- 1Henan Institute of Meteorological Science, Zhengzhou, China
- 2Key Laboratory of Agrometeorological Support and Applied Technique, China Meteorological Administration, Zhengzhou, China
- 3Chinese Academy of Agricultural Sciences Institute of Crop Sciences, Beijing, China
- 4Institute of Environmental & Agricultural Sciences, Faculty of Life Sciences, University of Okara, Renala Khurd, Punjab, Pakistan
- 5College of Resources and Environmental Sciences, China Agricultural University, Beijing, China
Introduction: Mid-season lodging in maize (Zea mays L.) often restricts grain yield potential and is a great hurdle in improving production efficiency. The aim of this research was to study the effects of lodging on photosynthesis, evapotranspiration, dry matter accumulation, and distribution in a maize population.
Methods: We examined the effects of lodging on photosynthesis, dry matter accumulation, and distribution of maize in Zhengzhou Agro-meteorological station in August 2016, following a strong wind lodging process. Based on observational data of crops, meteorology, and CO2/H2O flux from milk maturity to maturity of maize in a normal growth year (2017), a model of population photosynthesis and evapotranspiration of maize under normal growth conditions was constructed. The validated model was used to simulate the theoretical value of photosynthesis and evapotranspiration in lodging years (2016), then the measured value of population photosynthesis and evapotranspiration after lodging was calculated based on the measured data of CO2/H2O flux from milk maturity to maturity of maize, and the difference between the simulated value and the measured value of population photosynthesis and evapotranspiration in lodging years (2016) was compared and analyzed. The correlation between dry matter accumulation and population photosynthetic accumulation was examined in order to estimate the reduction of dry matter accumulation after lodging. The effect of lodging on dry matter accumulation, distribution, and yield was analyzed using field biomass data. The population photosynthesis model and evapotranspiration model could accurately simulate the characteristics of normal growth conditions of summer maize.
Results: The population photosynthesis model absolute error between the simulated value and the measured values in 2017 was −0.43 mg m−2 s−1, and the relative error was −3.3%; the evapotranspiration model absolute error between the simulated value and the measured values in 2017 was −0.005 mm·30 min−1, and the relative error was −10.7%. In 2016, the measured value of photosynthesis after lodging was significantly lower than the simulated value, and the daily average population photosynthesis rate decreased by 13.99 mg m−2 s−1 or 53%. Under the same condition, the daily average evapotranspiration decreased by 1.03 mm d−1 or 28%. The lodging process altered the accumulation and distribution of dry matter in maize. The dry weight of the stem and sheath increased by 5.5% and the ear weight decreased by 10.9% after lodging, compared to without lodging but there was no significant effect on leaf dry weight. After lodging, the proportion of stem sheath distribution increased by 3.0%, while the proportion of ear distribution decreased by 3.0%. After lodging, 100-grain weight and plant grain weight decreased by 2.8 and 10.8%, respectively. According to the lodging rate and density theory of computation yield, the yield of the entire field was reduced by 5.0%.
1. Introduction
Maize is the most widely cultivated food crop in the Huanghuai area, and its perennial sown area and yield account for more than 20% of maize in China (National Bureau Of Statistics Of China, 2018). In the growing season of maize, strong convective weather is frequent which makes lodging possible. Lodging is a critical meteorological disaster that affects the yield and quality of maize. Lodging alters the population structure and spatial distribution of leaves in maize, resulting in a mutual shielding of plants and leaves, thereby reducing the ventilation and light transmission between plants and the photosynthetic rate of the population (Alam et al., 2018; Wang et al., 2022). Furthermore, lodging destroys the grooming tissues of the stem and negatively affects the transportation of water and nutrients from roots to leaves; at the same time, the transportation of photosynthetic products to underground organs and ear filling are hindered (Elmore and Ferguson, 1999; Yoon-Sup et al., 2013; Jun et al., 2016), all of which directly affect yield and components of yield (Minami and Ujihara, 1991; Jun et al., 2017; Xue et al., 2021). Various studies have shown that lodging reduces the yield of maize by 5–25% every year, and the yield decreases by 90–120 kg hm−2 for every 1% increase in lodging rate (Elmore and Ferguson, 1999; Kang et al., 1999).
Lodging during grain filling can reduce the content of crude fat and crude protein in maize grains, thus affecting the quality of maize (Cao, 2016). Previous studies have made in-depth analyses of the effects of lodging on dry matter accumulation, distribution, yield, and quality of maize, as well as causes of lodging, remedial measures, and the evaluation of lodging resistance on varieties of maize (Zhang et al., 2009; Wang et al., 2011; Chen et al., 2012). However, few studies have been conducted on the various characteristics of maize photosynthetic rates and evapotranspiration after lodging; observation methods have been identified as one of the main reasons for limited research in this field. The plants become mutually shaded after lodging, so it is necessary to measure the population photosynthesis and evapotranspiration to reflect the real disaster situation on the field. At present, the recognized photosynthetic measurement systems such as LI-6800 are limited to the measurement of the photosynthetic capacity of a single leaf. Since summer maize is a high-stem crop, it is difficult to measure community photosynthesis directly. However, considerable numbers of research have been carried out on the photosynthesis of maize leaves, the characteristics of the population photosynthesis, and the establishment of photosynthesis and evapotranspiration models by numerical analysis (Yu et al., 1998; Zhang et al., 2004; Wang, 2006). The findings of this research provide new ideas for analyzing the characteristics of summer maize population photosynthesis and evapotranspiration changes after lodging.
In this study, we examined the effects of a maize lodging catastrophe at the Zhengzhou Agricultural Meteorological Experimental Station on photosynthesis, evapotranspiration, dry matter accumulation, and distribution of the maize population in August 2016. Based on the flux observation system and crop observation data of summer maize from the milk stage to the maturation stage without lodging in 2017, photosynthesis and evapotranspiration simulation models were constructed and verified under normal growth conditions. In 2016, the verified models were used to simulate the theoretical value without lodging. In addition, after lodging, according to the observed data of CO2 flux, the actual photosynthetic rate and evapotranspiration were determined by using the carbon and water cycles theory of farmland ecosystem as the measured value, and the decrease in population photosynthesis and evapotranspiration after lodging was compared and analyzed. The Richards dry matter accumulation model was used to simulate and analyze the relationship between daily photosynthesis and daily dry matter accumulation after lodging. The dry matter accumulation after lodging was calculated by the decrease in group photosynthesis. At last, we analyzed the effects of lodging on dry matter distribution and yield formation by using field biomass data.
2. Materials and methods
2.1. Experimental site
The experimental site was located at the Zhengzhou Agro-meteorological Station, Henan Province, China (34 ° 43 ′ N, 113 ° 39 ′ E, altitude 110.4 m). The annual average temperature and precipitation at the station were 14.9°C and 670 mm, respectively. The mid-mature hybrid variety “Xundan 20” was sown in 2016 and 2017. There were no meteorological disasters during the 2017 growing season, and there were no major meteorological disasters except for a strong wind on August 25, 2016. The station experienced a strong wind at a maximum wind speed of 18.1 m s−1 and an hourly average wind speed of 3.8 m s−1 at 12:20 on August 25, 2016 (the third day after milk maturity). This time, the wind was accompanied by precipitation, with daily precipitation of 23.8 mm. Approximately half of the entire field was lodged, with broken stems mainly occurring between the third and fourth nodes, and the broken parts were approximately 20 to 30 cm away from the ground.
2.2. CO2 flux data observation and data quality control
The CO2 flux observation system is based on the eddy covariance system (Campbell Scientific Company of America), which also incorporates a gradient observation system and a vorticity observation system. The gradient observation system consisted of a set of PAR LITE photosynthetically active radiation sensors, five layers of air temperature and humidity probes, and four layers of wind direction anemometers. The eddy covariance system was composed of an LI-7500 CO2/H2O analyzer (with a height of 3 m), a CSAT3 3D ultrasonic anemometer, a CNR2 net radiation sensor, and the HFP01 soil heat flux plate. The daily maintenance and flux data download of instruments were carried out in accordance with the data format by the standers of the China Meteorological Administration. The half-hour flux data was processed in accordance with the general flux data processing method through outlier elimination, coordinate axis rotation, and WPL transformation (Vickers and Mahrt, 1997; Li et al., 2008; Xu et al., 2008). The average day and night method and nonlinear regression method were used to interpolate for the data missing due to precipitation, power failure, and other factors. As a result, the continuity of the data was ensured (Falge et al., 2001; Guo et al., 2007).
The CO2 data was analyzed from August 22 to September 12 before and after lodging in 2016, and the data on the day of lodging (August 25) was excluded due to rainfall. In 2017, the corresponding data from milk maturity to maturity (August 28 to September 22) was selected for analysis, and the data from August 29 to 30 were excluded because of rainfall.
2.3. Investigation on lodging disaster of maize
On the second day after maize lodging (August 26, 2016), the lodging type and lodging rate were investigated. This lodging was caused by stem breakage, and 100 plants were continuously examined to record the lodging number. Based on statistical analysis and image recognition (Figure 1), it was determined that the lodging number divided by the total number of investigated plants resulted in a lodging rate of 46.0% recognition (Jia et al., 2015).
2.4. Crop observation
The data of maize growth was observed by manual methods from planting to harvest, and the agricultural meteorological observation data was completed in 2016 and 2017 (National Meteorological Administration, 1993). After lodging, lodging data was collected according to the disaster situation in 2016.
2.4.1. Observation of growth stage
This mainly included the observation of the three-leaf stage, seven-leaf stage, jointing stage, tasseling stage, milk-ripe stage, and mature stage.
2.4.2. Determination of dry matter
The samples were taken before lodging at the three-leaf stage, seven-leaf stage, jointing stage, tasseling stage, and milk ripe stage. After lodging occurred, samples were taken and determined according to “lodging” and “not lodging” in the mature period. Four measuring points were fixed in the field, 10 plants were selected continuously from each measuring point, and the height of 40 plants at each measuring point was measured, which was divided into five groups with the same date range according to the dispersion degree of data regarding plant height. Each measuring point was sampled according to the average plant height of each group as the reference height, and one plant was taken from each reference height; five plants were taken from each measuring point, which was cut off on the ground and brought back into the room. The leaves, stem sheaths, ears, and other organs were separated and dried before weighing.
Taking lodging into account, the average dry matter accumulation at the field level was calculated as follows:
where DLE is the average dry matter accumulation at the field level, Lr is the lodging rate (46.0%), DL is the dry matter accumulation of lodging plants, and DNL is the dry matter accumulation of non-lodging plants.
2.4.3. Determination of leaf area index
We collected leaves for leaf area measurement in the same manner for dry matter sampling as mentioned above using the length-width coefficient method:
where LSi is the leaf area per plant (cm2), Li is leaf length (cm), Di is leaf width (cm), and k is the leaf area correction coefficient, which is 0.70. The leaf area index LAI was obtained by multiplying the leaf area per plant by the number of plants per square meter.
2.4.4. Yield investigation and theoretical yield calculation
At the mature period of maize, four measuring points were randomly selected, and 10 plants were taken from each measuring point based on lodging and non-lodging. Plants were cut off evenly and dried in the sun and the yield component, ear length, ear diameter, total grain weight, 100-grain weight, and grain-to-stalk ratio were analyzed. The theoretical yield was calculated as:
where YR is the theoretical yield (kg·hm−2), YNL is the yield of the non-lodging part, and YL is the yield of the lodging part. SWNL is the grain weight of a non-lodging plant (kg·plant−1). Lr is the lodging rate, which is 46.0% according to statistics. ED is the total planting density (plant·hm−2), and SWL is the grain weight of the non-lodging plant (kg·plant−1).
According to the theoretical output, the yield reduction rate was calculated as follows:
Pr is the yield reduction rate (%). YE is the expected theoretical yield (kg·hm−2).
2.5. Simulation of population photosynthesis under normal growth conditions
The crop population photosynthetic rate was equal to the difference between the total population photosynthetic rate and dark respiration rate, otherwise known as the net population photosynthetic rate, which was the simulated population photosynthetic rate under normal growth conditions:
Acm is the simulated value of the net photosynthetic rate of the population, A is the total photosynthetic rate of the population (mg m−2 s−1), and Rd is the population dark breathing rate (mg m−2 s−1), which is calculated by COLLATZ G. JAMES (Collatz et al., 1991). The total photosynthetic rate (A) of the population was simulated by the scale expansion method (Yu and Sun, 2006). Firstly, according to the modified Farquhar biochemical model of photosynthesis (Farquhar et al., 1980), the total photosynthetic rate (P) of a single leaf was calculated as:
where α is the initial quantum efficiency (μmol μmol−1), I is light intensity (μmol m−2 s−1), Pmax is the maximum photosynthetic rate (μmol m−2 s−1), θ is convexity, and the value is 0.55 μmol μmol−1.
Using the photosynthetic rate of a single leaf, it was then extended to the scale of crop population, and the single-leaf model was applied. The total photosynthetic rate of the population was as follows:
where LAIL is the daily leaf area index. LAIL is simulated by a modified Logistic equation (Peng and Yang, 2018):
where LAImax is the measured maximum leaf area index during the growth period, DS is the normalized accumulated temperature, and a, b, and c are the fitting parameters.
2.6. Estimation of the photosynthetic rate of measured population
Based on the carbon cycle theory of farmland ecosystems, the population photosynthetic rate can be calculated from the CO2 flux and soil respiration as the measured value of population photosynthesis. CO2 flux mainly includes the net photosynthesis of crops and the respiration of soil. By using the black box method, the calculation formula for the net photosynthetic rate of crop population was (Liu et al., 2015):
where Acs is the net photosynthetic rate of population (mg m−2 s−1), and Fc is CO2 flux (mg m−2 s−1), which can be observed by the flux observation system. Rs is soil respiration rate (mg m−2 s−1) which increases exponentially with the increase of soil surface temperature (Ts) (Tjkelker et al., 2001; Wang et al., 2004):
where R0 is the soil respiration rate (0.11 mg m−2 s−1) when the soil temperature is 25°C, Q10 is a constant, the value is 2.14, and Ts is 5 cm soil temperature.
2.7. Shuttleworth–Wallace dual-source model
Shuttleworth and Wallace studied the evapotranspiration of soil surface under sparse soil cover conditions and developed a dual-source model (S-W model) consisting of vegetation and soil surface under vegetation cover with the assumption that the crop canopy was uniformly covered in vegetation.
where CcPMc is transpiration of summer maize, CsPMs is the evaporation of soil, Cc is canopy coefficient, Cs is soil surface coefficient, Rn is net canopy surface radiation (W m−2), G is soil heat flux, ρis is air density (kg m−3), Cp is the specific heat of the air (1,012 J kg−1 K−1), D is air saturation vapor pressure difference (hPa), γ is the dry and wet meter constant (0.67 hPa K−1), △ is the slope of saturation vapor pressure–temperature curve (hPa K−1), is the net radiation of soil surface (W m−2), is canopy resistance (s m−1), is the resistance of the canopy inner boundary (s m−1), is the height of the crop canopy, and is aerodynamic resistance between surface and canopy height (s m−1).
2.8. Model verification
The theoretical simulation value of the population photosynthetic rate calculated by Formula (8) was verified by using the measured value of the population photosynthetic rate calculated by Formula (12). The actual value of evapotranspiration is measured by the flux observation system, and the simulated value is calculated by the S-W model. The simulation result was evaluated by using Absolute Error (AE) and Relative Error (RE). The smaller the error, the higher the simulation accuracy will be.
where AE is the absolute relative error, RE is the relative error, Si is the ith simulated value, and Oi is the ith observed value.
2.9. Estimation of dry matter accumulation
According to Richards equation, the daily growth model of maize biomass was constructed by using the measured biomass at different development stages (Zhang et al., 2018):
where y is the daily dry matter weight per unit area and the unit is g m−2, A, B, C, and D are fitting parameters, and t is the normalized accumulated temperature.
As 2016 was a lodging year, according to the sampling data of lodging and non-lodging biomass, two types of daily growth biomass were simulated.
2.10. Data processing
All the data and statistical analyses were performed using SPSS Statistics package 19.0 (SPSS Inc., Chicago, IL, USA). Microsoft Excel 2016 was used for data processing and drawing.
3. Results
3.1. The effect of lodging on photosynthesis and evapotranspiration of maize population
3.1.1. Population photosynthesis simulation
Based on the observational data of maize in the normal growth year (2017) from the milk stage to the maturity stage, the simulation model of population photosynthesis was constructed, and the parameters of the model were calibrated to simulate the photosynthetic rate of the maize population under normal growth conditions (Figure 2). The simulated values of photosynthesis of normal maize farmland population were in accordance with the observed values. There was a significant positive correlation between the simulated value and the observed value, and the correlation coefficient was 0.95. The absolute error of the simulation was 0.43 mg m−2 s−1 and the relative error was −3.3%. The results showed that the model could accurately simulate the change rate in photosynthesis of the maize population from the milk stage to the mature stage under normal growth conditions.
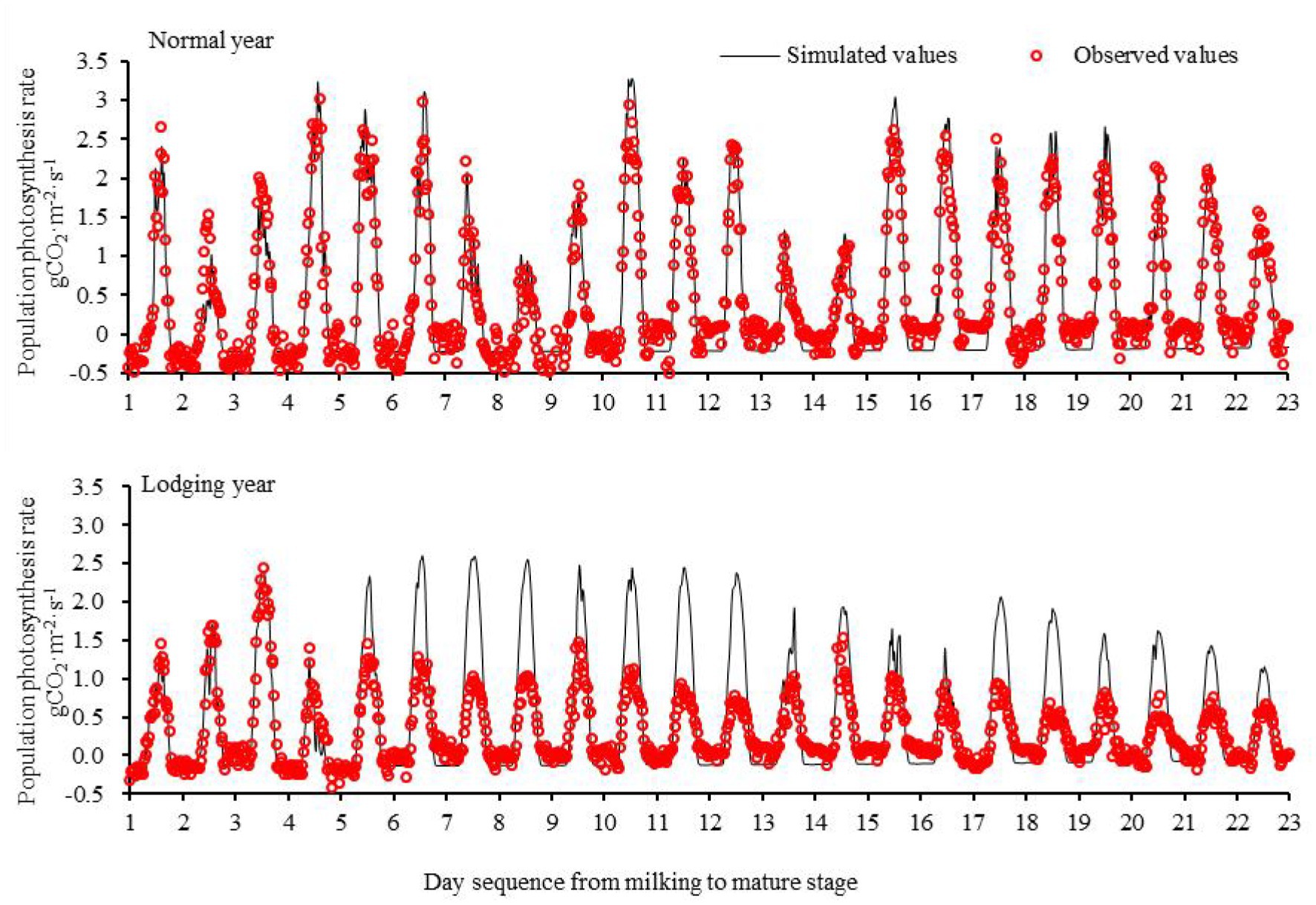
Figure 2. Population photosynthesis of maize from milking to mature stage in a normal year and lodging year.
Based on the constructed photosynthesis model of the maize population, the theoretical value of simulating the photosynthesis rate of the maize population from the milk stage to the mature stage in 2016 was taken as the control value. The differences between the measured population photosynthesis and simulated control value after lodging are shown in Figure 2. The photosynthesis of the observed population after maize lodging (August 25, 2016) was significantly lower than the simulated value, which indicated that the photosynthesis of maize farmland decreased significantly after lodging. In the period from lodging to mature harvest, the average daily photosynthesis rate decreased by 13.99 mg m−2 s−1, with an average decrease of 53%. Among the maize population, lodging was more significant under sunny conditions (sunshine hours ≥7 h), and the rates of average daily photosynthetic activity decreased by 60 cloudy days, resulting in weak photosynthesis as a whole, and the average daily photosynthetic rate decreased by 39% after lodging.
3.1.2. Simulation of evapotranspiration by the Shuttleworth–Wallace dual-source model
The S–W dual-source model was used to calculate the evapotranspiration for summer maize from the milking to maturity stage in a normal growth year type (2017; Figure 3). The simulated and measured evapotranspiration values of summer maize in a normal year were in good agreement; the correlation coefficient was 0.88, the simulated absolute error was −0.005 mm 30 min−1, and the relative error was −10.7%. The results showed that the model could accurately simulate the evapotranspiration variation from the milk stage to the maturity stage of summer maize under normal growth conditions. The S–W model was used to simulate the theoretical evapotranspiration of summer maize from the milk stage to the maturity stage in 2016 as a control, and the difference between the measured group evapotranspiration and the simulated control value after lodging was compared. After the lodging of summer maize (August 25, 2016), the measured evapotranspiration was significantly lower than the simulated value, which suggested that lodging significantly reduced the evapotranspiration of summer maize. On average, the daily evapotranspiration decreased by 1.03 mm d−1 or 28% from lodging to harvest.
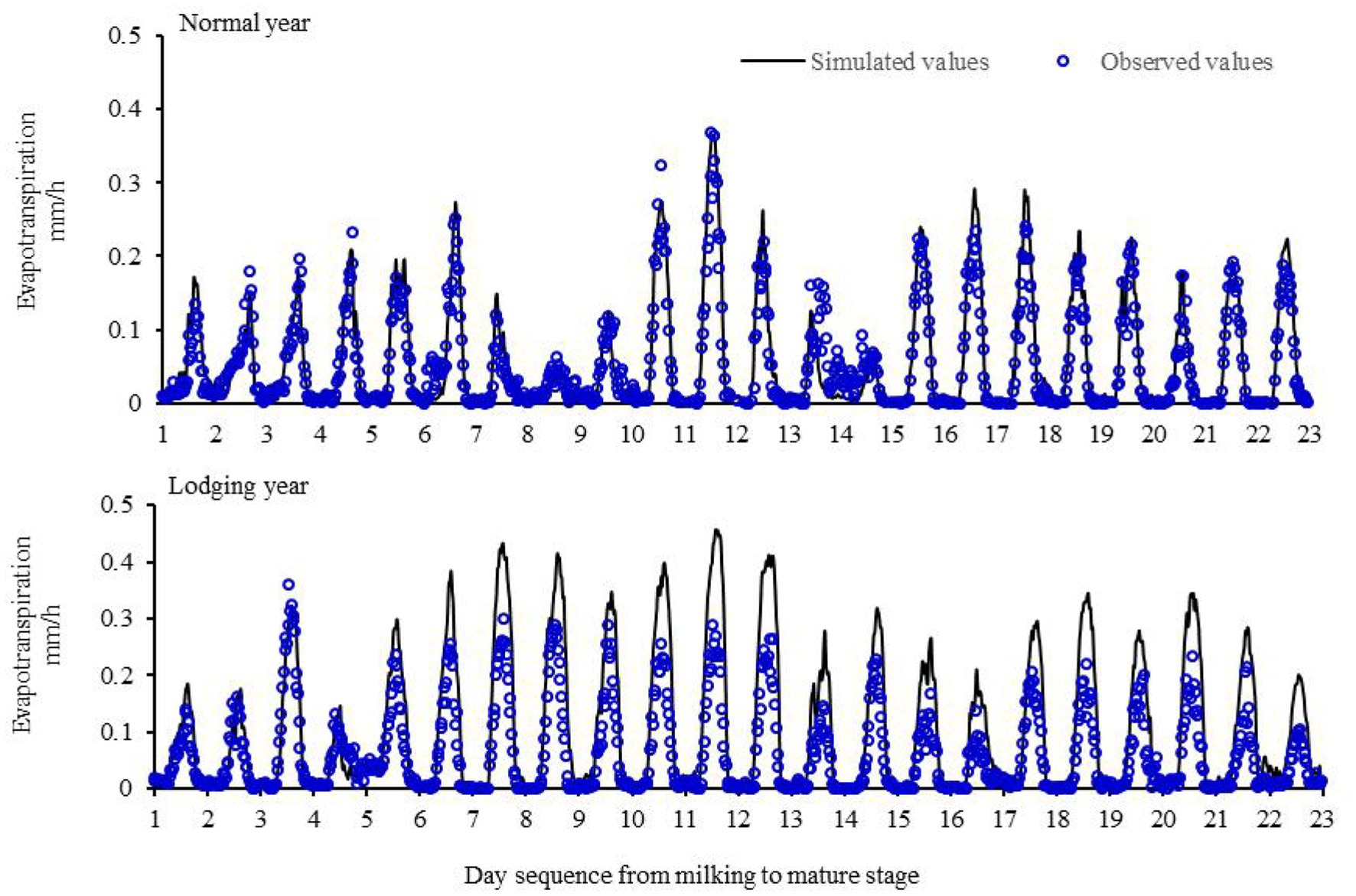
Figure 3. Evapotranspiration of maize from milking to mature stage in a normal year and lodging year.
3.2. The effect of lodging on dry matter accumulation of maize
3.2.1. Estimation of dry matter accumulation of maize after lodging based on population photosynthetic model
In the study, the dry matter accumulation was simulated using the Richards model (Formula (16)) of summer corn based on dry matter sampling data in a normal year (2017) at different growth stages. As a consequence, the measured and simulated values of population photosynthesis every half hour were converted into the cumulative values of daily photosynthesis, and the daily cumulative amounts of population photosynthesis were calculated accordingly. The data in Figure 4 shows the results of correlation analysis with the accumulated amount of dry matter over the corresponding period. Under normal growth conditions, the measured and simulated values of daily population photosynthetic accumulation were significantly positively correlated with daily dry matter accumulation. The correlation coefficient between measured and simulated values of daily population photosynthetic accumulation were 0.9977 and 0.9965, respectively, which indicated that the change of daily dry matter accumulation after the milk stage of maize could be estimated by using the accumulated value of population photosynthesis.
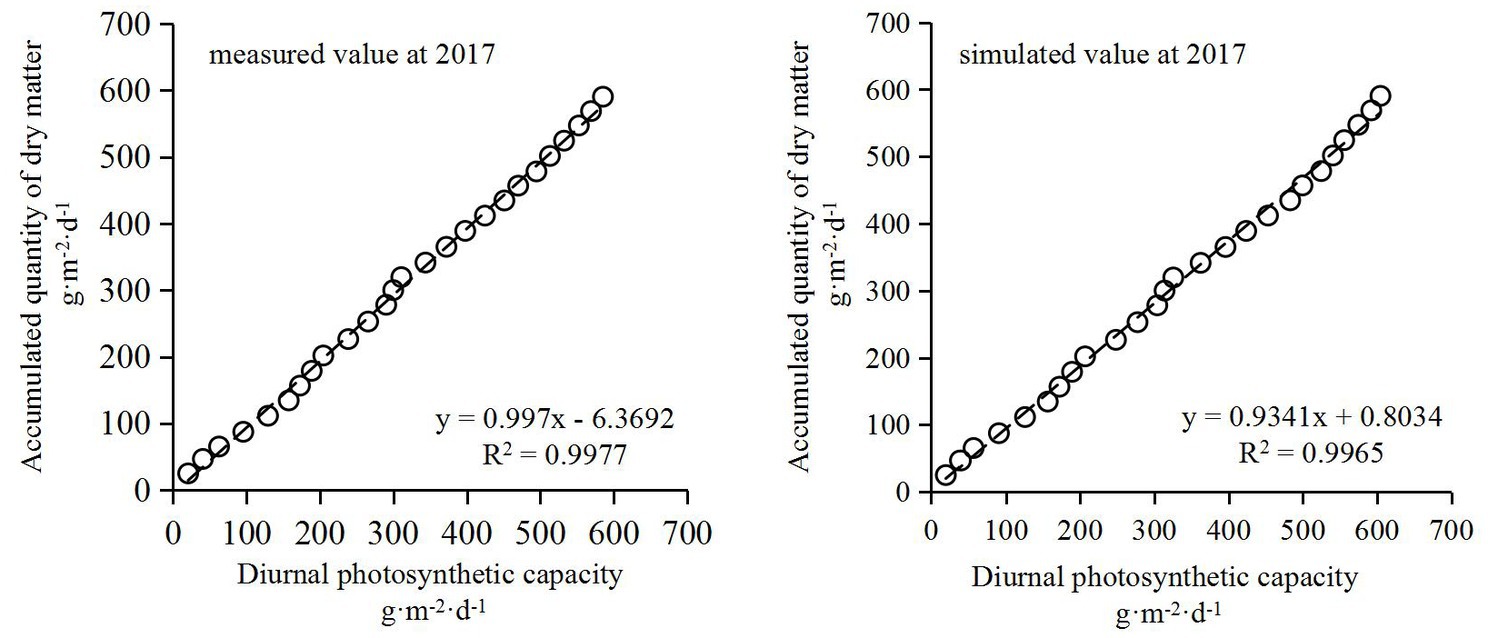
Figure 4. The fitting relationship between the accumulation of photosynthetic product and dry matter accumulation in normal summer maize after the milk stage.
Based on the positive correlation between photosynthesis accumulation and dry matter accumulation, the change in dry matter accumulation after lodging in 2016 was estimated. Taking the dry matter sampling data of non-lodging samples as the substitute for the Richards model of daily dry matter growth of maize, the daily dry matter growth of maize was simulated, which was taken as the “reference value of dry matter growth” under normal growth conditions. The calculated daily photosynthetic rate decreased in percentage after lodging, and multiplying the daily photosynthetic rate decreased percentage by the reference value of daily dry matter increase gave us the estimated amount of daily dry matter increase after lodging (Figure 5). After lodging, photosynthesis and daily average photosynthetic products decreased significantly, resulting in the decrease of dry matter accumulation. If lodging did not occur, the dry matter accumulation in the milk-ripe stage to the mature stage could increase by 368.2 g m−2. However, lodging occurred, and it was estimated that the dry matter accumulation increased by 299.6 g m−2 in the milk-ripe stage, which was 68.6 g m−2 lower than the theoretical value. Dry matter accumulation decreased by 18.6% (Table 1).
3.2.2. Dry matter accumulation of maize after lodging based on field sampling calculation
After the disaster, samples of dry matter were taken from lodging and non-lodging plants. The changes in dry matter in maize are shown in Table 2. The total dry matter weight per unit area in the mature period increased by 181.0 g m−2 (lodging) and 299.8 g m−2 (non-lodging), and the total dry matter weight of lodging plants decreased significantly by 118.8 g·m−2 (α = 0.05). According to Formula (1), the average total dry matter weight per unit area of the whole field after lodging was 245.2 g·m−2, which was 18.2% lower than the theoretical value without lodging. Moreover, the dry matter accumulation of field sampling results was basically consistent with the decreased range (18.6%) of dry matter accumulation estimated by population photosynthesis in 2.2.1.
3.3. The effect of lodging on dry matter distribution of maize
Compared with the milk-ripe period, the leaf dry weight at the mature stage decreased by 37.5 g m−2 (lodging) and 24.6 g m−2 (not lodging), and the stem sheath weight increased by 141.6 g m−2 (lodging) and 129.2 g m−2 (not lodging). The dry weight of ears in the mature stage increased by 77.0 g m−2 (lodging) and 195.2 g m−2 (not lodging) compared with the milk-ripe period, and the weight of ears in the lodging stage decreased significantly (α = 0.05) by 118.2 g m−2, with a decrease of 60.6%. It can be seen from the above that the decrease in the dry weight of the spike after lodging is the main reason for the decrease in total dry matter weight.
As a result of lodging, there was a significant decrease in dry matter accumulation and changes in the distribution ratio of dry matter in various organs (Table 2). After lodging, the dry matter partition ratio in stem and sheath increased significantly by 3.0%. The partition ratio of the ear significantly reduced by 3.0% after lodging than without lodging. The dry weight of aging leaves no longer increased because it was in the middle and late growth stages of maize, and the distribution ratio of lodging and non-lodging leaves had little difference. The total weight of maize plants decreased after lodging, the photosynthetic products were more distributed to the vegetative organs, and the dry matter accumulation in reproductive organs decreased, resulting in a decrease in maize yield.
3.4. The effect of lodging on maize yield
After harvest, samples were taken from lodging and non-lodging plants. The yield structure analysis of maize is shown in Table 3. As lodging occurred in the middle and late filling stages of maize, there was little difference in the ear length and ear diameter between lodging and non-lodging maize. After lodging, the weight of 100 grains of maize decreased by 0.88 g, which was significantly reduced by 2.8% (α = 0.05), compared with without lodging. After lodging, the total grain weight of the plant decreased by 18.0 g which was significantly lower than without lodging (α = 0.05). After lodging, the grain-to-stalk ratio of maize was significantly lower than that of non-lodging, indicating that more dry matter distribution was allocated to the stem and sheath, and the harvest index decreased. Compared with non-lodging, the theoretical yield of lodging plants decreased significantly by 1172.9 kg hm−2. The yield of a whole field decreased by 539.5 kg hm−2 when the lodging rate was about 46.0%, and the yield reduction rate was approximately 5.0% when the lodging rate was approximately 46.0%.
4. Discussion
The population photosynthesis model extends the physiological and ecological models at the leaf scale to the canopy scale by means of scale expansion, and the large leaf model, the two-leaf model, and the multi-layer model are widely used. In this study, the Farquhar biochemical model (1980), which is widely used, was extended to the canopy-scale photosynthesis model of large leaves. It is generally believed that the hypothesis of the big leaf model is basically valid when the leaf area index (L ≥ 3) of the canopy is taken into account (Ren et al., 2004). At present, canopy-scale photosynthesis models mostly focus on simulating crop responses to light, temperature, water, CO2, and other environmental factors, ignoring the simulation of the physiological and ecological processes that allow crops to adapt to the environment, and thus, the models cannot predict how crops will respond to the environment under adverse conditions. In this study, based on two aspects of crop photosynthesis and ecosystem carbon cycle combined with CO2 flux observation data of the vorticity correlation system, the characteristic changes of group photosynthesis of maize during normal growth from milk maturity to maturity were compared and analyzed by two methods, and the model parameters were calibrated. In 2017, the simulated values of milk maturity to maturity in the normal growing season of maize were consistent with comparative values, and the change characteristics of group photosynthesis after lodging in maize in 2016 were further analyzed.
Photosynthesis is the source of dry matter accumulation and yield formation in maize, and the accumulation of photosynthetic products is generally calculated using dry matter accumulation through a model. The CO2 assimilation module in the WOFOST model calculates the parameters of photosynthesis cumulative conversion to dry matter mass. Lizaso et al. (2005) introduced CO2 flux observation data and used the CERES-Maize model to simulate the changes in dry matter accumulation and parameter sensitivity at the canopy scale, obtaining good simulation results. After maize lodging in 2016, the accumulation of photosynthetic products and dry matter decreased. In addition, a previous study showed that (Liu et al., 2019) parameter correction was necessary for calculating the conversion of photosynthesis accumulation of other plants into dry matter mass of plants, and the correction coefficient was not fixed, so a certain error occurred when calculating the daily dry matter accumulation through the decrease percentage of photosynthetic rate in section (2.2.1). The accumulated amount of dry matter after lodging was reduced by 18.6% calculated by daily photosynthetic rate reduction percentage, and by field sampling, the accumulated amount of dry matter after lodging was reduced by 18.2%. Although there were some errors, they were consistent.
Lodging changes the cumulative distribution of dry matter in maize. Under normal growth conditions, the vegetative organs of maize generally stop growing at the late growth stage, and the increase of dry matter in the population is mainly manifested in the accumulation of grains. Several studies have shown that the stem is the main organ for the redistribution of photosynthetic products, and the redistribution amount of stem dry matter can reach 35% of its dry weight during grain filling (Ma and Zhou, 2016). This study shows that after maize lodging, not only the photosynthesis ability of plants decreases but the accumulation of total dry matter also decreases. Moreover, the cumulative distribution law of dry matter changes, and the plant grows uprightly and in reverse, and some dry matter transfers from ear to stem (Li et al., 2015a). Several studies have shown that the earlier lodging resulted in serious yield reduction (Li et al., 2015b). On the other hand, adversity accelerates the senescence of crops, which is beneficial for their reproductive growth process, thus avoiding adversity stress and maintaining population stability (Gao et al., 2017). In this study, maize lodging occurred at the milking stage and did not have any significant effect on grain number per ear, ear length, ear diameter, and leaf dry matter distribution. There was no significant effect of lodging on yield, but it did have an effect on grain weight per ear and 100-grain weight.
The photosynthesis model of the maize population has a strong mechanism, but there are many model parameters that make it more difficult to calculate the model accurately (Zhnag et al., 2001; Zhang, 2016; An et al., 2021). Meanwhile, crop dark respiration and soil respiration are regarded as functions only depending on air temperature and soil temperature, respectively, but the model parameters are too simple, resulting in the accumulation of simulation errors (Sun et al., 2013; Chen et al., 2017; Shah et al., 2017). In addition, due to the sudden and sporadic wind lodging disaster and the poor repeatability of natural lodging, there is still a lack of observation data after large-scale lodging to verify the simulation results of population photosynthesis.
5. Conclusion
The simulation model of population photosynthesis and evapotranspiration under normal growth conditions of maize was constructed by using the Eddy-Covariance system and crop observation data during the period from milk maturity to maturity in 2017 without lodging, and the model was verified by the measured population photosynthesis calculated by CO2/H2O flux observation data. The results showed that the absolute error of the population photosynthesis model simulation was −0.43 mg m−2 d−1 and the relative error was −3.3% under the normal growth of maize. Under normal growth conditions, the simulated values of photosynthesis were in good agreement with the measured values. The verified model was used to simulate the theoretical population photosynthesis of maize in the lodging year (2016). Compared with the calculated measured population photosynthesis, the average daily photosynthesis decreased by 13.99 mg m−2 s−1 and 53% after lodging.
Lodging reduced photosynthesis, evapotranspiration, and decreased dry matter accumulation. The dry matter accumulation of maize simulated by the Richards model was positively correlated with the population photosynthesis accumulation. The dry matter accumulation of lodging year (2016) was estimated from the population photosynthesis accumulation, and the dry matter accumulation decreased by 18.6%.
Lodging changed the distribution ratio of dry matter in maize. After lodging, the stem and sheath dry weight of maize increased by 5.5% and ear weight decreased by 10.9%, but there was no significant effect on the leaf dry weight. After lodging, the partition ratio of stem and sheath increased by 3.0% and the partition ratio of ear decreased by 3.0%. After lodging, the 100-grain weight significantly decreased by 2.8%, and the grain weight per plant decreased by 10.8%. Calculating theoretical yield according to lodging rate and density, lodging resulted in a yield reduction rate of approximately 5.0% for the whole field.
Experts have conducted extensive research on crop lodging, but the lodging process of summer maize is very complex, for example, various factors such as high or low stems. There are also meteorological factors such as strong winds or heavy precipitation and planting factors such as excessive planting density or unreasonable field management. The meteorologist, agronomists, physiologists, molecular actors, and breeders require further exploration to fix this challenging problem.
Data availability statement
The original contributions presented in the study are included in the article/supplementary materials, further inquiries can be directed to the corresponding authors.
Author contributions
JP is the first author. SL and WM are the corresponding authors. LL and MN have modified the grammar of the full text. WJ has provided technical guidance for the article. All authors contributed to the article and approved the submitted version.
Conflict of interest
The authors declare that the research was conducted in the absence of any commercial or financial relationships that could be construed as a potential conflict of interest.
Publisher’s note
All claims expressed in this article are solely those of the authors and do not necessarily represent those of their affiliated organizations, or those of the publisher, the editors and the reviewers. Any product that may be evaluated in this article, or claim that may be made by its manufacturer, is not guaranteed or endorsed by the publisher.
References
Alam, S., Aaqil, K., Umair, A., Liu, H. H., and Li, J. C. (2018). Characterization of the effect of increased plant density on canopy morphology and stalk lodging risk. Front. Plant Sci. 9, 1–12. doi: 10.3389/fpls.2018.01047
An, S., Tanveer, M., Abbas, A., Yildirim, M., Aa, S., Mi, A., et al. (2021). Combating dual challenges in maize under high planting density: stem lodging and kernel abortion. Front. Plant Sci. 12:699085. doi: 10.3389/fpls.2021.699085
Cao, Q. J.. Evaluation of stalk lodging resistance of different spring maize genotypes and mechanism of its improvement by plant growth regulators (Pgrs) [D]. University Of Chinese Academy Of Sciences (Northeast Institute Of Geography And Agroecology), (2016), 23–29. (In Chinese)
Chen, S. H., Chen, H. L., Shen, X. S., Wang, C. T., Zhang, Y. L., and Liu, D. H. (2012). Effects of planting density and nitrogen application on yield and lodging of mechanized sowing maize. Southwest China J. Agri. 25, 805–808. doi: 10.16213/j.cnki.scjas.2012.03.031 (In Chinese)
Chen, S. T., Zou, J. W., and Hu, Z. H. (2017). Measurements and modeling of soil respiration in terrestrial ecosystems. Ecol. Environ. Sci. 26, 1985–1996. doi: 10.16258/j.cnki.1674-5906.2017.11.020 (In Chinese)
Collatz, G., James, B. J., Timothy, G. C., and Berry, J. A. (1991). Physiological and environmental regulation of stomatal conductance, photosynthesis and transpiration: a model that includes a laminar boundary layer. Agricultural & Forest Meteorology, 54, 107–136. doi: 10.1016/0168-1923(91)90002-8
Elmore, R. W., and Ferguson, R. B. (1999). Mid-season stalk breakage in corn: hybrid and environmental factors. J. Prod. Agric. 12, 293–299. doi: 10.2134/jpa1999.0293
Falge, E., Baldocchi, D., Olson, R., and Anthoni, P. (2001). Gap-filling strategies for defensible annual sums of net ecosystem exchange. Agric. For. Meteorol. 107, 43–69. doi: 10.1016/S0168-1923(00)00225-2
Farquhar, G. D., Von Caemmere, S., and Berry, J. A. (1980). A biochemical model of photosynthetic Co2 assimilation in leaves of C3 species. Planta 149, 78–90. doi: 10.1007/BF00386231
Gao, J., Shi, J. G., Dong, S. T., Liu, P., Zhao, B., and Zhang, J. W. (2017). Effect of different light intensities on root characteristics and grain yield of maize (Zea Mays L.). Sci. Agric. Sin. 50, 2104–2113. doi: 10.3864/j.issn.0578-1752.2017.11.016 (In Chinese)
Guo, J. X., Bian, L. G., and Dai, Y. J. (2007). Measured Co2 concentration and flux at 16m height during corn growing period on the North China plain. Chin. J. Atmos. Sci. 31, 695–707. doi: 10.3878/j.issn.1006-9895.2007.04.14 (In Chinese)
Jia, H. L., Wang, G., Guo, M. Z., Shah, D., Jiang, X. M., and Zhao, J. L. (2015). Methods and experiments of obtaining corn population based on machine vision. Trans. Chin. Soc. Agri. Eng. 31, 215–220. doi: 10.3969/j.issn.1002-6819.2015.03.028 (In Chinese)
Jun, X., Ling, G., Yingshan, Z., Hesheng, Y., Jingshan, T., and Wangfeng, Z. (2016). Effects of light intensity within the canopy on maize lodging. Field Crop Res. 188, 133–141. doi: 10.1016/j.fcr.2016.01.003
Jun, X., Rui-Zhi, X., Wang-Feng, Z., Ke-Ru, W., Peng, H., Bo, M., et al. (2017). Research Progress on reduced lodging of high-yield and-density maize. J. Integr. Agric. 16, 2717–2725. doi: 10.1016/S2095-3119(17)61785-4
Kang, M. S., Kushairi Din, A., Zhang, Y., and Magari, R. (1999). Combining ability for rind puncture resistance in maize. Crop Sci. 39, 368–371. doi: 10.2135/cropsci1999.0011183X0039000200011x
Li, C., He, H. L., Liu, M., Su, W., Fu, Y. L., Zhabg, L. M., et al. (2008). The design and application of Co2 flux data processing system at China flux. Geo-Inf. Sci. 10, 557–565. (In Chinese)
Li, S. Y., Ma, W., Peng, J. Y., and Chen, Z. M. (2015a). Study on yield loss of maize due to lodging at the big flare stage and grain filling stage. Sci. Agri. Sin. 19, 3952–3964. doi: 10.3864/j.issn.0578-1752.2015.19.017 (In Chinese)
Li, S. Y., Wang, Y. X., Hu, C. D., and Yan, Y. (2015b). Effect of strong wind lodging at pre-and post-tasseling stages on growth and yield of maize. Chin. J. Appl. Ecol. 26, 2405–2413. doi: 10.13287/j.1001-9332.20150521.018 (In Chinese).
Liu, Y., Chen, P. M., and Chen, J. N. (2015). Progress and perspectives in studies on agro-ecosystem carbon cycle model. Trans. Chin. Soc. Agri. Eng. 31, 1–9. doi: 10.3969/j.issn.1002-6819.2015.03.001 (In Chinese)
Liu, Q., Xie, L. F., and Li, F. R. (2019). The spatial distribution of the needle area of planted Larix Olgensis trees. Forests 10, 1–17. doi: 10.3390/f10010028
Lizaso, J. I., Batchelor, W. D., Boote, K. J., and Westgate, M. E. (2005). Evaluating a leaf-level canopy assimilation model linked to Ceres-maize. Agron. J. 97, 734–740. doi: 10.2134/agronj2004.0172
Ma, X. Y., and Zhou, G. S. (2016). Maize biomass simulation based on dynamic Photosynthate allocation. Chin. J. Appl. Ecol. 27, 2292–2300. (In Chinese). doi: 10.13287/j.1001-9332.201607.026
Minami, M., and Ujihara, A. (1991). Effects of lodging on dry matter production, grain yield and nutritional composition at different growth stages in maize (Zea Mays L.). Japan. J. Crop Sci. 60, 107–115. doi: 10.1626/jcs.60.107
National Bureau Of Statistics Of China. China Statistical Yearbook. Beijing: China Statistics Press, (2018): 401–405. (In Chinese)
National Meteorological Administration. Agricultural Meteorological Observation Specifications (Volume I). Beijing: Meteorological Press (1993): 7–30. (In Chinese)
Peng, J. Y., and Yang, G. X. (2018). Improvement of evapotranspiration models in maize field and its sensitivities analysis to the resistance parameters. Agric. Res. Arid Areas. 36, 55–62. doi: 10.7606/j.issn.1000-7601.2018.02.09 (In Chinese)
Ren, C. Y., Yu, G. R., Wang, Q. F., and Guan, D. X. (2004). Study on coupling model of photosynthesis and transpiration of ecosystem at canopy scale. Sci. China Ser. D Earth Sci. 34, 142–151. In Chinese
Shah, A. N., Tanveer, M., Rehman, A. U., Anjum, S. A., Iqbal, J., and Ahmad, R. (2017). Lodging stress in cereal—effects and management: An overview. Environ. Sci. Pollut. Res. Vol. 24, 5222–5237. doi: 10.1007/s11356-016-8237-1
Sun, J. W., Yuan, F. H., Guan, D. X., and Wu, J. B. (2013). Dark respiration of terrestrial vegetations: a review. Chin. J. Appl. Ecol. 24, 1739–1746. (In Chinese).
Tjkelker, M. G., Oleksyn, J., and Reich, P. B. (2001). Modelling respiration of vegetation: evidence for a general temperature-dependent Q10. Glob. Chang. Biol. 7, 223–230. doi: 10.1046/j.1365-2486.2001.00397.x
Vickers, D., and Mahrt, L. (1997). Quality control and flux sampling problems for tower and aircraft data. J. Atmos. Ocean. Technol. 14, 512–526. doi: 10.1175/1520-0426(1997)014<0512:QCAFSP>2.0.CO;2
Wang, J. (2006). Study on the experiment and simulation of crop growth and water heat and Co2 transfer in agro-ecosystem. Beijing, Chin. Acad. Sci., 78–90. (In Chinese)
Wang, X., Li, Y., Han, W., Song, Z., Wang, S., and Yang, J. (2022). Evaluation of root lodging resistance during whole growth stage at the plant level in maize[J]. Sci. Rep. 12:10375. doi: 10.1038/s41598-022-14159-0
Wang, H. L., Wu, R. H., Zhu, K., Zhang, Y. C., Zhang, Y. J., and Sun, J. W. (2011). Reviews of causes and control of maize lodging. J. Henan Agri. Sci. 40, 1–5. doi: 10.15933/j.cnki.1004-3268.2011.10.015 (In Chinese)
Wang, J., Yu, Q., Li, X. G., Sun, X. M., and Zhu, Z. L. (2004). Diurnal variation of winter wheat water and heat fluxes of a simulation with photosynthesis-evapotranspiration coupled model. Chin. J. Appl. Ecol. 11, 2077–2082. (In Chinese)
Xu, Z. W., Liu, S. M., Gong, L. J., Wang, J. M., and Li, X. W. (2008). A study on the data processing and quality assessment of the Eddy covariance system. Adv. Earth Sci. 23, 357–370. doi: 10.3321/j.issn:1001-8166.2008.04.005 (In Chinese)
Xue, J., Qi, B., Ma, B., Li, B., and Gou, L. (2021). Effect of altered leaf angle on maize stalk lodging resistance[J]. Crop Sci. 61, 689–703. doi: 10.1002/csc2.20284
Yoon-Sup, S., Adetimirin Victor, O., and Soon-Kwon, K. (2013). Observational study on the recovery from root lodging at flowering time and yield reduction in maize (Zea Mays L.). Plant Breeding Biotechnol. 1, 171–177. doi: 10.9787/PBB.2013.1.2.171
Yu, G. R., and Sun, X. M.. Principles of Fluxes Measurement in Terrestrial Ecosystems. Beijing: Higher Education Press, (2006): 316–324. (In Chinese)
Yu, Q., Wang, T. D., Liu, J. D., and Sun, S. F. (1998). A mathematical study on crop architecture and canopy photosynthesis I. Model. Acta Agronomica Sinica 24, 7–15. (In Chinese)
Zhang, Z. (2016). Simulation on Hulunbeier meadow steppe ecosystem carbon cycle dynamic and future climate scenario analysis. Chin. Acad. Agri. Sci., 1–10. (In Chinese)
Zhang, J. Y., Liu, S., Song, C. Y., Gao, J. L., and Sun, L. J. (2009). Cause analysis and prevention measures of maize lodging. Shandong Agric. Sci. 11, 119–121. (In Chinese)
Zhang, D., Wang, J. C., Chen, H., Hunag, Z. J., and Sun, T. (2018). Effect of density on dry matter accumulation of maize and establishment of normalized simulation model. J. Maize Sci. 026, 58–64. doi: 10.13597/j.cnki.maize.science.20180510 (In Chinese)
Zhang, Y. Q., Yu, Q., Liu, C. M., and Wang, J. (2004). Coupled simulation of vegetation photosynthesis, canopy conductance and evapotranspiration. Sci. China Ser. D Earth Sci. 34, 152–160. (In Chinese)
Keywords: maize lodging, CO2 flux, evapotranspiration, population photosynthesis, dry matter partition
Citation: Peng J, Lu L, Noor MA, Li S, Ma W and Wang J (2023) Mid-season lodging modulates photosynthesis, evapotranspiration, and dry matter accumulation and distribution simulated by the optimized model in maize. Front. Ecol. Evol. 11:1178609. doi: 10.3389/fevo.2023.1178609
Edited by:
Guoqi Wen, Laval University, CanadaReviewed by:
Wang Huasen, Zhejiang Agriculture and Forestry University, ChinaYahui Guo, Beijing Normal University, China
Copyright © 2023 Peng, Lu, Noor, Li, Ma and Wang. This is an open-access article distributed under the terms of the Creative Commons Attribution License (CC BY). The use, distribution or reproduction in other forums is permitted, provided the original author(s) and the copyright owner(s) are credited and that the original publication in this journal is cited, in accordance with accepted academic practice. No use, distribution or reproduction is permitted which does not comply with these terms.
*Correspondence: Shuyan Li, lsy_126com@126.com; Wei Ma, mawei02@caas.cn