- 1College of Resources and Environment, Jilin Agricultural University, Changchun, China
- 2General Station of Agricultural Environmental Protection and Rural Energy Management of Jilin Province, Changchun, China
- 3Key Laboratory of Urban Stormwater System and Water Environment, Ministry of Education, Beijing University of Civil Engineering and Architecture, Beijing, China
Introduction: Composting is a highly effective method for managing the growing quantity of agricultural waste, promoting nutrient recycling, and advancing sustainable agriculture. However, the significant amounts of volatile inorganic compounds (VICs) and volatile organic compounds (VOCs) generated during the composting process cannot be ignored due to their serious ecological environmental hazards and detrimental impact on human health. The types and pathways of VICs and VOCs produced during the composting process remain not well understood.
Methods: In this study, we investigated the VOCs produced during chicken manure and straw composting using gas chromatography–mass spectrometry (GC/MS). We performed qualitative and quantitative analyses of VOCs in composting emissions gases and their correlation with odor.
Results: Our results showed that 44 VOCs were detected during composting, including 4 oxidation-containing compounds, 2 sulfur-containing organics, 5 alkanes, 19 halogenated compounds, and 14 aromatic compounds. Aromatic compounds were found to be the main component of VOCs, accounting for 59.14% of the total content. The highest VOC content was found in the pre-composting period, and the highest emitting VOC was p-Isopropyl Toluene, which reached 7870.50 μg/m3. We identified eight substances that were highly correlated with odor concentration (p < 0.01) and are the main odor-causing substances that need to be monitored and controlled.
Discussion: Our study provides important insights into the transformation of volatile gases during the composting process. It highlights the need for proper management practices to control VOCs emissions and reduce their environmental impact.
Highlights
– The highest VOC emission in composting is p-Isopropyl Toluene, 7870.50 μg/m3.
– The aromatic compounds were the main component of VOCs, accounting 59.14%.
– Eight VOCs were highly positive correlated with the total odor concentration.
– The conversion pathways of VOCs emitted were quantified.
1. Introduction
Aerobic composting has emerged as a popular technology for agricultural waste reutilization due to its advantages of low cost, high safety, and high treatment capacity (Awasthi et al., 2020). This process eliminates potentially dangerous viral organisms and helps address associated environmental problems, such as nutrient recycling (Wang et al., 2018; Yang C. et al., 2019), making it vital for achieving sustainable agricultural development (Zhao et al., 2022). The organic fertilizer produced through aerobic composting contains comprehensive crop-demand nutrients, such as nitrogen and phosphorus, and ample organic nutrients, including amino acids, proteins, and humus. As a result, it is commonly used in agricultural production. However, it is essential to note that aerobic composting can also lead to secondary pollution. Aerobic composting contributes to greenhouse gas emissions that damage the environment and thus affect the carbon cycle in the ecosystem (Ochoa-Hueso et al., 2017; Chen et al., 2022). During the decomposition of metabolites and nutrients by microorganisms, diverse Volatile Inorganic Compounds (VICs) and Volatile Organic Compounds (VOCs) are emitted, including ammonia, hydrogen sulfide, alkanes, sulfur-containing organics, and aromatic compounds. These emissions can result in environmental pollution, nutrient loss, and human health threats (Rincón et al., 2019; Zhang Z. et al., 2021).
Agricultural waste, including straw and livestock manure, is produced at an alarming rate of nearly 9 billion tonnes globally each year due to economic development and population growth (Zhang X. et al., 2021). Shockingly, one-third of this waste is not disposed of safely but rather dumped, landfilled, or burnt, leading to the soil, water, and atmospheric pollution (Yao et al., 2021). Northeast China, a central corn-producing region, total corn production reached 93.56 million tons, is responsible for large quantities of corn straw burning, contributing to China’s air pollution (Zhiyu et al., 2021; China Statistics Press, 2022). With the rapid development of China’s livestock industry, 12 billion poultry are slaughtered each year, producing chicken manure as the most representative organic waste with ~1.63%, 1.54%, 0.85% of trace elements such as nitrogen, phosphorus, and potassium (National Bureau of Statistics of China, 2020). However, improper treatment of chicken manure can lead to serious environmental problems, including strong odors and heavy metal pollution (Gay et al., 2003). Thus, properly treating chicken manure and straw is crucial for agroecological protection.
NH3 and H2S are recognized as the main components of malodorous air pollutants and precursors for haze formation among VICs during the aerobic composting process (Zheng et al., 2021). These gases can easily harm human cardiovascular and respiratory systems due to their low olfactory thresholds (Wu et al., 2017). NH3 emissions are the primary pathway for nitrogen loss during composting, reducing 20–60% of total nitrogen fertilization efficiency (Jiang et al., 2018; Chen et al., 2018a; Li et al., 2020). Recent studies have also shown that H2S is the primary constituent of malodor gas emitted during the composting process, with an odor threshold of only 0.5–2 ppb in air. This gas produces a unique ‘rotten egg’ smell and has attracted significant attention recently (Li et al., 2022). To mitigate these issues, researchers have explored biotrickling filters packed with polyhedral spheres, which have demonstrated the best efficiency in compost deodorization (Huan et al., 2021). Therefore, managing NH3 and H2S emissions during composting is crucial to reduce their negative impacts on human health and the environment.
Volatile organic compounds (VOCs) typically have small molecular weights and low melting points and can arise from the raw materials themselves or incomplete organic matter decomposition. Studies have revealed that VOCs and their emissions vary significantly throughout the composting process. A diverse range of VOCs can be generated during composting, with many appearing during the early stages of the process. The concentration of total volatile organic compounds (TVOCs) is highest in the early stages, with levels reaching 3,000–14,000 mg/m3, which is 1.5–2 times greater than those in the middle and late stages (Eitzer, 1995; Turan et al., 2007; Gao et al., 2010; Kumar et al., 2011). Many VOCs are malodorous, and some can be toxic, irritating, and carcinogenic (Sánchez-Monedero et al., 2019), posing threats to human health (Nicell, 2009), VOCs cause air pollution that further affects ecosystems (De Marco et al., 2019), and potential impacts on the greenhouse effect and global climate change (Shen et al., 2012). Studies have demonstrated that different compost materials can lead to the production of different VOCs, including methyl sulfide, dimethyl disulfide, methanethiol, ethylbenzene, methylamine, p-isopropyl toluene, ammonia, acetic acid, and dimethyl trisulfide, among others (Van Durme et al., 1992; Noble et al., 2001; Tsai et al., 2008; Zhang et al., 2012; Shen et al., 2016). Although research has focused on the generation period and types of VOCs, little research has explored the transformation pathways of VOCs during different periods. Therefore, a clear understanding of the transformation pathways of VOCs is crucial for further control VOCs pollution for an ecological environment sustainable future development exploration (Nie et al., 2019; Huang et al., 2020; Aryal et al., 2022; Guan et al., 2023).
The objectives of this study are, therefore to (1) clarify VICs and VOCs conversion paths at different periods in the composting process. (2) Gain insights into the release characteristics of VICs and VOCs during composting. (3) Identify the main odor-causing substances in volatile gases to provide an accurate reference for effective control of composting odor gas emissions to deliver a sustainable future.
2. Materials and methods
2.1. Composting materials and processes
2.1.1. Composting materials
Corn straw and chicken manure were used as composting material, corn straw and chicken manure were taken from the waste of Changchun Jiaxin farm. The composting unit was a solid fermenter type 30SS-2020. The chicken manure and corn straw were naturally dried and then prepared. Each sample was analyzed thrice within 24 h after collection, and the average concentration was used for analysis. The basic physical and chemical properties of the chicken manure and corn straw are shown in Table 1.
2.1.2. Composting processes
The composting material used in this study was a mixture of air-dried chicken manure and trampled sections of corn straw. To achieve an appropriate carbon-to-nitrogen ratio required for aerobic fermentation and to maintain good ventilation, the corn straw was crushed into small sections of approximately 1 cm (Figure 1A). Chicken manure and corn straw were mixed in a ratio of C:N = 25:1. The initial moisture content was controlled at 60%, and aeration was adjusted to maintain a concentration of oxygen in the exhaust gas of no less than 10%. Air samples were collected using the SOC-01 sampling system at the fermenter exhaust port (Figure 1B). The system was connected to an 8 l polyester sample bag operated according to the lung principle (Ding et al., 2007). A mass flow controller was used with a collection rate of 15 l/min. Each ambient air sample was collected over 20 s, resulting in a sample volume of approximately 5 l. Each sample bag was collected and vented for air cleaning 3 times at the sampling point before the sample was collected to minimize the effect of VOCs in the bag. The input and connection lines each contain a Teflon sampling tube. Each sample was analyzed thrice within 24 h after collection, and the average concentration was used for analysis.
The composting period was from 25 July 2022 to 20 August 2022, with a composting time of 26 days. Gas was collected during the pre-composting period (measured on day 3 of composting, 28 July 2022), mid-composting period (measured on day 14 of composting, 8 August 2022), and end-composting period (measured on day 21 of composting, 15 August 2022).
2.2. Gas determination methods
2.2.1. Devices and reagents
The Devices and reagents used for the test are listed in Table 2.
2.2.2. Measurement methods
To measure the concentration of ammonia (NH3) in the exhaust gas from the composting process, a gas wash bottle containing 100 mL of 2% boric acid solution was connected to the fermenter exhaust using a rubber tube. The gas wash bottle was sealed with a rubber stopper, and two glass tubes were inserted into the plug to allow the exhaust gas to enter and exit the bottle. The exhaust gas was directly discharged into the boric acid solution flask. After absorbing the ammonia in the exhaust gas for 15 min, the concentration of NH3 was measured using the H2SO4 titration method. This method ensured accurate measurement of NH3 concentration in the exhaust gas during the composting process.
To determine the concentration of H2S in the composting process, a biological gas measuring instrument was employed. The exhaust gas discharged from the fermenter exhaust port was first passed through a desiccant bottle and then directly connected to the gas receiving pipe of the biological measuring instrument. The instrument is equipped with a built-in pump, and the H2S content data stabilized after approximately 1 min of pumping. The concentration of H2S gas was then read directly from the data window of the measuring instrument.
The determination of VOCs in the composting process was carried out using gas chromatography–mass spectrometry (GC–MS) following the method reported by González et al. (2019) and Wang et al. (2013). The mass spectrometry method was qualitative in full scan mode, and the peak area external standard method was used for quantitative analysis. The experimental conditions of the instrument setup were as follows: a thermal desorption instrument was used with a transmission line temperature of 130°C; the initial temperature of the adsorbent tube and the focus tube was 35°C; the desorption temperature of the adsorbent tube and the focus tube was 325°C with a desorption time of 3 min and 5 min, respectively; the primary desorption flow rate was 40 ml/min; the aging temperature of the focus tube was 350°C. The GC setup conditions were as follows: the inlet temperature was set at 200°C with helium gas as the carrier gas and a shunt ratio of 5:1; the column flow rate was 1.2 ml/min with a ramp-up procedure of initial temperature at 30°C, hold for 3.2 min, ramp up to 200°C at 11°C/min, and hold for 3 min; the capillary column used was DB-624 with a dimension of 30 m × 0.25 mm × 1.4 μm film thickness. The mass spectrometer was fully scanned with a scanning range of 35 ~ 270 amu, ionization energy of 70 eV, and interface temperature of 280°C.
2.3. Statistical analysis
The statistical analyses were performed using IBM SPSS Statistics version 23 (IBM, Armonk, NY, USA), with a significance level set at p < 0.05. Correlations were calculated by Pearson correlation. Data visualization was conducted using Origin Pro 2021 (Origin Lab, Northampton, MA, USA). Microsoft Excel 2016 was used for basic calculations.
3. Results and discussion
3.1. NH3 and H2S emission characteristics
NH4+-N is a critical precursor of NH3 and a substrate for nitrification and assimilation, playing a vital role in the nitrogen conversion process of compost. The NH4+-N content can be reduced by increasing the rate of ammonia oxidation, which in turn reduces NH3 release. Figure 2A shows the measured NH3 concentrations during the pre-, mid-, and post-composting sampling. NH3 concentrations were highest in the early stage and gradually decreased as the composting progressed. In the early stage of composting, high water content resulted in the ammonia produced by the decomposition of organic nitrogen, such as amino acid nitrogen, mainly present in a pile as NH4+-N. During the high-temperature period, NH4+-N was converted into NH3 and released from the pile due to its high temperature and high pH. The production of NH3 is closely related to NH4+-N, and the more NH4+-N is produced, the more NH3 is released. Under poor ventilation conditions, denitrifying bacteria convert nitrite to N2O or N2 (Zhao et al., 2021). Nitrifying bacteria are predominantly mesophilic, and their activity is inhibited in the high-temperature phase (Hort et al., 2009), preventing the timely conversion of ammonium nitrogen to nitrate nitrogen and intensifying the volatilization of ammonia. However, as the composting progresses, the activity of nitrifying bacteria increases, with decreasing ammonium nitrogen and increasing nitrate nitrogen, significantly reducing the volatilization of NH3. Some incomplete nitrification of NH4+-N or denitrification of NO2-N and NO3-N in the local anaerobic environment will produce by-products, mainly N2O, NO2, and other nitrogen oxides (Jarvis et al., 2009; Yang F. et al., 2019). The volatilization of NH3 is mainly produced from the decomposition of nitrogenous organic matter in the material. Organic components such as proteins in livestock manure and straw are decomposed by ammonifying bacteria, producing a large amount of NH4+-N, which increases the pH and causes ammonia to volatilize directly in the presence of sufficient oxygen. As the temperature of the pile increases, the solubility of NH3 decreases, and NH3 is transformed from NH3-H2O in the aqueous phase to the gaseous state and volatilized to the atmosphere. The higher the temperature, the greater the volatilization. The emission of NH3 produces odor, and long-term exposure to excessive NH3 threatens human health and causes the loss of compost nutrients, so it should be controlled during the composting process.
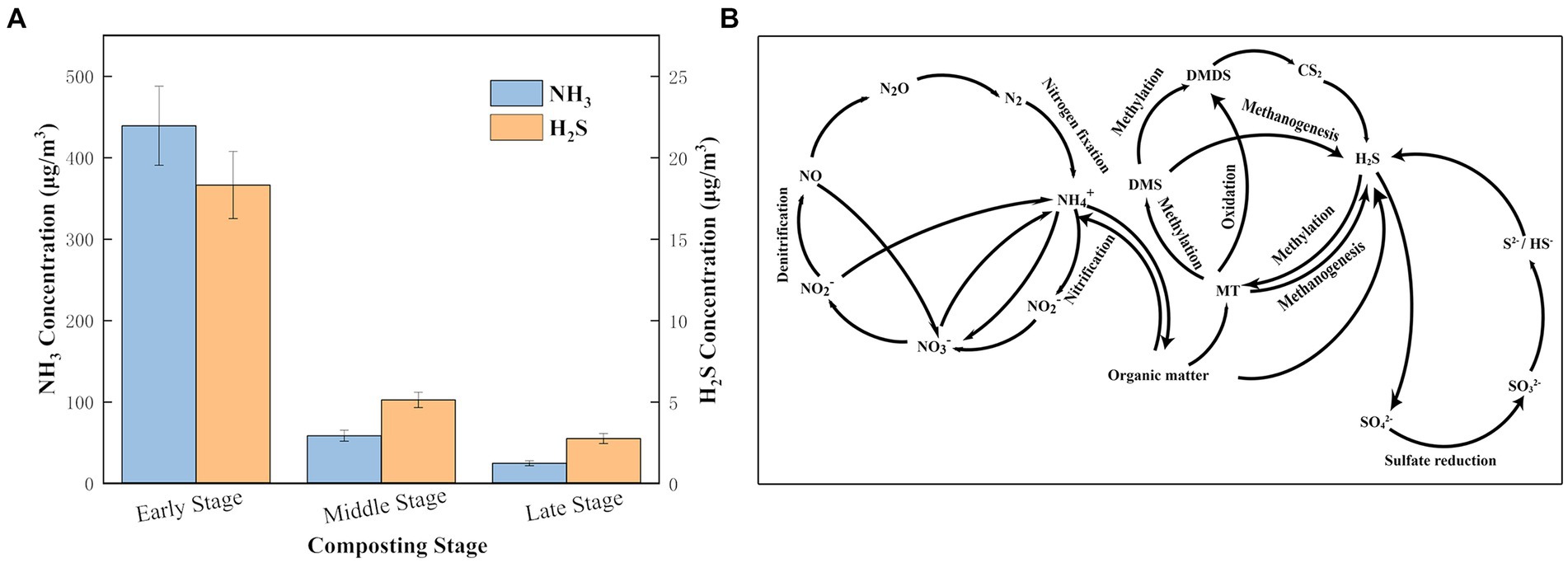
Figure 2. Concentration changes (A) and main transformation pathways (B) of NH3 and H2S in different composting stages.
The composting of corn straw and chicken manure under aerobic fermentation conditions in this study resulted in high oxygen consumption in the early stages, leading to the presence of local anaerobes in the pile. Anaerobic conditions during the decomposition of sulfate-reducing bacteria and sulfur-containing organic matter produced H2S, which has a low odor threshold and is highly malodorous. It is crucial to explore the release pattern of H2S gas. Figure 2A indicates that the H2S concentration was highest in the pre-composting period and gradually decreased in the middle and late composting periods. In the early stages, the temperature rose rapidly, causing local anoxia while producing sulfur-containing compounds, and microbial activity increased, leading to the rapid decomposition of easily decomposable organic matter. Sulfite compounds decomposed into S2−, combined with H+ to release H2S in gas form. In the middle and late stages, microbial activity weakened, and oxygen consumption decreased, gradually decreasing H2S gas concentration. Biodegradation of sulfur-containing amino acids such as cysteine and methionine in compost can form H2S and methyl mercaptan (MT). In contrast, methylation of H2S and MT can form MT and methyl sulfide (DMS), respectively (Figure 2B). MT can be oxidized to form dimethyl disulfide (DMDS) (Higgins et al., 2006; Arellano-García et al., 2018). Moreover, MT and DMS from sulfide methylation reactions produce H2S during methanogenesis by methanogens. Under low redox potential (ORP) conditions, H2S lacks electron acceptors such as dissolved oxygen (DO), Cl2, NO3−, Fe3+, ClO-, and KMnO4−, and sulfides are formed under anaerobic conditions (Delgado et al., 1999). The optimal ORP for H2S production in compost has been identified to be within the range of −100 to −250 mV (Boon, 1995). Thus, increasing the redox potential can reduce H2S production (Zhang et al., 2008) by adding favorable electron acceptor compounds such as oxidants.
3.2. Emissions and changes of VOCs in the composting process
3.2.1. Qualitative analysis of VOCs
Gas chromatography–mass spectrometry analysis was performed on compost samples collected from different stages of the composting process to identify and quantify volatile organic compounds (VOCs). A total of 44 VOCs were detected and identified based on chromatograms, standard spectra search, and manual analysis. The identified VOCs included 4 oxygen-containing compounds (tetrahydrofuran, acetone, 2-butanone, 2-ketone), 2 sulfur-containing organics (dimethyl disulfide, carbon disulfide), 5 alkanes (n-tridecane, n-tetradecane, n-pentadecane, n-eicosane, n-twenty-six), 19 halogenated compounds (dichlorodifluoromethane, chloromethane, bromo-methane, chloroform, carbon tetrachloride, trichloroethylene, 1,2-dichloropropane, 1,1,2-trichloroethane, tetrachloroethylene, dibromochloromethane, 1,2-dibromoethane, chlorobenzene, 1,3-dichlorobenzene, bromobenzene, 2-chlorotoluene, 1,2-dibromo-3-chloropropane, 1,2,4-trichlorobenzene, hexachlorobutadiene, 1,2,3-trichlorobenzene), and 14 aromatic compounds (benzene, toluene, ethylbenzene, m,p-xylene, styrene, isopropylbenzene, n-propylbenzene, 1,3,5-trimethylbenzene, tert-butylbenzene, 1,2,4-trimethylbenzene, sec-butylbenzene, p-Isopropyl Toluene, n-butylbenzene, naphthalene) (Supplementary Table S1).
The VOCs produced by different compost substrates are different. Schiavon et al. (2017) found that the VOCs produced by using different substrates for composting were different. The VOCs produced by sludge composting were mainly terpenes, organosulphur compounds and esters. Using municipal solid waste and green waste composting, the main VOCs detected were terpenes, which may originate from fruit and vegetable waste, and as intermediate products of aerobic degradation of organic matter. A variety of VOCs are generated in the process of using food waste to degrade diesel composting, and they can be classified as alkanes, cycloalkanes, aromatics, alcohols, esters, ketones, aldehydes, alkenes, sulfurs, amines, and terpenes (He et al., 2020). Since the diesel component consists of 79% alkanes and 20% benzene rings, a large amount of alkanes, cycloalkanes and benzene rings are produced in the pre-composting stage (Schiavon et al., 2017; Nie et al., 2019). Kitchen waste compost has a strong odor due to amines and sulfides (Bruno et al., 2007). Lin et al. (2023) showed that trimethylamine is the most common amine. Sulfide and amines may come from kitchen meat protein because it contains N and S elements, these are the primary source of compost odor (Koyama et al., 2018). González et al. (2019) research found that Terpenes, organosulphur compounds, ketones and carboxylic acids were found to be the most abundant VOCs present in the gaseous emissions. Nie et al. (2019) research found that the use of sludge composting produced VOC-like compounds, with the highest emissions of oxygenated compounds.
3.2.2. Quantitative analysis of VOCs
Figure 3 illustrates the total amount of gases released during different stages of composting, which were analyzed based on their emission patterns. The pre-composting phase exhibited the highest concentration of volatile organic compounds (VOCs), with 43 types of VOCs detected, and the total emitted gases reached 35537.76 μg/m3. In the middle and late stages, 23 and 17 types of VOCs were detected, respectively, with a significant reduction in both the types and the total amount of emitted gases, measuring 14,831.07 and 14,462.07 μg/m3, respectively. The high concentration of VOCs in the early phase of composting can be attributed to the intense microbial activity and rapid decomposition of easily degradable organic substances, which produce large amounts of VOCs coupled with low oxygen concentration and high moisture content in the composting material. As the easily degradable organic matter decreases in the later stages, the temperature also decreases, reducing the production of VOCs. The pre-composting period plays a crucial role in controlling gas emissions. The high temperature in composting can accelerate the decomposition of materials but leads to a higher emission of VOCs, which can significantly impact human health and the surrounding environment. Using a standard external method, 44 VOCs were quantified and analyzed in different periods of the composting process, with p-Isopropyl Toluene exhibiting the highest concentration of 7870.50 μg/m3 measured in the pre-composting period (Supplementary Table S2). These findings highlight the need to control VOC emissions during composting and implement measures to mitigate their impact on the environment and human health.
3.2.3. Analysis of the release characteristics and mechanism of VOCs in the composting process
The concentration of most VOCs during composting is highest in the initial stages of the process. However, due to the significant differences in the concentration levels of various types of VOCs during the same composting stage, the high-concentration VOCs can mask the variation patterns of the low-concentration VOCs. Therefore, it is important to investigate the concentration variations and emission mechanisms of different types of VOCs during composting. Understanding the various patterns and mechanisms of VOC emissions can help develop strategies to effectively control and reduce the emission of harmful VOCs during composting.
3.2.3.1. Oxygenated compounds
The composting process is known to produce various oxygenated compounds, such as acetone, 2-ketone, and 2-butanone, which are standard products in agricultural waste composting. However, the concentration and production patterns of these compounds can vary throughout the composting process. In this study, Figure 4A shows that tetrahydrofuran was only produced in the pre-composting period, indicating local anaerobic conditions and insufficient oxygen supply. Although this test is aerobic fermentation composting, in the early stage of composting, the water content of the pile is high, the microbial activity increases, the range of easily degradable organic matter is relatively abundant, and the frequent microbial activities lead to the relatively low oxygen concentration in the pile, which may have local anaerobic and produce ether tetrahydrofuran. On the other hand, acetone was mainly produced in the middle of the composting period, possibly due to the hydrolysis of compost raw materials such as sugar, starch, and cellulose in straw. Acetone is mostly produced by hydrolysis of compost raw materials such as sugar, starch, and cellulose in straw. Its potential source is the decomposition by-products of easily degradable organic matter in waste, and acetone is only produced in the middle of composting, probably because there is more pyruvate in the middle of composting, and the intermediate products produced by the tricarboxylic acid cycle are metabolized by ketone bodies to produce acetone, and later in composting, as the pH rises, pyruvate gradually decreases and does not produce acetone. In contrast, 2-ketone was only produced in the early stages and low concentrations. At the same time, 2-butanone had the highest concentration in the early stage of composting, which may be due to the abundance of soluble organic matter and free microbial activity. 2-ketone is produced by the conversion of complex carbohydrates in an acidic environment, and 2-ketone is only produced in the early stages and low concentrations because the high metabolic activity of microorganisms in the early stages leads to anoxic conditions, which means that the system gradually becomes anaerobic and 2-ketone is not oxidized, while as microbial activity decreases in the middle and late stages, 2-ketone is no longer oxidized. The highest concentration of 2-butanone in the early stage of composting may be due to the abundance of soluble organic matter (TOC) in the compost material used. Microbial activity is not limited by the concentration of substrate, resulting in the release of a large amount of butanone as a metabolic intermediate product, in the middle stage of composting, the temperature is lower than in the early stage of composting, and the solubility of 2-butanone increases and the emission decreases, while in the late stage of composting, the oxygen content increases, the pH increases, and a large amount of acetone was converted to butanone, which increased the emission compared with the middle stage of composting. These oxygenated compounds are essential contributors to composting emissions from agricultural waste such as vegetable and fruit, garden waste, and livestock manure. Understanding their production patterns can help improve composting practices and reduce emissions.
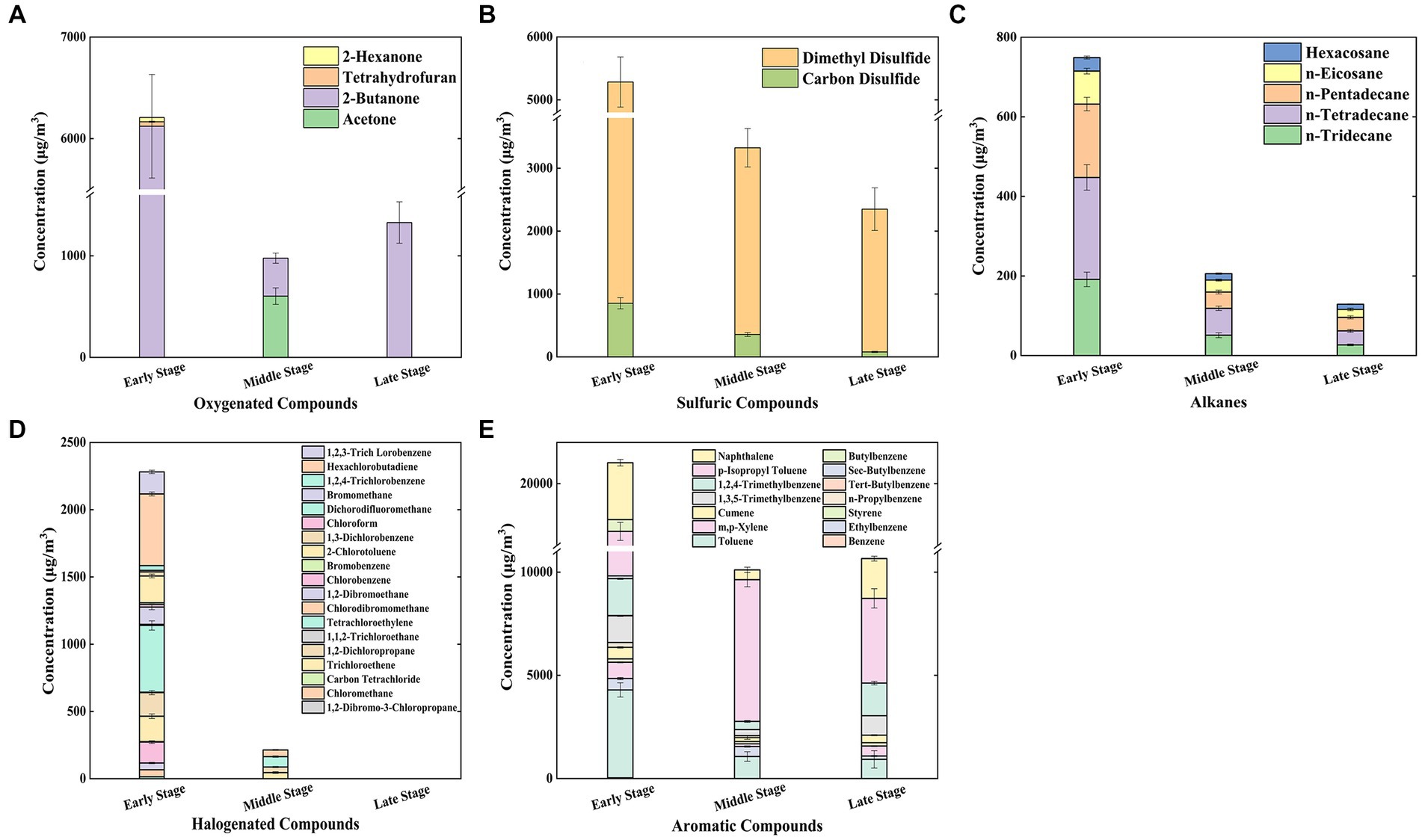
Figure 4. Concentration changes of Oxygenated Compounds (A), Sulfuric Compounds (B), Alkanes (C), Halogenated Compounds (D), Aromatic Compounds (E), in different composting stages.
3.2.3.2. Sulfuric compounds
Carbon disulfide and dimethyl disulfide have been detected in all stages of composting, with the highest concentrations found in the initial phases of composting and gradually decreasing as composting progresses. Sulfur-containing organic matter is mainly produced in semi-aerobic and anaerobic conditions. Degradation of sulfur-containing amino acids releases sulfur compounds, including dimethyl sulfide, carbon disulfide, and styrene, all categorized under the Emission Standards for Odorous Pollutants (GB 14554–93) by the Chinese Ministry of Environmental Protection. In this study, the main components of sulfur-containing compounds were dimethyl disulfide and carbon disulfide, which aligns with previous findings (Maulini-Duran et al., 2015). Methanethiol is produced during composting, and its oxidation results in the formation of dimethyl disulfide. The equation explains this reaction, where R is typically an aromatic compound (Han et al., 2018).
Figure 4B shows that sulfuric compound concentration changed in different composting stages. During the early stages of composting, volatile sulfides are rapidly formed from biodegradable organic sulfur, resulting in a significant increase in dimethyl disulfide and carbon disulfide emissions (Han et al., 2019; Cao et al., 2022). However, as composting progresses, the biodegradable organic sulfur content decreases, and the oxygen supply increases, reducing carbon disulfide and dimethyl disulfide emissions. Chicken manure, a common composting material, is rich in proteins containing -SH groups that generate sulfur-containing amino acids like cystine, cysteine, and methionine under enzyme action. These amino acids then undergo desulfurization under anaerobic conditions, resulting in the production of H2S, which can be oxidized to sulfate via sulfidation under aerobic conditions. However, when composting lacks sufficient oxygen supply, slow sulfidation leads to the accumulation of H2S, resulting in the generation of methyl sulfide and dimethyl sulfide as intermediate products of the desulfurization reaction. Therefore, ensuring adequate oxygen supply is crucial in controlling the production and emission of sulfur-containing VOCs (Fisher et al., 2018).
3.2.3.3. Alkanes
Figure 4C shows that alkane concentration changed in different composting stages. The concentration patterns of five alkanes (n-tridecane, n-tetradecane, n-pentadecane, n-eicosane, and n-twenty-six) were examined during composting. It was observed that the highest concentrations were present in the pre-composting stage, followed by a decline in concentration as composting progressed. Alkanes with low molecular weight (<C10) are considered short-chain alkanes, while those with high molecular weight (>C10) are classified as long-chain alkanes (Tassi et al., 2009; Duan et al., 2014). Long-chain alkanes, especially, have been linked to particulate matter (PM 2.5) pollution in urban areas. Alkanes are primarily associated with the decomposition of organic matter in livestock manure (Mustafa et al., 2017). Furthermore, alkanes will likely be released during fermentation at elevated internal temperatures and humidity (Komilis et al., 2004; Tan et al., 2017). During the pre-composting stage, the material undergoes adequate aerobic fermentation, leading to a higher concentration of alkane emission. The concentration of alkanes decreases as composting progresses due to two reasons. Firstly, long-chain molecules of hydrocarbons break down into various short-chain gaseous hydrocarbons and a small number of liquid hydrocarbons. Secondly, halogens are produced during the composting process, and the hydrogen atoms in the alkanes are replaced by halogens in halogenation reactions, leading to a reduction in the concentration of the alkanes. The temperature decrease during composting makes long-chain alkanes more stable. In contrast, the boiling point of alkanes increases with the increase of carbon atoms, making short-chain alkanes easier to separate than long-chain alkanes. This also explains why the gas concentration of n-twenty-six alkanes changes the least in the middle and late stages of composting.
3.2.3.4. Halogenated compounds
Halogenated compounds are a concerning class of pollutants that can be emitted during composting processes. Figure 4D shows that halogenated compound concentration changed in different composting stages. In this study, we observed that the concentration patterns of the 19 halogenated compounds seen were highest at the beginning of composting, decreasing as composting progressed, and no longer detected at the middle and end of composting. This may be due to the production of tetrachloroethylene and bromomethane by aerobic fermentation and composting of the material, which is mainly produced by the degradation of chlorine- and bromine-containing compounds (Liu et al., 2009). These compounds are commonly used in agriculture and can be present in chicken manure and straw. The production of halogenated compounds during composting is also attributed to chlorinated and brominated organic compounds and other solvents in the composted materials (Duan et al., 2014; Mustafa et al., 2017). The high temperature and microbial activity during the early stage of composting lead to the production of organic compounds containing chlorine and bromine, and a large number of hydrogen atoms on alkanes are replaced by organic compounds containing chlorine and bromine, generating a variety of halogenated compounds through substitution reactions. As composting proceeds, the decomposition reaction reduces, leading to a gradual decrease in the concentration of halogenated compounds in the later stages. Despite their highest emissions only at the beginning of composting, the emissions of halogenated compounds require attention because they have a low olfactory threshold and may pose a greater toxicological risk to the surrounding population.
3.2.3.5. Aromatic compounds
During the composting process, the concentrations of 8 out of the 14 aromatic compounds - including benzene, toluene, ethylbenzene, n-propylbenzene, tert-butylbenzene, sec-butylbenzene, p-Isopropyl Toluene, and n-butylbenzene - were highest in the early stage and gradually decreased over time or ceased to be produced altogether. On the other hand, the concentrations of the remaining 6 aromatic compounds - m,p-xylene, styrene, isopropylbenzene, 1,3,5-trimethylbenzene, 1,2,4-trimethylbenzene, and naphthalene - followed a different pattern. Their concentrations were highest in the early stage of composting, decreased in the middle stage, and then increased again in the late stage.
Figure 4E shows that aromatic compound concentration changed in different composting stages. Fourteen aromatic compounds were detected in the samples, with toluene being the primary aromatic compound, accounting for about 35% of the aromatic compound concentration on average (Sánchez-Monedero et al., 2018). Toluene dominates in aromatic compounds, and its concentration during composting decreases sequentially with composting time, consistent with previous findings (Moreno et al., 2014). Toluene is commonly used as an intermediate in the degradation of organic waste (Chiriac et al., 2011), and it is biological sources are very common in the composting process (Shao et al., 2014). Aromatic compounds are also classified as exogenous due to their biological sources in the composting process. The ratio of benzene to toluene (B:T ratio) is commonly used to identify sources of aromatic emissions. In this study, the average B:T ratio ranged from 0.01–0.02, significantly smaller than that of other studies, such as 0.4–1.0 from vehicle exhaust in Beijing city and 0.2–0.7 from Hangzhou landfill (Wang et al., 2012). Therefore, the sample’s concentration and composition of aromatic compounds were less affected by external influences. The most common aromatic compounds in the chicken manure composting process are benzene, toluene, ethylbenzene, and xylenes (m-xylene, p-xylene, and o-xylene). Exposure to these compounds can lead to various health problems (Durmusoglu et al., 2010). Thus, it is important to know the potential health risks to people exposed to these compounds in or near composting plants.
3.2.4. The main transformation pathways of VOCs in the composting process
Figure 5A depicts the percentage changes of various categories of VOCs during composting. It can be observed that the percentage of alkanes and halogenated compounds decreased gradually, whereas the percentage of aromatic compounds increased. The percentage of sulfur-containing compounds exhibited an initial increase followed by a decrease, while the percentage of oxygen-containing compounds first decreased and then increased.
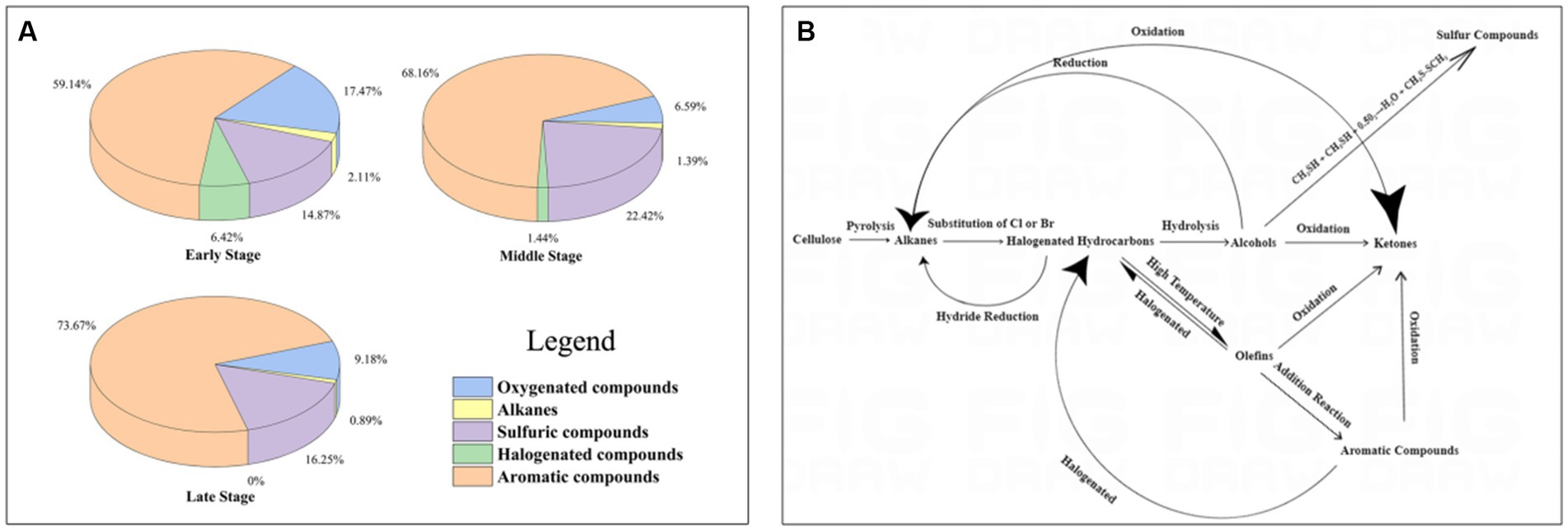
Figure 5. Percentage content (A) and main transformation paths (B) of various VOCs in different composting stages.
Figure 5B illustrates the transformation pathways of VOCs during composting. Our findings indicate that the emission of oxygenated compounds is mainly influenced by temperature and the degree of material fermentation. In the pre-composting period, microbial activity is more frequent, and the temperature rises faster when cellulose in the straw produces alkanes through pyrolysis (Mellouki et al., 2015). Alkanes can undergo substitution reactions with Cl or Br to produce halogenated hydrocarbons that subsequently undergo hydrolysis reactions to produce alcohols. Alcohols can be oxidized to produce ketones. O2 is a key factor in the degradation of organic matter. It is important that the microorganisms are provided with adequate oxygen to maintain their metabolic activities throughout composting. The oxygen consumption in the early stage of composting is high, leading to a semi-consumptive and semi-anaerobic state in the pile that produces a large amount of H2S. H2S can generate methanethiol with alcohols, and methanethiol can be oxidized to generate sulfur-containing compounds such as dimethyl disulfide (Equations 1, 2). The presence of oxygen can influence the composition classes of VOCs (Zheng et al., 2022). In the anaerobic zone of the composting, large amounts of dimethyl sulfide, dimethyl disulfide, H2S, carbonyl sulfide, carbon disulfide and methyl mercaptan are produced, and these compounds are generated by bacterial sulfate reduction and decomposition of sulfur-containing organic matter under anaerobic conditions (Dhamodharan et al., 2019; Duan et al., 2022). In the aerobic zone of the composting, aerobic microorganisms gradually come into play, with lower emissions of sulfur-containing compounds and higher emissions of alkanes and halogenated compounds, such as n-tridecane, n-tetradecane, 1,3-dichlorobenzene, bromobenzene, 2-chlorotoluene. Halogenated hydrocarbons can generate olefins at high temperatures, alkenes can form aromatic compounds by addition reaction (Atkinson and Arey, 2003). However, it is known that the conversion of olefins to aromatic compounds by addition reactions is more demanding (Sun et al., 2013; Chen et al., 2018b). Since the olefin emissions in this study were below the detection limit, we speculate that the emissions of aromatic compounds are related to the high organic compounds such as pesticides and chemical fertilizers left in the compost matrix (Rincón et al., 2019; Yang F. et al., 2019). In the middle and late stages of composting, oxygen consumption gradually decreased, leading to an increase in the concentration of oxygenated compounds. As the temperature of the pile gradually decreases and the straw in the material tends to decay, the concentration of alkanes produced through cellulose pyrolysis decreases. With the emission of gases containing Cl and Br in the pile, the concentration of halogenated hydrocarbons decreases to below the detection limit (He et al., 2019). Because aromatic compounds are structurally stable and do not break down easily, and the concentration of aromatic compounds increases as a percentage, accounting for up to 73.67% of the total VOCs emissions. However, the high toxicity of aromatic compounds suggests that although the total emissions of VOCs decreased in the middle and late stages of composting, the increase in the percentage of aromatic compounds does not mean that the toxicity of the emitted gas decreased. Instead, the toxicological risk to humans may increase.
3.3. Correlation analysis of the main odor-causing substances in VOCs with the total odor concentration
The odors that are produced due to the presence of volatile substances, such as VICs (NH3 and H2S) and VOCs (dimethyl sulfide, carbon disulfide, toluene, ethylbenzene, m,p-xylene, 1,3,5-trimethylbenzene, 1,2,4-trimethylbenzene, and naphthalene), during the composting of chicken manure have an impact on people’s lives due to their low olfactory threshold. According to the olfactory threshold criteria for odor-causing substances (Devos, 1995), although low, the concentration of an individual odor-causing substance is enough to cause an unpleasant experience. This is evidenced by the low olfactory threshold criteria, combined with the gases measured in different periods of composting, which resulted in the selection of 10 major odor-causing gases exceeding the limited olfactory threshold criteria.
The correlation analysis of the emission concentration of each malodorous substance and the total odor concentration conducted (Figure 6) revealed that, except for ethylbenzene and 1,2,4-trimethylbenzene, all the other eight malodorous substances showed a highly significant correlation (p < 0.01) with the odor concentration, indicating that these eight malodorous substances are the main odor-causing substances of the odor produced by chicken manure composting and should be monitored and controlled with emphasis (Hanajima et al., 2010). The correlation also showed that the eight main odoriferous substances could be divided into two groups, one comprising NH3, H2S, dimethyl disulfide, and carbon disulfide, and the other of toluene, m,p-xylene, 1,3,5-trimethylbenzene, and naphthalene, with a significant positive correlation (p < 0.05) between the concentrations of each odoriferous substance within the group. This indicates that there are similar emission patterns in the same group of substances and that the highest emissions of these eight main odor-causing substances are observed in the pre-composting period, making it the main period to focus on when monitoring and removing malodorous substances.
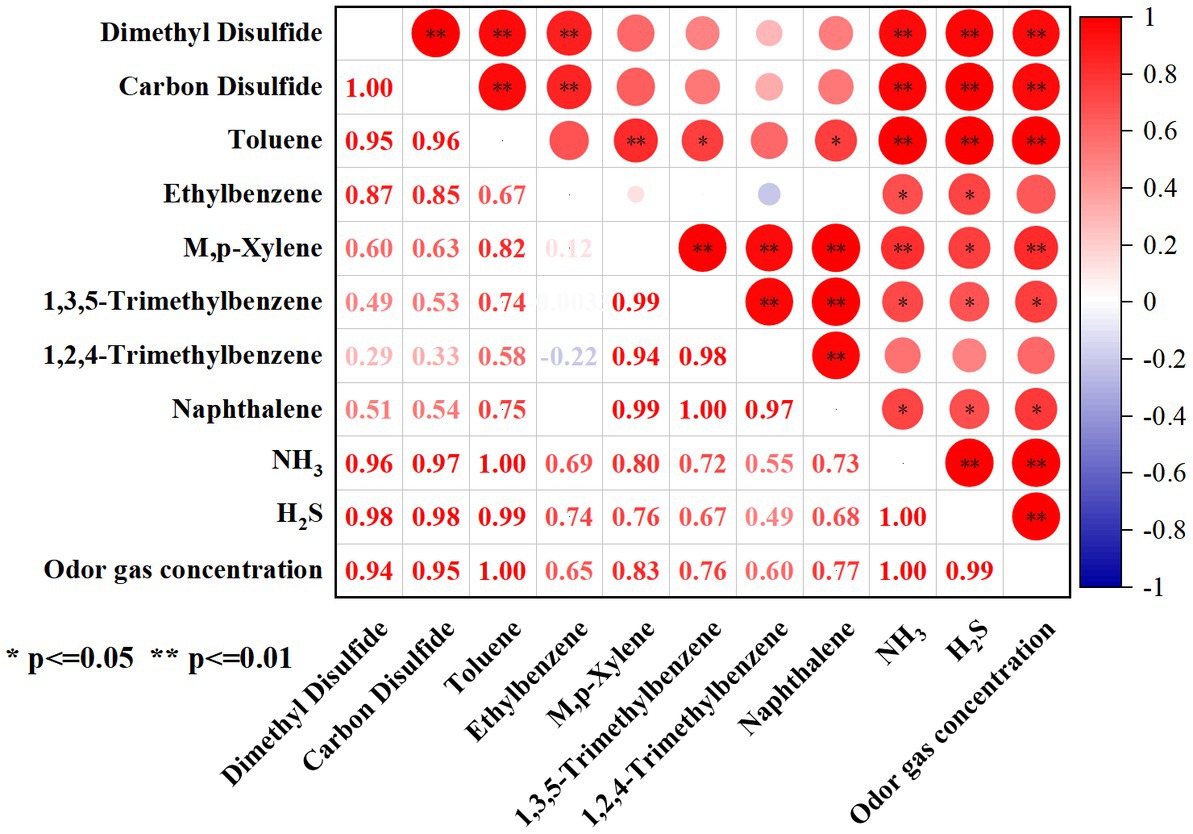
Figure 6. Correlation analysis of emission concentration of malodorous substances and total odor concentration.
NH3 and H2S are significantly positively correlated, with a low olfactory threshold and high sensitivity to humans, making them ideal indicators for odor pollution. Monitoring them, but also according to their concentrations, to test the effectiveness of odor removal. The main point as an indicator of odor pollution is that the concentration of the indicator should change with all or most of the major odor-causing substances with a high degree of consistency so that the indicator can respond to the emission of other malodorous substances, followed by the monitoring of odor indicators to facilitate the emission of odor indicators, odor pollution indicators should try to choose a higher concentration of gas to improve the accuracy of monitoring.
4. Conclusion
During the pre-composting period, the highest concentration of volatile gases and the largest concentration of major odoriferous substances were detected, with 44 kinds of VOCs identified, including 4 oxidized compounds, 2 sulfur-containing organics, 5 alkanes, 19 halogenated compounds, and 14 aromatic compounds. Aromatic compounds were the main component of VOCs, accounting for 59.14%. P-Isopropyl Toluene was found to have the highest content of VOCs, reaching 7870.50 μg/m3. Among the odors generated by the composting process, NH3, H2S, dimethyl disulfide, carbon disulfide, toluene, m,p-xylene, 1,3,5-trimethylbenzene, and naphthalene were found to have highly significant concentrations (p < 0.01) and are the main odor-causing substances. As composting proceeds, the percentage of alkanes and halogenated compounds decreases gradually, whereas the percentage of aromatic compounds increases. The percentage of sulfur-containing compounds exhibited an initial increase followed by a decrease, while the percentage of oxygen-containing compounds first decreased and then increased.
Data availability statement
The original contributions presented in the study are included in the article/Supplementary material, further inquiries can be directed to the corresponding authors.
Ethics statement
Ethical review and approval was not required for the animal study because this study used corn straw and chicken manure obtained from the waste of Changchun Jiaxin farm. Jilin Agricultural University did not require the study to be reviewed or approved by an ethics committee because these items were used for research purposes only and with permission. The study complied with relevant institutional, national, and international guidelines and legislation.
Author contributions
YJ: conceptualization, investigation, formal analysis, and writing—original draft. YY: data curation, methodology, and writing—original draft. HL: investigation. SZ: supervision. XB: visualization. XM: validation. YW: visualization, funding acquisition, and writing—review and editing. QR: funding acquisition and supervision. All authors contributed to the article and approved the submitted version.
Funding
This work was supported by the National Key Research and Development Program of China (2021YFD1500805) and the Natural Science Foundation of Jilin Province (20220101175JC).
Conflict of interest
The authors declare that the research was conducted in the absence of any commercial or financial relationships that could be construed as a potential conflict of interest.
Publisher’s note
All claims expressed in this article are solely those of the authors and do not necessarily represent those of their affiliated organizations, or those of the publisher, the editors and the reviewers. Any product that may be evaluated in this article, or claim that may be made by its manufacturer, is not guaranteed or endorsed by the publisher.
Supplementary material
The Supplementary material for this article can be found online at: https://www.frontiersin.org/articles/10.3389/fevo.2023.1192132/full#supplementary-material
References
Arellano-García, L., Le Borgne, S., and Revah, S. (2018). Simultaneous treatment of dimethyl disulfide and hydrogen sulfide in an alkaline biotrickling filter. Chemosphere 191, 809–816. doi: 10.1016/j.chemosphere.2017.10.096
Aryal, B., Gurung, R., Camargo, A. F., Fongaro, G., Treichel, H., Mainali, B., et al. (2022). Nitrous oxide emission in altered nitrogen cycle and implications for climate change. Environ. Pollut. 314:120272. doi: 10.1016/j.envpol.2022.120272
Atkinson, R., and Arey, J. (2003). Atmospheric degradation of volatile organic compounds. Chem. Rev. 103, 4605–4638. doi: 10.1021/cr0206420
Awasthi, M. K., Duan, Y., Liu, T., Awasthi, S. K., and Zhang, Z. (2020). Relevance of biochar to influence the bacterial succession during pig manure composting. Bioresour. Technol. 304:122962. doi: 10.1016/j.biortech.2020.122962
Boon, A. G. (1995). Septicity in sewers: causes, consequences and containment. Water Sci. Technol. 31, 237–253. doi: 10.2166/wst.1995.0240
Bruno, P., Caselli, M., de Gennaro, G., Solito, M., and Tutino, M. (2007). Monitoring of odor compounds produced by solid waste treatment plants with diffusive samplers. Waste Manag. 27, 539–544. doi: 10.1016/j.wasman.2006.03.006
Cao, T., Zheng, Y., Zhang, Y., Wang, Y., Cong, Q., Wang, Y., et al. (2022). Pilot study on gaseous pollution removal efficiency of acid scrubbing in a broiler house. Agric. Ecosyst. Environ. 335:108021. doi: 10.1016/j.agee.2022.108021
Chen, H., Awasthi, M. K., Liu, T., Zhao, J., Ren, X., Wang, M., et al. (2018a). Influence of clay as additive on greenhouse gases emission and maturity evaluation during chicken manure composting. Bioresour. Technol. 266, 82–88. doi: 10.1016/j.biortech.2018.06.073
Chen, H., Cheng, H., Zhou, F., Chen, K., Qiao, K., Lu, X., et al. (2018b). Catalytic fast pyrolysis of rice straw to aromatic compounds over hierarchical HZSM-5 produced by alkali treatment and metal-modification. J. Anal. Appl. Pyrolysis 131, 76–84. doi: 10.1016/j.jaap.2018.02.009
Chen, Z., Huang, G., Li, Y., Zhang, X., Xiong, Y., Huang, Q., et al. (2022). Effects of the lignite bioorganic fertilizer on greenhouse gas emissions and pathways of nitrogen and carbon cycling in saline-sodic farmlands at Northwest China. J. Clean. Prod. 334:130080. doi: 10.1016/j.jclepro.2021.130080
Chiriac, R., De Araujos Morais, J., Carre, J., Bayard, R., Chovelon, J. M., and Gourdon, R. (2011). Study of the VOC emissions from a municipal solid waste storage pilot-scale cell: comparison with biogases from municipal waste landfill site. Waste Manag. 31, 2294–2301. doi: 10.1016/j.wasman.2011.06.009
De Marco, A., Proietti, C., Anav, A., Ciancarella, L., D'Elia, I., Fares, S., et al. (2019). Impacts of air pollution on human and ecosystem health, and implications for the National Emission Ceilings Directive: insights from Italy. Environ. Int. 125, 320–333. doi: 10.1016/j.envint.2019.01.064
Delgado, S., Alvarez, M., Rodriguez-gomez, L. E., and Aguiar, E. (1999). H2S generation in a reclaimed urban wastewater pipe. Case study: Tenerife (Spain). Water Res. 33, 539–547. doi: 10.1016/j.wasman.2011.06.009
Dhamodharan, K., Varma, V. S., Veluchamy, C., Pugazhendhi, A., and Rajendran, K. (2019). Emission of volatile organic compounds from composting: a review on assessment, treatment and perspectives. Sci. Total Environ. 695:133725. doi: 10.1016/j.scitotenv.2019.133725
Ding, Y., Shi, J. Y., Wu, W. X., Yin, J., and Chen, Y. X. (2007). Trimethylamine (TMA) biofiltration and transformation in biofilters. J. Hazard. Mater. 143, 341–348. doi: 10.1016/j.jhazmat.2006.09.031
Duan, Z., Lu, W., Li, D., and Wang, H. (2014). Temporal variation of trace compound emission on the working surface of a landfill in Beijing, China. Atmos. Environ. 88, 230–238. doi: 10.1016/j.atmosenv.2014.01.051
Duan, Z., Lu, W., Mustafa, M. F., Du, J., and Wen, Y. (2022). Odorous gas emissions from sewage sludge composting windrows affected by the turning operation and associated health risks. Sci. Total Environ. 839:155996. doi: 10.1016/j.scitotenv.2022.155996
Durmusoglu, E., Taspinar, F., and Karademir, A. (2010). Health risk assessment of BTEX emissions in the landfill environment. J. Hazard. Mater. 176, 870–877. doi: 10.1016/j.jhazmat.2009.11.117
Eitzer, B. D. (1995). Emissions of volatile organic chemicals from municipal solid waste composting facilities. Environ. Sci. Technol. 29, 896–902. doi: 10.1021/es00004a009
Fisher, R. M., Le-Minh, N., Alvarez-Gaitan, J. P., Moore, S. J., and Stuetz, R. M. (2018). Emissions of volatile sulfur compounds (VSCs) throughout wastewater biosolids processing. Sci. Total Environ. 616-617, 622–631. doi: 10.1016/j.scitotenv.2017.10.282
Gao, D., Shen, Y., Chen, T., Zheng, G., Liu, H., Chen, J., et al. (2010). Generation and release of odoriferous substances during aerobic fermentation of sludge. China Water Supply Drain. 13, 117–119 (in Chinese). doi: 10.19853/j.zgjsps.1000-4602.2010.13.032
Gay, S. W., Schmidt, D. R., Clanton, C. J., Janni, K. A., Jacobson, L. D., and Weisberg, S. (2003). Odor, total reduced sulfur, and ammonia emissions from animal housing facilities and manure storage units in Minnesota. Appl. Eng. Agric. 19, 347–360. doi: 10.13031/2013.13663
González, D., Colón, J., Sánchez, A., and Gabriel, D. (2019). A systematic study on the VOCs characterization and odour emissions in a full-scale sewage sludge composting plant. J. Hazard. Mater. 373, 733–740. doi: 10.1016/j.jhazmat.2019.03.131
Guan, Y., Liu, X., Zheng, Z., Dai, Y., Du, G., Han, J., et al. (2023). Summer O3 pollution cycle characteristics and VOCs sources in a central city of Beijing-Tianjin-Hebei area, China. Environ. Pollut. 323:121293. doi: 10.1016/j.envpol.2023.121293
Han, Z., Qi, F., Wang, H., Li, R., and Sun, D. (2019). Odor assessment of NH3 and volatile sulfide compounds in a full-scale municipal sludge aerobic composting plant. Bioresour. Technol. 282, 447–455. doi: 10.1016/j.wasman.2018.05.049
Han, Z., Qi, F., Wang, H., Liu, B., Shen, X., Song, C., et al. (2018). Emission characteristics of volatile sulfur compounds (VSCs) from a municipal sewage sludge aerobic composting plant. Waste Manag. 77, 593–602. doi: 10.1016/j.wasman.2018.05.049
Hanajima, D., Kuroda, K., Morishita, K., Fujita, J., Maeda, K., and Morioka, R. (2010). Key odor components responsible for the impact on olfactory sense during swine feces composting. Bioresour. Technol. 101, 2306–2310. doi: 10.1016/j.biortech.2009.11.026
He, C., Cheng, J., Zhang, X., Douthwaite, M., Pattisson, S., and Hao, Z. (2019). Recent advances in the catalytic oxidation of volatile organic compounds: a review based on pollutant sorts and sources. Chem. Rev. 119, 4471–4568. doi: 10.1021/acs.chemrev.8b00408
He, P., Du, W., Xu, X., Zhang, H., Shao, L., and Lü, F. (2020). Effect of biochemical composition on odor emission potential of biowaste during aerobic biodegradation. Sci. Total Environ. 727:138285. doi: 10.1016/j.scitotenv.2020.138285
Higgins, M. J., Chen, Y. C., Yarosz, D. P., Murthy, S. N., Maas, N. A., Glindemann, D., et al. (2006). Cycling of volatile organic sulfur compounds in anaerobically digested biosolids and its implications for odors. Water Environ. Res. 78, 243–252. doi: 10.2175/106143005X90065
Hort, C., Gracy, S., Platel, V., and Moynault, L. (2009). Evaluation of sewage sludge and yard waste compost as a biofilter media for the removal of ammonia and volatile organic sulfur compounds (VOSCs). Chem. Eng. J. 152, 44–53. doi: 10.1016/j.cej.2009.03.026
Huan, C., Fang, J., Tong, X., Zeng, Y., Liu, Y., Jiang, X., et al. (2021). Simultaneous elimination of H2S and NH3 in a biotrickling filter packed with polyhedral spheres and best efficiency in compost deodorization. J. Clean. Prod. 284:124708. doi: 10.1016/j.jclepro.2020.124708
Huang, D., Sun, M., Han, M., Zhang, Z., Miao, Y., Zhang, J., et al. (2020). Volatile organic compounds (VOCs) regulate the spatial distribution of Lepidoptera insects in an orchard ecosystem. Biol. Control 149:104311. doi: 10.1016/j.biocontrol.2020.104311
Jarvis, A., Sundberg, C., Milenkovski, S., Pell, M., Smars, S., Lindgren, P. E., et al. (2009). Activity and composition of ammonia oxidizing bacterial communities and emission dynamics of NH3 and N2O in a compost reactor treating organic household waste. J. Appl. Microbiol. 106, 1502–1511. doi: 10.1111/j.1365-2672.2008.04111.x
Jiang, J., Kang, K., Chen, D., and Liu, N. (2018). Impacts of delayed addition of N-rich and acidic substrates on nitrogen loss and compost quality during pig manure composting. Waste Manag. 72, 161–167. doi: 10.1016/j.wasman.2017.11.025
Komilis, D. P., Ham, R. K., and Park, J. K. (2004). Emission of volatile organic compounds during composting of municipal solid wastes. Water Res. 38, 1707–1714. doi: 10.1016/j.watres.2003.12.039
Koyama, M., Nagao, N., Syukri, F., Rahim, A. A., Kamarudin, M. S., Toda, T., et al. (2018). Effect of temperature on thermophilic composting of aquaculture sludge: NH3 recovery, nitrogen mass balance, and microbial community dynamics. Bioresour. Technol. 265, 207–213. doi: 10.1016/j.biortech.2018.05.109
Kumar, A., Alaimo, C. P., Horowitz, R., Mitloehner, F. M., Kleeman, M. J., and Green, P. G. (2011). Volatile organic compound emissions from green waste composting: characterization and ozone formation. Atmos. Environ. 45, 1841–1848. doi: 10.1016/j.atmosenv.2011.01.014
Li, Y., Liu, T., Song, J., Lv, J., and Jiang, J. (2020). Effects of chemical additives on emissions of ammonia and greenhouse gas during sewage sludge composting. Process Saf. Environ. Prot. 143, 129–137. doi: 10.1016/j.psep.2020.05.056
Li, D., Yuan, J., Ding, J., Wang, H., Shen, Y., and Li, G. (2022). Effects of carbon/nitrogen ratio and aeration rate on the sheep manure composting process and associated gaseous emissions. J. Environ. Manag. 323:116093. doi: 10.1016/j.jenvman.2022.116093
Lin, T., Kuo, S., Hussain, A., Chen, Z., Huang, M., and Lin, C. (2023). Characterization of VOCs during diesel oil composting process. Bioresour. Technol. Rep. 22:101392. doi: 10.1016/j.biteb.2023.101392
Liu, Q., Li, M., Chen, R., Li, Z., Qian, G., An, T., et al. (2009). Biofiltration treatment of odors from municipal solid waste treatment plants. Waste Manag. 29, 2051–2058. doi: 10.1016/j.wasman.2009.02.002
Maulini-Duran, C., Abraham, J., Rodríguez-Pérez, S., Cerda, A., Jiménez-Peñalver, P., Gea, T., et al. (2015). Gaseous emissions during the solid state fermentation of different wastes for enzyme production at pilot scale. Bioresour. Technol. 179, 211–218. doi: 10.1016/j.biortech.2014.12.031
Mellouki, A., Wallington, T. J., and Chen, J. (2015). Atmospheric chemistry of oxygenated volatile organic compounds: impacts on air quality and climate. Chem. Rev. 115, 3984–4014. doi: 10.1021/cr500549n
Moreno, A. I., Arnáiz, N., Font, R., and Carratalá, A. (2014). Chemical characterization of emissions from a municipal solid waste treatment plant. Waste Manag. 34, 2393–2399. doi: 10.1016/j.wasman.2014.07.008
Mustafa, M. F., Liu, Y., Duan, Z., Guo, H., Xu, S., Wang, H., et al. (2017). Volatile compounds emission and health risk assessment during composting of organic fraction of municipal solid waste. J. Hazard. Mater. 327, 35–43. doi: 10.1016/j.jhazmat.2016.11.046
National Bureau of Statistics of China. (2020). China Statistical Yearbook, 2020. Beijing: China Statistics Press.
Nicell, J. A. (2009). Assessment and regulation of odour impacts. Atmos. Environ. 43, 196–206. doi: 10.1016/j.atmosenv.2008.09.033
Nie, E., Zheng, G., Gao, D., Chen, T., Yang, J., Wang, Y., et al. (2019). Emission characteristics of VOCs and potential ozone formation from a full-scale sewage sludge composting plant. Sci. Total Environ. 659, 664–672. doi: 10.1016/j.scitotenv.2018.12.404
Noble, R., Hobbs, P. J., Dobrovin-Pennington, A., Misselbrook, T. H., and Mead, A. (2001). Olfactory response to mushroom composting emissions as a function of chemical concentration. J. Environ. Qual. 30, 760–767. doi: 10.2134/jeq2001.303760x
Ochoa-Hueso, R., Munzi, S., Alonso, R., Arróniz-Crespo, M., Avila, A., Bermejo, V., et al. (2017). Ecological impacts of atmospheric pollution and interactions with climate change in terrestrial ecosystems of the Mediterranean Basin: current research and future directions. Environ. Pollut. 227, 194–206. doi: 10.1016/j.envpol.2017.04.062
Rincón, C. A., De Guardia, A., Couvert, A., Le Roux, S., Soutrel, I., Daumoin, M., et al. (2019). Chemical and odor characterization of gas emissions released during composting of solid wastes and digestates. J. Environ. Manag. 233, 39–53. doi: 10.1016/j.jenvman.2018.12.009
Sánchez-Monedero, M. A., Fernández-Hernández, A., Higashikawa, F. S., and Cayuela, M. L. (2018). Relationships between emitted volatile organic compounds and their concentration in the pile during municipal solid waste composting. Waste Manag. 79, 179–187. doi: 10.1016/j.wasman.2018.07.041
Sánchez-Monedero, M. A., Sánchez-García, M., Alburquerque, J. A., and Cayuela, M. L. (2019). Biochar reduces volatile organic compounds generated during chicken manure composting. Bioresour. Technol. 288:121584. doi: 10.1016/j.biortech.2019.121584
Schiavon, M., Martini, L. M., Corrà, C., Scapinello, M., Coller, G., Tosi, P., et al. (2017). Characterisation of volatile organic compounds (VOCs) released by the composting of different waste matrices. Environ. Pollut. 231, 845–853. doi: 10.1016/j.envpol.2017.08.096
Shao, L., Zhang, C., Wu, D., Lu, F., Li, T., and He, P. (2014). Effects of bulking agent addition on odorous compounds emissions during composting of OFMSW. Waste Manag. 34, 1381–1390. doi: 10.1016/j.wasman.2014.04.016
Shen, Y., Chen, T.-B., Gao, D., Zheng, G., Liu, H., and Yang, Q. (2012). Online monitoring of volatile organic compound production and emission during sewage sludge composting. Bioresour. Technol. 123, 463–470. doi: 10.1016/j.biortech.2012.05.006
Shen, Y., Zhang, P., Zhao, L., Meng, H., and Cheng, H. (2016). Analysis of volatile organic compounds components and determination of odor-causing factors during aerobic fermentation of pig manure. J. Agricult. Eng. 04, 205–210. doi: 10.11975/j.issn.1002-6819.2016.04.029 (in Chinese)
Sun, J.-L., Zeng, H., and Ni, H.-G. (2013). Halogenated polycyclic aromatic hydrocarbons in the environment. Chemosphere 90, 1751–1759. doi: 10.1016/j.chemosphere.2012.10.094
Tan, H., Zhao, Y., Ling, Y., Wang, Y., and Wang, X. (2017). Emission characteristics and variation of volatile odorous compounds in the initial decomposition stage of municipal solid waste. Waste Manag. 68, 677–687. doi: 10.1016/j.wasman.2017.07.015
Tassi, F., Montegrossi, G., Vaselli, O., Liccioli, C., Moretti, S., and Nisi, B. (2009). Degradation of C2–C15 volatile organic compounds in a landfill cover soil. Sci. Total Environ. 407, 4513–4525. doi: 10.1016/j.scitotenv.2009.04.022
Tsai, C. J., Chen, M. L., Ye, A. D., Chou, M. S., Shen, S. H., and Mao, I. F. (2008). The relationship of odor concentration and the critical components emitted from food waste composting plants. Atmos. Environ. 42, 8246–8251. doi: 10.1016/j.atmosenv.2008.07.055
Turan, N. G., Akdemir, A., and Ergun, O. N. (2007). Emission of volatile organic compounds during composting of poultry litter. Water Air Soil Pollut. 184, 177–182. doi: 10.1007/s11270-007-9406-0
Van Durme, G. P., McNamara, B. F., and McGinley, C. M. (1992). Bench-scale removal of odor and volatile organic compounds at a composting facility. Water Environ. Res. 64, 19–27. doi: 10.2175/WER.64.1.4
Wang, Y., Feng, L., Zhao, X., Ma, X., Yang, J., Liu, H., et al. (2013). Characteristics of volatile compounds removal in biogas slurry of pig manure by ozone oxidation and organic solvents extraction. J. Environ. Sci. 25, 1800–1807. doi: 10.1016/S1001-0742(12)60240-X
Wang, K., Mao, H., Wang, Z., and Tian, Y. (2018). Succession of organics metabolic function of bacterial community in swine manure composting. J. Hazard. Mater. 360, 471–480. doi: 10.1016/j.jhazmat.2018.08.032
Wang, Y., Ren, X., Ji, D., Zhang, J., Sun, J., and Wu, F. (2012). Characterization of volatile organic compounds in the urban area of Beijing from 2000 to 2007. J. Environ. Sci. 24, 95–101. doi: 10.1016/S1001-0742(11)60732-8
Wu, C., Liu, J., Zhao, P., Li, W., Yan, L., Piringer, M., et al. (2017). Evaluation of the chemical composition and correlation between the calculated and measured odour concentration of odorous gases from a landfill in Beijing, China. Atmos. Environ. 164, 337–347. doi: 10.1016/j.atmosenv.2017.06.010
Yang, F., Li, Y., Han, Y., Qian, W., Li, G., and Luo, W. (2019). Performance of mature compost to control gaseous emissions in kitchen waste composting. Sci. Total Environ. 657, 262–269. doi: 10.1016/j.scitotenv.2018.12.030
Yang, C., Miao, G., Pi, Y., Xia, Q., Wu, J., Li, Z., et al. (2019). Abatement of various types of VOCs by adsorption/catalytic oxidation: a review. Chem. Eng. J. 370, 1128–1153. doi: 10.1016/j.cej.2019.03.232
Yao, X., Zhou, H., Meng, H., Ding, J., Shen, Y., Cheng, H., et al. (2021). Amino acid profile characterization during the co-composting of a livestock manure and maize straw mixture. J. Clean. Prod. 278:123494. doi: 10.1016/j.jclepro.2020.123494
Zhang, L., De Schryver, P., De Gusseme, B., De Muynck, W., Boon, N., and Verstraete, W. (2008). Chemical and biological technologies for hydrogen sulfide emission control in sewer systems: a review. Water Res. 42, 1–12. doi: 10.1016/j.watres.2007.07.013
Zhang, X., Li, S., Cheng, W., Zhao, Y., Cui, H., Xie, X., et al. (2021). Oxytetracycline stress reconstruct the core microbial community related to nitrogen transformation during composting. Bioresour. Technol. 319:124142. doi: 10.1016/j.biortech.2020.124142
Zhang, Z., Liu, D., Qiao, Y., Li, S., Chen, Y., and Hu, C. (2021). Mitigation of carbon and nitrogen losses during pig manure composting: a meta-analysis. Sci. Total Environ. 783:147103. doi: 10.1016/j.scitotenv.2021.147103
Zhang, H., Zou, K., Yang, J., Li, G., Yang, Q., and Zhang, F. (2012). Analysis of malodorous substances in food waste composting process. Environ. Sci. 08, 2563–2568. doi: 10.13227/j.hjkx.2012.08.032 (in Chinese)
Zhao, S., Schmidt, S., Gao, H., Li, T., Chen, X., Hou, Y., et al. (2022). A precision compost strategy aligning composts and application methods with target crops and growth environments can increase global food production. Nature Food 3, 741–752. doi: 10.1038/s43016-022-00584-x
Zhao, Y., Zhou, Q., Hidetoshi, K., and Luo, L. (2021). Nitrogen flow characteristics of solid waste in China. Ecotoxicol. Environ. Saf. 208:111596. doi: 10.1016/j.ecoenv.2020.111596
Zheng, G., Cheng, Y., Zhu, Y., Yang, J., Wang, L., and Chen, T. (2022). Correlation of microbial dynamics to odor production and emission in full-scale sewage sludge composting. Bioresour. Technol. 360:127597. doi: 10.1016/j.biortech.2022.127597
Zheng, T., Li, L., Chai, F., and Wang, Y. (2021). Factors impacting the performance and microbial populations of three biofilters for co-treatment of H2S and NH3 in a domestic waste landfill site. Process Saf. Environ. Prot. 149, 410–421. doi: 10.1016/j.psep.2020.11.009
Keywords: composting, volatile inorganic compounds, volatile organic compounds, emission characteristics, transformation pathway
Citation: Jiang Y, Yao Y, Liu H, Zhang S, Bai X, Ma X, Wang Y and Ren Q (2023) Volatile organic compounds conversion pathways and odor gas emission characteristics in chicken manure composting process. Front. Ecol. Evol. 11:1192132. doi: 10.3389/fevo.2023.1192132
Edited by:
Junjie Lin, Chongqing Three Gorges University, ChinaReviewed by:
Sai Xu, Nanjing University of Science and Technology, ChinaLei Zhang, Chongqing Three Gorges University, China
Copyright © 2023 Jiang, Yao, Liu, Zhang, Bai, Ma, Wang and Ren. This is an open-access article distributed under the terms of the Creative Commons Attribution License (CC BY). The use, distribution or reproduction in other forums is permitted, provided the original author(s) and the copyright owner(s) are credited and that the original publication in this journal is cited, in accordance with accepted academic practice. No use, distribution or reproduction is permitted which does not comply with these terms.
*Correspondence: Yujun Wang, eXVqdW53QGpsYXUuZWR1LmNu; Qibiao Ren, MjQ1NjcxOTY2OUBxcS5jb20=
†These authors have contributed equally to this work and share first authorship
‡These authors have contributed equally to this work and share last authorship