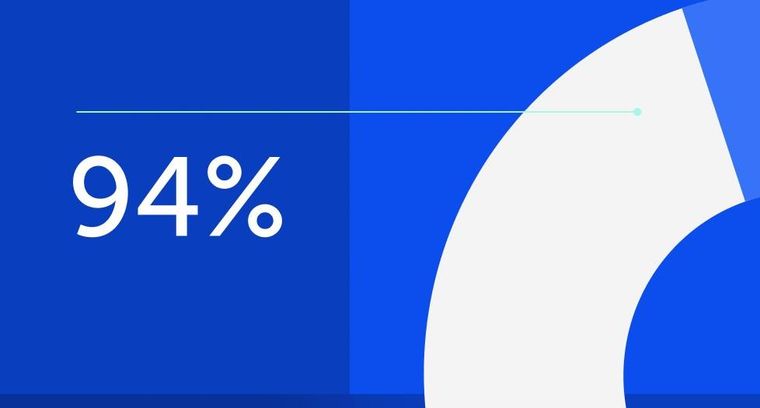
94% of researchers rate our articles as excellent or good
Learn more about the work of our research integrity team to safeguard the quality of each article we publish.
Find out more
ORIGINAL RESEARCH article
Front. Ecol. Evol., 31 August 2023
Sec. Population, Community, and Ecosystem Dynamics
Volume 11 - 2023 | https://doi.org/10.3389/fevo.2023.1203647
This article is part of the Research TopicEffects of Microplastics on Ecosystem Functioning of Eukaryotic Marine MicrobesView all 5 articles
Microplastics (MPs) provide persistent contaminants in freshwaters and the oceans from anthropogenic sources worldwide. MP contamination in ecosystems has emerged as a global environmental issue. While increasing research focused on the ecological consequences of plastic pollution, health-related implications of plastic pollution have been somewhat overlooked. In this study, we evaluated the effects of polyethylene MP contamination on microbial, physical, and biochemical characteristics of the hydrothermal vent crab Xenograpsus testudinatus over a 7-day food exposure. Different concentrations (0%, 0.3%, 0.6%, and 1.0%) of polyethylene MPs were used for feed intake experiments. Oxford Nanopore Technologies’ full-length sequencing of the 16S rDNA gene was used to explore the changes of the microbial composition in vent crab tissues. At the phylum level, the content of Firmicutes significantly decreased in the digestive gland tissue. Furthermore, the predicted functions of genes in the microbial community were significantly influenced by MPs. In contrast, there were eight functions in gill and 11 functions in digestive gland tissues identified at low and high intake levels. The dominant functions of methylotrophy, dark thiosulfate oxidation, dark oxidation of sulfur compounds, aromatic hydrocarbon degradation, and aromatic compound degradation were significantly increased at high intake levels in the digestive gland. These findings indicate that MP ingestion causes not only a short-term decrease in energy intake for crustaceans but also a change in microbial communities and their functions. This study provided a first account on the toxicity of MPs in a hydrothermal vent crab to aid in the assessment of health risks provided by polyethylene MP to marine invertebrates.
Hydrothermal vent (HV) systems are among the most challenging marine ecosystems on the planet. HV studies began in the 1970s, and soon thereafter, it has been hypothesized that life may have evolved in HVs because of the similarity with extreme conditions on early Earth after the planet was cooling down (Ka et al., 2011). Since the discovery of HVs in the deep sea of the Galapagos trench in 1977, several new biota were identified from this environment. These included higher trophic-level organisms inhabiting HVs, including invertebrates such as polychaetes, gastropods, and crustaceans that consume organic matter produced by free-living chemoautotrophic bacteria or microbiota that are associated with fauna (Van Dover, 2002; Wang et al., 2014). The ability of organisms to adapt to the extreme conditions of HVs may significantly be impacted by mutualism between bacteria and their host species. Bacteria and other life forms were found to provide a chemoautotrophic symbiosis within HVs. At HV sites, decapod crustaceans such as crabs were studied in terms of their reproductive biology, larval development, and behavior. HVs contain a unique microbial community compared to other environments because of environmental factors such as high temperature, high pressure, low oxygen concentrations, and the presence of heavy metals and other toxic compounds (Minic and Thongbam, 2011).
Twenty-two sites with HVs in shallow waters at depths of less than 200 m were meanwhile reported worldwide (Zhang et al., 2020). They are associated with volcanic arcs, back-arc basins, and mid-ocean ridges (Zhang et al., 2020). Several aspects of these distinct regions have been studied so far. Kueishan island (KST), located off the northeast coast of Taiwan, is a volcanic outcrop. A shallow-water HV habitat exists on one side of the island, emitting gases (mostly carbon dioxide and hydrogen sulfide) and toxic fluids. This HV area consists of two different types of vents: the yellow vents (which include sulfur mounds and chimneys) releasing fluids that are of temperatures between 48°C and 62°C and providing an acidic pH of 1.8 to the neutral value 7.0, whereas white vents provide fluids between 92°C and 116°C and pH values ranging from 1.5 to 6.3 (Chan et al., 2016). According to different reports, only a few sulfide-resistant aquatic organisms live in the KST vent area (Van Dover, 2019; Allen et al., 2020; Chiu et al., 2022). The HV crab Xenograpsus testudinatus, a dominant endemic species described by Lee et al. (2023), thrives abundantly in the HVs at KST, best explained by particular adaptations and the absence of competitors and predators. Japan’s Showa Iwo-jima in Kagoshima and Shikine-jima Island in the Izu archipelago have both reported it from shallow-water HV regions (Jeng et al., 2004; Hiroshi et al., 2019; Yang C.-H. et al., 2022).
Xenograpsus testudinatus lives in the immediate vicinity of HVs as an opportunistic species that feeds on plankton killed by vent plumes and other dead organisms in the vent region (Chan et al., 2016; Wu et al., 2022). Understanding the ecology and evolution of HV organisms requires knowledge of their diets. Several HV organisms are known to rely on microorganisms for energy (Zbinden and Cambon-Bonavita, 2003). Crabs at deep-sea HVs were observed feeding on chemoautotrophic bacteria (Watsuji et al., 2015). Free-living bacteria, including cyanobacteria and soil bacteria, were found in the stomach and intestines of X. testudinatus. These organisms may represent a significant source of nutrients for this species (Wang et al., 2014). Studies also suggest that the microbiota of X. testudinatus are species-specific (Ho et al., 2015).
Plastics turned out to be emerging pollutants of concern due to their negative impact on aquatic and terrestrial ecosystems (Usman et al., 2022; Truchet et al., 2023). Larger plastics degrade to microplastics (MPs) (size ≤5 mm). MPs were shown to be consumed by different aquatic organisms (Liu et al., 2019) and became an integral part of several food webs (Pironti et al., 2021). MPs, if ingested, can harm aquatic organisms by obstructing feeding appendages, impeding food passage, or causing pseudo-satiation, resulting in decreased food intake (Murphy and Quinn, 2018). Plastic particle size, shape, and density affect MP absorption in different feeding-type groups. Low-density polymers like polyethylene and polypropylene float and may be eaten by planktivorous surface water consumers (Jorquera et al., 2022).
High-density polyethylene (HDPE), polyethylene, and polyvinylchloride sink down to the sediment and are likely to be consumed by deposit feeders (Urbina et al., 2023). It was shown that after ingesting plastics, in crabs and other crustaceans, most of the plastics can be excreted, but this will increase the metabolic energy requirements of crabs (Watts et al., 2014; Ryan, 2019). In contrast to the growing number of field and lab research evaluating the effects of MP consumption on other crustaceans (Vermeiren et al., 2023), there are presently only a small number of studies evaluating the effects of MP ingestion on crabs. Despite the fact that the microbiota of X. testudinatus were the subject of some studies as yet (Wang et al., 2014; Ho et al., 2015), little is known about the negative repercussions of MPs on HV species and their microbiota.Our attempt to understand microbial life, such as microbial community composition, functional potential, and metabolic activities, has recently been greatly expanded due to the recent revolution in microbiology provided by culture-independent analyses and bioinformatic approaches (Ho et al., 2015; Alvarez-Yela et al., 2019). Previous research has shown that MPs and nanoplastics are capable of having a variety of negative effects on aquatic organisms, including physical damage (Yuan et al., 2022; Fang et al., 2023). The present study investigated the fate and impact of polyethylene microplastics (PE-MPs) on X. testudinatus and its microbiota determined by applying culture-independent analyses.
Crabs from HVs were captured in a chimney off KST, Taiwan. The HVs of KST near the northeastern Taiwan coast are about 60 miles away from the Okinawa Trough HVs. Healthy adult female crabs of X. testudinatus (n = 140) were captured at a depth of 10–15 m (121°57′E 24°50′N) on 28 July 2020 (Figure 1). The target species of this study, X. testudinatus, was not designated as endangered or protected and was not taken from a marine protected area in Taiwan, therefore, no special permission was required to capture these crabs.
Figure 1 Geographic location of the Kueishan Island hydrothermal vent system and photographs of hydrothermal vent crab sampling (station 1: 121°57′E 24°50′N).
Experiments were carried out at the Institute of Marine Biology, National Taiwan Ocean University. To avoid plastic pollution, X. testudinatus were raised in a 30 cm × 30 cm tempered glass tanks, filled with 6 L of filtered seawater (33 PSU 20°C, 12:12 light:dark), and adapted to the lab conditions for 72 h prior to the experiment. Fluorescent green polyethylene microspheres of 500–600-µm size (Cospheric Innovations in Microtechnology, Santa Barbara, CA, USA) were used for this experiment. The crabs were provided with food of 7% of their body weight in the form of homogenized squid mixed with gelatin. In four experimental groups, crabs were distributed at random and given feed containing different amounts of polyethylene particles: 0% (0 mg), 0.3% (0.6 mg), 0.6% (1.2 mg), and 1% (2.0 mg) supplemented to 2 g of feed. These concentrations were utilized to simulate the potential MP exposure levels that crabs might experience in their native benthic environment (Watts et al., 2016). The crabs were fed once a day, and water was changed daily to maintain hygienic conditions and ensure healthy growth of the crabs. After feeding, we observed that individual crabs had a different feed intake (Supplementary Table S1). It was suspected that certain crabs did not consume the desired MP quantities, thus they were regrouped. According to the observed intake level, they were categorized into three consumption types: low (0–≤5 × 10−3 g as a control group), intermediate (>5 × 10−3~≤2 × 10−2 g), and high (>2 × 10−2 g) (Figure 2).
Figure 2 Experimental design of polyethylene microplastics fed to the shallow-water hydrothermal vent crab (Xenograpsus testudinatus).
Crabs were rinsed three times with sterile seawater before being dissected. All dissection instruments were sanitized to prevent cross-contamination. A biological safety cabinet was used during the entire dissection. The digestive gland and gills were removed from the crab individually within the cabinet and put into 1.5-mL Eppendorf tubes with a DNA/RNA Shield (Zymo Research, CA, USA). The 1.5-mL Eppendorf tubes contained all tissue samples that were ground with plastic pestles that had been autoclaved. Gills and the digestive gland from dissected crabs were used to extract total DNA using a DNA Quick-DNA HMW MagBead kit from Zymo Research in California, USA, in accordance with the manufacturer’s instructions in triplicate. A NanoDrop 2000 spectrophotometer (Thermo Fisher Scientific, Wilmington, DE, USA) and 1% agarose gel electrophoresis were used to assess the DNA quantity and quality. Polymerase chain reaction (PCR) was carried out using primers 27F (5’-AGA GTT TGA TCC TGG CTC AG-3’) and 1492R (5’-GGT TAC CTT GTT ACG ACT T-3’) (Weisburg et al., 1991) amplifying variable regions of the 16S rRNA gene (V1–V9). The following conditions were used during the PCR amplification process: 95°C for 5 min, then 35 cycles of denaturation at 95°C for 30 s, annealing at 53°C for 30 s, extension at 72°C for 1 min and 30 s, and a final extension at 72°C for 10 min. The PCR was performed with a total reaction volume of 50 μL containing 5 μL of 10× Ex Tag buffer, 4 μL of dNTP, 0.5 µL of TaKaRa Ex Taq (5U/µL), 31 µL of ddH2O, 4 µL of primer sets (2 µL of forward and reverse 16s primers), and 1 µL of template DNA.
A MinION was used to perform third-generation sequencing (Oxford Nanopore Technologies, Oxford, UK) technology equipped with a R9.4.1 flow cell (MINION, MinKNOW 20.10.3) following the guidelines provided by the manufacturer. Concentrating full-length 16S rRNA gene products and purifying amplicons using the AMPure XP Purification Kit from Beckman Coulter Life Sciences, amplicons were combined with the AMPure XP Purification kit, and reactions were then let to sit at room temperature for 20 min. Before elution in 30 µL of sterilized ddH2O, one round of 70% fresh ethanol washing was followed by 1 min of room temperature air-drying. With a Qubit 4 Fluorometer dsDNA HS (Thermo Fisher Scientific), we measured the purity of each sample after it had been pooled in equal amounts (equimolar ratios) of roughly 2,000 ng. Following the SQK-LSK109 procedure, nanopore sequencing libraries were subsequently created (Oxford Nanopore Technologies). When no sequencing reads were gathered or all of the flow cell protein pores were unavailable, we ended the experiment. We loaded 75 µL of the library into the R9.4.1 MinION flow cells (Oxford Nanopore Technologies), ran for approximately 96 h, and were subsequently stopped. Following the sequencing process, reads were basecalled using the high-accuracy model (HAC) in Guppy (version 4.2.3), and the resulting DNA sequences with Q > 9 were stored as “fastq” files for further analysis.
For demultiplex, porechop package (version 0.2.4) was used to trim adapters and demultiplex sequence readings. Using NanoFilt (version 2.5.0, https://github.com/wdecoster/nanofilt), we eliminated reads shorter than 1.4 kbp and longer than 1.6 kbp. NanoStat (version 1.1.2, https://github.com/wdecoster/nanostat) was utilized to analyze read data such as quality scores and read durations, while Pistis (https://github.com/mbhall88/pistis) was utilized to construct quality control plots. A centrifuge was used to determine the taxonomic classification of the reads. The centrifuge database was created by sequencing the 16s rRNA of the type of strain from the National Center for Biotechnology Information (released on 17 February 2022). Pavian was used to visualize the results. After assigning the taxonomic information of the reads, we used “Vegan” package version 2.6.2 (https://cran.r-project.org/package=vegan) for rarefying the data and then eliminating the unclassified reads, reads of the cellular organism, and a total number of reads of less than 0.05% of total reads across all samples.
Change in the body weight of crabs was tested with a two-tailed Student’s t-test. Various statistics were carried out using R software (version 4.1.3), and the rarefaction function in the vegan package (version 2.6.2) was used for calibration before statistical analysis. The samples with the lowest reading were used as the calibration benchmark to ensure that all sample readings were consistent and comparable for rarefaction. Data integration was performed through Phyloseq package (version 1.36.0) (McMurdie and Holmes, 2013). Alpha and beta diversity was performed using Microbiota Process (version 1.4.4). Statistical Analysis of Metagenomic Profiles (STAMP) software was used to create extended error bar plots with a two-sided Welch’s t-test p-value <0.05. Afterward, the unique and shared genera were represented using an UpSet plot using the UpSetR software (Conway et al., 2017). Bacterial functional analysis was performed using Functional Annotation of Prokaryotic Taxa (FAPROTAX; version 1.2.4) software (Louca et al., 2016). Wald chi-square test of R package DESeq2 was used to search for significantly different bacterial and functional groups (Nearing et al., 2022).
The effects of PE-MP particles on vent crab wet weight were measured before and after the experiment (Figure 3). As a result of artificial feeding, a decrease in body weight of the crab was observed. For 7 days’ feeding, among the treatment groups, comparatively highest weight reduction was observed in 1% (0.62 g reduction) followed by 0.6% (0.44 g), 0.3% (0.42 g), and 0% (0.28 g) (Supplementary Figure S1). When considering different intake levels, we observed that the crabs in the low-intake group had more weight reduction (0.63 g), followed by the high-intake group (0.57 g). The intermediate group showed a slight reduction in their wet weight (0.26 g) (Figure 3). Results suggest that artificial feeding of X. testudinatus has slightly negatively affected the wet weight of the crabs at all conditions. However, none of the statistical results comparing the weights before and after feeding were significantly different.
Figure 3 Effects of polyethylene microplastics (MPs) on vent crab body weight change before and after feeding experiments with different intake levels (low, intermediate, high) of MP impact on vent crabs.
To investigate the impact of plastic particles on the bacterial communities of crabs, we calculated the alpha diversity indices of bacterial communities associated with X. testudinatus gills and digestive glands. The aim was to observe how the bacterial composition changed in each tissue or organ after being exposed to various concentrations of MPs (Supplementary Table S1) at different real intake levels (Figure 4). Alpha diversity indices such as species richness (Chao1), species diversity (Shannon), and species evenness values varied among different intake levels in the gill tissues (Figure 4A). A Kruskal–Wallis test (p ≤ 0.001) was used for statistical analysis. Examining alpha diversity indices, no significant differences were found. We observed significant differences in the digestive gland tissue alpha diversity index, even though the values varied between intake levels (Figure 4B). In the case of concentration groups in the gill tissue, the concentration group 1.00% showed significant differences in bacterial richness with control and concentration group 0.30% (Supplementary Figure S2A).
Figure 4 Alpha diversity represented by Chao1, Shannon, and Pielou indices in the three intake levels of gill and digestive gland samples. (A) Indicating the alpha diversity of gill samples. (B) Indicating the alpha diversity of digestive gland samples. Tukey test indicating significant differences (p < 0.05). Different colors indicating different real intake levels.
For comparison of the similarity of bacterial composition among the different feeding concentrations and intake levels of plastic particles, nonmetric multidimensional scaling (NMDS) based on the weighted Unifrac distances described shifts of bacterial communities at different conditions. The results of PERMANOVA verified significant effects of MP intake levels.
From the results of PERMANOVA tests of the gill tissue, the interpretation degree (variation: 21%, p = 0.174) among different treatments was low and there was no significant difference (Figure 5A). However, the digestive gland group showed a 64.6% variation (R2 = 0.646) and p ≤ 0.001. This result indicated a significantly different bacterial community between the different MP real-intake groups. The microbial community changed after different amounts of MP particles were ingested by the digestive gland. The figure shows that the low-intake group and the other two groups were clearly separated, while the medium- and high-intake groups overlapped (Figure 5B).
Figure 5 Comparison of beta diversity of bacterial communities among the different feeding intake levels of plastic particles shown by nonmetric dimensional scaling (NMDS) based on weighted UniFrac dissimilarities. (A) NMDS biplot of bacterial community in the gill among different real-intake groups. (B) NMDS biplot of bacterial community in the digestive gland among different real-intake groups. Different colors indicating different real intake levels.
The relative abundance of several bacterial taxa varied in gill and digestive gland samples (Figure 6). The 10 most abundant bacterial phyla in the gill samples were Proteobacteria, Bacteroidetes, Planctomycetes, Firmicutes, Tenericutes, Actinobacteria, Verrucomicrobia, Cyanobacterium, Lentisphaerea, and unclassified bacteria (Figure 6A). In the gill samples, there were two top dominant bacterial phyla: Proteobacteria (65.59%) and Bacteroidetes (27.14%) followed by Planctomycetes (1.98%) and Firmicutes (1.37%) (Figure 6A). These findings indicated that there was no significant difference between the intake level and bacterial phyla. At class level, the main representatives among all samples were Gammaproteobacteria (50.58%), Flavobacteria (45.60%), and Epsilonproteobacteria (37.95%), followed by Alphaproteobacteria (28.36%) that were dominant in all samples (Figure 6B). Notably, compared to low and intermediate intake levels, Epsilonproteobacteria (24.0%) were slightly decreased in the high-intake group.
Figure 6 Relative abundance of the top 10 bacterial phyla and classes in gill and digestive gland samples at different levels of microplastic intake groups. (A) Relative abundance of the top 10 bacterial phyla from gill samples. (B) Relative abundance of the top 10 bacterial classes from gill samples. (C) Relative abundance of the top 10 bacterial phyla from digestive gland samples. (D) Relative abundance of the top 10 bacterial classes from digestive gland samples.
Among the bacterial phyla found in the digestive gland samples, the 10 most abundant were Proteobacteria, Firmicutes, Tenericutes, Bacteroidetes, unclassified bacteria, Acidobacteria, Terrabacteria-group, Fusobacteria, Planctomycetes, and Deferribacteriales (Figure 6C).
In terms of phylum abundance, Proteobacteria (63.19%) emerged as being highly abundant in all samples. Firmicutes (12.6%), Tenericutes (6.46%), and Bacteroidetes (5.91%) were also present in significant quantities. Notably, when comparing the three intake levels (low, intermediate, high), significant differences were observed across all samples. In the low-intake group, the dominant phyla were Firmicutes (31.77%) and Proteobacteria (24.17%), followed by Tenericutes (18.76%), Acidobacteria (11.06%), and the Terrabacteria group (4.40%). In contrast, the intermediate-intake group displayed a different community composition, with Bacteroidetes (12.41%) emerging as the second dominant phylum. Particularly noteworthy is the fact that, compared to the low-intake group, the high- and intermediate-intake groups exhibited a significant decrease in certain phyla (Figure 6C).
At the class level, the two most prevalent bacterial communities in all digestive gland samples were Betaproteobacteria (24.29%) and Gammaproteobacteria (20.98%), followed by Alphaproteobacteria (12.50%), unclassified bacteria (6.89%), and Mollicutes (6.46%). Significant differences were observed across all intake groups. Comparing the low-, intermediate-, and high-intake groups, some phyla were consistently present in all replicates of the low-intake group, while experiencing decreases in the intermediate- and high-intake groups. Notably, Mollicutes (0.99%), Bacilli (1.98%), Actinomycetia (0.74%), and Clostridia (0.67%) were significantly decreased in the intermediate- and high-intake groups (Figure 6D). Furthermore, there were bacteria that exhibited decreased abundance specifically in the high-intake group compared to the low-intake group. Similarly, Mollicutes (0.99%), Bacilli (1.98%), Actinomycetia (0.74%), and Clostridia (0.67%) were significantly reduced in the intermediate- and high-intake groups (Figure 6D).
In order to enhance our understanding of the impact of MPs on bacterial communities, we conducted an analysis using STAMP. This analysis allowed us to identify and demonstrate significant differences in the average proportions of bacterial classes within gill and digestive gland samples across various intake groups. To assess these differences, we applied the Welch t-test, which helped us to draw meaningful conclusions about the relationship between MP exposure and changes in bacterial communities. The analysis of gill samples revealed significant differences in the relative abundance of Saprospria between the high–low and high–intermediate groups, with significantly higher proportions observed at the high-intake group (Figures 7A, B). In the digestive gland samples, significant differences were observed between the high–low and low–intermediate groups. Comparing the high- and low-intake groups, Gammaproteobacteria and Betaproteobacteria showed significantly higher abundances in the high-intake group. In the low–intermediate-intake group, only Gammaproteobacteria showed higher proportions in the intermediate group, while Bacilli and unclassified bacteria were significantly more abundant in the low-intake group (Figures 7C, D). These findings provide valuable insights into the specific bacterial classes that respond differently to MP exposure, both in gill and digestive gland samples, highlighting the effect of intake groups on bacterial community dynamics.
Figure 7 Extended error bar plot in STAMP illustrating significant differences between mean proportions of bacterial classes associated with gill and digestive gland samples between different intake levels using Welch’s t-test (two-sided. p-value <0.05). (A) Significantly different bacterial classes in gill samples between high and low intake levels. (B) Significantly different bacterial classes in gill samples between high and intermediate intake levels. (C) Bacterial classes that are significantly different in the digestive gland among high and low intake levels. (D) Bacterial classes being significantly different in the digestive gland among low and intermediate intake levels.
In order to comprehend present and absent bacteria among the different groups, we used the upset plot, which showed shared genera in different combinations. The analysis of the gill and digestive gland tissues revealed the presence of shared genera, indicating common bacterial compositions between the two tissue types (Figures 8A, B). Specifically, in the gill samples, a total of 53 genera were found to be shared among all three intake groups, indicating a core set of bacterial genera present across the different levels of MP intake. Additionally, 17 genera were identified as specific to either the low- or high-intake group, suggesting unique bacterial communities associated with different intake levels (Figure 8A). In the gill samples, a total of 16 genera were found exclusively in the low- and high-intake groups, while nine genera were specific to the high- and low-intake groups. Additionally, five genera were shared between the low- and intermediate-intake groups, and five genera were only present in the low-intake group (Figure 8A).
Figure 8 Upset plot indicating the dissimilarity of bacterial genera among different intake levels (A, Gill; B, Digestive gland). The plots representing shared and specific core microbiome genera in the between intake levels (low, intermediate, and high). Set size indicates the total number of biologically significant genera and the interaction size showing how many species were identified in one group. Red color indicating the counts of shared genera across all intake levels.
In contrast, the digestive gland samples exhibited a higher number of unique genera associated with MP intake. Specifically, 72 genera were exclusively present in the high-intake group, while the low- and intermediate-intake groups had eight and five specific genera, respectively. Furthermore, nine genera were shared between the intermediate- and high-intake groups, and five genera were shared between the low- and high-intake groups (Figure 8B). These findings indicate that the middle- and high-intake groups ingested a greater amount of plastic particles, resulting in a more diverse range of bacterial genera within the digestive gland. Interestingly, the gill samples did not show significant changes in bacterial composition as a result of MP particle intake (Figure 8B). These observations highlight the differential impact of MP ingestion on bacterial communities in different tissue types.
The FAPROTAX investigation discovered that a number of functional categories may be predicted and identified based on the taxonomic profiles of bacteria (Figure 9). In the gill tissue, 53.1% of the sequences were paired with the functional database, and there was no significant functional difference between the low- and intermediate-intake groups. There were eight significant functions observed in the low- and high-intake groups (Figure 9A). The five functions of reduced acetogenesis, iron respiration, dark sulfite oxidation, chemoheterotrophy, and aerobic chemoheterotrophy significantly increased, while the three functions of dark thiosulfate oxidation, dark sulfur oxidation, and dark oxidation of sulfur compounds significantly decreased (Figure 9A).
Figure 9 Log2 fold change plot showing differential expression of functional groups in the high-intake group compared with the low-intake group. (A) Log2 fold change plot showing the differential expression of bacterial functional groups in gill samples between high- and low-intake groups. (B) Log2 fold change plot showing the differential expression of bacterial functional groups in digestive gland samples between high- and low-intake groups. Different colors indicate intake levels.
A total of 60.63% of the sequences in the digestive gland were paired with functional directory, and this was also performing 11 functions. The high and low groups also showed significant functional differences. Plant pathogens, nitrate respiration, methylotrophy, dark thiosulfate oxidation, dark oxidation of sulfur compounds, aromatic hydrocarbon degradation, and aromatic compound degradation were significantly increased at high intake. Photoautotrophy, iron respiration chemoheterotrophy, and anoxygenic photoautotrophy were significantly increased at low intake (Figure 9B). The different bacterial functions present in the gill and digestive gland also provide information about how MPs influence the responses of the HV crabs.
Plastics are a prominent newly emerging marine pollutant. The term “plastics” is typically used to describe synthetic polymers made of different elements including carbon, nitrogen, oxygen, and sulfur. Concerns regarding the impact of MP particles on the health of aquatic organisms have been steadily increasing (Sharma and Chatterjee, 2017). In recent years, the detrimental effects of MPs on aquatic organisms have become a growing concern among researchers (Gola et al., 2021). Accumulation of MPs in crustaceans has been linked to physiological consequences such as developmental delays, delayed molting, and decreased fertility, as reported by Jeong et al. (2016). Previous studies have also indicated that the consumption of MPs hinders the feeding ability of marine organisms. This is attributed to the accumulation of MPs in the digestive tract, leading to blockage, reduced feeding capacity, and depletion of energy reserves (Li et al., 2021). This study presents initial evidence indicating that the presence of PE-MPs, categorized into different intake groups, can have an impact on the associated microbiota and their functioning in adult HV crabs (X. testudinatus). In our study, after 7 days of exposure to MPs, there was a noticeable trend of weight loss in vent crabs across all groups, although the differences were not statistically significant. Previous research has extensively examined the impact of MPs and nanoplastics on organism growth, biomass, and physical well-being. However, conflicting findings exist in the literature. While some studies have provided compelling evidence of the harmful effects of MPs and nanoplastics (Ziajahromi et al., 2017), others have not found significant impacts (Lo and Chan, 2018). These disparities can be attributed to variations in factors such as plastic concentration, duration of exposure, organism life stages, and environmental conditions across different studies. It is crucial to note that the expression of physical impacts from MPs depends on these variables. Additionally, studies have suggested that if MPs are able to penetrate biological cells, their effects on organisms may be amplified, resulting in weight loss, localized inflammation, and energy redistribution (Li B. et al., 2020).
Numerous investigations have explored the interactions between microbes and plastics in pelagic systems, as well as the consequences of plastics on marine life (Latva et al., 2022). For instance, Mattsson et al. (2014) observed several effects on feeding, shoaling behavior, and metabolism in crucian carp after exposure to 24- and 27-nm polystyrene nanoparticles through the food chain, from algae to the water flea Daphnia. Laboratory experiments have also demonstrated that the ingestion of MPs reduces species diversity, survival rates, and larval development in bivalves (Sussarellu et al., 2016). MP ingestion has been documented in over 180 marine organisms, with filter-feeding bivalves and crabs being particularly vulnerable (Waite et al., 2018). In the case of crustaceans, shore crabs have been observed to ingest MPs via their gills and digestive tract (Watts et al., 2016). Understanding how different levels of plastic uptake can affect aquatic animals and their microbiota is of utmost importance.
Previous studies have examined the impact of plastic on marine organisms, with a particular focus on brachyuran crustaceans, as highlighted by Watts et al. (2016). In a laboratory setting, Yang Z. et al. (2022) investigated Eriocheir sinensis, commonly known as the Chinese mitten crab, to understand its consumption of plastic particles made of different polymers and varying hardness and shapes. The study revealed that certain crabs exhibited individual differences and increased their intake of plastic-contaminated food to ensure adequate nourishment. This behavior can be attributed to the crabs’ ability to survive for longer periods without food, distinguishing them from other marine organisms. A similar feeding pattern has also been observed in the common shore crab Carcinus maenas (Watts et al., 2015). Given the hypothesis that life originated in HVs (Corliss et al., 1981), investigating the impact of plastics on aquatic life in these ecosystems becomes particularly relevant. In our study, we focused on investigating the impact of MPs on the gut microbiome of the HV crab X. testudinatus.
We selected this crab species, as it represents a dominant invertebrate group found near the outlets of HVs and is among the macrocrustaceans in KST (Ng et al., 2000; Yang Z. et al., 2022). The microbial populations residing in the intestine play a crucial role as environmental stress sensors that can be disrupted by various pollutants (Milan et al., 2018). In the ocean, microbes are essential for the survival and evolution of marine organisms (Pita et al., 2018). Numerous studies have demonstrated the accumulation of MPs in animal intestines and their impact on gut microbiota (Wang et al., 2018; Yan et al., 2021). The gut-associated microbiota uniquely influence the development of the gastrointestinal tract, nutritional status, immunological responses, and disease resistance of the host (Chaiyapechara et al., 2012). Overall, our study contributes to the understanding of how MPs can influence the gut microbiome of HV crabs. By investigating the microbial composition and potential disruptions caused by MPs, we shed light on the ecological implications and potential risks associated with plastic pollution in marine environments. Further research is needed to elucidate the specific mechanisms underlying the interactions between MPs, gut microbiota, and the overall health of marine organisms.
However, to the best of our knowledge, there have been no prior studies investigating the effects of MP exposure on the microbiome of vent crabs. It is worth noting that although polyethylene is the most prevalent plastic in environmental waters, the majority of MP exposure experiments (Li et al., 2020). While previous studies have focused on feeding behavior, community analysis, and behavioral studies in vent crabs (Hu et al., 2016; Yang et al., 2016), the direct investigation of MP effects on these organisms is limited. Therefore, the present study contributes unique insights for the understanding how MPs may influence vent crab populations. The findings highlight the ecological implications and potential risks associated with MP pollution, emphasizing the need for further research on mechanisms, long-term consequences, and mitigation strategies.
In our study, we found that the presence of PE-MPs significantly altered the microbial community composition of vent crabs. Our NMDS analysis revealed distinct variations in the distribution of microbial communities in the digestive gland and gill samples of X. testudinatus, particularly at different intake groups of MPs. Specifically, we observed a reduction in the abundance of Firmicutes and Tenericutes, accompanied by an increase in Proteobacteria in the digestive gland samples. This finding aligns with previous studies that reported an increase in Proteobacteria in the gut microbiomes of other organisms exposed to MPs, such as European sea bass and mice (Montero et al., 2022). Furthermore, our results are consistent with previous reports that have documented an increase in certain bacterial taxa following plastic ingestion in fish (Carassius carassius) and Chinese mitten crab (Eriocheir sinensis) (Liu et al., 2019; Hu et al., 2022). Along with their toxicity, MP ingestion has the potential to alter the gut microbiota by incorporating bacterial species attached to the MPs’ surface into the host (Yan et al., 2021). The HV crab X. testudinatus is closely related to other crab species, such as the euryhaline crab Eriocheir sinensis and crabs of the genus Hemigrapsus spp. The members of the phylum Proteobacteria are Gram-negative chemoautotrophic bacteria that have been previously reported from deep-sea crustaceans and from anoxic sediments (Longnecker and Reysenbach, 2001). These microorganisms are not abundant in the water column. Sulfate-reducing bacteria, on the other hand, were found to be very abundant in the upper oxic sediment layer despite being believed to be restricted to the anoxic zone (Tsuchida et al., 2011). Bacteroidetes species are commonly found in the gut microbiota of all organisms including shrimp, fish, and humans. They are thought to be indigenous to the host and maintain a complex and generally beneficial relationships with the host (Wexler, 2007). Changes in the composition and diversity of the gut microbiome can have detrimental effects on aquatic animals (Wan et al., 2019). Overall, our study provides evidence that PE-MPs significantly alter the microbial community structure of vent crabs, with implications for the abundance of specific bacterial phyla. These findings contribute to our understanding of the potential ecological consequences of MP pollution on the gut microbiota of marine organisms. Further research is warranted to elucidate the underlying mechanisms driving these changes and to investigate the long-term impacts of MP-induced alterations in the vent crab microbiome. Additionally, strategies for mitigating the adverse effects of MP pollution on marine ecosystems should be developed (Hu et al., 2016).
The extended bar plot analysis revealed significant differences in the proportions of bacterial classes between gill and digestive gland samples across different intake groups. In the gill samples, the high-intake group exhibited dominance of Saprospiria, a filamentous group of bacteria commonly found in activated sludges (De Carvalho and Fernandes, 2010). Previous research has demonstrated that Saprospiria can thrive on and degrade various polymer types, which could explain its higher abundance in the gill samples of individuals with high intake levels (Raulf et al., 2015). Moving to the digestive gland, we observed a different pattern. The high-intake group showed a higher representation of Gammaproteobacteria compared to the low-intake group. It is important to note that Gammaproteobacteria are typically associated with deep-sea environments, whereas Epsilonproteobacteria are known to thrive in sulfur-rich environments (Campbell et al., 2006). These findings indicate that the composition of bacterial classes in the digestive gland is influenced by the individual’s intake level, potentially reflecting distinct environmental conditions and microbial interactions. Overall, the results highlight the differential abundance of specific bacterial classes in gill and digestive gland samples, emphasizing the potential role of dietary intake in shaping the microbial communities in these anatomical regions.
The FAPROTAX analysis of X. testudinatus microbiota exposed to MPs indicated potential impacts on microbial functions. In the gill samples, the most dominant functions observed were chemoheterotrophy and aerobic chemoheterotrophy. These findings are consistent with previous reports highlighting chemoheterotrophy, fermentation, and nitrate reduction as prevalent functions in the gut microorganisms of fish, crabs, and shrimp. Specifically, the key bacterial groups responsible for executing chemoheterotrophy and aerobic chemoheterotrophy were identified as Gammaproteobacteria, Alphaproteobacteria, Firmicutes, Bacteroidetes, and Actinobacteria (Flint et al., 2012). Notably, the presence of MPs significantly increased the methylotrophy function in the digestive gland. Methylotrophy plays a significant role in the carbon cycle, and the high intake level of MPs might exert a stronger influence on this vital carbon cycling process (Chen et al., 2022). This suggests a potential link between MP pollution and alterations in carbon cycling dynamics within X. testudinatus microbiota. According to our findings, the dominant bacterial communities were identified, and notable alterations were observed in the intake groups, accompanied by a reduction in sulfur metabolism functions. The impact of MPs on the gill was evident, and in the digestive gland, 11 functions were identified at both low and high intake levels. Strikingly, an increase in 14 functions was observed, with a significant portion of these functions being associated with plastic degradation. This aligns with previous knowledge that Flavobacteria are proficient polymer degraders found in diverse environments, including sediments and seawater (Alonso et al., 2007). These results suggest that MP pollution can trigger specific changes in the microbial communities and metabolic functions within the studied organism. The increased presence of functions related to plastic degradation indicates that the MPs may serve as a substrate or induce the proliferation of bacterial groups capable of breaking down plastic compounds. This highlights the potential ecological consequences of MP contamination on marine organisms, as it may lead to alterations in microbe-mediated processes, such as carbon cycling, within the marine ecosystem. Further investigations are required to fully understand the mechanisms behind these observed effects and their broader implications for the environment.
Proteobacteria and Bacteroidetes are involved in degradation under anaerobic conditions. According to our findings, these bacteria were dominant in all samples. Gill and digestive gland of X. testudinatus are considered important organs of sulfur metabolism (Kodama and Watanabe, 2004). We compared the effects of plastic particle ingestion on the gill and digestive gland of vent crabs. The main metabolic organs in shrimp and crabs, the digestive glands, can store nutrients and provide energy. Other vital functions, including immunity, metabolism, growth, and development, depend on the digestive gland. Additionally, the gills of crabs have a large surface area and are in direct contact with moving seawater. The digestive gland regulates energy storage, digestion, metabolism, ovary development in crustaceans, and the absorption and storage of waste and metabolites (Shenghai et al., 2015). According to a previous report, the primary food source of X. testudinatus is zooplankton, which is probably destroyed by the hot fluid generated from the HV. It is assumed that they do so only during trans-vent migration, where they serve as pivotal species in energy transfer of the vent system (Chan et al., 2016). Xenograpsus testudinatus is physically suited to the vent environment and possesses proteolytic enzymes, which are active throughout wide ranges of temperature and pH values, even in the presence of heavy-metal inhibitors (Hu et al., 2012). This adaptation is for irregular food supplies. In addition, X. testudinatus is able to store significant quantities of lipids for use as a source of nutrition during times when food is scarce. Xenograpsus testudinatus is a secondary consumer that consumes zooplankton directly. The crab X. testudinatus is omnivorous and a scavenger, eating a variety of food, including microbes and different organic debris, according to previous studies (Tseng et al., 2020). Fang et al. (2023) reported that by intaking and absorbing suspended particles in Kueishantao waters, vent crabs absorb polycyclic aromatic hydrocarbons. Anthropogenic contaminant regulation should be maintained for this unique shallow hydrothermal ecosystem.
MPs were shown to be ingested by zooplankton or adsorbed on algae, as well as adhering to the exoskeleton and appendages of zooplankton with negative impact on their functioning and health (Besseling et al., 2013; Cole et al., 2013). Invertebrates and zooplankton with filter-feeding habits are believed to be more susceptible to swallowing MPs, since they may filter a large amount of seawater (Desforges et al., 2015). Therefore, MPs may be consumed by HV crabs indirectly through zooplankton. We here present firstly that PE-MPs of different intake levels can influence the microbiota and their function in the gut of HV crabs. However, to our knowledge, there was no study reporting physical impacts on microbiota and their function in the crab influenced by MPs. In fact, MPs can influence or interact with environmental microorganisms. Studies described that the effects of MPs on living organisms may be amplified if they are able to enter cells, where they may trigger weight loss, local inflammation, and distribution of energy (Li et al., 2020). However, vent crabs depend on a symbiotic relationship with microorganisms or bacteria in their bodies (Yang et al., 2016). It was reported recently that MPs have an impact on biogeochemical cycles and even animal health through their microbiome (Wang et al., 2021). Taken together, these findings add to the evidence that various chemicals can influence the gut microbiota of crabs.
In conclusion, this pioneering study sheds light on the presence of MPs in the shallow-water HV crab X. testudinatus and their potential impacts on the crab’s microbiome. As MPs are becoming pervasive contaminants in various ecosystems, understanding their effects on marine organisms and their associated microbial communities is of paramount importance. The research involved feeding X. testudinatus with different concentrations of polyethylene particles and utilizing third-generation sequencing technology to analyze changes in the bacterial communities of the gill and digestive gland. The results revealed significant differences in the microbiome communities, particularly in the digestive gland, at the high-intake groups of MPs. Notably, increases in Proteobacteria and Bacteroidetes, accompanied by decreases in Firmicutes and Tenericutes, were observed in response to MP exposure. The findings also indicated that MPs influenced the functions of both the gill and digestive gland. These alterations in the crab’s microbiome and physiological processes raise concerns about potential deleterious effects on the organism’s overall health and well-being. Importantly, this study underscores the need for further research to explore the broader implications of MP consumption by crabs. Understanding whether changes in the gut microbiota can impact the environment’s microbial communities, thereby affecting the biodiversity of shallow-water HVs, is crucial. Additionally, investigating the potential promotion of pathogens that could adversely affect various organ systems of vent biota, including crabs, warrants attention. Given the widespread presence of MPs and their potential impacts on marine organisms and ecosystems, it is imperative to address plastic pollution through effective management strategies and sustainable practices. Continued research into the ecological consequences of MPs on marine life will provide crucial insights for developing conservation measures and mitigating the adverse effects of plastic contamination on the delicate balance of marine ecosystems. Ultimately, safeguarding the health of marine organisms like X. testudinatus and preserving the biodiversity of shallow-water HV environments are vital steps in ensuring the long-term health of our oceans.
The data presented in the study are deposited in the NCBI Sequence Read Archive (SRA) repository, accession number PRJNA957573. The SRA accession numbers for the different sample types are as follows: for low gill samples SRR24288099–SRR24288102, for intermediate gill samples SRR24288124–SRR24288120, for high gill samples SRR24288119–SRR24288116, for low digestive gland samples SRR24288115–SRR24288112, for intermediate digestive gland samples SRR24288111–SRR24288107, and for high digestive gland samples SRR24288106–SRR24288103.
According to the Guide for Animal Use Protocol of the Institutional Animal Care and Use Committee (IACUC) of National Taiwan Ocean University, only experiments with vertebrates require ethical approval. Because crabs are invertebrates, no ethical approval is required.
PM authored the article, analyzed and interpreted the data, and reviewed and edited the article. Y-CL and Y-LC worked on the field sampling, experimentation, and data analysis. GKG assisted in data interpretation, data analysis, article review, and editing. Y-NH prepared the paper and conducted statistical analysis, data analysis and interpretation, and text revision and editing. J-SH supervised project finance, negotiations, and article writing. All authors contributed to the article and approved the submitted version.
Financial support from the National Science and Technology Council, Taiwan (Grant Nos. MOST 108-2621-M-019-003, MOST 109-2621-M-019-002, MOST 110-2621-M-019-001, and MOST 111-2621-M-019-001) and Center of Excellence for Ocean Engineering, NTOU, Taiwan (Grant Nos. 109J13801-51, 110J13801-51, 111J13801-51) to J-SH is acknowledged here. Grant Nos. MOST 109-2313-B-019-003-MY2, MOST 110-2621-M-019-003, and MOST 111-2628-M-019-001-MY3 are to Y-NH.
The authors would like to acknowledge the National Taiwan Ocean University, Institute of Marine Biology, for all the support and assistance in the conduct of this study.
The authors declare that the research was conducted in the absence of any commercial or financial relationships that could be construed as a potential conflict of interest.
All claims expressed in this article are solely those of the authors and do not necessarily represent those of their affiliated organizations, or those of the publisher, the editors and the reviewers. Any product that may be evaluated in this article, or claim that may be made by its manufacturer, is not guaranteed or endorsed by the publisher.
The Supplementary Material for this article can be found online at: https://www.frontiersin.org/articles/10.3389/fevo.2023.1203647/full#supplementary-material
Supplementary Figure 1 | Effects of polyethylene MPs on vent crab body weight change before and after feeding experiments showing the impact of different microplastic concentrations (0%, 0.30%, 0.60%, 1%) on the vent crab.
Supplementary Figure 2 | Alpha-diversity represented by Chao1, Shannon, and Pielou indices at the different concentration levels of gill and digestive gland samples. (A) Indicating alpha diversity of gill samples. (B) Indicating alpha diversity of digestive gland samples. Letters above bars indicate significant differences (P<0.05). Different colors indicate different concentration levels of gill and digestive gland.
Allen G. J. P., Kuan P. L., Tseng Y. C., Hwang P. P., Quijada-Rodriguez A. R., Weihrauch D. (2020). Specialized adaptations allow vent-endemic crabs (Xenograpsus testudinatus) to thrive under extreme environmental hypercapnia. Sci. Rep. 10 (1), 11720. doi: 10.1038/s41598-020-68656-1
Alonso C., Warnecke F., Amann R., Pernthaler J. (2007). High local and global diversity of Flavobacteria in marine plankton. Environ. Microbiol. 9 (5), 1253–1266. doi: 10.1111/j.1462-2920.2007.01244.x
Alvarez-Yela A. C., Mosquera-Rendón J., Noreña-P A., Cristancho M., López-Alvarez D. (2019). Microbial diversity exploration of marine hosts at serrana bank, a coral atoll of the seaflower biosphere reserve. Front. Mar. Science. 6. doi: 10.3389/fmars.2019.00338
Besseling E., Wegner A., Foekema E. M., Van Den Heuvel-Greve M. J., Koelmans A. A. (2013). Effects of microplastic on fitness and PCB bioaccumulation by the lungworm Arenicola marina (L.). Environ. Sci. Technol. 47 (1), 593–600. doi: 10.1021/es302763x
Campbell B. J., Engel A. S., Porter M. L., Takai K. (2006). The versatile epsilon-proteobacteria: key players in sulphidic habitats. Nat. Rev. Microbiol. 4 (6), 458–468. doi: 10.1038/nrmicro1414
Chaiyapechara S., Rungrassamee W., Suriyachay I., Kuncharin Y., Klanchui A., Karoonuthaisiri N., et al. (2012). Bacterial community associated with the intestinal tract of P. monodon in commercial farms. Microbial Ecol. 63 (4), 938–953. doi: 10.1007/s00248-011-9936-2
Chan B. K., Wang T. W., Chen P. C., Lin C. W., Chan T. Y., Tsang L. M. (2016). Community structure of macrobiota and environmental parameters in shallow water hydrothermal vents off Kueishan Island, Taiwan. PloS One 11 (2), e0148675. doi: 10.1371/journal.pone.0148675
Chen Y., Wang X., Wang X., Cheng T., Fu K., Qin Z., et al. (2022). Biofilm structural and functional features on microplastics surfaces in greenhouse agricultural soil. Sustainability 14 (12), 7024. doi: 10.3390/su14127024
Chiu L., Wang M. C., Tseng K. Y., Wei C. L., Lin H. T., Yang S. H., et al. (2022). Shallow-water hydrothermal vent system as an extreme proxy for discovery of microbiome significance in a crustacean holobiont. Front. Mar. Sci. 9. doi: 10.3389/fmars.2022.976255
Cole M., Lindeque P., Fileman E., Halsband C., Goodhead R., Moger J., et al. (2013). Microplastic ingestion by zooplankton. Environ. Sci. Technol. 47 (12), 6646–6655. doi: 10.1021/es400663f
Conway J. R., Lex A., Gehlenborg N. (2017). UpSetR: An R package for the visualization of intersecting sets and their properties. Bioinformatics 33, 2938–2940. doi: 10.1093/bioinformatics/btx364
Corliss J. B., Baross J. A., Hoffman S. E. (1981). A hypothesis concerning the relationships between submarine hot springs and the origin of life on earth. Oceanologica Acta. Special issue.
De Carvalho C. C. C. R., Fernandes P. (2010). Production of metabolites as bacterial responses to the marine environment. Mar. Drugs 8 (3), 705–727. doi: 10.3390/md8030705
Desforges J. P. W., Galbraith M., Ross P. S. (2015). Ingestion of microplastics by zooplankton in the Northeast Pacific Ocean. Arch. Environ. Contam. Toxicol. 69 (3), 320–330. doi: 10.1007/s00244-015-0172-5
Fang J. K. H., Tse T. W., Maboloc E. A., Leung R. K. L., Leung M. M. L., Wong M. W. T., et al. (2023). Adverse impacts of high-density microplastics on juvenile growth and behaviour of the endangered tri-spine horseshoe crab Tachypleus tridentatus. Mar. pollut. Bull. 187, 114535. doi: 10.1016/j.marpolbul.2022.114535
Flint H. J., Scott K. P., Louis P., Duncan S. H. (2012). The role of the gut microbiota in nutrition and health. Nat. Rev. Gastroenterol. Hepatol. 9 (10), 577–589. doi: 10.1038/nrgastro.2012.156
Gola D., Tyagi P. K., Arya A., Chauhan N., Agarwal M., Singh S. K., et al. (2021). The impact of microplastics on marine environment: A review. Environ. Nanotechnol. Monit. Manage. 16, 100552. doi: 10.1016/j.enmm.2021.100552
Hiroshi M., Oda A., Wada S., Kodaka T., Kurosawa K. (2019). First record of a shallow hydrothermal vent crab, Xenograpsus testudinatus, from Shikine-jima Island in the Izu archipelago. Biogeogr. 21, 31–36. doi: 10.11358/biogeo.21.31
Ho T. W., Hwang J. S., Cheung M. K., Kwan H. S., Wong C. K. (2015). Dietary analysis on the shallow-water hydrothermal vent crab Xenograpsus testudinatus using Illumina sequencing. Mar. Biol. 162 (9), 1787–1798. doi: 10.1007/s00227-015-2711-z
Hu M. Y., Guh Y. J., Shao Y. T., Kuan P. L., Chen G. L., Lee J. R., et al. (2016). Strong ion regulatory abilities enable the crab xenograpsus testudinatus to inhabit highly acidified marine vent systems. Front. Physiol. 7. doi: 10.3389/fphys.2016.00014
Hu M., Hagen W., Jeng M. S., Saborowski R. (2012). Metabolic energy demand and food utilization of the hydrothermal vent crab Xenograpsus testudinatus (Crustacea: Brachyura). Aquat. Biol. 15, 11–25. doi: 10.3354/ab00396
Hu J., Zuo J., Li J., Zhang Y., Ai X., Zhang J., et al. (2022). Effects of secondary polyethylene microplastic exposure on crucian carp (Carassius carassius) growth, liver damage, and gut microbiome composition. Sci. Total Environ. 802, 149736. doi: 10.1016/j.scitotenv.2021.149736
Jeng M. S., Ng N. K., Ng P. K. L. (2004). Hydrothermal vent crabs feast on sea ‘snow’. Nature 432 (7020), 969–969. doi: 10.1038/432969a
Jeong C. B., Won E. J., Kang H. M., Lee M. C., Hwang D. S., Hwang U. K., et al. (2016). Microplastic Size-Dependent Toxicity, Oxidative Stress Induction, and p-JNK and p-p38 Activation in the Monogonont Rotifer (Brachionus koreanus). Environ. Sci. Technol. 50 (16), 8849–8857. doi: 10.1021/acs.est.6b01441
Jorquera A., Castillo C., Murillo V., Araya J., Pinochet J., Narváez D., et al. (2022). Physical and anthropogenic drivers shaping the spatial distribution of microplastics in the marine sediments of Chilean fjords. Sci. Total Environ. 814, 152506. doi: 10.1016/j.scitotenv.2021.152506
Ka C., Kato S., Ohkuma M., Yamagishi A. (2011). Microbial insights into the evolution of hydrothermal vent communities. Front. Microbiol. 2. doi: 10.3389/fmicb.2011.00001
Kodama Y., Watanabe K. (2004). Sulfuricurvum kujiense gen. nov., sp. nov., a facultatively anaerobic, chemolithoautotrophic, sulfur-oxidizing bacterium isolated from an underground crude-oil storage cavity. Int. J. Syst. Evol. Microbiol. 54 (Pt 6), 2297–2300. doi: 10.1099/ijs.0.63243-0
Latva M., Dedman C. J., Wright R.J, Polin M., Christie-Oleza J. A. (2022). Microbial pioneers of plastic colonisation in coastal seawaters. Mar. Pollut. Bull. 179, 113701. doi: 10.1016/j.marpolbul.2022.113701
Lee F. J., Hwang J. S., Cheng J. O., Lin H. T., Ko F. C. (2023). Comparison of polycyclic aromatic hydrocarbon accumulation in crab tissues with ambient marine particles from shallow hydrothermal vents, northeast Taiwan. Environ. Res. 217, 114863. doi: 10.1016/j.envres.2022.114863
Li B., Ding Y., Cheng X., Sheng D., Xu Z., Rong Q. (2020). Polyethylene microplastics affect the distribution of gut microbiota and inflammation development in mice. Chemosphere 244, 125492. doi: 10.1016/j.chemosphere.2019.125492
Li Y., Sun Y., Li J., Tang R., Miu Y., Ma X. (2021). “Research on the influence of microplastics on marine life,” in 3rd International conference on air pollution and environmental engineering IOP Conf. Series: earth and environmental science, Vol. 631. (IOP Publishing), 012006. doi: 10.1088/1755-1315/631/1/012006
Liu Z., Yu P., Cai M., Wu D., Zhang M., Chen M., et al. (2019). Effects of microplastics on the innate immunity and intestinal microflora of juvenile Eriocheir sinensis. Sci. Total Environ. 685, 836–846. doi: 10.1016/j.scitotenv.2019.06.265
Lo H. K. A., Chan K. Y. K. (2018). Negative effects of microplastic exposure on growth and development of Crepidula onyx. Environ. pollut. 233, 588–595. doi: 10.1016/j.envpol.2017.10.095
Longnecker K., Reysenbach A. L. (2001). Expansion of the geographic distribution of a novel lineage of ϵ-Proteobacteria to a hydrothermal vent site on the Southern East Pacific Rise. FEMS Microbiol. Ecol. 35 (3), 287–293. doi: 10.1016/S0168-6496(01)00099-X
Louca S., Parfrey L. W., Doebeli M. (2016). Decoupling function and taxonomy in the global ocean microbiome. Science 353 (6305), 1272–1277. doi: 10.1126/science.aaf4507
Mattsson K., Ekvall M., Hansson L.-A., Linse S., Malmendal A., Cedervall T. (2014). Altered behavior, physiology, and metabolism in fish exposed to polystyrene nanoparticles. Environ. Sci. Technol. 49 (1), 553–561. doi: 10.1021/es5053655
McMurdie P. J., Holmes S. (2013). phyloseq: an R package for reproducible interactive analysis and graphics of microbiome census data. PloS One 8 (4), e61217. doi: 10.1371/journal.pone.0061217
Milan M., Carraro L., Fariselli P., Martino M. E., Cavalieri D., Vitali F., et al. (2018). Microbiota and environmental stress: how pollution affects microbial communities in Manila clams. Aquat. Toxicol. 194, 195–207. doi: 10.1016/j.aquatox.2017.11.019
Minic Z., Thongbam P. D. (2011). The biological deep sea hydrothermal vent as a model to study carbon dioxide capturing enzymes. Mar. Drugs 9 (5), 719–738. doi: 10.3390/md9050719
Montero D., Rimoldi S., Torrecillas S., Rapp J., Moroni F., Herrera A., et al. (2022). Impact of polypropylene microplastics and chemical pollutants on European sea bass (Dicentrarchus labrax) gut microbiota and health. Sci. Total Environ. 805, 150402. doi: 10.1016/j.scitotenv.2021.150402
Murphy F., Quinn B. (2018). The effects of microplastic on freshwater Hydra attenuata feeding, morphology & reproduction. Environ. pollut. 234, 487–494. doi: 10.1016/j.envpol.2017.11.029
Nearing J. T., Douglas G. M., Hayes M. G., MacDonald J., Desai D. K., Allward N., et al. (2022). Microbiome differential abundance methods produce different results across 38 datasets. Nat. Commun. 13 (1), 342. doi: 10.1038/s41467-022-28034-z
Ng N. K., Huang J. F., Ho P. H. (2000). National Taiwan Museum Special Publication Series No. 10: 191–199. Proceedings of the Intemational Symposium on Marine Biology in Taiwan - Crustacean and Zooplankton Taxonomy; 2000 March; Ecology and Living Resources; 1998 May 26-27; Taiwan.
Pironti C., Ricciardi M., Motta O., Miele Y., Proto A., Montano L. (2021). Microplastics in the environment: intake through the food web, human exposure and toxicological effects. Toxics 9 (9), 224. doi: 10.3390/toxics9090224
Pita L., Rix L., Slaby B. M., Franke A., Hentschel U. (2018). The sponge holobiont in a changing ocean: from microbes to ecosystems. Microbiome 6 (1), 46. doi: 10.1186/s40168-018-0428-1
Raulf F. F., Fabricius K., Uthicke S., de Beer D., Abed R. M. M., Ramette A. (2015). Changes in microbial communities in coastal sediments along natural CO2 gradient at a volcanic vent in Papua New Guinea. Environ. Microbiol. 17 (10), 3678–3691. doi: 10.1111/1462-2920.12729
Ryan P. G. (2019). “Ingestion of plastics by marine organisms,” in Hazardous chemicals associated with plastics in the marine environment. Eds. Takada H., Karapanagioti H. K. (Amsterdam: Springer International Publishing), 235–266. doi: 10.17159/sajs.2020/7678
Sharma S., Chatterjee S. (2017). Microplastic pollution, a threat to marine ecosystem and human health: a short review. Environ. Sci. pollut. Res. 24 (27), 21530–21547. doi: 10.1007/s11356-017-9910-8
Shenghai H., Zhonglie P., Jiang Z., Fan L., Zhongping C. (2015). <In vivo> microvascular network imaging of the human retina combined with an automatic three-dimensional segmentation method. J. Biomed. Optics 20 (7), 76003. doi: 10.1117/1.JBO.20.7.076003
Sussarellu R., Suquet M., Thomas Y., Lambert C., Fabioux C., Pernet M. E. J., et al. (2016). Oyster reproduction is affected by exposure to polystyrene microplastics. Proc. Natl. Acad. Sci. 113 (9), 2430–2435. doi: 10.1073/pnas.1519019113
Truchet D. M., Buzzi N. S., Moulatlet G. M., Capparelli M. V. (2023). Macroecotoxicological approaches to emerging patterns of microplastic bioaccumulation in crabs from estuarine and marine environments. Sci. Total Environ. 870, 161912. doi: 10.1016/j.scitotenv.2023.161912
Tseng L.-C., Yu P.-Y., Hwang J.-S. (2020). Distribution and sexual dimorphism of the crab Xenograpsus testudinatus from the hydrothermal vent field of Kueishan Island, northeastern Taiwan. PloS One 15 (3), e0230742. doi: 10.1371/journal.pone.0230742
Tsuchida S., Suzuki Y., Fujiwara Y., Kawato M., Uematsu K., Yamanaka T., et al. (2011). Epibiotic association between filamentous bacteria and the vent-associated galatheid crab, Shinkaia crosnieri (Decapoda: Anomura). J. Mar. Biol. Assoc. United Kingdom 91 (1), 23–32. doi: 10.1017/S0025315410001827
Urbina M. A., da Silva Montes C., Schäfer A., Castillo N., Urzúa Á., Lagos M. E. (2023). Slow and steady hurts the crab: Effects of chronic and acute microplastic exposures on a filter feeder crab. Sci. Total Environ. 857 (Pt 1), 159135. doi: 10.1016/j.scitotenv.2022.159135
Usman S., Abdull Razis A. F., Shaari K., Azmai M. N. A., Saad M. Z., Isa N. M., et al. (2022). Polystyrene microplastics induce gut microbiome and metabolome changes in Javanese medaka fish (Oryzias javanicus Bleeker 1854). Toxicol. Rep. 9, 1369–1379. doi: 10.1016/j.toxrep.2022.05.001
Van Dover C. (2002). Trophic relationships among invertebrates at the Kairei hydrothermal vent field (Central Indian Ridge). Mar. Biol. 141 (4), 761–772. doi: 10.1007/s00227-002-0865-y
Van Dover C. L. (2019). Inactive sulfide ecosystems in the deep sea: A review. Front. Mar. Sci. 6. doi: 10.3389/fmars.2019.00461
Vermeiren P., Ikejima K., Uchida Y., Muñoz C. C. (2023). Microplastic distribution among estuarine sedimentary habitats utilized by intertidal crabs. Sci. Total Environ. 866, 161400. doi: 10.1016/j.scitotenv.2023.161400
Waite H. R., Donnelly M. J., Walters L. J. (2018). Quantity and types of microplastics in the organic tissues of the eastern oyster Crassostrea virginica and Atlantic mud crab Panopeus herbstii from a Florida estuary. Mar. pollut. Bull. 129 (1), 179–185. doi: 10.1016/j.marpolbul.2018.02.026
Wan Z., Wang C., Zhou J., Shen M., Wang X., Fu Z., et al. (2019). Effects of polystyrene microplastics on the composition of the microbiome and metabolism in larval zebrafish. Chemosphere 217, 646–658. doi: 10.1016/j.chemosphere.2018.11.070
Wang T. W., Chan T. Y., Chan B. K. K. (2014). Trophic relationships of hydrothermal vent and non-vent communities in the upper sublittoral and upper bathyal zones off Kueishan Island, Taiwan: a combined morphological, gut content analysis and stable isotope approach. Mar. Biol. 161 (11), 2447–2463. doi: 10.1007/s00227-014-2479-6
Wang J., Peng C., Li H., Zhang P., Liu X. (2021). The impact of microplastic-microbe interactions on animal health and biogeochemical cycles: A mini-review. Sci. Total Environ. 773, 145697. doi: 10.1016/j.scitotenv.2021.145697
Wang A. R., Ran C., Ringø E., Zhou Z. G. (2018). Progress in fish gastrointestinal microbiota research. Rev. Aquac. 10 (3), 626–640. doi: 10.1111/raq.12191
Watsuji T., Yamamoto A., Motoki K., Ueda K., Hada E., Takaki Y., et al. (2015). Molecular evidence of digestion and absorption of epibiotic bacterial community by deep-sea crab Shinkaia crosnieri. ISME J. 9 (4), 821–831. doi: 10.1038/ismej.2014.178
Watts A. J. R., Lewis C., Goodhead R. M., Beckett S. J., Moger J., Tyler C. R., et al. (2014). Uptake and retention of microplastics by the shore crab Carcinus maenas. Environ. Sci. Technol. 48 (15), 8823–8830. doi: 10.1021/es501090e
Watts A., Urbina M., Corr S., Lewis C., Galloway T. (2015). Ingestion of plastic microfibers by the crab Carcinus maenas and its effect on food consumption and energy balance. Environ. Sci. Technol. 49 (24), 14597–14604. doi: 10.1021/acs.est.5b04026
Watts A. J., Urbina M. A., Goodhead R., Moger J., Lewis C., Galloway T. S. (2016). Effect of microplastics on the gills of the shore crab Carcinus maenas. Environ. Sci. Technol. 50 (10), 5364–5369. doi: 10.1021/acs.est.6b01187
Weisburg W. G., Barns S. M., Pelletier D. A., Lane D. J. (1991). 16S ribosomal DNA amplification for phylogenetic study. J. Bacteriol. 173 (2), 697–703. doi: 10.1128/jb.173.2.697-703.1991
Wexler H. M. (2007). Bacteroides: the good, the bad, and the nitty-gritty. Clin. Microbiol. Rev. 20 (4), 593–621. doi: 10.1128/cmr.00008-07
Wu J. Y., Lin S. Y., Huang J. F., Chen C. T. A., Hung J. J., Peng S. H., et al. (2022). Differential feeding habits of the shallow-water hydrothermal vent crab Xenograpsus testudinatus correlate with their resident vent types at a scale of meters. Biogeosci. Discuss 2022, 1–22. doi: 10.5194/bg-2022-177
Yan M., Li W., Chen X., He Y., Zhang X., Gong H. (2021). A preliminary study of the association between colonization of microorganism on microplastics and intestinal microbiota in shrimp under natural conditions. J. Hazard. Mater. 408, 124882. doi: 10.1016/j.jhazmat.2020.124882
Yang S.-H., Chiang P.-W., Hsu T.-C., Kao S.-J., Tang S.-L. (2016). Bacterial Community Associated with Organs of Shallow Hydrothermal Vent Crab Xenograpsus testudinatus near Kuishan Island, Taiwan. PloS One 11 (3), e0150597. doi: 10.1371/journal.pone.0150597
Yang C.-H., Wang T.-W., Ng P. K., Chan T.-Y., Lin Y.-Y., Chan B. K. (2022). Population genetic differentiation of the hydrothermal vent crab Xenograpsus testudinatus along depth and geographical gradients in the western pacific. Diversity 14 (3), 162. doi: 10.3390/d14030162
Yang Z., Zhu L., Liu J., Cheng Y., Khor W., Chen A., et al. (2022). Polystyrene microplastics increase Pb bioaccumulation and health damage in the Chinese mitten crab Eriocheir sinensis. Sci. Total Environ. 829, 154586. doi: 10.1016/j.scitotenv.2022.154586
Yuan Z., Nag R., Cummins E. (2022). Human health concerns regarding microplastics in the aquatic environment - From marine to food systems. Sci. Total Environ. 823, 153730. doi: 10.1016/j.scitotenv.2022.153730
Zbinden M., Cambon-Bonavita M. (2003). Occurrence of Deferribacterales and Entomoplasmatales in the deep-sea Alvinocarid shrimp Rimicaris exoculata gut. FEMS Microbiol. Ecol. 46 (1), 23–30. doi: 10.1016/s0168-6496(03)00176-4
Zhang Z., Fan W., Bao W., Chen C.-T. A., Liu S., Cai Y. (2020). Recent developments of exploration and detection of shallow-water hydrothermal systems. Sustainability 12, 9109. doi: 10.3390/su12219109
Keywords: polyethylene, Oxford Nanopore Technologies, chemoheterotrophy, functional group, Xenograpsus testudinatus
Citation: Muthu P, Lee Y-C, Girija GK, Chen Y-L, Ho Y-N and Hwang J-S (2023) Bacterial community responses of the hydrothermal vent crab Xenograpsus testudinatus fed on microplastics. Front. Ecol. Evol. 11:1203647. doi: 10.3389/fevo.2023.1203647
Received: 11 April 2023; Accepted: 03 August 2023;
Published: 31 August 2023.
Edited by:
Wen-tseng Lo, National Sun Yat-sen University, TaiwanReviewed by:
Kwee Siong Tew, NSYSU, TaiwanCopyright © 2023 Muthu, Lee, Girija, Chen, Ho and Hwang. This is an open-access article distributed under the terms of the Creative Commons Attribution License (CC BY). The use, distribution or reproduction in other forums is permitted, provided the original author(s) and the copyright owner(s) are credited and that the original publication in this journal is cited, in accordance with accepted academic practice. No use, distribution or reproduction is permitted which does not comply with these terms.
*Correspondence: Jiang-Shiou Hwang, anNod2FuZ0BtYWlsLm50b3UuZWR1LnR3; Ying-Ning Ho, eW5ob0BtYWlsLm50b3UuZWR1LnR3
Disclaimer: All claims expressed in this article are solely those of the authors and do not necessarily represent those of their affiliated organizations, or those of the publisher, the editors and the reviewers. Any product that may be evaluated in this article or claim that may be made by its manufacturer is not guaranteed or endorsed by the publisher.
Research integrity at Frontiers
Learn more about the work of our research integrity team to safeguard the quality of each article we publish.