- 1Department of Biology, University of Toronto Mississauga, Mississauga, ON, Canada
- 2Department of Ecology and Evolutionary Biology, University of Toronto, Toronto, ON, Canada
Urbanization is a key stressor of freshwater habitats, possibly contributing to global insect declines. However, scientific understanding of urbanization's effects on aquatic insect communities has largely been based on studies of temperate streams. We reviewed global urban freshwater macroinvertebrate community studies, classifying habitat type, location, urbanization metrics, biodiversity metrics, and focal taxa, drawing from 114 studies in 32 different countries. Our goals were to: (1) investigate the extent of research on urbanization across a variety of freshwater habitats, (2) examine the representation in empirical literature across the globe by comparing cities in different geographic regions, and (3) highlight how study approaches including taxonomic resolution and the inclusion of trait data impact interpretation of these patterns. Most studies were conducted in North America and Europe, but there is growing representation from other continents. Additionally, lentic environments were underrepresented in the literature on community responses to urbanization compared to lotic studies. Therefore, we suggest that lentic habitats should be investigated more thoroughly. We suggest that future empirical studies should incorporate traits of the taxa investigated to better predict how communities respond to urban stressors. The lack of consistent results from the reviewed studies showed that there is no single, predictable effect of urbanization, indicating that future meta-analyses and review papers should consider the potential context-dependency of freshwater insect responses to anthropogenic pressures. Our goal in highlighting understudied environmental and regional contexts is to move toward holistically addressing the ongoing challenges of urban freshwater insect conservation and freshwater ecology research.
1. Introduction
Human activities deplete biodiversity across the globe (Wagner, 2020; Wagner et al., 2021), and continued biodiversity loss is forecasted (Sala et al., 2000). Recently, reports have drawn attention to widespread insect declines (Leather, 2018; Sánchez-Bayo and Wyckhuys, 2019; Cardoso et al., 2020; Eggleton, 2020; Wagner et al., 2021). Insects comprise the majority of animal biomass, playing vital roles in food webs (Eggleton, 2020; Wagner et al., 2021) and providing ecosystem services (Kim, 1993; Losey and Vaughan, 2006; Vanbergen and the Insect Pollinators Initiative, 2013; DeWalt and Ower, 2019; Morse et al., 2019; Sánchez-Bayo and Wyckhuys, 2019; Cardoso et al., 2020), thus observed declines have potential to threaten the integrity of ecosystems around the world.
While most research on insect declines has occurred in terrestrial systems, freshwater ecosystems have also experienced persistent and emerging threats, such as habitat modification, invasive species, and pollution (Ricciardi and Rasmussen, 1999; Dudgeon et al., 2006; Vörösmarty et al., 2010; Reid et al., 2019). Research focusing on freshwater insect populations has shown declines in abundance and biomass (DeWalt et al., 2005; Bojková et al., 2014; Murray-Stoker et al., 2020; Roth et al., 2020; Stepanian et al., 2020), though conflicting conclusions have also been reached through meta-analysis (van Klink et al., 2020). More research on declines in freshwater insects is needed, especially with respect to community responses. Nuanced investigations into freshwater insects' responses to global change can help develop clear policies to minimize further degradation and aid recovery (Dornelas and Daskalova, 2020).
Here, we study the effects of urbanization on global communities of freshwater insects and other macroinvertebrates. The “urban stream syndrome” describes consistent changes in physical, chemical, and biological aspects of urbanized streams (Meyer et al., 2005; Walsh et al., 2005). However, this syndrome was characterized from studies primarily in North America and Australia, and symptoms underlying the urban stream syndrome may show inconsistent trends across cities differing in climatic, physiographic, and social conditions (Walsh et al., 2005; Booth et al., 2016). Similarly, meta-analyses and reviews of insect population changes typically lack data from the tropics (Stendera et al., 2012; Sánchez-Bayo and Wyckhuys, 2019; Eggleton, 2020), and tropical environments are expected to experience greater levels of land use change compared to other biomes (Sala et al., 2000). Additionally, whether urban pond environments will exhibit patterns of biological and chemical changes similar to streams has yet to be fully understood. While the identification of this syndrome has been useful in developing tractable approaches to understand temperate urban streams, these limitations should also motivate research efforts to encompass the diversity of freshwater habitats worldwide.
A recent review identified priorities with respect to macroinvertebrate communities and the effects of urbanization, including (1) investigating specific macroinvertebrate taxa, and (2) examining the impacts of urbanization on diversity in specific habitat types (Gál et al., 2019). Given these gaps, our review synthesizes the current status of the literature on global freshwater biodiversity in urban environments by examining: (1) the extent of research across freshwater habitats that span the gradient of lotic to lentic, as well as the metrics commonly used in these assessments, (2) the representation of studies from cities in different geographic regions, and (3) how study approaches including taxonomic resolution and the inclusion of trait data impact the interpretation of these patterns.
2. Methods
We conducted a literature review using Web of Science (© Clarivate 2022) and Google Scholar databases (https://scholar.google.ca/). We focused on empirical investigations of insects and co-existing macroinvertebrates (e.g., crustaceans, oligochaetes) at the community level in freshwater habitats. We selected studies that examined macroinvertebrate community responses (e.g., diversity, percent abundance, richness) to urban influences. Search terms included: “urban*,” “communit*,” “aquatic,” “freshwater,” “macroinvertebrate,” “diversity,” “river,” “wetland,” “pond,” and “stream.” To maximize geographic coverage, we included continents, countries, or biomes in search terms. We only evaluated English language papers and thus acknowledge we are not fully representing global literature with this review.
For each article, we noted the location, habitat type, focal taxa (whether this was the whole macroinvertebrate community or a specific taxon), the metric used to distinguish “urban” vs. “non-urban” habitats (e.g., percent impervious surface cover), whether the article specifically mentioned the presence of human-created aquatic habitats, the response variable(s) used (e.g., taxonomic richness, Shannon's diversity index), and the main finding(s) of the article. We used seven different geographic regions to divide the study locations: Africa, Asia, Central America/Caribbean, Europe, North America, Australia/New Zealand, and South America. We considered “human-created aquatic habitats” to include constructed stormwater ponds, garden ponds, significantly dammed or channelized rivers, or effluent-dominated streams.
We obtained GPS coordinates based on site descriptions outlined in each study. We first classified study locations as either temperate (>23.43631 N/S) or tropical (<23.43631 N/S), based on the latitudes of the Tropic of Cancer and the Tropic of Capricorn. Study regions, particularly those that fall just outside of these latitudes, were further assessed to include a subtropical category by examining the overlay of site points with the ESRI USGS World Climate Regions geospatial dataset (ESRI, USGS, TNC 2020; https://arcg.is/1jHifW; Sayre et al., 2020). We summarized the papers by calculating percentages of various categories. In total, we compiled 114 relevant articles (Supplementary Table 1) that spanned a 30-year period (1991–2021) and 32 countries (Figure 1).
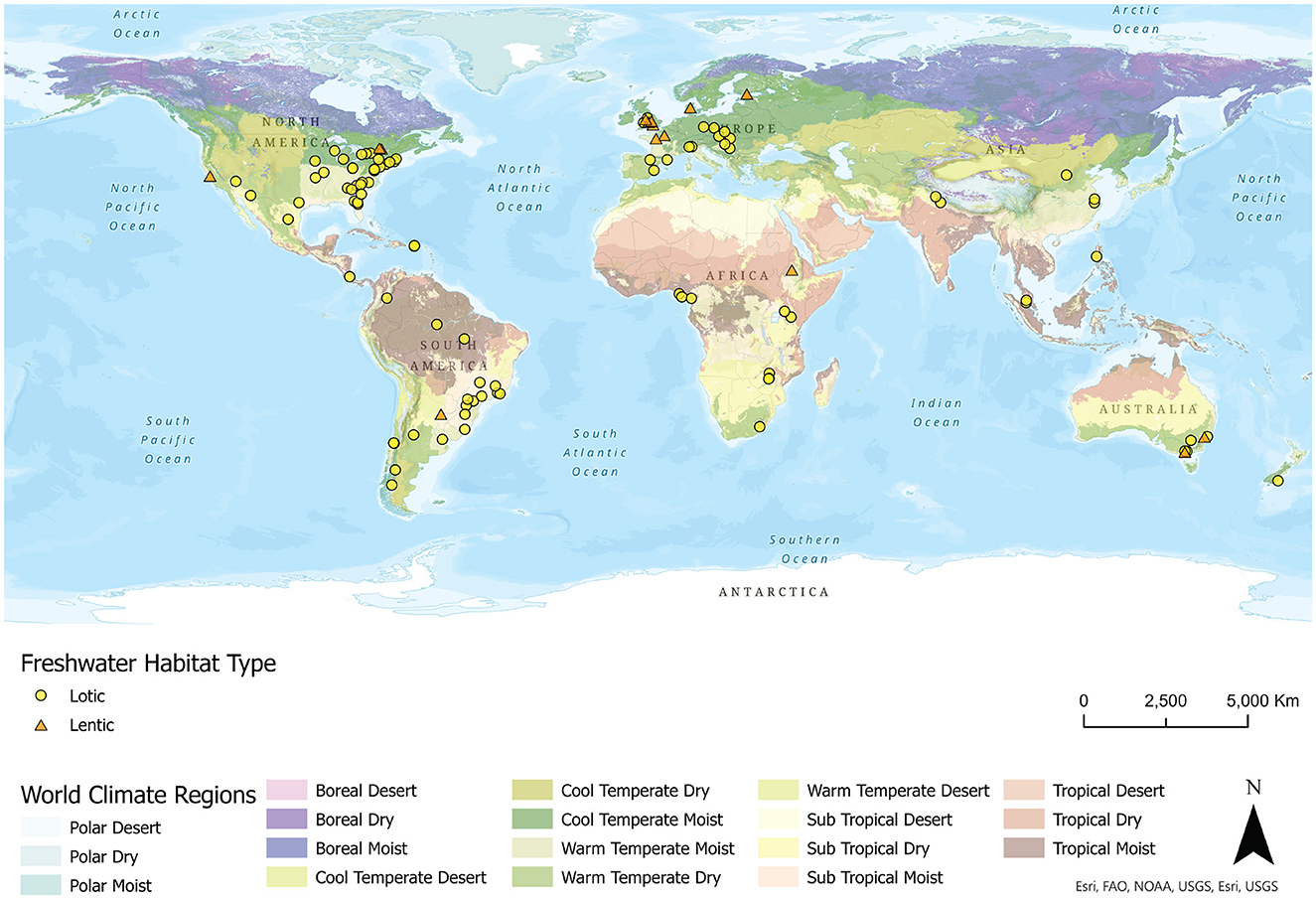
Figure 1. Locations of the empirical studies examined in this review (n = 114 papers) investigating freshwater macroinvertebrate community responses to urbanization. The freshwater habitat type investigated in each study and the climatic regions globally are provided in the legend.
3. Results
3.1. Global contexts of urban freshwater biodiversity
The studies we reviewed spanned Africa (8.77% of studies, Figure 1), Asia (7.02%), Central America/Caribbean (3.51%), Europe (22.81%), North America (33.33%), Australia/New Zealand (7.02%), and South America (17.54%). In terms of climate, 68.4% of evaluated articles were in temperate regions, 13.2% were in subtropical areas, and 18.4% were in tropical regions (Figures 1, 2B). Nearly all studies of lentic habitats were in temperate areas (Supplementary Table 1), with single articles reporting results from subtropical (Argentina; Gallardo et al., 2019) and tropical (Ethiopia; Gezie et al., 2017) lentic habitats.
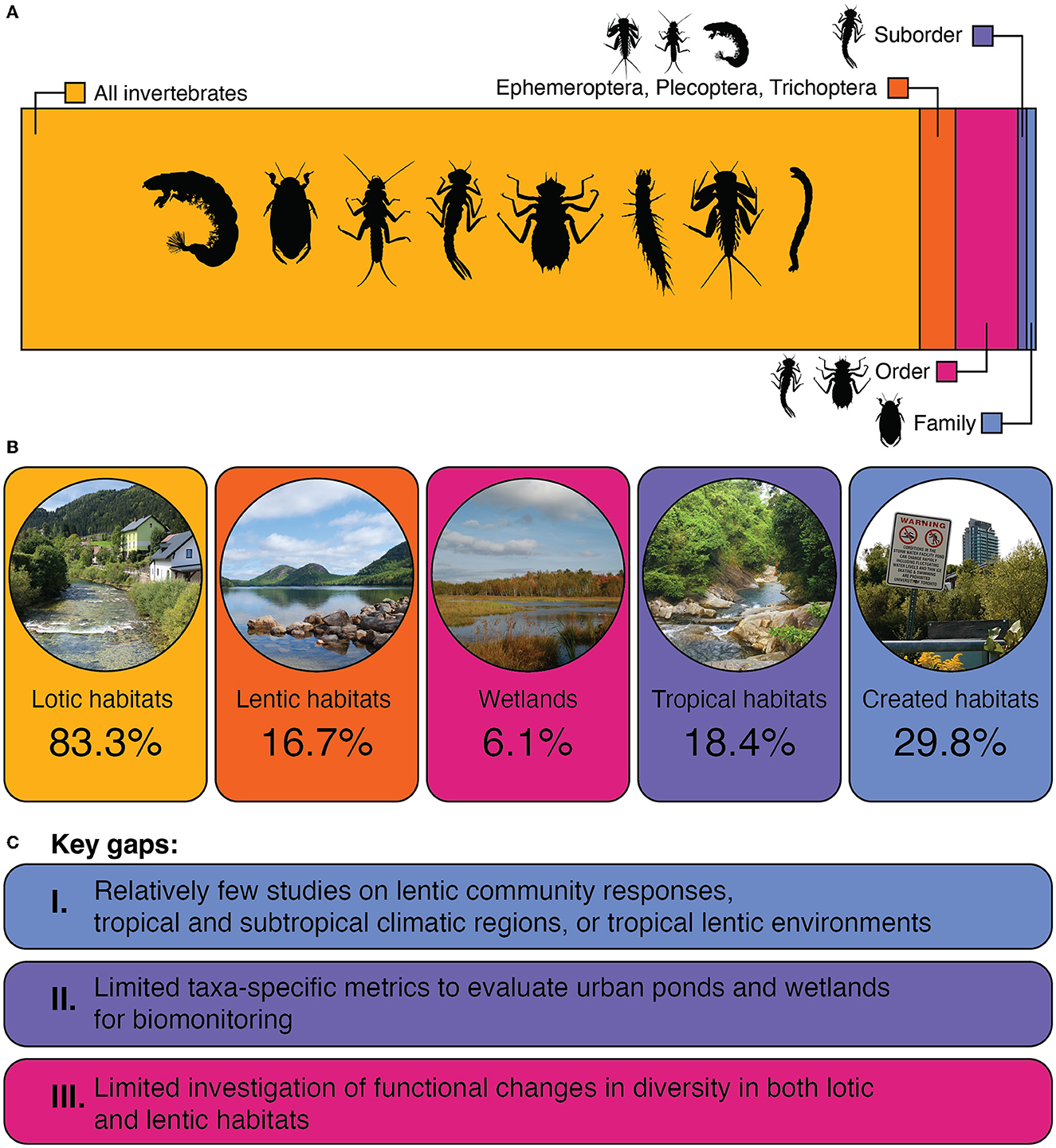
Figure 2. Summary of the 114 articles reviewed for this synthesis, highlighting: (A) the proportion of studies that analyzed all freshwater macroinvertebrates (88.59%), Ephemeroptera, Plecoptera, and Trichoptera (“EPT;” 3.51%), a single order (e.g., Odonata; 6.14%), a single suborder (i.e., one article focused on Zygoptera; 0.88%, or a single family (i.e., one article focused on Dytiscidae; 0.88%), and (B) the representation of various habitats, especially those that may have been previously underrepresented in urban freshwater research. All papers were classified as either focusing on “lotic” or “lentic” habitats. Wetland habitats are also included within the total lentic percentage. Created habitats include stormwater and other human-made ponds, effluent-dominated streams, and dammed and channelized streams. Figure photographs by K.M. Murray-Stoker. (C) Based on the representation of our review, we summarize key research gaps to be considered both for forming the context for current meta-analyses of global insect trends and for future research efforts examining the community responses to urbanization.
Since the development of the primarily temperate urban stream syndrome (Meyer et al., 2005; Walsh et al., 2005), there have been recent calls for more attention on tropical urban freshwater systems and the development of the “Southern urban hydrosystem syndrome,” which integrates urban lakes and wetlands in the Global South (Wantzen et al., 2019). Ramírez et al. (2009) pointed out that unique characteristics of tropical and island streams, such as their naturally flashier hydrograph, will result in these habitats experiencing urbanization differently. Indeed, climate, physical structure, and resident biota should be accounted for when predicting effects of urbanization, but our review indicates a community approach remains useful when analyzing urban and non-urban habitats around the globe. Correspondence analyses allowed for detection of important and region-specific stressors on aquatic insects and other macroinvertebrates such as heavy metal contamination (Bere et al., 2016), changes in flow regime (Chadwick et al., 2006), increased nitrogen (Docile et al., 2016), or reduced dissolved oxygen (Wan Abdul Ghani et al., 2018). Finally, seasonal determinants of freshwater insect communities in the context of urbanization were rarely studied (though see work in temperate streams: Walsh et al., 2007; Hepp et al., 2010; Violin et al., 2011). As impacts of region-specific stressors may change among seasons (e.g., road salt inputs in temperate winters), more insight is needed into how climate and seasonality may interact with urban pressures on freshwater biodiversity.
3.2. Lotic vs. lentic environments
Most studies investigated river and stream habitats (n = 95, 83.3%; Figure 2). Lentic environments comprised 16.7% of studies evaluated (n = 19), and wetlands were sparsely represented, at 6.1% of the total (n = 7; Figure 2). The predominance of lotic habitats in urban freshwater literature may be partially due to historical differences between the persistence of urban lotic and lentic environments. Destruction of ponds and wetlands through draining and filling has been historically common in cities (Davidson, 2014; Birch et al., 2022), while rivers have been maintained, albeit often in a highly impaired state, for urban usage for transportation, waste removal, and power (Winiwarter et al., 2016).
Encouragingly, there has been recent attention to urban stormwater management and wetland preservation, in part due to increasing urban sprawl (Oertli and Parris, 2019; Birch et al., 2022). Lentic studies reviewed here tended to focus on some type of created habitat, such as stormwater or garden ponds (84.2%, n = 16), while only 18.9% of lotic studies specifically mentioned the inclusion of created habitats (n = 18), such as effluent-dependent or channelized streams; across all studies, created habitats make up 29.8% of studies (n = 34; Figure 2). We noted that it was common for urban ponds to have comparable biodiversity to reference habitats (Le Viol et al., 2009; Hassall and Anderson, 2015; Liao et al., 2020). Increased habitat availability across the landscape may improve urban biodiversity for lentic specialists (Gledhill et al., 2008; Hill et al., 2016), thus the mere presence of urban pond habitats may mitigate the effects of anthropogenic impairment in certain contexts. From a biodiversity perspective, pollutants are a concern in stormwater runoff ponds, though manifestations are context-specific: odonate (dragonfly and damselfly) abundance decreased with increased chloride in Canadian ponds (Perron and Pick, 2020), whereas macroinvertebrate communities were unaffected by heavy metal accumulation in ponds of western Denmark (Stephansen et al., 2016).
Created habitats may also be beneficial to lotic specialists, depending upon regional context and level of modification. Bogan et al. (2020) found that urban wastewater effluent in an arid region (Santa Cruz River, Arizona, USA) reduced the risk of stream drying and facilitated odonate diversity. By contrast, however, Canobbio et al. (2009) reported that the pollutant load and hydrologic alterations introduced by wastewater in an Italian effluent-dominated stream resulted in reduced insect taxonomic richness and density. Commonly, a watershed approach has been used to study the effect of land use on lotic macroinvertebrate communities (Roy et al., 2003; Urban et al., 2006; Walsh et al., 2007; Pavel et al., 2016; White and Walsh, 2020), and dominant stressors can be specific to a watershed, as reported in a study of emerging contaminants (e.g., pharmaceuticals and pesticides) (De Castro-Català et al., 2015).
3.3. Taxon-specific metrics
Lotic and lentic studies also differed in the taxa-specific metrics used. Univariate biodiversity metrics have been developed for rivers and streams: percent community composition of richness or abundance of Ephemeroptera, Plecoptera, and Trichoptera (EPT; mayflies, stoneflies, and caddisflies) was a focus of 3.51% of total articles (n = 4/114, Figure 2), though 35.6% of studies (n = 36/101) examining the broader macroinvertebrate community also used an EPT-specific metric to complement other analyses. Family- or genus-level identifications of specimens was most common (but see for example Bozóki et al., 2018). EPT metrics are restricted to lotic systems, as stoneflies typically do not occur in lentic habitats. Lunde and Resh (2012) adjusted this approach for California wetlands (United States) by evaluating effects of urbanization using the percentage of Ephemeroptera, Odonata, and Trichoptera (EOT). However, in general, there is a lack of applicable taxon-specific metrics of biodiversity among urban lentic studies. This may hinder urban pond biomonitoring and conservation, as % EPT or EPT richness efficiently assesses the integrity of lotic environments due to the sensitivity of these taxa to disturbance. There remains some uncertainty regarding which macroinvertebrate taxa should be used as indicators of urbanization or anthropogenic pressure (e.g., whether the most sensitive or the most tolerant should be used). Some researchers suggest that organisms less sensitive to anthropogenic disturbance may be useful in the biomonitoring of urban waters (Bonada et al., 2004, 2005; Tszydel et al., 2015), because they would provide a more continuous measure of anthropogenic impacts.
While some authors provided specialized insight through examination of a particular taxonomic order (6.14%), suborder (one study on Amazonian damselflies; Brasil et al., 2017), or family (one study on dytiscid beetles in Finnish ponds, Liao et al., 2020), it was most common for studies to evaluate diversity metrics of the entire macroinvertebrate community (88.59%, Figure 1). Additional focal metrics often aimed to capture the degree of disturbance tolerance by highlighting specific taxa, such as the prevalence of Chironomidae or Oligochaeta (non-biting midge or aquatic worms, e.g., Marchamalo et al., 2018). Acknowledging the range of tolerance values within a single insect order, some studies specified the ratio of Hydropsychidae (a typically tolerant caddisfly family) to the rest of Trichoptera taxa in the benthic community (Rogers et al., 2002; Tszydel et al., 2015). Taxonomic resolution varied among the studies; species identification allows for more precise ecological conclusions about the habitat state (Resh and Unzicker, 1975), but this is typically not feasible unless focusing on only one order or family of insects.
3.4. Functional facets of diversity
Connecting stressors directly with freshwater insect communities might be best approached with functional traits. Studies that assessed overall diversity or richness of traits tended to find that urbanization results in functional homogenization (Barnum et al., 2017; Castro et al., 2018). Some literature suggests that specific traits should be expected for the “winners” of biotic homogenization, such as wide tolerance to environmental changes, low trophic specialization, and high fecundity (McKinney and Lockwood, 1999; Pavel et al., 2016). Tests of trait composition changes for urban lentic environments remain particularly limited, though Le Viol et al. (2009) showed that taxa with small body size, short life span, multivoltine, passive dispersal mode, and a range of feeding modes (scavengers, herbivores, shredders, piercers, and scrapers) were most associated with urban highway ponds in France. In this study, taxonomic diversity was comparable in highway ponds and surrounding reference ponds, with functional analyses providing additional insight into the changes in community structure in response to specific stressors, e.g., increased nutrients and salt (Le Viol et al., 2009). Edegbene et al. (2020) and Akamagwuna et al. (2021) determined traits that predicted urbanization sensitivity in South African and Nigerian streams, respectively, using a fourth-corner or RQL approach in which environment, taxa, and trait matrices are correlated (e.g., Legendre et al., 1997); however, these studies emphasize the importance of local context, as invertebrate body size was found by both studies to be significantly correlated with urban tolerance, but in opposite directions.
Various databases have been developed to aid researchers in associating traits with taxa for North American lotic insects (Poff et al., 2006), freshwater insects of the United States (Twardochleb et al., 2021), European freshwater organisms (Schmidt-Kloiber and Hering, 2015), European dispersal traits of aquatic macroinvertebrates (Sarremejane et al., 2020), freshwater invertebrates in North America, Europe, Australia, and New Zealand (Kunz et al., 2022), macroinvertebrates of the Three Parallel Rivers Region in China (Ao et al., 2022), macroinvertebrates in South African rivers (Odume et al., 2018), and freshwater macroinvertebrates of New Zealand (Phillips and Smith, 2018). Several were utilized by the articles evaluated in this review.
4. Conclusions and future directions
Our goal was to facilitate comparison between urban freshwater habitats so that perceptions of freshwater insect responses to human-induced environmental change are accurate to the habitat type encountered. Even after reviewing more than 100 studies, it is difficult to provide any definitive effects of urbanization on freshwater macroinvertebrate communities due to the disproportionate representation of all habitats in all climate zones (for example, the dearth of studies focusing on tropical and subtropical lentic habitats). When solely focusing on lotic studies, increasing impervious surface cover was generally associated with a decline in sensitive taxa. Overall, however, the studies investigated exhibited a range of effects of urbanization on biodiversity ranging from negative (e.g., Moyo and Rapatsa, 2016), to negligible (e.g., Hassall and Anderson, 2015), to positive (e.g., Bogan et al., 2020), to varied (e.g., Sitati et al., 2021), depending on the habitat, taxa, and stressors studied.
Given the current state of the field, we lack a comprehensive understanding of the impacts of urbanization on freshwater insects, and we argue that the complexity generated by unique contexts needs to be investigated to a greater degree before making definitive conclusions. Our review identified key gaps to be considered for future research examining community responses to urbanization (summarized in Figure 2). We therefore suggest that future reviews and meta-analyses distinguish patterns within these specific subgroups as opposed to treating all freshwater habitats and locations similarly. When researchers are focusing specifically on freshwater insect declines due to anthropogenic pressures, we argue that such nuanced approaches can also benefit from considering changes in regional community diversity and functional composition of freshwater communities rather than solely focusing on total abundances or biomass changes.
Beyond the explorations of this review, we suggest there is great potential for research on freshwater habitats in urban landscapes by studying location-specific social and historical elements. The field of ecology has been reluctant until recent years to evaluate urban environments in the same fashion as nonurban environments, which has led to biases against prioritizing assessment of biodiversity in created habitats (Clifford and Heffernan, 2018). Rather, we advocate for embracing a “natureculture” perspective (Haraway, 2016) in which heavily human-influenced habitats are not assigned a lesser intrinsic value than nonurban sites but are instead substantially included in the conversation about maintenance and protection of global biodiversity. Future urban freshwater ecology research may consider evaluating the effects of factors such as city organization and age through interdisciplinary collaboration with city planners, engineers, and architects. Additionally, the effects of systemic racism in cities, long a focus of environmental justice discourse (Hare, 1970), have been shown to affect biodiversity in terrestrial urban environments (Schell et al., 2020) and distribution of urban greenspaces (Boone et al., 2009) in North America. As global urbanization increases, we need to understand how legacies of social histories have shaped the lotic and lentic habitats of cities around the world and the biodiversity contained within them.
Finally, we argue that given differences in the way climate change and future global development will impact different biogeographic regions (e.g., temperate vs. tropical geographies), these distinctions are essential to appropriate, targeted conservation and protection of freshwater environments. By highlighting the existing literature on how specific taxonomic groups or functional traits might express differential responses to urbanization, we hope to move closer toward predicting community shifts to stressors associated with urban development in these different freshwater habitats and improving our understanding of the functional facets of freshwater diversity responses.
Author contributions
IF, KM-S, and SM conceived the idea for this manuscript and directed data collection. IF, KM-S, LH, CJ, VM, RS, GT, and OT collected data through literature searching and summarizing articles. IF and KM-S conducted analyses, created figures, and wrote the manuscript with input from LH, CJ, VM, RS, GT, OT, and SM. All authors contributed to the article and approved the submitted version.
Funding
We acknowledge the support of the Natural Sciences and Engineering Research Council of Canada (NSERC), (RGPIN-2019-06484). Additional funding was provided by the Department of Biology at the University of Toronto Mississauga. IF was supported by an NSERC CGS-D and KM-S was supported by a Connaught Graduate Fellowship and the University of Toronto Department of Ecology and Evolutionary Biology.
Acknowledgments
We thank R. Martin, Z. MacFarlane, R. Murray, A. Axiotis, and D. Murray-Stoker for thoughtful feedback and discussions on earlier drafts. This manuscript was improved by comments from the editor and two reviewers.
Conflict of interest
The authors declare that the research was conducted in the absence of any commercial or financial relationships that could be construed as a potential conflict of interest.
Publisher's note
All claims expressed in this article are solely those of the authors and do not necessarily represent those of their affiliated organizations, or those of the publisher, the editors and the reviewers. Any product that may be evaluated in this article, or claim that may be made by its manufacturer, is not guaranteed or endorsed by the publisher.
Supplementary material
The Supplementary Material for this article can be found online at: https://www.frontiersin.org/articles/10.3389/fevo.2023.1174166/full#supplementary-material
References
Akamagwuna, F. C., Ntloko, P., Edegbene, A. O., and Odume, O. N. (2021). Are Ephemeroptera, Plecoptera and Trichoptera traits reliable indicators of semi-urban pollution in the Tsitsa River, Eastern Cape Province of South Africa? Environ. Monit. Assess. 193, 309. doi: 10.1007/s10661-021-09093-z
Ao, S., Li, X., Tian, Z., Hu, J., and Cai, Q. (2022). Harmonizing and searching macroinvertebrate trait information in alpine streams: method and application–a case study in the three parallel rivers region, China. Front. Ecol. Evol. 10, 945824. doi: 10.3389/fevo.2022.945824
Barnum, T. R., Weller, D. E., and Williams, M. (2017). Urbanization reduces and homogenizes trait diversity in stream macroinvertebrate communities. Ecol. Appl. 27, 2428–2442. doi: 10.1002/eap.1619
Bere, T., Dalu, T., and Mwedzi, T. (2016). Detecting the impact of heavy metal contaminated sediment on benthic macroinvertebrate communities in tropical streams. Sci. Total Environ. 572, 147–156. doi: 10.1016/j.scitotenv.2016.07.204
Birch, W. S., Drescher, M., Pittman, J., and Rooney, R. C. (2022). Trends and predictors of wetland conversion in urbanizing environments. J. Environ. Manage. 310, 114723. doi: 10.1016/j.jenvman.2022.114723
Bogan, M. T., Eppehimer, D., Hamdhani, H., and Hollien, K. (2020). If you build it, they will come: rapid colonization by dragonflies in a new effluent-dependent river reach. PeerJ 8, e9856. doi: 10.7717/peerj.9856
Bojková, J., Rádková, V., Soldán, T., and Zahrádková, S. (2014). Trends in species diversity of lotic stoneflies (Plecoptera) in the Czech Republic over five decades. Insect Conserv. Divers. 7, 252–262. doi: 10.1111/icad.12050
Bonada, N., Zamora-Muñoz, C., Rieradevall, M., and Prat, N. (2004). Ecological profiles of caddisfly larvae in Mediterranean streams: implications for bioassessment methods. Environ. Pollut. 132, 509–521. doi: 10.1016/j.envpol.2004.05.006
Bonada, N. V., Rieradevall, M., and Prat, N. (2005). Relationship between pollution and fluctuating asymmetry in the pollution-tolerant caddisfly Hydropsyche exocellata (Trichoptera, Insecta). Arch. Für Hydrobiol. 162, 167–185. doi: 10.1127/0003-9136/2005/0162-0167
Boone, C. G., Buckley, G. L., Grove, J. M., and Sister, C. (2009). Parks and people: An environmental justice inquiry in Baltimore, Maryland. Ann. Assoc. Am. Geogr. 99, 767–787. doi: 10.1080/00045600903102949
Booth, D. B., Roy, A. H., Smith, B., and Capps, K. A. (2016). Global perspectives on the urban stream syndrome. Freshw. Sci. 35, 412–420. doi: 10.1086/684940
Bozóki, T., Krasznai-Kun, E. Á., Csercsa, A., Várbír,ó, G., and Boda, P. (2018). Temporal and spatial dynamics in aquatic macroinvertebrate communities along a small urban stream. Environ. Earth Sci. 77, 559. doi: 10.1007/s12665-018-7735-5
Brasil, L. S., Vieira, T. B., de Oliveira-Junior, J. M. B., Dias-Silva, K., and Juen, L. (2017). Elements of metacommunity structure in Amazonian Zygoptera among streams under different spatial scales and environmental conditions. Ecol. Evol. 7, 3190–3200. doi: 10.1002/ece3.2849
Canobbio, S., Mezzanotte, V., Sanfilippo, U., and Benvenuto, F. (2009). Effect of multiple stressors on water quality and macroinvertebrate assemblages in an effluent-dominated stream. Water Air Soil Pollut. 198, 359–371. doi: 10.1007/s11270-008-9851-4
Cardoso, P., Barton, P. S., Birkhofer, K., Chichorro, F., Deacon, C., Fartmann, T., et al. (2020). Scientists' warning to humanity on insect extinctions. Biol. Conserv. 242, 108426. doi: 10.1016/j.biocon.2020.108426
Castro, D. M. P., de Dolédec, S., and Callisto, M. (2018). Land cover disturbance homogenizes aquatic insect functional structure in neotropical savanna streams. Ecol. Indic. 84, 573–582. doi: 10.1016/j.ecolind.2017.09.030
Chadwick, M. A., Dobberfuhl, D. R., Benke, A. C., Huryn, A. D., Suberkropp, K., and Thiele, J. E. (2006). Urbanization affects stream ecosystem function by altering hydrology, chemistry, and biotic richness. Ecol. Appl. 16, 1796–1807. doi: 10.1890/1051-0761(2006)016(1796:UASEFB)2.0.CO;2
Clifford, C., and Heffernan, J. (2018). Artificial Aquatic Ecosystems. Water 10, 1096. doi: 10.3390/w10081096
Davidson, N. C. (2014). How much wetland has the world lost? Long-term and recent trends in global wetland area. Mar. Freshw. Res. 65, 934. doi: 10.1071/MF14173
De Castro-Català, N., Muñoz, I., Armendáriz, L., Campos, B., Barcel,ó, D., López-Doval, J., et al. (2015). Invertebrate community responses to emerging water pollutants in Iberian river basins. Sci. Total Environ. 503–504, 142–150. doi: 10.1016/j.scitotenv.2014.06.110
DeWalt, R. E., Favret, C., and Webb, D. W. (2005). Just how imperiled are aquatic insects? A case study of stoneflies (Plecoptera) in Illinois. An 98, 941–950. doi: 10.1603/0013-8746(2005)098(0941:JHIAAI)2.0.CO;2
DeWalt, R. E., and Ower, G. D. (2019). Ecosystem services, global diversity, and rate of stonefly species descriptions (Insecta: Plecoptera). Insects 10, 99. doi: 10.3390/insects10040099
Docile, T. N., Figueir,ó, R., Portela, C., and Nessimian, J. L. (2016). Macroinvertebrate diversity loss in urban streams from tropical forests. Environ. Monit. Assess. 188, 237. doi: 10.1007/s10661-016-5237-z
Dornelas, M., and Daskalova, G. N. (2020). Nuanced changes in insect abundance. Science. 368, 368–369. doi: 10.1126/science.abb6861
Dudgeon, D., Arthington, A. H., Gessner, M. O., Kawabata, Z.-I., Knowler, D. J., Lévêque, C., et al. (2006). Freshwater biodiversity: importance, threats, status and conservation challenges. Biol. Rev. 81, 163. doi: 10.1017/S1464793105006950
Edegbene, A. O., Arimoro, F. O., and Odume, O. N. (2020). Exploring the distribution patterns of macroinvertebrate signature traits and ecological preferences and their responses to urban and agricultural pollution in selected rivers in the Niger Delta ecoregion, Nigeria. Aquatic Ecol. 54, 553–573. doi: 10.1007/s10452-020-09759-9
Eggleton, P. (2020). The state of the world's insects. Annu. Rev. Environ. Resour. 45, 61–82. doi: 10.1146/annurev-environ-012420-050035
Gál, B., Szivák, I., Heino, J., and Schmera, D. (2019). The effect of urbanization on freshwater macroinvertebrates – Knowledge gaps and future research directions. Ecol. Indic. 104, 357–364. doi: 10.1016/j.ecolind.2019.05.012
Gallardo, L. I., Coronel, J. M., and Poi, A. S. G. (2019). Urban rain-fed lakes: macro-invertebrate assemblages associated with Egeria najas as indicators of biological integrity in wetlands of Corrientes Province (Argentina). Biodivers. Conserv. 28, 1549–1568. doi: 10.1007/s10531-019-01742-7
Gezie, A., Anteneh, W., Dejen, E., and Mereta, S. T. (2017). Effects of human-induced environmental changes on benthic macroinvertebrate assemblages of wetlands in Lake Tana Watershed, Northwest Ethiopia. Environ. Monit. Assess. 189, 152. doi: 10.1007/s10661-017-5853-2
Gledhill, D. G., James, P., and Davies, D. H. (2008). Pond density as a determinant of aquatic species richness in an urban landscape. Landsc. Ecol. 23, 1219–1230. doi: 10.1007/s10980-008-9292-x
Haraway, D. (2016). The companion species manifesto: Dogs, people, and significant otherness. In: Manifestly Haraway. Minneapolis, MN: University of Minnesota Press. p. 91–198.
Hassall, C., and Anderson, S. (2015). Stormwater ponds can contain comparable biodiversity to unmanaged wetlands in urban areas. Hydrobiologia 745, 137–149. doi: 10.1007/s10750-014-2100-5
Hepp, L. U., Milesi, S. V., Biasi, C., and Restello, R. M. (2010). Effects of agricultural and urban impacts on macroinvertebrates assemblages in streams (Rio Grande do Sul, Brazil). Zoologia. 27, 106–113. doi: 10.1590/S1984-46702010000100016
Hill, M., Ryves, D., White, J., and Wood, P. (2016). Macroinvertebrate diversity in urban and rural ponds: Implications for freshwater biodiversity conservation. Biol. Conserv. 201, 50–59. doi: 10.1016/j.biocon.2016.06.027
Kim, K. C. (1993). Biodiversity, conservation and inventory: why insects matter. Biodivers. Conserv. 2, 191–214. doi: 10.1007/BF00056668
Kunz, S., Kefford, B. J., Schmidt-Kloiber, A., Matthaei, C. D., Usseglio-Polatera, P., Graf, W., et al. (2022). Tackling inconsistencies among freshwater invertebrate trait databases: harmonising across continents and aggregating taxonomic resolution. Freshw. Biol. 67, 275–291. doi: 10.1111/fwb.13840
Le Viol, I., Mocq, J., Julliard, R., and Kerbiriou, C. (2009). The contribution of motorway stormwater retention ponds to the biodiversity of aquatic macroinvertebrates. Biol. Conserv. 142, 3163–3171. doi: 10.1016/j.biocon.2009.08.018
Leather, S. R. (2018). “Ecological Armageddon” - more evidence for the drastic decline in insect numbers: insect declines. Ann. Appl. Biol. 172, 1–3. doi: 10.1111/aab.12410
Legendre, P., Galzin, R., and Harmelin-Vivien, M. L. (1997). Relating behavior to habitat: solutions to the fourth-corner problem. Ecology 78, 547–562. doi: 10.2307/2266029
Liao, W., Venn, S., and Niemel,ä, J. (2020). Environmental determinants of diving beetle assemblages (Coleoptera: Dytiscidae) in an urban landscape. Biodivers. Conserv. 29, 2343–2359. doi: 10.1007/s10531-020-01977-9
Losey, J. E., and Vaughan, M. (2006). The economic value of ecological services provided by insects. Bioscience 56, 311. doi: 10.1641/0006-3568(2006)56(311:TEVOES)2.0.CO;2
Lunde, K. B., and Resh, V. H. (2012). Development and validation of a macroinvertebrate index of biotic integrity (IBI) for assessing urban impacts to Northern California freshwater wetlands. Environ. Monit. Assess. 184, 3653–3674. doi: 10.1007/s10661-011-2214-4
Marchamalo, M., Springer, M., Acosta, R., González-Rodrigo, B., and Vásquez, D. (2018). Responses of aquatic macroinvertebrates to human pressure in a tropical highland volcanic basin: Birrís River, Irazú Volcano (Costa Rica). Hidrobiológica 28, 179–190. doi: 10.24275/uam/izt/dcbs/hidro/2018v28n2/Marchamalo
McKinney, M. L., and Lockwood, J. L. (1999). Biotic homogenization: a few winners replacing many losers in the next mass extinction. Trends Ecol. Evol. 14, 450–453. doi: 10.1016/S0169-5347(99)01679-1
Meyer, J. L., Paul, M. J., and Taulbee, W. K. (2005). Stream ecosystem function in urbanizing landscapes. J. North Am. Benthol. Soc. 24, 602–612. doi: 10.1899/04-021.1
Morse, J. C., Frandsen, P. B., Graf, W., and Thomas, J. A. (2019). Diversity and ecosystem services of Trichoptera. Insects 10, 125. doi: 10.3390/insects10050125
Moyo, N. A. G., and Rapatsa, M. M. (2016). Impact of urbanization on the ecology of Mukuvisi River, Harare, Zimbabwe. Phy. Chem. Earth A/B/C 92, 14–19. doi: 10.1016/j.pce.2015.09.007
Murray-Stoker, K. M., Batzer, D. P., Murray-Stoker, D., and McHugh, J. V. (2020). Shifts in the community composition of caddisflies (Insecta: Trichoptera) in a subtropical river over three decades. Ecol. Entomol. 45, 514–524. doi: 10.1111/een.12822
Odume, O. N., Ntloko, P., Akamagwuna, F. C., Dallas, H. F., and Barber-James, H. (2018). Development of Macroinvertebrate Trait-Based Approach for Assessing and Managing Ecosystem Health in South African rivers – Incorporating a Case Study in the Tsitsa river and Its Tributaries, Eastern Cape. Pretoria: Water Research Commission.
Oertli, B., and Parris, K. M. (2019). Review: Toward management of urban ponds for freshwater biodiversity. Ecosphere 10, e02810. doi: 10.1002/ecs2.2810
Pavel, B., Alexandra, R., Fedor, C., and Ciamporová-Zatovičová, Z. (2016). Catchment land use as a predictor of the macroinvertebrate community changes between inlet and outlet of small water dams. Environ. Monit. Assess. 188, 550. doi: 10.1007/s10661-016-5552-4
Perron, M. A. C., and Pick, F. R. (2020). Water quality effects on dragonfly and damselfly nymph communities: a comparison of urban and natural ponds. Environ. Pollut. 263, 114472. doi: 10.1016/j.envpol.2020.114472
Phillips, N., and Smith, B. (2018). New Zealand Freshwater Macroinvertebrate Trait Database. National Institute of Water and Atmospheric Research Taihoro Nukurangi. Available online at: https://niwa.co.nz/freshwater/management-tools/aquatic-invertebrate-traits-database (accessed December 15, 2022).
Poff, N. L., Olden, J. D., Vieira, N. K. M., Finn, D. S., Simmons, M. P., and Kondratieff, B. C. (2006). Functional trait niches of North American lotic insects: traits-based ecological applications in light of phylogenetic relationships. J. North Am. Benthol. Soc. 25, 730–755. doi: 10.1899/0887-3593(2006)025(0730:FTNONA)2.0.CO;2
Ramírez, A., De Jesús-Crespo, R., Martinó-Cardona, D. M., Martínez-Rivera, N., and Burgos-Caraballo, S. (2009). Urban streams in Puerto Rico: what can we learn from the tropics? J. North Am. Benthol. Soc. 28, 1070–1079. doi: 10.1899/08-165.1
Reid, A. J., Carlson, A. K., Creed, I. F., Eliason, E. J., Gell, P. A., Johnson, P. T. J., et al. (2019). Emerging threats and persistent conservation challenges for freshwater biodiversity. Biol. Rev. 94, 849–873. doi: 10.1111/brv.12480
Resh, V. H., and Unzicker, J. D. (1975). Water quality monitoring and aquatic organisms: The importance of species identification. J. Water Pollut. Control Fed. 47, 9–19.
Ricciardi, A., and Rasmussen, J. B. (1999). Extinction rates of North American freshwater fauna. Conserv. Biol. 13, 1220–1222. doi: 10.1046/j.1523-1739.1999.98380.x
Rogers, C. E., Brabander, D. J., Barbour, M. T., and Hemond, H. F. (2002). Use of physical, chemical, and biological indices to assess impacts of contaminants and physical habitat alteration in urban streams. Environ. Toxicol. Chem. 21, 1156–1167. doi: 10.1002/etc.5620210607
Roth, N., Zoder, S., Zaman, A. A., Thorn, S., and Schmidl, J. (2020). Long-term monitoring reveals decreasing water beetle diversity, loss of specialists and community shifts over the past 28 years. Insect Conserv. Divers. 13, 140–150. doi: 10.1111/icad.12411
Roy, A. H., Rosemond, A. D., Paul, M. J., Leigh, D. S., and Wallace, J. B. (2003). Stream macroinvertebrate response to catchment urbanisation (Georgia, U.S.A.). Freshw. Biol. 48, 329–346. doi: 10.1046/j.1365-2427.2003.00979.x
Sala, O. E., Stuart Chapin, F. Iii, Armesto, J. J., Berlow, E., Bloomfield, J., et al. (2000). Global biodiversity scenarios for the year 2100. Science. 287, 1770–1774. doi: 10.1126/science.287.5459.1770
Sánchez-Bayo, F., and Wyckhuys, K. A. G. (2019). Worldwide decline of the entomofauna: a review of its drivers. Biol. Conserv. 232, 8–27. doi: 10.1016/j.biocon.2019.01.020
Sarremejane, R., Cid, N., Stubbington, R., Datry, T., Alp, M., Cañedo-Argüelles, M., et al. (2020). DISPERSE, a trait database to assess the dispersal potential of European aquatic macroinvertebrates. Sci. Data 7, 386. doi: 10.1038/s41597-020-00732-7
Sayre, R., Karagulle, D., Frye, C., Boucher, T., Wolff, N. H., Breyer, S., et al. (2020). An assessment of the representation of ecosystems in global protected areas using new maps of World Climate Regions and World Ecosystems. Glob. Ecol. Conserv. 21, e00860. doi: 10.1016/j.gecco.2019.e00860
Schell, C. J., Dyson, K., Fuentes, T. L., Roches, S. D., Harris, N. C., Miller, D. S., et al. (2020). The ecological and evolutionary consequences of systemic racism in urban environments. Science 369, eaay4497. doi: 10.1126/science.aay4497
Schmidt-Kloiber, A., and Hering, D. (2015). www.freshwaterecology.info – An online tool that unifies, standardises and codifies more than 20,000 European freshwater organisms and their ecological preferences. Ecol. Indic. 53, 271–282. doi: 10.1016/j.ecolind.2015.02.007
Sitati, A., Raburu, P. O., Yegon, M. J., and Masese, F. O. (2021). Land-use influence on the functional organization of Afrotropical macroinvertebrate assemblages. Limnologica 88, 125875. doi: 10.1016/j.limno.2021.125875
Stendera, S., Adrian, R., Bonada, N., Cañedo-Argüelles, M., Hugueny, B., Januschke, K., et al. (2012). Drivers and stressors of freshwater biodiversity patterns across different ecosystems and scales: a review. Hydrobiologia 696, 1–28. doi: 10.1007/s10750-012-1183-0
Stepanian, P. M., Entrekin, S. A., Wainwright, C. E., Mirkovic, D., Tank, J. L., and Kelly, J. F. (2020). Declines in an abundant aquatic insect, the burrowing mayfly, across major North American waterways. Proc. Natl. Acad. Sci. 117, 2987–2992. doi: 10.1073/pnas.1913598117
Stephansen, D. A., Nielsen, A. H., Hvitved-Jacobsen, T., Pedersen, M. L., and Vollertsen, J. (2016). Invertebrates in stormwater wet detention ponds — Sediment accumulation and bioaccumulation of heavy metals have no effect on biodiversity and community structure. Sci. Total Environ. 566–567, 1579–1587. doi: 10.1016/j.scitotenv.2016.06.050
Tszydel, M., Markowski, M., Majecki, J., Błońska, D., and Zieliński, M. (2015). Assessment of water quality in urban streams based on larvae of Hydropsyche angustipennis (Insecta, Trichoptera). Environ. Sci. Pollut. Res. 22, 14687–14701. doi: 10.1007/s11356-015-4638-9
Twardochleb, L., Hiltner, E., Pyne, M., and Zarnetske, P. (2021). Freshwater insects CONUS: a database of freshwater insect occurrences and traits for the contiguous United States. Glob. Ecol. Biogeogr. 30, 826–841. doi: 10.1111/geb.13257
Urban, M. C., Skelly, D. K., Burchsted, D., Price, W., and Lowry, S. (2006). Stream communities across a rural-urban landscape gradient. Divers. Distrib. 12, 337–350. doi: 10.1111/j.1366-9516.2005.00226.x
van Klink, R., Bowler, D. E., Gongalsky, K. B., Swengel, A. B., Gentile, A., and Chase, J. M. (2020). Meta-analysis reveals declines in terrestrial but increases in freshwater insect abundances. Science 368, 417–420. doi: 10.1126/science.aax9931
Vanbergen, A. J., and the Insect Pollinators Initiative. (2013). Threats to an ecosystem service: pressures on pollinators. Front. Ecol. Environ. 11, 251–259. doi: 10.1890/120126
Violin, C. R., Cada, P., Sudduth, E. B., Hassett, B. A., Penrose, D. L., and Bernhardt, E. S. (2011). Effects of urbanization and urban stream restoration on the physical and biological structure of stream ecosystems. Ecol. Appl. 21, 1932–1949. doi: 10.1890/10-1551.1
Vörösmarty, C. J., McIntyre, P. B., Gessner, M. O., Dudgeon, D., Prusevich, A., Green, P., et al. (2010). Global threats to human water security and river biodiversity. Nature 467, 555–561. doi: 10.1038/nature09440
Wagner, D. L. (2020). Insect declines in the Anthropocene. Annu. Rev. Entomol. 65, 457–480. doi: 10.1146/annurev-ento-011019-025151
Wagner, D. L., Grames, E. M., Forister, M. L., Berenbaum, M. R., and Stopak, D. (2021). Insect decline in the Anthropocene: death by a thousand cuts. Proc. Natl. Acad. Sci. 118, e2023989118. doi: 10.1073/pnas.2023989118
Walsh, C. J., Roy, A. H., Feminella, J. W., Cottingham, P. D., Groffman, P. M., and II, R. P. M. (2005). The urban stream syndrome: current knowledge and the search for a cure. J. North Am. Benthol. Soc. 24, 706–723. doi: 10.1899/04-028.1
Walsh, C. J., Waller, K. A., Gehling, J., and Nally, R. M. (2007). Riverine invertebrate assemblages are degraded more by catchment urbanisation than by riparian deforestation. Freshw. Biol. 52, 574–587. doi: 10.1111/j.1365-2427.2006.01706.x
Wan Abdul Ghani, W. M. H., Kutty, A. A., Mahazar, M. A., Al-Shami, S. A., and Hamid, S. A. (2018). Performance of biotic indices in comparison to chemical-based Water Quality Index (WQI) in evaluating the water quality of urban river. Environ. Monit. Assess. 190, 297. doi: 10.1007/s10661-018-6675-6
Wantzen, K., Alves, C., Badiane, S., Bala, R., Blettler, M., Callisto, M., et al. (2019). Urban stream and wetland restoration in the Global South—A DPSIR Analysis. Sustainability 11, 4975. doi: 10.3390/su11184975
White, J. Y., and Walsh, C. J. (2020). Catchment-scale urbanization diminishes effects of habitat complexity on instream macroinvertebrate assemblages. Ecol. Appl. 30, e2199. doi: 10.1002/eap.2199
Keywords: aquatic insects, urbanization, global change, community diversity, temperate, tropical
Citation: Ferzoco IMC, Murray-Stoker KM, Hasan LS, Javier CM, Modi V, Singh R, Tjan G, Toth O and McCauley SJ (2023) Freshwater insect communities in urban environments around the globe: a review of the state of the field. Front. Ecol. Evol. 11:1174166. doi: 10.3389/fevo.2023.1174166
Received: 25 February 2023; Accepted: 17 April 2023;
Published: 05 May 2023.
Edited by:
David Houghton, Hillsdale College, United StatesReviewed by:
Ed DeWalt, University of Illinois at Urbana-Champaign, United StatesTodd Hubbard, The University of Iowa, United States
Copyright © 2023 Ferzoco, Murray-Stoker, Hasan, Javier, Modi, Singh, Tjan, Toth and McCauley. This is an open-access article distributed under the terms of the Creative Commons Attribution License (CC BY). The use, distribution or reproduction in other forums is permitted, provided the original author(s) and the copyright owner(s) are credited and that the original publication in this journal is cited, in accordance with accepted academic practice. No use, distribution or reproduction is permitted which does not comply with these terms.
*Correspondence: Kelly M. Murray-Stoker, ay5tdXJyYXlzdG9rZXImI3gwMDA0MDt1dG9yb250by5jYQ==
†These authors have contributed equally to this work and share first authorship