- 1U.S. Geological Survey, Western Fisheries Research Center, Seattle, WA, United States
- 2San Francisco Estuary Institute, Richmond, CA, United States
- 3Washington Sea Grant, University of Washington, Port Angeles, WA, United States
- 4U.S. Geological Survey, Pacific Coastal and Marine Science Center, Santa Cruz, CA, United States
- 5Washington Department of Natural Resources, Olympia, WA, United States
- 6Lower Elwha Klallam Tribe, Port Angeles, WA, United States
Dam removal is used increasingly to restore aquatic ecosystems and remove unnecessary or high-risk infrastructure. As the number of removals increases, there is a growing understanding about the hydrologic, geomorphic, and ecological responses to these removals. Most dam removal studies, however, focus on river and watershed responses to dam removal. The removal of two dams on the Elwha River provided a unique opportunity to characterize the response of nearshore (coastal) ecosystems. We conducted SCUBA surveys between 2011 and 2022 to quantify trajectories of change in a nearshore ecosystem during and after dam removal. We focused on the degree to which the abundances of kelp, benthic invertebrates, and fish changed in response to patterns of sediment fluxes during and after dam removal. Our findings point to two pathways of response depending on the disturbance mechanism and species type. Sites with persistent sediment deposition were characterized by wholesale community changes that did not recover to a before dam removal condition. Instead, the sites were colonized by new species that were largely absent prior to dam removal. Sites that experienced high turbidity but lacked persistent seafloor deposition were primarily characterized by a reduction in the abundance of kelp and other algae during dam removal and a rapid recovery after sediment flux to the nearshore declined. Dam removal influences on invertebrates and fish at these sites were more variable, benefiting some species and disadvantaging others. In addition to dam removal, sea star wasting syndrome and a marine heatwave exerted distinct controls on subtidal communities during the same period. The loss of the predatory sea star Pycnopodia helianthoides was associated with gains in some of its prey species, and kelp community changes reflected regional trends in ocean temperature and kelp abundance. The results presented here have important implications for understanding the response of marine ecosystems to future dam removals and similar sediment perturbation events.
1 Introduction
In marine ecosystems, sediment plays an important role in determining community composition in time and space (Hall, 1993; Airoldi, 1998; Lohrer et al., 2004; Erftemeijer et al., 2012). Large disturbance events that alter sediment dynamics in marine systems—such as storms and debris flows—can result in high turbidity, bed scour, and burial of benthic communities. Studies from disturbance events in a wide range of ecosystem types tend to point to two pathways of response for both the physical and ecological aspects of the system. The first is the stable state response, whereby the system is “resilient” and returns to the pre-disturbance state over time (Holling, 1973; Gunderson, 2000; Fuller et al., 2019). The second is the alternative state response, wherein the system resets to a state different from the pre-disturbance condition (Beisner et al., 2003; Knowlton, 2004; Schröder et al., 2005; Phillips and Van Dyke, 2016). Disturbance events such as these are natural laboratories that can provide insights about effects, recovery, and resilience, including the magnitude of impact, types of species affected, and the duration and trajectory of the recovery (Paine and Levin, 1981; Sousa, 1984; Palumbi et al., 2008).
Dam removal is a relatively new sediment disturbance in aquatic and marine ecosystems as dam removal activities in the United States and elsewhere have increased, particularly in the last three decades (O’Connor et al., 2015). As such, there is a growing body of literature that describes the physical, hydraulic, geomorphic, and ecological changes that occur following dam removals of all sizes in a range of watershed types (Foley et al., 2017b). For small dam removals, the effect on freshwater benthic communities is often undetectable or short lived (Doyle et al., 2005; Tullos et al., 2014), while larger dam removals can result in benthic effects that can be detected multiple years after dam removal (Morley et al., 2020). Because many of the dam removals to date have been small and/or far upstream, there are few studies describing the ecological response in marine ecosystems following dam removal. Many of those that have been published in the last ten years (Rubin et al., 2017; Shaffer et al., 2017; Glover et al., 2019) are associated with dam removal on a single river, the Elwha, which is the subject of the study described in this paper.
In this paper we describe observations of change for kelp (brown algae in the order Laminariales), benthic invertebrates, and fish over a twelve-year period, between 2011-2022, in the nearshore ecosystem during and after the removal of two dams on the Elwha River. The analysis presented here builds on the observed changes to the nearshore system presented in Rubin et al. (2017) by following kelp, benthic invertebrate, and fish communities for an additional eight years after dam removal was complete. This paper focuses on the following dam-removal related hypotheses for kelp, benthic invertebrate, and fish communities: 1) fine suspended sediment load in the Elwha nearshore will decrease after the completion of dam removal and algal communities at unburied sites will return to conditions similar to those that existed before dam removal; 2) kelp, benthic invertebrate, and fish community composition at the buried sites will not return to those that existed before dam removal due to persistent changes in habitat characteristics; 3) decreased suspended sediment and (or) recovery of kelp communities at the unburied sites will benefit kelp-dependent benthic invertebrates that decreased during dam removal and hinder invertebrates that increased during dam removal due to increased food supply or relaxed competition for space with algae.
During the 2011-2022 period, additional regional phenomena occurred that likely affected the trajectory of ecological recovery. In 2013, sea star wasting syndrome (SSWS) decimated populations of a major predatory sea star, Pycnopodia helianthoides (hereafter referred to as Pycnopodia), coast-wide during our study period (Montecino-Latorre et al., 2016). In addition, a marine heatwave affected the eastern Pacific from 2014-2016 (Tolimieri et al., 2023). While the focus of the paper is on dam removal effects, we also tested the effects of SSWS and compared kelp recovery trajectories to regional kelp data, specifically focusing on two additional hypotheses: 4) SSWS will negatively impact sea star density and positively impact other benthic invertebrates, particularly those released from predation; and 5) kelp abundance at unburied and control sites will show similar patterns of loss during the marine heatwave compared to regional kelp surveys.
1.1 Background and study area
The Elwha River is a relatively short (72-km long), high gradient river flowing north out of the Olympic Mountains into the Strait of Juan de Fuca (Figure 1). Two dams constructed on the Elwha River, Washington State, USA (Figure 1B), in the early 1900s trapped approximately 30 million tonnes (Mt) of sediment by 2011 (Ritchie et al., 2018). The staged removal of the 32-m tall Elwha Dam (at river kilometer 8) and the 64-m tall Glines Canyon Dam (at river kilometer 21), began in September 2011 and was completed by 2014. Sediment flux through the lower river and to the coast increased dramatically in response to dam removal, starting in March 2012, during the deconstruction of the lower Elwha Dam (Ritchie et al., 2018). The reservoir upstream of the Glines Canyon Dam, Lake Mills, started spilling sediment past the dam site in October of 2012. During the first two years of dam removal, between September 2011 and September 2013, over 10 Mt of sediment were eroded from the two reservoirs. Turbidity in the river downstream of the dams increased nearly three orders of magnitude above background levels during the same period (Magirl et al., 2015). In the subsequent three-year period (September 2013 to September 2016), an additional ~9 Mt of sediment eroded from the reservoirs, thereby resulting in almost 20 Mt of sediment eroded since the beginning of the project (Ritchie et al., 2018).
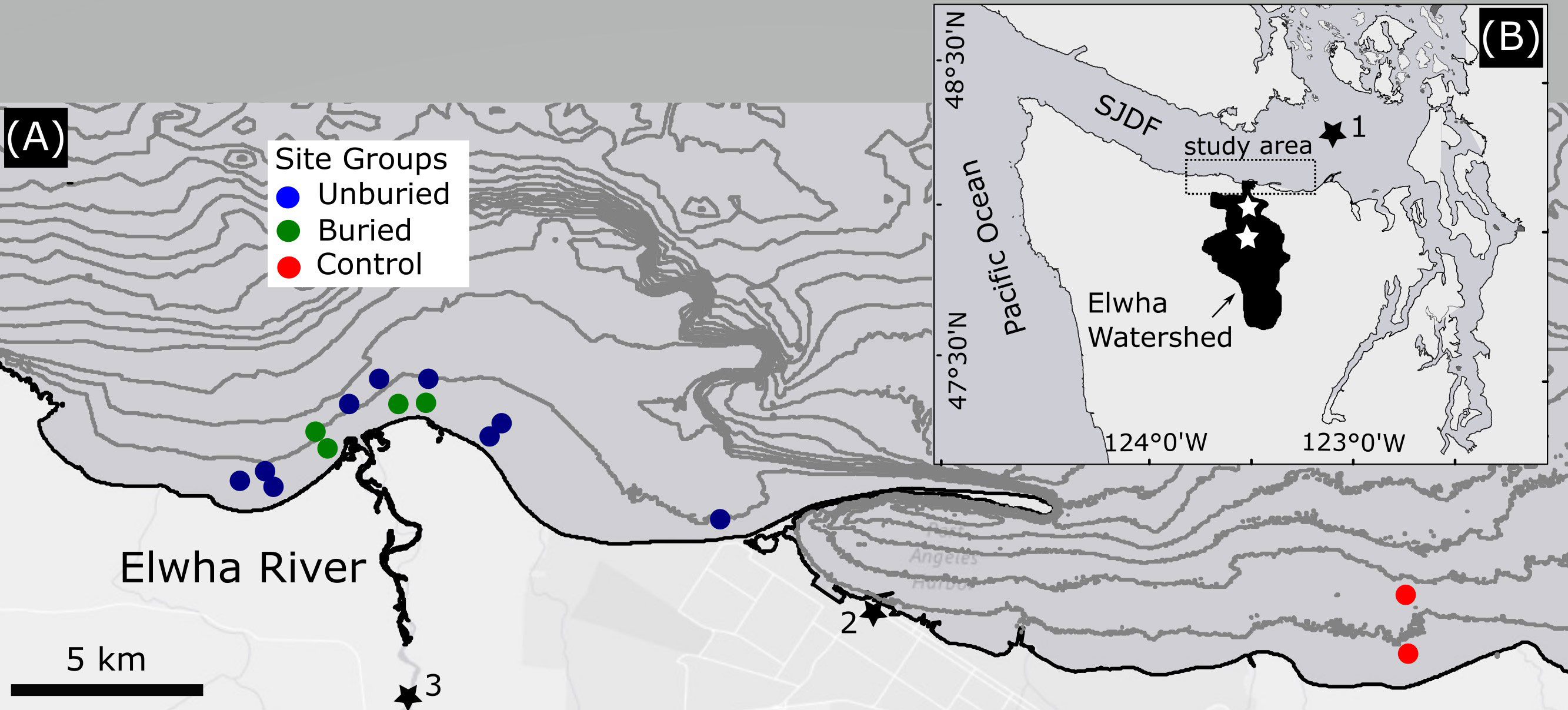
Figure 1 Study area (A) and regional context (B). The location of the two former dams on the Elwha River are shown with white stars in (B) Analysis of marine community response was based on three groups of subtidal survey sites (colored circles in A). Black stars mark the locations of environmental data discussed in the paper, including wave data from NDBC 46088 (1), water level data from NOAA 9444090 (2) and river flux and turbidity data from USGS 12046260 (3). Contour intervals in (A) are 10m and derived from pre-dam removal bathymetry.
Roughly 90% of the sediment eroded from the reservoirs during dam removal was transported rapidly through the river to the dynamic coastal environment of the Strait of Juan de Fuca. The diurnal tidal range, the difference between mean lower low and mean higher high water is 2.1 meters, and the exchange of tides through the Strait of Juan de Fuca generates large tidal currents that regularly exceed 1 m/s near the Elwha River delta (Warrick and Stevens, 2011). Wave energy in the Strait of Juan de Fuca near the Elwha River delta is a mix of locally forced wind waves and swell waves propagating from the Pacific Ocean, with a mean annual significant wave height of ~0.4 m and a bimodal distribution of dominant period, with peaks at 4 and 10 seconds (Miller et al., 2011).
The large increase in sediment supply associated with the dam removals resulted in dramatic physical changes in the nearshore, including: 1) growth of the river mouth delta landform and modification of a variety of coastal habitats (Foley et al., 2017c; Warrick et al., 2012; Gelfenbaum et al., 2015; Warrick et al., 2019), 2) increased turbidity in coastal waters near the river mouth (Foley and Warrick, 2017; Glover et al., 2019), and 3) persistent and intermittent sedimentation on the seafloor offshore of the delta (Eidam et al., 2016; Foley and Warrick, 2017; Glover et al., 2019). These dam removal-mediated habitat alterations had a variety of implications. Rubin et al. (2017) documented > 75% decrease in brown algal density and decreased benthic invertebrate species diversity at sites near the Elwha River mouth during dam removal compared to before dam removal surveys. Changes in algal abundance during dam removal were associated with the deposition of sediment and reductions in light driven by increased turbidity (Rubin et al., 2017; Glover et al., 2019).
2 Materials and methods
A suite of approaches for monitoring and tracking the influence of dam removal were developed prior to dam removal. Methods are briefly described below and in greater detail in previously published papers, including Magirl et al. (2015) for fluvial sediment fluxes, Gelfenbaum et al. (2015) for coastal morphology, and Rubin et al. (2017) for nearshore subtidal communities.
2.1 Grain size, bathymetry and sediment flux measures
Seafloor grain size and topographic and bathymetric measurements were collected annually on and around the Elwha River delta, typically over 3-4 days during the summer (Gelfenbaum et al., 2015; Stevens et al., 2017). Intertidal topographic measurements were collected with differentially corrected GNSS systems, mounted on backpacks, and walked by surveyors along the beach and the backshore during low tides. The GNSS system records positions and elevations in an auto-by-interval mode, in which observations were collected automatically as the surveyors moved over the beach and backshore. Measurements were concentrated on cross-shore oriented transects with approximately 50-m alongshore spacing, but surveyors also collected observations along slope breaks and irregular features.
Bathymetric observations were made during the same surveys using personal watercraft equipped with GNSS systems coupled with depths measured using single beam echosounders. Vessel operators collected bathymetric data at high tide along the same set of cross-shore oriented transects used by walking surveyors at low tide, and generally collect observations between mean lower low water (0.12 m NAVD88 at Elwha) and offshore depths of 15-20 m. The combined topographic and bathymetric data are interpolated onto a uniform grid with a resolution of 5 m. The resulting digital elevation model (DEM) has an estimated vertical uncertainty of 12 cm (Stevens et al., 2017).
Surface sediment samples were collected with a petite ponar grab sampler (Gelfenbaum et al., 2015). Samples were obtained along a series of cross-shore transects at sites that, prior to dam removal, were subtidal (elevations between -1 and -12 m, NAVD88). As the bathymetry of the Elwha River delta changed during and after dam removal (Ritchie et al., 2018), the sampling locations stayed the same, such that some sites sampled from a boat in 2012 were sampled by hand from the beach in subsequent years. For samples collected with the grab sampler, up to three attempts were made to collect a sample with adequate mass (approximately 50 g) from the seafloor; if no sample could be collected the site was assumed to be coarse-grained. Suitable samples were transported to a U.S. Geological Survey (USGS) laboratory in Santa Cruz, California, USA, and analyzed to determine the disaggregated grain size distributions using a combination of sieves and a Beckman Coulter LS 13 320 particle size analyzer (Stevens et al., 2017).
Sediment fluxes in the Elwha River for the period between September 2011 and September 2016 were estimated using the methods of Magirl et al. (2015) as calculated and tabulated by Ritchie et al. (2018) for the USGS gaging station 12046260 on the Elwha River (Figure 1), which captures the sediment inputs from both reservoirs upstream. Suspended sediment concentrations (SSC) were derived from a combination of standard flow-weighted suspended sediment samples, automated point-sampler daily composite samples, and three sediment-surrogate instruments—two optical turbidimeters and one acoustic Doppler velocity meter (ADVM)—to generate 15-min interval records of SSC during the 2012 to 2016 water years. These estimates include the proportions of the suspended sediment discharge within sand grain sizes (0.063 to 2 mm) and silt and clay grain sizes (less than 0.063 mm), which we termed fine suspended sediment load (FSSL). Sediment discharge was calculated by the product of SSC and measured river discharge, which were summarized into daily and annual sediment discharge values.
Turbidity measurements in the Elwha River were discontinued after the 2016 water year, so sediment fluxes after that time were estimated using a rating curve derived from measurements of 2016 water year average daily river discharge (Q, m3/s) and daily suspended-sediment discharge (Qs, t/d) using power-law formulations. Sediment rating curves were generated from least-squares regression for both sand grain-size fractions (Qs = 0.000188 Q3.3181; r2 = 0.77) and silt-to-clay grain size fractions (Qs = 0.000786 Q3.1366; r2 = 0.76) and applied to daily average discharge records (Warrick et al., 2012). Our computations assume that the sediment supply rates to the river continued to be dictated by river flow and that the sediment availability stayed relatively constant with time. These assumptions likely result in overestimates of sediment discharge because they do account for the observed decreased sediment availability over time after dam removal ended (Ritchie et al., 2018).
2.2 Subtidal SCUBA surveys
Subtidal marine community surveys were conducted annually between mid-July and early September at 13 sites near the Elwha River delta, and at two additional control sites at Green Point, approximately 20 km east in the Strait of Juan de Fuca (Figure 1). Before dam removal, each site was surveyed at least once between 2008 and 2011 (Rubin et al., 2017). Since 2012 all sites have been surveyed annually except the two control sites, which were not surveyed in 2018. Sites vary in depth between 5 and 17 m, and (excepting the control sites) are distributed up to 7.5 km east and 2.5 km west of the Elwha River mouth. Substrate type at sites is a mix of sand, gravel, cobbles, and boulders (Rubin et al., 2017) and has not changed measurably except where persistent deposition occurred.
Each site was marked on the seafloor with a stainless steel post, which identifies the center of two 50-m long transects, one trending east and one trending west, marked on either end by a cement block. Along each transect, divers enumerated benthic invertebrates greater than 2.5 cm along any dimension and kelp and acid kelp (Desmarestia sp) greater than 24 cm in length, within a 30-m long and 1-m wide swath. Divers also enumerated fish of any size within a 30-m long by 2-m wide by 2-m high tube. Additionally, a modified uniform point contact methodology was used to characterize the substrate type and algal cover at 0.5 m intervals along the transect (Rubin et al., 2017).
2.3 Data analysis
Kelp, invertebrates, and fish were analyzed separately. Species were grouped to the lowest taxonomic level practicable for consistency among observers and across years. Many fish were small, cryptic, and mobile, and are generally aggregated to taxonomic levels higher than those for kelp or invertebrates. Common rather that scientific names were used for fish. Data were averaged over transects within each site-year before analysis. All data collected between 2008 and 2011, before the start of dam removal, were consolidated and are presented here as belonging to 2011 (see Rubin et al., 2017 for full details). All data used in analyses are publicly available (Rubin et al., 2023).
Sites were categorized into three “site groups” prior to analysis: buried (4 sites), unburied (9 sites), and control (2 sites; Figure 1). Buried and unburied site groups were separated because previous analyses (Rubin et al., 2017) indicated fundamental differences in biological response between sites buried under persistent sediment deposits and unburied sites. We classified a site as buried if it experienced deposition greater than 15 cm based on analysis of bathymetry survey data or measurements of a post installed at each site that allowed divers to estimate changes in elevation unless the depth of deposition exceeded post height (Rubin et al., 2017). Sites classified as buried first became buried in 2013 or 2014 and remained buried for the duration of our study; unburied sites did not experience persistent burial.
2.4 Community analysis
Community analyses compared trajectories of change among communities at buried, unburied, and control sites. A difference in trajectory for the control sites compared to the other two site groups would suggest that change at the buried and unburied sites was due to dam removal. A trajectory difference between buried and unburied sites would suggest that communities were differentially affected by dam removal at buried compared to unburied sites. Community analyses addressed hypotheses 1, 2, and 3.
Multivariate community analyses were conducted using species that were present in at least five site-years. Analyses were performed on Bray-Curtis similarity matrices computed from square root-transformed species densities (Clarke et al., 2014). Analyses were conducted in Primer 7 (Clarke and Gorley, 2015).
Two-way analysis of similarity (ANOSIM) with factors survey year and site was used to investigate which major taxon (kelp, invertebrates, or fish)-by-site group combinations showed significant community change across years (Clarke et al., 2014). Site was included as a factor to account for (i.e., factor out) variation due to spatial differences among sites. Taxon-by-site group combinations that did not exhibit significant community change across years were not included in subsequent analyses, except for testing the effect of the density of the sea star Pycnopodia on community composition within the control and unburied site groups.
Two-way analysis of similarity percentages (SIMPER) with factors survey year and site was used to investigate which species contributed most to community change across years within each site group (Clarke et al., 2014). SIMPER generated percent contribution of species contributing most to the difference between each year pair, up to a cumulative contribution of 70%. We computed a weighted average of a species’ contribution to each year pair difference where the weights were the average community dissimilarity between each year pair. This gave a single mean percent contribution to community differences among years for each species. Species contributing most to differences over time were highlighted in subsequent analyses.
Nonmetric multidimensional scaling (NMDS) analysis was conducted on densities averaged across sites within site groups and was used to investigate when community change occurred and the trajectory of change (e.g., unidirectional with different start and end states, circular with similar start and end states). Species vectors, indicating the strength and direction of correlations between species densities and each NMDS axis were superimposed on NMDS plots (Clarke et al., 2014; Clarke and Gorley, 2015).
Species analyses (Somerfield and Clarke, 2013) were used to identify groups of species that changed similarly across years. Analyses were conducted on densities averaged over sites within site groups. Densities were standardized by total within species (density from each year was divided by total density across years). Then cluster analysis was performed on Bray-Curtis similarity matrices computed from standardized densities (no square root transformation). Species that did not contribute to community change between at least one pair of years according to SIMPER analysis were excluded. Species that did contribute to community change but only occurred in one year were also excluded.
2.5 Mechanisms driving change
Analyses of mechanisms driving change addressed whether communities or individual species were affected by variables directly or indirectly related to dam removal or other stressors. Variables considered were fine suspended sediment load (FSSL), algal cover, and Pycnopodia density. Mechanism analyses primarily addressed hypotheses 3 and 4.
We used regression approaches to test the three mechanisms, with FSSL, percent algal cover, and Pycnopodia density as independent variables. We chose FSSL in the river as the independent variable representing suspended sediment because suspended sediment in the nearshore was linked to FSSL in the river (Glover et al., 2019). We used cumulative FSSL between February 1 and July 31 because our observations of the system suggest that elevated suspended sediment during that period could suppress algal recruitment and growth in our study area.
Distance-based redundancy analysis (dbRDA) was used to investigate whether community change was related to FSSL, percent algal cover, or Pycnopodia density (McArdle and Anderson, 2001). dbRDA is akin to multivariate regression and is appropriate when the dependent variables, in our case densities of species, are represented by a Bray-Curtis similarity matrix as ours were. Site was included in dbRDAs as an independent variable (as a fixed effect because dbRDAs do not allow random effects) to account for spatial effects. dbRDAs were conducted in Permanova+ (Anderson et al., 2008).
Univariate regression analysis was used to investigate whether densities of single species were related to FSSL, algal cover, or Pycnopodia density. Species identified by SIMPER as contributing most to community change across years at Elwha unburied sites were chosen for this analysis. Species density was log-transformed before analysis to increase normality and decrease heteroskedasticity. Sites where the species did not occur in any year were excluded. Analyses were conducted in R (R Core Team, 2022). We used General Additive Models (GAM) to test which shape of a curved relationship fit best, or whether a linear relationship fit better than any curve, and also to test which of four error structures fit best: 1) including site as a random effect, 2) including an auto-correlation term (AR1) to account for any non-independence due to consecutive years of data, 3) including both site as a random effect and an auto-correlation term, and 4) including neither site nor an auto-correlation term (R package mgcv; calls gam or gamm; Wood, 2017). The model with the lowest AIC value was selected as the best fit. A final step was to test whether adding to the best fitting model a term allowing residual spread to vary as a function of the independent variable (VarExp variance structure; Zuur et al., 2009) improved model fit further (Pinheiro et al., 2023).
3 Results
3.1 Sediment flux and coastal turbidity
Between water years 2011 and 2015 the Elwha River discharged roughly 14 Mt of suspended sediment to the coastal waters, composed of ~58% silt and clay, and ~42% sand, and roughly 5 Mt of sand and gravel bedload. After dam removal ended in 2014 sediment discharge declined rapidly: A total of ~3 Mt of suspended sediment were discharged between water years 2016 and 2022, composed of ~62% silt and clay and 38% sand (Figure 2A). The seasonality of suspended discharge also changed during and after dam removal. Most of the suspended-sediment discharge occurred during winter high flow events both during and after dam removal. Suspended sediment fluxes during the lower flows of summer, though, decreased rapidly after dam removal ended as the availability of erodible sediment decreased (Figure 2B). These summer season decreases in sediment discharge were especially apparent after dam removal was completed (WY2015-2022; Figure 2B), whereas during dam removal (WY2012-2014), the suspended-sediment discharge during the summer was several orders of magnitude higher. For example, by early August (day of year 200) when many of the scuba surveys were conducted, the Elwha River was discharging 20 to 400 t/d during active dam removal (WY2012-2014) but only ~1 t/d following the completion of dam removal (WY2015-2022; Figure 2B). Thus, dam removal resulted in three distinct intervals of time with respect to river sediment and turbidity: 1) during dam removal (WY2012-2014), when suspended sediment discharge was high during both winter and summer seasons, 2) immediately following dam removal (WY2015-2016), when annual suspended-sediment discharge was high, but with lower turbidity and sediment discharge during the summer, and 3) several years following dam removal (WY2017-WY2022) when river sediment loads were relatively low in both winter and summer.
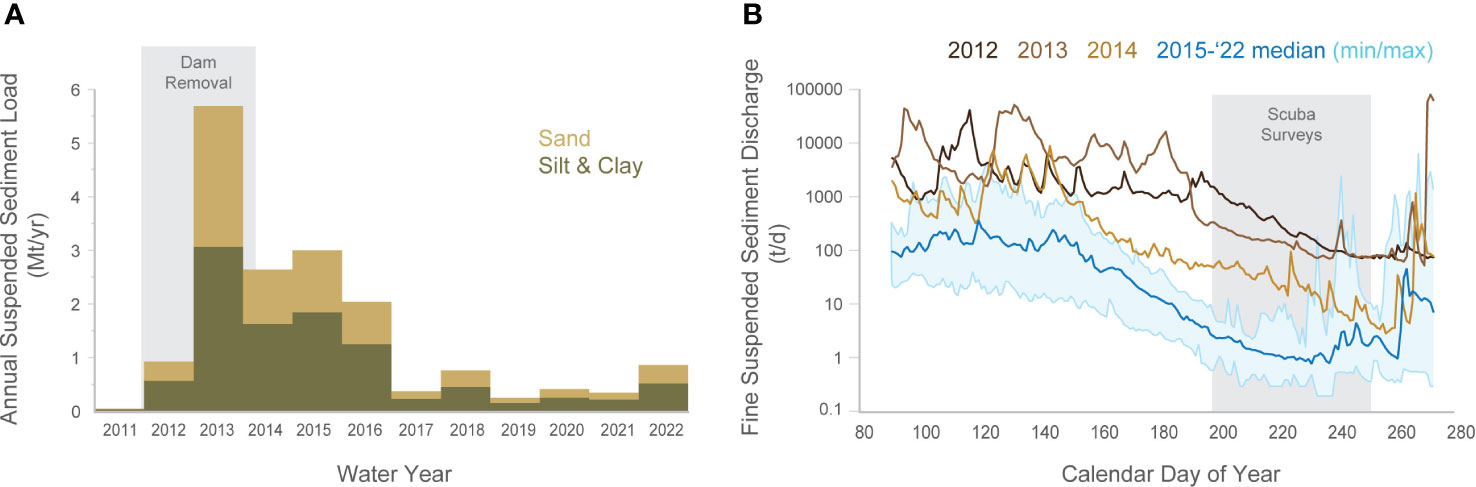
Figure 2 Annual suspended sediment (A) discharge from the Elwha River for the period 2011-2022, and daily fine suspended sediment (B) discharge during the estimated biologically relevant “growing season” (mid-March to October) for the years during dam removal (2012, 2013 and 2014) and after dam removal (2015-2022). Data for water years 2012-2016 are from Ritchie et al. (2018); and water years 2017-2022 from rating curves derived from WY2016 data.
3.2 Sea-floor morphology changes
Morphologic changes to the seafloor occurred near the Elwha River mouth during dam removal (Gelfenbaum et al., 2015), resulting in vertical sediment deposition exceeding 5 m in some locations (Figure 3E). Sediment deposits to the west of the river mouth were composed primarily of mud, and to the east of the river mouth of fine sand (Figure 3H). As sediment flux to the nearshore declined after dam removal ended (Figure 2A) these new sub-tidal deposits were eroded and re-worked in response to hydrodynamic forces (Figures 3C, F, I). Notably, the sand deposit on the east side of the delta eroded, and deposits at all points around the delta coarsened (Figures 3F, I). The four sites in the buried site group include those nearest to the river mouth, and at shallower depths. Two sites were in an area of mud deposition to the west of the river mouth, and two sites were in an area of fine sand deposition to the east of the river mouth (Figures 3E, F).
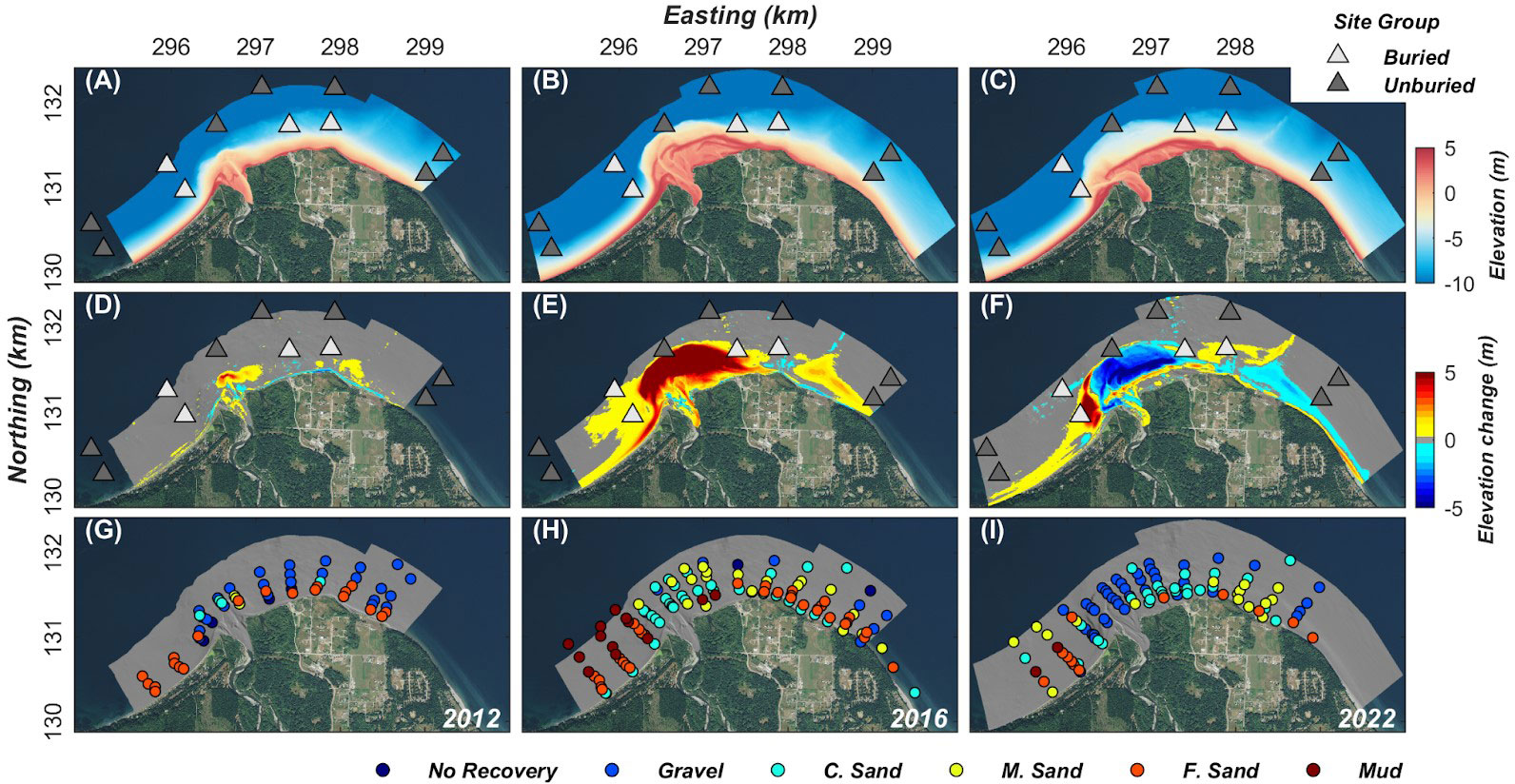
Figure 3 Bathymetry (A–C), elevation change (D–F), and mean surface sediment grain size (G–I) of the Elwha River delta in 2012 (left column), 2016 (middle column), and 2022 (right column). Elevation changes are calculated between 2012-2011 (D), 2016-2012 (E), and 2022-2016 (F). Sites where no sediment sample was recovered were interpreted to represent hard ground or a substrate so coarse that a sample could not be collected, and are marked on the map as “No Recovery”. Bathymetry and grain size data are from Stevens et al. (2017). The dive sites shown in Figure 1 are added in panels (A–F) for context, and shaded according to their site group (i.e., buried vs. unburied).
3.3 Change in density and richness of kelp, invertebrates, and fish
The earliest and most pronounced change during dam removal was the decrease of kelp density and species richness at both buried and unburied site groups beginning in 2012 (Figures 4A, B). This change is consistent with the timing of an increase in suspended sediment flux from the river following the breach of the Elwha Dam (Figure 2A; Rubin et al., 2017), increasing the turbidity of coastal waters (Foley and Warrick, 2017) and reducing the availability of light in the water column (Glover et al., 2019). The trajectory of change following the initial response of kelp to dam removal, however, was different for the buried and unburied site groups. Within the buried site group, density and species richness of kelp did not recover to pre-removal levels by 2022 (Figures 4A, B) despite a substantial reduction in suspended sediment flux between 2017 and 2022 (Figure 2).
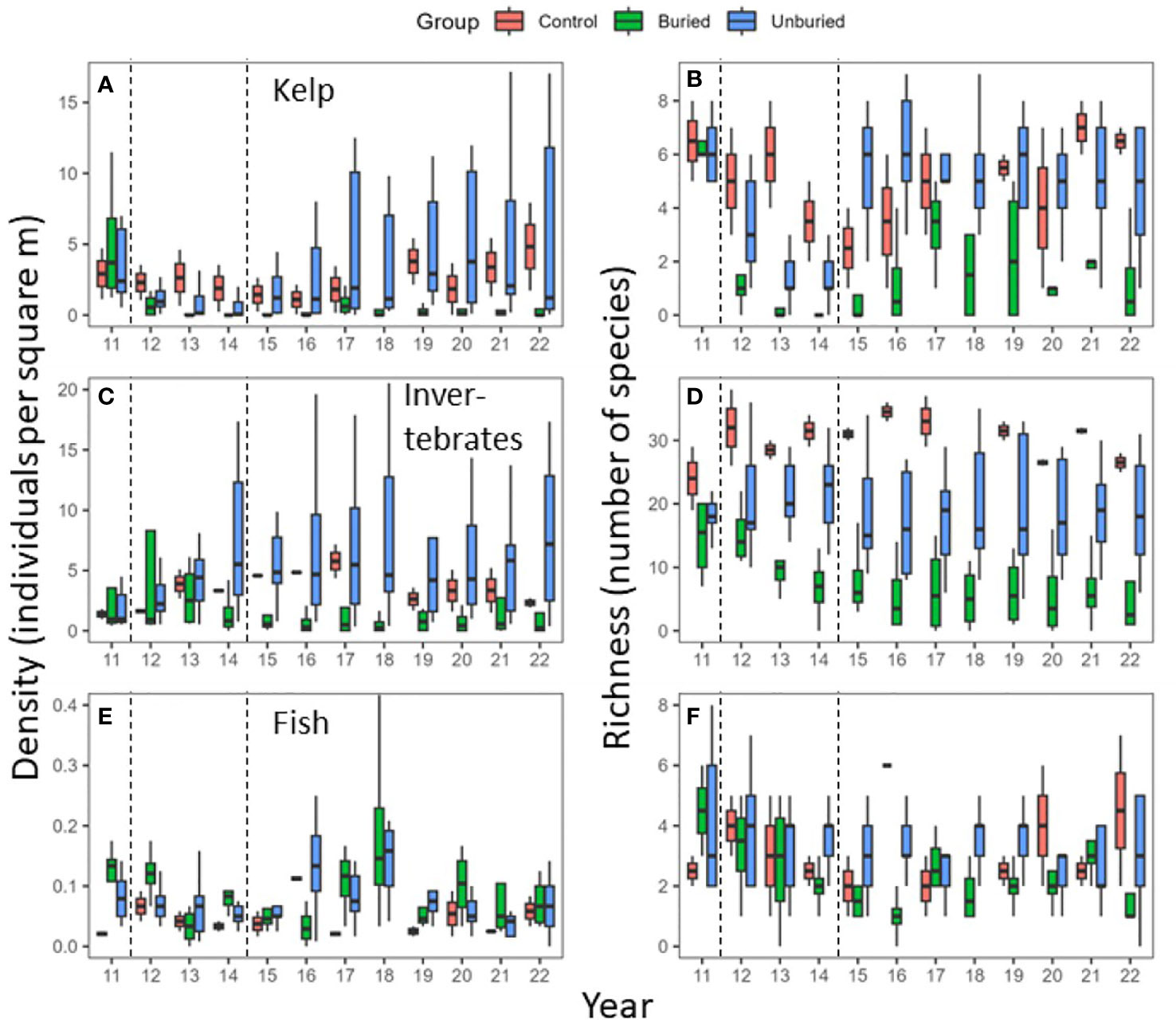
Figure 4 Box and whisker plots of density (left panels) and species richness (right panels) for kelp (A, B), invertebrates (C, D), and fish (E, F) by year and site group. Boxes were constructed from data for each site-year. The top and bottom of boxes are the upper and lower quartiles and the line is the median. The whiskers extend from the upper (or lower) quartile to the largest (or smallest) value no farther than 1.5 times the inter-quartile range. Outliers are not shown. Dotted lines indicate the start and end of dam removal.
At the unburied sites, kelp density and richness decreased immediately following dam removal when suspended sediment flux was highest (Figures 2, 4A, B). Unlike the buried sites, the density of kelp at unburied sites started to rebound in 2015 (Figure 4A), coinciding with reduced sediment flux from the river, especially during the spring and summer months (Figure 2B). The rate of kelp recovery was rapid at unburied sites, largely returning to pre-removal levels by 2015 for richness and 2017 for density, although variability among sites was considerable within the unburied group (Figures 4A, B). Density of Desmarestia sp., the other main group of brown algae in the study area besides kelp, total percent cover of algae, and percent cover of red algae, the other main algal group besides brown, showed trends across time generally like kelp at buried and unburied sites (Figures S1B, C, E, F).
For invertebrates and fish at sites within the buried group, density and species richness also decreased during dam removal and had not recovered to pre-removal levels by 2022 (Figures 4C–F). The decrease in invertebrate and fish density and species richness were most pronounced in 2013 (fish) and 2014 (invertebrates), which coincided with the period of sediment deposition in the buried site group (Figure 3; Gelfenbaum et al., 2015; Rubin et al., 2017). At buried sites, fish density increased briefly in 2017 and 2018, decreased in 2019, and then remained low through 2022 (Figure 4).
Invertebrate and fish density and species richness did not decrease within the unburied site group during dam removal (Figure 4; Rubin et al., 2017). Invertebrate density at unburied sites increased in 2014 and remained high through the end of the time series. Fish density within the unburied site group briefly increased from 2016-2018, but otherwise remained at a similar level throughout the time series. Invertebrate and fish density and species richness were highly variable within site groups between 2011-2022 without any clear trend over time.
At the control sites, density and species richness of kelp, invertebrates, and fish, and density for Desmarestia sp. and percent cover for red algae, varied annually between 2011 and 2022, but with no clear trend over time (Figures 4, S1A, D). The decrease in kelp richness at control sites in 2014-2016 is consistent with regional patterns of kelp decline due to a marine heatwave affecting the eastern Pacific (Tolimieri et al., 2023).
3.4 Community composition during and after dam removal
3.4.1 Kelp
Kelp community composition changed significantly from 2011-2022 at all site groups (Table 1; Figure 5A). For the buried site group, the loss of all kelp species drove the change in community composition between years (Figures 5B, 6A; Tables S1, S2). Community composition measured at the buried sites was most different between 2011 (before dam removal) and 2014 (end of dam removal; points on the NMDS plot that are closer together are more like each other than points farther apart). Community composition did move closer to starting conditions after 2014, mainly due to modest increases in Pterygophora californica and Nereocystis luetkeana (Figure 6A; Table S1), but did not return to before dam removal composition by 2022 within the buried group. Between-year variability became less pronounced between 2018 and 2022 (Figure 5B) when sediment flux from the river was lower than previous years (Figure 2A).
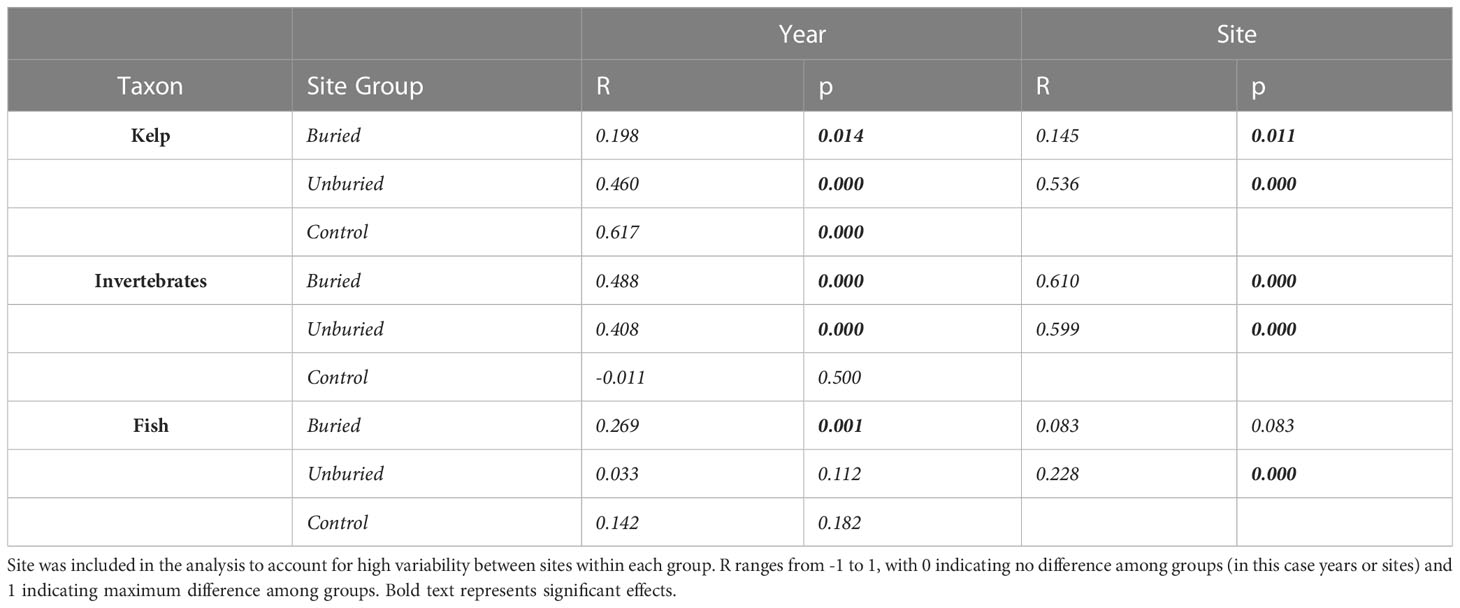
Table 1 Results of two-way crossed ANOSIM results for community differences among years and sites for kelp, invertebrates, and fish at buried, unburied, and control site groups.
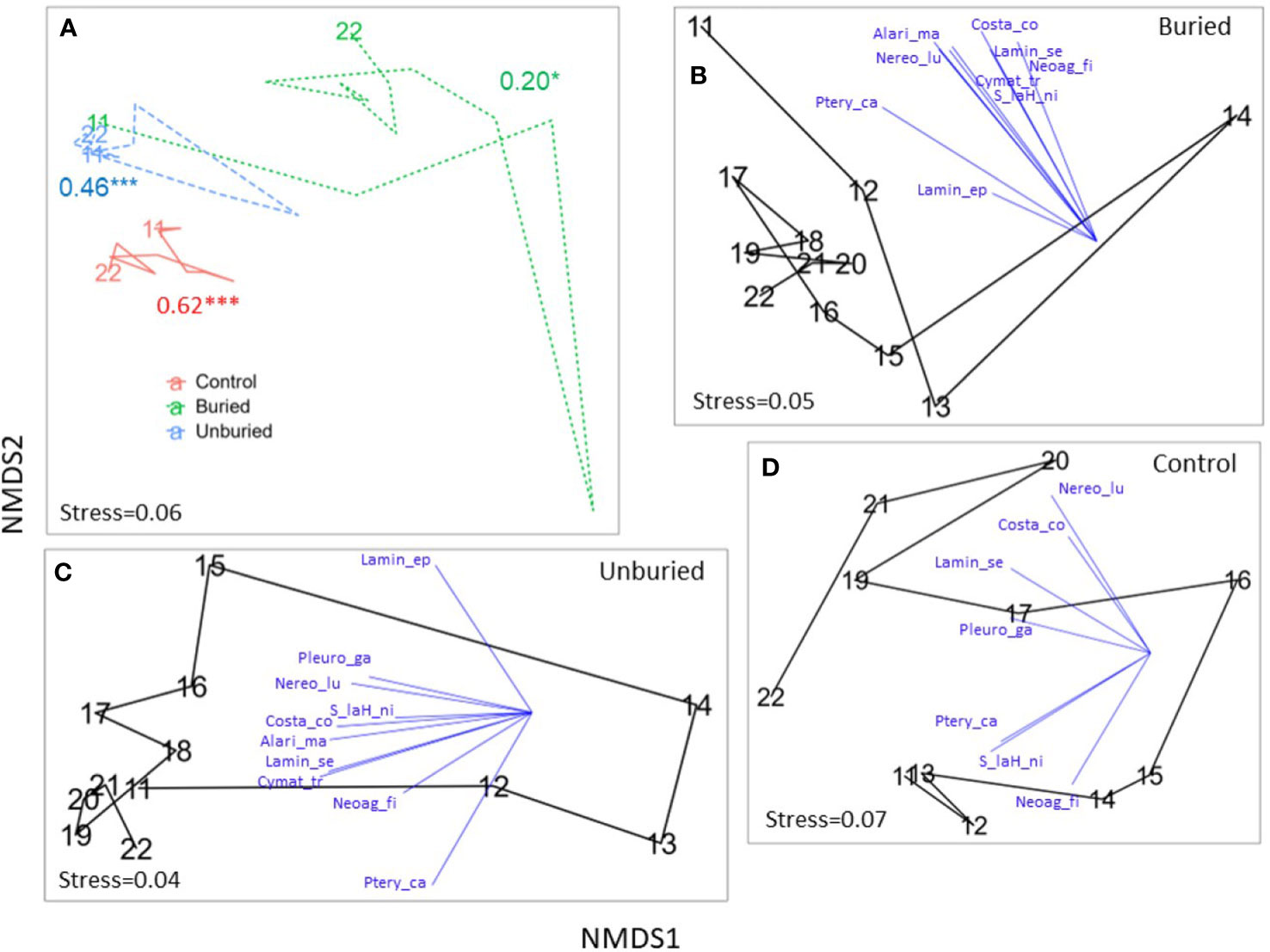
Figure 5 NMDS plots showing trajectories of kelp community change across years by site group. The communities—in terms of species composition and density—in the closer together points are more similar than farther away points. (A) Site groups are shown together with ANOSIM R and p-values (* p < 0.05, ** p < 0.01, *** p < 0.001) for each site group included (see Table 1) and the first and last years labeled. (B–D) Buried, unburied, and control site groups, respectively, are shown separately with blue vectors indicating the direction and strength (vector length) of correlations between density of kelp species and NMDS axes included for species contributing most to community change (see Table S1), and all years labeled. See Table 2 for full species names. Note that points in (A) were plotted with the goal of showing similarities (differences) among all year-by-site group combinations, whereas points in (B–D) were replotted to show similarities between years within a particular site group, so trajectories in A do not exactly match trajectories in (B–D).
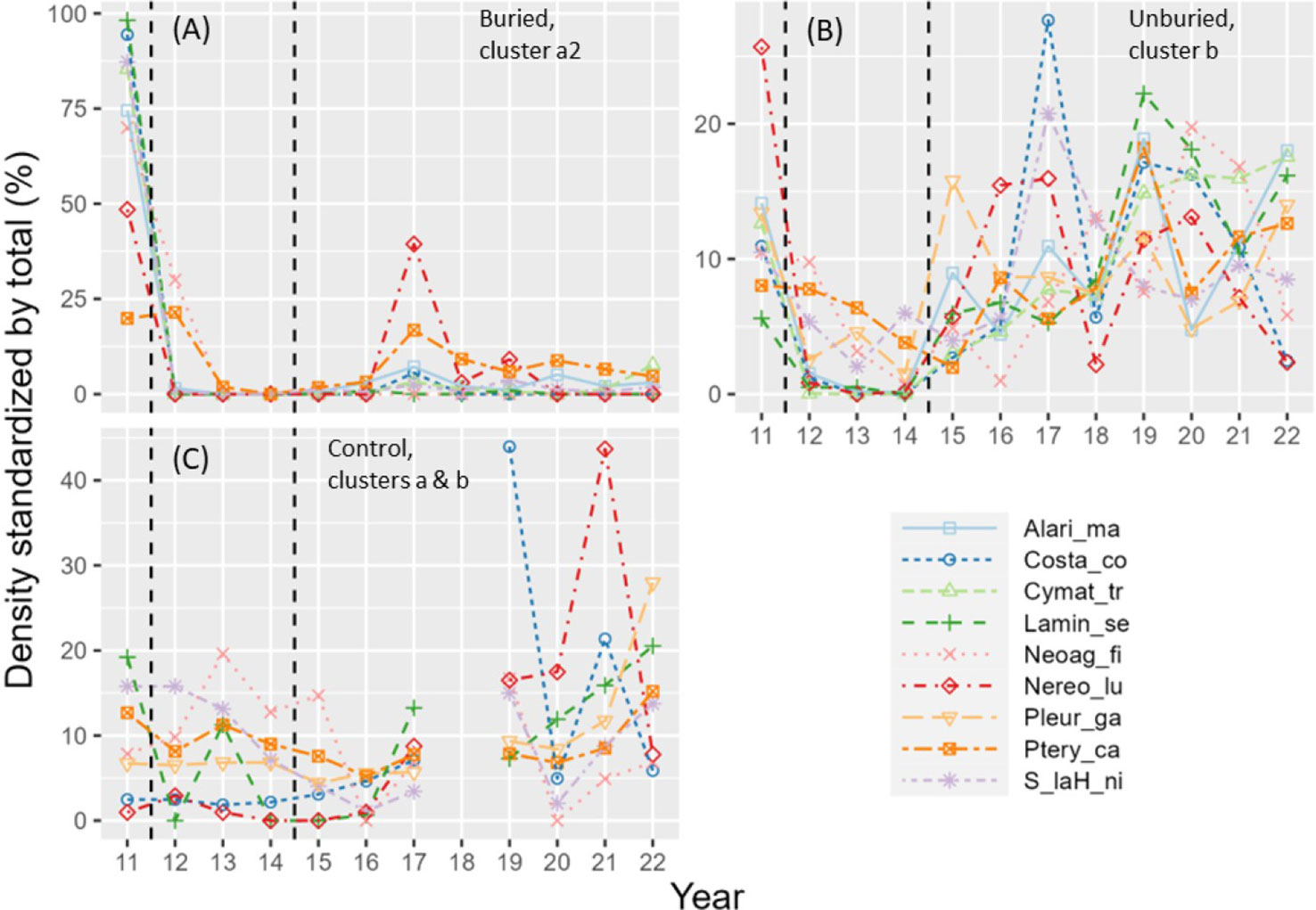
Figure 6 Standardized density (density in each year divided by total density across all years) versus year for kelp species that changed similarly across years according to cluster analyses (see Table S2 for clusters) for buried (A), unburied (B), and control (C) site groups. Vertical dashed lines show start and end of dam removal. See Table 2 for full species names.
For the unburied site group, the loss of all kelp species during dam removal drove the change in composition across years, particularly from 2012-2015 (Figure 6B). Consistent with the recovery of density and species richness (Figures 4A, B), kelp community composition returned to a state similar to that before dam removal by 2018 (Figure 5C). Individual species density was highly variable across years (Figure 6B), but most species started to increase in 2015.
Within the control group, changes in kelp community composition across years (Figure 5D), which was least variable over time of the three site groups (Figure 5A), was driven by the annually variable densities of kelp species (Figure 6C) rather than a complete loss of species as in the buried and unburied site groups. Note that there was little change in kelp community composition from 2011-2013 within the control group, which is when drastic changes occurred at the buried and unburied site groups (Figures 4–6), suggesting the effects of dam removal did not affect the control group.
3.4.2 Benthic invertebrates
For the invertebrate community, composition change across years was significant for the buried and unburied site groups but not for the control (Table 1; Figure 7A). Within the buried site group, species composition changed substantially following dam removal, driven by the loss of multiple species, including anemones (Halcampa spp. and Epiactis spp.), sabellid tubeworms (Schizobranchia spp., Chone aurantiaca, Eudystylia vancouveri), and an increase in crabs (Metacarcinus magister), geoduck (Panopea abrupta), and polychaete worms (Pista sp., Diopatra ornata) (Figures 7B, 8A, B; Tables S1, S2). Community composition did not return conditions like those before dam removal by 2022, but composition was similar in 2021 and 2022.
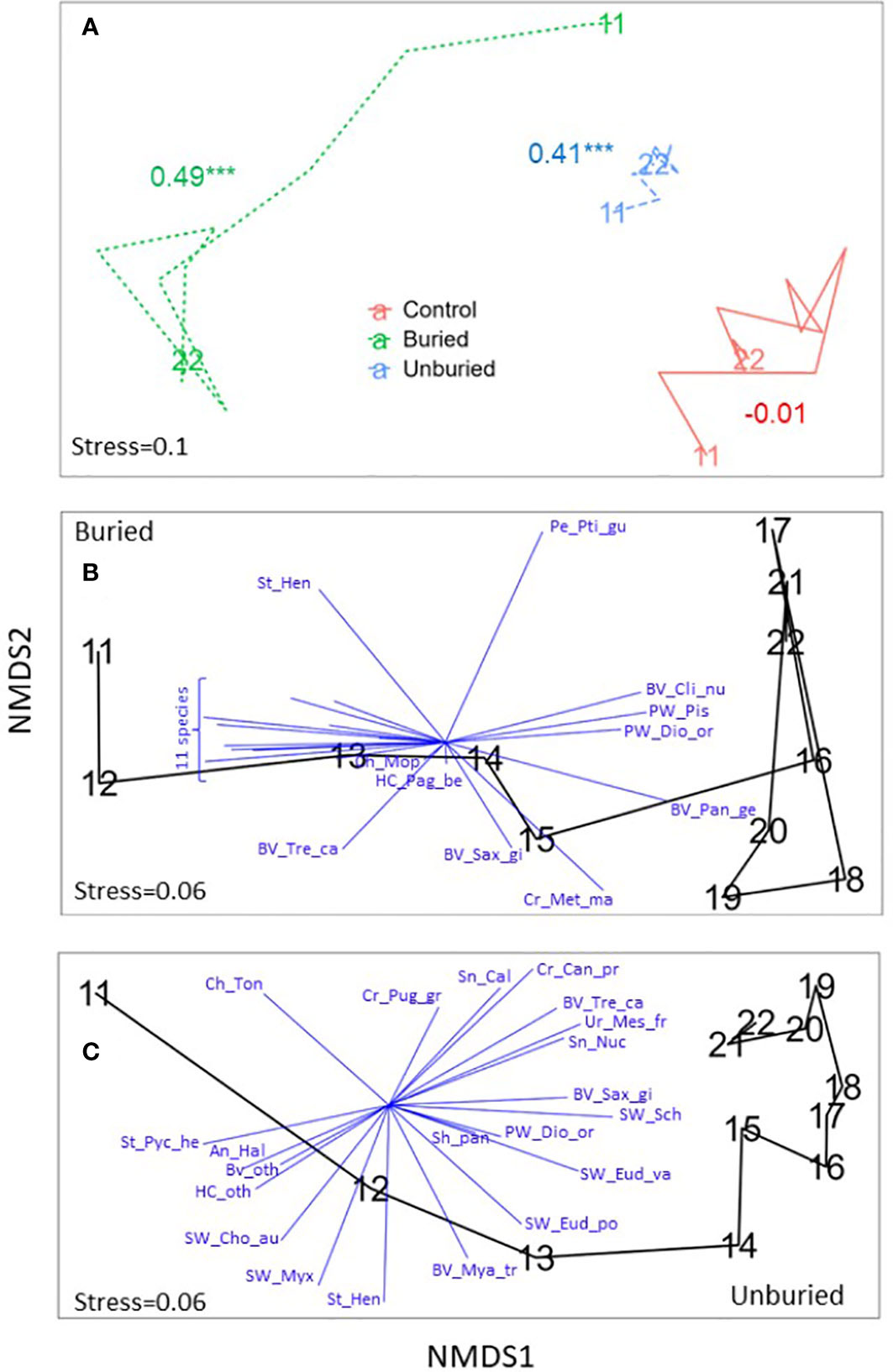
Figure 7 NMDS plots showing trajectories of invertebrate community change across years by site group. (A) Site groups are shown together with ANOSIM R and p-values (* p < 0.05, ** p < 0.01, *** p < 0.001) for each site group included (see Table 1) and the first and last years labeled. (B) and (C) Buried and unburied site groups, respectively, are shown separately with blue vectors indicating the direction and strength (vector length) of correlations between density of invertebrate species and NMDS axes included for species contributing most to community change (see Table S1), and all years labeled. The 11 bracketed species in (B) are: An_Epi, An_Hal, An_oth, Cr_Can_pr, Cr_Met_mj, Hc_oth, Sh_Cra, St_Pyc_he, SW_chone, Tw_Eud_va, and SW_Sch. See Table 2 for full species names.
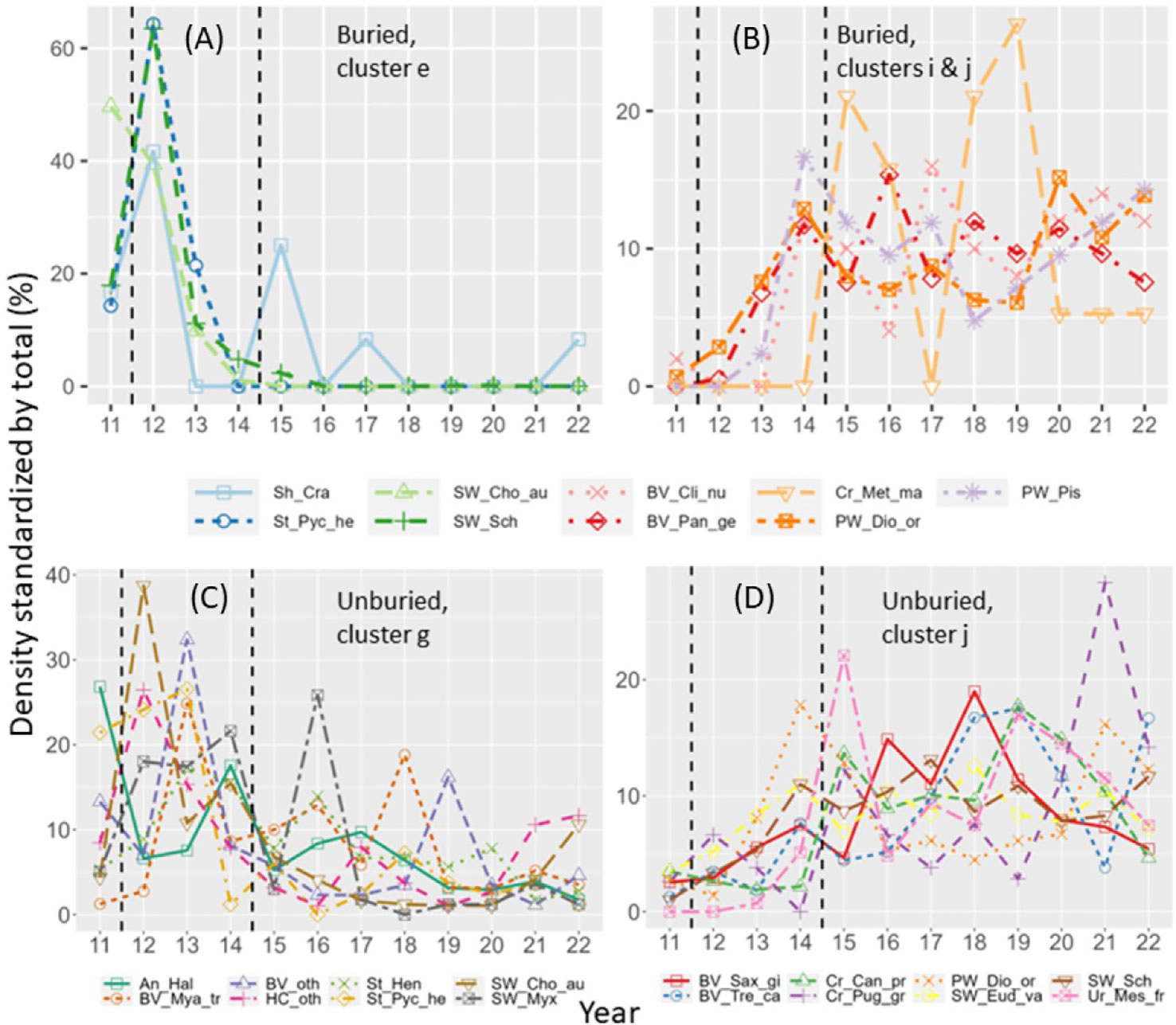
Figure 8 Standardized density (density in each year divided by total density across all years) versus year for invertebrate species that changed similarly across years according to cluster analyses (see Table S2 for clusters). (A) Species that decreased at buried sites. (B) Species that increased at buried sites. (C) Species that decreased at unburied sites. (D) Species that increased at unburied sites. See Table 2 for full species names. Vertical dashed lines show start and end of dam removal.
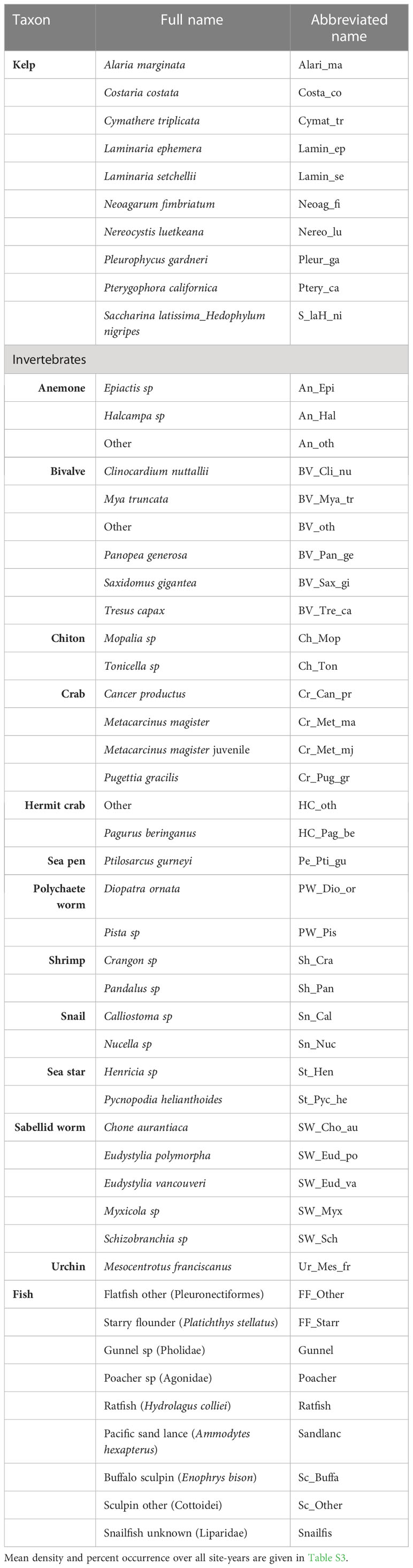
Table 2 Full names and abbreviations assigned to species, or lowest identified taxonomic level, in figures and tables.
Invertebrate community composition was comparatively less variable over time for the unburied site group than the buried site group and was driven by increases and decreases of a range of species (Figures 7C, 8C, D; Tables S1, S2). This response was fundamentally different from the response of the kelp community, which had the same trajectory for all species. There was a marked increase in two sabellid worms (Schizobranchia spp., Eudystylia vancouveri) and bivalves (Saxidomus gigantea, Tresus capax), and a decrease in anemones (Halcampa sp.), bivalves (Mya truncata, other), and two other sabellid worms (Chone aurantiaca, Myxicola sp.). The dissimilarity between invertebrate community composition across years in the unburied site group was much smaller than in the buried site group (Figure 7A), which was also the case for kelp (Figure 5A). However, invertebrate community composition in the unburied site group did not return to before dam removal conditions by 2022, but composition was relatively stable from 2019-2022 (Figure 7C).
In the unburied site group, there was also a marked decrease in sea star abundance, particularly Pycnopodia (Figure 8C). While negative effects from dam removal cannot be completely ruled out, the disappearance of this species and Henricia sp. was likely most attributable to sea star wasting syndrome (SSWS) that decimated sea star populations in the eastern Pacific beginning in 2014 (Montecino-Latorre et al., 2016). Note that Pycnopodia also decreased at buried sites but the decrease mainly occurred in 2013 (Figure 8A) when sites became buried but before SSWS started. The red urchin, Mesocentrotus franciscanus, only occurred at one unburied site, but at that site its abundance started to increase in 2014 concurrently with the loss of sea stars, reaching a peak in 2015 and continuing to be higher than before SSWS occurred (Figure 8D). Algae had not recovered at the site by 2014.
3.4.3 Fish
Fish community composition in the buried site group changed significantly between years (Table 1; Figure 9A). Like invertebrates in the buried site group, the density of many fish species—including gunnel (Pholidae), ratfish (Hydrolagus colliei), and sculpins (Cottoidei)—decreased when vast amounts of sediment were deposited near the river mouth in 2013-2014 and did not return to previous densities (Figure 10B). Some fish species, however, were able to take advantage of the newly created habitat at the buried sites near the river mouth, including flat fish (Pleuronectiformes), snailfish (Liparidae), and poacher (Agonidae), and increased in density after dam removal (Figure 10A). Similar to kelp and invertebrates, fish community composition did not return to a state similar to that prior to dam removal by 2022 but was relatively similar from 2020-2022 (Figure 9B).
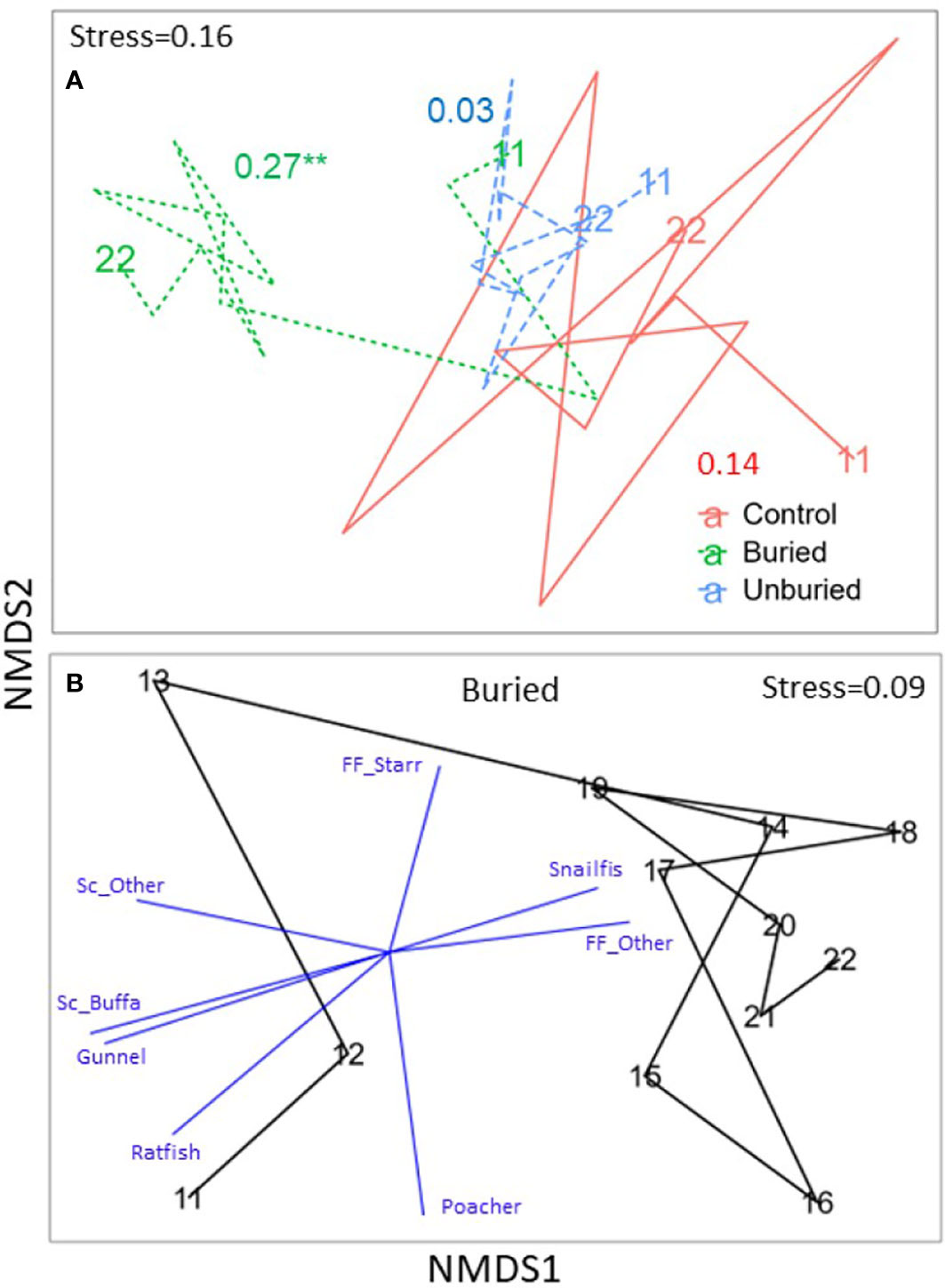
Figure 9 NMDS plots showing trajectories of fish community change across years by site group. (A) Site groups are shown together with ANOSIM R and p-values (* p < 0.05, ** p < 0.01, *** p < 0.001) for each site group included (see Table 1) and the first and last years labeled. (B) The buried site group is shown separately with blue vectors indicating the direction and strength (vector length) of correlations between density of fish species and NMDS axes included for species contributing most to community change (see Table S1), and all years labeled. See Table 2 for full species names.
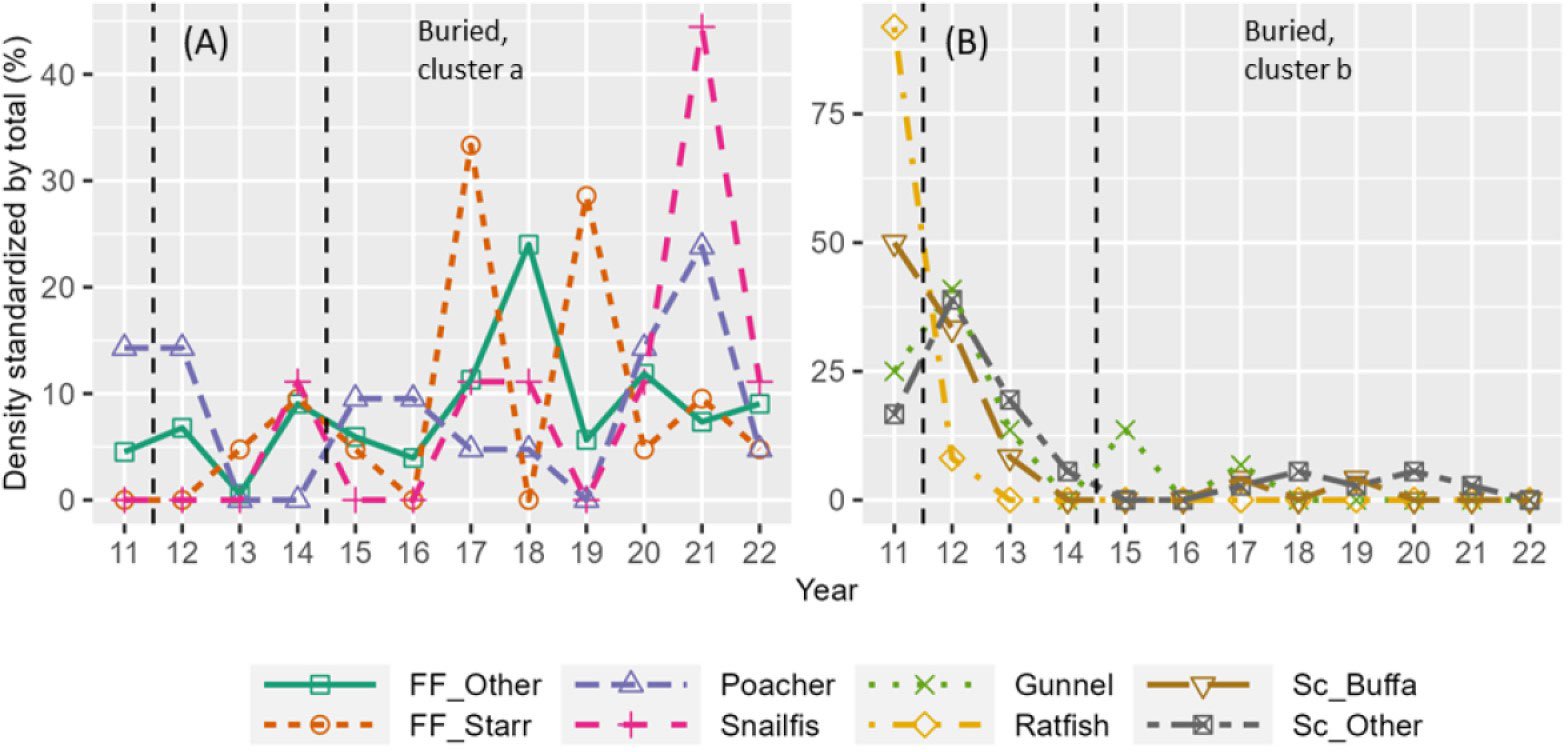
Figure 10 Standardized density (density in each year divided by total density across all years) versus year for fish species that changed similarly across years according to cluster analyses (see Table S2 for clusters). (A) Species that decreased at buried sites. (B) Species that increased at buried sites. See Table 2 for full species names. Vertical dashed lines show start and end of dam removal.
Pacific sand lance (Ammodytes hexapterus) were difficult to accurately count because they were either darting in and out of the sediment or schooling in the water column, so we only recorded them as present or absent. When sand lance were present there were usually many of them. We rarely encountered sand lance at unburied sites (4 occurrences in 108 site-years) or control sites (4 occurrences in 22 site-years).
3.5 Mechanisms driving change in the buried and unburied site groups
The major physical changes in the nearshore during dam removal included increased suspended sediment flux (Figure 2) and sediment deposition (Figure 3). For the buried site group, the dominant mechanism driving community change was burial and an accompanying change in grain size (Figures 3G–I). The change in grain size, however, was not the same at all buried sites. At the sites to the west of the river mouth, sand was buried by mud; to the east, gravel was buried by sand (Figures 3G–I).
This change in grain size resulted in changes in invertebrate and fish species present within the buried group (Figure 11). For invertebrates, the bivalves Clinocardium nuttallii (cockles) and Panopea generosa (geoduck clams) and the polychaete worms Diopatra ornata and Pista sp. were more abundant at the western buried sites (mud) following dam removal than before (Figure 11B). For fish, flatfish and snailfish became more abundant at the western sites when the habitat changed from sand to mud after burial. Poacher decreased after the western sites were buried but were still occasionally present.
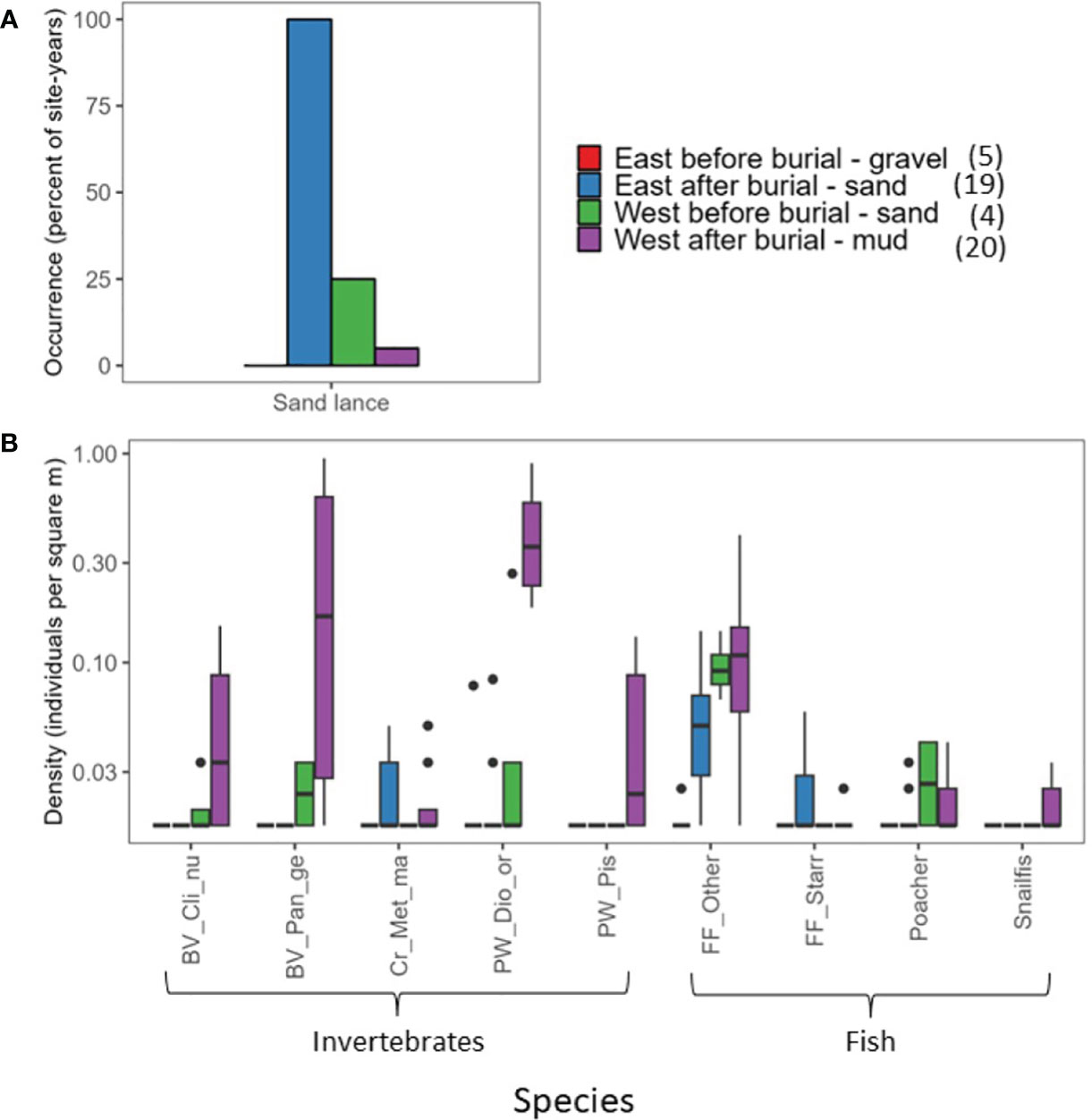
Figure 11 Occurrence or density at buried sites east and west of the river mouth before and after site burial for invertebrates and fish that were abundant after site burial. (A) Percent occurrence of Pacific sand lance. (B) Box and whisker plots for densities of invertebrates and fish other than sand lance; the top and bottom of boxes are the upper and lower quartiles, the line is the median, and the whiskers extends from the upper (or lower) quartile to the largest (or smallest) value no farther than 1.5 times the inter-quartile range. Numbers in parentheses in the legend are sample sizes (number of site-years).
For the buried sites on the eastern side of the delta where the habitat changed from gravel to sand, Metacarcinus magister (Dungeness crab) were recorded during our surveys after the sites were buried and had not been observed at those sites before. Sand lance were present during every survey in the new sand habitat at the eastern sites despite not being observed there before deposition (Figure 11A). Flatfish (other and starry flounder Platichthys stellatus) also became more abundant following burial at the east sites than before.
The mechanisms driving change in the unburied site group varied depending on taxonomic group. The only dam removal-related physical change at unburied sites was elevated suspended sediment. Kelp community composition was strongly related to FSSL in the river, which was correlated with suspended sediment in the nearshore (Glover et al., 2019), and total kelp density and percent algal cover were strongly negatively correlated with FSSL (Tables 3, 4; Figure S2A), suggesting that algal trajectories of change during and after dam removal were primarily driven by high suspended sediment levels during dam removal followed by water column clearing following dam removal (Figures 2, 4–6, S1). Kelp community composition was also related to Pycnopodia density at unburied sites (p < 0.05; Table 3). Kelp community composition (Table 3), kelp density, and algal cover were unrelated to FSSL within the control site group (p > 0.05).
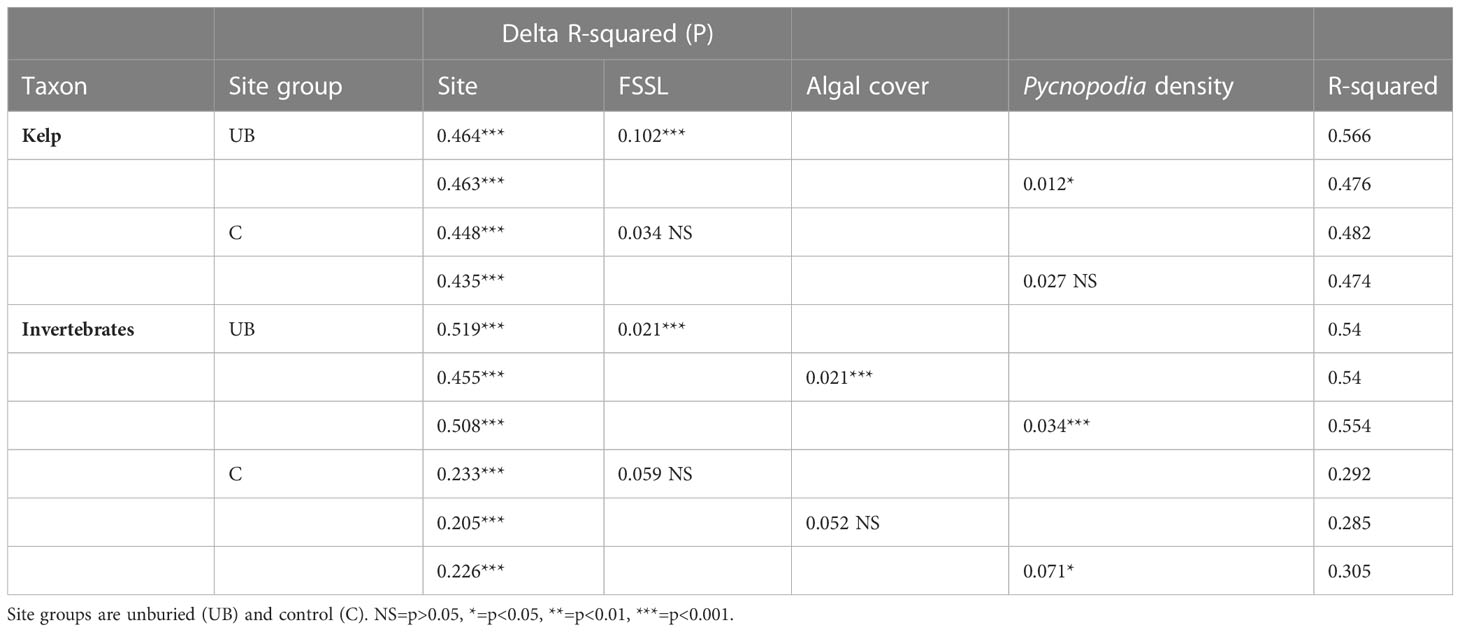
Table 3 Multivariate tests (distance based redundancy analyses) for associations between kelp community change and fine suspended sediment load (FSSL) or Pycnopodia density, and between invertebrate community change and FSSL, algal cover, or Pycnopodia density.
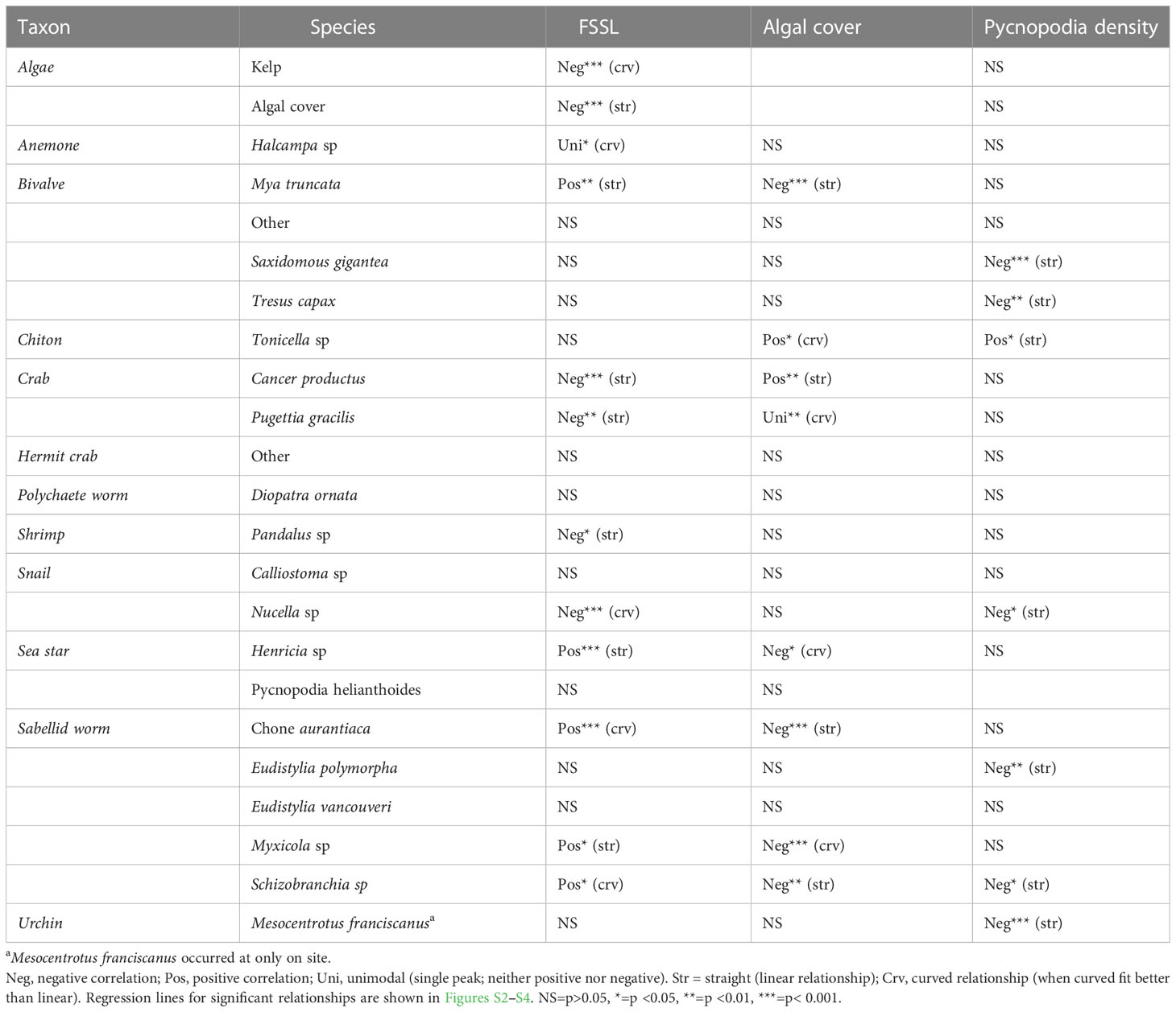
Table 4 For Elwha unburied sites only, univariate tests (regressions) for association of fine suspended sediment load (FSSL), algal cover, or Pycnopodia density with kelp density (summed over species), percent algal cover, or invertebrate species densities.
Invertebrates in the unburied site group were affected by changes in suspended sediment and (or) algal cover, and by changes in Pycnopodia density. Invertebrate community composition change within the unburied site group was significantly related to FSSL, algal cover, and the density of Pycnopodia (p < 0.001 for all three terms; Table 3). For invertebrates within the control site group, community change was not significantly related to FSSL or algal cover (p > 0.05) but was related to Pycnopodia density (p < 0.05; Table 3) even though the ANOSIM test did not detect significant community change across years at control sites (Table 1).
For individual invertebrate species that contributed most to community composition change within the unburied site group (Table S1; vectors in Figure 8C), the significance of associations with FSSL, algal cover, and the density of Pycnopodia varied widely based on the univariate regressions (Table 4). Except for the seastar Henricia sp., all species that were positively correlated with FSSL (Figure S2) were filter feeders (bivalves and sabellid worms). Most of those species also had a significant negative correlation with algal cover (Figure S3). Comparatively, Cancer productus (crab) density was negatively correlated with FSSL and positively correlated with algal cover. Multiple invertebrate species were negatively correlated with Pycnopodia density, including two species of bivalves, one snail, two sabellid worms, and one urchin (Figure S4). This correlation suggests that the loss of Pycnopodia from our sites between 2013 and 2014 could be driving the increase in abundance of those species (Figure 8D), particularly for species that were not correlated with FSSL or algal cover (bivalves, sabellid worm Eudistylia polymorpha, and red urchin Mesocentrotus franciscanus). Mean Pycnopodia density (averaged over site years) was higher at control than at unburied sites, and community composition change was significantly related to the density of Pycnopodia within the control and unburied site groups. However, different species were affected by Pycnopodia within those two site groups. All invertebrate species that were negatively correlated with the density of Pycnopodia within the unburied site group had no relationship with Pycnopodia density within the control site group, possibly because the density of these species was lower in the control than in the unburied site group (< 0.75 times as high).
4 Discussion
Sediment flux and deposition associated with dam removal can drive both short-lived and persistent changes to aquatic ecosystems (Foley et al., 2017b; Bellmore et al., 2019; Stanley and Doyle, 2003; O’Connor et al., 2015). During and immediately following dam removal on the Elwha River (2012-2016), nearly 90% of the 20 Mt of sediment eroded from behind the two dams was transported to the Strait of Juan de Fuca, resulting in significant deposition at the mouth of the river (Figure 3) and three orders of magnitude increase in suspended sediment concentration above background levels in the river. The physical changes associated with this sediment flux—deposition, changes in seabed substrate, and increased turbidity—were the dominant drivers of the multiple direct and indirect pathways that drove changes in algal, benthic invertebrate, and fish communities in the Elwha nearshore.
4.1 Two response trajectories: buried vs. unburied
Following disturbance events, systems can have a range of trajectories. In most cases, systems tend to either return to a condition closely resembling the pre-disturbance state or reset to a different state (Palumbi et al., 2008; Fryirs and Brierley, 2016). In the Elwha, Ritchie et al. (2018) described evidence for both models in the geomorphic system. Similarly, we found evidence for two trajectories of species and community change in the Elwha nearshore depending on the location of a site and whether a site was exposed to the joint stressors of increased turbidity and sediment deposition (buried site group, close to the river mouth) or only increased turbidity (unburied site group, farther away from the river mouth). Within each of these groups, we also found that different taxonomic groups responded differently to burial and increased turbidity, including differences in timing of response.
The geomorphic response in the coastal environment can explain, in part, the response trajectory of the marine community. Within the unburied site group, kelp responded directly to the increased flux of fine suspended sediment as soon as dam removal began (2012; Table 4) and started to rebound quickly once turbidity decreased shortly after dam removal was complete (Figures 4A, B, 5C). Increased suspended sediment decreased light availability at the unburied site group (Rubin et al., 2017), but may have also resulted in increased scour and temporary deposition, which is known to negatively affect algal recruitment and survival of juvenile sporophytes (Traiger and Konar, 2018; Picard et al., 2022). Kelp density and species richness at the unburied site group responded rapidly (Figure 4)—within a year—to the attenuation of FSSL after dam removal was complete. Overall kelp community composition at the unburied site group took longer—closer to ten years—to re-establish a state equivalent to the initial condition (Figure 5C). The close similarity between the initial kelp community and the community present at the end of our study, after a major disturbance, is notable. Watson and Estes (2011) followed recovery of kelp after sea otters reoccupied areas and removed red urchins, and found considerable variation in the re-established communities attributable to propagule availability, succession, and demography.
The invertebrate response within the unburied site group was not as universal across species as it was for kelp, nor was it directly tied to the timing of increased turbidity caused by dam removal for most species. Invertebrate density and richness did not immediately change when turbidity increased (Figures 4C, D), but overall community composition did change in 2012 (Figure 7C). Invertebrate density started to increase in 2013 and remained elevated compared to before dam removal throughout the time series (Figure 4C). For some species, increased turbidity and the loss of kelp seemed to allow them to gain a toehold at the unburied sites (Table 4; Figures S2, S3), and they were able to persist after kelp recovered and turbidity subsided (Figures 4C, 8D). Studies have shown that some filter feeders benefit from increased suspended sediment in the water column, particularly when it is correlated with an uptick in the availability of organic carbon (Dunton et al., 2006; McGovern et al., 2020). Decreases in understory algae can benefit sessile invertebrates by releasing them from competition for space (Arkema et al., 2009), or by increasing filter feeder access to food because the understory inhibits flow rates beneath it and possibly delivery of suspended particulate material as well (Eckman et al., 1989; Eckman and Duggins, 1991). For species whose abundance was positively correlated with algal cover, density tended to increase starting in 2015 (Figure 8D) when kelp started to recover.
There was no significant change in fish species composition within the unburied group, suggesting that increased turbidity and loss of kelp had little effect on the fish enumerated in our surveys. The lack of an effect of kelp density on fish abundance is contrary to other studies from kelp forest systems (Bodkin, 1988; Norderhaug et al., 2020). However, many of those studies focused on canopy-forming kelp (e.g., Macrocystis gigantea, Nereocystis luetkeana), while the kelp community in the Elwha nearshore is dominated by prostrate species, such as Alaria marginata, Costaria costata, and Laminaria spp. However, in some cases understory kelp too can be associated with increased fish abundance (Hamilton and Konar, 2007). There were fewer clear long-term invertebrate winners and losers within the unburied site group due to the transitory nature of the dam removal effects at these sites. The invertebrates that were able to sustain an advantage from short-term changes in turbidity and algal cover appear to be the long-term winners following dam removal.
The response of kelp, invertebrates, and fish within the buried site group was consistent with previous studies in other marine ecosystems following large sediment deposition events where communities tend to shift to an alternate state (Miller et al., 2002; Lohrer et al., 2006; Connell et al., 2008; Watanabe et al., 2016). The species response at the buried site group highlighted winners and losers due to dam removal. Species that were dependent on the gravel substrate at the eastern buried sites were lost locally and had not recovered by 2022. For the sand-dependent species, the area of new habitat created by deposition of relatively fine sediment associated with dam removal resulted in density increases. Within the buried site group, kelp responded initially to the increased turbidity in 2012 and recovery was precluded due to burial and a change in habitat suitability when the cobble substrate at the eastern buried sites was buried by sand (Figure 3I), which reduced or eliminated substrate suitable for holdfast attachment. For invertebrates, the effects were more species specific, although the overall trajectory was decreased density and species richness (Figure 4). The initial increase in turbidity resulted in an increase in filter feeding sabellid worms, followed by a steep decline once the sites were buried in 2014 (Figure 8A). For other species, burial provided new habitat that was rapidly colonized by species that rely on finer grained habitat than cobble. For fish, increased turbidity at the start of dam removal in 2012 did not result in drastic changes to species density and richness or overall community composition, but burial and related grain size changes in 2013 and 2014 resulted in decreased richness and a significant change in species composition (Figures 4, 9). For some species, particularly the ecologically important forage fish Pacific sand lance, the change in habitat at the river mouth was a boon for their population (Figure 11). Frick et al. (2022) also saw an increase in sand lance at their Elwha sites after dam removal, but the increase was not significant. For all three taxonomic groups at the burial sites, despite their different initial responses to turbidity and burial, they all reached a relatively stable level of density and richness, and consistent community composition by 2016, following the peak of sediment deposition at the buried sites (Figures 3–5, 7, 9). The evolution of seafloor deposits formed during dam removal is on-going (Figure 3), with important implications for the species found within the buried site group. As the deposits of mud and sand formed during dam removal are eroded by tides and waves and the substrate coarsens (Figure 3I), it is possible that the composition of the benthic community associated with those new habitats will continue to evolve until geomorphic processes stabilize.
Within the control site group, change was unrelated to the effects of dam removal. Kelp community composition did significantly change over the course of our study, but the trajectory of change was highly variable over time (Figure 5D) and changes in density and species richness did not correspond with the timing of changes within the buried and unburied site groups (Figure 4). There were regional changes that occurred at our sites (see Other drivers of change below) that likely had a stronger effect on the variability of kelp, invertebrates, and fish within the control site group.
4.2 Other drivers of change
While dam removal was the dominant driver of change for kelp and some invertebrate and fish species from 2012-2015 for the unburied and buried site groups, dam removal was not the only disturbance playing out in the Pacific Northwest during our study period. As mentioned previously, SSWS decimated populations of Pycnopodia in the Strait of Juan de Fuca between our 2013 and 2014 surveys (Montecino-Latorre et al., 2016; Harvell et al., 2023) and populations had not recovered by 2022 (Figure 8C). Correlation analysis showed that the changes in the density of Pycnopodia was significantly negatively correlated with densities of six invertebrate species in the unburied site group, including (from strongest effect to weakest) Mesocentrotus franciscanus, Saxidomus gigantea, Schizobranchia sp., Tresus capax, Eudistylia polymorpha, and Nucella sp. (Table 4; Figure S4). Pycnopodia is a generalist in most subtidal communities (Duggins, 1983), so the loss of Pycnopodia may have affected other invertebrate species besides those we included in our analysis. We limited our analysis to those species that contributed to the overall change in invertebrate community composition within the unburied site group during our study. To the north of our study sites, but still within the Salish Sea, Schultz et al. (Schultz et al., 2016) found evidence of a trophic cascade in the subtidal communities in Howe Sound following the loss of Pycnopodia, whereby the population of green urchins (Strongylocentrotus droebachiensis) increased, and kelp cover declined. Although invertebrate community composition did not change significantly across years within the control site group (Table 1; Figure 7A), community composition at control sites was significantly related to the density of Pycnopodia (Table 3). This mixed result provides some support for a more extensive regional effect of the loss of this important sea star predator.
For some species, density increases following the loss of Pycnopodia may have resulted from behavior changes rather than reduced predation mortality. Urchins sometimes come out of hiding and switch from passive to active foraging (Smith et al., 2021) and are preyed upon by and show an escape response to Pycnopodia (Duggins, 1983), however, susceptibility of red urchins (Mesocentrotus franciscanus) to Pycnopodia predation is greatly reduced when they reach full size (Duggins, 1983). Although we did not take size measurements, the red urchins we censused appeared to be full sized. They were usually aggregated on boulders or more spread out on gravel-cobble substrate, suggesting a change in foraging behavior rather than a release from predation.
The other regional event that coincided with the tail end of increased suspended sediment associated with the dam removal was the largest marine heatwave on record from winter 2013-2015 (Di Lorenzo and Mantua, 2016). Given the regional nature of this event, we would expect to be able to see the effects, if any, of the marine heatwave at our control sites. For kelp, there was significant change in community composition across years that was not temporally aligned with change at Elwha sites (little change from 2011-2013 at control sites compared to great change at Elwha sites; Figure 5). The waters along the outer coast of Washington, including the western entrance to the Strait of Juan de Fuca, experienced anomalously warm temperatures in 2013 and 2014 (Tolimieri et al., 2023), and canopy kelp extent was anomalously low on the outer coast and in the Strait of Juan de Fuca in 2014 (Pfister et al., 2018). Kelp density at our control sites decreased from 2013 to 2016 with some recovery in 2017 (Figure 4), and Nereocystis leutkeana and Laminaria setchellii were lost in 2014 but reappeared by 2016-2017 (Figure 6C), accounting for the large change in kelp community composition between 2013 and 2017 (Figure 5D). These results suggest some correspondence between kelp response at our control sites and regional trends in ocean temperatures and canopy kelp abundance. We would expect that regional oceanographic conditions would affect kelp at Elwha sites as well, but we were unable to separate regional from dam removal effects based on our sampling design because of the overlap of the two stressors.
The correlations we observed between community composition and the loss of sea stars (Tables 3, 4; Figure S4), along with the potential connection with climate variability (Tolimieri et al., 2023) cloud, to a degree, our ability to characterize the long-term dam removal response in the marine ecosystem, as well as to predict the ongoing trajectory of change. Invertebrate community composition within the unburied site group, for example, was indirectly related to dam removal (correlation to algal cover), but directly affected by SSWS. The relaxed predation pressure likely drove increased densities of bivalves, urchins, and some worms, suggesting that both dam removal and SSWS contributed to the response trajectory. It is possible that dam removal sparked changes in the marine community that were then further shaped by additional stressors in the system, some of which we could directly test and others that we could not.
4.3 Considerations for other dam removals
While dam removal is accelerating in the United States (O’Connor et al., 2015), the number of dam removals motivated by, or that considered, marine impacts or restoration in their management is small (Ralston et al., 2021; Cancel Villamil and Locke, 2022). The removal of the two dams on the Elwha was notable for its influence on multiple aspects of the marine system (Gelfenbaum et al., 2015; Rubin et al., 2017; Shaffer et al., 2017; Glover et al., 2019; Warrick et al., 2019). It is likely that a variety of factors contributed to those effects, including the characteristics of the impounded sediment (volume, erodibility, and grain size distribution), length and steepness of the river system, and distribution of dams within the watershed (i.e., tributary versus mainstem), which have been well characterized, particularly for dam removals in the western United States (Foley et al., 2017a; Major et al., 2017). The characteristics of marine waters into which the watershed drains—waves, currents, and bathymetry—influenced sediment dynamics in the nearshore. While data on the marine influence of dam removals besides Elwha is generally lacking, sediment dynamics in the nearshore from other sediment disturbances (e.g., land use change, landslides) have been studied (Crain et al., 2009; Erftemeijer et al., 2012; Booth, 2020; Rangel-Buitrago et al., 2023). Below we highlight processes that contributed to the geomorphic changes and ultimately ecological responses and how they may be used to consider effects of sediment disturbances, including dam removal, in other locations.
The Elwha River delivered 20 Mt of sediment to the nearshore during and immediately following dam removal, resulting in greater than 5 m of deposition near the river mouth (Figure 3E). The watershed steepness, proximity of dams to the coast, and lack of additional impoundments all contributed to the rapid flux of sediment to the nearshore and subsequent reduction following the completion of dam removal (Ritchie et al., 2018). The tidal currents in the Strait of Juan de Fuca are a dominant driver of sediment transport at the Elwha and likely contributed to a reduced amount of fine sediment retention (Warrick et al., 2008; Miller et al., 2011) compared to other systems with weaker tidal forcing. Of the 20 Mt of sediment delivered to the nearshore, approximately 60% was fine silt and clay (fine suspended sediment), which was predominantly dispersed away from the Elwha River delta by the strong tidal currents (Gelfenbaum et al., 2015). This sediment was transported predominantly to the east of the river mouth, affecting the spatial distribution of water column turbidity (Gelfenbaum et al., 2015; Foley and Warrick, 2017; Glover et al., 2019) and resulting impacts to nearshore communities (Rubin et al., 2017).
During the dam removals, the large flux of coarse sediment to the nearshore overwhelmed the littoral transport processes resulting in widespread accumulation of mostly sand-sized sediment (Figures 3E, H) that deposited on top of formerly eroding lag deposits of cobbles and boulders (Warrick et al., 2008). The two dams were operated as run of river dams, allowing for the passage of a substantial portion of fine sediment to reach the nearshore while the dams were in place, while retaining most of the sand and coarse sediment in the reservoirs. With the removal of the dams, it is likely that sand delivery to the nearshore will be greater than when the dams were in place. While the large influx of sediment initially resulted in deposition near the river mouth, those deposits are actively being reworked by tidal currents and waves, resulting in net erosion on the subaqueous delta (Warrick et al., 2019) and a coarsening of grain size (Figures 3C, F, I). The ongoing changes at the delta and the magnitude of deposition and erosion will likely affect future habitat suitability for the species in the new deposits.
Based on our observations of geomorphic and ecological response after the Elwha dam removals, the major factors to consider when evaluating the potential effects from dam removals or large sediment fluxes from watersheds to the marine environment (e.g., landslides, wildfire debris flows) include the type, magnitude, and duration of the disturbance, character of the sediment, and coastal sediment transport processes that result in dispersal and sorting of sediment in the nearshore. In the case of the Elwha, ecological change was driven by two types of effects, sediment deposition of coarser grain sediment and increased turbidity from finer grain sediment. The duration of the disturbance depended on the type of effect. Sediment deposition had longer lasting effects on the ecosystem than increased fine suspended sediment, in part due to the more variable effects of suspended sediment in space and time. In addition, species likely had different sensitivities to stressor types depending on their life history, morphological structure, mobility, and habitat needs. The magnitude of effect on the Elwha ecosystem was likely a function of both the overall sediment flux from the reservoirs to the coast as well as the hydrodynamics of the Elwha nearshore. Coastal areas without strong tidal currents would likely be most affected by burial due to a lack of transport capacity in the system, particularly if the amount of sediment being delivered is greater than background conditions. While the exact trajectories of community response to a disturbance are difficult to predict, assessing these conditions can be used to inform the development of conceptual models of potential outcomes that can inform monitoring and modeling efforts before and after dam removal.
Data availability statement
The datasets presented in this study can be found in online repositories. The names of the repository/repositories and accession number(s) can be found below: Rubin, S.P., Elder, N.E., Miller, I.M., Beirne, M.M., and Foley, M.M., 2023, Data collected in 2008-2022 to assess nearshore subtidal community responses to increased sediment load during and after removal of the Elwha River dams, Washington State, USA: U.S. Geological Survey data release, https://doi.org/10.5066/P9NCE4FE.
Author contributions
SR, IM, JW and GG contributed to the study concept and design, SR, MF, IM, AS, JW, NE, MB and GG participated in data collection, and SR, MF, IM, AS, NE and HB in sample processing and data analysis. SR, MF, IM, AS, JW, and HB contributed to drafting and review of the submitted manuscript. All authors contributed to the article and approved the submitted version.
Funding
This work was funded by the U.S. Geological Survey (USGS), Washington Sea Grant and the Lower Elwha Klallam Tribe, with support provided through the USGS’s Coastal Habitats in Puget Sound Project, USGS Mendenhall Research Fellowship Program, USGS Coastal Marine Hazards and Resources Program and Ecosystems Mission Areas, and the U.S. Environmental Protection Agency (EPA)/Puget Sound Partnership Puget Sound Protection and Restoration Tribal Implementation Assistance Program (CFDA#66.121) and the Technical Investigations and Implementation Assistance Program (CFDA#66.123). Additional funding was provided by the Washington State legislature, through contract OTGP-2021-LEKT-00072 administered through the Washington Department of Ecology Shorelands and Environmental Assistance Program to implement the Puget Sound Kelp Conservation and Recovery Plan.
Acknowledgments
The authors wish to acknowledge and thank the contributions made by a variety of partners and collaborators over the years, including Reginald Reisenbichler and Jeff Duda for contributions to study initiation, and dive team leadership early in the study. Chris Clark, John Gresham, Mike Hayes, Marshal Hoy, Mike McHenry, Tessa Code, Rusty Rodriguez, and Lisa Wetzel participated in dive data collection or dive boat operation. Many members of the EPA Region 10 Dive Team participated in dive data collection. Kristen Omori, Oleksandr Stefankiv, and Katherine Vittum assisted with dive database creation and management. David Duggins, Wendel Raymond, and Sarah Traiger provided helpful comments on the manuscript. The US Coast Guard Air Station Port Angeles provided dock space and small vessel security and support. Any use of trade, firm, or product names is for descriptive purposes only and does not imply endorsement by the U.S. Government.
Conflict of interest
The authors declare that the research was conducted in the absence of any commercial or financial relationships that could be construed as a potential conflict of interest.
Publisher’s note
All claims expressed in this article are solely those of the authors and do not necessarily represent those of their affiliated organizations, or those of the publisher, the editors and the reviewers. Any product that may be evaluated in this article, or claim that may be made by its manufacturer, is not guaranteed or endorsed by the publisher.
Supplementary material
The Supplementary Material for this article can be found online at: https://www.frontiersin.org/articles/10.3389/fevo.2023.1233895/full#supplementary-material
References
Airoldi L. (1998). Roles of disturbance, sediment stress, and substratum retention on spatial dominance in algal turf. Ecology 79 (8), 2759–2770. doi: 10.1890/0012-9658(1998)079[2759:RODSSA]2.0.CO;2
Anderson M., Gorley R. N., Clarke K. (2008). PERMANOVA+ for primer: Guide to software and statistical methods (Plymouth: Primer-E).
Arkema K. K., Reed D. C., Schroeter S. C. (2009). Direct and indirect effects of giant kelp determine benthic community structure and dynamics. Ecology 90 (11), 3126–3137. doi: 10.1890/08-1213.1
Beisner B. E., Haydon D. T., Cuddington K. (2003). Alternative stable states in ecology. Front. Ecol. Environ. 1 (7), 376–382. doi: 10.1890/1540-9295(2003)001[0376:ASSIE]2.0.CO;2
Bellmore J. R., Pess G. R., Duda J. J., O’Connor J. E., East A. E., Foley M. M., et al. (2019). Conceptualizing ecological responses to dam removal: if you remove it, what’s to come? BioScience 69 (1), 26–39. doi: 10.1093/biosci/biy152
Bodkin J. L. (1988). Effects of kelp forest removal on associated fish assemblages in central California. J. Exp. Mar. Biol. Ecol. 117 (3), 227–238. doi: 10.1016/0022-0981(88)90059-7
Booth J. D. (2020). Reviewing the far-reaching ecological impacts of human-induced terrigenous sedimentation on shallow marine ecosystems in a northern-New Zealand embayment. New Z. J. Mar. Freshw. Res. 54 (4), 593–613. doi: 10.1080/00288330.2020.1738505
Cancel Villamil J. J., Locke S. A. (2022). Fish assemblage response to removal of a low-head dam in the lower reach of a tropical island river. Freshw. Biol. 67 (5), 926–937. doi: 10.1111/fwb.13893
Clarke K., Gorley R. (2015). PRIMER version 7: User manual/tutorial (Plymouth, United Kingdom: PRIMER-E), 192.
Clarke K., Gorley R. N., Somerfield P., Warwick R. (2014). Change in Marine Communities: An Approach to Statistical Analysis (Plymouth, United Kingdom: PRIMER-E).
Connell S. D., Russell B. D., Turner D. J., Shepherd S. A., Kildea T., Miller D., et al. (2008). Recovering a lost baseline: missing kelp forests from a metropolitan coast. Mar. Ecol. Prog. Ser. 360, 63–72. doi: 10.3354/meps07526
Crain C. M., Halpern B. S., Beck M. W., Kappel C. V. (2009). Understanding and managing human threats to the coastal marine environment. Ann. New York Acad. Sci. 1162 (1), 39–62. doi: 10.1111/j.1749-6632.2009.04496.x
Di Lorenzo E., Mantua N. (2016). Multi-year persistence of the 2014/15 North Pacific marine heatwave. Nat. Climate Change 6 (11), 1042–1047. doi: 10.1038/nclimate3082
Doyle M. W., Stanley E. H., Orr C. H., Selle A. R., Sethi S. A., Harbor J. M. (2005). Stream ecosystem response to small dam removal: Lessons from the Heartland. Geomorphology 71 (1–2), 227–244. doi: 10.1016/J.GEOMORPH.2004.04.011
Duggins D. O. (1983). Starfish predation and the creation of mosaic patterns in a kelp-dominated community. Ecology 64 (6), 1610–1619. doi: 10.2307/1937514
Dunton K. H., Weingartner T., Carmack E. C. (2006). The nearshore western Beaufort Sea ecosystem: Circulation and importance of terrestrial carbon in arctic coastal food webs. Prog. Oceanogr. 71 (2–4), 362–378. doi: 10.1016/J.POCEAN.2006.09.011
Eckman J. E., Duggins D. O. (1991). Life and death beneath macrophyte canopies: effects of understory kelps on growth rates and survival of marine, benthic suspension feeders. Oecologia 87, 473–487.
Eckman J. E., Duggins D. O., Sewell A. T. (1989). Ecology of under story kelp environments. i. effects of kelps on flow and particle transport near the bottom. J. Exp. Mar. Biol. Ecol. 129, 173–187.
Eidam E. F., Ogston A. S., Nittrouer C. A., Warrick J. A. (2016). Tidally dominated sediment dispersal offshore of a small mountainous river: Elwha River, Washington State. Continental Shelf Res. 116, 136–148. doi: 10.1016/J.CSR.2016.01.009
Erftemeijer P. L. A., Riegl B., Hoeksema B. W., Todd P. A. (2012). Environmental impacts of dredging and other sediment disturbances on corals: A review. Mar. pollut. Bull. 64 (9), 1737–1765. doi: 10.1016/J.MARPOLBUL.2012.05.008
Foley M. M., Bellmore J. R., O’Connor J. E., Duda J. J., East A. E., Grant G. E., et al. (2017a). Dam removal: listening. Water Resour. Res. 53 (7), 5229–5246. doi: 10.1002/2017WR020457
Foley M. M., Magilligan F. J., Torgersen C. E., Major J. J., Anderson C. W., Connolly P. J., et al. (2017b). Landscape context and the biophysical response of rivers to dam removal in the United States. PloS One 12 (7), e0180107. doi: 10.1371/journal.pone.0180107
Foley M. M., Warrick J. A. (2017). Ephemeral seafloor sedimentation during dam removal: Elwha River, Washington. Continental Shelf Res. 150, 36–47. doi: 10.1016/J.CSR.2017.09.005
Foley M. M., Warrick J. A., Ritchie A., Stevens A. W., Shafroth P. B., Duda J. J., et al. (2017c). Coastal habitat and biological community response to dam removal on the Elwha River. Ecol. Monogr. 87 (4), 552–577. doi: 10.1002/ecm.1268
Frick K. E., Kagley A. N., Fresh K. L., Samhouri J. F., Ward L. S., Stapleton J. T., et al. (2022). Spatiotemporal variation in distribution, size, and relative abundance within a salish sea nearshore forage fish community. Mar. Coast. Fish. 14 (2), e10202. doi: 10.1002/mcf2.10202
Fryirs K. A., Brierley G. J. (2016). Assessing the geomorphic recovery potential of rivers: forecasting future trajectories of adjustment for use in management. Wiley Interdiscip. Reviews: Water 3 (5), 727–748.
Fuller I. C., Gilvear D. J., Thoms M. C., Death R. G. (2019). Framing resilience for river geomorphology: Reinventing the wheel? River Res. Appl. 35 (2), 91–106. doi: 10.1002/rra.3384
Gelfenbaum G., Stevens A. W., Miller I., Warrick J. A., Ogston A. S., Eidam E. (2015). Large-scale dam removal on the Elwha River, Washington, USA: Coastal geomorphic change. Geomorphology 246, 649–668. doi: 10.1016/J.GEOMORPH.2015.01.002
Glover H. E., Ogston A. S., Miller I. M., Eidam E. F., Rubin S. P., Berry H. D. (2019). Impacts of suspended sediment on nearshore benthic light availability following dam removal in a small mountainous river: in situ observations and statistical modeling. Estuaries Coasts 42 (7), 1804–1820. doi: 10.1007/s12237-019-00602-5
Gunderson L. H. (2000). Ecological resilience–in theory and application. Annu. Rev. Ecol. Sys. 31, 425–439. doi: 10.1146/annurev.ecolsys.31.1.425
Hall S. J. (1993). Physical disturbance and marine benthic communities: Life in unconsolidated sediments. Oceanogr. Mar. Biol. 32, 178–239.
Hamilton J., Konar B. (2007). Implications of substrate complexity and kelp variability for south-central alaskan nearshore fish communities. Fishery Bull. 105 (2), 189–196.
Harvell C. D., Montecino-Latorre D., Caldwell J. M., Burt J. M., Bosley K., Keller A., et al. (2023). Disease epidemic and a marine heat wave are associated with the continental-scale collapse of a pivotal predator (Pycnopodia helianthoides). Sci. Adv. 5 (1), eaau7042. doi: 10.1126/sciadv.aau7042
Holling C. S. (1973). Resilience and stability of ecological systems. Annu. Rev. Ecol. Sys. 4 (1), 1–23. doi: 10.1146/annurev.es.04.110173.000245
Knowlton N. (2004). Multiple “stable” states and the conservation of marine ecosystems. Prog. Oceanogr. 60 (2–4), 387–396. doi: 10.1016/J.POCEAN.2004.02.011
Lohrer A. M., Thrush S. F., Hewitt J. E., Berkenbusch K., Ahrens M., Cummings V. J. (2004). Response of marine macrobenthic communities to thin terrigenous deposits. Mar. Ecol. Prog. Ser. 273, 121–138. doi: 10.3354/meps273121
Lohrer A. M., Thrush S. F., Lundquist C. J., Vopel K., Hewitt J. E., Nicholls P. E. (2006). Response of two contrasting community types. Mar. Ecol. Prog. Ser. 307, 115–125. doi: 10.3354/meps307115
Magirl C. S., Hilldale R. C., Curran C. A., Duda J. J., Straub T. D., DOmanski M., et al. (2015). Large-scale dam removal on the Elwha River, Washington, USA: Fluvial sediment load. Geomorphology 246, 669–686. doi: 10.1016/J.GEOMORPH.2014.12.032
Major J. J., East A. E., O’Connor J. E., Grant G. E., Wilcox A. C., Magirl C. S., et al. (2017). Geomorphic responses to dam removal in the United States – a two-decade perspective. Gravel-Bed Rivers, 355–383. doi: 10.1002/9781118971437.ch13
McArdle B. H., Anderson M. J. (2001). Fitting multivariate models to community data: A comment on distance-based redundancy analysis. Ecology 82 (1), 290–297. doi: 10.1890/0012-9658(2001)082[0290:FMMTCD]2.0.CO;2
McGovern M., Poste A. E., Oug E., Renaud P. E., Trannum H. C. (2020). Riverine impacts on benthic biodiversity and functional traits: A comparison of two sub-Arctic fjords. Estuarine Coast. Shelf Sci. 240, 106774. doi: 10.1016/J.ECSS.2020.106774
Miller D. C., Muir C. L., Hauser O. A. (2002). Detrimental effects of sedimentation on marine benthos: what can be learned from natural processes and rates? Ecol. Eng. 19 (3), 211–232. doi: 10.1016/S0925-8574(02)00081-2
Miller I. M., Warrick J. A., Morgan C. (2011). Observations of coarse sediment movements on the mixed beach of the Elwha Delta, Washington. Mar. Geol. 282 (3–4), 201–214. doi: 10.1016/J.MARGEO.2011.02.012
Montecino-Latorre D., Eisenlord M. E., Turner M., Yoshioka R., Harvell C. D., Pattengill-Semmens C. V., et al. (2016). Devastating transboundary impacts of sea star wasting disease on subtidal asteroids. PloS One 11 (10), e0163190. doi: 10.1371/journal.pone.0163190
Morley S. A., Foley M. M., Duda J. J., Beirne M. M., Paradis R. L., Johnson R. C., et al. (2020). Shifting food web structure during dam removal—Disturbance and recovery during a major restoration action. PloS One 15 (9), e0239198. doi: 10.1371/journal.pone.0239198
Norderhaug K. M., Filbee-Dexter K., Freitas C., Birkely S. R., Christensen L., Mellerud I., et al. (2020). Ecosystem-level effects of large-scale disturbance in kelp forests. Mar. Ecol. Prog. Ser. 656, 163–180. doi: 10.3354/meps13426
O’Connor J. E., Duda J. J., Grant G. E. (2015). 1000 dams down and counting. Science 348 (6234), 496–497. doi: 10.1126/science.aaa9204
Paine R. T., Levin S. A. (1981). Intertidal landscapes: Disturbance and the dynamics of pattern. Ecol. Monogr. 51 (2), 145–178. doi: 10.2307/2937261
Palumbi S. R., McLeod K. L., Grünbaum D. (2008). Ecosystems in action: lessons from marine ecology about recovery, resistance, and reversibility. BioScience 58 (1), 33–42. doi: 10.1641/B580108
Pfister C. A., Berry H. D., Mumford T. (2018). The dynamics of Kelp Forests in the Northeast Pacific Ocean and the relationship with environmental drivers. J. Ecol. 106 (4), 1520–1533. doi: 10.1111/1365-2745.12908
Phillips J. D., Van Dyke C. (2016). Principles of geomorphic disturbance and recovery in response to storms. Earth Surface Processes Landforms 41 (7), 971–979. doi: 10.1002/esp.3912
Picard M. M. M., Johnson L. E., Côté I. M. (2022). Effects of sediment on spore performance as a potential constraint on kelp distribution. Mar. pollut. Bull. 185, 114336. doi: 10.1016/J.MARPOLBUL.2022.114336
Pinheiro J., Bates D., R Core Team (2023). nlme: Linear and nonlinear mixed effects models. R package version 3.1-162. https://CRAN.R-project.org/package=nlme.
Ralston D. K., Yellen B., Woodruff J. D. (2021). Watershed suspended sediment supply and potential impacts of dam removals for an estuary. Estuaries Coasts 44 (5), 1195–1215. doi: 10.1007/s12237-020-00873-3
Rangel-Buitrago N., Rizzo A., Neal W. J., Mastronuzzi G. (2023). Sediment pollution in coastal and marine environments. Mar. pollut. Bull. 192, 115023. doi: 10.1016/J.MARPOLBUL.2023.115023
R Core Team (2022). R: A language and environment for statistical computing. R Foundation for Statistical Computing, Vienna, Austria. https://www.R-project.org.
Ritchie A. C., Warrick J. A., East A. E., Magirl C. S., Stevens A. W., Bountry J. A., et al. (2018). Morphodynamic evolution following sediment release from the world’s largest dam removal. Sci. Rep. 8 (1), 13279. doi: 10.1038/s41598-018-30817-8
Rubin S. P., Elder N. E., Miller I. M., Beirne M. M., Foley M. M. (2023). Data collected in 2008-2022 to assess nearshore subtidal community responses to increased sediment load during and after removal of the Elwha River dams. Washington State, USA: U.S. Geological Survey data release. doi: 10.5066/P9NCE4FE
Rubin S. P., Miller I. M., Foley M. M., Berry H. D., Duda J. J., Hudson B., et al. (2017). Increased sediment load during a large-scale dam removal changes nearshore subtidal communities. PloS One 12 (12), e0187742. doi: 10.1371/journal.pone.0187742
Schröder A., Persson L., De Roos A. M. (2005). Direct experimental evidence for alternative stable states: a review. Oikos 110 (1), 3–19. doi: 10.1111/j.0030-1299.2005.13962.x
Schultz J., Cloutier R., Côté I. (2016). Evidence for a trophic cascade on rocky reefs following sea star mass mortality in British Columbia. PeerJ 4, e1980. doi: 10.7717/peerj.1980
Shaffer J. A., Juanes F., Quinn T. P., Parks D., McBride T., Michel J., et al. (2017). Nearshore fish community responses to large scale dam removal: implications for watershed restoration and fish management. Aquat. Sci. 79 (3), 643–660. doi: 10.1007/s00027-017-0526-3
Smith J. G., Tomoleoni J., Staedler M., Lyon S., Fujii J., Tinker M. T. (2021). Behavioral responses across a mosaic of ecosystem states restructure a sea otter–urchin trophic cascade. Proc. Natl. Acad. Sci. 118, e2012493118.
Somerfield P. J., Clarke K. R. (2013). Inverse analysis in non-parametric multivariate analyses: distinguishing groups of associated species which covary coherently across samples. J. Exp. Mar. Biol. Ecol. 449, 261–273. doi: 10.1016/J.JEMBE.2013.10.002
Sousa W. P. (1984). The role of disturbance in natural communities. Annu. Rev. Ecol. Sys. 15 (1), 353–391. doi: 10.1146/annurev.es.15.110184.002033
Stanley E. H., Doyle M. W. (2003). Trading off: the ecological effects of dam removal. Front. Ecol. Environ. 1 (1), 15–22. doi: 10.1890/1540-9295(2003)001[0015:TOTEEO]2.0.CO;2
Stevens A. W., Gelfenbaum G., Warrick J. A., Miller I. M., Weiner H. M. (2017). Bathymetry, topography, and sediment grain-size data from the Elwha River delta, Washington (U.S. Geological Survey data release). doi: 10.5066/F72N51GC
Tolimieri N., Shelton A. O., Samhouri J. F., Harvey C. J., Feist B. E., Williams G. D., et al. (2023). Changes in kelp forest communities off Washington, USA, during and after the 2014-2016 marine heatwave and sea star wasting syndrome. Mar. Ecol. Prog. Ser. 703, 47–66. doi: 10.3354/meps14220
Traiger S. B., Konar B. (2018). Mature and developing kelp bed community composition in a glacial estuary. J. Exp. Mar. Biol. Ecol. 501, 26–35. doi: 10.1016/J.JEMBE.2017.12.016
Tullos D. D., Finn D. S., Walter C. (2014). Geomorphic and ecological disturbance and recovery from two small dams and their removal. PloS One 9 (9), e108091. doi: 10.1371/journal.pone.0108091
Warrick J. A., Cochrane G. R., Sagy Y., Gelfenbaum G. (2008). Nearshore substrate and morphology offshore of the Elwha River, Washington. Northwest Sci. 82 (sp1), 153–163. doi: 10.3955/0029-344X-82.S.I.153
Warrick J. A., Duda J. J., Magirl C. S., Curran C. A. (2012). River turbidity and sediment loads during dam removal. Eos Trans. Am. Geophys. Union 93 (43), 425–426. doi: 10.1029/2012EO430002
Warrick J. A., Stevens A. W. (2011). A buoyant plume adjacent to a headland—Observations of the Elwha River plume. Continental Shelf Res. 31 (2), 85–97. doi: 10.1016/J.CSR.2010.11.007
Warrick J. A., Stevens A. W., Miller I. M., Harrison S. R., Ritchie A. C., Gelfenbaum G. (2019). World’s largest dam removal reverses coastal erosion. Sci. Rep. 9 (1), 13968. doi: 10.1038/s41598-019-50387-7
Watanabe H., Ito M., Matsumoto A., Arakawa H. (2016). Effects of sediment influx on the settlement and survival of canopy-forming macrophytes. Sci. Rep. 6 (1), 18677. doi: 10.1038/srep18677
Watson J., Estes J. A. (2011). Stability, resilience, and phase shifts in rocky subtidal communities along the west coast of Vancouver Island, Canada. Ecol. Monogr. 81 (2), 215–239. doi: 10.1890/10-0262.1
Wood S. N. (2017). Generalized Additive Models: An Introduction with R. 2nd ed (Boca Raton, Florida: Chapman and Hall/CRC).
Keywords: dam removal, nearshore, Elwha, sediment, disturbance, recovery
Citation: Rubin SP, Foley MM, Miller IM, Stevens AW, Warrick JA, Berry HD, Elder NE, Beirne MM and Gelfenbaum G (2023) Nearshore subtidal community response during and after sediment disturbance associated with dam removal. Front. Ecol. Evol. 11:1233895. doi: 10.3389/fevo.2023.1233895
Received: 02 June 2023; Accepted: 21 July 2023;
Published: 10 August 2023.
Edited by:
Laura Soissons, INRAE Bretagne Normandie, FranceReviewed by:
Sarah Traiger, U.S. Geological Survey, Alaska, United StatesDavid Duggins, Independent Researcher, Friday Harbor, WA, United States
Copyright © 2023 Rubin, Foley, Miller, Stevens, Warrick, Berry, Elder, Beirne and Gelfenbaum. This is an open-access article distributed under the terms of the Creative Commons Attribution License (CC BY). The use, distribution or reproduction in other forums is permitted, provided the original author(s) and the copyright owner(s) are credited and that the original publication in this journal is cited, in accordance with accepted academic practice. No use, distribution or reproduction is permitted which does not comply with these terms.
*Correspondence: Stephen P. Rubin, srubin@usgs.gov
†These authors have contributed equally to this work and share first authorship