- 1Key Laboratory of Artificial Intelligence Application Technology State Ethnic Affairs Commission, Qinghai Minzu University, Xining, China
- 2Tianjin Key Laboratory of Cognitive Computing and Application, Tianjin University, Tianjin, China
Several excavations at the Hemudu site in Zhejiang Province have led to the discovery of more than 100 pieces of bird bones that were likely used to create a kind of musical instrument. However, in contrast to the Jiahu bone flute, it is not clear for what the Hemudu bone whistle was used, although it was probably used for musical purposes. In this paper, the 7000-year-old Hemudu bone whistle is reproduced with 3D scanning and printing technology. Therefore, we propose a robust scan point detection and tracking method that includes three main stages. First, we conducted robust point detection and selection where noise and irrelevant points were removed. Then, we propose to track the key points and conduct 3D model optimization. With the above efforts, we obtained the 3D model of the bone whistle, and the audio of the bone whistle is replicated. The purpose of the bone whistles is inferred and evaluated through audio analyses. The experimental results show that the method is feasible.
1 Introduction
The Hemudu site is approximately 7,000 years old. Chinese archaeologists have conducted two archaeological excavations at the Hemudu site in Zhejiang Province. During the first excavation in 1973-1974, archaeologists discovered a total of 45 bone tools that were similar in shape to pipes. The bone pipes varied from 6 to 10cm in length, and they are hollow structures, that are slightly curved with round holes in their convex arcs (Anonymous, 1978). This kind of tubular bone tool with holes has also been discovered at other Neolithic sites in China. These bone tools can be divided into two categories: monoporous and porous. These single-hole bone artifacts have been discovered at various sites, including Luojiajiao in Tongxiang, Zhejiang (Ruzuo, 1996), Changge Shigu in Henan (Yanping, 1989), Meiyan in Wujiang, Jiangsu (Yuyin, 1963), and Dahezhuang in Yongjing, Gansu (Anonymous, 1974). Porous bone artifacts were found in Xiaoshan Kuahu Bridge in Zhejiang (Zhimao, 2004), Jiahu Wuyang in Henan (Xingbang, 2001), Zhongshanzhai in Ruzhou in Henan (Naiwu and Zhonghe, 1991), Nuomuhong Talitaliha in Qinghai (Ruxiang, 1963) and other sites.
Among these sites, a large number of bone flutes were unearthed at the Wuyang Jiahu site in Henan Province. These bone flutes were made of bird limb bones. They are khaki in color, constructed with excellent workmanship and can be used in various purposes. Furthermore, modern and simple music can be restored to some extent (Fei et al., 2004). However, compared with the Jiahu bone flute, which is more complex and mature in terms of craftsmanship and structure, most Hemudu bone whistles have only one or two purposes. Various archaeologists have speculated on the true use of the bone whistle, and no consistent conclusions have been drawn. To date, archaeologists have excavated many bone whistles and bone flutes at various Neolithic sites in China. These bone tools are a popular topic of research in the field of ancient Chinese musical instruments and the history of Chinese folk music. Historical research on folk music has shown that the bone flute played diverse roles in the Neolithic Age. The bone flute can be used not only as a sacrificial utensil but also as a musical instrument. Furthermore, compared with the bone whistles unearthed in the Jiangsu-Zhejiang area, these bone whistles have deeper application and usage value.
In 1979, Zhao Songting, a famous dizi player in Zhejiang, Lu Ji, the then chairman of the Chinese Musicians Association, and others tested the Hemudu bone whistle. After testing, they arrived at the conclusion that “the Hemudu bone whistle is the oldest musical instrument”. Furthermore, they compared the Hemudu bone whistle with the six-hole bamboo flute and found that the Hemudu bone whistle was a precursor to the six-hole bamboo flute. Since the six-hole bamboo flute was one of the first musical instruments associated with archaeological bone pipes, the Hemudu bone whistle is considered to be the originator of all wind instruments. These perforated bone pipes found in Hemudu reflect the transition to weaving in prehistoric human textile activities, indicating that a tubular twisted warp weaving method was likely used to produce long and narrow ribbon-shaped textiles 7,000 years ago. The study of history is extremely important (Yongjia, 2012). This work includes a novel and unique conjecture on the Hemudu bone whistle. The long Jiahu bone flute has multiple holes, can produce a variety of different tones, and has been proven to be a seven-tempered sound instrument. Regarding these wind instruments, some articles on bone whistle research include “Research on the “Bone Whistles” Unearthed at Hemudu Site” (Yongjia, 2012) and “Mystery of the Bone Whistles of Ancient Chinese Musical Instruments” (Ximin, 1986).With the robust approach of visual object trackers, problems such as blurred motion are prevented (Guo et al., 2021a, Guo et al., 2020, Jiping, 2005; Guo et al., 2021b). Different works have speculated that the bone whistle may have been a musical instrument. In addition, the bone whistle may have been a textile tool, a warning tool, or a birdsong imitation tool. Thus, there are different opinions on the use of bone whistles. Therefore, to study the vocal pattern of bone whistles, the ancient bone whistle must be scanned and analyzed. In this paper, the bone whistle is digitized and printed using 3D printing technology. As a result, permanent damage that may be caused by playing the bone whistle directly can be prevented. After the bird whistle was printed, recording equipment in a recording studio was used to record the sound in all directions. It is necessary to play, record, and analyze the acoustics and temperament by changing the playing method to speculate regarding the use of the bone whistle from the perspective of acoustics.
2 3D data and challenges with modeling bone whistles
2.1 Introduction of 3D scanning instruments
A three-dimensional scanner, also known as a three-dimensional stereo scanner, is a high-tech product that integrates optical, mechanical, electrical and computer technologies. 3D scanners are mainly used to obtain the 3D coordinates of the outer surfaces of objects and 3D digital models of objects. This equipment can be used not only in the fields of product reverse engineering and 3D inspection but also for the continuous development of 3D scanning technology, such as 3D film and television animation, digital exhibition halls, custom clothing, and computer virtual reality simulation and visualization. Many industries have begun to use 3D scanners as a convenient means for creating digital models of physical objects. Through the noncontact scanning of a physical model with the 3D scanner, accurate 3D point cloud data of the physical surface can be obtained, and a digital model of the physical object can be generated. This process is not only fast but also has high precision. The 3D form is a realistic reproduction of the real-world object.
2.2 3D bone whistle data
The scanner was connected to a laptop before the scan began. The necessary software, including Vison Studio and Geomagic Studio, was installed according to the instructions. After the software was installed, the format of the scanner and the clarity and brightness of the camera were adjusted and calibrated. All the above preparations should be set as shown in Figure 1.
In this paper, 9 representative bone whistles that were obtained during archaeological excavations in the Yuyao area were selected for scanning. Therefore, we scanned 9 bone whistles that were obtained in the Hemudu and Tianluoshan areas. The scanned data are shown in Table 1. Table 2 shows the definitions of the bone whistle labels.
Figures 2, 3 show schematic diagrams of the strata in the Hemudu and Tianluoshan areas.
The marked point paste method has several precautions that should be considered:
● The marking points should be randomly distributed on the surface of the measured object and clearly visible in the camera window. The marking points should not be on a straight line and should instead be distributed in a V shape. The marked points should remain on the surface of the object as much as possible.
● Before the marking point is placed on the object, it should be decided whether the marking point should be attached to the scanned object, around the scanned object, or both. The advantage of placing the marking points on the surface of the object is that the object can move freely; however, this slightly affects the 3D data of the surface that is covered by the marker points. When the marking point is placed around the scanned object, the 3D data of the surface of the object is not affected; however, during the acquisition process, the relative movement between the scanned object and the object attached to the marked point must be maintained.
● The size of the marking point should be selected appropriately. If the size is not properly selected, the marker may not be recognized and the data cannot be spliced.
● When the data of the flat objects are collected, it is necessary to attach the markers to the surface of the object and around the object or to place some additional fixtures to ensure the collection accuracy.
2.3 Robustness challenges faced by bone sentinel modeling
The accuracy of bone whistle modeling is directly related to the accuracy of the scanning points. In real scanning data, the scanning points often have the following problems:
● There are irrelevant points outside the scanned object;
● The scanning points on the surface of the object are discretized; and
● The scanning device itself causes unavoidable noise points. These problems result in low-quality scan data that affect the final modeling results. To address this issue, we propose a bone sentinel modeling method based on robust scan point tracking.
3 Bone whistle modeling based on robust scan point tracking
3.1 Algorithm ideas
3.1.1 Key point detection
A Gaussian kernel can generate a multiscale space. The scale space L(x,y,σ) of the image uses the original image I(x,y) and a variable 2-dimensional Gaussian function G(x,y,σ), which are defined as follows:
where σ is the scale space factor. To obtain stable feature points in different scale spaces, the convolution of the image I(x,y) and the Gaussian kernel G(x,y,σ) under different scale factors can be used to form a Gaussian pyramid, and the points can be passed through adjacent scale spaces in the Gaussian pyramid. The difference in the Gaussian (DoG) pyramid is obtained by subtracting the functions, which is represented by D(x,y,σ)
By comparing the point marked with a cross to the adjacent points in the upper and lower layers and the adjacent points in the same layer, the local extreme value of the DoG space can be determined. The direction parameter for each feature point is specified by using the gradient direction distribution characteristics, and the main direction of the key point is determined. Each detected feature point contains three pieces of information, namely, the position, the scale and the orientation, which ensures the translation, scaling and rotation invariance of the key points, respectively.
3.1.2 Description of the key points
The purpose of this description is to describe the detected key points as a set of feature vectors. The basic process is divided into three steps: first, the image area around the key point is divided into blocks; second, the gradient histogram within the block is calculated; and third, the feature vector is generated.
3.1.3 Key point matching
The similarity measurement is the first step in the key point matching process. In general, distance functions such as the Euclidean distance and Mahalanobis distance are used to obtain potential matches between images. Next, a commonly used data structure known as the k-d tree is implemented. Based on the key points in the target image, the feature points in the original image that are closest to the feature points in the target image and the adjacent feature points in the original image are searched. If the ratio of the nearest neighbor distance to the next nearest neighbor distance is less than a certain threshold, the pair of matching points is accepted. Since many mismatched points are found during the matching process, additional constraints are needed to eliminate mismatches. The random sample consensus (RANSAC) algorithm is often used to improve the robustness of the matching process.
3.2 Robust scan point selection
The main goal of point-stage data processing is to preprocess the point cloud sets of scanned objects to improve the quality and quantity of the data. The processing flow is divided into four main steps: the elimination of discrete and external points, the elimination of erroneous noise points introduced by the scanning equipment, the optimization of the number of point clouds, and the encapsulation of the objects.
3.2.1 Removal of the irrelevant points
When the bone whistle is scanned, since there is no filter processing, some objects in the background, such as computers, walls, and printers, may be scanned; thus, there may be objects around the detected object. Irrelevant points are collectively referred to as extracorporeal points. The geometric shapes represented by these points cannot be preserved. The presence of irrelevant points may lead to the deformation of the 3D objects; thus, these points should be removed. This process is divided into two steps that are completed by Geomagic Studio.
● Manual remove of the points. Some points outside the body are clearly too far from the measured object and can thus be regarded as discrete points. These points can be manually selected with tools. For example, discrete points that are clearly too far from the object can be manually selected with selection tools, such as the rectangle tool, ellipse tool or other regular shape selection tools or lassos. Tools such as the lasso tool and other irregular shape selection tools can be used to manually remove the selected discrete points.
● Automatic detection of the external points. Geomagic Studio software includes an automatic detection function. Through this software, the system can select points at the corners of the object that are far from the main point cloud and thus do not belong to the main point cloud. After the irrelevant points are removed, the 3D image of the bone whistle can be constructed.
3.2.2 Removal of the noise points
During the 3D scan, slight vibrations of the scanning instrument or errors in the scanning calibration process may result in inaccuracies, an unstable scanning environment or an untreated surface on the object to be measured (such as dust). The existence of these factors may introduce noise points into the scanned 3D image, which appear as an uneven or rough surface on the measured object. To reduce the impact of these factors on the printed object and eliminate errors, we can use a code to reduce noise. The Figure 6 shows a 3D schematic diagram of the bone whistle after the noise points are removed. According to Figures 5, 6, the number of noise points has been reduced.
3.3 Robust scan point tracking
3.3.1 Tracking of the points
Key point detection can be used to discern face, human body key points, and specific key points on various types of objects (such as hand bones). Moreover,3D object detection has been a popular research topic in recent years. Although the addition of prior knowledge can improve the accuracy of the algorithm to some extent, it also increases the difficulty of obtaining labels and the complexity of the algorithm design.
Although the bone whistle was treated as a cylinder in the previous steps, it is a polygonal object. When the point cloud data are too dense, the polygonization of the subsequent data may be affected. Therefore, we need to process the point cloud data to remove extra points, that is, we need to use a data filtering operation. The effect of the point cloud optimization process is shown in Figure 7.
3.3.2 Encapsulation of the point objects
After the above processing steps are performed on the point object, we can use the polygon mesh operation on the point object to encapsulate the object, as shown in the figure.
3.4 3D modeling and optimization
After the measured object has been encapsulated, a polygon is formed by splicing multiple small triangles, as shown in Figure 8. In the polygon stage, we need to repair the damaged holes in the measured object to ensure that the object resembles the original shape to facilitate future printing and analysis. The operations of the polygon stage are divided into the following steps: repairing the polygons, removing redundant parts on the polygons, adjusting the number and smoothness of the polygons, and detecting and correcting the polygons.
3.4.1 Hole filling
The plane is selected by bridging and filling. Then, 4 to 5 bridges are built inside the whistle through the edge features of the two mouths of the whistle. Next, the inner wall is gradually filled through the boundary hole/internal hole + curvature/tangent, focusing on the change in the thickness of the whistle to ensure uniformity and a smooth curvature. After the holes are filled, the inner wall is filled to obtain a schematic diagram of the 3D printed bone whistle, as shown in Figure 9.
3.4.2 3D model optimization of the bone whistle
During the repair process, some abnormal protrusions and folds on the surface of the bone whistle need to be repaired, as shown in Figure 10. The redundant parts need to be selected with the lasso tool, deleted, and filled with the curvature mode of the single-hole function. Each hole is filled and patched to ensure that the smooth appearance is restored to the maximum extent possible. The operation steps are provided as follows. Next, the model is optimized by simplifying the polygon command, which reduces the number of triangles in areas with smaller curvatures to maintain the number of triangles in areas with larger curvatures. Finally, the result shown in Figure 11 is obtained.
3.5 Implementation details and 3D printing
Geomagic Studio is a CAD software that is provided by Raindrop for public use. This program is a type of reverse engineering software that creates polygonal models and meshes point cloud data obtained from 3D scanning. In this paper, Geomagic Studio is used to process the 3D data of the bone whistle and to repair the model, mainly during the point stage and the polygon stage.
The file that has been processed by Geomagic Studio is opened with PreForm software, the appropriate printer is selected, the drop-down menu is used to set the material type as white, the slider is used to set the printing layer thickness to 0.05 mm, a sufficient amount of printing material is poured into the resin tank, and the resin is properly stirred with a spatula to ensure that the resin does not clump or stick to the walls. The Figure 12 shows the bone whistle after printing.
4 Acoustic analysis of the bone whistle
4.1 How to play the bone whistle
In terms of musical instrument classification, the bone whistle is a gas-sounding edge musical instrument; that is, the gas impacts the edge of the blowing hole, causing the air column in the tube to vibrate and produce sound. However, since the bone whistle is a historical instrument, there is no reference for how to play the bone whistle. Therefore, in this work, all possible blowing methods are analyzed. There are four types of blowing methods:
● Horizontal blowing: blow horizontally, with one of the holes of the bone whistle used as the blow hole.
● Oblique blowing: refer to the method of the eagle bone flute and tilt the pipe end and the lips at an angle of 45°.
● Vertical blowing: blow vertically, with the pipe end as the edge.
● Whistle blowing: use a hole near the pipe end as the blow hole and wrap the pipe end with the mouth to play.
Various tests showed that using Method 4 produced the best sound. Therefore, the acoustic analysis in this work was carried out with Method 4.
4.2 Recording experiment environment
The numbered bone whistle was played in a silent room at approximately 20 cm from the microphone. A *.wav file was saved every time the playing was completed, and all the sounds of each whistle were saved in a folder. The duration was approximately 4 s. Five whistle audio files were recorded. Audacity recording software was used. The recording equipment included a condenser microphone, a Roland Duo-Capture EX audio interface, and a ThinkPad notebook computer.
4.3 Recording analysis
The bone whistle has a single hole and is double sided. The excavation site was the Tianluoshan area, Yuyao, and the excavation layer was No. 7. The specific shape of the single-hole bone whistle is shown in Figure 13, and the 3D printed model is shown in Figure 14. The two holes at the ends of the bone whistle are labeled as Numbers 1 and 2 to distinguish the holes during the recordings and analyses.
regarding this bone whistle, we recorded and analyzed four different blowing methods:
● Blowing Method 1: blow on the 1st end and press and hold the 1st hole; the result is shown in the upper left spectrogram in Figure 15.
● Blowing Method 2: blow on the 1st end and press and hold the 2nd hole; the result is shown in the upper right spectrogram in Figure 15.
● Blowing Method 3: blow on the 2nd end and press and hold the 1st hole; the result is shown in the lower left spectrogram in Figure 15.
● Blowing Method 4: play on the 2nd end and hold down the 2nd hole; the result is shown in the bottom right spectrogram in Figure 15. Below, we analyze the audio obtained with these four blowing methods.
In the analysis, we found that the tones of the spectrograms of Figures 15C, D were fuller and clearer than those of Figures 15A, B. We believe that the reason for this result is that the whistle sounds of the third and fourth blowing methods is closer to the intended whistle sound, Moreover, the sounds are easier to produce. The nearly cylindrical bone whistle with double holes on both sides was found and recovered from Layer 4 A at the Hemudu site in Yuyao area. The specific shape of this bone whistle is shown in Figure 16, and the 3D model is shown in Figure 17. The two holes at the ends of the bone whistle are labeled as Numbers 1 and 2 to distinguish the holes during recordings and analyses.
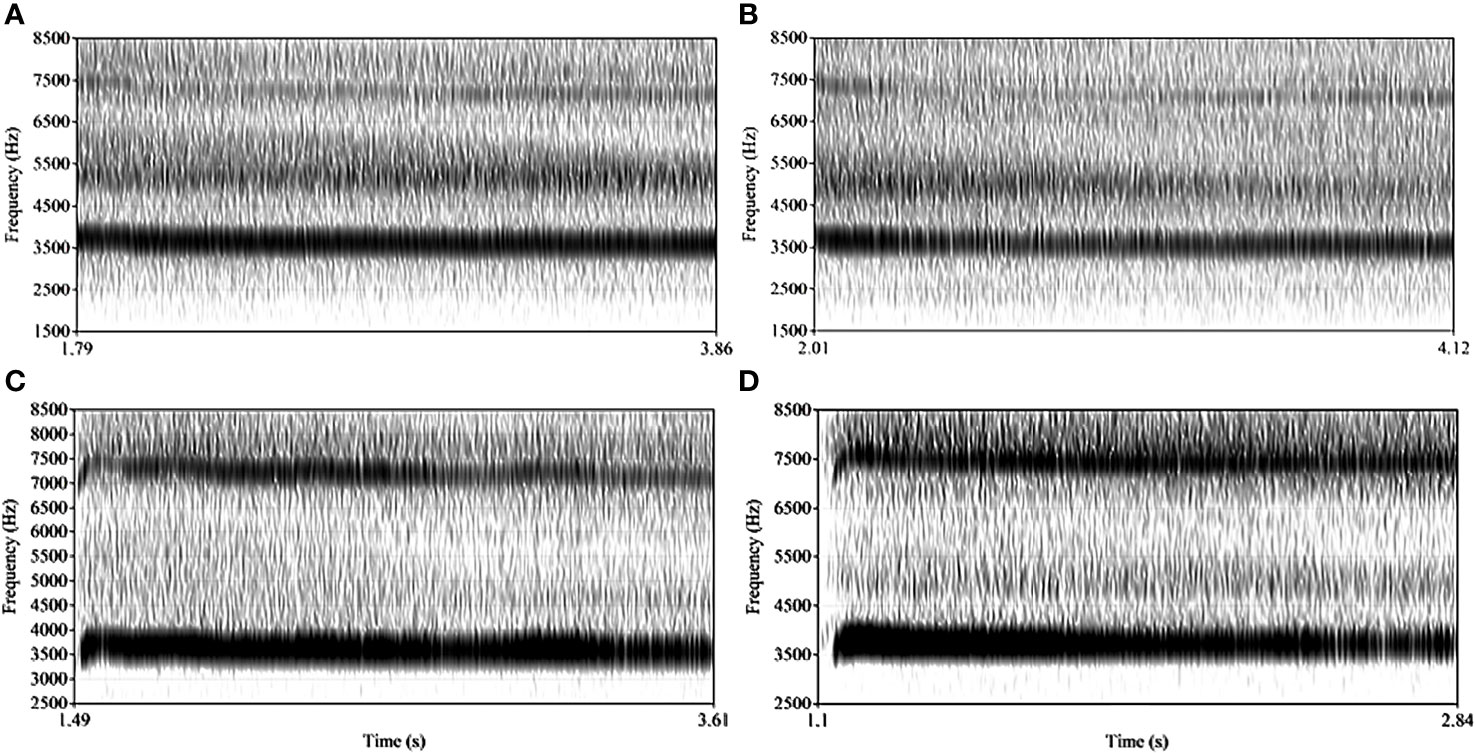
Figure 15 Spectrogram of the four blowing methods of single-hole bone whistle. (A) Spectrogram of Blowing Method 1: blow on the 1st end and press and hold the 1st hole; (B) Spectrogram of Blowing Method 2: blow on the 1st end and press and hold the 2nd hole; (C) Spectrogram of Blowing Method 3: blow on the 2nd end and press and hold the 1st hole; (D) Spectrogram of Blowing Method 4: blow on the 2nd end and press and hold the 2nd hole.
We recorded four kinds of blowing audio with this bone whistle.
● Blowing Method 1: blow Whistle 1 with gentle airflow and open hole 2; the result is shown in the spectrograms of Figure 18A.
● Blowing Method 2: blow Whistle 1 with turbulent airflow (overblowing) and open hole 2; the result is shown in the spectrograms of Figure 18B.
● Blowing Method 3: blow Whistle 2 with gentle airflow and open hole 1; the result is shown in the spectrograms of Figure 18C.
● Blowing Method 4: blow Whistle 2 with turbulent airflow (overblowing) and open hole 1; the result is shown in the spectrograms of Figure 18D.
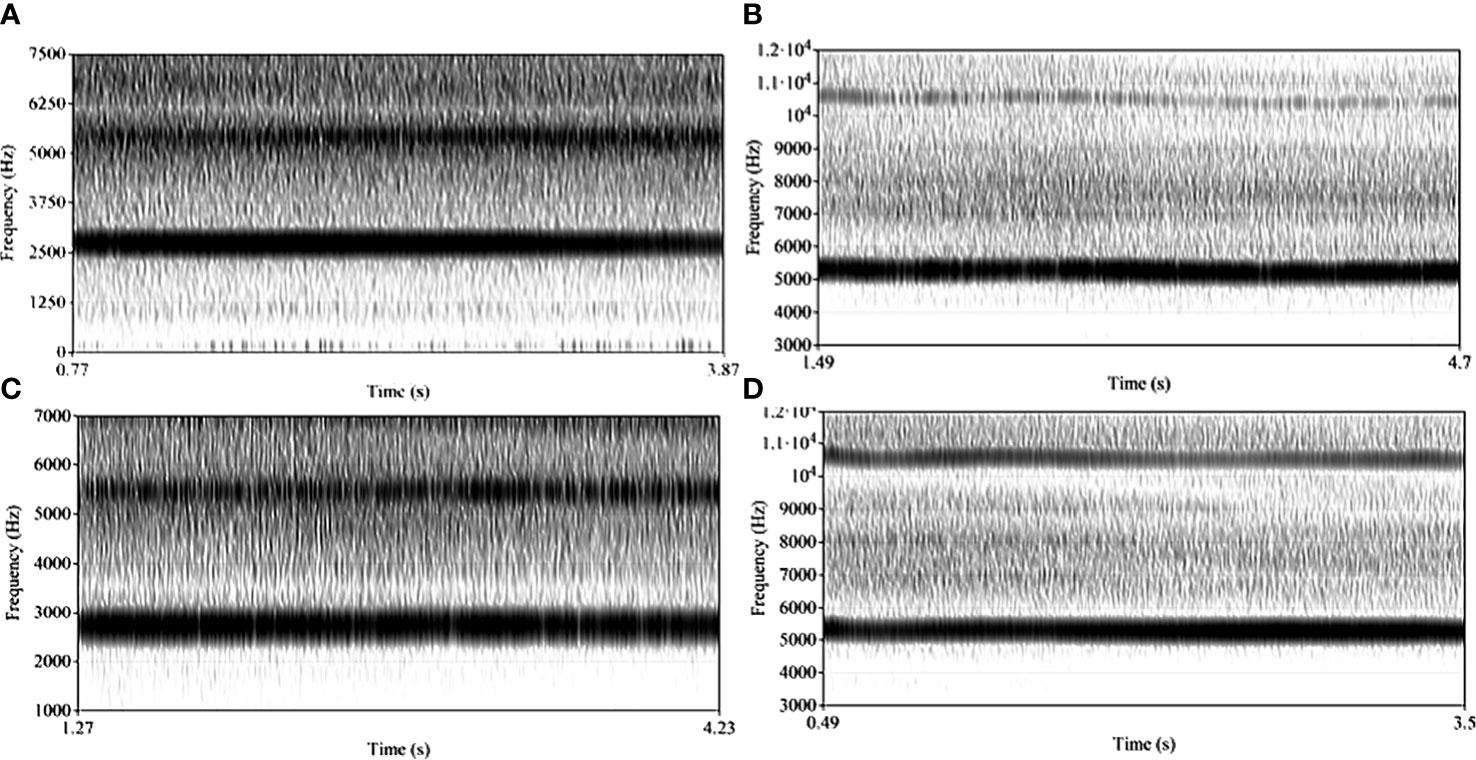
Figure 18 Spectrogram of the four blowing methods of the double-hole bone whistle. (A) Spectrogram of Blowing Method 1: blow Whistle 1 with gentle airflow and open hole 2; (B) Spectrogram of Blowing Method 2: blow Whistle 1 with turbulent airflow (overblowing) and open hole 2; (C) Spectrogram of Blowing Method 3: blow Whistle 2 with gentle airflow and open hole 1; (D) Spectrogram of Blowing Method 4: blow Whistle 2 with turbulent airflow (overblowing) and open hole 1.
The following is an analysis of the audio obtained with these four blowing methods.
According to the analysis of the spectrograms and pitch maps of these four blowing methods, when the bone whistle is blown by a gentle airflow, the sound is more pleasant to the ear, and when the bone whistle is overblown, the sound is a high-frequency whistle with a warning effect. The nearly cylindrical bone whistle with a single hole on one side of the whistle is slightly longer than the other whistles. This whistle was recovered from Hemudu site from Layer 4A in the Yuyao area. The specific shape of this bone whistle is shown in Figure 19, and the 3D model is shown in Figure 20. The ends of the bone whistle are labeled as Numbers 1 and 2 to distinguish the ends during recordings and analyses. There is only one method for producing a satisfactory sound with this bone whistle, that is, blowing from the whistle. Spectrogram in Figure 21 shows that the depth at 1500-2000 Hz is slightly shallower than that at 3000-4000 Hz. The low frequency at this point is due to the influence of the air when the whistle produces a relatively large audio sound. The frequency distribution of the spectrogram of the whistle is not particularly obvious. A possible reason for this result is that the bone whistle with only one hole is easier to blow than the bone whistle with two holes. This finding is consistent with the actual situation. Among the more than 100 bone whistles that were discovered in Hemudu, bone whistles with one hole at both ends were the most common. People at that time may have mastered the craftsmanship of the two-hole bone whistle, while the other forms were still being explored.
4.4 Analysis of the experimental results
After the audio analysis of the above bone whistles, we concluded that bone whistles can produce both musical tones and high-frequency whistles. We speculate that the bone whistle may have more than one use and that the various types of bone whistles may have different functions. We summarize the different blowing methods for producing musical sounds and whistles in the Table 3. The experiments show that the sounds of the GushaoT403 8 47 and Gushao1523 whistles with double holes at both ends are more inclined toward musical sound under normal airflow, while they produce higher frequency whistles when overblown. Under the same conditions, the single-hole whistles produced sounds that were more similar to whistles or bird songs. Most of the bone whistles produced in Hemudu have double holes; however, bone whistles with holes at both ends are more likely to be recovered, and these bone whistles have thus far not been connected. Even if these objects are combined, it is difficult to produce complete music. The role of the bone whistle may be closer to that of a flute, and its role can be roughly divided into the following three points:
● For ancient people, the bone whistle may have been used as a warning tool. For people who live in groups, different bone whistles may have represented different tribes, and they may have been a type of trophy.
● In special cases, the bone whistle may have been used as a musical instrument by ancient people to determine mutual positioning. It is speculated that in ancient times, people did not have sufficient items to communicate with each other and determine positioning because the environment differed from that of modern and post-feudal cities. Before humans settled, the use of such musical instruments may have contributed to their positioning; furthermore, the tool is convenient and easy to carry, and the sound does not disturb the environment. In forests where ancient people lived, only humans can recognize the sound, and it is thus a convenient and reliable tool.
● The bone whistle can also be used to imitate and trap birds and fowls.
5 Summary and outlook
In this paper, more than 100 bone whistles that were discovered in the Yuyao area were selected, scanned, restored, 3D printed, recorded and analyzed. This work provides a new understanding of music in the Neolithic Age. The experiments and analysis of the bone whistle have led us to speculate about the general purpose of the bone whistle, namely, that the bone whistle had hunting, positioning, and warning functions. This speculation may provide new insights for studying archaeological problems, as well as an inspiration for novel analysis methods.
In the analysis of the bone whistle, we used several methods, including vertical blowing and blowing with the mouth on the pipe end. Traditional musical instruments, including wind instruments such as the clarinet, suona, and cucurbit flute, are all played in this manner. In our study on the bone whistle, we used a similar whistle blowing method. The bone whistle is easy to operate, and the sound is relatively full, which is in line with the habits of ancient people. Finally, because the bone whistle does not have many holes, it is unlikely that there were too many fingering patterns. Although there is a technique for blowing different sounds by controlling the breath with modern musical instruments, the contrast is too low. Thus, the possibility that the bone whistle was a musical instrument is still low. In addition, although the rhythm of the bone whistle is not apparent, it can emit a very pleasant flute sound between 1500-2500 Hz, which demonstrates another possible application of the bone whistle. A whistle is a tool that mimics the chirping sound of a trapped object.
In addition, since only the bone whistle alone cannot fully verify the playing method of the bone whistle, there have also been external devices in traditional folk music that enable the instrument to provide more detailed tones. Moreover, in recording, due to the inability to fully restore the real environment, the initial timbre may not be obtained, and the pronunciation may also vary. Therefore, more information may be needed to verify in the future.
Although this work has carried out research on the bone whistle, the rigor of the research cannot be guaranteed. Thus, further research is needed to focus on the deeper characteristics of the bone whistle to obtain more complete experimental results.
Data availability statement
The raw data supporting the conclusions of this article will be made available by the authors, without undue reservation.
Ethics statement
Written informed consent was obtained from the individual(s) for the publication of any potentially identifiable images or data included in this article.
Author contributions
KK: contributed to the conception of the study, performed the experiment, performed the data analyses and wrote the manuscript. JW: helped perform the analysis with constructive discussions. All authors contributed to the article and approved the submitted version.
Funding
This work was supported by the supported by the National Natural Science Foundation of China(62261045) and Key R&D and Transformation Plan of Qinghai Province, 2022-QY-218.
Conflict of interest
The authors declare that the research was conducted in the absence of any commercial or financial relationships that could be construed as a potential conflict of interest.
Publisher’s note
All claims expressed in this article are solely those of the authors and do not necessarily represent those of their affiliated organizations, or those of the publisher, the editors and the reviewers. Any product that may be evaluated in this article, or claim that may be made by its manufacturer, is not guaranteed or endorsed by the publisher.
References
CPAM (1978). Chekiang Province and Chekiang Provincial Museum. the first excavation report of the hemudu site[J]. Journal of Archaeology 1978 (1), 72. doi: CNKI:SUN:KGXB.0.1978-01-002
Fei X., Xia X., Changsui W. (2004). A new exploration of the acoustic characteristics of jiahu bone flute music–research on the sound measurement of the newly unearthed jiahu bone flute. Music Research 01, 30–35.
Guo Q., Cheng Z., Juefei-Xu F., Ma L., Xie X., Liu Y., et al. (2021a). “Learning to adversarially blur visual object tracking,” in Proceedings of the IEEE/CVF International Conference on Computer Vision. 1812–1824.
Guo Q., Han R., Feng W., Chen Z., Wan L. (2020). Selective spatial regularization by reinforcement learned decision making for object tracking. IEEE Transactions on Image Processing 29, 2999–3013. doi: 10.1109/TIP.2019.2955292
Guo Q., Wang Z., Juefei-Xu F., Lin D., Ma L., Feng W., et al. (2021b). CarveNet: Carving Point-Block for Complex 3D Shape Completion. arXiv preprint arXiv:2107.13452. doi: 10.3389/fnins.2013.12345
Institute of Archaeology (1978). The excavation report of the dahezhuang site in Yongjing[J]. Journal of Archeology, 1974-02-029.
Jiping L. (2005). Archaeological experiment and analysis of bone flute. Journal of Tianjin Conservatory of Music (5), 13–17 doi: 02:1008-2530
Naiwu Z., Zhonghe L. (1991). Zhongshanzhai ruins in Ruzhou. henan. Journal of archaeology 01, 57–89. doi: 10.1016/B978-0-08-036390-5.50009-9
Ruxiang W. (1963). Investigation and trial excavation of the nuomuhongdaliha site in Dulan county, Qinghai province. Journal of archaeology 01, 17–44.
Ruzuo W. (1996). On the relationship between hemudu culture and majiabang culture. Cultural Relics in Southern China 03, 33–40.
Ximin H. (1986). The mystery of the ancient Chinese musical instrument bone whistle. Chinese Music 02, 89–95.
Yongjia L. (2012). Research on the bone whistle unearthed from the hemudu site. Southeast Culture 04, 89–95.
Keywords: bone whistle, 3D print, 3D scan, audio analysis, 3D image
Citation: Khysru K and Wei J (2023) Bone whistle modeling method based on robust scan point tracking. Front. Ecol. Evol. 11:1174739. doi: 10.3389/fevo.2023.1174739
Received: 28 February 2023; Accepted: 20 July 2023;
Published: 22 August 2023.
Edited by:
Bingwei He, Fuzhou University, ChinaReviewed by:
Cong Luo, Hohai University, ChinaJoshua Kumbani, University of Johannesburg, South Africa
Copyright © 2023 Khysru and Wei. This is an open-access article distributed under the terms of the Creative Commons Attribution License (CC BY). The use, distribution or reproduction in other forums is permitted, provided the original author(s) and the copyright owner(s) are credited and that the original publication in this journal is cited, in accordance with accepted academic practice. No use, distribution or reproduction is permitted which does not comply with these terms.
*Correspondence: Kuntharrgyal Khysru, Z3RqMTg2QHRqdS5lZHUuY24=