- 1Caesar Kleberg Wildlife Research Institute, Texas A&M University Kingsville, Kingsville, TX, United States
- 2East Foundation, San Antonio, TX, United States
Aim: Mitigating the effects of extreme conditions is a mechanism that can structure the activity patterns and habitat selection of a species and may particularly impact species at the extremes of their geographic distribution. Furthermore, changing climate patterns have the potential to influence biotic interactions between species in novel ways. As two species at the edges of their northern and southern distributions, respectively, ocelots and bobcats may face unique pressure compared to individuals in more central portions of their range. Our objective was to describe the selection of thermal cover by ocelots and bobcats to examine whether partitioning of thermal resources was occurring or if this was a source of potential interspecific competition.
Location: We monitored eight ocelots and 13 bobcats in South Texas, USA.
Methods: We compared selection within, above, and below their estimated thermoneutral zones to examine the effect of varying temperature on habitat selection. Additionally, we stationed 130 black globe thermal sensors to describe the thermal properties of the various cover types.
Results: We observed variation in habitat selection across temperatures and species. Ocelots and bobcats selected for shrub cover and vertical canopy cover when cold stressed. When heat stressed, both species avoided bare ground and selected for higher vertical canopy cover and were located closer to dense cover. Black globe measurements revealed differences in environmental temperature across cover types, with forest and shrub cover significantly lower than herbaceous or bare ground.
Main conclusions: Changing climates may influence interspecific competition and alter areas of sympatry through range shifts. Our results stress the importance of dense shrub cover and forested canopy as thermal refuge for ocelots and bobcats and suggest that abundant vegetation may mitigate the effects of interspecific competition during lower temperatures and niche partitioning may reduce interspecific competition during upper temperature limits, providing support for the interactive range-limit theory.
Introduction
Climatic conditions in a region are often dynamic and, as a result, may influence interactions between species as conditions change. When conditions such as temperature range outside of typical conditions (i.e., extreme climatic events), organism may experience heat or cold stress (Terrien et al., 2011) and may respond to these changes by altering their activity or habitat selection, creating the potential for increased competition as animals compete for resources in an attempt to thermoregulate in a stressful environment. Thermoregulation is an essential component to the ecology of all animals, ranging across herpetofauna (Blouin-Demers and Nadeau, 2005), birds (Whittow and Tazawa, 1991), and mammals (Chappell, 1980; Aujard et al., 2006; Hwang et al., 2007). In general, the objective of thermoregulation is to maximize the net gain in energy and maintain homeostasis (Nelson et al., 1984; Mitchel and Angilleta, 2009). Maintaining an internal temperature within a certain range often requires balancing the processes of thermogenesis (heat production) and thermolysis (heat dissipation; Terrien et al., 2011). Ectotherms regulate body temperature by transferring heat to and from their surrounding environment, while endotherms typically rely on internally produced heat (Nelson et al., 1984). An ideal temperature range, known as the thermal neutral zone, exists within which an organism can maintain internal temperatures without exerting physiological effort (i.e., such as increased metabolic rates; Smith and Kok, 2006a). Outside of this range lies the thermal zone of survival, requiring behavioral adaptations and physiological effort to maintain core temperature (Stryker, 2016). Eventually, temperatures become too extreme and biological processes within the organism begin to fail, referred to as the minimum and maximum critical temperatures (Deutsch et al., 2008; Stryker, 2016). Exposure to extreme temperatures can negatively impact fitness and may result in hyperthermia or hypothermia from excessively high or low temperatures, respectively (Schmitz, 1991; Terrien et al., 2011). As a result, animals have evolved a broad range of strategies to maintain temperature within a suitable range.
Thermoregulatory adaptations of animals include both autonomic and behavioral responses (Aujard et al., 2006). Autonomic reactions include vasomotor responses, shivering, panting, sweating, color changes, and erection of fur or feathers (Nelson et al., 1984). Behavioral thermoregulation includes changes in posture, changing activity levels or altering habitat selection (Stelzner and Hausfater, 1986; Jemifow et al., 2004; Terrien et al., 2011; Tanner et al., 2017). Selecting for specific areas of optimal temperature is a common behavioral adaptation and among the most fundamental methods of thermoregulation (Nelson et al., 1984). From an ultimate perspective, animals have evolved to inhabit particular geographic ranges at latitudes and longitudes or elevations that maintain specific temperature ranges. On a proximate scale, animals may select for areas on the landscape that can facilitate maintaining homeostasis while operating within the range of ultimate conditions. Selection for specific habitat features as a means of thermal refuge has been observed in a multitude of mammalian species. Ruffed lemurs increased use of sunny patches during lower ambient temperatures (Morland, 1993). Predatory species such as gray foxes (Urocyon cinereoargenteus) and ringtails (Bassariscus astutus) rely on habitat features (tree cavities, rocks) as a means of thermal refuge (Zielinski, 2015). Other carnivores such as American badgers (Taxidea taxus) and wolverines (Gulo gulo) rely on burrows and dens to regulate their temperature during both overly hot and cold periods (Zielinski, 2015; Tsonuda et al., 2018). Pumas (Puma concolor) similarly rely on the use of micro-environments, and variation in pelage thickness, to mitigate the effects of changing ambient temperature (Laundre, 2005).
In the past several decades, global climates have shifted dramatically due to anthropogenic influences, resulting in increasing sea levels and glacial melt, disruptions in seasonal processes, variation in wind and precipitation and increasing average temperatures (Stuart-Menteth et al., 2003; IPCC, 2022). Climate change can influence ecosystems in a variety of ways including altering hydrology, primary production and trophic interactions between species (Sattar et al., 2021). In regard to wildlife species, changing climates have the potential to increase human–wildlife conflicts (Abrahms, 2021), alter predator–prey interactions (Miller et al., 2017), cause shifts in geographic distributions (Shoo et al., 2006; Saikkonen et al., 2012), and alter fundamental ecological processes such as development, respiration rate, survival and reproduction (Zhao et al., 2014; Speights and Barton, 2019). As such, climate change can substantially alter the fundamental and realized niche of a species (MacArthur, 1968). Because temperatures at night are increasing more rapidly than daytime temperatures (Zhao et al., 2014), climate change may strongly impact nocturnal species at disparate rates. Documented adverse effects of climate change on predators (Miller et al., 2017; Speights and Barton, 2019), in combination with increasing nocturnal temperatures, may pose a particular threat to nocturnal predators such as many felid species.
Little is known of the thermoregulatory behavior of many wild felids. Thermoregulatory stress, coupled with limited prey abundance, resulted in mortality of Geoffroy’s cats (Leopardus geoffroyi; Pereira et al., 2010). Smith and Kok (2006a) estimated the thermoneutral zone of African lions (Panthera leo) through observational studies. Additionally, lions increased loin exposure to dissipate heat during warmer periods (Smith and Kok, 2006b). Cold adapted species like the Amur tiger (Panthera tigris altaica) rely on dense pelage to combat excessive cold and can increase insulation during winters (Langman et al., 2015). Bobcats (Lynx rufus) commonly occurred in energetically costly environments suggesting the use of microenvironments and behaviors such as sunning to aid in thermoregulation (Mautz and Pekins, 1989). Veterinary studies on felines have described the vascular and dermal adaptations involved in thermoregulation (Affolter and Moore, 1994). Additionally, prior research has been conducted on the thermoregulatory behavior of large captive felids (Young, 2010; Stryker, 2016). Large amounts of energy are required to maintain regular bodily processes in felines, and ambient temperatures can greatly influence energy expended on thermoregulation (Pereira et al., 2010). Furthermore, nocturnal predators typically expend greater energy on maintaining homeostasis (Chappell, 1980), suggesting that thermoregulation may be particularly vital to a nocturnal feline such as an ocelot (Leopardus pardalis).
The ocelot is a Neotropical felid found throughout south and central America, as well as the southern tip of Texas in the United States (Di Bitetti et al., 2006). Ocelots in South Texas are heavily dependent on dense vegetation (Shindle and Tewes, 1998; Harveson et al., 2004) and, as a result of reduced populations due to habitat loss and fragmentation, are listed as federally endangered in the United States (Haines et al., 2006). The availability of woody vegetation in South Texas has plummeted as a result of agricultural and urban development (Harveson et al., 2004). Despite this, selection for woody cover by ocelots has increased in recent years, showing an increasing dependence on woody vegetation (Veals et al., 2022). Future projections of land use in South Texas suggest continued urban and agricultural expansion and further decline in availability of woody vegetation (Lombardi et al., 2020a). Ocelots may use this dense woody vegetation to aid in thermoregulation; however, this relationship remains undocumented. Within this area, ocelots reduced activity during hotter temperatures (Leonard et al., 2020); however, habitat selection in the context of thermal extremes has not been examined in this species to our knowledge.
Ocelots in South Texas are sympatric with another similar-sized felid, the bobcat (Lombardi et al., 2020b; Sergeyev et al., 2023). As these species overlap in space and diet, there is a high potential for competition between species (MacArthur, 1968; Horne et al., 2009; Booth-Binczik et al., 2013; Lombardi et al., 2020b). However, bobcats are a more cold adapted species compared to ocelots (Newbury and Hodges, 2018; Newbury and Hodges, 2019), and South Texas represents an area toward the southern edge of their distribution that extends as far northward as southern Canada (Wigginton and Dobson, 1999). As such, the effects of extreme climate on habitat selection may differ between these two carnivores and may result in competition for thermal refuge or may conversely facilitate coexistence through spatial partitioning of thermal resources. The interactive range-limit theory (iRLT) would dictate that abiotic factors might reduce competition along lower range limits (in the case of bobcats) and that positive biotic conditions such as food and resources can mitigate the effects of abiotic stress at upper limits (in the case of the ocelot; Sirén and Morelli, 2019). The effects of snow cover and winter conditions on habitat selection of bobcats has been examined at the northern extent of its range (Newbury and Hodges, 2018; Scully et al., 2018; Newbury and Hodges, 2019; Sirén et al., 2021); however, to our knowledge habitat selection in the context of extreme temperature in the southern portions of their range has not been documented. Furthermore, to our knowledge this is the first study to examine coexistence of ocelots and bobcats in the context of thermal ecology and changing climates.
As a species on the northern periphery of its range, ocelots in South Texas may face a unique set of ecological challenges compared to individuals in more central portions of their distribution, specifically in dealing with extreme climatic conditions (Sexton et al., 2009). We compared habitat selection of ocelots and bobcats within, above and below their thermoneutral zone to examine the effect of abiotic stress on habitat selection and the potential for competition. In addition, we quantified the thermal characteristics of the various cover types available to both species. If microclimates on the landscape result in variation in operative temperature (Bakken et al., 1985), we would expect differences in habitat selection during periods of extreme temperature. Because ocelots are more heat adapted while bobcats are more cold adapted, we assume that dense cover acts as a means of thermal refuge and predicted that (1) ocelots would select for dense vegetation as a means of thermal refuge, particularly during extreme cold; (2) bobcats would show stronger selection for dense vegetation during extreme heat than cold; and (3) extreme climatic conditions would result in less competition through niche partitioning. Furthermore, we compared overlap in predicted high-use areas for each species during extreme heat and cold to examine the potential for competition over thermal resources. As a species of conservation concern, understanding how extreme climatic conditions influence habitat selection of ocelots, and competing carnivores, is essential to managing habitat to sustain populations. Furthermore, as instances of both extreme heat and cold will likely increase due to changing climates, these results may provide a guideline for future management.
Study area
We conducted this study on the East Foundation’s El Sauz Ranch and the Yturria San Francisco Ranch located in Willacy and Kenedy counties in the southern tip of Texas, USA (Figure 1). The Yturria San Francisco Ranch (25.9 km2) is a private ranch that prioritizes conservation of ocelots, land stewardship, and hunting of ungulates. The ranch features two conservation easements (1.98 km2) consisting of highly dense woody vegetation that are owned by the United States Fish and Wildlife Service Lower Rio Grande Valley National Wildlife Refuge Complex. These two easements are comprised of vegetation considered as optimal cover for ocelots in South Texas. Surrounding patches of restored native woody vegetation are managed by The Nature Conservancy. The El Sauz Ranch (113 km2) is an operational cattle ranch that prioritizes land stewardship and jointly manages land for cattle (Bos taurus indicus) and native wildlife. The ranch features a range of landscape features that includes grasslands, coastal estuarine wetlands, man-made water features, sand dunes, prairies, and areas of woody vegetation cover (Lombardi et al., 2020a). Woody vegetation in these areas is comprised of lime prickly ash (Zanthoxylum fagara), white brush (Aloysia gratissima), lotebush (Ziziphus obtusifola), desert olive (Forestiera angustifolia), crucita (Chromolaena odorata), honey mesquite (Neltuma glandulosa), live oak (Quercus virginiana), crucifixion thorn (Castela emoryi), snake-eyes (Phaulothamnus spinescens), huisache (Acacia farnesiana), and spiny hackberry (Celtis pallida; Leonard 2016; Lombardi et al., 2020a; Lombardi et al., 2021). Climate in the region is subtropical and semi-arid climate with annual temperatures that typically range from 10°C to 36°C and mean temperature of 23°C (Haines et al., 2006; Norwine and Kuruvilla, 2007). Rainfall in the region is inconsistent and often leads to episodic droughts; mean annual rainfall was 68 cm (Haines et al., 2006).
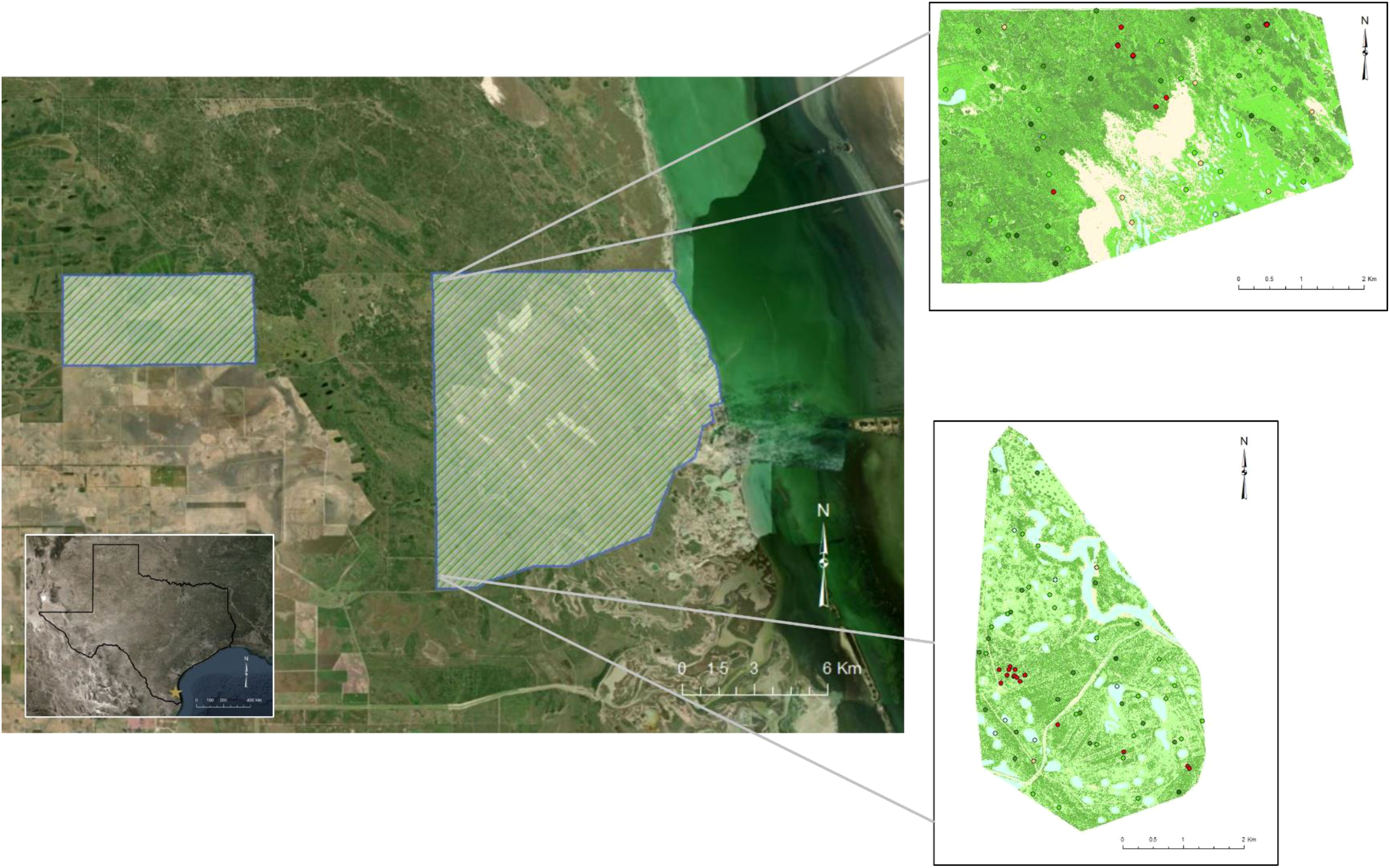
Figure 1 Study area for assessing selection of thermal cover of ocelots (Leopardus pardalis) and bobcats (Lynx rufus) in South Texas, USA from 2017 to 2021. Polygons denote the boundaries of the Yturria San Francisco Ranch (left) and the East Foundation’s El Sauz Ranch (right). Inset maps at right show placement of black globe thermal sensors across microclimates on El Sauz Ranch. Globes were positioned in wetland, bare, herbaceous, shrub, and forest cover, as well as randomly sampled locations of known use by ocelots during extreme heat (>33°C). The different colors in the inset maps represent the different land cover types in which globes were positioned (beige = bare ground, light green = herbaceous, green = shrub, dark green = forest, light blue = wetland, and red = “hot”). Landsat imagery courtesy of the USGS/NASA Landsat Program, reproduced under the Landsat Data Distribution Policy.
Methods
Animal capture
From January 2017 to May 2021, we captured eight (three males and five females) and 13 bobcats (seven male and six female) on the El Sauz Ranch and Yturria San Francisco Ranch. We captured animals using single-door Tomahawk box-traps (108 cm × 55 cm × 40 cm; Tomahawk Trap Co., Tomahawk, WI, USA). Box traps were baited with a live chicken (Gallus gallus) or pigeon (Columbia livia) contained within a separate compartment inaccessible from the trap. All captured animals were of adult age. We immobilized captured animals using a 4:1 mixture of tiletamine hydrochloride and zolazepam hydrochloride (Telazol™, Zoetis, Florham Park, NJ, USA) at a dose of 5mg/kg in 2017 and a mixture of ketamine hydrochloride (4–5 mg/kg) and medetomidine HCl (0.05 mg/kg) and used a reversal of 5 mg of atipamezole per 1-mg medetomidine (ZooPharm, Laramie, WY, USA) from 2019 to 2021 (Shindle and Tewes, 2000; Lombardi et al., 2021; Sergeyev et al., 2022). We fitted each captured individual with a Lotek Minitrack and Litetrack global positioning system (GPS) radio collar (Lotek™, New Market, ON, Canada). We programmed collars to record locations every 30–60 min and to automatically drop after either a 4- to 6-month or 1-year period. All capture and handling of wildlife were conducted following United States Fish and Wildlife Service permit (#PRT-676811), Texas Parks and Wildlife Department permit (#SP0190-600), and Texas A&M University Kingsville Institutional Animal Care and Use Committee protocol (2012-12-20B-A2).
Habitat selection during thermal extremes
We assessed habitat selection of ocelots and bobcats using a step selection framework (Muff et al., 2019). We partitioned our data into four thermal zones based on the values of thermoneutral zones for ocelots and bobcats [22°C–33°C for ocelots (n = 1) and 19°C–32°C for bobcats (n = 3); McNab, 2000]. As these temperatures elicited an ecophysiological response, they may lead to a behavioral response (Kearney et al., 2021). As such, we considered four temperature ranges: <19°C (a range below both species’ thermoneutral zones), 19°C–22°C (when temperatures are below the ocelot’s thermoneutral zone while being within the bobcat’s thermoneutral zone), 22°C–33°C (32°C for bobcats; when temperatures are within both species’ thermoneutral zones), and >33°C (when temperatures are above both species’ thermoneutral zones) and fitted the same model of habitat selection across these four thermal partitions. Climate data were obtained from the “rnoaa” package in program R (Version 4.1.2) for the nearest available weather station, located at the Brownsville International Airport in Brownsville, TX, approximately 72 km from our study area. Statewide land cover data were obtained from Ecological Mapping Systems of Texas (Elliott et al., 2009–2014) and cover types of collected GPS locations were reclassified into one of four categories: bare ground, herbaceous, shrub, and forest. Vegetation density and percent canopy cover were calculated using light detection and ranging (LiDAR) data flown by the United States Geological Survey (USGS, 2018), downloaded from the Texas Natural Resources Information System and classified using GeoCue LP360 software at a resolution of 10 m. We considered dense vegetation cover as greater than 75% vertical canopy cover as ocelots and bobcats showed interspecific partitioning of these areas (Horne et al., 2009); we similarly considered <25% as the opposite extreme referring to open areas. Distance to dense cover (>75% canopy; m), distance to open areas (<25% canopy; m), and patch size of dense cover (>75% canopy, m2) were all calculated from the canopy cover raster using ESRI ArcMap 10.8 and program R.
We evaluated selection using a mixed-effect conditional logistic regression model (Muff et al., 2019). We considered available steps based on the empirical distribution of step lengths and turning angle from each species and considered 10 random steps per true step. Our models included land cover type, vegetation density (vegetation points/cell), percent canopy cover, distance to dense vegetation cover, distance to open areas, and patch size of dense cover. Animal ID was included as a random intercept in all models. All variables in the model were scaled and centered by standard deviation. For each thermal partition, we compared an additive model that include the aforementioned variables and an interaction model that included those same variables as well as interactions of land cover type with vegetation density and canopy cover to examine if selection of dense cover differed between land cover types. We compared candidate models using AICc model selection (Akaike, 1973) and considered models with ΔAICc < 2.0 to be strongly competing models. To examine whether selection changed during periods of thermal stress, we fitted the same model across thermal partitions.
We created utilization distributions based on our top models using the simulation approach outlined in Signer et al. (2017). We used the R code available by Hofmann et al. (2023) to simulate the dispersal of 50,000 individuals based on previously fitted models for each thermal partition and species, resulting in a raster of habitat selection modeled across the El Sauz Ranch. We used GPS locations collected from ocelots from 2017 to 2021 to create 95% minimum convex polygons (MCPs) as the bounding area for the dispersal simulations, to mimic trapping efforts and known occurrence, and simulated 25,000 individuals within each MCP for each thermal partition and species. Random steps were drawn from a gamma distribution and turning angles were drawn from a von mises distribution where κ = 0 (Hofmann et al., 2023). We then compared these predicted surfaces across species and thermal zones and quantified niche overlap using Schoener’s D (Palaoro et al., 2013), which ranges in value from 0 (no overlap) to 1.0 (complete overlap).
Thermal variation across microclimates
To measure thermal variability across the landscape, we positioned black globe thermal sensors to record temperature within the various cover types available on the East Foundation’s El Sauz Ranch. We built 130 black globe sensors using 15.24-cm copper floats, fitted with a copper cap, and mounted on PVC pipe. Globes were painted matte black (emissivity = 1) to replicate the maximum temperature that an organism may experience on the landscape (Olsen et al., 2018). Globes were mounted 22 cm above the ground (the midpoint between the average shoulder height of an ocelot standing and laying on the ground) to approximate conditions at a height relevant to species of interest. We positioned a HOBO Max Temp MX2201 data logger (Onset, Bourne, MA, USA) inside of each globe and recorded temperature every 15 min for a full year.
We positioned black globes within areas known to be used by ocelots. We used GPS locations collected from ocelots from 2017 to 2021 to create 95% MCPs as the bounding area of two sampling arrays of black globes in the northwest and southwest areas of the ranch (Figure 1). We used a 1-m National Agriculture Imagery Program digital orthophoto quadrangle classified imagery created using ERDAS Imagine 2018 (Hexagon Geospatial Norcross, GA, USA) to quantify land cover types within our areas of interest. Using 2016 normalized difference vegetation indices, we defined six broad land cover categories: forest [live oak (Quercus virginiana); NDVI >0.35], thornshrub (NDVI >0.2 and <0.35), herbaceous (coastal prairie and grasslands, non-woody plants, and riparian vegetation; NDVI ≥0.05 and <0.2), bare ground (inland dunes, sand, caliche roads; NDVI <0.05), and water (wetlands and lagunas). To assess our classification, we followed ground-truth methodologies outlined by Lombardi et al. (2020a), where we collected 760 ground truth points in June and September 2016 and June 2020 using a Trimble a Trimble Nomad® 1050 Series Handheld Computer with GBSS 1-m precision and a Trimble® Geo 7 Series Handheld Computer with 1-m precision (Trimble Navigation, Ltd., Sunnyvale, CA, USA). We then completed an accuracy assessment using a confusion matrix to visually compare the observed field data and the classified data, achieving an overall accuracy of 87.5%.
We quantified the proportion of each cover type within the MCPs using ArcGIS to inform our sampling strategy. Of the 130 total black globes, 100 were sampled within the six cover types in the proportions observed within the MCPs, split evenly between the northern grid and the southern grid. Stratified sampling based on cover types yielded the following design: nine globes in bare ground, 38 in herbaceous cover, 36 in shrub cover, 10 in forest cover, and seven in wetlands (Figure 1); locations were randomly sampled using spatially balanced sampling methods (Brown et al., 2015). In addition, 30 black globes were positioned in areas of known use by ocelots during extreme temperatures from a random sample of 30 locations that were collected during extreme heat (when the temperature measured by an ocelot’s collar exceeded 33°C; McNab, 2000). This design was selected to ensure that areas that function as thermal refuge on the landscape were sampled. Because such areas may only be composed of several individual patches spread across the landscape, there was concern that a stratified sampling scheme based only on cover types may exclude the specific areas that are vital to providing refuge from extreme heat. Measurements from black globe sensors quantify thermal characteristics of microclimates and describe the environmental temperature of an organism within those specific microclimates. Models of habitat selection were used to identify landscape characteristics of areas of thermal refuge and the black globe sensors were used to describe thermal characteristics of the various microclimates available for use on the landscape. We compared black body temperature throughout the diel cycle and black body versus ambient temperature for each cover type sampled.
Results
Habitat selection—ocelots
We examined habitat selection of ocelots and bobcats across four thermal zones and found support for the interaction model over the additive model across all temperatures for both species (ΔAICc > 2.00). As such, our model was consistent across all species and thermal zones and included land cover type, vegetation density, distance to open areas, distance to heavy cover, patch size of heavy cover, percent canopy cover, and an interaction between shrub and forest cover and vegetation density and canopy cover. We omitted the bare cover class in the interactions, because the estimates would not be ecologically meaningful, given bare cover will have no change in vegetation density nor canopy cover. All results described are significant at α = 0.05 unless otherwise stated. Ocelots selected for shrub cover (logRSS = 0.267 below 19°C; 0.365 from 19°C to 22°C; 0.355 from 22°C to 33°C; and 0.426 above 33°C) and greater vertical canopy across all four thermal zones and all cover types (logRSS = 0.556 below 19°C; 0.579 from 19°C to 22°C; 0.615 from 22°C to 33°C; and 0.344 above 33°C; Table 1; Figure 2). We observed no difference in selection of forest cover compared to herbaceous cover across all temperatures. Within their thermoneutral zone (22°C–32°C), ocelots remained closer to heavy cover (logRSS = −0.695) and selected large patches (logRSS = 0.213, α = 0.10) and less dense vegetation (logRSS = −0.142, α = 0.06). As temperatures increased, ocelots exhibited stronger selection for shrub cover (logRSS increased from 0.267 below 19°C to 0.355 from 22°C to 33°C and 0.426 above 33°C). When heat stressed, ocelots avoided bare ground (logRSS = −13.950) and remained further from open areas (logRSS = −0.526). At temperatures above 33°C, we observed a negative interaction between shrub cover and vegetation density (logRSS = −0.104 when Shrub = 1 and 0.374 when Shrub = 0; Figure 3) and a positive interaction between shrub and canopy cover (logRSS = 0.633 when Shrub = 1 and 0.344 when Shrub = 0) and forest and canopy cover (logRSS = 0.977 when Forest = 1 and 0.344 when Forest = 0). When cold stressed (<22°C), ocelots remained further from open areas (logRSS = 0.203 from 19°C to 22°C and 0.267 below 19°C). From 19°C to 22°C, we observed a negative interaction between shrub and vegetation density (logRSS = −0.197 when Shrub = 1 and 0.063 when Shrub = 0) and forest and vegetation density (logRSS = −0.058 when Forest = 1 and 0.063 when Forest = 0).
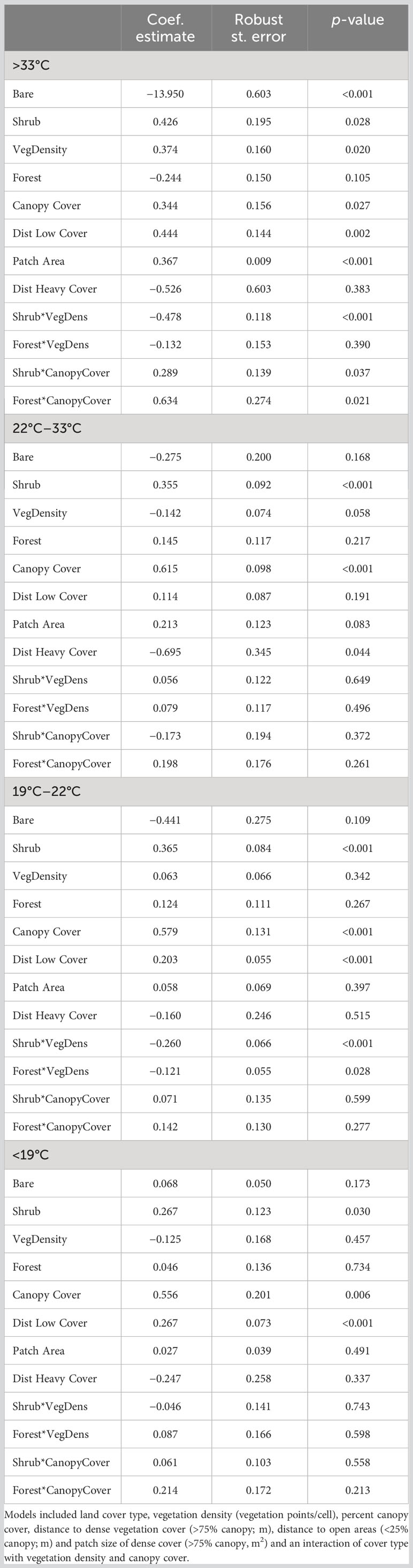
Table 1 Model output describing habitat selection of ocelots (Leopardus pardalis) in South Texas, USA from 2017 to 2021, across four thermal partitions (<19°C, 19°C–22°C, 22°C–33°C, and >33°C) comparing selection below, within and above the thermoneutral zone of ocelots (22°C–33°C).
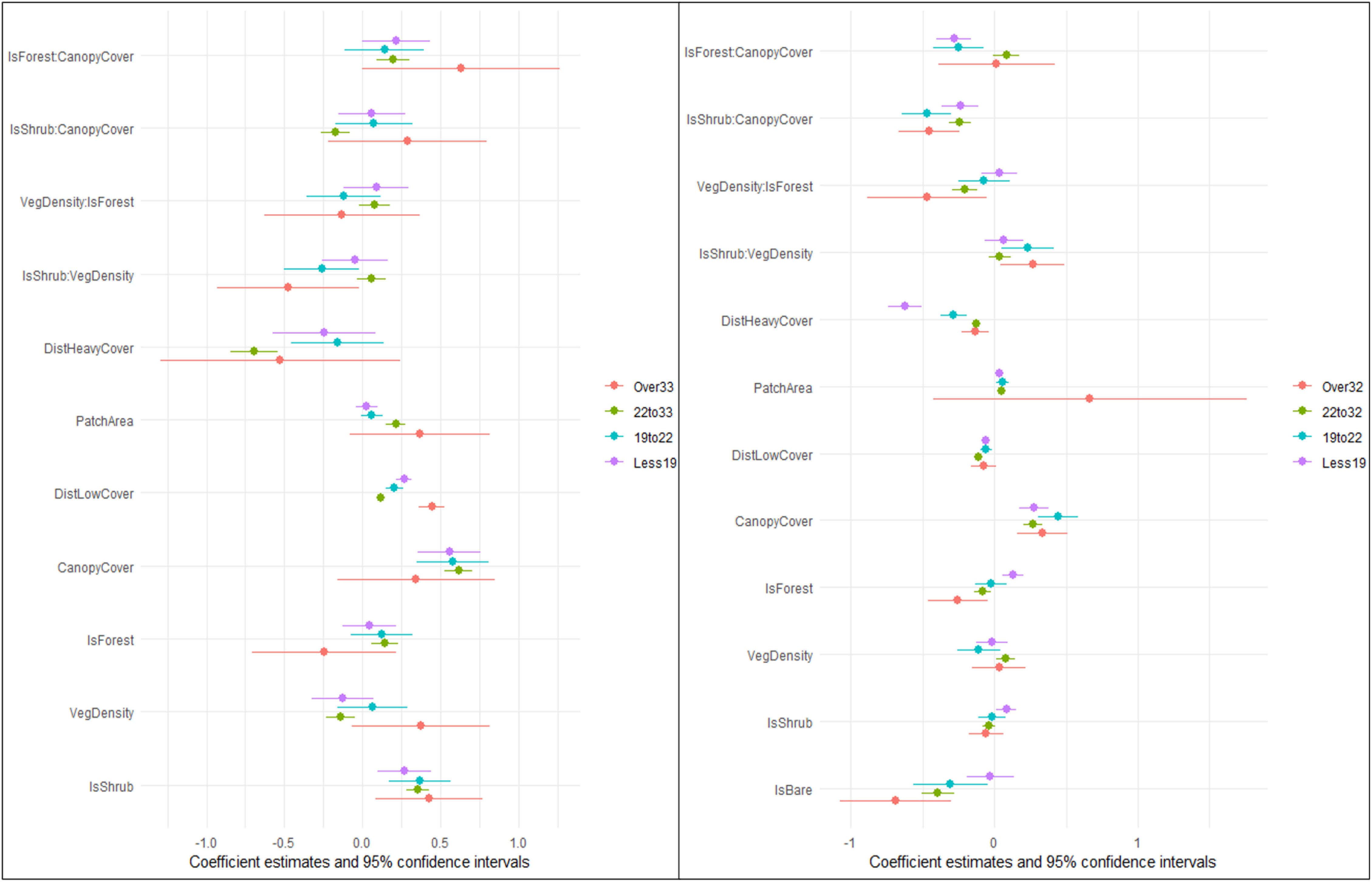
Figure 2 Model coefficients of habitat selection of ocelots (Leopardus pardalis; left panel) and bobcats (Lynx rufus; right panel) in South Texas, USA from 2017 to 2021, across four thermal partitions (below, within and above their thermoneutral zones [TNZ = 22°C–33°C]). Models included land cover type, vegetation density (vegetation points/cell), percent canopy cover, distance to dense vegetation cover (>75% canopy; m), distance to open areas (<25% canopy; m) and patch size of dense cover (>75% canopy, m2) and an interaction of cover type with vegetation density and canopy cover. Vertical zero line denotes no selection, values to the left denote avoidance while values to the right denote selection. We removed the variable for bare ground due to an extremely high negative coefficient during periods of heat stress skewing the rest of the figure and reducing interpretability.
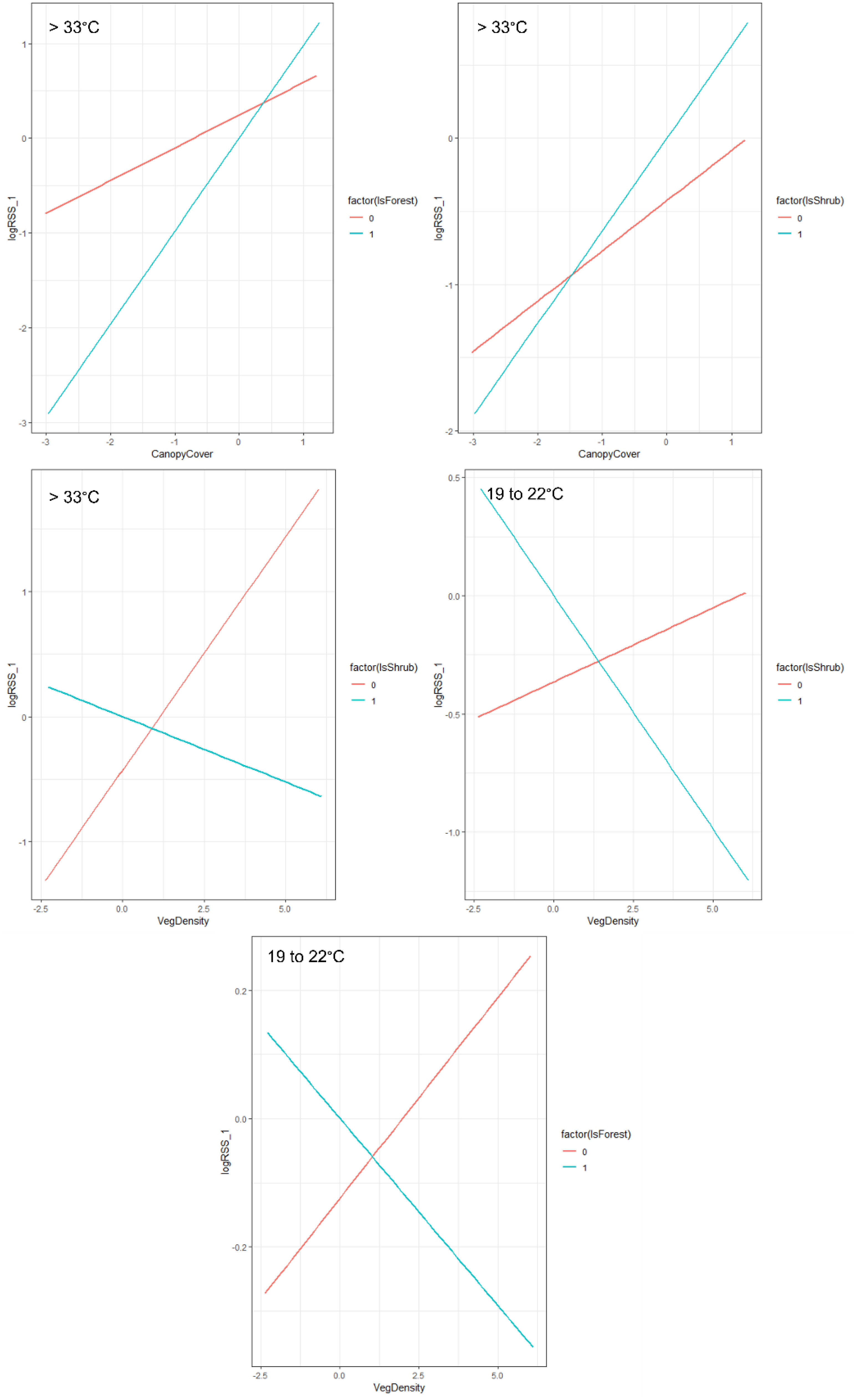
Figure 3 Interaction plots displaying the habitat selection of ocelots (Leopardus pardalis) in South Texas, USA from 2017 to 2021, across four thermal partitions (below, within, and above their thermoneutral zone [22°C–33°C]). Models included land cover type, vegetation density (vegetation points/cell), percent canopy cover, distance to dense vegetation cover (>75% canopy; m), distance to open areas (<25% canopy; m) and patch size of dense cover (>75% canopy, m2) and an interaction of cover type with vegetation density and canopy cover. The two lines represent selection within that specific cover type (Factor = 1) versus outside of that cover type (Factor = 0).
Habitat selection—bobcats
Across all temperature zones, bobcats selected locations closer to dense cover (logRSS = −0.620 below 19°C; −0.284 from 19°C to 22°C; −0.127 from 22°C to 32°C [α = 0.10]; and −0.135 above 32°C) and greater canopy cover (logRSS = 0.276 below 19°C; 0.444 from 19°C to 22°C; 0.270 from 22°C to 32°C; and 0.333 above 32°C; Table 2; Figure 2). We observed a negative interaction between shrub*canopy cover across thermal partitions (logRSS = 0.039 when Shrub = 1 and 0.276 when Shrub = 0 below 19°C; −0.027 when Shrub = 1 and 0.444 when Shrub = 0 from 19°C to 22°C; 0.032 when Shrub = 1 and 0.270 when Shrub = 0 from 22°C to 33°C; and −0.120 when Shrub = 1 and 0.332 when Shrub = 0 above 33°C; Figure 4). Bobcats avoided bare ground within (logRSS = −0.392 from 22°C to 32°C; α = 0.10) and above (logRSS = −0.688 above 32°C) their thermoneutral zone but used bare ground as available below 22°C. Within their thermoneutral zone (19°C–22°C and 22°C–32°C), bobcats used shrub and forest cover equal to herbaceous cover and selected large patches of dense cover (logRSS = 0.052 from 22°C to 32°C; α = 0.06). From 22°C to 32°C, we observed a negative interaction between forest and vegetation density (logRSS = −0.122 when Forest = 1 and 0.081 when Forest = 0). From 19°C to 22°C, we observed a positive interaction between shrub and vegetation density (logRSS = 0.124 when Shrub = 1 and −0.109 when Shrub = 0). When bobcats were heat stressed (>32°C), we observed a positive interaction between shrub and vegetation density (logRSS = 0.300 when Shrub = 1 and 0.032 when Shrub = 0) and a negative interaction between forest and vegetation density (logRSS = −0.437 when Forest = 1 and 0.033 when Forest = 0). When cold stressed (<19°C), bobcats selected for shrub (logRSS = 0.084) and forest cover (logRSS = 0.131) and larger patches (logRSS = 0.038). Below 19°C, we observed a negative interaction between forest and canopy cover (logRSS = −0.004 when Forest = 1 and 0.276 when Forest = 0).
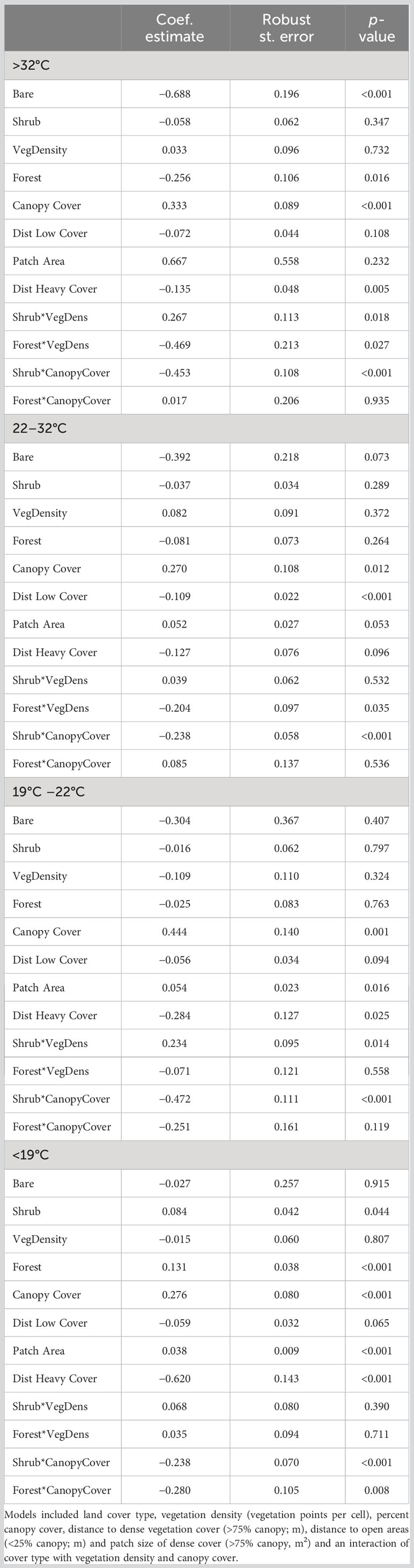
Table 2 Model output describing habitat selection of bobcats (Lynx rufus) in South Texas, USA from 2017 to 2021, across four thermal partitions (<19°C, 19°C–22°C, 22°C–32°C, >32°C) comparing selection below, within and above the thermoneutral zone of bobcats (19°C–32°C).
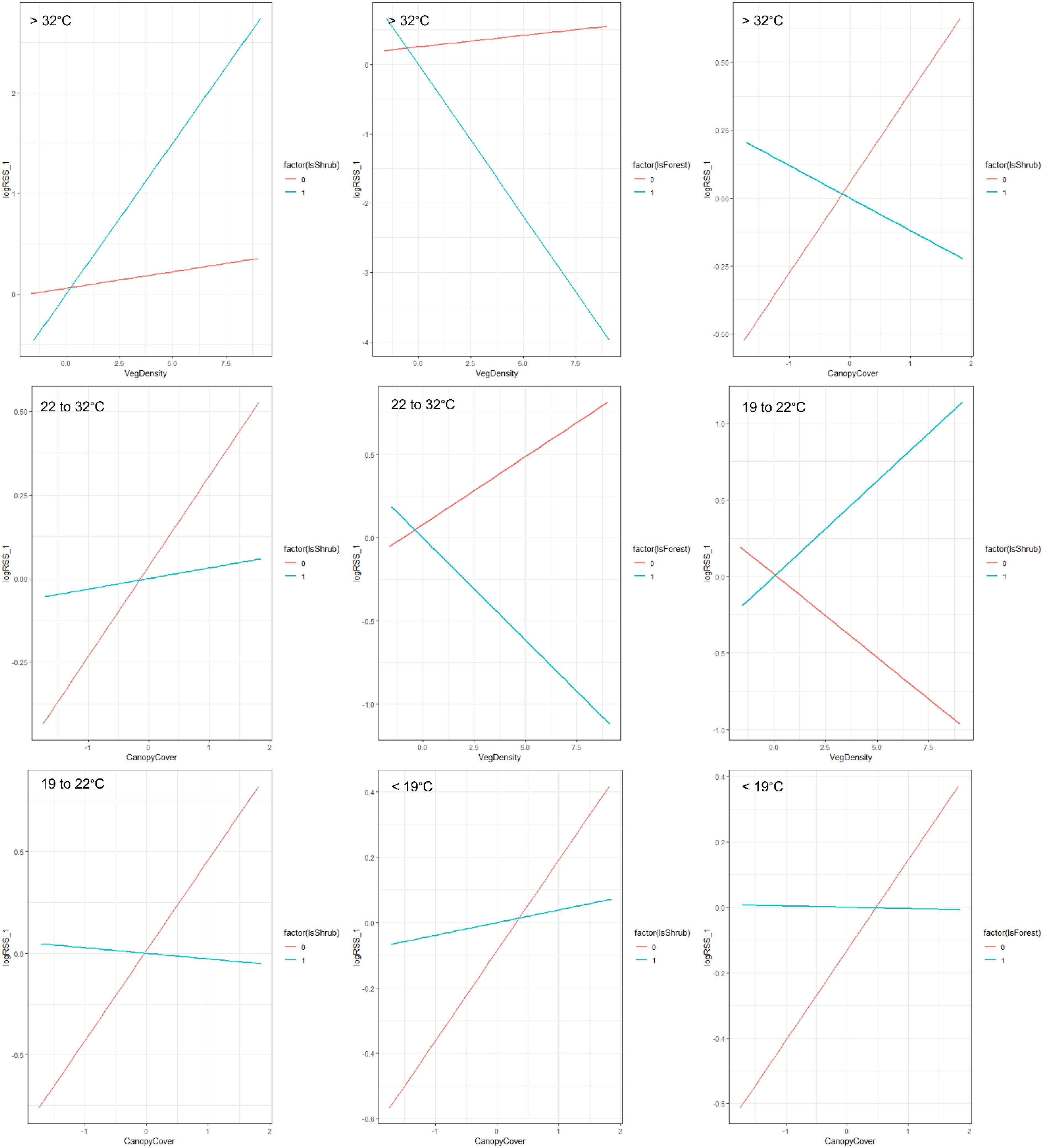
Figure 4 Interaction plots displaying the habitat selection of bobcats (Lynx rufus) in South Texas, USA from 2017 to 2021, across four thermal partitions (below, within, and above their thermoneutral zone [19°C–32°C]). Models included land cover type, vegetation density (vegetation points/cell), percent canopy cover, distance to dense vegetation cover (>75% canopy; m), distance to open areas (<25% canopy; m) and patch size of dense cover (>75% canopy, m2) and an interaction of cover type with vegetation density and canopy cover. The two lines represent selection within that specific cover type (Factor = 1) versus outside of that cover type (Factor = 0).
Comparison of selection across temperature and species
Habitat selection of ocelots varied across thermal partitions. Overlap was high when comparing selection above 33°C and between 19°C and 22°C (Schoener’s D = 0.81; Table 3; Figure 5). Similarly, overlap was high when cold stressed (<19°C) and when thermally neutral (22°C to 33°C, Schoener’s D = 0.70). Overlap was low between selection during heat stress and thermal neutrality (Schoener’s D = 0.35), and when comparing 19°C to 22°C to selection below 19°C (Schoener’s D = 0.37). Overlap in selection by bobcats was high across thermal partitions (Schoener’s D > 0.79; Table 3; Figure 6). Overlap between species was most similar above 33°C and from 19°C to 22°C (Schoener’s D = 0.62 and 0.66, respectively) and was low when thermally neutral and cold stressed (Schoener’s D = 0.28 and 0.27, respectively).
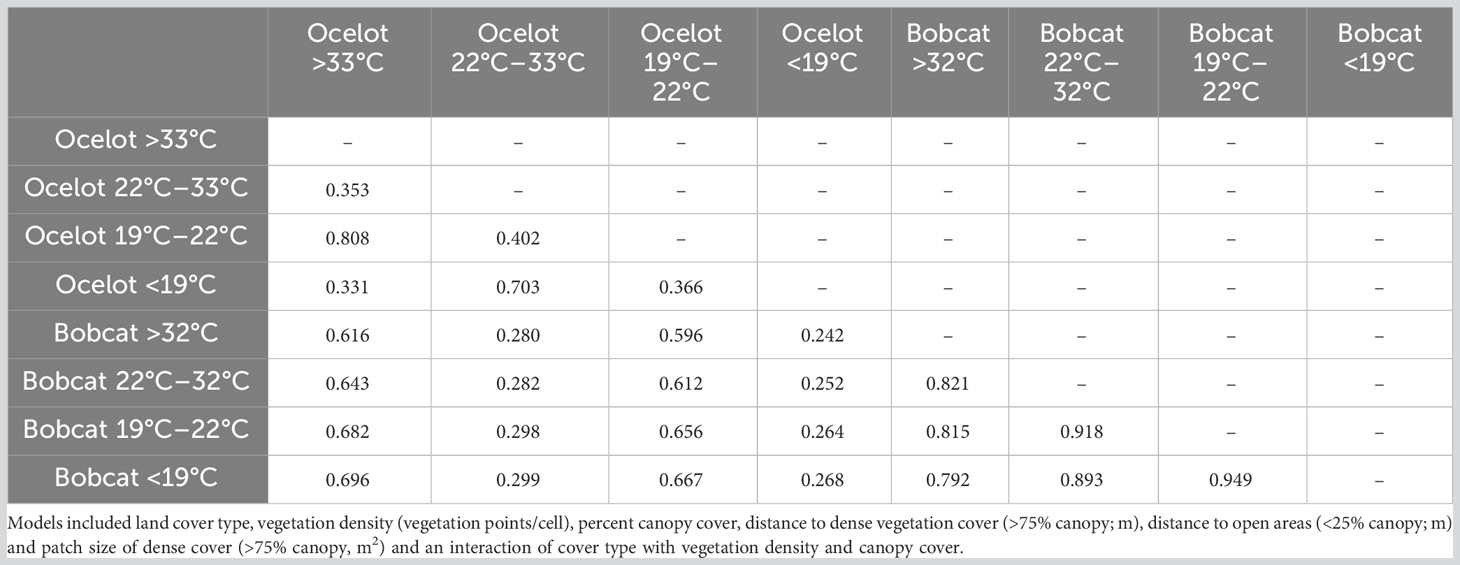
Table 3 Niche overlap between habitat selection of ocelots (Leopardus pardalis) and bobcats (Lynx rufus) in South Texas, USA from 2017 to 2021, across four thermal partitions, table denotes Schoener’s D for each pairwise combination.
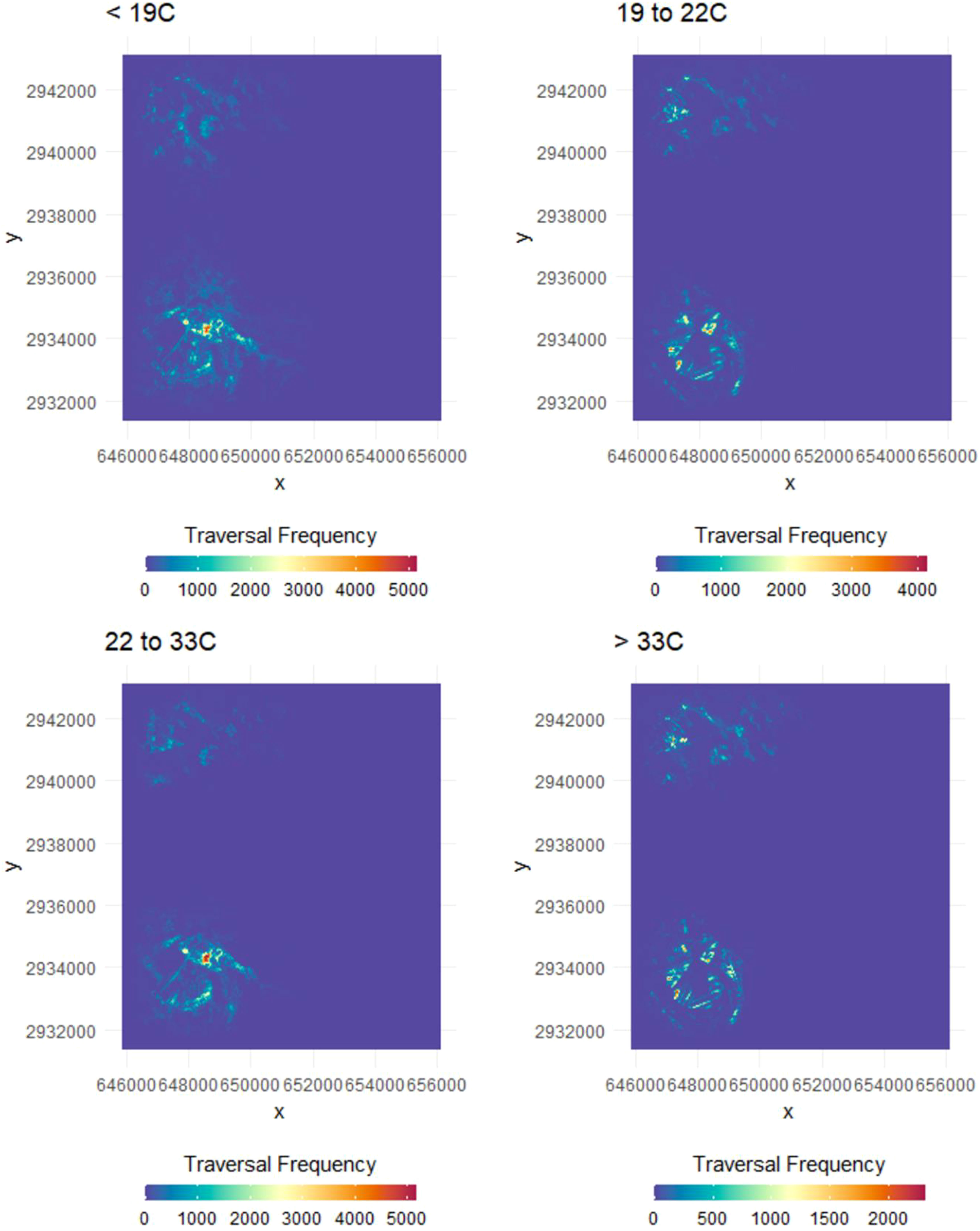
Figure 5 Heat maps displaying the relative probability of use by ocelots (Leopardus pardalis) in South Texas, USA from 2017 to 2021, across four thermal partitions [below (top panels) within (bottom left) and above (bottom right) their thermoneutral zone]. Models included land cover type, vegetation density (vegetation points/cell), percent canopy cover, distance to dense vegetation cover (>75% canopy; m), distance to open areas (<25% canopy; m) and patch size of dense cover (>75% canopy, m2), and an interaction of cover type with vegetation density and canopy cover.
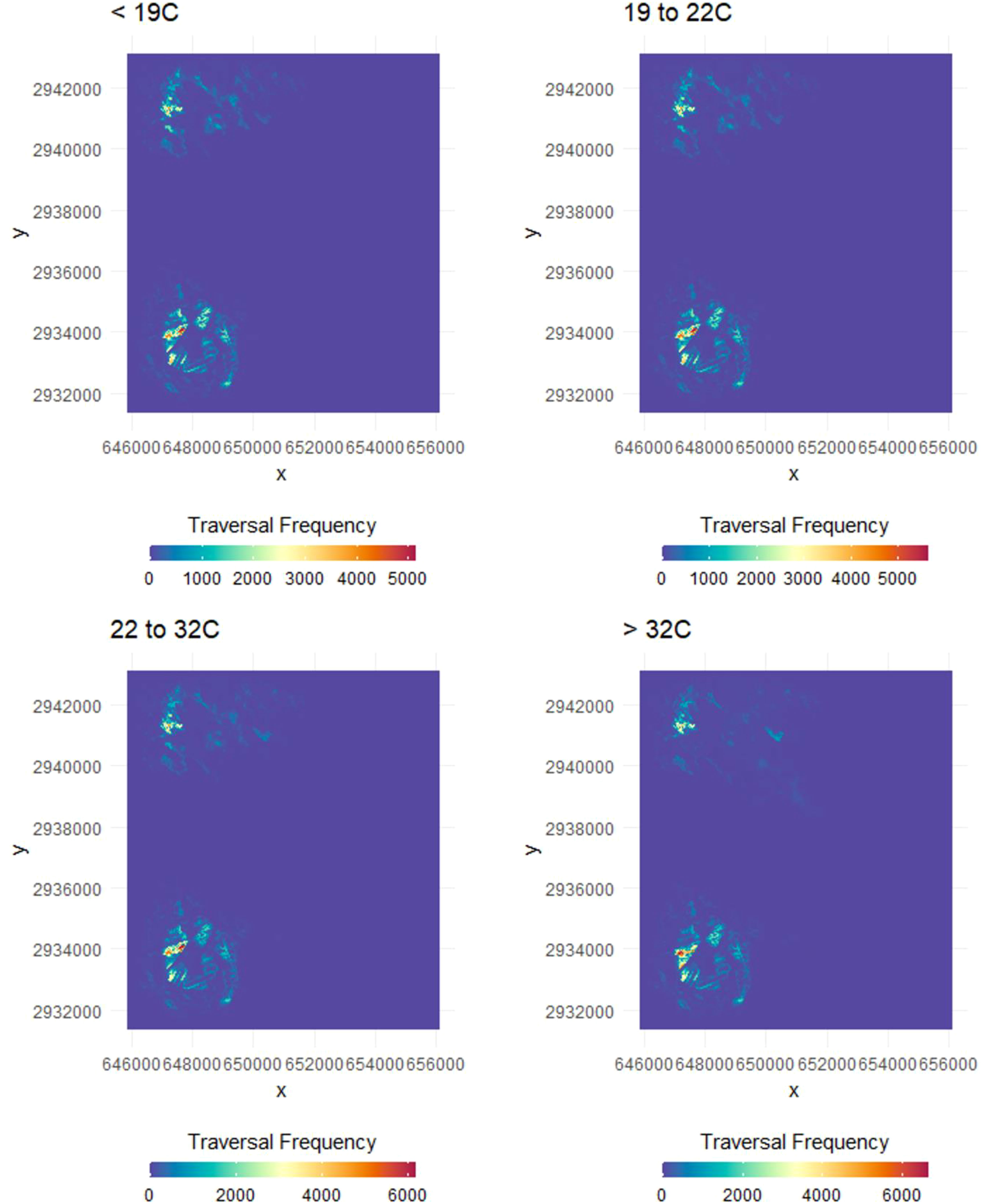
Figure 6 Heat maps displaying the relative probability of use by bobcats (Lynx rufus) in South Texas, USA from 2017 to 2021 across four thermal partitions [below (top left) within (top right and bottom left) and above (bottom right) their thermoneutral zone]. Models included land cover type, vegetation density (vegetation points/cell), percent canopy cover, distance to dense vegetation cover (>75% canopy; m), distance to open areas (<25% canopy; m) and patch size of dense cover (>75% canopy, m2) and an interaction of cover type with vegetation density and canopy cover.
Thermal variation of microclimates
Black globe measurements of temperature across microclimates showed substantial variation between cover types. When comparing black body temperature throughout the diel cycle, temperature peaked around 14:00–15:00 across all cover types but reached a temperature approximately 10°C higher in bare ground compared to forest cover (Figure 7). Herbaceous cover showed similar thermal characteristics to bare ground while shrub cover was approximately 8°C cooler. Areas used by ocelots during extreme heat functioned similar to forest cover but appeared to warm up at a slower rate. When comparing ambient temperature to black body temperature across cover types, we found that temperature was consistent across cover types at lower temperatures but diverged substantially around 25°C (Figure 8). At temperatures over 30°C, forest and shrub cover was approximately 15°C cooler than bare ground and 10°C cooler than herbaceous cover.
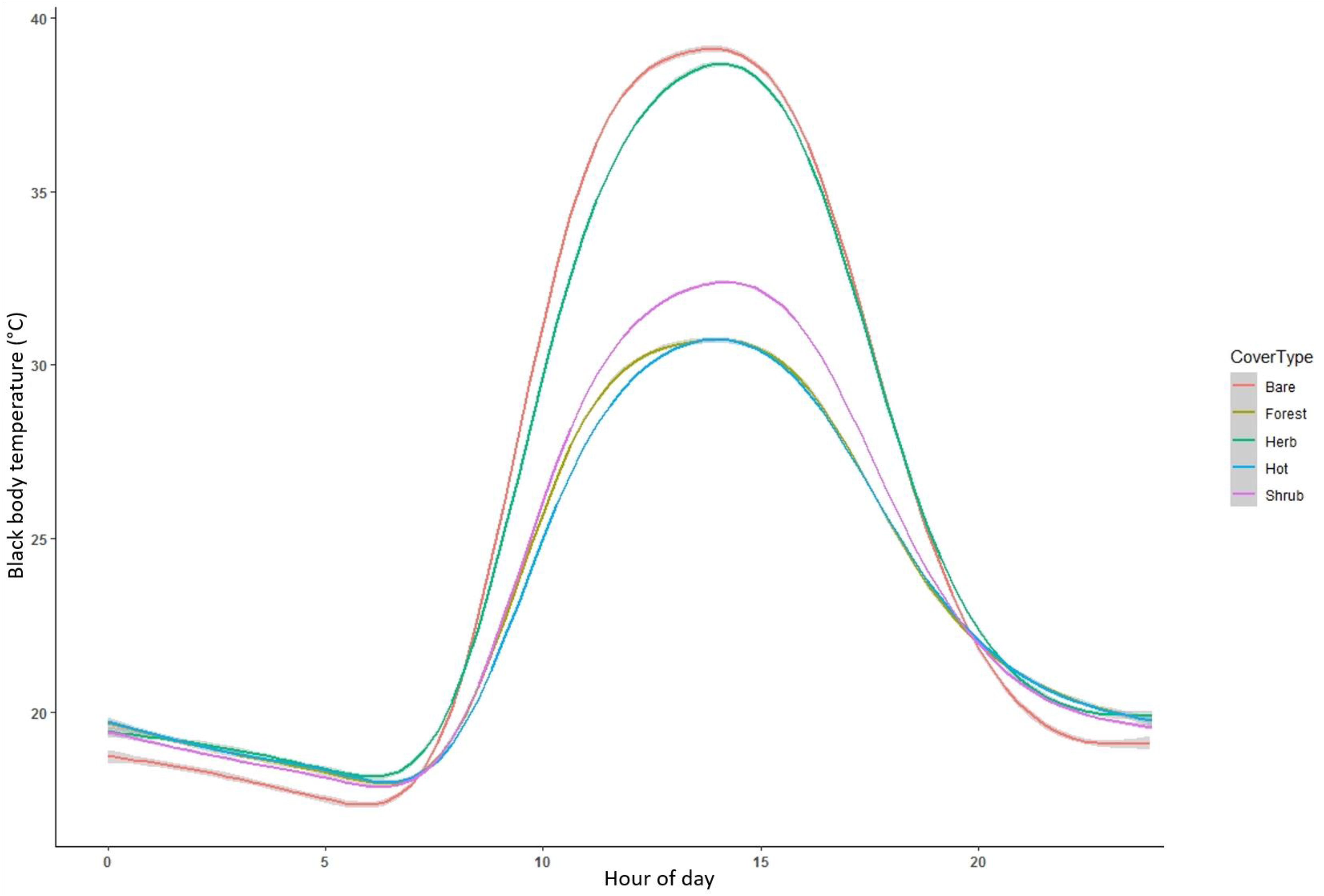
Figure 7 Black globe measurements of hourly temperature throughout the diel cycle, split by land cover type, in South Texas, USA from 2017 to 2021. Globes were positioned in wetland, bare, herbaceous, shrub, and forest cover, as well as randomly sampled locations of known use by ocelots (Leopardus pardalis) during extreme heat (>33°C, “Hot”).
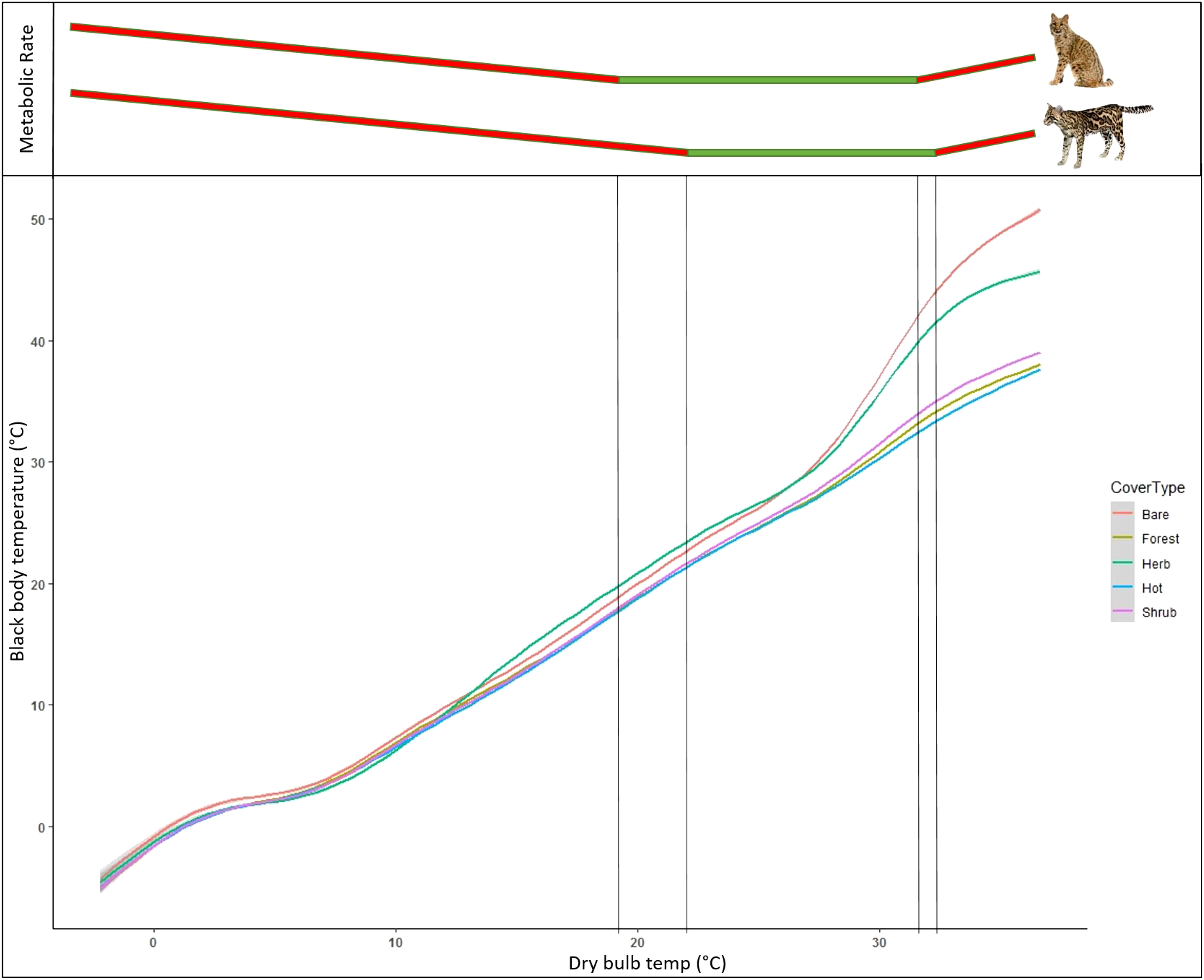
Figure 8 Ambient temperature versus black body temperature, as measured by black globe thermal sensors, split by cover type, in South Texas, USA from 2017 to 2021. Globes were positioned in wetland, bare, herbaceous, shrub, and forest cover, as well as randomly sampled locations of known use by ocelots (Leopardus pardalis) during extreme heat (>33°C, “Hot”).Top portion of graph is a conceptual representation of the change in metabolic rate of bobcats (Lynx rufus; top) and ocelots (bottom) and across temperature. Green portions denote thermoneutral zone while red are areas of cold stress (left) and heat stress (right).
Discussion
Extreme climate creates an energetically costly environment for wildlife and, as such, they may alter their habitat selection to mitigate these effects. Comparing selection across thermal conditions can identify the characteristics of thermal cover that are essential for thermoregulation. Furthermore, comparing the effect of climate across species can identify if abiotic conditions are alleviating interspecific competition for thermal resources or creating the potential for increased competition during energetically costly periods. When examining the selection of ocelots and bobcats within, above and below their thermoneutral zones, we observed extensive variation in selection across temperature by ocelots but more consistency by bobcats, suggesting bobcats may have a higher plasticity in selection while ocelots may be getting pinched into specific cover types. Overlap between species was highest when both species were heat stressed (>32/33°C) and when ocelots were cold stressed but bobcats were thermally neutral (19°C–22°C). When cold stressed, both species selected for shrub cover and greater vertical canopy cover, suggesting a high potential for interspecific competition for thermal resources; however, overlap between species was low below 19°C.
We observed differences in selection that may facilitate coexistence in that ocelots selected locations farther from open areas, whereas bobcats selected for larger patches and closer to open areas. When both species were thermally neutral, overlap between species was low; however, as ocelots began to get cold stressed, overlap between species was higher until temperature fell even lower, suggesting that there may be a threshold to how landscape structure mitigates thermal conditions. The iRLT would suggest that an ample quantity of resources on the landscape could alleviate the impact of interspecific competition (Sirén and Morelli, 2019). Ocelots and bobcats in South Texas exhibit a high degree of co-occurrence and overlap in habitat selection (Horne et al., 2009; Lombardi et al., 2020b; Sergeyev et al., 2023), potentially serving as further evidence that adequate resources exist on the landscape, to facilitate such a high degree of niche overlap. Niche theory would suggest that interspecific competition would be greatest for similar species, occurring in high densities and sharing similar food resources (MacArthur, 1968), conditions that are both present for populations of ocelots and bobcats in the region (Lombardi et al., 2022; Booth-Binczik et al., 2013). As selection between species overlapped highly during cold periods, this suggests a sufficient amount of thermal cover to reduce competition.
Habitat selection between species was highly consistent during periods of heat stress. Both species avoided bare ground in extreme heat and selected for vertical canopy cover and areas closer to heavy cover. We did observe selection for forest cover by bobcats but not ocelots, which instead selected shrub cover and large patches of dense cover, suggesting that dense shrub and forest vegetation functions as essential thermal cover for both species. Data from the black globe sensors confirm this pattern, showing differences of over 10°C across daily averages and over 15°C during extreme heat between open areas and dense vegetation, suggesting that use of these areas would have significant consequences on energy expenditure and thermal regulation of ocelots and bobcats. Habitat selection of ocelots in the context of thermal conditions has not been examined, to our knowledge. However, a preference for larger patches has been hypothesized to function as a means of mitigating extreme temperature (Shindle, 1995). Furthermore, ocelots were found to reduce activity during hotter periods (Leonard et al., 2020), suggesting varying climate can affect activity and potentially habitat selection of ocelots. Bobcats have been documented selecting for woody vegetation over grasslands during winter conditions (Kamler and Gipson, 2000; Kolowski and Woolf, 2002), consistent with our results indicating a preference for greater overstory. Furthermore, Newbury and Hodges (2019) found that bobcats had a 1.4× higher rate of metabolism during winter months, suggesting a need to mitigate the effects of an energetically costly environment. Both ocelots and bobcats were found to have a basal metabolic rate far higher than would be expected for their mass (McNab, 2000), suggesting energetic costs are high for these species even without additional stress.
As large amounts of energy are required to maintain regular bodily processes in felines, which can be greatly influenced by ambient temperature (Pereira et al., 2010), and even more so for nocturnal species (Chappell, 1980), both ocelots and bobcats can face severe stress from extreme climate and proper thermal cover is essential for the persistence of both species. The climate of South Texas can pose significant challenges to wildlife. Summer temperatures can exceed 38°C (Wiemers et al., 2014), creating an energetically costly environment. To our knowledge, this is the first study to examine habitat selection in the context of the thermal environment for ocelots or bobcats. Selection of thermal environments in Texas by northern bobwhite (Colinus virginianus; Guthery et al., 2005; Hiller et al., 2009), Texas horned lizards (Phrynosoma; Burrow et al., 2001), and white-tailed deer (Odocoileus virginianus; Wiemers et al., 2014) showed similar patterns of selection for shrub cover and taller vegetation as essential thermal cover, consistent with our results.
The iRLT posits that warm limits are dictated by competition (Sirén and Morelli, 2019), supported by our results as overlap between species was highest during high temperatures. Similarly, the iRLT suggests that cold limits are set by abiotic factors, supported by our study in that we observed the least overlap during periods of cold stress when abiotic stress was likely high. Under these circumstances, the cold adapted species will have more options in regard to habitat use while the warm adapted species is pinched into specific conditions. Coexistence between bobcats and another sympatric felid, the Canada lynx (Lynx canadensis), was examined at the interface of their respective ranges and bobcats (the more warm-adapted species) and was found to displace lynx during periods without snow (Sirén et al., 2021; Scully et al., 2018). This may suggest that, if climates continue warming, ocelots (as the more warm-adapted species) could outcompete bobcats, or conversely may highlight the competitive edge that bobcats may have over other felids. Furthermore, changing climates have the potential to shift the geographic distribution of species (Shoo et al., 2006; Saikkonen et al., 2012; HilleRisLambers et al., 2013), potentially altering areas of sympatry between ocelots and bobcats. South Texas represents the northern extent of the ocelot’s distribution (Di Bitetti et al., 2006), suggesting annual low temperatures during winters may be a limiting factor for the species. As temperatures warm, ocelots may experience range expansion northwards while bobcats could experience range contraction as temperatures become unsustainable for the species. As such, depending on the extent of the shift in range for each species, areas of sympatry between ocelots and bobcats could shift northwards and potentially increase or decrease. Furthermore, in areas with a high degree of competition that may already be acting to constrain populations, range shifts will respond to both climatic conditions and the effect of these changing conditions on competition (HilleRisLambers et al., 2013), suggesting a highly dynamic and complex process between species and both biotic and abiotic factors.
Coexistence of sympatric felids has often been examined in the context of spatial or temporal partitioning of habitat or differences in diet (Scognamillo et al., 2003; Grassman et al., 2005; Horne et al., 2009; Booth-Binczik et al., 2013; Gonzalez-Borrajo et al., 2016; Cruz et al., 2018; Lombardi et al., 2020b; Sergeyev et al., 2022); however, studies that examine habitat selection in the context of thermal conditions are fewer. Aside from the aforementioned climate-driven interactions between bobcats and Canada lynx (Scully et al., 2018; Sirén et al., 2021), snow leopards (Panthera uncia) and leopards (Panthera pardus) were found to micro-partition their habitat in central Himalaya and had higher dietary overlap higher in cold months compared to warm months, suggesting seasonal differences in habitat use and prey base with a potential for greater competition in winter months (Lovari et al., 2013). These studies highlight the potential for climate-driven interspecific competition between sympatric felids. Furthermore, they emphasize the dynamic nature of competition and how abiotic factors can influence biotic patterns such as habitat use and diet, thereby influencing competition. This dynamic nature suggests a high potential for adaptation and may help to explain some of the similarities observed between bobcats and ocelots. Although we may expect bobcats to be far more cold-adapted than ocelots based on their overall geographic distribution, ocelots and bobcats in South Texas have evolved under similar ecological constraints. As such, these two species likely overlap far more in ecology than might be expected of a bobcat at its northern limit compared to an ocelot at its southern limit, as a result of local adaptation (Joshi et al., 2001).
Our study applies a novel approach to examining coexistence between ocelots and bobcats and benefits from the use of high-frequency GPS data, high-resolution assessment of thermal microclimates and fine-scale landscape variables; yet, our study is not without its limitations. Despite a large number of locations collected from each individual, our sample size in terms of individuals collared was modest, although this is a common issue in studies on endangered carnivores. Weather data were collected at a location approximately 72 km from our study site and may potentially vary within that area; nevertheless, this variation is likely minor given the proximity and similarity in climates. Furthermore, our black globes were positioned for one full annual cycle; however, weather patterns likely fluctuated between years and this variation was uncaptured, thereby necessitating the use of the NOAA weather data to encompass the full span over which GPS data were collected. We considered thermoneutral zones based on a previous study by McNab (2000); however, the sample size for establishing these thermal limits was very small and these thermal niches likely vary substantially when comparing individuals from varying parts of their geographic range; yet, as the best available information on thermal limits of these two species, this provided some context for examining selection of thermal resources. In regard to comparing biotic interactions at range limits, we examine the interactions at one extreme for both species but lack a comparison of these two species at their other respective limit (northern limit for bobcats and southern limit for ocelots). Despite this, as the only area of sympatry between these ocelots and bobcats, South Texas provides a unique system for studying the effect of changing climate on these two ecologically similar with differing thermal adaptations.
Abiotic conditions can influence biotic interactions and the ecology of a species in a multitude of complex and dynamic ways (Sirén et al., 2020). As climates change, species may respond by altering activity or habitat selection (Tanner et al., 2017). As changes in climate continue to disrupt these processes, the potential for competition among species may increase during energetically costly periods. As such, understanding the selection of thermal cover by a species is a vital component of managing landscapes in a changing climate. Furthermore, comparing selection between species can provide a proxy for examining the potential for interspecific competition. Our study emphasizes the importance of large patches of heavy canopy cover, dense shrub, and forest canopy as thermal cover for ocelots and bobcats. We observed a high degree of overlap in habitat selection between ocelots and bobcats when heat stressed and observed greater niche partitioning when cold stressed. The presence of ample amounts of space and food resources may facilitate the high degree of overlap between species during high temperatures, while niche partitioning may reduce competition for resources during low temperatures. Our results support the iRLT in that we observed high competition at high temperatures, possibly contributing to the warm limit of ocelots, while abiotic stress may reduce competition during periods of cold stress. As climates continue to warm, ocelots and bobcats may exhibit shifts in geographic distribution, potentially leading to new areas of sympatry between species with unpredictable consequences on biotic interactions involving these species. Furthermore, as temperature extremes become more common, maintaining adequate thermal refuge for wildlife will become paramount for conservation. Management for ocelots and bobcats should ensure that larger patches of shrub and forest cover are available to provide refuge from excessive heat and dense forest canopy during periods of cold stress. Maintaining sufficient amounts of vegetation cover and managing for changing climatic conditions can increase likelihood of sustaining ocelots in Texas.
Data availability statement
Due to the sensitive nature of ocelots as an endangered species, the GPS location data used in this article are not readily available. Requests to access the data should be directed to the corresponding author. Datasets will only be made available with proper approval and permits.
Ethics statement
The animal study was approved by IACUC Texas A&M University Kingsville. The study was conducted in accordance with the local legislation and institutional requirements.
Author contributions
MS—Formal analysis, data acquisition, conceptualization, manuscript writing. ET—Conceptualization, editing, formal analysis. MC—Conceptualization, editing, formal analysis. MT—Conceptualization, project administration, funding. TC—Project administration, funding. JL—conceptualization, data acquisition, editing. All authors contributed to the article and approved the submitted version.
Funding
The author(s) declare financial support was received for the research, authorship, and/or publication of this article. Collaborative funding was provided by the East Foundation, the Feline Research Program at the Caesar Kleberg Wildlife Research Institute, the Brown Foundation, the Tim and Karen Hixon Foundation, and the United States Fish and Wildlife Service.
Acknowledgments
We would like to thank all of the technicians and research partners that made this project possible. This is manuscript number 094 of the East Foundation and manuscript 23-103 of the Caesar Kleberg Wildlife Research Institute.
Conflict of interest
The authors declare that the research was conducted in the absence of any commercial or financial relationships that could be construed as a potential conflict of interest.
Publisher’s note
All claims expressed in this article are solely those of the authors and do not necessarily represent those of their affiliated organizations, or those of the publisher, the editors and the reviewers. Any product that may be evaluated in this article, or claim that may be made by its manufacturer, is not guaranteed or endorsed by the publisher.
References
Abrahms B. (2021). Human-wildlife conflict under climate change. Science 373, 484–485. doi: 10.1126/science.abj4216
Affolter V. A., Moore P. F. (1994). Histologic features of normal canine and feline skin. Clinics Dermatol. 12, 491–497. doi: 10.1016/0738-081X(94)90215-1
Akaike H. (1973). “Information theory and an extension of the maximum likelihood principle,” in 2nd International Symposium on Information Theory (B. N. Petrov and F. Czaki, eds), Budapest. 267–281 (Akad. Kiadó).
Aujard F., Séguy M., Terrien J., Botalla R., Blanc S., Perret M. (2006). Behavioral thermoregulation in a non-human primate: effects of age and photoperiod on temperature selection. Exp. Gerontol. 41, 784–792. doi: 10.1016/j.exger.2006.06.001
Bakken G. S., Santee W. R., Erskine D. J. (1985). Operative and standard operative temperature: tools for thermal energetics studies. Am. Zool. 25, 933–943. doi: 10.1093/icb/25.4.933
Blouin-Demers G., Nadeau P. (2005). The cost-benefit model of thermoregulation does not predict lizard thermoregulatory behavior. Ecology 86, 560–566. doi: 10.1890/04-1403
Booth-Binczik S. D., Bradley R. D., Thompson C. W., Bender L. C., Huntley J. W., Harvey J. A., et al. (2013). Food habits of ocelots and potential for competition with bobcats in Southern Texas. Southwest. Nat. 58, 403–410. doi: 10.1894/0038-4909-58.4.403
Brown J. A., Robertson B. L., McDonald. T. (2015). Spatially balanced sampling: application to environmental surveys. Proc. Environ. Sci. 27, 6–9. doi: 10.1016/j.proenv.2015.07.108
Burrow A. L., Kazmaier R. T., Hellgren E. C., Ruthven D. C. III (2001). Microhabitat selection by Texas Horned Lizards in southern Texas. J. Wildl. Manage. 65, 645–652. doi: 10.2307/3803015
Chappell M. A. (1980). Thermal energetics and thermoregulatory costs of small arctic mammals. J. Mammol. 61, 278–291. doi: 10.2307/1380049
Cruz P., Iezzi M. E., De Angelo C., Varela D., Di Bitetti M. S., Paviolo A. (2018). Effects of human impacts on habitat use, activity patterns and ecological relationships among medium and small felids of the Atlantic Forest. PloS One. doi: 10.1371/journal.pone.0200806
Deutsch C. A., Tewksbury J. J., Huey R. B., Sheldon K. S., Ghalambor C. K., Haak D. C., et al. (2008). Impacts of climate warming on terrestrial ectotherms across latitude. Proc. Natural Acad. Sci. 105, 6668–6672. doi: 10.1073/pnas.0709472105
Di Bitetti M. S., Paviolo A., De Angelo C. (2006). Density, habitat use and activity patterns of ocelots (Leopardus pardalis) in the Atlantic Forest of Misiones, Argentina. J. Zool. 270, 153–163. doi: 10.1111/j.1469-7998.2006.00102.x
Elliott L. F., Treuer-Kuehn A., Blodgett C. F., Diane True C., German D., Diamond D. D. (2009–2014). Ecological Systcems of Texas: 391 Mapped Types. Phase 1 – 6, 10-meter resolution Geodatabase, Interpretive Guides, and Technical Type Descriptions (Austin, Texas: Texas Parks & Wildlife Department and Texas Water Development Board). Available at: http://www.tpwd.state.tx.us/gis/data/downloads#EMS-T.
Gonzalez-Borrajo N., López-Bao J. V., Palomares F. (2016). Spatial ecology of jaguars, pumas, and ocelots: a review of the state of knowledge. Mammal Rev. 47, 62–75. doi: 10.1111/mam.12081
Grassman L. I., Tewes M. E., Silby N. J., Kreetiyutanont K. (2005). Ecology of three sympatric felids in a mixed evergreen forest in north-central Thailand. J. Mammal. 86, 29–38. doi: 10.1644/1545-1542(2005)086<0029:EOTSFI>2.0.CO;2
Guthery F. S., Rybak A. R., Fuhlendorf S. D., Hiller T. L., Smith S. G., Puckett W. H. Jr., et al. (2005). Aspects of the thermal ecology of Bobwhites in North Texas. Wildl. Monogr. 159, 1–36. doi: 10.2193/0084-0173(2004)159[1:AOTTEO]2.0.CO;2
Haines A. M., Tewes M. E., Laack L. L., Horne J. S., Young J. H. (2006). A habitat-based population viability analysis for ocelots (Leopardus pardalis) in the United States. Biol. Conserv. 132, 424–436. doi: 10.1016/j.biocon.2006.04.035
Harveson P. M., Tewes M. E., Anderson G. L., Laack L. L. (2004). Habitat use by ocelots in South Texas: implications for restoration. Wildl. Soc. Bull. 32, 948–954. doi: 10.2193/0091-7648(2004)032[0948:HUBOIS]2.0.CO;2
Hiller T. L., Felix A. B., Guthery F. S. (2009). Association of northern Bobwhites with surface water in the semi-arid Texas panhandle. Wilson J. Ornithol. 121, 135–140. doi: 10.1676/08-033.1
HilleRisLambers J., Harsch M. A., Ettinger A. K., Ford K. R., Theobald E. J. (2013). How will biotic interactions influence climate change-induced range shifts? Ann. New York Acad. Sci. 1297, 112–125. doi: 10.1111/nyas.12182
Hofmann D. D., Cozzi G., McNutt J. W., Ozgul A., Behr D. M. (2023). A three-step approach for assessing landscape connectivity via simulated dispersal: African wild dog case study. Landscape Ecol. 38, 981–998. doi: 10.1007/s10980-023-01602-4
Horne J. S., Haines A. M., Tewes M. E., Laack L. L. (2009). Habitat partitioning by sympatric ocelots and bobcats: implications for recovery of ocelots in Southern Texas. Southwest. Nat. 54, 119–126. doi: 10.1894/PS-49.1
Hwang Y. T., Larivière S., Messier F. (2007). Energetic consequences and ecological significance of heterothermy and social thermoregulation in striped skunks (Mephitis mephitis). Physiol. Biochem. Zool.: Ecol. Evolution. Approaches 80, 138–145. doi: 10.1086/509211
IPCC (2022). Climate Change 2022: Impacts, Adaptation and Vulnerability. Contribution of Working Group II to the Sixth Assessment Report of the Intergovernmental Panel on Climate Change. Eds. Pörtner H.-O., Roberts D. C., Tignor M., Poloczanska E. S., Mintenbeck K., Alegría A., Craig M., Langsdorf S., Löschke S., Möller V., Okem A., Rama B. (Cambridge, UK and New York, NY, USA: Cambridge University Press). 3056 pp. doi: 10.1017/9781009325844
Jemifow M., Wojciechowski M., Tęgowska E. (2004). Seasonal changes in the thermoregulation of laboratory golden hamsters during acclimation to seminatural outdoor conditions. Comp. Biochem. Physiol. 139, 379–388. doi: 10.1016/j.cbpb.2004.10.007
Joshi J., Schmid B., Caldeira M. C., Dimitrakapoulos P. G., Good J., Harris R., et al. (2001). Local adaptation enhances performance of common plant species. Ecol. Lett. 4, 536–544. doi: 10.1046/j.1461-0248.2001.00262.x
Kamler J. F., Gipson P. S. (2000). Home range, habitat selection, and survival of bobcats, Lynx rufus, in a prairie ecosystem in Kansas. Can. Field-Naturalist 114, 388–394.
Kearney M. R., Jusup M., McGeoch M. A., Kooijman S. A. L. M., Chown S. L. (2021). Where do functional traits come from? role Theory models Funct. Ecol. 35, 1385–1396. doi: 10.1111/1365-2435.13829
Kolowski J. M., Woolf A. (2002). Microhabitat use by bobcats in southern Illinois. J. Wildl. Manage. 66, 822–832. doi: 10.2307/3803146
Langman V. A., Langman S. L., Ellifrit N. (2015). Seasonal acclimitization determined by non-invasive measurements of coat insulation. Zoo Biol. 34, 368–373. doi: 10.1002/zoo.21219
Laundre J. W. (2005). Puma energetics: a recalculation. J. Wildl. Manage. 69, 723–732. doi: 10.2193/0022-541X(2005)069[0723:PEAR]2.0.CO;2
Leonard J. P. (2016). Home range characteristics, activity patterns, and resource selection of sympatric ocelots (Leopardus pardalis) and bobcats (Lynx rufus) and major histocompatibility complex variation in ocelots. Dissertation, Texas A&M University-Kingsville.
Leonard J. P., Tewes M. E., Lombardi J. V., Wester D. W., Campbell T. A. (2020). Effects of sun angle, lunar illumination, and diurnal temperature on temporal movement rates of sympatric ocelots and bobcats in South Texas. PloS One 15 (4), e0231732. doi: 10.1371/journal.pone.0231732
Lombardi J. V., MacKenzie D. I., Tewes M. E., Perotto-Baldivieso H. L., Mata J. M., Campbell T. A. (2020b). Co-occurrence of bobcats, coyotes, and ocelots in Texas. Ecol. Evol. 10, 4903–4917. doi: 10.1002/ece3.6242
Lombardi J. V., Perotto-Baldivieso H. L., Tewes M. E. (2020a). Land cover trends in South Texas and potential implications for wild felids. Remote Sens. 12, 659. doi: 10.3390/rs12040659
Lombardi J. V., Perotto-Baldivieso H. L., Sergeyev M., Veals A. M., Schofield L., Young J. H. Jr., et al. (2021). Landscape structure of woody cover patches for endangered ocelots in Southern Texas. Remote Sens. 13, 4001. doi: 10.3390/rs13194001
Lombardi J. V., Sergeyev M., Tewes M. E., Schofield L. R., Neal Wilkins R. (2022). Spatial capture-recapture and LiDAR-derived vegetation metrics reveal highdensities of ocelots on Texas ranchlands. Front. Conserv. Sci. 3, 1003044. doi: 10.3389/fcosc.2022.1003044
Lovari S., Minder I., Ferretti F., Mucci N., Randi E., Pellizzi B. (2013). Common and snow leopards share prey, but not habitats: competition avoidance by large predators? J. Zool. 291, 127–135. doi: 10.1111/jzo.12053
MacArthur R. H. (1968). “The theory of the niche” in Population biology and evolution. Ed. Lewontin R. C. (Syracuse, N.Y.: Syracuse University Press), 159–176.
Mautz W. W., Pekins P. J. (1989). Metabolic rate of bobcats as influenced by seasonal temperatures. J. Wildl. Manage. 53, 202–205. doi: 10.2307/3801332
McNab B. K. (2000). The standard energetics of mammalian carnivores: felidae and hyaenidae. Can. J. Zool. 78, 2227–2239. doi: 10.1139/z00-167
Miller C. R., Barton B. T., Zhu L., Radeloff V. C., Oliver K. M., Harmon J. P., et al. (2017). Combined effects of night warming and light pollution on predator-prey interactions. Proc. R. Br. Soc. doi: 10.6084/m9
Mitchel W. A., Angilleta M. J. Jr. (2009). Thermal games: frequency-dependent models of thermal adaptation. Br. Ecol. Soc. 23, 510–520. doi: 10.1111/j.1365-2435.2009.01542.x
Morland H. S. (1993). Seasonal behavioral variation and its relationship to thermoregulation in ruffed lemurs (Varecia variegata variegata). Lemur Soc. Syst. Their Ecol. Basis, 193–194. doi: 10.1007/978-1-4899-2412-4_14
Muff S., Signer J., Fieberg J. (2019). Accounting for individual-specific variation in habitat-selection studies: efficient estimation of mixed-effects models using Bayesian or frequentist computation. J. Anim. Ecol. 89, 80–92. doi: 10.1111/1365-2656.13087
Nelson D. O., Heath J. E., Prosser C. L. (1984). Evolution of temperature regulatory mechanisms. Am. Zool. 24, 791–807. doi: 10.1093/icb/24.3.791
Newbury R. K., Hodges K. E. (2018). Regional differences in winter diet of bobcats in their northern range. Ecol. Evol. 8, 11100–11110. doi: 10.1002/ece3.4576
Newbury R. K., Hodges K. E. (2019). A winter energetics model for bobcats in a deep snow environment. J. Thermal Biol. 80, 56–63. doi: 10.1016/j.jtherbio.2019.01.006
Norwine J., Kuruvilla J. (2007). The changing climate of South Texas 1900 - 2100 - Problems and prospects, impacts and implications (Texas A&M University - Kingsville). 158 p.
Olsen B. R. L., Fulbright T. E., Hernandez F., Grahmann E. D., Wester D. B., Hehman M. W. (2018). Ground surface vs. black globe temperature in northern bobwhite resourceselection. Ecosphere 9, e02441.
Palaoro A. V., Dalosto M. M., Costa G. C., Santos S. (2013). Niche conservatism and the potential for the crayfish Procambarus clarkii to invade South America. Freshw. Biol. 58, 1379–1391. doi: 10.1111/fwb.12134
Pereira J. A., Fracassi N. G., Rago V., Ferreyra H., Marull C. A., McAloose D., et al. (2010). Causes of mortality in a Geoffrey’s cat population – a long-term survey using diverse recording methods. Eur. J. Wildl. Res. 56, 939–942. doi: 10.1007/s10344-010-0423-8
Saikkonen K., Taulavouri K., Hyvönen T., Gundel P. E., Hamilton C. E., Vänninen I., et al. (2012). Climate change-driven species’ range shifts filtered by photoperiodism. Nat. Climate Change 2, 239–242. doi: 10.1038/NCLIMATE1430
Sattar Q., Maqbool M. E., Ehsan R., Akhtar S. (2021). Review on climate and its effect on wildlife and ecosystem. Open J. Environ. Biol. 6 (1), 008–014. doi: 10.17352/ojeb.000021
Schmitz O. J. (1991). Thermal constrains and optimization of winter feeding and habitat choice in white-tailed deer. Holarctic Ecol. 14, 104–111.
Scognamillo D., Maxit I. E., Sunquist M., Polisar J. (2003). Coexistence of jaguar (Panthera onca) and puma (Puma concolor) in a mosaic landscape in Venezuelan llanos. J. Zool. Soc. London 259, 269–279. doi: 10.1017/S0952836902003230
Scully A. E., Fisher S., Miller D. A. W., Thornton D. H. (2018). Influence of biotic interactions on the distribution of Canada lynx (Lynx canadensis) at the southern edge of their range. J. Mammal. 99, 760–772. doi: 10.1093/jmammal/gyy053
Sergeyev M., Cherry M. J., Tanner E. P., Lombardi J. V., Tewes M. E., Campbell T. A. (2023). Multiscale assessment of habitat selection and avoidance of sympatric carnivores by the endangered ocelot. Sci. Rep. 13, 8882. doi: 10.1038/s41598-023-35271-9
Sergeyev M., Holbrook J. D., Lombardi J. V., Campbell T. A., Tewes M. E. (2022). Behaviorally mediated coexistence of ocelots, bobcats, and coyotes Using Hidden Markov Models. Oikos. doi: 10.1111/oik.09480
Sexton J. P., McIntyre P. J., Angert A. L., Rice K. J. (2009). Evolution and ecology of species range limits. Annu. Rev. Ecol. Evol. System. 40, 415–436. doi: 10.1146/annurev.ecolsys.110308.120317
Shindle D. B. (1995). Habitat use of ocelots in the Tamaulipan Biotic Province (Kingsville: Texas A&M University). M.S. thesis.
Shindle D. B., Tewes M. E. (1998). Woody species composition of habitats used by ocelots (Leopardus pardalis) in the Tamaulipan Biotic Province. Southwest. Nat. 43, 273–279.
Shindle D. B., Tewes M. E. (2000). Immobilization of wild ocelots with tiletamine and zolazepam in southern Texas. J. Wildl. Dis. 36, 546–550. doi: 10.7589/0090-3558-36.3.546
Shoo L. P., Williams S. E., Hero J. M. (2006). Detecting climate change induced range shifts: where and how should we be looking? Austral Ecol. 31, 22–29. doi: 10.1111/j.1442-9993.2006.01539.x
Signer J., Fieberg J., Avgar T. (2017). Estimating utilization distributions from fitted step-selection functions. Ecosphere 8, e01771. doi: 10.1002/ecs2.1771
Sirén A. P. K., Morelli T. L. (2019). Interactive range-limit theory (iRLT): an extension for predicting range shifts. J. Anim. Ecol. 89, 940–954. doi: 10.1111/1365-2656.13150
Sirén A. P. K., Sutherland C. S., Bernier C. A., Royar K. J., Kilborn J. R., Callahan C. B., et al. (2021). Abiotic stress and biotic factors mediate range dynamics on opposing edges. J. Biogeogr. 48, 1758–1772. doi: 10.1111/jbi.14112
Smith Y., Kok O. B. (2006a). Loin exposure as a means of complementing thermoregulation in Kalahari lions (Panthera leo Linneaus 1958). Pakistan J. Biol. Sci. 9, 2168–2172. doi: 10.3923/pjbs.2006.2168.2172
Smith Y., Kok O. B. (2006b). A suggested thermoneutral zone for African lions (Panthera leo Linneaus 1958) in the southwestern Kalahari, Namibia. Pakistan J. Biol. Sci. 9, 2535–2537. doi: 10.3923/pjbs.2006.2535.2537
Speights C. J., Barton B. T. (2019). Timing is everything: effects of day and night warming on predator functional traits. Food Webs 21, e00130. doi: 10.1016/j.fooweb.2019.e00130
Stelzner J. K., Hausfater G. (1986). Posture, microclimate, and thermoregulation in yellow baboons. Primates 27, 449–463. doi: 10.1007/BF02381890
Stryker J. A. (2016). Thermoregulatory Behavior Assessment and Thermal Imaging of Large Felids (University of Guelph: PhD Dissertation).
Stuart-Menteth A. C., Robinson I. S., Challenor P. G. (2003). A global study of diurnal warming using satellite-derived sea surface temperature. J. Geophys. Res. doi: 10.1029/2002JC001534
Tanner E. P., Elmore R. D., Fuhlendorf S. D., Davis C. A., Dahlgren D. K., Orange J. P. (2017). Extreme climatic events constrain space use and survival of a ground-nesting bird. Global Change Biol. 23, 1832–1846l. doi: 10.1111/gcb.13505
Terrien J., Perret M., Aujard F. (2011). Behavioral thermoregulation in mammals: a review. Front. Biosci. 16, 1428–1444. doi: 10.2741/3797
Tsonuda M. C., Buesching C. D., Macdonald D. W., Kaneko Y. (2018). Badger setts provide thermal refugia, buffering changeable surface weather conditions. J. Thermal Biol. 74, 226–233. doi: 10.1016/j.jtherbio.2018.04.005
Veals A. M., Holbrook J. D., Blackburn A., Anderson C. J., DeYoung R. W., Campbell T. A., et al. (2022). Multiscale habitat relationships of a habitat specialist over time: The case of ocelots (Leopardus pardalis) in South Texas from 1982–2017. Ecosphere. doi: 10.1002/ecs2.4204
Whittow G. C., Tazawa H. (1991). The early development of thermoregulation in birds. Physiol. Zool. 64, 1371–1390. doi: 10.1086/physzool.64.6.30158220
Wiemers D. W., Fulbright T. E., Wester D. B., Ortega-S. J. A., Rasmussen G. A., Hewitt D. G., et al. (2014). Role of thermal environment in habitat selection by male white-tailed deer during summer in Texas, USA. Wildl. Biol. 20, 47–56. doi: 10.2981/wlb.13029
Wigginton J. D., Dobson F. S. (1999). Environmental influences on geographic variation in body size of western bobcats. Can. J. Zool. 77, 802–813. doi: 10.1139/z99-037
Young T. (2010). Lions and tigers in zoos, oh my! An exploration of thermal comfort and its implications for zoo exhbit design (University of Guelph). M. S. Thesis.
Zhao F., Zhang W., Hoffmann A. A., Ma C. (2014). Night warming on hot days produces novel impacts on development, survival, and reproduction in a small arthropod. J. Anim. Ecol. 83, 769–778. doi: 10.1111/1365-2656.12196
Keywords: bobcats, climate change, habitat selection, interspecific competition, ocelots, spatial ecology, thermal ecology, range shifts
Citation: Sergeyev M, Tanner EP, Cherry MJ, Lombardi JV, Tewes ME and Campbell TA (2023) Influence of abiotic factors on habitat selection of sympatric ocelots and bobcats: testing the interactive range-limit theory. Front. Ecol. Evol. 11:1166184. doi: 10.3389/fevo.2023.1166184
Received: 14 February 2023; Accepted: 08 November 2023;
Published: 29 November 2023.
Edited by:
Piotr Nowicki, Jagiellonian University, PolandReviewed by:
Jan Janecka, Duquesne University, United StatesLevi Newediuk, University of Manitoba, Canada
Copyright © 2023 Sergeyev, Tanner, Cherry, Lombardi, Tewes and Campbell. This is an open-access article distributed under the terms of the Creative Commons Attribution License (CC BY). The use, distribution or reproduction in other forums is permitted, provided the original author(s) and the copyright owner(s) are credited and that the original publication in this journal is cited, in accordance with accepted academic practice. No use, distribution or reproduction is permitted which does not comply with these terms.
*Correspondence: Maksim Sergeyev, ecomaksimsergeyev@gmail.com
†Present address: Jason V. Lombardi, Wildlife Health Laboratory, California Fish and Wildlife Department, Rancho Cordova, CA, United States