- 1U.S. Geological Survey, Western Ecological Research Center, Reno, NV, United States
- 2Center for Innovation in Teaching and Learning, Bajada Ecology LLC, Seal Beach, CA, United States
In the Mojave Desert, timing and amounts of precipitation profoundly affect the availability of water and annual plant foods necessary for the threatened Agassiz’s desert tortoise (Gopherus agassizii) to survive, especially during prolonged droughts. As part of recovery actions to increase declining populations, we translocated 83 juvenile and young desert tortoises raised in head-start pens for 4–10 years to a new location 15 km away during the fall of 2013 and 2014. We tracked them for 9 years during a megadrought, during multiple years of low rainfall, and a few years when precipitation neared or exceeded long-term norms. We evaluated behaviors and how precipitation and forage availability affected survival. At the end of the study, 21.6% of tortoises were alive, and six had grown to adulthood. Annual models of survival indicated that tortoise size was the driving variable in most years, followed by the number of repeatedly used burrows during periods of temperature extremes. Other variables affecting survival in ≥1 year were vegetation, movements during the first 2 years post-translocation, and condition index, a measure of health. Tortoises moved more, expanded home ranges, and grew rapidly in years when winter rainfall approached or exceeded long-term norms and annual plants were available to eat. During dry years, movements and growth were limited. Exceptions to this pattern occurred in the last year of study, a dry year: tortoises grew and moved more, and home ranges increased. The increase in size and approaching adulthood may have stimulated greater traveling. Some left the study area, indicating a need for large release areas. We may have aided survival by offering water twice yearly when handling because some tortoises drank and increased in mass up to 40%. Prolonged droughts and hotter temperatures can limit the recovery of populations, reduce the survival of young tortoises, and increase the time to maturity.
1 Introduction
Throughout the globe, many species of tortoises and turtles are endangered or threatened with extinction (Rhodin et al., 2018). Agassiz’s desert tortoise (Gopherus agassizii), an iconic desert species in the American Southwest, is a federally listed, threatened species (U.S. Fish and Wildlife Service [USFWS] 1990). Numerous human activities contributed to the listing, e.g., collecting, vandalism, deaths on roads, diseases, and hyper-predation by expanding predator populations. Habitat was degraded, fragmented, or lost from livestock grazing; mining; development for agriculture, urbanization, road networks, solar energy, and utilities; military uses; invasive species and fires; and uncontrolled recreational vehicle use. Despite recovery efforts, the species continued to decline rapidly, and Allison and McLuckie (2018) reported that the desert tortoise was on the path to extinction under current conditions. In 2021, the International Union for Conservation of Nature placed G. agassizii on the Red List as critically endangered (Berry et al., 2021). Numerous anthropogenic activities and diseases resulted in continued population and habitat losses and habitat degradation (USFWS 2011, Berry and Murphy, 2019).
The latest test for survival is climate warming. According to Williams et al. (2020); Stahle (2020), and Cook et al. (2021), the worst megadrought occurred in the 16th century and the second worst from 1999 to 2020 in southwestern North America. Anthropogenic influences added to the severity. Without the anthropogenic influence, Stahle (2020:1,584) wrote: “…the 2000–2018 interval would have been just another episode of reduced precipitation, low soil moisture, and poor tree growth…” The warming temperatures drove greater aridity, including drier soil conditions, more severe droughts, and the die-off of trees (Overpeck and Udall, 2020). As climate warming continues, the American Southwest is expected to become more arid with “…widespread, prolonged, and severe dry spells and drought almost a sure bet” (Overpeck and Udall, 2020:11,857; Cook et al., 2021). More extreme heat waves and dust storms are part of the pattern.
Life in the Mojave, western Sonoran, and southern edge of the Great Basin deserts is harsh: rainfall is low and unpredictable, summer air temperatures exceed 46°C, and freezing days range from 2 to ~120 per year, depending on region (Rowlands et al., 1982; Rowlands, 1995). Desert tortoises have adaptations to survive but are vulnerable in part because of their k-selected life history traits: a long period of 17 to 20 years or more to reach sexual maturity, low fecundity, and an estimated longevity of >60 years (Woodbury and Hardy, 1948; Hardy, 1976; Turner et al., 1987; Medica et al., 2012; Berry and Murphy, 2019). Survival is low in the early years, increasing as tortoises grow from hatching to adulthood (Turner et al., 1987; Berry et al., 2020). Tortoises seek refuge in burrows, caves, and dens to avoid temperature extremes, lack of forage and moisture, and probably predators; they spend >95% of their lives underground (Woodbury and Hardy, 1948; Nagy and Medica, 1986; Henen et al., 1998).
The herbivorous tortoise depends on winter and summer rains for water for drinking and producing native annual forbs and herbaceous perennial plants for forage. Tortoises are highly selective in choosing species of plants to eat (Oftedal, 2002; Oftedal et al., 2002; Jennings and Berry, 2015). Growth occurs after emergence from brumation in late winter, if food is available, and ceases by late summer or fall (Nagy and Medica, 1986; Medica et al., 2012). Tortoises will emerge to drink with rain and when fresh forage is available (Medica et al., 1980; Henen et al., 1998).
Periods of drought and precipitation have profound effects on the physiology, health, and above-ground activities of adult desert tortoises (Henen et al., 1998; Christopher et al., 1999; Duda et al., 1999; Jennings and Berry, 2015). Drought can lead to dehydration and starvation and may have more severe effects on small, young tortoises because soft shells with developing bone and scute are vulnerable to desiccation and overheating (e.g., Berry et al., 2002; Longshore et al., 2003; Nagy et al., 2015a).
The augmentation of declining populations through head-starting and translocation was part of the revised recovery plan for the tortoise (U.S. Fish and Wildlife Service [USFWS], 2011). The International Union for Conservation of Nature (IUCN, International Union for the Conservation of Nature, Species Survival Commission, 2013) published guidelines on head-starting, translocation of animals, and conservation techniques used for endangered species. For desert tortoises, head-starting involves rearing juveniles in predator-proof pens experimentally either to learn more about early life stages or to grow them to predator-resistant sizes to augment depleted populations. Previous research indicated that survival and growth were negatively affected when rainfall was low (Berry et al., 2002; Medica et al., 2012). Existing knowledge of small tortoises involved studies of wild tortoise behaviors, use of burrows, and temperatures when active (Berry and Turner, 1984; 1986). Studies of tortoises kept in large, open pens provided data on growth, activities, survival (Nagy and Medica, 1986; Medica et al., 2012), and other traits. Research on head-started and translocated small tortoises has provided several advances in identifying important variables associated with survival (e.g., Nagy et al., 2015a; Nagy et al., 2015b; Nafus et al., 2016; Germano et al., 2017; Nagy et al., 2020). Most publications on the translocation of head-started tortoises were limited to 1–3 years.
Our overarching objective was to translocate tortoises from predator-proof head-start pens to an appropriate site, monitor behavioral responses to the megadrought for several years, and identify factors affecting survival. The tortoises were in pens from 2003 to 2014, where most experienced poor husbandry (Mack et al., 2018). Thus, the translocation began under conditions lacking the desirable protocols later published by the IUCN, International Union for the Conservation of Nature, Species Survival Commission (2013) and Swaisgood and Ruiz-Miranda (2019). We translocated tortoises in 2013 and 2014 and monitored them for 9 years. We asked four questions:
(1) Did timing and amounts of precipitation and forage availability using the normalized difference vegetation index (NDVI) affect tortoise behaviors, e.g., settling, movements and dispersal, and home ranges?
(2) Were growth and size affected by precipitation and forage availability?
(3) Was health a factor in survival?
(4) What variables affected survivorship annually and at the end of 9 years?
We addressed the questions by 1) evaluating amounts and timing of precipitation and NDVI during the study, 2) comparing behaviors with timing and amounts of rainfall and NDVI, 3) tracking size and growth during the life of each tortoise and evaluating relationships to precipitation and NDVI, and 4) modeling survival annually using multiple variables to identify important variables.
2 Study area
The study area was at Edwards Air Force Base (EAFB) in the western Mojave Desert, Kern County, California, USA. Because the tortoises were pen-raised on EAFB, a release there was a requirement. To maximize survival to adulthood, we used four criteria for site selection: an area formerly supporting a population of desert tortoises but severely reduced to one to two adults (Allison and McLuckie, 2018; Berry et al., 2020), ease of access (limited restrictions on use and surface disturbance), soils suitable for juveniles to dig burrows, and a vegetation association comparable to the head-start pens—a diverse creosote bush community with Larrea tridentata and western Joshua trees, Yucca brevifolia (Mack et al., 2018; https://www.wildlife.ca.gov/Data/VegCAMP/Natural-Communities, accessed 1 Oct 2022). We sought a site with large (2 m) creosote bushes and clones with well-developed coppice mounds where old and recently dug burrows by rodents and other animals were evident because wild juvenile tortoises select large shrubs, such as creosote bushes, for constructing burrows (Berry and Turner, 1984; 1986). We selected a 15-km2 site on the north-facing slope and alluvial fan of Leuhman Ridge at elevations of 750 to 850 m (Figure 1). Typical of the region, the site was fragmented by linear disturbances: 13.74 km of dirt roads (density of 0.91 km/km2 and in use), a fence forming the southern boundary at the base of Leuhman Ridge, a paved road on the west, and railroad tracks forming the eastern boundary. The site was within 2 km of a landfill, settlements, and military facilities. Historically, sheep grazed the area.
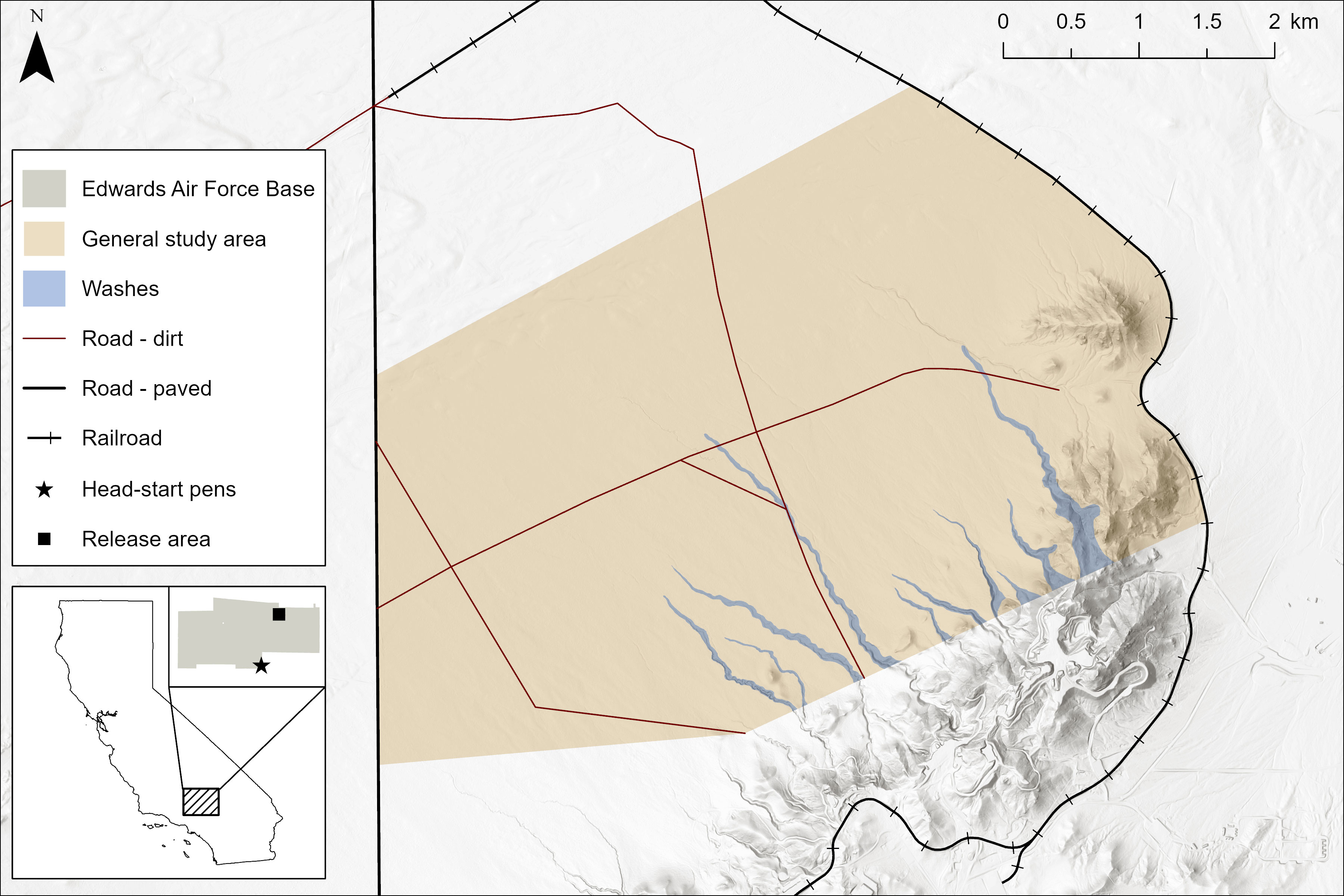
Figure 1 Locations of the head-start pens and study area where 83 small Agassiz’s desert tortoises (Gopherus agassizii) were translocated in 2013–2014 on Edwards Air Force Base in the western Mojave Desert, California, USA.
Predators likely to kill juvenile tortoises included gopher snakes (Pituophis melanoleucus), Mohave rattlesnakes (Crotalus scutulatus), antelope ground squirrels (Ammospermophilus leucurus), Mohave ground squirrels (Xerospermophilus mohavensis), loggerhead shrikes (Lanius ludovicianus), common ravens (Corvus corax), kit fox (Vulpes macrotis), and coyotes (Canis latrans). Ravens and the canids were common to abundant, supported by nearby anthropogenic sources of water, food, perches, and nest sites.
3 Materials and methods
3.1 Precipitation
In this region of the Mojave Desert, most precipitation falls between 1 Oct and 31 Mar, the fall and winter seasons, seasons with approximately 80 freezing days (Rowlands, 1995). We accessed total monthly precipitation data from the PRISM Climate Group in a 4-km grid cell raster dataset. We extracted data from the grid cell centered at latitude 34.9565, longitude −117.7047, and elevation 760 (prism_archive_ls function in package prism; Edmund et al., 2020). We calculated water year from 1 Oct to 30 Sep, and winter totals from 1 Oct to 31 Mar (Manning, 1992; Hereford et al., 2006). Our monthly series began Oct 1969 and ended Sep 2022 to allow the calculation of two long-term averages: 30 years prior to the study (1982–2012) and 30 years prior to the megadrought (1969–1999).
3.2 Vegetation
We obtained data on the NDVI through the U.S. Geological Survey’s (USGS’s) Earth Resources Observation and Science Center Science Processing Architecture On Demand Interface (U.S. Geological Survey [USGS], 2022). We used NDVI as a measure of food availability, given its strong correlation with the production of vegetation and productivity in a broad range of systems (Petorelli, 2013). We compiled Landsat NDVI and cloud cover (%) data (30-m spatial resolution, 16-day temporal resolution) from 2013 through 2022. All images were corrected following standard methods including radiometrically calibrated, orthorectified, and corrected for top of atmospheric reflectance. We extracted mean monthly NDVI values within each tortoise home range annually and derived a time series of two indices of vegetation production: mean growing season (MGS) NDVI and peak growing season (PGS) NDVI. We collected data at randomly selected sites on the composition and cover of perennial vegetation using 50 line intercepts (50 m each) during Apr 2017, a non-drought year. Also in April, the biomass of annual plants was collected from 20 randomly selected quadrats, 20 × 50 cm each from beneath shrub canopies and in intershrub spaces.
3.3 Desert tortoises
3.3.1 Histories and selection of tortoises
The tortoises were from the head-start program and pens and from cohorts hatched in summer or early fall annually between 2003 and 2010 (Mack et al., 2018; Figure 1). They were in three life stages or size classes (carapace length at the midline, MCL in mm): juvenile 1, <60; juvenile 2, 60–99; and immature 1, 100–139 (Berry and Christopher, 2001). In the fall of 2013, a few days prior to release, we removed 35 tortoises from the pens to prepare them for release. We collected metrics (mm, g) from each tortoise: MCL, carapace width at the fifth or sixth marginal scute, maximum height, and mass. The metrics were used to assess growth and calculate a body condition index developed by Nagy et al. (2002). The prime condition index was 0.64 g/cm3 (0.6–0.7 g/cm3). We also evaluated clinical signs of health and disease using a form modified by Berry and Christopher (2001). These tortoises were from cohorts hatched in 2003–2007, were 6–10 years old, and averaged ( ± SE) 85.2 ± 2.6 mm MCL (range, 69.0–132.0 mm). We repeated this process in the fall of 2014 and removed the 48 remaining tortoises from cohorts 2005, 2007–2010, and aged 4–9 years; they averaged 64.2 ± 1.5 mm MCL (range, 50.6–112.2 mm).
Prior to release, we attached radio transmitters of increasing size and lifespan as the tortoises grew larger, starting with the smaller sizes (BD-2, 2.4 g; PD-2, 3.6 g; and later, R1–2B, 10 g; Holohil Systems Ltd., Carp, Ontario, Canada). Transmitter weights were <10% of the body weights of the tortoises. Before release, tortoises were placed in individual containers in ~1.5 cm of water to drink for 15–20 min.
3.3.2 Site visits and tracking status of tortoises
Throughout the study, unless described elsewhere, we tracked and searched for live, missing, and dead tortoises monthly using two types of receivers (R2000, Advanced Telemetry Systems Inc., Isanti, MN, USA; R-1000, Communications Specialists, Inc., Orange, CA, USA), and recorded locations with Global Positioning Systems (GPS, Garmin GPS Map 62s, Garmin Ltd., Olanthe, KS, USA). Locations were accurate within the error range of the GPS (~3 m). After translocating the 2013 tortoises, we tracked them daily for 7 days, then on alternate days for 2 weeks, and then twice monthly through February. After that, the tortoises were tracked monthly. The 2014 tortoises were checked 24 h after initial release and then monitored monthly.
During each monthly visit, we recorded data on date, time (PST), weather conditions, location (Universal Transverse Mercator [UTM] system, NAD 83), details of location (whether the tortoise was above or below ground), description of the burrow (if at a burrow), and activities. If a tortoise was missing and pulses were not heard in the vicinity of known locations or from a high point in the study area, we searched previously used activity areas, nearby coyote or kit fox dens, and raven perches. If the tortoise was dead, we recorded the location (UTMs), condition of remains, and evidence for the cause of death; photographed the site and remains; and collected the remains for further analysis. We determined probable causes of death, drawing on previously described observations for clinical signs of poor health, forensic evidence of lesions from trauma (Berry and Christopher, 2001; Berry et al., 2002; Mack et al., 2018), and evidence of predation by ground squirrels and other rodents, ants, common ravens, kit foxes, and coyotes and other species (Boarman, 1993; Boarman and Berry, 1995; Berry et al., 2006; Mack et al., 2018). We used tracks, scats, the size of tooth marks, and the condition of the shell to determine the probable predator.
In spring and fall, we changed transmitters, collected data on metrics and health, took digital images (carapace, plastron, limbs, head, and posterior shell), and evaluated health and lesions from trauma or other sources. If a tortoise was deep in a burrow when the transmitter was to be changed, we tapped it to encourage emergence (Medica et al., 1980), sprayed water at the entrance to simulate rain, or returned later to determine accessibility. After handling, we offered the tortoises water to drink. From 2018 to the end of the study, we conducted more comprehensive health assessments (Berry and Christopher, 2001, updated). If the tortoise was injured, additional notes and photographs were taken. From the fall of 2019 through 2022 (end of the project), we measured the mass of tortoises before and after they were offered water to drink. When tortoises grew to >165 mm MCL, they were fitted with transmitters lasting approximately a year. Handling only occurred when ambient temperatures were ≤35°C and generally for <30 min.
3.4 Data analysis
3.4.1 Precipitation and vegetation
We compared precipitation totals for water year and winter for the study years (2013–2022) with long-term averages: 30-year norms for the 30 years prior to the study and the 30 years prior to the beginning of the megadrought in 1999 (Cook et al., 2021). We explored the linear relationship between precipitation totals (winter and water year) and measures of NDVI (MGS and PGS) using general linear models. We conducted all calculations and statistical analyses in R (version 4.1.0; R Core Team, 2021).
3.4.2 Desert tortoises
3.4.2.1 Settling behavior
We described initial settling behaviors for tortoises released in 2013, estimating the time to establish a first burrow (tortoise covered and stayed overnight), noting how many were self-dug or developed by expanding mammal or reptile burrows, dates of entering and staying in a burrow for brumation, and straight-line distances traveled from the release site to 1) first burrow and 2) burrow occupied for brumation. We calculated two measures of dispersal: 1) dispersal after 24 h and 2) dispersal after 1 year. For dispersal after 24 h, we calculated the straight-line distance from the release point to where a tortoise was located at the 24-h post-release check. For dispersal after 1 year, we calculated the straight-line distance from the release point to the center of the home range (see below) developed during the first year (Oct-to-Oct). For both releases, we compared dispersal distances using an unpaired two-sample t-test. For these analyses, we included tortoises that were alive at the end of both time intervals.
3.4.2.2 Movements and home range
We calculated the distance that a tortoise moved between observations (hereafter movements) as a straight-line distance (i.e., the minimum distance moved). We then calculated the total distance traveled as the sum of tortoise movements and average movement as the total movements divided by the number of observations.
Home ranges were estimated by fitting a minimum convex polygon (MCP) to monthly locations (mcp function in package adehabitatHR; Calenge, 2006). To calculate an area from the MCP, we removed tortoises with <3 unique locations. Traditional methods like MCP were recommended for studies of herpetofauna, even though these methods may include areas of unusable habitat or underestimate areas of habitat use (Row and Blouin-Demers, 2006; Fleming et al., 2015; Averill-Murray et al., 2020).
We compared differences in movements, home ranges, and growth between years by fitting a linear mixed-effects (LME) model that controlled for random variation between tortoises. Differences between years were explored with Tukey’s post-hoc multiple comparisons. LME models were fitted with the lme4 package (Bates et al., 2023).
3.4.2.3 Repeat burrows
We used periods of temperature extremes (i.e., fall–winter and summer) to quantify the number of repeatedly used burrows (hereafter repeat burrows) because locations recorded during these periods were likely to be below ground. To compensate for errors in GPS accuracy, we treated burrows within a 3-m radius as a single location (spDists function in package sp; Pebesma et al., 2021). We further defined a repeat burrow as one used among seasons with temperature extremes but not used during a single season within a single year.
3.4.2.4 Growth
We used carapace measurements recorded during transmitter changes to calculate growth rates. Because growth is dependent on food supply and foraging in spring, we focused on fall-to-fall measurements (i.e., water year cycle) and calculated annual growth rates for each tortoise after release until death or the end of the study. We compared growth between years with an LME model that controlled for variation between tortoises and explored differences with Tukey’s post-hoc multiple comparisons. For tortoises with a known status (i.e., live or dead) at the end of the study, we calculated an overall growth rate by fitting a linear model of size by time since release and compared overall growth rates between status with an unpaired two-sample t-test. For each tortoise smaller than the minimum adult size at the end of the study, we estimated years to reach adult size based on cohort year, years prior to release, and an average of post-release annual growth.
3.4.2.5 Status (live, dead, or missing) of tortoises and survivorship
We summarized the status and metrics for live, dead, and missing tortoises annually. We calculated death rates for single years as D/n, where D is the number of dead tortoises and n is the number of known dead and live tortoises. For annualized death rates for >1 year, we used the equation
where D is the number of dead tortoises with transmitters in a given period, n is the number of known live and dead tortoises with transmitters in a specific year, and t is the number of years. Annual or annualized survival was the reciprocal of the annual or annualized mortality rate. We summarized probable causes of death for dead tortoises. We summarized data on the status of tortoises annually.
3.4.2.6 Annual models of survival for tortoises
We identified important predictor variables of survival using generalized linear models (GLMs) with a logit link for binary outcomes. Models were run each year with cumulative data collected through December. We started modeling efforts in 2014 with 15 months of data collected for the tortoises released in October 2013. Tortoises were either removed from modeling efforts because they were missing, with unknown status, or missing a value for a variable included in the model. Only live and dead tortoises were included in each annual model.
Nine potential variables were considered for the survival models: age, size (MCL), condition index, total distance traveled, distance traveled per observation, size of home range, the total number of unique locations, number of repeat burrows, and average MGS NDVI within a tortoise home range. Before including all proposed variables, we conducted a Pearson’s correlation analysis to identify variables correlated with one another. We removed highly correlated variables to reduce redundant information and simplify the model structure without reducing the quality of prediction (Dormann et al., 2013). We removed the total distance traveled and size of the home range because of the correlation with distance traveled per observation, total number of unique locations because of the correlation with the number of repeat burrows, and age because of the correlation with size. Therefore, the final set of models considered five variables: size (MCL), mean MGS NDVI, condition index, distance traveled per observation, and number of repeat burrows. These variables created 32 main effects models with all possible variable combinations for each year.
Model fit was based on second-order Akaike’s information criterion (AICc; corrected for sample size). We identified the best-fit annual model as the model with the lowest AICc, then compared each model to the best-fit model, and ranked the models based on the difference between AICc values (ΔAICc). In addition to the best-fit model, we reported models with ΔAICc values <2 units, highlighting the most parsimonious model, or one with the simplest structure (i.e., fewest parameters) and a similar model fit (Burnham and Anderson, 2002). We also calculated additional metrics of model fit including Akaike weights and evidence ratios.
For each of the five explanatory variables, we estimated relative variable importance and model-averaged coefficients annually. Relative importance was calculated by taking the sum of the Akaike weights for all models in which a specific variable occurred. Similarly, model-averaged coefficients were calculated by averaging coefficient estimates for each variable over the models in which they occurred. We constructed the model set and ran the GLMs with the package ModelInference (version 1.70, https://www.usgs.gov/staff-profiles/mark-herzog, accessed Jul 2021). Additionally, we described two relationships with survival based on the size and number of repeat burrows by fitting separate logistic regression models. Based on model fits, we highlighted the size of tortoises and the number of repeat burrows that corresponded to a 50% level of survival.
4 Results
4.1 Precipitation and vegetation
During the study, rainfall exceeded the 30-year normal for the water year in 2 of 9 years (2018–2019 and 2019–2020) and for winter in 3 of 9 years (2016–2017, 2018–2019, and 2019–2020; Figure 2). However, amounts of precipitation reflected the megadrought. Average precipitation was 69.8% of normal for the water year (x̄ = 103.2 mm; range, 45.8–204.0 mm) and winter rain was 68.0% of normal (x̄ = 85.4; range, 38.5–163.6) when compared to the norms for the previous 30 years (water year, 147.8 mm; winter, 125.6 mm). Differences in precipitation (65.2, 65.1%) were more pronounced when totals were compared with norms from 30 years prior to the beginning of the megadrought (1999): water year and winter averages were 158.4 and 131.2 mm, respectively. Although totals of precipitation varied from the norms, timing remained consistent. Winter precipitation accounted for 82.7% of total precipitation during the study, which was comparable to 30 years prior to the study (85.0%) and prior to 1999 (82.8%).
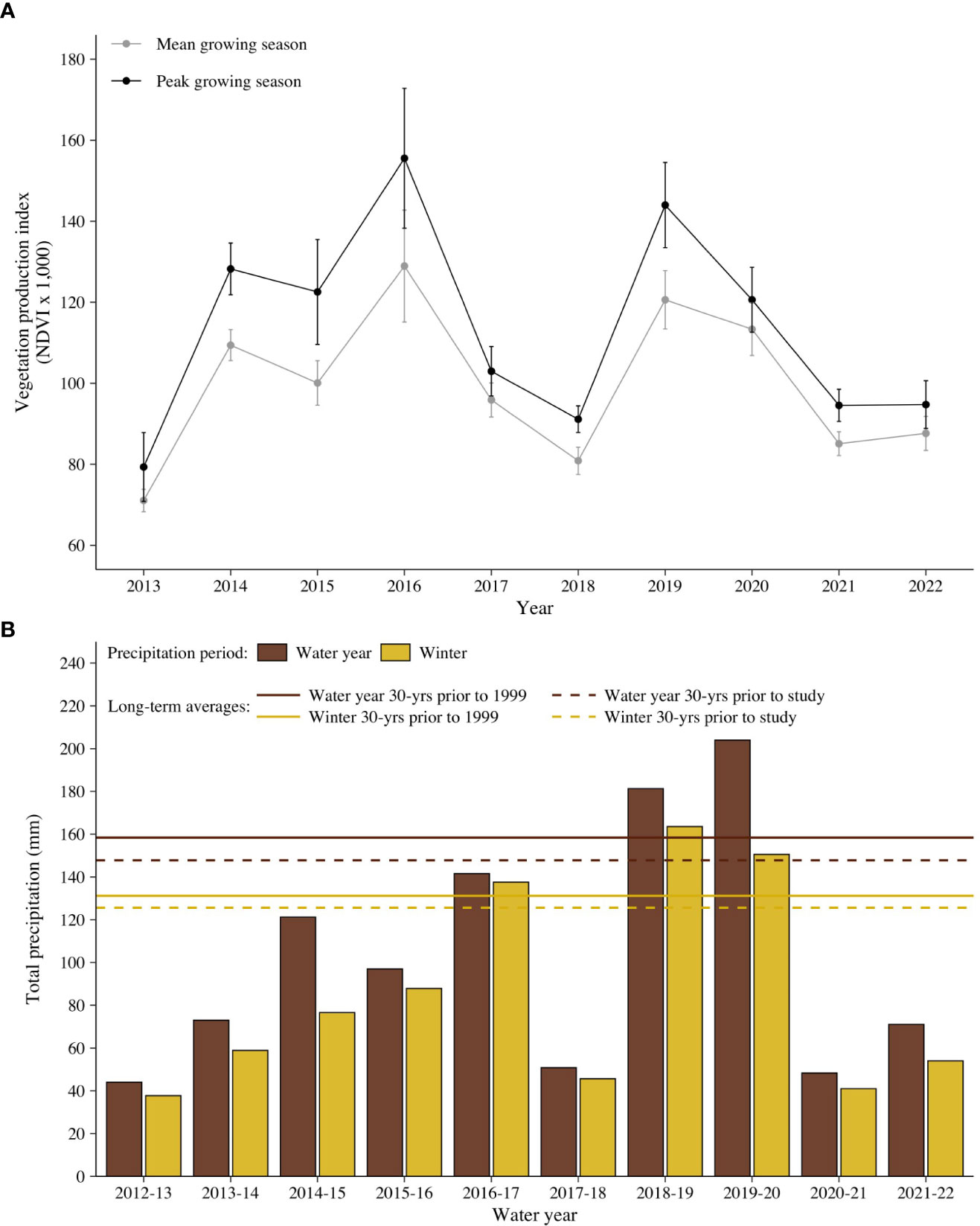
Figure 2 (A) Mean growing season and peak growing season of vegetation, part of the normalized difference vegetation index (NDVI), and (B) yearly precipitation for water year and winter precipitation for the 2013–2022 study of translocated small Agassiz’s desert tortoise (Gopherus agassizii) from head-start pens to a site on Edwards Air Force Base in the western Mojave Desert, California, USA. Norms for 30 years pre-megadrought (1999) and the 30 years prior to the study are shown.
Both vegetation indices (MGS and PGS NDVI) closely tracked precipitation (Figure 2). Overall, a linear relationship existed between precipitation and the average NDVI value within tortoise home ranges, which was significant for MGS (water year and winter: t = 2.5, df = 8, p ≤ 0.05). The average MGS value increased by 0.25 and 0.21 with a 1-mm increase in precipitation for winter and water year precipitation, respectively, and accounted for the same amount of variability (adjusted partial R2 = 0.360).
The composition of shrubs and trees was dominated by creosote bushes (76.2%) and white bur-sage (18.5%, Ambrosia dumosa). The western Joshua tree made up 2.3%, Cooper’s thornbush (Lycium cooperi) 1.2%, and cheesebush (Ambrosia salsola), California joint fir (Ephedra californica), and giant Eriastrum (Eriastrum densifolium ssp. elongatum) 0.6% each. Biomass of annual forbs and grasses averaged 107 g/m2 beneath shrub canopies and 18.1 g/m2 in the intershrub spaces. Non-native forbs (Brassica tournefortii and Erodium cicutarium) and grasses (Bromus spp., Schismus spp.) composed 23.4% of the biomass beneath canopies and 47.8% of the biomass in the intershrub spaces. During the study, African mustard (B. tournefortii) arrived along the paved road and rapidly spread into the study area.
4.2 Settling and dispersal
Between 2 Oct and 6 Dec 2013, each tortoise was observed an average (± SE) of 18.6 ± 0.4 times (range, 17.0–26.0) unless an unusual circumstance dictated otherwise. Most (58.8%, 20/34) tortoises established first burrows within ~24 h and an additional 38.2% (13/34) within 48 h. One exploring tortoise excavated its first burrow after 6 days. The average distance between the release and construction of the first burrow was 64.5 ± 19.0 m (range, 0–642.7 m). Tortoises began settling into burrows for brumation starting the day of release and continued through 17 Oct, although most settled after 6 days (58.8%, 20/34). We considered settling for brumation when the tortoise remained in a single burrow for the remainder of the fall and early winter unless rainfall caused a burrow to collapse or a tortoise emerged to drink. The average distance between release sites and burrows used for brumation was 75.8 ± 18.3 m (range, 0–603 m). Rain may have disturbed five settled tortoises in November, and they re-settled to additional burrows. Most burrows used by tortoises were modified rodent burrows, although some occupied larger mammal and reptile burrows. No rodent burrows were of sufficient size to support the three larger tortoises; overall ≥9 tortoises dug ≥1 of their own burrows. We observed one to six excavated or modified burrows for each tortoise; most were in coppice mounds under creosote bushes and camouflaged by overhanging live and dead branches and dried plants. Dispersal distances within 24 h were larger (p < 0.005) for tortoises released in 2013 (46.6 ± 7.2 m; range, 0.0–166.1 m) compared to tortoises released in 2014 (23.6 ± 3.0 m; range, 0.0–117.2). One year after release, dispersal distances were no longer different (p = 0.865) between tortoises released in 2013 (49.4 ± 6.7 m; range, 4.8–149.6) and those released in 2014 (47.5 ± 8.2 m; range, 5.5–204.6).
4.3 Movements, home ranges, and growth
Movements varied between years. Tortoises moved larger distances per observation in 2020 and 2022 compared to all other years (p < 0.05; Figure 3A). The first year of significantly larger movements (2020) followed two above-average years of precipitation and vegetation growth. Conversely, the second year (2022) followed two below-average years of precipitation (Table 1). Comparable to movements, home range size varied between years. Following the large movements in 2020 and 2022, the home range size significantly increased to its largest size in 2022 (p < 0.05; Figure 3B). Home ranges also grew consistently in size over time, even when movements were low, suggesting tortoises were exploring new areas. At the end of the study, tortoise size had a linear relationship with movement and home range, where both increased by 0.25 m and 0.001 km2, respectively, with an increase in 1-mm MCL (Figure 4; adjusted partial R2 movement = 0.454 and home range = 0.323). A marked increase in movements and home range size appeared to occur at approximately 145–150 mm MCL. Two tortoises missing at the end of the study undertook long trips and left the study area; one was last seen on a paved road >3 km to the south after moving little and having a small home range for 8 years.
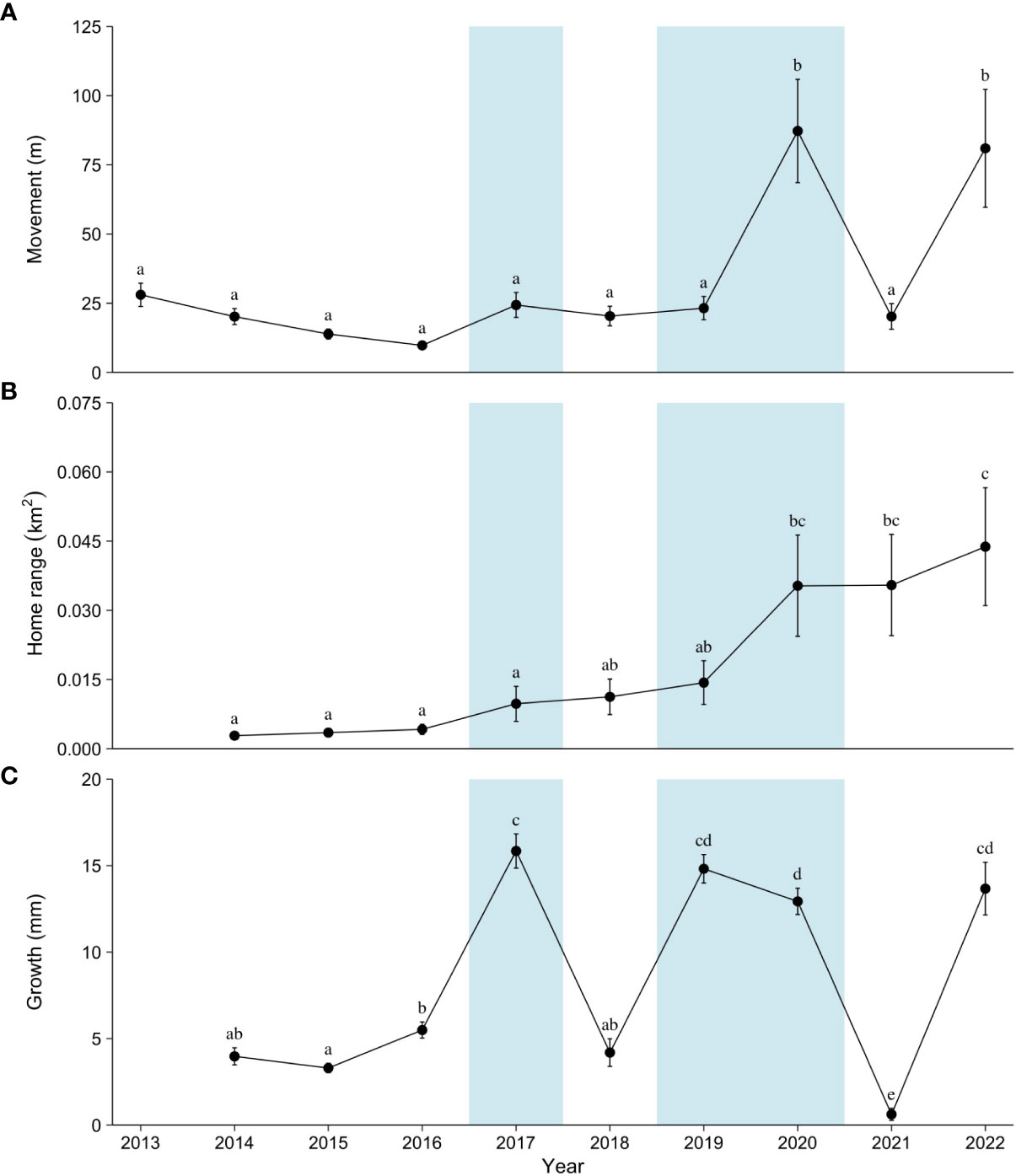
Figure 3 Annual averages of movements between observations (A), total home range (B), and growth (C) for small Agassiz’s desert tortoises (Gopherus agassizii) translocated to a study area at Edwards Air Force Base in the western Mojave Desert, California, USA. Blue boxes indicate years with precipitation above the long-term norms. Different small letters indicate significant differences in years within each figure (p < 0.05).
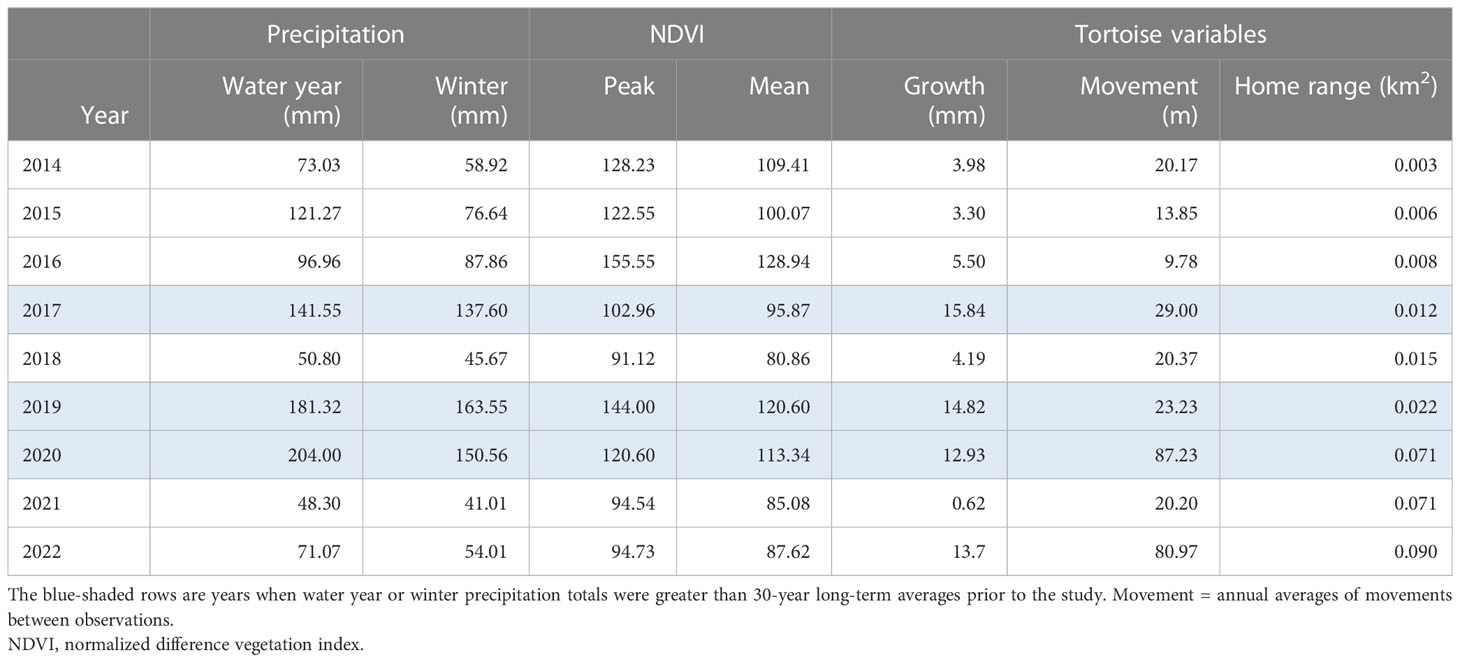
Table 1 Comparisons of yearly averages of precipitation data with growth, average movement, and home ranges of Agassiz’s desert tortoises (Gopherus agassizii) after translocation in 2013 and 2014 to a site on Edwards Air Force Base in the western Mojave Desert, California, USA.
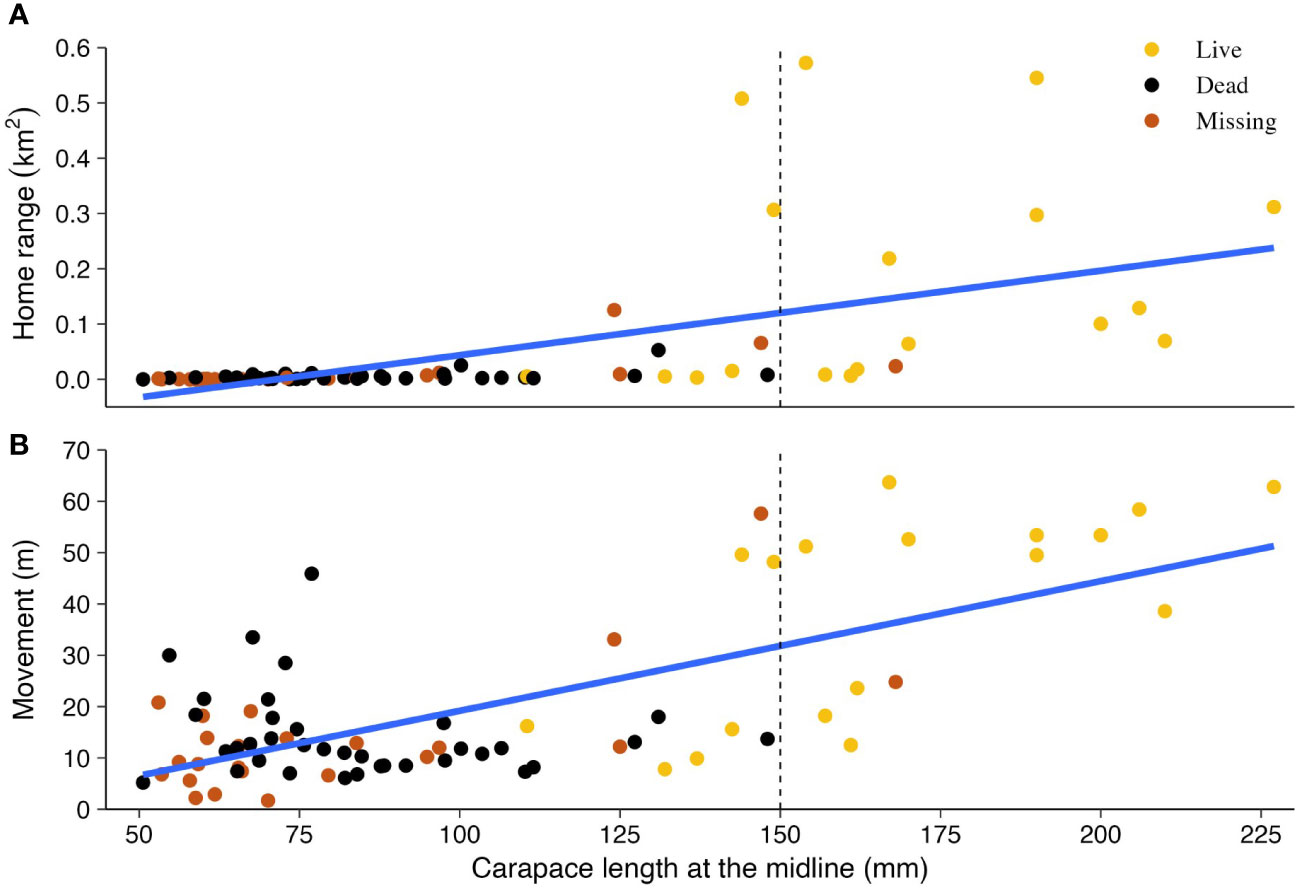
Figure 4 Annual averages of total home range (A) and movements between observations (B) by size (MCL in mm, carapace at the midline) of small Agassiz’s desert tortoises (Gopherus agassizii) translocated from head-start pens to a study area on Edwards Air Force Base in the western Mojave Desert, California, USA.
4.4 Growth
Growth varied between years and mostly tracked precipitation and vegetation patterns, except for 2022 (Table 1; Figure 3C). Growth was higher in years of above-average precipitation (2017, 2019, 2020, and 2022) compared to all other years (p < 0.05). The large growth in 2022 again suggested that tortoises had reached a critical size permitting increases in behavioral (movement) and physiological (growth) patterns. The fastest-growing tortoise, 80 mm MCL at release, grew 12.4 mm/year on average, whereas the slowest-growing tortoise (and still alive) was 92.3 mm MCL when released and averaged 6.1 mm/year. Growth rates calculated from linear model fits also showed that tortoises grew at different overall rates (Figure 5). On average, tortoises alive at the end of the study grew 9.42 mm/year (range, 5.16–12.4 mm/year), which was higher (p < 0.005) compared to those that died, which grew 4.26 mm/year (range, 0–11.6 mm/year). Differences in hatching year (cohort year) and individual growth rates resulted in differences in years to reach a minimum size at sexual maturity, 180 mm MCL. At the end of the study, six female tortoises (77.5 to 126 mm MCL at release) reached and surpassed 180 mm MCL at 14–17 years of age. The remaining live tortoises probably will require up to 25 years post-hatching to achieve minimum adult size.
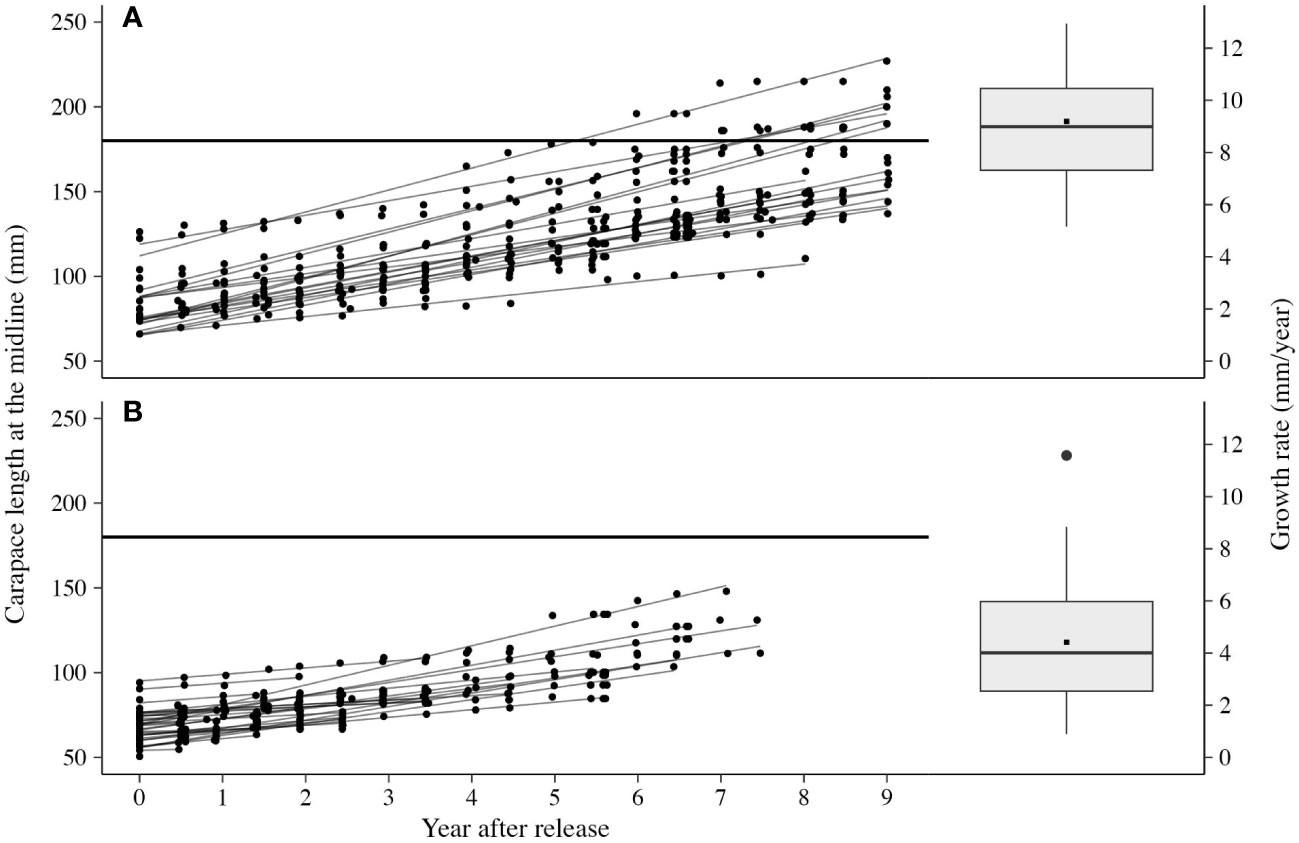
Figure 5 Growth rates and linear model fits of live (A) and dead (B) small Agassiz’s desert tortoises (Gopherus agassizii) translocated from head-start pens to a study area on Edwards Air Force Base in the western Mojave Desert, California, USA.
4.5 Hydration
Each tortoise was offered opportunities to drink after handling and responded in a variety of ways from not drinking to drinking copiously. For tortoises that drank, gains in body mass ranged from 0.97% to 39.7% and varied by year (Table 2). Tortoises drank and gained mass during years when precipitation was above the norm and during droughts. The proportions of non-drinking tortoises were similar in drought and wet years.
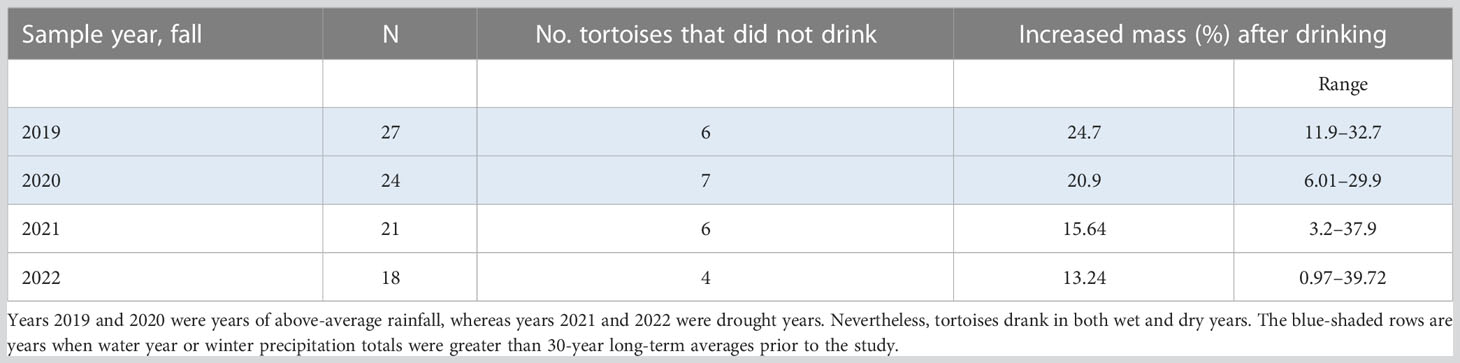
Table 2 Annual gains in mass following handling and hydration for juvenile, immature, and young adult Agassiz’s desert tortoises (Gopherus agassizii) in fall for 4 years (2019–2022) at the study site on Edwards Air Force Base in the western Mojave Desert of California, USA.
4.6 Status of tortoises
By the end of the study, 18 tortoises were alive, 41 were dead, and 24 were missing with unknown status (Figure 6). Fifteen of 18 tortoises surviving to the end of the study were <100 mm MCL when released, averaging 81.3 mm MCL (range, 61.6–99.0 mm). Annualized survivorship for all the tortoises for 2013–2022 was 92.97%; concomitantly, mortality rates were 7.03% (Table 3). When evaluated by year or groups of years, mortality rates ranged from 4.51 to 18.60%. Mortality in the 1.25 years between Oct 2014 and Dec 2015 was high (15.91%) following the translocation of the 48 remaining tortoises from the head-start pens; an additional 14 tortoises were lost during this time. Other years with high mortality rates were 2017 and 2021.
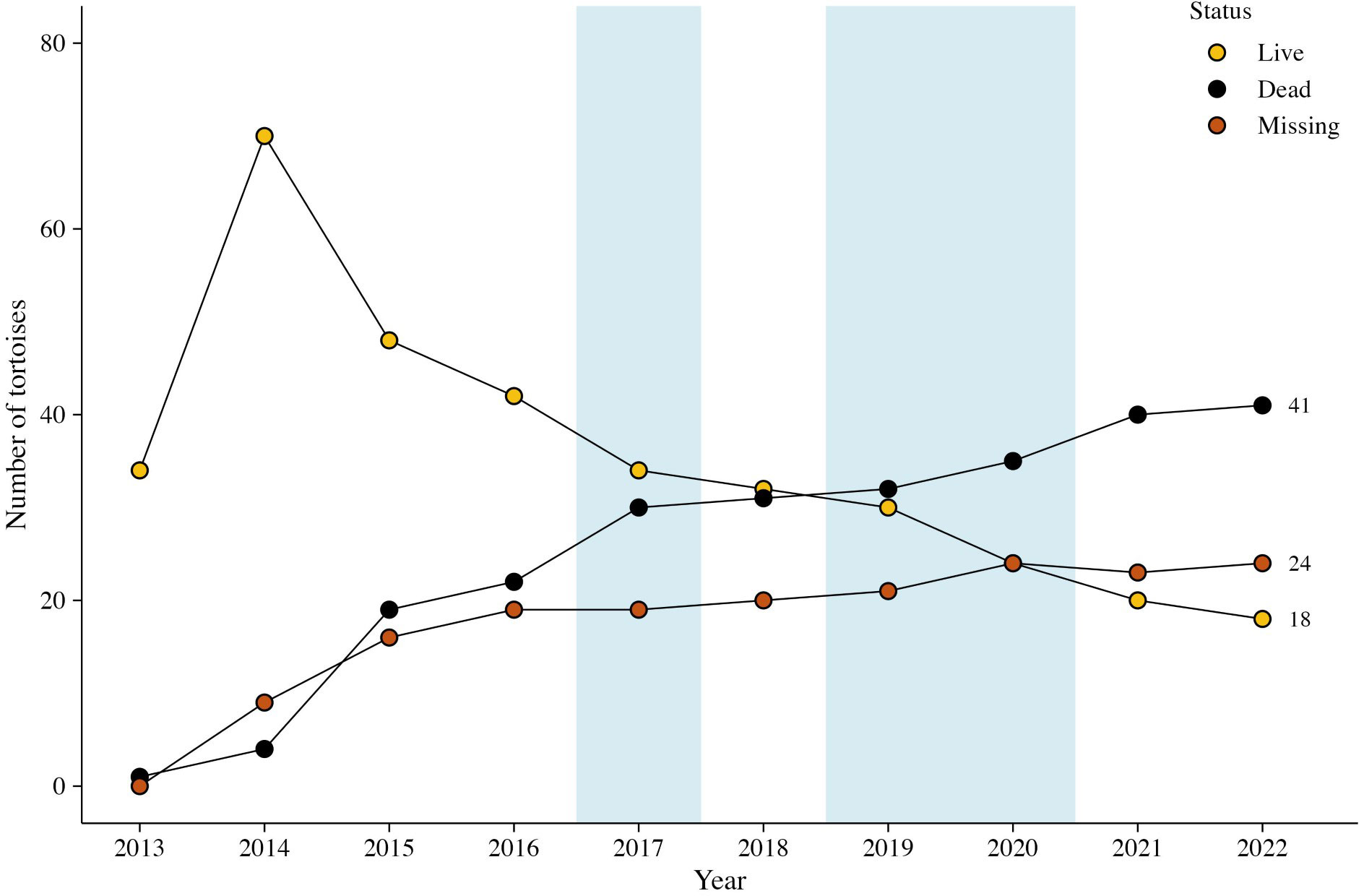
Figure 6 Status (live, dead, and missing) of small Agassiz’s desert tortoises (Gopherus agassizii) translocated from head-start pens to a study area on Edwards Air Force Base in 2013–2014 in the western Mojave Desert, California, USA. The shaded blue boxes indicate years with precipitation above the long-term norms.
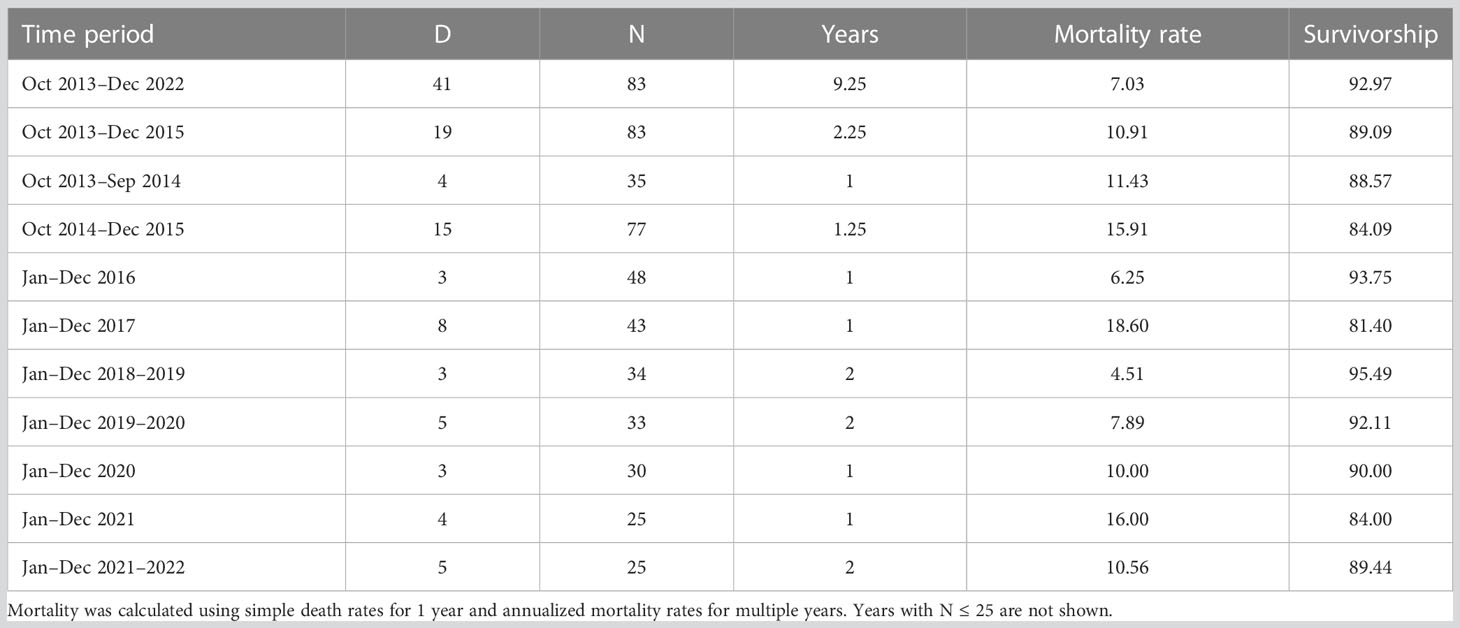
Table 3 Death rates, annualized mortality rates, and survivorship of translocated small Agassiz’s desert tortoises between 1 October 2013 and 31 December 2022 at Edwards Air Force Base in the western Mojave Desert, California, USA.
Tortoises found dead were <100 mm MCL when released, and most (36/41) were ≤100 mm MCL at death. Most missing tortoises also were <100 mm MCL when released (22/24) and when lost (Table 4). Most small missing tortoises were probably prey, carried off the study area, although some tortoises may have left the plot. Most surviving tortoises (15/18) were older when released, from 7 to 10 years old; only three were 4 to 6 years old.
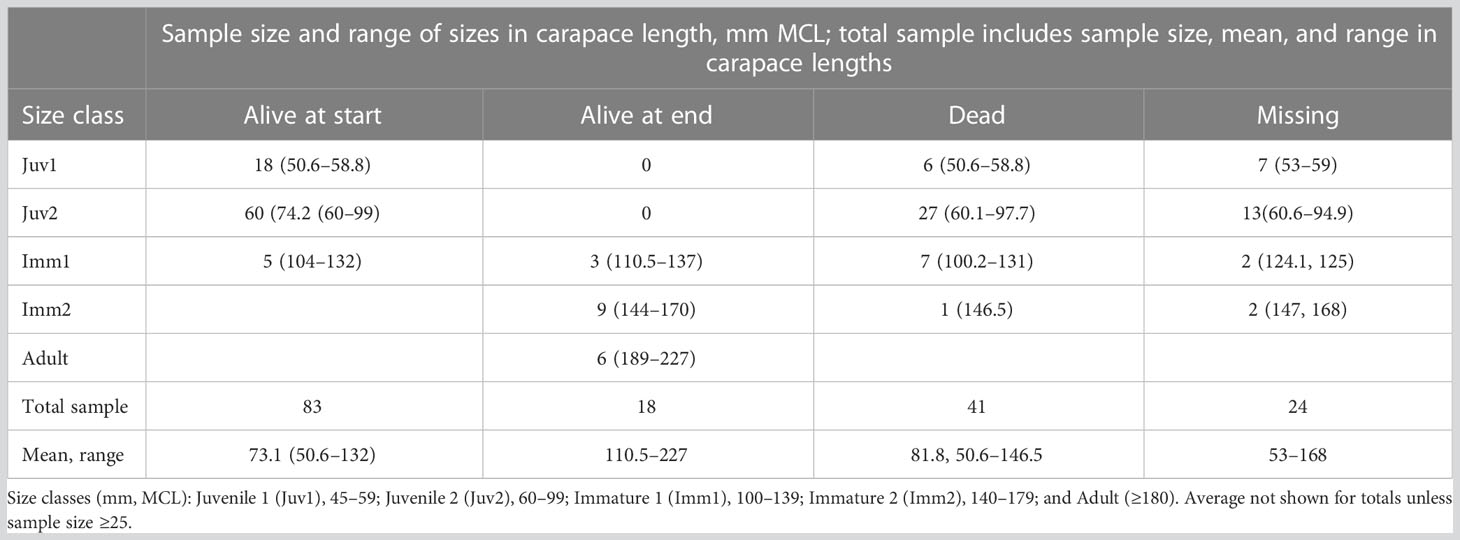
Table 4 Size classes and sizes (mm carapace lengths at the mid-line, MCL) of Agassiz’s desert tortoises (Gopherus agassizii) at release and fates of live, dead, and missing at the end of the project at Edwards Air Force Base in the western Mojave Desert, California, USA.
Probable causes of death included predation by avian and mammalian predators; a combination of starvation, dehydration, and exposure; and unknown (Table 5). For all dead tortoises, more died from predators than other causes: common ravens, 31.7% (13/41); and mesocarnivores [kit fox, coyote, and generic canid (kit fox or coyote)], 48.78% (20/41). Sizes of tortoises differed depending on the cause of death. Tortoises dying of a combination of dehydration, starvation, and exposure were the smallest and averaged 58.1 mm MCL (n = 6; range, 50.6–65.2), whereas for kills by common ravens, the mean was 74.4 mm MCL (n = 13; range, 54.7–106.5). Kills by mesocarnivores (kit fox, coyote, and canids) were larger, averaging 90.3 mm MCL (n = 21; range, 63–148). The distances that dead tortoises were found from the last known locations when alive depended on the cause of death and predator. Tortoises dying of starvation, dehydration, or exposure were at or close to the last known location when alive, x̄ = 0.8 m (n = 6; range, 0–5.8 m), whereas predators appeared to transport their prey at greater distances. On average, tortoises killed by mammals were 113.4 m (range, 3.2–1,109.2 m) distant from the last known locality, whereas those killed by common ravens were an average of 450.6 m (8.9–2,405 m) distant. More tortoises died in spring (19/41), the season when most likely to be above ground, than in summer (10/41), fall (9/41), or winter (3/41).
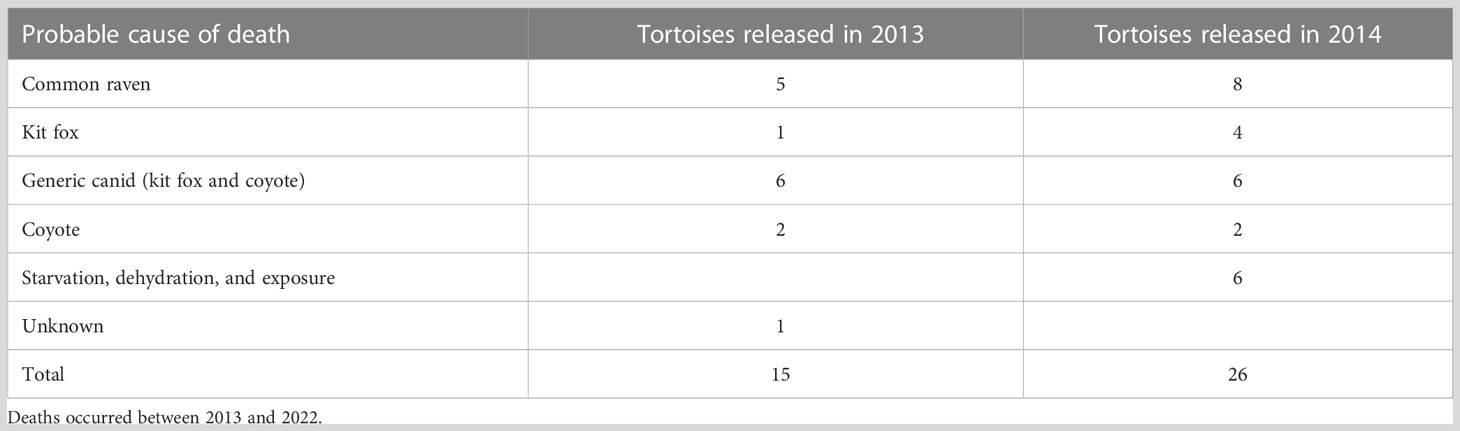
Table 5 Probable causes of death for 41 small, head-started Agassiz’s desert tortoises (Gopherus agassizii) translocated in 2013 and 2014 to the study site at Edwards Air Force Base in the western Mojave Desert, California, USA.
4.7 Annual models of survival for tortoises
The sample sizes of tortoises in the annual models of survival ranged from 31 in 2014, which included only the first release of tortoises, to 65 in 2015, after the second release of tortoises. By the end of the study, the final model included 57 tortoises (18 alive and 39 dead; Table 6). In terms of variable importance, tortoise size (MCL) ranked highest in 8 of 9 years with positive model coefficients, indicating that larger tortoises were more likely to survive (Table 7). The logistic regression model indicated that tortoises that reached a size (MCL) of 125 mm had a 50% chance of survival (Figure 7A). The importance value of repeat burrows was greater than 0.5 in 6 years of the study (2014–2017, 2019, and 2021); tortoises with more repeat burrows were more likely to survive; however, this pattern was not consistent across years, with a negative model coefficient in 2014 (Table 6). Model coefficients in this first year, however, had very high standard errors, which were likely caused by low sample sizes between live and dead tortoises (28 live and three dead) and should be interpreted with caution. The logistic regression model indicated that tortoises with approximately four repeat burrows had a 50% chance of survival, and chances of survival increased with increasing numbers of repeat burrows (Figure 7B). Additional variables with importance values ≥0.50 in one or more years were distance traveled per observation in the first 2 years, MGS NDVI in 2 years of low rainfall, and condition index in 2015, the year after 48 tortoises were released in 2014 and deaths were high (Table 7). Model fit and the number of models that performed similarly to the best-fit model (i.e., ∆AICc < 2) varied by year (Table 6). Starting in 2018, a model with size as the sole variable became the most parsimonious model.
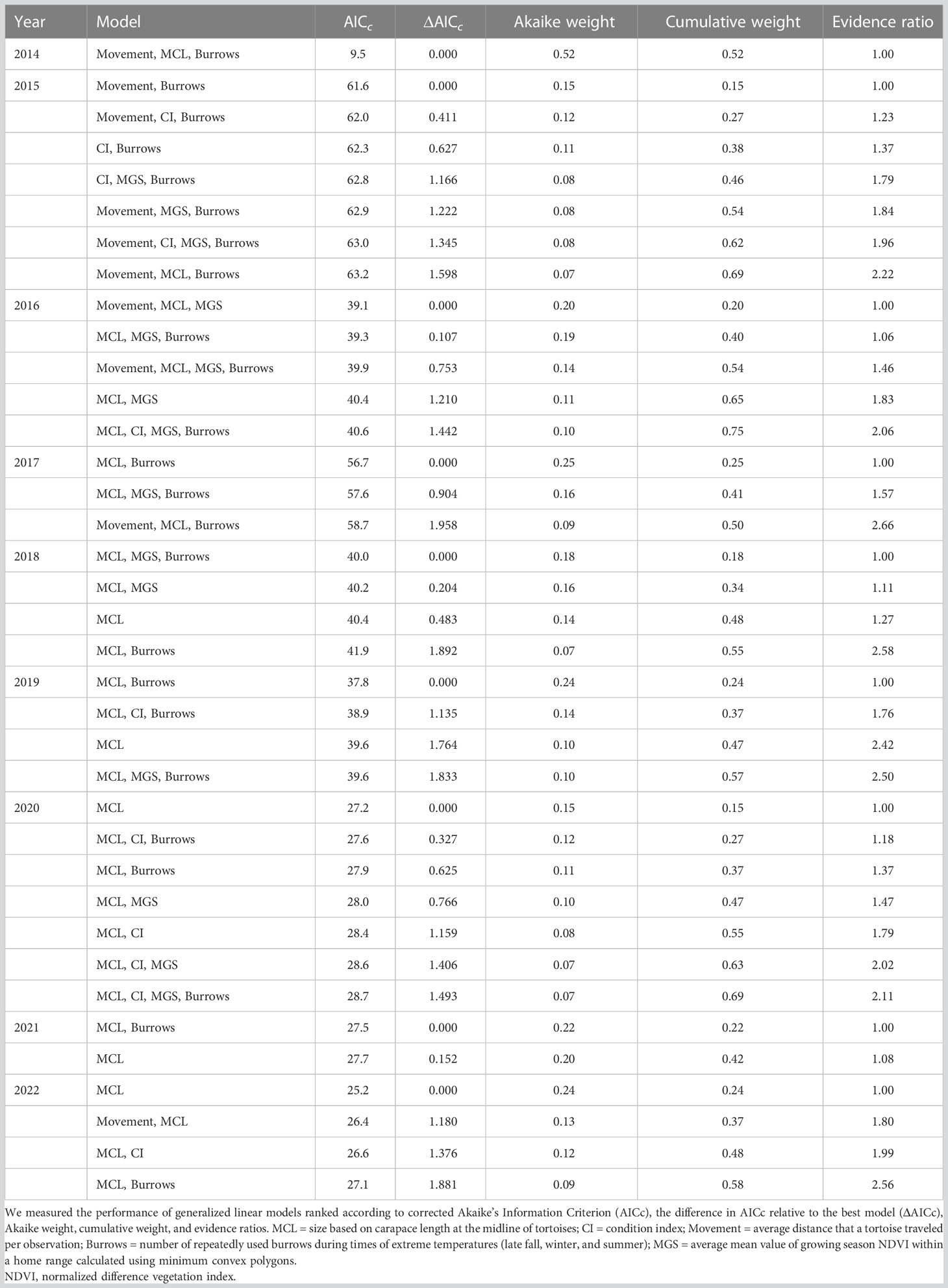
Table 6 Models for evaluating variables associated with survival of translocated juvenile and young desert tortoises raised in head-start pens at Edwards Air Force Base, California, USA.
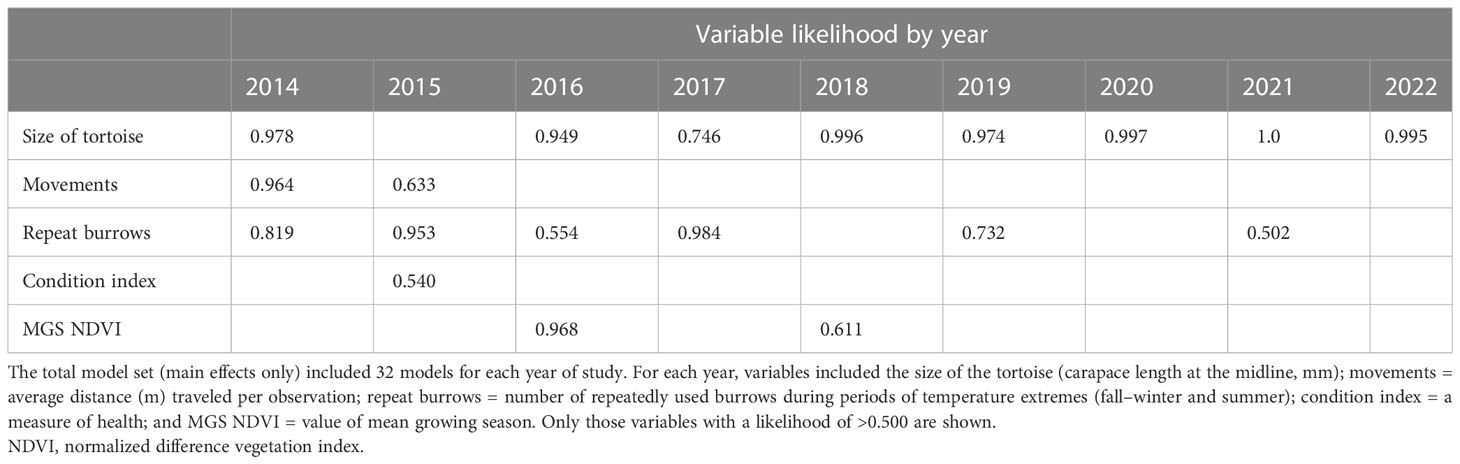
Table 7 Importance values of predictor variables used to explain the survival of small Agassiz’s desert tortoises (Gopherus agassizii) released in 2013 and 2014 at a study area on Edwards Air Force Base, in the western Mojave Desert, California, USA.
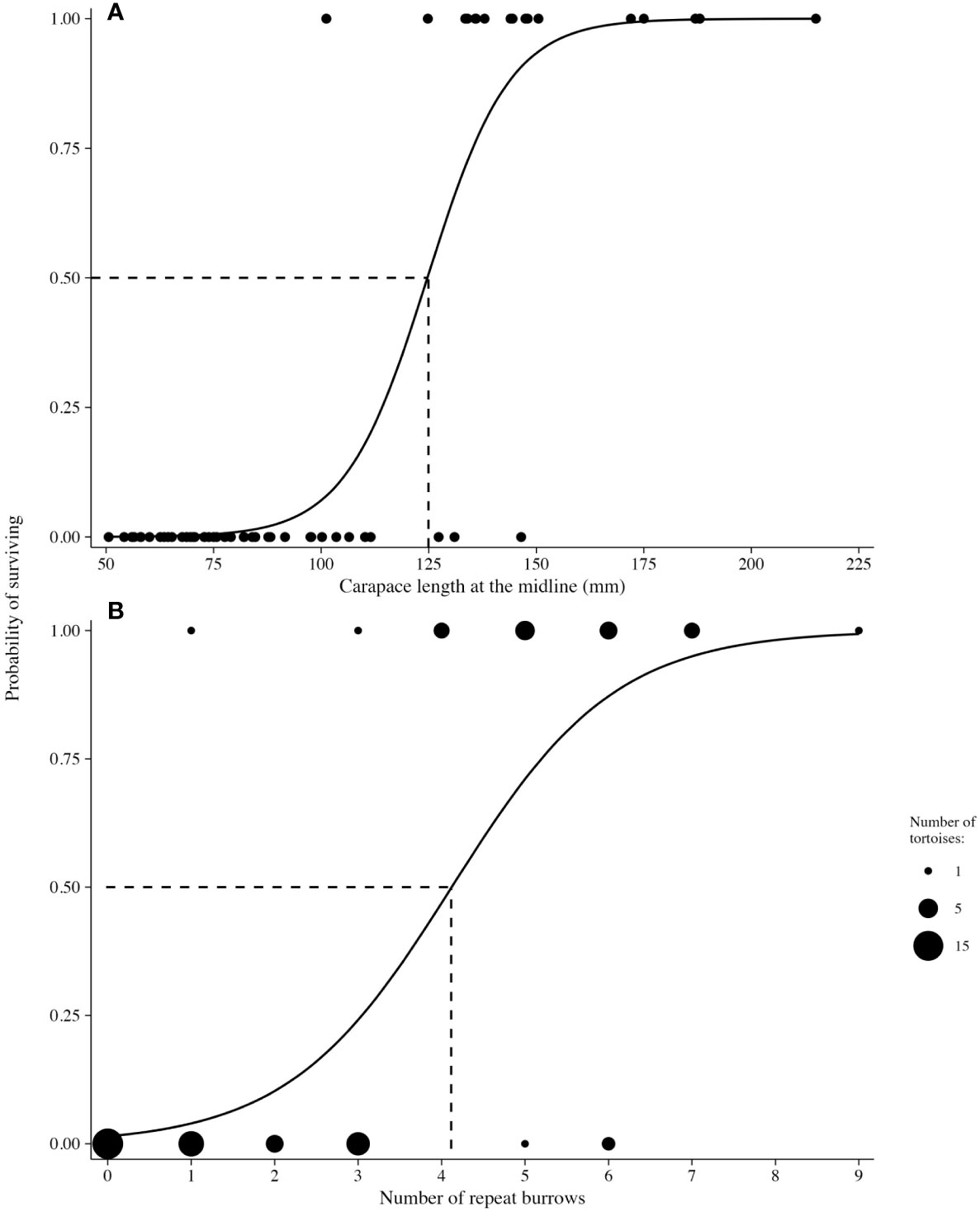
Figure 7 Probability of survival for 83 small Agassiz’s desert tortoises (Gopherus agassizii) based on (A) size (carapace length at the midline, MCL, mm) and (B) number of repeat burrows (repeatedly used burrows during periods of temperature extremes) after translocation to a site on Edwards Air Force Base in the western Mojave Desert, California, USA. Size of the tortoise was the principal driver of survival in annual models of survival, followed by repeatedly used burrows.
5 Discussion
5.1 Translocation during the megadrought and a warming climate
The megadrought resulted in prolonged years of drought with precipitation 65% to 70% below pre-megadrought and pre-study norms. The megadrought resulted in hotter air temperatures, hot droughts, evaporative losses of soil moisture, and stressed plants, presenting a bleak outlook for tortoises (Dannenberg et al., 2022). According to Cook et al. (2021), an estimated 50% chance exists for another similar drought before the end of the century. Low levels of precipitation and hotter temperatures affect almost every aspect of life for all sizes of tortoises, from the reduced cover of shrubs used for protection (Hereford et al., 2006) to health and physiology (Henen et al., 1998; Christopher et al., 1999). With this study and previous publications on adults, above-ground activities, such as movements and home range size, are limited (Duda et al., 1999), more years may be required to achieve reproductive maturity (Medica et al., 2012), and egg production is more limited (Turner et al., 1986; Turner et al., 1987; Henen, 1997; Henen, 2002a; Henen, 2002b). Higher death rates are likely for all sizes of tortoises, and juveniles in head-start pens may be especially vulnerable (Berry et al., 2002: Mack et al., 2018). More females than males may be produced, as suggested by Nagy et al. (2016), and this has been observed in other species of turtles (Roberts et al., 2023).
5.2 Survival and contributing factors
Survival of juvenile tortoises to adult sizes is essential to achieve viable populations (Congdon et al., 1993, Turner et al., 1987; Berry et al., 2020). Despite challenging circumstances for the small tortoises coupled with the megadrought, 21.7% survived. Most survivors were <100 mm MCL when released, and six reached an adult size at 14–17 years old, slightly younger than reported in a long-term study of penned tortoises in Nevada (Medica et al., 2012) but within the ages suggested for a population in the eastern Mojave Desert (Turner et al., 1987). Low rainfall and persistent drought did not appear to slow growth overall or increase the time to maturity for some survivors. The larger tortoises followed a pattern of tortoises in Nevada pens: little or no growth during drought and rapid growth when winter rains produced annual plant foods (Medica et al., 2012). Nevertheless, this pattern changed at EAFB in 2021 and 2022, both dry years: home ranges increased, and in 2022, movements, home range, and growth were comparable to those during wet years. The cause may be size-related, genetics, or physiology, with tortoises in the large immature class (140–179 mm MCL) approaching or becoming adults. Larger tortoises have more mass and nutritional resources and can travel more to obtain food. Forbs were uncommon and widely spaced in those dry years, and tortoises sought them out and ate them.
The availability of drinking water twice per year during handling may have partially offset the effects of drought. During the last 2 years of study, available drinking water possibly contributed to increased growth and movements. The added water had an important physiological function, potentially allowing tortoises to dump concentrated wastes from the bladder, refilling it with diluted urine, rehydrating, and ridding them of concentrations of waste in the blood. Consumption of dried plants could follow, thereby improving nutritional status (Peterson, 1996; Oftedal, 2002).
Models of survival indicated that size (MCL) ranked the highest among the variables, and larger tortoises were more likely to survive. Some tortoises <100 mm MCL at release survived to large immature and adult sizes, suggesting that release may be possible at smaller sizes, i.e., 75–80 mm MCL. Nagy et al, 2011; Nagy et al, 2015a; Nagy et al, 2015b) considered size and shell hardness to be vital components of decisions on timing for releases of small, head-started tortoises and suggested sizes >100 mm MCL. For 50% survival based on size, our findings suggest 125 mm MCL.
The number of repeatedly used burrows during periods of temperature extremes ranked second in model importance for survival, not surprisingly, considering the importance of burrows in the lives of adults and the fidelity that adults show to these retreats (Woodbury and Hardy, 1948; Burge, 1978; Freilich et al., 2000; Drake et al., 2015). Juvenile and immature tortoises rapidly prepared burrows during settling and showed behaviors comparable to those of adults, constructing and using burrows during temperature extremes. Burrow use also figured prominently in short-term experimental studies of translocated juvenile and immature tortoises; burrow abundance (Nafus et al., 2016) and use (Germano et al., 2017) were powerful predictors of survival. Burrows will have an important role in buffering against the increasingly hot and dry conditions forecast for the entire range of the tortoise, especially if long and deep burrows allow a reduction in metabolic rates (Nagy et al., 1997). Repeatedly used burrows were also an important component of survival for translocated adults in the central Mojave Desert in a long-term study (Mack and Berry, 2023).
Other variables with high importance values contributed to post-release survival in ≥1 year: distance traveled per observation (high in the first 2 years post translocation) and mean growing season ranked high in 2 dry years. Condition index held a high value in 2015 only, the year following the release of small tortoises in 2014 and when mortality rates were high and many tortoises disappeared.
5.3 Reducing dispersal in the future
Finding methods to anchor animals to the release site can be important for positive outcomes for translocation because excessive dispersal is associated with higher mortality rates (e.g., Field et al., 2003; Nussear et al., 2012; Swaisgood and Ruiz-Miranda, 2019). In our study, the timing of the release in early October was likely a factor in reducing dispersal distances, homing, and rejection of the release site in the short-term (Stamps and Swaisgood, 2007; Berger-Tal et al., 2020). The fall release coincided with the time that tortoises enter brumation (Woodbury and Hardy, 1948; Rautenstrauch et al., 2002; Mack et al., 2015). Comparable to the pattern for wild adults, the small tortoises settled into burrows within several days of release in October and remained until late winter, unless precipitation stimulated brief emergence (Medica et al., 1980; Henen et al., 1998).
The choice of a release site can provide an anchor for settling, particularly if the habitat is comparable to the home site. In a long-distance translocation study, Nussear et al. (2012) observed less dispersal and more rapid settling when tortoises were translocated to typical habitats. We selected a site like the head-start pens and where large creosote bushes had live and dead overhanging branches and soils contained numerous rodent burrows, evidence of easy digging. Wild tortoises of all sizes selected canopies of large shrubs to place burrows throughout much of the geographic range (Burge, 1978; Berry and Turner, 1984; 1986). Nafus et al. (2016) reported that the abundance of small mammal burrows was the most powerful predictor of survival among translocated juvenile desert tortoises in a Nevada study. However, if tortoises 70–100 mm MCL are released, some are likely to require larger rodent burrows than used by heteromyid rodents, i.e., burrows used by ground squirrels, because many rodent burrows are too small for larger juvenile and immature tortoises. The presence of rodent burrows beneath shrubs signals easy digging.
5.4 Selecting translocation sites
Locating places to translocate tortoises for recovery purposes presents major challenges because habitats are degraded and fragmented due to historic, recent, and current anthropogenic disturbances typical throughout the geographic range and generally in the West (U.S. Fish and Wildlife Service [USFWS], 1994; Leu et al., 2008; U.S. Fish and Wildlife Service [USFWS], 2011; Berry and Murphy, 2019). Importantly, substantial blocks of land, whether managed by agencies within the Departments of Defense or the Interior, have histories of uses and current commitments often limiting compatibility for recovery (e.g., U.S. Bureau of Land Management, 2019).
The USFWS (2011) has a goal of restoring tortoise populations to viability, and head-starting is one method of augmenting diminished populations. Translocated tortoises need space. Based on our study, the 15-km2 site was too small because some tortoises dispersed after reaching 145–150 mm MCL (or smaller). Depending on location, the translocation site may need fencing to reduce unauthorized uses (e.g., Berry et al., 2014a; Berry et al., 2020) or protect the tortoises from vehicle kills on paved and well-used dirt roads (von Seckendorff Hoff and Marlow 2002; Nafus et al., 2013).
Tortoise habitats will gain from the reduction of non-native grasses and harmful non-native forbs (e.g., African mustard). Grasses, whether native or non-native, are poor forage and harmful for juveniles and all sizes of tortoises if forbs are not consumed as part of the diet (Hazard et al., 2009; 2010; Drake et al., 2016). Much of the geographic range, including the study area on EAFB, has invasive, non-native annual grasses, and African mustard has rapidly invaded parts of the Mojave Desert (Brooks and Berry, 2006; Minnich and Sanders, 2000). Non-native, annual grasses are flammable and have contributed to severe damage from fires to critical habitat units for the tortoise, particularly in the central, eastern, and northeastern Mojave Desert regions (Brooks and Matchett, 2006; Klinger et al., 2021). Non-native grasses and forbs effectively compete with the native forbs preferred by tortoises (Brooks, 2000; Brooks, 2003; Berry et al., 2014b; Jennings and Berry, 2015). The situation is acute during drought years because non-native species (e.g., Schismus spp., Bromus spp.) composed 91% of the biomass in dry years in the western Mojave (Brooks and Berry, 2006). Production of annual forbs following winter rains is essential for the growth of small tortoises: forbs provide energy, nutrients, and minerals (Nagy and Medica, 1986; Hazard et al., 2009; Hazard et al., 2010). The altered food supply, when coupled with prolonged years of drought, reduces the capacity of juveniles to grow and increases the time to reach sexual maturity. Habitat restoration is likely essential to the recovery of tortoise populations (Abella et al., 2023).
5.5 Hyper-predation by ravens and coyotes
Reductions in predators that kill tortoises would benefit the survival of small, translocated tortoises. Populations of ravens and coyotes have grown in the Mojave Desert because of subsidies from anthropogenic sources of food and water and, for ravens, the addition of nest and perch sites (Kristan and Boarman., 2003; Esque et al., 2010; Cypher et al., 2018). At EAFB, the two species of predators benefitted locally from animal kills on nearby roads and highways, trash, and other food and water sources available from nearby settlements and on-base housing, military offices and facilities, and perches in Joshua trees and cultivated trees. These predators were the sources of most deaths of tortoises in our study and other projects (e.g., Nagy et al., 2015b). Recent efforts to reduce the population growth of ravens throughout the Mojave Desert in California have focused on lethal measures and oiling of eggs (e.g., U.S. Fish and Wildlife Service [USFWS] 2008; Shields et al., 2019), but neither measure occurred on EAFB during the time frame of the study (W.E. King, 412 CEG/CEVA, EAFB, personal communication, 2 Feb 2023).
6 Implications
The translocation of head-started tortoises began under difficult circumstances because of several factors, including the condition of tortoises to be translocated (Mack et al., 2018). In the future, head-starting projects would benefit from guidelines in IUCN, International Union for the Conservation of Nature, Species Survival Commission (2013) and Swaisgood and Ruiz-Miranda (2019). These publications provide roadmaps to successful projects, especially when a team of knowledgeable and committed parties and multiple government agencies can be assembled in advance of project initiation and meet frequently as conditions warrant.
Climate change, with increasing temperatures, megadroughts, and more hot and dry years forecasted, poses new challenges to survival for tortoises, especially when existing and projected climate variations are coupled with long-term habitat degradation. Although tortoises have physiological and behavioral adaptations for living in deserts, these adaptations may be insufficient during prolonged droughts. For small tortoises, deeper burrows with more soil cover and longer tunnels may allow greater protection from increasing temperatures and declining soil moisture but may be beyond the capacity of small tortoises to dig. Experimental testing of pre-dug, deep burrows, and drinking water is another option, especially when associated with telemetered individuals.
Protection and restoration of habitats and controlling subsidized predators are potential routes forward. Improvements in head-start methods (e.g., Nagy et al., 2020) with translocations delayed until juveniles are ≥70–100 mm MCL are likely to aid in higher survival. Fall translocations to appropriate sites can allow for rapid construction of burrows, settling, and brumation. Fall releases result in tortoises moving underground, whereas spring releases contribute to above-ground activity and likely predation. Release sites of high quality (large, protected from ongoing and future surface disturbances) could enhance survival. Future options include the exploration of sites beyond the current distributional limits in the northern Mojave at higher elevations. Desirable sites likely to benefit the tortoise would be free of historic and recent livestock grazing, feral burros, horses, mining, and other developments, especially if those areas support food plants preferred by the tortoises.
Data availability statement
The senior author will need to obtain permission from the U.S. Air Force base at Edwards, California, before sharing the data. Research is continuing on the remaining live study animals by Dr. Ron Swaisgood’s team at the San Diego Zoo Wildlife Alliance, California and he should also be contacted, at least to approve sharing. Requests to access the datasets should be directed to kristin_berry@usgs.gov.
Ethics statement
The animal study was reviewed and approved by U.S. Fish and Wildlife Service Endangered Species Permits. We worked under USFWS Permits for Endangered Species and the study plan received multiple reviews. All permit numbers are listed in the paper.
Author contributions
The authors are listed in order of the level of contributions. KB is first, JM is second, and KA is third. All authors contributed to the article and approved the submitted version.
Funding
Edwards Air Force Base provided funds to the U.S. Geological Survey for the project.
Acknowledgments
We thank D. Essary, R. Newman, T. Shields, A. Spenceley, and M. Tuma for assistance in the field; M. Tuma for assistance with two reports; R. Klinger for assistance with NDVI; R. Swaisgood for sharing plant data; and R. Swaisgood, D. Elam, and three reviewers for constructive reviews. We appreciated the support of T. Mull early in the project and W. King, who managed long-term monitoring; both were employees of EAFB. Handling during translocation and subsequent monitoring were under permits from the U.S. Fish and Wildlife Service: TE-32004C-0-2, TE-87850B-0, TE-45827D-0, and TE-46010D-0. Any use of trade firm or product names is for descriptive purposes only and does not imply endorsement by the U.S. government.
Conflict of interest
Author KA was employed by the company Bajada Ecology LLC.
The remaining authors declare that the research was conducted in the absence of any commercial or financial relationships that could be construed as a potential conflict of interest.
Publisher’s note
All claims expressed in this article are solely those of the authors and do not necessarily represent those of their affiliated organizations, or those of the publisher, the editors and the reviewers. Any product that may be evaluated in this article, or claim that may be made by its manufacturer, is not guaranteed or endorsed by the publisher.
References
Abella S. R., Berry K. H., Ferrazzano S. (2023). Techniques for restoring damaged Mojave and western Sonoran habitats, including those for threatened desert tortoises and Joshua trees. Desert Plants 38, 4–52.
Allison L. J., McLuckie A. M. (2018). Population trends in Mojave desert tortoises (Gopherus agassizii). Herpetol. Conserv. Biol. 13, 433–452.
Averill-Murray R. C., Fleming C. H., Riedle J. D. (2020). Reptile home ranges revisited: a case study of space use of Sonoran desert tortoises (Gopherus morafkai). Herpetol. Conserv. Biol. 15, 253–271.
Bates D., Maechler M., Bolker B., Walker S., Christensen R. H. B., Singmann H., et al. (2023) lme4: linear mixed-effects models using ‘‘Eigen’’ and S4. Available at: https://cran.r-project.org/package=lme4 (Accessed Jun 2023).
Berger-Tal O., Blumstein D. T., Swaisgood R. R. (2020). Conservation translocations: a review of common difficulties and promising directions. Anim. Conserv. 23, 121–131. doi: 10.1111/acv.12534
Berry K. H., Allison L. J., McLuckie A. M., Vaughn M., Murphy R. W. (2021). Gopherus agassizii, Mojave Desert tortoise. The International Union for Conservation of Nature Red List of Threatened Species™ (IUCN 2021). Available at: https://www.iucnredlist.org/species/97246272/3150871 (Accessed July 14, 2023).
Berry K. H., Bailey T. Y., Anderson K. M. (2006). Attributes of desert tortoise populations at the National Training Center, central Mojave Desert, California, USA. J. Arid Environ. 67 (Supplement), 165–191. doi: 10.1016/j.jaridenv.2006.09.026
Berry K. H., Christopher M. M. (2001). Guidelines for the field evaluation of desert tortoise health and disease. J. Wildl. Dis. 37, 427–450. doi: 10.7589/0090-3558-37.3.427
Berry K. H., Gowan T. A., Miller D. M., Brooks M. L. (2014b). Models of invasion and establishment for African mustard (Brassica tournefortii). Invasive Plant Sci. Manage. 7, 599–616. doi: 10.1614/IPSM-D-14-00023.1
Berry K. H., Lyren L. M., Yee J. L., Bailey T. Y. (2014a). Protection benefits desert tortoise (Gopherus agassizii) abundance: the influence of three management strategies on a threatened species. Herpetological Mongr. 28, 66–92. doi: 10.1655/HERPMONOGRAPHS-D-14-00002
Berry K. H., Murphy R. W. (2019). Gopherus agassizii (Cooper 1861) – Mojave Desert Tortoise, Agassiz’s Desert Tortoise. Chelonian Res. Monogr. 5, 1–45. doi: 10.3854/crm.5.109.agassizii.v1.2019
Berry K. H., Spangenberg E. K., Homer B. L., Jacobson E. R. (2002). Deaths of desert tortoises following periods of drought and research manipulation. Chelonian Conserv. Biol. 4, 436–448.
Berry K. H., Turner F. B. (1984). “Notes on the behavior and habitat preferences of juvenile desert tortoises (Gopherus agassizii) in California,” in Proc. Ninth Ann. Desert Tortoise Council Symp (Lake Havasu City, AZ, USA: The Desert Tortoise Council), 111–130. Available at: https://deserttortoise.org (Accessed January 2, 2023).
Berry K. H., Turner F. B. (1986). Spring activities and habits of juvenile desert tortoises, Gopherus agassizii, in California. Copeia 4), 1010–1012. doi: 10.2307/1445302
Berry K. H., Yee J. L., Shields T. A., Stockton L. (2020). The catastrophic decline of tortoises at a fenced Natural Area. Wildl. Monogr. 205, 1–53. doi: 10.1002/wmon.1052
Boarman W. I. (1993). “When a native predator becomes a pest: A case study,” in Conservation and Resource Management. Eds. Majumdar S. K., Miller E. W., Baker D. E., Brown E. K., Pratt J. R., Schmalz R. F. (Easton, PA: The Pennsylvania Academy of Science), 191–206.
Boarman W. I., Berry K. H. (1995). “Common ravens in the southwestern United States 1968–92,” in Our Living Resources. A Report to the Nation on the Abundance, and Health of U.S. Plants, Animals, and Ecosystems. Eds. LaRoe E. T., Farris G. S., Puckett C. E., Doran P. D., Mac M. J. (Washington, DC: U.S. Department of the Interior, National Biological Service), 73–75.
Brooks M. L. (2000). Competition between alien annual grasses and native annual plants in the Mojave Desert. Am. Midl. Nat. 144, 92–108. doi: 10.1674/0003-0031(2000)144[0092:CBAAGA]2.0.CO;2
Brooks M. L. (2003). Effects of increased soil nitrogen on the dominance of alien annual plants in the Mojave Desert. J. Appl. Ecol. 40, 344–353. doi: 10.1046/j.1365-2664.2003.00789.x
Brooks M. L., Berry K. H. (2006). Dominance and environmental correlates of alien annual plants in the Mojave Desert, USA. J. Arid Environ. 67 (Supplement), 100–124. doi: 10.1016/j.jaridenv.2006.09.021
Brooks M. L., Matchett J. R. (2006). Spatial and temporal patterns of wildfires in the Mojave Deser–2004. J. Arid Environ. 67 (Supplement), 148–164. doi: 10.1016/j.jaridenv.2006.09.027
Burge B. L. (1978). “Physical characteristics and patterns of utilization of cover sites used by Gopherus agassizi in southern Nevada,” in Proc. 3rd Ann. Desert Tortoise Council Symp (Las Vegas, Nevada, USA: The Desert Tortoise Council), 80–111. Available at: https://desertortoise.org (Accessed January 2, 2023).
Burnham K. P., Anderson D. R. (2002). Model Selection and Multimodel Inference: a Practical Information-theoretic Approach. 2nd ed. (New York: Spring Science Business Media).
Calenge C. (2006). The package adehabitat for the R software: tool for the analysis of space and habitat use by animals. Ecol. Modell. 197, 1035. doi: 10.1016/j.ecolmodel.2006.03.017
Christopher M. M., Berry K. H., Wallis I. R., Nagy K. A., Henen B. T., Peterson C. C. (1999). Reference intervals and physiologic alternations in hematologic and biochemical values of free-ranging desert tortoises in the Mojave Desert. J. Wildl. Dis. 35, 212–238. doi: 10.7589/0090-3558-35.2.212
Congdon J. D., Dunham A. E., Van Loben Sels R. C. (1993). Delayed sexual maturity and demographics of Blanding’s turtles (Emydoidea blandingii): implications for conservation and management of long-lived organisms. Conserv. Biol. 7, 826–833. doi: 10.1046/j.1523-1739.1993.740826.x
Cook B. I., Mankin J. S., Williams A. P., Marvel K. D., Smerdon J. E., Liu H. (2021). Uncertainties, limits, and benefits of climate change mitigation for soil moisture drought in southwestern North America. Earth’s Future 9, e2021ER002013. doi: 10.1029/2021EF002014
Cypher B. L., Kelly E. C., Westall T. L., Van Horn Job C. L. (2018). Coyote diet patterns in the Mojave Desert: implications for threatened desert tortoises. Pac. Conserv. Biol. 24, 44–54. doi: 10.1071/PC17039
Dannenberg M. P., Yan D., Barnes M. L., Smith W. K., Johnston M. R., Scott R. L., et al. (2022). Exceptional heat and atmospheric dryness amplified losses of primary production during the 2020 U.S. Southwest hot drought. Global Change Biol. 22, 4794–4806. doi: 10.111/gcb.16214
Dormann C. F., Elith J., Bacher S., Buchmann C., Carl G., Carré G., et al. (2013). Collinearity: a review of methods to deal with it and a simulation study evaluating their performance. Ecography 5, 027–046. doi: 10.1111/j.1600-0587.2012.07348.x
Drake K. K., Bowen L., Nussear K. E., Esque T. C., Berger A. J., Custer N. A., et al. (2016). Negative impacts of invasive plants on conservation of sensitive desert wildlife. Ecosphere 7, e01531. doi: 10.1002/ecs2.1531
Drake K. K., Esque T. C., Nussear K. E., DeFalco L. A., Scoles-Sciulla S. J., Modlin A. T., et al. (2015). Desert tortoise use of burned habitat in the eastern Mojave Desert. J. Wildl. Manage. 79, 618–629. doi: 10.1002/jwmg.874
Duda J. J., Krzysik A. J., Freilich J. E. (1999). Effects of drought on desert tortoise movement and activity. J. Wildl. Manage. 63, 1181–1192. doi: 10.2307/3802836
Edmund H., Bell K., Butler A. (2020) Prism: access data from the Oregon State Prism Climate Project. Available at: https://github.com/ropensci/prism.
Esque T. C., Nussear K. E., Drake K. K., Walde A. D., Berry K. H., Averill-Murray R. C., et al. (2010). Effects of subsidized predators, resource variability, and human population density on desert tortoise populations in the Mojave Desert, USA. Endangered Species Res. 12, 167–177. doi: 10.3354/esr00298
Field K. J., Tracy C. R., Medica P. A., Marlow R. W., Corn P. S. (2003). “Spring, fall, or winter? Success of desert tortoise translocation as affected by season of release,” in Proc. 27th Ann. Desert Tortoise Council Symp (Las Vegas, Nevada, USA: The Desert Tortoise Council), 107–109. Available at: https://deserttortoise.org (Accessed October 10, 2021).
Fleming C. H., Fagan W. F., Mueller T., Olson K. A., Leimgruber P., Calabrese J. M. (2015). Rigorous home range estimation with movement data: a new autocorrelated kernel density estimator. Ecology 96, 1182–1188. doi: 10.1890/14-2010.1
Freilich J. E., Burnham K. P., Collins C. M., Garry C. A. (2000). Factors affecting population assessments of desert tortoises. Conserv. Bio. 14, 1479–1489. doi: 10.1046/j.1523-1739.2000.98360.x
Germano J. M., Nafus M. G., Perry J. A., Hall D. B., Swaisgood R. R. (2017). Predicting translocation outcomes with personality for desert tortoises. Behav. Ecol. 28, 1075–1084. doi: 10.1093/beheco/arx064
Hardy R. (1976). “The Utah population—a look in the 1970’s,” in Proc. First Ann. Desert Tortoise Council Symp., 23–24 March 1976 (Las Vegas, Nevada, USA: The Desert Tortoise Council), 84–88. Available at: https://deserttortoise.org (Accessed August 15, 2022).
Hazard L. C., Shemanski D. R., Nagy K. A. (2009). Nutritional quality of natural foods of juvenile desert tortoises (Gopherus agassizii): energy, nitrogen, and fiber digestibility. J. Herpetol. 43, 38–48. doi: 10.1670/07-160R1.1
Hazard L. C., Shemanski D. R., Nagy K. A. (2010). Nutritional quality of natural foods of juvenile and adult desert tortoises (Gopherus agassizii): calcium, phosphorus, and magnesium digestibility. J. Herpetol. 44, 135–147. doi: 10.1670/08-134.1
Henen B. T. (1997). Seasonal and annual energy budgets of female desert tortoises (Gopherus agassizii). Ecology 78, 283–296. doi: 10.1890/0012-9658(1997)078[0283:SAAEBO]2.0.CO;2
Henen B. T. (2002a). Energy and water balance, diet, and reproduction of female desert tortoises (Gopherus agassizii). Chelonian Conserv. Biol. 4, 319–329.
Henen B. T. (2002b). Reproductive effort and reproductive nutrition of female desert tortoises: essential field methods. Integr. Comp. Biol. 42, 43–50. doi: 10.1093/icb/42.1.43
Henen B. T., Peterson C. C., Wallis I. R., Berry K. H., Nagy K. A. (1998). Effects of climatic variation on field metabolism and water relations of desert tortoises. Oecologia 117, 365–373. doi: 10.1007/s004420050669
Hereford R., Webb R. H., Longpré C. I. (2006). Precipitation history and ecosystem response to multidecadal precipitation variability in the Mojave Desert regio–2001. J. Arid Environ. 67 (Supplement), 13–34. doi: 10.1016/j.jaridenv.2006.09.019
IUCN, International Union for the Conservation of Nature, Species Survival Commission. (2013). Guidelines for reintroductions and other conservation translocations. The Reintroduction and Invasive Species Specialist Groups’ Task Force on Moving Plants and Animals for Conservation Purposes. Version 1.0 (Gland, Switzerland: IUCN Species Survival Commission). Available at: https://www.iucn.org/content/guidelines-reintroductions-and-other-conservation-translocations (Accessed 11 Jun 2021).
Jennings W. B., Berry K. H. (2015). Desert tortoises (Gopherus agassizii) are selective herbivores that track the flowering phenology of their preferred food plants. PloS One 10, e0116716. doi: 10.1371/journal.pone.0116716
Klinger R., Underwood E. C., McKinley R., Brooks M. L. (2021). Contrasting geographic patterns of ignition probability and burn severity in the Mojave Desert. Front. Ecol. Evol. 9, 593167. doi: 10.3389/fevo.2021.593167
Kristan W. B. III, Boarman. W. I. (2003). Spatial pattern of risk of common raven predation on desert tortoises. Ecology 84, 2432–2443. doi: 10.1890/02-0448
Leu M., Hanser S. E., Knick S. T. (2008). The human footprint in the West: A large-scale analysis of anthropogenic impacts. Ecol. Appl. 18, 1119–1139. doi: 10.1890/07-0480.1
Longshore K. M., Jaeger J. R., Sappington J. M. (2003). Desert tortoise (Gopherus agassizii) survival at two eastern Mojave Desert sites: death by short-term drought? J. Herpetol. 37, 169–172. doi: 10.1670/0022-1511(2003)037[0169:DTGASA]2.0.CO;2
Mack J. S., Berry K. H. (2023). Drivers of survival of translocated tortoises. J. Wildl. Manage. 87, e22352. doi: 10.1002/jwmg.22352
Mack J. S., Berry K. H., Miller D. M., Carlson A. S. (2015). Factors affecting the thermal environment of Agassiz’s desert tortoise (Gopherus agassizii) cover sites in the Central Mojave Desert during periods of temperature extremes. J. Herpetol. 49, 405–414. doi: 10.1670/13-080
Mack J. S., Schneider H. E., Berry K. H. (2018). Crowding affects health, growth, and behavior in headstart pens for Agassiz’s desert tortoise. Chelonian Conserv. Biol. 17, 14–26. doi: 10.2744/CCB-1248.1
Medica P. A., Bury R. B., Luckenbach R. A. (1980). Drinking and construction of water catchments by the desert tortoise, Gopherus agassizii, in the Mojave Desert. Herpetologica 36, 301–304.
Medica P. A., Nussear K. E., Esque T. C., Saethre M. B. (2012). Long-term growth of desert tortoises (Gopherus agassizii) in a southern Nevada population. J. Herpetol. 46, 213–220. doi: 10.1670/11-327
Minnich R. A., Saunders A. C. (2000). “Brassica tournefortii Gouan,” in Invasive Plants of California’s Wildlands. Eds. Bossard C. C., Randall J. M., Hoshovsky M. C. (Berkeley, CA: University of California Press), 68–72.
Nafus M. G., Esque T. C., Averill-Murray R. C., Nussear K. E., Swaisgood R. R. (2016). Habitat drives dispersal and survival of translocated juvenile desert tortoises. J. Appl. Ecol. doi: 10.1111/1365–2664.12774
Nafus M. F., Tuberville T. D., Buhlmann K. A., Todd B. D. (2013). Relative abundance and demographic structure of Agassiz’s desert tortoise (Gopherus agassizii) along roads of varying size and traffic. Biol. Conserv. 162, 100–106. doi: 10.1016/j.biocon.2013.04.009
Nagy K. A., Henen B. T., Hillard L. S. (2020). Head-started Agassiz’s desert tortoise Gopherus agassizii achieved high survival, growth, and body condition in natural field enclosures. Endangered Species Res. 43, 305–321. doi: 10.3354/esr01067
Nagy K. A., Henen B. T., Vyas D. B., Wallis I. R. (2002). A condition index for the desert tortoise (Gopherus agassizii). Chelonian Conserv. Biol. 4, 425–429.
Nagy K. A., Hillard S., Dickson S., Morafka D. J. (2015a). Effects of artificial rain on survivorship, body condition, and growth of head-started desert tortoises (Gopherus agassizii) released to the open desert. Herpetological Conserv. Biol. 10, 535–549.
Nagy K., Hillard L. S., Tuma M. W., Morafka D. J. (2015b). Head-started desert tortoises (Gopherus agassizii): Movements, survivorship and mortality causes following their release. Herpetological Conserv. Biol. 10, 203–215.
Nagy K. A., Kuchling G., Hillard L. S., Henen B. T. (2016). Weather and sex ratios of head-started Agassiz’s desert tortoise Gopherus agassizii juveniles hatched in natural habitat enclosures. Endangered Species Res. 30, 145–155. doi: 10.3354/esr00737
Nagy K. A., Medica P. A. (1986). Physiological ecology of desert tortoises in southern Nevada. Herpetologica 42, 73–92.
Nagy K. A., Morafka D. J., Yates R. A. (1997). Young desert tortoise survival—energy, water, and food requirements in the field. Chelonian Conserv. Biol. 2, 396–404.
Nagy K. A., Tuma M. W., Hillard L. S. (2011). Shell hardness measurement in juvenile desert tortoises, Gopherus agassizii. Herpetological Rev. 42, 191–195.
Nussear K. E., Tracy C. R., Medica P. A., Wilson D. S., Marlow R. W., Corn P. S. (2012). Translocation as a conservation tool for Agassiz’s desert tortoises: survivorship, reproduction, and movements. J. Wildl. Manage. 76, 1341–1353. doi: 10.1002/jwmg.390
Oftedal O. T. (2002). “Nutritional ecology of the desert tortoise in the Mohave and Sonoran deserts,” in The Sonoran Desert Tortoise: Natural History, Biology, and Conservation. Ed. Van Devender T. R. (Tucson, AZ: University of Arizona Press and the Arizona-Sonora Desert Museum), 194–241.
Oftedal O. T., Hillard S., Morafka D. J. (2002). Selective spring foraging by juvenile desert tortoises (Gopherus agassizii) in the Mojave Desert: evidence of an adaptive nutritional strategy. Chelonian Conserv. Biol. 4, 341–352.
Overpeck J. T., Udall B. (2020). Climate change and the aridification of North America. Proc. Natl. Acad. Sci. U.S.A. 117, 11856–11858. doi: 10.1073/pnas.2006323117
Pebesma E., Bivand R., Rowlingson B., Gomez-Rubio V., Hijmans R., Sumner M., et al. (2021). Sp: classes and methods for spatial data. R package version 1.4.5. Available at: https://github.com/edzer/sp/ (Accessed September 2022).
Peterson C. C. (1996). Anhomeostasis: seasonal water and solute relations in two populations of the desert tortoise (Gopherus agassizii) during chronic drought. Physiol. Zool. 69, 1324–1358. doi: 10.1086/physzool.69.6.30164263
Petorelli N. (2013). The normalized difference vegetation index (Oxford, UK: Oxford University Press).
Rautenstrauch K. R., Rakestraw D. L., Brown G. A., Boone J. L., Lederle P. E. (2002). Patterns of burrow use by desert tortoises (Gopherus agassizii) in southcentral Nevada. Chelonian Conserv. Biol. 4, 398–405.
R Core Team. (2021). R: a language and environment for statistical computing (Vienna, Austria: R Foundation for Statistical Computing).
Rhodin A. G. J., Stanford C. B., van Dijk P. P., Eisemberg C., Goode E. V., Luiselli L., et al. (2018). Global conservation status of turtles and tortoises (Order Testudines). Chelonian Conserv. Biol. 17, 135–161. doi: 10.2744/ccb-1348.1
Roberts H. P., Willey L. L., Jones M. T., Akre T. S. B., King D. I., Kleopfer J., et al. (2023). Is the future female for turtles? Climate change and wetland configuration predict sex ratios of a freshwater species. Global Change Biol. 29, 2643–2654. doi: 10.1111/gcb.16625
Row J. R., Blouin-Demers G. (2006). Kernels are not accurate estimators of home-range size for herpetofauna. Copeia 2006 (4), 797–802. doi: 10.1643/0045-8511(2006)6[797:KANAEO]2.0.CO;2
Rowlands P. G. (1995). “Regional bioclimatology of the California Desert,” in The California Desert: An Introduction to Natural Resources and Man’s Impact. Eds. Latting J., Rowlands P. G. (Riverside, CA: June Latting Books), 95–134.
Rowlands P., Johnson H., Ritter E., Endo A. (1982). “The Mojave Desert,” in Reference Handbook on the Deserts of North America. Ed. Bender G. (Westport, CT: Greenwood Press), 103–162.
Shields T., Currylow A., Hanley B., Boland S., Boarman B., Vaughn M. (2019). Novel management tools for subsidized avian predators and a case study in the conservation of a threatened species. Ecosphere 10 (10), e02895. doi: 10.1002/ecs2.2895
Stahle D. W. (2020). Anthropogenic megadrought. Science 368 (6488), 238–239. doi: 10.1126/science.abb6902
Stamps J. A., Swaisgood R. R. (2007). Someplace like home: experience, habitat selection and conservation biology. Appl. Anim. Behav. Sci. 102, 392–409. doi: 10.1016/j.applanim.2006.05.038
Swaisgood R. R., Ruiz-Miranda C. (2019). “Moving animals in the right direction: making conservation translocation an effective tool,” in International Wildlife Management: Conservation Challenges in a Changing World. Eds. Koprowski J. L., Krausman P. R. (Baltimore, MD: Johns Hopkins Univ Press), 141–156.
Turner F. B., Berry K. H., Randall D. C., White G. C. (1987). Population ecology of the desert tortoise at Goffs, Californ–1986 (Rosemead, California, USA: Southern California Edison).
Turner F. B., Hayden P., Burge B. L., Roberson J. B. (1986). Egg production by the Desert Tortoise (Gopherus agassizii) in California. Herpetologica 42, 93–104.
U.S. Bureau of Land Management. (2019). West Mojave route network project. Final supplemental environmental impact statement for the California Desert District (California, USA: USBLM, Moreno Valley).
U.S. Fish and Wildlife Service [USFWS]. (1990). Endangered and threatened wildlife and plants; determination of threatened status for the Mojave population of the desert tortoise. Fed. Regist. 55, 12178–12191.
U.S. Fish and Wildlife Service [USFWS]. (1994). esert tortoise (Mojave population) recovery plan (Portland, Oregon. USA: USFWS).
U.S. Fish and Wildlife Service [USFWS]. (2008). Environmental assessment to implement a desert tortoise recovery plan task; reduce common raven predation on the desert tortoise (Ventura, California, USA: U.S. Department of the Interior, Fish and Wildlife Service).
U.S. Fish and Wildlife Service [USFWS]. (2011). Revised Recovery Plan for the Mojave Population of the desert tortoise (Gopherus agassizii) (Reno, Nevada, USA: USFWS).
U.S. Geological Survey [USGS]. (2022). User guide. Earth Resources Observation and Science (EROS) Center Science Processing Architecture (ESPA) On Demand Interface, version 13.0. Department of the Interior, U.S. Geological Survey. Available at: https://www.usgs.gov/media/files/eros-science=processing-architecture-demand-interface-user-guide.
von Seckendorff Hoff K., Marlow R. W. (2002). Impacts of vehicle road traffic on Desert Tortoise populations with consideration of conservation of tortoise habitat in southern Nevada. Chelonian Conserv. Biol. 4, 449–456.
Williams A. P., Cook E. R., Smerdon J. E., Cook B. I., Abatzoglou J. T., Bolles K., et al. (2020). Large contribution from anthropogenic warming to an emerging North American megadrought. Science 368, 314–318. doi: 10.1126/science.aaz9600
Keywords: Gopherus agassizii, growth, behavior, juvenile, Mojave Desert, precipitation
Citation: Berry KH, Mack JS and Anderson KM (2023) Variations in climate drive behavior and survival of small desert tortoises. Front. Ecol. Evol. 11:1164050. doi: 10.3389/fevo.2023.1164050
Received: 11 February 2023; Accepted: 04 July 2023;
Published: 28 July 2023.
Edited by:
Clare Aslan, Northern Arizona University, United StatesReviewed by:
Amanda Goldberg, Virginia Tech, United StatesCameron Barrows, University of California, Riverside, United States
Copyright © 2023 Berry, Mack and Anderson. This is an open-access article distributed under the terms of the Creative Commons Attribution License (CC BY). The use, distribution or reproduction in other forums is permitted, provided the original author(s) and the copyright owner(s) are credited and that the original publication in this journal is cited, in accordance with accepted academic practice. No use, distribution or reproduction is permitted which does not comply with these terms.
*Correspondence: Kristin H. Berry, a3Jpc3Rpbl9iZXJyeUB1c2dzLmdvdg==
†Present address: Jeremy S. Mack, Lehigh University, Bethlehem, PA, United States