- 1Ministry of Education Key Laboratory of Ecology and Resource Use of the Mongolian Plateau and Inner Mongolia Key Laboratory of Grassland Ecology, School of Ecology and Environment, Inner Mongolia University, Hohhot, China
- 2School of Public Administration, South China Agricultural University, Guangzhou, China
Appropriate grazing pressure and fertilizer application of nitrogen (N) and phosphorus (P) are effective measures to increase grassland productivity. In this study, we report on the interactive effects of grazing intensity and fertilizer application on soil properties, enzyme characteristics, and soil bacterial community compositions. The experiment was set up in a typical grassland in Xilingol, Inner Mongolia, and had 12 treatments (CC, CN, CP, CNP, LC, LN, LP, LNP, HC, HN, HP, and HNP). These consisted of three grazing intensity levels crossed with four fertilizer application treatments: no fertilizer, N fertilizer, P fertilizer addition alone, and both N and P fertilizers addition, subjected to field sampling and laboratory analysis. The results showed that soil alkaline hydrolysis nitrogen was increased by 15 and 13.6% in LN over LC in the 0–10 and 10–20 cm soil depth layers, respectively. Soil available P was 135.6% higher in LP than in LC at 0–10 cm but similar between LP and LC at 10–20 cm. Soil urease activity rose by 46.8 and 39.3% in 0–10 cm soil and was augmented by 63.1 and 60.3% in 10–20 cm soil of LN and LP relative to LC, respectively. Soil catalase (CAT) activity was decreased in response to LNP by 29.4, 23.5, and 26.5% vis-à-vis LC, LN, and LP in the 0–10 cm layer. Soil CAT activity also decreased in 0–20 cm layer for HN and HNP in comparison with HP. The relative abundance of Actinobacteria increased by 38.1 and 45.0% in HC over that in CC and LC, respectively, in 0–10 cm soil; compared with LC, it increased by 35.8 and 21.7% in LN and LNP, respectively. The relative abundance of Proteobacteria was increased in LNP versus LC in 0–10 cm soil. Overall, fertilizer application coupled with a light grazing intensity promoted key soil properties and the relative abundance of a dominant bacterial phylum.
1. Introduction
Grassland ecosystems provide many goods and services for human beings all over the world (Zhao et al., 2020). Grazing is one of the main modes of utilizing the grassland ecosystem. However, with an increasing human population and more livestock, nearly one-third of the world’s grassland areas are now seriously degraded due to overutilization, resulting in a decline in the productivity and biodiversity of grassland ecosystems, which is seriously affecting their functions and services (Eldridge and Delgado-Baquerizo, 2017). Grazing pressure and soil nutrient enrichment are two key factors that affect grassland’s soil health and its sustainable utilization, with grazing intensity usually having adverse impacts (Li W.H. et al., 2017).
The grazing of livestock can change plant communities, and herding animal waste and trampling behavior also alters soil’s physical and chemical properties (Zhang et al., 2017). Grazing can also modulate soil functioning by changing soil microbial community composition (Eldridge et al., 2017). In addition, the application of N and phosphorus (P) fertilizers is an effective measure in improving the general soil quality of grassland (Wang C.L. et al., 2021). The use of N and P fertilizers is known to significantly affect the composition and diversity of soil bacterial communities (Li M. et al., 2020). Further, bacterial community composition in soil often changes with environmental factors (Yang W. et al., 2020; Jiang et al., 2021). Bacteria accelerate the decomposition of soil organic matter and promote material circulation by producing soil enzymes (Bhagat and Kokitkar, 2021). In this respect, phosphatase and urease are closely related to the degradation of soil P and organic matter, while catalase indicates the strength of microbial activities and oxidation processes in soil (Sun et al., 2021).
Because the bacterial community composition responds strongly to grassland management measures such as grazing and fertilization practices, it is often used to assess the soil health status of grassland (Li J.Y. et al., 2017). Numerous studies have discussed the effects of grazing intensity or fertilization on soil microbial community structure and their underlying mechanisms (He et al., 2017; Liu et al., 2021; Wang et al., 2022a). Kang et al. (2021) found that soil TC and SOC decreased with increasing soil depth in alpine meadows. According to one grassland study, bacterial diversity declines with increasing soil depth (Xu et al., 2021). More disturbance by grazing and fertilizer applications in 0–10 cm soil than in 10–20 cm soil resulted in the different response of soil properties and soil bacterial community structure in 0–10 and 10–20 cm. The bacterial abundance of non-rhizospheric soil was bolstered under light grazing yet fell rapidly under moderate or heavy grazing (Zhang et al., 2019). However, the study by Zhang Y.T. et al. (2020) found that heavy grazing significantly increased the relative abundance of Actinobacteria. The microbial compositions responded to grazing, mainly attributable to the changes in soil physicochemical properties induced by grazing (Zhang Y.T. et al., 2020). The N application alone has been shown to inhibit the soil N cycle, but the joint application of N and P fertilizers promoted the metabolic activities of soil microorganisms (Che et al., 2018; Liu et al., 2023); however, long-term N application negatively impacted the abundance and community-level diversity of bacteria in soil (Zhang et al., 2018). Some studies have pointed out that N and P fertilizer application is capable of increasing the relative abundance of Actinobacteria but reduces that of Acidobacteria (Wang Z.H. et al., 2021).
Although the respective effects of grazing and fertilizer applications on the bacterial community structure of grassland ecosystems have been increasingly studied (Li et al., 2018; Xu et al., 2020), their interactive effects on microbial community structures in typical grasslands remain unclear (Wang et al., 2022b). Furthermore, which management practices in terms of grazing and fertilizer applications are most beneficial for accumulating soil nutrients and stabilizing the soil microbial community in typical grasslands? In this study, we hypothesized that (1) soil properties and soil bacterial community structure respond differently in 0–10 vs. 10–20 cm depth layer (Xu et al., 2021); (2) different grazing intensity and different fertilizer application change the soil properties and soil bacterial community composition by changing soil nutrients, animal waste, and urine inputs (Wang C.L. et al., 2021); and (3) the effects of grazing intensity and fertilizer application on soil microbial composition is related to locally changed soil properties (Cheng et al., 2021).
2. Materials and methods
2.1. Study area
The field experiment was carried out in a semiarid steppe ecosystem of typical grassland at the Grassland Ecosystem Research Station of Inner Mongolia University (44°15′N, 116°31′E), situated 54 km east of Xilinhot in Inner Mongolia, China (Supplementary Figure A1). The study area is characterized by a classical semi-arid climate in a mid-temperate zone, with an annual average temperature of 0.5–1.0°C and 280.5 mm of precipitation (year range: 1967 to 2017). The rainfall was concentrated from May to September (Yin et al., 2022). The soil type here is mainly chestnut soil (Gao et al., 2017). The dominant plant species of the natural grassland community are Achnatherum splendens, Caragana microphylla, Leymus chinensis, and Stipa grandis.
2.2. Experimental design
The experimental site was freely grazed until 2012 and then fenced for 7 years before conducting this experiment. It had 12 treatments, each with three replicates (Figure 1): (1) Without grazing: ① Control (CC); ② Nitrogen fertilizer application alone without grazing (CN); ③ Phosphorus fertilizer application alone without grazing (CP); ④ Nitrogen and phosphorus application without grazing (CNP). (2) Light grazing: ⑤ Light grazing without fertilizer application (LC); ⑥ Nitrogen fertilizer application alone under light grazing (LN); ⑦ Phosphorus fertilizer application alone under light grazing (LP); ⑧ Nitrogen and P application under light grazing (LNP). (3) Heavy grazing: ⑨ Heavy grazing without fertilizer application (HC); ⑩ Nitrogen fertilizer application alone under heavy grazing (HN); ⑪ Phosphorus fertilizer application alone under heavy grazing (HP); ⑫ Nitrogen and P application under heavy grazing (HNP). Each replicate plot was 6 m × 8 m, with 36 plots used in total. In each treatment, the distance between plots was 2 m. The light and heavy grazing plots were grazed by three and nine sheep, respectively, in the same way: rotationally once a month, beginning on the 20th of June to August of each year, from 2019 to 2021. The experimental sheep were selected to be of similar weight to 2-year-old native sheep. Grazing intensity was controlled to a light grazing level by leaving a grassland stubble ca. 6 cm in height each time. Chemical fertilizers consisting of urea and calcium superphosphate were applied to the plots at the beginning of each month, from June to August of each year. Nitrogen and P fertilizers were applied to the plots at 100 and 50 kg ha−1 (Soares et al., 2013; Ma et al., 2018), respectively, once a month from June to August, from 2019 to 2021 in the research plots. Not to disturb the grassland, there was no irrigation or plowing done after each chemical fertilizer application. The chemical fertilizers were dissolved after precipitation.
2.3. Collection and analyses of soil samples
Soil samples were collected in July 2021. Five soil samples were collected from each 0–10 and 10–20 cm depth layer in each plot and then mixed homogenously (on per layer per plot basis). These composite soil samples were then sieved (2 mm), homogenized, and divided into three parts. One part was air-dried to analyze the soil’s physical and chemical properties; one part was stored at 4°C to measure enzymatic activities; the other part was stored at 80°C for DNA extractions (Liu et al., 2020). For high-throughput sequencing, the extracted DNA from each sample was sent to Gene Denovo Biotechnology Co., Ltd. (Guangzhou, China). DNA library sequencing was performed on the Illumina HiSeqTM 2500/4000. The methods used to determine the soil’s physical, chemical, and biological properties are presented in Table 1. The high-throughput sequencing raw data of bacteria in 0–10 and 10–20 cm soil layers in 2021 have been uploaded to the NCBI database under SRA accession numbers PRJNA856452 and PRJNA856463, respectively.
2.4. Statistical analysis
Statistical analyses were conducted in SPSS 24.0 Software. For the analysis of soil parameters, each was tested for normal distribution. One-way ANOVA was carried out to determine significant differences in soil indicators among the 12 treatments. Pairwise differences between the treatments’ mean values for soil properties and the relative abundances of soil bacterial community structures were tested using Fisher’s LSD at a p < 0.05 significance level. The differences between treatments in terms of their alpha (α) diversity metrics were tested using Tukey HSD or the Kruskal–Wallis test (at p < 0.05 significance level). Pearson correlations between the soil indicators and the dominant bacterial phylum were conducted in SPSS 24.0. Data were visualized using Origin 9.1 software. Two-way ANOVA was performed after grazing and fertilizer application using R 4.0.4 software. Redundancy analysis (RDA) and linear discriminant analysis effect size (Lefse) analysis were carried out online, using the Majorbio Cloud Platform (https://cloud.majorbio.com/; Yan et al., 2021).
3. Results
3.1. Soil properties
Between the two soil layers (i.e., 0–10 and 10–20 cm), soil total carbon (TC), total nitrogen (TN), and total phosphorus (TP) were significantly decreased by 22.0, 13.0, and 16.0% at 0–10 cm compared with that at 10–20 cm in response to LN (Supplementary Table S1). Soil-dissolved organic carbon (DOC) decreased significantly by 9.0% at 0–10 vs. 10–20 cm in HN. Compared with the 10–20 cm, soil alkaline hydrolysis nitrogen (AN) and available phosphorus (AP) significantly increased by 21.4, 6.9, and 6.0% at 0–10 cm under the CN, CNP, and LNP treatments. Soil pH was 8.3–18.1% lower in the 0–10 cm than in the 10–20 cm layer, irrespective of treatments.
Under the three different grazing treatments (no grazing, light grazing, and heavy grazing), soil DOC increased with greater grazing intensity by 44.2 and 60.0% in LC and HC, respectively, than in CC at 0–10 cm. Soil AP was 122.5% higher in LC than in CC at 10–20 cm (Table 2). Soil AN and AP were both reduced by LN and HN compared with CN in the 0–10 cm layer. Soil AN was 9.5% lower in HN than in CN at 10–20 cm, but their soil AP contents were similar at 10–20 cm. Soil AP under HP fell by 36.0 and 31.8% relative to CP and LP at 0–10 cm, respectively, and was reduced even more (by 46.7%) vs. LP at 10–20 cm. Soil AP was decreased by 25.5 and 19.7%, respectively, in LNP and HNP relative to CNP in the 0–10 cm depth layer.
Under differing fertilizer application treatments—no fertilizer addition, N fertilizer addition alone, P fertilizer addition alone, and both N and P fertilizer addition—soil TC, TN, and TP were similar among CN, CP, CNP, and CC at 0–10 cm. However, compared with LC, soil TC increased by 9.8 and 16.9% in the 0–10 and 10–20 cm layers, respectively, under the LN treatment. Soil AN was augmented by N fertilizer application, and soil AP was increased by CP in comparison with CC at 0–10 cm. Soil pH decreased in CNP relative to CC at 0–10 cm. Both soil AN and pH were lower in CNP than CC in the 10–20 cm layer. At 0–10 cm, soil TP was higher in LP than either LN or LNP. Soil AP was bolstered by 75.7 and 72.4% in HP and HNP, respectively, over HN in 0–10 cm soil. Two-way ANOVA was used to investigate the interactive effects of grazing and fertilization on soil properties (Table 3). Grazing and fertilization both had significant effects on TN and AP at 10–20 cm.
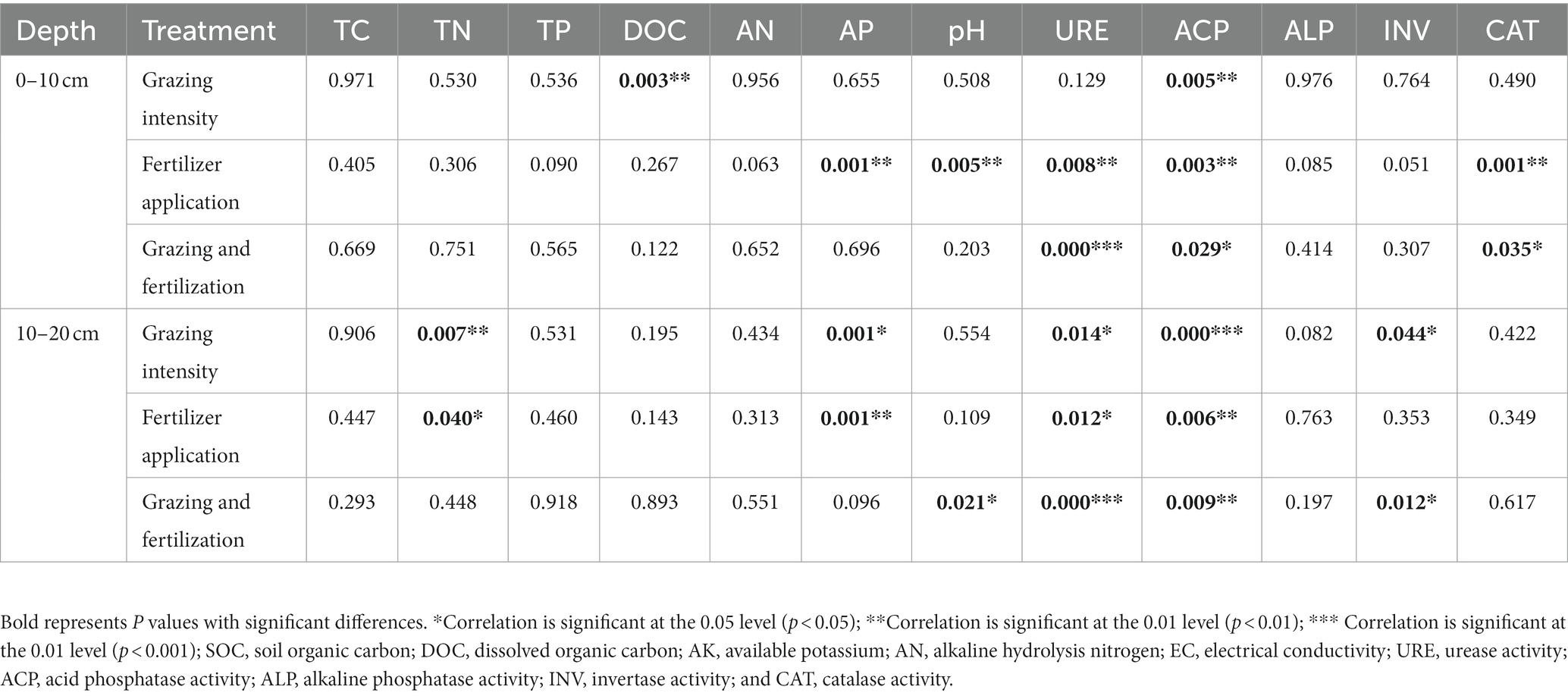
Table 3. p values of the two-way ANOVA of grazing intensity and fertilizer application on soil properties.
3.2. Soil enzyme activities
Between soil depth layers, soil urease (URE) activity significantly decreased by 29.8 and 38.1% at 0–10 cm compared with 10–20 cm in both CN and HN treatments (Supplementary Table S2). Soil alkaline phosphatase (ALP) activity was significantly decreased by 34.4 and 20.4% in CNP and HNP at 0–10 cm relative to 10–20 cm. Soil invertase (INV) activity was significantly increased by 19.2 and 43.1% at 0–10 cm versus 10–20 cm in CNP and LNP, respectively. Soil catalase (CAT) activity significantly increased by 23.2 and 23.1% at 0–10 cm relative to 10–20 cm in CP and LP, respectively.
Comparing the three grazing treatments, soil ALP activity was reduced by 29.4 and 30.1% in LC and HC, respectively, relative to CC in the 0–10 cm layer, falling by 16.1% in HC than CC in deeper soil (10–20 cm; Figures 2, 3). At 0–10 cm, soil INV activity was 15.9 and 23.0% lower in HC than CC and LC, respectively (Figure 2). Under the different fertilizer application treatments, in the 10–20 cm layer, soil URE activity increased by 63.1, 60.3, and 55.3% in LN, LP, and LNP, respectively, over LC but decreased by 12.5, 16.4, and 18.0% in HN, HP, and HNP, respectively, over HC (Figure 3). Soil acid phosphatase (ACP) activity was 27.5 and 33.5% lower in LN than LC at 0–10 and 10–20 cm, respectively. Soil ALP activity was decreased by 38.4, 32.2, and 37.1% in CN, CP, and CNP, respectively, when compared with CC in the 0–10 cm layer. Soil ALP activity was 21.9 and 13.9% higher in HP than HN at 0–10 and 10–20 cm, respectively. Soil CAT activity fell by 29.4, 23.5, and 26.5% in LNP relative to LC, LN, and LP, respectively, at 0–10 cm. There was less soil CAT activity in HN and HNP than both HC and HP in the 0–10 cm layer, and likewise for HN and HNP than for HP in deeper soil (10–20 cm). Two-way ANOVA analysis showed that grazing and fertilization had a significant interactive effect on URE as well as ACP in 0–20 cm soil (Table 3).
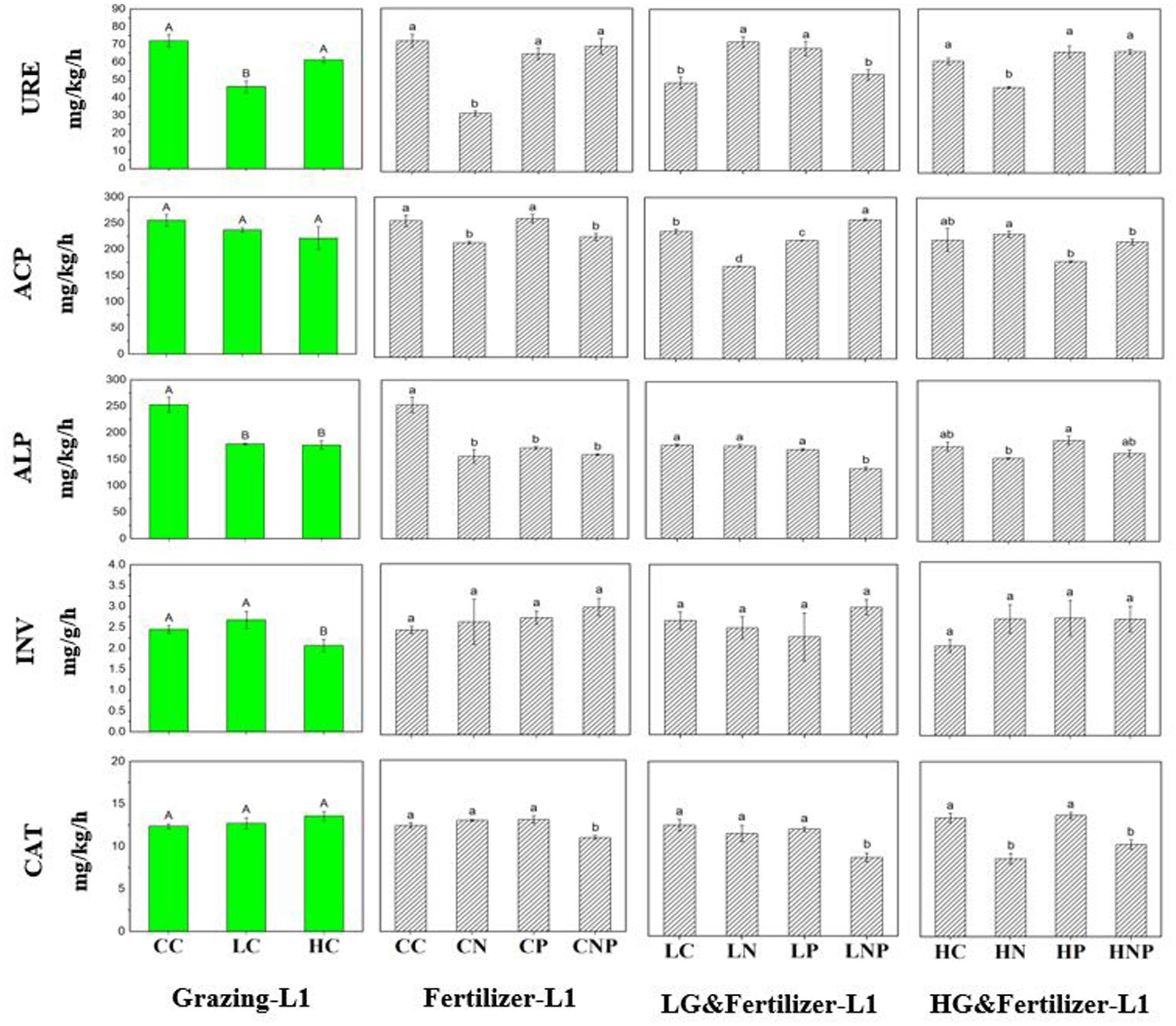
Figure 2. Soil enzyme activity at different grazing intensities and fertilizer application treatments in 0–10 cm (L1) soil. Different lowercase letters indicate significant differences between soil properties in different fertilizer treatments at the same grazing intensity at p < 0.05. Different uppercase letters indicate significant differences between soil properties in different grazing intensity treatments at the same fertilization treatments at p < 0.05; n = 3. URE, urease activity; ACP, acid phosphatase activity; ALP, alkaline phosphatase activity; INV, invertase activity; and CAT, catalase activity.
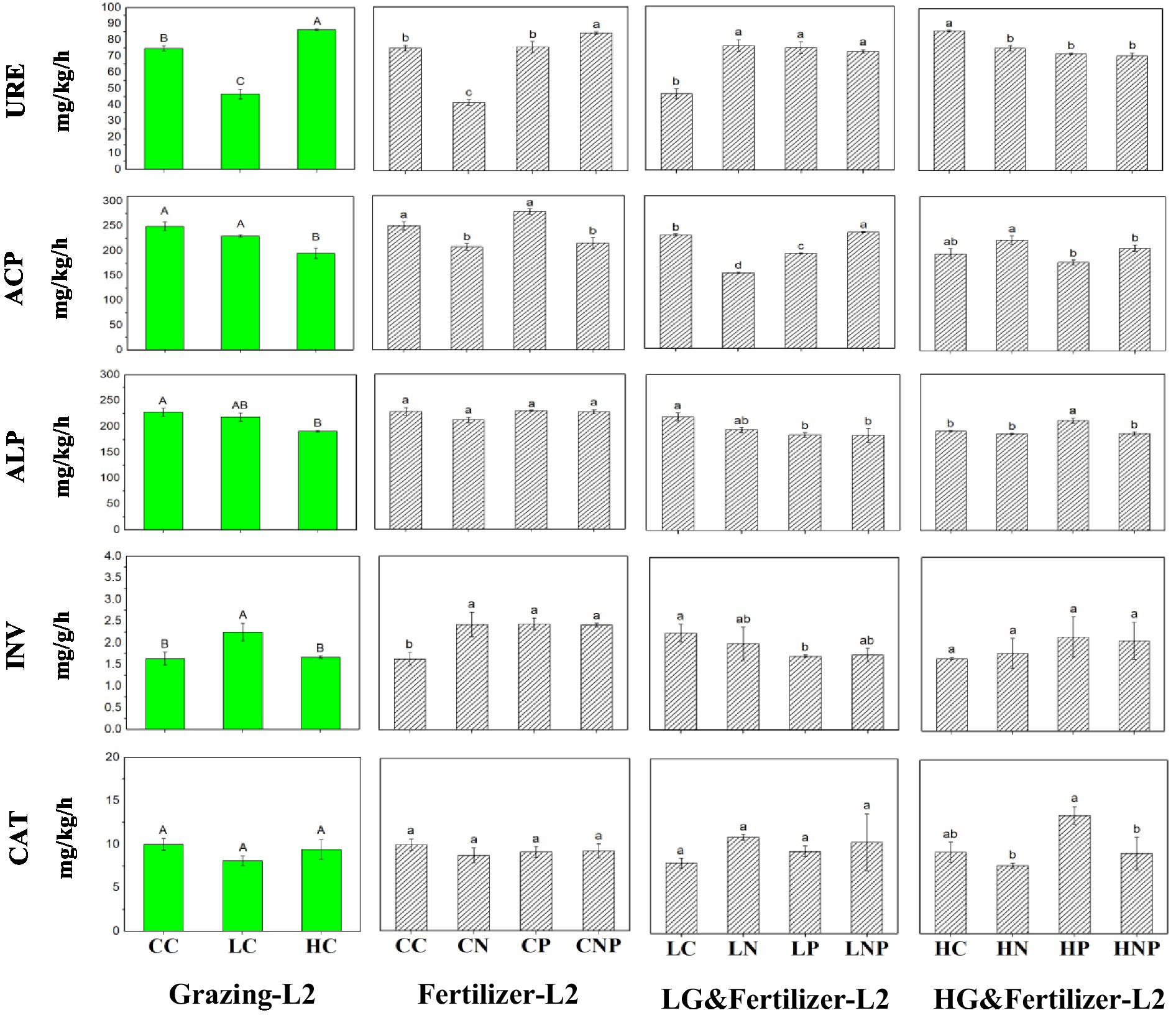
Figure 3. Soil enzyme activity at different grazing intensities and fertilizer application treatments in 10–20 cm (L2) soil. Different lowercase letters indicate significant differences between soil properties in different fertilizer treatments at the same grazing intensity at p < 0.05. Different uppercase letters indicate significant differences between soil properties in different grazing intensity treatments at the same fertilization treatments at p < 0.05; n = 3. URE, urease activity; ACP, acid phosphatase activity; ALP, alkaline phosphatase activity; INV, invertase activity; and CAT, catalase activity.
3.3. Bacterial community composition and structure
The dominant bacterial phylum was Acidobacteria, followed by Proteobacteria, Actinobacteria, Verrucomicrobia, Bacteroidetes, Planctomycetes, and Patescibacteria, in the 0–10 and 10–20 cm soil layer samples (Figure 4). Under different soil layers, the relative abundance of Bacteroidetes was 27.0–35.1% higher at 0–10 than 10–20 cm under light grazing and fertilizer application (Supplementary Table S3). The diversity index values (Sobs, Shannon, Chao1, ACE, and PD-tree diversity metrics) were higher in the 0–10 cm than in the 10–20 cm layer, regardless of treatments (Table 4). Specifically, the Sobs, Shannon, and Chao1 indexes were higher in 0–10 cm than in 10–20 cm in both HP and HC (Supplementary Table S4). The Chao1, ACE, and PD-tree indexes were higher in 0–10 cm than in 10–20 cm in the LP treatment.
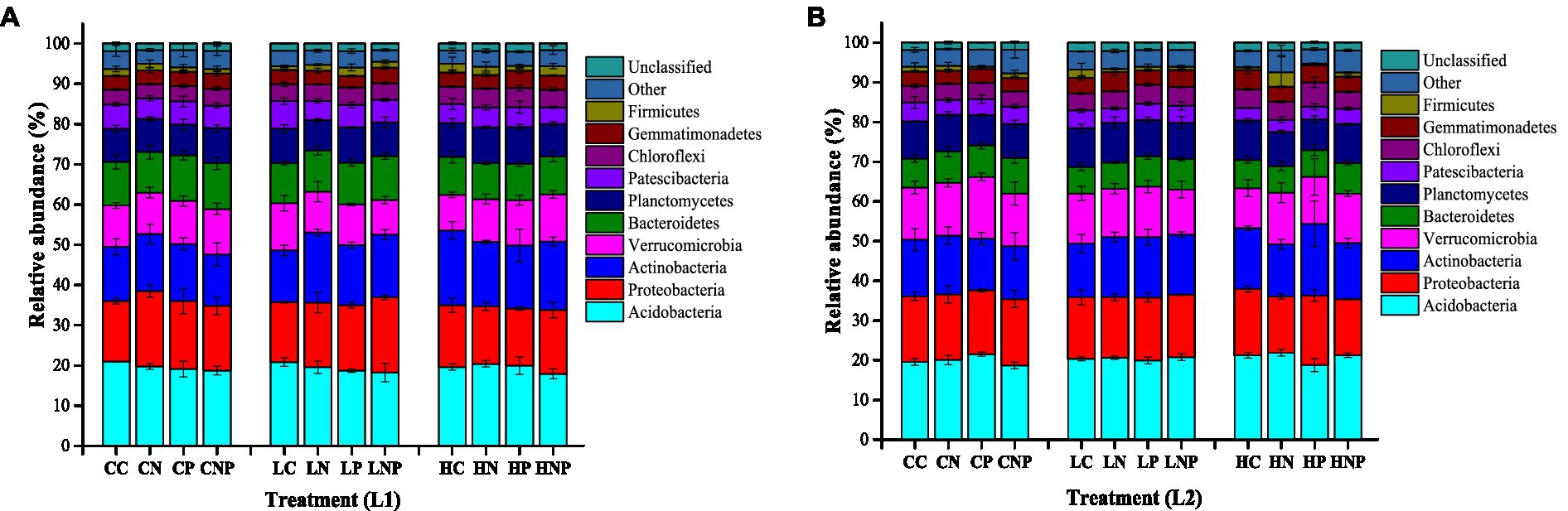
Figure 4. The relative abundance of the bacteria at phylum level under different grazing intensity and fertilizer application treatments (A) in 0–10 cm (L1) and (B) 10–20 cm (L2) soil. The treatments were: CC, control; CN, nitrogen fertilizer application alone without grazing; CP, phosphorus fertilizer application alone without grazing; CNP, nitrogen and phosphorus application without grazing; Light grazing: LC, light grazing without fertilizer application; LN, nitrogen fertilizer application alone under light grazing; LP, phosphorus fertilizer application alone under light grazing; LNP, nitrogen and phosphorus application under light grazing; Heavy grazing: HC, heavy grazing without fertilizer application; HN, nitrogen fertilizer application alone under heavy grazing; HP, phosphorus fertilizer application alone under heavy grazing; HNP, nitrogen and phosphorus application under heavy grazing. n = 3.
Comparing the no grazing, light grazing, and heavy grazing treatments, the relative abundance of Actinobacteria increased by 38.1 and 45.0% in response to HC vis-à-vis CC and LC, respectively. For Verrucomicrobia, its relative abundance increased by 36.6% in HNP when compared with LNP at 0–10 cm. The relative abundance of Acidobacteria rose by 11.1 and 13.7% in LNP and HNP, respectively, over CNP. Under the four different fertilizer application treatments, in 0–10 cm soil, the relative abundance of Actinobacteria was 35.8 and 21.7% higher in LN and LNP, respectively, than LC, while that of Proteobacteria increased by 25.1% in LNP relative to LC. The relative abundance of Verrucomicrobia was 28.1 and 33.0% greater in HP and HNP, respectively than in HC. For Acidobacteria, its relative abundance was reduced by 11.4, 14.2, and 11.6% in HP in comparison to HC, HN, and HNP. In the 10–20 cm soil, the relative abundance of Planctomycetes was 19.1 and 17.8% lower in CP than CC and CN, respectively, and in HC, it dropped by 21.7 and 21.0% versus HC and HNP, respectively.
3.4. Indicator bacteria in different treatments
In the 0–10 cm soil, the indicator bacteria of the HC group belonged to the genera Hymenobacter (phylum Bacteroidetes) and Phaselicystis and Phyllobacterium (phylum Proteobacteria; Supplementary Figure A2). The indicator bacteria of the LNP group at 0–10 cm were the genera Stenotrophomonas (phylum Acidobacteria) and Pseudomonas (phylum Proteobacteria). For the HN group, its indicator bacteria belonged to the genus Kosakonia (phylum Proteobacteria) in 10–20 cm soil (Supplementary Figure A2). The indicator bacteria of the HNP group were of the genus Methylobacterium (phylum Proteobacteria) in the 10–20 cm layer. The LEfSe analysis showed that the indicator bacteria were distributed among Actinobacteria in both LN and LNP at 0–10 cm and distributed among Proteobacteria in HP and HNP at 10–20 cm (Figure 5).
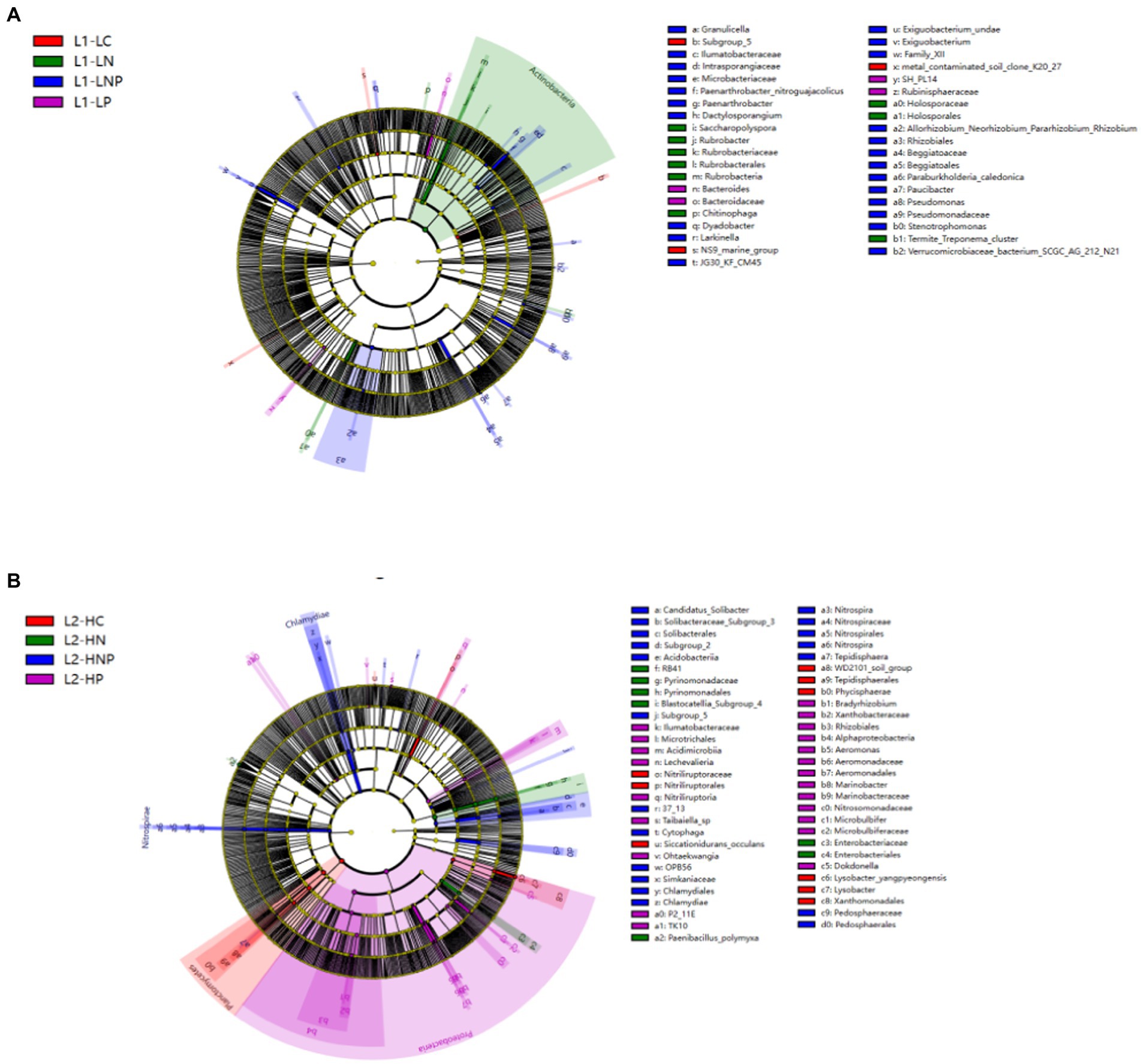
Figure 5. LEfSe analysis of each treated group in (A) bacterial community in 0–10 cm (L1) and (B) bacterial community in 10–20 cm (L2) soil. Cladograms were the phylogenetic distribution of bacteria under different treatment lineages associated with soils from the two treated groups.
3.5. Relationships of bacteria and soil properties
In the RDA, the first 20 bacterial phyla (whose summed relative abundances >97%) served as biological parameters, while the physicochemical properties of TC, TN, TP, DOC, AN, AP, and pH were used as the environmental parameters (Figure 6). The first two axes of the RDA model accounted for 70.21 and 74.36% of the total variance in the bacterial communities in the 0–10 and 10–20 cm depth layers, respectively. The results showed that AP had a stronger influence on the overall bacterial community at 0–10 cm, followed by TP, DOC, TC, TN, and AN. At 10–20 cm, TC, TN, and AN all had more pronounced effects on the overall bacterial community, followed by DOC, pH, TP, and AP.
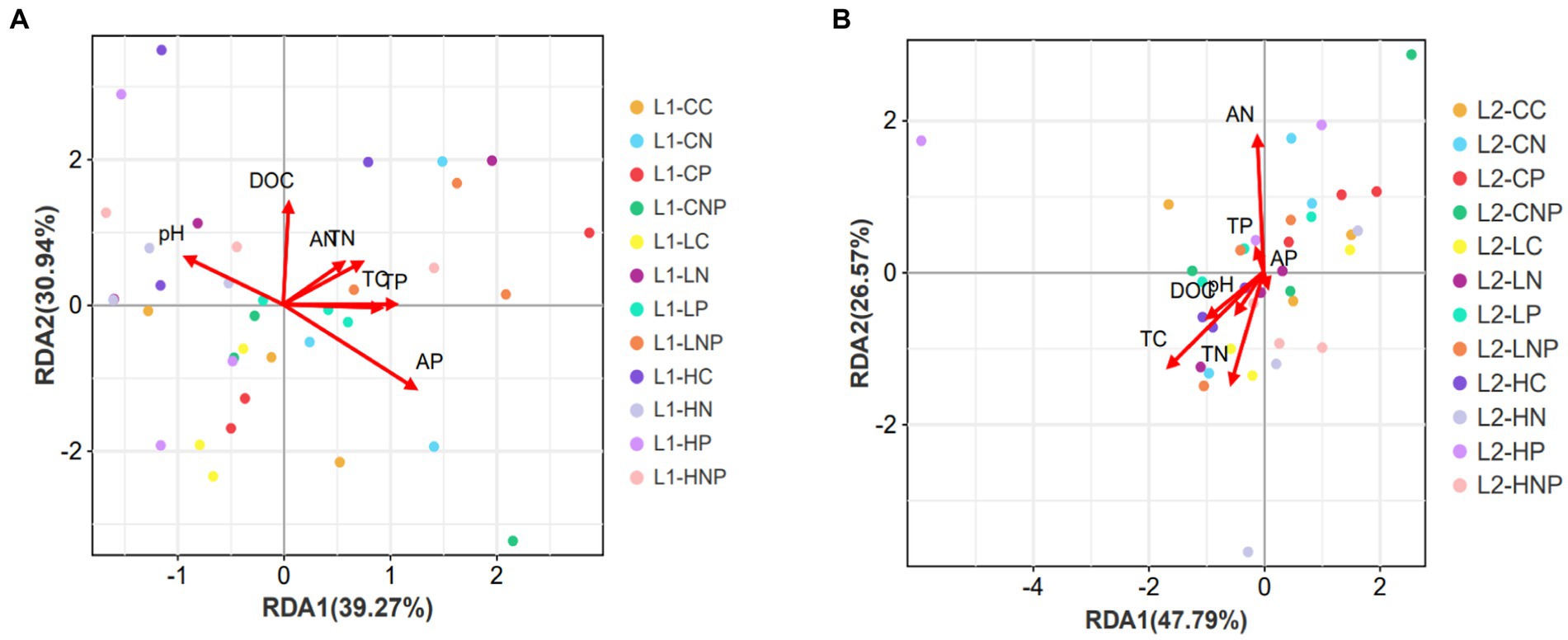
Figure 6. Redundancy analysis (RDA) between the 20 dominant bacteria phyla and seven soil properties (A) in 0–10 cm (L1) and (B) 10–20 cm (L2) soil. The arrow was the influence of environmental factors on biological factors; the length of which is proportional to the importance.
Pearson’s correlation analysis revealed that Chloroflexi was positively correlated with DOC in 0–10 cm soil, as was Nitrospirae with AN (Figure 7). At 0–10 cm, Bacteroidetes and Fibrobacteres were each positively correlated with AP, yet Acidobacteria was negatively correlated with TP. Soil pH was negatively correlated with Acidobacteria, Elusimicrobia, and Omnitrophicaeota in the 0–10 cm layer and likewise for Nitrospirae, Rokubacteria, and Thaumarchaeota. At 10–20 cm, soil TC was negatively correlated with Verrucomicrobia, Bacteroidetes, Armatimonadetes, Elusimicrobia, and Patesibacteraia, yet it was positively correlated with Rokubacteria. Soil TN was negatively correlated with Armatimonadetes but positively correlated with Gemmatimonadetes, Acidobacteria, and Nitrospirae in the 10–20 cm layer. Both Firmicutes and Omnitrophicaeota were negatively correlated with AN in 10–20 cm soil; in this layer, Cyanobacteria was negatively correlated with TP.
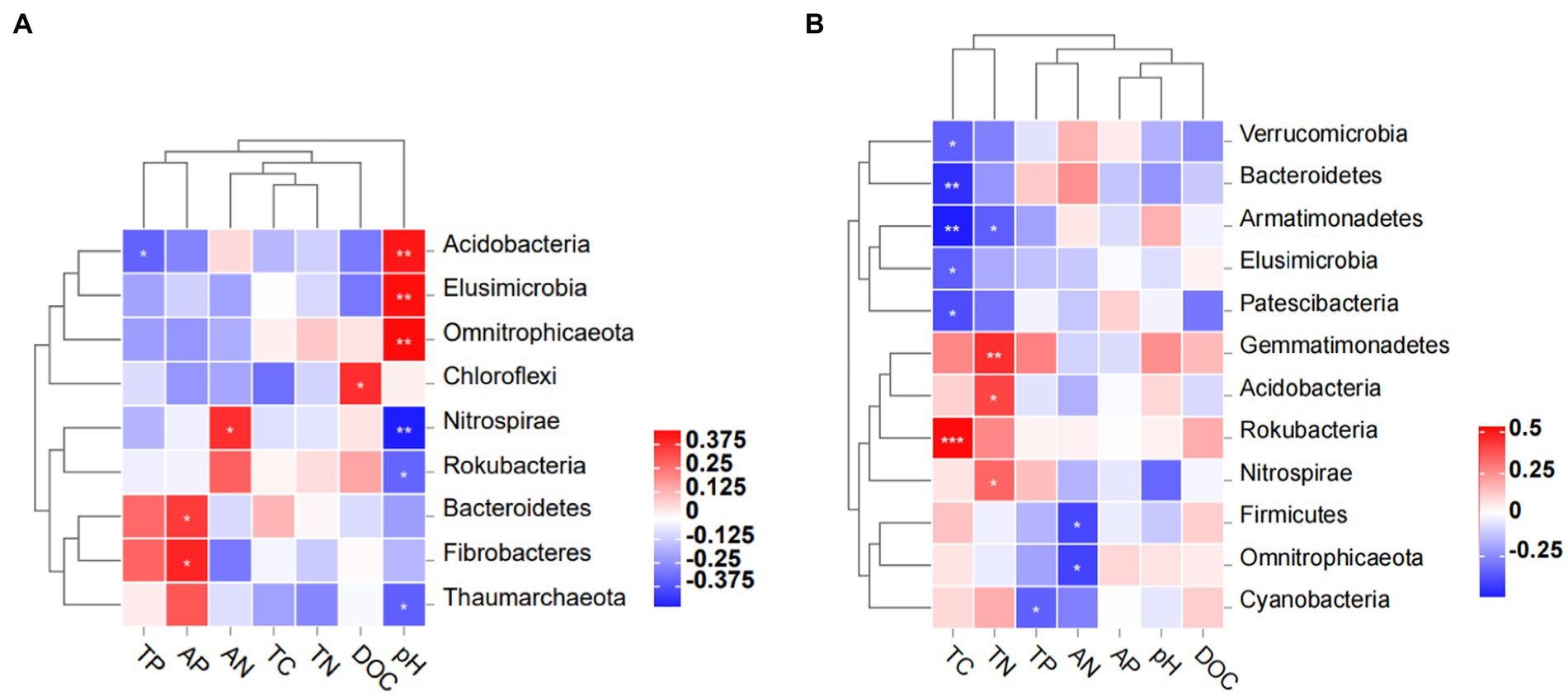
Figure 7. Pearson’s correlation analysis between the bacterial community and soil properties (A) in 0–10 cm (L1) soil and (B) in 10–20 cm (L2) soil depth. *, p < 0.05; **, p < 0.01; ***, p < 0.001.
The correlations between soil enzymes and dominant bacterial taxa showed that the relative abundance of Acidobacteria was positively correlated with ALP in the 0–10 cm layer (Table 5). At 10–20 cm, the relative abundance of Actinobacteria was negatively correlated with ACP as well as CAT (Table 6). The relative abundance of Verrucomicrobia was positively correlated with both ACP and ALP in 10–20 cm soil, but the relative abundance of Acidobacteria was negatively correlated with that of Proteobacteria in 10–20 cm soil.
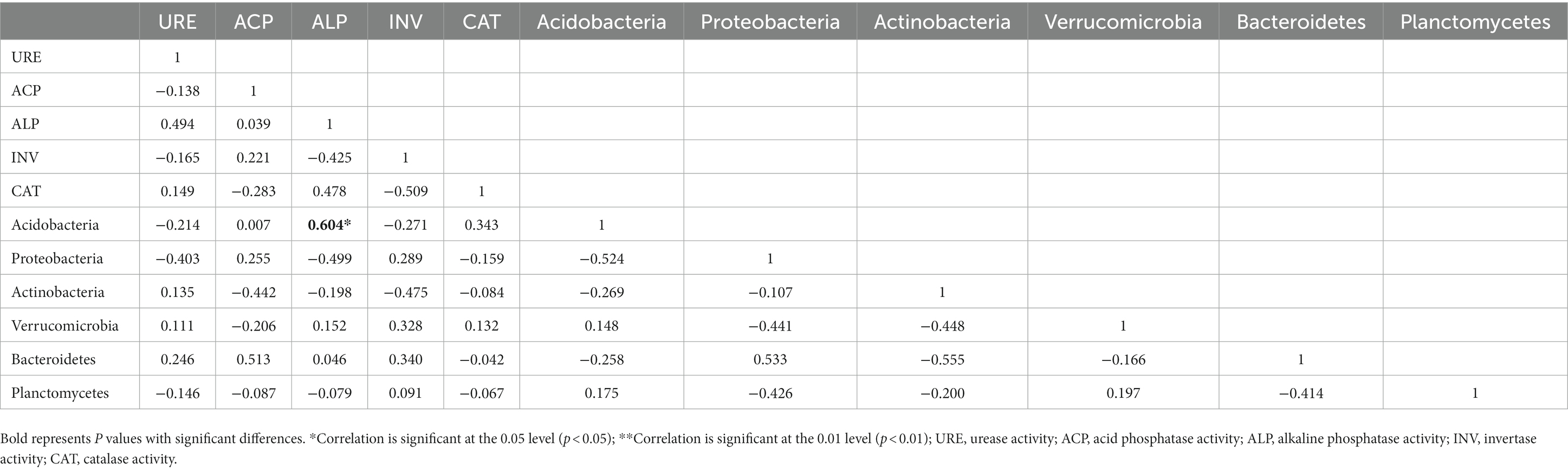
Table 5. Correlation analysis of the relative abundance of the dominant bacteria and soil enzyme activity in 0–10 cm soil.
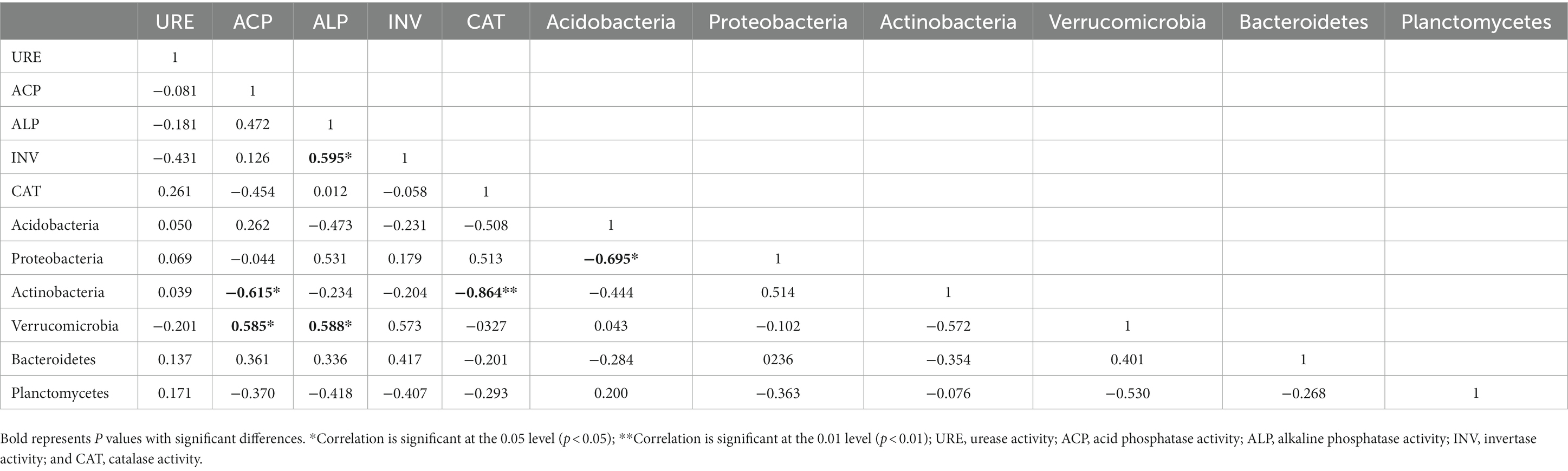
Table 6. Correlation analysis of the relative abundance of the dominant bacteria and soil enzyme activity in 10–20 cm soil.
4. Discussion
4.1. Soil properties
In response to LN, soil total nutrients (TC, TN, and TP) were lower at 0–10 cm than at 10–20 cm depths because the augmented bacterial diversity consumed more nutrients under light grazing with N fertilizer application in 0–10 cm than 10–20 cm soil (Supplementary Table S4; Wang et al., 2019; Wu et al., 2020). Soil available nutrients (AN and AP) were all bolstered in 0–10 cm relative to 10–20 cm under CN, CNP, and LNP because the application of fertilizers contributed more to AN and AP (Zi et al., 2017). Soil DOC increased in response to greater grazing intensity, likely because the labile C released into the rhizosphere by grazed plants stimulated extracellular enzyme activities, thereby enhancing soil C mineralization (Sun et al., 2017). The trampling of vegetation by livestock increased the physical fragmentation of litter, which accelerated its decomposition and carbon turnover (Wang et al., 2020). The AP was 122.5% higher in LC than CC because the urine of grazing sheep entered the soil via trampling effects, which in tandem also increased the input of N and P elements into the soil (Liu et al., 2018). Fertilizer application is an effective measure for grasslands to increase the yield of pasture (Chen et al., 2018). The enrichment of available nutrients in soil suggests a high metabolic efficiency of the belowground microenvironment (Legout et al., 2020). In this study, light grazing after N fertilizer application contributed to the accumulation of C in soil and the consumption of N and P because the N fertilizer application promoted soil carbon metabolism (Wang Z.H. et al., 2021). Light grazing after P fertilizer application was conducive to AP accumulation; this could be attributed to the fact that an increased nutrient input was positively correlated with the leaching of nitrate, ammonium, and phosphate (Apostolakis et al., 2022). The interactive effect of grazing intensity and fertilizer application significantly affected soil TC, TP, AN, and AP, suggesting that grazing affects the general soil nutrient structure by increasing the aboveground inputs from plant litter and animals’ excreta and the latter’s pulverization of the ground surface (Pavlu et al., 2019; Zhang et al., 2022).
4.2. Soil enzyme activity
Soil URE and ALP activities were 20–40% lower in 0–10 cm than in 10–20 cm in this study, which was consistent with the study of Yu et al. (2019). The reason was that the URE and ALP activities were affected by soil properties and microbial communities (Zhou et al., 2019), as well as the inputs and outputs of organic matter into the soils (Fanin et al., 2020) by the disturbance of grazing and fertilizer applications. Soil INV and CAT activities were 19–44% higher in 0–10 cm than in 10–20 cm in this study because soil INV and CAT activities had a higher organic matter in 0–10 cm than in 10–20 cm (Yang S.H. et al., 2020). Soil enzymes regulated microbial metabolic activities and energy conversion dynamics, mainly originating from plant roots and microbial secretions (Fan et al., 2020). Soil URE activity was higher in HC than in LC at 0–20 cm due to the input of AN from sheep manure (Cui and Holden, 2015). Soil INV activity was higher in LC than in CC at 10–20 cm because the beneficial nutrient for INV activity was moved to 10–20 cm by light grazing (Wu et al., 2020). Soil INV activity was higher in CN, CP, and CNP than in CC in 10–20 cm because the fertilizer application increased the root weight which bolstered the INV activity (Coura et al., 2020; Shao et al., 2020). That soil URE activity was higher in LN or LP than LC at 0–10 cm and also higher in LN, LP, and LNP than LC at 10–20 cm, demonstrating that fertilizer application and light grazing intensity increased the utilization rate of urea. In the 0–10 cm layer, soil CAT activity was lower in both HN and HNP than in HC. Further, soil URE activity was lower in HN than HC at 0–10 cm and also lower in HN, HP, and HNP than HC at 10–20 cm. This is likely because heavy grazing destroyed the soil surface structure, resulting in less soil macroaggregate and more soil microaggregate (Koppe et al., 2021; Luan et al., 2021).
4.3. Bacterial community compositions
The interactive effect of grazing and fertilization was beneficial to Actinobacteria, Proteobacteria, and Verrucomicrobia in 0–10 cm soil. The relative abundance of soil bacteria varied due to differences in soil properties, soil layers (Kang et al., 2021), and soil enzyme activities (Wang L. et al., 2022). In this study, the relative abundance of Bacteroidetes increased by 27.0–35.1% in 0–10 cm than in 10–20 cm under light grazing and fertilizer application (Supplementary Table S3), and the diversity indexes were higher in 0–10 cm than in 10–20 cm in some grazing and fertilizer application treatments (Supplementary Table S4). This was due to the fact that grazing changed bacterial diversity because of the change in soil moisture (Zhao et al., 2010). In addition, the addition of N changed bacterial communities by the decrease in soil pH in 0–10 cm than in 10–20 cm (Table 2; Chen et al., 2019). That heavy grazing increased the relative abundance of Actinobacteria is consistent with our finding that it also increased in HC than in CC and LC (Zhang Y.T. et al., 2020) The reason may be because of greater fecal matter deposition, which is high in partially digested plant residues with heavier grazing intensity provided substrate for Actinobacteria to decompose (Zhang Y.T. et al., 2020). Long-term overgrazing had an irreversible impact on soil properties and soil bacterial communities (Li et al., 2021), but the bacterial community was relatively stable under light-intensity grazing (Figures 4, 5; Beneduzi et al., 2019).
The relative abundances of Actinobacteria and Proteobacteria were higher in LNP than LC in 0–10 cm soil, probably because both taxa were regulated by N and P nutrients, whose addition via fertilizers was conducive to the survival and reproduction of these bacteria (Dai et al., 2018). In addition, RDA analysis showed that soil AN and AP were closely related to soil bacterial communities in this study because soil bacterial diversity was affected by the direct effects of N and P fertilizer application as well as the indirect effects of N and P fertilizer application through AN, AP, and the ratio of N to P (Dong et al., 2020). Soil bacterial diversity indexes were affected by N and P fertilizer applications (Wang Z.H. et al., 2021). Bacterial communities had a greater mean Shannon index in the 0–10 cm than 10–20 cm soil layer because the nutrient availability (such as available N and P) was higher in the soil upper layer than in the soil subsurface layer, which influenced the abundance and composition of microbial communities (Wang et al., 2019). The RDA analysis showed that AP was the highest explanation power to influence soil bacterial community in 0–10 cm (Figure 6A) due to its low mineralization in typical grassland, which resulted in a P limitation type for bacteria in this study area (Liu et al., 2022). Soil AP, pH, TP, DOC, TC, and TN all contributed to shaping the overall bacterial community in 0–10 cm because N and P found in animal excreta and the modification of N uptake and C exudation by frequently defoliated plants could jointly augment soil fertility in addition to enhancing microbial activities (Li Y. et al., 2020).
5. Conclusion
Grazing and fertilizer application had significant interactive effects on soil TC, URE activity, ACP activity, and CAT activity at 0–10 cm and significant interactive effects on soil pH, URE, ACP, and INV activity at 0–20 cm. Soil AN was increased by light grazing. Soil AP was increased by light grazing and P fertilizer application than in light grazing alone but was not different between HP and HC. Grazing intensity and fertilizer application both increased soil bacterial diversity by 0–10 cm more than in the 10–20 cm depth layer. Grazing and fertilizer application each had positive effects on the relative abundances of Actinobacteria, Proteobacteria, and Verrucomicrobia, yet it exerted negative effects on the relative abundances of Acidobacteria and Planctomycetes. The bacterial community responses to grazing and fertilization were closely correlated with soil URE, ALP, and AN. Fertilization with N and P could offset the negative effect of heavy grazing on grassland soil biodiversity. Therefore, light grazing with N or P fertilizer applications was appropriate to help soil nutrient accumulation and soil microbial community stability maintenance in the typical grassland. Furthermore, our results highlight the need for incorporating both grazing intensity and fertilizer application into the design and execution of future long-term biogeochemical studies.
Data availability statement
The original contributions presented in the study are included in the article/Supplementary material, further inquiries can be directed to the corresponding author.
Author contributions
WR: conceptualization, resources, and supervision. WR, LQ, and MZ: formal analysis and methodology. MZ, JY, SS, ZC, and TY: investigation and data collection. LQ, MZ, SS, and LG: writing. All authors contributed to the article and approved the submitted version.
Funding
This study was financially supported by the National Natural Science Foundation of China (No. 31872407), the National Natural Science Regional Foundation of China (Nos. 32060407 and 32260289), the National Natural Science Foundation of Inner Mongolia (Nos. 2020MS03029 and 2021BS03004), the Major Special Foundation of Science and Technology Plan of Inner Mongolia (Nos. 2022JBGS004003, 2021ZD00804, 2020ZD0020, and 2020GG0063), and the Project for the Innovation Team Development from the Department of Education of Inner Mongolia (NMGIRT2316).
Conflict of interest
The authors declare that the research was conducted in the absence of any commercial or financial relationships that could be construed as a potential conflict of interest.
Publisher’s note
All claims expressed in this article are solely those of the authors and do not necessarily represent those of their affiliated organizations, or those of the publisher, the editors and the reviewers. Any product that may be evaluated in this article, or claim that may be made by its manufacturer, is not guaranteed or endorsed by the publisher.
Supplementary material
The Supplementary material for this article can be found online at: https://www.frontiersin.org/articles/10.3389/fevo.2023.1174866/full#supplementary-material
References
Apostolakis, A., Schöning, I., Klaus, V. H., Michalzik, B., Bischoff, W. A., Boeddinghaus, R. S., et al. (2022). Direct and plant community mediated effects of management intensity on annual nutrient leaching risk in temperate grasslands. Nutr. Cycl. Agroecosyst. 123, 83–104. doi: 10.1007/s10705-022-10209-1
Bajouco, R., Fraga, I., Pinheiro, J., and Coutinho, J. (2020). Acid phosphomonoesterase and β-glucosidase activities in volcanic soils under permanent fertilized pastures: distribution profile and microbial effort toward P acquisition. Soil Sci. Plant Nutr. 66, 734–744. doi: 10.1080/00380768.2020.1814114
Beneduzi, A., Borges, L. G. D., Alvarenga, S. M., Faoro, H., Souza, E. M., Vargas, L. K., et al. (2019). Distinct grazing pressure loads generate different impacts on bacterial community in a long-term experiment in Pampa biome. Appl. Soil Ecol. 137, 167–177. doi: 10.1016/j.apsoil.2019.02.005
Bhagat, S. A., and Kokitkar, S. S. (2021). Isolation and identification of bacteria with cellulose-degrading potential from soil and optimization of cellulase production. J. Appl. Biol. Biotechnol. 9, 1–6. doi: 10.7324/JABB.2021.96020
Che, R. X., Qin, J. L., Tahmasbian, I., Wang, F., Zhou, S. T., Xu, Z. H., et al. (2018). Litter amendment rather than phosphorus can dramatically change inorganic nitrogen pools in a degraded grassland soil by affecting nitrogen-cycling microbes. Soil Biol. Biochem. 120, 145–152. doi: 10.1016/j.soilbio.2018.02.006
Chen, Y. L., Liu, J. T., and Liu, S. T. (2018). Effect of long-term mineral fertilizer application on soil enzyme activities and bacterial community composition. Plant Soil Environ. 64, 571–577. doi: 10.17221/658/2018-PSE
Chen, D. M., Xing, W., Lan, Z., Saleem, M., Wu, Y., Hu, S., et al. (2019). Direct and indirect effects of nitrogen enrichment on soil organisms and carbon and nitrogen mineralization in a semi-arid grassland. Funct. Ecol. 33, 175–187. doi: 10.1111/1365-2435.13299
Cheng, X. Y., Yun, Y., Wang, H. M., Ma, L. Y., Tian, W., Man, B. Y., et al. (2021). Contrasting bacterial communities and their assembly processes in karst soils under different land use. Sci. Total Environ. 751:142263. doi: 10.1016/j.scitotenv.2020.142263
Coura, F. T. V., Fries, D. D., Quoos, R. D., Teixeira, F. A., Pires, A. J. V., and Figueiredo, A. J. D. (2020). Activity invertase and amylase in Marandu grass under shading and nitrogen fertilization. Acta Sci. Agron. 42:e42496. doi: 10.4025/actasciagron.v42i1.42496
Cui, J. F., and Holden, N. M. (2015). The relationship between soil microbial activity and microbial biomass, soil structure and grassland management. Soil Tillage Res. 146, 32–38. doi: 10.1016/j.still.2014.07.005
Dai, Z. M., Su, W. Q., Chen, H. H., Barberán, A., Zhao, H. C., Yu, M. J., et al. (2018). Long-term nitrogen fertilization decreases bacterial diversity and favors the growth of Actinobacteria and Proteobacteria in agro-ecosystems across the globe. Glob. Chang. Biol. 24, 3452–3461. doi: 10.1111/gcb.14163
Dong, J. F., Che, R. X., Jia, S. G., Wang, F., Zhang, B., Cui, X. Y., et al. (2020). Responses of ammonia-oxidizing archaea and bacteria to nitrogen and phosphorus amendments in an alpine steppe. Eur. J. Soil Sci. 71, 940–954. doi: 10.1111/ejss.12911
Eldridge, D. J., and Delgado-Baquerizo, M. (2017). Continental-scale impacts of livestock grazing on ecosystem supporting and regulating services. Land Degrad. Dev. 28, 1473–1481. doi: 10.1002/ldr.2668
Eldridge, D. J., Delgado-Baquerizo, M., Travers, S. K., Val, J., Oliver, I., Hamonts, K., et al. (2017). Competition drives the response of soil microbial diversity to increased grazing by vertebrate herbivores. Ecology 98, 1922–1931. doi: 10.1002/ecy.1879
Fan, Z. Z., Lu, S. Y., Liu, S., Li, Z. R., Hong, J. X., Zhou, J. X., et al. (2020). The effects of vegetation restoration strategies and seasons on soil enzyme activities in the karst landscapes of Yunnan, Southwest China. J. For. Res. 31, 1949–1957. doi: 10.1007/s11676-019-00959-0
Fan, D. W., Wang, S. Y., Guo, Y. H., Liu, J., Agathokleous, E., Zhu, Y. L., et al. (2021). The role of bacterial communities in shaping cd-induced hormesis in ‘living’soil as a function of land-use change. J. Hazard. Mater. 409:124996. doi: 10.1016/j.jhazmat.2020.124996
Fanin, N., Alavoine, G., and Bertrand, I. (2020). Temporal dynamics of litter quality, soil properties and microbial strategies as main drivers of the priming effect. Geoderma 377:114576. doi: 10.1016/j.geoderma.2020.114576
Gao, Y. F., Liu, H. L., and Liu, G. X. (2017). The spatial distribution and accumulation characteristics of heavy metals in steppe soils around three mining areas in Xilinhot in Inner Mongolia. Environ. Sci. Pollut. Res. 24, 25416–25430. doi: 10.1007/s11356-017-0113-0
He, Y. T., Xu, M. G., Qi, Y. C., Dong, Y. S., He, X. H., Li, J. W., et al. (2017). Differential responses of soil microbial community to four-decade long grazing and cultivation in a semi-arid grassland. Sustain. For. 9:128. doi: 10.3390/su9010128
Jiang, H. M., Chen, Y. C., Hu, Y., Wang, Z. W., and Lu, X. Y. (2021). Soil bacterial communities and diversity in alpine grasslands on the Tibetan plateau based on 16S rRNA gene sequencing. Front. Ecol. Evol. 9:630722. doi: 10.3390/su9010128
Kang, B. T., Bowatte, S., and Hou, F. J. (2021). Soil microbial communities and their relationships to soil properties at different depths in an alpine meadow and desert grassland in the Qilian mountain range of China. J. Arid Environ. 184:104316. doi: 10.1016/j.jaridenv.2020.104316
Koppe, E., Rupollo, C. Z., de Queiroz, R., Puschmann, D. U., Peth, S., and Reinert, D. (2021). Physical recovery of an oxisol subjected to four intensities of dairy cattle grazing. Soil Tillage Res. 206:104813. doi: 10.1016/j.still.2020.104813
Legout, A., Hansson, K., van der Heijden, G., Laclau, J. P., Mareschal, L., Nys, C., et al. (2020). Chemical fertility of forest ecosystems. part 2: towards redefining the concept by untangling the role of the different components of biogeochemical cycling. For. Ecol. Manag. 461:117844. doi: 10.1016/j.foreco.2019.117844
Li, J. J., Huang, Y., Xu, F. W., Wu, L. J., Chen, D. M., and Bai, Y. F. (2018). Responses of growing-season soil respiration to water and nitrogen addition as affected by grazing intensity. Funct. Ecol. 32, 1890–1901. doi: 10.1111/1365-2435.13118
Li, Y., Liu, Y. W., Pan, H., Hernández, M., Guan, X. M., Wang, W., et al. (2020). Impact of grazing on shaping abundance and composition of active methanotrophs and methane oxidation activity in a grassland soil. Biol. Fertil. Soils 56, 799–810. doi: 10.1007/s00374-020-01461-0
Li, M., Wang, G. X., Kang, X. M., Hu, H. L., Wang, Y., Zhang, X. R., et al. (2020). Long-term fertilization alters microbial community but fails to reclaim soil organic carbon stocks in a land-use changed soil of the Tibetan plateau. Land Degrad. Dev. 31, 531–542. doi: 10.1002/ldr.3469
Li, S. X., Wang, Y. L., Wang, Y. Q., and Yin, Y. L. (2021). Response of soil bacterial community characteristics to alpine meadow degradation. Biodivers. Sci. 29, 53–64. doi: 10.17520/biods.2020137
Li, W. H., Xu, F. W., Zheng, S. X., Friedhelm, T., and Bai, Y. F. (2017). Patterns and thresholds of grazing-induced changes in community structure and ecosystem functioning: species-level responses and the critical role of species traits. J. Appl. Ecol. 54, 963–975. doi: 10.1111/1365-2664.12806
Li, J. Y., Zhang, Q. C., Li, Y., Liu, J., Pan, H., Guan, X. M., et al. (2017). Impact of mowing management on nitrogen mineralization rate and fungal and bacterial communities in a semiarid grassland ecosystem. J. Soils Sediments 17, 1715–1726. doi: 10.1007/s11368-016-1620-1
Liu, S. B., He, F. K., Kuzyakov, Y., Xiao, H. X., Hoang, D. T. T., Pu, S. Y., et al. (2022). Nutrients in the rhizosphere: a meta-analysis of content, availability, and influencing factors. Sci. Total Environ. 826:153908. doi: 10.1016/j.scitotenv.2022.153908
Liu, W. L., Jiang, Y. L., Wang, G. X., Su, Y., Smoak, J. M., Liu, M. Y., et al. (2021). Effects of N addition and clipping on above and belowground plant biomass, soil microbial community structure, and function in an alpine meadow on the Qinghai-Tibetan plateau. Eur. J. Soil Biol. 106:103344. doi: 10.1016/j.ejsobi.2021.103344
Liu, F. D., Mo, X., Kong, W. J., and Song, Y. (2020). Soil bacterial diversity, structure, and function of suaeda salsa in rhizosphere and non-rhizosphere soils in various habitats in the yellow river delta, China. Sci. Total Environ. 740:140144. doi: 10.1016/j.scitotenv.2020.140144
Liu, D., Song, X. Y., Lin, Y., and Wang, C. T. (2023). Effects of phosphorus application on soil phosphorus forms and phoD-harboring microbial communities in an alpine grassland on the Qinghai-Tibetan plateau. Front. Ecol. Evol. 11:1131408. doi: 10.3389/fevo.2023.1131408
Liu, C., Wang, L., Song, X. X., Chang, Q., Frank, D. A., Wang, D. L., et al. (2018). Towards a mechanistic understanding of the effect that different species of large grazers have on grassland soil N availability. J. Ecol. 106, 357–366. doi: 10.1111/1365-2745.12809
Luan, H. A., Shuo, Y. U. A. N., Wei, G. A. O., Tang, J. W., Li, R. N., Zhang, H. Z., et al. (2021). Changes in organic C stability within soil aggregates under different fertilization patterns in a greenhouse vegetable field. J. Integr. Agric. 20, 2758–2771. doi: 10.1016/S2095-3119(21)63646-8
Ma, Z. Q., Zhang, X. Y., Zhang, C., Wang, H. M., Chen, F. S., Fu, X. L., et al. (2018). Accumulation of residual soil microbial carbon in chinese fir plantation soils after nitrogen and phosphorus additions. J. For. Res. 29, 953–962. doi: 10.1007/s11676-017-0522-4
Pavlu, K., Kassahun, T., Nwaogu, C., Pavlu, L., Gaisler, J., Homolka, P., et al. (2019). Effect of grazing intensity and dung on herbage and soil nutrients. Plant Soil Environ. 65, 343–348. doi: 10.17221/177/2019-PSE
Qi, L., Pokharel, P., Chang, S. X., Zhou, P., Niu, H. D., He, X. H., et al. (2020a). Biochar application increased methane emission, soil carbon storage and net ecosystem carbon budget in a 2-year vegetable–rice rotation. Agric. Ecosyst. Environ. 292:106831. doi: 10.1016/j.agee.2020.106831
Qi, L., Zhou, P., Yang, L. S., and Gao, M. (2020b). Effects of land reclamation on the physical, chemical, and microbial quantity and enzyme activity properties of degraded agricultural soils. J. Soils Sediments 20, 973–981. doi: 10.1007/s11368-019-02432-1
Shao, K., Bai, Z. Q., Li, M. H., Yu, C., Shao, J. W., Sun, Y. Q., et al. (2020). Sucrose metabolism enzymes affect sucrose content rather than root weight in sugar beet (Beta vulgaris) at different growth stages. Sugar Tech 22, 504–517. doi: 10.1007/s12355-019-00781-2
Soares, C. V., Cecato, U., Ribeiro, O. L., Roma, C. F., Jobim, C. C., Beloni, T., et al. (2013). Root system and root and stem base organic reserves of pasture tanzania grass fertilizer with nitrogen under grazing. Semina Ciencias Agrarias 34:2415. doi: 10.5433/1679-0359.2013v34n5p2415
Sun, X. D., Ye, Y. Q., Ma, Q. X., Guan, Q. W., and Davey, L. J. (2021). Variation in enzyme activities involved in carbon and nitrogen cycling in rhizosphere and bulk soil after organic mulching. Rhizosphere 19:100376. doi: 10.1016/j.rhisph.2021.100376
Sun, G., Zhu-Barker, X., Chen, D. M., Liu, L., Zhang, N. N., Shi, C. G., et al. (2017). Responses of root exudation and nutrient cycling to grazing intensities and recovery practices in an alpine meadow: an implication for pasture management. Plant Soil 416, 515–525. doi: 10.1007/s11104-017-3236-7
Tao, D. X., Chen, T. P., Luo, Y., Wu, H. H., Wang, X. Y., Wang, J., et al. (2021). Belowground net primary productivity and biomass allocation in response to different restoration measures in a salt-alkali-degraded Songnen meadow. Ecol. Indic. 130:108038. doi: 10.1016/j.ecolind.2021.108038
Wang, Z., Ding, Y., Jin, K., Struik, P. C., Sun, S. X., Ji, B. M., et al. (2022a). Soil bacterial and fungal communities are linked with plant functional types and soil properties under different grazing intensities. Eur. J. Soil Sci. 73:e13195. doi: 10.1111/ejss.13195
Wang, Z., Jiang, S. Y., Struik, P. C., Wang, H., Jin, K., Wu, R. L. G., et al. (2022b). Plant and soil responses to grazing intensity drive changes in the soil microbiome in a desert steppe. Plant Soil 02. doi: 10.1007/s11104-022-05409-1
Wang, Y. N., Li, F. Y., Song, X., Wang, X. S., Suri, G., and Baoyin, T. G. T. (2020). Changes in litter decomposition rate of dominant plants in a semi-arid steppe across different land-use types: soil moisture, not home-field advantage, plays a dominant role. Agric. Ecosyst. Environ. 303:107119. doi: 10.1016/j.agee.2020.107119
Wang, Z. H., Wang, Z. R., Li, T. P., Wang, C., Dang, N., Wang, R. Z., et al. (2021). N and P fertilization enhanced carbon decomposition function by shifting microbes towards an r-selected community in meadow grassland soils. Ecol. Indic. 132:108306. doi: 10.1016/j.ecolind.2021.108306
Wang, L., Yu, X. J., Xu, C. L., Jing, Y. Y., and Song, M. J. (2022). Grazing by Tibetan sheep enhances soil bacterial and fungal diversity in cold season pastures of alpine meadows on the northern Qinghai–Tibetan plateau. J. Soil Sci. Plant Nutr. 22, 2434–2456. doi: 10.1007/s42729-022-00819-7
Wang, Z., Zhang, Q., Staley, C., Gao, H. L., Ishii, S., Wei, X. R., et al. (2019). Impact of long-term grazing exclusion on soil microbial community composition and nutrient availability. Biol. Fertil. Soils 55, 121–134. doi: 10.1007/s00374-018-01336-5
Wang, C. L., Zhang, R., Vilonen, L., Qu, Y. N., Fu, X., Shi, B. K., et al. (2021). Grazing and nitrogen addition restructure the spatial heterogeneity of soil microbial community structure and enzymatic activities. Funct. Ecol. 35, 2763–2777. doi: 10.1111/1365-2435.13926
Wu, J. Q., Wang, H. Y., Li, G., Ma, W. W., Wu, J. H., Gong, Y., et al. (2020). Vegetation degradation impacts soil nutrients and enzyme activities in wet meadow on the Qinghai-Tibet plateau. Sci. Rep. 10, 21271–21217. doi: 10.1038/s41598-020-78182-9
Xu, T., Chen, X., and Hou, Y. H. (2021). Changes in microbial biomass, community composition and diversity, and functioning with soil depth in two alpine ecosystems on the Tibetan plateau. Plant Soil 459, 137–153. doi: 10.1007/s11104-020-04712-z
Xu, F. W., Li, J. J., Wu, L. J., Lu, X. M., Xing, W., Chen, D. M., et al. (2020). Resource enrichment combined with biomass removal maintains plant diversity and community stability in a long-term grazed grassland. J. Plant Ecol. 13, 611–620. doi: 10.1093/jpe/taa046
Yan, T. T., Xue, J. H., Zhou, Z. D., and Wu, Y. B. (2021). Biochar-based fertilizer amendments improve the soil microbial community structure in a karst mountainous area. Sci. Total Environ. 794:148757. doi: 10.1016/j.scitotenv.2021.148757
Yang, W., Cai, A. D., Wang, J. S., Luo, Y. Q., Cheng, X. L., and An, S. Q. (2020). Exotic Spartina alterniflora Loisel. invasion significantly shifts soil bacterial communities with the successional gradient of saltmarsh in eastern China. Plant Soil 449, 97–115. doi: 10.1007/s11104-020-04470-y
Yang, S. H., Chen, X., Jiang, Z. W., Ding, J., Sun, X., and Xu, J. Z. (2020). Effects of biochar application on soil organic carbon composition and enzyme activity in paddy soil under water-saving irrigation. Int. J. Environ. Res. Public Health 17:333. doi: 10.3390/ijerph17010333
Yin, J. J., Guo, H. Q., Ellen, L. F., Jonathan, R. L., Tang, S. M., Yuan, T., et al. (2022). Plant roots send metabolic signals to microbes in response to long-term overgrazing. Sci. Total Environ. 842:156241. doi: 10.1016/j.scitotenv.2022.156241
Yu, P. J., Tang, X. G., Zhang, A. C., Fan, G. H., and Liu, S. W. (2019). Responses of soil specific enzyme activities to short-term land use conversions in a salt-affected region, northeastern China. Sci. Total Environ. 687, 939–945. doi: 10.1016/j.scitotenv.2019.06.171
Zhang, Y. T., Gao, X. L., Hao, X. Y., Alexander, T. W., Shi, X. J., Jin, L., et al. (2020). Heavy grazing over 64 years reduced soil bacterial diversity in the foothills of the Rocky Mountains, Canada. Appl. Soil Ecol. 147:103361. doi: 10.1016/j.apsoil.2019.09.011
Zhang, G. G., Huang, J., Jia, M. Q., Huang, W., Sui, X., Wang, Z. W., et al. (2019). Effect of different grazing intensities on bacterial community composition and diversity in rhizosphere and non-rhizosphere soils in desert steppe of China. Chil. J. Agri. Res. 79, 531–546. doi: 10.4067/S0718-58392019000400531
Zhang, T. R., Li, F. Y., Shi, C. J., Li, Y. L., Tang, S. M., and Baoyin, T. G. T. (2020). Enhancement of nutrient resorption efficiency increases plant production and helps maintain soil nutrients under summer grazing in a semi-arid steppe. Agric. Ecosyst. Environ. 292:106840. doi: 10.1016/j.agee.2020.106840
Zhang, N. N., Sun, G., Liang, J., Wang, E. T., Shi, C. G., He, J., et al. (2018). Response of ammonium oxidizers to the application of nitrogen fertilizer in an alpine meadow on the Qinghai-Tibetan plateau. Appl. Soil Ecol. 124, 266–274. doi: 10.1016/j.apsoil.2017.11.018
Zhang, X. R., Zhang, W. Q., Sai, X., Chun, F., Li, X. J., Lu, X. X., et al. (2022). Grazing altered soil aggregates, nutrients and enzyme activities in a Stipa kirschnii steppe of Inner Mongolia. Soil Tillage Res. 219:105327. doi: 10.1016/j.still.2022.105327
Zhang, J., Zuo, X. A., Zhou, X., Lv, P., Lian, J., and Yue, X. Y. (2017). Long-term grazing effects on vegetation characteristics and soil properties in a semiarid grassland, northern China. Environ. Monit. Assess. 189, 216–213. doi: 10.1007/s10661-017-5947-x
Zhao, Y. Y., Liu, Z. F., and Wu, J. G. (2020). Grassland ecosystem services: a systematic review of research advances and future directions. Landsc. Ecol. 35, 793–814. doi: 10.1007/s10980-020-00980-3
Zhao, Y., Peth, S., Horn, R., Krümmelbein, J., Ketzer, B., Gao, Y. Z., et al. (2010). Modeling grazing effects on coupled water and heat fluxes in Inner Mongolia grassland. Soil Tillage Res. 109, 75–86. doi: 10.1016/j.still.2010.04.005
Zhou, J. Q., Zhang, F. G., Huo, Y. Q., Wilson, G. W. T., Cobb, A. B., Xu, X. X., et al. (2019). Following legume establishment, microbial and chemical associations facilitate improved productivity in degraded grasslands. Plant Soil 443, 273–292. doi: 10.1007/s11104-019-04169-9
Keywords: bacterial community structures, fertilizer application, grazing, soil enzyme activity, soil nutrients
Citation: Qi L, Zhang M, Yin J, Ren W, Sun S, Chen Z, Yuan T and Guo L (2023) The interactive effect of grazing and fertilizer application on soil properties and bacterial community structures in a typical grassland in the central Inner Mongolia Plateau. Front. Ecol. Evol. 11:1174866. doi: 10.3389/fevo.2023.1174866
Edited by:
Dima Chen, China Three Gorges University, ChinaReviewed by:
Bing Wang, China Three Gorges University, ChinaFengwei Xu, Chinese Academy of Forestry, China
Copyright © 2023 Qi, Zhang, Yin, Ren, Sun, Chen, Yuan and Guo. This is an open-access article distributed under the terms of the Creative Commons Attribution License (CC BY). The use, distribution or reproduction in other forums is permitted, provided the original author(s) and the copyright owner(s) are credited and that the original publication in this journal is cited, in accordance with accepted academic practice. No use, distribution or reproduction is permitted which does not comply with these terms.
*Correspondence: Weibo Ren, cnBwY2F1Y2F1QDE2My5jb20=
†These authors have contributed equally to this work