- 1College of Geography and Environmental Science, Henan University, Kaifeng, China
- 2School of Urban Economics and Public Administration, Capital University of Economics and Business, Beijing, China
- 3Xinyang Vocational and Technical College, Xinyang, China
- 4School of Medicine, Case Western Reserve University, Cleveland, OH, United States
In the context of urban expansion and climate change, the world is under pressure from multiple ecological risks. Key ecological protection areas play a pivotal role in preserving ecological stability and promoting development. Due to its unique geographical conditions, the Yellow River basin has been facing huge ecological risk pressure. In the affected area of the Lower Yellow River (AALYR) as an agricultural hub, ecological protection has gradually become a key factor restricting the development of cities and agriculture. Taking AALYR as an example, the landscape ecological risk assessment (LERA) system is established based on three aspects “natural environment—human society—landscape pattern”. We construct a comprehensive cumulative resistance surface based on the risk assessment results as the basis for the future study. Ecological corridors are identified by minimum cumulative resistance (MCR) models to establish and optimize Ecological security pattern (ESP) in the AALYR. We found that the landscape ecological risks (LER) in the study area show a uniform spatial distribution, with a slightly higher distribution in the northeast than the southwest. The ecological risk levels are generally high in AALYR, indicating a more severe risk problem in this area. A total of 56 ecological sources were identified, with a total area of 21176 km2. The ecological sensitivity of AALYR was high, and 99 ecological corridors and 59 ecological nodes were extracted. Ecological corridors and nodes were consistently and densely distributed throughout the study area. The network analysis method improves the stability of the network structure after optimization. Based on the key components of the ESP, with the combination of geographical characteristics and local policy planning guidance, we constructed the “One Belt and One Axis, Two Cores and Two Corridors, Four zones” ESP. The study results may offer guidance and suggestions for the construction of ESP and ecological environment protection system in the world’s major river basins, and may also provide information for ecological planning of other similar river basins in the world.
1 Introduction
Technological advances and population growth have changed the global environment and led to a range of ecological problems such as global warming (Schiermeier et al., 2019; Rong et al., 2022), deteriorating air quality (Castells-Quintana et al., 2021) and declining biodiversity (Mupepele et al., 2021). Ecological problems in many watershed areas have the potential to worsen ecological security risks (Luo et al., 2018). Ecological risk assessment has also become a hot topic of discussion and research for domestic and international scholars. Landscape ecological risk assessment (LERA) as an important subfield of ecological risk at the regional scale is widely used for risk area identification. LER refers to the possible negative effects of the interaction between landscape pattern and ecological process (Gong et al., 2020). Recently, researchers have carried out a lot of fruitful exploration of LERA by choosing relevant indicators, methods and models for different regions and different assessment purposes (Kayumba et al., 2021). However, there have been few studies on the multi-faceted quantitative assessment of ecological risks in basin landscapes from both “natural-social” dimensions (Zhu et al., 2022). Therefore, the combination of the landscape pattern index and the “natural-social” multi-source elements of the basin into the basin LERA system could offer a basis for building safety patterns (Chen et al., 2022). As an ecological space consisting of key locations, positions, and spatial connections in the landscape (Yang et al., 2022c), the Ecological Security Pattern (ESP) should prioritize resource elements that link human well-being and construct relevant ecological sources, nodes and corridors, etc. to optimize regional ESP in a targeted manner and make practical suggestions. By constructing a reasonable land ESP, the ecological security problems brought about by river basin development planning can be effectively guaranteed (Gao et al., 2021).
Concepts similar to ESP include urban growth boundaries (Dawkins and Nelson, 2002), ecological networks (Chen et al., 2023), green infrastructure (Zhang et al., 2022b), ecological control lines (Chen et al., 2021). In 1960s. Warntz and Woldenberg (1967) point, line and surface model for constructing flow surfaces provides a good picture of the “ecological flow” process in the landscape. McHarg (1969) used a “lasagna” superimposed model to reveal vertical links between vegetation, animals, soils and human activities in the landscape. Odum and Barrett (1971) proposed a regional ecosystem development strategy based on systems theory. In 1990s, Forman (1995) proposed a “patch-corridor-matrix” model of landscape ecology based on theories of landscape and regional ecology, laying the foundation for the study of landscape patterns. Subsequent research has focused mainly on ecological reserve zoning and regional landscape planning from the perspective of biodiversity. For example, Budaeva et al. (2021) planned the nature park reserve using a multi-criteria decision analysis method, which provided a reference for the nature conservation and tourism development of the park through sensitive zoning results. Otuoze et al. (2021) used cellular automata theory to construct a dynamic model to simulate and quantitatively predict urban growth, strengthened scientific urban planning and control of green space patterns. Zhelonkina et al. (2021) promoted regional conservation planning by identifying key spatial components of ecological conservation and evolutionary processes from the perspective of biodiversity conservation. Saleh and Abeer (2021) and Saleem et al. (2022) used correlation to assess land suitability and provided a theoretical basis for regional urban and rural land planning and forestry planning.
Following the proposal of the concept of landscape ESP for biological conservation by Yu (1999), Chinese scholars have conducted in-depth exploration and research on regional LER and the ESP based on theories and methods such as “source and sink” and landscape patterns. Existing research areas in LERA are mainly focused on ecologically fragile areas. Examples include large urban areas (Zhang et al., 2021), river basins (Wei et al., 2022), industrial, mining areas (Xu et al., 2021) and Wetland Nature Reserve (Yang et al., 2022a). Early LERA mainly continued the “source-sink” theory of regional ecological risk assessment (Zhu et al., 2020), calculating the ecological risk of a target unit by predicting the spatial dispersion effect of risk sources in a given landscape pattern (Li et al., 2023b). In the aspect of ESP, the study paradigm of “identifying sources—establishing resistance surfaces—extracting corridors—discerning the ESP” has been developed in the development practice (Zhang et al., 2022a). More research results have been achieved at national (Li et al., 2022b), provincial and municipal scales (Li et al., 2022c), as well as in urban clusters (Ran et al., 2022), basins (Wei et al., 2022a) and counties (Fan et al., 2021). The main research methods are graph theory (Urban and Keitt, 2001), circuit theory (Wang et al., 2022a) and the minimum cumulative resistance (MCR) model (Chen et al., 2020). The MCR model is the most commonly used and the study gradually attempted to establish a “bridge” between LERA and the construction of the ESP (Li et al., 2023a). Previous studies have identified sources and constructed general ideas for ESP by analyzing morphological spatial patterns and the importance of ecological services (Lin et al., 2021). There are relatively few studies on the construction of ESP from the perspective of LERA (Chen et al., 2022). Therefore, this study uses the “source—sink” theory to assess the LER areas in basin areas, construct ESP and propose locally appropriate protection strategies.
The affected area of the Lower Yellow River (AALYR) is currently relatively ecologically fragile, with low flows in the lower basin and shrinking estuarine wetlands starting to occur in some places. Five of the 14 concentrated contiguous destitute areas across the country involve the Yellow River basin (Wohlfart et al., 2016). The AALYR as the basin continues to develop socially and economically, the basin will be further exposed to ecological pressures and risks caused by economic growth and land use expansion (Zhang et al., 2020; Tang et al., 2023). Due to the interference and destruction of human activities in recent years, the spatial differentiation characteristics of landscape pattern in the AALYR are significant (Lou et al., 2022). Serious problems such as severe alkalinization, desertification, drought, waterlogging and poor drainage have occurred in the basin, resulting in serious damage to the ecological risk and the ESP of the basin. Ecological security issues pose a serious challenge to maintaining the LER issues in the AALYR. As one of the key areas for the ecological civilization construction in the Yellow River basin, the construction and optimization of ESP is of strong practical significance for the management of the AALYR. Therefore, the aim of this study is to assess the ecological risk of the AALYR using a LERA method. Overall, the study takes the AALYR as an example. Firstly, The SPCA is used to analyze the spatial distribution of LER in the AALYR. Then, the method of ecological sensitivity assessment is combined to extract high-value areas, which are considered as ecological sources. Finally, the ecological corridors are extracted using the MCR model to build an ESP (Figure 1). Research results can provide important information and reference for the development, utilization and conservation planning of the Yellow River basin.
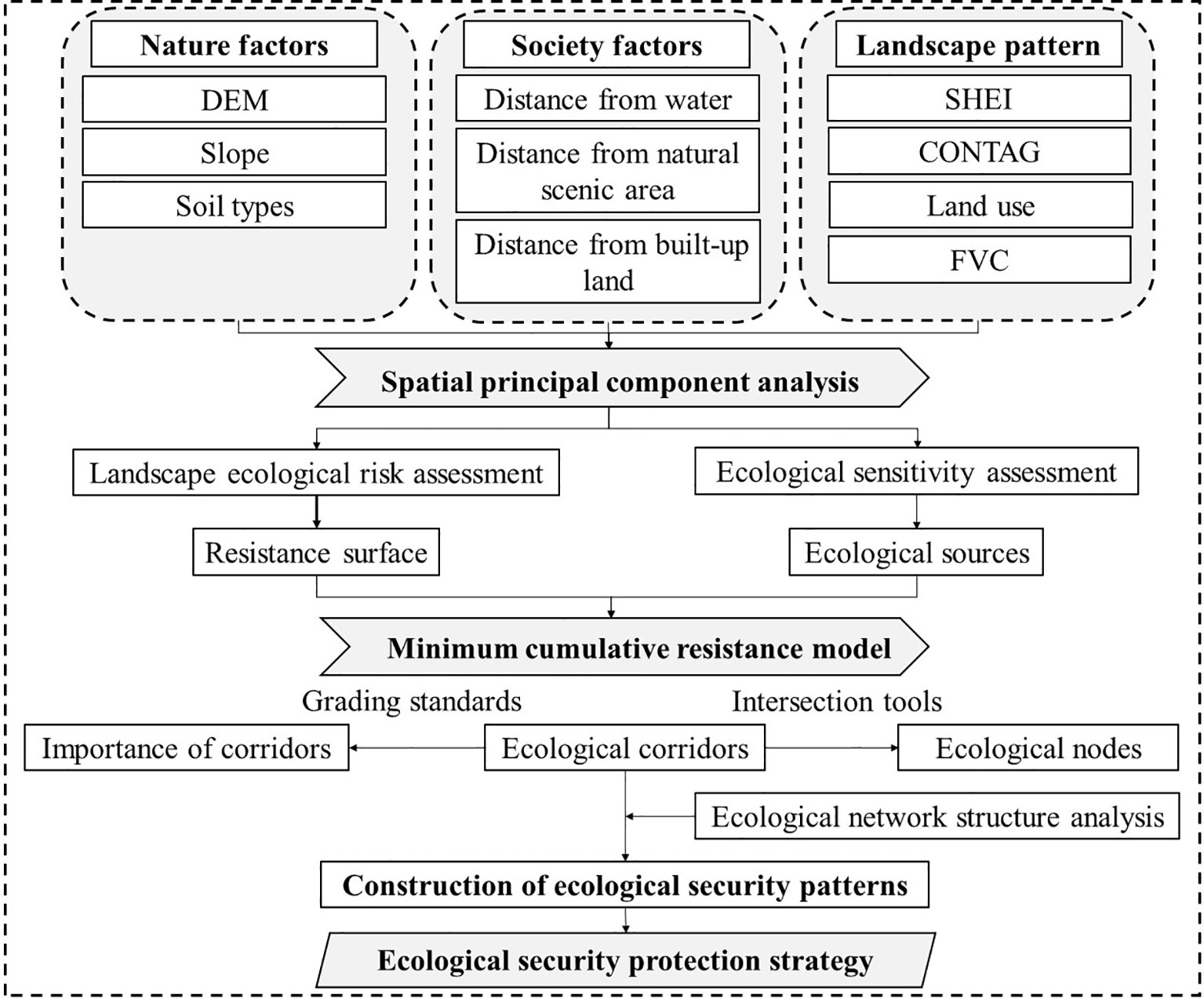
Figure 1 Research framework. (DEM, Digital Elevation Model; SHEI, Shannon’s evenness index; CONTAG, Contagion; FVC, Fraction Vegetation Coverage).
2 Materials and methods
2.1 Study area
Lower Yellow River is 785.6 km in length. According to existing studies on the division of the AALYR (Cen et al., 2019), and taking into account the Yellow River basin irrigation area, the integrity of regional urban development, and administrative divisions, 20 prefecture-level cities in Henan and Shandong provinces with a total area of 148,100 km2 are considered as the affected areas of the lower Yellow River (Figure 2). It is a flat plain area that contains seven major landscape types: cultivated land, forest, shrub, wetland, water, bare land and construction land. The cultivated land accounts for 68.67% of the total area; followed by built-up land, which accounts for 18.94% of total area; Forestland and grassland account for only 7.12% of the total area. Considering the development of the lower Yellow River area in recent years and the availability of data, this study selected 2018 data to analyze. Since Laiwu, Shandong Province was approved by the State Council to be abolished as a district in 2019, it is still considered to be a prefecture-level city in our study. The terrain of the affected area of the AALYR is dominated by plains, mountains and hills. At the end of 2018, the total GDP of the study area reached 6.63 trillion RMB, accounting for>40 percent of the total GDP of the basin. In 2019, ecological protection and high-quality development of the Yellow River basin were upgraded as a major national strategy, providing unprecedented opportunities for regional development. Therefore, it is urgent to study the status of ecological risk in the lower reaches of the Yellow River area and to analyze the influencing factors in order to provide relevant policy suggestions for the study of regional ecological protection research in small and medium-sized basins and similar areas.
2.2 Data sources
The study data include land use, digital elevation model (DEM), soil types and other geographic data in 2018.
(1) Land use from the Resource and Environmental Science Data Center (http://www.resdc.cn). Land use is divided into cultivated land, forestland, grassland, built-up land, water, and unused land. The spatial resolution is 30 m.
(2) DEM from Geospatial Data Cloud (http://www.gsclound.cn). The spatial resolution is 30m.
(3) Vegetation cover from United States Land Processes Distributed Data Archive center’s (https://e4ftl01.cr.usgs.gov/MOLT/MOD13Q1.006/) MOD13A3 dataset product. Data products have a time resolution of 16 days and a spatial resolution of 250 m.
(4) Soil types data from the Chinese soil dataset (http://www.fao.org/home/en/) of the World Soil Database. The spatial resolution is 1 km.
(5) Other basic geographic data from the Global Geographic Information Resources Directory Service System (https://www.webmap.cn). The scale of natural scenic spots, built-up land and water are 1: 250000. The spatial resolution is 30 m.
2.3 Methods
This study mainly aiming at the current situation of ecological environment in the study area, and selects indicators from the natural environment, human society, and landscape pattern as the evaluation elements of the LER of the study area, and constructs a three-dimensional comprehensive LREA system, which is based on the “nature-human society-landscape pattern”. Construct a three-dimensional comprehensive LERA system of “nature—human society—landscape pattern”. The results of LREA were used as evaluation factors for landscape pattern resistance, and the ecological source was identified by combining with ecological sensitivity assessment, and ESP optimization was carried out by using the MCR model and network structure analysis method.
2.3.1 Selection of ecological risk assessment indexes
According to the natural environment and economic development of the AALYR, and considering the scientific soundness and data availability, we selected 10 influencing factors from three perspectives: nature, human disturbance, and landscape pattern, to assess the LER of the study area. These include: DEM, Slope, Soil types, Distance from water, Distance from built-up land, Distance from natural scenic, Shannon’s evenness index (SHEI), Contagion (CONTAG), Land use, Fraction vegetation coverage (FVC). Indicators of natural factors: the DEM, the higher the risk of landscape ecology; the higher the slope, the higher the risk of the existence of landscape ecology; soil type can reflect the growing condition of crops, which has a good improvement effect. Due to human interference and highway construction, the original ecological conditions have been altered and the original landscape pattern has been disturbed. The closer the distance from the built-up land, the higher the LER; The greater distance from the water and distance from the natural scenic higher ecological risk to the landscape; Landscape factor indicators: The higher the Shannon evenness index, the higher the ecological stability of the area; The lower contagion index corresponds to the vulnerability of the disturbance to external activities in the landscape pattern; Vegetation cover indicates the degree of greening of an area, with larger values indicating a better ecological environment. Land use is classified according to the first-level reclassification. The selection of assessment indicators is based on relevant literature (Chi et al., 2022) and the environmental conditions of the AALYR. The ecological security classification standards for each factor in the study area are formulated using the natural breakpoint method, which divides the factors into 5 levels (Table 1). Figure 3 classifies the indicators by using the reclassification tool.
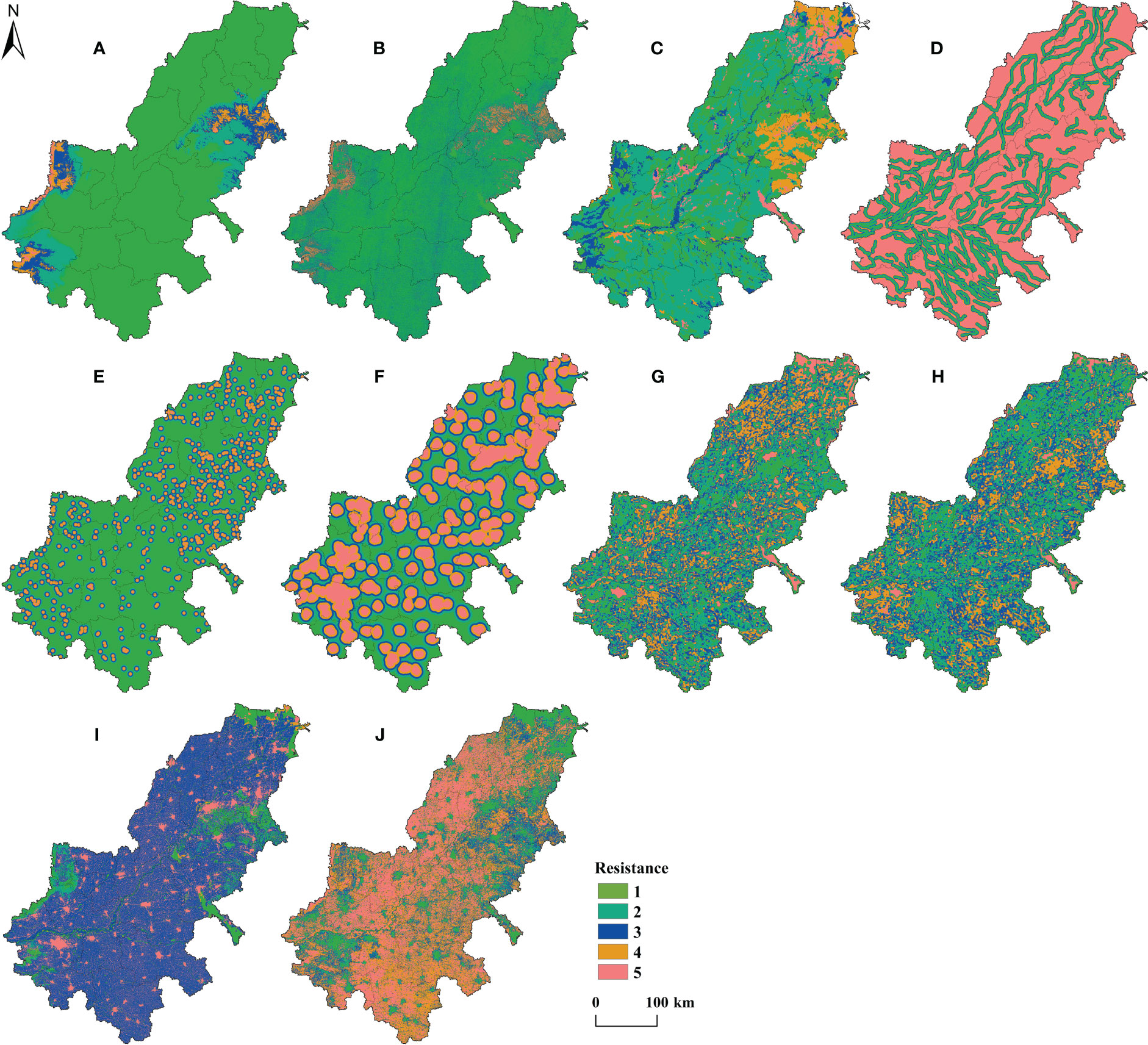
Figure 3 Spatial visualization of ecological risk levels of assessment index in the AALYR. (A) DEM, (B) Slope, (C) Soil types, (D) Distance from water, (E) Distance from natural scenic area, (F) Distance from built-up land, (G) SHEI, (H) CONTAG, (I) Land use, (J) FVC).
2.3.2 Spatial principal component analysis
Spatial principal component analysis (SPCA) is widely used in ecological risk assessment (Wei et al., 2020). The key risk factors are identified by extracting the principal components of the original assessment factors to remove the correlation and redundancy of the influencing factors, so that the calculation results of the principal component factors are uniformly presented on each corresponding raster in space, with good spatial visualization effect (Wang et al., 2021). The principal component factors are weighted and superimposed using the raster calculation tool in ArcGIS 10.6 software. Equation is as follows:
Where E is the comprehensive assessment results of LER. aij is the j principal component corresponding to the i raster. Fj indicates the eigenvalue contribution rate of the j principal component.
2.3.3 Construction and optimization of ESP
The MCR model is often used to simulate the minimum cost path of the cost of species crossing different landscape substrates from the source (Yi et al., 2022). Early on, the concept of MCR model was proposed by Dutch scholars (Knaapen et al., 1992), and then it was improved by Chinese scholar Yu (1999) and has been widely studied in the field of regional ESP. Its advantages include convenient data processing, comprehensive process analysis and visual results. In addition, the MCR model not only analyzes the superposition of vertical factors, but also uses the geographic information system to analyze the horizontal flow trend of land landscape units, making it one of the important models for connectivity and suitability analysis of large-scale space. Equation is as follows:
Where MCR is the cumulative value of the minimum resistance between the ecological source j and any grid i. Dij is the path distance of species form source j to another possible destination i of a source or region. Wi is the resistance value of the i grid on the landscape resistance surface to the ecological flow.
(1) Identification of ecological sources. Source areas, as habitat patches, are crucial for improving the optimization of landscape patterns. They serve as source points for species migration and maintenance, with good stability and expansion potential (Ding et al., 2022). In this study, we employ the ecological sensitivity assessment method to identify ecological sources. Ecological sensitivity refers to the ability of ecological factors to adapt to external pressure or human disturbance when the environmental quality does not decrease (Wang et al., 2017b). Specifically, this method first constructs the index system and assigns a weight to each index, then superposes and analyzes each ecological factor, and finally obtains the ecological sensitivity distribution of a region in space. Considering the ecological characteristics of the lower Yellow River and the applicability of the data, a total of 5 ecological factors were selected (DEM, slope, FVC, water, and land use). Combined with analytic hierarchy process (CR=0.0356<0.1, pass the test) to determine the weight of each factor (0.10, 0.15, 0.29, 0.22, and 0.24). The areas with higher ecological sensitivity are relatively rich in ecological resources, and most of the current situation is dominated by woodlands and hills with higher slopes, which have high ecosystem service values (Du et al., 2020; Cui et al., 2022). Finally, taking into account the description of ecological sensitivity classification in the National Ecological Function Zoning issued by the Ministry of Environmental Protection of China in 2015 (No.61 of 2015) and related studies (Jin et al., 2021). on this basis, we extracted a large area of patches in highly sensitive areas as ecological sources.
(2) Determination of resistance surfaces. The resistance surface is calculated using the results of the basin LERA and the screened sources as the basis for the generation of the resistance surface of the landscape pattern, and the size of the integrated resistance surface is classified into 1-5 grades using the natural breaks method. Table 2 shows the graded criteria.
(3) Extraction of ecological corridors. The shortest consumption paths for the exchange of materials and energy between different ecological source areas are referred to as corridors (Li et al., 2022d). The length of the corridor is divided into 3 levels, the first level corridor is greater than 50 km, the second level corridor is between 30 km and 50 km, and the third corridor is less than 30 km.
(4) Identification of ecological nodes. Nodes are areas on the ecological corridor where ecological functions are weakest and need to be identified and protected as a priority (Yu et al., 2021). The extraction of ecological nodes was performed by utilizing the intersection tool of hydrological analysis.
(5) Assessment of ecological network structure. The assessment indicators mainly include: closure index (α), connectivity rate (β) and linkage index (γ). These indicators show the relationship between the number of corridors and nodes, and also the complexity of the network structure, with larger values indicating a more complex network structure and a better ecological environment. Closure index is used to indicate the extent to which network loops occur (Equation 3), the range of change is between 0-1, and the larger the number, the more species transport paths and the better the circulation of the network. Connectivity indicates the degree of connectivity of the intersection points in the ecological network (Equation 4), the range of change is between 0-1, connectivity rate represents the average connectivity probability between the nodes of the ecological network (Equation 5), β<1 shows that the network structure is dendritic, β=1 explain that the network is the structure of a one-route circuit, and β>1 indicates a complicated network shape. Equation is as follows:
Where L is used to mean the number of corridors, V is used to mean the number of nodes.
3 Result
3.1 LERA
3.1.1 Assessment of ecological resistance of landscape pattern
In this study, 10 influencing factors were selected, including DEM, Slope, Soil types, Distance from water, Distance from natural scenic, Distance from built-up land, SHEI, CONTAG, Land use, and FVC. Table 3 shows the characteristic roots and cumulative contribution rates. We extracted factors with characteristic roots and cumulative contribution rate above 85% to improve the reasonableness of the ecological resistance pattern of the AALYR. Table 4 shows the values of the loadings of the assessment factors that correspond to every principal component. From a natural factors point of view, the results indicate that DEM, slope, and soil types have lower loadings on the third principal component compared to the other index factors. Specifically, the loading value of soil types is 0.590, indicating a higher loading on the sixth principal component. This suggests that natural indicators have a weaker effect on LER, and that soil types have a more significant impact on the integrated risk. Among the indicators of human interference, the Distance from water factor had the highest factor load in the second principal component with a value of 0.937, indicating the strongest influence on the comprehensive LER. The Distance from natural scenic area index had a higher factor load of 0.739 in the first principal component, indicating a significant impact on the comprehensive risk. As for the aspects Landscape pattern, the SHEI has the highest loading of 0.596 in the third principal component factor; the FVC has a higher loading of 0.559 in the fourth principal component factor and the CONTAG has a loading of 0.731 in the tenth principal component factor. This shows that CONTAG, FVC and SHEI contribute significantly to the integrated ecological risk of the landscape.
3.1.2 Spatial analysis of LER
Figure 4A shows the spatial distribution characteristics shown by the results of the LERA in the AALYR region: (1) Highest ecological risk area. The risk index was 3.30-4.95, and the area was 26106.36 km2. It is centered in the southwest area of the AALYR and some areas with built-up land and settlement distribution in the northeast region. This pattern is mainly caused by the municipality being the administrative center unit and the large interference from human activities, resulting in a serious LER. (2) Higher ecological risk area. The risk index was 2.85-3.30 and the area was 44466.70 km2. Such regions are mainly located at the edge of high-risk areas and are affected by parts of industrial land and urban built-up land. (3) Middle ecological risk area. The risk index is 2.39-2.85 and the area is 43751.21 km2. This risk area is uniformly distributed throughout the study area and occupies the largest area. (4) Lower ecological risk area. The risk index was 1.07-2.39, and the area was 20926.18 km2. These risk areas are mostly located at the edge of the medium ecological risk area. (5) Lowest ecological risk area. The risk index is 0-1.07, and the area is 12849.55 km2, which is the smallest proportion of the study area. This area is mainly located in regions with high vegetation cover and along river corridors, where ecosystem services have a high value and human interference is relatively low. These conditions are conducive to the gathering of natural species and the maintenance of ecological balance in this type of habitat.
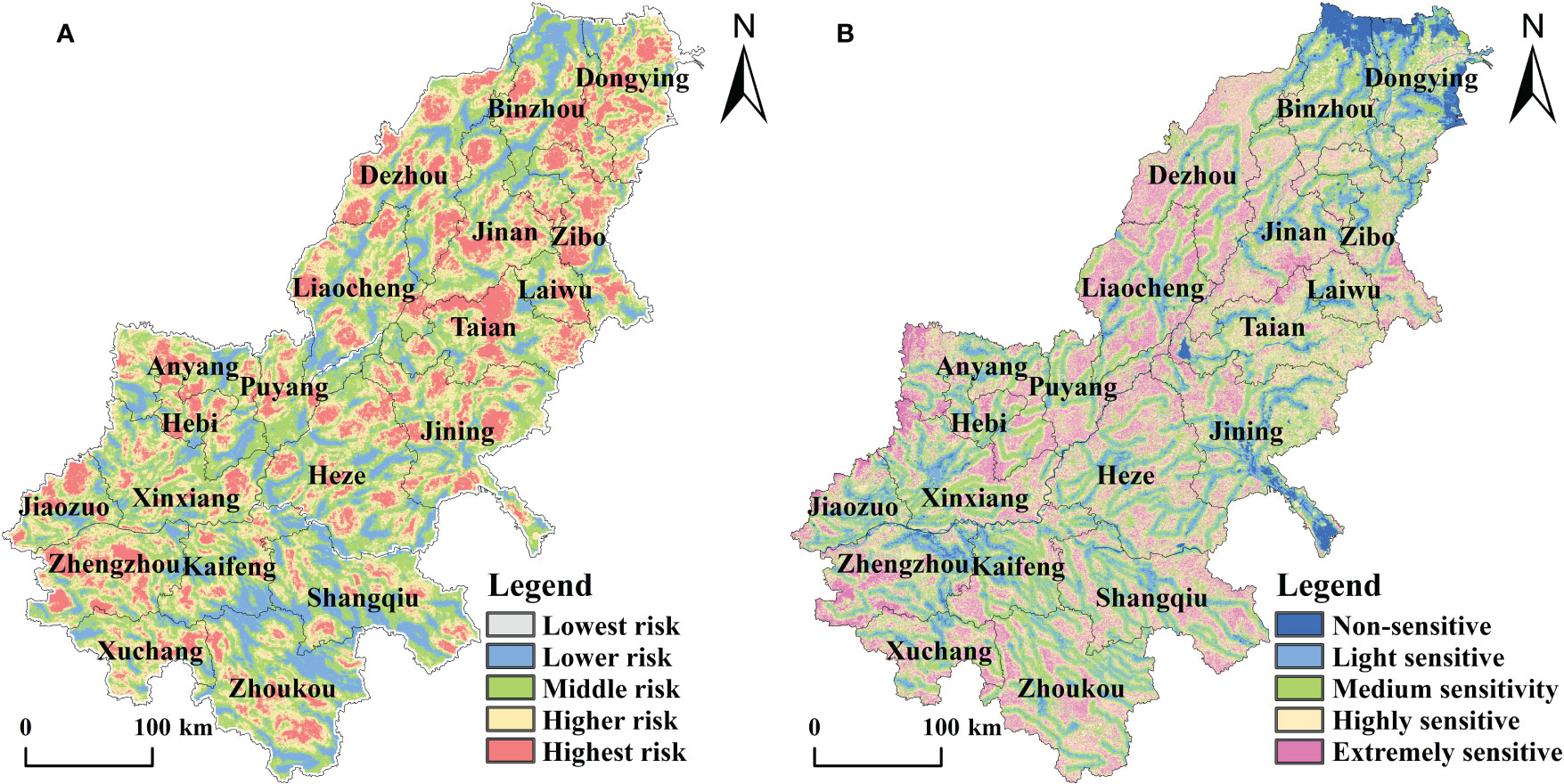
Figure 4 (A) Classification of landscape ecological risks in the AALYR; (B) Spatial distribution of ecological sensitivity in the AALYR.
3.2 Construction of the ESP
3.2.1 Identify ecological sources
Figure 4B shows the integrated ecological sensitivity assessment obtained by AALYR after weighting and superimposing single-factor ecological sensitivity indicators. The Natural Break was used for grading treatment, and five grades were obtained: non-sensitive, light sensitive, medium sensitivity, highly sensitive and extremely sensitive. From Figure 4B, highly sensitive areas are distributed in Dezhou, Liaocheng, Puyang, and Zhengzhou, mainly because the area is close to the Yellow River. The landscape substrate is mainly forestland and grassland, the landscape types are rich, and the ecological environment is high and sensitive. Areas with low ecological risk sensitivity are located in populated areas, urban built-up land and settlements are densely distributed, and the ecological sensitivity is relatively low due to the destruction of human activities. In order to ensure the relevance and integrity of source areas within the AALYR, as well as the appropriate patch size, a careful screening process was carried out to identify ecological sources. After comparing different patch area sizes, patches with high sensitivity areas larger than 200 km2 were selected as ecological sources. A total of 56 sources were identified, covering an area of 21176 km2, which represents 14.30% of the total study area. Figure 5A shows that most of the sources are concentrated in the northeastern and southwestern parts of the AALYR, where the high vegetation cover and rich biodiversity are conducive to the dispersal and conservation of species.
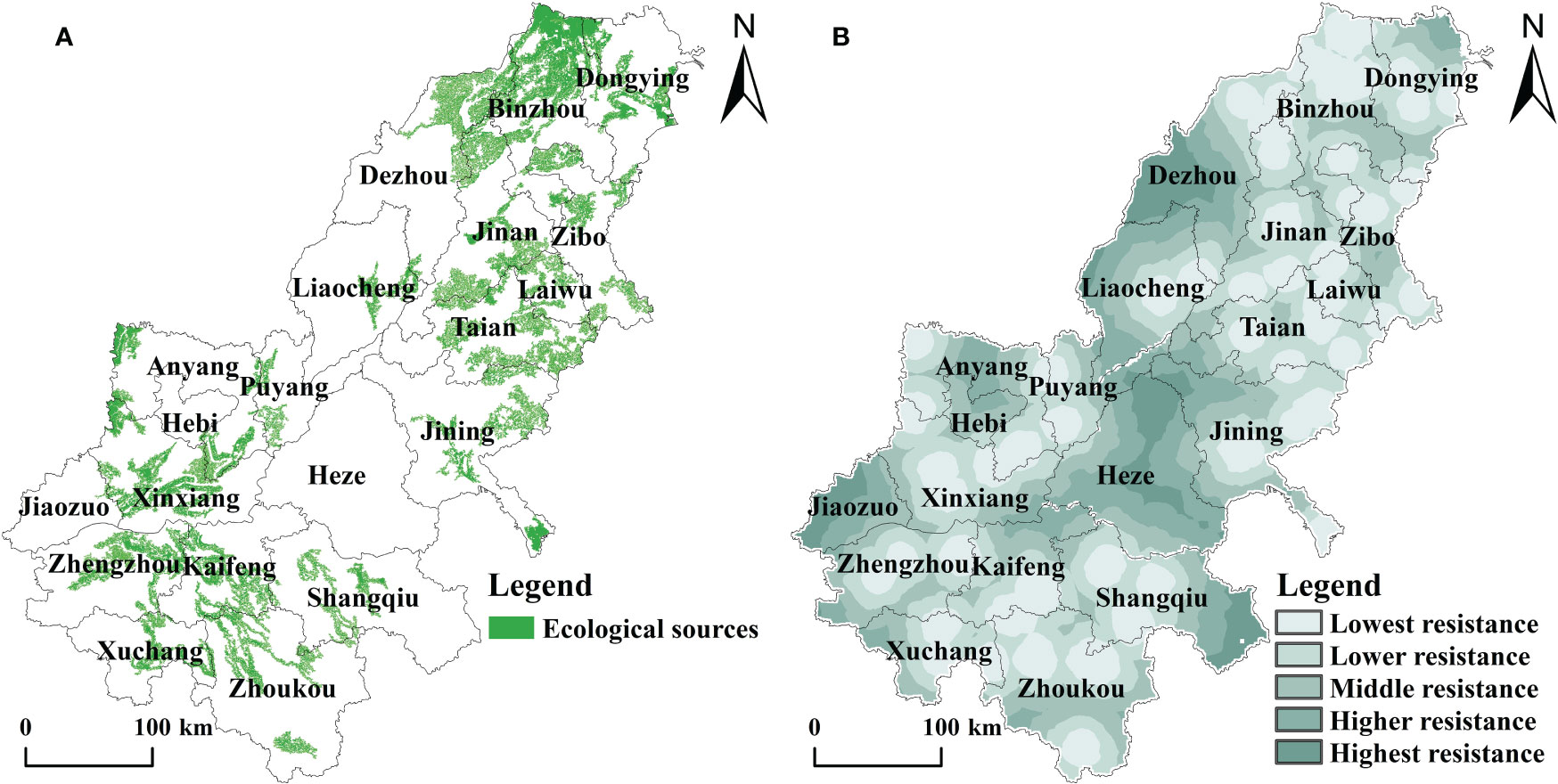
Figure 5 (A) Spatial distribution of ecological source area of the AALYR; (B) Spatial distribution of resistance in landscape patterns in the AALYR.
3.2.2 Integrated ecological resistance surface
Based on the ecological source and integrated resistance surface as the reference surface, the MCR surface of the AALYR is calculated by using the cost distance tool. Figure 5B shows the spatial distribution characteristics of the resistance surface: (1) The lowest resistance area is the largest in range, with a total area of 38847.90 km2, mostly distributed in the northeast and southwest regions and in areas with high vegetation cover. (2) Lower resistance area of 7159.58 km2, 3.13% of the total study area, mostly distributed in Zhengzhou, Xuchang, Kaifeng, Xinxiang, Zhoukou, Shangqiu, Jinan, Taizhou, Liaocheng, Binzhou, and Dongying. (3) Middle resistance area is 32800.07 km2, 22.15% of the total study area, mainly located in the peripheral edge region of lower resistance. (4) Higher resistance area is mostly located in the study area in various types of areas such as cultivated land, grassland and unused land, 14.31%, and the area was 21186.08 km2. (5) Highest resistance area makes up the smallest percentage, accounting for 6.19%. The area is 9,164.50 km2, mostly concentrated in the central areas of Dezhou and Heze in the AALYR. The main reason is that the region has historically seen several major Yellow River migrations, influenced by factors such as floodway, flow velocity and wind, resulting in a spatial distribution characterized by higher and undulating terrain and poorer connectivity between source sites in high resistance areas and larger patches, creating greater resistance to the flow between species. The lowest resistance values occur in the middle of the Henan and Shandong regions of the basin, so it is necessary to build corresponding ecological corridors to make the resistance surface of the AALYR connected to the ecological source and to obtain the exchange between energy.
3.2.3 Extraction of ecological corridors
Corridor extraction is based on the technical principle of using the geometric centroid of the source site as the source input and clustering the remaining n-1 (where n means the number of ecological source geometric centroids) to create target output clusters. Figure 6A shows that Cost path tool was used to extract the shortest costly paths for clusters of source centroids and target points to obtain ecological corridors between ecological source sites. 99 corridors were built in the study area, with a total length of 3670 km, 51.65% of the total length. The results show: (1) There are 27 first-level ecological corridors whose total length is 1933.004 km, and most of the first-level ecological corridors are concentrated in the area of dense source patches, mainly in Dongying, Binzhou, Jinan, Liaocheng, Jining, Shangqiu, and Zhengzhou, and have a high degree of connectivity. The primary ecological corridor mainly connects the entire the AALYR with its length, thus facilitating movement of species and exchange of energy within the study area. This forms an important hub for maintaining ecological balance in the relevant areas. (2) There are 32 second-level ecological corridors, which are short and scattered, but they are distributed in the AALYR, effectively linking the scattered ecological sources in the study area with smaller regions, improving biodiversity and increasing the value of ecological services. The total length is 1268.14 km, 33.88%. Second-level ecological corridors are mainly distributed with the cities of Laiwu, Taian, Xinxiang, and Zhoukou. (3) There are 41 three-level ecological corridors, with a total length of 541.65 km, only 14.47% of the length of all corridors. Due to the lack of a relatively perfect ecological corridor network system, the ecological connection is weak and cannot adapt to the circulation between “ecological flows”.
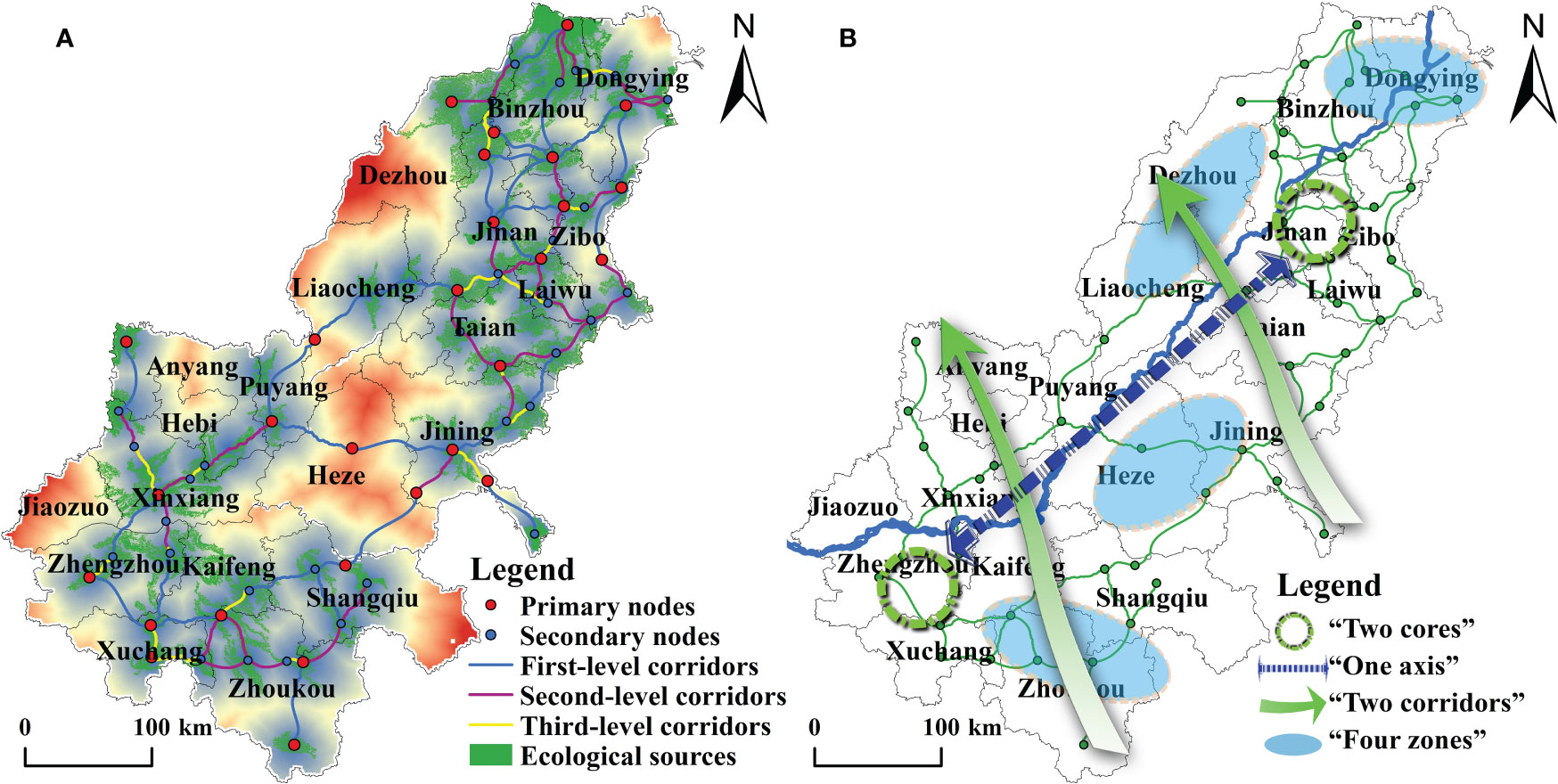
Figure 6 (A) Length of ecological corridors and number of ecological nodes in the AALYR; (B) The optimized framework to better balance ecological conservation and economic development in the AALYR.
3.2.4 Identify ecosystem nodes
Figure 6A shows the ecological nodes. The identification of nodes is obtained by extracting the valley lines of the cumulative resistance surface and intersecting the corridors. We identified 59 ecological nodes, including 30 primary and 29 secondaries nodes. The primary ecological nodes intersect the first-level corridors mainly with the least costly paths and play a strategic position in the construction of ESP. The secondary ecological nodes intersect with the second-level and three-level corridors with the maximum cumulative resistance path, forming a radiation driving effect on the surrounding fragile ecological pattern. Ecological nodes are key points of weak ecological functions and should be given key ecological protection and construction.
3.2.5 Optimization of ecological network structure
Table 5 shows the calculation results of ecological network indexes before and after optimization. Optimized connectivity was significantly improved, which promoted the stability of ESP in the study area. γ before and after optimization are 0.5789, 0.5819, indicating that the ecological network nodes before optimization are sparse and poorly connected to each other, and the connection of the ecological network nodes after optimization is improved. α before and after optimization are 0.3628, 0.3675. It reflected that there were fewer ways of species migration and diffusion in the AALYR before optimization, and the circulation was poor, which increased the material, energy and information exchange capacity between landscape patches after optimization, and promoted the interoperability between ecological patches. β before and after optimization are 1.6780, 1.5885, indicating that the ecological network is no longer a tree or a single loop network, but a more complex network structure. The stability of the network structure is enhanced by proposing optimized paths for adding some ecological sources, corridors and nodes in the study area.
3.2.6 Optimization framework of the ESP
Identifying and preventing LER is an important prerequisite for improving regional ecological security. From this perspective, this paper proposes to construct a trans-administrative boundary basin ESP, which is of great significance for solving large-scale regional ecological security problems. Combine various landscape elements to optimize ESP. The distribution of corridors, the construction of sources and the selection of nodes are important parts of the construction of ESP. The analysis showed that the AALYR is most affected by human interference, as well as the relatively complex and heterogeneous land use types within the ecological source region. Corridors selection aspect, unlike other research methods, this paper only connects the edges of the source’s region, ignoring the connectivity within the source region. In addition, the role of primary nodes is to focus more on the protection and adjustment of current environmental conditions and to provide optimal conditions for species movement, while secondary nodes are mainly used to improve the balance between human destruction and ecological protection and to enhance the basic requirements for species movement between source regions. Based on this, the ESP strategy for the AALYR was established by combining the spatial distribution of ecology, agriculture and cities in Shandong and Henan provinces, as well as the ecological networks constructed. Specifically, with the Yellow River basin as the guiding main line, Zhengzhou and Jinan as the main cores have established an optimization framework based on “One Belt and One Axis, Two Cores and Two corridors, Four zones” (Figure 6B).
4 Discussion
4.1 Comparison and connection with similar studies
Based on the results of ecological security assessment, the present situation of ESP and ecological risks in the AALYR were further clarified. We conclude that the LER in the study area continues to intensify, and the northeast is higher than the southwest, and the LER in the basin area is increased by the influence of man-made destruction, which is consistent with the research results of other similar basins (Ai et al., 2022). At present, China’s ecological protection policy is based on the ecological protection red line (Gao et al., 2020), evaluating important ecological sources according to the importance of ecosystem services and ecological sensitivity, and dividing areas far from the scope of human activities according to their importance (Wang et al., 2017a). Source areas are identified by analyzing methods of ecological sensitivity assessment and taking into account the impact of environmental and human activities when selecting sources. This paper mainly draws on the research ideas of other similar basins (Li et al., 2020), compared with the method of directly selecting a nature reserve or a fixed patch of forestland as an ecological source, this study overcomes the limitations of single-factor assessments, enabling a more comprehensive evaluation of ecosystem benefits and providing valuable insights. This is similar to the ESP of the Minjiang River Basin (Wang et al., 2022b). A total of 56 ecological source areas were extracted, and 99 ecological corridors and 59 ecological nodes were identified. The distribution characteristics of the corridors were mostly concentrated in areas with low resistance accumulation and dense source patches, which provided convenience for the exchange of matter and energy, which was consistent with the research results of other similar basins (Li et al., 2022a). Moreover, there is growing evidence that fertile agricultural and forestland areas are often taken up by construction activities, resulting in urban sprawl and ecological land loss (Yang et al., 2022b; Zhou et al., 2023). At present, the methods and standards for constructing resistance surfaces are not unified (Su et al., 2016), and some scholars construct resistance value coefficient by biodiversity conservation (Fu et al., 2019). Therefore, the construction of the resistance surface should fully consider regional differences to improve the reliability of simulation results. In addition, biologists (Salviano et al., 2021) have shown that an ecological corridor of 60-100 m was the best width for species migration and had a better ecological conservation effect. Narrow corridors do not meet the basic needs of migrating species, while wide corridors increase the need for additional land and elevate conflict with landowners (Dong et al., 2020). Therefore, we set the width as 100 m for the extracted first-level ecological corridors and 60 m for the other ecological corridors. In summary, our constructed ESP is scientifically sound and can provide more accurate information for maintaining ecological security when compared to existing research and ecological policies.
4.2 Reasonableness of the LERA
With further urban development and use, landscape fragmentation is not only limited to change the shape of the landscape, but further affect the internal environment, eventually leading to changes in landscape structure and function. Research has shown that changes in landscape patterns and internal relationships are dynamic processes that cannot simply be analyzed in terms of landscape fragmentation and connectivity. Rather, it is important to consider these patterns in relation to specific temporal and spatial changes (Yang et al., 2023; Zou et al., 2022). Therefore, in the management and planning of urban space in the AALYR, corresponding measures are required according to the different development stages of urbanization, not only to coordinate the contradiction of land use, but also to optimize the internal structure, especially to improvement of cultivated land quality. For the more developed Jinan and Zhengzhou, the need for socio-economic development needs to be seriously considered. At the same time, it is important to protect areas where natural habitats are concentrated, especially habitat margins and corridors, in order to achieve a positive balance between natural and economic interests (Liu et al., 2022). This approach can promote regional planning and support the healthy development of the city.
4.3 Limitations and future research directions
This research not only ensures the integrity of the ecosystem, but also provides direct insights for policy makers and planners. However, a number of important issues related to the ESP need to be further explored. Firstly, in establishing the resistance surface factors, although an attempt was made to adequately reflect the natural and socio-economic elements, the socio-economic factors were still generalized due to the lack of regionally corrected ecosystem service values, and the values quoted only reflect the relative differences between the factors rather than reflecting the absolute values (Geng et al., 2022). The selection of ecological sources only relied on what can be ecological sources, and there is no in-depth research on the issue of the scale of ecological sources and how large an area can meet the function of ecological land. Meanwhile, the construction of ecological corridors will inevitably change the land use structure, and the resulting changes in the environment are also a research direction that should be concerned in the future. Our study only analyzed the current ESP based on land use data, and researchers can predict future pattern trends in the context of the corresponding development status.
This study takes the AALYR as the research object. On the one hand, it is because the study area is rich in natural conditions and biodiversity resources; on the other hand, the rapid urbanization and frequent anthropogenic damage have led to environmental degradation, which seriously threatens the regional ecological security. The results of this study expand the understanding of the ecological characteristics of the area and provide suggestions for urban planning and environmental protection in the AALYR and other areas facing similar challenges.
5 Conclusions
Planning urban ecological space is effective in weakening threats to ecological security from urban sprawl at the landscape scale. In this study, we analyzed the ESP of the basin with the by the aid of LERA and MCR model. Figure 6B shows our proposed optimal ecological optimization framework of “One Belt and One Axis, Two Cores and Two Corridors, Four zones”. We followed the sequence of “determining the sources, building the resistance surface, selecting corridors and nodes, constructing and optimizing the ESP”, which is a more scientific approach. This allowed us to propose specific countermeasures for optimizing the ecological service pattern. It is recommended that a part of the plantation forestland be added, the protective zone on both sides of the river be expanded and an ecological reserve be built to enhance the ecological protection of the AALYR. In order to optimize the ecological environment in the AALYR, local government departments should focus on the construction of ESP and reasonably plan and allocate control measures such as ecological land and built-up land. In addition, in the context of promoting ecological conservation and ecological restoration in the Yellow River basin, it is necessary consider the effectiveness of differentiated policy development at different scales.
Data availability statement
The original contributions presented in the study are included in the article/supplementary material. Further inquiries can be directed to the corresponding authors.
Author contributions
YH: Conceptualization, Methodology, Writing – original draft. JZ: Writing – review & editing, Data curation, Investigation. PZ: Funding acquisition, Methodology, Project administration, Writing – review & editing. ZC: Writing – review & editing. XZ: Data curation, Writing – review & editing. RL: Data curation, Writing – review & editing. ML: Data curation, Writing – review & editing. GX: Data curation, Writing – review & editing. YS: Funding acquisition, Project administration, Writing – review & editing.
Funding
The author(s) declare financial support was received for the research, authorship, and/or publication of this article. This research was funded by the National Natural Science Foundation of China, grant number 41601175, 41801362, the Program for Innovative Research Talent in University of Henan Province, grant number 20HASTIT017, 2020 Philosophy and Social Science Planning Project of Henan Province, grant number 2020BJJ020, and 2022 Program for youth talent of Zhongyuan, The Belt and Road Special Foundation of the State Key Laboratory of Hydrology-Water Resources and Hydraulic Engineering, grant number 2021490111, Funding for the construction of the key Laboratory for Agricultural non-point Source pollution Control on the Huang-Huai-Hai Plain in 2023, grant number 2023HNNPSCL-HNDX001. We also thank the Geographical Science Data Center of The Greater Bay Area for providing the relevant data in this study.
Conflict of interest
The authors declare that the research was conducted in the absence of any commercial or financial relationships that could be construed as a potential conflict of interest.
Publisher’s note
All claims expressed in this article are solely those of the authors and do not necessarily represent those of their affiliated organizations, or those of the publisher, the editors and the reviewers. Any product that may be evaluated in this article, or claim that may be made by its manufacturer, is not guaranteed or endorsed by the publisher.
References
Ai J. W., Yu K. Y., Zeng Z., Yang L. Q., Liu Y. F., Liu J. (2022). Assessing the dynamic landscape ecological risk and its driving forces in an island city based on optimal spatial scales: Haitan Island, China. Ecol. Indic. 137, 108771. doi: 10.1016/j.ecolind.2022.108771
Budaeva D. G., Sharaldaeva V. D., Maksanova L. (2021). “Some peculiarities of functional zoning in specially protected natural territories: case study of the Tunkinsky National Park, Russia,” in IOP Conference Series: Earth and Environmental Science, Vol. 885. 012051. doi: 10.1088/1755-1315/885/1/012051
Castells-Quintana D., Dienesch E., Krause M. (2021). Air pollution in an urban world: a global view on density, cities and emissions. Ecol. Econ. 189, 107153. doi: 10.1016/j.ecolecon.2021.107153
Chen C. L., Shi L., Lu Y., Yang S., Liu S. F. (2020). The optimization of urban ecological network planning based on the minimum cumulative resistance model and granularity reverse method: a case study of Haikou, China. IEEE Access. 8, 43592–43605. doi: 10.1109/ACCESS.2020.2976548
Chen J., Wang S. S., Zou Y. T. (2022). Construction of an ecological security pattern based on ecosystem sensitivity and the importance of ecological services: a case study of the Guanzhong Plain urban agglomeration, China. Ecol. Indic. 136, 108688. doi: 10.1016/j.ecolind.2022.108688
Chi J. Y., Xu G. L., Yang Q. Q., Liu Y. T., Sun J. X. (2022). Evolutionary characteristics of ecosystem services and ecological risks at highly developed economic region: A case study on Yangtze River Delta, China. Environ. Sci. Pollut. Res. doi: 10.1007/s11356-022-22313-4
Chen X. Q., Guan Q. H., Li F. L., Liu D., Han C. H., Zhang W. J. (2021). Study on the ecological control line in the major leakage area of Baotu spring in Shandong province, eastern China. Ecol. Indic. 133, 108467. doi: 10.1016/j.ecolind.2021.108467
Chen X. Q., Kang B. Y., Li M. Y., Du Z. B., Zhang L., Li H. Y. (2023). Identification of priority areas for territorial ecological conservation and restoration based on ecological networks: a case study of Tianjin City, China. Ecol. Indic. 146, 109809. doi: 10.1016/j.ecolind.2022.109809
Cui X. F., Deng W., Yang J. X., Huang W., de Vries Walter T. (2022). Construction and optimization of ecological security patterns based on social equity perspective: A case study in Wuhan, China. Ecol. Indic. 136, 108714. doi: 10.1016/j.ecolind.2022.108714
Cen Y. F., Zhang P. Y., Yan Y. H., Jing W. L., Zhang Y., Li Y. Y., et al. (2019). Spatial and temporal agglomeration characteristics and coupling relationship of urban built-up land and economic hinterland—a case study of the Lower Yellow River, China. Sustainability. 11, 5218. doi: 10.3390/su11195218
Dawkins C. J., Nelson A. C. (2002). Urban Containment Policies and Housing Prices: an international comparison with implications for future research. Land Use Policy. 19, 1–12. doi: 10.1016/S0264-8377(01)00038-2
Ding M. M., Liu W., Xiao L., Zhong F. X., Lu N., Zhang J., et al. (2022). Construction and optimization strategy of ecological security pattern in a rapidly urbanizing region: a case study in central-south China. Ecol. Indic. 136, 108604. doi: 10.1016/j.ecolind.2022.108604
Dong J. Q., Peng J., Liu Y. X., Qiu S. J., Han Y. N. (2020). Integrating spatial continuous wavelet transform and kernel density estimation to identify ecological corridors in megacities. Landsc Urban Plan. 199, 103815. doi: 10.1016/j.landurbplan.2020.103815
Du T. F., Qi W., Zhu X. C., Wang X., Zhang Y., Zhang L. (2020). Precise identification and control method of natural resources space based on ecological security pat tern in mountainous hilly area. J. Ecol. Nat. Resour. 35 (5), 1190–1200. doi: 10.31497/zrzyxb.20200514
Fan F. F., Wen X. J., Feng Z. M., Gao Y., Li W. J. (2021). Optimizing urban ecological space based on the scenario of ecological security patterns: The case of central Wuhan, China. Appl. Geogr. 138, 102619. doi: 10.1016/j.apgeog.2021.102619
Forman R. T. T. (1995). Some general principles of landscape and regional ecology. Landsc Ecol. 10, 133–142. doi: 10.1007/BF00133027
Fu C. H., Xu Y., Bundy A., Gruss A., Coll M., Heymans J. J., et al. (2019). Making ecological indicators management ready: Assessing the specificity, sensitivity, and threshold response of ecological indicators. Ecol. Indic. 105, 16–28. doi: 10.1016/j.ecolind.2019.05.055
Gao J. X., Wang Y., Zou C. X., Xu D. L., Lin N. F., Wang L. X., et al. (2020). China’s ecological conservation redline: A solution for future nature conservation. Ambio 49 (9), 1519–1529. doi: 10.1007/s13280-019-01307-6
Gao J. B., Du F. J., Zuo L. Y., Jiang Y. (2021). Integrating ecosystem services and rocky desertification into identification of karst ecological security pattern. Landsc Ecol. 36, 2113–2133. doi: 10.1007/s10980-020-01100-x
Geng W. L., Li Y. Y., Zhang P. Y., Yang D., Jing W. L., Rong T. Q. (2022). Analyzing spatio-temporal changes and trade-offs/synergies among ecosystem services in the Yellow River Basin, China. Ecol. Indic. 138, 108825. doi: 10.1016/j.ecolind.2022.108825
Gong J., Cao E. J., Xie Y. C., Xu C. X., Li H. Y., Yan L. L. (2020). Integrating ecosystem services and landscape ecological risk into adaptive management: Insights from a western mountain-basin area, China. J. Environ. Manage 281, 111817. doi: 10.1016/j.jenvman.2020.111817
Jin X., Wei L., Wang Y. I., Lu Y. (2021). Construction of ecological security pattern based on the importance of ecosystem service functions and ecological sensitivity assessment: a case study in Fengxian County of Jiangsu Province, China. Environ. Dev. Sustain. 23 (1), 563–590. doi: 10.1007/s10668-020-00596-2
Kayumba P. M., Chen Y. N., Mingje R., Mindje M., Li X. Y., Maniraho A. P., et al. (2021). Geospatial land surface-based thermal scenarios for wetland ecological risk assessment and its landscape dynamics simulation in Bayanbulak Wetland, Northwestern China. Landsc Ecol. 36, 1699–1723. doi: 10.1007/s10980-021-01240-8
Knaapen J. P., Seheffer M., Harms B. (1992). Eetimating habitat isolation in landscape planning. Landsc Urban Plan. 23, 1–16. doi: 10.1016/0169-2046(92)90060-D
Li S. K., He W. X., Wang L., Zhang Z., Chen X. Q., Lei T. C., et al. (2023a). Optimization of landscape pattern in China Luojiang Xiaoxi basin based on landscape ecological risk assessment. Ecol. Indic. 146, 109887. doi: 10.1016/j.ecolind.2023.109887
Li L., Huang X. J., Wu D. F., Wang Z. L., Yang H. (2022a). Optimization of ecological security patterns considering both natural and social disturbances in China’s largest urban agglomeration. Ecol. Eng. 180, 106647. doi: 10.1016/j.ecoleng.2022.106647
Li Z. T., Li M., Xia B. C. (2020). Spatio-temporal dynamics of ecological security pattern of the Pearl River Delta urban agglomeration based on LUCC simulation. Ecol. Indic. 114, 106319. doi: 10.1016/j.ecolind.2020.106319
Li H. F., Su F. L., Guo C. J., Dong L. L., Song F., Wei C., et al. (2023b). Landscape ecological risk assessment and driving mechanism of coastal estuarine tidal flats—a case study of the liaohe estuary wetlands. Front. Environ. Sci. 10. doi: 10.3389/fenvs.2022.1070009
Li C., Wu Y. M., Gao B. P., Zheng K. J., Wu Y., Wang M. J. (2022b). Construction of ecological security pattern of national ecological barriers for ecosystem health maintenance. Ecol. Indic. 146, 109801. doi: 10.1016/j.ecolind.2022.109801
Li H., Zhang T., Cao X. S., Zhang Q. Q. (2022c). Establishing and optimizing the ecological security pattern in Shaanxi province (China) for ecological restoration of land space. Forests. 13, 766. doi: 10.3390/f13050766
Li Y. M., Zhao J. Z., Yuan J., Ji P. K., Deng X. L., Yang Y. M. (2022d). Constructing the ecological security pattern of Nujiang Prefecture Based on the framework of “Importance–Sensitivity–Connectivity”. Int. J. Env. Res. Pub He. 19, 10869. doi: 10.3390/ijerph191710869
Lin Q. X., Eladawy A., Sha J. M., Li X. M., Wang J. L., Kurbanov E., et al. (2021). Remotely sensed ecological protection redline and security pattern construction: a comparative analysis of Pingtan (China) and Durban (South Africa). Remote Sens. 13, 2865. doi: 10.3390/rs13152865
Liu H. M., Xing L., Wang C. X., Zhang H. Y. (2022). Sustainability assessment of coupled human and natural systems from the perspective of the supply and demand of ecosystem services. Front. Earth Sci. 10. doi: 10.3389/feart.2022.1025787
Lou Y. Y., Yang D., Zhang P. Y., Zhang Y., Song M. L., Huang Y. C., et al. (2022). Multi-Scenario simulation of land use changes with ecosystem service value in the Yellow River Basin. Land. 11, 992. doi: 10.3390/land11070992
Luo F. H., Liu Y. X., Peng J., Wu J. S. (2018). Assessing urban landscape ecological risk through an adaptive cycle framework. Landsc Urban Plan. 180, 125–134. doi: 10.1016/j.landurbplan.2018.08.014
Mupepele A. C., Bruelheide H., Bruhl C., Dauber J., Fenske M., Freibauer A., et al. (2021). Biodiversity in European agricultural landscapes: transformative societal changes needed. Trends Ecol. Evol. 36, 1067–1070. doi: 10.1016/j.tree.2021.08.014
Otuoze S. H., Hunt D. V. L., Jefferson I. F. (2021). Monitoring spatial-temporal transition dynamics of transport infrastructure space in urban growth phenomena: a case study of Lagos—Nigeria. Front. Future Transp. 2. doi: 10.3389/ffutr.2021.673110
Ran Y. J., Lei D. M., Li J., Gao L. P., Mo J. X., Liu X. (2022). Identification of crucial areas of territorial ecological restoration based on ecological security pattern: a case study of the central Yunnan urban agglomeration, China. Ecol. Indic. 143, 109318. doi: 10.1016/j.ecolind.2022.109318
Rong T. Q., Zhang P. Y., Zhu H. R., Jiang L., Li Y. Y., Liu Z. Y. (2022). Spatial correlation evolution and prediction scenario of land use carbon emissions in China. Ecol. Inform. 71, 101802. doi: 10.1016/j.ecoinf.2022.101802
Saleem J., Ahmad S. S., Shabbir R. (2022). Evaluating land suitability analysis for urban services planning in coal clusters of punjab using AHP, WOM, and TOPSIS method. Arab. J. Geosci. 15, 324. doi: 10.1007/s12517-022-09510-8
Saleh D., Abeer A. E. K. (2021). Problematic soil risk assessment approach for sustainable spatial suitability of urban land use in new cities by using GIS and remote sensing: a case study of new East Port Said city. Int. J. Sus. Dev. Plann 16, 615–628. doi: 10.18280/IJSDP.160402
Salviano I. R., Gardon F. R., dos Santos R. F. (2021). Ecological corridors and landscape planning: a model to select priority areas for connectivity maintenance. Landscape Ecol. 36 (11), 3311–3328. doi: 10.1007/s10980-021-01305-8
Schiermeier Q., Atkinson K., Mega E. R., Padma T. V., Stoye E., Tollefson J., et al. (2019). Scientists worldwide join strikes for climate change. Nature. 573, 472–473. doi: 10.1038/d41586-019-02791-2
Su Y. X., Chen X. Z., Liao J. S., Zhang H. O., Wang C. J., Ye Y. Y., et al. (2016). Modeling the optimal ecological security pattern for guiding the urban constructed land expansions. Urban For Urban Green. 19, 35–46. doi: 10.1016/j.ufug.2016.06.013
Tang J. J., Zhou L., Dang X. W., Hu F. N., Yuan B., Yuan Z. F., et al. (2023). Impacts and predictions of urban expansion on habitat quality in the densely populated areas: A case study of the Yellow River Basin, China. Ecol. Indic. 151, 110320. doi: 10.1016/j.ecolind.2023.110320
Urban D., Keitt T. (2001). Landscape connectivity: a graph-theoretic perspective. J. Ecol. 82, 1205–1218. doi: 10.2307/2679983
Wang Y., Zou C. X., Xu D. L., Wang L. X., Jin Y., Wu D. (2017a). Identifying ecologically valuable and sensitive areas: a case study analysis from China. J. Nat. Conserv 40, 49–63. doi: 10.1007/s13280-019-01307-6
Wang Y., Gao J. X., Zou C. C., Xu D. L., Wang L. X., Jin Y., et al. (2017b). Identifying ecologically valuable and sensitive areas—A case study analysis from China. J. Nat. Conserv. 40, 1617–1381. doi: 10.1016/j.jnc.2017.08.005
Wang Y. J., Qu Z., Zhong Q. C., Zhang Q. P., Zhang L., Zhang R., et al. (2022a). Delimitation of ecological corridors in a highly urbanizing region based on circuit theory and MSPA. Ecol. Indic. 142, 109258. doi: 10.1016/j.ecolind.2022.109258
Wang Z. Y., Shi P. J., Zhang X. B., Tong H. L., Zhang W. P., Liu Y. (2021). Research on landscape pattern construction and ecological restoration of Jiuquan city based on ecological security evaluation. Sustainability. 13, 5732. doi: 10.3390/su13105732
Wang X. K., Xie X. Q., Wang Z. F., Lin H., Liu Y., Xie H. L., et al. (2022b). Construction and optimization of an ecological security pattern based on the MCR model: a case study of the Minjiang River Basin in Eastern China. Int. J. Environ. Health 19, 8370. doi: 10.3390/ijerph19148370
Warntz W., Woldenberg M. (1967). Geography and the properties of surface, concepts and applications-spatial order (Harvard Papers in Theoretical Geography: Harvard university cambridge mass lab for computer graphics).
Wei Q. Q., Halike A., Yao K. X., Chen L. M., Balati M. (2022a). Construction and optimization of ecological security pattern in Ebinur Lake Basin based on MSPA-MCR models. Ecol. Indic. 138, 108857. doi: 10.1016/j.ecolind.2022.108857
Wei W., Shi S. H., Zhang X. Y., Zhou L., Xie B. B., Zhou J. J., et al. (2020). Regional-scale assessment of environmental vulnerability in an arid inland basin. Ecol. Indic. 109, 105792. doi: 10.1016/j.ecolind.2019.105792
Wei L., Zhou L., Sun D. Q., Yuan B., Hu F. N. (2022b). Evaluating the impact of urban expansion on the habitat quality and constructing ecological security patterns: a case study of Jiziwan in the Yellow River Basin, China. Ecol. Indic. 145, 109544. doi: 10.1016/J.ECOLIND.2022.109544
Wohlfart C., Kuenzer C., Chen C., Liu G. H. (2016). Social–ecological challenges in the Yellow River basin (China): a review. Environ. Earth Sci. 75, 1866–6280. doi: 10.1007/s12665-016-5864-2
Xu W. X., Wang J. M., Zhang M., Li S. L. (2021). Construction of landscape ecological network based on landscape ecological risk assessment in a large-scale opencast coal mine area. J. Clean. Prod. 286, 125523. doi: 10.1016/j.jclepro.2020.125523
Yang C., Deng W., Yuan Q. Z., Zhang S. Y. (2022a). Changes in landscape pattern and an ecological risk assessment of the Changshagongma wetland nature reserve. Front. Ecol. Evol. 10. doi: 10.3389/fevo.2022.843714
Yang D., Lou Y. Y., Zhang P. Y., Jiang L. (2022b). Spillover effects of built-up land expansion under ecological security constraint at multiple spatial scales. Front. Ecol. Evol. 10. doi: 10.3389/fevo.2022.907691
Yang K., Wang S. F., Cao Y. G., Li S. P., Zhou W. X., Liu S. H., et al. (2022c). Ecological restoration of a loess open-cast mining area in China: perspective from an ecological security pattern. Forests. 13, 269. doi: 10.3390/f13020269
Yang D., Zhang P. Y., Liu Z. Y., Huang Y. C., Chen Z., Chang Y. H., et al. (2023). How does urbanization process affect ecological landscape pattern? an empirical analysis based on scale effects. Ecol. Indic. 154, 110921. doi: 10.1016/j.ecolind.2023.110921
Yi S. Q., Zhou Y., Li Q. (2022). A new perspective for urban development boundary delineation based on the MCR model and CA-Markov model. Land. 11, 401. doi: 10.3390/land11030401
Yu K. J. (1999). Landscape ecological security patterns in biological conservation. Acta Eco. Sin. 1, 10–17. doi: 10.1016/S0169-2046(96)00331-3
Yu H. C., Huang J., Ji C. N., Li Z. A. (2021). Construction of a landscape ecological network for a large-scale energy and chemical industrial base: a case study of Ningdong, China. Land. 10, 344. doi: 10.3390/land10040344
Zhang Y. Z., Jiang Z. Y., Li Y. Y., Yang Z. G., Wang X. H., Li X. B. (2021). Construction and optimization of an urban ecological security pattern based on habitat quality assessment and the minimum cumulative resistance model in Shenzhen city, China. Forests. 12, 847. doi: 10.3390/f12070847
Zhang X. Y., Liu G., Zheng Z. (2022a). Construction of an ecological security pattern based on functional wetland theory: A case study in a landscape city. Front. Environ. Sci. 10. doi: 10.3389/fenvs.2022.955230
Zhang P. Y., Yang D., Qin M. Z., Jing W. L. (2020). Spatial heterogeneity analysis and driving forces exploring of built-up land development intensity in Chinese prefecture-level cities and implications for future Urban Land intensive use. Land Use Policy. 99, 104958. doi: 10.1016/j.landusepol.2020.104958
Zhang J. B., Zhu H. R., Zhang P. Y., Song Y. P., Zhang Y., Li Y. Y., et al. (2022b). Construction of GI network based on MSPA and PLUS model in the main urban area of Zhengzhou: a case study. Front. Environ. Sci. 10. doi: 10.3389/fenvs.2022.878656
Zhelonkina E. E., Pafnutova I. D., Emelyanova T. A. (2021). Conditions for territorial biodiversity conservation affected by technogenesis in the North. IOP Conf. Environ. Earth Sci. 867, 12075. doi: 10.1088/1755-1315/867/1/012075
Zhou T. Y., Liu H. M., Gou P., Xu N. (2023). Conflict or Coordination? measuring the relationships between urbanization and vegetation cover in China. Ecol. Indic. 147, 109993. doi: 10.1016/j.ecolind.2023.109993
Zhu K. W., Chen Y. C., Zhang S., Yang Z. M., Huang L., Lei B., et al. (2020). Identification and prevention of agricultural non-point source pollution risk based on the minimum cumulative resistance model. Glob. Ecol. Conserv. 23, e01149. doi: 10.1016/j.gecco.2020.e01149
Zhu Z. Y., Mei Z. K., Xu X. Y., Feng Y. Z., Ren G. X. (2022). Landscape ecological risk assessment based on land use change in the Yellow River Basin of Shaanxi, China. Int. J. Env. Res. Pub He. 19, 9547. doi: 10.3390/ijerph19159547
Keywords: landscape ecological risk assessment, ecological security patterns, spatial principal component analysis, minimum cumulative resistance model, the affected area of the Lower Yellow River
Citation: Huang Y, Zhang J, Zhang P, Chen Z, Zhang X, Lu R, Li M, Xing G and Song Y (2023) Construction and optimization of ecological security pattern based on landscape ecological risk assessment in the affected area of the Lower Yellow River. Front. Ecol. Evol. 11:1271352. doi: 10.3389/fevo.2023.1271352
Received: 02 August 2023; Accepted: 18 October 2023;
Published: 01 November 2023.
Edited by:
Xiao Ouyang, Hunan University of Finance and Economics, ChinaReviewed by:
Kunlun Qi, China University of Geosciences Wuhan, ChinaLiang Zhou, Lanzhou Jiaotong University, China
Yue Liu, Guangzhou Institute of Geography, China
Copyright © 2023 Huang, Zhang, Zhang, Chen, Zhang, Lu, Li, Xing and Song. This is an open-access article distributed under the terms of the Creative Commons Attribution License (CC BY). The use, distribution or reproduction in other forums is permitted, provided the original author(s) and the copyright owner(s) are credited and that the original publication in this journal is cited, in accordance with accepted academic practice. No use, distribution or reproduction is permitted which does not comply with these terms.
*Correspondence: Yongpeng Song, MTAxMzAwODVAdmlwLmhlbnUuZWR1LmNu; Pengyan Zhang, cGVuZ3lhbnpoQDEyNi5jb20=