- 1Department of Palynology and Climate Dynamics, Albrecht-von-Haller-Institute for Plant Sciences, University of Göttingen, Göttingen, Germany
- 2College of Life and Environmental Sciences, University of Exeter, Exeter, United Kingdom
- 3Department of Soil Science, University of Jambi (UNJA), Jambi, Indonesia
- 4Department of Soil Science and Land Resource, Bogor Agriculture University (IPB), Bogor, Indonesia
Riparian wetland ecosystems provide important ecological services but are also vulnerable to climate change and human activities. To understand the influence of natural factors (e.g. climate change, flooding, drought) and human activities (e.g. agriculture) as well as to support management strategies, reconstructions of past vegetation and environmental changes are needed. To achieve this, we conducted a multi-proxy paleoecological analysis, including pollen and spores, macro-charcoal and radiocarbon dating, on a sediment core taken from a riparian area in the Harapan forest of Sumatra. Three distinct periods were identified: i) AD 1100 – 1400: Upland and swamp forest with riparian and herbaceous vegetation, possibly part of a riparian buffer zone (e.g. riverbank), was present in the study area under a stronger dry season regime; ii) AD 1400 – 1870: freshwater swamps expanded to the study site; iii) later, from AD 1870 to present, upland forests dominated in the study area with a strong dry season. The presence of cereal cultivation from AD 1300 – 1450, and oil palm (Elaeis guineensis) since the mid-19th century AD indicates the presence of small-scale agriculture in the study area. This study of riparian vegetation dynamics and environmental changes in the Harapan forest of Sumatra shows the development from a riparian forest to a freshwater swamp and upland forest under the impact of climate change and human activities.
1 Introduction
Riparian wetlands are wetland ecosystems located in floodplains next to rivers or streams, which are periodically flooded by the adjacent waterways. This unique surface flow is specific to riparian wetlands, making them distinct from other types of wetland (Mitsch and Gosselink, 2007). Riparian wetlands are important for filtering pollutants, providing habitats for wildlife, and controlling floods (Lowrance, 1998; National Research Council, 2002). Riparian vegetation provides important sources of forage for livestock grazing (Kauffman and Krueger, 1984). The shade of riparian vegetation and wood fragments generate ideal living conditions for fish (Singh et al., 2021). The vegetation also plays a vital role in protecting and preventing shoreline erosion caused by strong flooding and promoting landform stability (Capon, 2020). Riparian wetland areas also play an important role in carbon storage (Hennings et al., 2021) However, today, human activities and climate change have made riparian wetlands among the most threatened ecosystems on Earth (Tockner and Stanford, 2002; Schneider et al., 2017). Owing to their location with frequently high settlement densities, riparian wetlands are subject to a high degree of land- use change and modification (e.g. dam construction, transportation, industrial and urban development, agriculture; National Research Council, 2002; Mitsch and Gosselink, 2007; Fitri et al., 2018). It is estimated that up to 90% of North American and European floodplains are ecologically dysfunctional due to human activities, and in developing countries, the degradation and disappearance of riparian ecosystems is accelerating (Tockner and Stanford, 2002). In Asia, where approximately 1.5 billion people live in floodplains (Devitt et al., 2023), more than 5000 km2 of wetland area, including riparian wetlands, are lost annually due to the expansion of agricultural activities, rapid urbanization and irrigation (McAllister et al., 2001; Dudgeon, 2009). Over the past few decades, the construction of dams in Asia and Africa has had a profound impact on the morphologies of rivers and the ecosystems of riparian wetlands (Dudgeon, 2009; Khan et al., 2014; Schneider et al., 2017). This extensive development has resulted in a significant decline, estimated at around 21%, in the number of remaining free-flowing rivers (Zarfl et al., 2015). Dams obstruct water flow, impede sediment movement, and disrupt the natural flow regime, affecting floods, low flows, and overall river dynamics (Poff et al., 1997; Cui et al., 2020). In addition, climate change, e.g. changes in temperature, hydrological regime, droughts, and fires also impact the morphology of rivers and their riparian ecosystems (Tockner and Stanford, 2002; Dwire et al., 2018; Shrestha et al., 2020).
Indonesia has one of the world’s most extensive riparian wetland areas, with more than 5700 rivers, 131 official river basins and approximately 8000 watersheds (Asian Development Bank, 2016). Indonesia’s riparian wetlands are a biodiversity hotspot, supporting a variety of flora and fauna, including iconic megafaunas such as the Sumatran rhinoceros, orangutans, tigers, and elephants (Dudgeon, 2000a; Dudgeon, 2000b; von Rintelen et al., 2017). The mix of terrestrial and aquatic features found in riparian zones creates a unique environment that supports a variety of specialized species, many of which are adapted to living in both wet and dry conditions. The results of an experimental study conducted in Jambi, Sumatra, comparing amphibians in different habitats, revealed that rare amphibians were much more abundant in riparian forests than in upland forests, oil palm and rubber plantations in riparian areas (Paoletti et al., 2018).
The targeting of rivers and modification of riparian wetlands by humans has a long history in Indonesia. Throughout Indonesian history, riparian wetland areas were often chosen to place the capitals of kingdoms, and rivers were landmarks dividing the territories, for example, the Malayu Kingdom and the Batanghari River, Srivijaya and the Musi River, the Langkat Kingdom and the Langkat River, the Siak Kingdom and the downstream section of the Siak River, the Deli Kingdom which was close to the Deli River, and the Serdang Kingdom with the Serdang River. Many other kingdoms are also close to, and bounded by rivers (Sutihat, 2014). Nowadays, Indonesia ranks as the fifth most populous country in the world (Nitisastro, 2006). The high population density leading to the growth of urban areas and changing agricultural practices in Indonesia has put more pressure on the already vulnerable riparian ecosystems. Many riparian wetlands have been converted into settlements and farmland (Fitri et al., 2018).
In 2012, Indonesia is the country with the highest rate of deforestation in the world (Margono et al., 2014). In particular, Sumatra island, which is experiencing the fastest loss of primary rainforest cover in Indonesia, is undergoing a marked conversion of rainforest to large-scale agricultural use (Laumonier et al., 2010; Miettinen et al., 2011; Margono et al., 2014). Over past decades, the rapid conversion of tropical lowland rainforests and extensive traditional production systems into rubber and oil palm plantations has been particularly evident in Sumatra’s Jambi Province (Laumonier et al., 2010). Rapid land-use changes for settlements and agriculture in riparian wetland areas in Jambi have already had long-term environmental consequences. For example, an experimental study in Jambi Province, Sumatra, showed that the land-use change from forests to monoculture plantation in the Tembesi watershed have caused increases in flood frequencies and intensity (Merten et al., 2020). In addition, the establishment of monoculture oil palm and rubber plantations in riparian wetlands in Jambi has reduced soil carbon content compared to natural riparian areas (Hennings et al., 2021).
Riparian wetlands, which are complex and highly dynamic ecosystems (Wantzen et al., 2008), are disappearing at an accelerating rate globally (Schneider et al., 2017), making their preservation critical for human well-being (Wantzen et al., 2008). Understanding the effects of natural factors (e.g. flooding, drought) and human activities (e.g. agriculture, water diversion and socioeconomically local communities) on riparian wetlands is essential for developing management strategies. For instance, understanding ecosystem recovery times after disturbance could help with allocating sufficient restoration periods (Hapsari et al., 2018). However, our understanding of riparian wetlands is still limited, and little attention has been paid to these remarkable ecosystems, particularly in the tropics (Wantzen et al., 2008; Junk et al., 2022; Rodríguez-González et al., 2022). In general, riparian wetland ecosystems are challenging to study due to their intricate environmental influences, unpredictable flooding, and seasonal processes (Lakitan et al., 2018b; Rodríguez-González et al., 2022). This difficulty is further hindered by the challenging nature of riparian wetland study sites in tropical areas, including the presence of poisonous snakes, mosquitoes, and dense thorny vegetation (Wantzen et al., 2008).
Harapan forest in Jambi Province, with its dominant river system and indigenous population that have lived in this forest for hundreds of years offers a unique opportunity to study riparian vegetation dynamics and how land uses have been practiced by the indigenous people. Therefore, aiming to understand the effects of climate and human activities on the riparian wetlands, we conducted a paleoecological study of the Harapan forest using palynological and charcoal analyses. The main aim was to understand: 1) how the riparian vegetation changed in the Harapan forest over time and 2) the possible factors (climate and humans) that may have influenced vegetation in the riparian zones.
2 Study area
The Harapan forest is located about 60 km southwest of Jambi city (Figure 1B). The average annual temperature near the study site is about 26°C, and the mean rainfall is about 2235 mm (Sultan Thaha Airport climatic station, Jambi; Drescher et al., 2016). The climate is influenced by El Niño–Southern Oscillation (ENSO) and the Indian Ocean Dipole (IOD) (Saji et al., 1999; Aldrian and Susanto, 2003). When the warm (cold) phase of ENSO dominates, Sumatra receives less (higher) rainfall than in other years (Philander, 1990; Cane, 2005). Resembling ENSO, the IOD is a climate phenomenon that occurs in the Indian Ocean, characterized by a fluctuation of sea surface temperature and wind patterns across the region. It also has two phases, positive and negative. During a negative (positive) phase, the western Indian Ocean is colder (warmer) than usual, while the eastern part of the ocean is warmer (colder) than usual. This creates a gradient of temperature across the ocean that results in increased (decreased) rainfall over western Indonesia (Saji et al., 1999; Iskandar et al., 2022).
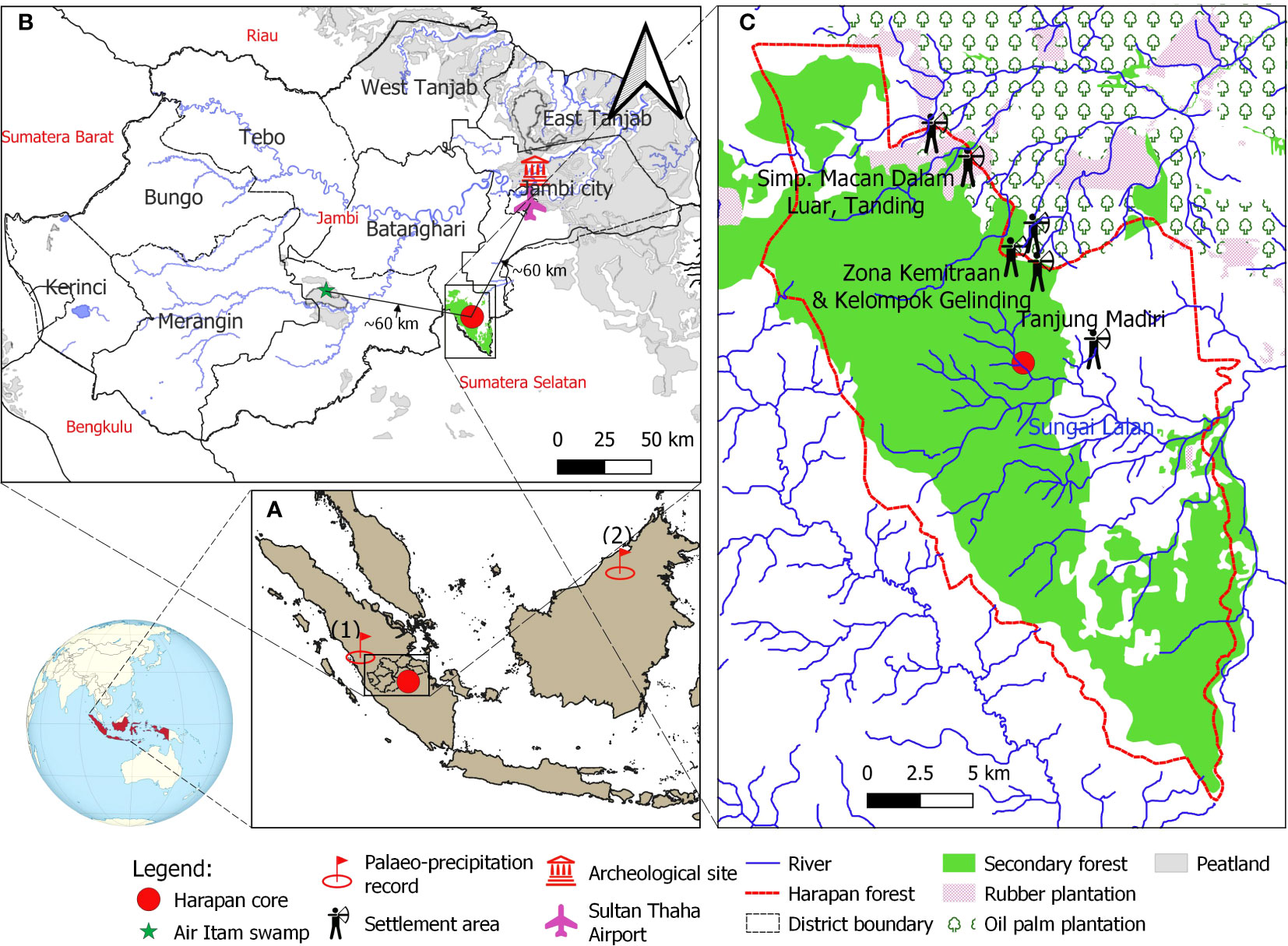
Figure 1 (A) Map of the study site and the site of paleo-precipitation record from Tangga Cave (1, Wurtzel et al., 2018) and Bukit Assam Cave, Gunung Buda, Sarawak (2; Chen et al., 2016); (B) Climatic measurement tower at Sultan Thaha Airport in Jambi City (Drescher et al., 2016) and Air Itam paleo-vegetation study (green star) near the study site (Biagioni et al., 2015); (C) The core location is indicated by a red circle, the land-use statement in Harapan forest and adjacent areas in 2013 is indicated by Hein and Faust (2013). The locations of the indigenous Batin Sembilan’s settlement areas are based on the survey of Hauser-Schäublin and Steinebach (2014); Widianingsih et al. (2016); Widianingsih et al. (2019) and Hein (2019). The map was created in QGIS version 3.16.7-Hannover.
Part of the Harapan forest is a watershed of many river systems and their tributaries. Some pass through the Harapan forest, such as Sungai Kapas, Meranti River, Sungai Lalan, Masse River, Sungai Telang and Sungai Kandang. The coring site is about 50 m a.s.l. and located 100 m distant from the nearest large river, the Lalan, and about 200 m from the upland area. The Harapan forest is generally dominated by the Dipterocarpaceae tree family and riparian communities, for example, Shorea macroptera, Intsia palembanica, Eusideroxylon zwageri, Calamus (rattan), Glochidion, Semecarpus (Briggs et al., 2012; Harrison and Swinfield, 2015), which were regrowth following the intensive legal logging concession during the 1960s – 1970s (Lambertini, 2008). Located in one of the global biodiversity hotspots, this forest hosts ca. 133 critically endangered species on IUCN Red List, including the sumatran tiger (Panthera tigris sumatrae), orangutan (Pongo abelii), the storm’s stork (Ciconia stormi), the sumatran elephant (Elephas maximus sumatranus), the helmeted hornbill (Rhinoplax vigil), and the rhinoceros hornbill (Buceros rhinoceros) (Jain et al., 2018; Utomo and Walsh, 2018). Since AD 2008, the Harapan forest has been restored and managed by PT REKI – Restorasi Ekosistem Indonesia, with a license for up to 95 years to restore ecosystems/conservation concession (Ministerial Regulation No. P. 61/Menhut-II/2008, Ministry of Forestry). This is the first rainforest ecosystem restoration project in Indonesia (Harrison and Swinfield, 2015).
Harapan forest is well-known as home to the Batin Sembilan indigenous people practicing shifting cultivation (Forbes, 1885; Steinebach, 2013; Hein et al., 2015). Near the coring site, there are villages of the Batin Sembilan people, such as Tanjung Mandiri, Zona Kemitraan, Kel Gelinding, Macan Dalam, Luar, and Tangding (Figure 1C). They are thought to have originated from proto-Malay people who have fled into the forest since the 7th century to avoid the wars between rulers of Jambi and adjacent kingdoms (Anderbeck, 2008; Steinebach, 2013, https://hutanharapan.id/batin-sembilan/). According to historical records, the Batin Sembilan people are semi-nomadic. Their livelihood mainly relies on fishing, logging, harvesting non-timber forest products (e.g. jernang, dammar (resin), honey, rattan, bamboo, forest fruits) and practising agriculture (e.g. rice, sugarcane and vegetables; Wardah, 2013; Hein, 2019; Wulandari, 2021).
3 Materials and methods
3.1 Core sampling, sediment description and dating
A 150 cm-long core from a riparian area (2.173186°S 103.365748°E, ca. 50 m a.s.l.) of the Harapan forest (HRF) in Jambi province was collected in the summer of 2017 using a Russian peat corer (Jowsey, 1966). The lowermost part of the core from 150 – 100 cm consists of white and light-brownish sand and sandy clay with wood remains. The sediments between 100 – 40 cm consist of peaty clay and woody remains. The uppermost part, 40 – 0 cm, is composed of brown-humified sandy mud with a high proportion of woody and plant remains. Sedlog (Zervas et al., 2009) was used to create the graphic sediment profile (Figure 2). Four samples consisting of organic bulk sediment and fine plant material were sent to Poznan Radiocarbon Laboratory in Poland for radiocarbon dating (Table 1). Ages were calibrated using the southern Hemisphere SHCal13.14C calibration curve (Hogg et al., 2013), and the age-depth model was built and illustrated using Clam 2.3.2 (Blaauw, 2010) in R (R Core Team, 2018).
3.2 Pollen and spore analysis
Palynological analyses were conducted to reconstruct past vegetation in the Harapan forest. A total of 33 samples of 0.5 cm3 from the 150 cm-long core were chosen for pollen and spore analysis at 4 cm interval; each sample was processed using the standard pollen analytical method of Faegri and Iversen (1986). Lycopodium clavatum spores were added to each sample as a “spike” to estimate the concentration of pollen and spores (Stockmarr, 1971). The remaining organic residue was sieved at 120 µm mesh and mounted on a slide in glycerin.
Pollen and spores were counted to a minimum of 300 pollen grains for each sample under a light microscope at a magnification of 400x. The total number of pollen grains counted (100%) was used to calculate the pollen percentages, whilst the spore percentages were calculated based on the total number of pollen and spore grains counted. The pollen and spore identification was based on the modern tropical pollen reference collections of the Department of Palynology and Climate Dynamics, University of Göttingen (available at https://www.uni-goettingen.de/de/97306.html) and online databases of the Australasian Pollen and Spore Atlas (APSA) (available at https://apsa.anu.edu.au). Some taxa that have similar morphologies and are difficult to separate were grouped together, such as Moraceae/Urticaceae, excluding Ficus, Lithocarpus/Castanopsis, Macaranga/Mallotus. In accordance with the literature of vegetation distribution and ecology in Indonesia and adjacent areas (e.g. Whitmore and Tantra, 1986; Laumonier, 1997; Whitten and Damanik, 2000; Morley, 2013; Biagioni et al., 2015; Cole et al., 2015; Prosea collection: https://uses.plantnet-project.org/en/Category : PROSEA and Plant of SE Asia: https://asianplant.net), the pollen and spore taxa were grouped into six main categories: i) Upland forest (UF), representing pollen from plants that are restricted to, or commonly found in, areas less influenced by flooding; ii) riparian forest (RF), representing pollen from plants that are restricted to or commonly found in riverine, floodplain areas, and open vegetation (OV), OV representing pollen produced by herbaceous, pioneering plants found in open areas along the rivers or that tend to grow after disturbance; iii) Swamp forest (SF) representing the pollen of plants commonly found in swampy environments; iv) cultivated plants (e.g. oil palms, cereals); v) ubiquitous vegetation (UV), representing taxa that can grow in a wide range of habitats such as upland and floodplain vegetation; and vi) ferns. Some taxa typical of the UF also occur in SF and RF and vice versa, e.g. Dipterocarpaceae, Elaeocarpaceae, Macaranga/Mallotus. However, the PCA analysis can identify pollen taxa related to particular habitats as well as those related to environmental changes. Furthermore, in the Sundaland region (currently the islands of Sumatra, Borneo, Java and Peninsular Malaysia), Poaceae pollen grains with a paired threshold of 39.5 µm of grain diameter and 8.5 µm of annulus diameter can be used to detect common/major cereal pollen with 98% specificity (Hapsari and Ballauff, 2022). Therefore, in our study, Poaceae pollen grains with diameters <40 µm and >40 µm, but with an annulus diameter <8.5 µm were considered to be wild grasses. Poaceae pollen with a pair of grain diameters ≥40 µm and an annulus diameter ≥8.5 µm were counted separately as potential cereal cultivation. Pollen zonation is identified based on cluster analysis of terrestrial pollen using CONISS (Grimm, 1987). The StrataBugs®v.3 (Stratadata, 2014) was used to construct the pollen diagram, species richness and Shannon diversity index (Shannon, 1948). Only taxa showing significant changes in proportion and specific ecological relationships (UF, SF, RF, OV, and ferns) are displayed on the pollen diagram.
3.3 Macro-charcoal analysis
Macro-charcoal (>125 µm) analysis was performed to reconstruct local fire regimes based on the method of Stevenson and Haberle (2005). One cm3 subsamples at one cm contiguous intervals along the core (total 147 samples) were taken and soaked in sodium hexametaphosphate solution (5%, overnight) to help disaggregate the samples and separate charcoal particles from other material. The sample was then bleached in a Javel water solution (2% sodium hypochlorite) to increase the contrast between charcoal and dark organic matter. The residue was sieved using 125µm diameter mesh to remove smaller particles for a more local charcoal signal (Clark et al., 1998; Carcaillet et al., 2001). Large charcoal particles (>125µm) were counted under a stereomicroscope and analyzed with CharAnalysis software (Higuera et al., 2009). Charcoal accumulation rates are expressed as number of particles cm−2 yr−1.
3.4 Statistical analysis
Unconstrained ordination principal component analysis (PCA) and detrended correspondence analysis (DCA) were carried out using CANOCO 5 (Simpson and Birks, 2012; Šmilauer and Lepš, 2014) to display the frequencies of the original pollen and spore percentage data on spatial coordinate axes. All the pollen was square root transformed to minimize the effects of large and small values in the record. The results from DCA and PCA were expected to detect the dominant environmental factors driving the vegetation changes over time.
4 Results
4.1 Age-depth modelling
The age-depth model indicates that the HRF recorded the last 1000 years. The depth versus age relationship (Figure 2) suggests irregular sediment accumulation through time. From ca. AD 1100 to 1400 (150 to 95 cm) the core records an average sediment accumulation rate of 0.18 cm yr−1. From ca. AD 1400 – 1750 (95 – 75 cm) it indicates an average sediment accumulation rate of 0.05 cm yr−1. Later, from the ca. AD 1750 – present (75 – 0 cm) interval, the sediment accumulation rate is around 0.29 cm yr−1.
4.2 Palynological results
In total, 127 pollen and 12 spore taxa were identified. The pollen and spores have good preservation and high concentrations along the core (average 69,000 grains cm−3). The bottom part of the core (150 – 95 cm) records the highest pollen concentration (average 74,000 grains cm−3), which then decreases in the middle part (95 – 35 cm; average 64,000 grains cm−3) and slightly increases again at the top of the sediment core (35 – 0 cm; average 70,000 grains cm−3). Based on cluster analysis, the pollen diagram of the HRF core is divided into three zones according to the following main changes in pollen composition (Figure 3; all the following % values are averages):
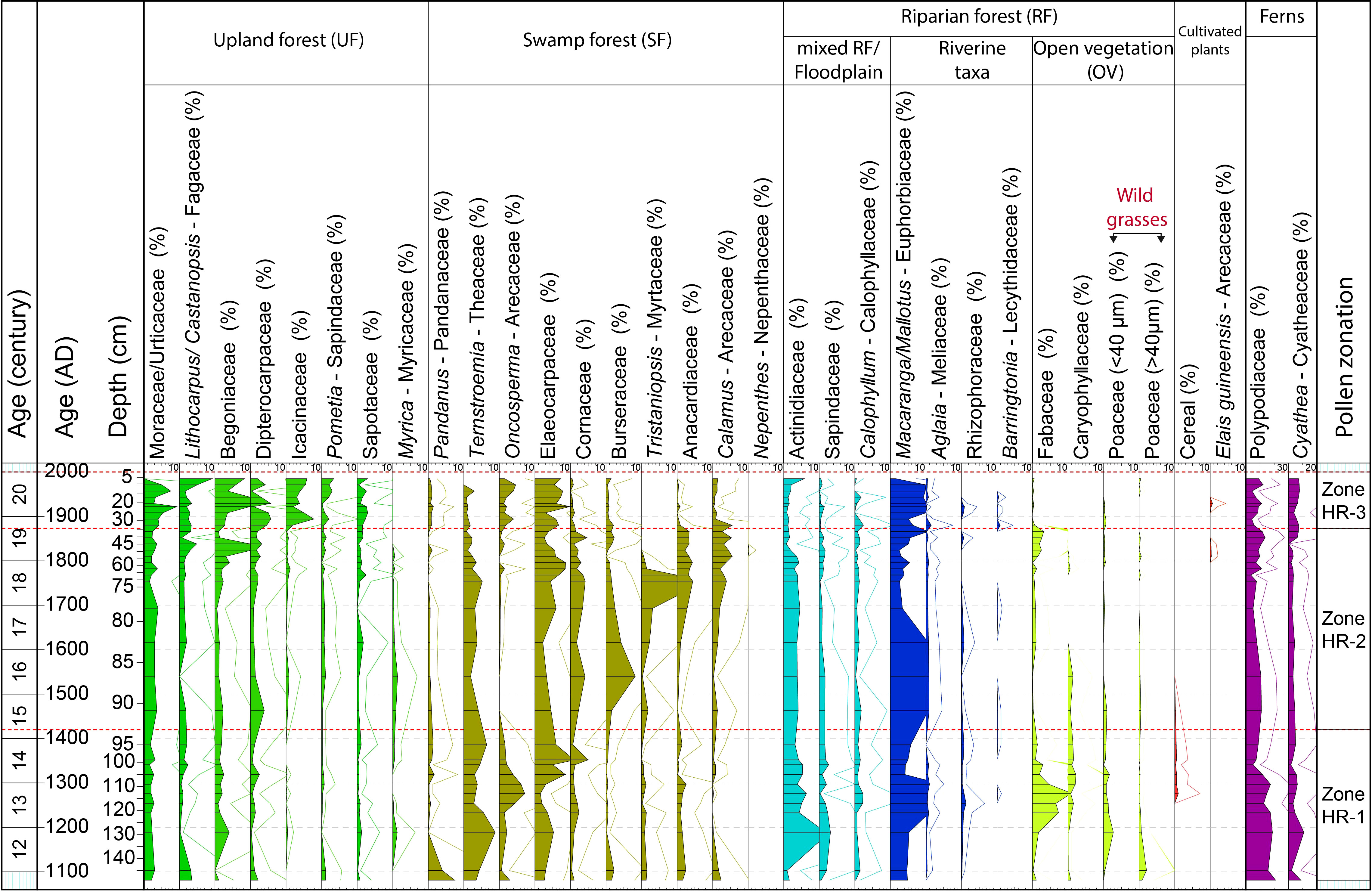
Figure 3 Pollen and spore diagram of the HRF core with age scales, representative taxa of each group (%), and pollen zones.
4.2.1 Zone HR-1 (150 – 95 cm, AD 1100 – 1400)
The UF taxa account for 20% of all pollen, mainly represented by Moraceae/Urticaceae (2%), Begoniaceae (2%), Lithocarpus/Castanopsis (1%) and Dipterocarpaceae (1%). The SF pollen taxa are dominant (30%), mainly represented by Elaeocarpaceae (5%), Ternstroemia (5%), Oncosperma (3%), and Pandanus (2%), Burseraceae (<1%). The RF accounts for 41%, characterized by Macaranga/Mallotus (8%), Actinidiaceae (5%), Fabaceae (4%), Poaceae (wild grasses, 1%). Cereal pollen (1%) starting in the second part of the zone. The UV taxa account for around 7% and stay stable along the core, and are mainly dominated by Rutaceae (2%), and Melastomataceae (5%). These two taxa are therefore not shown in the diagram (except in the subtotal in Figure 4).
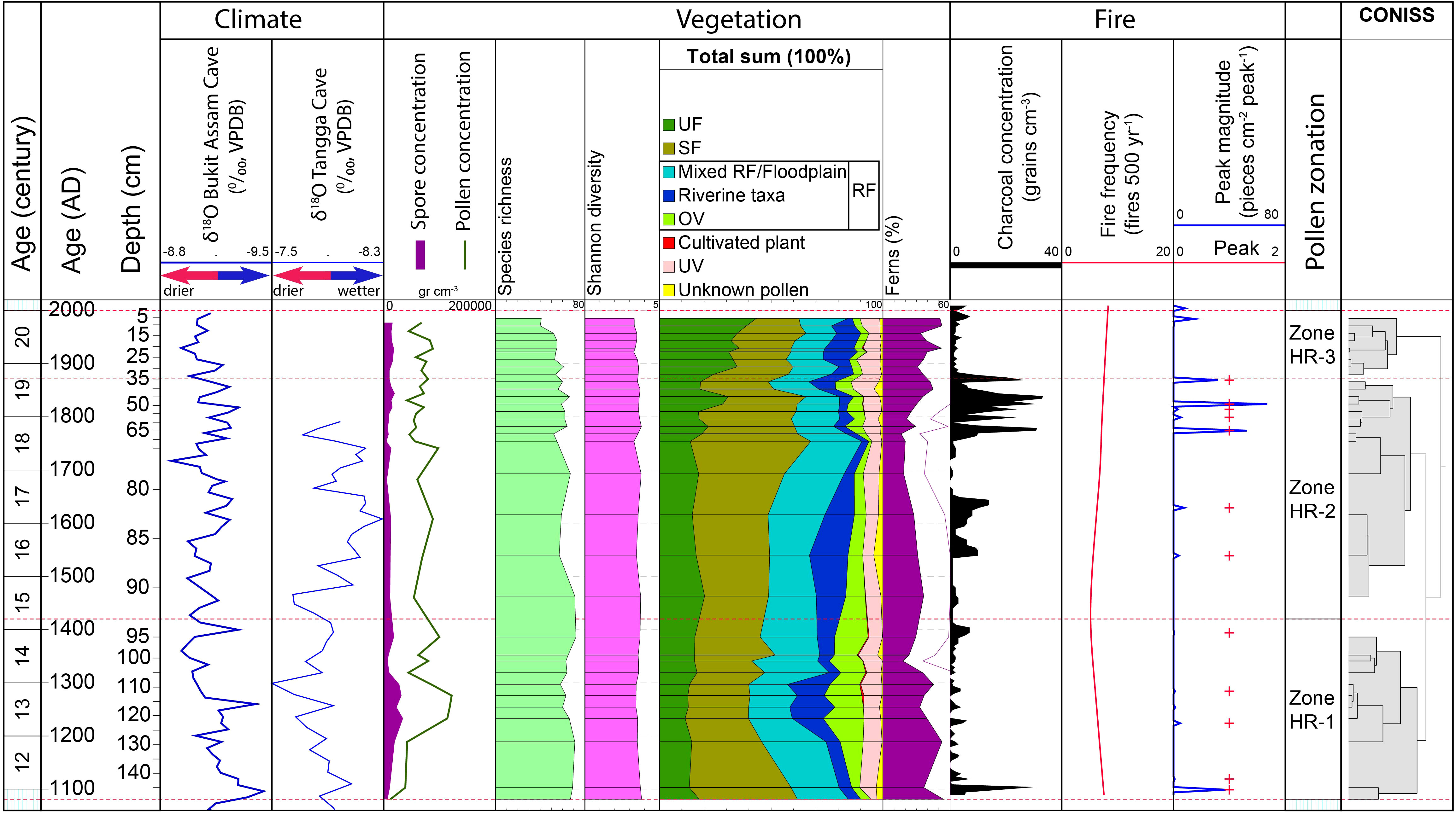
Figure 4 Comparison between various records: Age scale, δ18O recorded in the Bukit Assam Cave, Gunung Buda, Sarawak (Chen et al., 2016) and Tangga Cave (Wurtzel et al., 2018)); pollen and spore concentration, species richness, Shannon diversity index, total sum of pollen and spore of each ecological group in percentages, CharAnalysis results (e.g. macro-charcoal concentration, fire peaks, peaks magnitude, and fire frequency), pollen zonation and CONISS.
4.2.2 Zone HR-2 (95 – 35 cm, AD 1400 – 1870)
The UF pollen taxa increase (to 22%); Moraceae/Urticaceae (to 3%), Begoniaceae (to 3%), Dipterocarpaceae (to 2%) and Lithocarpus/Castanopsis (to 2%). The SF pollen taxa increase (to 37%), Tristaniopsis (to 3%), Anacardiaceae (to 3%), and Calamus (to 3%), Burseraceae (to 2%). However, Oncosperma decrease (3 to 1%), Ternstroemia (to 3%) and Pandanus (to < 1%). Elaeocarpaceae is stable at 5%. The RF pollen decrease (to 32%), Macaranga/Mallotus (to 6%), Fabaceae (to 1%). Cereals are almost absent in this zone.
4.2.3 Zone HR-3 (35 – 0 cm, AD 1870 – present)
The UF pollen taxa continuously increase (to 38%), Icacinaceae (to 5%), Moraceae/Urticaceae (3 to 5%), Begoniaceae (to 7%), Dipterocarpaceae (to 3%), Lithocarpus/Castanopsis (to 4%), and Sapotaceae (to 2%). The SF pollen taxa decrease (to 25%), led by the decrease in Tristaniopsis (to 0%), Ternstroemia (to 1%), Anacardiaceae (to <1%). However, Elaeocarpaceae increases (to 7%). The RF pollen continuously decrease (to 28%) due to the decrease in Actinidiaceae (to 2%). However, some taxa increase in proportion, such as Macaranga/Mallotus (6 to 9%). The OV pollen taxa represent about 1%, mainly grasses. At the end of this zone, the presence of Elaeis guineensis is important despite its low values (<1%).
4.3 Macro-charcoal and fire regime
The CharAnalysis results show the global signal-to-noise index is high (4.4). The local signal-noise index (SNI) values fluctuate above 3, indicating potential local fires (Kelly et al., 2011). The raw charcoal counting is interpolated into 4 year intervals (the median temporal resolution). The mean fire return interval (mFRI) is 70 with a mean fire frequency of about 7 peaks per 500 years. Overall, a total of 11 peaks are modelled along the core (Figure 4); in general the macro-charcoal concentration is low in zone 1 (150 – 95 cm) with an average of 3 particles cm−3; the highest charcoal concentration is in zone 2 (95 – 35 cm) with an average 7 particles cm−3, and the second highest charcoal concentration is in zone 3 (35 – 0 cm) with an average 5 particles cm−3.
4.4 Principal component analysis
The unimodular DCA showed a gradient length of 1.1 SD units (<2.5 SD). Therefore, a linear response model (PCA) was considered more appropriate to summarize the pollen and spore composition changes over time. All pollen and spore taxa were included in the statistical analysis and the most dominant and important (40 taxa) were selected to be shown in Figure 5. The first two components, PC1 and PC2 represent the most dominant and common pollen types and explain 29% of the total variance. The relatively low explained variance suggests the presence of other influential factors, such as hydrological processes (e.g. seasonal precipitation patterns, changes in sediment transport dynamics, alterations in the flow regime), geomorphological dynamics (e.g. river channel adjustments, meander dynamics, floodplains that influence the spatial distribution of pollen types, etc.), anthropogenic disturbances (cultivation, open landscape, logging, etc.), and spatial/temporal variability (e.g. localized microclimatic conditions affecting pollen production or dispersal), which may contribute significantly to the unexplained variance and overall variability observed in the study site. PC1 (18%) likely represents the development of wetland – upland taxa, whereas PC2 (11%) represents the presence of riparian – freshwater swamp taxa. The PCA biplot shows a clear separation between three periods of riparian forest dynamics. The first period, from AD 1100 – 1400, shows a period of riparian environment dominated by OV taxa such as wild grasses, cereals and Caryophyllaceae, and riverine taxa such as Macaranga/Mallotus, and Rhizophoraceae. From AD 1400 – 1870, the vegetation is dominated by SF (e.g. Calamus, Tristaniopsis, Burseraceae). Later, from about AD 1870 – present, the forest is dominated by upland taxa such as Dipterocarpaceae, Icacinaceae, Lithocarpus/Castanopsis, Sapotaceae, and Begoniaceae
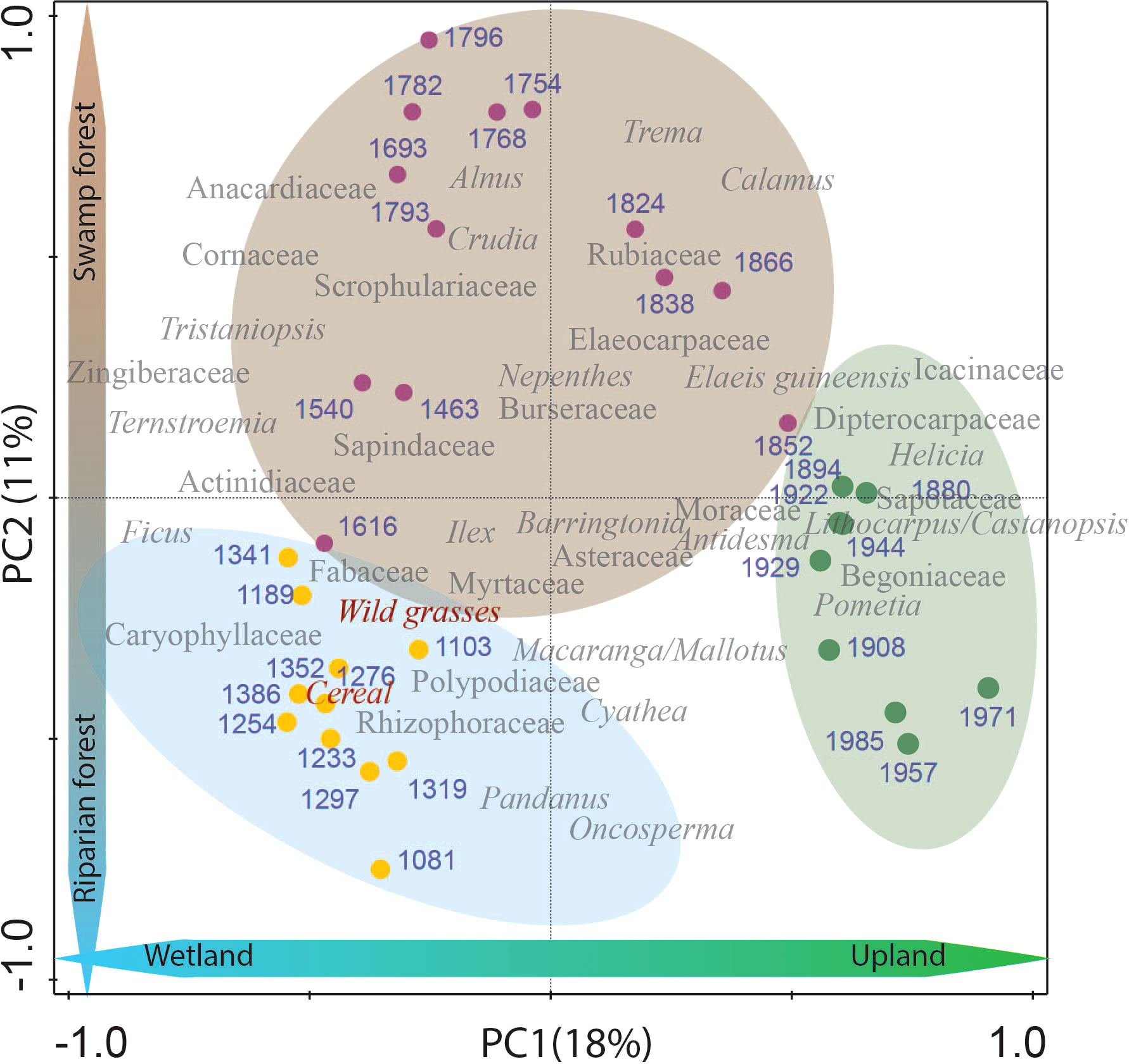
Figure 5 PCA plot for percentage data of identified pollen and spores obtained from the Harapan forest since AD 1100. Biplots of representative species and samples show a relationship between samples and taxa assemblage in three different periods of the Harapan forest. The blue circle represents the period of dominant RF (AD 1100 – 1400). The brown circle represents the period of typical SF (AD 1400 – 1870), and the green circle represents the period of common UF (AD 1870 – present). Blue numbers are the estimated age (AD) of each sample.
5 Interpretation and discussion
5.1 Dynamics of the Harapan riparian forest, the role of climate and humans
The pollen data record of the last millennium shows the occurrence of Upland forest (UF), Swamp forest (SF) and Riparian forest (RF) taxa, which include mixed RF/Floodplain, Riverine and Open vegetation (OV) (Figures 3, 4) in the study area of the Harapan forest. The proportions of the different vegetation types were relatively stable (except for the increase in UF at the end of the record), but taxa show stronger changes within the groups and three distinct periods were identified by CONISS.
5.1.1 Period from AD 1100 – 1400 (150 – 95 cm, zone HR-1)
The core lithology from this period shows an accumulation of fine-grained sandy layers (Figure 2), suggesting deposition from flowing water. Additionally, this zone facilitated the deposition of pollen from UF taxa, such as Lithocarpus/Castanopsis, Dipterocarpaceae, Sapotaceae, Begoniaceae, and Icacinaceae, which were possibly transported into the coring site.
This period is characterized by a high frequency of RF taxa, such as Actinidiaceae, Macaranga/Mallotus, Callophyllum, Rhizophoraceae, and Baringtonia which well-represent riparian habitats. The occurrence of wild grasses (Figure 3) indicates the possible presence of herbaceous marshes or grassy swamp nearby. Nowadays, herbaceous marshes is found in freshwater swamps in Sabah, Malaysia (Ismail et al., 2021) and in the lebak swamps (lebak swamps are non-tidal swamps) along the Musi river in the Pampangan sub-district, South Sumatra where grass swamps are utilized for grazing Pampangan buffaloes and for cultivation (Laumonier, 1997; Ali et al., 2014). However, the increase in OV may also be related to human activity (see below).
In the SF, a high proportion of Pandanus is found at the beginning of the record, while other groups such as Ternstroemia, Oncosperma, Elaeocarpaceae, and Calamus occurred less frequently (Figure 3), suggesting a Pandanus-dominated swamp near the coring site. Nowadays, Pandanus can be found in swamp environments and often colonises submerged/flooded riverbanks in Sumatra (Laumonier, 1997; Whitten and Damanik, 2000; Biagioni et al., 2015). Later, Ternstroemia, followed by Oncosperma, increased in the SF. At the end of this period (from ca. AD 1300 to 1400), Elaeocarpaceae became more frequent. In Southeast (SE) Asia, Elaeocarpus is documented as pioneer of the early development of peat swamp forests (Cole et al., 2015). Increased Elaeocarpaceae abundance indicates an early successional phase or disturbed vegetation, as seen in the Air Hitam peatland (Figure 1B; Biagioni et al., 2015).
Since riparian wetlands can respond to climate change and to human disturbance (Wantzen et al., 2008; Pandey et al., 2022), it is difficult to disentangle the vegetation dynamics affected by climate change and human impacts. At the beginning of our record from AD 1100 to 1250, paleo-precipitation records in the Tangga Cave (Figure 1A) showed relatively high δ18O values (Figure 4), indicating high precipitation (Chen et al., 2016; Wurtzel et al., 2018). Around the same time, there were two fire events, in AD 1100 and 1120 (Figure 4). The wetter climatic conditions led to extensive growth of wild grass (Figure 3), which in turn increased the availability of fuel and resulted in more fire frequent occurrences, probably caused by natural ignition sources (e.g. lightning). Later, during AD 1250 – 1350, the δ18O in the Tangga Cave recorded maximum values, indicating that Sumatra received less precipitation (Wurtzel et al., 2018), which could have suppressed the growth of SF (Nishimua et al., 2007) as seen in our record (Figure 4). On the other hand, in the late Holocene, the occurrence of local and regional burning in SF related to dry climate has been documented in Sumatra (Chen et al., 2016; Hapsari et al., 2021; Hapsari et al., 2022). This coincides with the presence of charcoal with fire events in our record in AD 1220 and 1280 (Figure 4). However, the fire events that occurred during high rainfall (AD 1100, 1120) and drier seasons (Figure 4) might also be related to human activities.
In addition to the occurrence of fire events in wetlands, caused by natural factors as discussed above, the presence of charcoal in paleoecological records or fire events in wetlands also strongly indicates human activity (Bush et al., 2007; Åkesson et al., 2023). Historically, human-caused fires intended to maintain and to open landscapes are common across many regions and periods (Bowman et al., 2011). For example, a paleoecological study of Lake Ayauchi, a tributary of the Marañón River in Amazonian Ecuador, by Åkesson et al. (2023) revealed that fire events were occurring linked to human activity and did not align with dry periods. In Indonesia, fire is commonly utilized as the cheapest and fastest way to open land for agriculture (Simorangkir, 2007; Cattau et al., 2016; Cole et al., 2019) and fishing (Hope et al., 2005). Thus, in our record, fire events from the beginning of this period indicate that the indigenous people might also have used fire for multiple purposes, e.g. cooking, fishing, and opening lands.
The occurrence of cereal pollen around AD 1300 – 1450 (Figure 3) indicates that agricultural activities have been conducted in the study area of the Harapan forest. Some common/major cereal species widely cultivated in Sundaland are Oryza, Sorghum, Setaria and Echinochloa (Hapsari and Ballauff, 2022). Historically, since moving to the forest, the Batin Sembilan people have used the forests to construct their huts and to practice cultivation (Wardah, 2013). One of the staple crops that they have cultivated in the Harapan forest is dry rice (Hein et al., 2015). Merten et al. (2020) documented that in Jambi, the riparian floodplains were only cultivated during the dry season with dry rice, corn or soybean. The proportion of Fabaceae and Caryophyllaceae also increased during the interval of cereal pollen grain occurrence in our record (Figure 3). Some species of Fabaceae (e.g. Aeschynomene indica, A. aspera) and Caryophyllaceae (e.g. Polycarpon sp.) are the most common weeds in lowland paddy fields documented in SE Asia (e.g. Lao, Cambodia, Indonesia; Moody, 1989; Huelma et al., 1996; Caton et al., 2010; Kosaka et al., 2013; Martin et al., 2017). Therefore, the occurrence of cereal pollen grains and higher proportions of Fabaceae and Caryophyllaceae, might indicate that the indigenous people, probably Batin Sembilan, conducted dry rice cultivation. The relatively high proportion of OV and other disturbance indicators during this period, such as Macaranga/Mallotus in the RF, could therefore possibly be related to human activity. Furthermore, in the upland, the slight decrease in UF in the middle part of this period (Figure 3) may also be related to human presence. Dipterocarpaceae and Lithocarpus/Castanopsis were not so frequent during this period. Nowadays, they are widely used for housing or boat construction (Lemmens et al., 1995) and it is possible that they might have also been used for wood by the indigenous people in the past.
5.1.2 Period from AD 1400 – 1870 (95 – 35 cm, zone HR-2)
At the beginning of this period, the sediments changed to include a higher proportion of clay and mud, indicating that the area was more frequently flooded, since the clay and muddy sediments could be deposited during flooding (Whitemore, 1982). During this period, SF increased continuously but only very slightly (Figure 4) with a stronger presence of Burseraceae followed by other SF taxa. Tristaniopsis is common in permanently flooded forests (Sosef et al., 1998; Aribal and Fernando, 2018). The increase in Tristaniopsis, together with dominant Calamus and Anacardiaceae species (Figure 3) toward the end of this period (from ca AD 1700 – 1780) suggests an expansion of rattan mixed freshwater swamp forests. At the end of this period (ca. AD 1780 – 1870), the presence of Nepenthes suggests that the swampy environment had become nutrient-poor (Sim et al., 1992; Rawi and Rohani, 2021), which may have contributed to the decline of the SF vegetation in the area due to the different nutritional requirements of the plant species involved.
Climate seems to be the main factor driving the shift in SF vegetation during this period. The stronger occurrence of SF vegetation between AD 1540 and 1740 (Figure 3) is consistent with low δ18O values recorded in the Tangga Cave during AD 1350 – 1420, 1540 – 1650 and 1700 – 1740 (Figure 4), indicating high precipitation (Wurtzel et al., 2018). The high rainfall season can promote waterlogged conditions (Sayama et al., 2015; Yamamoto et al., 2021) and encourage the growth of SF (e.g. Burseraceae) as seen in our record (Figures 3, 4), and particularly favors taxa adapted to inundated conditions and frequent floods such as Tristaniopsis and Calamus (Figure 3; Sosef et al., 1998). After AD 1760, the δ18O values in the Tangga Cave increased (Figure 4), indicating the climate changed to drier conditions. Macro-charcoal analysis revealed fire events at about AD 1770, 1800, 1810, 1820, and 1870, dates which are in accordance with the highest δ18O values recorded in the Tangga Cave and Bukit Assam Cave, and Gunung Buda, Sarawak (about 1300 km from the Harapan forest; Chen et al., 2016) (Figure 4), suggesting the occurrence of regional burning related to a drier climate. Around the same time, El Niño events with strong (S) and very strong (VS) intensities were globally documented in the years 1761 (S), 1775 (S), 1803-1804 (S), 1814 (S), 1828 (VS) and 1871 (S) (Quinn et al., 1987), coinciding with severe droughts in East Java (Rodysill et al., 2013). An observational study by Hamid et al. (2001) shows increased lightning flashes during the El Niño events over Indonesia. Page et al. (2002) reported that the El Niño-linked dry spells can even cause peatlands to dry up, potentially promoting wildfires in Indonesia. In our study, the long dry season and wildfires – possibly related to El Niño events and droughts – may interrupt the development of SF at the end of this period. However, the frequent fire events and riparian ecosystem dynamics recorded at the site are also manifestly related to human activities.
The decrease in cereal presence since AD 1400 (Figure 3) suggests that the indigenous people no longer cultivated cereal near the coring site. The reduction of cereal cultivation could be explained by climatic flooding conditions. High rainfall and inundated conditions can damage cereal cultivation (Hartono et al., 2020; Merten et al., 2020). Nowadays, in Indonesia, local farmers grow rice once a year in riparian wetlands due to the occurrence of unpredictable, prolonged flooding (Lakitan et al., 2018a; Lakitan et al., 2019). Flooding can last for up to six months during the rainy season, during which time no agricultural activities take place (Wati et al., 2015; Siaga et al., 2017). In our record (Figures 3, 4), the increase in rainfall during AD 1350 – 1420 may have suppressed the development of cereals which usually are cultivated in the floodplain during the dry season (Merten et al., 2020). The indigenous Batin Sembilan farmers with a tradition of dry rice cultivation (Hein and Faust, 2013) could also have changed their lifestyles, adapting to the flooding season during this period, for example by fishing, and harvesting non-timber forest products, perhaps collecting rattan and selling it to traders as seen nowadays (Indrizal and Anwar, 2023). At the end of this period (ca. AD 1850), the presence of the exotic oil palm Elaeis guineensis (Figure 3) indicates oil palm cultivation on a small scale in the study area. The presence of oil palm is in accordance with the increase in fire with frequent fire events and the decrease in SF (Figure 4), suggesting that humans may use fire to open the swampy area to cultivate oil palm in the Harapan forest. In addition, the SF vegetation record shows that Tristaniopsis has sharply decreased since AD 1800. Ternstroemia has also decreased at the same time. Nowadays, the hard and durable wood of Tristaniopsis is widely used for heavy constructions such as bridges, wharves and jetties (Sosef et al., 1998). Ternstroemia is also widely used for house interior constructions, such as ceilings, window frames, and doors (Soerianegara and Lemmens, 1994). The indigenous people may therefore be exploiting these taxa from the SF of the past.
5.1.3 Period from AD 1870 – present (35 – 0 cm, zone HR-3)
Since AD 1870, the UF portion of the assemblage has increased markedly, by the stronger occurrence of Lithocarpus/Castanopsis, Dipterocarpaceae, Icacinaceae, Begoniaceae and Sapotaceae, suggesting that the upland Harapan forests have been increasingly dominant. This can be explained by an expansion of UF vegetation to the study site, or by the UF being less disturbed by humans than forests in swampy areas (see below). Macaranga/Mallotus, which can adapt well in the UF, also dominate the pollen assemblage.
It is difficult to distinguish the influences of climate and humans on the vegetation dynamics during this period. Since 1900, the annual temperature in Indonesia has increased by ca. 0.3°C, and annual precipitation has decreased by about 2 – 3% (Hulme and Sheard, 1999; Case and Spector, 2007). This may have caused less inundation and promoted the expansion of UF.
However, local human activities, such as opening forests by cultivation and cutting trees, may also have contributed to the change in vegetation during this period. The exotic oil palm (Elaeis guineensis) pollen indicates its cultivation in the study area, but probably at a certain distance from the coring site. On the other hand, SF vegetation shows that Calamus, Anacardiaceae, and Burseraceae are very rare during this period. Nowadays, rattans (Calamus) are intensively collected for trading due to their high value (Dransfield and Manokaran, 1994). Due to its light weight, durability, flexibility, and attractive natural appearance, rattan has numerous applications, including furniture, handicrafts, and construction (Dransfield and Manokaran, 1994). Furthermore, Anacardiaceae and Burseraceae are important families for major commercial timber production (Soerianegara and Lemmens, 1994). Therefore, the indigenous people may also have been utilizing more of these taxa from SF during this period.
6 Summary and conclusion
The riparian vegetation in the Harapan forest underwent remarkable dynamics, reflecting a complex interplay of vegetation and environmental changes, climatic fluctuations, and human activities during the recorded last millennium. Our paleoecological study identified three distinct periods of vegetation development.
Between AD 1100 and 1400, the forest displayed a combination of mixed open, riverine and swamp vegetation, indicating the possible occurrence of an open forested riparian buffer zone. The occurrence of fires may indicate human activities since the beginning of this period. Later from AD 1300 to 1450, evidence of cereal cultivation document agricultural activities carried out by the Batin Sembilan people. The indigenous use of slash-and-burn farming played an important role in shaping the vegetation composition in the riparian zone of the forest.
From AD 1400 to 1870, freshwater swamps were dominant, and both climate fluctuations and human activities remarkably impacted the dynamics of the swampy vegetation. At the beginning of this period, the high rainfall season played a crucial role as it promoted waterlogged conditions, providing an environment for the growth of swamp forests. Towards the end of this period, the development of swamp forest vegetation faced suppression, likely due to strong fires that were correlated with regional dry seasons. Furthermore, human activities had a persistent impact by fires, especially with changes in land-use practices like oil palm plantations and logging.
Since AD 1870 to the present, upland forests have become the prevailing vegetation type in the study area. This change could be attributed to various factors, including the increase in annual temperatures and a decrease in annual precipitation in Indonesia, which may have led to reduced inundation and facilitated the expansion of upland forests. Additionally, human activities, such as forest clearance for cultivation and extensive tree-cutting in the swamp forest, might have also played an important role in driving the vegetation shift during this period.
Overall, our research shows the complex relationship between vegetation and environmental changes, including climatic fluctuations as well as human activities by fire and agriculture, that have influenced the riparian vegetation dynamics of the Harapan forest over the past millennia. This study emphasizes the importance of understanding both natural and anthropogenic factors that have shaped the present-day riparian vegetation of the Harapan ecosystem.
Data availability statement
The original contributions presented in the study are included in the article/Supplementary Material. Further inquiries can be directed to the corresponding author.
Author contributions
Conceptualization, CN, KH, and HB. Methodology, CN, KH, and HB. Writing – original draft preparation, CN. Writing – review and editing, CN, KH and HB. Supervision, KH, HB, AS and SS. Project administration, HB, AS and SS. Funding acquisition, HB. All authors have read and agreed to the published version of the manuscript.
Funding
The authors are very grateful for our financial supported by the German Research foundation (DFG) within the CRC 990 (192626868) subproject A01 and by the German Academic Exchange Service (DAAD).
Acknowledgments
This study was conducted using the research permit (RISTEK; 23/EXT/SIP/FRP/E5/Dit.KI/VI/2017) from the Ministry of Research and Technology of Indonesia and sample export permit (B-1127/IPH.1/KS.02.04/111/2019) based on the recommendation of the Indonesian Institute of Sciences (LIPI). Furthermore, we gratefully acknowledge logistic support from the EFForTS coordination team and the Indonesian partners, Institut Pertanian Bogor (IPB) and University of Jambi (UNJA), the Ministry of Education in Jakarta (DIKTI) and the Indonesian Institute of Sciences (LIPI). We also thank Siria Biagioni and the Indonesian team for her help with the fieldwork and sample collection. Furthermore, we express our sincere gratitude to the two reviewers for their invaluable suggestions, which contributed to the improvement of the manuscript during the review process. Last but not least, we acknowledge support by the Open Access Publication Funds of the Göttingen University.
Conflict of interest
The authors declare that the research was conducted in the absence of any commercial or financial relationships that could be construed as a potential conflict of interest.
Publisher’s note
All claims expressed in this article are solely those of the authors and do not necessarily represent those of their affiliated organizations, or those of the publisher, the editors and the reviewers. Any product that may be evaluated in this article, or claim that may be made by its manufacturer, is not guaranteed or endorsed by the publisher.
Supplementary material
The Supplementary Material for this article can be found online at: https://www.frontiersin.org/articles/10.3389/fevo.2023.1224160/full#supplementary-material
References
Åkesson C. M., McMichael C. N. H., León-Yánez S., Bush M. B. (2023). Late-Holocene maize cultivation, fire, and forest change at Lake Ayauchi, Amazonian Ecuador. Holocene 33 (5), 550–561. doi: 10.1177/09596836231151833
Aldrian E., Susanto R. D. (2003). Identification of three dominant rainfall regions within Indonesia and their relationship to sea surface temperature. Int. J. Climatology 23 (12), 1435–1452. doi: 10.1002/joc.950
Ali A., Sandi S., Muhakka M., Riswandi, Budianta D. (2014). The grazing of pampangan buffaloes at non tidal swamp in South Sumatra of Indonesia. APCBEE Proc. 8, 87–92. doi: 10.1016/j.apcbee.2014.03.006
Anderbeck K. R. (2008). Malay Dialects of the Batanghari River Basin (Jambi, Sumatra). Ed. Wise M. R. (SIL International). Available at: https://www.sil.org/resources/publications/entry/9245.
Aribal L., Fernando E. (2018). Plant diversity and structure of the Caimpugan peat swamp forest on Mindanao Island, Philippines. Mires Peat 22, 1–16. doi: 10.19189/MaP.2017.OMB.309
Asian Development Bank (2016). Indonesia country water assessment. Malina, Philippines: Asian Development Bank (ADB).
Biagioni S., Krashevska V., Achnopha Y., Saad A., Sabiham S., Behling H. (2015). 8000years of vegetation dynamics and environmental changes of a unique inland peat ecosystem of the Jambi Province in Central Sumatra, Indonesia. Palaeogeography Palaeoclimatology Palaeoecol. 440, 813–829. doi: 10.1016/j.palaeo.2015.09.048
Blaauw M. (2010). Methods and code for ‘classical’ age-modelling of radiocarbon sequences. Quaternary Geochronology 5 (5), 512–518. doi: 10.1016/j.quageo.2010.01.002
Bowman D. M. J. S., Balch J., Artaxo P., Bond W. J., Cochrane M. A., D’Antonio C. M., et al. (2011). The human dimension of fire regimes on Earth. J. Biogeography 38 (12), 2223–2236. doi: 10.1111/j.1365-2699.2011.02595.x
Briggs M., de Kok R., Moat J., Whaley O., Williams J. (2012). Vegetation mapping for reforestation and carbon capture in the harapan rainforest. Published Rep. to DEFRA grant: DX11-04. Available at: https://www.researchgate.net/publication/265913379_Vegetation_Mapping_for_Reforestation_and_Carbon_Capture_in_the_Harapan_Rainforest/citations
Bush M. B., Silman M. R., Listopad C. M. C. S. (2007). A regional study of Holocene climate change and human occupation in Peruvian Amazonia. J. Biogeography 34 (8), 1342–1356. doi: 10.1111/j.1365-2699.2007.01704.x
Cane M. A. (2005). The evolution of El Niño, past and future. Earth Planetary Sci. Lett. 230 (3), 227–240. doi: 10.1016/j.epsl.2004.12.003
Capon S. J. (2020). “Riparian Ecosystems,” in Encyclopedia of the World's Biomes. Eds. Goldstein M. I., DellaSala D. A. (United State: Elsevier), 170–176. doi: 10.1016/B978-0-12-409548-9.11884-6
Carcaillet C., Bouvier M., Fréchette B., Larouche A. C., Richard P. J. H. (2001). Comparison of pollen-slide and sieving methods in lacustrine charcoal analyses for local and regional fire history. Holocene 11 (4), 467–476. doi: 10.1191/095968301678302904
Case M., Spector E. (2007). Climate Change in INDONESIA Implications for Humans and Nature. Indonesia: Word Wide Fund for Nature (WWF), 1–13. Available at: https://www.researchgate.net/publication/237325800_Climate_Change_in_Indonesia_Implications_for_Humans_and_Nature.
Caton B. P., Mortimer M., Hill J. E., Johnson D. E. (2010). A practical field guide to Weeds of Rice in Asia. 2nd ed. (Laguna, Philippines: International Rice Research Institute).
Cattau M. E., Harrison M. E., Shinyo I., Tungau S., Uriarte M., DeFries R. (2016). Sources of anthropogenic fire ignitions on the peat-swamp landscape in Kalimantan, Indonesia. Global Environ. Change 39, 205–219. doi: 10.1016/j.gloenvcha.2016.05.005
Chen S., Hoffmann S. S., Lund D. C., Cobb K. M., Emile-Geay J., Adkins J. F. (2016). A high-resolution speleothem record of western equatorial Pacific rainfall: Implications for Holocene ENSO evolution. Earth Planetary Sci. Lett. 442, 61–71. doi: 10.1016/j.epsl.2016.02.050
Clark J. S., Lynch J., Stocks B. J., Goldammer J. G. (1998). Relationships between charcoal particles in air and sediments in west-central Siberia. Holocene 8 (1), 19–29. doi: 10.1191/095968398672501165
Cole L. E. S., Bhagwat S. A., Willis K. J. (2015). Long-term disturbance dynamics and resilience of tropical peat swamp forests. J. Ecol. 103 (1), 16–30. doi: 10.1111/1365-2745.12329
Cole L., Bhagwat S., Willis K. (2019). Fire in the swamp forest: Palaeoecological insights into natural and human-induced burning in intact tropical peatlands. Front. Forests Global Change 2. doi: 10.3389/ffgc.2019.00048
Cui T., Tian F., Yang T., Wen J., Khan M. Y. A. (2020). Development of a comprehensive framework for assessing the impacts of climate change and dam construction on flow regimes. J. Hydrology 590, 125358. doi: 10.1016/j.jhydrol.2020.125358
Devitt L., Neal J., Coxon G., Savage J., Wagener T. (2023). Flood hazard potential reveals global floodplain settlement patterns. Nat. Commun. 14 (1), 2801. doi: 10.1038/s41467-023-38297-9
Dransfield J., Manokaran N. (1994). “Rattans,” in Plant Resources of South-East Asia, vol. 6 . Eds. Westphal E., Jansen P. C. M. (Bogor, Indonesia: Prosea Foundation).
Drescher J., Rembold K., Allen K., Beckschäfer P., Buchori D., Clough Y., et al. (2016). Ecological and socio-economic functions across tropical land use systems after rainforest conversion. Philos. Trans. R. Soc. B: Biol. Sci. 371, 20150275. doi: 10.1098/rstb.2015.0275
Dudgeon D. (2000a). The ecology of tropical asian rivers and streams in relation to biodiversity conservation. Annu. Rev. Ecol. Systematics 31, 239–263. doi: 10.1146/annurev.ecolsys.31.1.239
Dudgeon D. (2000b). Riverine biodiversity in Asia: a challenge for conservation biology. Hydrobiologia 418 (1), 1–13. doi: 10.1023/A:1003998519910
Dudgeon D. (2009). Large-scale hydrological changes in tropical Asia: Prospects for riverine biodiversity. BioScience 50, 793–806. doi: 10.1641/0006-3568(2000)050[0793:LSHCIT]2.0.CO;2
Dwire K. A., Mellmann-Brown S., Gurrieri J. T. (2018). Potential effects of climate change on riparian areas, wetlands, and groundwater-dependent ecosystems in the Blue Mountains, Oregon, USA. Climate Serv. 10, 44–52. doi: 10.1016/j.cliser.2017.10.002
Faegri K., Iversen J. (1989). Textbook of pollen analysis. Ed. Knut Faegri, Peter Emil Kaland, Knut (Great Britain UK: John Wiley and Sons).
Fitri M., Triadi S., Harun I. B. (2018). Preferences for settlement improvement in urban Riparian Indonesia. Ecology Environ. Conserv. 24, 663–672.
Forbes H. O. (1885). On the kubus of Sumatra. J. Anthropological Institute Great Britain Ireland 14, 121–127. doi: 10.2307/2841849
Grimm E. (1987). CONISS: A FORTRAN 77 program for stratigraphically constrained cluster analysis by the method of incremental sum of squares. Comput. Geosciences 13, 13–35. doi: 10.1016/0098-3004(87)90022-7
Hamid E., Kawasaki Z.-I., Mardiana R. (2001). Impact of the 1997-98 El Nino Event on lightning activity over Indonesia. Geophysical Res. Lett. - GEOPHYS Res. Lett. 28, 147–150. doi: 10.1029/2000GL011374
Hapsari K. A., Ballauff J. (2022). Distinguishing pollen grains of cereal from wild grasses in the Sundaland region using size separation. Rev. Palaeobotany Palynology 301, 1–10. doi: 10.1016/j.revpalbo.2022.104648
Hapsari K. A., Biagioni S., Jennerjahn T. C., Reimer P., Saad A., Sabiham S., et al. (2018). Resilience of a peatland in Central Sumatra, Indonesia to past anthropogenic disturbance: Improving conservation and restoration designs using palaeoecology. J. Ecol. 106 (6), 2473–2490. doi: 10.1111/1365-2745.13000
Hapsari K. A., Biagioni S., Jennerjahn T. C., Saad A., Sabiham S., Corre M. D., et al. (2021). Late Holocene ENSO-related fire impact on vegetation, nutrient status and carbon accumulation of peatlands in Jambi, Sumatra, Indonesia. Rev. Palaeobotany Palynology 293, 104482. doi: 10.1016/j.revpalbo.2021.104482
Hapsari K. A., Jennerjahn T., Nugroho S., Yulianto E., Behling H. (2022). Sea level rise and climate change acting as interactive stressors on development and dynamics of tropical peatlands in coastal Sumatra and South Borneo since the Last Glacial Maximum. Global Change Biol. doi: 10.1111/gcb.16131
Harrison R., Swinfield T. (2015). Restoration of logged humid tropical forests: An experimental programme at harapan rainforest, Indonesia. Trop. Conserv. Sci. 8, 4–16. doi: 10.1177/194008291500800103
Hartono A., Wijdan H., Nurrochmad F., Pratiwi E., Setyawan C. (2020). “Precipitation and flood impact on rice paddies: Statistics in Central Java, Indonesia,” in The 6th International Conference on Water Resource and Environment August 23rd to 26th, 2020, IOP Publishing ltd conference. Vol. 612. doi: 10.1088/1755-1315/612/1/012040
Hauser-Schäublin B., Steinebach S. (2014). Harapan: A "No Man's Land" Turned into a Contested Agro-Industrial Zone (Göttingen, Germany: EFForTS Discussion Paper). Available at: http://hdl.handle.net/10419/117316.
Hein J. I. (2019). Political Ecology of REDD+ in Indonesia: Agrarian Conflicts and Forest Carbon (London, UK: Routledge). doi: 10.4324/9781351066020
Hein J. I., Adiwibowo S., Dittrich C., Soetarto E., Faust H. (2015). Rescaling of access and property relations in a frontier landscape: Insights from Jambi, Indonesia. Prof. Geographer 68, 1–10. doi: 10.1080/00330124.2015.1089105
Hein J., Faust H. (2013). Conservation, REDD+ and the struggle for land in Jambi, Indonesia. Pac. Geogr. 41, 20–25.
Hennings N., Becker J. N., Guillaume T., Damris M., Dippold M. A., Kuzyakov Y. (2021). Riparian wetland properties counter the effect of land-use change on soil carbon stocks after rainforest conversion to plantations. CATENA 196, 104941. doi: 10.1016/j.catena.2020.104941
Higuera P. E., Brubaker L. B., Anderson P. M., Hu F. S., Brown T. A. (2009). Vegetation mediated the impacts of postglacial climate change on fire regimes in the south-central Brooks Range, Alaska. Ecol. Monogr. 79 (2), 201–219. doi: 10.1890/07-2019.1
Hogg A. G., Hua Q., Blackwell P. G., Niu M., Buck C. E., Guilderson T. P., et al. (2013). SHCal13 Southern hemisphere calibration, 0–50,000 years cal BP. Radiocarbon 55 (4), 1889–1903. doi: 10.2458/azu_js_rc.55.16783
Hope G., Chokkalingam U., Anwar S. (2005). The stratigraphy and fire history of the Kutai Peatlands, Kalimantan, Indonesia. Quaternary Res. 64 (3), 407–417. doi: 10.1016/j.yqres.2005.08.009
Huelma C., Moody K., Mew T. (1996). Weed seeds in rice seed shipments: A case study. Int. J. Pest Manage. - Int. J. Pest MANAGE 42, 147–150. doi: 10.1080/09670879609371986
Hulme M., Sheard N. (1999). Climate Change Scenarios for Indonesia (Norwich, UK: University of East Anglia, Climatic Research Unit).
Indrizal E., Anwar H. (2023). The indigenous people Suku Anak Dalam Batin Sembilan livelihood: Adaptation and socio-cultural dynamics. ETNOSIA Jurnal Etnografi Indonesia 8, 24–43. doi: 10.31947/etnosia.v8i1.23836
Iskandar I., Lestari D. O., Saputra A. D., Setiawan R. Y., Wirasatriya A., Susanto R. D., et al. (2022). Extreme Positive Indian Ocean Dipole in 2019 and its impact on Indonesia. Sustainability 14 (22), 1–15. doi: 10.3390/su142215155
Ismail P., Tariq Mubarak H., Hyrul Izwan M. H. (2021). An overview of wetlands in Malaysia Vol. 153 (Malaysia: Forest research institute Malaysia).
Jain A., Lee J. G. H., Chao N., Lees C., Orenstein R., Strange B. C., et al. (2018). Helmeted hornbill (Rhinoplax vigil: status review, range-wide conservation strategy and action plan, (2018 - 2027) (Sarawak, Malaysia: IUCN Species Survival Commission Hornbill Speciallist Group).
Jowsey P. C. (1966). AN IMPROVED PEAT SAMPLER. New Phytol. 65 (2), 245–248. doi: 10.1111/j.1469-8137.1966.tb06356.x
Junk W. J., Piedade M. T. F., Schöngart J., da Cunha C. N., Goncalves S. R. A., Wantzen K. M., et al. (2022). Riparian wetlands of low-order streams in Brazil: extent, hydrology, vegetation cover, interactions with streams and uplands, and threats. Hydrobiologia. doi: 10.1007/s10750-022-05056-8
Kauffman J., Krueger W. (1984). Livestock impacts on riparian ecosystems and streamside management implications... A review. J. Range Manage. 37, 430. doi: 10.2307/3899631
Kelly R. F., Higuera P. E., Barrett C. M., Hu F. S. (2011). A signal-to-noise index to quantify the potential for peak detection in sediment–charcoal records. Quaternary Res. 75 (1), 11–17. doi: 10.1016/j.yqres.2010.07.011
Khan O., Mwelwa-MuteKenya E., Crosato A., Zhou Y. (2014). Effects of dam operation on downstream river morphology, the case of the Middle Zambezi River. Water Manage. 167, 596–611. doi: 10.1680/wama.13.00122
Kosaka Y., Xayvongsa L., Vilayphone A., Chanthavong H., Takeda S., Kato M. (2013). Wild edible herbs in paddy fields and their sale in a mixture in Houaphan province, the lao people’s democratic republic. Economic Bot. 67 (4), 335–349. doi: 10.1007/s12231-013-9251-6
Lakitan B., Alberto A., LIndiana L., Kartika K., Herlinda S., Kurnianingsih A. (2018a). The benefits of biochar on rice growth and yield in tropical riparian wetland, South Sumatra, Indonesia. Chiang Mai Univ. J. Natural Sci. 17 (2), 111–126. doi: 10.12982/CMUJNS.2018.0009
Lakitan B., Hadi B., Herlinda S., Siaga E., Widuri L. I., Kartika K., et al. (2018b). Recognizing farmers’ practices and constraints for intensifying rice production at Riparian Wetlands in Indonesia. NJAS - Wageningen J. Life Sci. 85, 10–20. doi: 10.1016/j.njas.2018.05.004
Lakitan B., Siaga E., Kartika K., Yunindyawati Y. (2019). Use of Scleria poaeformis as biomaterial in etno-agricultural practice at riparian wetlands in Indonesia. Bulgarian J. Agric. Sci. 25 (2), 320–325.
Lambertini M. (2008). Long term conservation of the Harapan rainforest in Sumatra (Cambrige, UK: Final report to the Nando Peretti Foundation).
Laumonier Y. (1997). The Vegetation and Physiography of Sumatra Vol. 22 (The Netherlands: kluwer Academic).
Laumonier Y., Uryu Y., Stüwe M., Budiman A., Setiabudi B., Hadian O. (2010). Eco-floristic sectors and deforestation threats in Sumatra: identifying new conservation area network priorities for ecosystem-based land use planning. Biodiversity Conserv. 19 (4), 1153–1174. doi: 10.1007/s10531-010-9784-2
Lemmens R. H. M. J., Soerianegara I., Wong W. C. (1995). “Timber trees: Minor commercial timbers,” in Plant Resources of South-East Asia. Eds. Lemmens R. H. M. J., Soerianegara I., Wong W. C. (Bogor, Indonesia: Prosea Foundation).
Lowrance R. (1998). “Riparian Forest Ecosystems as Filters for Nonpoint-Source Pollution,” in Successes, Limitations, and Frontiers in Ecosystem Science. Eds. Pace M. L., Groffman P. M. (New York, USA: Springer New York), 113–141. doi: 10.1007/978-1-4612-1724-4_5
Margono B. A., Potapov P. V., Turubanova S., Stolle F., Hansen M. C. (2014). Primary forest cover loss in Indonesia over 2000–2012. Nat. Climate Change 4 (8), 730–735. doi: 10.1038/nclimate2277
Martin R. J., Van Ogtrop F., Henson Y., Broeum K., Rien R., Srean P., et al. (2017). A survey of weed seed contamination of rice paddy in Cambodia. Weed Res. 57 (5), 333–341. doi: 10.1111/wre.12265
McAllister D., Craig J., Davidson N., Delany S., Seddon M. (2001). Biodiversity impacts of large dams. International Union for Conservation of Nature and Natural Resources and the United Nations Environmental Programme. Available at: https://www.researchgate.net/publication/255643683_Biodiversity_Impacts_of_Large_Dams.
Merten J., Stiegler C., Hennings N., Purnama E. S., Röll A., Agusta H., et al. (2020). Flooding and land use change in Jambi Province, Sumatra: integrating local knowledge and scientific inquiry. Ecol. Soc. 25 (3), 14. doi: 10.5751/ES-11678-250314
Miettinen J., Shi C., Liew S. C. (2011). Deforestation rates in insular Southeast Asia between 2000 and 2010. Global Change Biol. 17 (7), 2261–2270. doi: 10.1111/j.1365-2486.2011.02398.x
Moody K. (1989). Weeds reported in Rice in South and Southeast Asia (Laguna, Philippines: International Rice Research Institute).
Morley R. J. (2013). Cenozoic ecological history of South East Asian peat mires based on the comparison of coals with present day and Late Quaternary peats. J. Limnology 72, 36–59. doi: 10.4081/jlimnol.2013.s2.e3
National Research Council. (2002). Riparian Areas: Functions and Strategies for Management. (Washington, D.C. USA: The National Academies Press). doi: 10.17226/10327
Nishimua T. B., Suzuki E., Kohyama T., Tsuyuzaki S. (2007). Mortality and growth of trees in peat-swamp and heath forests in central kalimantan after severe drought. Plant Ecol. 188 (2), 165–177. doi: 10.1007/s11258-006-9154-z
Page S. E., Siegert F., Rieley J. O., Boehm H.-D. V., Jaya A., Limin S. (2002). The amount of carbon released from peat and forest fires in Indonesia during 1997. Nature 420 (6911), 61–65. doi: 10.1038/nature01131
Pandey S., Kumari T., Verma P., Singh R., Raghubanshi A. S. (2022). “Chapter 16 - Impact of anthropogenic stresses on riparian ecosystem and their management perspectives,” in Ecological Significance of River Ecosystems. Eds. Madhav S., Kanhaiya S., Srivastav A., Singh V., Singh P. (Netherlands: Elsevier), 299–324. doi: 10.1016/B978-0-323-85045-2.00004-2
Paoletti A., Darras K., Jayanto H., Grass I., Kusrini M., Tscharntke T. (2018). Amphibian and reptile communities of upland and riparian sites across Indonesian oil palm, rubber and forest. Global Ecol. Conserv. 16, e00492. doi: 10.1016/j.gecco.2018.e00492
Philander S. G. H. (1990). “El Niño, La Niña, and the Southern Oscillation,” in International Geophysics Series. Eds. Holton J., Dmowska R. (USA: Academic Press), 289.
Poff N. L., Allan J. D., Bain M. B., Karr J. R., Prestegaard K. L., Richter B. D., et al. (1997). The natural flow regime. BioScience 47 (11), 769–784. doi: 10.2307/1313099
Quinn W. H., Neal V. T., Antunez De Mayolo S. E. (1987). El Niño occurrences over the past four and a half centuries. J. Geophysical Research: Oceans 92 (C13), 14449–14461. doi: 10.1029/JC092iC13p14449
Rawi N.-H., Rohani S. (2021). Species richness and soil properties in Nepenthes habitat at Bris Ecosystem in Setiu. Terengganu UNIVERSITI MALAYSIA TERENGGANU J. OF UNDERGRADUATE Res. 3, 67–72. doi: 10.46754/umtjur.2021.10.007
R Core Team. (2018). R: A language and environment for statistical computing (R Foundation for Statistical Computing). Available at: https://www.R-project.org/.
Rodríguez-González P., Abraham E., Aguiar F., Andreoli A., Berisha N., Bernez I., et al. (2022). Bringing the margin to the focus: 10 challenges for riparian vegetation science and management. Wiley Interdiscip. Reviews: Water 9 (5), 1–14. doi: 10.1002/wat2.1604
Rodysill J. R., Russell J. M., Crausbay S. D., Bijaksana S., Vuille M., Edwards R. L., et al. (2013). A severe drought during the last millennium in East Java, Indonesia. Quaternary Sci. Rev. 80, 102–111. doi: 10.1016/j.quascirev.2013.09.005
Saji N. H., Goswami B. N., Vinayachandran P. N., Yamagata T. (1999). A dipole mode in the tropical Indian Ocean. Nature 401 (6751), 360–363. doi: 10.1038/43854
Sayama T., Tatebe Y., Iwami Y., Tanaka S. (2015). Hydrologic sensitivity of flood runoff and inundation: 2011 Thailand floods in the Chao Phraya River basin. Nat. Hazards Earth Syst. Sci. 15 (7), 1617–1630. doi: 10.5194/nhess-15-1617-2015
Schneider C., Flörke M., De Stefano L., Petersen-Perlman J. D. (2017). Hydrological threats to riparian wetlands of international importance – a global quantitative and qualitative analysis. Hydrol. Earth Syst. Sci. 21 (6), 2799–2815. doi: 10.5194/hess-21-2799-2017
Shannon C. E. (1948). A mathematical theory of communication. Bell System Tech. J. 27 (3), 379–423. doi: 10.1002/j.1538-7305.1948.tb01338.x
Shrestha S., Imbulana N., Piman T., Chonwattana S., Ninsawat S., Babur M. (2020). Multimodelling approach to the assessment of climate change impacts on hydrology and river morphology in the Chindwin River Basin, Myanmar. CATENA 188, 104464. doi: 10.1016/j.catena.2020.104464
Siaga E., Lakitan B., Bernas S. M., Wijaya A., Lisda R., Ramadhani F., et al. (2017). Application of floating culture system in chili pepper (Capsicum annum L.) during prolonged flooding period at riparian wetland in Indonesia. Aust. J. Crop Sci. 12 (5), 808–816. doi: 10.21475/ajcs.18.12.05.PNE1007
Sim J. W. S., Tan H. T. W., Turner I. M. (1992). Adinandra belukar: an anthropogenic heath forest in Singapore. Vegetatio 102 (2), 125–137. doi: 10.1007/BF00044729
Simorangkir D. (2007). Fire use: Is it really the cheaper land preparation method for large-scale plantations? Mitigation Adaptation Strategies Global Change 12 (1), 147–164. doi: 10.1007/s11027-006-9049-2
Simpson G., Birks H. (2012). Statistical Learning in Palaeolimnology, Vol. 5. 249–327. doi: 10.1007/978-94-007-2745-8_9
Singh R., Tiwari A. K., Singh G. S. (2021). Managing riparian zones for river health improvement: an integrated approach. Landscape Ecol. Eng. 17 (2), 195–223. doi: 10.1007/s11355-020-00436-5
Šmilauer P., Lepš J. (2014). Multivariate Analysis of Ecological Data using CANOCO 5. 2 ed (UK: Cambridge University Press). doi: 10.1017/CBO9781139627061
Soerianegara I., Lemmens R. H. M. J. (1994). “Timber trees: Major commercial timbers,” in Plant Resources of South-East Asia, vol. 5 . Eds. Soerianegara I., Lemmens R. H. M. J. (Bogor, Indonesia: Prosea Foundation).
Sosef M. S. M., Hong L. T., Prawirohatmodjo S. (1998). Plant resources of South-East Asia (PROSEA) Vol. 5 (Bogor, Indonesia: Prosea foundation).
Steinebach S. (2013). “Today we Occupy the Plantation - Tomorrow Jakarta" - Indigenity, Land and Oil Palm Plantations in Jambi,” in Adat and Indigenity in Indonesia, Culture and Entitlements between Heteronomy and Self-Ascription, vol. 7 . Ed. Hauser-Schäublin B. (Universitätsverlag Göttingen: Göttingen Studies in Cultural Property).
Stevenson J., Haberle S. (2005). Macro Charcoal Analysis: A Modified Technique Used by the Department of Archaeology and Natural History (Australia: Australian National University (ANU).
Stockmarr J. (1971). Tablets with spores used in absolute pollen analysis. Pollen Spores 13, 615–621.
Stratadata (2014) Stratabugs biostratigraphic data management software. Available at: http://www.stratadata.co.uk.
Sutihat A. (2014). Types of river transportation in the east coast of Sumatra before the 19th century: an analysis based on the findings of boats in North Sumatera Province and Siak districts, Riau Province. MUA collection.. Available at: http://www.themua.org/collections/items/show/1640.
Tockner K., Stanford J. A. (2002). Riverine flood plains: present state and future trends. Environ. Conserv. 29 (3), 308–330. doi: 10.1017/S037689290200022X
Utomo A., Walsh T. (2018). Hutan Harapan ecosystem restoration concession, Sumatra, Indonesia: A potential OECM? Parks 24, 61–68. doi: 10.2305/IUCN.CH.2018.PARKS-24-SIABU.en
von Rintelen K., Arida E., Häuser C. (2017). A review of biodiversity-related issues and challenges in megadiverse Indonesia and other Southeast Asian countries. Res. Ideas Outcomes 3, e20860. doi: 10.3897/rio.3.e20860
Wantzen K. M., Yule C. M., Tockner K., Junk W. J. (2008). “Riparian Wetlands of Tropical Streams,” in Tropical Stream Ecology. Ed. Dudgeon D. (USA: Academic Press), 199–217. doi: 10.1016/B978-012088449-0.50009-1
Wardah N. (2013). Harapan rainforest: Cultivating or Ignoring Hopes? The Livelihood of the Forest Dwelling People and Their Perceptions (A case Study in Harapan forest, Jambi Indonesia) (The Netherland: Wageningen University]).
Wati I., Ehara H., Suwignyo R., Sakagami J.-I. (2015). Swamp rice cultivation in South Sumatra, Indonesia: an overview. Trop. Agric. Dev. 59, 35–39. doi: 10.11248/jsta.59.35
Whitmore T. C., Tantra I. G. M. (1986). Tree flora of Indonesia: check list for Sumatra (Indonesia: Bogor).
Whitten T., Damanik S. J. (2000). The Ecology of Sumatra (Singapore: Periplus Editions (HK) Limited).
Widianingsih N. N., David W., Pouliot M., Theilade I. (2019). Land use, income, and ethnic diversity in the margins of Hutan Harapan – A rainforest restoration concession in Jambi and South sumatra, Indonesia. Land Use Policy 86, 268–279. doi: 10.1016/j.landusepol.2019.05.006
Widianingsih N. N., Theilade I., Pouliot M. (2016). Contribution of forest restoration to rural livelihoods and household income in Indonesia. Sustainability 8 (9), 1–22. doi: 10.3390/su8090835
Wulandari R. A. (2021). Livelihood dynamics among the semi-sedentary Kubu Batin Sembilan of Anak Dalam Tribe in Sumatra. (Master thesis). Wageningen University and Research, Wageningen, Netherlands.
Wurtzel J. B., Abram N. J., Lewis S. C., Bajo P., Hellstrom J. C., Troitzsch U., et al. (2018). Tropical Indo-Pacific hydroclimate response to North Atlantic forcing during the last deglaciation as recorded by a speleothem from Sumatra, Indonesia. Earth Planetary Sci. Lett. 492, 264–278. doi: 10.1016/j.epsl.2018.04.001
Yamamoto K., Sayama T., Apip A. (2021). Impact of climate change on flood inundation in a tropical river basin in Indonesia. Prog. Earth Planetary Sci. 8, 1–15. doi: 10.1186/s40645-020-00386-4
Zarfl C., Lumsdon A. E., Berlekamp J., Tydecks L., Tockner K. (2015). A global boom in hydropower dam construction. Aquat. Sci. 77 (1), 161–170. doi: 10.1007/s00027-014-0377-0
Keywords: Late Holocene, riparian wetlands, riparian and swamp forest dynamics, climate change, human impact
Citation: Nguyen CH, Hapsari KA, Saad A, Sabiham S and Behling H (2023) Late Holocene riparian vegetation dynamics, environmental changes, and human impact in the Harapan forest of Sumatra, Indonesia. Front. Ecol. Evol. 11:1224160. doi: 10.3389/fevo.2023.1224160
Received: 17 May 2023; Accepted: 28 July 2023;
Published: 15 August 2023.
Edited by:
Anna Maria Mercuri, University of Modena and Reggio Emilia, ItalyReviewed by:
Eleonora Clò, University of Modena and Reggio Emilia, ItalyConstantin Nechita, National Institute for Research and Development in Forestry Marin Dracea (INCDS), Romania
Copyright © 2023 Nguyen, Hapsari, Saad, Sabiham and Behling. This is an open-access article distributed under the terms of the Creative Commons Attribution License (CC BY). The use, distribution or reproduction in other forums is permitted, provided the original author(s) and the copyright owner(s) are credited and that the original publication in this journal is cited, in accordance with accepted academic practice. No use, distribution or reproduction is permitted which does not comply with these terms.
*Correspondence: Chung Hoai Nguyen, Y2h1bmctaG9haS5uZ3V5ZW5AYmlvbG9naWUudW5pLWdvZXR0aW5nZW4uZGU=