- 1Kansas Biological Survey and Center for Ecological Research, and Department of Ecology and Evolutionary Biology, Lawrence, KS, United States
- 2Riverine Landscapes Research Laboratory, University of New England, Armidale, NSW, Australia
- 3Large River Studies Center, Biology Department, Winona State University, Winona, MN, United States
- 4Department of Community and Ecosystem Ecology, Leibniz Institute of Freshwater Ecology and Inland Fisheries, Berlin, Germany
Opportunities to understand and protect natural aquatic diversity in both relatively pristine and managed rivers can be enhanced with a comprehensive, system-wide understanding of a river’s hydrogeomorpholgy and its effects on ecological structure and functioning from the river’s headwaters to its terminus in an ocean, lake, or natural endorheic basin. While a moderate number of macrosystem ecology studies have been undertaken recently in headwaters, comparable ecological approaches to studying whole rivers or at least their larger components from upstream to downstream are relatively rare. This is partially correlated with the paucity of applicable river ecosystem models developed over the last half century which could otherwise provide diverse, testable tenets (hypotheses). This manuscript focuses on a 15+ year updated, system-wide analysis of the applicability of the 17 tenets included in our previously published, lotic model - the Riverine Ecosystem Synthesis, or RES. We also propose here four new tenets and analyze the system-wide applicability of the revised RES. Those new tenets hypothesize that: (H-18) “The range and degree of impacts of a Functional Process Zone on biodiversity and ecological processes differ among several factors, including types of FPZs, total river area covered, and dependent variables examined, even in the same river network position”; (H-19) “The degree of ecological differences among types of FPZs vary seasonally with the process being examined while also differing among types of life history characteristics - especially when contrasting responses among seasonal periods of either maximum or minimum growth and reproduction”; (H-20) “The relative importance of in-stream versus watershed drivers of ecological processes in streams can vary within macrosystems and among ecoregions and partially depends on elevation, terrestrial characteristics (natural or human modified), and FPZ type and extent”; and (H-21) “The provision of ecosystem services varies significantly with FPZ type, river size, and location vis-à-vis human populations”. Where appropriate, we also evaluate aspects of several other models published by colleagues that pertain to river ecology.
Introduction
Rivers and their watersheds span some of the largest inland macrosystems on earth (Thorp, 2014; Thorp et al., 2021), yet the environmental knowledge of how they function through space and time often seems to lag behind that are available for lakes, wetlands, and many terrestrial systems. Causes for this relative scarcity of information may include the following randomly listed limitations to some degree.
● The scientific challenges and dangers to humans associated with conducting aquatic research tend to rise with increases in the size of the river site being investigated.
● Knowledge of how lotic systems function can be limited by seasonal sampling constraints of ambient temperature or precipitation related to human comfort or safety or can be diminished by sampling only in periods of maximum secondary production and species diversity.
● Based on a general survey of the scientific literature over the last few decades, it is apparent that fewer scientists investigate medium to large rivers compared to headwater studies, and much of the larger-system research is focused on practical rather than conceptual questions.
● Research costs can be prohibitive in large aquatic systems because of frequent needs for larger boats and special sampling equipment.
Because the preponderance of descriptive and conceptual lotic studies is typically limited to headwater systems, the ecological knowledge of how whole riverine macrosystems function is likewise constrained or even distorted because of relative differences in the physicochemical habitat and community characteristics. Consequently, a goal of the present treatise is to evaluate models of whole riverine landscapes by describing critical environmental elements that change from upstream to downstream and from the main channel to the riverscape and floodscape. We especially focus here on revisions of, and ecological extensions to the Riverine Ecosystem Synthesis (or RES: Thorp et al., 2006; Thorp et al., 2008), while also integrating some theories proposed by other scientists. We include a relatively short analysis of some of the original model tenets (≈ hypotheses) from our 2006 journal article and our 2008 book along with a presentation of four new ecologically oriented tenets. The task of developing new tenets to supplement the 14-17 previously published has benefitted from studies of the RES in different biomes on at least five continents and discussion of the RES in various college textbooks and journal articles worldwide. Our coverage includes geographical (longitudinal and lateral) and seasonal changes as they affect diversity, density, and ecological processes, but it does not explicitly include endorheic (terminal basin) rivers nor do we have the journal space to focus on many other important aspects of river science, including the nature and distribution among rivers of important hydrogeomorphic zones that form what we have called ecological “Functional Process Zones” (= FPZs).
Macrosystem models need to recognize longitudinal and seasonal effects
Before commenting on the validity of a subset of our previous tenets and proposing four new ones, we review below some critical abiotic and biotic factors potentially affecting species diversity and ecological function from upstream to downstream that could influence the nature, applicability, and validity of lotic theories used by many river ecologists.
Hydrogeomorphology is the keystone, physical factor in the RES as it embodies the interaction of the landform (the physical template) and hydrology (flow regime and water retention). The major focus of the RES model is on the pervasive ecological responses to river hydrogeomorphology (as delineated by our FPZs), including how changes in flow pathways influence community and ecosystem properties. Development of a hydrogeomorphic focus of the RES was influenced by earlier river models (e.g., Thoms and Sheldon, 1997; Dollar et al., 2007; Thoms and Parsons, 2002; Thoms and Parsons, 2003; Thoms et al., 2018). The RES contrasts markedly with the well-known River Continuum Concept (or RCC; Vannote et al., 1980; Sedell et al., 1989) which emphasized continuous and gradual physical and ecological changes in rivers related to downstream position.
The ecological importance of hydrogeomorphology has been shown for rivers on multiple continents by various researchers. This is principally based on the concept that the hydrogeomorphologic character of a river network provides the template upon which evolution acts to create unique biological communities (Thoms et al., 2017). For example, the “Inshore Retention Concept” (Schiemer et al., 2001) emphasized the importance of low-flow areas in the main channel borders and lateral side channels of the riverscape based on studies of the modern-day Danube River in Austria. Food web studies of communities in the central and side channels of the mainstem of the Upper Mississippi River (Roach et al., 2009; Thorp and Bowes, 2017; Delong et al., 2019) have confirmed their theory on the importance of the lateral riverscape, as have research on the density and diversity of zooplankton in the St. Lawrence River (Casper and Thorp, 2007) and studies of fish communities in Andean river networks (Elgueta et al., 2019), to mention only a few publications. In a related geomorphic approach, the landscape view of the Network Dynamics Hypothesis (NDH; Benda et al., 2004) focused on the hierarchical nature of branching river networks and interactions with watershed disturbances (fires, storms, and floods) that imposed a non-uniform distribution of riverine habitats, with consequences for biological diversity and productivity.
The ecological roles of hydrogeomorphology and related processes are emphasized in the remainder of the current manuscript, but we acknowledge the need for more research to elucidate effects of the type, size, and location (longitudinal and lateral) of FPZs on lotic ecology for a variety of river channel forms (e.g., Nicholas, 2013), riverscape-floodscape interactions, and climatic conditions.
Seasonality in ecological responses
River discharge and available resources for biotic communities both vary seasonally, and these changes could easily influence the construction, interpretation, and validity of riverine models as they pertain to ecological structure and function. With a few exceptions (e.g., Junk, 1999; Humphries et al., 2014), however, seasonal environmental changes have not been explicitly incorporated into lotic diversity and trophic energy theories, including our own. Lacking from most riverine models is a comprehensive treatment of how periods of reduced diversity, lower population and individual growth rates, depressed metabolic rates, and changes to other environmental factors influence other yearly community and ecosystem processes and how such changes relate to river hydrogeomorphology and downstream position.
Oxygen and temperature as responses to river hydrogeomorphology
While variation in the levels of oxygen and temperature are rarely incorporated in large spatial scale models of river ecology, they can be important in understanding biotic responses to river hydrogeomorphology. For example, it is widely acknowledged that oxygen content varies with temperature (inverse relationship), elevation (often but not exclusively inverse), turbulence (direct and strong), and often water depth. The type of FPZ can be used in part to predict the relative amount of turbulence, but this motion can vary among and within FPZs. For example, in an anastomosing FPZ, water velocity generally decreases from the main channel to the lateral riverscape, with related effects on turbulence, oxygen tension, temperature, substrate size and type, food availability, and eventually biotic communities. Thus, the relative ecological importance of downstream variation in hydrogeomorphic conditions (as emphasized in the RES) and “continuum” position (as highlighted by the RCC) need to be evaluated in light of the degree of changes to stream elevation, turbulence, discharge, and flow pathways (e.g., positions within the main channel vs. slackwaters).
Food type and availability along a river’s path
One of the most prominent and controversial components of stream models is the relative importance of autochthonous (primarily algae) versus allochthonous (terrestrial plants) carbon to system metabolism and organismal growth and diversity. The basic proposition - at least since publication of the RCC - has been that algae dominate food webs in grassland headwaters and shallow mid-sized rivers, but that terrestrial carbon is most important in wooded headwaters and in rivers where solar radiation does not reach the stream bottom because of depth and/or turbidity. However, recent studies using the most accurate analytical techniques (CSIA-AA, or “compound specific stable isotope analyses of amino acids”) have shown the greater importance of autochthonous carbon for assimilation into tissues of organisms within Southeast Asian streams (Liew et al., 2019) and in both forested and open canopy headwaters in temperate steppes of the USA and Mongolia (Arsenault et al., 2022). This has also been demonstrated for periods of maximum consumer growth in main channels and slackwaters of large rivers like the Upper Mississippi (e.g., Thorp and Bowes, 2017). Additional research is needed to understand how the relative importance of autochthonous carbon varies in large rivers with depth and helical flow in the main channel and with water retention in side channels. In contrast, yearly ecosystem metabolism in lotic systems as a whole seems to be driven primarily by allochthonous inputs (e.g., Bernhardt et al., 2017), though the importance of autochthonous inputs can vary with stream size and season (e.g., Bertuzzo et al., 2022).
New perspectives on rivers based on recent and historical, ecological models
While we personally argue that the paradigm of linear, predictable changes from headwaters to a river’s terminus contrasts with the majority of available biophysical, geochemical, and ecosystem data from hydrogeomorphologists and ecologists, it appears that this perspective is still taught with seemingly minimal challenges in many colleges and universities and thus may negatively impact future research. All environmental concepts, in our opinion, should be discussed in light of alternative river theories. Clearly one paper alone cannot adequately explain longitudinal and lateral changes in the ecological structure and function of lotic systems, but our approach here is to present a broader perspective of river macrosystems by analyzing and sometimes updating some widely known theories and by emphasizing missing data and conceptual constructs.
Many models have been developed that describe and evaluate spatially or functionally linked subsets of river ecology and river basins. Three that are often taught in academia emphasize major ecological changes in rivers from headwaters to a river’s terminus and also best fit within the concept of macrosystem ecology, as defined elsewhere (Heffernan et al., 2014; Thorp, 2014; Thorp et al., 2021). Those are the RCC, the RES, and the Stream Biome Gradient Concept (Dodds et al., 2015), the last of which was later expanded as the Freshwater Biome Gradient Framework (FBGC; Dodds et al., 2019). As you evaluate these and other conceptual models, keep in mind the published sentiment from the preface of the 2008 RES book: “Theories should be viewed as formed of unfired clay. They need a lot of shaping and remolding before they accurately model the real world, and sometimes you need to toss them out and start again.” This should be a goal of both the originators and external evaluators of those theories. Consistent with this philosophy, we welcome suggestions for improving this expanded edition of the RES.
A brief historical perspective on models classifying rivers from upstream to downstream
Prior to publication of the RES, ecological analyses of rivers often assumed that the structure and function of ecological systems vary with stream size in a roughly consistent, continuous, and predictable manner from upstream to downstream both within and among basins. Similar assumptions were commonly made when teaching about rivers theories in university ecology classes. Indeed, as pointed out near the end of the last century by Fisher (1997) - and which remains widely debated a quarter century later - the major paradigms and research foci in stream ecology “… have been based upon a linear ideogram - an image which is at best incomplete and at worst incorrect.” With this continuum or clinal approach - as most commonly portrayed in the RCC and the Process Domain Concept (Montgomery, 1999) - the characteristics of a stream with an order of “x” are assumed to be more similar to another stream of the same order within that basin and different from attributes in streams of orders “x+1… n”. The prima facie values of this important and influential approach are that it is very easy to present in a classroom lecture and to use as a putative model for testing river functioning and perhaps managing rivers. The RCC has been beneficial in focusing ecological and academic attention on rivers - ecosystems which have often been neglected in research and teaching. However, the inherent concern with this approach is that it often does not explicitly acknowledge habitat and hydrological differences among streams positioned within the same network size, but which occupy different portions of the same or different basins. Crucial differences could include numerous factors such as elevation, bed slope, hydrogeomorphic type, riparian extent, valley morphology, and character of adjacent river zones or segments. The importance of these factors along with light availability, precipitation, and stream temperature are partially discussed in models by (Dodds et al., 2015; Dodds et al., 2019). Moreover, a continuum approach is obviously not applicable to many endorheic rivers, though these can still be analyzed by the RES.
In contrast to this “clinal” approach, the RES is a heuristic model of lotic biocomplexity applicable to diverse spatiotemporal scales from headwaters to large rivers. Its simultaneous advantage and disadvantage compared to most clinal models is that it is much more complex, and thus more challenging to test in the field and to teach in college classrooms (especially by professors who are not aquatic ecologists). We contend that the RES provides a more accurate framework for understanding broad, often discontinuous patterns along longitudinal and lateral dimensions of river networks as well as local ecological patterns that vary across various temporal and smaller spatial scales. The RES portrays rivers as downstream arrays of large hydrogeomorphic patches (e.g., constricted, braided, and anastomosing channels) formed by catchment geomorphology and climate (Figure 1). The sizes and longitudinal distribution of these patches, which are identifiable using standard geomorphic techniques (e.g., Williams et al., 2013), vary among rivers but sometimes can be difficult to forecast, especially above ecoregional scales (Thoms and Sheldon, 1997; Thoms and Parsons, 2002; Thoms and Parsons, 2003). Some types of hydrogeomorphic patches may reoccur along this downstream passage and may vary considerably in size from upstream to downstream, with consequent relative effects on lotic ecology. We suggest that species types and ecological processes are unlikely to respond equally to such longitudinal changes, but field research testing this proposition largely remains insufficient (but see, for example, Maasri et al., 2021a; Maasri et al., 2021b). However, recent research has shown that the location and strength of discontinuities within river networks are associated with the spatial organisation of FPZs (Scown and Thoms, 2023), which presumably influences ecological communities and functions along rivers from headwaters to lowland reaches.
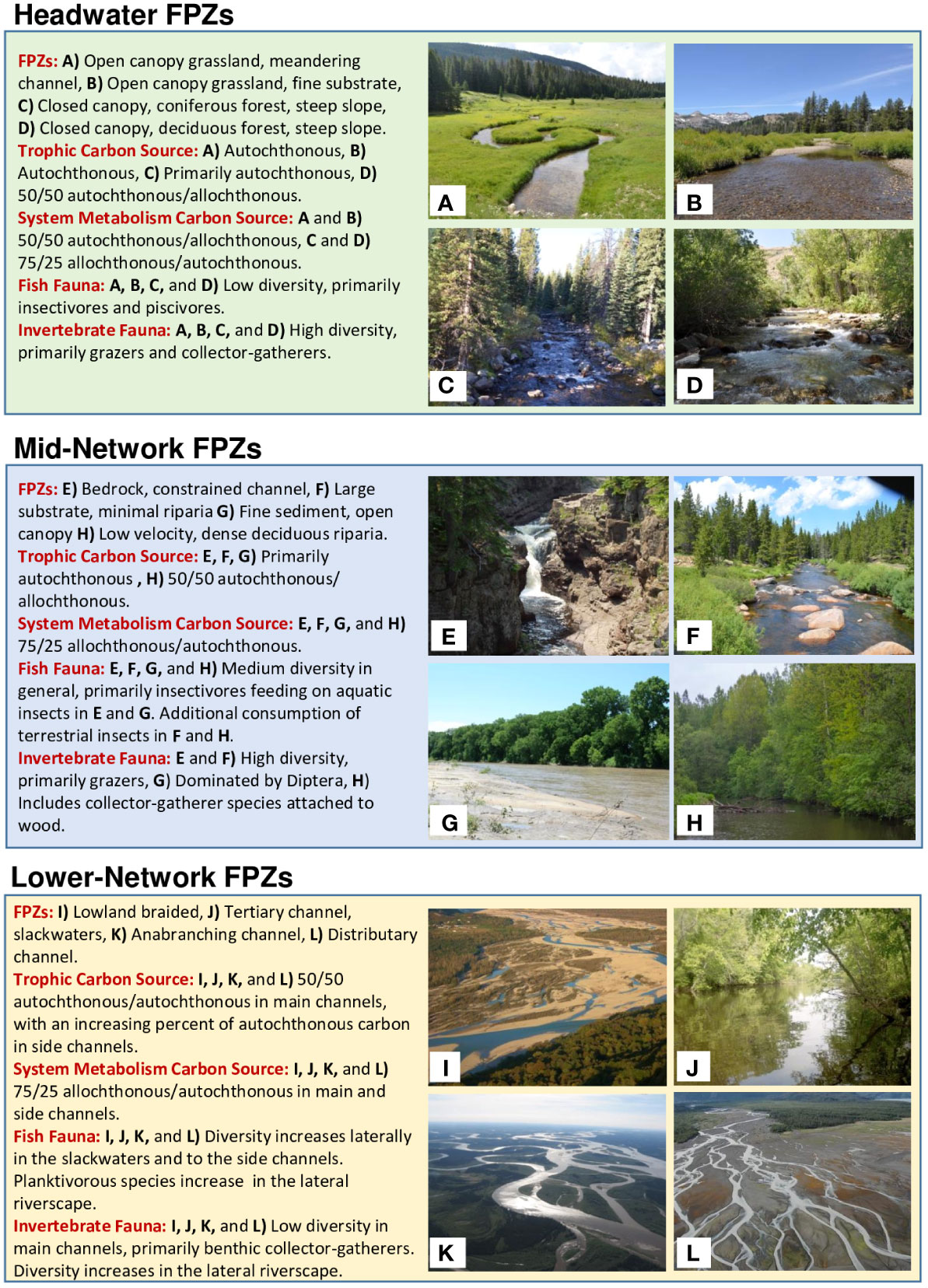
Figure 1 Examples of many of the possible Functional Process Zones (FPZs) within headwaters, mid-order reaches, and large rivers – a perspective emphasized in the Riverine Ecosystem Synthesis (RES) that contrasts sharply with a clinal viewpoint that rivers gradually and predictably change in biodiversity and ecological function from upstream to downstream. Some of the various possible ecological effects of these diverse FPZs are shown, including how they influence trophic carbon sources and system metabolism. An overview of likely functional dominant fish and invertebrate assemblages are also described. Actual ecological processes and biodiversity will vary depending on the specific nature of the river section studied. Photographs are from the authors and open-source figures on the web.
Ecological communities and processes may vary among types of hydrogeomorphic patches (FPZs) because of their unique physicochemical and other ecological habitat characteristics. These FPZs are formed in response to the interactions of many physical characteristics of a basin and river that are not acknowledged in RCC-based models. The RES provides a more precise and accurate portrayal of river structure via hydrogeomorphic analyses compared to the use of simple stream order metrics (e.g., stream orders 1, 2, 3, etc.) - the latter of which can vary greatly in meaning among and within basins even in the same macrosystem for a variety of reasons including data availability, substrate type, and riparian differences. While FPZs can be identified and delineated most accurately with GIS-based hydrogeomorphic models (e.g., Thoms and Parsons, 2002; Thoms and Parsons, 2003; Williams et al., 2013), the river state can also be characterized less rigorously by other common methods used by river scientists if the methods take into account some valley-scale variables as well as stream hydrogeomorphic features. The elevation of the stream section and both the downstream length and lateral extent of the FPZ can sometimes be important predictive variables to consider along with other ecological constraints within the stream and in the adjacent terrestrial habitats. And, of course, anthropogenic factors that can alter river structure and biotic community health need to be considered when testing any lotic model.
Other scientists have also emphasized the importance of structural characteristics of streams and their surrounding watersheds. For example, Poole (2010) verified the critical role in stream ecology of hydrogeomorphic conditions, and Hoeinghaus et al. (2007) emphasized the significant effects of landscape-scale hydrologic characteristics on carbon flow in large-river food webs. Also, Gilvear et al. (2016) integrated river structure with both management needs and related effects on river functioning, and Hanna et al. (2017) evaluated research gaps in riverine ecosystem services.
Evaluation of selected RES hypotheses
In our original journal article (Thorp et al., 2006) and later book (Thorp et al., 2008), we described 14 and 17 tenets, respectively (see abbreviated form in Table 1). Some of these tenets are well supported in field studies conducted by various scientists around the world, but many others still need considerable research confirmation, modification, or perhaps even rejection. Where data are available, however, they generally support the importance of FPZs, with some apparent differences in their applicability based on stream size and the focal dependent variable. The most significant attribute missing is an analysis of the relative importance of different FPZs throughout entire river macrosystems from headwaters to lower sections of large rivers in multiple biomes. Below we briefly review six of the more prominent hypotheses from our book (original tenet number is listed) that have strong support in the ecological literature. [To avoid excessive duplication, refer in part to Thorp et al., 2006; Thorp et al., 2008 for literature support.] We then propose four new hypotheses for the RES in a subsequent section of the current manuscript.
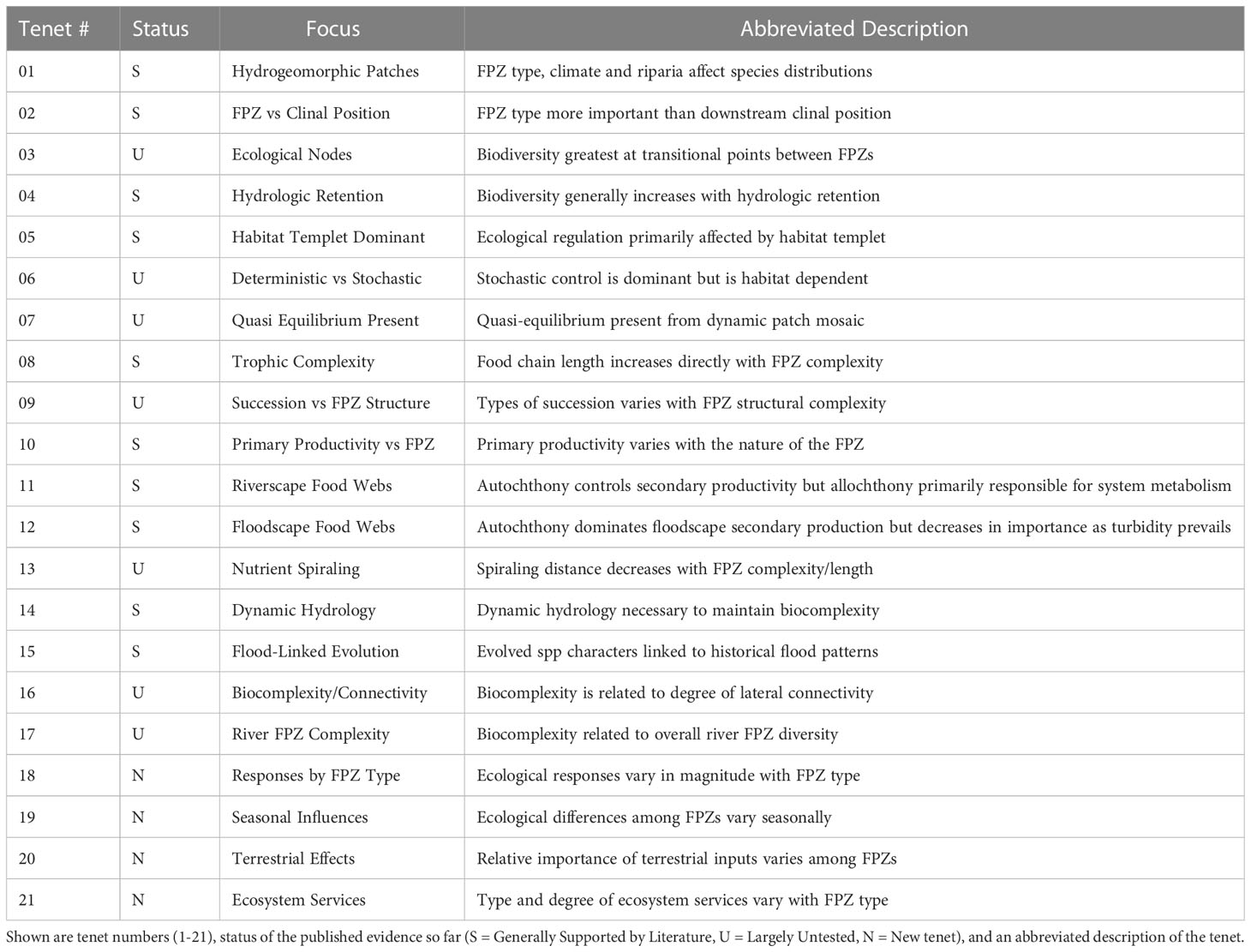
Table 1 Tenets below are abbreviated from our previous publications (Thorp et al., 2006; Thorp et al., 2008) or are discussed only in the current text (tenets #18-21).
Distribution of species and community characteristics
H-01. Hydrogeomorphic patches
Species distributions are associated primarily with the distribution of small to large spatial patches formed principally by hydrogeomorphic forces and modified by climate and vegetation.
Rationale
The ecological importance of FPZs seems likely to be influenced by two main factors (along with a series of lesser elements of nature): (1) local FPZ type and its physical extent longitudinally and laterally; and (2) elevation, because this restricts species composition, range of dependent variables, and sometimes the lateral and downstream extent of the FPZ. For example, Maasri et al. (2019) delineated FPZs across three endorheic drainages in the U.S. Great Basin and found discrete macroinvertebrate communities associated with specific FPZs across multiple drainages. In that study, elevation prevailed over valley confinement in structuring these communities. Overall, environmental filters exerted a stronger control on the functional organization of a community than did the specific taxonomic composition.
H-02. Importance of functional process zone over clinal position
Diversity and distributions of species and ecotypes primarily reflect the nature of the FPZ rather than a clinal position along the river’s longitudinal dimension.
Rationale
The ecological importance of longitudinal position is not controversial at a broad level, but downstream transitions are rarely likely to be smooth, continuous, and equivalent among rivers, especially when flow crosses distinct biomes. For example, the RCC was based on a forested headwater model, but some rivers transition from grasslands in upper watersheds to forested headwaters and from mostly sandy sediments to rocky bottoms and eventually back to gravel-sand bottoms downstream. Continuum models also emphasize single channel systems and the diversity and ecological processes within them, but many medium-to-large rivers historically have had significant lateral anastomosing FPZs where the biotic structure and ecological processes differ from those in the main channel. Evidence indicates that fish community organization is strongly associated with the physical character of FPZs (e.g., Elgueta et al., 2019; Maasri et al., 2021a), as is the structure of zoobenthos communities (e.g., Casper and Thorp, 2007; Maasri et al., 2021b) and food web complexity (Thoms et al., 2017). The extent of differences in species diversity and densities among FPZ types also seem to be influenced by physical heterogeneity, stream elevation, and mean discharge.
Ecological functioning in general and ecosystem services have been altered in many rivers worldwide by the presence of dams and many types of bank structures that control lateral access of river currents and in the process alter the river’s natural hydrogeomorphic structure and basic ecological character. The ecological impacts have been widely studied (e.g., Schmutz and Moog, 2018), including as alterations to the natural flow regime of streams (Poff et al., 1997).
H-04. Hydrologic retention
Overall community complexity varies directly with the diversity of hydrologic habitats in a functional process zone and increases directly with hydrologic retention until other abiotic environmental conditions (e.g., light, oxygen, temperature, substrate type, and nutrient availability) become restrictive.
Rationale
The strong relationship between habitat diversity and community composition has been known for many decades (e.g., Southwood, 1977) and has been demonstrated for both vertebrates and invertebrates in lotic (e.g., Poff and Ward, 1990), lentic, marine, and terrestrial habitats. And thus, while this may be intuitively obvious for lotic ecosystems, we do not fully know to what extent this is important, how it varies with type and size of the FPZ, what effects ecoregional differences have, and where that FPZ is situated from headwaters to the river’s mouth. Nonetheless, it is clear that retaining organic matter in the lateral riverscape with its slower flow rates can substantially affect biodiversity and productivity (e.g., Schiemer et al., 2001). Moreover, we hypothesize that habitat-niche diversity should rise with an increase in an FPZ’s physical complexity.
Community regulation
H-08. Trophic complexity
Food chain length (maximum trophic position) increases directly with the hydrogeomorphic complexity of an FPZ in response to multiple factors related to habitat heterogeneity, diversity and abundance of food resources, and dynamic stability.
Rationale
The scientific literature strongly supports the concept that habitat complexity affects species diversity in aquatic systems (e.g., Power and Dietrich, 2002; Tokeshi and Arakaki, 2012; Brauns et al., 2021) and enhances, therefore, both food chain length and complexity. This has been demonstrated from headwaters to the mouths of rivers and for multiple FPZs (Casper and Thorp, 2007; Roach et al., 2009; O'Neill and Thorp, 2011; Thoms and Delong, 2018; Thoms et al., 2022).
Ecosystem and riverine landscape processes
H-11. Riverscape food web pathways
On an average annual basis, autochthonous production provides, through an algal-grazer food web pathway, the trophic basis for most metazoan productivity for the riverscape as a whole, but allochthonous organic matter may be more important for some species and seasons and in shallow, heavily canopied headwaters; however, a collateral and weakly linked, decomposer food pathway (the microbial-viral loop) is primarily responsible with algal respiration in some cases for a river’s heterotrophic state (P/R < 1).
Rationale
Arguments against this hypothesis are often based on the abundant presence of allochthonous material in the alimentary tract of aquatic insects and the relatively heterotrophic state of many rivers (e.g., Cole and Solomon, 2012). The former essentially assumes that ingestion is a reliable measure of tissue assimilation, when in fact only some plant shredding insects can derive substantial nutritional benefit from plant polysaccharides, although not without microbial mediation (Sinsabaugh et al., 1985). In contrast, Smock and Roeding (1986) found in low-order, blackwater coastal plain streams of the southeastern USA that production in some abundant collector-gatherer species was supported predominantly by algae. Analyses of metazoan food webs using the much more precise but expensive (Bowes and Thorp, 2015) “compound specific stable isotope analyses of amino acids” (CSIA-AA) have demonstrated the dominant importance of autotrophy in large rivers, at least during seasons of maximum secondary production (e.g., Delong and Thorp, 2006; Thorp and Bowes, 2017; Bowes et al., 2020). Moreover, recent work using these highly exact CSIA-AA methods (Arsenault et al., 2022) to study temperate steppe rivers of the USA and Mongolia have provided strong evidence that algal grazer pathways in headwaters may dominate the food web energy pathway for metazoans in both forested and open headwaters.
As an alternative trophic pathway in rivers, Sedell et al. (1989) significantly modified predictions of the RCC for food sources in downstream sections of large rivers worldwide based on the Flood Pulse Concept (FPC; Junk et al., 1989). They concluded that food webs in the lower main channel of large rivers were often supported by organic carbon entering the channel via a floodplain flood pulse rather than by particulate carbon from upstream processing (though both sources are largely composed of recalcitrant carbon). However, and most importantly, the roles of algae in these flooded tropical floodplains and adjacent rivers were not examined in the FPC nor in the modified RCC (see our Tenet 12).
In contrast to metazoan food webs, abundant evidence exists that system metabolism as a whole in lotic systems is characterized by net heterotrophy (Findlay et al., 1991). This occurs via a microbial-viral, decomposer loop that processes both allochthonous and autochthonous carbon (e.g., Thorp and Delong, 2002).
In a related conceptual paper, Humphries et al. (2014) constructed the River Wave Concept (RWC) with the laudable goal of linking various river models into an overall explanation of river food webs in mostly large floodplain rivers. Their basic premise was that all models of food sources in rivers (portions of the RCC, RES, and FPC) were correct but for different times of the year based on prevailing flow patterns and seasons. They hypothesized that the autochthonous carbon derived from algae and riverine macrophytes were most important when river flows were low. We agree with this portion of their model because this is also commonly linked temporally to high primary and secondary production in rivers. They also hypothesized that allochthonous carbon from upstream and floodplains was the dominant source of energy during the periods surrounding maximum flow rates. While we agree that the abundance of recalcitrant carbon peaks at this time in both temperate zone and tropical rivers and that its contribution to food webs may be relatively greater than at other seasons of the year, we contend that there is a dearth of convincing evidence that allochthonous carbon is still relatively more important to food webs on an annual basis and perhaps not even for the high flow periods, especially in temperate zone rivers.
H-12. Floodscape food web pathways
Algal production is the primary source of organic energy fueling aquatic metazoan food webs in floodscapes of most riverine landscapes, especially in rivers with seasonal, warm-weather floods; the relative importance of autochthonous production in floodscapes decreases with a rise in the amount and temporal extent of inorganic turbid conditions.
Rationale
This tenet could be one of the most controversial, in great part because of insufficient field studies. The relative importance of autochthonous and allochthonous carbon as basal energy sources for metazoa in flooded floodscapes almost certainly depends partially on the seasonality (vs. maximum period of consumer growth and reproduction), temporal length, spatial extent, and predictability of floods. If floods tend to be relatively short: (a) opportunities for evolving flood-associated food webs would be diminished; (b) turbid water conditions would reduce the depth of photosynthetic active radiation; and (c) the negative effects from relying on less nutritious food sources (refractile POC) would be short-lived. For example, the flood period in the Amazon is long and predictable, and thus life history adaptations over time to this pattern would be expected. In contrast, the seasonal spring floods in the Mississippi River are largely based on upstream, spring snow melt and are shorter, and often seasonally inappropriate as a major food web contributor because primary and secondary productivity are maximum in the summer months in the adjacent river channels. It is worth noting that the authors of the Flood Pulse Concept (Junk et al., 1989) - upon which floodplain contributions were based - apparently assumed that algae were not important in the metazoan food webs of floodplains and thus did not quantify their potential contribution. Consequently, evidence for or against this hypothesis may depend on the latitude and river system examined, as evidence seems to exist for and against this hypothesis. Some other authors have verified the importance of algae in the central channel of floodplain river food webs, including studies by Hamilton et al. (1992) for the neotropical Orinoco River, and both Thorp and Delong (2002) and Bowes et al. (2020) for the main channel and lateral riverscape of the temperate Mississippi River. However, Jeffries et al. (2020) demonstrated that the relative importance of algae as the primary base of the food web for river salmon varied with the physical location within the lateral river components and the prey components of the diet in those areas.
New RES hypotheses
We are proposing below four new tenets to the 14-17 listed in our previous publications (Thorp et al., 2006; Thorp et al., 2008).
H-18. The overall degree of ecological responses varies with FPZ type, surface area, and species or process examined
The range and degree of impacts of a Functional Process Zone on biodiversity and ecological processes differ among several factors, including types of FPZs, total river area covered, and dependent variables examined, even in the same river network position.
Rationale
The physical complexity of an FPZ is directly associated with the type and abundance of aquatic habitats and, to some degree, the interactions with the adjacent terrestrial habitat during normal flows and floods. For example, in comparison to a meandering FPZ (a medium complexity condition), a constricted channel FPZ (Figure 1E) provides the least habitat complexity (thus supporting fewer niches and a lower species diversity), while anastomosing and anabranching FPZs (Figures 1J, K) afford the greatest diversity of habitats and niches and thus the highest species diversity. These laterally complex FPZs provide more varied niches compared to less complex FPZs based on the greater production in lateral slackwater habitats with their slower current velocities, more abundant hard surface habitats, greater diversity of habitats in general, and deeper light penetration compared to the river’s main channel. Unfortunately, ecological impacts from anthropogenic modification (e.g., channelization, dams, and levees) are likely greater in rivers sections formerly characterized by abundant anastomosing channels.
FPZs that are relatively short and narrow should have fewer opportunities to influence a river’s ecological conditions. Furthermore, FPZ types that occur often, or constitute a high relative percentage of the river network, are obviously expected to have a greater contribution to the overall functioning and organization of a riverine macrosystem. The greater the difference between FPZ types in a river, the more significant on average will be the corresponding effects on biodiversity and ecological processes for the river as a whole. However, not all ecologically dependent variables may change in the same way and degree to differences in the type, elevation, and longitudinal position of FPZs. For example, species richness and secondary production seem more responsive to differences in FPZ type than does system metabolism, which might be driven more by ecoregion and biome scale variables.
H-19. Seasonal influences
The degree of ecological differences among types of FPZs vary seasonally with the process being examined while also differing among types of life history characteristics - especially when contrasting responses among seasonal periods of either maximum or minimum growth and reproduction.
Rationale
Growth and reproductive processes vary to some degree seasonally in rivers at all latitudes, and the degree of seasonal impacts may vary among FPZ types and location within the river from headwaters to the mouth. Thus, the relative ecological importance of abiotic conditions - such as flooding, current velocities, turbidity, light penetration, and water temperatures on biotic processes (e.g., growth rates, reproduction, and system metabolism) - may also vary to different degrees over an annual cycle. Food source hypotheses on this topic were explored in greater detail by Humphries et al. (2014). However, some relationships (e.g., the importance of autochthonous carbon to food webs or allochthonous carbon to system metabolism) may be consistently dominant on a yearly average but vary seasonally in the relative degree of importance to growth and reproduction vs simple maintenance compared to other factors.
H-20. Relative impact of terrestrial inputs vary with FPZ type, stream size, and watershed flora
The relative importance of in-stream versus watershed drivers of ecological processes in streams can vary within macrosystems and among ecoregions and partially depends on elevation, terrestrial characteristics (natural or human modified), and FPZ type and extent.
Rationale
The relative effects of a stream’s watershed on lotic structure and function vary directly with the interactive watershed area and valley characteristics. As the percentage of interactive borders between the stream and the adjacent land increases, the terrestrial influence on lotic processes should rise. This will be true from both multi-channel headwaters through anastomosing great rivers. Moreover, terrestrial influences increase with the permanence of the riverscape’s slackwater habitat and with longer and more predictable flood periods that occur during seasons that are most suitable for secondary production.
The impact of riparian vegetation will naturally vary with the labile vs. recalcitrant nature of the vegetation but should also increase where riparian trees provide physical structure within the stream from projecting roots that can serve as hard substrate for algal growth, attachment sites for invertebrates, and refuges from larger aquatic predators (Thorp et al., 1985).
Floodplain rivers by their nature interact more closely with the main channel community than do non-floodplain rivers over periods ranging from days and weeks to multiple months (in some tropical locations). Interaction of the communities in streams located in high relief watersheds (e.g., those passing through gorges) tend to be relatively short but more intense because of rapid changes in current velocities and resulting shear stress. Finally, in mixed vegetation areas, the diversity of inputs of detritus and live animals falling into the stream may be more diverse than in areas with a constant vegetative cover.
H-21. Ecosystem services
The provision of ecosystem services varies significantly with FPZ type, river size, and location vis-à-vis human populations.
Rationale
It is well established that lotic ecosystems provide numerous ecosystem services to humans including water (e.g., for drinking, crops, manufacturing), power (dams), transportation of people and material, food, recreation, and diverse cultural activities (Costanza et al., 2014; Rieb and Bennett, 2020). In most cases the maximum values of these services vary directly (but not necessarily proportionally) with river discharge, but even small streams can provide most of these benefits to at least a limited degree. In many cases, the degree and types of services also can vary among FPZ types (Thorp et al., 2010; Bajracharya et al., 2023). For example, constrained channels are superior sites for impoundments to generate electricity, whereas the side channels of anastomosing FPZs provide better locations for recreational houseboats than in most main channel habitats. While rocky, turbulent FPZs are more ideal for some fishes (such as trout), lateral slackwaters allow better access for fishes requiring slow velocities. Anabranching and anastomosing FPZs also provide more water storage sites during floods.
Conclusions and a guide for future conceptual models
In this manuscript, we have provided research support for 6 of our previous 17 tenets (≈ hypotheses) and have added four new ones. Additional research is required for all 21 tenets, and we encourage our colleagues to test these ideas even if they disagree with our initial conclusions in some way.
Rigorous examination of these tenets should be undertaken in a great diversity of lotic habitats. For example, more research is needed in large rivers, in slackwater habitats of all sizes of rivers, and especially in understudied habitats globally, such as dryland rivers in Africa. Finally, we need an enhanced focus on floodplain ecology (= floodscape studies) in rivers spanning the range from systems characterized by short intermittent floods to ones with highly predictable and long-lasting flood inundation.
Conceptual models help ecologists better understand nature, but progress in elucidating critical factors controlling riverine populations through macrosystems are hindered by numerous limitations, including: (a) insufficient funding by governments around the world; (b) a narrow spatiotemporal focus of most aquatic studies; (c) over reliance on individual research versus team research; (d) educating the next generation using overly simple ecological models; and (e) the inherent weakness in all of us to be willing to challenge not only other prominent models but also our own. Finally, we need to acknowledge that published models – including ones that we propose - are rarely the finished product, and thus we must continue testing and remolding them over time as we gain additional understanding about the functioning of natural systems.
Data availability statement
The original contributions presented in the study are included in the article/supplementary material. Further inquiries can be directed to the corresponding author.
Author contributions
All authors listed have made a substantial, direct, and intellectual contribution to the work and approved it for publication.
Funding
Funding for JHT and AM was provided by the National Science Foundation MacroSystems Biology grant number: 1442595.
Conflict of interest
The authors declare that the research was conducted in the absence of any commercial or financial relationships that could be construed as a potential conflict of interest.
Publisher’s note
All claims expressed in this article are solely those of the authors and do not necessarily represent those of their affiliated organizations, or those of the publisher, the editors and the reviewers. Any product that may be evaluated in this article, or claim that may be made by its manufacturer, is not guaranteed or endorsed by the publisher.
References
Arsenault E. R., Thorp J. H., Polito M. J., Minder M., Dodds W. K., Tromboni F., et al. (2022). Intercontinental analysis of temperate steppe stream food webs reveals consistent autochthonous support of fishes. Ecol. Lett. 25, 2624–2636. doi: 10.1111/ele.14113
Bajracharya S., Thoms M. C., Parsons M. (2023). The heterogeneity of ecosystem services across the riverine landscape of the koshi river basin, Nepal. Ann. Am. Assoc. Geographers 1–23. doi: 10.1080/24694452.2023.2187338
Benda L., Poff N. L., Miller D., Dunne T., Reeves G., Pess G., et al. (2004). The network dynamics hypothesis how channel networks structure riverine habitats. BioScience 54, 413–427. doi: 10.1641/0006-3568(2004)054[0413:TNDHHC]2.0.CO;2
Bernhardt E. S., Heffernan J. B., Grimm N. B., Stanley E. H., Harvey J. W., Arroita M., et al. (2017). The metabolic regimes of flowing waters. Limnol. Oceanogr. 63, 99–118. doi: 10.1002/lno.10726
Bertuzzo E., Hotchkiss E. R., Argerich A., Kominoski J. S., Oviedo-Vargas D., Savoy P., et al. (2022). Respiration regimes in rivers partitioning source-specific respiration from metabolism time series. Limnol. Oceanogr. 67, 2374–2388. doi: 10.1002/lno.12207
Bowes R. E., Thorp J. H. (2015). Consequences of employing amino acid vs. bulk-tissue, stable isotope analysis a laboratory trophic position experiment. Ecosphere 6 (1), 1–12. doi: 10.1890/ES14-00423.1
Bowes R. E., Thorp J. H., Delong M. D. (2020). Reweaving river food webs through time. Freshw. Biol. 65, 390–402. doi: 10.1111/fwb.13432
Brauns M., Kneis D., Brabender M., Weitere M. (2021). Habitat availability determines food chain length and interaction strength in food webs of a large lowland river. River Res. Appl. 2021, 1–11. doi: 10.1002/rra.3908
Casper A. F., Thorp J. H. (2007). Diel and lateral patterns of zooplankton distribution in the st. Lawrence river. River Res. Appl. 23, 73–86. doi: 10.1002/rra.966
Cole J. J., Solomon C. T. (2012). Terrestrial support of zebra mussels and the Hudson river food web a multi-isotope, Bayesian analysis. Limnol. Oceanogr. 57, 1802–1815. doi: 10.4319/lo.2012.57.6.1802
Costanza R., de Groot R., Sutton P., van der Ploeg S., Anderson S. J., Kubiszewski I., et al. (2014). Changes in the global value of ecosystem services. Glob. Environ. Change 26, 152–158. doi: 10.1016/j.gloenvcha.2014.04.002
Delong M. D., Thoms M. C., Sorenson E. (2019). Interactive effects of hydrogeomorphology on fish community structure in a large floodplain river. Ecosphere 10, 1–19. doi: 10.1002/ecs2.2731
Delong M. D., Thorp J. H. (2006). Significance of instream autotrophs in trophic dynamics of the upper Mississippi river. Oecologia 147, 76–85. doi: 10.1007/s00442-005-0241-y
Dodds W. K., Bruckerhoff L., Batzer D., Schechner A., Pennock C., Renner E., et al. (2019). The freshwater biome gradient framework predicting macroscale properties based on latitude, altitude, and precipitation. Ecosphere 10, 1–33. doi: 10.1002/ecs2.2786
Dodds W. K., Gido K., Whiles M. R., Daniels M. D., Grudzinski B. P. (2015). The stream biome gradient concept factors controlling lotic systems across broad biogeographic scales. Freshw. Sci. 34, 1–19. doi: 10.1086/679756
Dollar E. S. J., James C. S., Rogers K. H., Thoms M. C. (2007). A framework for interdisciplinary understanding of rivers as ecosystems. Geomorphology 89, 147–162. doi: 10.1016/j.geomorph.2006.07.022
Elgueta A., Thoms M. C., Górski K., Díaz G., Habit E. L. (2019). Functional process zones and their fish communities in temperate Andean river networks. River Res. Appl. 2019, 1–10. doi: 10.1002/rra.3557
Findlay S., Pace M. L., Lints D., Cole J. J., Caraco N. F., Peierls B. (1991). Weak coupling of bacterial and algal production in a heterotrophic ecosystem the Hudson river estuary. Limnol. Oceanogr. 36, 268–278. doi: 10.4319/lo.1991.36.2.0268
Fisher S. G. (1997). Creativity, idea generation, and the functional morphology of streams. J. North Am. Benthological Soc. 16, 305–318. doi: 10.2307/1468020
Gilvear D. J., Greenwood M. T., Thoms M. C., Wood P. J. (2016). River science research and management for the 21st century (Chichester: John Wiley and Sons).
Hamilton S. K., Lewis W. M. Jr., Sippe S. J. (1992). Energy sources for aquatic animals in the Orinoco river floodplain evidence from stable isotopes. Oecologia 89, 324–330. doi: 10.1007/BF00317409
Hanna D. E. L., Tomscha S. A., Dallaire C. O., Bennett E. M. (2017). A review of riverine ecosystem service quantification research gaps and recommendations. J. Appl. Ecol. 55, 1299–1311. doi: 10.1111/1365-2664.13045
Heffernan J. B., Soranno P. A., Angilletta M. J. (2014). Macrosystems ecology understanding ecological patterns and processes at continental scales. Front. Ecol. Environ. 12, 5–14. doi: 10.1890/130017
Hoeinghaus D. J., Winemiller K. O., Agostinho A. A. (2007). Landscape-scale hydrologic characteristics differentiate patterns of carbon flow in large-river food webs. Ecosystems 10, 1019–1033. doi: 10.1007/s10021-007-9075-2
Humphries P., Keckeis H., Finlayson B. (2014). The river wave concept integrating river ecosystem models. BioScience 64, 870–882. doi: 10.1093/biosci/biu130
Jeffries C. A., Holmes E. J., Sommer T. R., Katz J. V. E. (2020). Detrital food web contributes to aquatic ecosystem productivity and rapid salmon growth in a managed floodplain. PloS One 15 (9), e0216019. doi: 10.1371/journal.pone.0216019
Junk W. J. (1999). The flood pulse concept of large rivers learning from the tropics. Archiv fur Hydrobiologie/Suppl. Large Rivers 11, 261–280. doi: 10.1127/lr/11/1999/261
Junk W. J., Bayley P. B., Sparks R. E. (1989). “The flood-pulse concept in river-floodplain systems,” in Proceedings of the international Large river symposium, Canadian special publications in fisheries and aquatic sciences, vol. 106 . Ed. Dodge D. P., 110–127.
Liew J. H., Chua K. W. J., Arsenault J., Thorp J. H., Suvarnaraksha A., Amirrudin A., et al. (2019). Quantifying the role of terrestrial carbon in freshwater food webs using amino acid isotope analysis - a case study with an endemic cave fish. Methods Ecol. Evol. 10, 1594–1605. doi: 10.1111/2041-210X
Maasri A., Pyron M., Arsenault E. R., Thorp J. H., Mendsaihan B., Tromboni F., et al. (2021a). Valley-scale hydrogeomorphology drives river fish assemblage variation in Mongolia. Ecol. Evol. 11, 6527–6535. doi: 10.1002/ece3.7505
Maasri A., Thorp J. H., Gelhaus J. K., Tromboni F., Chandra S., Kenner S. J. (2019). Communities associated with functional process zone scale a case of stream macroinvertebrates in endorheic drainages. Sci. Total Environ. 677, 184–193. doi: 10.1016/j.scitotenv.2019.04.394
Maasri A., Thorp J. H., Kotlinski N., Kiesel J., Erdenee B., Jähnig S. (2021b). Variation in macroinvertebrate community structure of functional process zones along the river continuum new elements for the interpretation of the river ecosystem synthesis. River Res. Appl. 37, 665–674. doi: 10.1002/rra.3784
Montgomery D. R. (1999). Process domains and the river continuum. J. Am. Water Resour. Assoc. 35, 397–410. doi: 10.1111/j.1752-1688.1999.tb03598.x
Nicholas A. P. (2013). Modelling the continuum of river channel patterns. Earth Surface Process Landforms 38, 1187–1196. doi: 10.1002/esp.3431
O'Neill B. J., Thorp J. H. (2011). Zoobenthos of a sand bed river coping with hydrogeomorphic fluctuations. J. North Am. Benthol. Soc. 30, 546–558. doi: 10.1899/10-083.1
Poff N. L. R., Allan J. D., Bain M. B., Karr J. R., Prestegaard K. L., Richter B. D., et al. (1997). The natural flow regime; a paradigm for river conservation and restoration. Bioscience 47, 769–784. doi: 10.2307/1313099
Poff N. L. R., Ward J. V. (1990). Physical habitat template of lotic systems recovery in the context of historical pattern of spatiotemporal heterogeneity. Environ. Manage. 14, 629–645. doi: 10.1007/BF02394714
Poole G. C. (2010). Stream hydrogeomorphology as a physical science basis for advances in stream ecology. J. North Am. Benthological Soc. 29, 12–25. doi: 10.1899/08-070.1
Power M. E., Dietrich W. E. (2002). Food webs in river networks. Ecol. Res. 17, 451–471. doi: 10.1046/j.1440-1703.2002.00503.x
Rieb J. T., Bennett E. M. (2020). Landscape structure as a mediator of ecosystem service interactions. Landscape Ecol. 35, 2863–2880. doi: 10.1007/s10980-020-01117-2
Roach K. A., Thorp J. H., Delong M. D. (2009). Influence of lateral gradients of hydrologic connectivity on trophic position of fishes in the upper Mississippi river. Freshw. Biol. 54, 607–620. doi: 10.1111/j.1365-2427.2008.02137.x
Schiemer F., Keckeis H., Reckendorfer W., Winkler G. (2001). The "inshore retention concept" and its significance for large rivers. Arch. für Hydrobiologie suppl.)135, 509–516. doi: 10.1127/lr/12/2001/509
Schmutz S., Moog O. (2018). “Dams ecological impacts and management,” in Riverine ecosystem management, vol. 8 . Eds. Schmutz S., Sendzimir J. (Cham: Springer). doi: 10.1007/978-3-319-73250-3_6
Scown M. W., Thoms M. C. (2023). The discontinuum of river networks the importance of geomorphic boundaries. Landscape Ecol. 38, 1307–1319. doi: 10.1007/s10980-023-01626-w
Sedell J. R., Richey J. E., Swanson F. J. (1989). “The river continuum concept a basis for the expected behavior of very large rivers?” in Proceedings of the international Large river symposium. Ed. Dodge D. P. (Canadian Special Publications in Fisheries and Aquatic Sciences), 106.
Sinsabaugh R. L., Linkins A. E., Benfield E. F. (1985). Cellulose digestion and assimilation by three leaf-shredding aquatic insects. Ecology 66, 1464–1471. doi: 10.2307/1938009
Smock L. A., Roeding C. E. (1986). The trophic basis of production of the macroinvertebrate community of a southeastern U.S.A. blackwater stream. Holarctic Ecol. 9, 165–174.
Southwood T. R. E. (1977). Habitat, the templet for ecological strategies? J. Anim. Ecol. 46, 337–365. doi: 10.2307/3817
Thoms M. C., Delong M. D. (2018). Ecosystem responses to water resource developments in a large dryland river. Water Resour. Res. 54, 6643–6655. doi: 10.1029/2018WR022956
Thoms M. C., Delong M. D., Flotemersch J. E., Collins S. E. (2017). Physical heterogeneity and aquatic community function in river networks a case study from the kanawha river basin, USA. Geomorphology 290, 277–287. doi: 10.1016/j.geomorph.2017.02.027
Thoms M. C., Parsons M. (2002). Eco-geomorphology an interdisciplinary approach to river science. Int. Assoc. Hydrological Sci. 276, 113–120.
Thoms M. C., Parsons M. (2003). Identifying spatial and temporal patterns in the hydrological character of the condamine-balonne river, Australia, using multivariate statistics. River Res. Appl. 19, 443–457. doi: 10.1002/rra.737
Thoms M. C., Rayburg S., Neave M., Parsons M., Chiew F. (2022). The physical diversity and assessment of a large river system the Murray-darling basin, Australia. Ed. Gupta (Chichester: Large Rivers. Wiley), p 587–p 608.
Thoms M., Scown M., Flotemersch J. (2018). Characterization of river networks a GIS approach and its applications. J. Am. Water Resour. Assoc. 54, 899–913. doi: 10.1111/1752-1688.12649
Thoms M. C., Sheldon F. (1997). “River channel complexity and ecosystem processes the barwon–darling river (Australia),” in Frontiers in ecology building the links. Eds. Klomp N., Lunt I. (Oxford: Elsevier Science), p.193–p.205.
Thorp J. H. (2014). Metamorphosis in river ecology from reaches to macrosystems. Freshw. Biol. 59, 200–210. doi: 10.1111/fwb.12237
Thorp J. H., Bowes R. E. (2017). Carbon sources in riverine food webs new evidence from amino acid techniques. Ecosystems 20, 1029–1041. doi: 10.1007/s10021-016-0091-y
Thorp J. H., Delong M. D. (2002). Dominance of autochthonous autotrophic carbon in food webs of heterotrophic rivers. Oikos 96, 543–550. doi: 10.1034/j.1600-0706.2002.960315.x
Thorp J. H., Dodds W. K., Robbins C. J., Maasri A., Arsenault E. R., Lutchen J. A., et al. (2021). A framework for lotic macrosystem research. Ecosphere 12 (2), e03342. doi: 10.1002/ecs2.3342
Thorp J. H., Flotemersch J. E., Delong M. D., Casper A. F., Thoms M. C., Ballantyne F., et al. (2010). Linking ecosystem services, rehabilitation, and river hydrogeomorphology. BioScience 60, 67–74. doi: 10.1525/bio.2010.60.1.11
Thorp J. H., McEwan E. F., Flynn M. F., Hauer F. R. (1985). Invertebrate colonization of submerged wood in a cypress-tupelo swamp and blackwater stream. Am. Midland Naturalist. 113, 56–68. doi: 10.2307/2425347
Thorp J. H., Thoms M. C., Delong M. D. (2006). The riverine ecosystem synthesis biocomplexity in river networks across space and time. River Res. Appl. 22 (2), 123–147. doi: 10.1002/rra.901
Thorp J. H., Thoms M. C., Delong M. D. (2008). The riverine ecosystem synthesis (Boston, MA, USA: Academic Press), 208 p.
Tokeshi M., Arakaki S. (2012). Habitat complexity in aquatic systems fractals and beyond. Hydrobiologia 685, 27–47. doi: 10.1007/s10750-011-0832-z
Vannote R. L., Minshall G. W., Cummins K. W., Sedell J. R., Cushing C. E. (1980). The river continuum concept. Can. J. Fisheries Aquat. Sci. 37, 130–137. doi: 10.1139/f80-017
Keywords: macrosystem ecology, river continuum concept, riverine ecosystem synthesis, river ecology theories, river functioning
Citation: Thorp JH, Thoms MC, Delong MD and Maasri A (2023) The ecological nature of whole river macrosystems: new perspectives from the riverine ecosystem synthesis. Front. Ecol. Evol. 11:1184433. doi: 10.3389/fevo.2023.1184433
Received: 11 March 2023; Accepted: 05 June 2023;
Published: 16 June 2023.
Edited by:
Tao Tang, Institute of Hydrobiology (CAS), ChinaReviewed by:
Yixin Zhang, Soochow University, ChinaRobert Fournier, University of California, Berkeley, United States
Samuel Dias, University of Arkansas, United States in collaboration with reviewer [RF]
Copyright © 2023 Thorp, Thoms, Delong and Maasri. This is an open-access article distributed under the terms of the Creative Commons Attribution License (CC BY). The use, distribution or reproduction in other forums is permitted, provided the original author(s) and the copyright owner(s) are credited and that the original publication in this journal is cited, in accordance with accepted academic practice. No use, distribution or reproduction is permitted which does not comply with these terms.
*Correspondence: James H. Thorp, thorp@ku.edu