- 1Institute of Environmental Health and Ecological Security, School of Environment and Safety Engineering, Jiangsu University, Zhenjiang, China
- 2Ministry of Education Key Laboratory of Pollution Processes and Environmental Criteria, Nankai University, Tianjin, China
- 3Key Laboratory of Environmental Biotechnology, Research Center for Eco-Environmental Sciences, Chinese Academy of Sciences, Beijing, China
- 4School of Environment and Safety Engineering, Jiangsu University, Zhenjiang, China
- 5Department of Civil and Environmental Engineering, College of Engineering, Seoul National University, Seoul, Republic of Korea
- 6School of Management, Shandong Technology and Business University, Yantai, Shandong, China
- 7School of Emergency Management, Jiangsu University, Zhenjiang, China
Invasive alien plants pose severe threats to agroecosystems. Microplastic (MP) contamination in farmland soil is also concerning, as it causes crop stress and reduces productivity. However, the effects of the interactions between invasive alien plants and MP in the soil impact crops remain unclear. Herein, belowground plant characteristics associated with stress responses were examined in a pot experiment using root scan analyzes of rice plants exposed to Solidago canadensis L. invasion, polyethylene MP contamination, and a combined treatment. The observed changes in root growth traits under Canada goldenrod (Solidago canadensis L.) invasion were the least adverse, whereas S. canadensis invasion combined with soil MP contamination had the most adverse effects on root growth. Solidago canadensis L. invasion increased all belowground indices except root height and mean root diameter, which was upregulated in the soil MP contamination treatment. The combined treatment (S. canadensis invasion and soil MP contamination) reduced the belowground root growth traits more than the other treatments. The root growth traits may have been affected by changes in the antioxidant enzyme activity of the roots caused by the treatments. The combined effects of S. canadensis invasion and MP toxicity on rice root growth traits raise concerns regarding potential yields, financial damage, and consequences related to a potential move into the food web.
1. Introduction
Alien plant invasion is considered to be one of the most severe global environmental issues, as it poses significant threats to native ecosystems by modifying the structure and internal processes that affect the ecosystems (Powell et al., 2013; Yuan et al., 2013). Owing to intensive human activity and exhaustive farming, agroecosystems suffer more from alien plant invasions compared to other ecosystems (Yang et al., 2008; Wang et al., 2016). Release of active metabolites and toxic compounds into the soil, referred to as allelochemicals, by invasive plants may result in differentiated allelopathy effects on agroecosystems, particularly of those related to crop growth performance, seed germination, and seedling growth (Sun and He, 2010; Yuan et al., 2013; Wang et al., 2016; Lu et al., 2020). Cropland in particular is strongly affected by invasive plant species (Wang et al., 2018b), owing to their often unimpeded spread (Wang et al., 2018a). Once the alien plants have successfully invaded agricultural land, they can negatively affect crop productivity (Lu et al., 2007, 2020; Wang et al., 2016). Furthermore, alien plant invasion can affect plant hormone pathways, which are the most important mechanisms through which crop plants adjust to environmental changes (Agathokleous et al., 2019) and can cause highly detrimental effects to crop physiology and root growth traits (Wang et al., 2016).
Annual increases in untreated plastic trash have made plastic contamination a serious concern, particularly on agricultural land (Lian et al., 2021a,b). However, plastic is still an essential component of daily life worldwide owing to its stability, durability, light weight, and low cost (Phuong et al., 2016). Microplastic (MP) pollution has become a fundamental problem, as unmanaged plastic waste increases steadily (Lian et al., 2021a). Owing to the ongoing increases in plastic production, which reached 368 million tons in 2019 as reported by Plastics Europe (2020), such “new generation” pollutants are a global concern (De Souza Machado et al., 2018). The emergence and spread of plastic pollutants into the environment and their extremely low biodegradability are also major global concerns. Global plastic pollution reached 6.3 billion metric tons in 2015 and is predicted to increase to 12 billion metric tons by 2050 (Geyer et al., 2017). Crop cultivation may be affected by MP contamination in the soil; however, the contaminating effects on crop plants remain unclear, as numerous studies have reported contradictory results, suggesting that MP contamination can exert negative or positive impacts on crop performance (Khalid et al., 2020; Lian et al., 2020a,b). MPs occur in various shapes, including fragments, films, and fibers, and in different polymer types, including polyester, polypropylene, polyacrylic, and polyethylene (Lian et al., 2020b). MPs can be distributed in terrestrial ecosystems, particularly agroecosystems, through various channels, including plastic film mulching, distribution of biosolids, and atmospheric deposition (Ng et al., 2018; Wang et al., 2019, 2020; Kumar et al., 2020).
High MP contamination in agricultural soil is predominantly attributed to the excessive use of plastic mulching film, which has caused severe problems by altering the soil biota (Rezania et al., 2018; Zhang Y. et al., 2022). Therefore, MPs affect nutrient cycling, exert pressure on soil biota, and directly influence plant growth and development (Bosker et al., 2019; Li S. et al., 2021; Zhao et al., 2022). However, MP contamination has the most substantial effects on crop growth, beginning with seed germination, which is the first and most crucial phase of a crop’s life span, followed by root development, on which the crop body structure and shoot, stem, and leaf development depends (Gao et al., 2019; Yuan et al., 2019; Sun et al., 2020; Li B. et al., 2021). Therefore, MPs are a substantial threat to terrestrial ecosystems (De Souza Machado et al., 2018; Khalid et al., 2020).
Belowground roots primarily absorb water and nutrients from the soil to accommodate plant requirements and promote plant growth, thereby significantly enhancing crop yields (Iqbal et al., 2023b). However, modifications to their distribution and morphological characteristics may enhance nutrient acquisition, which further affects plant performance (Xia et al., 2020). Plant roots in diverse environments and those specifically in the vicinity of invasive ecosystems propagate several branches and grow horizontally, guaranteeing intense competition for nutrients and water (Su et al., 2018). As a result, when competing with the surrounding plants for such a confined nutrient availability, Chinese fir seedlings are known to increase their specific root length to improve nutrient acquisition and affect plant growth and development (Xu et al., 2019). Moreover, when combined with the arbuscular mycorrhizal network, the invasive plant exhibits considerable advantages related to nutrient availability, root growth traits, and root development process (Xia et al., 2020). However, understanding how invasive species interact with crops is necessary to better acclimate root growth traits to environmental pollutants and stressors. Moreover, MP contamination in the soil adversely affects root growth traits, performance, and MP movement in the crop body (Chen et al., 2022; Zhang Y. et al., 2022).
Potential interactions between MPs and other adverse ecological issues may result in synergistic, additive, or antagonistic effects. The highly invasive Canada goldenrod (Solidago canadensis L.) is dispersed widely throughout most of China’s provinces, especially in southern China. It is considered to be one of the most harmful and pervasive invasive species (Zhao et al., 2015) and has attracted increasing research attention worldwide. However, both S. canadensis and MP treatments have been observed frequently on agricultural land, which substantially affected crop productivity (Wang et al., 2016; Du et al., 2020; Zhang Y. et al., 2022; Zhao et al., 2022). However, potential changes in root growth traits under S. canadensis invasion and soil MP contamination remain largely unknown. The objectives of this study were to identify the response of belowground growth traits to Solidago canadensis L. invasion and soil MP contamination. Root scanning experiments were carried out to explore the belowground root system and its correlations with previously published root antioxidant enzymatic activities (Zhao et al., 2022) related to competition for water, nutrient uptake, and enhancing crop productivity. Identifying S. canadensis and MP effects on belowground root growth traits is critical for directing future research on the potential adverse effects of such treatments on root growth traits, which are intended to increase the rice crop biomass and in turn facilitate yields.
2. Materials and methods
2.1. Preparation of materials
Polyethylene MPs are widely used in farmland ecosystems owing to the application of plastic mulch film, which constitutes a major proportion of MP pollution in farmland soil (De Souza Machado et al., 2018). Plastic mulch film has been used widely in global agriculture to improve water use efficiency and crop yields (Dong et al., 2020; Kumar et al., 2020; Gao et al., 2021; Lozano et al., 2021). MPs occur in various shapes in natural farmland soil, and three shapes of polyethylene MPs (i.e., pellets, fragments, and fibers) were used in this study. MP pellets were purchased from the Dongwan Zhangmutou Huahuang plastic material firm (Dongguan, Guangdong, China), and MP fragments and fibers were produced by cutting a polyethylene plate (Shanghai Dayou Hardware Co.) and rope (0.20 mm diameter) into fiber particles <2.00 mm in length, respectively. Pellet and fragment MP particles were produced by sieving through 18-mesh (1.00 mm) and 30-mesh (0.60 mm) screens. MP particles of all three shape categories were mixed at a proportion of 30% pellets, 40% fragments, and 30% fibers, and the mixture was then applied to experimental pot soil. The ratio used herein is commonly observed in the environment, with minor variations between crops (Zhao et al., 2022). Prior to use, the MPs were sterilized and kept at 4°C for storage. MP treatments were executed as described previously (Pignattelli et al., 2020).
A hybrid of the conventional indica rice variety Ning Japonica-12 was used as the test crop in June 2021. The seedlings were obtained from the Baima research station of Nanjing Agricultural University. However, a random collection of S. canadensis seeds occurred in Zhenjiang City in December 2020. The seedlings were grown in a greenhouse in April 2021, and identical sizes were carefully selected for the experiments.
In April 2021, soil samples were taken from the surface of green space (0–20 cm) without MP exposure or S. canadensis invasion at the Jiangsu University campus in Zhenjiang City (32°12′ N, 119°30′ E). The air-dried soil was sieved through a 10-mesh (2.00 mm) sieve after being cleared of plant debris and stones and was then kept in the dark until the experiment.
2.2. Experimental design and crop collection
The experiment was performed in pots at the greenhouse of Jiangsu University from the middle of June to the end of October 2021. The complete factorial design was maintained, with four treatments and 14 replicates for a total of 56 plots, which comprised control, S. canadensis invasion (SI), MP contamination (MPc), and combined (SI × MP) Treatments. All treatment placements in the corresponding pots and the transferal of seedlings to the pots followed the procedures described previously (Zhao et al., 2022). After transplantation, every pot was put in a protected environment with ample sunlight. After careful growing following agronomic practices, the crops were uprooted 50 and 80 days after transplanting (DAT). At each step, samples from seven pots per treatment were collected.
2.3. Root scanning
Root scanning was performed on the uprooted rice plants. To get rid of impurities like soil, the roots were cut off and properly cleansed with distilled water. The roots of each plant were scanned using a WinRhizoTM scanner-based system (v.2007; Reagent Instruments Inc., Quebec City, QC, Canada) to measure the root area (RA), root width (RW), root height (RH), mean root diameter (MRD), root surface area (RSA), root volume (RV), root length (RL), and numbers of root tips, branches, and crossings.
2.4. Statistical analysis
The direct impacts of Solidago canadensis L. invasion, soil MP contamination, growth time, and their interactions on root growth characteristic metrics were examined using repeated measures analysis of variance (RMANOVA) at 50 and 80 DAT. The effects of the treatments on all indices were examined using a one-way ANOVA and Fisher’s least significant difference test. Rice root growth traits were compared between treatments across the sampling dates using principal component analysis (PCA). Redundancy analysis (RDA) was used to analyze differences in the root traits related to antioxidant enzyme activity in the roots (based on data from Zhao et al., 2022). Pearson’s correlation test was used to assess correlations between the root growth traits and antioxidant enzyme activity. The R software package (ver. 4.1.1) was used to perform all analyzes (R Core Team, 2013).
3. Results
3.1. Effects on root growth traits
The SI, MPc, and SI × MP treatments significantly affected the RA, RW, and RH (Table 1). Compared with the control, RA and RW were higher in the SI treatment and lower in the MPc and SI × MP treatments. The SI × MP treatment caused more substantial reductions in RA, RW, and RH. RH was higher in the MPc treatment than in the control. RA, RW, and RH were higher at 80 DAT than those at 50 DAT for all treatments. The RMANOVA indicates that the different treatments and DAT had highly significant effects on RA, RW, and RH (Supplementary Table S1). Compared with the control, the SI × MP treatment exhibited the most pronounced reductions (~33.02, 22.17, and 15.15%) in RA, RW, and RH, respectively, at 50 DAT and 80 DAT (39.84, 24.39, and 22.76%, respectively). The SI treatment had significantly higher RSA, RV, and RL values, whereas the MPc treatment had higher MRDs compared with those in the control. The RMANOVA showed a highly significant effect of different treatments and DAT on the crop’s MRD, RSA, and RL (Supplementary Table S1). The RMANOVA indicates that the SI treatment had significant effects on MRD (p < 0.001), RSA (p < 0.001) and RL (p < 0.05), but did not have a significant effect on RV (p > 0.05). The MPc treatment had highly significant effects on RSA (p < 0.001), RV (p < 0.001), and RL (p < 0.001), but not on MRD. The SI × MP treatment had highly significant effects on MRD, RSA, RV, and RL (all p < 0.001; Supplementary Table S1).
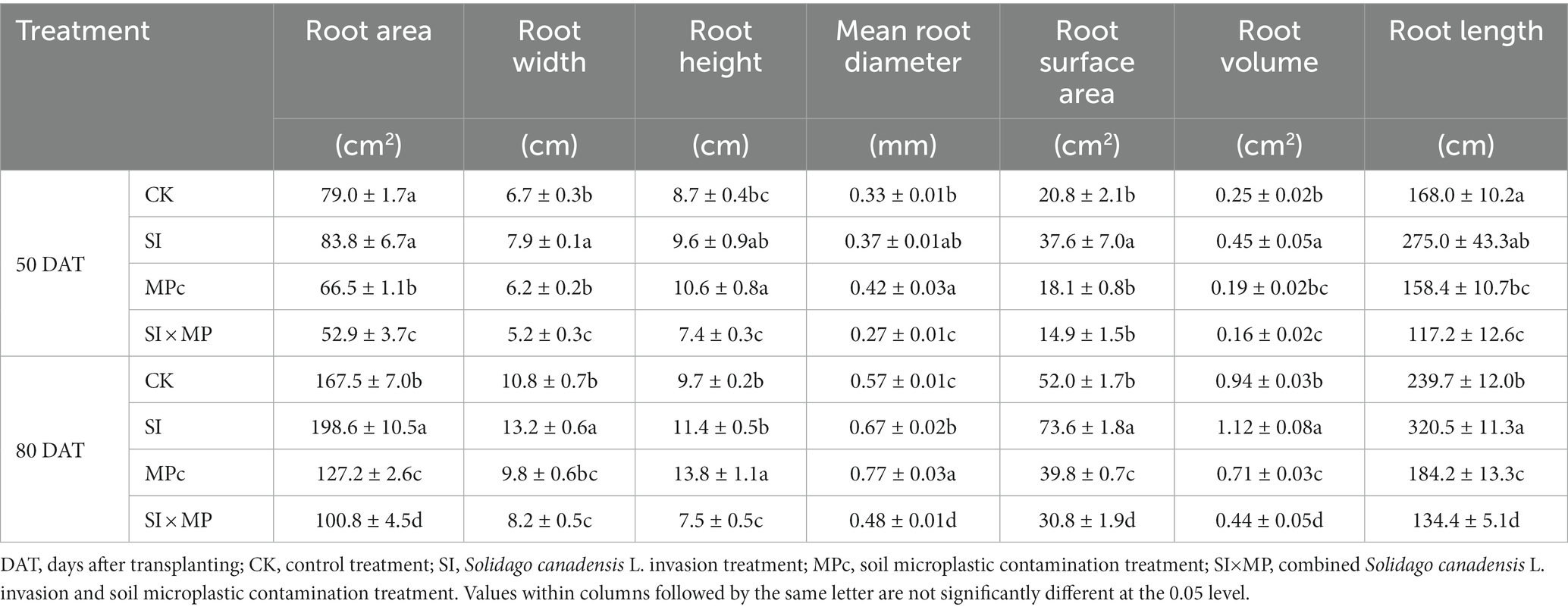
Table 1. Mean root areas, widths, heights, diameters, surface areas, volumes, and lengths of rice plants under different treatments at 50 and 80 days after transplanting (presented as mean ± standard error, n = 7).
The SI, MPc, and SI × MP treatments significantly affected the root branches, crossings, and tips compared with those of the control (Table 2). The SI treatment significantly increased the numbers of root branches and tips, whereas the MPc and SI × MP treatments decreased these properties compared with the control. Root crossings were less abundant in all treatments. The SI treatment produced a weaker effect on root crossings compared with the control, but a larger reduction was observed in the SI × MP treatment. The numbers of root branches, crossings, and tips were higher at 80 DAT than at 50 DAT. The treatment type and sampling time significantly affected the numbers of root branches, crossings, and tips (Supplementary Table S1). The SI × MP treatment caused more significant reductions in the root traits. The RMANOVA indicates that the SI treatment had a highly significant effect on the number of root crossings (p < 0.001) and no significant effects on the numbers of root branches and tips, whereas the MPc treatment had highly significant effects on the numbers of root branches, crossings, and tips (all p < 0.001). The SI × MP treatment also had highly significant effects on the numbers of root branches and tips (both p < 0.001) and a significant effect on the number of root crossings (p < 0.05; Supplementary Table S1). Compared with the control, the SI × MP treatment yielded fewer root branches, crossings, and tips at 50 DAT (decreases of approximately 48.9, 79.8, and 40.7%, respectively) and 80 DAT (decreases of 52.2, 79.2, and 56.1%, respectively).
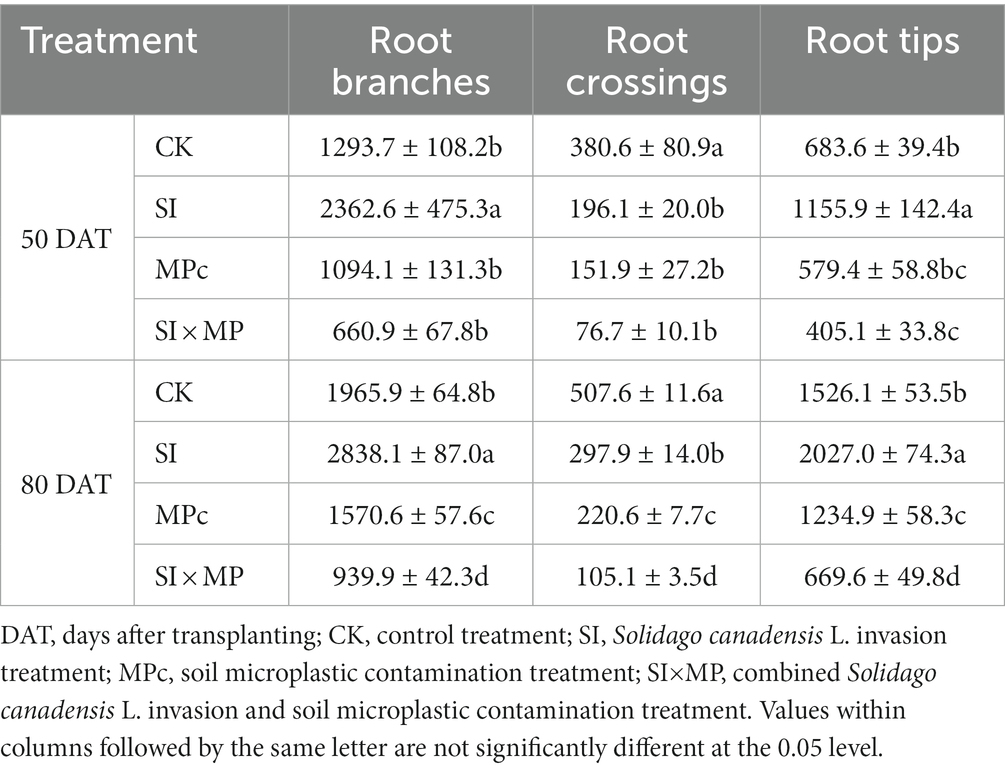
Table 2. Mean numbers of root branches, crossings, and tips of the rice plants in the different treatments at 50 and 80 days after transplanting (presented as mean ± standard error, n = 7).
The two-dimensional PCA indicates that PC1 and PC2 explained 72 and 19% of the variation, respectively, by combining all of the belowground root growth traits. Moreover, the PCA indicates that a clear shift in root growth traits occurred along the first axis of the PCA, which was induced by treatment type and DAT (Figure 1).
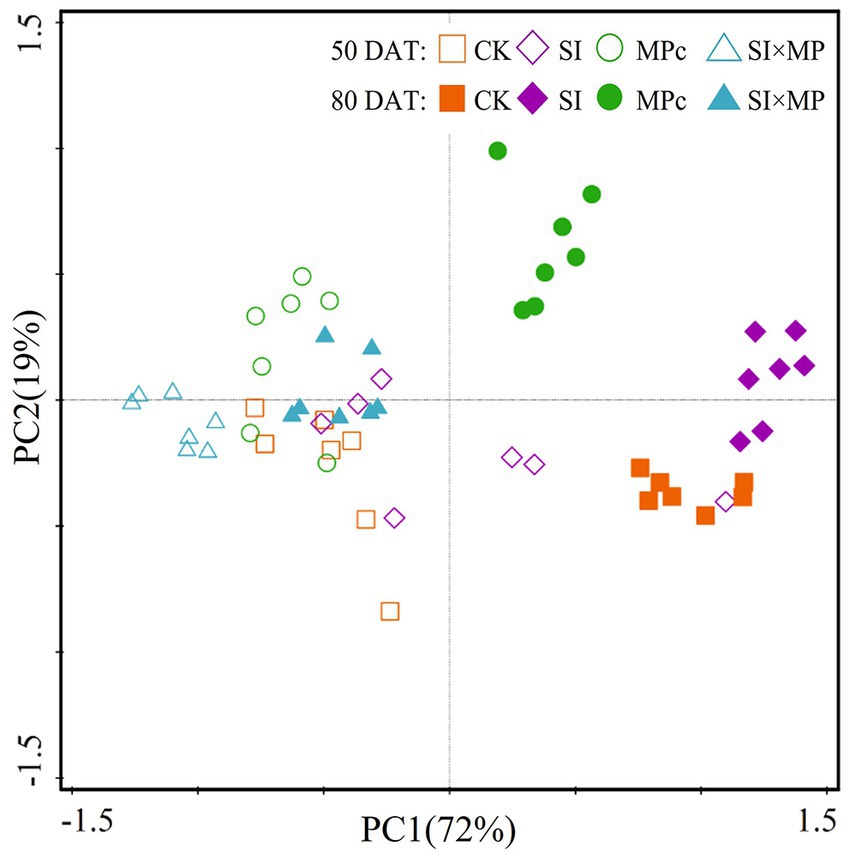
Figure 1. Changes in root growth traits in the SI, MPc, and SI × MP treatments. Principal component analysis (PCA) of root growth traits at 50 and 80 days after transplanting (DAT). CK = control; SI = Solidago canadensis L. invasion; MPc = soil microplastic contamination; and SI × MP = combined Solidago canadensis L. invasion and soil microplastic contamination.
3.2. Interactions between antioxidant enzymes activities and root growth indices
A two-dimensional PCA of root antioxidant enzyme activity indicates that PC1 and PC2 explained 51 and 34% of the variations, respectively. Furthermore, the PCA revealed significant changes in the root antioxidant enzyme activities owing to the treatment type and DAT (Figure 2).
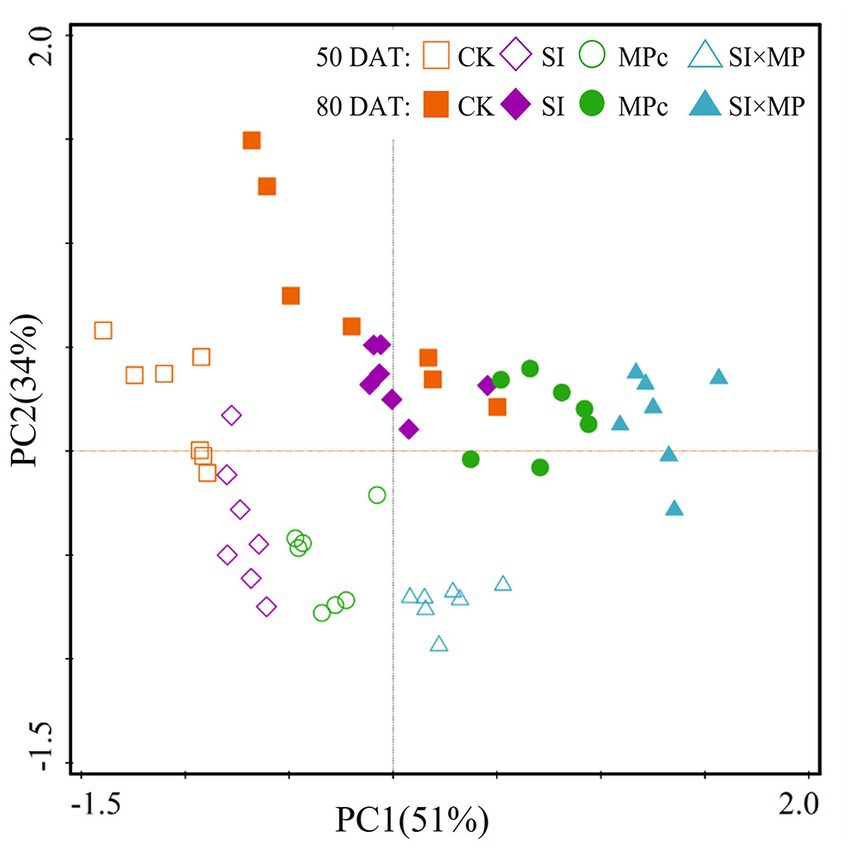
Figure 2. Changes in root antioxidant enzyme activities in the SI, MPc, and SI × MP treatments. PCA of root antioxidant enzyme activities at 50 and 80 DAT. CK = control; SI = Solidago canadensis L. invasion; MPc = soil microplastic contamination; and SI × MP = combined Solidago canadensis L. invasion and soil microplastic contamination.
The redundancy analysis (RDA) indicates that the root growth traits and antioxidant enzyme activities explained 34.4 and 4.9% of the variance, respectively (Figure 3). The correlations between the root growth traits and antioxidant enzyme activities are shown in Figure 4. Overall, the antioxidant enzyme activities had the most substantial impacts on root growth traits at 50 and 80 DAT. Thus, the variations in the root growth traits were directly proportional to the antioxidant enzyme activities in the roots, which were noticeable in the rice plants at 50 and 80 DAT.
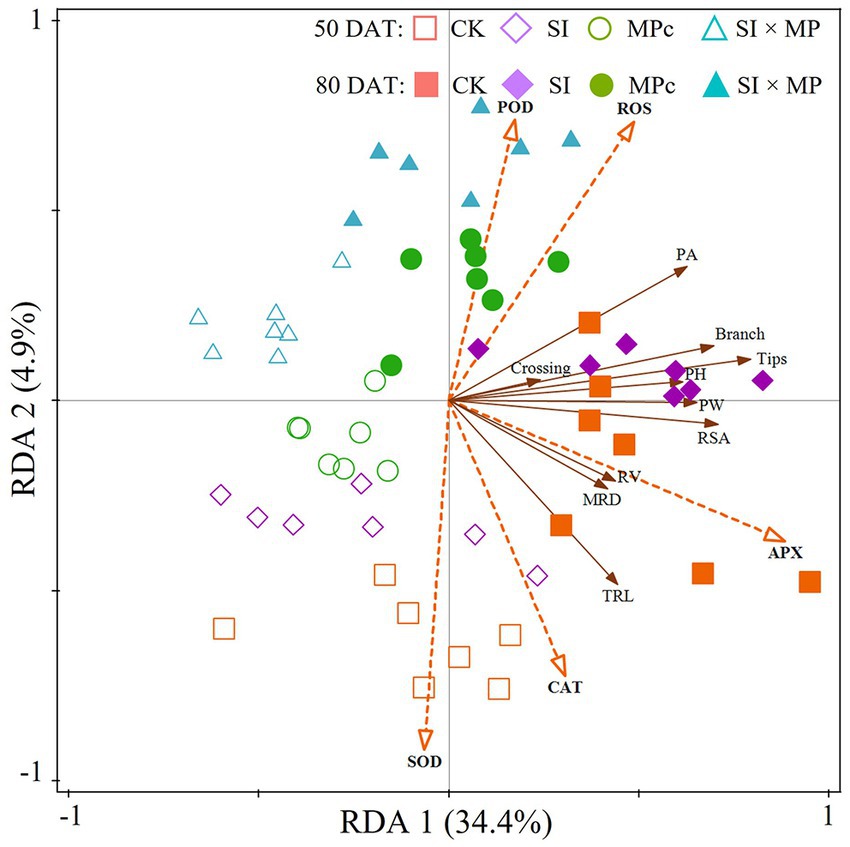
Figure 3. Redundancy analysis (RDA) based on root growth traits and antioxidant enzyme activities. RA = root area; RW = root width; RH = root height; MRD = mean root diameter; RSA = root surface area; RV = root volume; RL = root length; APX = ascorbate peroxidase; CAT = catalase; POD = peroxidase; SOD = superoxide dismutase; ROS = reactive oxygen species; CK = control; SI = Solidago canadensis L. invasion; MPc = soil microplastic contamination; and SI × MP = combined Solidago canadensis L. invasion and soil microplastic contamination.
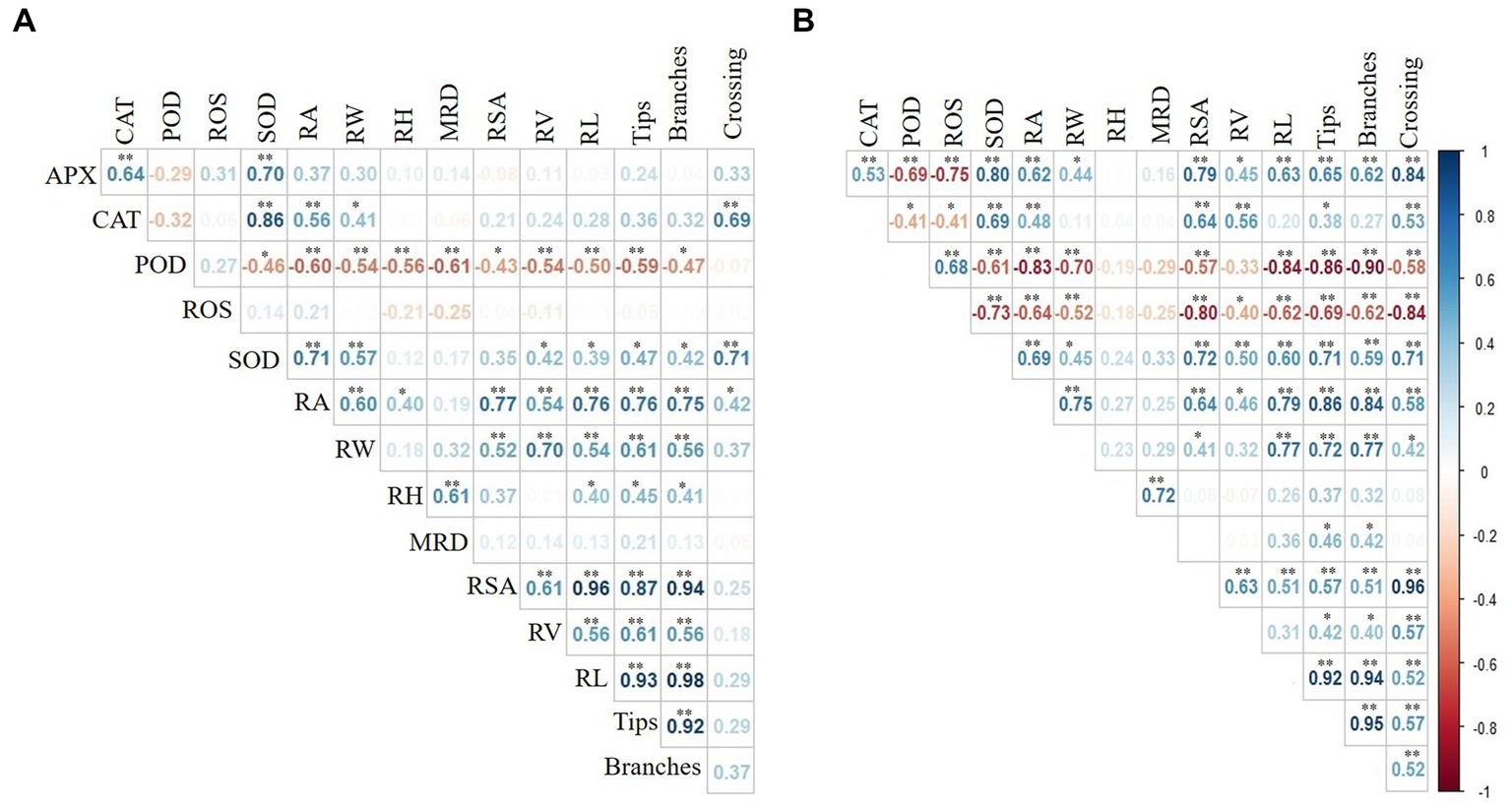
Figure 4. Pearson correlation of rice root growth traits and antioxidant enzyme activities at (A) 50 and (B) 80 DAT in the SI, MPc, and SI×MP treatments, as represented by a heatmap. RA = root area; RW = root width; RH = root height; MRD = mean root diameter; RSA = root surface area; RV = root volume; RL = root length; APX = ascorbate peroxidase; CAT = catalase; POD = peroxidase; SOD = superoxide dismutase; ROS = reactive oxygen species. “**” represents highly significant (p < 0.01) and “*” represents significant (p < 0.05) interactions among the different parameters.
4. Discussion
We investigated the individual and combined effects of Solidago canadensis L. invasion (SI) and microplastic soil contamination (MPc) on rice root growth traits. The findings indicate varying effects on rice root growth traits, including minimal and severe adverse effects. Our findings demonstrate that S. canadensis enhanced root growth traits, except for RH and MRD. The counter-impacts of the various antioxidant enzyme activities in the rice roots augmented some root growth traits, while decreasing them when combined with soil MP contamination. In general, S. canadensis invasion was less adverse to root growth traits, which is consistent with the findings of previous studies (Wang et al., 2016; Lu et al., 2020).
Our results indicate that S. canadensis invasion and MP contamination in farmland considerably affected the root growth traits. Changes in root growth traits were S. canadensis invasion- and MP-specific. They might vary depending on the competition between species for nutrients and water in the soil, which promoted the effects on crop root growth traits (i.e., root area, width, volume, and numbers of branches and tips). However, when combined with MP, the severity of pollution increased and negatively affected the crop root growth traits (Table 1). Furthermore, the crop root growth indices increased more at 80 DAT than at 50 DAT, likely owing to higher nutrient requirements for enhancing crop growth and productivity (Iqbal et al., 2020). Such differences in root growth traits in rice crops may be explained partly by the fact that S. canadensis invasion promotes the root growth traits (i.e., RA, RW, RSA, RV, RL, and the numbers of tips and branches) owing to high competition for water and nutrients (Chen et al., 2013; Mncube and Mloza Banda, 2018). In contrast, MPs are known to negatively impact root growth (Qi et al., 2018; Zang et al., 2020; Meng et al., 2021; Javed et al., 2023), which severely reduces the belowground growth of the crop. Furthermore, plastic particles may interfere with the root system and root hair progression, thereby restricting nutrient uptake (Bosker et al., 2019). MPs affect root growth and functions and may further hinder water and nutrient absorption. Direct negative impacts on root growth in plants exposed to MPs in hydroponic systems have been observed (Urbina et al., 2020).
Changes in the antioxidant enzyme activities in the presence of these adverse factors may have caused changes in the root growth traits. Unbalanced antioxidant enzyme activities in crop roots were caused by the interaction of S. canadensis invasion and MP pollution (Zhao et al., 2022) resulted in negative impacts on crop root growth traits (Figure 2). The results also indicate that the root growth traits correlated with the antioxidant enzyme activities (Figure 3). Peroxidase (POD) had a negative effect on root growth traits at 50 DAT. In comparison, both POD and reactive oxygen species (ROS) had negative impacts at 80 DAT (Figure 4). Slight decreases in photosynthetic pigments under stressful conditions is known to contribute to varying membrane permeability and oxidative stress-induced component destruction (Jain et al., 2010; Li et al., 2018; Aihemaiti et al., 2019; Zhao et al., 2022). Enzymatic antioxidants such as ascorbate peroxidase (APX), superoxide dismutase (SOD), catalase (CAT), and POD are potent antioxidants that help plants recover from environmental stress. Thus, these enzymes are considered to be crucial components of the antioxidative defense system, as they significantly reduce and restore oxidative injury caused by ROS (Jain et al., 2010; Zhang X. M. et al., 2022; Iqbal et al., 2023a). However, enzymatic activities are still considered the cause of limited root growth traits in the SI, MPc, and SI × MP treatments (Figure 3).
In general, the negative effects of crop antioxidant enzyme activities were associated with the detrimental impact of S. canadensis invasion and MP toxic contamination on the yield of crops (Zhao et al., 2022). The most substantially adverse effects occurred in the combined treatment, which exhibited the most pronounced changes in the root growth indices. In contrast, the less adverse SI treatment exhibited intermediate effects and promoted crop root growth traits (except for RH and number of root crossings). In this study, the SI treatment-induced changes in the root growth traits interacted with soil MP contamination. However, there is no appropriate way to detect the specific chemical exudates from the root system of plants in the SI treatment and their impacts on the crop when combined with MP contamination. As a result, the data obtained from the invasive plant roots in the SI treatment could have been more precise and reliable. Therefore, all parameters of the rice crop were examined, focusing on the belowground characteristics.
5. Conclusion
Rice plants were subjected to S. canadensis invasion and soil MP contamination. The combined treatment exerted the most adverse effects on crop root growth traits. In contrast, the effects of S. canadensis invasion were the least harmful. The combined impact of SI × MP toxicity was linked to changes in the root growth traits. This root growth trait evaluation offers comprehensive perspectives on the combined ecological consequences of S. canadensis and soil MP contamination. These findings can be used to scrutinize the ecological consequences of other invasive crops contaminated by MPs. However, additional research is required to clarify the interrelations between the root growth traits, antioxidant enzyme activities, and crop biomass using long-term field experiments. In addition, the potential integration of MP contamination in the food chain and the resulting impacts on food safety and health consequences should be explored.
Data availability statement
The raw data supporting the conclusions of this article will be made available by the authors, without undue reservation.
Author contributions
GL, BI, JL, and DD: conceptualization. XiaZ and JL: data curation and formal analysis. GL, XiaZ, XinZ, and DD: funding acquisition. XiaZ, XinZ, and QJ: investigation. GL and JL: methodology. GL, XinZ, JL, and DD: supervision. XiaZ and BI: visualization, roles, and writing-original draft. XinZ, JL, and QJ: writing-review and editing. All authors contributed to the article and approved the submitted version.
Funding
This work was supported by the Open Project of Key Laboratory of Environmental Biotechnology, CAS (Grant No kf2020006), the Opening Foundation of Ministry of Education Key Laboratory of Pollution Processes and Environmental Criteria (2021a02); the Carbon Peak and Carbon Neutrality Technology Innovation Foundation of Jiangsu Province (BK20220030), the National Natural Science Foundation of China (32271587; 31800342), the Basic Science Research Program through the National Research Foundation of Korea (NRF) funded by the Ministry of Education (NRF-2022R1I1A1A01055443), the Yantai City school-land integration development project (2022YB14), the Talent Project from the “Double-Entrepreneurial Plan” in Jiangsu Province, the Postgraduate Research and Practice Innovation Program of Jiangsu Province (SJCX21_1708), and the Senior Talent Foundation of Jiangsu University (18JDG039).
Acknowledgments
This research was supported by Institute of Construction and Environmental Engineering at Seoul National University. The authors wish to express their gratitude for the support.
Conflict of interest
The authors declare that the research was conducted in the absence of any commercial or financial relationships that could be construed as a potential conflict of interest.
Publisher’s note
All claims expressed in this article are solely those of the authors and do not necessarily represent those of their affiliated organizations, or those of the publisher, the editors and the reviewers. Any product that may be evaluated in this article, or claim that may be made by its manufacturer, is not guaranteed or endorsed by the publisher.
Supplementary material
The Supplementary material for this article can be found online at: https://www.frontiersin.org/articles/10.3389/fevo.2023.1172093/full#supplementary-material
References
Agathokleous, E., Kitao, M., Harayama, H., and Calabrese, E. J. (2019). Temperature-induced hormesis in plants. J. For. Res. 30, 13–20. doi: 10.1007/s11676-018-0790-7
Aihemaiti, A., Jiang, J., and Blaney, L. (2019). The detoxification effect of liquid digestate on vanadium toxicity to seed germination and seedling growth of dog’s tail grass. J. Hazard. Mater. 369, 456–464. doi: 10.1016/j.jhazmat.2019.01.091
Bosker, T., Bouwman, L. J., Brun, N. R., Behrens, P., and Vijver, M. G. (2019). Microplastics accumulate on pores in seed capsule and delay germination and root growth of the terrestrial vascular plant Lepidium sativum. Chemosphere 226, 774–781. doi: 10.1016/j.chemosphere.2019.03.163
Chen, G., Li, Y., Liu, S., Junaid, M., and Wang, J. (2022). Effects of micro(nano)plastics on higher plants and the rhizosphere environment. Sci. Total Environ. 807:150841. doi: 10.1016/j.scitotenv.2021.150841
Chen, G. Q., Zhang, C. B., Ling, M. A., Sheng, Q., Silander, J. A., and Li, L. Q. (2013). Biotic homogenization caused by the invasion of Solidago canadensis in China. J. Integr. Agric. 12, 835–845. doi: 10.1016/S2095-3119(13)60302-0
De Souza Machado, A. A. S., Kloas, W., Zarfl, C., Hempel, S., and Rillig, M. C. (2018). Microplastics as an emerging threat to terrestrial ecosystems. Glob. Chang. Biol. 24, 1405–1416. doi: 10.1111/gcb.14020
Dong, Y. M., Gao, M. L., Song, Z. G., and Qiu, W. W. (2020). Microplastic particles increase arsenic toxicity to rice seedlings. Environ. Pollut. 259:113892. doi: 10.1016/j.envpol.2019.113892
Du, C., Liang, H., Li, Z., and Gong, J. (2020). Pollution characteristics of microplastics in soils in southeastern suburbs of Baoding city, China. Int. J. Environ. Res. Public Health 17:845. doi: 10.3390/ijerph17030845
Gao, M., Liu, Y., and Song, Z. (2019). Effects of polyethylene microplastic on the phytotoxicity of di-n-butyl phthalate in lettuce (Lactuca sativa L. var. ramosa Hort). Chemosphere 237:124482. doi: 10.1016/j.chemosphere.2019.124482
Gao, X. H., Xie, D., and Yang, C. (2021). Effects of a PLA/PBAT biodegradable film mulch as a re-placement of polyethylene film and their residues on crop and soil environment. Agric. Water Manag. 255:107053. doi: 10.1016/j.agwat.2021.107053
Geyer, R., Jambeck, J. R., and Law, K. L. (2017). Production, use, and fate of all plastics ever made. Sci. Adv. 3:e1700782. doi: 10.1126/sciadv.1700782
Iqbal, B., Khan, I., Alabbosh, K. F., Javed, Q., Inamullah, I., Zhiguo, Z., et al. (2023b). The high phosphorus incorporation promotes the soil enzymatic activity, nutritional status, and biomass of the crop. Polish J. Environ. Stud. 32:158765. doi: 10.15244/pjoes/158765
Iqbal, B., Kong, F., Ullah, I., Ali, S., Li, H., Wang, J., et al. (2020). Phosphorus application improves the cotton yield by enhancing reproductive organ biomass and nutrient accumulation in two cotton cultivars with different phosphorus sensitivity. Agronomy 10:153. doi: 10.3390/agronomy10020153
Iqbal, B., Zhao, T. T., Yin, W. Q., Zhao, X., Xie, Q. J., Khan, K. Y., et al. (2023a). Impacts of soil microplastics on crops: A review. Appl. Soil Ecol. 181:104680. doi: 10.1016/j.apsoil.2022.104680
Jain, R., Srivastava, S., Solomon, S., Shrivastava, A., and Chandra, A. (2010). Impact of excess zinc on growth parameters, cell division, nutrient accumulation, photosynthetic pigments and oxidative stress of sugarcane (Saccharum spp.). Acta Physiol. Plant. 32, 979–986. doi: 10.1007/s11738-010-0487-9
Javed, Q., Sun, J., Rutherford, S., Li, J., Iqbal, B., Xiang, Y., et al. (2023). Soil pollution and the invasion of congener Sphagneticola in crop lands. J. Environ. Manag. 340:118013. doi: 10.1016/j.jenvman.2023.118013
Khalid, N., Aqueel, M., and Noman, A. (2020). Microplastics could be a threat to plants in terrestrial systems directly or indirectly. Environ. Pollut. 267:115653. doi: 10.1016/j.envpol.2020.115653
Kumar, M., Xiong, X., He, M., Tsang, D. C., Gupta, J., Khan, E., et al. (2020). Microplastics as pollutants in agricultural soils. Environ. Pollut. 265:114980. doi: 10.1016/j.envpol.2020.114980
Li, B., Huang, S., Wang, H., Liu, M., Xue, S., Tang, D., et al. (2021). Effects of plastic particles on germination and growth of soybean (Glycine max): a pot experiment under field condition. Environ. Pollut. 272:116418. doi: 10.1016/j.envpol.2020.116418
Li, S., Wang, T., Guo, J., Dong, Y., Wang, Z., Gong, L., et al. (2021). Polystyrene microplastics disturb the redox homeostasis, carbohydrate metabolism and phytohormone regulatory network in barley. J. Hazard. Mater. 415:125614. doi: 10.1016/j.jhazmat.2021.125614
Li, G., Kim, S., Han, S. H., Chang, H., Du, D., Son, Y., et al. (2018). Precipitation affects soil microbial and extracellular enzymatic responses to warming. Soil Biol. Biochem. 120, 212–221.
Lian, J., Liu, W., Meng, L., Wu, J., Chao, L., Zeb, A., et al. (2021a). Foliar-applied polystyrene nanoplastics (PSNPs) reduce the growth and nutritional quality of lettuce (Lactuca sativa L.). Environ. Pollut. 280:116978. doi: 10.1016/j.envpol.2021.116978
Lian, J., Liu, W., Meng, L., Wu, J., Zeb, A., Cheng, L., et al. (2021b). Effects of microplastics derived from polymer-coated fertilizer on maize growth, rhizosphere, and soil properties. J. Clean. Prod. 318:128571. doi: 10.1016/j.jclepro.2021.128571
Lian, J., Wu, J., Xiong, H., Zeb, A., Yang, T., Su, X., et al. (2020a). Impact of polystyrene nanoplastics (PSNPs) on seed germination and seedling growth of wheat (Triticum aestivum L.). J. Hazard. Mater. 385:121620. doi: 10.1016/j.jhazmat.2019.121620
Lian, J., Wu, J., Zeb, A., Zheng, S., Ma, T., Peng, F., et al. (2020b). Do polystyrene nanoplastics affect the toxicity of cadmium to wheat (Triticum aestivum L.)? Environ. Pollut. 263:114498. doi: 10.1016/j.envpol.2020.114498
Lozano, Y. M., Lehnert, T., Linck, L. T., Lehmann, A., and Rillig, M. C. (2021). Microplastic shape, polymer type, and concentration affect soil properties and plant biomass. Front. Plant Sci. 12:616645. doi: 10.3389/fpls.2021.616645
Lu, Y., Wang, Y., Wu, B., Wang, S., Wei, M., Du, D., et al. (2020). Allelopathy of three compositae invasive alien species on indigenous Lactuca sativa L. enhanced under cu and Pb pollution. Sci. Hortic. 267:109323. doi: 10.1016/j.scienta.2020.109323
Lu, J. Z., Weng, E. S., Wu, X. W., Weber, E., Zhao, B., and Li, B. (2007). Potential distribution of Solidago canadensis in China. Acta Phytotaxon. Sin. 45, 670–674. doi: 10.1360/aps06200
Meng, F., Yang, X., Riksen, M., Xu, M., and Geissen, V. (2021). Response of common bean (Phaseolus vulgaris L.) growth to soil contaminated with microplastics. Sci. Total Environ. 755:142516. doi: 10.1016/j.scitotenv.2020.142516
Mncube, T. L., and Mloza Banda, H. R. (2018). Evaluation of chemical and non-chemical weed control practices on weed communities and maize yield in two agroecological zones of Swaziland. Afr. J. Agric. Res. 13, 1708–1718. doi: 10.5897/AJAR2018.13311
Ng, E. L., Lwanga, E. H., Eldridge, S. M., Johnston, P., Hu, H. W., Geissen, V., et al. (2018). An overview of microplastic and nanoplastic pollution in agroecosystems. Sci. Total Environ. 627, 1377–1388. doi: 10.1016/j.scitotenv.2018.01.341
Phuong, N. N., Zalouk-Vergnoux, A., Poirier, L., Kamari, A., Châtel, A., Mouneyrac, C., et al. (2016). Is there any consistency between the microplastics found in the field and those used in laboratory experiments? Environ. Pollut. 211, 111–123. doi: 10.1016/j.envpol.2015.12.035
Pignattelli, S., Broccoli, A., and Renzi, M. (2020). Physiological responses of garden cress (L. sativum) to different types of microplastics. Sci. Total Environ. 727:138609. doi: 10.1016/j.scitotenv.2020.138609
Plastics Europe. (2020). Plastics–the facts 2020. An analysis of European plastics production, demand and waste data. Available at: https://www.plasticseurope.org/en/resources/publications/4312-plastics-facts-2020_versionJun21_final.pdf.
Powell, K. I., Chase, J. M., and Knight, T. M. (2013). Invasive plants have scale-dependent effects on diversity by altering species-area relationships. Science 339, 316–318. doi: 10.1126/science.1226817
Qi, Y. L., Yang, X., Pelaez, A. M., Huerta Lwanga, E., Beriot, N., Gertsen, H., et al. (2018). Macro- and micro- plastics in soil-plant system: effects of plastic mulch film residues on wheat (Triticum aestivum L.) growth. Sci. Total Environ. 645, 1048–1056. doi: 10.1016/j.scitotenv.2018.07.229
R Core Team. (2013). R: A language and environment for statistical computing, 4.1.1. R foundation for statistical computing. Vienna, Austria: R Core Team. Available at: http://www.R-project.org/.
Rezania, S., Park, J., Md Din, M. F., Taib, S. M., Talaiekhozani, A., Yadav, K. K., et al. (2018). Microplastics pollution in different aquatic environments and biota: a review of recent studies. Mar. Pollut. Bull. 133, 191–208. doi: 10.1016/j.marpolbul.2018.05.022
Su, L., Du, H., Wang, H., Zeng, F. P., Song, T. Q., Peng, W. X., et al. (2018). Root architecture of the dominant species in various vegetation restoration processes in karst peak-cluster depression. Acta Bot. Boroccid. Sin. 38, 150–157. doi: 10.7606/j.issn.1000-4025.2018.01.0150
Sun, Z. K., and He, W. M. (2010). Evidence for enhanced mutualism hypothesis: Solidago canadensis plants from regular soils perform better. PLoS One 5:e15418. doi: 10.1371/journal.pone.0015418
Sun, X. D., Yuan, X. Z., Jia, Y., Feng, L. J., Zhu, F. P., Dong, S. S., et al. (2020). Differentially charged nanoplastics demonstrate distinct accumulation in Arabidopsis thaliana. Nat. Nanotechnol. 15, 755–760. doi: 10.1038/s41565-020-0707-4
Urbina, M. A., Correa, F., Aburto, F., and Ferrio, J. P. (2020). Adsorption of polyethylene microbeads and physiological effects on hydroponic maize. Sci. Total Environ. 741:140216. doi: 10.1016/j.scitotenv.2020.140216
Wang, W., Ge, J., Yu, X., and Li, H. (2020). Environmental fate and impacts of microplastics in soil ecosystems: progress and perspective. Sci. Total Environ. 708:134841. doi: 10.1016/j.scitotenv.2019.134841
Wang, C. Y., Jiang, K., Liu, J., Zhou, J. W., and Wu, B. D. (2018b). Moderate and heavy Solidago canadensis L. invasion are associated with decreased taxonomic diversity but increased functional diversity of plant communities in East China. Ecol. Eng. 112, 55–64. doi: 10.1016/j.ecoleng.2017.12.025
Wang, C. Y., Jiang, K., Wu, B. D., and Zhou, J. W. (2018a). The combined treatments of Canada goldenrod leaf extracts and cadmium pollution confer an inhibitory effect on seed germination and seedling development of lettuce. Aust. J. Bot. 66, 331–337. doi: 10.1071/BT18046
Wang, J., Liu, X., Li, Y., Powell, T., Wang, X., and Wang, G. (2019). Microplastics as contaminants in the soil environment: a mini-review. Sci. Total Environ. 691, 848–857. doi: 10.1016/j.scitotenv.2019.07.209
Wang, C. Y., Xiao, H., Zhao, L., Liu, J., Wang, L., Zhang, F., et al. (2016). The allelopathic effects of invasive plant Solidago canadensis on seed germination and growth of Lactuca sativa enhanced by different types of acid deposition. Ecotoxicology 25, 555–562. doi: 10.1007/s10646-016-1614-1
Xia, T., Wang, Y., He, Y., Wu, C., Shen, K., Tan, Q., et al. (2020). An invasive plant experiences greater benefits of root morphology from enhancing nutrient competition associated with arbuscular mycorrhizae in karst soil than a native plant. PLoS One 15:e0234410. doi: 10.1371/journal.pone.0234410
Xu, J. J., Li, Q., and Wu, W. J. (2019). Effects of vertical phosphorus competition on root growth and biomass distribution of Chinese fir seedlings. Acta Ecol. Sin. 39, 2071–2081. doi: 10.5846/stxb201803230577
Yang, R. Y., Yu, G. D., Tang, J. J., and Chen, X. (2008). Effects of metal lead on growth and mycorrhizae of an invasive plant species (Solidago canadensis L.). J. Environ. Sci. 20, 739–744. doi: 10.1016/S1001-0742(08)62121-X
Yuan, Y. G., Wang, B., Zhang, S. S., Tang, J. J., Tu, C., Hu, S. J., et al. (2013). Enhanced allelopathy and competitive ability of invasive plant Solidago canadensis in its introduced range. J. Plant Ecol. 6, 253–263. doi: 10.1093/jpe/rts033
Yuan, W., Zhou, Y., Liu, X., and Wang, J. (2019). New perspective on the nanoplastics disrupting the reproduction of an endangered fern in artificial freshwater. Environ. Sci. Technol. 53, 12715–12724. doi: 10.1021/acs.est.9b02882
Zang, H., Zhou, J., Marshall, M., Chadwick, D., Wen, Y., and Jones, D. (2020). Microplastics in the agroecosystem: are they an emerging threat to the plant-soil system? Soil Biol. Biochem. 148:107926. doi: 10.1016/j.soilbio.2020.107926
Zhang, X. M., Cao, X. X., He, L. X., Xue, W., Gao, J. Q., Lei, N. F., et al. (2022). Soil heterogeneity in the horizontal distribution of microplastics influences productivity and species composition of plant communities. Front. Plant Sci. 13:1075007. doi: 10.3389/fpls.2022.1075007
Zhang, Y., Yang, X., Luo, Z., Lai, J., Li, C., and Luo, X. (2022). Effects of polystyrene nanoplastics (PSNPs) on the physiology and molecular metabolism of corn (Zea mays L.) seedlings. Sci. Total Environ. 806:150895. doi: 10.1016/j.scitotenv.2021.150895
Zhao, S. Y., Sun, S. G., Dai, C., Gituru, R. W., Chen, J. M., and Wang, Q. F. (2015). Genetic variation and structure in native and invasive Solidago canadensis populations. Weed Res. 55, 163–172. doi: 10.1111/wre.12130
Keywords: Solidago canadensis L., rice, soil microplastics, toxicity, root growth traits
Citation: Li G, Zhao X, Iqbal B, Zhao X, Liu J, Javed Q and Du D (2023) The effect of soil microplastics on Oryza sativa L. root growth traits under alien plant invasion. Front. Ecol. Evol. 11:1172093. doi: 10.3389/fevo.2023.1172093
Edited by:
Kaixiong Xing, Hainan Normal University, ChinaReviewed by:
Xiao Guo, Qingdao Agricultural University, ChinaIrum Raza, University of Georgia, United States
Copyright © 2023 Li, Zhao, Iqbal, Zhao, Liu, Javed and Du. This is an open-access article distributed under the terms of the Creative Commons Attribution License (CC BY). The use, distribution or reproduction in other forums is permitted, provided the original author(s) and the copyright owner(s) are credited and that the original publication in this journal is cited, in accordance with accepted academic practice. No use, distribution or reproduction is permitted which does not comply with these terms.
*Correspondence: Babar Iqbal, babar@ujs.edu.cn; Jingjing Liu, seajingjing@126.com
†These authors have contributed equally to this work and share first authorship