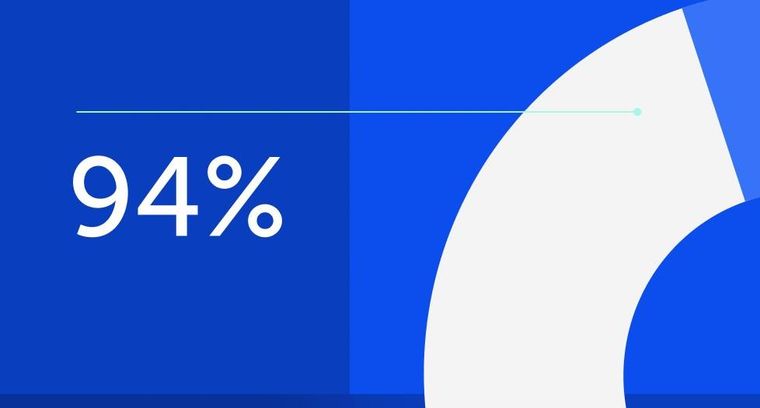
94% of researchers rate our articles as excellent or good
Learn more about the work of our research integrity team to safeguard the quality of each article we publish.
Find out more
ORIGINAL RESEARCH article
Front. Ecol. Evol., 18 August 2023
Sec. Conservation and Restoration Ecology
Volume 11 - 2023 | https://doi.org/10.3389/fevo.2023.1198085
This article is part of the Research TopicKey Determinants of Biodiversity, Ecosystem Functioning and Restoration in Climate Change Sensitive EcosystemsView all 24 articles
In the montane forest-grassland mosaics of the Western Ghats, land cover conversion to silviculture and agriculture over the last five decades has resulted in both loss of natural habitats and widespread invasion of remnant habitat patches. While invasion of the grassland habitats of the mosaic has been relatively well studied, there have been few attempts to understand the extent to which forest habitats (locally known as sholas) have been affected by the spread of exotic species. Here we examine the patterns and impacts of invasion of shola forest understoreys by Cestrum aurantiacum Lindl., an exotic shrub species. At the landscape scale, we demonstrate that the presence and abundance of this invasive in shola understories is negatively related to distance from tea plantations. Further, the intensity of invasion is higher in areas with greater seasonality of temperature and lower mean annual precipitation. At the patch scale, invasion is greatest at shola edges and away from stream courses. We find that C. aurantiacum abundance has negatively affected the regeneration of native shola tree species as well as the abundance of native shola understorey shrubs. Fifty three percent of invaded plots had no native shrubs present. In plots where both C. aurantiacum and native shrubs were present in large enough numbers, we found evidence of negative spatial dependence between stem locations of C. aurantiacum and native shrubs. Our findings have important implications for the management and conservation of these mosaics.
Tropical montane ecosystems occur on all continents across the globe, and are thought to be especially vulnerable to multiple drivers of global change (Loeffler et al., 2011; Salinas et al., 2021). These include climatic changes such as warming and altered precipitation regimes, but also pervasive land-use changes such as the intensification of agriculture, expansion of silviculture and built-up areas for human habitation (He et al., 2023). With complex topographies that support a diversity of natural vegetation types and multiple interacting change drivers, the responses of these ecosystems to ongoing and future global change are complex and difficult to predict (Loeffler et al., 2011; Salinas et al., 2021). For example, rates of invasion of montane ecosystems have been increasing rapidly across the globe, but the reasons for this remain poorly understood (Iseli et al., 2023), and are likely to vary across regions.
The “sky-islands” of the mountain tops of the Western Ghats in southern India, a global biodiversity hotspot, are a tropical montane ecosystem that typifies the above scenario. These forest–grassland mosaics consist of distinctive stunted evergreen forests (locally known as sholas) set in a matrix of grasslands. They are rich in endemic biodiversity and hold great significance, not only from an evolutionary perspective, but also for their provision of critical ecosystem services including climate and hydrological regulation for the entire southern peninsular region (Sukumar et al., 1995; Bose et al., 2016; Joshi et al., 2018). Over the course of the past hundred and fifty years, but accelerating over the past five decades, large sections of these mosaic habitats have been converted to other land uses such as agricultural and silvicultural plantations, mainly at the expense of grasslands (Prabhakar, 1994; Joshi et al., 2018). This has resulted in extensive land-cover change across the region, with more than sixty percent of the grassland habitats converted to exotic tree plantations, and also the widespread invasion of remnant patches of natural habitats (Joshi et al., 2018; Arasumani et al., 2019; Sriramamurthy et al., 2022).
One visible and widely acknowledged effect of the conversion of grasslands to exotic tree plantations has been the increase in invasive alien species in the remnant grasslands (Thomas and Palmer, 2007, pers. obs.). While one of main species of exotic plantation trees, Acacia mearnsii, itself is a dominant and aggressive invader of the remnant natural grasslands (Thomas and Palmer, 2007; Arasumani et al., 2019), other woody invasive shrubs, including scotch broom Cytisus scoparius and common gorse Ulex europaeus have also invaded the grasslands extensively in recent years (Sriramamurthy et al., 2022). While these woody invasions of the grasslands have received lot of research attention (Joshi et al., 2018; Arasumani et al., 2019; Sriramamurthy et al., 2020), far less research attention has been paid to the less visible invasions, often in the understoreys, of shola forest patches. This is an important knowledge gap, as the patterns and consequences of invasions within shola forests are likely to differ from those in grasslands, such that the management of invasives in the sholas versus the grasslands, will require different strategies.
Over the past few decades, shola forests have witnessed the spread of an exotic woody invader, Cestrum aurantiacum Lindl. The genus Cestrum is native to Central and South America where it thrives in montane forests (Monro, 2012). The abundant, attractive and fragrant flowers of this genus are the reason it has been introduced as an ornamental plant in many regions, where it has subsequently become naturalized, and in several cases, turned invasive, including in parts of Africa, Asia, Australia and multiple oceanic islands (Henderson, 2007; Harvey et al., 2012; Junaedi, 2012; Gardener et al., 2013; Padmanaba et al., 2017; Makokha, 2018). In the Indian subcontinent, C. aurantiacum has been reported across many montane regions including the Himalaya, the Western Ghats and in Sri Lanka (Kunwar, 2003; Sajeev et al., 2012; Wijesundara, 2012; Moktan and Das, 2013; Mandal and Joshi, 2015; Nayak et al., 2020). In general, Cestrum spp. are fast-growing and capable of vegetative reproduction (Symon, 1981). C. aurantiacum tends to form dense mats which can suppress the regeneration of other plant species (USDA, 2013; Witt and Luke, 2017). However, few studies have investigated the impacts of invasion by C. aurantiacum on native forest communities, and there is little primary data on the ecology and impacts of this particular species.
Here, we investigated the correlates and consequences of the invasion of shola forest communities by C. aurantiacum. Because C. aurantiacum was introduced as an ornamental plant in tea plantations, we hypothesized that sholas near tea plantations would be more heavily invaded by C. aurantiacum than sholas further away. We also expected that C. aurantiacum abundance within sholas would be related to other climatic and habitat factors such as rainfall and local topography, which influence stand structure and soil moisture, and thereby the optimal conditions for this species. Finally, we expected that C. aurantiacum invasion has led to reduced native shola tree regeneration, as well as reduced abundances of native shola understorey shrubs, possibly through negative competitive interactions.
The study was conducted across 60 km2 in the western and southern parts of the Upper Nilgiris Plateau (11.17°N,76.77°E and 11.50°N, 76.43°E), that still hold large areas of natural shola–grassland mosaics, dating to at least 40,000 years ago (Caner et al., 2007). Please see Supplementary Figure S1 for a map of the study area. Other dominant land cover types in the region, such as non-native tree plantations and commercial tea plantations, were established relatively recently (Prabhakar, 1994), predominantly through the conversion of natural grasslands (Joshi et al., 2018). The region is rich in endemic plants (Blasco, 1971) and has extraordinary vertical and horizontal physiographic differentiation. Mean annual rainfall ranges from above 2500 mm on the Western side to 1200 mm towards the east (Von Lengerke, 1977; Caner et al., 2007). The dry season lasts for 3–4 months mainly between December and March. Temperature ranges from a mean maximum of 24 °C in April to a mean minimum of 5 °C in December. Frost occurs between November and March and mainly in the valleys rather than the higher hill slopes (Von Lengerke, 1977; Caner et al., 2007). The elevation range covered in this study extends from 1750–2400 m ASL.
The genus Cestrum in the family Solanaceae has 175 known species of shrubs, vines and small trees (de Rojas and D’Arcy, 1998; Monro, 2012). The native range for this genus is Central and South America (Monro, 2012). Here most Cestrum species occur in montane areas, above 800 m elevation, in cloud forests and conifer and oak forests (de Rojas and D’Arcy, 1998; Monro, 2012). Introduced as ornamentals in various parts of the world, many species of this genus have now become invasive. Most Cestrum spp. bear berries with small seeds that remain viable in the seed bank and are bird-dispersed (Marambe et al., 2001; Geldenhuys, 2004; Gardener et al., 2013). They are also shade-tolerant (Geldenhuys, 2004), drought-tolerant, capable of growing on poor soils and have invaded a range of habitats from coastal dunes to savannahs, grasslands, plantations and closed forest (Henderson, 2007). Most are quite toxic to livestock, native mammals and humans (de Rojas and D’Arcy, 1998; Makokha, 2018). For these reasons, they are labelled as noxious weeds with moderate to high invasive potential (Nel et al., 2004; Henderson, 2007). In South Africa and Australia, extensive programs have been undertaken to clear areas of Cestrum species (Macdonald and Jarman, 1985; Stockard, 1996; Marais and Wannenburgh, 2008).
C. aurantiacum is an evergreen climbing shrub 1–6 m tall, with thin, unpleasant smelling leaves that are toxic to livestock. It is native to central America (Costa Rica, Guatemala, Honduras, Mexico and Nicaragua; CABI, 2023). In many parts of its invaded range, C. aurantiacum occurs in montane forests, between 1500 to above 2000m (Junaedi, 2012; Sajeev et al., 2012; Wijesundara, 2012; Moktan and Das, 2013; Makokha, 2018; Witt et al., 2018). In the Nilgiris, it has successfully invaded native forest fragments and the understorey of tree plantations above 2000 m (Saravanan et al., 2014, Figure 1). It appears to have spread from settled areas and tea plantations, where its abundance is highest (AAD pers. obs) and was likely imported as an ornamental plant for the estate managers’ bungalows. In its native range, it appears to be well adapted to the cloud forest environment (de Rojas and D’Arcy, 1998; Monro, 2012), which would allow it to thrive in the dense shade of sholas.
Figure 1 (A) Shola understorey with native shrubs (Psychotria spp.) present. (B) Shola understorey invaded by Cestrum aurantiacum with no native shrubs visible.
Shola woody communities were sampled using 0.04 ha plots (n = 87), that were located using a stratified random sampling design based on topography and surrounding land cover. Field data were collected between 2010–2012 (Das et al., 2017). We sampled a total of 52 shola forest patches in varying landscape contexts (i.e., natural grassland, tea plantation and non-native tree plantations). Within forest patches, plots were spaced at least 50 m apart. Species identity, height and diameter at breast height (dbh) were recorded for individuals >1 cm dbh. We also recorded the position of individual trees and shrubs within the plot by dividing it into 5m blocks and mapping the location of each stem within each block. Two transects of four 1 × 1 m seedling plots each were laid across each vegetation plot. Seedlings (individuals <50 cm height) of all woody species within these plots were censused. Distance to the nearest forest edge, GPS location of the plot corner, elevation, slope and aspect were also recorded in the field. We confirmed species identities using published flora (Gamble, 1923; Ramesh et al., 2008) and the help of an experienced taxonomist.
We modelled C. aurantiacum presence as a function of distance to the closest tea plantation edge using a GLM with binomial error and a logit link function (McCullach and Nelder, 1989). The results were used to find a threshold distance from tea plantations beyond which the probability of C. aurantiacum occurrence approached zero. We used this threshold to identify a subset of study plots within which C. aurantiacum presence was likely based on their distance from a tea plantation edge (n = 54). This was done to ensure that subsequent analysis of the correlates of C. aurantiacum abundance was free from the issue of zero-inflation (Martin et al., 2005). We used data from these plots to model C. aurantiacum abundance as a function of bio-climatic and habitat variables listed as follows: temperature seasonality (standard deviation of monthly temperature averages), mean annual precipitation, CV of precipitation (i.e., variation in monthly precipitation within a year), distance to tea plantation, distance to nearest shola edge, distance to stream. Bioclimatic predictors were sourced from Hijmans et al. (2005). This data is derived from interpolations of existing weather station data at a 1 km2 spatial resolution. The values represent long term averages between 1950 and 2000. Distance from tea plantation was measured using high resolution imagery in Google Earth (Google Earth, 2013). Distance from nearest forest edge was recorded in the field and distance to stream was calculated in QGIS after deriving a stream network from a DEM with 30m resolution (METI and NASA, 2011). All predictors were checked for collinearity and standardized prior to running the models. A set of competing models using these predictors were compared using Akaike Information Criteria to identify the model that best predicted C. aurantiacum abundance.
To assess impacts of C. aurantiacum invasion on native woody plants, we tested whether the number of shola tree seedlings and native shrubs in the plot were related to C. aurantiacum abundance using GLMs with a Poisson error term and a log link function. Analysis of spatial point patterns has been used to assess the presence of competitive interactions between plants (Gray and He, 2009; Pescador et al., 2020). Here, we assessed evidence for competitive interactions between C. aurantiacum and native shrub species by testing whether native shrubs (individuals belonging to three genera: Psychotria, Lasianthus and Tarenna) were located farther from C. aurantiacum individuals than what would be expected if their distributions were independent at the plot level. As most of the plots invaded by C. aurantiacum had no native shrubs present in the understorey, this test was run on only three plots which had sufficient sample size for both C. aurantiacum as well as native shrubs. We first tested whether the point pattern of C. aurantiacum and native shrubs within the plot conformed to a homogenous Poisson point process by dividing each of the three plots into nine sub plots and conducting a x2 test to assess whether the point pattern departed from complete spatial randomness. After confirming homogeneity of the observed point pattern, we used the cross-type L-function (Lcross), a linearized version of Ripley’s K function for multitype point patterns (Baddeley et al., 2016), to assess whether the point locations of native shrubs showed evidence of competitive inhibition relative to a null hypothesis in which their locations were independent of those of C. aurantiacum within each plot. The Ripley’s K function for multitype points quantifies spatial aggregation between points of different types within a circle of radius r around a given focal point (Baddeley et al., 2016). The null hypothesis was modelled by splitting the data into the sub-patterns of points of each type and randomly shifting each of these sub-patterns, independently of the other using a toroidal shift and then calculating Lcross for the plot (Baddeley et al., 2016). As we had a square plot (20 × 20 m), we also used a toroidal shift to correct for edge effects while estimating Lcross (Baddeley et al., 2016). We used Monte Carlo simulations to test the significance of Lcross at the α = 0.05 level (Baddeley et al., 2016). All analyses were conducted in QGIS v.3.22 (QGIS Development Team, 2022) and statistical software R v.4.2.1 (R Core Team, 2022) using the packages: ‘spatstat’ (Baddeley et al., 2016), ‘maptools’ (Bivand and Lewin-Koh 2022).
C. aurantiacum was present in 17 of the 87 (19.5%) study plots. In these plots, the number of mature individual C. aurantiacum stems ranged from 1–54 (mean of 17.6). The probability of C. aurantiacum presence was greatest between 0–2 km from a tea plantation edge and fell to near zero beyond 4 km from a tea plantation edge (Figure 2). Within plots ≤ 4 km from a tea plantation edge (n = 54), C. aurantiacum abundance was influenced by both bio-climatic (temperature seasonality and annual precipitation) and habitat factors (i.e., distance to tea edge, distance to shola fragment edge, distance to stream; Table 1). The β for seasonality of temperature (0.015 [0.0009]) indicates that C. aurantiacum abundance is higher in areas with greater seasonality of temperature. Cestrum abundance decreases with mean annual precipitation (−0.004 [0.0005]). Abundance decreases with distance from the shola edge (−0.014 [0.002]) and tea plantations (−0.0001 [0.0001]) and increases with distance from stream (0.0003 [0.0007]) (Figure 3).
Figure 2 Probability of Cestrum aurantiacum presence in shola forest patches in the Upper Nilgiris modelled as a function of distance from tea plantation edge in meters. Modelled as a GLM with binomial error.
Table 1 Results of model selection using GLMs with Poisson error to model Cestrum aurantiacum abundance in sholas as a function of bio-climatic and distance variables.
Figure 3 Cestrum aurantiacum abundance in sholas modelled as a function of distance to tea plantation edge, distance to nearest shola fragment edge and distance to stream, modelled using GLMs with Poisson errors.
Most of the plots (53%) where C. aurantiacum was present did not have any individuals belonging to native shrub genera. The number of individuals of native shrub species in the plot was significantly negatively related to Cestrum abundance (β = −0.07 [0.005], P < 0.001). Cestrum abundance had a significant but weak negative relationship with the number of native shola tree seedlings in a plot (−0.009 [0.002], P < 0.001; Figure S2). However, the number of shola tree saplings in a plot did not show a significant relationship to C. aurantiacum abundance (0.00006 [0.0007], P > 0.1).
The results of the point pattern analysis indicate support for competitive inhibition of native shrubs by C. aurantiacum. The Lcross metric indicates greater separation between native shrub locations and C. aurantiacum locations than expected under spatial independence, at scales of approximately 1–5 meters in two of the three plots, and some evidence in support of spatial dependence at the 0.5–1.5 m scale in the third plot (Figure 4).
Figure 4 Lcross function for point locations of Cestrum aurantiacum (CA) and native shrub species (NS) in three plots in the Upper Nilgiris shola forests. In each case, the black solid line shows observed value while the red dashed line represents the expectation under complete spatial independence between the locations of CA and NS. Values of L (CA, NS) r > r indicate spatial aggregation, while L (CA, NS) r < r indicates spatial regularity. The grey shaded area represents the 95% confidence envelope of the Lcross function under the null expectation of spatial independence, calculated using Monte Carlo simulations.
More than half (53%) of the woody species encountered in this study are endemic to the Western Ghats (Ramesh and Pascal, 1997). Woody invasive shrubs such as Lantana camara (< 2000 m; Najar et al., 2019) and C. aurantiacum (> 2000m) threaten this unique biodiversity. Here we show that the invasive spread of C. aurantiacum in the Upper Nilgiris is associated with the presence of tea plantations, as native shola forests embedded within a matrix of tea estates or within 4 km from a tea plantation edge, were more likely to have this species in the understorey, with its abundance increasing in sholas closer to tea plantations. Further, we found that increasing C. aurantiacum abundance appeared to negatively impact the presence and abundance of dominant native shrub genera Psychotria, Lasianthus and Tarenna as well as shola seedling regeneration. A number of studies including Bartuszevige et al. (2006); González-Moreno et al. (2013); Chen et al. (2017); Shiferaw et al. (2019), and the synthetic review by Vilà and Ibáñez (2011) have similarly found that landscape attributes play an important role (or a more important role than local site factors) in driving the presence of invasive species or the variation in invasion risk across space, while Milbau et al. (2009) outline a hierarchical framework where “…factors operating at a smaller scale are subordinate to factors operating at a larger scale, but if conditions at higher levels are satisfied, the small-scale factors may become indispensable for making accurate predictions”.
C. aurantiacum abundance was positively related to annual temperature seasonality – which in turn is highly negatively correlated to the elevation gradient in this study, indicating lower bio-climatic suitability at the highest part of the elevation gradient in this study (2200–2400 m). This species is susceptible to frost damage (AAD pers. obs.), which could explain why it does not occur in open grasslands (where frost occurs; Joshi et al., 2020) but rather along roads (Nayak et al., 2020) and other edges where some shrub or tree cover is present (Jobin et al., 2023). The conversion of large expanses of native grasslands to timber plantations, tea and other landuses (Prabhakar, 1994; Joshi et al., 2018) may thus have facilitated the spread of this species, by creating connected edge habitats with suitable microclimatic conditions (reduced extent and intensity of frost; Von Lengerke, 1977) across the landscape.
Land cover changes and associated changes in anthropogenic disturbances may also favour the spread of this invasive species through the opening of canopies that increase light availability in the understory (Lozano and MacIsaac, 1997; Iseli et al., 2023). Junaedi (2012) found that C. aurantiacum presence was positively related to light intensity. Wijesundara (2012) reports it spreading in montane forest die-back gaps in Sri Lanka. Here we found the species to be more abundant in plots close to shola–tea plantation edges, which are more likely to have greater light penetration due to human disturbance. Finally, land cover changes are often associated with changes in the composition of pollinator and disperser communities (Raman, 2006), which in turn can facilitate invasive spread. In the Nilgiris, C. aurantiacum seeds are dispersed by common bird species that thrive in anthropogenic habitats, like the red-whiskered bulbul (AAD pers. obs.). In Sri Lanka, its seeds are dispersed by the yellow-eared bulbul (Wijesundara, 2012), while flowers are reported to be pollinated by the Sri Lankan white-eye (Zosterops ceylonensis; Wijesundara, 2012).
Shola seedling regeneration was found to be lower in C. aurantiacum invaded sites. A similar finding has been reported for Lantana invaded sites in the Upper Nilgiris (Najar et al., 2019). The negative relationship between C. aurantiacum and native seedling regeneration could be due to either direct competitive effects or allelopathic interactions (Callaway and Ridenour, 2004). Cestrum spp. are reported to have anti-microbial properties (Prasad et al., 2013), which may lead to altered soil microbial communities in invaded sites (Elgersma and Ehrenfeld, 2011), thereby affecting native seedling regeneration. Alternatively, this association could also arise due to greater human disturbance in sholas near tea plantations, leading to lower levels of native species regeneration, while also allowing C. aurantiacum to spread faster (Lozano and MacIsaac, 1997). Further research is needed to elucidate the mechanisms behind this observation.
We found a strong negative relationship between C. aurantiacum abundance and the dominant native shrubs of the shola understorey, with some evidence in support of negative spatial interactions within the plot. There are several factors that could contribute to the impact of C. aurantiacum on native shrub populations. For instance, C. aurantiacum is native to cloud forest understoreys of central America and may therefore be well adapted to the microclimatic conditions of shola forest understoreys. This could enhance its impact within the context of this habitat (Kestrup and Ricciardi, 2009). It also grows in denser stands than native species, which has been associated with stronger impacts (Hejda et al., 2009). The combination of such environmental matching and greater fecundity (discussed below), could lead to large increases in abundance of C. aurantiacum in shola understories, sufficient to exclude native shrubs in parts of their range (MacDougall et al., 2009; Ricciardi et al., 2013).
While C. aurantiacum and native shrubs share common abiotic habitat requirements, they differ phylogenetically and also in key traits linked to growth and resource acquisition (trait divergence or phylogenetic distinctiveness; Ricciardi and Atkinson, 2004). Native shola understorey dominants all belong to the family Rubiaceae, while C. aurantiacum is a member of Solanaceae. Further, it displays traits associated with fast growth and rapid resource capture in comparison to native shrubs (high specific leaf area (SLA) – thinner, larger, more easily bruised leaves, low stem specific density; AAD pers. obsv.). Therefore, C. aurantiacum may avoid the effects of competitive interactions with native shrub species (Levine et al., 2003) by being sufficiently different from them in terms of phylogeny and key traits (Sofaer et al., 2018; Pearse et al., 2019).
Finally, C. aurantiacum exhibits characteristics associated with greater fecundity compared to native shrubs, i.e., more frequent and profuse flowering and fruiting (AAD pers. obs.). A South African study found it had comparable levels of fruit set to Lantana (Rambuda, 2001). Further research is required to assess the presence and relative contribution of each of the factors discussed above to the magnitude of C. aurantiacum impact on native shrubs.
Upper montane forests in the tropics and subtropics, like the sholas, often occur as relatively small patches (<10 ha), that are separated by native grasslands, tea plantations or non-native timber stands (Wijesundara, 2012; Das et al., 2017). Hence, these habitats may be more vulnerable to impacts of invasion in the same way that islands are, due to their restricted area and isolation (Pyšek et al., 2012). In particular, they may exhibit a different form of the relationship between invader abundance and per capita impact compared to continuous forest ecosystems, with associated implications for the timing and nature of management interventions (Sofaer et al., 2018; Strayer, 2020).
Tea estates constitute approximately 26% of the Upper Nilgiris Plateau above 1400 m ASL (Arasumani et al., 2019) and therefore potentially pose a serious threat to native forests through propagule rain from invasive plants. We observed wide variation in the quality of tea estate (holdings >100 ha) management across the landscape, ranging from abandonment of large areas planted with tea to intensely managed tea plantations. Shola patches in Korakundah tea estate, which has numerous certifications for ecological sustainability and fair trade, did not have C. aurantiacum in the understorey, indicating that estate management based on best practices could be effective in controlling the spread of this species. The COVID pandemic and related restrictions have probably hampered estate upkeep and management through shortages of tea estate labour and management personnel in areas important for conservation (Bates et al., 2021).
More recent work (Jobin et al., 2023) indicates that C. aurantiacum is also growing in the understorey of non-native timber plantations. It is also common in settlements and along road margins (AAD pers obs),. Together these landcover types probably contribute massive amounts of seed rain from C. aurantiacum across the Upper Nilgiris. Therefore, urgent attention to control of this species (particularly along road margins) in production and forestry landscapes surrounding natural shola forests – specifically targeting the interface between sholas and the surrounding land cover – is critical to mitigate the impacts of invasion. Experimental studies comparing the relative effectiveness of control methods for this species at different levels of invasion should be prioritized along with the restoration of native shola species (Mohandass et al., 2016; Najar et al., 2019).
The raw data supporting the conclusions of this article will be made available by the authors, without undue reservation.
AAD conceptualized the research, collected the primary field data, ran the analysis and wrote and edited the manuscript. JR helped with conceptualization of the manuscript, and wrote and edited the manuscript. DJ helped with collection and analysis of field data, visualized the results, and edited the manuscript. All authors contributed to the article and approved the submitted version.
Funding for field data collection was provided by the ATREE-NORAGRIC grant. Further financial support for AAD from the DBT-RA programme in Biotechnology & Life Sciences is gratefully acknowledged.
We thank Tamil Nadu Forest Department for granting fieldwork permits, and the management of Korakundah, Royal Valley, Thia Shola and Prospect Tea Estates for field assistance. We thank Uma Ramakrishnan for supporting AAD during her postdoctoral work. We are grateful to K.S. Bawa and T. Ganesh for guidance and support. We also thank Kartik Shanker and Siddharth Krishnan for their assistance with logistics during field data collection and R. Ganesan for taxonomic assistance. Paul Dorai, V. Rathish, Kishore and Thorthai Gooden assisted with field data collection. We are grateful to Mandira Banerji for her support. Finally, we thank the reviewers for their comments on the manuscript.
The authors declare that the research was conducted in the absence of any commercial or financial relationships that could be construed as a potential conflict of interest.
All claims expressed in this article are solely those of the authors and do not necessarily represent those of their affiliated organizations, or those of the publisher, the editors and the reviewers. Any product that may be evaluated in this article, or claim that may be made by its manufacturer, is not guaranteed or endorsed by the publisher.
The Supplementary Material for this article can be found online at: https://www.frontiersin.org/articles/10.3389/fevo.2023.1198085/full#supplementary-material
Arasumani M., Khan D., Vishnudas C. K., Muthukumar M., Bunyan M., Robin V. V., et al. (2019). Invasion compounds an ecosystem-wide loss to afforestation in the tropical grasslands of the Shola Sky Islands. Biol. Conserv. 230, 141–150. doi: 10.1016/j.biocon.2018.12.019
Baddeley A., Rubak E., Turner R. (2016). Spatial point patterns: methodology and applications with R (Boca Raton, Florida, USA: CRC press).
Bartuszevige A. M., Gorchov D. L., Raab L. (2006). The relative importance of landscape and community features in the invasion of an exotic shrub in a fragmented landscape. Ecography 29, 213–222. doi: 10.1111/j.2006.0906-7590.04359.x
Bates A. E., Primack R. B., Biggar B. S., Bird T. J., Clinton M. E., Command R. J., et al. (2021). Global COVID-19 lockdown highlights humans as both threats and custodians of the environment. Biol. Conserv. 263, 109175. doi: 10.1016/j.biocon.2021.109175
Bivand R., Lewin-Koh N. (2022). maptools: Tools for Handling Spatial Objects. R package version 1.1-6. Available at: https://CRAN.R-project.org/package=maptools.
Blasco F. (1971). Montagnes du Sud de l’Inde: forêts, savanes, écologie (Pondicherry: Institut Français de Pondicherry. French Institute of Pondicherry).
Bose R., Munoz F., Ramesh B. R., Pelissier R. (2016). Past potential habitats shed light on the biogeography of endemic tree species of the Western Ghats biodiversity hotspot, South India. Journal of Biogeography 43, 899–910.
Callaway R. M., Ridenour W. M. (2004). Novel weapons: invasive success and the evolution of increased competitive ability. Front. Ecol. Environ. 2, 436–443. doi: 10.1890/1540-9295(2004)002[0436:NWISAT]2.0.CO;2
Caner L., Seen D. L., Gunnell Y., Ramesh B. R., Bourgeon G. (2007). Spatial heterogeneity of land cover response to climatic change in the Nilgiri highlands (southern India) since the Last Glacial Maximum. Holocene 17, 195–205. doi: 10.1177/0959683607075833
Chen C., Wu S., Meurk C. D., Ma M., Zhao J., Tong X. (2017). Effects of local and landscape factors on exotic vegetation in the riparian zone of a regulated river: Implications for reservoir conservation. Landscape Urban Plann. 157, 45–55. doi: 10.1016/j.landurbplan.2016.06.003
Das A. A., John R., Anand M. (2017). Does structural connectivity influence tree species distributions and abundance in a naturally discontinuous tropical forest formation? J. Vegetation Sci. 28, 7–18. doi: 10.1111/jvs.12474
de Rojas C. B., D’Arcy W. G. (1998). The genera cestrum and sessea (Solanaceae: cestreae) in Venezuela. Ann. Missouri Bot. Garden 85, 273–351. doi: 10.2307/2992010
Elgersma K. J., Ehrenfeld J. G. (2011). Linear and non-linear impacts of a non-native plant invasion on soil microbial community structure and function. Biol. Invasions 13, 757–768. doi: 10.1007/s10530-010-9866-9
Gardener M. R., Trueman M., Buddenhagen C., Heleno R., Jäger H., Atkinson R., et al. (2013). A Pragmatic Approach to the Management of Plant Invasions in Galapagos | SpringerLink. Plant Invasions in Protected Areas: Patterns, Problems and Challenges, Invading Nature (Dordrecht: Springer).
Geldenhuys C. J. (2004). Concepts and process to control invader plants in and around natural evergreen forest in South Africa1. Weed Technol. 18, 1386–1391. doi: 10.1614/0890-037X(2004)018[1386:CAPTCI]2.0.CO;2
QGIS Development Team. (2022). QGIS Geographic Information System. QGIS Association. Available at: http://www.qgis.or.
González-Moreno P., Pino J., Carreras D., Basnou C., Fernández-Rebollar I., Vila M. (2013). Quantifying the landscape influence on plant invasions in Mediterranean coastal habitats. Landscape Ecol. 28, 891–903. doi: 10.1007/s10980-013-9857-1
Google Earth. (2013). Satellite images for southern India. Data providers: Digital Globe and Cnes/SPOT. Imagery dated 2008-2013. Available at: www.googleearth.com (Accessed July and August 2013).
Gray L., He F. (2009). Spatial point-pattern analysis for detecting density-dependent competition in a boreal chronosequence of Alberta. For. Ecol. Manage. 259, 98–106. doi: 10.1016/j.foreco.2009.09.048
Harvey K. J., Nipperess D. A., Britton D. R., Hughes L. (2012). Australian family ties: does a lack of relatives help invasive plants escape natural enemies? Biol. Invasions 14, 2423–2434. doi: 10.1007/s10530-012-0239-4
He X., Ziegler A. D., Elsen P. R., Feng Y., Baker J. C., Liang S., et al. (2023). Accelerating global mountain forest loss threatens biodiversity hotspots. One Earth 6, 303–315. doi: 10.1016/j.oneear.2023.02.005
Hejda M., Pyšek P., Jarošík V. (2009). Impact of invasive plants on the species richness, diversity and composition of invaded communities. J. Ecol. 97, 393–403. doi: 10.1111/j.1365-2745.2009.01480.x
Henderson L. (2007). Invasive, naturalized and casual alien plants in southern Africa: a summary based on the Southern African Plant Invaders Atlas (SAPIA). Bothalia 37, 215–248. doi: 10.4102/abc.v37i2.322
Hijmans R. J., Cameron S. E., Parra J. L., Jones P. G., Jarvis A. (2005). Very high resolution interpolated climate surfaces for global land areas. Int. J. Climatol. 25, 1965–1978. doi: 10.1002/joc.1276
Iseli E., Chisholm C., Lenoir J., Haider S., Seipel T., Barros A., et al. (2023). Rapid upwards spread of non-native plants in mountains across continents. Nat. Ecol. Evol. 7, 405–413. doi: 10.1038/s41559-022-01979-6
Jobin V., Das A., Harikrishnan C. P., Chanda R., Lawrence S., Robin V. V. (2023). Patterns of understory invasion in invasive timber stands of a tropical sky island. Ecol. Evolution. 13 (4), e9995. doi: 10.1002/ece3.9995
Joshi A. A., Ratnam J., Sankaran M. (2020). Frost maintains forests and grasslands as alternate states in a montane tropical forest–grassland mosaic; but alien tree invasion and warming can disrupt this balance. J. Ecol. 108, 122–132. doi: 10.1111/1365-2745.13239
Joshi A. A., Sankaran M., Ratnam J. (2018). ‘Foresting’ the grassland: Historical management legacies in forest-grassland mosaics in southern India, and lessons for the conservation of tropical grassy biomes. Biol. Conserv. 224, 144–152. doi: 10.1016/j.biocon.2018.05.029
Junaedi D. I. (2012). Invasive plants in mountainous remnant forest: recommendation for choosing best decision for invasive species management of Cestrum aurantiacum Lindl. Buletin Kebun Raya 15, 37–47.
Kestrup Å. M., Ricciardi A. (2009). Environmental heterogeneity limits the local dominance of an invasive freshwater crustacean. Biol. Invasions 11, 2095–2105. doi: 10.1007/s10530-009-9490-8
Kunwar R. M. (2003). Invasive alien plants and Eupatorium: Biodiversity and livelihood. Himalayan J. Sci. 1, 129–133. doi: 10.3126/hjs.v1i2.213
Levine J. M., Vila M., Antonio C. M. D., Dukes J. S., Grigulis K., Lavorel S. (2003). Mechanisms underlying the impacts of exotic plant invasions. Proc. R. Soc. London Ser. B.: Biol. Sci. 270, 775–781. doi: 10.1098/rspb.2003.2327
Loeffler J., Anschlag K., Baker B., Finch O. D., Diekkrueger B., Wundram D., et al. (2011). Mountain ecosystem response to global change. Erdkunde 65, 189–213. doi: 10.3112/erdkunde.2011.02.06
Lozano J. D., MacIsaac H. J. (1997). Biological invasions: are they dependent on disturbance? Environ. Rev. 5, 131–144. doi: 10.1139/a97-007
Macdonald I. A. W., Jarman M. (1985). Invasive alien plants in the terrestrial ecosystems of Natal, South Africa (National Scientific Programmes Unit: CSIR).
MacDougall A. S., Gilbert B., Levine J. M. (2009). Plant invasions and the niche. J. Ecol. 97, 609–615. doi: 10.1111/j.1365-2745.2009.01514.x
Makokha J. (2018). Invasion of Cestrum aurantiacum Lindl. in Kenya. J. Environ. Prot. 9, 671–690. doi: 10.4236/jep.2018.96042
Mandal G., Joshi S. P. (2015). Estimation of above-ground biomass and carbon stock of an invasive woody shrub in the subtropical deciduous forests of Doon Valley, western Himalaya, India. J. Forestry Res. 26, 291–305. doi: 10.1007/s11676-015-0038-8
Marais C., Wannenburgh A. (2008). Restoration of water resources (natural capital) through the clearing of invasive alien plants from riparian areas in South Africa—costs and water benefits. South Afr. J. Bot. 74, 526–537. doi: 10.1016/j.sajb.2008.01.175
Marambe B., Bambaradeniya C., Kumara D. P., Pallewatta N. (2001). Human dimensions of invasive alien species in Sri Lanka. The Great Reshuffling: Human Dimensions of Invasive Alien Species IUCN, Cambridge. 135–144.
Martin T. G., Wintle B. A., Rhodes J. R., Kuhnert P. M., Field S. A., Low‐Choy S. J., et al. (2005). Zero tolerance ecology: improving ecological inference by modelling the source of zero observations. Ecol. Lett. 8, 1235–1246. doi: 10.1111/j.1461-0248.2005.00826.x
METI, NASA. (2011). ASTER Global DEM version 2. Available at: http://gdem.ersdac.jspacesystems.or.jp (Accessed July 12th 2013).
Milbau A., Stout J. C., Graae B. J., Nijs I. (2009). A hierarchical framework for integrating invasibility experiments incorporating different factors and spatial scales. Biol. Invasions 11, 941–950. doi: 10.1007/s10530-008-9306-2
Mohandass D., Chhabra T., Pannu R. S., Beng K. C. (2016). Recruitment of saplings in active tea plantations of the Nilgiri Mountains: Implications for restoration ecology. Trop. Ecol. 57, 101–118.
Moktan S., Das A. (2013). Diversity and distribution of invasive alien plants along the altitudinal gradient in Darjiling Himalaya, India. Pleione 7, 305–313.
Monro A. K. (2012). Eight new species of Cestrum (Solanaceae) from Mesoamerica. PhytoKeys 8, 49–82. doi: 10.3897/phytokeys.8.2238
Najar M. U. I., Puyravaud J.-P., Davidar P. (2019). Shola tree regeneration is lower under Lantana camara L. thickets in the upper Nilgiris plateau, India. J. Threatened Taxa 11, 14562–14568. doi: 10.11609/jott.4918.11.12.14562-14568
Nayak R., Verma A. K., Manika N., Bargali K., Pandey V. N., Behera S. K., et al. (2020). Alien species in the flora of Sikkim Himalaya, India. J. Economic Taxonomic Bot. 4, 119–137.
Nel J. L., Richardson D. M., Rouget M., Mgidi T. N., Mdzeke N., Le Maitre D. C., et al. (2004). A proposed classification of invasive alien plant species in South Africa: towards prioritizing species and areas for management action: working for water. South Afr. J. Sci. 100, 53–64. doi: 10.10520/EJC96213
Padmanaba M., Tomlinson K. W., Hughes A. C., Corlett R. T. (2017). Alien plant invasions of protected areas in Java, Indonesia. Sci. Rep. 7, 1–11. doi: 10.1038/s41598-017-09768-z
Pearse I. S., Sofaer H. R., Zaya D. N., Spyreas G. (2019). Non-native plants have greater impacts because of differing per-capita effects and nonlinear abundance–impact curves. Ecol. Lett. 22, 1214–1220. doi: 10.1111/ele.13284
Pescador D. S., de la Cruz M., Chacón-Labella J., Pavón‐García J., Escudero A. (2020). Tales from the underground: Soil heterogeneity and not only above-ground plant interactions explain fine-scale species patterns in a Mediterranean dwarf-shrubland. J. Vegetation Sci. 31, 497–508. doi: 10.1111/jvs.12859
Prabhakar R. (1994). Resource, Use, Culture And Ecological Change: A Case Study Of The Nilgiri Hills Of Southern India (PhD Thesis) (Bangalore: Indian Institute of Science).
Prasad M., Prabhu A., Thakur M. S., Ruparel Y. M. (2013). Phytochemical screening, anti-oxidant potential and antimicrobial activities in three species of Cestrum plants. Int. J. Pharma Bio Sci. 4, B673–B678.
Pyšek P., Jarošík V., Hulme P. E., Pergl J., Hejda M., Schaffner U., et al. (2012). A global assessment of invasive plant impacts on resident species, communities and ecosystems: the interaction of impact measures, invading species’ traits and environment. Global Change Biol. 18, 1725–1737. doi: 10.1111/j.1365-2486.2011.02636.x
Raman T. R. S. (2006). Effects of habitat structure and adjacent habitats on birds in tropical rainforest fragments and shaded plantations in the Western Ghats, India. In: Hawksworth D. L., Bull A. T. (eds) Forest Diversity and Management. Topics in Biodiversity and Conservation, vol 2. Springer, Dordrecht. doi: 10.1007/978-1-4020-5208-8_28
Rambuda T. D. (2001). Pollination and breeding systems of alien invasive plants in KwaZulu-Natal in South Africa. (M.Sc. Thesis) (Pietermaritzburg: University of Natal).
Ramesh B. R., Ayappan N., Grard P., Prosperi J., Aravajy S., Pascal J.-P. (2008). BIOTIK: Western Ghats (Pondicherry: French Institute of Pondicherry).
Ramesh B. R., Pascal J. P. (1997). Atlas of endemics of the Western Ghats, India. French Institute of Pondicherry, Pondicherry, India.
R Core Team (2022). R: A language and environment for statistical computing (Vienna, Austria: R Foundation for Statistical Computing). Available at: https://www.R-project.org/.
Ricciardi A., Hoopes M. F., Marchetti M. P., Lockwood J. L. (2013). Progress toward understanding the ecological impacts of nonnative species. Ecol. Monogr. 83, 263–282. doi: 10.1890/13-0183.1
Ricciardi A., Atkinson S. K. (2004). Distinctiveness magnifies the impact of biological invaders in aquatic ecosystems. Ecology letters 7, 781–784.
Sajeev T., Sankaran K., Suresh T. (2012). Are alien invasive plants a threat to forests of Kerala. KFRI occasional papers, Forest Health Programme Division Kerala Forest Research Institute, Peechi.
Salinas N., Cosio E. G., Silman M., Meir P., Nottingham A. T., Roman-Cuesta R. M., et al. (2021). Tropical montane forests in a changing environment. Front. Plant Sci. 12, 712748. doi: 10.3389/fpls.2021.712748
Saravanan V., Santhi R., Kumar P., Balasubramanian A., Damodaran A. (2014). Influence of forest fire on floral diversity of the degraded shola forest ecosystem. Int. Res. J. Biol. Sci. 3 (1), 49–56.
Shiferaw H., Schaffner U., Bewket W., Alamirew T., Zeleke G., Teketay D., et al. (2019). Modelling the current fractional cover of an invasive alien plant and drivers of its invasion in a dryland ecosystem. Sci. Rep. 9, 1576. doi: 10.1038/s41598-018-36587-7
Sofaer H. R., Jarnevich C. S., Pearse I. S. (2018). The relationship between invader abundance and impact. Ecosphere 9, e02415. doi: 10.1002/ecs2.2415
Sriramamurthy R. T., Bhalla R. S., Sankaran M. (2020). Fire differentially affects mortality and seedling regeneration of three woody invaders in forest–grassland mosaics of the southern Western Ghats, India. Biol. Invasions 22, 1623–1634. doi: 10.1007/s10530-020-02207-7
Sriramamurthy R. T., Sankaran M., Bhalla R. S. (2022). Wildfires and aliens: differenced Normalized Burn Ratios (dNBR) indicate that woody invasive plants increase fire intensities in montane forest-grassland mosaics of the Western Ghats, India, 23 March 2022, PREPRINT (Version 1) Available at Research Square doi: 10.21203/rs.3.rs-1382178/v1
Stockard J. D. (1996). Restoration of Wingham brush 1980-1996. In Eleventh Australian Weeds Conference Proceedings (ed. Shepherd R. C. H.). Weed Science Society of Victoria Inc., Melbourne, pp. 432–436.
Strayer D. L. (2020). Non-native species have multiple abundance–impact curves. Ecol. Evol. 10, 6833–6843. doi: 10.1002/ece3.6364
Sukumar R., Suresh H. S., Ramesh R. (1995). Climate change and its impact on tropical montane ecosystems in southern India. Journal of Biogeography 22:533–536.
Symon D. (1981). The solanaceous genera, Browalia, Capsicum, Cestrum, Cyphomandra, Hyoscyamus, Lycopersicon, Nierembergia, Physalis, Petunia, Salpichroa and Withania, naturalised in Australia. J. Adelaide Bot. Garden 3, 133–166.
Thomas S. M., Palmer M. W. (2007). The montane grasslands of the Western Ghats, India: Community ecology and conservation. Community Ecol. 8, 67–73. doi: 10.1556/ComEc.8.2007.1.9
USDA (2013). Weed Risk Assessment for Cestrum laevigatum Schltdl. (Solanaceae) – Inkberry (NC, USA: Raleigh).
Vilà M., Ibáñez I. (2011). Plant invasions in the landscape. Landscape Ecol. 26, 461–472. doi: 10.1007/s10980-011-9585-3
Von Lengerke H. J. (1977). The Nilgiris: weather and climate of a mountain area in south India (Wiesbaden, Germany: Steiner).
Wijesundara S. (2012). Present Status of Montane Forests in Sri Lanka. The National Red List 2012 of Sri Lanka; Conservation Status of the Fauna and Flora (Colombo, Sri Lanka: Ministry of Environment).
Witt A., Beale T., Van Wilgen B. W. (2018). An assessment of the distribution and potential ecological impacts of invasive alien plant species in eastern Africa. Trans. R. Soc. South Afr. 73, 217–236. doi: 10.1080/0035919X.2018.1529003
Keywords: tropical montane forest, Western Ghats, shola, land cover change, invasion, Cestrum aurantiacum Lindl., multitype point pattern analysis
Citation: Das AA, Ratnam J and Jathanna D (2023) Patterns and consequences of invasion of tropical montane forests by Cestrum aurantiacum Lindl. in the Western Ghats. Front. Ecol. Evol. 11:1198085. doi: 10.3389/fevo.2023.1198085
Received: 31 March 2023; Accepted: 01 August 2023;
Published: 18 August 2023.
Edited by:
Xiang Liu, Lanzhou University, ChinaReviewed by:
Yunquan Wang, Zhejiang Normal University, ChinaCopyright © 2023 Das, Ratnam and Jathanna. This is an open-access article distributed under the terms of the Creative Commons Attribution License (CC BY). The use, distribution or reproduction in other forums is permitted, provided the original author(s) and the copyright owner(s) are credited and that the original publication in this journal is cited, in accordance with accepted academic practice. No use, distribution or reproduction is permitted which does not comply with these terms.
*Correspondence: Arundhati A. Das, YXJ1bmRoYXRpZDc0QGdtYWlsLmNvbQ==
‡ORCID: Jayashree Ratnam, orcid.org/0000-0002-6568-8374
Disclaimer: All claims expressed in this article are solely those of the authors and do not necessarily represent those of their affiliated organizations, or those of the publisher, the editors and the reviewers. Any product that may be evaluated in this article or claim that may be made by its manufacturer is not guaranteed or endorsed by the publisher.
Research integrity at Frontiers
Learn more about the work of our research integrity team to safeguard the quality of each article we publish.