- 1College of Civil Engineering, Fuzhou University, Fuzhou, China
- 2School of Public Administration, Zhongnan University of Economics and Law, Wuhan, China
- 3School of Construction and Ecology, Shantou Polytechnic, Shantou, China
Biomineralization technology offers an eco-friendly and efficient method for stabilizing heavy metals (HMs) in ecosystem. This technology comprises two primary methods: microbially induced carbonate precipitation (MICP) and enzyme-induced carbonate precipitation (EICP). Biomineralization provides a superior alternative to stabilize heavy metals due to its low energy consumption, reduced carbon dioxide emissions, and superior biocompatibility. In the process of biomineralization, heavy metal ions precipitate and co-precipitate with calcium carbonate, forming a solidified and stabilized product. Despite its many advantages, little attention has been paid to the impact of biomineralization on mitigation of ammonia nitrogen of bio-treated polluted water and the strength of contaminated soil, limiting its further applications in ecological environment restoration. This paper summarizes recent advancements in biomineralization for solidifying and stabilizing (S/S) heavy metals in contaminated water and soil. Key factors inhibiting this method’s application include the concentration and combinations of heavy metal ions, the concentration of ammonia nitrogen in polluted water, and the properties of contaminated soil. Finally, this paper offers recommendations on the optimization of further research and experimental design of biomineralization on S/S polluted water and contaminated soil.
Introduction
Heavy metal pollution poses a risk to the global environment and human health, which has received attention in recent years. The S/S method based on cement has been used to immobilize HMs contaminated soil due to its high efficiency. However, the use of cement has raised a series of environmental concerns, especially regarding high carbon emissions in its production (Morales et al., 2019). More than 4.2Gt/year of greenhouse gases from ordinary Portland cement (OPC) production are emitted into the atmosphere, which accounts for around 12% of total manmade carbon dioxide production (Hassan et al., 2020). Electrochemistry is another promising method to remove HMs in contaminated soil (Xu et al., 2019), but the capacity of power supply restricts the wide spread of this method. Besides, the restoration methods such as chemical precipitation (Benalia et al., 2022), ion exchange and membrane filtration (Fu et al., 2022) were also adopted for immobilizing the HMs in polluted water. However, they caused serious environmental problems due to abuse and excessive use of chemical reagents (Fu et al., 2012).
Biomineralization technology has experienced promising developments in soil improvement and HMs immobilization, which has been characterized by non-carbon and high efficiency. Two main methods have been widely reported in recent years: microbially induced carbonate precipitation (MICP) and enzyme-induced carbonate precipitation (EICP). The difference between these two methods lies solely in the source of urease (i.e., the urease from bacteria or plants). Both methods make use of urease to hydrolyze the urea to generate the CO32− and NH4+. The resulting increase in pH for NH3 hydrolysis facilitates calcium carbonate precipitation. The process can be expressed as follows:
Besides, the biomineralization technology has been widely applied in soil improvement (Li et al., 2022), ground anti-liquefaction, cracks anti-seepage (Chu et al., 2014), fugitive dust control (Meng et al., 2021), coastal erosion control (Wang Y. -J. et al., 2022) and heavy metal stabilization (Liu J. et al., 2021), etc. The solutions of biomineralization can be injected at lower pressure, permeating in soil matrix (Morales et al., 2019). Additionally, the calcium carbonate (CaCO3) generated in biomineralization process has fine biocompatibility (Jiang et al., 2020), compared with traditional cement-based materials. In comparison to traditional methods for removing HMs in polluted water, such as chemical precipitation or electrochemical methods (Xu et al., 2019), the biomineralization method requires fewer types of chemical reagents and is virtually nontoxic to the ecosystem. However, the ammonia nitrogen and high pH values caused by the biomineralization process have not been thoroughly investigated. Additionally, the mechanical properties of biomineralization-S/S contaminated soil have also not been comprehensively studied. These gaps hinder the advancement of efficient reutilization of wastewater and contaminated soils. This paper aims to summarize the potential limitations of biomineralization-S/S contaminants, in the aspects of HMs stabilization in polluted water and the S/S of contaminated soil. Furthermore, we also recommend some methodologies aiming to comprehensively evaluate the durability and long-term stability of biomineralization-S/S heavy metal contaminated pollutants.
Application and challenges of biomineralization technology in removing HMs from polluted water
Biomineralization has demonstrated a remarkable effect on stabilizing HMs (Kang et al., 2016; Mwandira et al., 2017; Chen et al., 2021). However, the toxicity of heavy metal ions to bacteria and urease presents a significant challenge. Thus, the tolerance of HMs and the stabilization of solid precipitation are critical factors in further promoting the application of biomineralization-S/S polluted water. It found that lead ions (Pb2+) were deposited first in the form of (PbCl)2CO3 and then PbCO3, resulting in the formation of a multilayer carbonate structure in the presence of low urea concentrations (Jiang et al., 2019). Xue et al. (2022) introduced the MICP to stabilize Pb2+ in aqueous solution, the results showed that CO32− was sufficient to promote the Pb2+ precipitation in higher concentration of urea. Song et al. (2022) proposed a method that the pH of wastewater treated by MICP could be adjusted to neuter by phosphoric acid, and then the treated wastewater could be used for irrigation. Notably, most reporters focused on single HMs (e.g., Pb, Cd, Cr, and Cu) stabilized by biomineralization technology. Only a limited number of studies have investigated the effectiveness of biomineralization-S/S multiple HMs. Kang et al. (2016) used MICP to immobilize Pb, Cd, and Cu simultaneously and indicated that the removal rate of Pb and Cd reached 85% but that of Cu was only 5%. Qiao et al. (2021) proved that the MICP method immobilized multiple HMs through biological precipitation and co-precipitation, and the toxicity of HMs to bacteria was in the order of Cd > Zn > Ni > Cu. The research of Han et al. (2023) showed that phytase hydrolyzed magnesium ascorbyl phosphate (MAP) to produce the ascorbic acid (AA) and MgHPO4·3H2O precipitation. AA reduced Cr(VI) to Cr(III), which subsequently formed chromium hydroxide and chromium phosphate precipitates.
In summary, the immobilization of HMs through biomineralization can be achieved through two main processes: one is directly to form heavy metal carbonate (e.g., PbCO3, CrCO3, etc.) and/or the HMs replace the Ca2+ and then co-precipitate with calcium carbonate or hydroxyapatite (Achal et al., 2012; Zeng et al., 2021), as shown in Figure 1. Another is that HMs are absorbed by calcite and/ or hydroxyapatite. Besides, for the mineralization reaction involving bacteria, the cell wall and extracellular polymer of bacteria can adsorb, complex, chelate, and crystallize with heavy metal ions (Zhang et al., 2019).
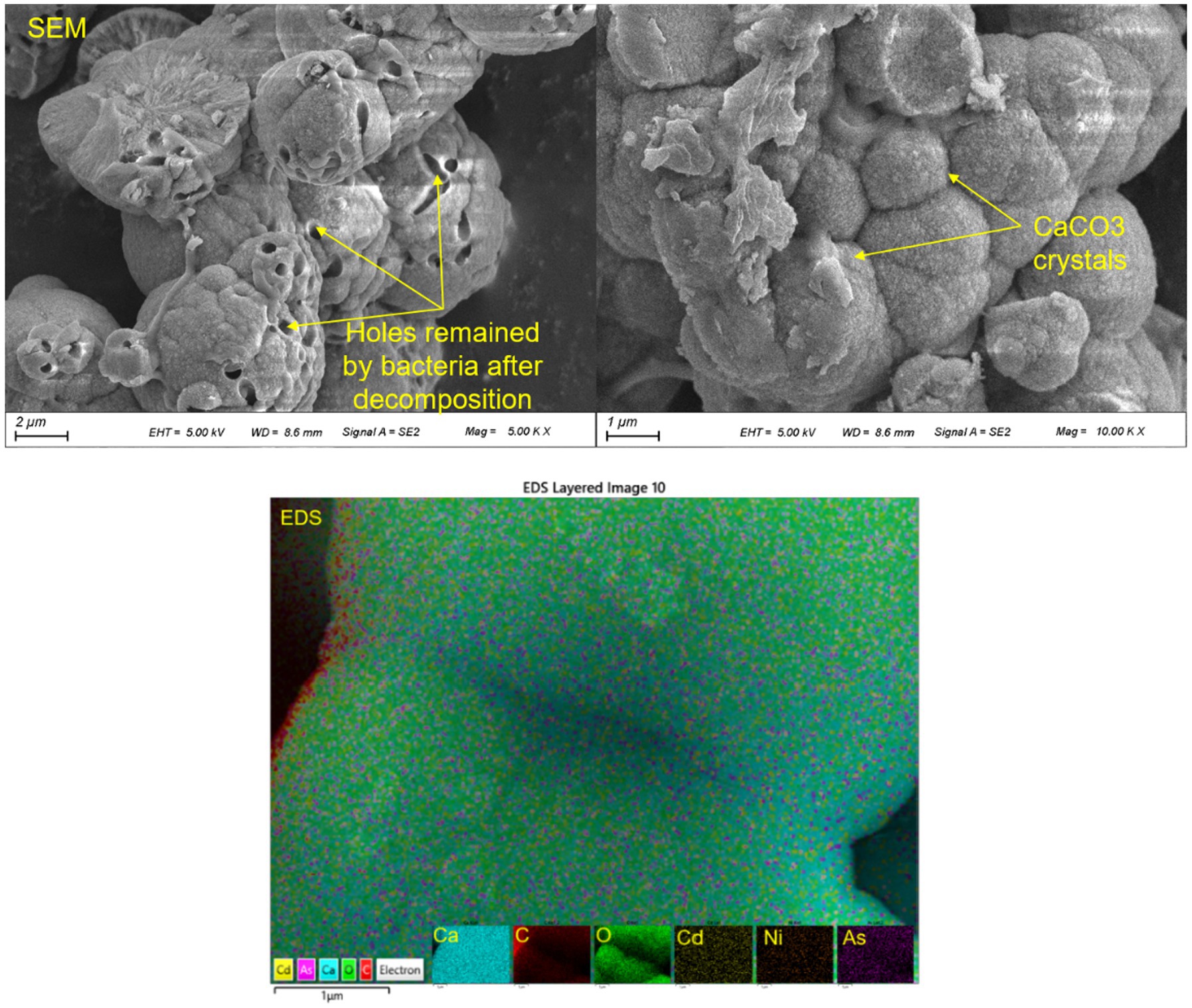
Figure 1. Precipitation/ co-precipitation of HMs (Cd, Ni, and As) in polluted water depicted by scanning electron microscope (SEM) and energy dispersive spectrometer (EDS).
Most of the researches on the MICP S/S HMs aim to investigate and identify microbial strains with specific functionalities. While the risk of introducing foreign strains into the ecological environment can be avoided, selecting and cultivating bacterial strains remains heavy work, and these strains may also experience reduced tolerance to HMs. The research of Wang Z. et al. (2022) provides a new perspective for revealing the mechanism of MICP stabilized HMs in polluted water via microfluidic experiments. Moreover, further investigation is required to determine the removal efficiency and long-term stability of biomineralization technology for immobilizing multiple HMs.
Limitations of the biomineralization-S/S HMs contaminated soil
The restoration of contaminated soil by biomineralization-S/S requires the enhancement of mechanical properties while immobilizing the HMs, which facilitates the secondary utilization of the contaminated soil and improves resource utilization efficiency. Han et al. (2022) reported that enzyme-induced phosphate precipitation (EIPP) could efficiently immobilize Pb and Zinc (Zn) in tailing sand, and it also indicated that the product of EIPP was MgHPO4(H2O)3 and Pb9(PO4)6. The tailing sand particles were bonded and the pores were filled by MgHPO4(H2O)3, enhancing the strength of EIPP-treated tailing sand, and the result was consistent with the research of Han et al. (2023). Besides, the decrease in porosity due to the filling effect of carbonate (Makinda et al., 2021), reduced hydraulic conductivity. This phenomenon can be explained by the reduction in pore size and increased tortuosity, leading to a decrease in the effective pore size. But Chen et al. (2021) found that the aggregate structure and porosity of the soil increased through MICP S/S contaminated soil improving the mobility of air and water within the soil, which is attributed to the addition of organic matter and microorganisms. Moreover, the long-term stability of biomineralization S/S heavy metal contaminated soil was significantly superior to chemical treatment methods (Liu P. et al., 2021).
The mechanical properties of biomineralization-S/S heavy metal contaminated soil remain largely unexplored. The effect of soil properties on the biomineralization-S/S HMs contaminated soil has not been fully considered. For instance, the silt and granite residual soil composed of numerous clay minerals can absorb and/ or desorb the heavy metal ions (Li et al., 2023), thereby affecting the process of biomineralization-S/S HMs. Furthermore, the generation of a large volume of dredged sludge, with high water content, minuscule pores and low permeability, from urban rivers and lakes has led to significant ecological problems (Mymrin et al., 2019). Biomineralization technology emerged as a promising and feasible method for solidification and stabilization of sludge or clay soils (Gowthaman et al., 2022). However, the poor strength of dredged sludge poses a challenge to the survival of bacteria. In this regard, EICP has several advantages over MICP in S/S contaminated sludge due to the smaller size of urease (~12 nm; Blakeley and Zerner, 1983) compared to that of bacteria (0.5 ~ 3 μm; Tsesarsky et al., 2016).
Conclusions and future perspective
Although the study on the biomineralization-S/S heavy metal contaminates has received much attention, there is still a lack of a deeper understanding of the mechanisms involved in the simultaneous immobilization of multiple HMs, the variation of pollutant concentration in water treated by biomineralization and the mechanical properties enhancement of treated contaminated soil. Limitations related to microbial strains, pollutant concentrations, and contaminated water/soil properties must also be considered. It should be paid attention to the interaction among microorganism/urease, heavy metal ions, mineral products, and water/soil particles. Additionally, more effective methods for removing the ammonia nitrogen and high pH produced by biomineralization in polluted water is urgent to be explored. Besides, the experiments of biomineralization-S/S contaminated soil with varying engineering properties should be carried out to understand the variation patterns of strength of biomineralization-treated contaminated soil. Long-term stability and durability of biomineralization-S/S treated contaminates should also be investigated by analyzing variations in fixation rate of HMs and mechanical properties of contaminated soil. By obtaining the basic theory of biomineralization-S/S heavy metal contaminates, it will provide a new option for the remediation and engineering reuse of wastewater and contaminated soil, which can achieve significant economic and ecological benefits.
Data availability statement
The raw data supporting the conclusions of this article will be made available by the authors, without undue reservation.
Author contributions
SL: formal analysis, methodology, writing – original draft, and writing – review and editing. XW: investigation, formal analysis, and writing – review and editing. JX: supervision and writing – review and editing. All authors contributed to the article and approved the submitted version.
Funding
This work was financially supported by the Project of Young Innovative Talents for General Colleges in Guangdong Province (2022KQNCX2372022) and the Scientific Research Project in Shantou Polytechnic (SZK2023Z01).
Conflict of interest
The authors declare that the research was conducted in the absence of any commercial or financial relationships that could be construed as a potential conflict of interest.
Publisher’s note
All claims expressed in this article are solely those of the authors and do not necessarily represent those of their affiliated organizations, or those of the publisher, the editors and the reviewers. Any product that may be evaluated in this article, or claim that may be made by its manufacturer, is not guaranteed or endorsed by the publisher.
References
Achal, V., Pan, X., Fu, Q., and Zhang, D. (2012). Biomineralization based remediation of as (III) contaminated soil by Sporosarcina ginsengisoli. J. Hazard. Mater. 201–202, 178–184. doi: 10.1016/j.jhazmat.2011.11.067
Benalia, M. C., Youcef, L., Bouaziz, M. G., Achour, S., and Menasra, H. (2022). Removal of heavy metals from industrial wastewater by chemical precipitation: mechanisms and sludge characterization. Arab. J. Sci. Eng. 47, 5587–5599. doi: 10.1007/s13369-021-05525-7
Blakeley, R. L., and Zerner, B. (1983). Jack bean urease: the first nickel enzyme. Inorg. Chim. Acta 79:11. doi: 10.1016/S0020-1693(00)95029-1
Chen, M., Li, Y., Jiang, X., Zhao, D., Liu, X., Zhou, J., et al. (2021). Study on soil physical structure after the bioremediation of Pb pollution using microbial-induced carbonate precipitation methodology. J. Hazard. Mater. 411:125103. doi: 10.1016/j.jhazmat.2021.125103
Chu, J., Ivanov, V., Naeimi, M., Stabnikov, V., and Liu, H.-L. (2014). Optimization of calcium-based bioclogging and biocementation of sand. Acta Geotech. 9, 277–285. doi: 10.1007/s11440-013-0278-8
Fu, Z.-J., Jiang, S.-K., Chao, X.-Y., Zhang, C.-X., Shi, Q., Wang, Z.-Y., et al. (2022). Removing miscellaneous heavy metals by all-in-one ion exchange-nanofiltration membrane. Water Res. 222:118888. doi: 10.1016/j.watres.2022.118888
Fu, F., Xie, L., Tang, B., Wang, Q., and Jiang, S. (2012). Application of a novel strategy – advanced Fenton-chemical precipitation to the treatment of strong stability chelated heavy metal containing wastewater. Chem. Eng. J. 189–190, 283–287. doi: 10.1016/j.cej.2012.02.073
Gowthaman, S., Chen, M., Nakashima, K., Komatsu, S., and Kawasaki, S. (2022). “Biocementation technology for stabilization/solidification of organic peat” in Low Carbon Stabilization and Solidification of Hazardous Wastes (Amsterdam, The Netherlands: Elsevier), 49–64.
Han, L., Li, J., Fei, X., Wang, M., Liu, S., Zhang, X., et al. (2023). Stabilization and strengthening of chromium (VI)-contaminated soil via magnesium ascorbyl phosphate (MAP) and phytase addition. J. Hazard. Mater. 448:130860. doi: 10.1016/j.jhazmat.2023.130860
Han, L.-J., Li, J.-S., Xue, Q., Guo, M.-Z., Wang, P., and Poon, C. S. (2022). Enzymatically induced phosphate precipitation (EIPP) for stabilization/solidification (S/S) treatment of heavy metal tailings. Constr. Build. Mater. 314:125577. doi: 10.1016/j.conbuildmat.2021.125577
Hassan, A., Arif, M., and Shariq, M. (2020). A review of properties and behaviour of reinforced geopolymer concrete structural elements-a clean technology option for sustainable development. J. Clean. Prod. 245:118762. doi: 10.1016/j.jclepro.2019.118762
Jiang, N.-J., Liu, R., Du, Y.-J., and Bi, Y.-Z. (2019). Microbial induced carbonate precipitation for immobilizing Pb contaminants: toxic effects on bacterial activity and immobilization efficiency. Sci. Total Environ. 672, 722–731. doi: 10.1016/j.scitotenv.2019.03.294
Jiang, N.-J., Tang, C.-S., Hata, T., Courcelles, B., Dawoud, O., and Singh, D. N. (2020). Bio-mediated soil improvement: the way forward. Soil Use Manag. 36, 185–188. doi: 10.1111/sum.12571
Kang, C.-H., Kwon, Y.-J., and So, J.-S. (2016). Bioremediation of heavy metals by using bacterial mixtures. Ecol. Eng. 89, 64–69. doi: 10.1016/j.ecoleng.2016.01.023
Li, S., Liu, D., Garg, A., Lin, P., and Huang, M. (2022). Mechanical properties of cement soil improved using microbial-induced calcite precipitation. Arab. J. Geosci. 15:1053. doi: 10.1007/s12517-022-09752-6
Li, H., Zhang, J., Zhang, Y., Huang, H., Ou, H., and Zhang, Y. (2023). In-situ adsorption-conversion recovery of heavy metal cadmium by natural clay mineral for multi-functional photocatalysis. Sep. Purif. Technol. 319:124058. doi: 10.1016/j.seppur.2023.124058
Liu, J., Ali, A., Su, J., Wu, Z., Zhang, R., and Xiong, R. (2021). Simultaneous removal of calcium, fluoride, nickel, and nitrate using microbial induced calcium precipitation in a biological immobilization reactor. J. Hazard. Mater. 416:125776. doi: 10.1016/j.jhazmat.2021.125776
Liu, P., Zhang, Y., Tang, Q., and Shi, S. (2021). Bioremediation of metal-contaminated soils by microbially-induced carbonate precipitation and its effects on ecotoxicity and long-term stability. Biochem. Eng. J. 166:107856. doi: 10.1016/j.bej.2020.107856
Makinda, J., Kassim, K. A., Ahmad, K., Muhammed, A. S., and Zango, M. U. (2021). “Hydraulic conductivity and calcium carbonate content of biocemented heavy-metal contaminated mine waste soil” in E3S Web of Conferences, vol. 331 (Padang, Indonesia: EDP Sciences), 03001.
Meng, H., Gao, Y., He, J., Qi, Y., and Hang, L. (2021). Microbially induced carbonate precipitation for wind erosion control of desert soil: field-scale tests. Geoderma 383:114723. doi: 10.1016/j.geoderma.2020.114723
Morales, L., Garzón, E., Romero, E., and Sánchez-Soto, P. J. (2019). Microbiological induced carbonate (CaCO3) precipitation using clay phyllites to replace chemical stabilizers (cement or lime). Appl. Clay Sci. 174, 15–28. doi: 10.1016/j.clay.2019.03.018
Mwandira, W., Nakashima, K., and Kawasaki, S. (2017). Bioremediation of lead-contaminated mine waste by Pararhodobacter sp. based on the microbially induced calcium carbonate precipitation technique and its effects on strength of coarse and fine grained sand. Ecol. Eng. 109, 57–64. doi: 10.1016/j.ecoleng.2017.09.011
Mymrin, V., Pan, R. C., Alekseev, K., Avanci, M. A., Stella, J. C., Scremim, C. B., et al. (2019). Overburden soil and marine dredging sludge utilization for production of new composites as highly efficient environmental management. J. Environ. Manag. 236, 206–213. doi: 10.1016/j.jenvman.2019.01.065
Qiao, S., Zeng, G., Wang, X., Dai, C., Sheng, M., Chen, Q., et al. (2021). Multiple heavy metals immobilization based on microbially induced carbonate precipitation by ureolytic bacteria and the precipitation patterns exploration. Chemosphere 274:129661. doi: 10.1016/j.chemosphere.2021.129661
Song, H., Kumar, A., Ding, Y., Wang, J., and Zhang, Y. (2022). Removal of Cd2+ from wastewater by microorganism induced carbonate precipitation (MICP): an economic bioremediation approach. Sep. Purif. Technol. 297:121540. doi: 10.1016/j.seppur.2022.121540
Tsesarsky, M., Gat, D., and Ronen, Z. (2016). Biological aspects of microbial-induced calcite precipitation. Environ. Geotech. 5, 69–78. doi: 10.1680/jenge.15.00070
Wang, Y.-J., Jiang, N.-J., Han, X.-L., Liu, K., and Du, Y.-J. (2022). Biochemical, strength and erosional characteristics of coral sand treated by bio-stimulated microbial induced calcite precipitation. Acta Geotech. 17, 4217–4229. doi: 10.1007/s11440-022-01491-y
Wang, Z., Yang, Z., Fagerlund, F., Zhong, H., Hu, R., Niemi, A., et al. (2022). Pore-scale mechanisms of solid phase emergence during DNAPL remediation by chemical oxidation. Environ. Sci. Technol. 56, 11343–11353. doi: 10.1021/acs.est.2c01311
Xu, J., Liu, C., Hsu, P.-C., Zhao, J., Wu, T., Tang, J., et al. (2019). Remediation of heavy metal contaminated soil by asymmetrical alternating current electrochemistry. Nat. Commun. 10:2440. doi: 10.1038/s41467-019-10472-x
Xue, Z.-F., Cheng, W.-C., Wang, L., and Hu, W. (2022). Effects of bacterial inoculation and calcium source on microbial-induced carbonate precipitation for lead remediation. J. Hazard. Mater. 426:128090. doi: 10.1016/j.jhazmat.2021.128090
Zeng, G., Qiao, S., Wang, X., Sheng, M., Wei, M., Chen, Q., et al. (2021). Immobilization of cadmium by Burkholderia sp. QY14 through modified microbially induced phosphate precipitation. J. Hazard. Mater. 412:125156. doi: 10.1016/j.jhazmat.2021.125156
Keywords: heavy metal, biomineralization, polluted water, contaminated soil, precipitation/ coprecipitation
Citation: Li S, Wu X and Xie J (2023) Biomineralization technology for solidification/stabilization of heavy metals in ecosystem: status and perspective. Front. Ecol. Evol. 11:1189356. doi: 10.3389/fevo.2023.1189356
Edited by:
Jian Luo, Inner Mongolia University, ChinaCopyright © 2023 Li, Wu and Xie. This is an open-access article distributed under the terms of the Creative Commons Attribution License (CC BY). The use, distribution or reproduction in other forums is permitted, provided the original author(s) and the copyright owner(s) are credited and that the original publication in this journal is cited, in accordance with accepted academic practice. No use, distribution or reproduction is permitted which does not comply with these terms.
*Correspondence: Jiamin Xie, jmxie@stpt.edu.cn