- 1Department of Biology, Ecology and Conservation, St. George’s University, True Blue, St. George’s, Grenada
- 2Natural Resources Institute, University of Manitoba, Winnipeg, MB, Canada
As anthropogenic noise becomes increasingly widespread in natural habitats, noise addition and removal studies have become an important and commonly used method to assess the effects of noise on wildlife. Despite their wide implementation, it is difficult to determine whether the results from these studies translate to natural environments. Oil extraction operations provide a unique opportunity to conduct large-scale natural and experimental noise studies because they emit high-amplitude noise and are subject to interruptions or cessation of operations. We conducted a 6-year study aimed at identifying the effects of different types of oil infrastructure and noise on four species of grassland songbirds in the Canadian prairies. We measured abundance and nesting success of our focal species and compared between a large-scale playback experiment (i.e., noise addition) and in response to real infrastructure (i.e., noise removal). We also conducted an in-depth species analysis of reproductive output, stress, and population structure of an endangered species, Chestnut-collared Longspur (Calcarius ornatus). This multi-species and species-specific approach enabled us to assess noise impacts that may vary among species and for different life-history traits. Overall, our comparative study suggests that noise addition experiments can result in different conclusions regarding the ecological impacts of noise generated from in situ field studies in sites with associated disturbances. While noise clearly impacts birds in many ways, when layered with the many other ecosystem changes associated with real-world oil extraction activity, the effects of noise may be less prominent. As such, caution must be taken when applying results of noise experiments to conservation and management plans and regulations, as effects of noise predicted by lab and field noise addition experiments may simply not be realized under real-world conditions.
Introduction
As anthropogenic noise becomes increasingly widespread in natural habitats (e.g., Barber et al., 2010; Shannon et al., 2016; Buxton et al., 2017), noise addition and removal studies have become an important and commonly used method to assess the effects of noise on wildlife. Noise addition studies that reproduce the anthropogenically disturbed soundscapes have identified effects on wild populations of birds (e.g., Blickley et al., 2012a; McClure et al., 2013; Cinto Mejia et al., 2019), bats (Finch et al., 2020), and whales (e.g., Goldbogen et al., 2013). Similarly, noise removal experimental setups that compare responses to real noise-producing infrastructure when it is active and inactive (e.g., Bayne et al., 2008; Francis et al., 2009; Bunkley et al., 2015) aid in disentangling observed impacts of noise from other disturbances. Both types of studies have provided a greater understanding of the mechanisms underlying the effects of noise on wildlife, such as changes in hormone levels (e.g., Kight and Swaddle, 2011; Blickley et al., 2012b) or shifts in community composition (e.g., Senzaki et al., 2020). Thus, noise addition and removal experiments have been used as a tool for assessing the consequences of anthropogenic noise in many habitats.
Despite the broad application of noise addition and removal experiments, it is difficult to determine whether the results from these studies can translate to natural environments. In noise addition studies, the playback noise may not accurately recreate the spectral or temporal characteristics of the noise stimuli or may broadcast it at higher amplitudes, leading to altered behavioral and physiological responses not indicative of responses to the actual noise source (Francis and Barber, 2013; Shannon et al., 2016). Conversely, species may have developed coping mechanisms or habituated to noise sources and their associated infrastructure (e.g., Francis and Barber, 2013; Potvin, 2017; Rosa and Koper, 2018). Hence, decoupling the actual source of the noise-producing infrastructure from the noise it produces may lead to impacts on wildlife that are not necessarily representative of natural conditions. While most noise addition studies aim to assess noise impacts on wildlife, the mitigation measures that stem from those studies may not be accounting for these discrepancies.
The increasing prevalence of noise may lead to greater impacts when compared to physical habitat disturbances (Francis et al., 2011), or conversely, species may have become habituated or employ effective coping mechanisms that enable them to minimize impacts related to human-generated noise (e.g., Warren et al., 2006; Francis and Barber, 2013; Rosa and Koper, 2018). To develop effective mitigations to aid potentially vulnerable species, such as those heavily reliant on vocal communication, it is essential to disentangle effects from noise to those related to other anthropogenic disturbances (Francis and Barber, 2013). Species may be more resilient than others, and noise addition studies may not be representative of actual noise impacts that noise removal studies could help uncover. By comparing the responses of wildlife in noise addition and removal experiments, researchers can not only better understand the effects of anthropogenic noise, but also propose more appropriate mitigative measures and use limited conservation efforts and funds more effectively.
Oil extraction operations provide a unique opportunity to conduct large-scale natural and experimental noise studies. These operations produce high levels of noise amplitude, particularly during drilling activities, which can have negative impacts on residing wildlife. Further, the oil industry’s lag as it relates to the reclamation of abandoned oil wells (e.g., Weber, 2021, 2022) and interruptions in activities during periods of low oil demand and service equipment allows researchers to test the effects of noise removal. Additionally, since noise is relatively cheap to mitigate when compared to other disturbances, such as physical habitat destruction or alterations, they have shown an interest in isolating impacts of noise from all other potential impacts when tasked with mitigating threats to migratory grassland songbirds. Hence, by grouping these two sets of circumstances, we were able to conduct a study that assessed the scale of noise impacts on migratory grassland songbirds, which is particularly important considering the increasing demand for oil globally (e.g., Cinto Mejia et al., 2019).
Here, we conducted a 6-year grassland songbird study aimed at identifying the effects of oil infrastructure and noise on grassland songbirds in the Canadian prairies. We conducted a large-scale noise addition experiment (i.e., Rosa and Koper, 2022), in which the anthropogenic soundscape is recreated without other associated disturbances (e.g., physical infrastructure, roads, pollutants), and a noise removal experiment with real oil infrastructure. We predicted that the effects of noise would be similar between the two types of experiments, while the impacts associated with the physical infrastructure would differ. We focused on comparing abundance and nesting success of four grassland songbird species declining in North America (Sauer et al., 2015), including two listed species: Chestnut-collared Longspur (Calcarius ornatus; Endangered) and Sprague’s Pipit (Anthus spragueii; Threatened), and two unlisted species: Savannah Sparrow (Passerculus sandwichensis) and Vesper Sparrow (Pooecetes gramineus). Additionally, we conducted an in-depth species analysis of Chestnut-collared Longspur that focused on comparing the impacts of noise and associated infrastructure on reproductive output, stress, and population structure. Since noise impacts can vary among species and for different life-history traits, we provide a multi-species and species-specific perspective to better our understanding of the extent of oil extraction noise on migratory grassland songbirds.
Materials and methods
Study area and focal species
From 2012 to 2018, we conducted noise addition and removal studies during migratory grassland bird breeding season (i.e., May to August) near Brooks, County of Newell, Alberta (50°33′51″N, 111°53′56″W), an area with a high concentration of oil activity (Figure 1). Sites spanned 64.7-ha (i.e., quarter section, 800 × 800 m) and consisted of mixed-grass prairies. Predominant grass were native species such as needle and thread (Hesperostipa comata), Junegrass (Koeleria macrantha), and western wheatgrass (Pascopyrum smithii; Nenninger and Koper, 2018). We chose to study Chestnut-collared Longspur, Savannah Sparrow, Sprague’s Pipit, and Vesper Sparrow because they were common enough for us to evaluate the effects of noise on abundance and nesting success, and represented a variety of habitat preferences, from short to tall grassland vegetation. These species also have different vocalizations, which may result in different responses to drilling and industrial noise. Additionally, we conducted an in-depth synthesis of the effects on Chestnut-collared Longspur as their status has recently changed from Threatened to Endangered (Committee on the Status of Endangered Wildlife in Canada (COSEWIC), 2019).
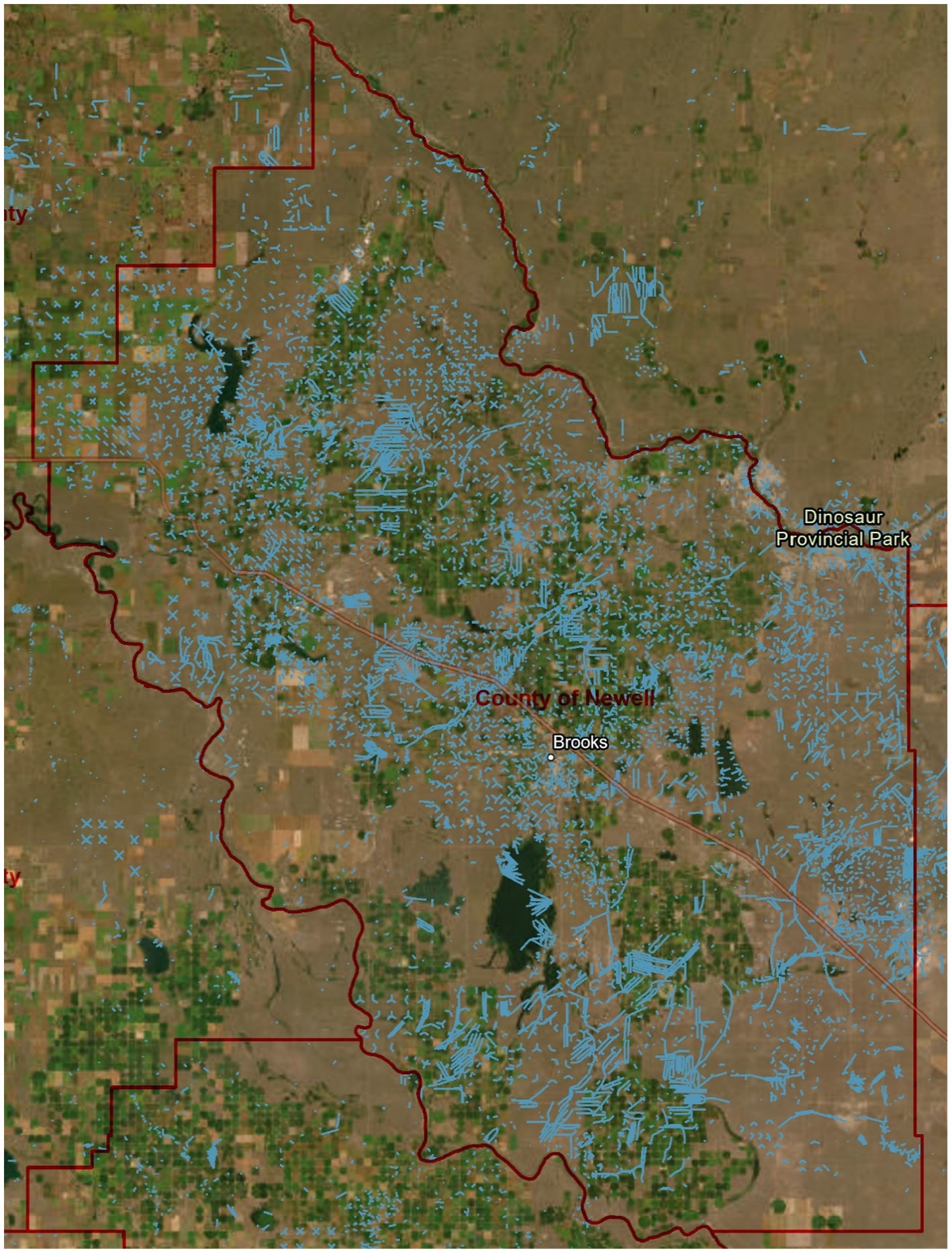
Figure 1. Map of general study area located within the County of Newell, and more specifically, areas surrounding Brooks, Alberta, Canada (scale 1:500,000). Blue lines show oil and gas wells [as of January 18, 2023 (Alberta Energy Regulator (AER), 2023)]. Government of Alberta, Esri Canada, Esri, HERE, Garmin, SafeGraph, FAO, METI/NASA, USGS, EPA, NRCAN, Parks Canada.
Study design
For the noise addition experiment, we selected sites without any oil infrastructure or activity. Instead, we broadcast high-fidelity recordings throughout the duration of the breeding seasons using a solar-powered playback system (Rosa et al., 2015; Rosa and Koper, 2022). Broadcasting systems were installed at the center of the site, except controls that contained no infrastructure. We used a Brüel and Kjær 2,250 SPL meter/frequency analyzer to measure sound pressure levels along transects in four cardinal directions at all study site types (see Rosa et al., 2015), opting for LCeq measurements of broadband sound due to its flatter frequency filter when compared to A-weighting to reduce bias towards human hearing frequencies (Pater et al., 2009). Noise addition consisted of drilling playbacks (88 ± 4.0 dB(C); n = 6), generator-powered pumpjack playbacks (73 ± 5.2 dB(C); n = 6), power-grid pumpjack playbacks (62 ± 2.8 dB(C); n = 6), infrastructure-only playbacks that mimicked the noise-producing ones, but did broadcast sound (n = 6), and controls (n = 17), with no infrastructure (i.e., playbacks or real oil wells; Rosa and Koper, 2022). During the approximately 90-day breeding season, pumpjack operating noise was broadcasted 24 h a day, while drilling noise was played back 24 h a day during two distinct 10-day periods, with at least 15 days between them (Rosa and Koper, 2022). The start of the drilling playback periods was staggered by 3 days among different replicates to distinguish the effects of date and drilling noise (Rosa and Koper, 2022). For the noise removal/real oil infrastructure experiment, we identified sites that had minimal additional anthropogenic infrastructure (e.g., paved roads, buildings) or topographical features that could affect birds (e.g., wetlands, trees, hills; Nenninger and Koper, 2018). Active, noise-producing wells were either powered by generators (73 ± 5.2 dB(C); n = 17) or were connected to the power-grid (quieter, 62 ± 2.8 dB(C); n = 9), while inactive wells (n = 21) were silent and were not actively frequented by industry personnel (Nenninger and Koper, 2018) and control sites had no infrastructure (n = 25). It is important to note that we designed this experimental design using methods and data from previously published papers and theses. We carefully structured our study to integrate and analyze these data sources, allowing us to generate new insights into the overarching impact of noise on our focal species.
Field surveys
Abundance and nesting searching of four grassland songbird species
During the breeding seasons from 2012 to 2015, we conducted two surveys on each transect. The surveys took place between mid-May and early July, in conditions of winds no greater than 20 km/h, no precipitation, and little to no fog, from dawn to 1,000 h (Nenninger and Koper, 2018). Each survey was conducted by two observers who sampled half of the transect each, with each transect running 400-m from the infrastructure or center-point at control sites. The observers recorded all birds that they saw and heard along the center line of the transect during a period of 40 min (Leston et al., 2015).
We estimated the location of birds using the estimated distance and bearing of the bird from the observer along the transect line (Nenninger and Koper, 2018). We used the rope-dragging method (Winter et al., 2003) to search for nests from late May to late July of each breeding season from 2012 to 2015. We conducted two (Rosa and Koper, 2022) or three rounds (Bernath-Plaisted and Koper, 2016) of nest searches at each site starting between 0630 and 0800 h and completing before 1,200 h (Bernath-Plaisted and Koper, 2016). In 2016 and 2017, we used adult behavioral cues (i.e., singing, carrying nesting material or food, territorial displays) to locate nests at our study sites (e.g., Heathcote, 2019). To determine the nest outcome, we monitored nests every 2–4 days (Winter et al., 2003) and used indices of predation, including indicators of predation incidents, for instance, an empty, damaged nest found during the early nest stage, while concurrently observing nestling development for around 10 days post-hatching (Ehrlich et al., 1988). These predation indices offered a dependable method for estimating nest outcomes, where a successful nest was defined as one in which a minimum of one juvenile fledged (Jones and Geupel, 2007).
Reproductive output, corticosterone, and age and size of Chestnut-collared Longspurs
For clutch size, we used the final number of eggs laid by the female or the number of nestlings as an estimate for nests found after hatching (Rosa and Koper, 2022). To determine nestling sex-ratio, we used blood samples to extract DNA from nestlings using methods developed by Ivanova et al. (2006) and PCR amplification of chromo-helicase-DNA (CHD1) binding genes from avian W and Z sex chromosomes (Griffiths et al., 1998; Cyr, 2021). To estimate fledgling survival, we radio-tagged (PicoPicAG339; Lotek Wireless Inc., New Market Ontario) and analyzed data from 138 8-day-old nestlings (Jonsomjit et al., 2007) from 83 nests from May to July in 2017 and 2018 (Carey, 2021). The location of tagged nestlings was tracked daily using receivers from Communications Specialist Inc. and Advanced Telemetry Systems Inc. until the battery of the radio tag ran out, or the individual died or went missing (see Carey, 2021). For corticosterone levels, we collected blood samples from females and males captured using mist nets (Des Brisay et al., 2022) and 7-day-old nestlings (Heathcote, 2019) within 3-min of capture (Romero and Reed, 2005) for baseline levels of corticosterone (i.e., basal CORT) and another sample after 12-min following a capture-restraint protocol to determine stress levels of corticosterone (i.e., stress CORT; Wingfield et al., 1992; Lynn and Wingfield, 2003, see Heathcote, 2019; Des Brisay et al., 2022). For adults, we captured birds to determine age [i.e., Second Year (SY) or After Second Year (ASY; Pyle et al., 2008)], sex, mass, and tarsus length (Des Brisay et al., 2022). For nestlings, we estimated age based on hatch date or using visual characteristics (Jonsomjit et al., 2007) and measured weight, tarsus length, and the length of the outer primary feather of 6–8 day old nestlings (see Heathcote, 2019; Des Brisay et al., 2022).
Statistical analyses
For abundance, we used unadjusted avian point-counts (Leston et al., 2015; Koper et al., 2016) and ran generalized linear mixed models (Bolker et al., 2009) to estimate the relative abundance of each focal species using the lme4 package (Bates et al., 2014) in Program R (R Core Team, 2014) and PROC GLIMMIX in SAS (SAS Institute, Inc., 2012; see Nenninger and Koper, 2018; Rosa and Koper, 2022). For nesting success, we used logistic-exposure models (Shaffer, 2004) using the lme4 package (Bates et al., 2014) in Program R (R Core Team, 2014) and PROC NLMIXED in SAS (SAS Institute, Inc., 2012; see Bernath-Plaisted and Koper, 2016; Des Brisay et al., 2022; Rosa and Koper, 2022). For detailed species analysis of Chestnut-collared Longspur, we used generalized linear models and mixed models [e.g., package lmerTest (Kuznetsova et al., 2017) in Program R (R Core Team, 2019)] to measure reproductive output, corticosterone response, and differences in age and sex among the treatments (see Heathcote, 2019; Ng et al., 2019; Carey, 2021; Cyr, 2021; Des Brisay et al., 2022; Rosa and Koper, 2022). For each species, we compared the impacts of noise and infrastructure on abundance and nesting success, and determined whether the effects were larger closer to the source of disturbance or generalized across the entire site (e.g., Rosa and Koper, 2022). From a management perspective, distance-dependent effects of noise would imply that reducing noise amplitude at the source would benefit species negatively impacted by noise, while effects occurring across the site, the scale at which management occurs in our study area, would indicate that effects are related to the presence of noise, and even the type of noise produced.
Results
Abundance and nesting searching of four grassland songbird species
In the noise addition experiment, all 3 of the 4 focal species were affected by playback noise (Figure 2A). In the noise addition experiment, drilling noise (loudest playback) led to decreased abundance of Chestnut-collared Longspurs (distance) and Savannah Sparrows (distance and quarter-section), and reduced nesting success of Savannah Sparrows (quarter-section) and Sprague’s Pipits (quarter-section; Figure 2A). We found no impacts of chronic generator oil well noise on abundance or nesting success of our focal species, however, Chestnut-collared Longspurs experienced decreased nesting success in the chronic power-grid oil well noise (quieter) treatment (distance; Figure 2A). The presence of the playback unit had a negative impact on the abundance of Sprague’s Pipits (distance) and nesting success of Vesper Sparrows (quarter-section; Figure 2A). However, Vesper Sparrows were more abundant in the presence of silent playback units (distance and quarter-section; Figure 2A).
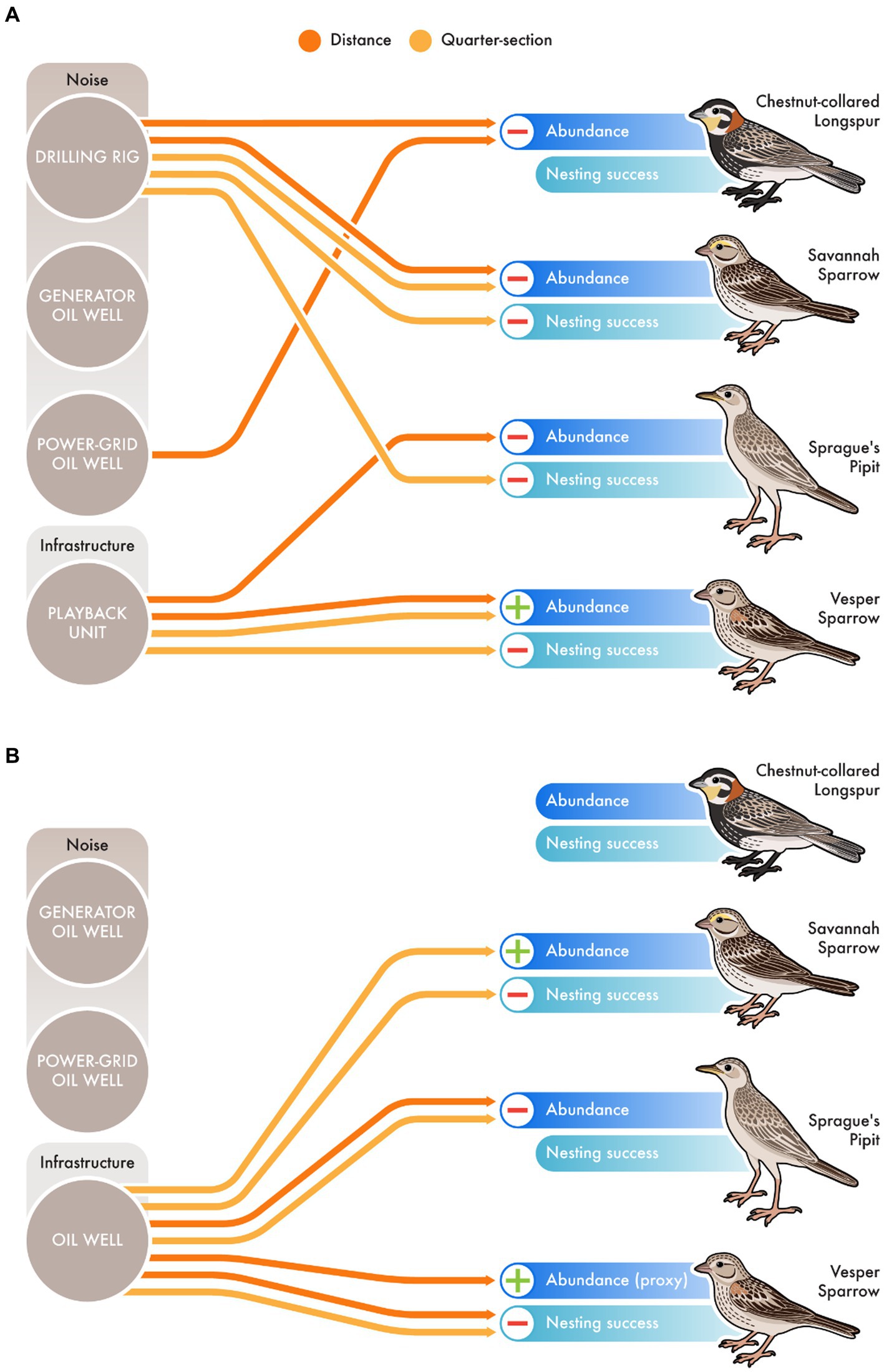
Figure 2. Comparison of effects on four species of grassland songbirds in a (A) noise addition and (B) noise removal/real oil wells experiment. For Vesper Sparrow (Pooecetes gramineus) abundance in B, nest density was used as a proxy for abundance. © 2023 Linden Pederson, St. George’s University CC-BY-ND.
In the noise removal experiment, only effects of infrastructure were detected, and for 3 of the 4 focal species (Figure 2B). Savannah Sparrows and Vesper Sparrows had greater abundances in responses to oil well infrastructure (quarter-section and distance respectively), while they both experienced reduced nesting success in presence of the physical infrastructure (Figure 2B). Oil well infrastructure negatively impacted abundance of Sprague’s Pipits (distance and quarter-section), while no effects were detected for Chestnut-collared Longspurs (Figure 2B). See Supplementary Tables S1, S2 for additional details.
Reproductive output, corticosterone, and age and size of Chestnut-collared Longspurs
For the detailed comparison of impacts on Chestnut-collared Longspurs of noise playback and real oil wells, we found similar effects between the two experiments (Figure 3). In both experiments, generator oil well noise resulted in lower basal and stress corticosterone levels in nestlings, and older and heavier females (Figure 3). Also, the presence of physical infrastructure [i.e., playback unit (Figure 3A) and oil well (Figure 3B)] skewed nestling sex-ratio in favor of females (i.e., fewer males). However, in the presence of chronic oil generator noise, females had greater basal corticosterone levels closer to the source of the playback noise (Figure 3A) and lower basal corticosterone levels closer to the real oil well noise (Figure 3B) Additionally, oil generator noise did lead to a skewed nestling sex-ratio in favor of females (i.e., fewer males) in response to real oil well noise (Figure 3B), while that effect was not detected in the playback study (Figure 3A). Acute drilling rig noise led to increased clutch size (distance and quarter-section) in the noise addition experiment (Figure 3A), however, we do not have a similar treatment with real infrastructure. See Supplementary Tables S3, S4 for additional details.
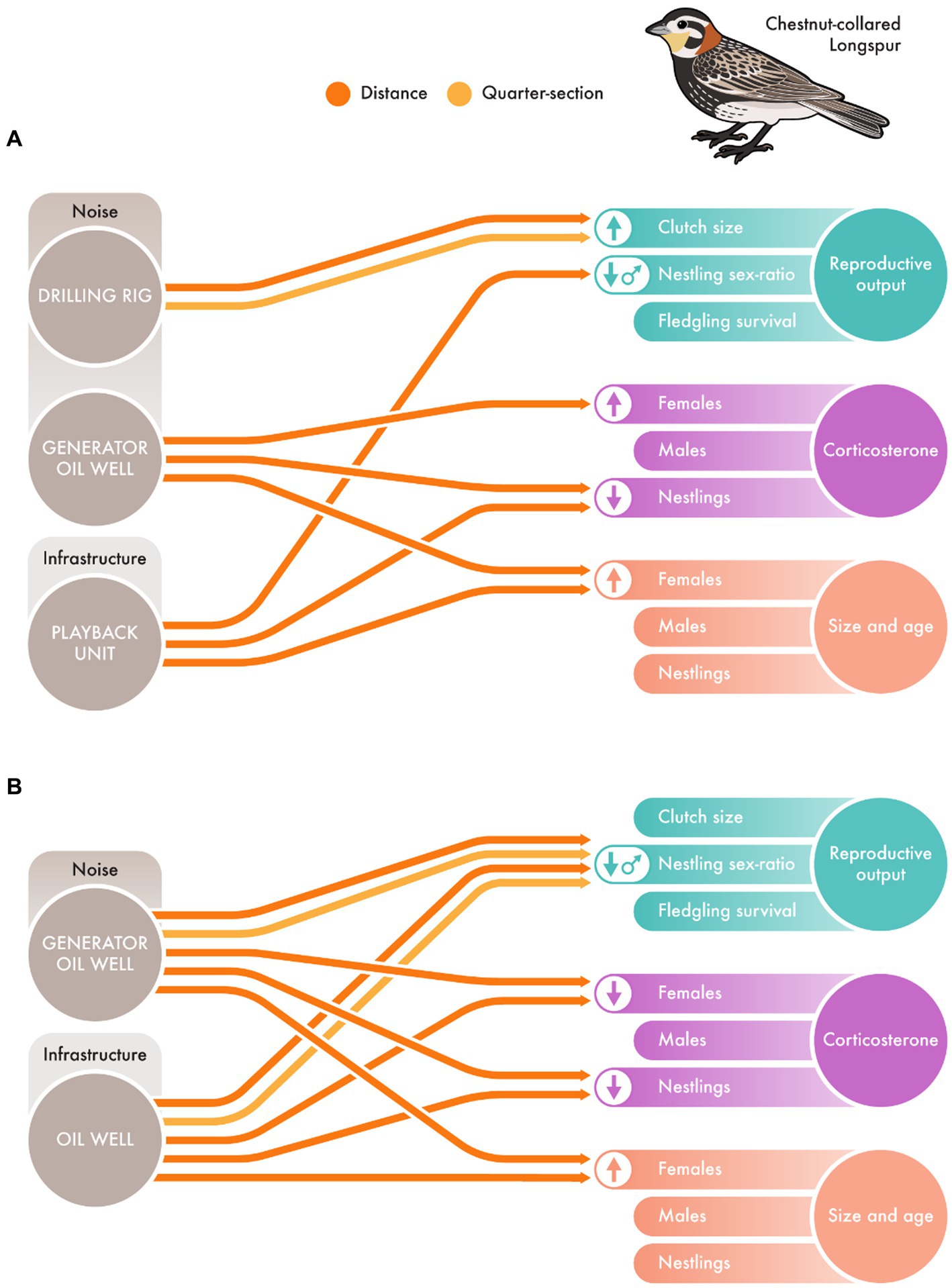
Figure 3. Comparison of effects on reproductive output, stress hormone (i.e., basal corticosterone for adults females and males, and basal and stress corticosterone for nestlings), and physiology (i.e., size and age) on Chestnut-collared Longspur (Calcarius ornatus) in a (A) noise addition experiment (i.e., landscape playback) and (B) noise removal/real oil wells experiment. © 2023 Linden Pederson, St. George’s University CC-BY-ND.
Discussion
Overall, our comparative study suggests that manipulative noise addition experiments can result in different conclusions regarding the ecological impacts of noise generated from in situ field studies in sites with associated disturbances. For example, we found no impacts of power-grid well noise on the abundance of Chestnut-collared Longspurs in our noise removal experiment (Figure 2B), despite detecting impacts in the noise addition experiment (Figure 2A). Additionally, impacts of generator-powered oil well noise on corticosterone levels of female Chestnut-collared Longspurs differed between noise addition and in situ oil well infrastructure studies (Figures 3A,B). This demonstrates the power of noise addition experiments in helping us understand the effects of noise per se on birds. However, it also demonstrates that impacts of noise on wildlife interact with real-world, cumulative effects of anthropogenic infrastructure and activities in complex ways such that impacts of noise may not carry over to individual or population-level declines. While noise clearly impacts birds in many ways, when layered with the many other ecosystem changes associated with real-world oil extraction activity, such as changes in vegetation structure, resource availability, display sites, human disturbance, and predator distributions, the effects of noise may be overwhelmed. This suggests that we must be cautious in applying results of noise experiments to conservation and management plans and regulations, as effects of noise predicted by lab and field noise-addition experiments may simply not be realized under real-world conditions.
Nonetheless, we note some consistent conclusions among experiments regarding the impacts of noise on grassland birds. First, when evaluating the effects of chronic well noise (not drilling), it is clear from both noise addition and noise removal experiments that the physical presence of above-ground infrastructure has a substantially greater impact on abundance and nesting success than noise (Figure 2). Impacts of the presence of infrastructure, independent of noise, on abundance varied among species, such that Sprague’s Pipits consistently responded negatively to the presence of infrastructure, while Vesper Sparrows and Savannah Sparrows had higher abundances near infrastructure. However, the presence of infrastructure consistently negatively impacted nesting success, such that Vesper Sparrows were attracted to all types of infrastructure but had lower nesting success in these sites, suggesting that anthropogenic infrastructure in these prairie landscapes may function as ecological traps for this species, perhaps contributing to its negative population trends (Atuo et al., 2018).
Our conclusions about the impacts of chronic well operating noise also differ between our multi-species, community-based approach (e.g., Bernath-Plaisted and Koper, 2016; Nenninger and Koper, 2018; Rosa and Koper, 2022), compared with the species-specific population analysis (e.g., Heathcote, 2019; Ng et al., 2019; Cyr, 2021). We found several characteristics related to health and long-term fitness that both noise playback and natural experiments suggest are impacted by noise (e.g., Bernath-Plaisted and Koper, 2016; Des Brisay et al., 2022). Well operating noise (generator oil well; Figure 3) impacted corticosterone and the distribution of birds across the landscape. Surprisingly, in both types of experiment, noise from generator-powered oil wells was associated with decreased corticosterone levels in nestlings [positively correlated with nestling mass in this system (Heathcote, 2019; cf. Kleist et al., 2018)], as well as larger or heavier adult females. All of these characteristics are associated with outcomes we generally interpret as positive, such as longer lives and higher reproductive output (Kleist et al., 2018; Des Brisay et al., 2022), counterintuitively suggesting that generator oil well noise may positively impact individual health, or may attract better-quality, more dominant adults (e.g., Fretwell and Lucas, 1969). We speculate that consistent, chronic noise might mask acoustic cues from predators (e.g., Rosa and Koper, 2018), leading to the erroneous interpretation by birds that these areas are relatively safe; this could attract dominant females and result in lower corticosterone levels in nestlings that divert energy to rapid growth instead (Chin et al., 2009). However, our study was not designed to identify the reason for these patterns, and more research must be conducted to understand the mechanisms behind these patterns. Noise also affected corticosterone levels of adult females, but the direction of the effect differed between experiments. Effects of noise interact with other environmental cues and challenges to result in complex and sometimes unpredictable impacts on avian health, including hormone levels. Nestling sex ratio was surprisingly strongly impacted by both noise and the presence of infrastructure, which were correlated with the production of fewer male nestlings per brood (Figure 3; see Cyr, 2021). Again, the reason for this is unknown (i.e., Hasselquist and Kempenaers, 2002); reproductive output of males tends to be more variable than females (Griffith et al., 2002) and male-biased broods may be more costly to produce (Westneat et al., 2002), so perhaps there is a selection pressure for producing more females in riskier environments. Cumulatively, these results suggest that noise per se has a range of effects on health, productive potential, and distribution of Chestnut-collared Longspurs across the landscape, and thus likely long-term fitness. Although an in-depth analysis of all focal species would have been ideal, our study does illustrate the importance of measuring the impacts of noise on health and fitness of individual birds as well as assessing its effects on wildlife communities, despite the challenges of doing so.
We note that it seems likely that there are substantially stronger negative impacts of oil well drilling noise on grassland birds compared with the effects of operating noise. We were unable to safely monitor active and inactive drilling rig operations, and thus, must depend on noise playback experiments to understand the effects of drilling noise on our focal species. However, we had evidence of impacts of well drilling noise on numerous species; drilling noise negatively impacted abundance, nesting success, or both, of three of our four focal species. Taken together with other research that has shown negative impacts of well drilling noise on wildlife, shown both through experimental playbacks (e.g., Blickley et al., 2012a) and in situ well drilling activity on wildlife (e.g., Northrup et al., 2021), we conclude that ecological impacts of well drilling, and its associated noise are probably significant, albeit locally temporary. Larger clutch sizes in drilling noise playback conditions could indicate that nesting birds are less energetically stressed (Stearns, 1976), and previous studies have reported smaller clutch sizes in the presence of urban and traffic noise when compared to quieter environments (Halfwerk et al., 2011; Des Huet Aunay et al., 2017). Various hypotheses suggest that variations in the trade-offs within and between species, particularly in the investment in offspring number, are often due to selective pressures arising from changes in environmental factors, availability of resources, the quality of the parents, and offspring mortality (e.g., Martin, 1987; Smith and Moore, 2003; Dillon and Conway, 2018). However, based on the theory of diversified bet-hedging (Olofsson et al., 2009), larger clutch sizes could be a strategy employed in unpredictable environments to increase the likelihood of at least one offspring having a phenotype that will enable it to survive (Simons, 2007). Overall, birds are likely unable to habituate to unpredictable drilling noise (e.g., pipes banging, machinery turning on and off, trucks backing up and honking; Rosa and Koper, 2022). With a projected increased drilling of 15% for 2023 (Canadian Association of Energy Contractors (CAOEC), 2022), reducing noise amplitude at drilling sites would likely benefit many species of wildlife.
Anthropogenic noise is often accompanied by many associated disturbances, to which species may positively or negatively respond to. We suggest that decoupling noise from its associated stimuli in manipulative experiments is extremely valuable for better understanding landscape-scale impacts on avian individuals and populations, and clearly, landscape-scale noise playback experiments have led to significant progress in increasing our understanding of ecological responses to anthropogenic noise (e.g., Blickley et al., 2012a; McClure et al., 2013; Cinto Mejia et al., 2019). However, playback experiments might also lead to an overestimation of the potential impacts of noise on wildlife in real, anthropogenically disturbed landscapes because they control for influential factors within the built environment that normally co-occur with anthropogenic noise. These factors may interact with noise in surprising ways, resulting in unpredictable outcomes, such as noise produced by the drilling rigs, which occurs during a short period, had greater impacts on nesting success than constant oil well noise produced by oil wells. While this study did not focus on disentangling the effects on life-history traits of interspecific interactions among our focal species, we suggest future research should endeavor to evaluate the indirect effects of noise in relation to interactions within species communities (see Francis et al., 2009). Overall, we recommend that managers be cautious when attributing findings from experimental research in controlled environments to real-world implications when developing management plans.
Data availability statement
The original contributions presented in the study are included in the article/Supplementary material, further inquiries can be directed to the corresponding author.
Ethics statement
The animal study was reviewed and approved by University of Manitoba Animal Care Protocol F15-005 Canadian Bird Banding Permit 10,840(A) Canadian Wildlife Service Permit #11-MB/SKL/AB-SC007 Alberta Environment and Sustainable Research Development Research Permit #56016 and Collection License #56017.
Author contributions
PR and NK designed the experiment. PR collected and analyzed the field data and led the writing of the manuscript. NK supervised all work and the overall research program. All authors contributed to the article and approved the submitted version.
Funding
Funding was provided by the National Science and Engineering Research Council of Canada (RGPIN-2017-04038), Cenovus Energy, Alberta Conservation Association (D000000563-S007), Canadian Foundation for Innovation (18787), Manitoba Research and Innovations Fund, and Clayton H. Riddell Endowment Fund of the University of Manitoba. NK and PR received partial funding for the research from Cenovus Energy, but the company had no involvement in study design, data analysis, interpretation of findings, or any other related work. Their contribution was limited to providing safety training and access to study sites, and they were not consulted in writing the manuscript to ensure the study’s integrity.
Acknowledgments
The authors thank all the people who contributed to this study, particularly Claire M. Curry, Paulson G. Des Brisay, Heather R. Nenninger, Jacy Bernath-Plaisted, Marie-Ève Cyr, Hannah Carey, Christoph S. Ng, Alexandra Heathcote, and many field technicians and volunteers. The authors thank Cenovus Energy for providing safety training and aid with site logistics and Eastern Irrigation District for granting us access to study sites.
Conflict of interest
The authors declare that the research was conducted in the absence of any commercial or financial relationships that could be construed as a potential conflict of interest.
Publisher’s note
All claims expressed in this article are solely those of the authors and do not necessarily represent those of their affiliated organizations, or those of the publisher, the editors and the reviewers. Any product that may be evaluated in this article, or claim that may be made by its manufacturer, is not guaranteed or endorsed by the publisher.
Supplementary material
The Supplementary material for this article can be found online at: https://www.frontiersin.org/articles/10.3389/fevo.2023.1168585/full#supplementary-material
References
Alberta Energy Regulator (AER) . (2023). ST37: List of wells in Alberta (monthly updates). Available at: https://www.aer.ca/providing-information/data-and-reports/statistical-reports/st37 (accessed January 18, 2023).
Atuo, F. A., Saud, P., Wyatt, C., Determan, B., Crose, J. A., and O'Connell, T. J. (2018). Are oil and natural gas development sites ecological traps for nesting killdeer? Wildl. Biol. 2018:wlb.00476, 1–8. doi: 10.2981/wlb.00476
Barber, J. R., Crooks, K. R., and Fristrup, K. M. (2010). The costs of chronic noise exposure for terrestrial organisms. Trends Ecol. Evol. 25, 180–189. doi: 10.1016/j.tree.2009.08.002
Bates, D., Maechler, M., Bolker, B. M., and Walker, S. (2014). lme4: Linear mixed-effects models using Eigen and S4. J. Stat. Softw.. 67. pp. 1–7.
Bayne, E. M., Habib, L., and Boutin, S. (2008). Impacts of chronic anthropogenic noise from energy-sector activity on abundance of songbirds in the boreal forest. Conserv. Biol. 22, 1186–1193. doi: 10.1111/j.1523-1739.2008.00973.x
Bernath-Plaisted, J., and Koper, N. (2016). Physical footprint of oil and gas infrastructure, not anthropogenic noise, reduces nesting success of some grassland songbirds. Biol. Conserv. 204, 434–441. doi: 10.1016/j.biocon.2016.11.002
Blickley, J. L., Blackwood, D., and Patricelli, G. L. (2012a). Experimental evidence for the effects of chronic anthropogenic noise on abundance of greater sage-grouse at leks. Conserv. Biol. 26, 461–471. doi: 10.1111/j.1523-1739.2012.01840.x
Blickley, J. L., Word, K. R., Krakauer, A. H., Phillips, J. L., Sells, S. N., Taff, C. C., et al. (2012b). Experimental chronic noise is related to elevated fecal corticosteroid metabolites in lekking male greater sage-grouse (Centrocercus urophasianus). PLoS One 7:e50462. doi: 10.1371/journal.pone.0050462
Bolker, B. M., Brooks, M. E., Clark, C. J., Geange, S. W., Poulsen, J. R., Stevens, M. H. H., et al. (2009). Generalized linear mixed models: a practical guide for ecology and evolution. Trends Ecol. Evol. 24, 127–135. doi: 10.1016/j.tree.2008.10.008
Bunkley, J. P., McClure, C. J., Kleist, N. J., Francis, C. D., and Barber, J. R. (2015). Anthropogenic noise alters bat activity levels and echolocation calls. Glob. Ecol. Conserv. 3, 62–71. doi: 10.1016/j.gecco.2014.11.002
Buxton, R. T., McKenna, M. F., Mennitt, D., Fristrup, K., Crooks, K., Angeloni, L., et al. (2017). Noise pollution is pervasive in US protected areas. Science 356, 531–533. doi: 10.1126/science.aah4783
Canadian Association of Energy Contractors (CAOEC) . (2022). CAOEC announces the release of its Q4 2022 and 2023 drilling forecast. Available at: https://caoec.ca/content.asp?admin=Y&contentid=505 (accessed February 2, 2023).
Carey, H. (2021). Survival of chestnut-collared longspurs (Calcarius ornatus) and Baird’s sparrow (Centronyx bairdii) on the breeding grounds in southeastern Alberta. master’s thesis. Winnipeg (MB): University of Manitoba.
Chin, E. H., Love, O. P., Verspoor, J. J., Williams, T. D., Rowley, K., and Burness, G. (2009). Juveniles exposed to embryonic corticosterone have enhanced flight performance. Proc. R. Soc. B 276, 499–505. doi: 10.1098/rspb.2008.1294
Cinto Mejia, E., McClure, C. J., and Barber, J. R. (2019). Large-scale manipulation of the acoustic environment can alter the abundance of breeding birds: evidence from a phantom natural gas field. J. Appl. Ecol. 56, 2091–2101. doi: 10.1111/1365-2664.13449
Committee on the Status of Endangered Wildlife in Canada (COSEWIC) . (2019). COSEWIC Assessment and Status Report on the Chestnut-Collared Longspur Calcarius ornatus in Canada. Committee on the Status of Endangered Wildlife in Canada. Ottawa.
Cyr, M. E. (2021). Effects of anthropogenic noise on songbirds and how mitigation may differ in high and low-income countries. master’s thesis. Winnipeg (MB): University of Manitoba.
Des Brisay, P. G., Burns, L. D., Ellison, K., Anderson, W. G., Leonard, M., and Koper, N. (2022). Stress and habitat selection suggest that oil infrastructure is more impactful than noise for grassland songbirds. Environ. Manag. 71, 393–404. doi: 10.1007/s00267-022-01752-2
Des Huet Aunay, G., Grenna, M., Slabbekoorn, H., Nicolas, P., Nagle, L., Leboucher, G., et al. (2017). Negative impact of urban noise on sexual receptivity and clutch size in female domestic canaries. Ethology 123, 843–853. doi: 10.1111/eth.12659
Dillon, K. G., and Conway, C. J. (2018). Nest predation risk explains variation in avian clutch size. Behav. Ecol. 29, 301–311. doi: 10.1093/beheco/arx130
Ehrlich, P. R., Dobkin, D. S., and Wheye, D. (1988). The birder's handbook: A field guide to the natural history of north American birds. New York: Simon and Schuster.
Finch, D., Schofield, H., and Mathews, F. (2020). Traffic noise playback reduces the activity and feeding behaviour of free-living bats. Environ. Pollut. 263:114405. doi: 10.1016/j.envpol.2020.114405
Francis, C. D., and Barber, J. R. (2013). A framework for understanding noise impacts on wildlife: an urgent conservation priority. Front. Ecol. Environ. 11, 305–313. doi: 10.1890/120183
Francis, C. D., Ortega, C. P., and Cruz, A. (2009). Noise pollution changes avian communities and species interactions. Curr. Biol. 19, 1415–1419. doi: 10.1016/j.cub.2009.06.052
Francis, C. D., Paritsis, J., Ortega, C. P., and Cruz, A. (2011). Landscape patterns of avian habitat use and nest success are affected by chronic gas well compressor noise. Landsc. Ecol. 26, 1269–1280. doi: 10.1007/s10980-011-9609-z
Fretwell, S. D., and Lucas, H. L. (1969). On territorial behavior and other factors influencing habitat distribution in birds. Acta Biotheor. 19, 16–36. doi: 10.1007/BF01601953
Goldbogen, J. A., Southall, B. L., DeRuiter, S. L., Calambokidis, J., Friedlaender, A. S., Hazen, E. L., et al. (2013). Blue whales respond to simulated mid-frequency military sonar. Proc. R. Soc. B 280:20130657. doi: 10.1098/rspb.2013.0657
Griffith, S. C., Owens, I. P. F., and Thuman, K. A. (2002). Extra pair paternity in birds: a review of interspecific variation and adaptive function. Mol. Ecol. 11, 2195–2212. doi: 10.1046/j.1365-294X.2002.01613.x
Griffiths, R., Double, M. C., Orr, K., and Dawson, R. J. (1998). A DNA test to sex most birds. Mol. Ecol. 7, 1071–1075. doi: 10.1046/j.1365-294x.1998.00389.x
Halfwerk, W., Holleman, L. J., Lessells, C. K. M., and Slabbekoorn, H. (2011). Negative impact of traffic noise on avian reproductive success. J. Appl. Ecol. 48, 210–219. doi: 10.1111/j.1365-2664.2010.01914.x
Hasselquist, D., and Kempenaers, B. (2002). Parental care and adaptive brood sex ratio manipulation in birds. Philos. Trans. R. Soc. Lond., B. Biol. Sci. 357, 363–372. doi: 10.1098/rstb.2001.0924
Heathcote, A. L. (2019). Effects of oil infrastructure and associated noise on the stress physiology, growth and development of an altricial grassland songbird nestling. master’s thesis. Winnipeg (MB): University of Manitoba.
Ivanova, N. V., Dewaard, J. R., and Hebert, P. D. (2006). An inexpensive, automation-friendly protocol for recovering high-quality DNA. Mol. Ecol. Notes 6, 998–1002. doi: 10.1111/j.1471-8286.2006.01428.x
Jones, S. L., and Geupel, G. R. (2007). Beyond Mayfield: measurement of nest-survival data. Stud. Avian Biol. 34, 1–159.
Jonsomjit, D., Jones, S. L., Gardali, T., Geupel, G. R., and Gouse, P. J. (2007). A Guide to Nestling Development and Aging in Altricial Passerines. US Fish & Wildlife Publications, Washington, DC
Kight, C. R., and Swaddle, J. P. (2011). How and why environmental noise impacts animals: an integrative, mechanistic review. Ecol. Lett. 14, 1052–1061. doi: 10.1111/j.1461-0248.2011.01664.x
Kleist, N. J., Guralnick, R. P., Cruz, A., Lowry, C. A., and Francis, C. D. (2018). Chronic anthropogenic noise disrupts glucocorticoid signaling and has multiple effects on fitness in an avian community. Proc. Natl. Acad. Sci. U. S. A. 115:201709200. doi: 10.1073/pnas.1709200115
Koper, N., Leston, L., Baker, T. M., Curry, C., and Rosa, P. (2016). Effects of ambient noise on detectability and localization of avian songs and tones by observers in grasslands. Ecol. Evol. 6, 245–255. doi: 10.1002/ece3.1847
Kuznetsova, A., Brockhoff, P. B., and Christensen, R. H. B. (2017). lmerTest package: tests in linear mixed effects models. J. Stat. Softw. 82, 1–26. doi: 10.18637/jss.v082.i13
Leston, L., Koper, N., and Rosa, P. (2015). Perceptibility of prairie songbirds using double-observer point counts. Gt. Plains Res. 25, 53–61. doi: 10.1353/gpr.2015.0021
Lynn, S. E., and Wingfield, J. C. (2003). Male chestnut-collared longspurs are essential for nestling survival: a removal study. Condor 105, 154–158. doi: 10.1093/condor/105.1.154
Martin, T. E. (1987). Food as a limit on breeding birds: a life-history perspective. Annu. Rev. Ecol. Syst. 18, 453–487. doi: 10.1146/annurev.es.18.110187.002321
McClure, C. J., Ware, H. E., Carlisle, J., Kaltenecker, G., and Barber, J. R. (2013). An experimental investigation into the effects of traffic noise on distributions of birds: avoiding the phantom road. Proc. R. Soc. B 280:20132290. doi: 10.1098/rspb.2013.2290
Nenninger, H. R., and Koper, N. (2018). Effects of conventional oil wells on grassland songbird abundance are caused by presence of infrastructure, not noise. Biol. Conserv. 218, 124–133. doi: 10.1016/j.biocon.2017.11.014
Ng, C. S., Des Brisay, P. G., and Koper, N. (2019). Chestnut-collared longspurs reduce parental care in the presence of conventional oil and gas development and roads. Anim. Behav. 148, 71–80. doi: 10.1016/j.anbehav.2018.12.001
Northrup, J. M., Anderson, C. R. Jr., Gerber, B. D., and Wittemyer, G. (2021). Behavioral and demographic responses of mule deer to energy development on Winter range. Wildl. Monogr. 208, 1–37. doi: 10.1002/wmon.1060
Olofsson, H., Ripa, J., and Jonzén, N. (2009). Bet-hedging as an evolutionary game: the trade-off between egg size and number. Proc. R. Soc. B 276, 2963–2969. doi: 10.1098/rspb.2009.0500
Pater, L. L., Grubb, T. G., and Delaney, D. K. (2009). Recommendations for improved assessment of noise impacts on wildlife. J. Wildl. Manag. 73, 788–795. doi: 10.2193/2006-235
Potvin, D. A. (2017). Coping with a changing soundscape: avoidance, adjustments and adaptations. Anim. Cogn. 20, 9–18. doi: 10.1007/s10071-016-0999-9
Pyle, P., Jones, S. L., and Ruth, J. M. (2008). Molt and Aging Criteria for Four North American Grassland Passerines. US Fish & Wildlife Publications, Washington, DC
R Core Team . (2014). R: A Language and Environment for Statistical Computing. R Foundation for Statistical Computing, Vienna.
R Core Team . (2019). R: A Language and Environment for Statistical Computing. R Foundation for Statistical Computing, Vienna.
Romero, L. M., and Reed, J. M. (2005). Collecting baseline corticosterone samples in the field: is under 3 min good enough? Comp. Biochem. Physiol. A Mol. Integr. Physiol. 140, 73–79. doi: 10.1016/j.cbpb.2004.11.004
Rosa, P., and Koper, N. (2018). Integrating multiple disciplines to understand effects of anthropogenic noise on animal communication. Ecosphere 9:e02127. doi: 10.1002/ecs2.2127
Rosa, P., and Koper, N. (2022). Impacts of oil well drilling and operating noise on abundance and productivity of grassland songbirds. J. Appl. Ecol. 59, 574–584. doi: 10.1111/1365-2664.14075
Rosa, P., Swider, C. R., Leston, L., and Koper, N. (2015). Disentangling effects of noise from presence of anthropogenic infrastructure: design and testing of system for large-scale playback experiments. Wildl. Soc. Bull. 39, 364–372. doi: 10.1002/wsb.546
Sauer, J., Hines, J., Fallon, J., Pardieck, K., Ziolowski, D. J., and Link, W. (2015). The North American Breeding Bird Survey (BSS), Results and Analysis 1966–2013. USGS Patuxent Wildlife Research Center, Laurel, MD.
Senzaki, M., Kadoya, T., and Francis, C. D. (2020). Direct and indirect effects of noise pollution alter biological communities in and near noise-exposed environments. Proc. R. Soc. B 287:20200176. doi: 10.1098/rspb.2020.0176
Shaffer, T. L. (2004). A unified approach to analyzing nest success. Auk 121, 526–540. doi: 10.1642/0004-8038(2004)121[0526:AUATAN]2.0.CO;2
Shannon, G., McKenna, M. F., Angeloni, L. M., Crooks, K. R., Fristrup, K. M., Brown, E., et al. (2016). A synthesis of two decades of research documenting the effects of noise on wildlife. Biol. Rev. 91, 982–1005. doi: 10.1111/brv.12207
Simons, A. M. (2007). Selection for increased allocation to offspring number under environmental unpredictability. J. Evol. Biol. 20, 813–817. doi: 10.1111/j.1420-9101.2006.01270.x
Smith, R. J., and Moore, F. R. (2003). Arrival fat and reproductive performance in a long-distance passerine migrant. Oecologia 134, 325–331. doi: 10.1007/s00442-002-1152-9
Stearns, S. C. (1976). Life-history tactics: a review of the ideas. Q. Rev. Biol. 51, 3–47. doi: 10.1086/409052
Warren, P. S., Katti, M., Ermann, M., and Brazel, A. (2006). Urban bioacoustics: it's not just noise. Anim. Behav. 71, 491–502. doi: 10.1016/j.anbehav.2005.07.014
Weber, B. (2021). Abandoned oil and gas wells put unfair burden on Alberta landowners, taxpayers, study says. CBC News Calgary. Available at: https://www.cbc.ca/news/canada/calgary/abandoned-wells-oil-gas-alberta-cost-report-1.6033830 (accessed January 18, 2023).
Weber, B. (2022). $1B orphaned oil and gas well cleanup bill estimate leaves out 'most expensive part,' critics say. CBC News Calgary. Available at: https://www.cbc.ca/news/canada/calgary/orphaned-wells-liability-alberta-1.6326942 (Accessed January 18, 2023).
Westneat, D. F., Stewart, I. R., Woeste, E. H., Gipson, J., Abdulkadir, L., and Poston, J. P. (2002). Patterns of sex ratio variation in house sparrows. Condor 104, 598–609. doi: 10.1093/condor/104.3.598
Wingfield, J. C., Vleck, C. M., and Moore, M. C. (1992). Seasonal changes of the adrenocortical response to stress in birds of the Sonoran Desert. J. Exp. Zool. 264, 419–428. doi: 10.1002/jez.1402640407
Keywords: anthropogenic noise, grassland songbirds, energy development, playback study, comparative study
Citation: Rosa P and Koper N (2023) Comparison of impacts of oil infrastructure on grassland songbirds between landscape-scale noise addition and noise removal experiments. Front. Ecol. Evol. 11:1168585. doi: 10.3389/fevo.2023.1168585
Edited by:
Alejandro Ariel Ríos-Chelén, Autonomous University of Tlaxcala, MexicoReviewed by:
Tinglei Jiang, Northeast Normal University, ChinaLuis Diaz-Balteiro, Polytechnic University of Madrid, Spain
Copyright © 2023 Rosa and Koper. This is an open-access article distributed under the terms of the Creative Commons Attribution License (CC BY). The use, distribution or reproduction in other forums is permitted, provided the original author(s) and the copyright owner(s) are credited and that the original publication in this journal is cited, in accordance with accepted academic practice. No use, distribution or reproduction is permitted which does not comply with these terms.
*Correspondence: Patricia Rosa, cHJvc2FAc2d1LmVkdQ==