- 1College of Life Sciences, Zhejiang Normal University, Jinhua, China
- 2Management Department of Maolan National Nature Reserve, Libo, China
When measuring plant functional traits across geomorphologies, 5–10 healthy individuals of a plant species are commonly sampled. However, whether more individuals should be sampled in habitat-heterogeneous karst vegetation remains unknown. In this study, two dominant tree species (Clausena dunniana and Platycarya strobilacea) in karst evergreen and broadleaved mixed forests in Southwestern China were selected. On the basis of a large quantity of individuals of the two species grown in different peak clumps and slope positions, variations of 10 morphological traits in the two species were statistically analyzed. The suggested sampling number of individuals, which could mostly represent the common trait characteristics, was further explored. All traits showed significant differences between the two species (p < 0.05). The traits of P. strobilacea displayed larger intraspecific variations than those of C. dunniana, except for twig dry matter content. The bark thickness (BT), leaf area (LA), and specific leaf area (SLA) of C. dunniana and the BT, SLA, LA, leaf tissue density, and bark tissue density of P. strobilacea presented large intraspecific variations. Most traits exhibited significant differences between peak clumps and/or among slope positions (p < 0.05). Random sampling analysis indicated that the suggested sampling numbers of individuals for the 10 traits are 6–23 in C. dunniana and 9–29 in P. strobilacea. The common accepted sample size in normal geomorphologies is not sufficiently large in most cases. Larger sample sizes are recommended for traits, such as SLA, BT, and LA, with larger intraspecific variations. Therefore, under sufficient labor, material, and time, more individuals should be sampled when measuring plant functional traits in habitat-heterogeneous karst vegetation.
1. Introduction
Plant functional traits (PFTs) are the morphological and physiological characters highly connected with the survival, growth, reproduction, and death dynamics of plants (Violle et al., 2007). PFTs, especially the morphological traits such as leaf area (LA), bark thickness (BT), and wood density, are easy to measure and could reveal the relationships between plants and environments; For example, plants grow in harsh environments display small leaves, thick barks, and hard woods (Wright et al., 2004; Liu et al., 2022; Kambach et al., 2023). The study of PFTs offers an alternative approach to comprehend community species coexistence and predict the effects of global environmental changes on plant distribution (Thuiller et al., 2004; Kraft et al., 2008; Cui et al., 2020). Currently, the morphological traits of leaves, barks, and twigs (especially leaves) of various vegetation types in diverse geomorphologies worldwide have been considerably explored (Wright et al., 2010; Auger and Shipley, 2013; Myers-Smith et al., 2019; Jin et al., 2023; Liu et al., 2023).
When measuring PFTs in the field across geomorphologies, 5–10 healthy individuals of a species are commonly selected, and leaf, bark, twig, and root samples are further collected from the selected individuals (Cornelissen et al., 2003; Pérez-Harguindeguy et al., 2013). However, PFTs vary among species (interspecific variation) and individuals within a species (intraspecific variation). Interspecific trait variations are determined by species identity (genetic factors), and intraspecific trait variations are more influenced by environmental factors (Weiher and Keddy, 1995; Westoby et al., 2002; Bolnick et al., 2011). Plant scientists generally agree that PFTs show large interspecific variations among species (Cornelissen et al., 2003; Wright et al., 2004). In the last decade, a growing body of studies suggested that intraspecific trait variations could not be ignored (Albert et al., 2010; Auger and Shipley, 2013; Niu et al., 2020). Climate, soil, altitude, aspect, illumination, and many other environmental factors all cause intraspecific trait variations (Hultine and Marshall, 2000; McDonald et al., 2003; Peppe et al., 2011). Plants of a certain species growing in diverse environments may present large intraspecific trait variations (Liu et al., 2023). A global meta-analysis indicated that intraspecific trait variations occupy an average of 32% of the total trait variations (Siefert et al., 2015). Intraspecific trait variations may even be larger than interspecific trait variations in some studies (Lecerf and Chauvet, 2008; Messier et al., 2010). Thus, the traits of different species and those of individuals within a species growing in different environments vary inevitably. The trait characteristics (especially for traits with large interspecific and intraspecific variations) may be accompanied by much uncertainty on the basis of a unified sample individual number without regard to species identity and environmental conditions.
Karst is a fantastically particular geomorphology formed from the solution of carbonate rocks (such as limestone and dolomite) by underground and surface water. This geomorphology is sporadically distributed over the world, with an area of 22 million km2, occupying approximately 14.8% of the global land area (Jiang et al., 2014). Southwestern China, Southern America, and the Mediterranean coast of Europe are the three major areas with widespread distribution of karst geomorphology (Sweeting, 1972). Due to long-range geologic functions of weathering, denudation, and leaching, the karst geomorphology in Southwestern China is well known for its high habitat heterogeneity. Karst mountain areas have various landforms, such as dissolved gully, stone bud, peak clump, peak forest, isolated peak, doline funnel, and solution depression (Song, 2000). Rock exposure, soil depth and nutrients, light intensity, and water conditions relatively differ among mountains and slope positions within a mountain (Pan, 2003; Peng et al., 2019; Liu et al., 2021). Researchers have studied the PFT characteristics and PFT-based adaptation strategies of karst plants and plant communities in this region and found that PFTs, such as morphological traits and ecological stoichiometry, in karst plants presented large interspecific and intraspecific variations due to highly heterogeneous environments/habitats (Jiang et al., 2016; Geekiyanage et al., 2018; Yang et al., 2020; Liu et al., 2022).
However, most PFT studies conducted in karst geomorphology in Southwestern China sampled a similar small individual number of plants (for example, five plant individuals were sampled) in normal geomorphologies (Pang et al., 2019; Yu et al., 2021; Shui et al., 2022). The results from such sampling method may not indicate the entire trait characteristics in plants, and plant communities grow in considerably heterogeneous environments/habitats. For example, plants grow in stone gullies, on stone surfaces and soil surfaces in a karst forest presented significantly different leaf trait values (Zhou et al., 2022). Thus, researchers may raise a question of whether more individuals should be sampled when measuring PFTs in habitat-heterogeneous karst regions.
In this study, two dominant tree species (an evergreen species and a deciduous species) in habitat-heterogeneous primary forests in peak clump depression karst geomorphology in Southwestern China were used as samples. The morphological traits of leaf, bark, and twig in a large quantity of individuals of the two tree species were measured. Trait differences between peak clumps and among slope positions within a mountain were analyzed. This study aimed to answer whether more individuals should be sampled when measuring PFTs of tree species in habitat-heterogeneous karst forests. It could provide theoretical support for the sampling quantity of PFT measurements in karst regions in future studies.
2. Materials and methods
2.1. Study area
Peak clump depression karst geomorphology is one of the eight karst geomorphological types in Southern China and mainly distributed in Southern Guizhou Province and Northern Guangxi Autonomous Region. Libo County, located in Southern Guizhou, is one of the representative areas of the region, especially its Maolan National Nature Reserve (107°52′–108°05′ E, 25°09′–25°21′ N) with an area of 21,285 km2 (Figure 1). This terrain is located in mid-subtropical China and has a humid monsoon climate. According to records from the Libo meteorological station (107°53′ E, 25°25′ N, 429 m), the mean annual temperature is 18.3°C, with temperatures of 8.5°C and 26.5°C in January and July, respectively. The mean annual precipitation is 1,269 mm, of which 86% falls between April and October. The mean annual sunshine duration is 1,273 h, with a low sunshine percentage of 26%. The altitude of the reserve is between 430 and 1,078 m above sea level, with a relative height of most hills at 100–200 m. Limestone and dolomite (especially the former) are distributed everywhere in the reserve. Black limestone soil is shallow and discontinuous. The continuous evergreen and deciduous broadleaved mixed forests in this reserve are the most widely distributed, best protected, and most original karst forest in the world. The forests here are known as the gene bank of biological resources with the best preserved biodiversity in the subtropical karst geomorphology. Primary evergreen and deciduous broadleaved mixed forests are distributed in the peak clumps, and rice fields are the key land use in the depressions.
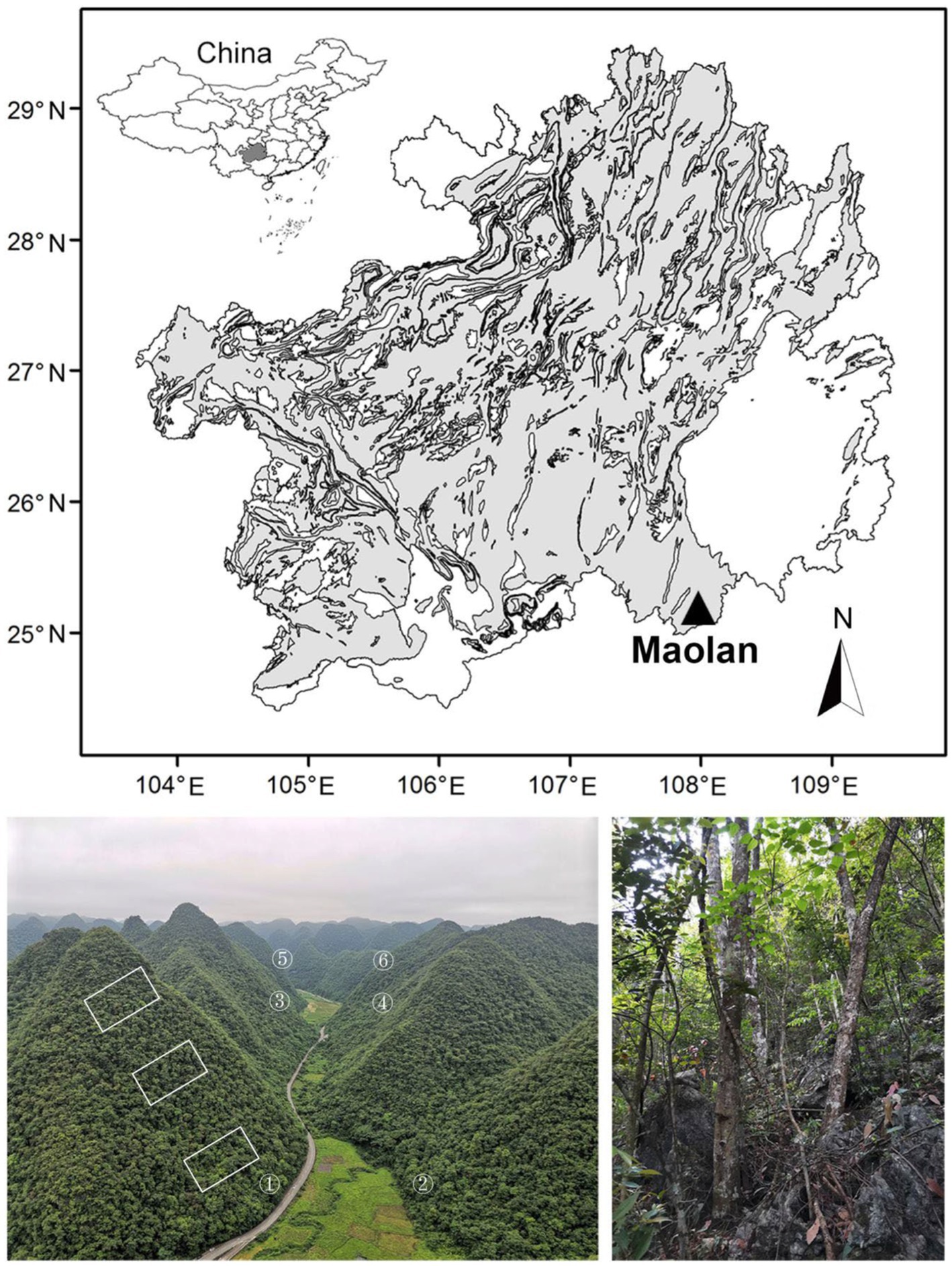
Figure 1. Location of Maolan National Nature Reserve, physiognomy of peak clump depression karst geomorphology and plot setting (left bottom), and interior view of karst forests (right bottom) in the distribution map of karst geomorphology (grey) in Guizhou Province, Southwestern China.
2.2. Vegetation survey and plant functional trait measurements
After a vegetation survey in the reserve was completed, six mountains in two opposite peak clumps (three mountains on each side peak clumps separated by a depression) at Poling site (107°56′ E, 25°17′ N) representing the karst peak clump depression geomorphology, vegetation, and soil of the whole reserve were selected. In each mountain, three plots (each with an area of 20 m × 30 m) at the upper, middle, and lower slopes (18 plots in total) were established (Figure 1). The species identity, diameter at breast height (DBH), and height of each woody plant with DBH ≥ 1 cm were recorded. Clausena dunniana, Platycarya strobilacea, Cyclobalanopsis glauca, Lindera communis, and Swida parviflora are the dominant tree species of the primary forests.
Habitats, such as slope, aspect, rock exposure rate, and soil depth and nutrient, are highly heterogeneous between peak clumps and among slope positions (Pan, 2003; Peng et al., 2019; Liu et al., 2021). Two typical dominant karst tree species, C. dunniana and P. strobilacea, distributed in all plots were selected as samples to explore the effects of highly heterogeneous habitats on PFTs and whether more individuals should be sampled when measuring PFTs of tree species in karst forests. Ten healthy individuals per species in each plot were randomly sampled (180 individuals of each tree species were sampled). Four branches in different positions of the sunlit side of the tree canopy in each sampled individual were cut using a long reach chain saw. Twenty healthy mature leaves and three approximately 20 cm-length terminal twigs were collected from the four branches by using a scissor. Three bark samples at the DBH position of each individual were collected by using a sickle.
Fresh and dry (dried at 85°C for 72 h in an oven) masses of leaf, bark, and twig (peeled) samples were weighed using an electronic balance. Leaf thicknesses (LTs) and BT were measured using an electronic Vernier caliper. LA was scanned using the WinFOLIA multipurpose leaf area meter (Regent Instruments, Canada). The volumes of bark and twig samples were determined using the drainage method, and those of leaf samples were calculated as the product of LA and LT. The values of specific leaf area (SLA), leaf dry matter content (LDMC), leaf tissue density (LTD), bark dry matter content (BDMC), bark tissue density (BTD), twig dry matter content (TDMC), and twig tissue density (TTD) were calculated using their corresponding equations (Cornelissen et al., 2003).
2.3. Data analysis
All statistical analyses were performed using SPSS version 20 and the R software version 3.5.1 (Xue, 2017; R Core Team, 2018). The coefficients of intraspecific variation (standard deviation divided by mean times 100%) were used to characterize the varying degrees of PFTs within a species. Independent sample t-test was conducted to determine trait differences between the two species and between peak clumps. One-way ANOVA was used to analyze trait differences among slope positions. Sampling without replacement (2,000 times of duplicate sampling under each sample size from two to the suggested sample individual number) was conducted for each trait (each trait of each species had 180 values from 180 individuals, Supplementary Table S1) by using the “Sample” package in the R software to determine the suggested individual numbers when measuring the PFTs of tree species in karst forests. Then, independent sample t-test was conducted to determine trait differences between object samples and total samples, and the corresponding p values were obtained (2,000 p values were obtained for each trait). When the number of p > 0.05 exceeded 1,900 of 2,000 times p values (95%), this sample size is the suggested sample individual number for the trait of this tree species (Gao et al., 2018).
3. Results
All traits showed significant differences between the two species (p < 0.05). C. dunniana presented significantly higher LT, LA, BDMC, BTD, TDMC, and TTD values and significantly lower SLA, LDMC, LTD, and BT values than P. strobilacea (p < 0.05). The coefficients of intraspecific trait variation of C. dunniana ranged from 6.32% to 35.95%. The BT, LA, and SLA of C. dunniana showed large intraspecific variations, indicated by large coefficients of intraspecific variation (Figure 2). The coefficients of intraspecific trait variation of P. strobilacea ranged from 8.13% to 45.62%. The BT, SLA, LA, LTD, and BTD of P. strobilacea displayed large intraspecific variations, indicated by large coefficients of intraspecific variation (Figure 2). In general, the traits of P. strobilacea displayed larger intraspecific variations than those of C. dunniana, except for TDMC (Figure 2).
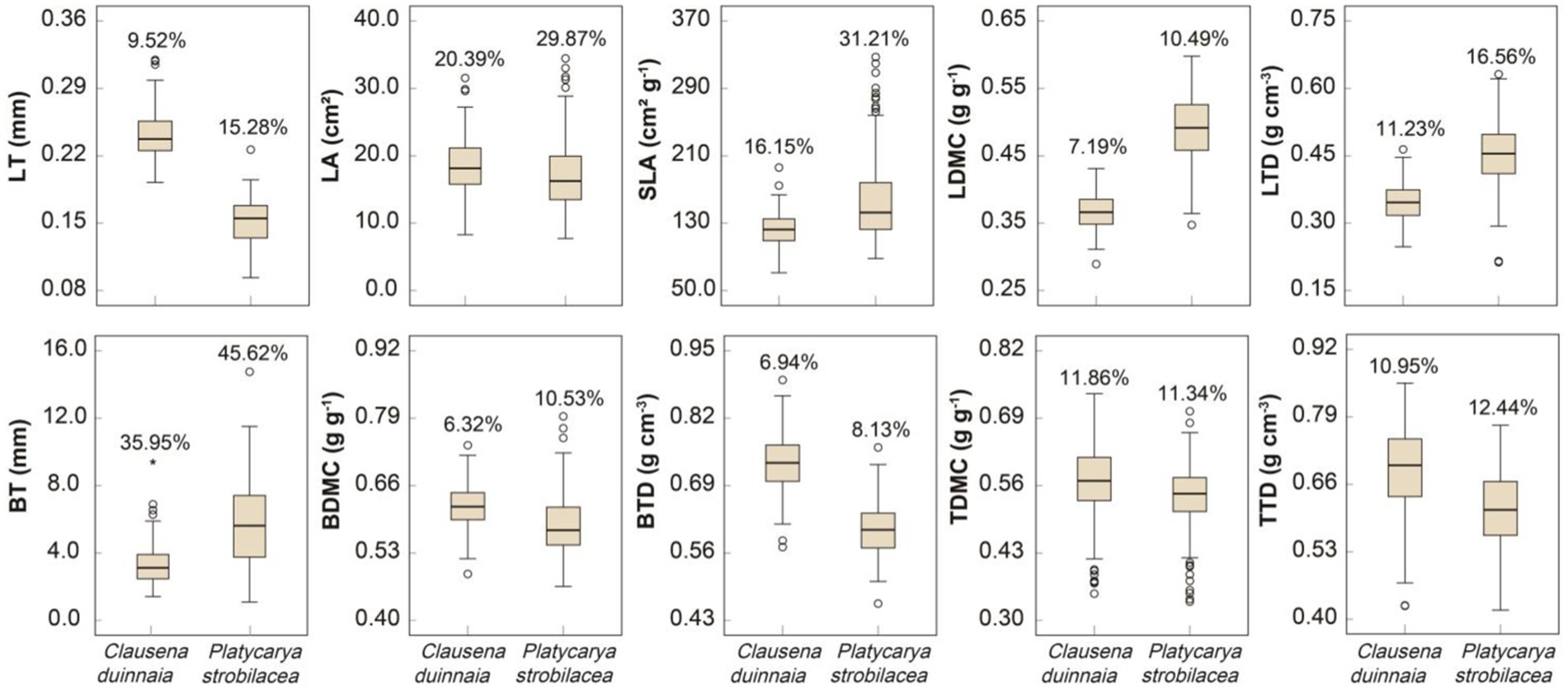
Figure 2. Plant functional traits and their intraspecific variations in two tree species in karst forests in Guizhou Province, Southwestern China. The circles in the box plots indicate abnormal values. The percentages of data in the box plots are the coefficients of intraspecific trait variation. LT, leaf thickness; LA, leaf area; SLA, specific leaf area; LDMC, leaf dry matter content; LTD, leaf tissue density; BT, bark thickness, BDMC, bark dry matter content; BTD, bark tissue density; TDMC, twig dry matter content; TTD, twig tissue density.
The LA and LTD of C. dunniana showed no differences between peak clumps and among slope positions (p > 0.05). The SLA, BT, and BTD of P. strobilacea also displayed no differences between peak clumps (p > 0.05). Other traits presented significant differences between peak clumps and/or among slope positions (p < 0.05) (Figure 3). The LTD, BT, BDMC, and TDMC of C. dunniana and the LDMC, LTD, BT, BDMC, and BTD of P. strobilacea increased with increasing slope position. The SLA of the two tree species decreased with increasing slope position (Figure 3).
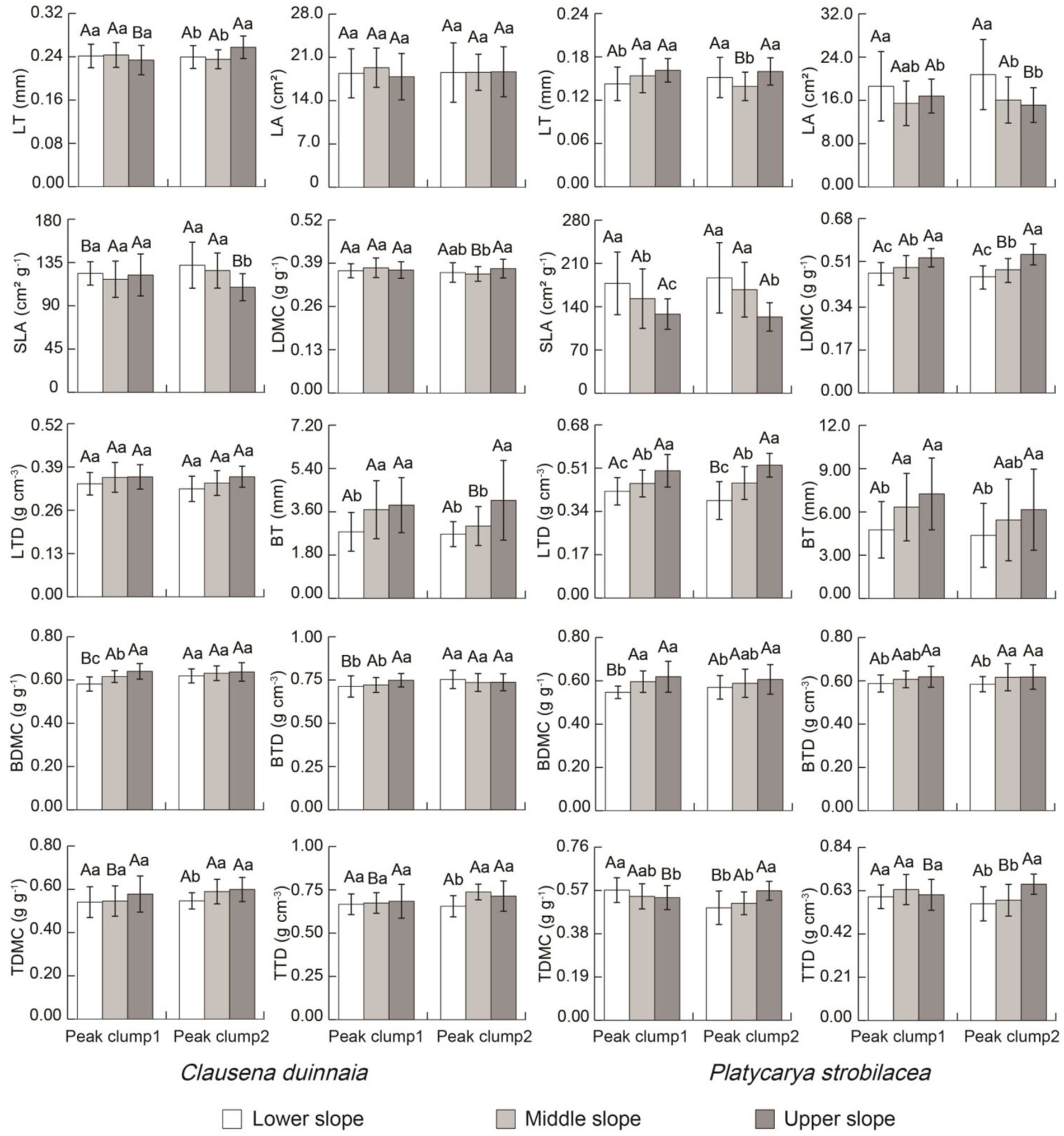
Figure 3. Plant functional trait differences between peak clumps and among slope positions in two tree species in karst forests in Guizhou Province, Southwestern China. Trait values with different capital letters between peak clumps in the same slope position are significantly different (independent sample t-test, p < 0.05). Trait values with different small letters among slope positions in a peak clump are significantly different (one-way ANOVA, p < 0.05). LT, leaf thickness; LA, leaf area; SLA, specific leaf area; LDMC, leaf dry matter content; LTD, leaf tissue density; BT, bark thickness, BDMC, bark dry matter content; BTD, bark tissue density; TDMC, twig dry matter content; TTD, twig tissue density.
Based on the results of sampling without replacement and independent sample t-test, the average LT values of object samples and total samples showed no significant differences when the sample sizes for the LT of C. dunniana and P. strobilacea exceeded 18 and 14, respectively. That is, the suggested sample individual numbers for the LT of C. dunniana and P. strobilacea are 18 and 14, respectively (Figure 4). The corresponding suggested sample individual numbers for the LA, SLA, LDMC, LTD, BT, BDMC, BTD, TDMC, and TTD of C. dunniana were 12, 8, 10, 13, 23, 9, 6, 12, and 15, respectively (Figure 4), and those for P. strobilacea were 20, 29, 12, 13, 22, 23, 11, 15, and 9, respectively. In general, more individuals of P. strobilacea should be sampled than C. dunniana, and more individuals should be sampled when measuring traits with larger intraspecific variations.
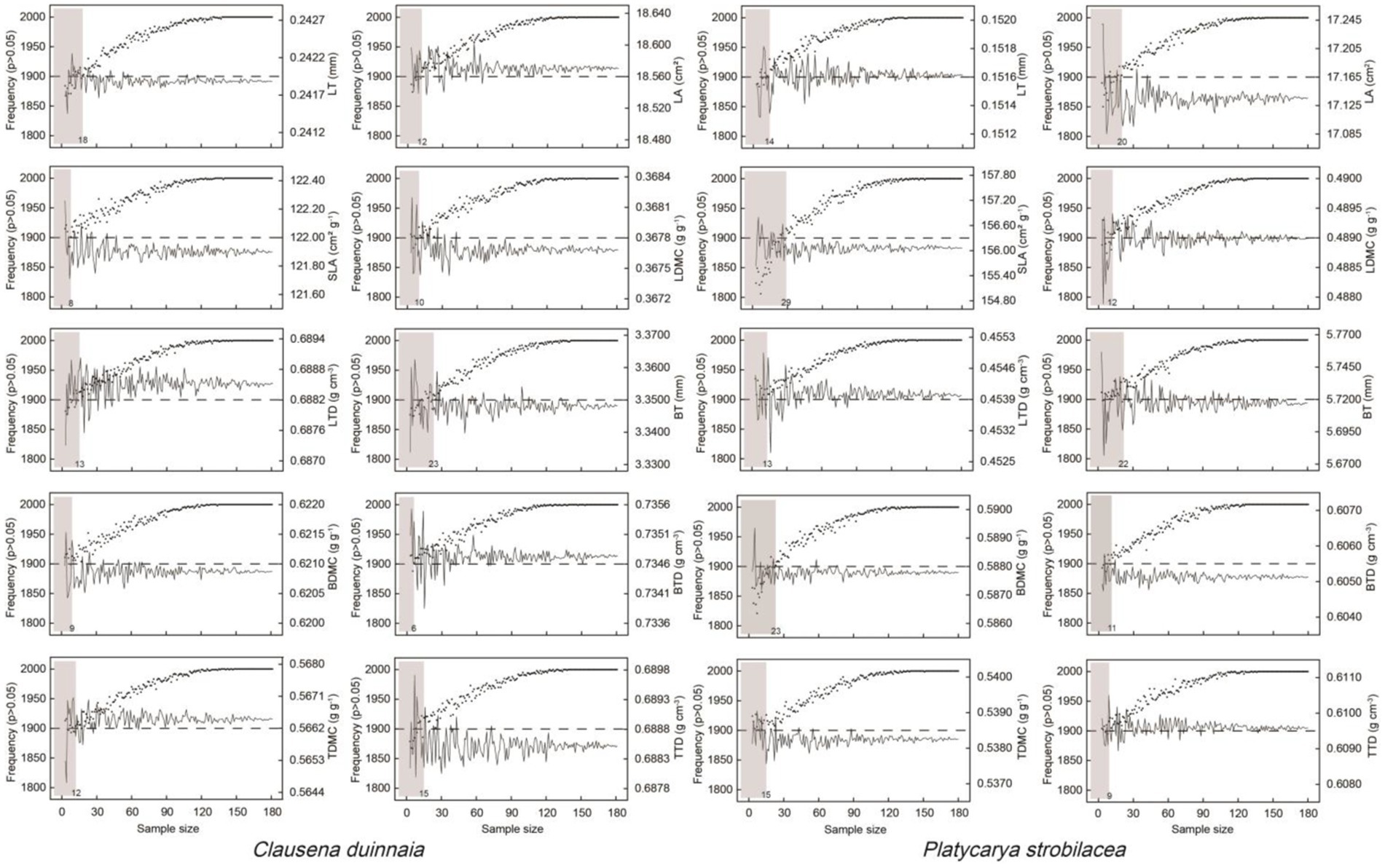
Figure 4. Mean of random sampling, frequency, and significant test of plant functional traits in two tree species in karst forests in Guizhou Province, Southwestern China. The solid line indicates the mean trait value of random sampling. The dots indicate frequencies of p > 0.05. The grey blocks are the areas of significant differences between object samples and total samples, indicating that the sample size of the right position of the block is the suggested sampling number of individuals. LT, leaf thickness; LA, leaf area; SLA, specific leaf area; LDMC, leaf dry matter content; LTD, leaf tissue density; BT, bark thickness, BDMC, bark dry matter content; BTD, bark tissue density; TDMC, twig dry matter content; TTD, twig tissue density.
4. Discussion
The PFTs of various vegetation types worldwide across geomorphologies have been considerably measured with reference to the handbooks for standardized measurements of PFTs (Cornelissen et al., 2003; Pérez-Harguindeguy et al., 2013). However, the PFT characteristics of some vegetation in remote or certain topographical areas have not been fully determined yet, such as those of the karst vegetation in Southwestern China. Most of the existing studies adopted the same sampling method as conducted in normal geomorphology (Geekiyanage et al., 2018; Pang et al., 2019; Yu et al., 2021; Shui et al., 2022). With regard to the effects of highly heterogeneous habitats on PFTs in karst geomorphology, in the present study, whether more individual numbers should be sampled when measuring PFTs in karst vegetation was explored for the first time. This study could provide not only basic data to the TRY and the China plant trait databases but also methodology for future detailed PFT measurements (especially for intraspecific trait variation measurements) in vegetation types grow in highly heterogeneous environments/habitats worldwide other than in karst vegetation in Southwestern China (He et al., 2019; Kattge et al., 2020).
Taxonomic status (genetic factors) and environmental conditions codetermine PFT characteristics (Weiher and Keddy, 1995; Westoby et al., 2002; Bolnick et al., 2011). In the present study, considerably different trait values between two karst tree species, and among individuals within a species but under different habitats were observed. Thus, the sampling quantities are supposed to be different when measuring PFTs in different species and within a species, but individuals grow in different environments/habitats. Besides, traits in different organs vary to some degree (Liu et al., 2022). The sampling quantities should also differ when measuring PFTs in different organs.
The common accepted practice considers that the number of individuals is often 5–10 when measuring PFTs across geomorphologies. In the present study, the suggested sampling quantities for 10 morphological traits in two tree species (C. dunniana and P. strobilacea) in karst forests in Southwestern China are 6–23 and 9–29, respectively. The common accepted sample size across geomorphologies is not sufficiently large in most cases. Therefore, more individuals should be sampled when measuring PFTs in habitat-heterogeneous karst vegetation, and the traits in different organs and species should be treated differently. C. dunniana is an evergreen species with long leaf life. It increases resource investments for survival. Large intraspecific variations in PFTs are not conducive for evergreen species to adapt to environments. P. strobilacea is a deciduous species with short leaf life. It increases resource investments for growth (Aerts, 1995; Givnish, 2002). Thus, more individuals should be sampled when measuring PFTs in deciduous species than evergreen ones. Larger sample sizes are also recommended for traits with larger intraspecific variations (such as LA, SLA, and BT) than smaller intraspecific variations (such as LDMC, LTD, and BTD).
Other PFTs, such as root specific length, C:N:P stoichiometry, leaf net photosynthetic rate, and water use efficiency, of tree species, shrubs, and herbs in karst vegetation also displayed large interspecific and intraspecific variations (Zhong et al., 2018; Zhang et al., 2020, 2021; Jing et al., 2021; Liu et al., 2022; Dong et al., 2023). The same method is also recommended to explore the sample sizes of those PFTs. In conclusion, when measuring PFTs in habitat-heterogeneous karst vegetation, the sample size should not be limited to 5–10. More plant individuals should be sampled under sufficient labor, material, and time.
Data availability statement
The original contributions presented in the study are included in the article/Supplementary material, further inquiries can be directed to the corresponding author.
Author contributions
LL and JN conceived and designed the research. CW, XL, LL, TY, YZ, and LC contributed to the field work. CW and LL analyzed the data and wrote the first draft with substantial input from JN. All authors contributed to the article and approved the submitted version.
Funding
This study was funded by the Zhejiang Provincial Natural Science Foundation of China (LQ20C030003).
Acknowledgments
The authors thank the Management Department of Maolan National Nature Reserve for assistance in all field work.
Conflict of interest
The authors declare that the research was conducted in the absence of any commercial or financial relationships that could be construed as a potential conflict of interest.
Publisher’s note
All claims expressed in this article are solely those of the authors and do not necessarily represent those of their affiliated organizations, or those of the publisher, the editors and the reviewers. Any product that may be evaluated in this article, or claim that may be made by its manufacturer, is not guaranteed or endorsed by the publisher.
Supplementary material
The Supplementary material for this article can be found online at: https://www.frontiersin.org/articles/10.3389/fevo.2023.1175031/full#supplementary-material
References
Aerts, R. (1995). The advantages of being evergreen. Trends Ecol. Evol. 10, 402–407. doi: 10.1016/S0169-5347(00)89156-9
Albert, C. H., Thuiller, W., and Yoccoz, N. G. (2010). Intraspecific functional variability: extent, structure and sources of variation. J. Ecol. 98, 604–613. doi: 10.1111/j.1365-2745.2010.01651.x
Auger, S., and Shipley, B. (2013). Inter-specific and intra-specific trait variation along short environmental gradients in old-growth temperate forest. J. Veg. Sci. 24, 419–428. doi: 10.1111/j.1654-1103.2012.01473.x
Bolnick, D. I., Amarasekare, P., Araojo, M. S., Burger, R., Levine, J. M., Novak, M., et al. (2011). Why intraspecific trait variation matters in community ecology. Trends Ecol. Evol. 26, 183–192. doi: 10.1016/j.tree.2011.01.009
Cornelissen, J. H. C., Lavorel, S., Garnier, E., Díaz, S., Buchmann, N., Gurvich, D. E., et al. (2003). A handbook of protocols for standardised and easy measurement of plant functional traits worldwide. Aust. J. Bot. 51, 335–380. doi: 10.1071/BT02124
Cui, E. Q., Weng, E. S., Yan, E. R., and Xia, J. Y. (2020). Robust leaf trait relationships across species under global environmental changes. Nat. Commun. 11:2999. doi: 10.1038/s41467-020-16839-9
Dong, Y. P., Wang, B., Wei, Y. L., He, F., Lu, F., Li, D. X., et al. (2023). Leaf micromorphological, photosynthetic characteristics and their ecological adaptability of dominant tree species in a karst seasonal rain forest in Guangxi, China. Guihaia 42, 415–428. doi: 10.11931/guihaia.gxzw202203031
Gao, S. H., Ge, Y. X., Zhou, L. Y., Zhu, B. L., Ge, X. Y., Li, K., et al. (2018). What is the optimal number of leaves when measuring leaf area of tree species in a forest community? Chin. J. Plant Ecol. 42, 917–925. doi: 10.17521/cjpe.2018.0087
Geekiyanage, N., Goodale, U. M., Cao, K. F., and Kitajima, K. (2018). Leaf trait variations associated with habitat affinity of tropical karst tree species. Ecol. Evol. 8, 286–295. doi: 10.1002/ece3.3611
Givnish, T. J. (2002). Adaptive significance of evergreen vs. deciduous leaves: solving the triple paradox. Silva Fenn. 36, 703–743. doi: 10.14214/sf.535
He, N. P., Liu, C. C., Piao, S. L., Sack, L., Xu, L., Luo, Y. Q., et al. (2019). Ecosystem traits linking functional traits to macroecology. Trends Ecol. Evol. 34, 200–210. doi: 10.1016/j.tree.2018.11.004
Hultine, K. R., and Marshall, J. D. (2000). Altitude trends in conifer leaf morphology and stable carbon isotope composition. Oecologia 123, 32–40. doi: 10.1007/s004420050986
Jiang, Y., Chen, X. B., Ma, J. M., Liang, S. C., Huang, J., Liu, R. H., et al. (2016). Interspecific and intraspecific variation in functional traits of subtropical evergreen and deciduous broadleaved mixed forests in karst topography, Guilin, Southwest China. Trop. Conserv. Sci. 9, 194008291668021–194008291668029. doi: 10.1177/1940082916680211
Jiang, Z. C., Lian, Y. Q., and Qin, X. Q. (2014). Rocky desertification in Southwest China: impacts, causes, and restoration. Earth-Sci. Rev. 132, 1–12. doi: 10.1016/j.earscirev.2014.01.005
Jin, Y. L., Wang, H. Y., Xia, J., Ni, J., Li, K., Hou, Y., et al. (2023). TiP-leaf: a dataset of leaf traits across vegetation types on the Tibetan plateau. Earth Syst. Sci. Data 15, 25–39. doi: 10.5194/essd-15-25-2023
Jing, H. X., Sun, N. X., Umair, M., Liu, C. J., and Du, H. M. (2021). Leaf stoichiometric response of two typical herbaceous plants to water additions in degraded karst areas in southern Yunnan, China. J. Yunnan Univ. Nat. Sci. Ed. 43, 1254–1263. doi: 10.7540/j.ynu.20200450
Kambach, S., Sabatini, F. M., Attorre, F., Biurrun, I., Boenisch, G., Čarni, A., et al. (2023). Climate-trait relationships exhibit strong habitat specificity in plant communities across Europe. Nat. Commun. 14:712. doi: 10.1038/s41467-023-36240-6
Kattge, J., Bönisch, G., Díaz, S., Lavorel, S., Prentice, I. C., and Wirth, C. (2020). TRY plant trait database-enhanced coverage and open access. Glob. Chang. Biol. 26, 119–188. doi: 10.1111/gcb.14904
Kraft, N. J. B., Valencia, R., and Ackerly, D. D. (2008). Functional traits and niche-based tree community assembly in an Amazonian forest. Science 322, 580–582. doi: 10.1126/science.1160662
Lecerf, A., and Chauvet, E. (2008). Intraspecific variability in leaf traits strongly affects alder leaf decomposition in a stream. Basic Appl. Ecol. 9, 598–605. doi: 10.1016/j.baae.2007.11.003
Liu, L. B., Hu, J., Chen, X. Y., Xu, X., Yang, Y., and Ni, J. (2022). Adaptation strategy of karst forests: evidence from the community-weighted mean of plant functional traits. Ecol. Evol. 12:e8680. doi: 10.1002/ece3.8680
Liu, L. B., Wu, Y. N., Zhong, Q. L., Guo, Y. M., Xu, X., Yang, Y., et al. (2021). Tree species influences soil microbial community diversity but not biomass in a karst forest in southwestern China. J. Plant Ecol. 14, 280–290. doi: 10.1093/jpe/rtaa096
Liu, L. B., Xia, H. J., Quan, X. H., and Wang, Y. Q. (2023). Plant trait-based life strategies of overlapping species vary in different succession stages of subtropical forests. Eastern China. Front. Ecol. Evol. 10:1103937. doi: 10.3389/fevo.2022.1103937
McDonald, P. G., Fonseca, C. R., Overton, J., and Westoby, M. (2003). Leaf-size divergence along rainfall and soil-nutrient gradients: is the method of size reduction common among clades? Funct. Ecol. 17, 50–57. doi: 10.1046/j.1365-2435.2003.00698.x
Messier, J., McGill, B. J., and Lechowica, M. J. (2010). How do traits vary across ecological scales? A case for trait-based ecology. Ecol. Lett. 13, 838–848. doi: 10.1111/j.1461-0248.2010.01476.x
Myers-Smith, I. H., Thomas, H. J. D., and Bjorkman, A. D. (2019). Plant traits inform predictions of tundra responses to global change. New Phytol. 221, 1742–1748. doi: 10.1111/nph.15592
Niu, K. C., Zhang, S., and Lechowicz, M. (2020). Harsh environmental regimes increase the functional significance of intraspecific variation in plant communities. Funct. Ecol. 34, 1666–1677. doi: 10.1111/1365-2435.13582
Pan, Z. (2003). Habitat Heterogeneity of Karst Ecological System and Plant Adaptability. Ph.D. thesis. Guilin: Guangxi Normal University.
Pang, Z. Q., Lu, W. L., Jiang, L. S., Jin, K., and Qi, Z. (2019). Leaf traits of different growing plants in karst area of Shilin, China. Guihaia 39, 1126–1138. doi: 10.11931/guihaia.gxzw201810009
Peng, X., Dai, Q., Ding, G., and Li, C. (2019). Role of underground leakage in soil, water and nutrient loss from a rock-mantled slope in the karst rocky desertification area. J. Hydrol. 578:124086. doi: 10.1016/j.jhydrol.2019.124086
Peppe, D. J., Royer, D. L., Cariglino, B., Oliver, S. Y., Newman, S., Leight, E., et al. (2011). Sensitivity of leaf size and shape to climate: global patterns and paleoclimatic applications. New Phytol. 190, 724–739. doi: 10.1111/j.1469-8137.2010.03615.x
Pérez-Harguindeguy, N., Díaz, S., Garnier, E., Lavorel, S., Poorter, H., Jaureguiberry, P., et al. (2013). New handbook for standardised measurement of plant functional traits worldwide. Aust. J. Bot. 61, 167–234. doi: 10.1071/BT12225
R Core Team. (2018). R: A Language and Environment for Statistical Computing. R Foundation for Statistical Computing. Vienna.
Shui, W., Guo, P. P., Zhu, S. F., Feng, J., Sun, X., and Li, H. (2022). Variation of plant functional traits and adaptive strategy of woody species in degraded karst tiankeng of Yunnan Province. Sci. Geogr. Sin. 42, 1295–1306. doi: 10.13249/j.cnki.sgs.2022.07.016
Siefert, A., Violle, C., Chalmandrier, L., Albert, C. H., Taudiere, A., Fajardo, A., et al. (2015). A global meta-analysis of the relative extent of intraspecific trait variation in plant communities. Ecol. Lett. 18, 1406–1419. doi: 10.1111/ele.12508
Song, L. H. (2000). Progress and trend of karst geomorphology study. Prog. Geogr. 19, 193–202. doi: 10.11820/dlkxjz.2000.03.001
Thuiller, W., Lavorel, S., Midgley, G., Lavergne, S., and Rebelo, T. (2004). Relating plant traits and species distributions along bioclimatic gradients for 88 Leucadendron taxa. Ecology 85, 1688–1699. doi: 10.1890/03-0148
Violle, C., Navas, M. L., Vile, D., Kazakou, E., Fortunel, C., Hummel, I., et al. (2007). Let the concept of trait be functional! Oikos 116, 882–892. doi: 10.1111/j.0030-1299.2007.15559.x
Weiher, E., and Keddy, P. A. (1995). Assembly rules, null models, and trait dispersion: new question front old patterns. Oikos 74, 159–164. doi: 10.2307/3545686
Westoby, M., Falster, D. S., Moles, A. T., Vesk, P. A., and Wright, I. J. (2002). Plant ecological strategies: some leading dimensions of variation between species. Annu. Rev. Ecol. Syst. 33, 125–159. doi: 10.1146/annurev.ecolsys.33.010802.150452
Wright, S. J., Kitajima, K., Kraft, N. J. B., Reich, P. B., Wright, I. J., Bunker, D. E., et al. (2010). Functional traits and the growth-mortality trade-off in tropical trees. Ecology 91, 3664–3674. doi: 10.1890/09-2335.1
Wright, I. J., Reich, P. B., Westoby, M., Ackerly, D. D., Baruch, Z., Bongers, F., et al. (2004). The worldwide leaf economics spectrum. Nature 428, 821–827. doi: 10.1038/nature02403
Yang, Y., Xu, X., Xu, Y., and Ni, J. (2020). Adaptation strategies of three dominant plants in the trough-valley karst region of northern Guizhou Province, southwestern China, evidence from associated plant functional traits and ecostoichiometry. Earth Environ. 48, 413–423. doi: 10.14050/j.cnki.1672-9250.2020.48.061
Yu, Y. H., Zhong, X. P., Zheng, W., Chen, Z. X., and Wang, J. X. (2021). Species diversity, functional traits, stoichiometry and correlation of plant community in different succession stages of karst forest. Acta Ecol. Sin. 41, 2408–2417. doi: 10.5846/stxb202005031089
Zhang, S. H., Zhang, Y., Xiong, K. N., Yu, Y. H., and Min, X. Y. (2020). Changes of leaf functional traits in karst rocky desertification ecological environment and the driving factors. Glob. Ecol. Conserv. 24:e01381. doi: 10.1016/j.gecco.2020.e01381
Zhang, Q. W., Zhu, S. D., Jansen, S., and Cao, K. F. (2021). Topography strongly affects drought stress and xylem embolism resistance in woody plants from a karst forest in Southwest China. Funct. Ecol. 35, 566–577. doi: 10.1111/1365-2435.13731
Zhong, Q. L., Liu, L. B., Xu, X., Yang, Y., Guo, Y. M., Xu, H. Y., et al. (2018). Variations of plant functional traits and adaptive strategy of woody species in a karst forest of Central Guizhou Province, southwestern China. Chin. J. Plant Ecol. 42, 562–572. doi: 10.17521/cjpe.2017.0270
Keywords: morphological traits, interspecific and intraspecific variations, sampling number, habitat heterogeneity, evergreen and deciduous broadleaved mixed forest, karst geomorphology
Citation: Wang C, Lu X, Yang T, Zheng Y, Chen L, Liu L and Ni J (2023) Should more individuals be sampled when measuring functional traits of tree species in habitat-heterogeneous karst forests? Front. Ecol. Evol. 11:1175031. doi: 10.3389/fevo.2023.1175031
Edited by:
Xiang Liu, Lanzhou University, ChinaCopyright © 2023 Wang, Lu, Yang, Zheng, Chen, Liu and Ni. This is an open-access article distributed under the terms of the Creative Commons Attribution License (CC BY). The use, distribution or reproduction in other forums is permitted, provided the original author(s) and the copyright owner(s) are credited and that the original publication in this journal is cited, in accordance with accepted academic practice. No use, distribution or reproduction is permitted which does not comply with these terms.
*Correspondence: Libin Liu, liulibin@zjnu.cn