- 1Research Institute of Forestry, Chinese Academy of Forestry, Key Laboratory of Forest Silviculture of the State Forestry and Grassland Administration, Beijing, China
- 2Co-Innovation Center for Sustainable Forestry in Southern China, Nanjing Forestry University, Nanjing, China
- 3Hubei Academy of Forestry, Wuhan, China
- 4Hubei Shennongjia Forestry Ecosystem Research Station, Shennongjia, China
Nitrogen (N) deposition is an important environmental factor that can change soil chemical properties. It can also alter the characteristics of microbial communities. The incorporation of biochar into soils is considered a potential strategy to enhance carbon (C) storage in soil and modify the impacts of N deposition. However, the impacts of biochar on the microbial characteristics of soil after short-term N deposition in subtropical plantations remain poorly understood. Here, we investigated the effects of biochar application (0, 5, 10 t ha−1) on soil chemical traits and microbial characteristics (extracellular enzyme activities, microbial community and microbial biomass) in a Larix kaempferi plantation in Shennongjia, China, under N addition (0, 50, 100 kg N ha−1 yr−1) during two growing seasons. We found that simulated N deposition significant increased soil total nitrogen (TN), nitrate nitrogen (NO3−-N) and total phosphorus (TP) concentrations, while heavy N deposition (100 kg N ha−1 yr−1) significant decreased soil microbial biomass nitrogen (MBN) concentration and β-glucosidase (β-GC) activity. Biochar amendment significantly increased soil microbial biomass, TN and soil organic carbon (SOC) concentrations. Both N addition and biochar amendment significantly altered Ascomycota and Basidiomycota relative abundance, with biochar amendment increasing Ascomycota relative abundance and decreasing Mortierellomycota relative abundance under heavy N deposition. Fungal diversity showed a positive correlation to TN, TP and NO3−-N concentrations, but a negative correlation to MBN. Biochar addition inhibited the increase in soil NO3−-N concentration caused by high N addition in the plantation, and influenced the change in the composition of microbial community caused by N addition. Our piecewise structural equation model suggested that N addition affected MBN and fungal diversity directly or indirectly via its effects on soil enzyme activities and properties. In contrast, there were no significant direct or indirect effects on bacterial diversity among all factors. These results improve our understanding of the influence and mechanisms of N addition and biochar amendment on soil microbial characteristics in subtropical coniferous plantations in the short term, and can provide a valuable reference for predicting the future effects of N deposition on soils in this region’s plantation.
1 Introduction
The atmospheric nitrogen (N) deposition resulting from human activities has significantly impacted terrestrial ecosystems globally (Lilleskov et al., 2019; Yan et al., 2020), with soil biogeochemical processes, microbial biomass and activities being greatly affected (Lingli and L, 2009; Li et al., 2019a). In China, agriculture and fossil fuels are major sources of N deposition (Janssens et al., 2010; Liu et al., 2013), and studies have calculated that the total N storage in Chinese forests is 14.45 ± 8.42 t N hm–2, with soil containing roughly 94% of this total (Xu and He, 2020). Given China’s status as the largest developing country, it is expected that there will be a significant increase in N deposition within its borders (Yu et al., 2019). N deposition, along with stand density and age, is a crucial factor in modulating forest growth (Sophia et al., 2020). This increase in N can negatively impact soil pH (Dai et al., 2018) and organic carbon (C) content (Chen et al., 2018), with changes in various edaphic properties such as microbial biomass and microbial communities (Zhu et al., 2016; Chen et al., 2020; Jia et al., 2020; Tang et al., 2020; Zhu et al., 2020), which can affect soil quality, C storage capacity and permanent changes in soil C accumulation over time (Chen et al., 2018; Dai et al., 2018; Yan et al., 2019; Flechard et al., 2020). Anthropogenic N inputs, whether due to climate change or forest management practices, may significantly affect belowground processes, particularly microbial activity, in subtropical forests in China (Yan et al., 2020). For example, N addition caused a change in the N/P ratio, which significantly increases the biomass of fungi and the activity of certain enzymes in a subtropical Castanopsis forest (Fan et al., 2020), while N addition-induced changes in soil N availability and soil pH significantly altered the bacterial and fungal community structures of subtropical natural forest (Wang et al., 2021). However, the difference in the direction of the effect of N addition on the bacterial and fungal communities of typical subtropical plantation soils and the mechanisms responsible for this difference need further investigation.
Biochar is a carbon-rich substance with a highly porous (Lehmann and Joseph, 2015), could enhance the growth and colonization of soil microbes (Gul et al., 2015). Due to its short term mineralization and long term stability (Smith et al., 2010; Hassan et al., 2020), biochar is often used to adsorb pollutants (Zhang et al., 2019) and nutrient elements (Zhang et al., 2020) in water to altering water environment, as well as to remediating contaminated soils (Zama et al., 2018; Chang et al., 2019). In addition, in order to enhance soil carbon storage and improve soil fertility, biochar is frequently utilized as a soil amendment, and several studies have examined the impact of incorporating biochar into soil for the purpose (Palviainen et al., 2018; Bilgili et al., 2019). For example, the application of poultry litter biochar can reduce soil P limitation due to N inputs, resulting in increased pasture yields (Van Zwieten et al., 2019). According to a meta-analysis (Zhou et al., 2017), adding biochar does not have a significant impact on soil carbon storage. However, studies conducted in subtropical bamboo forests in China have shown that adding biochar can effectively enhance soil organic carbon sequestration (Ge et al., 2019), the reasons may be biochar application led to an increase in the recalcitrance of soil organic carbon and a decrease in carbon-degrading enzyme activities (Li et al., 2018b). The results suggest that biochar can effectively improve soil health by enhancing carbon sequestration and reducing carbon loss. In a subtropical Torreya grandis orchard, biochar application has led to a significant increase in soil SOC and TN (Li et al., 2020), while inducing a significant decrease in the levels of soil NH4+-N and NO3−-N, and a decline in the activities of soil enzymes associated with the N cycle (Song et al., 2019). Additionally, microbial biomass has been found to increase as a result of biochar application (Lehmann et al., 2011; Teutscherova et al., 2018; Xu et al., 2018). However, due to the differences in the properties of biochar itself and the limited application in typical subtropical coniferous plantations, the direction and mechanism of biochar application to improve the impact of N deposition on soil microbial characteristics remains unclear.
Soils are the largest pool of carbon in terrestrial ecosystems, in fact, the amount of organic carbon in soil organic matter exceeds that of the combined global vegetation and atmosphere (Johannes and Markus, 2015). Soils also harbor a significant proportion of the Earth’s biodiversity, which plays a vital role in supporting many essential ecosystem processes (Guerra et al., 2020). Forests play an important role in terrestrial ecosystems (Xu and He, 2020), and understanding soil carbon dynamics and soil microbial changes in forests is crucial for ecological implications. In China’s forest resources, plantations play an increasingly important role in improving the environment and sequestering carbon (Piao et al., 2009; Song et al., 2022). However, both N deposition and biochar application can alter the soil chemical properties and microbial characteristics of plantation soils (Tu et al., 2011; Li et al., 2018b). Although simulated N deposition and biochar amendment experiments have been conducted in plantations, there is insufficient research on their interaction effects on soil microbial characteristics and soil chemical properties of subtropical plantations in the short term. Shennongjia, located in the Qinba Mountains of China, is an outstanding representative of biodiversity, ecosystem types, and biological evolution at the same latitude in the world, and its ecological status is of great importance. The plantation area accounts for about 30% of the forest area of Shennongjia, with Larix kaempferi as the main plantation tree species. In this study, simulated N deposition and biochar amendment experiments were conducted on L. kaempferi plantation in Shennongjia, and the effects of their interaction and independence on soil chemical properties and microbial characteristics were analyzed. We hypothesize that biochar addition will ameliorate the effects of N addition on soil microbial community structure and composition and other microbial characteristics, and mitigate the changes in soil organic C caused by simulated N deposition.
2 Materials and methods
2.1 Study site
The study site is situated in Shennongjia Forestry District (109°56’–110°58’ E, 31°15’–31°57’ N), Hubei Province, China. The main part of Shennongjia, with an altitude of 398–3105 m, belongs to the northern subtropical monsoon climate zone. The average annual sunshine duration and temperature are 1858.3 h and 12.2°C, respectively, with January and July having the lowest and highest temperatures, respectively. The average annual rainfall is 800–2500 mm, and the growing season is about 220 days.
The L. kaempferi plantation near the National Positioning Observation and Research Station of Forest Ecosystem in Shennongjia Forestry District was selected as the research object. The stand is approximately 40 years old and is located in mountain yellow-brown soil. The main shrubs are Viburnum dilatatum, Smilax china, Rubus hirsutus, and the main herbs include Euphorbia pekinensis, Aquilegia Viridiflora, Cryptotaenia japonica. The basic information of forest land is shown in Table 1.
2.2 Experimental design, fertilization and soil sampling
In April 2019, nine standard experimental plots (10 m × 20 m) were established for N addition in L. kaempferi plantations. The plots were located in forest sites, and adjacent plots with a 10 m buffer zone. According to the amount of N addition, the standard plots were divided into 3 treatments, with 3 replicates per treatment. At the same time, 3 subplots (3 m × 3 m) for biochar amendment were established in each standard experimental plot, with adjacent subplots having a 4 m buffer zone. The 3 subplots in each standard plot were treated with three gradients of biochar. Combined N and biochar addition treatments to form a total of 9 treatments in the split-zone experimental design, including a control (CK: neither N nor biochar addition), low and high N addition (N1 and N2: 50 and 100 kg N ha−1 yr−1), low and high biochar addition (BC5 and BC10: 5 and 10 t biochar ha−1), and combinations of N and biochar treatments (N1BC5, N1BC10, N2BC5 and N2BC10).
In both 2019 and 2020, we simulated N deposition with urea once a month from May to September. On clear days, the urea solution was sprayed evenly on the soil with a precipitation rate of 0.01 cm. The amount of N applied each time was 1/5 of the total annual addition, while the same amount of clean water was sprayed on the CK. Biochar is a 200-mesh sieved coal-based powdered carbon made from coconut shells burnt at 350 °C for 4 hours, specially designed for soil substrate remediation (Lvzhiyuan Activated Carbon Co., Ltd., Henan, China). On 11 July 2019, for practical use in plantation forests and to avoid disturbing the soil and root system (Palviainen et al., 2018), biochar was added by spreading it on the soil surface. Meanwhile, to reduce the scouring of biochar by surface runoff, we sowed most of the biochar on the higher slopes within the subplots. The biochar had a basal composition of organic carbon, total nitrogen, total phosphorus and total potassium of 778.37, 9.84, 10.89 and 0.67 g·kg−1, respectively.
On 30 September 2020, we collected five random soil cores (0–15 cm depth) from each subplot. These were then combined into a homogenized sample, picked out the gravel and roots and the samples were passed through a sieve with a 2 mm mesh. Part of the fresh soil samples were held at −80°C for the determination of soil ammonium nitrogen (NH4+-N), nitrate nitrogen (NO3−-N), soil microbial community structural and diversity, microbial biomass carbon (MBC) and microbial biomass nitrogen (MBN). Another part of the soil samples was air-dried for the determination of soil enzyme activities, pH, soil organic carbon (SOC), total nitrogen (TN) and total phosphorus (TP).
2.3 Measurement of chemical properties, microbial biomass and enzyme activities of the soil
SOC concentration was determined using an enzyme-labelled instrument (Multiskan GO 1510; Vantaa Finland), with ferrous sulfate as a standard solution, the volumetric method is changed to a photometric method, and the SOC is colorimetric at a wavelength of 585 nm. TN concentration was determined by Kjeldahl nitrogen determination (KD310A KjelROC Automatic Kjeldahl Analyzer; Sweden). Soil pH was measured with a pH measuring instrument (pH 211; Hana, Italy) using a soil:water suspension (1:2.5 w:v) prepared with a shaker for 30 minutes (LY/T 1239–1999). The acid-molybdenum antimony anti-colorimetric method was used for the quantification of TP. (NH4+-N and NO3−-N concentrations were determined using an enzyme-labelled instrument (Multiskan GO 1510; Vantaa Finland). The ethanol fumigation-extraction method was used to determine MBC and MBN. The activities of soil acid phosphatase (ACP), β-glucosidase (β-GC), N-acetyl-β-D-glucosidase (NAG) and polyphenol oxidase (PPO) were determined using an enzyme-labelled instrument (Multiskan GO 1510; Vantaa Finland), and the activities of these enzymes were measured colorimetrically at wavelengths of 660, 400, 400 and 430 nm, respectively.
2.4 Soil DNA extraction, amplification, sequencing, and bioinformatics analysis
The Kit used for DNA extraction was the same as in Li et al. (2019b). Extracted DNA was assessed for purity and quality using 1% agarose gel electrophoresis and a NanoDrop spectrophotometer, respectively.
The bacterial 16S rRNA gene amplifies the V3–V4 hypervariable region, as previously described in Mumbi et al. (2015). The protocol described in Zhang et al. (2015) was used to amplify the fungal internal transcribed spacer (ITS) region. The addition of sample primers, PCR amplification and subsequent purification of the PCR products were as described in the method of Li et al. (2022b).
The Illumina MiSeqPE250 platform (Allwegene Company, Beijing, China) was used for deep sequencing. Following the run, bioinformatic analysis including image analysis, base calling and error estimation using the Illumina Analysis Pipeline version 2.6.
2.5 Statistical analysis
After sequencing the PCR products, we screened the raw data and performed operational taxonomic units (OTUs) clustering, referring to the study by Edgar and Robert (2013). Based on the OTU information obtained from each sample, clustering analysis and PCA were conducted (Wang et al., 2012) using R 4.1.3.
Excel 2019 for initial analysis of data; R 4.1.3 for analysis of variance, significance test, correlation analysis, Mantel tests and piecewise structural equation model (piecewiseSEM). Differences of soil chemical properties, enzyme activities, microbial biomass and microbial community composition (phyla and genus level) among all treatments were tested using one-way analysis of variance (ANOVA). Independent and combined effects of N and biochar additions on the above soil properties were tested using two-way ANOVA. Pearson’s correlation analysis and Mantel tests were used to identify the relationships among biotic and abiotic factors and to assess the impact of environmental factors on microbial composition and diversity (Li et al., 2022a). We employed Mantel tests and Pearson’s correlation analysis to identify the most significant predictors of microbial diversity to be included in the piecewiseSEM. The SEM was performed using the ‘nlme’, ‘lme4’ and ‘piecewiseSEM’ packages in R (Liu et al., 2022). To simplify the graphical presentation, different categories of factors, such as soil enzyme activities, soil properties, MBC and MBN, and soil microbial diversity, were placed in the same box. It is important to note that these boxes did not represent latent variables (Lefcheck and Freckleton, 2015; Zhao et al., 2022).
3 Results
3.1 Soil chemical and microbial properties
In N-free addition treatments, both BC5 and BC10 significantly increased SOC and TN concentrations compared to CK (P< 0.05), and with increasing biochar addition, SOC and TN concentrations increased significantly. However, both did not significantly affect TP, NH4+-N and NO3−-N concentrations compared to CK (P > 0.05, Table 2). The addition of biochar can increase soil carbon stocks in the short term. In biochar-free amendment treatments, both N1 and N2 induced a significant increase in TN and NO3−-N concentrations compared to CK (P< 0.05), but did not significantly affect NH4+-N concentration (P > 0.05). In addition, compared to CK, N2 significantly increased TP concentration (P< 0.05). When compared to N2, both N2BC5 and N2BC10 induced a significant increase in TN concentration (P< 0.05), while significantly decreasing NO3−-N concentration (P< 0.05), but not significantly affecting NH4+-N concentration (P > 0.05). Compared to BC5, both N1BC5 and N2BC5 induced a significant increase in TN, TP and NO3−-N concentrations (P< 0.05), but not in SOC and NH4+-N concentrations (P > 0.05). However, compared to BC10, both N1BC10 and N2BC10 caused a significant increase in NO3−-N concentration (P< 0.05) and a significant decrease in SOC and TN concentrations (P< 0.05). This suggests that biochar mitigated the effects of N on some soil chemical properties. Moreover, compared to CK, soil pH only increased under N1 (P< 0.05).
A two-way ANOVA indicated that N and biochar additions, either independently or in combination, significantly affected SOC, TN, NH4+-N, NO3−-N concentrations and pH (P< 0.05, Supplementary Table S1). However, biochar addition did not have a significant independent effect on TP (P > 0.05).
In N-free addition treatments, both BC5 and BC10 significantly increased MBC and MBN concentrations compared to CK (P< 0.05, Table 3). Additionally, compared to CK and BC5, BC10 significantly increased NAG concentration (P< 0.05). In biochar-free amendment treatments, N2 significantly decreased MBN concentration compared to CK and N1 (P< 0.05), and significantly decreased β-GC concentration compared to CK (P< 0.05). Compared to BC5, both N1BC5 and N2BC5 significantly decreased MBC and MBN concentrations (P< 0.05), while having no significant effect on enzyme concentrations (P > 0.05). Compared to BC10, both N1BC10 and N2BC10 significantly decreased MBC concentration (P< 0.05). However, N2BC10 significantly decreased MBN concentration (P< 0.05) and increased PPO concentration (P< 0.05) compared to BC10 and N1BC10. Biochar was shown to ameliorate the effect of N on soil microbial biomass. Compared to CK, treatments with BC10 and N2, both separately and in combination, significantly decreased β-GC concentration (P< 0.05), while other treatments didn’t have a significant effect on β-GC concentration (P > 0.05). In addition, NAG increased only under BC10 compared to CK (P< 0.05).
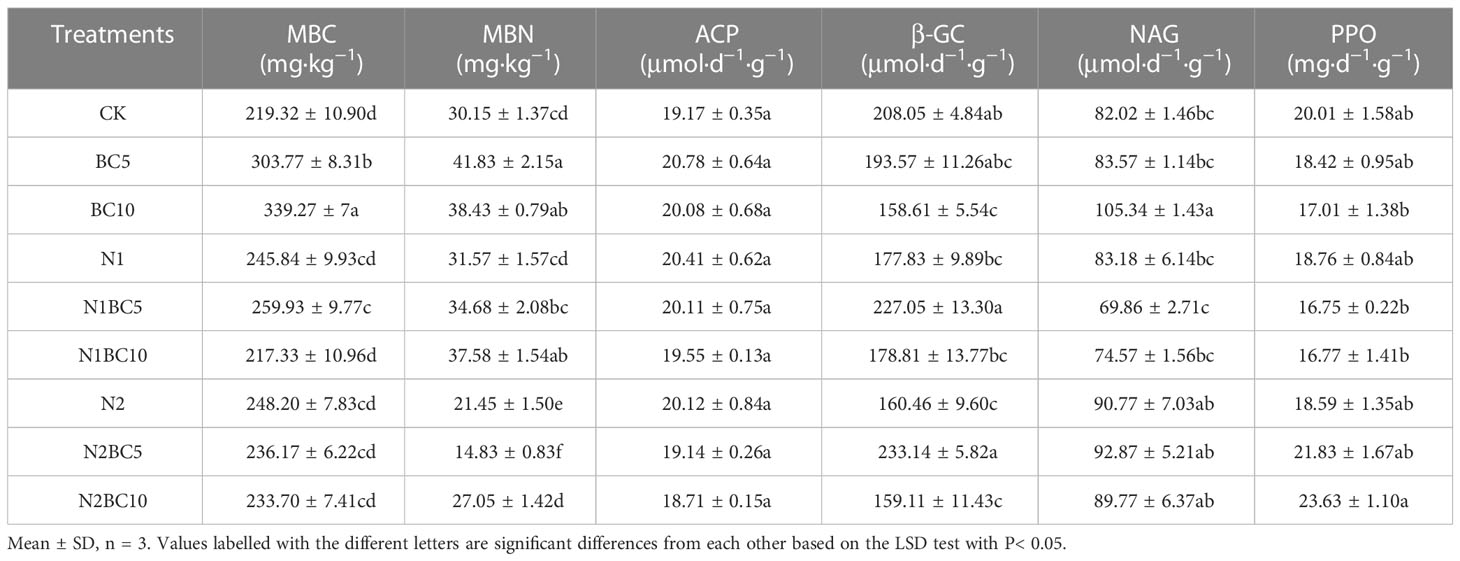
Table 3 Soil microbial biomass and soil enzyme activities a Larix kaempferi plantation in Shennongjia.
A two-way ANOVA indicated that N and biochar additions significantly affected MBC and MBN concentrations, either independently or in combination (P< 0.01, Table S2). Furthermore, β-GC, NAG and PPO were significantly affected by the combined effect of N and biochar addition (P< 0.05), while no significant effect was observed on ACP (P > 0.05). Independently, biochar amendment significantly affected soil β-GC independently (P< 0.001), but no significant effect was observed on ACP, NAG and PPO (P > 0.05). Similarly, N addition significantly affected soil NAG and PPO independently (P< 0.01), but did not affect ACP and β-GC significantly (P > 0.05).
3.2 Composition of soil microbial communities
At the phylum level, Proteobacteria (28.14%), Acidobacteriota (25.70%), Chloroflexi (8.61%), Actinobacteriota (5.42%), Verrucomicrobiota (5.41%), Gemmatimonadota (4.66%) and Planctomycetota (4.29%) were the most dominant phyla of the bacteria (Figure 1A; Supplementary Table S3). The relative abundance of these phyla had no significant differences with N and biochar addition (P > 0.05, Table S3).
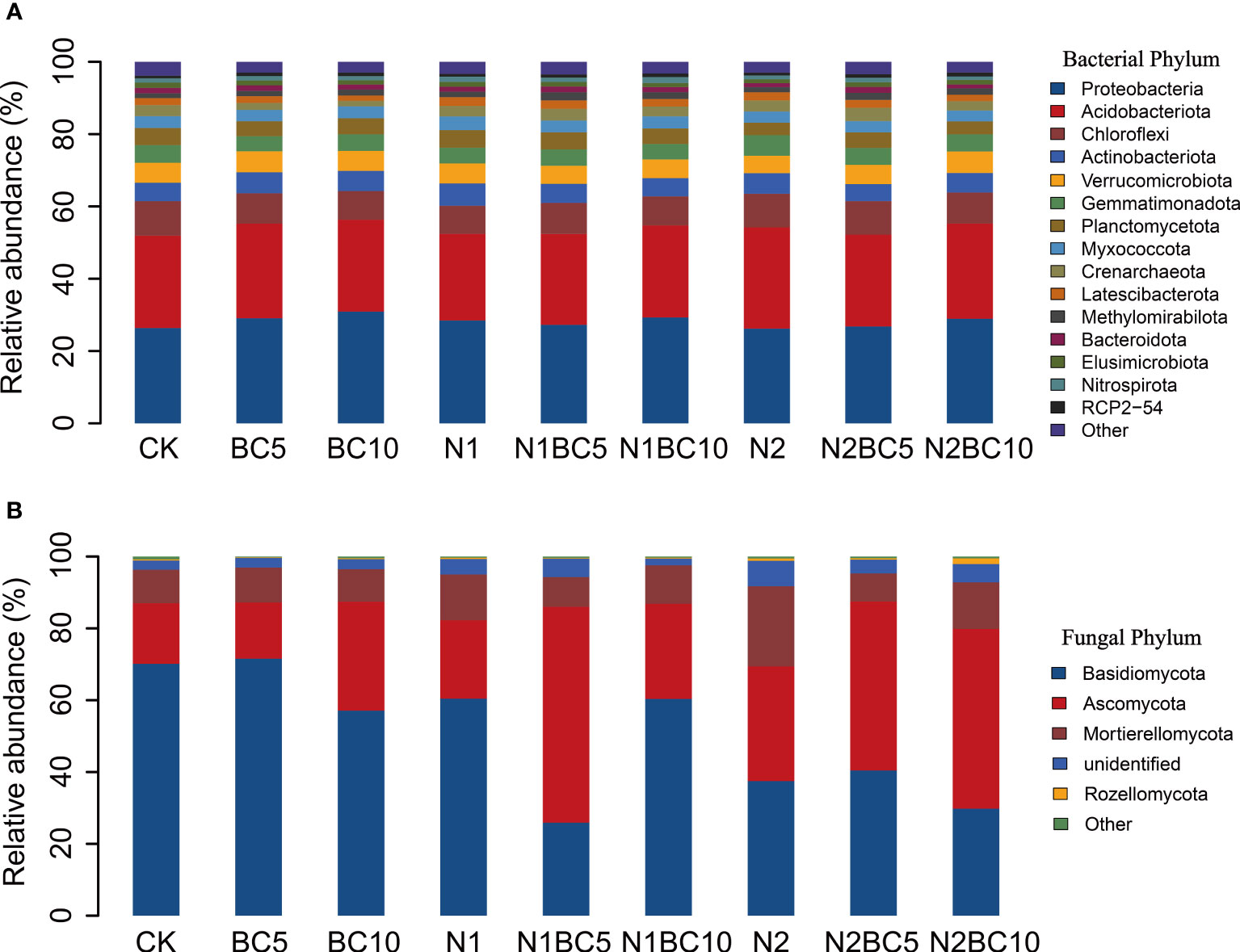
Figure 1 Relative abundance of bacterial (A) and fungal (B) phyla in response to the N and biochar additions.
Basidiomycota (50.35%), Ascomycota (33.37%), Mortierellomycota (11.44%), Rozellomycota (0.47%) and Kickxellomycota (0.12%) were the most dominant fungal phyla at the phylum level (Figure 1B; Supplementary Table S4). In addition, 3.93% of the fungal phyla were unidentified. In N-free addition treatments, the relative abundance of Basidiomycota was lower in BC10 than in CK and BC5 (P< 0.05, Supplementary Table S4), while Ascomycota showed the opposite trends. This indicated that there was a threshold value for the amount of biochar added to affect the fungal community. In biochar- free amendment treatments, both N1 and N2 significantly decreased Basidiomycota compared to CK (P< 0.05), and it decreased significantly with increasing N addition. Basidiomycota may be used as an indicator organism for N deposition in the region. However, Ascomycota and Mortierellomycota were higher in N2 than in CK and N1 (P< 0.05). Compared to N2, both N2BC5 and N2BC10 significantly increased Ascomycota (P< 0.05) and decreased Mortierellomycota and unidentified fungi (P< 0.05). Compared to BC5, both N1BC5 and N2BC5 significantly decreased Basidiomycota (P< 0.05) while increasing Ascomycota (P< 0.05). Similarly, N2BC10 showed a significant decrease in Basidiomycota compared to BC10, while increasing Ascomycota (P< 0.05).
At the genus level, unidentified and uncultured bacteria accounted for 34.20% and 26.99% of all bacterial taxa, respectively (Figure 2A; Supplementary Table S5), and the relative abundance of these groups had no significant differences (P > 0.05; Supplementary Table S5). The relative abundance of Acidibacter was significantly higher in N2BC10 (4.33%) than in N1 (2.44%, P< 0.05). In addition, Bradyrhizobium was significantly higher in BC5 (2.52%) than in N1BC5 (1.39%) and N2BC5 (1.22%, P< 0.05).
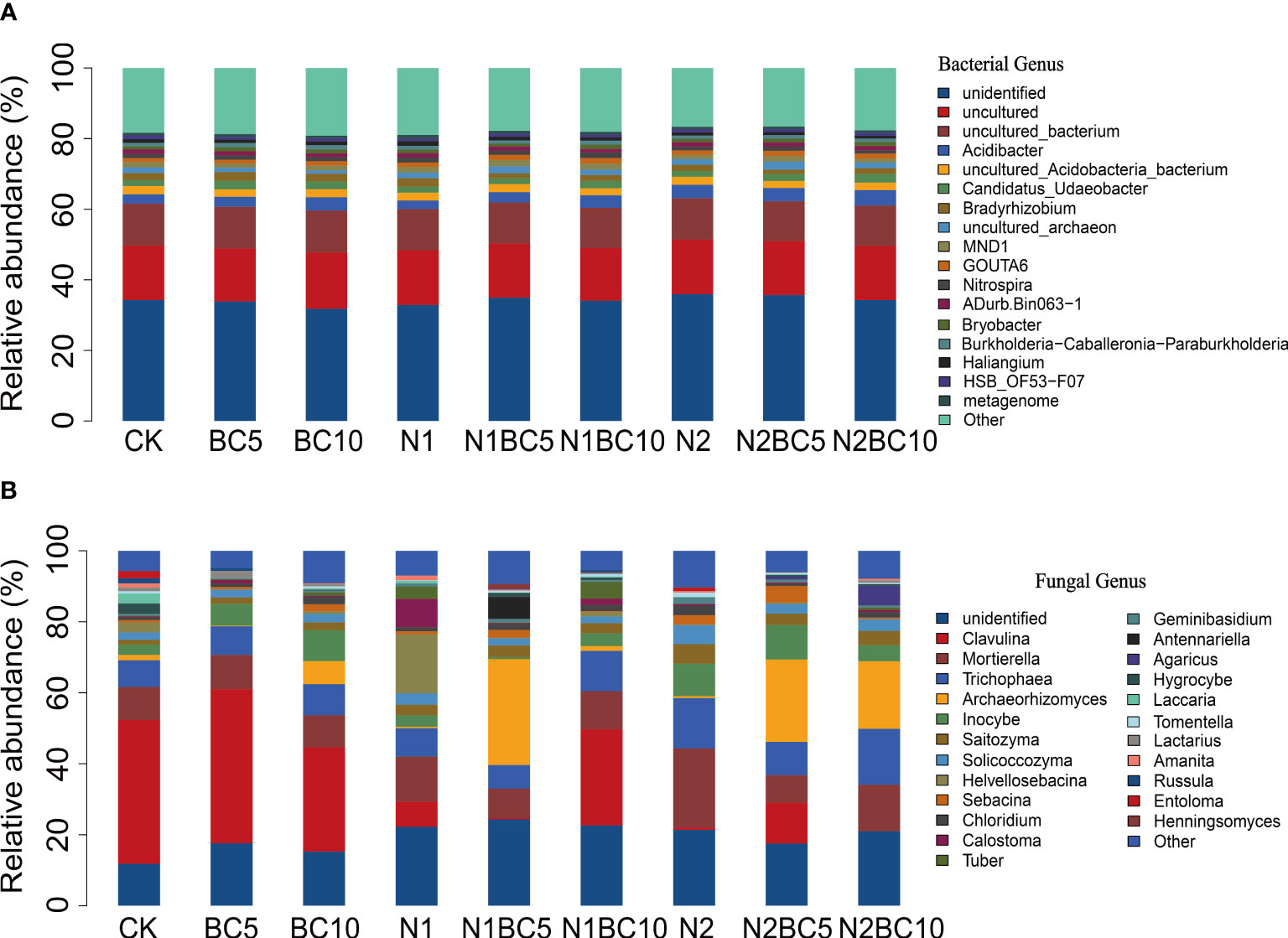
Figure 2 Relative abundance of bacterial (A) and fungal (B) genera in response to the N and biochar additions.
Clavulina (17.77%), Mortierella (11.42%), Trichophaea (9.97%), Archaeorhizomyces (9.16%) and Inocybe (5.41%) were the most dominant fungal taxa in all treatments (Figure 2B; Supplementary Table S6). In addition, 19.32% of the fungal genera were unidentified. In N-free addition treatments, the relative abundance of Clavulina was lower in BC10 than in CK and BC5 (P< 0.05, Table S6), while Inocybe exhibited the opposite trends. In biochar-free amendment treatments, both N1 and N2 significantly decreased Clavulina compared to CK (P< 0.05), with a significant decrease observed with increasing N addition. Additionally, N2 significantly increased Mortierella, Trichophaea and Inocybe compared to CK (P< 0.05). Furthermore, compared to N2, both N2BC5 and N2BC10 significantly decreased Mortierella (P< 0.05) and increased Archaeorhizomyces and unidentified fungal genus (P< 0.05).
3.3 Diversity of soil microbial communities
N1, N1BC5 and CK significantly increased Shannon’s index of bacteria compared to N2BC10 (P< 0.05, Figure 3A). However, Chao1 of bacteria did not show a significant difference among all treatments (P > 0.05, Figure 3C). Only N2BC10 significantly decreased PD_whole_tree of bacteria compared to CK, (P< 0.05, Figure 3E). Two-way ANOVA revealed that only N addition significantly affected Shannon’s index of bacteria (P< 0.05, Supplementary Table S7). Combination of high N and high biochar addition altered bacterial community diversity.
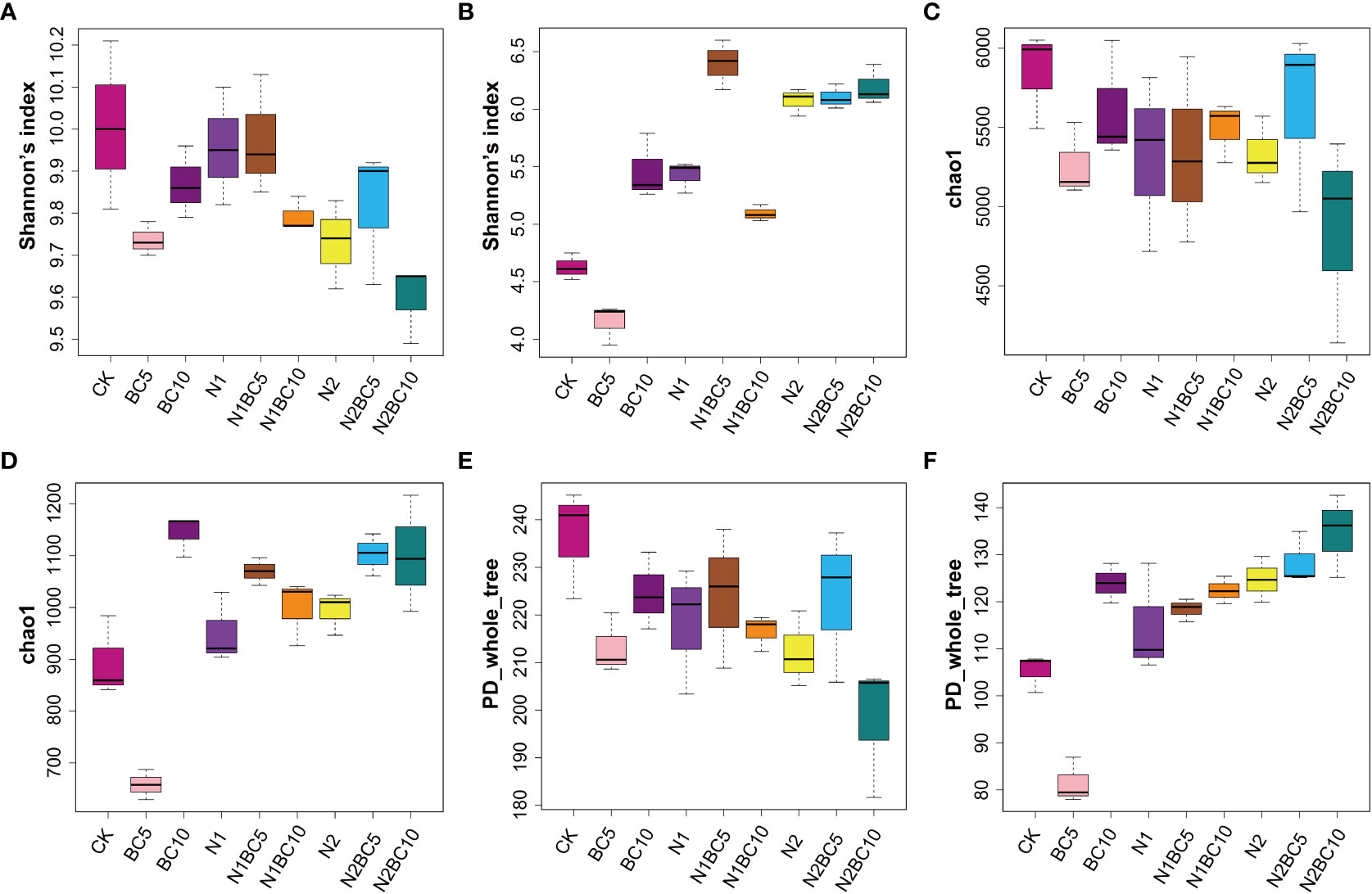
Figure 3 α-diversity indexes of soil bacteria and fungi under different N and biochar additions. Including Shannon's index of bacteria (A) and fungal (B), Chao1 of bacteria (C) and fungal (D), PD_whole_tree of bacteria (E) and fungal (F).
In N-free addition treatments, all of the α-diversity indexes (including Shannon’s index, Chao1 and PD_whole_tree) of fungi followed the significant order: BC10, CK, BC5 (P< 0.05, Figures 3B, D, F). The results showed that low biochar addition reduced fungal diversity, while high biochar addition increased it. In biochar-free amendment treatments, N addition significantly increased the Shannon’s index compared to CK (P< 0.05), which increased with increasing N addition. However, there was no significant effect on Chao1 of fungal (P > 0.05) and only N2 significantly increased PD_whole_tree of fungal compared to CK (P< 0.05). Compared to BC5, both N1BC5 and N2BC5 significantly increased all of the α-diversity indices of fungal (P< 0.05). Two-way ANOVA analysis revealed that N and biochar addition had a significant effect on Shannon’s index, Chao1 and PD_whole_tree of fungi, either separately or in combination (P< 0.05, Supplementary Table S8).
The first and second principal components of the PCA explained 17.95% and 47.94% of the variability of bacterial (Figure 4A) and fungal (Figure 4B) communities, respectively. The bacterial community was irregularly distributed among all treatments. while the fungal community displayed a clear clustering pattern, mainly based on the level of N addition and the interactive effect of N and biochar.
3.4 Relationships between microbial communities and biotic and abiotic environmental factors
As shown in Figure 5A, the bacterial community composition was not correlated with any biotic or abiotic factors. However, the fungal community composition correlated significantly with TN, C/N, TP, NO3−-N, MBN and β-GC. Additionally, MBC correlated positively with SOC, TN and NH4+-N (P< 0.05), while MBN correlated negatively with PPO (P< 0.05). Significant positive correlations were observed between TP and TN as well as NO3−-N (P< 0.05), and pH correlated positively with C/N (P< 0.05). However, there were no significant correlations between the soil enzyme activities studied (P > 0.05). Notably, bacterial diversity was only significantly correlated with TP (Figure 5B), whereas fungal diversity was significantly correlated with TN, TP, NO3−-N and MBN.
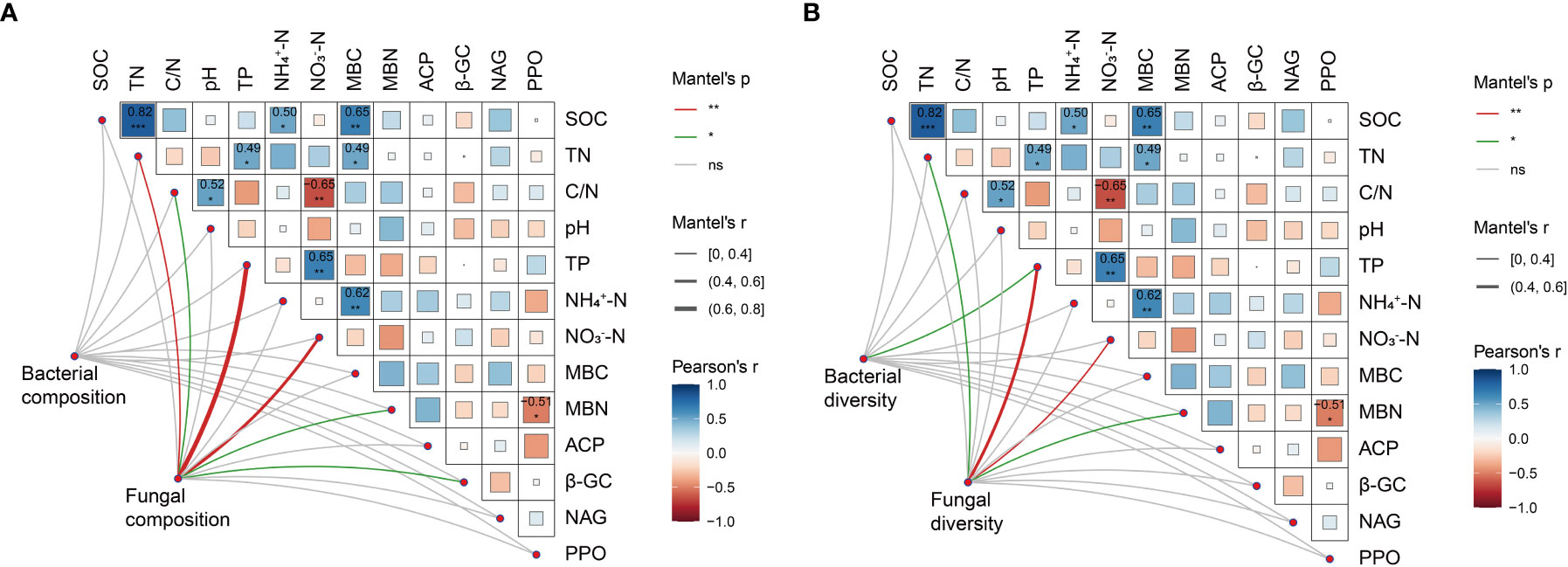
Figure 5 Relationships among soil chemical properties, MBC, MBN, enzyme activities and the composition (A) and diversity (B) of microbial communities. Pearson’s correlations among all environmental factors, Pearson’s r value among all environmental factors, with color gradients indicate significance level (*P< 0.05, **P< 0.01 and ***P< 0.001). Mantel test between environmental factors and microbial phyla composition (A) and microbial diversity (B), where the width and color of the connecting lines indicate Mantel’s P and r values, respectively.
N addition directly positively affected TP, NO3−-N and fungal diversity, it also had a directly negative effect on MBN (Figure 6). There had no significant direct or indirect effects on bacteria diversity among all factors. TN and β-GC positively affected fungal diversity, and ACP positively affected MBN. furthermore, N addition, biochar amendment and environmental factors explained 12% and 72% of the variance in the bacterial and fungal diversity, respectively.
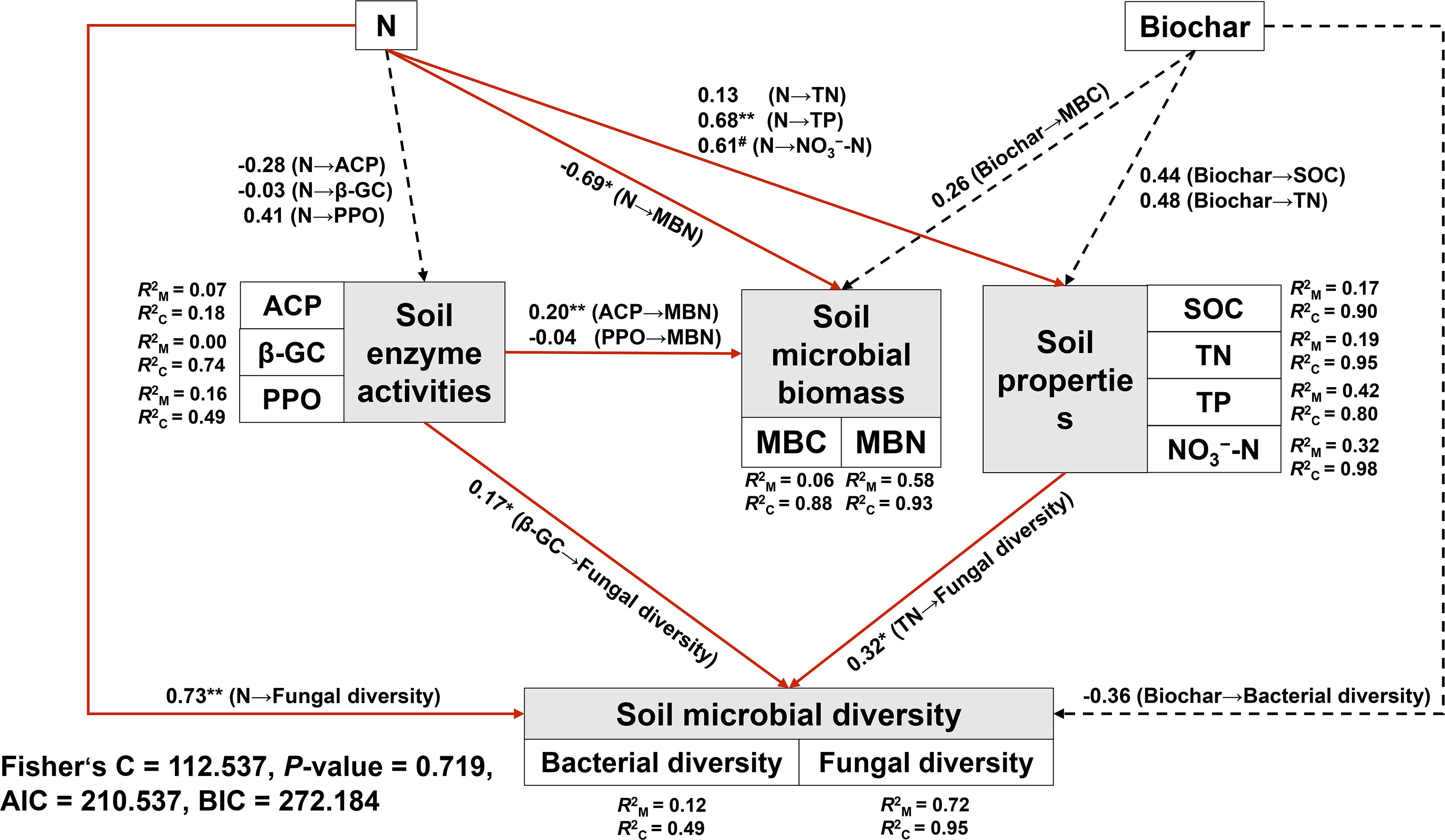
Figure 6 piecewiseSEM (Piecewise structural equation model) illustrating the multivariate influences of N addition, biochar amendment, soil enzyme activities and soil properties on soil microbial biomass and soil microbial diversity. All predictors were treated as separate variables and measured independently, with standardized path coefficients denoted by numbers next to the arrows. Red arrows indicate significant paths, while dashed arrows indicate non-significant paths (P > 0.1). N, nitrogen addition; Biochar, biochar amendment. AIC and BIC (akaike and bayesian information criterion), and (marginal R2 and conditional R2, used to determine the proportion of variance explained by the fixed and random effects). * P< 0.05 (marginally significant), ** P< 0.01 and # 0.05< P< 0.1.
4 Discussion
4.1 Effects of N addition
Previous research has demonstrated that N addition can positively affect N availability (Li et al., 2020) and concentrations of NH4+-N and NO3−-N in subtropical plantation soils (Yu et al., 2018). We observed that simulated N deposition significantly increased soil TN, NO3−-N and TP concentrations, but did not affect NH4+-N concentration in our study (Table 2). Our Mantel tests showed that the changes in chemical properties of the soil caused by the addition of N, especially TN, NO3−-N and TP concentrations, were significantly related to the fungal community composition and diversity (Figure 5). These results suggest that N addition alters TN, NO3−-N and TP concentrations of soil, and the observed changes in these properties may contribute to the mode of action of N on soil fungal communities (Figure 6). Similarly, a simulated N deposition experiment (last 5 years) in a warm temperate coastal wetland also indicated that TN and NO3−-N had greater impact on the microbial communities than NH4+-N (Lu et al., 2021). While the impact of N enrichment on soil microbes was found to be influenced by various factors (Jia et al., 2020), both our result and that of Lu et al. (2021) suggest that the effect of N addition on soil microbial communities maybe achieved by changing TN and NO3−-N. Meanwhile, the above results suggest that the effect of N on these soil N characteristics should be little influenced by climate and soil type, and the relationship between the change and experimental duration and soil type should be further studied. The addition of N may have promoted the increase of fungi with high P acquisition capacity, which in turn increased the availability of soil P (Fan et al., 2020). The increase of soil TP concentration caused by N addition in this experiment may come from the promotion of litter decomposition or the effect on plant roots by N, but our experiment did not cover these two aspects of research. Thus, we do not know where the increased phosphorus comes from, and this part needs further research in follow-up experiments.
The meta-analysis showed that N fertilization inhibited MBC by 9.5% (Jian et al., 2016).Two field experimental studies conducted in a subtropical plantation (Xiao et al., 2020) and in subtropical camphor and pine forests (Yan et al., 2020) in China found that short-term N addition reduced soil MBC concentration, which may be related to changes in soil heterotrophic respiration (Wei et al., 2015). Short-term N addition in L. kaempferi plantations had no significant effect on soil MBC concentration. However, heavy N deposition (100 kg N ha−1 yr−1) significantly reduced soil MBN concentration (Table 3), suggesting that short-term N addition caused a decrease in soil microbial biomass in subtropical L. kaempferi plantations. The results are consistent with those of studies in N-rich tropical forests (Wang et al., 2018). Additionally, heavy N deposition also significantly reduced soil β-GC activity in our short-term experiments, while mantel analysis showed that MBN concentration and β-GC activity were significantly correlated with fungal community composition (Figure 5). In subtropical forests, fungal community was more sensitive to N addition than bacterial community (Wang et al., 2021). We found that the effects of N deposition on soil microbial communities in subtropical L. kaempferi plantations were also mainly due to changes in fungal community composition and diversity. Furthermore, the changes in the fungal communities were significantly related to the changes in TN, -N, TP and MBN and β-GC activity (Figure 5).
4.2 Effects of biochar addition
The results of our research indicate that biochar amendment resulted in a significant increase in soil microbial biomass (MBC and MBN concentrations) in the 0–15cm layer (Table 3). This suggests that biochar application may be beneficial for enhancing soil microbial biomass in the region. Our findings are similar to the application of holm oak biochar in Acrisol soil in a short-term (Teutscherova et al., 2018) and other biochar amendment experiments (Li et al., 2018a; Xu et al., 2018; Ge et al., 2019). However, differences in the effects of biochar addition on soil microbial biomass may be due to the difference in biochar action time and application rate. For example, our microbial biomass was measured after one and a half years of biochar addition, and the sampling time of Ge et al. (2019) is about one year later than ours. Thus, our sampling time and the way in which the biochar was added may be more conducive to the various organic molecules released from the fresh biochar (Lehmann et al., 2011) to act on the soil surface layer and alter its microbial abundance and activity, which is more conducive to the increase of surface soil microbial biomass. And, it should be caused by differences in biochar production methods and feedstock characteristics (Lehmann et al., 2011; Yuan et al., 2018; Zhang et al., 2019). Furthermore, biochar amendment resulted in a significant increase in SOC and TN concentrations, with greater increases observed at higher levels of biochar amendment. These findings are consistent with other studies (Wang et al., 2014; Li et al., 2018b; Ge et al., 2019) and indicate that the addition of biochar may be beneficial for improving soil carbon storage in the plantations. Biochar addition reduced β-GC associated with the decomposition of carbohydrates and cellulose in soil (Li et al., 2018b), it took 10 t ha−1 of biochar addition to significantly affect β-GC and NAG in our experiments.
4.3 Interaction of N addition and biochar amendment
Biochar addition leads to a decrease in soil NO3−-N concentration (Song et al., 2019). Compared to high N addition alone, the combination of N and biochar addition significantly increased TN concentration and decreased NO3−-N concentration, which may be a result of biochar interacting with additional N through its effect on soil N, P and K availability (Zhang et al., 2017), indicates that biochar mitigate the effect of N addition on the elevated soil NO3−-N concentration in L. kaempferi plantation (Table 2). Microbial biomass will increase due to the addition of biochar (Lehmann et al., 2011). Our study found that the combination of N and biochar additions significantly decreased soil MBC concentration compared to biochar amendment treatments, and heavy N and biochar additions combination significantly decreased MBN concentration (Table 3), indicating that N addition inhibited soil microbial biomass increase which caused by biochar addition in the plantation. Furthermore, we observed that heavy N and biochar addition, separately or in combination, significantly decreased soil β-GC activity compared to the control, while the application of biochar in alkaline soils also led to a reduction in β-GC activity (Teutscherova et al., 2018). Biochar addition did not improve or antagonize the effects of N on soil enzymes. These results differ from a previous study, which found that biochar application mitigated the effects of N addition on soil enzyme activities in a subtropical Torreya grandis orchard (Li et al., 2020). Similarly, we found that N addition and biochar amendment had no significant effect on ACP activities both independently and in combination. This contrasts with the results of a study in a Moso bamboo plantation, where biochar amendment alone (40 t ha−1) significantly reduced ACP activities (Peng et al., 2019). The discrepancy in results could be explained by differences in the amount of biochar added, which they had four times more than we did. Finally, we found that only high biochar amendment increased soil NAG compared to the control.
N additions cause soil acidification (Zhang et al., 2017; Zhou et al., 2018; Liu et al., 2020). However, the significant increase in soil pH in this study with low N addition may be due to the elevated soil phosphorus increasing the soil pH (Mao et al., 2017). It was found that the composition and diversity of soil microbial communities, especially bacterial communities, were closely related to soil pH (Li et al., 2018a; Liu et al., 2020; Shuang-Li et al., 2020; Xiao et al., 2020; Zhenjiang et al., 2021). However, our results (Figure 5) differed from the above findings, which may be one of the reasons why the addition of N and biochar had no significant effect on bacterial communities in our study (Liu et al., 2021). As we observed, at the phylum level, there were no significant differences in bacterial taxa among the N addition and biochar amendment treatments (Figure 1; Table S3). However, these bacterial groups have been identified as dominant in other studies (Dai et al., 2018; Li et al., 2018a; Li et al., 2020; Shuai et al., 2021; Wang et al., 2022). Our study suggests that the effects on soil microbial structure caused by N addition and biochar amendment are mainly reflected in changes in the structure of the fungal community. Ascomycota and Basidiomycota could be used as biomarkers of specific successional sequences (Shuai et al., 2021; Wang et al., 2022). In our study, the combination of heavy N and biochar additions resulted in a significant decrease in the relative abundance of Basidiomycota and an increase in Ascomycota, while both heavy N deposition and high biochar amendment independently affected the relative abundance of Basidiomycota and Ascomycota. This suggests that heavy N deposition and biochar amendment may have promoted fungal succession in this L. kaempferi plantation. Furthermore, the combination of heavy N deposition and biochar amendment had a significant impact on the relative abundance of fungi such as Mortierella and Archaeorhizomyces compared to heavy N deposition alone, indicating that the fungal community is more sensitive to N deposition than the bacterial community (Figures 3, 4), which conclusion is consistent with the results of Wang et al. (2021).
Biochar addition improved the effect of N deposition on bacterial community diversity in subtropical plantation forests (Li et al., 2018a; Li et al., 2020), but in our study, biochar addition did not improve the effect of N deposition on bacterial community diversity (Figure 3). In addition, the Shannon’s index for fungi increased with increasing N addition. And compared to low biochar amendment, the treatments with added N significantly increased α-diversity of fungal. These effects indicate that, in line with results from other simulated N deposition experiments (Lilleskov et al., 2019; Liu et al., 2020; Sophia et al., 2020), N is also an important driver of changes in fungal diversity in L. kaempferi plantation.
5 Conclusions
N addition and biochar amendment had different effects under independent and interactive on soil chemical properties and microbial characteristics of L. kaempferi plantation in Shennongjia in short-term. N addition mainly affected soil TN, -N, TP and MBN concentrations and β-GC activity, as well as the fungal community. On the other hand, biochar amendment had obvious effects on increasing SOC, TN and microbial biomass, with high biochar addition increasing fungal community diversity and low biochar addition reducing fungal community diversity. In addition, biochar addition inhibited the increase in soil NO3−-N concentration caused by high N addition in the plantation and influenced the change in composition of the microbial community caused by adding N. Fungal community changes were significantly correlated with soil TN, NO3−-N, TP, MBN concentrations and β-GC activity. The piecewiseSEM showed that N addition affected microbial biomass and fungal diversity directly or indirectly through its effects on soil enzyme activities and soil properties. In contrast, there were no significant direct or indirect effects on bacterial diversity among all factors. Our research will provide a reference for improving soil fertility and coping with possible future increases in N deposition in plantations in this region.
Data availability statement
The datasets presented in this study can be found in online repositories. The name of the repository and accession number can be found below: NCBI; PRJNA972654.
Author contributions
JL and HC provided design ideas, guidance and funding for the study. CH completed the experimental operation, data analysis and manuscript writing. ZM, JG wrote and revised part of the manuscript. All authors participated in the revision and improvement of the manuscript and agreed to submit the final version.
Funding
This research was supported by the Projects of National Forestry and Grassland Administration: Technical support for UNFI Implementation in China, and the Science and Technology Research Project of Hubei Academy of Forestry (2021YGG05)
Acknowledgments
We are very grateful to Jinglong Yang and Guofei Ma for their help with the field work and to Jinlian Huang for her help with the soil experiments.
Conflict of interest
The authors declare that the research was conducted in the absence of any commercial or financial relationships that could be construed as a potential conflict of interest.
The reviewer XG declared a shared affiliation with the authors CH, ZM, JG and JL to the handling editor at the time of review.
Publisher’s note
All claims expressed in this article are solely those of the authors and do not necessarily represent those of their affiliated organizations, or those of the publisher, the editors and the reviewers. Any product that may be evaluated in this article, or claim that may be made by its manufacturer, is not guaranteed or endorsed by the publisher.
Supplementary material
The Supplementary Material for this article can be found online at: https://www.frontiersin.org/articles/10.3389/fevo.2023.1220111/full#supplementary-material
References
Bilgili A. V., Aydemir S., Altun O., Sayğan E. P., Yalçın H., Schindelbeck R. (2019). The effects of biochars produced from the residues of locally grown crops on soil quality variables and indexes. Geoderma 345, 123–133. doi: 10.1016/j.geoderma.2019.03.010
Chang R., Sohi S. P., Jing F., Liu Y., Chen J. (2019). A comparative study on biochar properties and Cd adsorption behavior under effects of ageing processes of leaching, acidification and oxidation. Environ. Pollut. 254 (2019), 113123. doi: 10.1016/j.envpol.2019.113123
Chen J., Luo Y., van Groenigen K. J., Hungate B. A., Cao J., Zhou X., et al. (2018). A keystone microbial enzyme for nitrogen control of soil carbon storage. Sci. Adv. 4 (8), eaaq1689. doi: 10.1126/sciadv.aaq1689
Chen J., van Groenigen K. J., Hungate B. A., Terrer C., van Groenigen J.-W., Maestre F. T., et al. (2020). Long-term nitrogen loading alleviates phosphorus limitation in terrestrial ecosystems. Global Change Biol. 26 (9), 5077–5086. doi: 10.1111/gcb.15218
Dai Z., Su W., Chen H., Barberán A., Zhao H., Yu M., et al. (2018). Long-term nitrogen fertilization decreases bacterial diversity and favors the growth of actinobacteria and proteobacteria in agro-ecosystems across the globe. Global Change Biol. 24 (8), 3452–3461. doi: 10.1111/gcb.14163
Edgar, Robert C. (2013). UPARSE: highly accurate OTU sequences from microbial amplicon reads. Nat. Methods 10 (10), 996–998. doi: 10.1038/nmeth.2604
Fan Y., Yang L., Zhong X., Yang Z., Lin Y., Guo J., et al. (2020). N addition increased microbial residual carbon by altering soil P availability and microbial composition in a subtropical castanopsis forest. Geoderma 375, 114470. doi: 10.1016/j.geoderma.2020.114470
Flechard C. R., Oijen M.V., Cameron D. R., Vries W.D., Ibrom A., Buchmann N., et al. (2020). Carbon–nitrogen interactions in European forests and semi-natural vegetation – Part 2: untangling climatic, edaphic, management and nitrogen deposition effects on carbon sequestration potentials. Biogeosciences 17 (6), 1621–1654. doi: 10.5194/bg-17-1621-2020
Ge X., Cao Y., Zhou B., Wang X., Yang Z., Li M.-H. (2019). Biochar addition increases subsurface soil microbial biomass but has limited effects on soil CO2 emissions in subtropical moso bamboo plantations. Appl. Soil Ecol. 142, 155–165. doi: 10.1016/j.apsoil.2019.04.021
Guerra C. A., Heintz-Buschart A., Sikorski J., Chatzinotas A., Guerrero-Ramírez N., Cesarz S., et al. (2020). Blind spots in global soil biodiversity and ecosystem function research. Nat. Commun. 11 (1), 3870. doi: 10.1038/s41467-020-17688-2
Gul S., Whalen J. K., Thomas B. W., Sachdeva V., Deng H. (2015). Physico-chemical properties and microbial responses in biochar-amended soils: mechanisms and future directions. Agriculture Ecosyst. Environ. 206, 46–59. doi: 10.1016/j.agee.2015.03.015
Hassan M., Liu Y., Naidu R., Parikh S. J., Du J., Qi F., et al. (2020). Influences of feedstock sources and pyrolysis temperature on the properties of biochar and functionality as adsorbents: a meta-analysis. Sci. Total Environ. 744, 140714. doi: 10.1016/j.scitotenv.2020.140714
Janssens I. A., Dieleman W., Luyssaert S., Subke J.-A., Reichstein M., Ceulemans R., et al. (2010). Reduction of forest soil respiration in response to nitrogen deposition. Nat. Geosci. 3 (5), 315–322. doi: 10.1038/ngeo844
Jia X., Zhong Y., Liu J., Zhu G., Shangguan Z., Yan W. (2020). Effects of nitrogen enrichment on soil microbial characteristics: from biomass to enzyme activities. Geoderma 366, 114256. doi: 10.1016/j.geoderma.2020.114256
Jian S., Li J., Chen J., Wang G., Mayes M. A., Dzantor K. E., et al. (2016). Soil extracellular enzyme activities, soil carbon and nitrogen storage under nitrogen fertilization: a meta-analysis. Soil Biol. Biochem. 101, 32–43. doi: 10.1016/j.soilbio.2016.07.003
Johannes L., Markus K. (2015). The contentious nature of soil organic matter. Nature 528 (7580), 60–68. doi: 10.1038/nature16069
Lefcheck J. S., Freckleton R. (2015). piecewiseSEM: piecewise structural equation modelling in r for ecology, evolution, and systematics. Methods Ecol. Evol. 7 (5), 573–579. doi: 10.1111/2041-210x.12512
Lehmann J., Joseph S. (2015). Biochar for environmental management, 2nd edition. London: Routledge. 1–11.
Lehmann J., Rillig M. C., Thies J., Masiello C. A., Hockaday W. C., Crowley D. (2011). Biochar effects on soil biota – a review. Soil Biol. Biochem. 43 (9), 1812–1836. doi: 10.1016/j.soilbio.2011.04.022
Li Q., Lei Z., Song X., Zhang Z., Ying Y., Peng C. (2018a). Biochar amendment decreases soil microbial biomass and increases bacterial diversity in moso bamboo (Phyllostachys edulis) plantations under simulated nitrogen deposition. Environ. Res. Lett. 13 (4), 044029. doi: 10.1088/1748-9326/aab53a
Li Y., Li Y., Chang S. X., Yang Y., Fu S., Jiang P., et al. (2018b). Biochar reduces soil heterotrophic respiration in a subtropical plantation through increasing soil organic carbon recalcitrancy and decreasing carbon-degrading microbial activity. Soil Biol. Biochem. 122, 173–185. doi: 10.1016/j.soilbio.2018.04.019
Li J., Li S., Huang X., Tang R., Zhang R., Li C., et al. (2022a). Plant diversity and soil properties regulate the microbial community of monsoon evergreen broad-leaved forest under different intensities of woodland use. Sci. Total Environ. 821, 153565. doi: 10.1016/j.scitotenv.2022.153565
Li Y., Nie C., Liu Y., Du W., He P. (2019b). Soil microbial community composition closely associates with specific enzyme activities and soil carbon chemistry in a long-term nitrogen fertilized grassland. Sci. Total Environ. 654, 264–274. doi: 10.1016/j.scitotenv.2018.11.031
Li X., Romanyà J., Li N., Xiang Y., Yang J., Han X. (2022b). Biochar fertilization effects on soil bacterial community and soil phosphorus forms depends on the application rate. Sci. Total Environ. 843, 157022. doi: 10.1016/j.scitotenv.2022.157022
Li Q., Song X., Chang S. X., Peng C., Xiao W., Zhang J., et al. (2019a). Nitrogen depositions increase soil respiration and decrease temperature sensitivity in a moso bamboo forest. Agric. For. Meteorology 268, 48–54. doi: 10.1016/j.agrformet.2019.01.012
Li Q., Song X., Yrjälä K., Lv J., Li Y., Wu J., et al. (2020). Biochar mitigates the effect of nitrogen deposition on soil bacterial community composition and enzyme activities in a Torreya grandis orchard. For. Ecol. Manage. 457, 117717. doi: 10.1016/j.foreco.2019.117717
Lilleskov E. A., Kuyper T. W., Bidartondo M. I., Hobbie E. A. (2019). Atmospheric nitrogen deposition impacts on the structure and function of forest mycorrhizal communities: a review. Environ. Pollut. 246, 148–162. doi: 10.1016/j.envpol.2018.11.074
Lingli L., L G. T. (2009). A review of nitrogen enrichment effects on three biogenic GHGs: the CO2 sink may be largely offset by stimulated N2O and CH4 emission. Ecol. Lett. 12 (10), 1103–1117. doi: 10.1111/j.1461-0248.2009.01351.x
Liu W., Jiang L., Yang S., Wang Z., Tian R., Peng Z., et al. (2020). Critical transition of soil bacterial diversity and composition triggered by nitrogen enrichment. Ecology 101 (8), e03053. doi: 10.1002/ecy.3053
Liu S., Sun Z., Tian P., Zhao X., Zhou G., Dietrich P., et al (2022). Temperature legacies predict microbial metabolic quotient across forest biomes. Global Ecol. Biogeography 32 (1), 107–119. doi: 10.1111/geb.13609
Liu S., Wang Z., Niu J., Dang K., Zhang S., Wang S., et al. (2021). Changes in physicochemical properties, enzymatic activities, and the microbial community of soil significantly influence the continuous cropping of Panax quinquefolius L. (American ginseng). Plant Soil 463, 427–446. doi: 10.1007/s11104-021-04911-2
Liu X., Zhang Y., Han W., Tang A., Shen J., Cui Z., et al. (2013). Enhanced nitrogen deposition over China. Nature 494 (7438), 459–462. doi: 10.1038/nature11917
Lu G., Xie B., Cagle G. A., Wang X., Han G., Wang X., et al. (2021). Effects of simulated nitrogen deposition on soil microbial community diversity in coastal wetland of the Yellow River delta. Sci. Total Environ. 757 (143825). doi: 10.1016/j.scitotenv.2020.143825
Mao Q., Lu X., Zhou K., Chen H., Zhu X., Mori T., et al. (2017). Effects of long-term nitrogen and phosphorus additions on soil acidification in an N-rich tropical forest. Geoderma 285, 57–63. doi: 10.1016/j.geoderma.2016.09.017
Mumbi M. P., Eissa N., Noah B. C., Ehsan K., Jean-Eric G., Heimesaat M. M. (2015). Antepartum antibiotic treatment increases offspring susceptibility to experimental colitis: a role of the gut microbiota. PloS One 10 (11), e0142536. doi: 10.1371/journal.pone.0142536
Palviainen M., Berninger F., Bruckman V. J., Köster K., Assumpção C. R. M., Aaltonen H., et al. (2018). Effects of biochar on carbon and nitrogen fluxes in boreal forest soil. Plant Soil 425 (1), 71–85. doi: 10.1007/s11104-018-3568-y
Peng C., Li Q., Zhang Z., Wu Z., Song X., Zhou G., et al. (2019). Biochar amendment changes the effects of nitrogen deposition on soil enzyme activities in a moso bamboo plantation. J. For. Res. 24 (5), 275–284. doi: 10.1080/13416979.2019.1646970
Piao S., Fang J., Ciais P., Peylin P., Huang Y., Sitch S., et al. (2009). The carbon balance of terrestrial ecosystems in China. Nature 458 (7241), 1009–1013. doi: 10.1038/nature07944
Shuai J., Yajuan X., Guancheng L., Chunyi H., Xiaochun W., Guoyong Y., et al. (2021). Changes in soil bacterial and fungal community composition and functional groups during the succession of boreal forests. Soil Biol. Biochem. 161, 108393. doi: 10.1016/j.soilbio.2021.108393
Shuang-Li H., Stephan H., Jun-Jie Y., Seeta S., Hai-Wei W., Zhi-Wei Z., et al. (2020). Increasing rates of long-term nitrogen deposition consistently increased litter decomposition in a semi-arid grassland. New Phytol. 229 (1), 296–307. doi: 10.1111/nph.16854
Smith J. L., Collins H. P., Bailey V. L. (2010). The effect of young biochar on soil respiration. Soil Biol. Biochem. 42 (12), 2345–2347. doi: 10.1016/j.soilbio.2010.09.013
Song W., Feng Y., Wang Z. (2022). Ecological restoration programs dominate vegetation greening in China. Sci. Total Environ. 848, 157729. doi: 10.1016/j.scitotenv.2022.157729
Song Y., Li Y., Cai Y., Fu S., Luo Y., Wang H., et al. (2019). Biochar decreases soil N2O emissions in moso bamboo plantations through decreasing labile N concentrations, N-cycling enzyme activities and nitrification/denitrification rates. Geoderma 348, 135–145. doi: 10.1016/j.geoderma.2019.04.025
Sophia E., Marco F., Jan R. G., Svein S., Arthur G., Peter W., et al. (2020). Nitrogen deposition is the most important environmental driver of growth of pure, even-aged and managed European forests. For. Ecol. Manage. 458, 117726. doi: 10.1016/j.foreco.2019.117762
Tang H., Xiao X., Li C., Pan X., Wang K. (2020). Microbial carbon source utilization in rice rhizosphere and nonrhizosphere soils with short-term manure N input rate in paddy field. Entific Rep. 10 (1), 6487. doi: 10.1038/s41598-020-63639-8
Teutscherova N., Lojka B., Houška J., Masaguer A., Benito M., Vazquez E. (2018). Application of holm oak biochar alters dynamics of enzymatic and microbial activity in two contrasting Mediterranean soils. Eur. J. Soil Biol. 88, 15–26. doi: 10.1016/j.ejsobi.2018.06.002
Tu L.-H., Hu T.-X., Zhang J., Li R.-H., Dai H.-Z., Luo S.-H. (2011). Short-term simulated nitrogen deposition increases carbon sequestration in a Pleioblastus amarus plantation. Plant Soil 340 (1-2), 383–396. doi: 10.1007/s11104-010-0610-0
Van Zwieten L., Kimber S., Morris S., Macdonald L. M., Rust J., Petty S., et al. (2019). Biochar improves diary pasture yields by alleviating P and K constraints with no influence on soil respiration or N2O emissions. Biochar. 1, 115–126. doi: 10.1007/s42773-019-00005-6
Wang L., Deng D., Feng Q., Xu Z., Pan H., Li H. (2022). Changes in litter input exert divergent effects on the soil microbial community and function in stands of different densities. Sci. Total Environ. 845, 157297. doi: 10.1016/j.scitotenv.2022.157297
Wang Z., Li Y., Chang S. X., Zhang J., Jiang P., Zhou G., et al. (2014). Contrasting effects of bamboo leaf and its biochar on soil CO2 efflux and labile organic carbon in an intensively managed Chinese chestnut plantation. Biol. Fertility Soils 50 (7), 1109–1119. doi: 10.1007/s00374-014-0933-8
Wang C., Lu X., Mori T., Mao Q., Zhou K., Zhou G., et al. (2018). Responses of soil microbial community to continuous experimental nitrogen additions for 13 years in a nitrogen-rich tropical forest. Soil Biol. Biochem. 121, 103–112. doi: 10.1016/j.soilbio.2018.03.009
Wang Y., Sheng H. F., He Y., Wu J. Y., Jiang Y. X., Tam F. Y., et al. (2012). Comparison of the levels of bacterial diversity in freshwater, intertidal wetland, and marine sediments by using millions of illumina tags. Appl. Environ. Microbiol. 78, 8264–8271. doi: 10.1128/AEM.01821-12
Wang J., Shi X., Zheng C., Suter H., Huang Z. (2021). Different responses of soil bacterial and fungal communities to nitrogen deposition in a subtropical forest. Sci. Total Environ. 755 (Pt 1), 142449. doi: 10.1016/j.scitotenv.2020.142449
Wei H., Chen X., Xiao G., Guenet B., Vicca S., Shen W. (2015). Are variations in heterotrophic soil respiration related to changes in substrate availability and microbial biomass carbon in the subtropical forests? Sci. Rep. 5 (1), 18370. doi: 10.1038/srep18370
Xiao S., Wang G. G., Tang C., Fang H., Duan J., Yu X. (2020). Effects of one-year simulated nitrogen and acid deposition on soil respiration in a subtropical plantation in China. . Forests 11 (2), 235. doi: 10.3390/f11020235
Xu L., He N. (2020). Nitrogen storage and allocation in China's forest ecosystems. Sci. China (Earth Sciences) 63 (10), 1475–1484. doi: 10.1007/s11430-019-9600-5
Xu Y., Seshadri B., Sarkar B., Wang H., Rumpel C., Sparks D., et al. (2018). Biochar modulates heavy metal toxicity and improves microbial carbon use efficiency in soil. Sci. Total Environ. 621, 148–159. doi: 10.1016/j.scitotenv.2017.11.214
Yan W., Chen X., PENG Y., ZHU F., ZHEN W., ZHANG X. (2020). Response of soil respiration to nitrogen addition in two subtropical forest types. Pedosphere 30 (4), 478–486. doi: 10.1016/S1002-0160(17)60471-5
Yan G., Xing Y., Lv X., Xu L., Zhang J., Dai G., et al. (2019). Effects of artificial nitrogen addition and reduction in precipitation on soil CO2 and CH4 effluxes and composition of the microbial biomass in a temperate forest. Eur. J. Soil Sci. 70 (6), 1197–1211. doi: 10.1111/ejss.12812
Yu G., Cheng S., Fang H., Tian J., Xu M., Yu G., et al. (2018). Responses of soil nitrous oxide flux to soil environmental factors in a subtropical coniferous plantation: a boundary line analysis. Eur. J. Soil Biol. 86, 16–25. doi: 10.1016/j.ejsobi.2018.02.002
Yu G., Jia Y., He N., Zhu J., Chen Z., Wang Q., et al. (2019). Stabilization of atmospheric nitrogen deposition in China over the past decade. Nat. Geosci. 12 (6), 424–429. doi: 10.1038/s41561-019-0352-4
Yuan H.-Y., Ding L.-J., Zama E. F., Liu P.-P., Hozzein W. N., Zhu Y.-G. (2018). Biochar modulates methanogenesis through electron syntrophy of microorganisms with ethanol as a substrate. Environ. Sci. Technol. 52 (21), 12198–12207. doi: 10.1021/acs.est.8b04121
Zama E. F., Reid B. J., Arp H. P. H., Sun G.-X., Yuan H.-Y., Zhu Y.-G. (2018). Advances in research on the use of biochar in soil for remediation: a review. J. Soils Sediments 18 (7), 2433–2450. doi: 10.1007/s11368-018-2000-9
Zhang M., Song G., Gelardi D. L., Huang L., Khan E., Mašek O., et al. (2020). Evaluating biochar and its modifications for the removal of ammonium, nitrate, and phosphate in water. Water Res. 186, 116303. doi: 10.1016/j.watres.2020.116303
Zhang Q., Wang J., Lyu H., Zhao Q., Jiang L., Liu L. (2019). Ball-milled biochar for galaxolide removal: sorption performance and governing mechanisms. Sci. Total Environ. 659, 1537–1545. doi: 10.1016/j.scitotenv.2019.01.005
Zhang W., Yuan Y., Yang S., Huang J., Huang L. (2015). ITS2 secondary structure improves discrimination between medicinal "Mu Tong" species when using DNA barcoding. PloS One 10 (7), e0131185. doi: 10.1371/journal.pone.0131185
Zhang R., Zhang Y., Song L., Song X., Hänninen H., Wu J. (2017). Biochar enhances nut quality of Torreya grandis and soil fertility under simulated nitrogen deposition. For. Ecol. Manage. 391, 321–329. doi: 10.1016/j.foreco.2017.02.036
Zhao X., Tian P., Liu S., Yin P., Sun Z., Wang Q. (2022). Mean annual temperature and carbon availability respectively controlled the contributions of bacterial and fungal residues to organic carbon accumulation in topsoil across China's forests. Global Ecol. Biogeography 32 (1), 120–131. doi: 10.1111/geb.13605
Zhenjiang C., Yuanyuan J., Xiang Y., Xuekai W., Xiuzhang L., Chunjie L., et al. (2021). Gene analysis reveals that leaf litter from epichloë endophyte-infected perennial ryegrass alters diversity and abundance of soil microbes involved in nitrification and denitrification. Soil Biol. Biochem. 154, 108123.
Zhou K., Lu X., Mori T., Mao Q., Wang C., Zheng M., et al. (2018). Effects of long-term nitrogen deposition on phosphorus leaching dynamics in a mature tropical forest. Biogeochemistry 138 (2), 215–224. doi: 10.1007/s10533-018-0442-1
Zhou G., Zhou X., Zhang T., Du Z., He Y., Wang X., et al. (2017). Biochar increased soil respiration in temperate forests but had no effects in subtropical forests. For. Ecol. Manage. 405, 339–349. doi: 10.1016/j.foreco.2017.09.038
Zhu J., Chen Z., Wang Q., Xu L., He N., Jia Y., et al. (2020). Potential transition in the effects of atmospheric nitrogen deposition in China. Environ. pollut. 258, 113739. doi: 10.1016/j.envpol.2019.113739
Keywords: biochar amendment, nitrogen addition, soil microbial properties, soil chemical properties, microbial communities
Citation: Hu C, Ma Z, Gong J, Lei J and Cui H (2023) The responses of soil microbial characteristics to nitrogen addition and biochar amendment in a Larix kaempferi plantation. Front. Ecol. Evol. 11:1220111. doi: 10.3389/fevo.2023.1220111
Received: 10 May 2023; Accepted: 20 June 2023;
Published: 10 July 2023.
Edited by:
Shuai Ouyang, Central South University of Forestry and Technology, ChinaReviewed by:
Xiaogai Ge, Chinese Academy of Forestry, ChinaYelin Zeng, Central South University Forestry and Technology, China
Xinzhang Song, Zhejiang Agriculture and Forestry University, China
Copyright © 2023 Hu, Ma, Gong, Lei and Cui. This is an open-access article distributed under the terms of the Creative Commons Attribution License (CC BY). The use, distribution or reproduction in other forums is permitted, provided the original author(s) and the copyright owner(s) are credited and that the original publication in this journal is cited, in accordance with accepted academic practice. No use, distribution or reproduction is permitted which does not comply with these terms.
*Correspondence: Jingpin Lei, bGVpanBAY2FmLmFjLmNu; Hongxia Cui, Y2h4bGt5QDE2My5jb20=