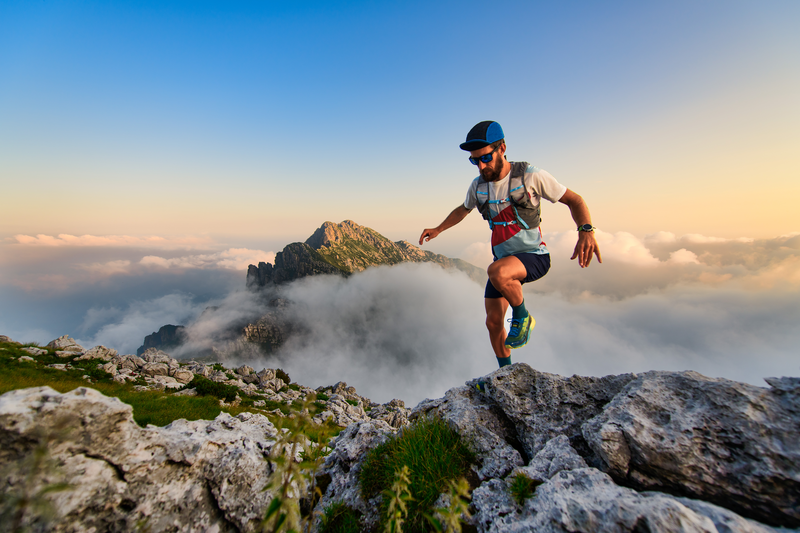
94% of researchers rate our articles as excellent or good
Learn more about the work of our research integrity team to safeguard the quality of each article we publish.
Find out more
ORIGINAL RESEARCH article
Front. Ecol. Evol. , 03 January 2024
Sec. Conservation and Restoration Ecology
Volume 11 - 2023 | https://doi.org/10.3389/fevo.2023.1250892
This article is part of the Research Topic Large-Scale Dam Removal and Ecosystem Restoration View all 25 articles
The contribution of two basal energy sources – detrital organic matter and primary producers – as part of aquatic food webs varies typically along river continua. A host of barriers to river flow increase the water residence time and sediment and nutrient retention in reservoirs worldwide, and potentially alter the balance between detritus-based and algae-based energy pathways in the downstream food webs. We explored this issue on the Sélune River (Normandy, France), a small coastal stream that drains an agricultural catchment with high sediment runoff. Seasonal measurements of the following parameters were compared upstream and downstream of the reservoirs of two large dams (16 m and 36 m high): sediment fluxes, nutrient and chlorophyll a concentrations, algal communities in the epilithic biofilm (taxonomic composition, biomass and growth), and benthic invertebrate communities (abundance and trophic guild structure). As anticipated, annual sediment fluxes were much lower downstream of the reservoirs, where significant decreases in water turbidity, phosphate and silicate concentrations were recorded. A higher chlorophyll a concentration in water and a higher contribution of pelagic algae taxa to the photosynthetic biofilm suggested drifting and deposition of reservoir-borne phytoplankton downriver. Photosynthetic biofilm growth was higher downstream of the reservoirs in spring and fall, and so was the abundance of herbivores in the invertebrate community, notably scrapers and algae eaters. Energy pathways within riverine food webs were traced using stable isotope analyses of carbon (C) and nitrogen in the tissues of aquatic consumers (invertebrates and fish). Mixing models revealed a discontinuity in the origin of the C entering the food webs along the river continuum, confirming a greater contribution of algal C to aquatic consumers downstream of the reservoirs. These results illustrate mechanisms whereby large reservoirs can modulate C flow in food webs along a small coastal river with high sediment loads, and make it possible to anticipate the effects of dam removal on the future river ecosystem.
Two major energy pathways exist in river food webs. On the one hand, dissolved nutrients and inorganic carbon (C) fuel primary producers, namely phytoplankton, benthic algae and aquatic plants (Elser et al., 2007; Bumpers et al., 2017). The organic C resulting from photosynthesis is called autochthonous C. It is available to herbivores and other consumers at higher trophic levels, and fuels the algae-based pathway. The dissolved inorganic carbon (DIC) that enters this energy pathway is a mix of terrestrial (HCO3 and dissolved CO2 from chemical weathering, soils and groundwater), aquatic (ecosystem respiration) and atmospheric (diffusion of CO2 at the air–water interface) sources (Mook and Tan, 1991; Finlay, 2003; Liu et al., 2011). Algae provide high-quality food resources to primary consumers (Guo et al., 2016a; Guo et al., 2016b), and the prevalence of algae-derived C in aquatic consumers has been reported in many river food webs (Mayer and Likens, 1987; Thorp and Delong, 2002; Lau et al., 2009; Brett et al., 2017). On the other hand, the detritus-based pathway involves primary consumers (fungi, bacteria and invertebrates) relying on dissolved or particulate organic C, notably fragments of dead plants, animals and feces. The available pool of detrital C is a combination of autochthonous C from aquatic organisms and allochthonous C from terrestrial ecosystem inputs, notably leaf litter from riparian trees (review by Marks, 2019). The influence of the detritus-based pathway on aquatic food web functioning is expected to vary along the river continuum. In theory, detrital C should prevail in headwater reaches receiving high allochthonous inputs from riparian trees (“river continuum concept” (RCC), Vannote et al., 1980). For instance, in small tributaries where a dense tree canopy strongly limits solar radiation, aquatic consumers mostly rely on C derived from riparian inputs (Roussel et al., 2021). According to the RCC, aquatic primary producers mostly support river food webs further downstream in intermediate reaches, while detrital and terrestrial C become dominant again in large rivers where primary production is hampered by water depth and turbidity. This pattern has been debated for large river ecosystems subjected to seasonal flooding, where macrophytes and/or floodplain plants can be a major C source in food webs (“flood pulse concept”, Junk et al., 1989). Various case studies in the literature support different predictions on the predominant C sources and energy pathways in large river food webs (Hoeinghaus et al., 2007; Roach, 2013; Soto et al., 2019; Wang et al., 2021).
In addition to natural variations along the river continuum, human activities can modify the expected balance between the algae-based and detritus-based pathways in river food webs. By stimulating primary production, anthropogenic nutrient inputs – notably N and P – have major effects on freshwater ecosystems, including algal blooms (Galloway et al., 2004; Diaz and Rosenberg, 2008; Paerl et al., 2011; Penuelas et al., 2013). Such an increase of the primary producer biomass can reduce the DIC concentration, promote the diffusion of atmospheric CO2 into the water (Portielje and Lijklema, 1995; Schindler et al., 1997), and ultimately change the origin of C cycling in aquatic environments (Brenner et al., 1999; Roussel et al., 2014). Anthropogenic nutrients from non-point-source pollution also change microbial activity on detrital C and its assimilation by primary consumers (Rosemond et al., 2015; Guo et al., 2016b) and modulate the detritus-based pathway. Another obvious impact of human activity on river food webs is observed when a large amount of organic waste offers alternative resources of high nutritional value to primary consumers in benthic invertebrate communities (Camargo, 1992; Guilpart et al., 2012) and enhances the detritus-based pathway downstream of the inputs (Roussel et al., 2018; de Carvalho et al., 2020). The algae-based and detritus-based pathways within river food webs are under the influence of a complex balance that depends on the position along the river continuum and anthropogenic pressures. Therefore, the origin of the C inputs at the base of river food webs and C flow to higher trophic levels can significantly change when the availability of detrital C and/or nutrients to aquatic organisms is altered.
A host of barriers constrain natural river flows worldwide (Grill et al., 2019). In a recent review, Belletti et al. (2020) concluded to a mean density of 0.74 barrier per kilometer of river in Europe. By creating impoundments, barriers to river flow increase the water residence time and have major consequences on the natural process of sediment transfer. Using satellite imagery analyses on major rivers, Dethier et al. (2022) estimated that dams have halved the global sediment flux from lands to seas in the northern hemisphere. Large dams and reservoirs can trap and store huge quantities of suspended materials, including detrital C that is no longer available to downstream river ecosystems, estuaries and marine coastal areas (Syvitski et al., 2005; Zarfl and Dun, 2022). Moreover, large reservoirs affect nutrient biogeochemistry and strongly modulate the downriver transfer of dissolved nutrients (review by Maavara et al., 2020). Phosphorus (P) is essential to freshwater primary producers in its dissolved inorganic form, but uptake by phytoplankton in reservoirs alter its availability to downstream food webs (Lu et al., 2016; Bao et al., 2018). After decades of impoundment, sediments trapped in reservoirs contain large quantities of legacy P, which can be released through various physical and biogeochemical processes (Orihel et al., 2017). Similarly, the blooming and sinking of silica diatoms in reservoirs deplete the dissolved silicon (DSi) available downriver and in marine areas (Tréguer and de la Rocha, 2013) during specific periods of the year (Chen et al., 2014). The intensity of depletion is correlated with the water residence time in reservoirs (Ma et al., 2018). In some cases, large dams and reservoirs massively reduce all dissolved nutrients (P, N, DSi) down to the ocean (Gupta et al., 2021). The diversity of patterns reported in the literature outlines that the complex biochemical processes occurring in large reservoirs alter both particulate and solute fluxes, and in turn (theoretically) the balance between the algae-based and detritus-based pathways and C flow in downriver aquatic food webs. However, literature on this issue mostly relies on the study of dams and reservoirs on large rivers, but the ecological processes at play in small river catchments have retained less attention to date.
We explored the effect of two large dams and their reservoirs on aquatic communities and the energy flow in the food web of a small coastal stream – the Sélune River, an 85-km stream discharging into the Bay of Mont Saint-Michel (Normandy, France). In the early 20th century, two large hydropower dams (16 and 36 m high) were constructed in the lower third of the catchment, resulting in two consecutive reservoirs that covered 19 km of the initial river course. Agriculture is a dominant activity in the catchment; annual rainfall and erosion result in high quantities of suspended sediment and dissolved nutrients reaching the river (Fovet et al., 2020). In this context, we expected that high inputs of terrestrial organic matter and water turbidity would drive the aquatic ecosystem toward detritus-based functioning. We predicted that by hindering the transfer of suspended sediment, the dams and reservoirs could enhance the algae-grazer pathway and the contribution of algal C to aquatic biota downriver. Seasonal measurements of sediment fluxes, nutrient concentrations, photosynthetic activity, benthic algae and invertebrate communities together with stable isotope analyses of aquatic fauna were performed upstream and downstream of the reservoirs to test these assumptions. The broader goal of this work was to establish a baseline pattern of the food web of the Sélune River with its two reservoirs in order to evaluate river recovery after the scheduled removal of the two dams.
The Sélune is a 85 km long river that drains a 1,083 km2 watershed and flows into the Mont Saint-Michel Bay in Normandy (France) (Figure 1). Climate is oceanic, with mean annual rainfall of ca. 800 mm, and high and low flow periods occurring in winter and early fall, respectively. The median daily flow is 5 m3·s−1, the total monthly flow varies from 106 m3·month−1 during severe summer drought up to 120·106 m3·month−1 during high flood in winter (Fovet et al., 2020). The bedrock is composed of granite and Brioverian schist. Mixed crop–livestock farming covers 85% of the watershed area, with arable land covering 46% of this surface and grassland 39% (https://www.theia-land.fr/en/product/land-cover-map/). The watershed is vulnerable to soil erosion, hence high water turbidity and sediment loads are recorded (Vongvixay et al., 2018). In the early 20th century, two large hydropower dams (Figure 1) were built on the river: La-Roche-qui-Boit (16 m high and 125 m long, reservoir volume 90·103 m3) and Vezins (36 m high and 278 m long, 18·106 m3). Depending on the season, the mean water residence time varied from 1 to 4 days in La-Roche-Qui-Boit, and from 14 to 47 days in Vezins (Fovet et al., 2020). The two reservoirs covered a total distance of 19 km, which is more than 20% of the initial river course (Figure 1). Owing their volume, spatial coverage and high water residence time, they were considered as large reservoirs on such a watershed. Water in the reservoirs was nutrient-rich; the mean summer nutrient concentrations varied between 7.4 and 16.5 μg·L−1 P-PO4 and between 4.0 and 5.3 mg·L−1 N-NO3 along the water column (Fovet et al., 2020).
Figure 1 The Sélune River watershed, with two dams (black rectangles), two reservoirs (in green) and the study sites (red dots, S1 to S9). Distances, from source (0 km) to bay (85 km): S1 (15 km), S2 (23 km), S3 (29 km), S4 (34 km), S5 (39 km), S6 (42 km), Vezins dam (57 km), La-Roche-Qui-Boit dam (61 km), S7 (62 km), S8 (65 km), S9 (70 km).
Considering the low hydropower capacity (27 GWh) of the two dams, potential safety issues because of the condition of their structure, periodic toxicity events in summer associated with cyanobacterial blooms in the reservoirs, and law on diadromous fish species, dismantling was decided by the French government in 2009. Operations started in spring 2017 by emptying Vezins reservoir, while dismantling of Vezins dam was completed by summer 2020. The same procedure for La-Roche-Qui-Boit started in 2021 and ended in early 2023. A long-term multidisciplinary scientific program (2012–2027) aims at evaluating the Sélune River restoration processes after dam removal (https://programme-selune.com). The present study is part of this program, and focuses on the pre-removal period, i.e. before spring 2017. The overall work took place at nine flowing sites located along the river continuum (Figure 1). For financial reasons it was not possible to record all variables (i.e. water discharge, sediment and nutrient concentrations, algal communities in epilithic biofilm and benthic invertebrate communities; see below for description) at each site. However, for each variable, the sampling design allowed comparisons between sites located upstream and downstream of the reservoirs. The choice of a site was constrained by specific criteria. For sediment and water chemistry, auto-samplers needed power supply and support made of concrete on bankside, whereas biofilm was studied with fragile glass slides installed mid-channel in sunny, shallow but not too fast-flowing habitats to avoid damage. For benthic invertebrate collection, we favored easily wadable sites with a great variety of substrates. An overall criterion was that the habitat conditions at upstream and downstream sites were as similar as possible for each variable. This explains why S5 or S6 (upstream), and S7 or S8 (downstream) sites were chosen depending on the variable to record (Figure 1). These sites (S5 and S6; S7 and S8) were very close (about 2 km) one from another, and we did not expect any bias coming out of this. The Airon tributary increases the Sélune River discharge between S5 and S6, but it does not cause major differences in dissolved nutrients as it drains an area with similar soils and land use pattern on the Sélune River catchment. Finally, we extended our sampling to eight sites for stable isotope analysis on animal tissues to get a clearer picture of food web shifts along the river gradient (see Section 2.5).
Water, sediment and solutes were monitored at S6 (upstream the reservoirs) and S8 (downstream the reservoirs) from January 2015 to March 2017 (Figure 1). The water level, temperature and turbidity were recorded at a (sub-)hourly time step (1h at S6; 6 min at S8) using dedicated sensors (Hach Lange SOLITAX sc). Water discharge was computed from the measured water level using a rating curve established and kindly provided by DREAL 50 (State office in charge of hydrometric monitoring) and EDF (Electricité de France, the hydropower company). At both sites, grab samples were collected weekly, and ISCO autosamplers collected 10–20 samples (800 mL each) during storm events (see Fovet et al., 2020 for details). In the laboratory, a subsample from each sample was filtered at 0.45 µm on nitrate cellulose filters, dried at 105°C and weighed. The filtered volume (at least 350 mL) was measured to determine the suspended solids (SS) concentrations (EN 872:2005, 2005). A 30-mL aliquot of filtered water was used to measure NO3 concentrations by ionic chromatography (Dionex ICS3000, EN 10304-1:1995, 1995), and dissolved silica (DSi) and orthophosphate (PO4) concentrations by colorimetry (Seal Analytical AQ2, NF T 90-007:2001, 2001; EN 6878:2004, 2004). Another subsample was filtered at 0.45 µm on glass fiber filters and used for analyzing the carbon (C) and nitrogen (N) contents in SS particles using an Elemental Analyzer (Thermo Finnigan FLASH EA 1112).
Data were pooled by site (S6 and S8) and season: winter (January–March), spring (April–June), summer (July–September), and fall (October–December). Specific discharge was calculated by dividing the discharge values (m3·s−1) by the drained surface (629 km2 at S6 and 777 km2 at S8) to compare discharge between sites. The molar C:N ratio of SS was used as a proxy of the origin of particulate organic matter, considering that values above or below eight suggest terrigenous or phytoplanktonic origin, respectively (Hedges et al., 1997; Kendall et al., 2001; Balakrishna and Probst, 2005). We fitted a regression between the SS concentrations and the turbidity values at S6 and S8 to estimate the SS fluxes from turbidity time series. Regression coefficients and their 95% confidence intervals were used to compute instantaneous loads of SS and uncertainty associated with the regression coefficients, and then cumulated over the water years. “Site” (upstream or downstream the reservoirs) and “season” (winter, spring, summer and fall) effects were tested with two-way ANOVAs followed by Tukey’s post-hoc test (p < 0.05) on the following response variables: water discharge, turbidity, SS concentrations, the C:N ratio, phosphate, nitrate and dissolved silicon.
Phytoplankton biomass was measured monthly at S5 (upstream the reservoirs) and S8 (downstream the reservoirs) by fluorescence using a multiparametric probe (Idronaut Ocean Seven 316Plus CTD, Milan, Italy) from January 2015 to October 2016. Water samples were collected monthly at the sub-surface and in mid-channel to measure chlorophyll a concentrations after filtration on Whatman GF/F glass-fiber filters and extraction overnight at 4°C in 90% acetone (Lorenzen, 1967). Absorbance was measured at 665 nm and 750 nm with a spectrophotometer, before and after acidification, following Lorenzen’s method (Lorenzen, 1967).
The photosynthetic biofilm was studied from January 2015 to October 2016 on a monthly basis. Four glass slides (30 × 10 × 3 mm) were installed vertically in opened plastic boxes with perforated sides to allow water flow. The boxes were covered with a plastic mesh (2 cm mesh size) to prevent large debris from entering. They were anchored in the middle of the riverbed at S5 and S8, and the glass slides were changed every month, i.e. after one month of incubation (Biggs, 1988; Morin et al., 2008). The boxes were occasionally inaccessible due to high water flow, or damaged. However, we successfully retrieved glass slides in winter (once at S8), spring (six times at S5 and six times at S8), summer (six times at S5 and six times at S8), and fall (one and two times at S5 and S8, respectively). Chlorophyll a, ash-free dry mass (AFDM), taxa and photosynthetic activity were evaluated on separate glass slides.
For chlorophyll a, the glass slide was scraped on both sides using a razor blade to retrieve the biofilm, which was immediately stored in a plastic tube, kept in the dark in a cooling box in the field, and then freeze-dried in the laboratory. Overnight extraction with acetone was performed before spectrophotometric measurements following Lorenzen’s method (Lorenzen, 1967). The chlorophyll a concentration (µg·L−1), the incubation duration and the glass slide surface were used to calculate the biofilm biomass increase in time, expressed in µg·cm−²·d−1. For AFDM, the biofilm was scraped in the same way as for chlorophyll a, oven-dried at 105°C for 48 h to get total dry mass (algae, bacteria, fungi, and detritus, in g·m−²), and then burnt for 1 h at 500°C to obtain AFDM. The percentage of organic matter was expressed as the AFDM:dry mass ratio. The photosynthetic biomass:total biomass ratio corresponding to the autotrophic index (Weber, 1973) was calculated to describe the trophic nature of the biofilm (autotrophic versus heterotrophic). For taxonomic identification, the biofilm was scraped off from the slide, immediately fixed in Lugol solution and kept in the dark at 4°C. Microalgae were identified and counted using a light microscope (Leica DM4000B) and a Nageotte counting chamber. Taxa were classified as benthic, benthopelagic, planktonic, or unknown according to the literature (Germain, 1981; Bourrelly, 1990; John et al., 2002; Rimet and Bouchez, 2012) and the Diatoms of North America online database (https://diatoms.org/).
Finally, we measured the photosynthetic activity of the biofilm on the fourth glass slide right after its removal from the river. Repeated measurements of fluorescence signals were performed using a four-wavelength-excitation pulse amplitude modulation fluorometer (Phyto-PAM, Heinz Walz® GmbH, Effeltrich, Germany) equipped with an emitter-detector fiberoptics unit. The fiber device was placed at 2 mm from the glass slide surface with a 4-cm diameter adapter to control irradiance. The PAM calculates photosynthesis as the relative electron transport rate (rETR) through photosystem II (µmol e−·m−2·s−1) at different levels of light (Kromkamp and Forster, 2003). After 15 min of dark adaptation, rapid photosynthesis-light curves (RLC) were performed in triplicate with increasing light intensities (16 to 265 mmol photons·m−2·s−1), with a 20 s time interval (Jakob et al., 2005). The light-saturated maximum rETR was calculated by applying the nonlinear least squares regression model of Eilers and Peeters (1988) to fit the rETR-irradiance curves. “Site” and “season” effects were tested separately with two-way ANOVAs followed by Tukey’s post-hoc test (p < 0.05) for chlorophyll a, AFDM, taxonomic identification and photosynthetic activity.
A Surber net sampler (0.05 m², 0.5 mm mesh size) was used to collect benthic invertebrates at S5 (upstream the reservoir) and S7 (downstream the reservoir) in fall (October 2014 and 2015) and spring (April 2015 and 2016), following the NFT 90-333 protocol and the Water Framework Directive (2000/60/EC). Twelve samples were collected per date and site to encompass the diversity of aquatic habitats in the river channel (96 samples in total, all dates and sites included). The samples were fixed with 96% ethanol and stored. In the laboratory, each sample was sorted under a binocular microscope, and taxa were identified down to the genus or species level (crustaceans, molluscs, insect larvae) or family/tribe levels (Diptera); Nematoda and Hydracarina were not identified further.
Following Tachet et al. (2010), two traits associated to invertebrate diet were considered: food items (9 modalities) and feeding behavior (8 modalities). For a given taxon and diet trait, an affinity score was assigned to each modality, ranging from “no affinity” (zero) to “high affinity” (3 for feeding behavior, 5 for food items). These values provide information on the intensity of the link between the taxa and each modality (i.e. low or high affinity) and the amplitude of the preference or tolerance of the taxon (i.e. the number of modalities used). We retained 8 modalities connected to the diet of primary consumer taxa for further analysis: deposit feeder, shredder, filter feeder, scraper (feeding behavior traits), small (<1 mm) and large (>1 mm) organic debris, and algae and macrophytes (food item traits). For each site and season, the diet trait modality affinities of the taxa (expressed as frequencies) weighted by their abundances were summed to determine the number of individuals in each modality at the community level (see Usseglio-Polatera et al., 2000 for further details). We run PERMANOVA on log(x +1) transformed data to test differences in community structure according to the diet traits (feeding behavior and food items). Then we tested for differences in the number of individuals in each modality to identify which modalities drove the difference observed for each diet trait, using non-parametric analysis of variance of aligned rank transformed data (Wobbrock et al., 2011) since the data did not meet the assumptions of parametric ANOVA. “Site” (2 levels), “season” (2 levels) and their interactions were considered in both PERMANOVA and non-parametric analysis of variance. The vegan 2.5-7 (Oksanen et al., 2020) and ARTool 0.11.1 (Kay et al., 2021) R packages were used for PERMANOVA and variance of aligned rank transformed data, respectively.
Primary and secondary consumers were sampled in summer (July 2014 and July 2015) at sites upstream (S1, S2, S3, S4, S5) and downstream (S7, S8, S9) of the reservoirs (Figure 1). A Surber sampler was used to collect benthic invertebrates, which were identified at the family level and sorted into three feeding behavior categories: herbivores, detritivores, and omnivores (see Table S1 for list of taxa) following Tachet et al. (2010) and Usseglio-Polatera et al. (2000). Each invertebrate was cleaned and stored individually in a microtube. For the smallest taxa, up to 10 specimens were pooled to reach the minimum weight for stable isotope analyses (see below). Six to 18 samples per feeding group were collected at each site and date, depending on availability. Small invertivorous fish were caught by electrofishing in shallow, coarse substratum habitats (see Table S1 for list of taxa). Depending on their availability, 10–30 individuals were collected at each site, their total body length ranging between 50 and 129 mm. Lamprey larvae were caught by electrofishing in the soft substrate habitats where they had buried; catch success and body length varied greatly among sites (1–10 individuals, 60–160 mm, respectively). Crayfish were caught by electrofishing at sites S1 and S5 only (60 and 54 samples, respectively, body length 15–41 mm from rostrum to telson). Fish and lamprey were anaesthetized using a benzocaïne bath, and all animals (fish, lamprey, crayfish) were euthanized and kept in a cooler in the field. The experiment complies with the French regulations on animal care and ethics (license number R-2012-JLB-02 and 201602051204637 delivered to INRAE) and electrofishing survey (permit number 2014-DDTM-SE-0036 and 2015-DDTM-SE-0019 delivered to INRAE).
Dorsal muscle (fish) or abdominal muscle (crayfish) samples were dissected in the laboratory, with special attention to avoid skin, scales and bones. Invertebrate and muscle samples were freeze-dried, ground to a homogenous powder using a mixer mill, weighed (ca. 0.9–1.1 mg) and encapsulated in tin foil. 13C:12C, 15N:14N and C:N ratios were measured by continuous-flow isotope-ratio mass spectrometry, using mass spectrometers (Delta Plus XP and Delta V Plus, Thermo Finnigan) interfaced with elemental analyzers (Carlo Erba NC2500 and Costech 4010, Thermo Finnigan). Isotopic ratios were expressed using the conventional δ notation as parts per thousand (‰). Five hundred and five samples were run in four separate batches. Repeat analyses of 12–15 certified standards showed maximum standard deviations (SD) of 0.29 (δ13C) and 0.38 (δ15N). Thirty-two samples were analyzed twice (i.e. 32 duplicates); the SD values of the duplicates were constantly lower than the SD values of the standards. All stable isotope analyses were performed at the Stable Isotopes in Nature Laboratory, University of New Brunswick (Canada).
C:N ratios were checked to identify possible lipid bias in δ13C values (Post et al., 2007). When C:N ≥ 3.5, the δ13C value was corrected using the equations of Post et al. (2007) for aquatic organisms. δ13C–δ15N biplot and the standard ellipse areas (SEAc, sample size-corrected for n < 30) that integrated 75% of the sample variance (also called isotopic niche breadths) were drawn to visualize the isotopic niches of aquatic consumers upstream (S1 to S5) and downstream (S7 to S9) of the reservoirs, using SIBER (Jackson et al., 2011). The tRophicPosition package (Quezada-Romegialli et al., 2018) was used to estimate the trophic position of consumers and the discrimination factor between C of algal and detrital origins (α), i.e. the contribution of each C source to the feed of consumers. Calculations were based on Bayesian models using stable C and N isotopes and combined with Markov chain Monte Carlo simulations (60,000 iterations). A two-baseline model with trophic enrichment factors on C and N was run for each site separately, using isotopic values of herbivores (primary consumers of algal C) and detritivores (primary consumers of detrital organic C) as end-members. The diet-tissue fractionation values of +3.7 ‰ δ15N between mixed invertebrate diet and fish proposed by Bunn et al. (2013) for streams and rivers, and of +0.4 ‰ δ13C per trophic level (Post, 2002) were used. Because mixing models are highly sensitive to variations in discrimination factors (Bond and Diamond, 2011), we kept the high SDs (± 2.4 for δ15N and ±1.3 for δ13C) proposed as priors in Bayesian models in the literature to better reflect uncertainties in posterior estimates.
The median seasonal values of specific discharge were similar downstream (S8) and upstream (S6) of the reservoirs (Figure 2A), in line with the dam management rules whereby the water flow downstream of the dams should mimic the natural flow variations recorded upstream. Median seasonal turbidity varied between 0 and 1098 NTU and was lower downstream (Figure 2B). Similarly, the seasonal suspended solid (SS) concentrations were 85–88% lower downstream (Figure 2C). R-square values for the fitted regressions between turbidity and SS were 0.96 and 0.84 at S6 and S8, respectively. Using regressions, the estimated sediment fluxes were 19.2 t·km−2·yr−1 (±11%) upstream and 11.6 t·km−2·yr−1 (±14%) downstream for the September 2015–August 2016 water year (Table 1). The average (± SD) C:N molar ratios of SS were higher upstream, notably in spring (11.1 ± 0.97 vs. 7.54 ± 0.83) and summer (9.43 ± 0.94 vs. 7.82 ± 1.47), indicating a greater contribution of phytoplankton-derived particles to organic matter in the SS downstream of the reservoirs (Figure 2D). The mean NO3, PO4 and DSi concentrations (Figures 2E–G) varied seasonally. P concentrations were significantly and systematically lower downstream (p < 0.001) except in winter, whereas between-site variations in N concentrations were not consistent. Similarly, DSi was lower downstream in all seasons except winter (p < 0.001).
Figure 2 Seasonal variations of specific discharge (A), water turbidity (B), the suspended solid concentration (C), the molar ratio of particulate C:N (D), phosphates (E), nitrates (F) and dissolved silicon (G) concentrations in the Sélune River, upstream (white) and downstream (gray) of the reservoirs. Each box plot shows the 25th and 75th percentiles around the median; vertical lines represent 1.5 times the interquartile range and dots are outliers. Stars indicate significant differences between upstream and downstream sites (***p < 0.001; *p < 0.05).
Table 1 Total rainfall and estimated runoff and sediment flux upstream and downstream of the Sélune River dams from January 2015 to March 2017.
The chlorophyll a concentration in the water was higher downstream of the reservoirs irrespective of season, and the difference was significant in summer (Figure 3A; p < 0.01). The N:P molar ratio based on N-NO3 and P-PO4 concentrations ranged from 180 to 10,300 between sites and seasons (Figure 3B) and were significantly higher downstream of the reservoirs in summer and fall (p < 0.001), indicating potential P limitation for primary producers downstream. The percentage of organic matter in the biofilm samples was significantly lower upstream in spring (p < 0.001), indicating more inorganic sediment embedded in the biofilm matrix (Figure 3C). The biofilm algal community upstream of the dams mostly contained benthic species (85.6 ± 10.9%) and a few planktonic (2.9 ± 1.2%) and benthopelagic (6.1 ± 5.8%) species (Figure S2). Conversely, the contribution of planktonic and benthopelagic species increased downstream to average 8.2 ± 6.6% and 17.6 ± 19.4%, respectively (Figure S2). Algal biomass growth (chlorophyll a, μg·cm–2·d–1) and photosynthetic activity (maximum rETR, µmol e–·m–2·s–1) in the biofilm decreased in fall and winter to reach values close to zero (Figures 3D, E). Biomass growth was higher in spring and fall downstream of the reservoirs, yet not significantly so. The maximum rETR values were very similar upstream and downstream of the reservoirs. Median values of the autotrophic index ranged between 1.9 and 2.3 in spring and summer upstream and downstream the reservoirs, indicating a biofilm where autotrophy and heterotrophy were balanced (Figure 3F), while values tended to increase toward heterotrophy in fall and winter upstream of the reservoirs (p < 0.02).
Figure 3 Seasonal variations of the chlorophyll a concentration in water (A) and of the dissolved N:P molar ratio based on N-NO3 and P-PO4 concentrations (B), and, for the photosynthetic biofilm: the percentage of organic matter (C), the biomass increase per day (D), photosynthetic activity (E) and the autotrophic index (F) in the Sélune River, upstream (white) and downstream (gray) of the reservoirs. Stars indicate significant differences between the upstream and downstream sites (*p < 0.05).
Irrespective of season, the abundance of benthic invertebrates caught in Surber net samples was higher downstream (12,273 individuals in spring; 17,267 in fall) than upstream (8,927 in spring; 6,613 in fall). A total of 45,080 individuals were sorted and identified. Among them, 39,653 (88.0%) and 36,332 (80.6%) were identified as primary consumers according to their feeding behavior trait (deposit feeder, shredder, filter feeder, scraper) or food item trait (small or large organic debris, algae, macrophytes), respectively. The most influential taxa are listed in Table S3.
The community structure of invertebrate primary consumers significantly differed between sites for the food item trait (PERMANOVA, Site pseudo-F = 15.26 and p = 0.001; Season pseudo-F = 1.41 and p = 0.2; Station × Season pseudo-F = 0.69 and p = 0.5) and for the feeding behavior trait (Site pseudo-F = 16.59 and p = 0.001; Season pseudo-F = 1.28 and p = 0.2; Station × Season pseudo-F = 1.0 and p = 0.3) but not between seasons (Figure 4). Primary consumer taxa were significantly more abundant downstream than upstream of the reservoirs, regardless of the modalities and traits (analysis of variance on aligned rank transformed data, see Table 2). No significant seasonal variation was detected except for filter feeders that were more abundant downstream the reservoirs in fall only. Scrapers and algae eaters were the two most abundant modalities found among primary consumers irrespective of season.
Figure 4 Abundance of invertebrate primary consumers in the Sélune River upstream (white) and downstream (gray) of the reservoirs. Box-plots display the spring (A, B) and fall (C, D) values according to the food items they ingest (A, C) and their feeding behavior (B, D). Each box-plot shows the 25th and 75th percentiles around the median; the vertical line represents 1.5 times the interquartile range, and dots are outliers.
Table 2 Analysis of variance (aligned rank transformed data) of the food items and feeding behavior of benthic invertebrates showing the effect of “site” (upstream or downstream of the dams), “season” (spring or fall) and their interaction.
The isotopic values of the samples collected along the Sélune River ranged from –21.3‰ to –32.6‰ and from 0.8‰ to 16.9‰ for δ13C and δ15N, respectively (Figure 5A). Invertebrate herbivores (grazers and scrapers pooled) were consistently 13C-depleted compared to detritivores (shredders), while omnivores and crayfish displayed intermediate values. As expected, invertivorous fish were 15N-enriched compared with invertebrates. The isotopic niche breadths (ellipses including 75% of the variance) upstream of the dams (sites S1 to S5 pooled) and downstream of the dams (sites S7 to S9 pooled) mostly overlapped for invertebrates but not for fish and lamprey (Figure 5A).
Figure 5 δ13C and δ15N values of samples collected in the Sélune River (A). Isotopic niche breadths (SEAc) of each invertebrate consumer group (iherb, herbivores; idetr, detritivores; iomn, omnivores), crayfish (cray), lamprey (lamp) and fish are depicted for sites located upstream (up, solid ellipses) and downstream (dw, dashed ellipses) of the dams. Simulated values of the trophic position and carbon-origin discrimination coefficient (α) obtained from end-members Bayesian mixing models for consumers upstream and downstream of the dams (B). The dual contributions of algal vs. detrital C origin to aquatic fauna is displayed along the Sélune River profile (C), each box indicating 50%, 75% and 95% Bayesian credibility intervals around the median (dot); the dashed line shows the position of the reservoirs.
Using invertebrate herbivores and detritivores as end-members for Bayesian mixing models, invertebrate omnivores showed trophic positions of 2.5, and C of algal and detrital origin equally contributed to their tissues (Figure 5B). Crayfish followed a similar pattern, with a slightly higher trophic position (2.7). Simulations confirmed that fish had the highest trophic position (>3), and revealed that fish and juvenile lamprey shifted toward a higher algal-C contribution downstream of the dams. Posterior distributions of coefficient α at each site showed that detrital C dominated in aquatic fauna only at the most upstream site S1, whereas algal C prevailed downstream of the dams at S7 and S8, and equal contributions of detrital and algal C were observed at S2, S3, S4, S5 and S9 (Figure 5C).
In the study, we explored the mechanisms whereby two sequential large dams and their reservoirs can control the balance between detritus-based and algae-based energy pathways in aquatic food webs along a river continuum. Investigations were made on the Sélune River (Normandy, France), a small-coastal stream draining an agricultural catchment with high sediment runoff and discharging into the Mont Saint-Michel Bay (France). The river has been impounded by two dams (16 and 36 m high) in its lower part for a century. As anticipated, the two reservoirs significantly retained sediments, phosphates and silicates for which concentrations were much lower downstream of the reservoirs. There were no large tributaries flowing into the reservoirs, nor was there a specific land use pattern in the vicinity of the reservoirs, that could have influenced water discharge and dissolved nutrient concentrations. Measures of the photosynthetic activity in epilithic biofilm showed no clear differences, but their biomass growth tended to be higher downstream of the reservoirs in spring and fall, and so was the abundance of herbivores in the benthic invertebrate community. A greater contribution of algae-based energy to aquatic invertebrates and fish downstream of the reservoirs was corroborated by stable isotopes analysis, clearly suggesting that the dams caused a major discontinuity in the origin of C entering and flowing in aquatic food webs along the river continuum.
Identifying the relative importance of different energy sources is a basic prerequisite for understanding river food web dynamics and ecosystem restoration. Aquatic primary consumers rely on two major basal sources defined by the origin of their C component – aquatic (autochthonous) or terrestrial (allochthonous) primary producers. The contribution of these two C sources to higher trophic levels, notably fish, depends on the position along the upstream–downstream gradient (Vannote et al., 1980; Finlay, 2001; Hoeinghaus et al., 2007). Several studies have illustrated significant contributions of terrestrial C to small headwater streams and large rivers, where terrestrial organic matter inputs from riparian trees (headwater streams) and floodplains (large rivers) supply the aquatic food webs (Reid et al., 2008; Wang et al., 2014; Soto et al., 2019; Roussel et al., 2021; Wang et al., 2021). Seasonal variations in discharge, local stream geomorphology and riparian communities also drive the food web reliance on C sources (Ou and Winemiller, 2016; Venarsky et al., 2020), outlining the complex balance of allochthonous and autochthonous C supply to the biomass of primary consumers and transfer to higher trophic levels. In this context, artificial barriers to river flow and related impoundments have major effects on the downstream ecosystem metabolism. Chowanski et al. (2020) observed that the gross primary production in a large, temperate, oligotrophic river increased downstream of a large reservoir, and postulated that sediment retention and lower water turbidity downriver increased light availability to aquatic producers. Such a pattern seems to be commonplace in literature on dams. In Mediterranean rivers, artificially stable flow regimes below dams promoted a shift from detritus-based to algae-based food webs (Mor et al., 2018). In large tropical rivers as in the Mekong basin, a seasonal combination of algae and terrestrial energy sources supports fish production, but the contribution of algal C appears greater below dams (Ou and Winemiller, 2016). Similarly, algae contribute more to the diet of macroinvertebrates in the Colorado River below Glen Canyon dam, while terrestrial-based food increases further downstream with increasing water turbidity and organic matter inputs from tributaries (Wellard Kelly et al., 2013). Various case studies in both temperate and tropical ecosystems highlight a shift toward greater assimilation of algal C by aquatic consumers downstream of reservoirs in large rivers. However, little attention has been given to the pattern of C flow in small stream food webs below such large reservoirs.
Carbon and N stable isotope ratios of invertebrate and fish tissue samples were used to estimate the contribution of basal sources supporting aquatic food webs along the course of the Sélune River. Using long-lived (>6 months) primary consumers in mixing models to limit stochastic isotopic signatures of basal sources (Cabana and Rasmussen, 1996; Vander Zanden and Rasmussen, 1999), we found a 3-fold variation in the contribution of the algal energy pathway within a 70-km-long stretch of river. At 15 km from the source, 75% of the food web C was derived from terrestrial detritus assimilated by invertebrate shredder species, notably Limnephilidae (Halesus sp., Chaetopteryx sp., Limnephilus sp., Potamophylax sp.) and Sericostomatidae (Sericostoma sp.). Consistent with theory (Vannote et al., 1980; Junk et al., 1989; Finley 2001; Hoeinghaus et al., 2007), this result confirms the dominant role of terrestrial supplies in the upstream reaches of the Sélune River (Site S1, Figures 1, 5C). However, the contribution of algal C equalled the detrital contribution at most sites, indicating that autochthonous and allochthonous sources of energy together sustained aquatic food webs in most of the river course. This result was somehow unexpected owing to the high sediment load and water turbidity in the river supposed to hamper primary production. Our simulations may have exaggerated the contribution of algae-derived C in the river food webs because we collected our samples in shallow, fast-flowing habitats where primary production is known to be at its maximum in rivers (Finlay, 2004). However, many studies have shown that agriculture in industrialized countries is a major source of nutrients in rivers and coastal waters, involving a number of effects including the increase in biomass of primary producers (e.g. Paerl et al., 2011; Grizzetti et al., 2012; Dupas et al., 2015; Boardman et al., 2019). Accordingly, the high concentration in nutrients is a favorable background to aquatic primary producers in the Sélune River, and this likely promotes the influence of the algae-based pathway in aquatic food webs despite high water turbidity and sediment loads.
The annual flux of sediment estimated in the Sélune River upstream of the reservoirs (19.2 t.km−2.yr−1) is consistent with the observed pattern of European Atlantic rivers with a similar catchment size (Vanmaercke et al., 2011). Despite greater uncertainty about the sediment load estimates downstream of the dam where suspended sediment quantities were sometimes very low and more difficult to measure accurately, the annual flux of sediment dropped (11.6 t.km−2·yr−1) downstream of the reservoirs. This corresponds to an amount of 12,077 t·yr−1 and 9,013 t·yr−1 upstream and downstream of the dams, respectively, meaning that the reservoirs trapped and stored about 3·103 tons of sediment from September 2015 to August 2016. With 758 mm rainfall, this water year was consistent with the inter-annual variability observed on the river. One immediate consequence was a drastic drop in water turbidity downstream of the reservoirs irrespective of season (Figure 2B) that set off a chain reaction in the entire river food web, from epilithic biofilm to invertivorous fish. The percentage of inorganic sediment in the epilithic biofilm samples was lower downstream of the reservoirs. Seasonal biofilm dynamics were substantial everywhere, with higher biomass and productivity in spring and summer. Biofilm growth on a virgin glass support measured as the increase in chlorophyll a per day was slightly, but not significantly, higher downstream than upstream of the reservoirs in spring and fall. Moreover, the trophic nature of the biofilm estimated using the autotrophic index (Weber, 1973) remained similar to the autotrophic threshold downstream irrespective of season, whereas heterotrophy prevailed upstream of the reservoirs in fall. This result was corroborated by measurements of the C:N molar ratio of suspended sediment. The ratio of organic matter exported from soils was close to the expected values (Kendall et al., 2001) upstream of the reservoirs, but it strongly decreased downstream in spring and summer when solar radiation, water turbidity and water temperature were most favorable to primary producers. It indicates that the reservoirs produced a fraction of organic matter – algae-derived C drifting downstream – as also supported by continuous monitoring of the chlorophyll a concentration in the water column downstream of the reservoirs (Fovet et al., 2020), hence the presence of pelagic forms (notably diatoms) in the epilithic biofilm matrix downriver. Therefore, the composition of the epilithic biofilm differed remarkably downstream of the reservoirs as a response to lower water turbidity, siltation, and the input of pelagic microalgae drifting from the reservoirs.
In the Sélune River, the higher abundance of herbivores (algae eaters, scrapers) within invertebrate communities downstream of the reservoirs suggests an enhancement of the algal pathway. The shift from detritivores to herbivores in invertebrate communities has been reported in river food webs downstream of large reservoirs (Mor et al., 2018). Stable isotope analysis of aquatic consumers, invertebrates and fish, gives support to this assumption. We estimated that an average 75% of the C flowing into the river food web 1 km downstream of the reservoirs was derived from aquatic primary producers, i.e. + 25% compared to the river food web just upstream of the reservoirs. This effect was still perceived 4 km downstream of the reservoirs (60% of algae-derived C in the food web) and stopped further downstream where equal contributions of autochthonous and allochthonous C were found. Phytoplankton that drifted from the reservoirs and deposited on the epilithic biofilm likely contributed to higher algal C contribution in the downstream food web, as reported in other studies (Angradi, 1993; Hoeinghaus et al., 2007; Doi et al., 2008; Ru et al., 2020). According to methodological recommendations (Cabana and Rasmussen, 1996; Vander Zanden and Rasmussen, 1999), invertebrate primary consumers (herbivores and shredders) were used in our mixing models, and it was not possible to estimate the contribution of a specific basal food sources (i.e. phytoplankton vs. phytobenthos). However, nine kilometers downstream of La-Roche-Qui-Boit reservoir (Site S9, Figure 1), equal contributions of algal C and detrital C to aquatic consumers were reported, meaning that the effect of the dams on C flow in the aquatic food webs had disappeared. This suggests that terrestrial-based food sources increased in the lower reaches of the Sélune River, outlining the possible influence of organic matter inputs from downstream tributaries, notably the Beuvron River (Figure 1).
In our analysis, values of nutrient concentrations, benthic algal and invertebrate communities and stable isotope ratios on aquatic organisms were considered jointly, revealing subtle interactions between C and nutrient biogeochemical cycles in the Sélune River. It also outlines mechanisms whereby large dams and reservoirs can affect these cycles and food web functioning in a small agricultural stream. Primary producers rely upon dissolved inorganic C (DIC) that originates from the terrestrial ecosystem (e.g. HCO3 and dissolved CO2 from chemical weathering and soils), respiration of the aquatic ecosystem, and diffusion of atmospheric CO2 into water (Mook & Tan, 1991; Finlay, 2003; Liu et al., 2011). During photosynthesis, algae preferentially assimilate 12C (Hecky and Hesslein, 1995), but when productivity is high and DIC concentration is depleted, less discrimination against 13C can lead to higher δ13C values in primary producers. Moreover, a lower DIC concentration can promote the diffusion of atmospheric CO2 into water. Since the δ13C value of atmospheric CO2 is high (about −8‰, Keeling et al., 2010), primary producers are expected to be 13C-enriched in more productive rivers (Roussel et al., 2014). In the Sélune River, the contribution of algal C to aquatic food webs was higher downstream of the reservoirs, but we did not find higher δ13C values in herbivorous invertebrates (Figure 5A). Fluorescence measurements on the epilithic biofilm did not reveal higher photosynthetic activity downstream of the reservoirs, and it is likely that the observed higher algal-C contribution to the aquatic food web downstream of the reservoirs was a consequence of a lower detrital-C contribution rather than higher primary production. Indeed, both PO4 measurements and the N:P molar ratios in water samples showed that phosphorus availability decreased downstream of the reservoirs. Phosphorus is essential to freshwater primary producers, but the blooming and sinking of phytoplankton in reservoirs can massively reduce its availability to downstream food webs during specific periods of the year (Chen et al., 2014; Lu et al., 2016; Bao et al., 2018), notably in large reservoirs where water residence time is high (Ma et al., 2018). Therefore, we argue that low phosphorus availability restricted primary production in reaches downstream of the reservoirs in the Sélune River, despite less water turbidity and better light availability. Taken together, those results illustrate how large reservoirs on a small, agricultural stream with high nutrient concentrations and sediment loads could affect the balance between the algae-based and the detritus-based energy pathways, and modulate C flow in the riverine food webs from primary producers to predators.
Finally, our study outlines some of the drivers of the reliance on different C sources in the food web of a small, coastal stream with high sediment loads. We notably highlighted mechanisms whereby large dams and reservoirs can induce a major discontinuity in the natural process of C flow in aquatic food webs and along the river continuum. By changing the downstream transfer of suspended particulate matter and dissolved nutrients, the two dams on the Sélune River artificially maintained environmental conditions that promoted the contribution of the algae-based pathway the riverine food webs. This conclusion comes from data and samples collected between 2015 and 2017, before the removal of the two dams. The dismantling operations were completed by early 2023, and the downstream transfer of sediment and solutes are being restored after a century of disruption (Fovet et al., 2023). Profound impacts on the river ecosystem are expected from now, and our results will help understand how nutrients and C flow in food webs will change during the river restoration process. We anticipate that the prevalence of algal C in aquatic consumers should fade out rapidly downstream of the reservoirs. Except in the upstream reaches and headwater tributaries where detrital C may prevail, equal contributions of algal and detrital C to the aquatic food web should be observed along the Sélune River continuum. Moreover, the expected return of diadromous species, e.g. Atlantic salmon and sea lamprey (Salmo salar, Petromyzon marinus), will disseminate marine-derived nutrients throughout the watershed, as observed in the Elwha River and the Penopscot River (Duda et al., 2011; Tonra et al., 2015; Zydlewski et al., 2023). European eel (Anguilla anguilla) will also colonize the headwaters, and this species is known to have a large impact on other fishes in the Sélune River (Lizé et al., 2023). These new driving forces will require attention in order to understand how the river ecosystem and the aquatic food webs will be restored along the Sélune River over the next decade.
The raw data supporting the conclusions of this article will be made available by the authors, without undue reservation.
The animal study was approved by “Comité rennais en matière d’éthique et d’expérimentation animale”. The study was conducted in accordance with the French legislation and institutional requirements on animal care and ethics.
J-MR conceived the idea, supervised the project and wrote the manuscript. OF and AC (sediment and solutes), AP and CG (photosynthetic biofilm), HR-P and CP (macroinvertebrates) and J-MR (stable isotopes and food web) designed the project, collected the samples, co-supervised the work and wrote the corresponding text sections of early drafts. MP, CG, GF, DH contributed to data and sample collection in the field, processed the samples in the laboratory and prepared the data. OF, CG, AP, HR-P, OD and J-MR analyzed the data, prepared the illustrations, and revised the manuscript. AT and MC developed and managed the geographic information system SISélune; SF and LS managed the project. All authors contributed to the article and approved the submitted version.
The author(s) declare financial support was received for the research, authorship, and/or publication of this article. The Water Agency (Agence de l’Eau Seine Normandie) funded this project under contracts INRAE (AESN-INRA 1050492, 1074268, 1033863, 1044888, 152504, 1066353) and CNRS (AESN-CNRS 1053864-1, 1085108-2019).
The Water Agency (Agence de l’Eau Seine Normandie) is thanked for funding. We thank Florian Boucard (EDF) who provided access to the water chemistry data recorded at La-Roche-Qui-Boit dam, and to the rating curve we used to calculate water discharge from the measured water levels. Bi-Eau engineering consultants (Angers, France) performed taxonomic identification and counting of diatoms. Yannick Fauvel, Béatrice Trinkler and Laurence Carteaux (INRAE) conducted the chemical analyses at UMR SAS laboratory (N, P, Si, C concentrations); Claude Legonidec, Mamadou Ndom and Nicolas Gillet installed and managed the sensors and water sampling for sediment and solute monitoring. Two reviewers and the handling editor are sincerely acknowledged for helpful comments on the earlier version of the manuscript.
The authors declare that the research was conducted in the absence of any commercial or financial relationships that could be construed as a potential conflict of interest.
All claims expressed in this article are solely those of the authors and do not necessarily represent those of their affiliated organizations, or those of the publisher, the editors and the reviewers. Any product that may be evaluated in this article, or claim that may be made by its manufacturer, is not guaranteed or endorsed by the publisher.
The Supplementary Material for this article can be found online at: https://www.frontiersin.org/articles/10.3389/fevo.2023.1250892/full#supplementary-material
Angradi T. (1993). Stable carbon and nitrogen isotope analysis of seston in a regulated Rocky Mountain river, USA. Regulated Rivers: Res. Manage. 8, 251–270. doi: 10.1002/rrr.3450080305
Balakrishna K., Probst J. (2005). Organic carbon transport and C/N ratio variations in a large tropical river: Godavari as a case study, India. Biogeochemistry 73, 457–473. doi: 10.1007/s10533-004-0879-2
Bao L., Li X., Cheng P. (2018). Phosphorus retention along a typical urban landscape river with a series of rubber dams. J. Environ. Manage. 228, 55–64. doi: 10.1016/j.jenvman.2018.09.019
Belletti B., Garcia de Leaniz C., Jones J., Bizzi S., Börger L., Segura G., et al. (2020). More than one million barriers fragment Europe’s rivers. Nature 588, 436–441. doi: 10.1038/s41586-020-3005-2
Biggs B. J. (1988). Artificial substrate exposure times for periphyton biomass estimates in rivers. New Z. J. Mar. Freshw. Res. 22, 507–515. doi: 10.1080/00288330.1988.9516321
Boardman E., Danesh-Yazdi M., Foufoula-Georgiou E., Dolph C. L., Finlay J. C. (2019). Fertilizer, landscape features and climate regulate phosphorus retention and river export in diverse Midwestern watersheds. Biogeochemistry 146, 293–309. doi: 10.1007/s10533-019-00623-z
Bond A. L., Diamond A. W. (2011). Recent Bayesian stable-isotope mixing models are highly sensitive to variation in discrimination factors. Ecol. Appl. 21, 1017–1023. doi: 10.1890/09-2409.1
Bourrelly P. (1990). Les algues d'Eau douce, tome I: les algues vertes (Paris, France: N. Boubee et Cie).
Brenner M., Whitmore T. J., Curtis J. H., Hodell D. A., Schelske C. L. (1999). Stable isotope (d13C and d15N) signatures of sedimented organic matter as indicators of historic lake trophic state. J. Paleolimnology 22, 205–221. doi: 10.1023/A:1008078222806
Brett M. T., Bunn S. E., Chandra S., Gallowa A. W. E., Guo F., Kainz M. J., et al. (2017). How important are terrestrial organic carbon inputs for secondary production in freshwater ecosystems? Freshw. Biol. 62, 833–853. doi: 10.1111/fwb.12909
Bumpers P. M., Rosemond A. D., Maerz J. C., Benstead J. P. (2017). Experimental nutrient enrichment of forest streams increases energy flow to predators along greener foodweb pathways. Freshw. Biol. 62, 1794–1805. doi: 10.1111/fwb.12992
Bunn S. E., Leigh C., Jardine T. D. (2013). Diet-tissue fractionation of δ15N by consumers from streams and rivers. Limnol. Oceanogr. 58, 765–773. doi: 10.4319/lo.2013.58.3.0765
Cabana G., Rasmussen J. B. (1996). Comparison of aquatic food chains using nitrogen isotopes. Proc. Natl. Acad. Sci. United States America 93, 10844–10847. doi: 10.1073/pnas.93.20.10844
Camargo J. A. (1992). Structural and trophic alterations in macrobenthic communities downstream from a fish farm outlet. Hydrobiologia 242 pp, 41–49. doi: 10.1007/BF00017642
Chen N. W., Wu Y. Q., Wu J. Z., Yan X. L., Hong H. S. (2014). Natural and human influences on dissolved silica export from watershed to coast in southeast China. J. Geophys. Research-Biogeosci. 119 (1), 95–109. doi: 10.1002/2013jg002429
Chowanski K., Kunza L., Hoffman G., Genzoli L., Stickney E. (2020). River management alters ecosystem metabolism in a large oligotrophic river. Freshw. Sci. 39 (3), 534–548. doi: 10.1086/710082
de Carvalho D. R., Alves C. B. M., Moreira M. Z., Pompeu P. S. (2020). Trophic diversity and carbon sources supporting fish communities along a pollution gradient in a tropical river. Sci. Total Environ. 738, 139878. doi: 10.1016/j.scitotenv.2020.139878
Dethier E. N., Renshaw C. E., Magilligan F. J. (2022). Rapid changes to global river suspended sediment flux by humans. Science 376, 1447–1452. doi: 10.1126/science.abn7980
Diaz R. J., Rosenberg R. (2008). Spreading dead zones and consequences for marine ecosystems. Science 321, 926–929. doi: 10.1126/science.1156401
Doi H., Chang K. H., Ando T., Imai H., Nakano S., Kajimoto A., et al. (2008). Drifting plankton from a reservoir subsidize downstream food webs and alter community structure. Oecologia 156, 363–371. doi: 10.1007/s00442-008-0988-z
Duda J. J., Coe H. J., Morley S. A., Kloehn K. K. (2011). Establishing spatial trends in water chemistry and stable isotopes (δ15N and δ13C) in the Elwha River prior to dam removal and salmon recolonization. River Res. Applic. 27, 1169–1181. doi: 10.1002/rra.1413
Dupas R., Delmas M., Dorioz J.-M., Garnier J., Moatar F., Gascuel-Odoux C. (2015). Assessing the impact of agricultural pressures on N and P loads and eutrophication risk. Ecol. Indic. 48, 396–407. doi: 10.1016/j.ecolind.2014.08.007
Eilers P., Peeters J. (1988). A model for the relationship between light intensity and the rate of photosynthesis in phytoplankton. Ecol. Model. 42, 199–215. doi: 10.1016/0304-3800(88)90057-9
Elser J. J., Bracken M. E. S., Cleland E. E., Gruner D. S., Harpole W. S., Hillebrand H., et al. (2007). Global analysis of nitrogen and phosphorus limitation of primary producers in freshwater, marine and terrestrial ecosystems. Ecol. Lett. 10, 1135–1142. doi: 10.1111/j.1461-0248.2007.01113.x
EN 10304-1:1995. Water quality — Determination of dissolved anions by liquid chromatography of ions — Part 1: Determination of bromide, chloride, fluoride, nitrate, nitrite, phosphate and sulfate.
EN 6878:2004. Water quality — Determination of phosphorus — Ammonium molybdate spectrometric method.
EN 872:2005 F. Water Quality- Determination of suspended solids – Method by filtration through glass fiber filters.
Finlay J. C. (2001). Stable-carbon-isotope ratios of river biota: implications for energy flow in lotic food webs. Ecology 82, 1052–1064. doi: 10.2307/2679902
Finlay J. C. (2003). Controls of streamwater dissolved inorganic carbon dynamics in a forested watershed. Biogeochemistry 62, 231–252. doi: 10.1023/A:1021183023963
Finlay J. C. (2004). Patterns and controls of lotic algal stable carbon isotope ratios. Limnology Oceanography 49 (3), 850–861. doi: 10.4319/lo.2004.49.3.0850
Fovet O., Meric F., Crave A., Cador J. M., Rollet A. J. (2023). Early assessment of effects of dam removal on abiotic fluxes of the Sélune River, France. Front. Ecol. Evol. 11. doi: 10.3389/fenvs.2023.1231721
Fovet O., Ndo M., Crave A., Pannard A. (2020). Influence of dams on river water-quality signatures at event and seasonal scales: The Sélune River (France) case study. River Res. Appl. 36, 1267–1278. doi: 10.1002/rra.3618
Galloway J. N., Dentener F. J., Capone D. G., Boyer E. W., Howarth R. W., Seitzinger S. P., et al. (2004). Nitrogen cycles: past, present, and future. Biogeochemistry 70, 153–226. doi: 10.1007/s10533-004-0370-0
Germain H. (1981). Flore des diatomées: Diatomophycées: eaux douces et saumâtres du Massif Armoricain et des contrées voisines d’Europe occidentale. Société Nouvelle Des Editions Boubée. ISBN 10: 2850040231 / ISBN 13: 9782850040238. p. 444.
Grill G., Lehner B., Thieme M., Geenen B., Tickner D., Antonelli F., et al. (2019). Mapping the world’s free-flowing rivers. Nature 569 (0), 215–221. doi: 10.1038/s41586-019-1111-9
Grizzetti B., Bouraoui F., Aloe A. (2012). Changes of nitrogen and phosphorus loads to European seas. Glob Change Biol. 18, 769–782. doi: 10.1111/j.1365-2486.2011.02576.x
Guilpart A., Roussel J. M., Aubin J., Caquet T., Marle M., Le Bris H. (2012). The use of benthic invertebrate community and water quality analyses to assess ecological consequences of fish farm effluents in rivers. Ecol. Indic. 23, 356–365. doi: 10.1016/j.ecolind.2012.04.019
Guo F., Kainz M. J., Sheldon F., Bunn S. E. (2016a). The importance of high-quality algal food sources in stream food webs – current status and future perspectives. Freshw. Biol. 61, 815–831. doi: 10.1111/fwb.12755
Guo F., Kainz M. J., Valdez D., Sheldon F., Bunn S. E. (2016b). High-quality algae attached to leaf litter boost invertebrate shredder growth. Freshw. Sci. 35 (4), 1213–1221. doi: 10.1086/688667
Gupta H., Reddy S. K. K., Chiluka M., Gandla V. (2021). Nutrient retention behind a tropical mega-dam: a case study of the Sardar Sarovar Dam, India. SN Appl. Sci. 3, 16. doi: 10.1007/s42452-020-04118-7
Hecky R. E., Hesslein H. (1995). Contributions of benthic algae to lake food webs as revealed by stable isotope analysis. J. North Am. Benthological Soc. 14, 631–653. doi: 10.2307/1467546
Hedges J. I., Keil R. G., Benner R. (1997). What happens to terrestrial organic matter in the ocean? Organic Geochemistry 27 (5–6), 195–212. doi: 10.1016/S0146-6380(97)00066-1
Hoeinghaus D. J., Winemiller K. O., Agostinho A. A. (2007). Landscape-scale hydrologic characteristics differentiate patterns of carbon flow in large-river food webs. Ecosystems 10, 1019–1033. doi: 10.1007/s10021-007-9075-2
Jackson A. L., Inger R., Parnell A. C., Bearhop S. (2011). Comparing isotopic niche widths among and within communities: SIBER–Stable Isotope Bayesian Ellipses in R. J. Anim. Ecol. 80 (3), 595–602. doi: 10.1111/j.1365-2656.2011.01806.x
Jakob T., Schreiber U., Kirchesch V., Langner U., Wilhelm C. (2005). Estimation of chlorophyll content and daily primary production of the major algal groups by means of multiwavelength-excitation PAM chlorophyll fluorometry: performance and methodological limits. Photosynthesis Res. 83, 343–361. doi: 10.1007/s11120-005-1329-2
John D. M., Whitton B. A., Brook A. J. (2002). The freshwater algal flora of the British Isles: An identification guide to freshwater and terrestrial algae (Cambridge, UK: Cambridge University Press).
Junk W. J., Bayley P. B., Sparks R. E. (1989). “The flood pulse concept in river-floodplain systems,” in Proceedings of the international large rivers symposium, vol. 106. Ed. Dodge D. P. (Ottawa: Canadian Special Publication in Fisheries and Aquatic Sciences), 110–127.
Kay M., Elkin L., Higgins J., Wobbrock J. (2021). ARTool: aligned rank transform for nonparametric factorial ANOVAs. doi: 10.5281/zenodo.594511
Keeling R. F., Piper S. C., Bollenbacher A. F., Walker S. J. (2010). “Monthly atmospheric 13C/12C isotopic ratios for 11 SIO stations,” in Trends: A compendium of data on global change (Oak Ridge, USA: Carbon Dioxide Information Analysis Center, Oak Ridge National Laboratory, U.S. Department of Energy).
Kendall C., Silva S. R., Kelly V. J. (2001). Carbon and nitrogen isotopic compositions of particulate organic matter in four large river systems across the United States. Hydrol. Process. 15, 1301–1346. doi: 10.1002/hyp.216
Kromkamp J. C., Forster R. M. (2003). The use of variable fluorescence measurements in aquatic ecosystems: differences between multiple and single turnover measuring protocols and suggested terminology. Eur. J. Phycol. 38, 103–112. doi: 10.1080/0967026031000094094
Lau D. C., Leung K. M., Dudgeon D. (2009). Are autochthonous foods more important than allochthonous resources to benthic consumers in tropical headwater streams? J. North Am. Benthological Soc. 28, 426–439. doi: 10.1899/07-079.1
Liu Z., Dreybrodt W., Liu H. (2011). Atmospheric CO2 sink: silicate weathering or carbonate weathering? Appl. Geochemistry 26, S292–S294. doi: 10.1016/j.apgeochem.2011.03.085
Lizé A., Teichert N., Roussel J. M., Acou A., Feunteun E., Carpentier A. (2023). Isotopic niches of diadromous fishes inform on interspecific competition in an obstructed catchment. Frontier Ecol. Evol. 11. doi: 10.3389/fevo.2023.1242452
Lorenzen C. J. (1967). Determination of chlorophyll and pheo-pigments: spectrophotometric equations 1. Limnology Oceanography 12, 343–346. doi: 10.4319/lo.1967.12.2.0343
Lu T., Chen N., Duan S., Chen Z., Huang B. (2016). Hydrological controls on cascade reservoirs regulating phosphorus retention and downriver fluxes. Environ. Sci. Pollut. Res. 23, 24166–24177. doi: 10.1007/s11356-016-7397-3
Ma N., Li Z., Xia S., Zhu Z., Song Z. (2018). Retention effects of river damming on dissolved silicon. Inland Waters 8 (2), 207–215. doi: 10.1080/20442041.2018.1457852
Maavara T., Chen Q., Van Meter K., Brown L. E., Zhang J., Ni J., et al. (2020). River dam impacts on biogeochemical cycling. Nat. Rev. Earth Environ. 1, 103–116. doi: 10.1038/s43017-019-0019-0
Marks J. C. (2019). Revisiting the fates of dead leaves that fall into streams. Annu. Rev. Ecol. Evol. Systematics 50 (1), 547–568. doi: 10.1146/annurev-ecolsys-110218-024755
Mayer M. S., Likens G. E. (1987). The importance of algae in a shaded headwater stream as food for an abundant caddisfly (Trichoptera). J. North Am. Benthological Soc. 6, 262–269. doi: 10.2307/1467313
Mook W. G., Tan F. C. (1991). “Stable carbon isotopes in rivers and estuaries,” in Biogeochemistry of major world rivers SCOPE 42 scientific committee on problems of the environment. Eds. Degens E. T., Kempe S., Richey J. E. (Chichester, UK: Wiley), 245–262.
Mor J.-R., Ruhí A., Tornés E., Valcárcel H., Muñoz I., Sabater S. (2018). Dam regulation and riverine food-web structure in a Mediterranean river. Sci. Total Environ. 625, 301–310. doi: 10.1016/j.scitotenv.2017.12.296
Morin S., Duong T. T., Dabrin A., Coynel A., Herlory O., Baudrimont M., et al. (2008). Long-term survey of heavy-metal pollution, biofilm contamination and diatom community structure in the Riou Mort watershed, South-West France. Environ. pollut. 151, 532–542. doi: 10.1016/j.envpol.2007.04.023
NF T 90-007:2001. Water quality - Determination of soluble silicates - Molecular absorption spectrometric method.
Oksanen J., Blanchet F. G., Friendly M., Kindt R., Legendre P., McGlinn D., et al. (2020) Vegan: community ecology package. Version 2.5-7. Available at: https://CRAN.R-project.org/package=vegan.
Orihel D. M., Baulch H. M., Casson N. J., North R. L., Parsons C. T., Seckar D. C. M., et al. (2017). Internal phosphorus loading in Canadian fresh waters: a critical review and data analysis. Can. J. Fisheries Aquat. Sci. 74 (12), 2005–2029. doi: 10.1139/cjfas-2016-0500
Ou C., Winemiller K. O. (2016). Seasonal hydrology shifts production sources supporting fishes in rivers of the Lower Mekong Basin. Can. J. Fisheries Aquat. Sci. 73 (9), 1342–1362. doi: 10.1139/cjfas-2015-0214
Paerl H. W., Hall N. S., Calandrino E. S. (2011). Controlling harmful cyanobacterial blooms in a world experiencing anthropogenic and climatic-induced change. Sci. Total Environ. 409, 1739–1745. doi: 10.1016/j.scitotenv.2011.02.001
Penuelas J., Poulter B., Sardans J., Ciais P., van der Velde M., Bopp L., et al. (2013). Human-induced nitrogen–phosphorus imbalances alter natural and managed ecosystems across the globe. Nat. Commun. 4, 2934. doi: 10.1038/ncomms3934
Portielje R., Lijklema L. (1995). Carbon dioxide fluxes across the air-water interface and its impact on carbon availability in aquatic systems. Limnology Oceanography 40, 690–699. doi: 10.4319/lo.1995.40.4.0690
Post D. (2002). Using stable isotopes to estimate trophic position: models, methods, and assumptions RID A-6987-2009. Ecology 83, 703–718. doi: 10.1890/0012-9658(2002)083[0703:USITET]2.0.CO;2
Post D. M., Layman C. A., Arrington D. A., Takimot G., Quattrochi J., Montana C. G. (2007). Getting to the fat of the matter: models, methods and assumptions for dealing with lipids in stable isotope analyses. Oecologia 152, 179–189. doi: 10.1007/s00442-006-0630-x
Quezada-Romegialli C., Jackson A. L., Hayden B., Kahilainen K. K., Lopes C., Harrod C. (2018). tRophicPosition, an r package for the Bayesian estimation of trophic position from consumer stable isotope ratios. Methods Ecol. Evol. 9, 1592–1599. doi: 10.1111/2041-210X.13009
Reid D. J., Quinn G. P., Lake P. S., Reich P. (2008). Terrestrial detritus supports the food webs in lowland intermittent streams of south-eastern Australia: a stable isotope study. Freshw. Biol. 53, 2036–2050. doi: 10.1111/j.1365-2427.2008.02025.x
Rimet F., Bouchez A. (2012). Life-forms, cell-sizes and ecological guilds of diatoms in European rivers. Knowl. Managt. Aquat. Ecosyst. 406), 1. doi: 10.1051/kmae/2012018
Roach K. A. (2013). Environmental factors affecting incorporation of terrestrial material into large river food webs. Freshw. Sci. 32, 283–298. doi: 10.1899/12-063.1
Rosemond A. D., Benstead J. P., Bumpers P. M., Gulis V., Kominoski J. S., Manning D. W., et al. (2015). Experimental nutrient additions accelerate terrestrial carbon loss from stream ecosystems. Science 347 (6226), 1142–1145. doi: 10.1126/science.aaa1958
Roussel J. M., Covain R., Vigouroux R., Allard L., Treguier A., Papa Y., et al. (2021). Fish communities critically depend on forest subsidies in small neotropical streams with high biodiversity value. Biotropica 53, 1096–1108. doi: 10.1111/btp.12949
Roussel J. M., Guilpart A., Huteau D., Perdriau M., Roucaute M., Le Bris H. (2018). Feeding the river: The fate of feed-pellet-derived material escaping from land-based trout farms. Aquaculture 495, 172–178. doi: 10.1016/j.aquaculture.2018.05.050
Roussel J. M., Perrier C., Erkinaro J., Niemelä E., Cunjak R. A., Huteau D., et al. (2014). Stable isotope analyses on archived fish scales reveal long-term effect of dissolved inorganic nitrogen loads on carbon cycling in rivers. Global Change Biol. 20, 523–530. doi: 10.1111/gcb.12293
Ru H., Li Y., Sheng Q., Zhong L., Ni Z. (2020). River damming affects energy flow and food web structure: a case study from a subtropical large river. Hydrobiologia 847, 679–695. doi: 10.1007/s10750-019-04130-y
Schindler D. E., Carpenter S. R., Cole J. J. (1997). Influence of food web structure on carbon exchange between lakes and the atmosphere. Science 277, 248–250. doi: 10.1126/science.277.5323.248
Soto D. X., Decru E., Snoeks J., Verheyen E., Van de Walle L., Bamps J., et al. (2019). Terrestrial contributions to Afrotropical aquatic food webs: The Congo River case. Ecol. Evol. 9, 10746–10757. doi: 10.1002/ece3.5594
Syvitski J. P. M., Vörösmarty C. J., Kettner A. J., Green P. (2005). Impact of humans on the flux of terrestrial sediment to the global coastal ocean. Science 308, 376–380. doi: 10.1126/science.1109454
Tachet H., Richoux P., Bournaud M., Usseglio-Polatera P. (2010). Invertébrés d'eau douce. Systématique, Biologie, Écologie (Paris: CNRS Éditions), ISBN: 978-2-271-06945-0.
Thorp J. H., Delong M. D. (2002). Dominance of autochthonous autotrophic carbon in food webs of heterotrophic rivers. Oikos 96, 543–550. doi: 10.1034/j.1600-0706.2002.960315.x
Tonra C. M., Sager-Fradkin K., Morley S. A., Duda J. J., Marra P. P. (2015). The rapid return of marine-derived nutrients to a freshwater food web following dam removal Vol. 192 (Biological Conservation), 130–134. doi: 10.1016/j.biocon.2015.09.009
Tréguer P. J., de la Rocha C. L. (2013). The world ocean silica cycle. Annu. Rev. Mar. Sci. 5 (1), 477–501. doi: 10.1146/annurev-marine-121211-172346
Usseglio-Polatera P., Bournaud M., Richoux P., Tachet H. (2000). Biological and ecological traits of benthic freshwater macroinvertebrates: relationships and definition of groups with similar traits. Freshw. Biol. 43, 175–205. doi: 10.1046/j.1365-2427.2000.00535.x
Vander Zanden M. J., Rasmussen J. B. (1999). Primary consumer d13C and d15N and the trophic position of aquatic consumers. Ecology 80, 1395–1404. doi: 10.1890/0012-9658(1999)080[1395:PCCANA]2.0.CO;2
Vanmaercke M., Poesen J., Verstraeten G., de Vente J., Ocakoglu F. (2011). Sediment yield in Europe: Spatial patterns and scale dependency. Geomorphology 130 (3–4), 142–161. doi: 10.1016/j.geomorph.2011.03.010
Vannote R. L., Minshall G. W., Cummins K. W., Sedell J. R., Cushing C. E. (1980). The river continuum concept. Can. J. Fish Aquat Sci. 37, 130–137. doi: 10.1139/f80-017
Venarsky M. P., Stewart-Koster B., Hunt R. J., Jardine T. D., Bunn S. E. (2020). Spatial and temporal variation of fish community biomass and energy flow throughout a tropical river network. Freshw. Biol. 65, 1782–1792. doi: 10.1111/fwb.13581
Vongvixay A., Grimaldi C., Dupas R., Fovet O., Birgand F., Gilliet N., et al. (2018). Contrasting suspended sediment export in two small agricultural catchments: Cross-influence of hydrological behaviour and landscape degradation or stream bank management. Land Degrad Dev. 29, 1385–1396. doi: 10.1002/ldr.2940
Wang J., Gu B., Huang J., Han X., Lin G., Zheng F., et al. (2014). Terrestrial contributions to the aquatic food web in the middle yangtze river. PloS One 9 (7), e102473. doi: 10.1371/journal.pone.0102473
Wang S., Wang T.-T., Xia W.-T., Chen Z.-B., Stewart S. D., Yang F.-J., et al. (2021). Longitudinal pattern of resource utilization by aquatic consumers along a disturbed subtropical urban river: Estimating the relative contribution of resources with stable isotope analysis. Ecol. Evol. 11, 16763–16775. doi: 10.1002/ece3.8304
Weber C. (1973). “Biological monitoring of the aquatic environment,” in Biological methods for the assessment of water quality (ASTM International). doi: 10.1520/STP34716S
Wellard Kelly H. A., Rosi-Marshall E. J., Kennedy T. A., Hall R. O. Jr., Cross W. F., Baxter C. V. (2013). Macroinvertebrate diets reflect tributary inputs and turbidity-driven changes in food availability in the Colorado River downstream of Glen Canyon Dam. Freshw. Sci. 32 (2), 397–410. doi: 10.1899/12-088.1
Wobbrock J., Findlater L., Gergle D., Higgins J. (2011). “The aligned rank transform for nonparametric factorial analyses using only ANOVA procedures,” in Proceedings of the ACM Conference on Human Factors in Computing Systems (CHI '11). 143–146. doi: 10.1145/1978942.1978963
Zarfl C., Dun F. E. (2022). The delicate balance of river sediments. Science 376, 1385–1386. doi: 10.1126/science.abq6986
Keywords: nutrient concentrations, sediment fluxes, photosynthetic biofilm, benthic invertebrates, fish, stable isotope analysis, mixing models, carbon flow
Citation: Roussel J-M, Fraisse S, Dézerald O, Fovet O, Pannard A, Rodriguez-Perez H, Crave A, Gorzerino C, Poupelin M, Forget G, Huteau D, Thomas A, Chevé M, Soissons L and Piscart C (2024) Effects of large dams on the aquatic food web along a coastal stream with high sediment loads. Front. Ecol. Evol. 11:1250892. doi: 10.3389/fevo.2023.1250892
Received: 30 June 2023; Accepted: 07 December 2023;
Published: 03 January 2024.
Edited by:
Diana Hamilton, Mount Allison University, CanadaReviewed by:
Rachelle Johnson, U.S. Geological Survey, United StatesCopyright © 2024 Roussel, Fraisse, Dézerald, Fovet, Pannard, Rodriguez-Perez, Crave, Gorzerino, Poupelin, Forget, Huteau, Thomas, Chevé, Soissons and Piscart. This is an open-access article distributed under the terms of the Creative Commons Attribution License (CC BY). The use, distribution or reproduction in other forums is permitted, provided the original author(s) and the copyright owner(s) are credited and that the original publication in this journal is cited, in accordance with accepted academic practice. No use, distribution or reproduction is permitted which does not comply with these terms.
*Correspondence: Jean-Marc Roussel, amVhbi1tYXJjLnJvdXNzZWxAaW5yYWUuZnI=
‡ORCID: Roussel JM, 0000-0002-6301-3234
Dézerald O, 0000-0002-9987-9865
Pannard A, 0000-0003-2997-5744
Soissons L, 0000-0002-0922-3206
Piscart C, 0000-0002-4054-4542
Disclaimer: All claims expressed in this article are solely those of the authors and do not necessarily represent those of their affiliated organizations, or those of the publisher, the editors and the reviewers. Any product that may be evaluated in this article or claim that may be made by its manufacturer is not guaranteed or endorsed by the publisher.
Research integrity at Frontiers
Learn more about the work of our research integrity team to safeguard the quality of each article we publish.