- 1College of Life Sciences, Xinyang Normal University, Xinyang, China
- 2Henan Dabieshan National Field Observation and Research Station of Forest Ecosystem, Zhengzhou, China
- 3Xinyang Academy of Ecological Research, Xinyang, China
- 4Wuhan Botanical Garden, Chinese Academy of Sciences, Wuhan, China
Soil nitrogen can significantly affect the morphology, biomass, nutrient allocation, and photosynthesis of alien vs. native plants, thereby changing their coexistence patterns; however, the effect of soil nitrogen on the interspecific relationship between alien plants is currently unclear. We conducted a nitrogen addition experiment in a greenhouse to explore the effect of soil nitrogen on the interspecific relationship between invasive alien weed Alternanthera philoxeroides and the noninvasive alien horticultural plant Oxalis articulata. We set three experimental factors—nitrogen treatment, planting type, and species and measured the morphology, biomass, carbon (C) and nitrogen (N) content, physiological traits, and photosynthetic fluorescence of the studied plant species. We then used multi-way ANOVA and multiple comparisons to examine the differences in the above indicators among treatment combinations. We found that, in mixed cultures, nitrogen addition significantly increased the root area of O. articulata by 128.489% but decreased the root length by 56.974% compared with the control, while it significantly increased the root length of A. philoxeroides by 130.026%. Nitrogen addition did not affect the biomass accumulation of these two plant species; however, the biomass and root/shoot ratio of O. articulata were significant higher than those of A. philoxeroides. Nitrogen addition significantly increased the N content of A. philoxeroides by 278.767% and decreased the C:N ratio by 66.110% in mixed cultures. Nitrogen addition caused a significant trade-off between flavonoid and anthocyanin in O. articulata, and decreased the initial fluorescence (F0) and maximal fluorescence (Fm) of A. philoxeroides by 18.649 and 23.507%, respectively, in mixed cultures. These results indicate that nitrogen addition increased the N absorption and assimilation ability of A. philoxeroides in deep soil; furthermore, it significantly enhanced the advantages for O. articulata in terms of morphology, physiological plasticity, and photosynthetic efficiency. In addition, O. articulata had better individual and underground competitive advantages. Under intensified nitrogen deposition, the biotic replacement effect of O. articulata on A. philoxeroides in natural ecosystems could be further enhanced.
1 Introduction
Under rapid global environmental change, the negative effects of plant invasion on the environment, economic development, and human health continue to increase (Wu and Ding, 2019; Fantle-Lepczyk et al, 2022). In natural ecosystems, limited available resources cause intense bio-interactions among plant species that have similar resource requirements, thereby weakening their growth, survival, and reproduction, which leads to interspecific competition (Prass et al., 2022; Tumber-Davila et al., 2022). Invasive plants usually have higher rates of growth, diffusion, and reproduction than native plant species, which can make them easily become the dominant or even constructive species and thus enhance their competitive advantages, and this will strongly affect the community structure and interspecific competition patterns (Gu et al., 2021; Cai et al., 2022). Recent studies have found that the competitive relationship between invasive and native plants can also change with the invasion process and species coevolution (Liu et al, 2018). For instance, the negative effect of the invasive species Heracleum mantegazzianum has decreased over time (Dostal et al., 2013), while the native species Desmodium heterocarpon and Senna tora can replace the invasive species Mikania micrantha in the field due to their strong environmental tolerance (Jia et al., 2022). Exploring the competitive relationships among invasive plants and other plants under environmental changes can help in optimizing the biocontrol measures for plant invasions.
The current amount of atmospheric nitrogen deposition around the globe has increased by four times compared to the 18th century, and China has become the third largest nitrogen deposition area in the world, following closely behind Europe and North America, with a maximum volume of 63.53 kg N ha−1 yr−1 in South China (Lv and Tian, 2007; Liu et al., 2013). Nitrogen is a crucial element that limits plant growth and photosynthesis, and nitrogen supply levels significantly affect the plant invasion process (Pan et al, 2016; Slate et al., 2022). Invasive plants usually have higher nitrogen utilization efficiency due to their high nitrate reductase activity, and thus have higher growth rates than native plant species (Ren et al., 2019; Ren et al., 2022). Previous studies have found that nitrogen addition enhances the competitiveness of some invasive plants, such as Centaurea stoebe, Galinsoga quadriradiata, and Ambrosia artemisiifolia (Liu et al., 2018; Ren et al., 2022; Slate et al., 2022), and promote biomass accumulation in the invasive species Plantago virginica, but has no effect on its native congener P. asiatica (Luo et al., 2020). However, different nitrogen supply levels will change the competitive advantage of invasive plants. For example, the growth advantage of the invasive species Solidago canadensis compared to the native species Artemisia argyi was found to decrease with increasing soil nitrogen availability (Ren et al., 2021), and under low nitrogen levels, the invasive species Robinia pseudoacacia strongly inhibits the growth of native accompanying species Quercus acutissima, while high nitrogen levels reduce the competitiveness of R. pseudoacacia (Lou et al., 2014). Some studies have also found that nitrogen addition inhibits the growth of both invasive and native species (Ross et al., 2011). However, studies of interspecific competition between invasive plants and noninvasive alien plants are relatively rare (but see Wang et al., 2019a). Comparing the different responses of these two plant types to nitrogen addition can help to clarify plant invasion mechanisms and predict the succession dynamics of invaded communities.
Alternanthera philoxeroides, a noxious weed native to South America with clonal growth, has widely invaded many countries including the United States, China, New Zealand, Australia, and India (Wu et al., 2016; Wu et al., 2017a). Due to its strong phenotypic plasticity, A. philoxeroides has invaded terrestrial and aquatic habitats in more than 20 provinces in China, causing serious damage to ecosystem function and native plant diversity (Tanveer et al., 2018; Yan et al., 2020). Previous studies have shown that A. philoxeroides has a strong allelopathic effect on the seed germination, seedling growth, and biomass accumulation of native plants, and the allelopathic effect of the underground parts is higher than that of the aboveground parts (Cui et al., 2022; Hu et al., 2023). Global warming increases the interspecific competitiveness of A. philoxeroides with the native co-occurring species Digitaria sanguinalis (Wu et al., 2017b). Nitrogen addition significantly increases the aboveground biomass accumulation of A. philoxeroides, which benefits its rapidly invasion in new habitats (Wang et al., 2015). A few studies have found that some native plants have a stronger resistance to A. philoxeroides invasion (Wang et al., 2021; Zhang et al., 2021). Like A. philoxeroides, Oxalis articulata is also native to South America; it has strong environmental tolerance and has been introduced into China as a horticultural and ornamental plant, and is now widely used in urban greening, landscape configuration, and ecological corridor construction (Oberlander et al., 2009). O. articulata is a typical noninvasive alien species in China; its strong tuber propagation capacity and developed root system enable it to quickly occupy vacant niches (Shiraishi et al., 2005; Li et al., 2021). In addition, O. articulata has a lower leaf construction cost and allocates more nitrogen to photosynthesis (Feng et al., 2008). In natural ecosystems, O. articulata can coexist with A. philoxeroides in large areas (Figure 1). Based on niche theory, screening noninvasive plants with strong competitiveness to occupy vacant niches, thus controlling replacement by invasive plants, would be beneficial for achieving sustainable ecological management of bioinvasions (Jia et al., 2022).
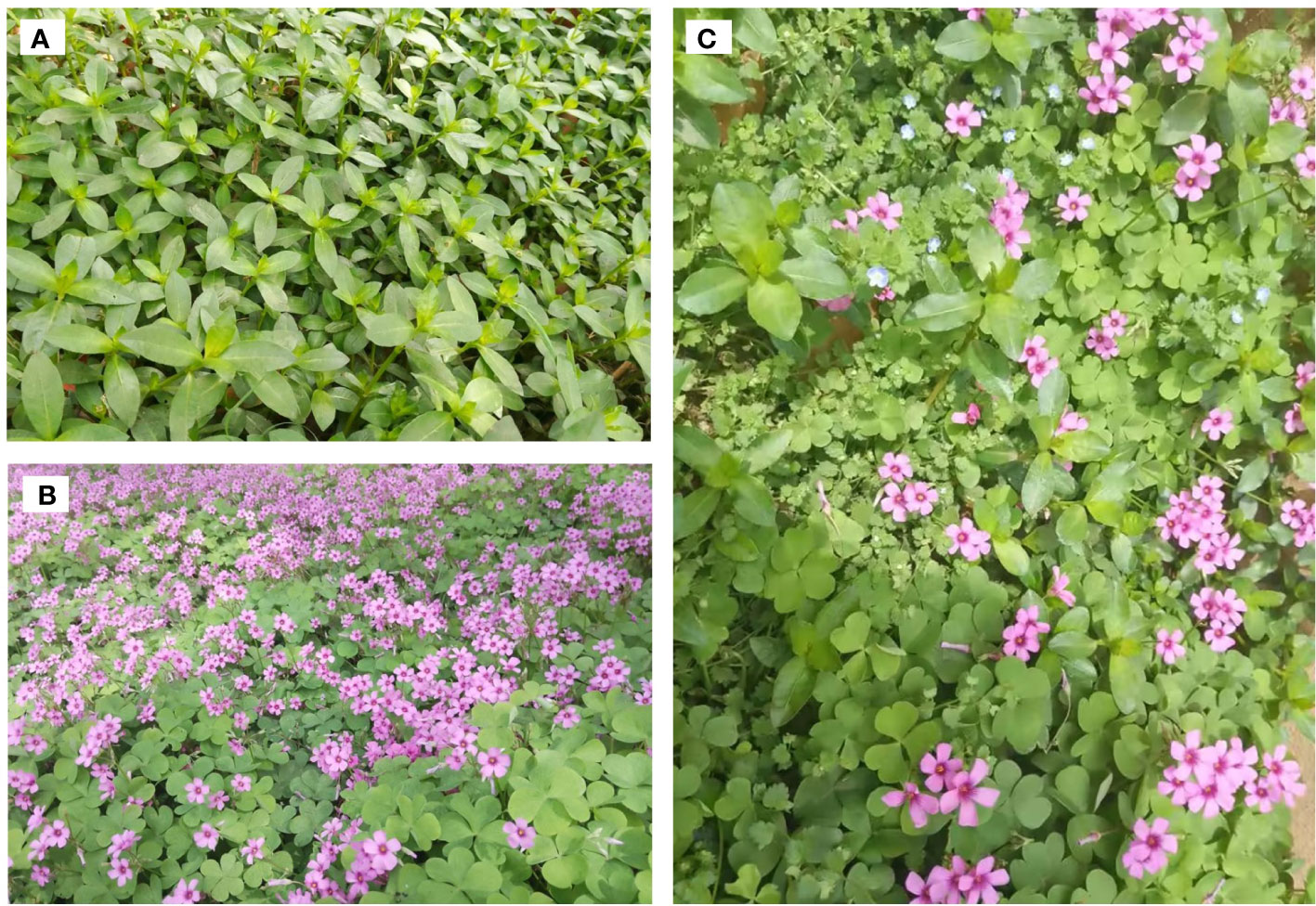
Figure 1 Single-dominant species community of (A) Alternanthera philoxeroides, (B) Oxalis articulata, and (C) their symbiotic communities in natural ecosystem. Photo: Hao Wu; location: roadside of Xinyang City, Henan Province, China.
Currently, there are many studies on the competition between A. philoxeroides and native plants; however, competition between A. philoxeroides and noninvasive alien plants remains unclear, and it is crucial to understand this in order to predict situations of A. philoxeroides under increasing global nitrogen deposition. We conducted a greenhouse experiment to explore the effect of nitrogen addition on the performance of these two plants under different cultivation methods (monocultures and mixed cultures). We hypothesized that nitrogen would significantly affect their interspecific competitiveness. Specifically, we posed the following questions: (1) Do the biomass, nutritional, physiological, and photosynthetic traits of A. philoxeroides and O. articulata cause them to have heterogeneous responses to nitrogen addition? (2) Does nitrogen addition increase the resistance of O. articulata to A. philoxeroides invasion?
2 Materials and methods
2.1 Plant materials
In this study, an original population of A. philoxeroides and tubers of O. articulata were collected from a symbiotic community of these two plants in the field. The method of stem node cutting was used for A. philoxeroides propagation: we cut a 2 cm stem of A. philoxeroides with the node and buried it in a plug for seedling cultivation (Wu et al., 2017b). Healthy tubers of O. articulate were selected from the soil of the symbiotic community for disinfection and sterilization, and we then sowed them in the plug for seedling cultivation (Shiraishi et al., 2005). After A. philoxeroides and O. articulata grew 2 true leaves, the seedlings of 2 plants that had similar growth were transplanted into plastic pots for experimental treatments.
2.2 Experiment design
Three factors were set in the greenhouse experiment: nitrogen treatment (control, nitrogen addition), planting type (monocultures, mixed cultures), and species (A. philoxeroides, O. articulata). Each treatment combination was replicated 5 times. We collected field soil from the A. philoxeroides–O. articulata symbiotic ecosystem (content of ammonium nitrogen, nitrate nitrogen, available phosphorus and available potassium was 103.029, 105.830, 125.724, and 44.667 mg·kg−1, respectively, and pH was 7.611) and mixed it with perlite and sand at a ratio of 4:2:1 to prepare the experimental soil. We sterilized the experimental soil by autoclaving it at 121°C for 120 min in a vertical sterilizer (BKQ-B12011, China) to eliminate the interference of soil microorganism and seeds from other plant species. Based on the de Wit replacement series experiment (Wu et al., 2017b), 2 individuals were planted in each plastic pot (20 cm dia × 17 cm h), and the proportions of O. articulata and A. philoxeroides in the pots were 1:0, 1:1, and 0:1.
All treatment combinations were cultured in a smart greenhouse to eliminate the interference of nitrogen from rainfall. After transplanting all plant seedlings, each pot was covered with a mesh net (30 cm dia × 50 cm h) to prevent herbivores from feeding and to eliminate mutual interference between pots. The cultivation conditions in the greenhouse were set as 14 h light with temperature, humidity, and light intensity of 27 °C, 60%, and 70%, respectively, and 10 h darkness with temperature, humidity, and light intensity of 20 °C, 60%, and 0%, respectively.
We used ammonium nitrate (NH4NO3) for the nitrogen treatment in this study, and the nitrogen addition standard was the maximum deposition amount of 63.53 kg N ha−1 yr−1 in South China (Liu et al., 2013); converted for our experiment, this was 0.570 g NH4NO3 per pot. The NH4NO3 crystals were dissolved in distilled water to prepare the solution, and we added the solution to the experimental treatment pots 3 times (10th, 20th, and 30th day after plants were cultured in the greenhouse), while equal amounts of distilled water were added to the control pots. After nitrogen was added 3 times, we continued to cultivate the plants for 10 days and then measured their various indicators. The entire cultivation time in the greenhouse was 40 days (May 17 to June 27, 2022).
2.3 Data collection
We randomly selected 3 healthy leaves from each plant and measured their nitrogen balance index (NBI), chlorophyll a index (Chla), flavonoid index (Flav), and anthocyanin index (Anth) using a handheld leaf-clip instrument (Dualex, France). After 15 min of adaptation in the dark for the plants in each pot, we used a chlorophyll fluorescence imaging system (FluorCam, Czech Republic) to measure the fluorescence parameters of each plant, including initial fluorescence (F0), maximal fluorescence (Fm), maximum photochemical quantum yield (QY_max), photochemical quenching coefficient (qP_Lss), steady-state fluorescence decay efficiency (Rfd_Lss), and steady-state photochemical quantum (QY_Lss).
We used a flexible ruler to measure the maximum stem length of each plant. We randomly selected 3 healthy leaves from each plant and used the ScanMaker i800 scanner (Microtek, China) to measure their area and took the average value. We dug out all plants from soil and washed them clean, and measured the total root length and area of each plant using the ScanMaker i800 scanner. We then placed the entire plants in a drying oven (Leirun 101-4A, China) at 75 °C for 48 h to constant weight, and measured their total biomass, stem biomass, and root biomass and calculated the root/shoot ratio. After grinding and crushing the plants and passing them through a 70 mesh sieve, we measured their total carbon (C) and total nitrogen (N) content using the vario TOC cube (Elementar, Germany), and calculated the carbon/nitrogen (C:N) ratio.
2.4 Statistical analysis
We conducted multi-way ANOVA using SPSS 16.0 software (SPSS Inc., USA) to examine the effects of nitrogen treatment, planting type, species, and their interactions on the various indicators of O. articulata and A. philoxeroides that were measured. The normality and homogeneity of the data were verified by using the “Homogeneity of variance test”, and data with non-random distribution were square-root transformed before conducting the ANOVA. When the ANOVA indicated significant effects (P < 0.05), the means of treatment combinations were compared using multiple comparisons with Fisher’s protected least significant difference (LSD) test (subset for a = 0.05) in SPSS 16.0. We preferentially analyzed the results with significant three-factor and two-factor interactive effects between nitrogen and other factors and significant single effect of nitrogen treatment.
3 Results
3.1 Effect of nitrogen addition on plant morphology
The interaction of nitrogen treatment, species, and planting type had a significant effect on maximum stem length (F1, 32 = 4.393, P = 0.044) and total root length (F1, 32 = 21.138, P < 0.001). The interaction of nitrogen treatment and species had a significant effect on total root area (F1, 32 = 4.455, P = 0.043). Nitrogen treatment had a significant effect on leaf area (F1, 32 = 24.356, P < 0.001) (Table 1).
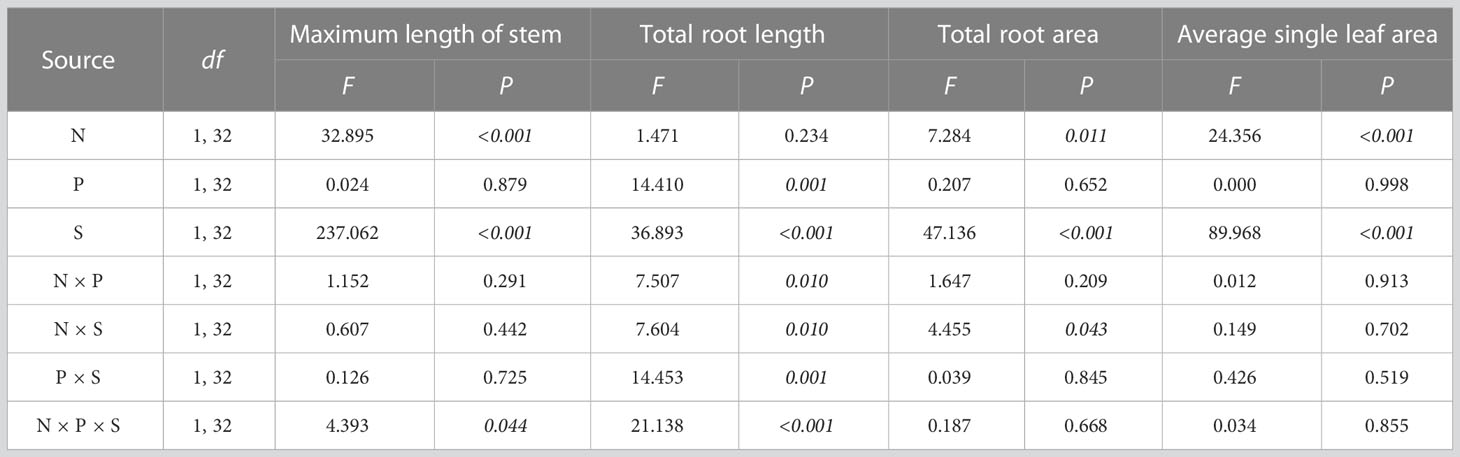
Table 1 Multi-way ANOVA for effects of nitrogen treatment (N), planting type (P), and species (S) and their interactions on plant morphology.
Multiple comparisons showed that nitrogen addition significantly increased the maximum stem length of O. articulata and A. philoxeroides in monocultures by 43.682 and 131.828%, and in mixed cultures by 8.406 and 225.350%, respectively (Figure 2A). In monocultures, nitrogen addition significantly decreased the root length of A. philoxeroides by 7.510%, while in mixed cultures, nitrogen addition significantly decreased the root length of O. articulata by 56.974% but increased the root length of A. philoxeroides by 130.026% (Figure 2B). Nitrogen addition significantly increased the total root area of O. articulata by 47.331 and 128.489% in the monocultures and mixed cultures, respectively (Figure 2C). Nitrogen addition significantly increased the leaf area of plants by 59.110% compared to the control (Figure 2D).
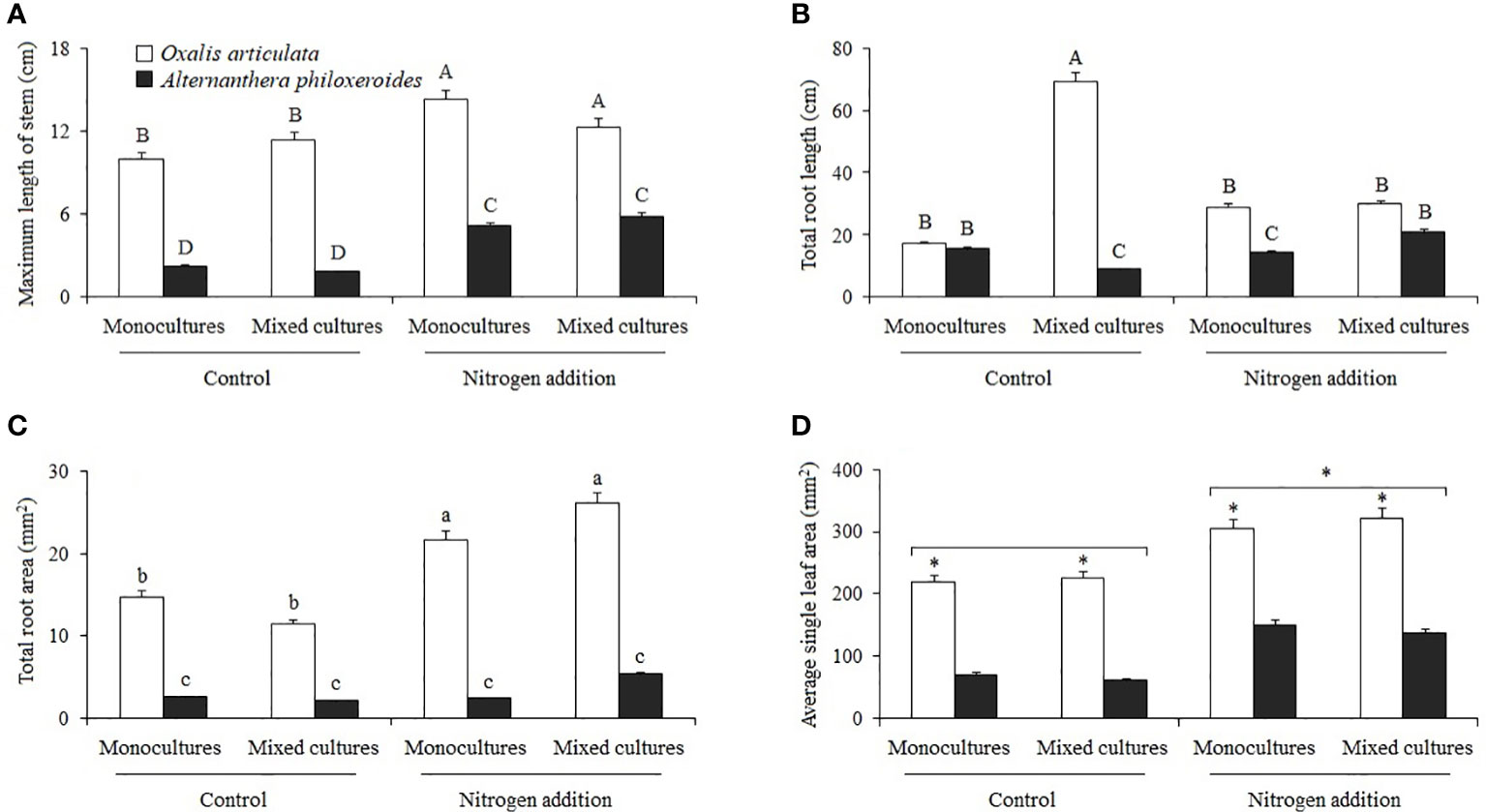
Figure 2 Multiple comparisons of (A) maximum length of stem, (B) total root length, (C) total root area, and (D) average single leaf area among treatments. Different capital letters indicate the significant three-factor interactive effects among nitrogen treatment, planting type, and species (P < 0.05), different lowercase letters indicate significant two-factor interactive effects between nitrogen treatment and other factors (P < 0.05), and stars indicate significant differences between single factors (P < 0.05). The same applies below.
3.2 Effect of nitrogen addition on plant biomass
Nitrogen treatment and its interaction with other factors had no significant effect on plant biomass; the differences in biomass mainly came from the effect of species (Table 2). Multiple comparisons showed that the total biomass, shoot biomass, root biomass, and root/shoot ratio of O. articulata were significantly higher than those of A. philoxeroides by 301.887, 35.289, 563.960, and 469.917%, respectively (Figure 3).
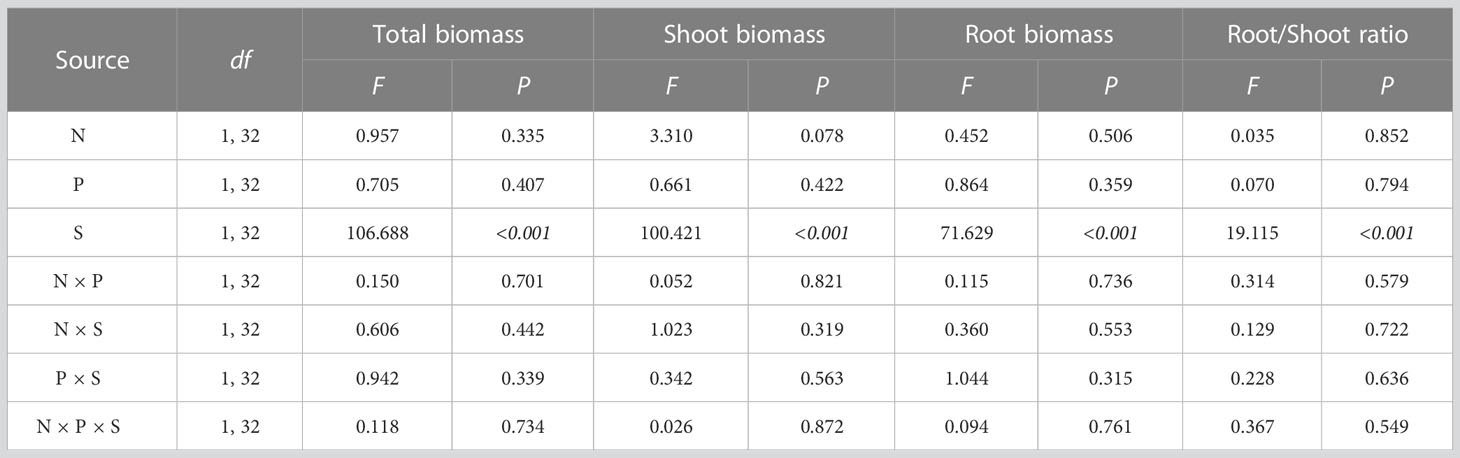
Table 2 Multi-way ANOVA for effects of nitrogen treatment (N), planting type (P), and species (S) and their interactions on plant biomass.
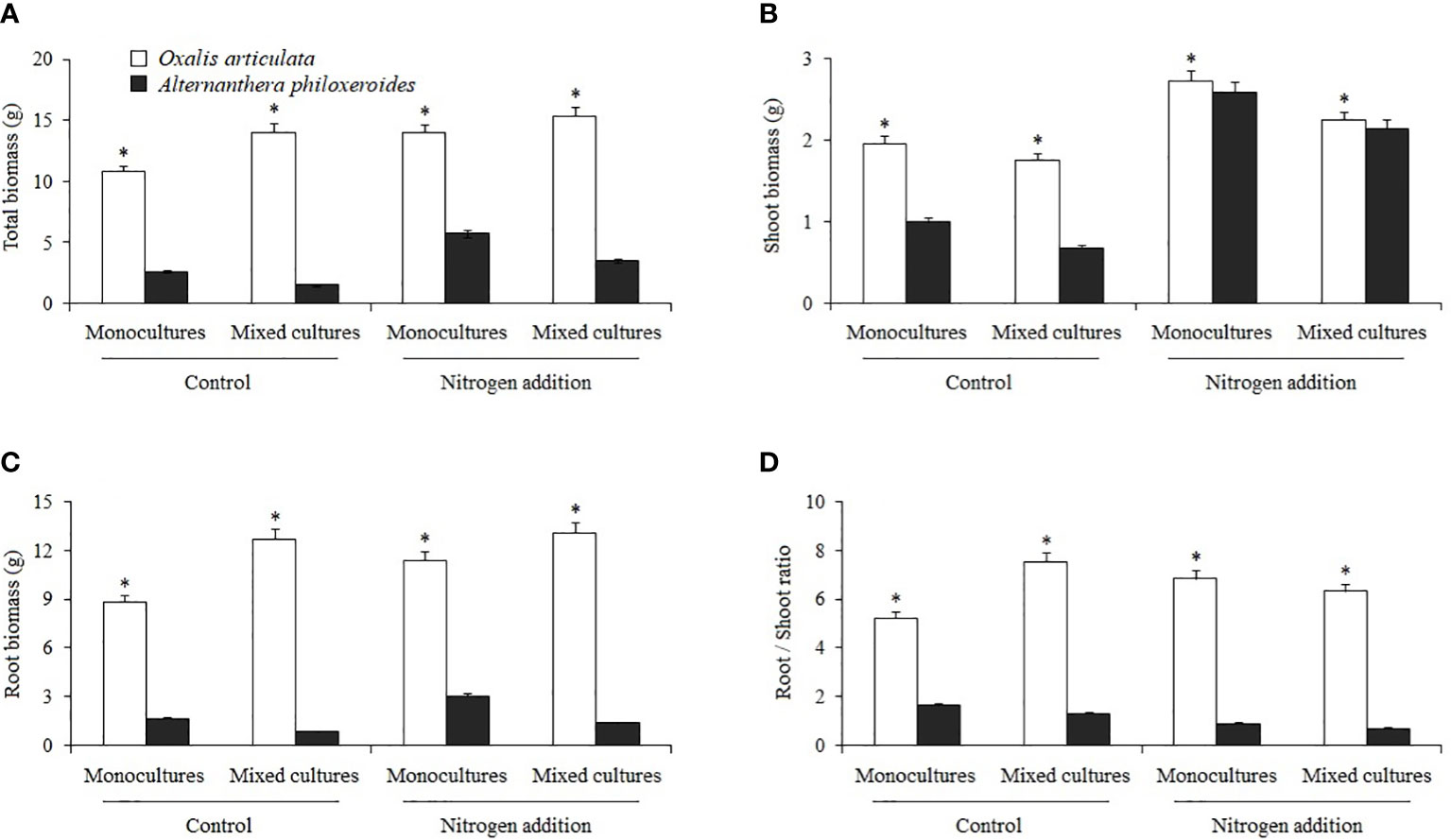
Figure 3 Multiple comparisons of (A) total biomass, (B) shoot biomass, (C) root biomass, and (D) root/shoot ratio among treatments. “*” indicates the significant differences between species (P < 0.05).
3.3 Effect of nitrogen addition on plant nutrients
Nitrogen treatment and its interaction with other factors had no significant effect on total C in plants (Figure 4A). The interaction of nitrogen treatment and species had a significant effect on the total N (F1, 32 = 5.157, P = 0.030) and C:N ratio (F1, 32 = 28.780, P < 0.001) (Table 3). Multiple comparisons showed that nitrogen addition significantly increased the total N in A. philoxeroides by 278.767% compared to the control, and caused A. philoxeroides to have 60.134% more total N than O. articulata the mixed cultures (Figure 4B). Compared to the control, nitrogen addition significantly decreased the C:N ratio of A. philoxeroides by 52.263 and 66.110% in monocultures and mixed cultures, respectively (Figure 4C).
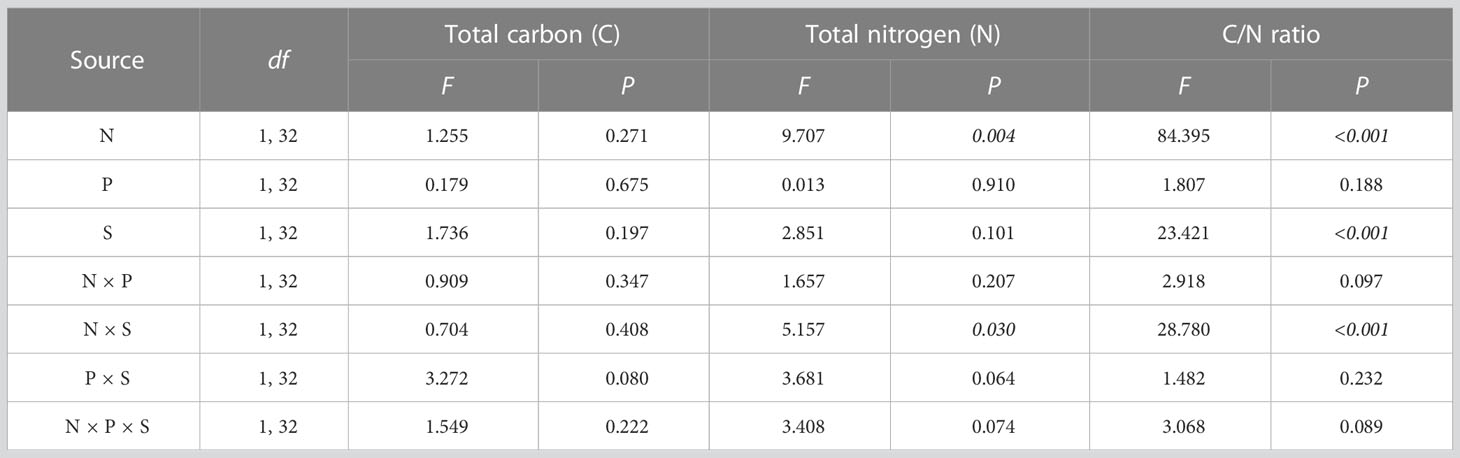
Table 3 Multi-way ANOVA for effects of nitrogen treatment (N), planting type (P), and species (S) and their interactions on plant nutrients.
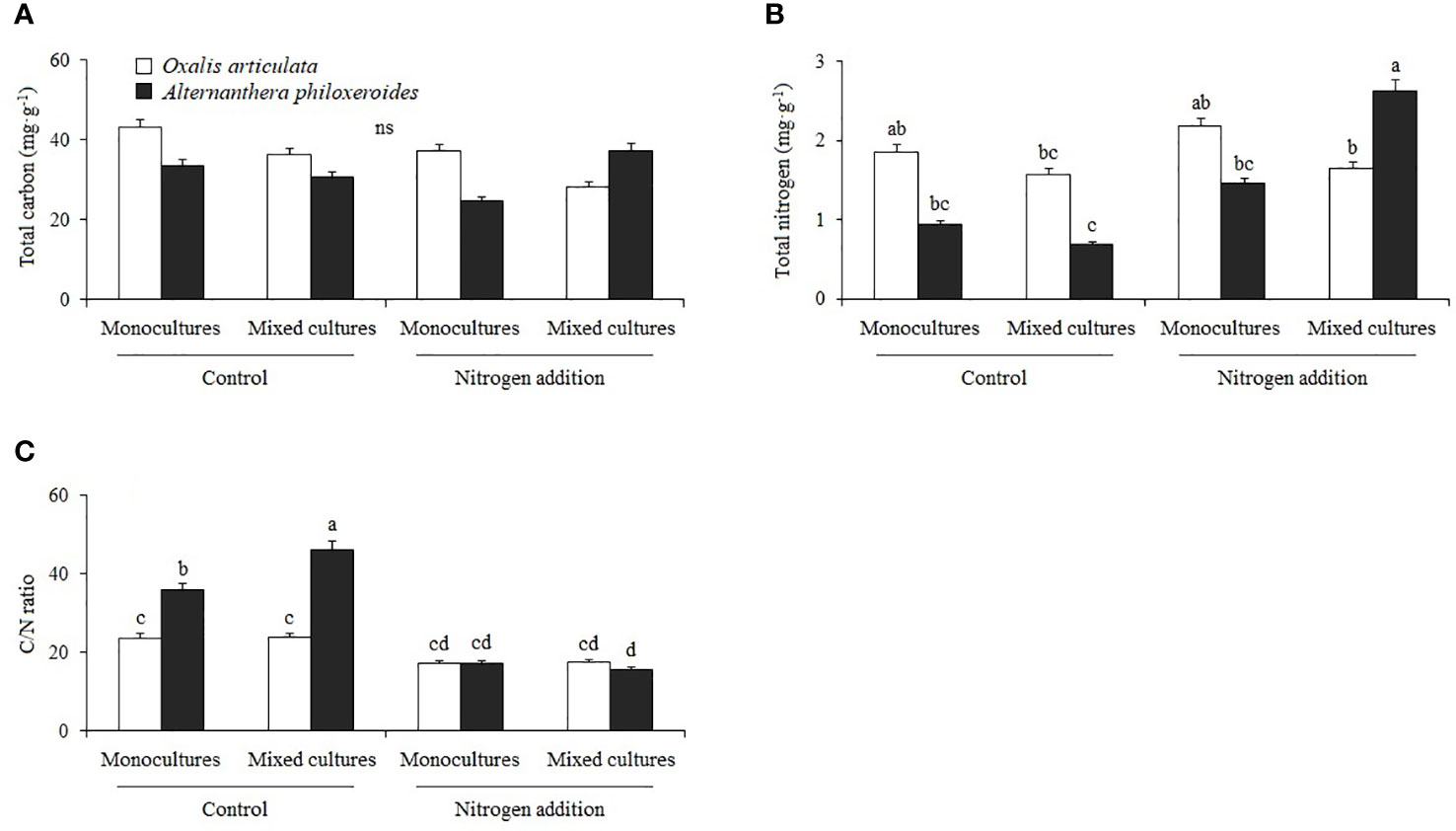
Figure 4 Multiple comparisons of (A) total carbon, (B) total nitrogen, and (C) carbon/nitrogen ratio among treatments. Different lowercase letters indicate significant two-factor interactive effects between nitrogen treatment and species (P < 0.05), and “ns” indicates no significant difference.
3.4 Effects of nitrogen addition on plant physiology
Nitrogen treatment had a significant effect on NBI (F1, 32 = 7.522, P = 0.011). The interaction of nitrogen treatment and species had a significant effect on Flav (F1, 32 = 14.299, P = 0.001) and Anth (F1, 32 = 12.551, P = 0.001). The interaction of nitrogen treatment and other factors had no significant effect on Chla (Table 4; Figure 5B). Multiple comparisons showed that nitrogen addition significantly increased the NBI of plants by 113.526% compared to the control (Figure 5A). Nitrogen addition significantly decreased the Flav of O. articulata by 69.361 and 60.671% in monocultures and mixed cultures, respectively (Figure 5C). Nitrogen addition significantly increased the Anth of O. articulata by 37.931 and 39.091% in monocultures and mixed cultures, respectively (Figure 5D).
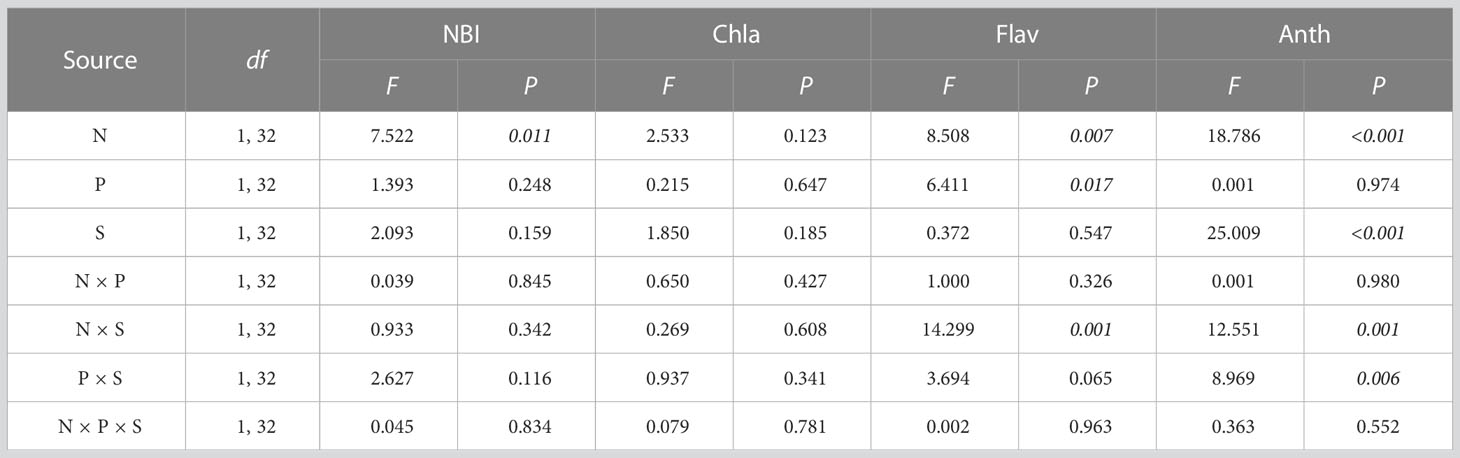
Table 4 Multi-way ANOVA for effects of nitrogen treatment (N), planting type (P), and species (S) and their interactions on plant physiology.
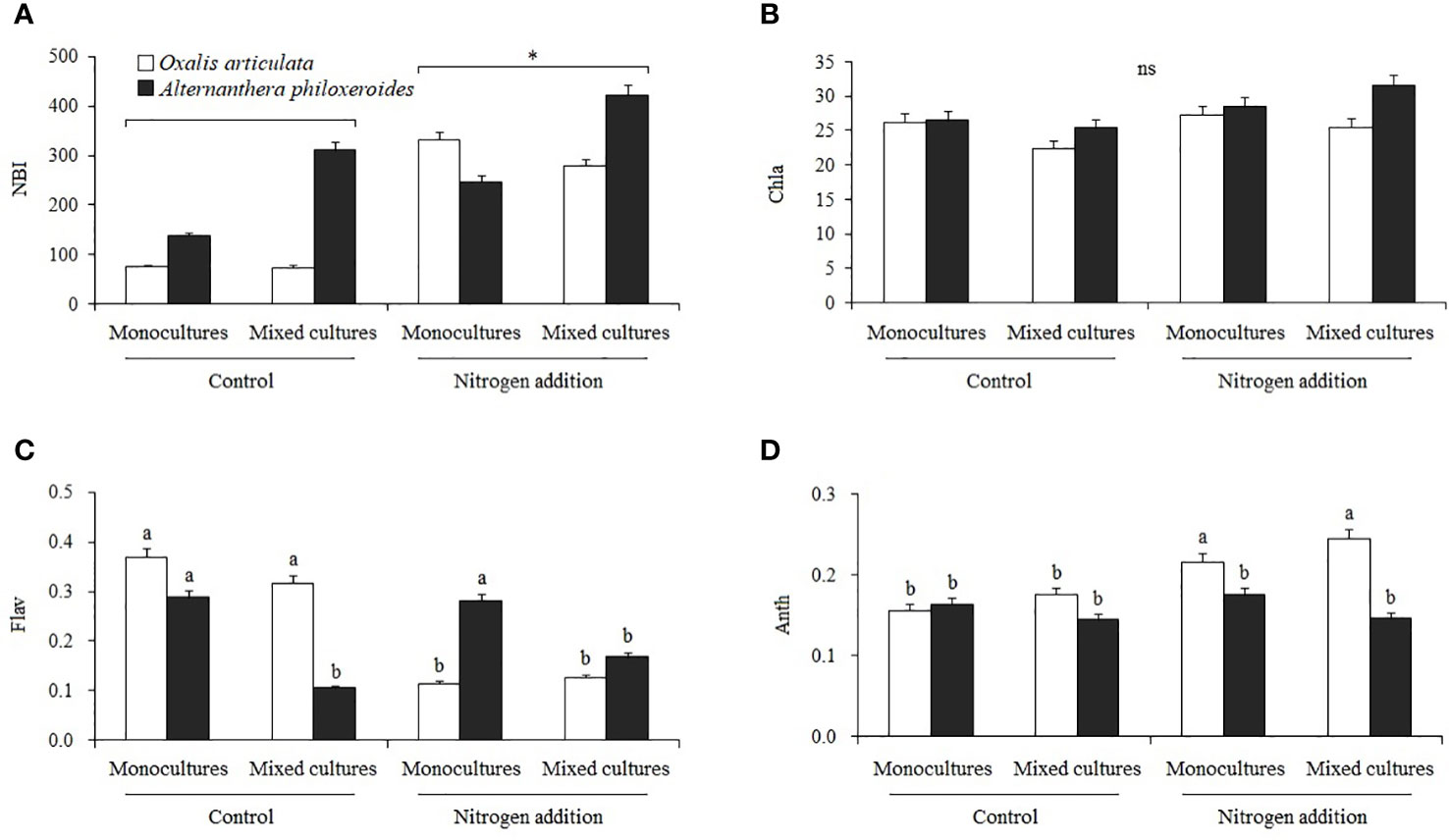
Figure 5 Multiple comparisons of (A) nitrogen balance index (NBI), (B) chlorophyll a index (Chla), (C) flavonoid index (Flav), and (D) anthocyanin index (Anth) among treatments. Different lowercase letters indicate significant two-factor interactive effects between nitrogen treatment and species (P < 0.05), and “*” indicates the significant differences between nitrogen treatment (P < 0.05).
3.5 Effect of nitrogen addition on plant photosynthetic fluorescence
Photosynthetic fluorescence imaging is shown in Figure 6. The interaction of nitrogen treatment and planting type (F1, 32 = 4.630, P = 0.039) and of nitrogen treatment and species (F1, 32 = 5.618, P = 0.024) had a significant effect on F0. The interaction of nitrogen treatment and species had a significant effect on Fm (F1, 32 = 13.880, P = 0.001). Nitrogen treatment had a significant effect on both QY_ max(F1, 32 = 5.818, P = 0.022) and QY_ Lss (F1, 32 = 4.411, P = 0.044). The interaction of nitrogen treatment and other factors had no significant effect on qP_ Lss and Rfd_ Lss (Table 5).
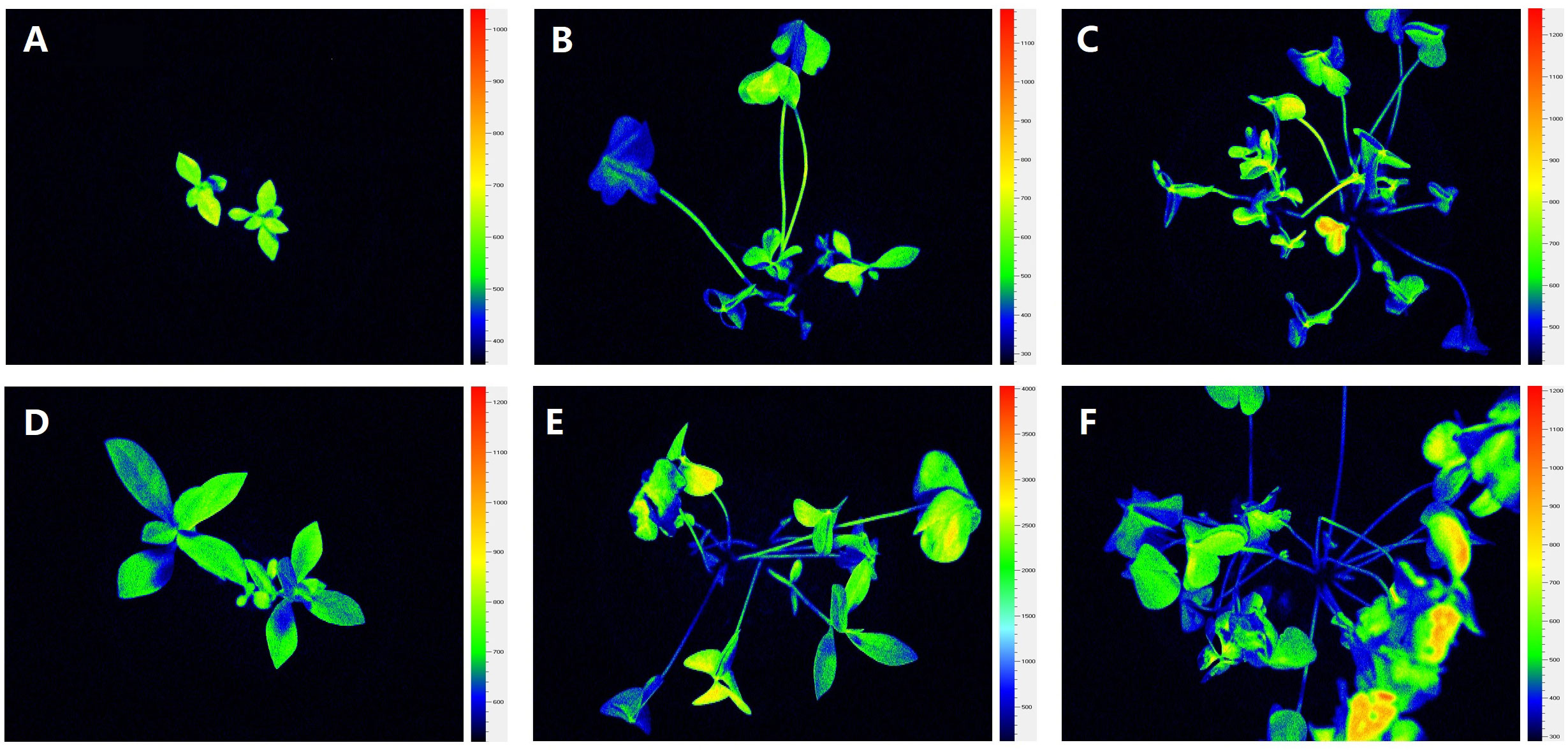
Figure 6 Use of chlorophyll fluorescence imaging in different treatment combinations: (A–C) Alternanthera philoxeroides in monocultures, Oxalis articulata in monocultures, and A. philoxeroides and O. articulata in mixed cultures under control treatment; (D–F) A. philoxeroides in monocultures, O. articulata in monocultures, and A. philoxeroides and O. articulata in mixed cultures under nitrogen addition. Images of leaves of A. philoxeroides and O. articulata show spatiotemporal variation in chlorophyll fluorescence at steady state of induction curve with actinic illumination of 200 μ mol photons m−2 s−1 after 15 min dark adaptation. Color scale to the right of each image indicates range of fluorescence values (from black to pink), with brighter color indicating higher value.
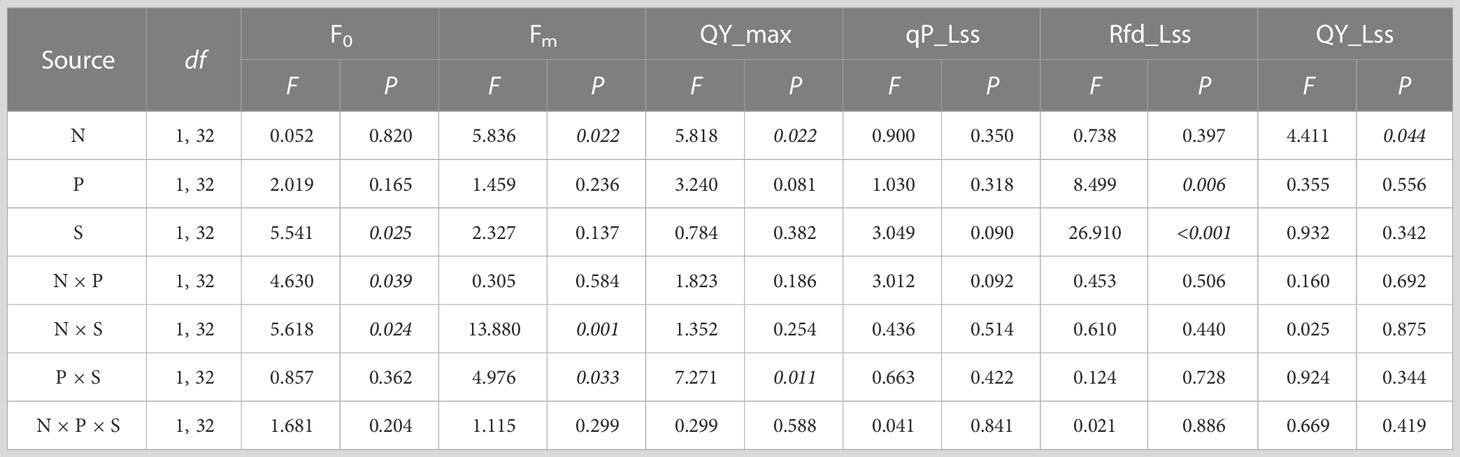
Table 5 Multi-way ANOVA for effects of nitrogen treatment (N), planting type (P), and species (S) and their interactions on plant photosynthetic fluorescence.
Multiple comparisons showed that nitrogen addition significantly decreased the F0 of A. philoxeroides by 18.649% compared to control in mixed cultures (Figure 7A). Compared to control, nitrogen addition significantly decreased the Fm of A. philoxeroides by 16.234 and 23.507% in monocultures and mixed cultures, respectively (Figure 7B). Nitrogen addition significantly decreased the QY_max and QY_Lss by 15.551 and 26.830% compared to control, respectively (Figures 7C–F). The Rfd_Lss of A. philoxeroides was significantly higher than that of O. articulata by 128.368%, and the Rfd_Lss of plants in mixed cultures was significantly higher than that in monocultures by 56.312% (Figure 7E).
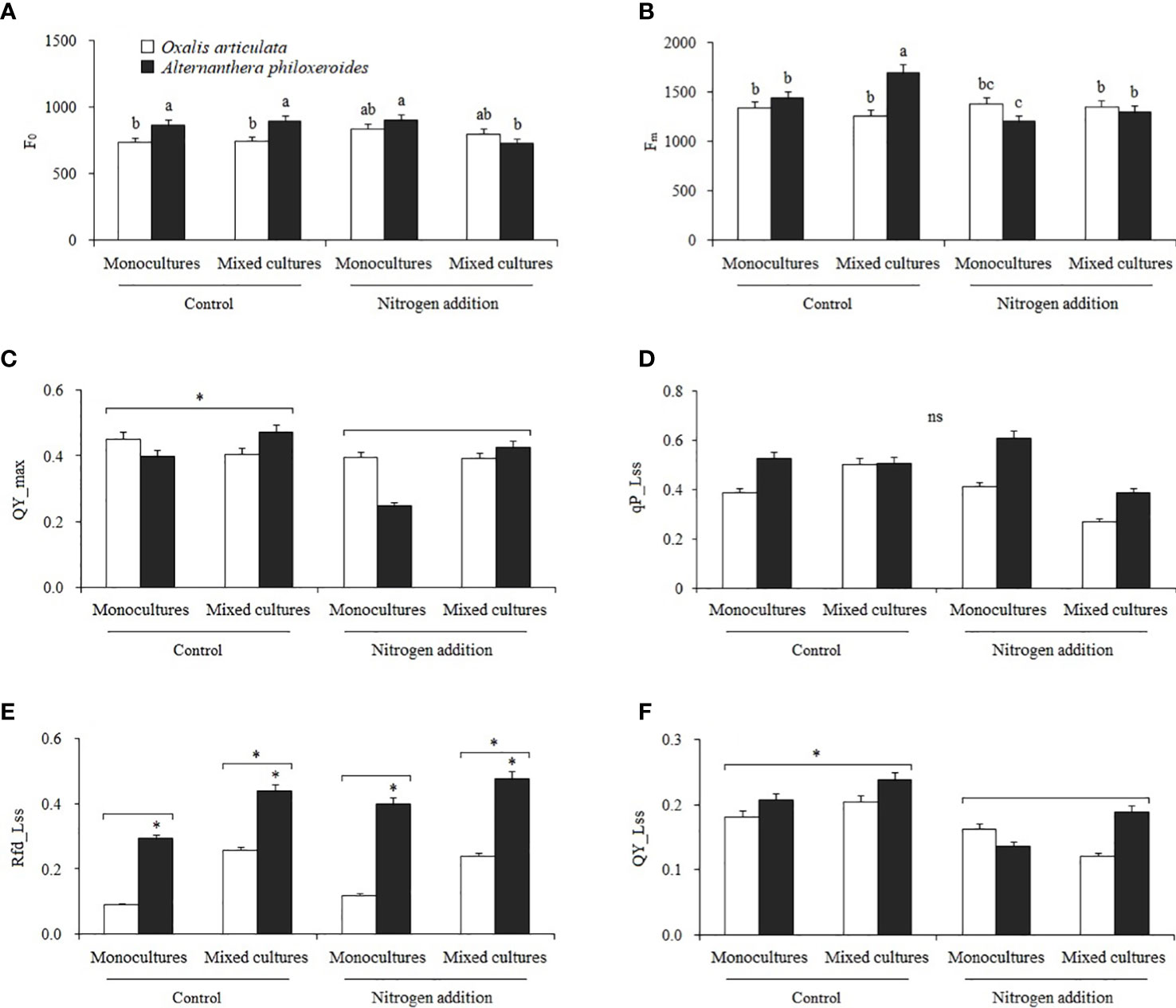
Figure 7 Multiple comparisons of (A) initial fluorescence (F0), (B) maximal fluorescence (Fm), (C) maximum photochemical quantum yield (QY_max), (D) photochemical quenching coefficient (qP_Lss), (E) steady-state fluorescence decay efficiency (Rfd_Lss), and (F) steady-state photochemical quantum (QY_Lss) among treatments. Different lowercase letters indicate significant two-factor interactive effects between nitrogen treatment and species (P < 0.05), “*” indicates significant differences between single factors (P < 0.05), and “ns” indicates no significant difference.
4 Discussion
Soil N enrichment could significantly alter the structure of ecosystems and considered as the third largest killer seriously threatening species diversity, after land-use changes and biotic exchanges (Phoenix et al., 2006). We found that nitrogen addition significantly increased the maximum stem length and leaf area of O. articulata and A. philoxeroides in this study, indicating that it could relieve the constraints of limited resources on the growth of exotic plants and thus benefit their aboveground growth, as well as expand their living space and improve light utilization efficiency (Rickey and Anderson, 2004; Pan et al, 2016; Wang et al, 2020). However, nitrogen addition could also intensify the competition between coexisting plants for light resources (Xiao et al., 2021). The root is an important organ for plants to absorb soil nutrients (Xu et al., 2017), nitrogen addition decreased the root length but increased the root area of O. articulata in mixed cultures, which might be because nitrogen addition increases the availability of nitrogen in deep soil to the plant’s root system thus the plant reduces its resource investment in root length but increases the contact areas between the root and the soil to absorb more nitrogen (Xu et al., 2017; Wang et al., 2020; Zhang et al., 2020a). Overall, nitrogen addition led to greater advantages for morphological traits of O. articulata, but also increased the root length of A. philoxeroides in mixed cultures, indicating that A. philoxeroides tends to absorb more nitrogen in deep soils to resist the competition of O. articulata.
Intuitive impact of interspecific competition on plant growth was preferentially reflected in biomass change (Poorter et al., 2012). Previous studies found that nitrogen addition could significantly promote the biomass accumulation of alien plants and thus rapidly accelerate their invasion process (Davis and Pelsor, 2001; Daehler, 2003). However, in our study we found that nitrogen addition did not increase the biomass of O. articulata and A. philoxeroides. The reason for this might be that although invasive plants usually have higher nitrogen utilization rates, once invaded, they tend to evolve similar nitrogen adaptability to the co-occurring native plant species (Godoy et al., 2012). Particularly, the two plant species in this study were both alien species, thus the effect of nitrogen addition on their biomass allocation was not significant. Similar studies also showed that nitrogen addition did not increase the biomass of some invasive weeds (e.g., Centaurea diffusa) in North America (LeJeune et al., 2006; Cleland et al., 2011). However, we found that the biomass and root/shoot ratio of O. articulata were significantly higher than those of A. philoxeroides, which demonstrates the strong individual and underground competitive advantages of O. articulata.
The C:N ratio reflects the resource utilization strategy and efficiency of plants (Zhang et al., 2020b; Liu et al, 2021a). We found that nitrogen addition significantly increased the N content of A. philoxeroides in mixed cultures, making it higher than that of O. articulata, indicating that A. philoxeroides has stronger N accumulation ability than O. articulata. This is because nitrogen addition increases soil N enrichment and thus might alleviate the interspecific competition between plants (Liu et al., 2020). Moreover, the growth of invasive plants can usually better accumulate the increased soil nutrient pool, which makes them more capable of obtaining N under fluctuating resources (Liu et al., 2019). The C:N ratio of A. philoxeroides was higher than that of O. articulata in the control treatments, indicating that A. philoxeroides tends to evolve higher N utilization efficiency in an N-limited environment. Nitrogen addition decreased the C:N ratio of A. philoxeroides, suggesting that this plant might enhance protein synthesis in vivo at the cost of reducing N utilization efficiency. This trade-off of N utilization in A. philoxeroides in our study strongly supports the adaptive growth hypothesis (AGH), which is that maintaining a high C:N ratio in a low N environment will help invasive plants to improve their nutrient utilization efficiency and prioritize survival, while maintaining a low C:N ratio in a high N environment will enable invasive plants to obtain more resources and achieve rapid growth (Sun et al., 2020; Zhang et al., 2020b; Geng et al., 2023). However, a high N content in plants will increase their palatability and thus their susceptibility to herbivores by changing the interactions with high-trophic-level organisms; this might weaken the defensive ability of A. philoxeroides to resist insect feeding compared to O. articulata in their symbiotic communities (Lu et al., 2015; Liu et al., 2018; Waterton et al., 2022).
NBI, the chlorophyll/flavonoid ratio of plants, is an important indicator for evaluating plant growth (Ilyas et al., 2022). In this study, nitrogen addition led to an increase in NBI, which increased the chlorophyll content of the plants and benefitted their absorption and transformation of light energy, thus enhancing their growth (Deng et al., 2019). Flavonoids are secondary polyphenolic metabolites that exist widely in plants and have strong antibacterial, insect-resistant, and free radical scavenging activity, which can significantly increase the stress resistance and defense ability of plants (Agati et al., 2012; Cesco et al., 2012). Flav is usually used as an important indicator for evaluating the level of plant chemical defense (Agati et al., 2012; Landi et al., 2015). Nitrogen addition significantly decreased the Flav of O. articulata, possibly because the vegetative growth of O. articulata prioritizes its secondary metabolism to obtain more resources when growing in high-nutrient environment, that is, it reduces its chemical defense ability but enhances its growth to resist A. philoxeroides invasion in high-N soil. This also supports the growth-differentiation balance hypothesis (GDBH) regarding plant defense (Liu et al., 2022). In addition, significantly increased rates of plant growth and development and protein synthesis will also inhibit the secondary metabolic pathway of phenylalanine and thus reduce flavonoid synthesis (Zaghdoud et al., 2016; Liu et al., 2021b).
Anthocyanin, a water-soluble glycoside derivative that is widely distributed in plants, plays a crucial role in plants’ resistance to exogenous abiotic stresses (e.g., ultraviolet radiation) and biotic stresses (e.g., insect feeding and interspecific competition) (Gonzalez-Teuber et al., 2017). Landi et al. (2015) found that nitrogen addition can significantly increase the content of anthocyanins, which will absorb excess light energy and dissipate it in the form of heat energy, thus protecting the photosynthetic membrane of plants. In this study, we also found that nitrogen addition significantly increased the Anth of O. articulata, indicating that this alien species could synthesize a large amount of anthocyanins in an N-enriched environment to increase its environmental tolerance, thereby effectively responding to the interspecific competition caused by A. philoxeroides invasion (Zhang et al., 2017). In addition, nitrogen addition caused a significant trade-off between the Flav and Anth of O. articulata, indicating that soil nitrogen enrichment can enhance the physiological plasticity of alien plants and thus increase their competitiveness (Wu et al., 2023). However, nitrogen addition did not have a significant effect on the Flav and Anth of A. philoxeroides in our study, which might be due to its high internal eco-stoichiometric stability (Sun et al., 2019), and this may help A. philoxeroides rapidly evolve suitable growth strategies in environments with different nutrient levels (Geng et al., 2023). Furthermore, global climate changes have exacerbated the escape of A. philoxeroides from the bio-control of its natural enemy Agasicles hygrophila, and it can thus utilize the resources it originally used to resist natural enemies to eliminate the interference of soil N fluctuations on its growth (Lu et al., 2015; Wu et al., 2016).
Chlorophyll fluorescence imaging can comprehensively reflect the primary reaction processes of photosynthesis in plants, such as light energy absorption, excitation energy transfer, and photochemical reactions (Dong et al., 2019). Previous studies showed that applying appropriate amounts of N can increase the chlorophyll content and promote photosynthesis, while N stress (including its concentration and morphology) will lead to a decline in photosynthesis (Chen et al., 2023). In addition, different plants have different levels of energy consumption in the process of absorbing, transporting, and assimilating N, which might lead to excess light energy and accumulation of reactive oxygen (ROS) and thus damage the photosynthetic electron transport chain (Landi et al., 2015; Wang et al., 2019b). F0 and Fm represent the fluorescence yield when the PS II reaction center of plants is fully opened and closed, respectively, and they characterize the relationship between the plant’s physiological status and habitat (Noor et al., 2022). We found that nitrogen addition decreased both F0 and Fm of A. philoxeroides in mixed cultures, and significantly weakened the advantage of F0 and Fm of A. philoxeroides over O. articulata compared with the control, indicating that nitrogen addition reduced the light absorption capacity of A. philoxeroides in interspecific competition. This might be due to the high soil N inhibiting the electron transfer of the plant’s PS II reaction center (Cesco et al., 2012), whereas the PS II reaction center of O. articulata might be more sensitive to irritation, and can effectively respond to high soil N stress by increasing the anthocyanin content to absorb the excess energy that is dissipated in the form of heat and fluorescence under nitrogen addition (Landi et al., 2015).
QY_max represents the maximum potential photochemical quantum efficiency and utilization efficiency of the PS II reaction center, and is an important indicator for evaluating the effect of environmental stress on photosynthesis, while QY_Lss represents the light harvesting ability of plants, which reflects the actual photochemical efficiency of chloroplasts (Fei et al., 2019; Zhang et al., 2021). In our study, nitrogen addition significantly decreased both the QY_max and QY-Lss of plants compared with control, indicating that high soil N weakened the potential vitality of leaves and the electron transfer chain was interrupted due to decreased PS II activity, and the site of injury may be located at the PS II reaction center or primary electron receptor (Singh et al., 2017). qP_Lss represents the portion of light energy absorbed by the PSII reaction center that is actually used for photochemical electron transfer, which reflects the level of plant photosynthetic activity, and Rfd_Lss is used to evaluate the resistance of plants under environmental stress (Kramer et al., 2004). Nitrogen addition did not affect the qP_Lss and Rfd_Lss of O. articulata and A. philoxeroides, indicating that alien plants might have a more stable photosynthetic regulation strategy than native plants under soil nutrient fluctuations (Qing et al., 2012).
5 Conclusions
We found that nitrogen addition significantly increased the maximum stem length, root area, and leaf area of both A. philoxeroides and O. articulata, which was benefitical for enhancing their competitiveness. Nitrogen addition alleviated the nitrogen limitation in soil, leading to increased root length of A. philoxeroides but decreased root length of O. articulata in mixed cultures; however, O. articulata met the demand for nutrients and water by expanding its root area. Nitrogen addition significantly increased the total N content but decreased the C:N ratio of A. philoxeroides. Nitrogen addition significantly decreased Flav but increased Anth of O. articulata in mixed cultures. Nitrogen addition significantly decreased both F0 and Fm in mixed cultures, which relatively the increased photosynthetic efficiency of O. articulata. These results indicate that nitrogen addition significantly affects the nutrient absorption and competition patterns of A. philoxeroides vs. O. articulata, in high soil N environment, A. philoxeroides achieves higher nitrogen enrichment capacity by increasing its root length and rapid growth by reducing its C:N ratio, while O. articulata has higher biomass accumulation and root/shoot ratios, and nitrogen addition relatively increased its root area and photosynthetic fluorescence. Moreover, nitrogen addition also promoted the physiological plasticity in the Flav–Anth trade-off for O. articulata. Therefore, under intensified global nitrogen deposition, the biotic resistance of O. articulata to A. philoxeroides invasion might be further enhanced.
Data availability statement
The original contributions presented in the study are included in the article/supplementary material. Further inquiries can be directed to the corresponding authors.
Author contributions
HW and BR: conceptualization. HW and SD: methodology and formal analysis. SD, LW, YZ, and SJ: investigation and data curation. HW and SD: writing—original draft preparation and writing—review and editing. HW and BR: funding acquisition. All authors contributed to the article and approved the submitted version.
Funding
This work was supported by the National Natural Science Foundation of China (31800460), the Xinyang Academy of Ecological Research Open Foundation (2023XYMS16), the Science and Technology Research Project of Henan Province (232102110062), and the Nanhu Scholars Program for Young Scholars of Xinyang Normal University (XYNU) (2023A017).
Conflict of interest
The authors declare that the research was conducted in the absence of any commercial or financial relationships that could be construed as a potential conflict of interest.
Publisher’s note
All claims expressed in this article are solely those of the authors and do not necessarily represent those of their affiliated organizations, or those of the publisher, the editors and the reviewers. Any product that may be evaluated in this article, or claim that may be made by its manufacturer, is not guaranteed or endorsed by the publisher.
References
Agati G., Azzarello E., Pollastri S., Tattini M. (2012). Flavonoids as antioxidants in plants: location and functional significance. Plant Sci. 196, 67–76. doi: 10.1016/j.plantsci.2012.07.014
Cai M. L., Chen L. H., Ke W. Q., Chen M. H., Zhang J. J., Huang J. D., et al. (2022). Understanding the invasion mechanism of malignant alien weed Mikania micrantha from the perspective of photosynthetic capacity of stems. Biol. Invasions 25 (4), 1–15. doi: 10.1007/s10530-022-02973-6
Cesco S., Mimmo T., Tonon G., Tomasi N., Pinton R., Terzano R., et al. (2012). Plant-borne flavonoids released into the rhizosphere: impact on soil bio-activities related to plant nutrition. a review. Biol. Fertility Soils 48 (2), 123–149. doi: 10.1007/s00374-011-0653-2
Chen H. H., Hu W. L., Wang Y. W., Zhang P., Zhou Y., Yang L. T., et al. (2023). Declined photosynthetic nitrogen use efficiency under ammonium nutrition is related to photosynthetic electron transport chain disruption in citrus plants. Scientia Hortic. 308, 111594. doi: 10.1016/j.scienta.2022.111594
Cleland E. E., Clark C. M., Collins S. L., Fargione J. E., Gough L., Gross K. L., et al. (2011). Patterns of trait convergence and divergence among native and exotic species in herbaceous plant communities are not modified by nitrogen enrichment. J. Ecol. 99 (6), 1327–1338. doi: 10.1111/j.1365-2745.2011.01860.x
Cui Y. Y., Zhang Q. Y., Tang T. W., Deng X. X., Zhang L., Liu P., et al. (2022). Intra- and interspecifific competition altered the competitive strategies of Alternanthera philoxeroides and Trifolium regens under cadmium contamination. Forests 13 (12), 2105. doi: 10.3390/f13122105
Daehler C. C. (2003). Performance comparisons of co-occurring native and alien invasive plants: implications for conservation and restoration. Annu. Rev. Ecol. Evol. Systematics 34, 183–211. doi: 10.1146/annurev.ecolsys.34.011802.132403
Davis M. A., Pelsor M. (2001). Experimental support for a resource-based mechanistic model of invasibility. Ecol. Lett. 4 (5), 421–428. doi: 10.1046/j.1461-0248.2001.00246.x
Deng B. L., Liu X. S., Zheng L. Y., Liu Q., Guo X. M., Zhang L., et al. (2019). Effects of nitrogen deposition and UV-b radiation on seedling performance of Chinese tallow tree (Triadica sebifera): a photosynthesis perspective. For. Ecol. Manage. 433, 453–458. doi: 10.1016/j.foreco.2018.11.038
Dong Z. F., Men Y. H., Li Z. M., Zou Q. Y., Ji J. W. (2019). Chlorophyll fluorescence imaging as a tool for analyzing the effects of chilling injury on tomato seedlings. Scientia Hortic. 246, 490–497. doi: 10.1016/j.scienta.2018.11.019
Dostal P., Mullerova J., Pysek P., Pergl J., Klinerova T. (2013). The impact of an invasive plant changes over time. Ecol. Lett. 16 (10), 1277–1284. doi: 10.1111/ele.12166
Fantle-Lepczyk J. E., Haubrock P. J., Kramer A. M., Cuthbert R. N., Turbelin A. J., Crystal-Ornelas R., et al. (2022). Economic costs of biological invasions in the united states. Sci. Total Environ. 806, 151318. doi: 10.1016/j.scitotenv.2021.151318
Fei C., Su J. X., Li Y. Y., Li Z. F., Wang K. Y., Fan H., et al. (2019). Light-response characteristics of photosynthesis of drip-irrigated sugar beet under different nitrogen fertilizer managements. Photosynthetica 57 (3), 804–811. doi: 10.32615/ps.2019.089
Feng Y. L., Fu G. L., Zheng Y. L. (2008). Specific leaf area relates to the differences in leaf construction cost, photosynthesis, nitrogen allocation, and use efficiencies between invasive and noninvasive alien congeners. Planta 228 (3), 383–390. doi: 10.1007/s00425-008-0732-2
Geng Q. H., Ma X. C., Peng F. X., Zhu Z., Li Q., Xu D. D., et al. (2023). Consistent responses of the c: n: p stoichiometry of green leaves and fine roots to n addition in poplar plantations in eastern coastal China. Plant Soil 485, 377–394. doi: 10.1007/s11104-022-05836-0
Godoy O., Valladares F., Castro-Diez P. (2012). The relative importance for plant invasiveness of trait means, and their plasticity and integration in a multivariate framework. New Phytol. 195 (4), 912–922. doi: 10.1111/j.1469-8137.2012.04205.x
Gonzalez-Teuber M., Quiroz C., Concha-Bloomfield I., Cavieres L.. (2017). Enhanced fitness and greater herbivore resistance: implications for dandelion invasion in an alpine habitat. Biol. Invasions 19 (2), 647–653. doi: 10.1007/s10530-016-1309-9
Gu C. J., Tu Y. I., Liu L. S., Bo W., Zhang Y. L., Yu H. B., et al. (2021). Predicting the potential global distribution of Ageratina adenophora under cur-rent and future climate change scenarios. Ecol. Evol. 11 (17), 12092–12113. doi: 10.1002/ece3.7974
Hu S. Y., Gao H., Li J., Wang Y. H., Gao A. G., Wen J. H., et al. (2023). The latitudinal and longitudinal allelopathic patterns of an invasive alligator weed (Alternanthera philoxeroides) in China. PloS One 18 (1), e0280866. doi: 10.1371/journal.pone.0280866
Ilyas M., Shah S. K. W., Lai Y. W., Sher J., Bai T., Zaman F., et al. (2022). Leaf functional traits of invasive grasses conferring high-cadmium adaptation over natives. Front. Plant Sci. 13, 869072. doi: 10.3389/fpls.2022.869072
Jia P., Wang J. Y., Liang H. L., Wu Z. H., Li F. L., Li W. H. B, et al. (2022). Replacement control of Mikania micrantha in orchards and its eco-physiological mechanism. Front. Ecol. Evol. 10, 1095946. doi: 10.3389/fevo.2022.1095946
Kramer D. M., Johnson G., Kiirats O., Edwards G. E. (2004). New fluorescence parameters for the determination of Q(A) redox state and excitation energy fluxes. Photosynthesis Res. 79 (2), 209–218. doi: 10.1023/B:PRES.0000015391.99477.0d
Landi M., Tattini M., Gould K. S. (2015). Multiple functional roles of anthocyanins in plant-environment interactions. Environ. Exp. Bot. 119, 4–17. doi: 10.1016/j.envexpbot.2015.05.012
LeJeune K. D., Suding K. N., Seastedt T. R. (2006). Nutrient availability does not explain invasion and dominance of a mixed grass prairie by the exotic forb Centaurea diffusa lam. Appl. Soil Ecol. 32 (1), 98–110. doi: 10.1016/j.apsoil.2005.01.009
Li J. X., Chen L., Chen Q. H., Miao Y. H., Peng Z., Huang B. S., et al. (2021). Allelopathic effect of Artemisia argyion the germination and growth of various weeds. Sci. Rep. 11 (1), 4303. doi: 10.1038/s41598-021-83752-6
Liu W. X., Feng Y., Yu S. H., Fan Z. Q., Li X. L., Li J. Y., et al. (2021b). The flavonoid biosynthesis network in plants. Int. J. Mol. Sci. 22 (23), 12824. doi: 10.3390/ijms222312824
Liu J. G., Liu W. G., Long X. E., Chen Y. G., Huang T. W., Huo J. S., et al. (2020). Effects of nitrogen addition on c: n: p stoichiometry in moss crust-soil continuum in the n-limited gurbantunggut desert, Northwest China. Eur. J. Soil Biol. 98, 103174. doi: 10.1016/j.ejsobi.2020.103174
Liu Y. Y., Luo W. M., Mu G. T., Wu X. L., Su S. C., Zhang Z. M.. (2021a). C:N:P stoichiometric characteristics of the soil–vegetation system of three rare tree species growing on mount fanjing in southwest China. Global Ecol. Conserv. 32, e01893. doi: 10.1016/j.gecco.2021.e01893
Liu Y. Y., Sun Y., Muller-Scharer H., Yan R., Zhou Z. X., Wang Y. J., et al. (2019). Do invasive alien plants differ from non-invasives in dominance and nitrogen uptake in response to variation of abiotic and biotic environments under global anthropogenic change? Sci. Total Environ. 672, 634–642. doi: 10.1016/j.scitotenv.2019.04.024
Liu G., Yang Y. B., Zhu Z. H. (2018). Elevated nitrogen allows the weak invasive plant Galinsoga quadriradiata to become more vigorous with respect to inter-specific competition. Sci. Rep. 8 (1), 1–8. doi: 10.1038/s41598-018-21546-z
Liu X. J., Zhang Y., Han W. H., Tang A. H., Shen J. L., Cui Z. L., et al. (2013). Enhanced nitrogen deposition over China. Nature 494 (7438), 459–462. doi: 10.1038/nature11917
Liu M., Zhou F., Pan X. Y., Zhang Z. J., Li B. (2022). Effects of soil nitrogen levels on growth and defense of the native and introduced genotypes of alligator weed. J. Plant Ecol. 15 (3), 461–472. doi: 10.1093/jpe/rtab068
Lou Y. J., Gou W. H., Yuan Y. F., Liu J., Du N., Wang R. Q. (2014). Increased nitrogen deposition alleviated the competitive effect of the introduce invasive plant Robinia pseudoacacia on the native tree quercus acutissima. Plant Soil 385, 63–75. doi: 10.1007/s11104-014-2227-1
Lu X. M., Siemann E., Wei H., Shao X., Ding J. Q. (2015). Effects of warming and nitrogen on above-and below-ground herbivory of an exotic invasive plant and its native congener. Biol. Invasions 17 (10), 2881–2892. doi: 10.1007/s10530-015-0918-z
Luo X., Zheng Y., Xu X. H., Xiao R., Guo H. (2020). The impacts of warming and nitrogen addition on competitive ability of native and invasive populations of plantago virginica. J. Plant Ecol. 13 (6), 676–682. doi: 10.1093/jpe/rtaa055
Lv C. Q., Tian H. Q. (2007). Spatial and temporal patterns of nitrogen deposition in China: synthesis of observational data. J. Geophysical Research-Atmospheres 112, 1–10. doi: 10.1029/2006JD007990
Noor H., Sun M., Algwaiz H. I. M., Sher A., Fiaz S., Attia K.A., et al. (2022). Chlorophyll fluorescence and grain filling characteristic of wheat (Triticum stivum l.) in response to nitrogen application level. Mol. Biol. Rep. 49 (7), 7157–7172. doi: 10.1007/s11033-022-07612-w
Oberlander K. C., Emshwiller E., Bellstedt D. U., Dreyer L. L. (2009). A model of bulb evolution in the eudicot genus Oxalis (Oxalidaceae). Mol. Phylogenet. Evol. 51, 54–63. doi: 10.1016/j.ympev.2008.11.022
Pan Y. M., Tang S. C., Wei C. Q., Li X. Q. (2016). Effects of global risks-nitrogen additions on growth and competitive relations among invasive and native congeneric species-bidens frondosa. Polish J. Ecol. 64 (4), 443–452. doi: 10.3161/15052249PJE2016.64.4.001
Phoenix G. K., Hicks W. K., Cinderby S., Kuylenstierna J. C. I., Stock W. D., Dentener F. J., et al. (2006). Atmospheric nitrogen deposition in world biodiversity hotspots: the need for a greater global perspective in assessing n deposition impacts. Global Change Biol. 12 (3), 470–476. doi: 10.1111/j.1365-2486.2006.01104.x
Poorter H., Niklas K. J., Reich P. B., Oleksyn J., Poot P., Mommer L.. (2012). Biomass allocation to leaves, stems and roots: meta-analyses of interspecific variation and environmental control. New Phytol. 193 (1), 30–50. doi: 10.1111/j.1469-8137.2011.03952.x
Prass M., Ramula S., Jauni M., Setala H., Kotze D. J. (2022). The invasive herb Lupinus polyphyllus can reduce plant species richness independently of local invasion age. Biol. Invasions 24 (2), 425–436. doi: 10.1007/s10530-021-02652-y
Qing H., Cai Y., Xiao Y., Yao Y. H., An S. Q. (2012). Leaf nitrogen partition between photosynthesis and structural defense in invasive and native tall form Spartina alterniflora populations: effects of nitrogen treatments. Biol. Invasions 14 (10), 2039–2048. doi: 10.1007/s10530-012-0210-4
Ren G. Q., Li Q., Li Y., Li J., Adomako M. O., Dai Z. C., et al. (2019). The enhancement of root biomass increases the competitiveness of an invasive plant against a co-occurring native plant under elevated nitrogen deposition. Flora 261, 151486. doi: 10.1016/j.flora.2019.151486
Ren G. Q., Yang B., Cui M. M., Dai Z. C., Xiang Y., Zhang H.Y., et al. (2022). Warming and elevated nitrogen deposition accelerate the invasion process of Solidago canadensis l. Ecol. Processes 11, 62. doi: 10.1186/s13717-022-00407-8
Ren G. Q., Zou C. B., Wan L. Y., Johnson J.H., Li J., Zhu L., et al. (2021). Interactive effect of climate warming and nitrogen deposition may shift the dynamics of native and invasive species. J. Plant Ecol. 14 (1), 84–95. doi: 10.1093/jpe/rtaa071
Rickey M. A., Anderson R. C. (2004). Effects of nitrogen addition on the invasive grass Phragmites australis and a native competitor spartina pectinata. J. Appl. Ecol. 41 (5), 888–896. doi: 10.1111/j.0021-8901.2004.00948.x
Ross K. A., Ehrenfeld J. G., Patel M. V. (2011). The effects of nitrogen addition on the growth of two exotic and two native forest understory plants. Biol. Invasions 13 (10), 2203–2216. doi: 10.1007/s10530-011-0034-7
Shiraishi S., Watanabe I., Kuno K., Fujii Y. (2005). Evaluation of the allelopathic activity of five oxalidaceae cover plants and the demonstration of potent weed suppression by oxalis species. Weed Biol. Manage. 5 (3), 128–136. doi: 10.1111/j.1445-6664.2005.00167.x
Singh A. S., Jones A. M. P., Shukla M. R., Saxena P. K. (2017). High light intensity stress as the limiting factor in micropropagation of sugar maple (Acer saccharum marsh.). Plant Cell 129 (2), 209–221. doi: 10.1007/s11240-017-1170-2
Slate M. L., Matallana-Mejia N., Aromin A., Callaway R. M. (2022). Nitrogen addition, but not pulse frequency, shifts competitive interactions in favor of exotic invasive plant species. Biol. Invasions 24 (10), 3109–3118. doi: 10.1007/s10530-022-02833-3
Sun X., Guo J. X., Guo S. W., Guo H., Hu S. J. (2019). Divergent responses of leaf N:P:K stoichiometry to nitrogen fertilization in rice and weeds. Weed Sci. 67 (3), 339–345. doi: 10.1017/wsc.2019.7
Sun Y., Wang C. T., Chen H. Y. H., Ruan H. H. (2020). Responses of C:N stoichiometry in plants, soil, and microorganisms to nitrogen addition. Plant Soil 456, 277–287. doi: 10.1007/s11104-020-04717-8
Tanveer A., Ali H. H., Manalil S., Raza A., Chauhan B.S. (2018). Eco-biology and management of alligator weed [Alternanthera philoxeroides (Mart.) griseb.]: a review. Wetlands 38, 1067–1079. doi: 10.1007/s13157-018-1062-1
Tumber-Davila S. J., Schenk H. J., Du E. Z., Du E. Z., Jackson R. B. (2022). Plant sizes and shapes above and belowground and their interactions with climate. New Phytol. 235 (3), 1032–1056. doi: 10.1111/nph.18031
Wang Y., Fan Z. W., Shen Y. D., Li X.X., Liu Y., Huang Q. Q. (2019a). Comparative performance of the highly invasive mimosa invisa and its less invasive subspecies under different nutrient and light conditions. Weed Res. 59 (6), 419–426. doi: 10.1111/wre.12379
Wang F., Gao J. W., Shi S. M., He X. H., Dai T. B. (2019b). Impaired electron transfer accounts for the photosynthesis inhibition in wheat seedlings (Triticum aestivum l.) subjected to ammonium stress. Physiologia Plantarum 167 (2), 159–172. doi: 10.1111/ppl.12878
Wang A., Jiang X. X., Zhang Q. Q., Zhou J., Li H. L., Luo F. L., et al. (2015). Nitrogen addition increases intraspecific competition in the invasive wetland plant Alternanthera philoxeroides, but not in its native congener alternanthera sessilis. Plant Species Biol. 30 (3), 176–183. doi: 10.1111/1442-1984.12048
Wang L. C., Liu Y., Zhu X. M., Zhang Z., Huang X. Q. (2021). Identify potential allelochemicals from Humulus scandens (Lour.) merr. root extracts that induce allelopathy on Alternanthera philoxeroides (Mart.) griseb. Sci. Rep. 11 (1), 1–8. doi: 10.1038/s41598-021-86656-7
Wang S., Wei M., Wu B. D., Cheng H. Y., Wang C. Y. (2020). Combined nitrogen deposition and cd stress antagonistically affect the allelopathy of invasive alien species Canada goldenrod on the cultivated crop lettuce. Scientia Hortic. 261, 108955. doi: 10.1016/j.scienta.2019.108955
Waterton J., Hammond M., Lau J. A. (2022). Evolutionary effects of nitrogen are not easily predicted from ecological responses. Am. J. Bot. 109 (11), 1741–1756. doi: 10.1002/ajb2.16095
Wu H., Carrillo J., Ding J. Q. (2016). Invasion by alligator weed, Alternanthera philoxeroides, is associated with decreased species diversity across the latitudinal gradient in china. J. Plant Ecol. 9 (3), 311–319. doi: 10.1093/jpe/rtv060
Wu H., Carrillo J., Ding J. Q. (2017a). Species diversity and environmental determinants of aquatic and terrestrial communities invaded by alternanthera philoxeroides. Sci. Total Environ. 581, 666–675. doi: 10.1016/j.scitotenv.2016.12.177
Wu H., Ding J. Q. (2019). Global change sharpens the double-edged sword effect of aquatic alien plants in China and beyond. Front. Plant Sci. 10, 787. doi: 10.3389/fpls.2019.00787
Wu H., Ismail M., Ding J. Q. (2017b). Global warming increases the interspecific competitiveness of the invasive plant alligator weed, alternanthera philoxeroides. Sci. Total Environ. 575, 1415–1422. doi: 10.1016/j.scitotenv.2016.09.226
Wu M., Liu H. Y., Zhang Y., Li B. B., Zhu T., Sun M. (2023). Physiology and transcriptome analysis of the response mechanism of Solidago canadensis to the nitrogen addition environment. Front. Plant Sci. 14, 1005023. doi: 10.3389/fpls.2023.1005023
Xiao Y., Liu X., Zhang L., Song Z. P., Zhou S. R. (2021). The allometry of plant height explains species loss under nitrogen addition. Ecol. Lett. 24 (3), 553–562. doi: 10.1111/ele.13673
Xu Z. W., Ren H. Y., Li M. H., Brunner I., Yin J. F., Liu H. Y., et al. (2017). Experimentally increased water and nitrogen affect root production and vertical allocation of an old-field grassland. Plant Soil 412, 369–380. doi: 10.1007/s11104-016-3071-2
Yan H. Y., Feng L., Zhao Y. F., Feng L., Wu D., Zhu C. P. (2020). Prediction of the spatial distribution of Alternanthera philoxeroides in China based on ArcGIS and MaxEnt. Global Ecol. Conserv. 21, e00856. doi: 10.1016/j.gecco.2019.e00856
Zaghdoud C., Carvajal M., Moreno D. A., Ferchichi A., Martinez-Ballesta M.D.. (2016). Health-promoting compounds of broccoli (Brassica oleracea l. var. italica) plants as affected by nitrogen fertilisation in projected future climatic change environments. J. Sci. Food Agric. 96 (2), 392–403. doi: 10.1002/jsfa.7102
Zhang J. H., He N. P., Liu C. C., Xu L., Chen Z., Li Y., et al. (2020b). Variation and evolution of C:N ratio among different organs enable plants to adapt to n-limited environments. Global Change Biol. 26 (4), 2534–2543. doi: 10.1111/gcb.14973
Zhang Y. Q., Liu Z. J., Liu J. P., Lin S., Wang J. F., Lin W. X., et al. (2017). GA-DELLA pathway is involved in regulation of nitrogen deficiency-induced anthocyanin accumulation. Plant Cell Rep. 36 (4), 557–569. doi: 10.1007/s00299-017-2102-7
Zhang X. L., Yu H. W., Yu H. H., Liu C. H., Fan S. F., Yu D. (2021). Highly competitive native aquatic species could suppress the growth of invasive aquatic species with similar traits. Biol. Invasions 23 (1), 267–280. doi: 10.1007/s10530-020-02370-x
Keywords: Alternanthera philoxeroides, carbon/nitrogen ratio, interspecific competition, nitrogen addition, Oxalis articulata, plant invasion, photosynthetic physiology
Citation: Wu H, Dong S, Wang L, Zhu Y, Jia S and Rao B (2023) Nitrogen enrichment alters the resistance of a noninvasive alien plant species to Alternanthera philoxeroides invasion. Front. Ecol. Evol. 11:1215191. doi: 10.3389/fevo.2023.1215191
Received: 01 May 2023; Accepted: 26 June 2023;
Published: 11 July 2023.
Edited by:
Hongwei Yu, Henan University, ChinaCopyright © 2023 Wu, Dong, Wang, Zhu, Jia and Rao. This is an open-access article distributed under the terms of the Creative Commons Attribution License (CC BY). The use, distribution or reproduction in other forums is permitted, provided the original author(s) and the copyright owner(s) are credited and that the original publication in this journal is cited, in accordance with accepted academic practice. No use, distribution or reproduction is permitted which does not comply with these terms.
*Correspondence: Hao Wu, d3VoYW84Njg2ODY4NkAxNjMuY29t; Benqiang Rao, cmJxeHlAMTYzLmNvbQ==
†These authors have contributed equally to this work and share first authorship