- 1Engineering Research Center of Agricultural Microbiology Technology, Ministry of Education & Heilongjiang Provincial Key Laboratory of Ecological Restoration and Resource Utilization for Cold Region & Key Laboratory of Microbiology, College of Heilongjiang Province & School of Life Sciences, Heilongjiang University, Harbin, China
- 2Center for Ecological Research, Northeast Forestry University, Harbin, China
With the development of agriculture and industry, the increase in nitrogen (N) deposition has caused widespread concern among scientists. Although emission reduction policies have slowed N releases in Europe and North America, the threat to biodiversity cannot be ignored. Arbuscular mycorrhizal (AM) fungi play an important role in the establishment and maintenance of plant communities in forest ecosystems, and both their distribution and diversity have vital ecological functions. Therefore, we analyzed the effects of long-term N addition on AM fungi and understory herbaceous plants in a Korean pine plantation in northern China. The soil properties, community structure, and diversity of AM fungi and understory herbaceous plants were detected at different concentrations of NH4NO3 (0, 20, 40, 80 kg N ha−1 year−1) after 7 years. The results showed that long-term N deposition decreased soil pH, increased soil ammonium content, and caused significant fluctuations in P elements. N deposition improved the stability of soil aggregates by increasing the content of glomalin-related soil protein (GRSP) and changed the AM fungal community composition. The Glomus genus was more adaptable to the acidic soil treated with the highest N concentration. The species of AM fungi, understory herbaceous plants, and the biomass of fine roots were decreased under long-term N deposition. The fine root biomass was reduced by 78.6% in the highest N concentration treatment. In summary, we concluded that long-term N deposition could alter soil pH, the distribution of N, P elements, and the soil aggregate fractions, and reduce AM fungal and understory herb diversity. The importance of AM fungi in maintaining forest ecosystem diversity was verified under long-term N deposition.
1 Introduction
The dramatic increase in atmospheric emissions of reactive nitrogen (N) from human activities since the Industrial Revolution (industrial and agricultural activities and fossil fuel combustion) has led to a rapid increase in N deposition to terrestrial and aquatic ecosystems (Ackerman et al., 2019). N deposition provides a new source of fertilizer for plants, but excessive N input can also affect biogeochemical cycles and alter ecosystem structure and function (Yu et al., 2019). Forest ecosystem species diversity is an important indicator reflecting the relationship between plants and the environment, determining the structure and ecological functions of forest communities, which are mutually constrained and synergistic with environmental factors. Moderate N input will alleviate forest N limitation to a certain extent and promote vegetation growth. On the other hand, excessive N deposition induces ecological problems such as soil acidification, reduction of biodiversity, and degradation of forest functions (Vuorenmaa et al., 2018; Gao Y. et al., 2019; Hong et al., 2019). The results of a worldwide network of long-term monitoring of N deposition showed that China has faced more severe N deposition than the United States, European countries, and other countries in East Asia since the twentieth century (Zhang et al., 2021). N deposition remains a threat to the biodiversity and stability of forest ecosystems (Weldon et al., 2022).
Herbaceous plants have the highest species diversity in forest ecosystems and contribute significantly to forest ecosystem structure and function, but are more sensitive to atmospheric changes and N deposition (Gilliam, 2007; Thrippleton et al., 2016; McDonnell et al., 2022). It has been demonstrated that excessive N input can have complex effects on herbaceous community structure and plant biological characteristics, such as reducing herbaceous diversity and altering herbaceous root chemical components and biomass (Li et al., 2015). However, most studies have focused on tropical forests and temperate grasslands, and not enough studies have been conducted on herbaceous plants in temperate forests.
The functions of soil microorganisms in forest ecosystems should not be underestimated. Among these, arbuscular mycorrhizal (AM) fungi can establish symbiotic relationships with most herbaceous plants and play an important role in the establishment and maintenance of plant communities (Smith and Read, 2008; Mariotte et al., 2013; Wang et al., 2019). AM fungi can promote plant growth (Hoeksema et al., 2010; Xie et al., 2022) and improve plant resilience (Ruiz-Lozano et al., 2016; Chen et al., 2018). Glomalin-related soil protein (GRSP) in mycelium secretions can also promote soil aggregation (Gao W. et al., 2019) and affect host plants in a range of direct and indirect ways. Most AM fungi depend on the host plant for existence and reproduction, and AM fungi are thought to be susceptible to environmental conditions, such as climate (Compant et al., 2010), plant species (Kivlin et al., 2011), and soil properties. Excessive N input indirectly affects the structure and diversity of AM fungi through changes in soil factors (Ma et al., 2021; Boeraeve et al., 2022) and thus affects the ecological function of AM fungi. The results of the studies are not the same around the world, which is due to the influence of experimental conditions. A global meta-analysis showed that the negative effect of N addition on AM fungi was mainly a reduction in total AM fungal abundance as well as the diversity and structure of AM fungi (Han et al., 2020). However, in a tropical simulated N deposition experiment, it was found that N addition mainly reduced the diversity and abundance of AM fungi (Camenzind et al., 2014). AM fungi have been shown to contribute to the maintenance of forest herbaceous diversity under N application conditions (Smith and Stephan, 2021).
In this experiment, AM fungi, herbaceous plants, and soil properties were investigated in a temperate Korean pine plantation ecosystem in northeastern China that was subjected to a 7-year N addition experiment. The AM fungi and the understory herbaceous plants that coexisted with them were investigated by morphological identification and high-throughput sequencing, respectively. We hypothesized that: (1) long-term N fertilization would change the soil pH value, increase soil GRSP content, and thus improve the stability of soil aggregates; (2) long-term N addition would change the structure and diversity of AM fungal communities, thus affecting the ecological functions of AM fungi; and (3) long-term N addition would change AMF diversity directly by reducing pH and indirectly by changing herbaceous plant composition.
2 Materials and methods
2.1 Study site
The experiment sample site was located in a Korean pine plantation in Liangshui National Nature Reserve, Heilongjiang Province, China (128°53′20″E, 47°10′50″N); natural N deposition is 12.93 kg N ha−1 year−1. The area has a distinct temperate continental monsoon climate with an average annual temperature of –0.3°C, of which the average annual precipitation of 676 mm, a frost-free period of 100–120 d, and an annual snowpack period of 130–150 d. The Korean pine plantation in this test site was established in 1954 after a clear-cutting of the mixed broadleaved-Korean pine forest. The dominant plant species are Korean pine and undergrowth-associated herbs (Oxalis corniculate and Mitella nuda).
2.2 Experimental design
The simulated N deposition experiment commenced in 2014, and the sample plots were treated with N application by understory manual spraying from June to September (four times per year). Each sample plot was 5 m × 30 m, bounded by a 10 m wide buffer strip. The required NH4NO3 was dissolved in 20 L water when it was needed. There were four treatments as follows: CK (0 kg N ha−1 year−1), LN (20 kg N ha−1 year−1), MN (40 kg N ha−1 year−1), and HN (80 kg N ha−1 year−1), which were approximately twice, four times, and eight times the level of bulk N deposition in the region, respectively (Song et al., 2019). There were three replicates in each treatment with a completely randomized design.
2.3 Soil sample collection and pre-treatment
Soil samples were collected in July 2020. After further removal of the top layer of plastic material, three small random sample squares (1 m×1 m) were set in each sample plot, and five random samples were collected from each plot and mixed into a composite sample (15 cm3, a total of 12 composite samples). Soil samples were sieved and divided into three parts: one part was stored at –80°C for soil microbial DNA extraction, another part was air-dried and used for soil chemical property determination, and the remaining part was used to determine the composition of soil aggregates and measures the content of GRSP.
2.4 Soil physicochemical properties
The air-dried soil samples were sieved through soil sieves with apertures of 2 mm, 1 mm, and 0.25 mm to obtain four groups of soil samples (>2 mm, 1–2 mm, 0.25–1 mm, <0.25 mm) (Muruganandam et al., 2009). Each group of soil samples was weighed. Soil structural stability was evaluated by the mean weight diameter (MWD) of water-stable aggregates (Zhao et al., 2017). The contents of easily extractable glomalin-related soil protein (EE-GRSP) and total glomalin-related soil protein (T-GRSP) were determined (Janos et al., 2008). EE-GRSP was extracted from 0.5 g of soil with 4 ml of 20 mmol L−1 sodium citrate (pH=7.0) and autoclaved at 121°C for 30 min. T-GRSP was extracted from 0.5 g of soil with 4 ml of 50 mmol L−1 sodium citrate (pH=8.0) and autoclaved at 121°C for 60 min. The supernatant was collected at 4°C, 10,000 rpm for 10 min. T-GRSP extraction was performed six times until the solution turned straw-colored (Gao et al., 2017). The GRSP content was determined using a spectrophotometer (595 nm) with bovine serum albumin as the standard.
The soil pH of the air-dried soil samples was determined using a pH meter (FiveEasy FE20) on a mixture with a water-to-soil ratio of 2.5:1. TC (total carbon), TOC (total organic carbon), and total N (TN) were determined by an analyzer (multi N/C 2100 TOC/TN Analyze, Analytik Jena, Germany). Soil ammonium N (NH4+-N) and nitrate N (NO3–N) were measured using a continuous flow analyzer (BRAN+LUEBBEAA3, Germany). Soil total phosphorus (TP) and available phosphorus (AP) were determined using the molybdenum antimony colorimetric method determined by the molybdenum antimony anti-colorimetric method.
2.5 Herbaceous plant diversity, root biomass, and AM fungal colonization rate
We explored herbaceous plant diversity in July 2020. Three small random sample squares (1 m×1 m) were set in each sample plot, and the species of herbaceous plants, the number of individuals of the species (counted as a single plant in the above-ground part), height, and cover were investigated. The fine roots (<2 mm in diameter) of herbaceous plants were selected, and the root biomass was measured (Li et al., 2021). We determined the AM fungal colonization rate of roots from herbs by a modified aniline blue staining method (Zubek et al., 2011). In addition, we randomly selected 50 mixed herbaceous roots from each sample site for the infestation rate experiment.
2.6 AM fungal community structure and diversity
2.6.1 Isolation and identification of AM fungal spores
Spores were obtained by wet sieving (three-layer soil sifters are 100, 200, and 400 mesh) method from the soil (10 g) around the roots of herbaceous plants. The spores were placed under a stereomicroscope for count, observation, and preliminary classification. The spores were counted and transferred to polyvinyl alcohol-lactic acid-glycerol (PVLG) to observe the morphological characteristics, including spore color, shape, diameter size, sporiferous saccule, spore wall thickness, spore wall type, and subtending hyphae width and shape. The spores were identified according to the photos and feature descriptions from the VA mycorrhizal fungi identification manual (Schenck and Perez, 1990), INVAM (https://invam.ku.edu/), and international AM fungal classification system (http://www.amf-phylogeny.com/).
AM fungal spore density, species richness, relative abundance (RA), frequency, importance value (I), and species alpha diversity index were calculated per 10 g of air-dried soil sample. The degree of AM fungal dominance was assigned into four categories according to their important values (I): I > 50% means dominant species, 30% < I ≤ 50% means subdominant species, 10% < I ≤ 30% means accompanying species, and I ≤ 10% means rare species.
2.6.2 High throughput analysis of AM fungi
Total DNA was extracted from mixed soil (0.5 g) using Fast DNA® Spin Kit for Soil. Then AM fungus-specific primers AML1 and AML2 were selected as the first round of primers (Lee et al., 2008), and AMV4.5NF (AAGCTCGTAGTTGAATTTCG) and AMDGR (CCCAACTATCCCTAT-TAATCAT) were selected as the second round of primers for amplification with the nested PCR amplification method (Sato et al., 2005). PCR was performed using TransGen AP221-02: TransStart Fastpfu DNA Polymerase, 20 μl reaction system. The PCR amplification products were detected and recovered by 2% agarose gel electrophoresis, and Illumina’s MiseqPE300 platform was used for sequencing (commissioned by Shanghai Meiji Bio-Pharmaceutical Technology Co). Raw sequences were uploaded to the NCBI Sequence Read Archive (SRP426700).
2.7 Statistical analysis
One-way ANOVA and Duncan’s multiple tests (P < 0.05) were performed using IBM SPSS Statistics 26 (IBM Corporation, New York) software to compare the means of plant, AM fungi, and soil physicochemical property data. The correlation between soil physicochemical properties and herbaceous plant diversity was calculated by Pearson’s test (two-tailed) at two significant levels, P < 0.05 and P < 0.01. RDA analysis was used to reveal the effect of soil properties on the community composition of AM fungi. Structural equation modeling (SEM) was used to predict causal pathways of N addition, key soil physicochemical indicators, and AM fungi on herbaceous plant diversity. The fit of models that had been previously built was evaluated using chi-squared test, root mean square error of approximation (RMSEA), and goodness-of-fit index (GFI).
3 Results
3.1 Soil physical and chemical properties
3.1.1 Soil chemical properties
The soil pH gradually decreased with the increase of N addition concentration, and the pH value in HN treatment significantly decreased to 5.24 (P < 0.05) (Table 1). TP content was significantly increased by 16.7% in the MN treatment. The AP content was significantly reduced after N addition. There were significant changes in NH4+-N and NO3–N content. The NH4+-N content increased with the concentration of N addition, and there was a significant increase in MN and HN treatments. The NO3–N content was significantly increased in the LN treatment and significantly decreased in the HN treatment.
3.1.2 Soil aggregate and glomalin-related soil protein
The weight percentage of soil aggregate varied with its size fractions (Figure 1). In all N addition treatments, MWD values ranged from 1.48–1.74 mm. The content of large aggregates (2–5 mm) and MWD value were significantly higher in the HN treatment than in the CK treatment.
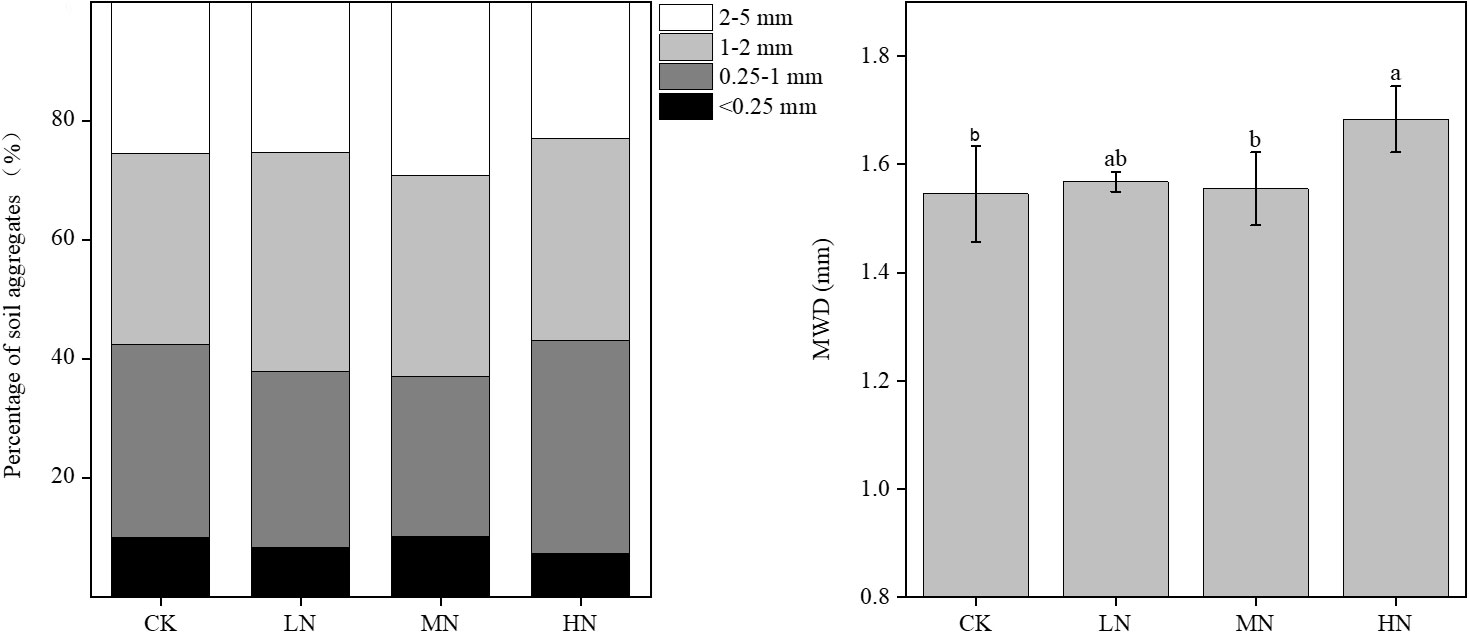
Figure 1 Soil aggregate size fractions and MWD value under different N addition treatments. Percentage of soil aggregate size fractions and MWD (mean weight diameter) value. Mean (n=3) of each parameter followed by a different letter is significantly different between four treatments (a, b) at P < 0.05.
N addition exerted a significant effect on the EE-GRSP and T-GRSP content of soil aggregate. The contents of EE-GRSP ranged from 1.36 to 4.29, and T-GRSP ranged from 3.73 to 13.50 mg·g−1 (Figure 2). GRSP content varied according to the amount of N addition. N addition increased the content of EE-GRSP and T-GRSP in soil aggregates and reached the maximum in the MN treatment. N addition increased the EE-GRSP content of soil aggregates by 60.6% to 118.7%, 12.0% to 77.2%, 5.0% to 60.5%, and 16.3% to 56.2% for each particle size (<0.25 mm, 0.25–1 mm, 1–2 mm, and 2–5 mm), respectively. N addition increased the T-GRSP content of soil aggregates by 45.5% to 87.6%, 25.5% to 88.4%, 44.5% to 105.2%, and 51.1% to 86.4% for each particle size, respectively.
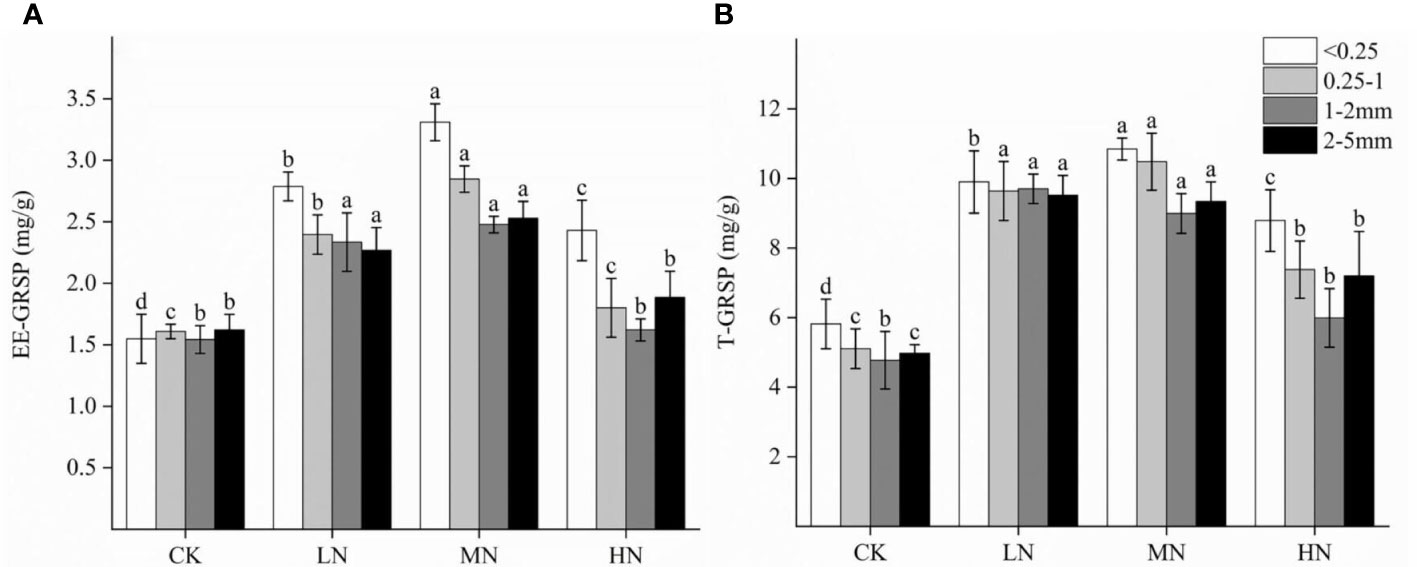
Figure 2 Effects of different N application rates on EE-GRSP (A) and T-GRSP (B) content in soil aggregates of each particle size. EE-GRSP represents easily extractable glomalin-related soil protein, and T-GRSP represents total glomalin-related soil protein. Mean (n=3) of each parameter followed by a different letter is significantly different between four treatments (a, b, c, d) at P < 0.05.
3.1.3 Relationship between the stability of soil aggregates and the content of GRSP content under N addition conditions
In the present study, Korean pine plantation soils were dominated by medium aggregates (1–2 mm), with MWD values ranging from 1.48 to 1.74 mm, which has been identified as an indicator of soil stability, and long-term (7 years) HN treatment (80 kg N ha−1 year−1) increased the soil macro-aggregates content and thus improved soil stability. Pearson correlation analysis of undisturbed soil GRSP content and MWD showed that both EE-GRSP and T-GRSP were significantly positively correlated with MWD values (P < 0.01) (Figure 3), where R2 is the coefficient of determination.
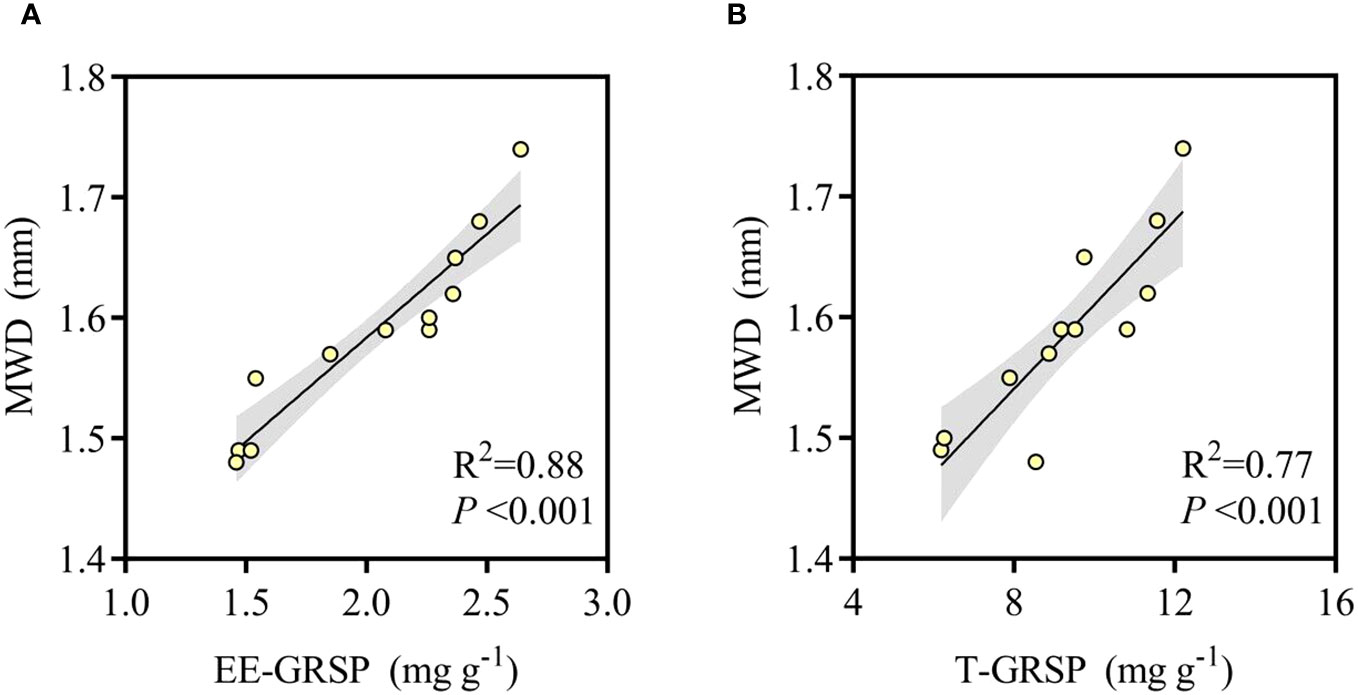
Figure 3 Relationships between the MWD and GRSP under different treatments. MWD represents mean weight diameter, EE-GRSP represents easily extractable glomalin-related soil protein (A). T-GRSP represents total glomalin-related soil protein (B).
3.2 Understory herbs
3.2.1 Herb plants communities
There were 33 species of herb plants were investigated in this study (Table S1). N addition significantly altered the dominance of five plants in Table S1 (P < 0.05): Chrysosplenium sinicum, Aegopodium alpestre, Dryopteris crassirhizoma, Eranthis stellate, and Phryma leptostachya. The species of herb plants were not detected in the LN treatment (9.1%), MN treatment (27.3%), and HN treatment (33.3%) compared with the CK treatment.
3.2.2 Herb diversity, fine root biomass, and AM fungal colonization rate
The Shannon index and Simpson index of herb plants decreased gradually with increasing N addition concentration, and the MN and HN treatments were significantly lower than CK (Table 2). The root biomass and AM fungal colonization rate decreased significantly with the increase of N addition concentration. The biomass decreased by 34.43 g/m2 in the HN treatment. The AM fungal colonization rate decreased significantly by 21.95% in HN treatment. This indicated that herb diversity and AM fungal colonization rate were significantly reduced when the applied N concentration exceeded 40 kg N ha−1 year−1 in this experiment. The fine root biomass of herb plants was also significantly reduced when the applied N concentration exceeded 20 kg N ha−1 year−1.
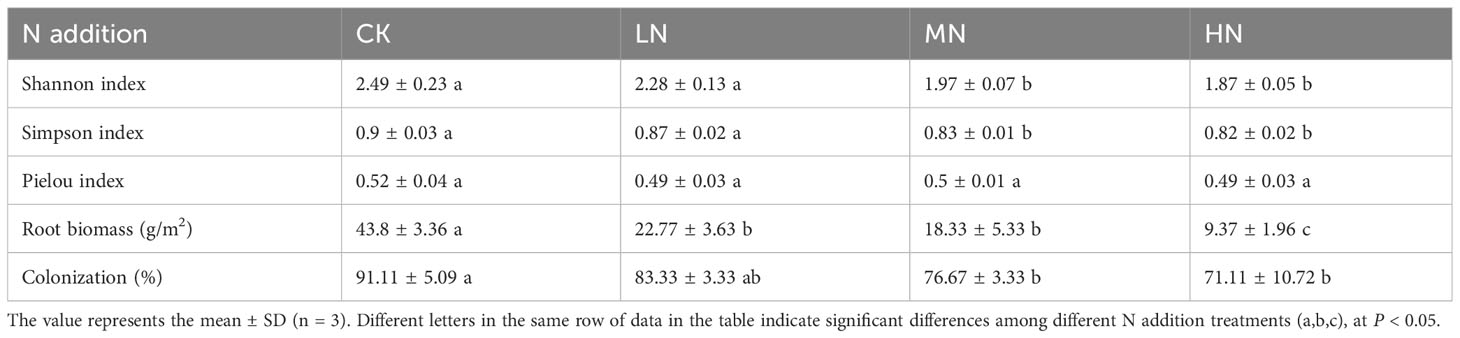
Table 2 Effects of N addition on the diversity of undergrowth herb plants, root biomass, and infection rate of AM fungi.
3.3 AM fungal community structure and diversity
3.3.1 Morphological identification of AM fungi
There were 4,461 AM fungal spores isolated from all soil samples, and nine genera and 20 species were identified morphologically. There were seven species belonging to Acaulospora, accounting for 35% of the total number of species, followed by four species of Glomus genus, accounting for 20% of the total number of species.
The relative abundance (RA) and importance values (I) of AM fungal spores were analyzed under the N application treatment (Table 3). The soil samples had the most abundant spore species in the CK treatment, with 17 AM fungal species. Moreover, Acaulospora spinosa and Claroideoglomus lamellosum species were only found in the CK treatment. The spore species in soil samples were gradually reduced with increasing N addition concentration. There were 14 AM fungal species in the LN treatment and MN treatment. Sclerocystis sinuosa was only found in the MN treatment. However, there were only 10 AM fungal species in the HN treatment.
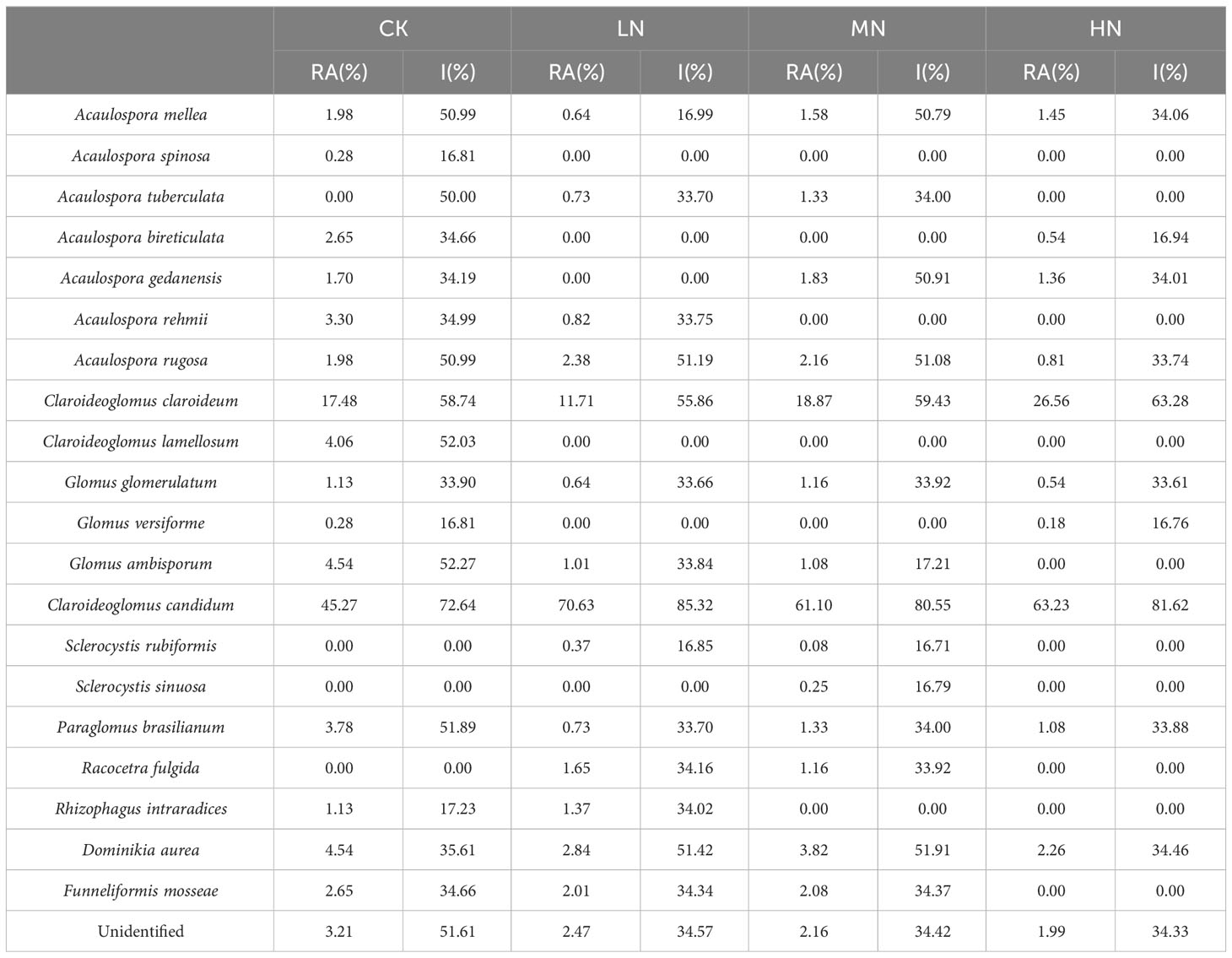
Table 3 Relative abundance (RA) and importance value (I) of Mycorrhizal fungal spores under four N addition treatments.
3.3.2 Spore density, species abundance, and diversity index of AM fungi
The highest AM fungal spore density was found in the MN treatment, with 40.13 spores g−1 (Table 4), followed by the HN treatment, LN treatment, and CK treatment with 36.9, 36.43, and 35.27 spores g−1, respectively. With increasing N addition concentration, the species abundance of AM fungi decreased gradually. There were only 8 species g−1 in the HN treatment. The diversity of AM fungal species was significantly different between the N addition treatments and the CK treatment, which had the highest Simpson’s index of 0.76. The LN treatment had a significantly lower diversity index than the CK and MN treatments, while the HN treatment had the lowest Simpson’s index of 0.49. Similarly, the CK treatment had the highest Shannon index of 1.85, whereas the HN treatment had the lowest Shannon index of 1.02.

Table 4 Spore densities, species richness, Shannon index, and Simpson index of AM fungi in four N addition treatments.
3.3.3 Bioinformatical analyses
In the present study, we identified 398,299 sequences from the total dataset, which optimized to 391,960 sequences. The coverage of operational taxonomic units (OTUs) in all soil types was more than 99%. The largest OTU richness of AM fungi was in the CK treatment (Table 5). The highest diversity of AM fungi was found in the CK treatment indicated by Shannon and Simpson’s indices, which were 2.27 and 0.59, respectively. The lowest diversity of AM fungi was found in the HN treatments, which were 0.84 and 0.16, respectively.
The results of the community structural composition showed that the majority of OTU sequences belonged to Glomus (Figure 4). The relative abundance of Glomus increased with N addition and was significantly higher in the HN treatment than in the CK treatment. In contrast, the relative abundance of Paraglomus was reduced by 96.71% in the HN treatment compared to the CK treatment.
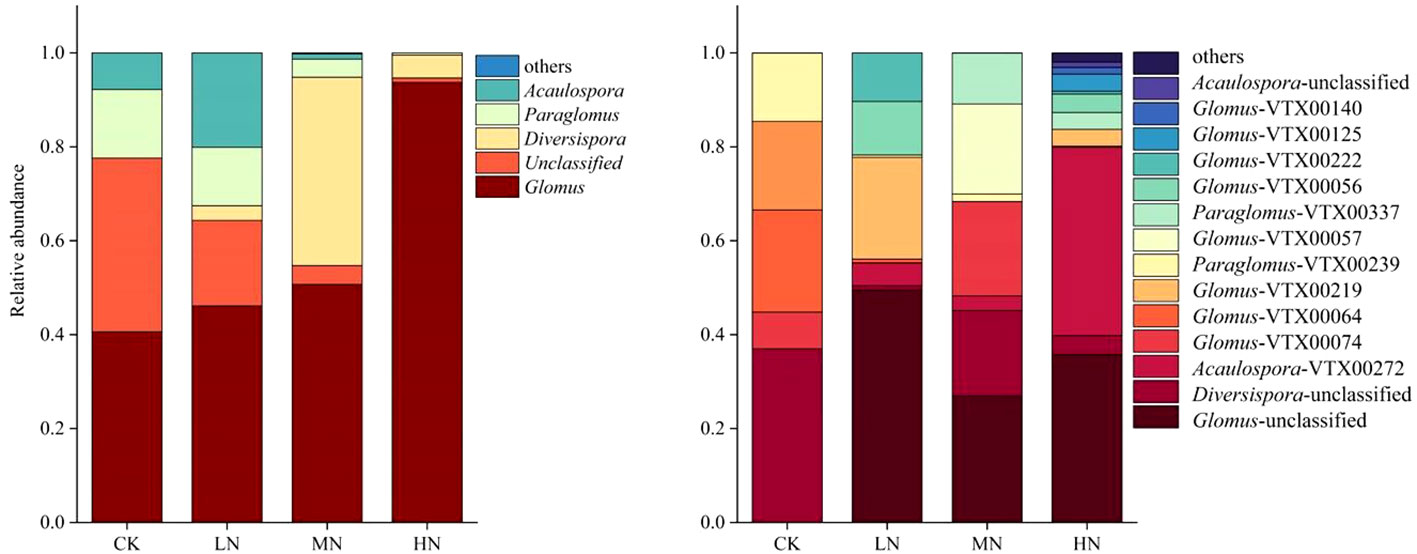
Figure 4 Relative abundances of AM fungal communities in Korean pine plantations with different N addition at genus and species level.
The AM fungal community composition and relative abundance at the species level are shown in Figure 4. The dominant species was Glomus-Glo3-VTX00074 in the CK treatment, Acaulospora-VTX00272 in the LN treatment, Diversispora-unclassified in the MN treatment, and Glomus-MO-G5-VTX00219 in the HN treatment. N addition had a significant effect on AM fungal community composition in the Korean pine plantation. Glomus-MO-G18-VTX00064 was only found in the CK treatment. Glomus-group-B-Glomus-acnaGlo7-VTX00057 was only found in the LN treatment. Glomus-MO-G22-VTX00125 and Glomus-Wirsel-OTU13-VTX00140 were unique species in the MN treatment.
3.3.4 The morphological and molecular identification of AM fungi
The result of morphological identification showed that there were nine genera and 20 species of AM fungi after wet sieving, while high-throughput sequencing amplified and sequenced the DNA extracted from the mixed soil from each small sample, and four genera and 11 virtual species were identified. The genus Diversispora was detected only by high-throughput sequencing in contrast to the morphological identification, while morphological identification revealed six genera that were not identified by high-throughput sequencing. However, the AM fungal diversity index had a similar change trend by high-throughput sequencing and morphological identification under different treatments.
3.4 Relationship between soil physicochemical properties and AM fungal community composition under N addition conditions
RDA analysis showed that six soil physicochemical factors explained 91.12% of the variation in the AM fungal community, as shown in Figure 5. Soil pH and NO3–N, AP, NH4+-N, and TN content significantly influenced the distribution of AM fungal species. In addition, Glomus was negatively correlated with pH and positively correlated with NH4+-N and NO3–N content. Paraglomus was positively correlated with AP content and pH value.
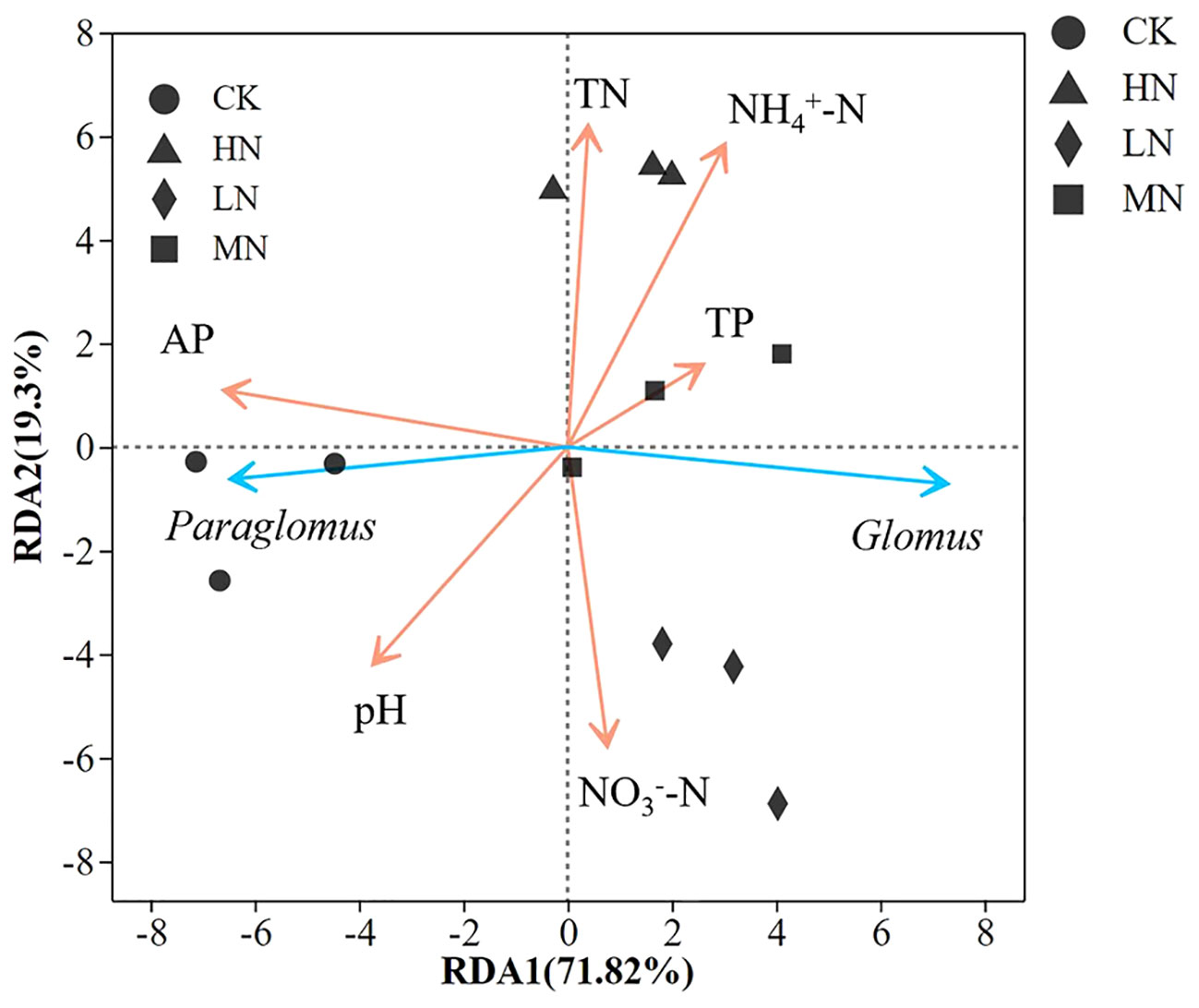
Figure 5 Redundancy analysis (RDA) of AM fungi species, genus distribution, and environmental characteristics. TP represents soil total phosphorus, AP represents soil effective phosphorus, TN represents soil total nitrogen.
3.5 Relationship between environmental factors and herb diversity under N addition conditions
Long-term N addition reduced the diversity of understory herbaceous plants. The correlation was analyzed between α diversity index of herbs and biochemical factors by Pearson correlation. The diversity of understory herbaceous plants was significantly and positively correlated with the diversity of AM fungi (P < 0.05, Figure 6) and the colonization rate of AM fungi (R2 = 0.66). The diversity of herbs was significantly and positively correlated with total N content (P < 0.05), significantly and negatively correlated with NH4+-N content (P < 0.01), and significantly and positively correlated with pH (R2 = 0.67). Additionally, herbaceous plant fine root biomass was significantly and positively correlated with their diversity (P < 0.01).
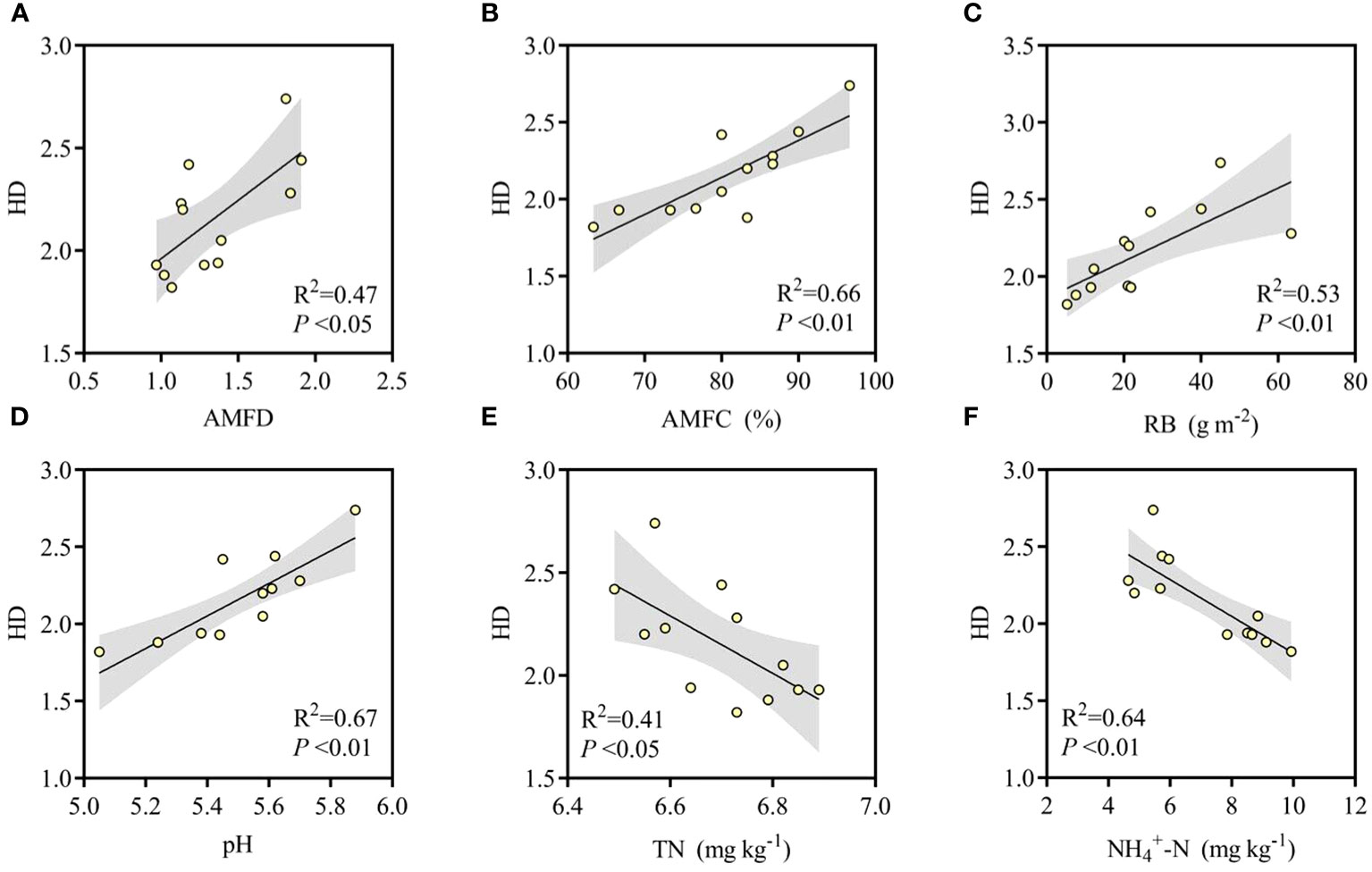
Figure 6 Linear correlation between herb Shannon diversity (HD) and (A) AM fungi Shannon diversity (AMFD), (B) AM fungi colonization (AMFC), (C) root biomass (RB), (D) soil pH, (E) soil total N, (F) soil NH4+-N.
After correlation analysis of soil physical and chemical indicators, herbaceous plants, and AM fungi, we built SEM models for important indicators and obtained the following models after multiple fitting (Figure 7). The P-value of χ2 was higher than 0.05, indicating that the model structure was reasonable. The SEM throughput analysis largely explained the effects of biotic as well as abiotic factors on herb diversity (R2 > 90%). N addition indirectly affected AM fungal diversity and plant diversity through soil pH value, ammonium N, and AP content. Among these, AM fungal diversity was the strongest pathway affecting plant diversity.
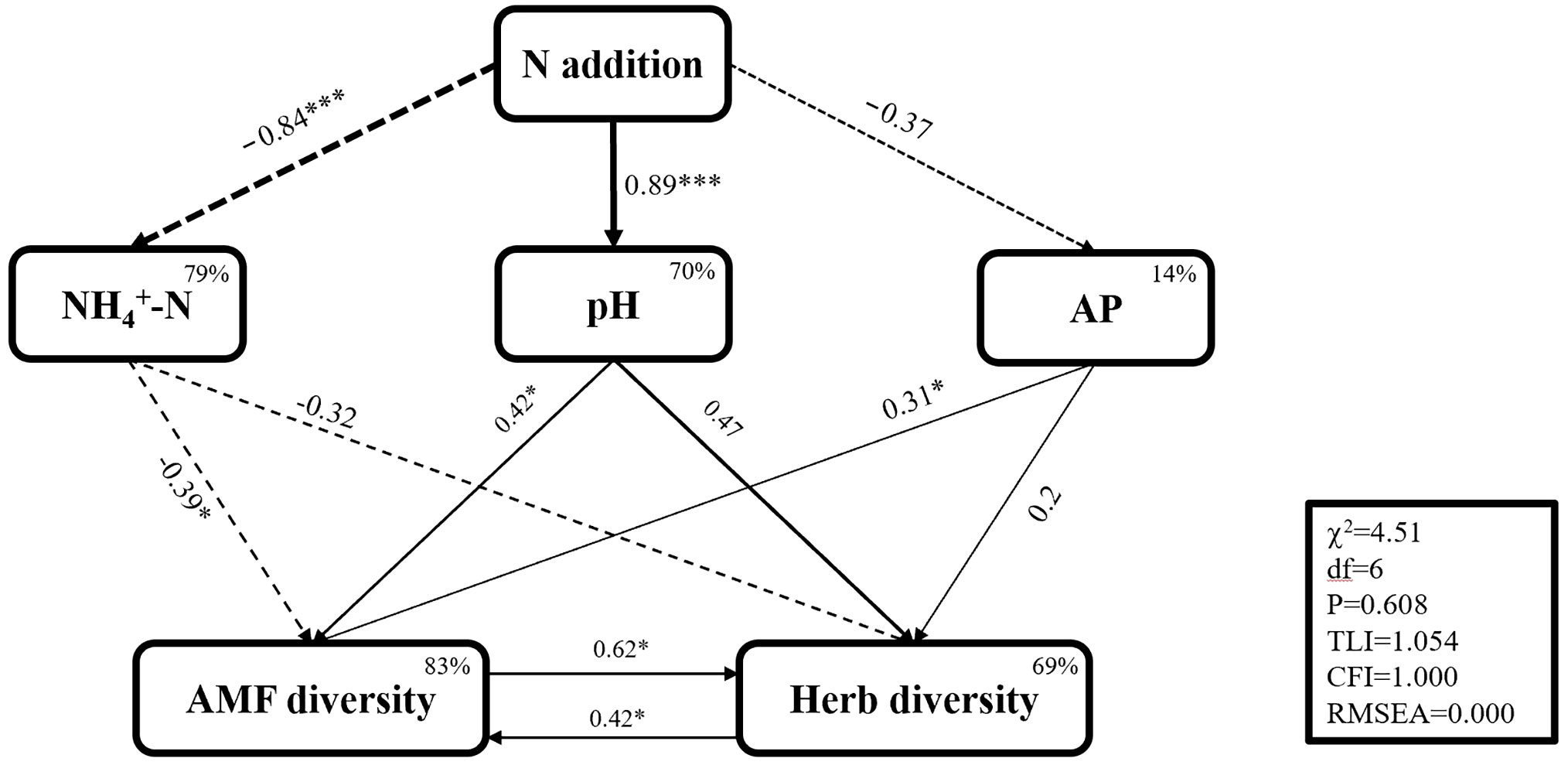
Figure 7 Structural equation model simulates the effects of various response variables on plant diversity of Korean pine plantation. Valid paths are indicated by arrows, and continuous and dashed arrows indicate positive and negative relationships, respectively. The numbers next to the paths indicate the normalized path coefficients, positive path coefficients indicate positive relationships, and negative path coefficients indicate negative relationships. The higher the absolute value of the path coefficient, the more important the path is and the thicker the line. * indicates the significance level of each fitting factor: *P < 0.05, **P < 0.01, ***P < 0.001. The number in the upper-right corner of the box is R2 and shows the percentage of explained variance for each endogenous variable. The fit of the model was determined using chi-squared test (c2), degrees of freedom (df), probability level (P), comparative fit index (CFI), Ticker-Lewis value (TLI), and root mean square error of approximation (RMSEA) expressed. Among them, high P values for c2 (P > 0.05); CFI, TLI > 0.90; RMSEA < 0.05 indicate a well-fitting model.
4 Discussion
4.1 Effect of N addition on soil properties
Soil acidification is caused by N deposition, and soil pH decreases linearly with increasing N application (Tian and Niu, 2015). In this study, long-term HN treatment (80 kg N ha−1 year−1) reduced soil pH, while LN and MN treatments had no significant effect on soil pH. The reason may be that soils in terrestrial ecosystems are more sensitive to N inputs, while forest ecosystems are more stable than other types of ecosystems (Roth et al., 2021).
MN treatment had a significant effect on soil TP content, while AP content decreased to varying degrees with N addition. This may be because soil acidification activates aluminum ions and iron ions in the soil, causing more phosphorus to be absorbed by the soil and resulting in a decrease in AP content. When plants and microorganisms absorb and utilize AP, they get a corresponding supplement from the phosphorus element of other components, which leads to the reduction of AP content.
Soil TN content was stable, while ammonium content increased linearly with N application. The results of Qin et al. (2022) showed that long-term N application can change the soil ammonium and nitrate content. In addition, total N increased significantly with the increase of N addition. The stability of the whole N content in this experiment may be because the forest ecosystem is more stable and can resist a certain concentration of N addition.
There was an increased tendency for EE-GRSP content as the N addition concentration was increased, and the T-GRSP content was significantly higher in the HN treatment than in the CK treatment, which was probably due to the significant acidification of the soil (Agnihotri et al., 2022). On the contrary, short-term N addition reduced the GRSP content in the soil (Jia et al., 2021; Huang et al., 2022). The reason might be that short-term N additions could not significantly change soil acidity and alkalinity, but lead to a decrease in GRSP by changing soil dissolved organic carbon and N/P ratio. The proportion of large soil aggregates and GRSP content were significantly elevated in the HN treatment. Pearson correlation analysis of in-situ soil GRSP content and MWD showed that both EE-GRSP and T-GRSP were significantly positively correlated with MWD values (Figure 3).
4.2 Effect of N addition on the structure and diversity of AM fungi community
N addition and local soil conditions affect plants and soil microorganisms (Cao et al., 2020; Zeng et al., 2021). In this study, both high-throughput sequencing and traditional spore morphological identification were used to investigate the community structure and diversity of woodland AM fungi. Morphological identification is inconsistent, limited, and subjective, but can be improved by means such as increasing the sample size. High-throughput sequencing technology has been widely used by researchers but still has some limitations. Due to the variety of microorganisms, the current NCBI database cannot cover all the microorganisms, or lacks accurate species information, resulting in the omission of some low-abundance species. Thus, we combined the two methods to accurately reflect the diversity and structural characteristics of AM fungi (Chaudhary et al., 2020). Both high-throughput sequencing and morphological identification results showed that N addition altered AM fungi community composition and reduced AM fungal alpha diversity.
RDA analysis showed that the community composition of AM fungi could be significantly affected by pH in the Korean pine plantation (Figure 5). Studies have reported that soil pH is one of the most important abiotic factors affecting the ecological distribution of AM fungi (Davison et al., 2021). As the N concentration increased, the abundance of Glomus gradually increased and was significantly higher in the HN treatment (Figure 4). The main reason for this phenomenon might be that Glomus showed better ecological advantages in acidic soils. Studies have shown that AM fungi alleviate the negative effect of nitrogen deposition on ecosystem functions (Kang et al., 2020). In the future, attention should be paid to the ecological functions of Glomus under the background of nitrogen deposition. Short-term HN treatment (80 kg N ha−1 year−1) also reduced fungal diversity and changed community composition (Cao et al., 2020). This phenomenon is mainly due to the changes in phosphorus elements in the soil caused by N addition, causing plants to select AM fungi with high phosphorus uptake ability.
4.3 Effect of N addition on understory herb plants
Cases of reduced plant diversity caused by soil acidification induced by N deposition have been reported (Kimmel et al., 2020). In this study, we found that the diversity of understory herbaceous plants in forests also decreased with lower soil pH. Additionally, excess N can also create nutrient imbalances. Large inputs of exogenous N will break the original stable stoichiometry, causing nutrient imbalances in soil and plants, and leading to the loss of certain plant species. N deposition indirectly affects plant species diversity by changing the composition of soil microbial communities (Bobbink et al., 2010). This study also found that changes in understory plant diversity were linearly positively correlated with AM fungal diversity and AM fungal colonization rate (Figure 6), which also verified the role of AM fungi in maintaining the stability of understory plants. In addition, long-term N application will significantly reduce plant fine root biomass, while a small amount of N application will increase plant root biomass (Frew, 2022). SEM clearly showed that AM fungal diversity was the strongest influencing pathway affecting herb diversity under N addition conditions (Figure 7), which further proved the ability of AM fungi to maintain understory herb diversity.
5 Conclusion
N addition in temperate Korean pine plantation ecosystems altered the AM fungal community structure, reduced the diversity of AM fungi and herbaceous plants, decreased soil pH, increased inorganic N concentration, and reduced soil available phosphorus content. We showed that N addition could change soil properties, the composition and diversity of soil AM fungi, and the AM fungal communities, which ultimately affected the distribution of soil aggregates. The above biotic and abiotic factors in the underground part affected plant communities and diversity. This paper showed the correlation between the changes of AM fungi and herbaceous plants under the background of N deposition and used SEM to prove the correlation. Future research should address issues such as soil acidification, biodiversity loss, and changes in ecosystem stability due to increased nitrogen deposition. Given the importance of AM fungi in ecosystems, future research should explore the pathways by which AM fungi regulate ecological functions under N addition in order to improve our understanding of the ecological functions of AM communities under conditions of global environmental change.
Data availability statement
The datasets presented in this study can be found in online repositories. The names of the repository/repositories and accession number(s) can be found below: https://www.ncbi.nlm.nih.gov/, SRP426700.
Author contributions
All authors made significant contributions to this study. FS and GJ provided the conceptualization. FS and WW framed the methodology. WW and YF wrote and prepared the original draft. XW, RW, and XH conducted the review and editing. MZ and KL oversaw the project administration. FS was responsible for funding acquisition. All authors contributed to the article and approved the submitted version.
Funding
This work was financally supported by the National Natural Science Foundation of China (31971527).
Acknowledgments
We acknowledge the editor and reviewers for their comments and suggestions to improve our manuscript.
Conflict of interest
The authors declare that the research was conducted in the absence of any commercial or financial relationships that could be construed as a potential conflict of interest.
The reviewer FF declared a shared affiliation with the author GJ at the time of review.
Publisher’s note
All claims expressed in this article are solely those of the authors and do not necessarily represent those of their affiliated organizations, or those of the publisher, the editors and the reviewers. Any product that may be evaluated in this article, or claim that may be made by its manufacturer, is not guaranteed or endorsed by the publisher.
Supplementary material
The Supplementary Material for this article can be found online at: https://www.frontiersin.org/articles/10.3389/fevo.2023.1192267/full#supplementary-material
References
Ackerman D., Millet D. B., Chen X. (2019). Global estimates of inorganic nitrogen deposition across four decades. Global Biogeochem. Cycles. 33 (1), 100–107. doi: 10.1029/2018GB005990
Agnihotri R., Sharma M. P., Prakash A., Ramesh A., Bhattacharjya S., Patra A. K., et al. (2022). Glycoproteins of arbuscular mycorrhiza for soil carbon sequestration: review of mechanisms and controls. Sci. Total Environ. 806 (Pt 2), 150571. doi: 10.1016/j.scitotenv.2021.150571
Bobbink R., Hicks K., Galloway J., Spranger T., Alkemade R., Ashmore M., et al. (2010). Global assessment of nitrogen deposition effects on terrestrial plant diversity: a synthesis. Ecol. Appl. 20 (1), 30–59. doi: 10.1890/08-1140.1
Boeraeve M., Kohout P., Ceulemans T., Cajthaml T., Tedersoo L., Jacquemyn H. (2022). Changes in the root microbiome of four plant species with different mycorrhizal types across a nitrogen deposition gradient in ombrotrophic bogs. Soil Biol. Biochem. 169, 108673. doi: 10.1016/j.soilbio.2022.108673
Camenzind T., Hempel S., Homeier J., Horn S., Velescu A., Wilcke W., et al. (2014). Nitrogen and phosphorus additions impact arbuscular mycorrhizal abundance and molecular diversity in a tropical montane forest. Glob. Change Biol. 20 (12), 3646–3659. doi: 10.1111/gcb.12618
Cao J., Lin T., Yang Z., Zheng Y., Xie L., Xiong D., et al. (2020). Warming exerts a stronger effect than N addition on the soil arbuscular mycorrhizal fungal community in a young subtropical cunninghamia lanceolata plantation. Geoderma 367, 114273. doi: 10.1016/j.geoderma.2020.114273
Chaudhary V. B., Nolimal S., Sosa-Hernandez M. A., Egan C., Kastens J. (2020). Trait-based aerial dispersal of arbuscular mycorrhizal fungi. New Phytol. 228 (1), 238–252. doi: 10.1111/nph.16667
Chen M., Arato M., Borghi L., Nouri E., Reinhardt D. (2018). Beneficial services of arbuscular mycorrhizal fungi – from ecology to application. Front. Plant Sci. 9. doi: 10.3389/fpls.2018.01270
Compant S., van der Heijden M., Sessitsch A. (2010). Climate change effects on beneficial plant-microorganism interactions. FEMS Microbiol. Ecol. 73 (2), 197–214. doi: 10.1111/j.1574-6941.2010.00900.x
Davison J., Moora M., Semchenko M., Adenan S. B., Ahmed T., Akhmetzhanova A. A., et al. (2021). Temperature and ph define the realised niche space of arbuscular mycorrhizal fungi. New Phytol. 231 (2), 763–776. doi: 10.1111/nph.17240
Frew A. (2022). Root herbivory reduces species richness and alters community structure of root-colonising arbuscular mycorrhizal fungi. Soil Biol. Biochem. 171, 108723. doi: 10.1016/j.soilbio.2022.108723
Gao W., Wang P., Wu Q. (2019). Functions and application of glomalin-related soil proteins: a review. Sains Malays. 48 (1), 111–119. doi: 10.17576/jsm-2019-4801-13
Gao Y., Jia Y., Yu G., He N., Zhang L., Zhu B., et al. (2019). Anthropogenic reactive nitrogen deposition and associated nutrient limitation effect on gross primary productivity in inland water of China. J. Clean. Prod. 208, 530–540. doi: 10.1016/j.jclepro.2018.10.137
Gao Y., Zhou Z., Ling W., Hu X., Chen S. (2017). Glomalin-related soil protein enhances the availability of polycyclic aromatic hydrocarbons in soil. Soil Biol. Biochem. 107, 129–132. doi: 10.1016/j.soilbio.2017.01.002
Gilliam F. S. (2007). The ecological significance of the herbaceous layer in temperate forest ecosystems. Bioscience 57 (10), 845–858. doi: 10.1641/B571007
Han Y., Feng J., Han M., Zhu B. (2020). Responses of arbuscular mycorrhizal fungi to N addition: a meta-analysis. Glob. Change Biol. 26 (12), 7229–7241. doi: 10.1111/gcb.15369
Hoeksema J. D., Chaudhary V. B., Gehring C. A., Johnson N. C., Karst J., Koide R. T., et al. (2010). A meta-analysis of context-dependency in plant response to inoculation with mycorrhizal fungi. Ecol. Lett. 13 (3), 394–407. doi: 10.1111/j.1461-0248.2009.01430.x
Hong S., Gan P., Chen A. (2019). Environmental controls on soil ph in planted forest and its response to nitrogen deposition. Environ. Res. Lett. 172, 159–165. doi: 10.1016/j.envres.2019.02.020
Huang B., Yan G., Liu G., Sun X., Wang X., Xing Y., et al. (2022). Effects of long-term N addition and precipitation reduction on glomalin-related soil protein and soil aggregate stability in a temperate forest. Catena 214, 106284. doi: 10.1016/j.catena.2022.106284
Janos D. P., Garamszegi S., Beltran B. (2008). Glomalin extraction and measurement. Soil Biol. Biochem. 40 (3), 728–739. doi: 10.1016/j.soilbio.2007.10.007
Jia S., Liu X., Lin W., Zheng Y., Li J., Hui D., et al. (2021). Decreased glomalin-related soil protein with nitrogen deposition in a 3-year-old cunninghamia lanceolata plantation. J. Soils Sediments. 22, 931–941. doi: 10.1007/s11368-021-03115-6
Kang F., Yang B., Wu J., Yang X., Wang L., Guo J., et al. (2020). Arbuscular mycorrhizal fungi alleviate the negative effect of nitrogen deposition on ecosystem functions in meadow grassland. Land Degrad Dev. 31, 748–759. doi: 10.1002/ldr.3491
Kimmel K., Furey G. N., Hobbie S. E., Isbell F., Tilman D., Reich P. B. (2020). Diversity-dependent soil acidification under nitrogen enrichment constrains biomass productivity. Glob. Change Biol. 26 (11), 6594–6603. doi: 10.1111/gcb.15329
Kivlin S. N., Hawkes C. V., Treseder K. K. (2011). Global diversity and distribution of arbuscular mycorrhizal fungi. Soil Biol. Biochem. 43 (11), 2294–2303. doi: 10.1016/j.soilbio.2011.07.012
Lee J., Lee S., Young J. (2008). Improved pcr primers for the detection and identification of arbuscular mycorrhizal fungi. FEMS Microbiol. Ecol. 65 (2), 339–349. doi: 10.1111/j.1574-6941.2008.00531.x
Li W., Jin C., Guan D., Wang Q., Wang A., Yuan F., et al. (2015). The effects of simulated nitrogen deposition on plant root traits: a meta-analysis. Soil Biol. Biochem. 82, 112–118. doi: 10.1016/j.soilbio.2015.01.001
Li X., Zhang C., Zhang B., Wu D., Zhu D., Zhang W., et al. (2021). Nitrogen deposition and increased precipitation interact to affect fine root production and biomass in a temperate forest: implications for carbon cycling. Sci. Total Environ. 765, 144497. doi: 10.1016/j.scitotenv.2020.144497
Ma X., Geng Q., Zhang H., Bian C., Chen H. Y. H., Jiang D., et al. (2021). Global negative effects of nutrient enrichment on arbuscular mycorrhizal fungi, plant diversity and ecosystem multifunctionality. New Phytol. 229 (5), 2957–2969. doi: 10.1111/nph.17077
Mariotte P., Meugnier C., Johnson D., Thébault A., Spiegelberger T., Buttler A. (2013). Arbuscular mycorrhizal fungi reduce the differences in competitiveness between dominant and subordinate plant species. Mycorrhiza 23 (4), 267–277. doi: 10.1007/s00572-012-0465-8
McDonnell T. C., Clark C. M., Reinds G. J., Sullivan T. J., Knees B. (2022). Modeled vegetation community trajectories: effects from climate change, atmospheric nitrogen deposition, and soil acidification recovery. Environ. Advances. 9, 100271. doi: 10.1016/j.envadv.2022.100271
Muruganandam S., Israel D. W., Robarge W. P. (2009). Activities of nitrogen-mineralization enzymes associated with soil aggregate size fractions of three tillage systems. Soil Sci. Soc Am. J. 73 (3), 751–759. doi: 10.2136/sssaj2008.0231
Qin S., Yang G., Zhang Y., Song M., Sun L., Cui Y., et al. (2022). Mowing did not alleviate the negative effect of N addition on the arbuscular mycorrhizal fungal community in a temperate meadow grassland. Front. Plant Sci. 13. doi: 10.3389/fpls.2022.917645
Roth M., Michiels H. G., Puhlmann H., Sucker C., Winter M. B., Hauck M. (2021). Responses of temperate forests to nitrogen deposition: testing the explanatory power of modeled deposition datasets for vegetation gradients. Ecosystems 24, 1222–1238. doi: 10.1007/s10021-020-00579-4
Ruiz-Lozano J. M., Aroca R., Zamarreño Á.M., Molina S., Andreo-Jiménez B., Porcel R., et al. (2016). Arbuscular mycorrhizal symbiosis induces strigolactone biosynthesis under drought and improves drought tolerance in lettuce and tomato. Plant Cell Environ. 39 (2), 441–452. doi: 10.1111/pce.12631
Sato K., Suyama Y., Saito M., Sugawara K. (2005). A new primer for discrimination of arbuscular mycorrhizal fungi with polymerase chain reaction-denature gradient gel electrophoresis. Grassl. Sci. 51 (2), 179–181. doi: 10.1111/j.1744-697X.2005.00023.x
Schenck N., Perez Y. (1990). “Manual for the identification of va mycorrhizal fungi,” in Manual for the Identification of Va Mycorrhizal Fungi. (Synergistic, Gainesville, USA)
Smith S. E., Read D. J. (2008). Mycorrhizal symbiosis. 3rd edition. (San Diego: Academic Press). 148-158,174-175,182-186.
Smith L. J., Stephan K. (2021). Nitrogen fertilization, stand age, and overstory tree species impact the herbaceous layer in a central appalachian hardwood forest. Forests 12 (7), 829. doi: 10.3390/f12070829
Song L., Zhang J., Müller C., Jin G. (2019). Responses of soil n transformations and n loss to three years of simulated n deposition in a temperate Korean pine plantation in northeast China. Appl. Soil Ecol. 137, 49–56. doi: 10.1016/j.apsoil.2019.01.008
Thrippleton T., Bugmann H., Kramer-Priewasser K., Snell R. S. (2016). Herbaceous understorey: an overlooked player in forest landscape dynamics? Ecosystems 19 (7), 1240–1254. doi: 10.1007/s10021-016-9999-5
Tian D. S., Niu S. L. (2015). A global analysis of soil acidification caused by N addition. Environ. Res. Lett. 10 (2). doi: 10.1088/1748-9326/10/2/024019
Vuorenmaa J., Augustaitis A., Beudert B., Bochenek W., Clarke N., de Wit H. A., et al. (2018). Long-term changes, (1990–2015) in the atmospheric deposition and runoff water chemistry of sulphate, inorganic nitrogen and acidity for forested catchments in Europe in relation to changes in emissions and hydrometeorological conditions. Sci. Total Environ. 625, 1129–1145. doi: 10.1016/j.scitotenv.2017.12.245
Wang J., Wang G. G., Zhang B., Yuan Z., Fu Z., Yuan Y., et al. (2019). Arbuscular mycorrhizal fungi associated with tree species in a planted forest of eastern China. Forests 10 (5), 424. doi: 10.3390/f10050424
Weldon J., Merder J., Ferretti M., Grandin U. (2022). Nitrogen deposition causes eutrophication in bryophyte communities in central and northern European forests. Ann. For. Sci. 79 (1), 24. doi: 10.1186/s13595-022-01148-6
Xie K., Ren Y., Chen A., Yang C., Zheng Q., Chen J., et al. (2022). Plant nitrogen nutrition: the roles of arbuscular mycorrhizal fungi. J. Plant Physiol. 269, 153591. doi: 10.1016/j.jplph.2021.153591
Yu G., Jia Y., He N., Zhu J., Chen Z., Wang Q., et al. (2019). Stabilization of atmospheric nitrogen deposition in China over the past decade. Nat. Geosci. 12 (6), 424–429. doi: 10.1038/s41561-019-0352-4
Zeng H., Yu L., Liu P., Wang Z., Chen Y., Wang J. (2021). Nitrogen fertilization has a stronger influence than cropping pattern on amf community in maize/soybean strip intercropping systems. Appl. Soil Ecol. 167, 104034. doi: 10.1016/j.apsoil.2021.104034
Zhang Q., Li Y., Wang M., Wang K., Meng F., Liu L., et al. (2021). Atmospheric nitrogen deposition: a review of quantification methods and its spatial pattern derived from the global monitoring networks. Ecotoxicol. Environ. Saf. 216, 112180. doi: 10.1016/j.ecoenv.2021.112180
Zhao J., Chen S., Hu R., Li Y. (2017). Aggregate stability and size distribution of red soils under different land uses integrally regulated by soil organic matter, and iron and aluminum oxides. Soil Tillage Res. 167, 73–79. doi: 10.1016/j.still.2016.11.007
Keywords: nitrogen deposition, AM fungi, herbs, biodiversity, soil aggregates, glomalin-related soil protein
Citation: Wang W, Feng Y, Wu R, Wang X, He X, Zhang M, Li K, Jin G and Song F (2023) Long-term N addition reduced the diversity of arbuscular mycorrhizal fungi and understory herbs of a Korean pine plantation in northern China. Front. Ecol. Evol. 11:1192267. doi: 10.3389/fevo.2023.1192267
Received: 23 March 2023; Accepted: 13 November 2023;
Published: 29 November 2023.
Edited by:
Mauro Fois, University of Cagliari, ItalyReviewed by:
Fujuan Feng, Northeast Forestry University, ChinaMuhammad Atif Muneer, Fujian Agriculture and Forestry University, China
Garvin Schulz, University of Göttingen, Germany
Copyright © 2023 Wang, Feng, Wu, Wang, He, Zhang, Li, Jin and Song. This is an open-access article distributed under the terms of the Creative Commons Attribution License (CC BY). The use, distribution or reproduction in other forums is permitted, provided the original author(s) and the copyright owner(s) are credited and that the original publication in this journal is cited, in accordance with accepted academic practice. No use, distribution or reproduction is permitted which does not comply with these terms.
*Correspondence: Guangze Jin, taxus@126.com; Fuqiang Song, 0431sfq@163.com