 2009). Potential explanations for the absence of response in our study include unfamiliarity with the recorded individuals, attenuation/degradation of signals, or pseudoreplication (Rosenthal, 2019). Alternatively, the clear visibility of corn on the feeding platforms could have served as a sufficient stimulus to attract Goffins. Furthermore, as these foraging locations were potentially already known to the locally residing Goffins, playback may have been ineffective in attracting additional individuals to these locations.
Interestingly, the feeding platforms reliably attracted only Goffins, despite several other psittacine species (Blue-streaked lory, Eos reticulata; Eclectus parrot, Eclectus roratus riedeli; Great-billed parrot, Tanygnathus megalorynchos subaffinis; and Red-cheeked parrot, Geoffroyus geoffroyi timorlaoensis; Bishop and Brickle, 1998; Eaton et al., 2021) living on the Tanimbar Islands. A few instances of occasional visits by Barred doves (Geopelia maugeus) and Cinnamon-banded kingfishers (Todiramphus Australasia), as well as a single visit of a civet (Paradoxurus spp.) were recorded (none of these species appeared to feed on the corn). The other psittacine species were occasionally present within the area surrounding the feeding platforms (within 100m). However, they never landed on the platforms, and no active monopolisation by Goffins was observed. While corn might not be suitable for nectarivore and mainly frugivore species (Blue-streaked lorries and Red-cheeked parrots; Collar, 1997; Juniper and Parr, 2010), this foraging limitation should not apply to the larger psittacine species, as they can readily feed on corn in captivity (e.g., Eclectus parrot; Rachmatika et al., 2020). However, the other psittacine species on the Tanimbar Islands seem to typically forage on easily accessible food sources (such as fruit, nectar, or small seeds; Collar, 1997; Juniper and Parr, 2010) and might not need to explore novel foraging opportunities (such as agriculture fields or feeding platforms; Mioduszewska et al., 2022). A similar adaptation to anthropogenic food sources (crops or ornamental plants) by only some potential species in an area was observed in insects (for a review, see Sih et al., 2011).
The exclusive use of the feeding platforms by Goffins may be attributable to them being opportunistic feeding generalists with reduced neophobia (hand-raised individuals in a laboratory setting are explorative and mildly neophobic; O’Hara et al., 2017). The relationship between birds and their environment is evident through their active search, exploration, and response to novel habitat features, food sources, and other potential resources (Greenberg and Mettke-Hofmann, 2001). Some wild animals can adapt their foraging strategies to exploit anthropogenic habitats, such as agriculture fields (for a review, see Hockings and McLennan, 2012). Specifically, generalist species inhabiting variable environments were proposed to exploit more resources from various habitats and be more behaviourally flexible, more explorative, and less neophobic than dietary/habitat specialist species relying on more stable resources (for reviews, see Greenberg and Mettke-Hofmann, 2001; Mettke-Hofmann, 2014). In apes, provisioning success was suggested to depend on their pre-experience with foraging on anthropogenic food sources (e.g., crop-raiding; McGrew, 1992). Any novel feeding location must first be approached, explored, and sampled before the visiting individuals can assess its potential costs and benefits (Greenberg and Mettke-Hofmann, 2001). Therefore, opportunistic feeding generalists (such as Goffins) may gain more from reduced neophobia and increased exploration of potential novel foraging locations. Foraging generalism and opportunism were also proposed as one of the characteristics adaptive for utilising anthropogenic food sources in cercopithecine monkeys, a subfamily of the Old World monkeys (including baboons, Papio anubis; or vervets, Chlorocebus pygerythrus). These species are some of the most frequent primate crop raiders (for a review, see Warren et al., 2011). Similarly, many various crop-raiding species are generalist foragers (e.g., elephants, Loxodonta Africana; or racoons, Procyon lotor; for a review, see Hockings and McLennan, 2012) and introduced Rose-ringed parakeets (Psittacula krameri) were observed to use bird feeders opportunistically (Clergeau and Vergnes, 2011).
4.2 Evaluation of the feeding platforms
Fieldwork studies provide less rigorous control and involve more potentially confounding factors (e.g., weather changes or distractions) than the laboratory setting (Pritchard et al., 2016). The natural habitat of an animal was even compared to “an arena where almost all behaviours are confounded by countless variables” (p. 42; Goodall, 1986). However, field research is invaluable for understanding the evolutionary drivers of behaviours and cognition (Janmaat, 2019). Feeding platforms that can reliably attract wild psittacines offer multiple advantages for research in their natural habitat. However, certain limitations are also present, and potential risks should be carefully considered and evaluated.
Importantly, to successfully attract a target species, the design and placement of feeding stations should consider their natural behavioural routines. For example, wild New Caledonian crows were recorded foraging on the ground (Rutz et al., 2007). Therefore, low feeding tables (1m above the ground) constructed from branches/forest materials are sufficient to reliably attract this species for detailed behavioural observations (e.g., Hunt and Gray, 2004; Holzhaider et al., 2010). Wild Goffins land on the agriculture fields (Mioduszewska et al., 2018; O’Hara et al., 2018) and have even been observed descending to the ground to forage on dropped corn kernels (B.M. & M.O., personal observations). However, they are typically highly vigilant and easily startled in such circumstances. Therefore, developing elevated feeding platforms for this particular species was considered more promising for a reliable and wildlife welfare-oriented approach (Lindsjö et al., 2019; Beaulieu, 2023).
4.2.1 Advantages
Generally, research programs involving feeding wildlife contribute to our understanding of the natural world and fulfil multiple criteria promoting the welfare of studied animals (Dubois and Fraser, 2013). These include the feasibility of managing the feeding schedules and the fact that relatively few animals are affected during a relatively small portion of their lifespan. As feeding research projects are generally short-term, they do not permanently disrupt natural foraging patterns (avoid permanent food-conditioning). The main advantage of baited locations is the enhanced opportunities for close observations of wild, free-ranging animals. However, it was suggested that behavioural data collected through this method do not reflect natural foraging conditions (McGrew, 1992; Rutz et al., 2007). Although providing corn on the feeding platforms temporarily affected Goffins’ activity patterns, the baiting schedule resembled the natural harvest times on the Tanimbar Islands. The presence of Goffin aggregations on agriculture fields and their reported corn consumption (Cahyadin et al., 1994b) confirm the ecological validity of using feeding platforms baited with corn to attract wild Goffins.
The naturalistic baiting schedule also did not modify the abundance of a valuable, ephemeral food source. Therefore, it did not create dependency on the feeding platforms among the local Goffin population. Even species relying on anthropogenic food sources for most of their diet maintain wild-foraging skills (McKinney, 2011). Importantly, our approach did not introduce a novel food source (corn has been present on the Tanimbar Islands since around the 16th century; Pesireron et al., 2021) and did not modify the behavioural repertoire of wild Goffins. Regarding the nutrition of crop-raiding psittacines, Carnaby’s cockatoos feeding on canola seem to avoid increasing foraging on agriculture fields relative to native vegetation (Riley et al., 2023). Therefore, at least some psittacines seem to regulate the intake of specific dietary nutrients by managing the proportional consumption of different types of food in their diet (e.g., high-energy anthropogenic crops vs high-protein native seeds).
The suitability of free-ranging animals as research candidates in the wild depends on their reliability, observability, and amenability (Pritchard et al., 2016). Reliable species can be encountered on multiple occasions and exhibit the studied behaviour frequently enough, thus allowing adequate data collection. Observable animals can be identified, and their behaviours can be recorded, whereas amenability relates to their willing participation in field experiments (motivation to engage with provided tasks). The novel design of feeding platforms allows for the reliable recording of wild Goffins’ behaviours. Goffins are also likely amenable as multiple individuals manipulated the artificial elements of the setup (plastic feeding plates or elements of the hoisting system). However, more research is needed on how wild individuals react to novel objects and tasks provided on the platforms. Furthermore, feeding platforms may facilitate the investigation of mechanisms underlying complex behaviours (such as tool use) and their social transmission under free-ranging conditions (which might not easily be reproduced in a laboratory setting; Pritchard et al., 2016).
4.2.2 Limitations and potential risks
Limitations of the presented approach relate to the context specificity, generalizability, lack of control over the number of visiting individuals, and interferences. Feeding platforms, by definition, attract only individuals engaged in foraging. Therefore, they are unsuitable for observing other behaviours, such as breeding or movement patterns. In terms of generalisability, conclusions derived from this study might apply only to Goffins experienced with anthropogenic food sources, either wild or introduced in different locations. Groups predominantly dwelling deep in the primary forest may be less familiar with agriculture fields and react differently to artificial foraging opportunities. Furthermore, the feeding platforms were strategically positioned in clearings at the forest edges to ensure their conspicuousness. The current design might not effectively attract Goffins within the forest due to restricted visibility in the dense vegetation (developing platforms suspended from the trees might offer a more promising approach). A further challenge emerges as the number of visiting Goffins cannot be controlled. Goffins seem to form fission-fusion flocks (O’Hara et al., 2018) and, as the majority of psittacine species (Bradbury, 2003), are likely non-territorial (B.M. & M.O., personal observations). Therefore, the feeding platforms can attract either few individuals or large groups (>10; e.g., during the first days after baiting) instead of consistently attracting smaller family units as is the case in less social (e.g., New Caledonian crows; Holzhaider et al., 2011) or territorial (e.g., Common buzzards; Mioduszewska et al., 2021) species. This variability in the number of visiting individuals holds significant implications for the type of research questions that can be posed, with the current design bound to group-level paradigms. Finally, due to the free-ranging aspect of the setup, intra- and inter-specific disturbances may occur during data collection. These challenges inherent to all fieldwork studies (for a review, see Pravosudov, 2022) cannot be controlled and should be considered during the preparatory phase.
Regarding potential risks, transmission of pathogens is a concern associated with animal aggregations at supplementary food sources (through artificially concentrating individuals and directly/indirectly increasing contact rates; IUCN/SSC, 2013; Sorensen et al., 2014; Fogell, 2019). Although the feeding platforms elicit natural patterns of Goffin flocking observed at agriculture fields on the Tanimbar Islands (Cahyadin et al., 1994a; Jepson et al., 2001), the density of individuals is higher on a feeding platform (artificially clustered food source) than on trees bordering agriculture fields. Regarding the biosecurity protocol, although the feeding platforms were constructed from durable materials that facilitated easy cleaning (plastic feeding plates, hardwood base), removing food remnants was restricted to the rebaiting events. However, between cleaning, we could also rely on the strong UV radiation of the sun for daily disinfection. The presence of the PBFD virus on supplemental feeding stations used for Echo parakeets endemic to the Mauritius islands (Fogell, 2019) might stem from a potential lack of continuous intense UV radiation and the stations’ design. Each feeding station consisted of two main parts: feeders (closed with lids to prevent water from entering) and perches. Feeders accumulated significantly more virions than their associated perches, and the most closed/sheltered design had the largest PBFD load. Importantly, viral accumulation was not progressive (attributed to exposure to wind and rain that might naturally remove infected feather dust) and was not influenced by the density of individuals visiting the feeding stations. The open design of the feeding platforms used in this study did not prevent UV from reaching both the feeding and perching areas, and it allowed for the potential cleaning effects of wind and rain. Furthermore, food on the feeding platforms was not consistently provided throughout the year (as with Echo parakeets), thus further reducing the potential risk of pathogen transmission. Finally, as the feeding platforms attracted mainly Goffins, there was a limited risk of spreading pathogens across avian species, a common concern related to other wildlife feeding methods (e.g., bird feeders; Becker et al., 2018).
4.2.3 Alternative approaches
Alternative approaches to constructing feeding platforms to facilitate behavioural observations include monitoring agriculture fields or building capture-release aviaries. Close-range behavioural observations of free-ranging Goffins could be conducted on local agriculture fields, where visibility is less limited by dense vegetation. However, such data collection may induce an observer effect and deploying motion-detection cameras could be challenging due to difficulties in predicting the exact location where individuals will aggregate. Furthermore, observations from a distance provide limited behavioural details and can often lead to conflicts with local farmers (who often either trap or scare Goffins away to protect crops). Thus, conducting behavioural observations or providing cognitive tests on the agriculture fields entails the risk of human interference. Additionally, local farmers may also decide to switch to crops that are less attractive to Goffins (such as chillies or bananas; B.M. & M.O., personal observation), and the presence of cornfields and harvesting times are becoming increasingly unpredictable due to variable weather (potentially due to climate change; for reviews, see Loo et al., 2015; Praveen and Sharma, 2019).
Another alternative approach is the use of field capture-release aviaries, which provide the opportunity to conduct detailed observations of temporarily-captive wild birds in semi-controlled and semi-naturalistic conditions within their natural surroundings (e.g., New Caledonian crows; Klump et al., 2015; St Clair et al., 2018; Goffins; Rössler et al., 2020; O’Hara et al., 2021). This method allows researchers to systematically investigate the behaviour and cognitive abilities of wild individuals, although in unnatural captive conditions that are susceptible to interference from the external environment (i.e., changeable weather, vocalising nearby conspecifics and heterospecifics) and deprive individuals of the freedom of movement and social interactions (McGrew, 1992). Furthermore, constructing field aviaries is not always feasible (logistically and financially) or desirable (difficulty in capturing the target species, potentially splitting mated pairs/families, biosafety concerns). This approach also does not exclude the potential for a captivity bias (Haslam, 2013), where both short- and long-term captivity might impact the behaviour and performance of subjects (for a review, see Rössler et al., 2020). However, field and captive studies could complement each other (Rutz, 2018). Experimental setups on the feeding platforms can be used to assess the ecological validity of cognitive findings from captivity in a free-ranging environment. Conversely, field aviaries provide an opportunity for more controlled setups.
4.3 Future directions
The next step for collecting behavioural data and conducting cognitive tests on the feeding platforms is establishing individual recognition. The identification and quantification of cognitive abilities within a population rely on repeated recording of the performance of the same individuals (Pritchard et al., 2016). Automated individual recognition systems, such as Radio Frequency Identification (RFID) tags (e.g., Farine and Lang, 2013; Aplin et al., 2015) or application of QR-tags, would allow identification of individuals repeatedly feeding in the same area (home ranges), participating in social interactions and engaging with provided cognitive tasks. Footage of tagged individuals may further act as a training set for an open-box convolutional neural network, which can subsequently be applied to identify untagged individuals (Ferreira et al., 2020). Additionally, the use of Artificial Intelligence (AI) for behavioural scoring (Bohnslav et al., 2021) has the potential to provide a mostly automated data collection system. Individual identification would also benefit population monitoring, as it was suggested that investigations of population dynamics should also include models of foraging by individuals (for a review, see Pyke, 2019).
Conservation of this species could also benefit from developing an individual recognition system. Social dynamics may offer valuable information about broader species trends, with demographic measures crucial in evaluating a species’ overall vitality and potential future population trends (Anders and Marshall, 2005; Mills, 2013). Long-term monitoring over wet and dry seasons, as well as breeding and non-breeding seasons (e.g., Cameron, 2005; Berg et al., 2007; Contreras-González et al., 2009; De Labra-Hernández and Renton, 2019), would further provide information on seasonal changes in the population and foraging patterns (likely affected by the distribution and abundance of food; Noske, 1980), as well as motivation to explore experimental tasks. Feeding platforms could also be used in behavioural research laboratory facilities that house groups of psittacines to allow a standardised, direct comparison between the behaviours and cognitive skills of captive and wild populations.
5 Conclusions
Fieldwork studies are fundamental for extending phylogenetic comparisons across the animal kingdom, ultimately contributing to a deeper understanding of the factors influencing the evolution of behaviours/cognitive skills (Janmaat, 2019). However, “observation in nature is really negotiation” (p. 34; McGrew, 1992) between researchers and the target species. This study offers a novel feeding platform design and a naturalistic baiting schedule for reliably attracting wild psittacine species that are opportunistic feeding generalists. It offers an approach alternative to captive studies by enabling research on behaviour and cognitive skills in an ecologically valid setting. Studies combining behavioural and weather data can also provide insights about avian responses to climate change (Mainwaring et al., 2021). However, potential ethical considerations should be thoroughly evaluated on a species-by-species basis to assess the feasibility and potential benefits of implementing feeding platforms. We hope to stimulate field research on wild, free-ranging psittacines in their natural environments and consider the presented approach a promising platform for unveiling behavioural and cognitive treasures hidden in the dense tropical forest.
Data availability statement
The datasets presented in this study can be found in online repositories. The names of the repository/repositories and accession number(s) can be found below: https://github.com/Mark-OHara/Goffin_visitation_rates_on_platforms.
Ethics statement
Our research plans were reviewed and approved (Ref No.: 047/KE.02/SK/9/2022) by the Animal Research Ethics Committee of the Indonesian National Research and Innovation Agency (NRIA), and all our research activities were following the Indonesian Ministry of Environment and Forestry Regulation No. 92 of 2018.
Author contributions
MO: Conceptualization, Data curation, Formal analysis, Funding acquisition, Investigation, Methodology, Project administration, Visualization, Writing – original draft, Writing – review & editing, Resources. AA: Funding acquisition, Resources, Writing – review & editing. DP: Project administration, Writing – review & editing. LH: Supervision, Writing – review & editing. BM: Conceptualization, Data curation, Investigation, Methodology, Project administration, Visualization, Writing – original draft, Writing – review & editing.
Funding
The author(s) declare financial support was received for the research, authorship, and/or publication of this article. This research was funded in whole by the Messerli Foundation and the Austrian Science Fund (FWF) grants awarded to MO (FWF project: J4169) and AA (FWF START project: Y01309).
Acknowledgments
We are grateful to the Republic of Indonesia for allowing us to conduct this research and acknowledge the National Research and Innovation Agency for granting this permission (No.: 191/SIP/IV/FR/9/2022 and 192/SIP/IV/FR/9/2022). We thank the Research Centre for Biosystematics and Evolution of the National Research and Innovation Agency (Badan Riset dan Inovasi Nasional; BRIN) as the supporting institution. We thank the Natural Resources Conservation Agency (Balai Konservasi Sumber Daya Alam; BKSDA) in Maluku and its regional office in Saumlaki (Johan M. Nendissa, Franston L. Kunu, Cardolin Latuputy). We are also grateful to the members of the Hurlatu clan (Wilhelmus Samangun, Vera Samangun, Petrus Sainyakit, Yakobus Sainyakit) from Lorulun village for their support in the field. We thank Theresa Rössler, Peter Füreder, and Gerlinde Hofstetter for fruitful discussions and Remco Folkertsma for statistical advice. Chat Generative Pre-Training Transformer (ChatGPT; default: GPT–3.5; OpenAI, 2023) was used during the editing of the draft (used prompts: “rephrase”, “synonym”, “check stylistics”).
Conflict of interest
The authors declare that the research was conducted in the absence of any commercial or financial relationships that could be construed as a potential conflict of interest.
Publisher’s note
All claims expressed in this article are solely those of the authors and do not necessarily represent those of their affiliated organizations, or those of the publisher, the editors and the reviewers. Any product that may be evaluated in this article, or claim that may be made by its manufacturer, is not guaranteed or endorsed by the publisher.
Supplementary material
The Supplementary Material for this article can be found online at: https://www.frontiersin.org/articles/10.3389/fevo.2023.1275949/full#supplementary-material
References
Anders A. D., Marshall M. R. (2005). Increasing the accuracy of productivity and survival estimates in assessing landbird population status. Conserv. Biol. 19 (1), 66–74. doi: 10.1111/j.1523-1739.2005.00543.x
Aplin L. M., Farine D. R., Morand-Ferron J., Cockburn A., Thornton A., Sheldon B. C. (2015). Experimentally induced innovations lead to persistent culture via conformity in wild birds. Nature 518 (7540), 538–541. doi: 10.1038/nature13998
Aplin L. M., Major R. E., Davis A., Martin J. M. (2021). A citizen science approach reveals long-term social network structure in an urban parrot, Cacatua galerita. J. Anim. Ecol. 90 (1), 222–232. doi: 10.1111/1365-2656.13295
Auersperg A. M. I., von Bayern A. M. P. (2019). Who’s a clever bird — now? A brief history of parrot cognition. Behaviour 156 (5–8), 391–407. doi: 10.1163/1568539X-00003550
Balsby T. J. S., Bradbury J. W. (2009). Vocal matching by orange-fronted conures (Aratinga canicularis). Behav. Processes. 82 (2), 133–139. doi: 10.1016/j.beproc.2009.05.005
Barr D. J., Levy R., Scheepers C., Tily H. J. (2013). Random effects structure for confirmatory hypothesis testing: Keep it maximal. J. Mem Lang. 68 (3), 255–278. doi: 10.1016/j.jml.2012.11.001
Beaulieu M. (2023). Capturing wild animal welfare: A physiological perspective. Biol. Rev., brv.13009. doi: 10.1111/brv.13009
Beck H. E., Zimmermann N. E., McVicar T. R., Vergopolan N., Berg A., Wood E. F. (2018). Present and future Köppen-Geiger climate classification maps at 1-km resolution. Sci. Data. 5 (1), 180214. doi: 10.1038/sdata.2018.214
Becker D. J., Hall R. J., Forbes K. M., Plowright R. K., Altizer S. (2018). Anthropogenic resource subsidies and host–parasite dynamics in wildlife. Philos. Trans. R Soc. B Biol. Sci. 373 (1745), 20170086. doi: 10.1098/rstb.2017.0086
Beissinger S. R., Snyder N. F. R. (1992). New World parrots in crisis (Washington, D.C.: Smithsonian Institution Press).
Berg K. S., Delgado S., Okawa R., Beissinger S. R., Bradbury J. W. (2011). Contact calls are used for individual mate recognition in free-ranging green-rumped parrotlets, Forpus passerinus. Anim. Behav. 81 (1), 241–248. doi: 10.1016/j.anbehav.2010.10.012
Berg K. S., Socola J., Angel R. R. (2007). Great Green Macaws and the annual cycle of their food plants in Ecuador. J. Field Ornithol. 78 (1), 1–10. doi: 10.1111/j.1557-9263.2006.00080.x
Bishop K. D., Brickle N. W. (1998). An annotated checklist of the birds of the Tanimbar Islands. Kukila 10, 115–150.
Bohnslav J. P., Wimalasena N. K., Clausing K. J., Dai Y. Y., Yarmolinsky D. A., Cruz T., et al. (2021). DeepEthogram, a machine learning pipeline for supervised behavior classification from raw pixels. eLife 10, e63377. doi: 10.7554/eLife.63377.sa2
Bomford M. (1992). “Review of research on control of bird pests in Australia,” in Proceedings of the Fifteenth Vertebrate Pest Conference. 93–96.
Bond A., Diamond J. (2019). Thinking like a parrot: Perspectives from the wild (Chicago, IL: University of Chicago Press).
Bradbury J. W. (2003). “Vocal communication in wild parrots,” in Animal social complexity: Intelligence, culture and individualized societies. Eds. de Waal F. B. M., Tyack P. L. (Cambridge, MA: Harvard University Press), 293–316.
Bradbury J. W., Vehrencamp S. L. (2011). “Social integration,” in Principles of animal communication. 2nd ed (Sunderland, MA: Sinauer Associates), 515–560.
Brightsmith D. J. (2004). Effects of weather on parrot geophagy in Tambopata, Peru. Wilson Bull. 116 (2), 134–145. doi: 10.1676/03-087B
Brooks M. E., Kristensen K., van Benthem K. J., Magnusson A., Berg C. W., Nielsen A., et al. (2017a). Modeling zero-inflated count data with glmmTMB. bioRxiv, 132753. doi: 10.1101/132753
Brooks M. E., Kristensen K., van Benthem K. J., Magnusson A., Berg C. W., Nielsen A., et al. (2017b). glmmTMB balances speed and flexibility among packages for zero-inflated generalized linear mixed modeling. R J. 9 (2), 378–400. doi: 10.32614/RJ-2017-066
Buhrman-Deever S. C., Hobson E. A., Hobson A. D. (2008). Individual recognition and selective response to contact calls in foraging brown-throated conures, Aratinga pertinax. Anim. Behav. 76 (5), 1715–1725. doi: 10.1016/j.anbehav.2008.08.007
Cahyadin Y., Jepson P., Manoppo B. I. (1994a). The status of Cacatua goffini and Eos reticulata on the Tanimbar Islands (Bogor, Indonesia: PHPA/BirdLife International Indonesia Programme).
Cahyadin Y., Manoppo B. I., Jepson P. (1994b). The status of Goffin’s cockatoo on the Tanimbar Islands and its impact on agriculture. Psittascene 6 (3), 14–15.
Calzada Preston C. E., Pruett-Jones S. (2021). The number and distribution of introduced and naturalized parrots. Diversity 13 (9), 412. doi: 10.3390/d13090412
Cameron M. (2005). Group size and feeding rates of Glossy Black-Cockatoos in central New South Wales. Emu — Austral Ornithol. 105 (4), 299–304. doi: 10.1071/MU04043
Clergeau P., Vergnes A. (2011). Bird feeders may sustain feral Rose-ringed parakeets Psittacula krameri in temperate Europe. Wildl Biol. 17 (3), 248–252. doi: 10.2981/09-092
Collar N. J. (1997). “Family Psittacidae (parrots),” in Handbook of the birds of the world. Vol. 4: Sandgrouse to cuckoos. Eds. del Hoyo J., Elliott A., Sargatal J. (Barcelona, Spain: Lynx Editions), 286–324.
Contreras-González A. M., Rivera-Ortíz F. A., Soberanes-González C., Valiente-Banuet A., Arizmendi M. C. (2009). Feeding ecology of Military Macaws (Ara militaris) in a semi-arid region of central México. Wilson J. Ornithol 121 (2), 384–391. doi: 10.1676/08-034.1
Coohill T. P., Sagripanti J. (2009). Bacterial inactivation by solar ultraviolet radiation compared with sensitivity to 254 nm radiation. Photochem. Photobiol. 85 (5), 1043–1052. doi: 10.1111/j.1751-1097.2009.00586.x
Curio E. (1978). The adaptive significance of avian mobbing: I. Teleonomic hypotheses and predictions. Z Für Tierpsychol. 48 (2), 175–183. doi: 10.1111/j.1439-0310.1978.tb00254.x
Das T. K. (2002). Evaluating the life cycle environmental performance of chlorine disinfection and ultraviolet technologies. Clean Technol. Environ. Policy. 4 (1), 32–43. doi: 10.1007/s10098-002-0139-x
De Labra-Hernández M. A., Renton K. (2019). Learning-by-consequence foraging model of the Northern Mealy Amazon in a modified landscape of tropical moist forest. J. Ornithol. 160 (2), 497–507. doi: 10.1007/s10336-019-01629-y
De Rosa A., Castro I., Marsland S. (2022). The acoustic playback technique in avian fieldwork contexts: A systematic review and recommendations for best practice. Ibis 164 (2), 371–387. doi: 10.1111/ibi.13033
Diamond J., Bond A. B. (1999). Kea, bird of paradox: The evolution and behavior of a New Zealand parrot (Berkeley, CA: University of California Press).
Dubois S., Fraser D. (2013). A framework to evaluate wildlife feeding in research, wildlife management, tourism and recreation. Animals 3 (4), 978–994. doi: 10.3390/ani3040978
Dunkley L., Cattet M. R. L. (2003). A comprehensive review of the ecological and human social effects of artificial feeding and baiting of wildlife. Can. Coop Wildl Health Cent Newsl Publ. 21, 1–68.
Eaton J. A., van Balen B., Brickle N., Rheindt F. E. (2021). Birds of the Indonesian Archipelago. Greater Sundas and Wallacea. 2nd ed (Barcelona, Spain: Lynx Edicions).
Edwards E. K., Mitchell N. J., Ridley A. R. (2015). The impact of high temperatures on foraging behaviour and body condition in the Western Australian Magpie Cracticus tibicen dorsalis. Ostrich 86 (1–2), 137–144. doi: 10.2989/00306525.2015.1034219
F10 Products (2016). Health and hygiene data sheet. Product: F10SC Veterinary Disinfectant (Somerset, England: F10 Products).
Fair J. M., Paul E., Jones J., Clark A. B., Davie C., Kaiser G. (2010). Guidelines to the use of wild birds in research (Washington, D.C.: Ornithological Council), 1–215.
Farine D. R., Lang S. D. J. (2013). The early bird gets the worm: Foraging strategies of wild songbirds lead to the early discovery of food sources. Biol. Lett. 9 (6), 20130578. doi: 10.1098/rsbl.2013.0578
Fedigan L. M. (2010). Ethical issues faced by field primatologists: Asking the relevant questions. Am. J. Primatol. 72 (9), 754–771. doi: 10.1002/ajp.20814
Ferreira A. C., Silva L. R., Renna F., Brandl H. B., Renoult J. P., Farine D. R., et al. (2020). Deep learning-based methods for individual recognition in small birds. Methods Ecol. Evol. 11 (9), 1072–1085. doi: 10.1111/2041-210X.13436
Fischer J. (2022). “Studying primate cognition: From the wild to captivity and back,” in Primate cognitive studies, 1st ed. Eds. Schwartz B. L., Beran M. J. (Cambridge, England: Cambridge University Press), 609–631.
Fogell D. J. (2019). Measuring the effects of supplementary feeding and biosecurity on the trajectory of a threatened avian population (Kent, England: University of Kent).
Forshaw J. M., Knight F. (2017). Vanished and vanishing parrots: Profiling extinct and endangered species (Ithaca, NY: Comstock Publishing Associates).
Forstmeier W., Schielzeth H. (2011). Cryptic multiple hypotheses testing in linear models: Overestimated effect sizes and the winner’s curse. Behav. Ecol. Sociobiol. 65 (1), 47–55. doi: 10.1007/s00265-010-1038-5
Fox J., Weisberg S. (2019). An R companion to applied regression. 3rd ed (Thousand Oaks, CA: Sage Publications).
Fraga R. E., Santos C. M. R., Silva R. S., Teixeira R. E. R., Tomazi L., Da Silva M. B., et al. (2023). Reintroduction and monitoring of the bird Amazona aestiva (Psittaciformes: Psittacidae) in Brazil. Rev. Biol. Trop. 71 (1), e53145. doi: 10.15517/rev.biol.trop..v71i1.53145
Gajdon G. K., Fijn N., Huber L. (2004). Testing social learning in a wild mountain parrot, the kea (Nestor notabilis). Anim. Learn Behav. 32 (1), 62–71. doi: 10.3758/BF03196007
Gilardi J. D., Munn C. A. (1998). Patterns of activity, flocking, and habitat use in parrots of the Peruvian Amazon. Condor. 100 (4), 641–653. doi: 10.2307/1369745
Giraldeau L. A., Pyke G. H. (2019). “Group foraging,” in Encyclopedia of animal behavior (Amsterdam, Netherlands: Elsevier), 191–200.
Godinho L., Marinho Y., Bezerra B. (2020). Performance of blue-fronted amazon parrots (Amazona aestiva) when solving the pebbles-and-seeds and multi-access-box paradigms: Ex situ and in situ experiments. Anim. Cogn. 23 (3), 455–464. doi: 10.1007/s10071-020-01347-6
Goldberg T. L., Ewald P. W. (1991). Territorial song in the Anna’s hummingbird, Calypte anna: Costs of attraction and benefits of deterrence. Anim. Behav. 42 (2), 221–226. doi: 10.1016/S0003-3472(05)80553-6
Goodall J. (1986). The chimpanzees of Gombe. Patterns of behavior (Cambridge, MA: Harvard University Press).
Greenberg R., Mettke-Hofmann C. (2001). “Ecological aspects of neophobia and neophilia in birds,” in Current Ornithology, vol. 16 . Eds. Nolan V., Thompson C. F. (Boston, MA: Springer), 119–178.
Greggor A. L., McIvor G. E., Clayton N. S., Thornton A. (2016). Contagious risk taking: Social information and context influence wild jackdaws’ responses to novelty and risk. Sci. Rep. 6 (1), 27764. doi: 10.1038/srep27764
Hangartner S., Laurila A. (2012). Effects of the disinfectant Virkon S on early life-stages of the moor frog (Rana arvalis). Amphib-Reptil 33 (3–4), 349–353. doi: 10.1163/15685381-00002837
Haslam M. (2013). ‘Captivity bias’ in animal tool use and its implications for the evolution of hominin technology. Philos. Trans. R Soc. B Biol. Sci. 368 (1630), 20120421. doi: 10.1098/rstb.2012.0421
Healy S. D., Hurly T. A. (1995). Spatial memory in rufous hummingbirds (Selasphorus rufus): A field test. Anim. Learn Behav. 23 (1), 63–68. doi: 10.3758/BF03198016
Heinsohn R., Zdenek C. N., Cunningham R. B., Endler J. A., Langmore N. E. (2017). Tool-assisted rhythmic drumming in palm cockatoos shares key elements of human instrumental music. Sci. Adv. 3 (6), e1602399. doi: 10.1126/sciadv.1602399
Hill D. A. (2017). “Provisioning,” in The international encyclopedia of primatology. Ed. Fuentes A. (Hoboken, NJ: John Wiley & Sons), 1–2.
Hockings K. J., McLennan M. R. (2012). From forest to farm: Systematic review of cultivar feeding by chimpanzees – Management implications for wildlife in anthropogenic landscapes. PLoS One 7 (4), e33391. doi: 10.1371/journal.pone.0033391
Holzhaider J. C., Hunt G. R., Gray R. D. (2010). Social learning in New Caledonian crows. Learn Behav. 38 (3), 206–219. doi: 10.3758/LB.38.3.206
Holzhaider J. C., Sibley M. D., Taylor A. H., Singh P. J., Gray R. D., Hunt G. R. (2011). The social structure of New Caledonian crows. Anim. Behav. 81 (1), 83–92. doi: 10.1016/j.anbehav.2010.09.015
Hubregtse V. (2020). Little and Long-billed Corellas feeding on seeds from cypress cones at Notting Hill, Victoria. Vic Nat. 137 (2), 48.
Hunt G. R. (2000). Tool use by the New Caledonian crow Corvus moneduloides to obtain Cerambycidae from dead wood. Emu — Austral Ornithol. 100 (2), 109–114. doi: 10.1071/MU9852
Hunt G. R., Abdelkrim J., Anderson M. G., Holzhaider J. C., Marshall A. J., Gemmell N. J., et al. (2007). Innovative pandanus-tool folding by New Caledonian crows. Aust. J. Zool. 55 (5), 291. doi: 10.1071/ZO07048
Hunt G. R., Gray R. D. (2004). Direct observations of pandanus-tool manufacture and use by a New Caledonian crow (Corvus moneduloides). Anim. Cogn. 7 (2), 114–120. doi: 10.1007/s10071-003-0200-0
Hunt G. R., Gray R. D. (2007). Parallel tool industries in New Caledonian crows. Biol. Lett. 3 (2), 173–175. doi: 10.1098/rsbl.2006.0603
Hunt G. R., Gray R. D., Taylor A. H. (2013). “Why is tool use rare in animals?,” in Tool use in animals. Eds. Sanz C., Call J., Boesch C. (Cambridge, England: Cambridge University Press), 89–118.
Hunt G. R., Rutledge R. B., Gray R. D. (2006). The right tool for the job: What strategies do wild New Caledonian crows use? Anim. Cognit. 9 (4), 307–316. doi: 10.1007/s10071-006-0047-2
IUCN/SSC (2013). Guidelines for reintroductions and other conservation translocations (Gland, Switzerland: IUCN Species Survival Commission).
Janmaat K. R. L. (2019). What animals do not do or fail to find: A novel observational approach for studying cognition in the wild. Evol. Anthropol Issues News Rev. 28 (6), 303–320. doi: 10.1002/evan.21794
Jepson P., Brickle N., Chayadin Y. (2001). The conservation status of Tanimbar corella and blue-streaked lory on the Tanimbar Islands, Indonesia: Results of a rapid contextual survey. Oryx 35 (3), 224–233. doi: 10.1046/j.1365-3008.2001.00179.x
Jones C. G. (2004). “Conservation management of endangered birds,” in Bird ecology and conservation: A handbook of techniques. Eds. Sutherland W. J., Newton I., Green R. (Oxford, England: Oxford University Press), 269–302.
Juniper T., Parr M. (2010). Parrots: A guide to the parrots of the world (New Haven, CT: Yale University Press). Original work published 1998.
Klump B. C., Martin J. M., Wild S., Hörsch J. K., Major R. E., Aplin L. M. (2021). Innovation and geographic spread of a complex foraging culture in an urban parrot. Science 373 (6553), 456–460. doi: 10.1126/science.abe7808
Klump B. C., van der Wal J. E. M., St Clair J. J. H., Rutz C. (2015). Context-dependent ‘safekeeping’ of foraging tools in New Caledonian crows. Proc. R Soc. B Biol. Sci. 282 (1808), 20150278.
Kondo N., Watanabe S. (2009). Contact calls: Information and social function. Jpn Psychol. Res. 51 (3), 197–208. doi: 10.1111/j.1468-5884.2009.00399.x
Koutsos E. A., Matson K. D., Klasing K. C. (2001). Nutrition of birds in the order Psittaciformes: A review. J. Avian Med. Surg. 15 (4), 257–275. doi: 10.1647/1082-6742(2001)015[0257:NOBITO]2.0.CO;2
Laumonier Y., Nasi R. (2018). The last natural seasonal forests of Indonesia: Implications for forest management and conservation. Appl. Veg Sci. 21 (3), 461–476. doi: 10.1111/avsc.12377
Li P., Feng Z., Jiang L., Liao C., Zhang J. (2014). A review of swidden agriculture in southeast Asia. Remote Sens. 6 (2), 1654–1683. doi: 10.3390/rs6021654
Lindsjö J., Cvek K., Spangenberg E. M. F., Olsson J. N. G., Stéen M. (2019). The dividing line between wildlife research and management — implications for animal welfare. Front. Vet. Sci. 6, 1–5. doi: 10.3389/fvets.2019.00013
Loepelt J., Shaw R. C., Burns K. C. (2016). Can you teach an old parrot new tricks? Cognitive development in wild kaka (Nestor meridionalis). Proc. R Soc. B Biol. Sci. 283 (1832), 20153056. doi: 10.1098/rspb.2015.3056
Loo Y. Y., Billa L., Singh A. (2015). Effect of climate change on seasonal monsoon in Asia and its impact on the variability of monsoon rainfall in Southeast Asia. Geosci Front. 6 (6), 817–823. doi: 10.1016/j.gsf.2014.02.009
Lytle C. D., Sagripanti J. L. (2005). Predicted inactivation of viruses of relevance to biodefense by solar radiation. J. Virol. 79 (22), 14244–14252. doi: 10.1128/JVI.79.22.14244-14252.2005
Mainwaring M. C., Nord A., Sharp S. P. (2021). Editorial: The impact of weather on the behavior and ecology of birds. Front. Ecol. Evol. 9, 777478. doi: 10.3389/fevo.2021.777478
McGraw W. S., Zuberbühler K. (2007). “The monkeys of the Taï Forest: An introduction,” in Monkeys of the Taï Forest: An African primate community. Eds. McGraw W. S., Zuberbühler K., Noë R. (Cambridge, England: Cambridge University Press).
McGrew W. C. (1992). “Studying chimpanzees,” in Chimpanzee material culture: Implications for human evolution (Cambridge, England: Cambridge University Press), 15–39.
McKinney T. (2011). The effects of provisioning and crop-raiding on the diet and foraging activities of human-commensal white-faced capuchins (Cebus capucinus). Am. J. Primatol. 73 (5), 439–448. doi: 10.1002/ajp.20919
Menteri Lingkungan Hidup dan Kehutanan (2018). Peraturan Menteri Lingkungan Hidup dan Kehutanan Republik Indonesia Nomor P.92/MENLHK SETJEN/KUM.1/8/2018 tentang perubahan atas peraturan Menteri Lingkungan Hidup dan Kehutanan Nomor P.20/MENLHK/SETJEN/KUM.1/6/2018 tentang jenis tumbuhan dan satwa yang dilindungi. (Jakarta, Indonesia: Menteri Lingkungan Hidup dan Kehutanan), 1–29.
Mettke-Hofmann C. (2014). Cognitive ecology: Ecological factors, life-styles, and cognition. Wiley Interdiscip Rev. Cognit. Sci. 5 (3), 345–360. doi: 10.1002/wcs.1289
Mills L. S. (2013). Conservation of wildlife populations: Demography, genetics, and management. 2nd ed (Hoboken, NJ: Wiley-Blackwell).
Mioduszewska B., Auersperg A. M. I., O’Hara M. (2022). Treasure islands: Foraging ecology and the emergence of tool use in wild Goffin’s cockatoos. Curr. Opin. Behav. Sci. 45, 101118. doi: 10.1016/j.cobeha.2022.101118
Mioduszewska B., O’Hara M., Haryoko T., Auersperg A. M. I., Huber L., Prawiradilaga D. M. (2018). Notes on ecology of wild Goffin’s cockatoo in the late dry season with emphasis on feeding ecology. Treubia 45, 85–102. doi: 10.14203/treubia.v45i0.3706
Mioduszewska B., O’Hara M., Rheindt F. E., Auersperg A. M. I. (2023). Dynamic object–fruit combinations by introduced Tanimbar corellas (Cacatua goffiniana) in Singapore. Behaviour 160 (8-9), 837–856. doi: 10.1163/1568539X-bja10231
Mioduszewska B., O’Hara M., Stryjek R. (2021). Novelty at dawn: Exploration, low neophobia and crepuscular activity in a wild Common Buzzard (Buteo buteo). Avian Biol. Res. 14 (4), 143–148. doi: 10.1177/17581559211052422
Monk K. A., De Fretes Y., Reksodiharjo-Lilley G. (1997). The ecology of Nusa Tenggara and Maluku (Singapore: Periplus Editions).
Morand-Ferron J., Cole E. F., Quinn J. L. (2016). Studying the evolutionary ecology of cognition in the wild: A review of practical and conceptual challenges. Biol. Rev. 91 (2), 367–389. doi: 10.1111/brv.12174
Nicastro F., Sironi G., Antonello E., Bianco A., Biasin M., Brucato J. R., et al. (2021). Solar UV-B/A radiation is highly effective in inactivating SARS-CoV-2. Sci. Rep. 11 (1), 14805. doi: 10.1038/s41598-021-94417-9
Nieuwenhuis R. (2012). “influence.ME: Tools for detecting influential data in mixed effects models,” in R J. 4 (2), 38–47.
Noske S. (1980). Aspects of the behaviour and ecology of the White cockatoo (Cacatua galerita) and Galah (C. roseicapilla) in croplands in North-East New South Wales (Armidale, Australia: University of New England).
Norwegian Scientific Committee for Food and Environment (2020). VKM Report: Status and trade assessment of parrots listed in CITES Appendix I (Oslo, Norway: Norwegian Scientific Committee for Food and Environment; VKM), 1–129.
O’Connell A. F., Nichols J. D., Karanth K. U. (Eds.) (2011). Camera traps in animal ecology: Methods and analyses (Tokyo, Japan: Springer).
O’Hara M., Mioduszewska B., Haryoko T., Prawiradilaga D. M., Huber L., Auersperg A. M. I. (2018). Extraction without tooling around – the first comprehensive description of the foraging- and socio-ecology of wild Goffin’s cockatoos (Cacatua goffiniana). Behaviour 156 (5–8), 661–690. doi: 10.1163/1568539X-00003523
O’Hara M., Mioduszewska B., Mundry R., Yohanna, Haryoko T., Rachmatika R., et al. (2021). Wild Goffin’s cockatoos flexibly manufacture and use tool sets. Curr. Biol. 31 (20), 4512–4520. doi: 10.1016/j.cub.2021.08.009
O’Hara M., Mioduszewska B., von Bayern A., Auersperg A., Bugnyar T., Wilkinson A., et al. (2017). The temporal dependence of exploration on neotic style in birds. Sci. Rep. 7 (1), 4742. doi: 10.1038/s41598-017-04751-0
Olah G., Butchart S. H. M., Symes A., Guzmán I. M., Cunningham R., Brightsmith D. J., et al. (2016). Ecological and socio-economic factors affecting extinction risk in parrots. Biodivers Conserv. 25 (2), 205–223. doi: 10.1007/s10531-015-1036-z
Perry S., Manson J. H. (2008). Manipulative monkeys: The capuchins of Lomas Barbudal (Cambridge, MA: Harvard University Press).
Pesireron M., Senewe R. E., Gaffar A., Yusuf (2021). The characteristics of Tanimbar Island local maize in Maluku Province. IOP Conf Ser. Earth Environ. Sci. 648 (1), 012048. doi: 10.1088/1755-1315/648/1/012048
Pitter E., Christiansen M. B. (1995). Ecology, status and conservation of the Red-fronted Macaw Ara rubrogenys. Bird Conserv. Int. 5 (1), 61–78. doi: 10.1017/S0959270900002951
Praveen B., Sharma P. (2019). A review of literature on climate change and its impacts on agriculture productivity. J. Public Aff. 19 (4), e1960. doi: 10.1002/pa.1960
Pravosudov V. V. (2022). Cognitive ecology in the wild — advances and challenges in avian cognition research. Curr. Opin. Behav. Sci. 45, 101138. doi: 10.1016/j.cobeha.2022.101138
Presiden Republik Indonesia (1999a). Peraturan Pemerintah Republik Indonesia Nomor 7 Tahun 1999 tentang pengawetan jenis tumbuhan dan satwa (Jakarta, Indonesia: Presiden Republik Indonesia), 1–26.
Presiden Republik Indonesia (1999b). Jenis-jenis tumbuhan dan satwa yang dilindungi (Jakarta, Indonesia: Presiden Republik Indonesia), 1–26.
Pritchard D. J., Hurly T. A., Tello-Ramos M. C., Healy S. D. (2016). Why study cognition in the wild (and how to test it)? J. Exp. Anal. Behav. 105 (1), 41–55. doi: 10.1002/jeab.195
Pyke G. H. (2019). “Optimal foraging theory: An introduction,” in Encyclopedia of animal behavior (Amsterdam, Netherlands: Elsevier), 111–117.
Rachmatika R., Prijono S. N., Sari A. P., Suparno S. (2020). Chick growth and nutrient requirement during rearing period on eclectus parrot (Eclectus roratus, Müller 1776). BIO Web of Conferences 19, 00013. doi: 10.1051/bioconf/20201900013
R Core Team (2023). R: A language and environment for statistical computing. (Vienna, Austria: R Foundation for Statistical Computing).
Rehfuess E. (2002). Global solar UV index: A practical guide (Geneva, Switzerland: World Health Organization).
Riley K. J., Warren K., Armstrong N., Yeap L., Dawson R., Mawson P. R., et al. (2023). Accelerometry reveals limits to use of an energy-saving anthropogenic food source by a threatened species: A case of Carnaby’s cockatoos (Zanda latirostris) and canola. Ecol. Evol. 13 (10), e10598. doi: 10.1002/ece3.10598
Rosati A. G., Machanda Z. P., Slocombe K. E. (2022). Cognition in the wild: Understanding animal thought in its natural context. Curr. Opin. Behav. Sci. 47, 101210. doi: 10.1016/j.cobeha.2022.101210
Rosenthal G. G. (2019). “The use of playbacks in behavioral experiments,” in Encyclopedia of animal behavior (Amsterdam, Netherlands: Elsevier), 529–534.
Rössler T., Mioduszewska B., O’Hara M., Huber L., Prawiradilaga D. M., Auersperg A. M. I. (2020). Using an Innovation Arena to compare wild-caught and laboratory Goffin’s cockatoos. Sci. Rep. 10 (1), 8681.
Rovero F., Zimmermann F. (Eds.) (2016). Camera trapping for wildlife research (Exeter, England: Pelagic Publishing).
Rutz C. (2018). “Studying corvids in lab and field,” in Field and laboratory methods in animal cognition: A comparative guide. Eds. Bueno-Guerra N., Amici F. (Cambridge, England: Cambridge University Press), 127–129. doi: 10.1126/science.1146788
Rutz C., Bluff L. A., Weir A. A. S., Kacelnik A. (2007). Video cameras on wild birds. Science 318 (5851), 765–765.
Saunders D. A. (1983). Vocal repertoire and individual vocal recognition in the short-billed white-tailed black cockatoo, Calyptorhynchus funereus latirostris Carnaby. Wildl Res. 10 (3), 527–536. doi: 10.1071/WR9830527
Schielzeth H. (2010). Simple means to improve the interpretability of regression coefficients. Methods Ecol. Evol. 1 (2), 103–113. doi: 10.1111/j.2041-210X.2010.00012.x
Schielzeth H., Forstmeier W. (2009). Conclusions beyond support: Overconfident estimates in mixed models. Behav. Ecol. 20 (2), 416–420. doi: 10.1093/beheco/arn145
Schindelin J., Arganda-Carreras I., Frise E., Kaynig V., Longair M., Pietzsch T., et al. (2012). Fiji: An open-source platform for biological-image analysis. Nat. Methods 9 (7), 676–682. doi: 10.1038/nmeth.2019
Schneider L., Serbena A. L., Guedes N. M. R. (2006). Behavioral categories of hyacinth macaws (Anodorhynchus hyacinthinus) during the reproductive period, at South Pantanal, Brazil. Rev. Etol. 8 (2), 71–80.
Shaw R. C., Boogert N. J., Clayton N. S., Burns K. C. (2015). Wild psychometrics: Evidence for ‘general’ cognitive performance in wild New Zealand robins, Petroica longipes. Anim. Behav. 109, 101–111. doi: 10.1016/j.anbehav.2015.08.001
Sih A., Ferrari M. C. O., Harris D. J. (2011). Evolution and behavioural responses to human-induced rapid environmental change. Evol. Appl. 4 (2), 367–387. doi: 10.1111/j.1752-4571.2010.00166.x
Sorensen A., Van Beest F. M., Brook R. K. (2014). Impacts of wildlife baiting and supplemental feeding on infectious disease transmission risk: A synthesis of knowledge. Prev. Vet. Med. 113 (4), 356–363. doi: 10.1016/j.prevetmed.2013.11.010
St Clair J. J. H., Burns Z. T., Bettaney E. M., Morrissey M. B., Otis B., Ryder T. B., et al. (2015). Experimental resource pulses influence social-network dynamics and the potential for information flow in tool-using crows. Nat. Commun. 6 (1), 7197. doi: 10.1038/ncomms8197
St Clair J. J. H., Klump B. C., Sugasawa S., Higgott C. G., Colegrave N., Rutz C. (2018). Hook innovation boosts foraging efficiency in tool-using crows. Nat. Ecol. Evol. 2 (3), 441–444. doi: 10.1038/s41559-017-0429-7
Stolwijk A. M., Straatman H., Zielhuis G. A. (1999). Studying seasonality by using sine and cosine functions in regression analysis. J. Epidemiol. Community Health 53 (4), 235–238. doi: 10.1136/jech.53.4.235
Struhsaker T. T. (1975). “Red colobus: General description and survey techniques,” in The red colobus monkey (Chicago, IL: University of Chicago Press), 3–9.
Styche A. (2000). Distribution and behavioural ecology of the sulphur-crested cockatoo (Cacatua galerita L.) in New Zealand (Wellington, New Zealand: Victoria University of Wellington).
Swift K. N., Marzluff J. M. (2015). Wild American crows gather around their dead to learn about danger. Anim. Behav. 109, 187–197. doi: 10.1016/j.anbehav.2015.08.021
Terborgh J., Diamond J. M. (1970). Niche overlap in feeding assemblages of New Guinea birds. Wilson Bull. 82 (1), 29–52.
Thévenaz P., Ruttimann U. E., Unser M. (1998). A pyramid approach to subpixel registration based on intensity. IEEE Trans. Image Process. 7 (1), 27–41. doi: 10.1109/83.650848
Thomas N. J., Hunter D. B., Atkinson C. T. (Eds.) (2007). Infectious diseases of wild birds (Hoboken, NJ: Blackwell Publishing).
Thornton A. (2014). How and why are some species so smart? A comment on Rowe and Healy. Behav. Ecol. 25 (6), 1294–1295. doi: 10.1093/beheco/aru115
Tollington S., Ewen J. G., Newton J., McGill R. A. R., Smith D., Henshaw A., et al. (2019). Individual consumption of supplemental food as a predictor of reproductive performance and viral infection intensity. J. Appl. Ecol. 56 (3), 594–603. doi: 10.1111/1365-2664.13303
Trolliet F., Huynen M.-C., Vermeulen C., Hambuckers A. (2014). Use of camera traps for wildlife studies. A review. Biotechnol. Agron. Soc. Env. 18 (3), 446–454.
Troscianko J., Rutz C. (2015). Activity profiles and hook-tool use of New Caledonian crows recorded by bird-borne video cameras. Biol. Lett. 11 (12), 20150777. doi: 10.1098/rsbl.2015.0777
Warren Y., Higham J. P., MacLarnon A. M., Ross C. (2011). “Crop-raiding and commensalism in olive baboons: The costs and benefits of living with humans,” in Primates of Gashaka: Socioecology and conservation in Nigeria’s biodiversity hotspot. Eds. Sommer V., Ross C. (New York, NY: Springer), 307–332.
Weladji R. B., Tchamba M. N. (2003). Conflict between people and protected areas within the Bénoué Wildlife Conservation Area, North Cameroon. Oryx 37 (1), 72–79. doi: 10.1017/S0030605303000140
Wickham H. (2016). Ggplot2: Elegant graphics for data analysis. 2nd ed. (New York, NY: Springer-Verlag).
Keywords: behaviour, feeding platform, field methods, group foraging, playback, psittacines, temporal distribution, visitation rate
Citation: O’Hara M, Auersperg AMI, Prawiradilaga DM, Huber L and Mioduszewska B (2024) A novel feeding platform design for behavioral research on wild Tanimbar corellas (Cacatua goffiniana). Front. Ecol. Evol. 11:1275949. doi: 10.3389/fevo.2023.1275949
Received: 10 August 2023; Accepted: 11 December 2023;
Published: 08 January 2024.
Edited by:
Michael H. Parsons, Fordham University, United StatesReviewed by:
Birgit Szabo, Macquarie University, AustraliaYasushi Kiyokawa, The University of Tokyo, Japan
Copyright © 2024 O’Hara, Auersperg, Prawiradilaga, Huber and Mioduszewska. This is an open-access article distributed under the terms of the Creative Commons Attribution License (CC BY). The use, distribution or reproduction in other forums is permitted, provided the original author(s) and the copyright owner(s) are credited and that the original publication in this journal is cited, in accordance with accepted academic practice. No use, distribution or reproduction is permitted which does not comply with these terms.
*Correspondence: Mark O’Hara, mark.ohara@vetmeduni.ac.at