- 1Department of Geobiology, University of Göttingen, Göttingen, Germany
- 2Xishuangbanna Tropical Botanical Garden, Chinese Academy of Sciences, Menglun, Yunnan, China
- 3Amber Study Group, c/o Geological-Palaeontological Museum of the University of Hamburg, Hamburg, Germany
The fossil fern species Cretacifilix fungiformis from mid-Cretaceous Kachin amber from Myanmar was previously assigned to eupolypod ferns for having sporangia with a vertical annulus and sporangial stalks 2–3 cells thick, along with monolete spores with a distinct perine. However, due to the insufficient documentation of character states and the unavailability of the holotype, the proposed affiliation of this fossil to derived families of eupolypods required further study. The recent discovery of additional leaf fragments of C. fungiformis from the same amber deposit enabled us to re-evaluate the relationships of this fossil taxon. Newly documented morphological evidence corroborated its placement within the eupolypod ferns. This was achieved by evaluating identifiable features present in the fossil species through an ancestral character state reconstruction utilizing a comprehensive, time-calibrated phylogeny of eupolypod ferns. Our character state reconstruction of extant eupolypod families and genera recovered morphological similarity of Cretacifilix to the closely related genera Arachniodes and Dryopteris, supporting an assignment of this fossil genus to the species-rich family Dryopteridaceae within the Polypodiineae.
1. Introduction
The eupolypod ferns represent the largest clade not only in the order Polypodiales but among all ferns. With ~7,000 species, Polypodiales includes 66% of all living fern species (Schneider et al., 2004; PPG I, 2016; Nitta et al., 2022). Distributed from tropical to temperate regions, this group of ferns inhabits most of the ecosystems occupied by ferns including epiphytic, climbing, and saxicolous growth habits (Smith et al., 2006; Rothfels et al., 2012).
DNA-sequence-based divergence time estimates differed substantially in the age of the crown group of eupolypods by considering a time of early divergence as young as the Early Cretaceous (Schneider et al., 2004; Schuettpelz and Pryer, 2009; Rothfels et al., 2015; Lehtonen et al., 2017), or as old as the Middle to Late Jurassic (Du et al., 2021) or even the Early Jurassic (Testo and Sundue, 2016; Nitta et al., 2022). Despite these discrepancies, caused by differences in the data utilized, fossil calibrations employment, and applied analytical procedures, all these studies were congruent in the estimated ages of the majority of extant eupolypod families. Thus, the majority of extant eupolypod fern diversity dates back to diversification events occurring from the Late Cretaceous to the Cenozoic.
For a long time, the Mesozoic fossil record of eupolypod ferns, as well as other groups of polypod ferns, was considered ambiguous because the unequivocal assignment of the rather sparse fossils was challenging due to the incompleteness of diagnostic morphological characters. For that reason, only a few macrofossils have been accepted to represent eupolypods in the Cretaceous, e.g., Athyrium cretaceum Chen and Meng, Dryopterites beishanensis Ren and Sun, Thelypteris goldianum (Lesquereux) Crabtree, and Woodwardia sp. (Crabtree, 1987; Chen et al., 1997; Upchurch and Mack, 1998; Ren et al., 2022). However, unambiguous taxonomic assignments of these fossils to extant families and genera require further assessment and documentation (e.g., Li et al., 2022).
Kachin amber from Myanmar has emerged as the most important source of information on the fossil diversity of Cretaceous polypod ferns, with ten species representing four of six Polypodiales suborders described to date (Poinar and Buckley, 2008; Schneider et al., 2016; Regalado et al., 2017a,b, 2018, 2019; Li et al., 2020; Poinar, 2021; Long et al., 2023a,b).
Among them, the monotypic fern genus Cretacifilix, with the fossil species Cretacifilix fungiformis G. O. Poinar and R. Buckley, was described from a single fertile leaf fragment preserved in mid-Cretaceous amber from Myanmar (Poinar and Buckley, 2008). The specimen was originally identified as a eupolypod fern, characterized by having sporangia with a vertical annulus interrupted by the stalk, along with monolete spores with a distinct perine. However, the proposed affiliation of this fossil to derived families of eupolypods has not been widely accepted so far because of the insufficient photographic documentation of character states and the unavailability of the holotype (refer to Schneider et al., 2016; Regalado et al., 2018).
Access to new specimens of mid-Cretaceous Kachin amber that preserved several leaf fragments of Cretacifilix fungiformis allowed us to reappraise the relationship of this fossil taxon by providing new morphological evidence. This evidence enabled us to confirm its placement within the eupolypod ferns. Furthermore, we evaluate the combination of character states of this fossil on a recent reconstruction based on a comprehensive, time-calibrated phylogeny of eupolypod ferns (Nitta et al., 2022; https://fernphy.github.io) and discuss the possible assignment of C. fungiformis to the family Dryopteridaceae.
2. Materials and methods
Kachin amber (traditionally called Burmese amber) derives from outcrops at Noije Bum (“Banyan Mountain”), a hill ~20 km SW of the municipality of Tanai, located on the Ledo Road ~105 km N of Myitkyina in Kachin State, N Myanmar (Grimaldi et al., 2002; Cruickshank and Ko, 2003). Biostratigraphic studies (Cruickshank and Ko, 2003) and U-Pb dating of zircons (Shi et al., 2012) revealed a late Albian to earliest Cenomanian age of Kachin amber, with a minimum age of 99 Ma.
Four amber pieces containing fertile inclusions of Cretacifilix were discovered from the Geoscientific Collection of the University of Göttingen, Germany (GZG), and the amber collection of the citizen scientist Patrick Müller (Zweibrücken, Germany).
Amber pieces were ground and polished manually using a series of wet silicon carbide papers with grits ranging from FEPA P 600 (25.8 μm grain size) to 4,000 (5 μm grain size, Struers company) to produce smooth surfaces (Sadowski et al., 2021; Schmidt et al., 2022). Prepared amber inclusions were examined using a Carl Zeiss Stemi 508 dissecting microscope and a Carl Zeiss AxioScope A1 compound microscope, both of them equipped with a Canon 5D digital camera. To capture the three-dimensionality of the inclusions, all light-microscopic images were digitally stacked to produce photomicrographic composites merged from up to 120 individual focal planes using the software package HeliconFocus version 6.3.3 Pro (Sadowski et al., 2021).
The morphological features provided in the original description of Cretacifilix fungiformis (Poinar and Buckley, 2008) were compared to those of the newly discovered fossils. They were also compared to published morphological descriptions of extant fern families and genera (Kramer and Green, 1990; Smith et al., 2006; Rothfels et al., 2012; Liu et al., 2013; Zhou et al., 2018; Fawcett and Smith, 2021) with taxonomic updates according to PPG I (2016), Zhou et al. (2018), and Fawcett and Smith (2021). The technical terms related to gross morphology follow the general definitions provided by Lellinger (2002).
The morphological similarity of the fossil to extant eupolypod families and genera was further assessed through an ancestral state reconstruction of 12 morphological characters (Table 1). The character state combinations observed in the fossils was compared to the reconstructed states at family and genus ranks among eupolypod ferns. Character states of extant species of eupolypod ferns were scored based on their observations and literature (Supplementary Table 1).
The eupolypod clade of the fern tree of life (FTOL) (Nitta et al., 2022; https://fernphy.github.io) was used as the backbone for the analyses. Species with unresolved taxonomy or uncertain phylogenetic position, in particular some non-monophyletic genera of Thelypteridaceae, were removed from the phylogeny. Species for which some of the morphological characters were unavailable were also removed. Our resulting dataset consisted of 1,457 species and 157 genera, representing the 22 eupolypod families and covering 84% of the eupolypod generic diversity.
To reconstruct character evolution, the best model for the substitution matrix was selected for each character utilizing the corrected Akaike information criterion (AIC) to select one out of three transition models: equal rates = ER, symmetrical rates = SYM, and all rates different = ARD. This was carried out using the fitDiscrete function from the R package geiger (Harmon et al., 2008). When identical AIC values were retrieved, the model with the lowest number of parameters was chosen. Ancestral states were estimated through stochastic character mapping (Bollback, 2006), running 500 iterations of the function make.simmap from the R package phytools v. 1.2-0 (Revell, 2012) employing the best-fit models (Supplementary Table 1). Finally, the 500 inferred possible evolutionary histories resulting from stochastic character mapping were summarized, calculating the posterior probabilities at nodes with the function summary from phytools (Revell, 2012). Character states of the most recent common ancestors of eupolypod families and genera were compared to the states observed in Cretacifilix with two thresholds: posterior probabilities > 0.75 and > 0.90 in all evaluated characters.
The phylogenetic signal of each character was assessed via δ-statistic (Borges et al., 2019). As the phylogenetic signal can vary at different taxonomic ranks, we calculated the δ-statistic from suborder to genus ranks. The significance of the δ-value was evaluated by comparing estimated δ-values to the random δ-value resultant of permuting the character vector along the phylogeny for 100 iterations. The phylogenetic signal was considered significant with the probability of random δ-value > the inferred δ-value of p < 0.05. The genus rank in families with phylogenies including < 20 species (e.g., Arthropteridaceae, Desmophlebiaceae, Didymochlaenaceae, Diplaziopsidaceae, Hemidictyaceae, Hypodematiaceae, Nephrolepidaceae, Oleandraceae, Onocleaceae, Pteridryaceae, Rhachidosoraceae, and Woodsiaceae) could not be assessed due to limitations in the calculations of the δ-statistic (Borges et al., 2019). Autapomorphic characters were excluded.
In addition, a second dataset comprising 818 species representing all genera of Dryopteridaceae was evaluated for the same characters except for the two characters related to spore morphology (Table 1). For this dataset, we followed the above-described methodology.
3. Results
3.1. Systematic paleontology
Classification: Eukaryota, Viridiplantae, Streptophyta, Embryophyta, Tracheophyta, Polypodiopsida, Polypodiidae, Polypodiales, Polypodiineae, Dryopteridaceae.
Cretacifilix G.O. Poinar and R. Buckley. Journal of the Botanical Research Institute of Texas 2: 1176. 2008, emended here.
Type species: Cretacifilix fungiformis G.O. Poinar and R. Buckley.
Emended diagnosis: Blade at least pinnate-pinnatifid, probably further divided; rachis or costa adaxially grooved, black sclerotized, glabrous. Veins free, not reaching the segment margin, tips not swollen, not ending in hydathodes. Tissue glabrous, leaves hypostomatic. Sori medial, round, with receptacle terminal on the veinlet. Indusia reniform, laterally attached at a sinus. Sporangia with a long vertical oriented annulus; stalks 2–3 cells thick. Spores bilateral, monolete with lophate perine (Figures 1–3).
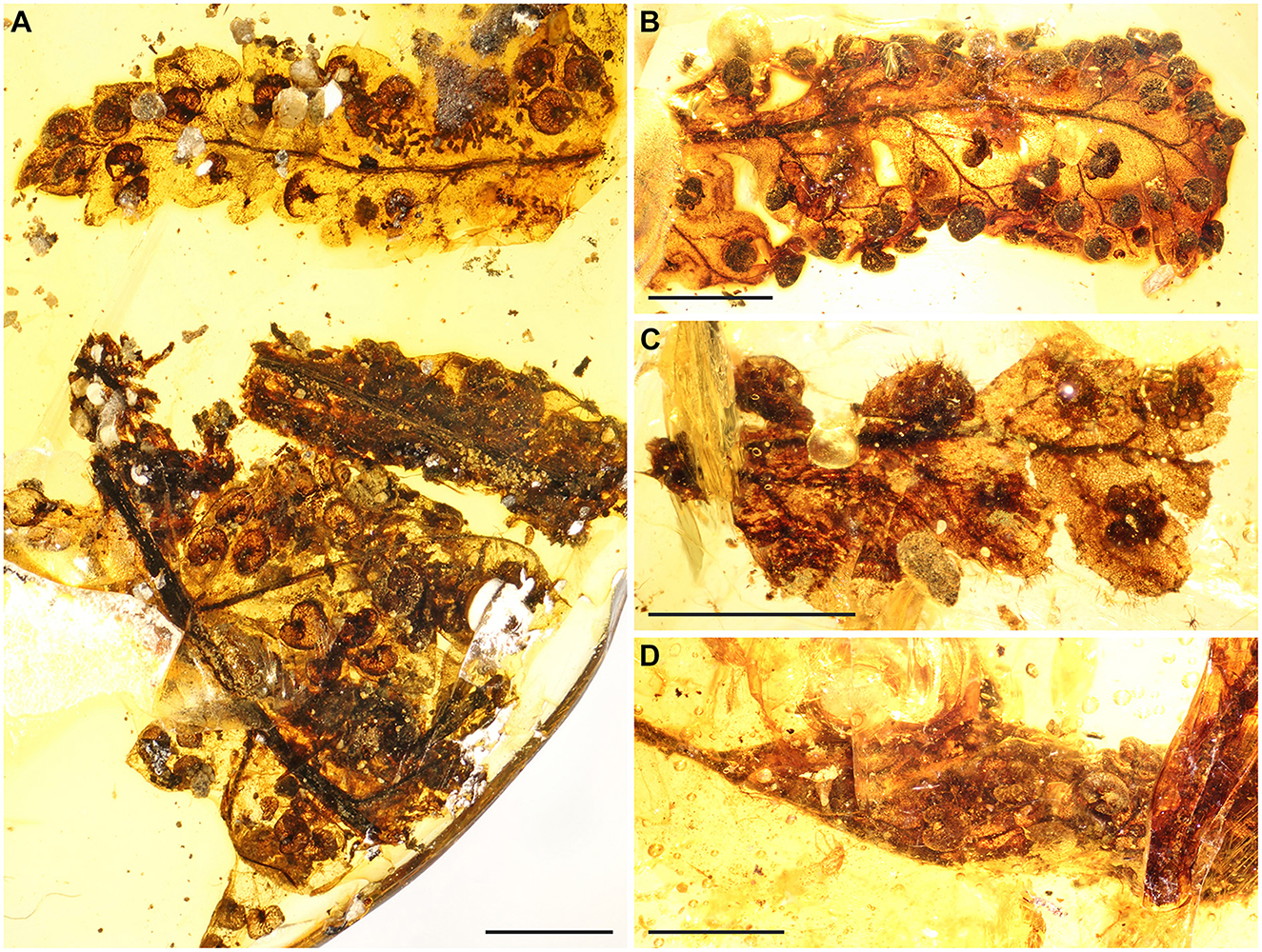
Figure 1. Overview of fossils of Cretacifilix fungiformis from Kachin amber. (A) Amber specimen GZG.BST.22036, (B) GZG.BST.22079, (C) BuB3488, and (D) BuB3479. All fragments show abaxial surfaces except for the smallest fragment in (A). Scale bars = 2 mm (A–D).
Cretacifilix fungiformis G.O. Poinar & R. Buckley. Journal of the Botanical Research Institute of Texas 2: 1176. 2008.
Holotype: Myanmar, Kachin, Noije Bum summit site in the Hukawng Valley, SW of Maingkhwan in the state of Kachin, collector unknown, catalog number B-11, amber collection of Deniz Erin in Istanbul 34744, Turkey (not available for study).
Because the holotype is not accessible, six leaf fragments of different amber specimens were examined: Three are enclosed in amber piece GZG.BST.22036, and the other three in pieces GZG.BST.22079, BuB3479, and BuB3488, respectively. Amber piece GZG.BST.22036 contains two leaf fragments showing a main axis (rachis, costa, or costula) with a rolled-up blade (13.4 × 2.0 mm and 9.7 × 3.5 mm, respectively) and the other is a fragment of a pinna or pinnula apex with 14 segments (9.7 × 3.5 mm in size; Figure 1A). The leaf fragment preserved in amber piece GZG.BST.22079 has 13.2 × 4.8 mm and 10 segments (Figure 1B). Amber pieces BuB3479 (Figure 1D) and BuB3488 (Figure 1C) contain a broken leaf fragment each, measuring 11.8 × 3.6 mm and 5.0 × 2.5 mm (eight segments), respectively. The inclusion preserved in the amber piece BuB3479 is a rolled-up fragment.
Emended description: Blade at least pinnate-pinnatifid, probably further divided; rachis, costa, or costula adaxially grooved, black sclerotized, glabrous (Figure 2A). Pinna or pinnula fragments oblong or narrowly elliptic, slightly tapering toward the apex, ending in a deltoid segment. Ultimate segments 1.0–2.5 (−3.8) × 0.9–1.8 (−2.5 mm), the basal ones oblong to narrowly elliptic with lobate margins, the medial and apical ones deltoid with entire margins. Veins free, simple, not reaching the segment margin, tips not swollen, not ending in hydathodes, with a catadromous arrangement in lateral veins (in specimen GZG.BST.22079). Tissue glabrous. Leaves hypostomatic, stomata polocytic, with guard cells connected to the distal, marginopolar side of the single subsidiary cell, this one with undulate anticlinal walls, guard cells 35–45 μm long, epidermal cells in both surfaces with undulate anticlinal walls (Figure 2B). Sori round, 280–700 (−900) × 200–600 (−700) μm, with the receptacle terminal on the veinlet (Figures 3C, D). Indusia reniform, laterally attached at a sinus, glabrous, having entire margins, composed of polygonal cells with thickened somewhat undulate walls (Figures 3A, B). Sporangia 120–170 μm, with a vertically oriented annulus (Figure 3E), of at least 12–14 indurated cells, extending the half perimeter of sporangia; stalks 2–3 cells thick (Figure 3F). Spores 23–30 μm in major equatorial diameter, bilateral, monolete. Perine surface lophate, with anastomosing sharp folds (Figures 3G, H).
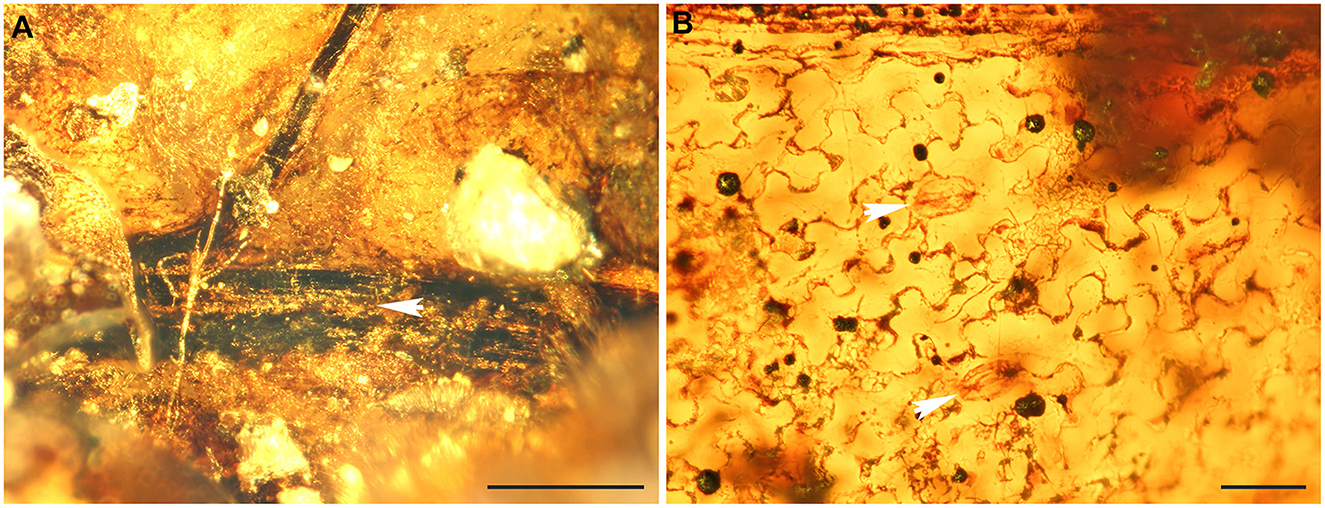
Figure 2. Details of the epidermis of Cretacifilix fungiformis from Kachin amber (GZG.BST.22036). (A) Adaxial blade surface showing the grooved axis (arrowhead). (B) Abaxial epidermis showing the polocytic stomata (arrowheads). Scale bars = 500 μm (A) and 50 μm (B).
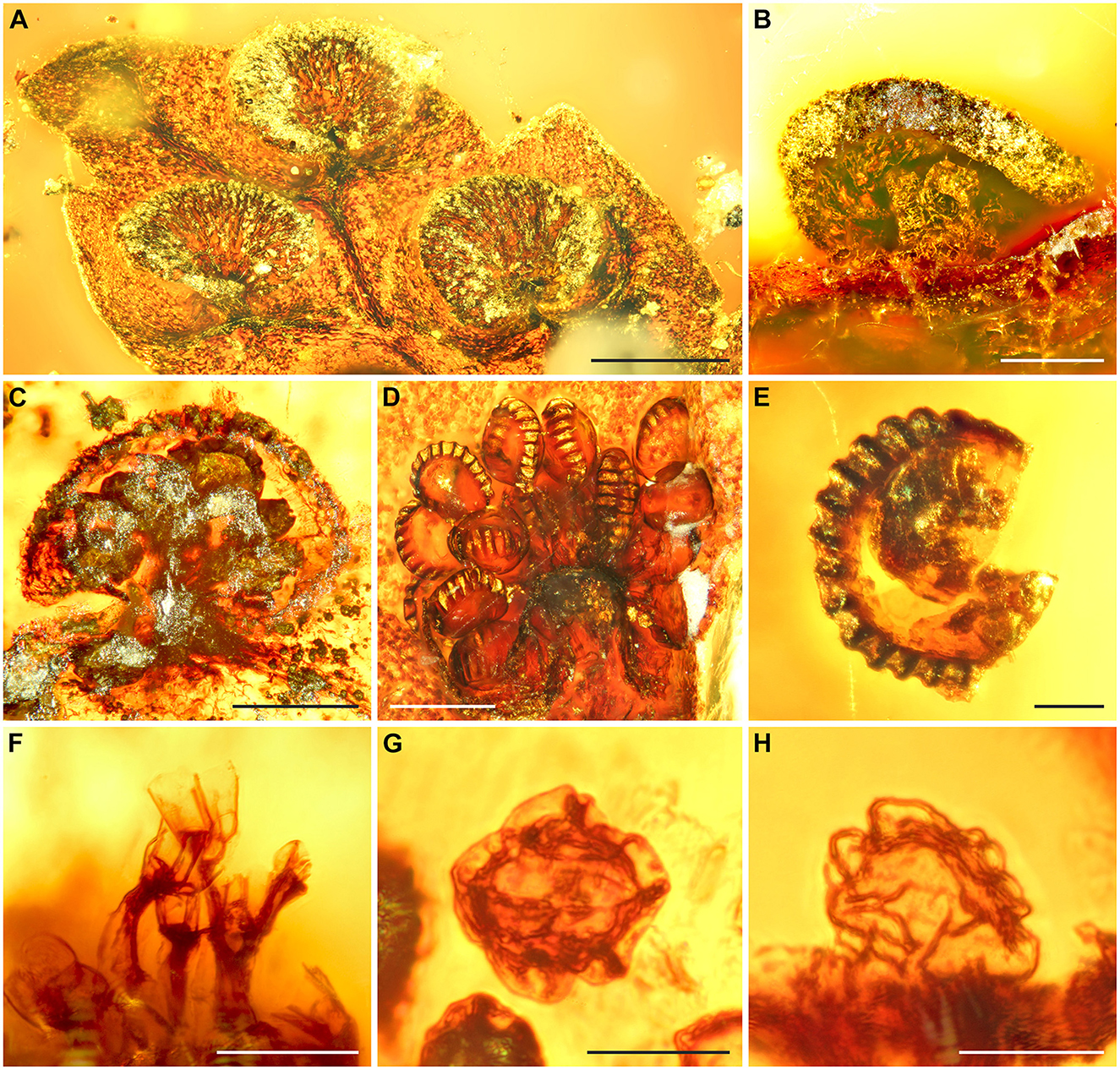
Figure 3. Fertile structures of Cretacifilix fungiformis from Kachin amber. (A) An apical segment with three mature indusia. (B) Lateral view of indusium. (C) Sorus in lateral view. A portion of the indusium and several sporangia lack, due to the initial preparation of the amber specimen. (D) Typical sorus in top view (indusium not preserved). (E) Sporangium with vertical annulus. (F) Sporangium stalks possessing 2–3 cells at the attachment site of the seceded sporangium. (G, H) Spores in equatorial view showing the lophate perine with anastomosing sharp folds. (A, C, F–H) GZG.BST.22036, (B, E) GZG.BST.22079, and (D) BuB3488. Scale bars = 500 μm (A), 200 μm (B–D), 50 μm (E, F), and 20 μm (G, H).
Additional specimens examined: mid-Cretaceous Kachin amber of Myanmar mined from outcrops at Noije Bum near Tanai, four amber pieces: Geoscientific Collection of the University of Göttingen GZG.BST.22036, GZG.BST.22079, Patrick Müller Amber Collection BuB3479, BuB3488.
3.2. Character states of the most recent common ancestors of eupolypod families and genera compared to the states observed in Cretacifilix
Cretacifilix was characterized by having divided fertile leaves; lateral veins free, arising from the mid-vein of a segment; secondary axes with adaxial grooves; sporangia arranged in discrete sori, organized in a medial line between the margin and the mid-vein of the segments; and indusia laterally attached, reniform, and monolete spores with a lophate perine.
The ARD model was determined as the best-fit model for the 12 characters evaluated for the eupolypod dataset (Supplementary Table 1). None of the recent common ancestors of eupolypod fern families showed a character combination of ancestral character states coinciding with the fossil taxon reaching a posterior probability > 0.75 in all the evaluated characters (Figures 4, 5). The closest combinations of character states were found in the families Thelypteridaceae, Dryopteridaceae, and Hypodematiaceae. In Thelypteridaceae, all ancestral character states were similar to those of the fossil taxon, except for the indusium presence which reached a posterior probability of 0.67 (Figure 4, Supplementary Table 1). The ancestral state combination found in Dryopteridaceae differed from the fossil by having a posterior probability of 0.95 for the sori organized in several lines between the mid-vein and the margin instead of having only one medial line, and the indusium laterally attached with a probability of 0.58 (Figure 5, Supplementary Table 1). Hypodematiaceae had the sori organized in one line situated in the medial position with a posterior probability of 0.73 and a lophate perine with a probability of 0.44 (Figure 5, Supplementary Table 1).
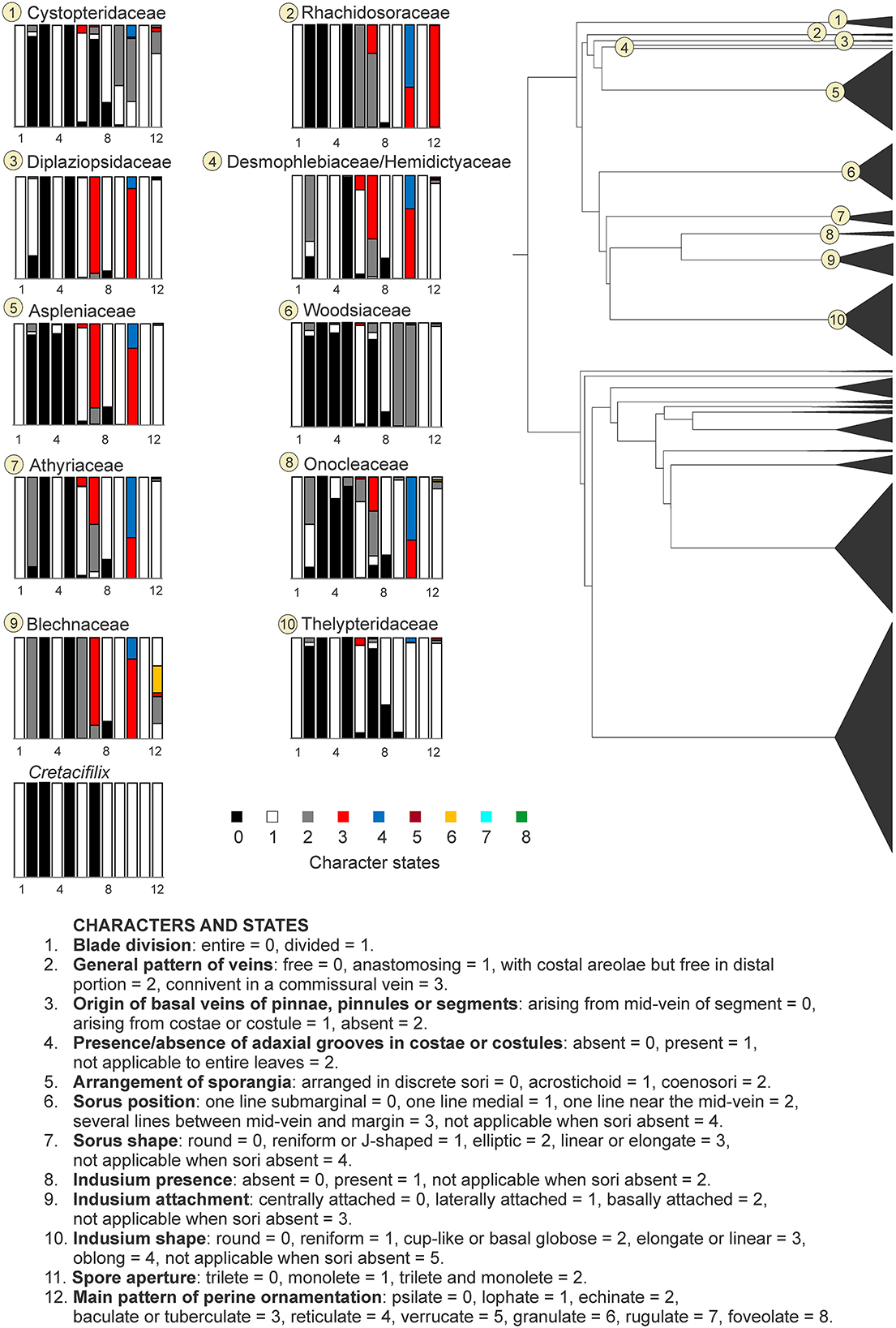
Figure 4. Ancestral state reconstruction of morphological characters in the eupolypod fern families of the suborder Aspleniineae. Rectangles represent proportional likelihoods for characters 1–12 in each family.
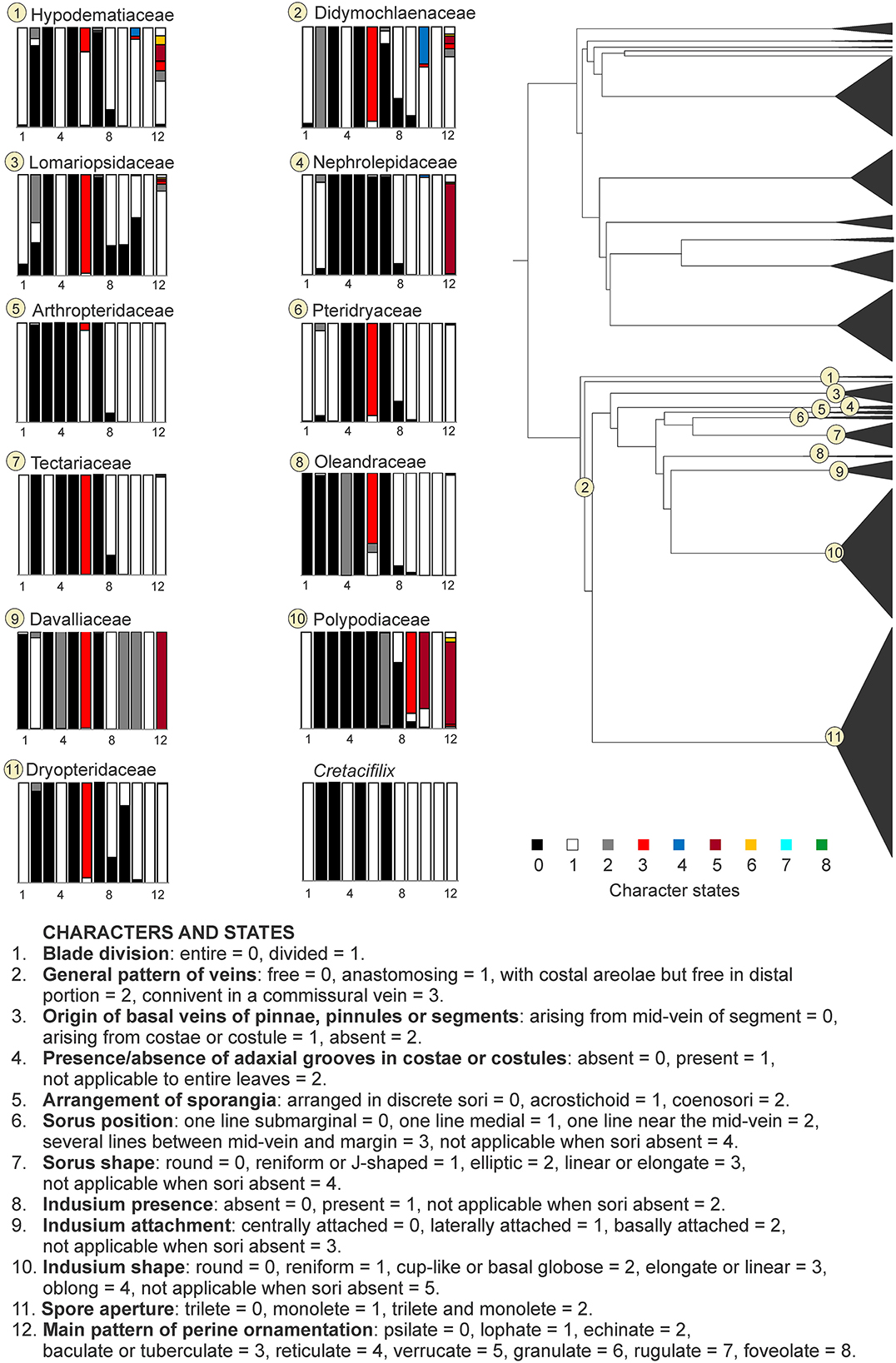
Figure 5. Ancestral state reconstruction of morphological characters in the eupolypod fern families of the suborder Polypodiineae. Rectangles represent proportional likelihoods for characters 1–12 in each family.
Within the genus rank, four genera, namely Dryopteris and Arachniodes (Dryopteridaceae), and Coryphopteris and Pelazoneuron (Thelypteridaceae), shared all ancestral character states with those of Cretacifilix, with a posterior probability > 0.75 in all the examined characters (Figure 6 and Supplementary Figures SM1–SM13, Supplementary Table 1). In Hypodematiaceae, Hypodematium and Leucostegia differed from the fossil in the perine main ornamentation, having a probability of 0.44 for a lophate perine in Hypodematium and a probability of 1 for a verrucate perine in Leucostegia (Supplementary Table 1).
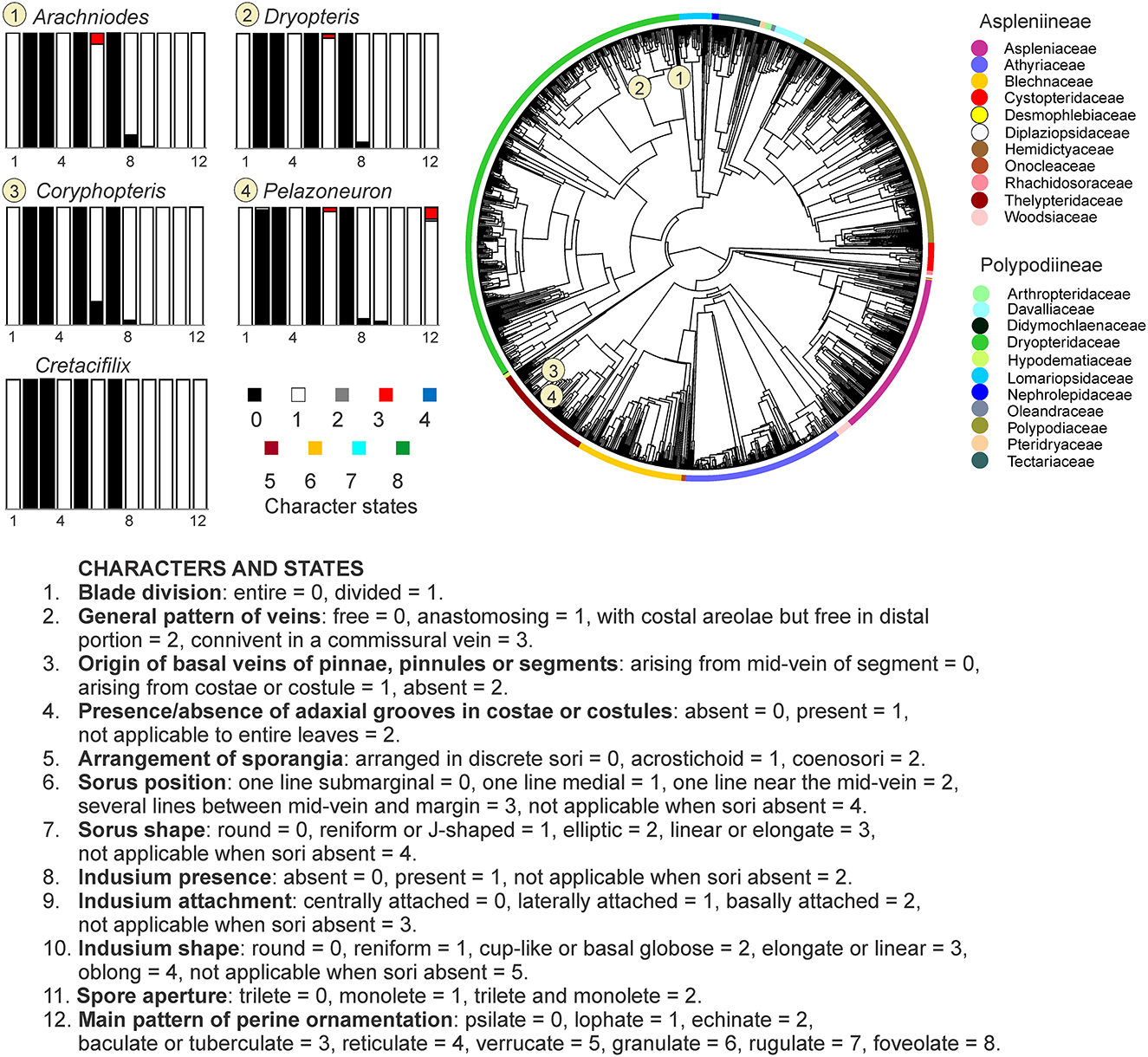
Figure 6. Ancestral state reconstruction of morphological characters in the eupolypod fern genera that showed the closest morphological affinities to Cretacifilix fungiformis. Rectangles represent proportional likelihoods for characters 1–12 in each genus.
Considering a threshold of the posterior probability > 0.9 in the evaluated characters, only the genus Dryopteris from Dryopteridaceae achieved posterior probability values higher than this value in all of the examined characters.
Based on this result, further analyses were carried out using an extended dataset of the family Dryopteridaceae. In this case, the ARD model was likewise determined as the best model for the substitution matrix in all examined characters, except for the arrangement of sporangia and sorus shape, in which the ER model was applied (Supplementary Table 1). Two genera, namely Dryopteris and Arachniodes, showed a combination of the ancestral character states coincident with the fossil taxa reaching a posterior probability > 0.9 in all the evaluated characters (Figure 7 and Supplementary Figures SM14–SM23, Supplementary Table 1). These two genera were also the only ones within the family Dryopteridaceae having posterior probability values > 0.75 in all the assessed characters.
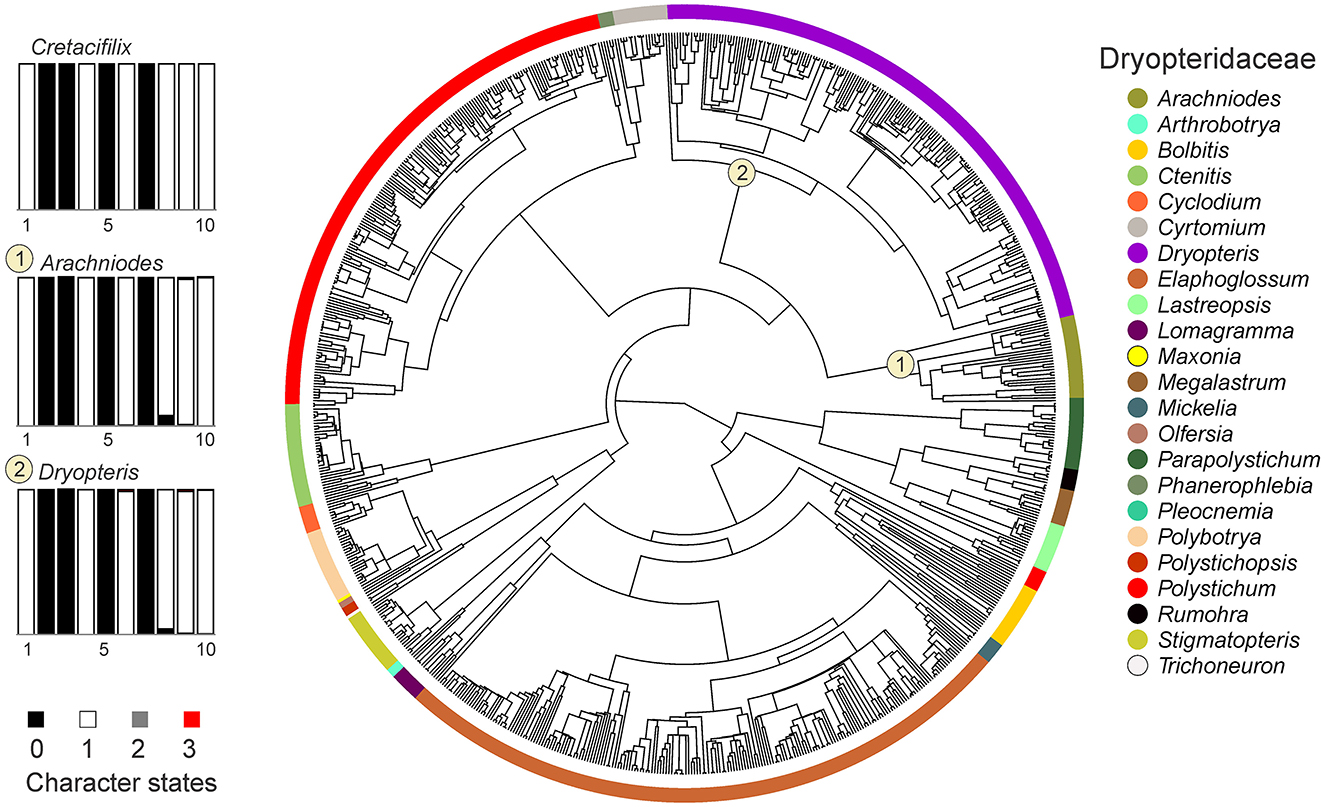
Figure 7. Ancestral state reconstruction of morphological characters in an extended dataset of Dryopteridaceae. Rectangles represent proportional likelihoods for characters 1–10 in each genus.
3.3. Phylogenetic signal
The phylogenetic signal was not significant for most of the evaluated characters at suborder, family, and genus ranks (Supplementary Table 1). Exceptions were the blade division at the family rank within the eupolypods II (δ = 7.37, p = 0.03), the general pattern of veins at the genus rank within Blechnaceae (δ = 38.59, p = 0.02), the indusium shape at the genus rank within Lomariopsidaceae (δ = 216.76, p = 0.04), the type of spore aperture at the genus rank of Polypodiaceae (δ = 10.20, p = 0.03), and the origin of basal veins of pinnae, pinnules, or segments at the genus rank within Tectariaceae (δ = 282.43, p = 0.04).
4. Discussion
4.1. New morphological evidence for Cretacifilix fungiformis
The overall morphology of the newly discovered fossil fragments is largely congruent with the general morphology of Cretacifilix fungiformis described by Poinar and Buckley (2008). However, the new leaf fragments are larger than the holotype; therefore, we were able to document further morphological details, e.g., the blade division degree of the plant is at least pinnate-pinnatifid, the presence of adaxial grooves in the sclerotized secondary axes, the hypostomatic condition of this fern, the presence of polocytic stomata in the abaxial epidermis, the sporangial annulus situated in a vertical position, and the sporangial stalks of 2–3 cells thick.
In their generic and specific descriptions, Poinar and Buckley (2008) mentioned the presence of a few scattered unicellular hairs on the surface and margins of the blade, ranging from 28 to 195 μm in length (Figure 2C in Poinar and Buckley, 2008). However, the putative hairs shown in their Figure 2C seem to have at least two cells, which would indicate pluricellular instead unicellular structures. Unfortunately, the images provided by Poinar and Buckley (2008) are not clear enough to achieve a conclusion about the nature of these structures. After examining the six-leaf fragments available for this study, we could not find any signs of the presence of hairs, not even remains of hair bases at the epidermis. Instead, we found evidence of darkly pigmented mycelia at the leaf surface of the fern fragment enclosed in amber piece BuB3488. These fungi possessed conidiophores at the edge of the leaf fragment (Figure 8). Their size (up to ca. 200 μm in length) ranges within the dimensions of the supposed hairs reported by Poinar and Buckley (2008). Similar fungi were reported from Cenozoic ambers (e.g., Kettunen et al., 2019). These conidiophores without preserved conidia may be considered reminiscent of plant hairs when not preserved in amber in cellular fidelity. Based on this evidence, we consider C. fungiformis a glabrous taxon.
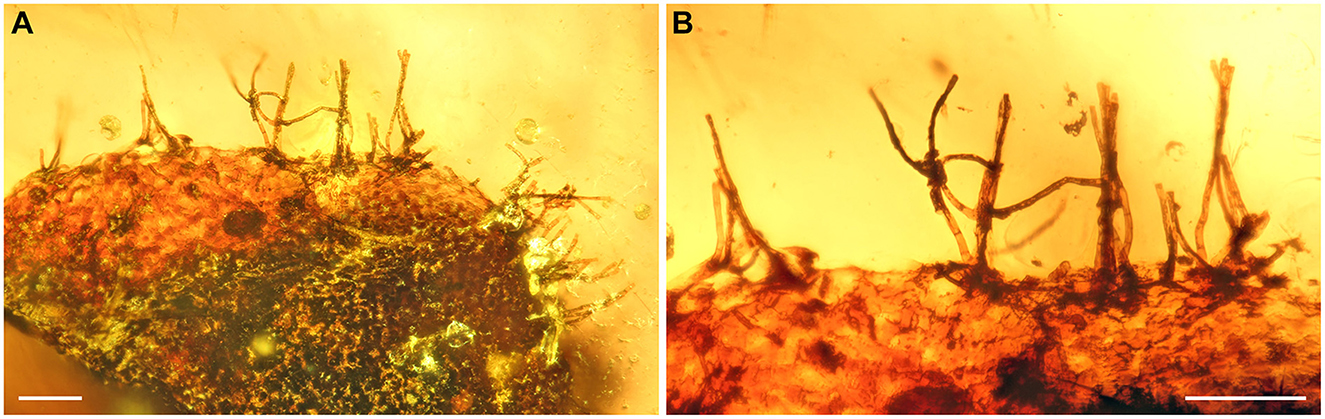
Figure 8. Darkly pigmented fungi at the margin of a pinnule of Cretacifilix fungiformis from Kachin amber. (A) Overview of mycelium and conidiophores. (B) Detail of septate conidiophores. BuB3488. Scale bars = 100 μm.
4.2. Comparison to other eupolypod ferns described from Kachin amber
Two other eupolypod genera, Holttumopteris (Regalado et al., 2018) and Prosperifilix (Long et al., 2023a), have been described from Kachin amber so far. Both taxa can be clearly distinguished from Cretacifilix. Holttumopteris possesses lanceolate to falcate ultimate segments, and the secondary veins are once forked, ending in clavate tips (Regalado et al., 2018), while Cretacifilix has deltoid ultimate segments, and simple secondary veins, with no swollen tips. Prosperifilix has been recently assigned to Dryopteridaceae by Long et al. (2023a). This fossil possesses typical polypod sporangia with a vertical annulus interrupted by the stalk and monolete spores with a distinct perine that characterizes eupolypod ferns. Compared to Cretacifilix, the new genus differs by having exindusiate sori and a blade indument consisting of scattered acicular ca. 0.3 mm long hairs (Long et al., 2023a). Although Prosperifilix may be related to Dryopteridaceae, this assignment should be further evaluated. According to the published data, the character combination rather suggests an assignment to Thelypteridaceae.
4.3. Systematic position of Cretacifilix
The order Polypodiales is characterized by a vertical annulus interrupted by the stalk and the stomium, with sporangial stalks of 1–3 cells thick (Smith et al., 2006; Schneider et al., 2009). These characters are unequivocally present in Cretacifilix fungiformis. The new amber inclusions reveal sporangia with a vertically oriented annulus of 12–14 indurated cells that extend half of the perimeter of sporangia (Figure 3E), with sporangial stalks of 2–3 cells thick (Figure 3F). The monolete spores occurring in the fossil sporangia possess a prominently lophate perine (Figures 3G, H). Within the Polypodiales, this character is only present in the eupolypod ferns (Tryon and Lugardon, 1991), indicating a likely affiliation to either Polypodiineae “eupolypod I” or Aspleniineae “eupolypod II” (PPG I, 2016). These two lineages can mostly be distinguished by the vasculature of the petioles (Schuettpelz and Pryer, 2007), with the only exception of Blechnaceae (Kramer et al., 1990b). Unfortunately, the petiole was not preserved together with the fossil leaf fragments. To assess the relationships of the incompletely preserved fossils, we, therefore, compared the specimen to extant species of both suborders.
4.3.1. Suborder Aspleniineae
Morphological affinities of Cretacifilix to those families within the suborder Aspleniineae that possess linear to elongate sori are discarded. These families include Aspleniaceae, Athyriaceae p.p., Blechnaceae, Desmophlebiaceae, Diplaziopsidaceae, Hemidictyaceae, and Rhachidosoraceae (Kramer and Viane, 1990; Kramer et al., 1990a,b; Smith et al., 2006; Rothfels et al., 2012; Mynssen et al., 2016). Extant Cystopteridaceae differ from the fossil by either the absence of indusia or the presence of hood-like basally attached indusia (Rothfels et al., 2012). Members of Woodsiaceae can be ruled out because of their cup-shaped indusia, which are attached around the base of the receptacle, or by having a ring of hairs extending from around the sporangia (Kramer et al., 1990a; Shmakov, 2015). Within the family Onocleaceae, only the genera Matteuccia and Pentarhizidium are characterized by free veins. The former differs from the fossil by having indurated fertile leaf lamina with reflexed margins that cover the sori, and the latter lacks adaxial grooves in the axes and possesses sori that are fused to form linear coenosori when mature (Kramer et al., 1990a; Smith et al., 2006). Members of the Athyriaceae with round reniform sori are differentiated from the fossil by having free veins often ending to tooth tips in lamina margins in Athyrium p.p. (Kramer et al., 1990a; Wang et al., 2013) or by possessing spores with echinate perine in Deparia p.p. (Tryon and Lugardon, 1991).
A possible affiliation of Cretacifilix to Thelypteridaceae appears to be unlikely as well, although the ancestral state reconstruction showed a combination of characters rather similar to the one observed in the fossil species (Figure 4). Members of this family can be recognized by having hyaline, acicular hairs usually on adaxial rachis and costae, and two strap-shaped vascular bundles in the stipe, distally uniting into a U-shape (Holttum, 1982; Smith, 1990; Fawcett and Smith, 2021). Even though unicellular hairs were described for Cretacifilix (Poinar and Buckley, 2008), we did not find them in any of the six examined leaf fragments and assume that this character is based on a misinterpretation of fungal conidiophores. Furthermore, the combination of characters, the upper surface of secondary axes grooved and all secondary veins terminating before reaching the segment margins, is not found in any extant member of this family (Holttum, 1982; Smith, 1990; Fawcett and Smith, 2021).
In our ancestral state reconstruction, when assessing the genus rank, Coryphopteris and Pelazoneuron (Thelypteridaceae) share all ancestral character states with those of Cretacifilix, with a posterior probability > 0.75 in all the examined characters (Figure 6). However, these genera can be well-differentiated from the fossil species by several other morphological features that were not included in the morphological matrix for the analyses. Coryphopteris has lateral veins reaching the margins and bears hyaline acicular hairs, filiform scales, or multicellular hairs along abaxial axes, and on the laminar tissue between the veins (Fawcett and Smith, 2021). In contrast, Pelazoneuron is characterized by possessing connivent veins at or just below the sinus in adjacent segments or the distal pair meeting the segment margin just above the sinus. Furthermore, the indument of stipes, rachises, costae, veins, and sometimes laminar tissue between veins is composed of hyaline acicular, unicellular hairs in the latter genus (Fawcett and Smith, 2021). None of these features are present in the fossils.
4.3.2. Suborder Polypodiineae
Within the suborder Polypodiineae, some of the families can be a priori ruled out due to their obvious morphological differences from the fossil species. These are Polypodiaceae, characterized by exindusiate sori (Smith et al., 2006), Oleandraceae, by having undivided blades (Kramer, 1990a; Smith et al., 2006), and Nephrolepidaceae, distinguished by its once-pinnate blades with articulate pinnae and its mostly low tuberculate spores (Kramer, 1990b; Tryon and Lugardon, 1991).
Members of Davalliaceae sensu PPG I (2016) differ from the fossils by having verrucate or tuberculate spores (Kramer, 1990c; Tryon and Lugardon, 1991). Didymochlaenaceae can also be discarded by possessing elliptical-oblong sori covered by a centrally attached indusium and spores with a tuberculate or echinate perine (Zhang and Zhang, 2015). Lomariopsidaceae differ from the fossil in its acrostichoid arrangement of the sporangia (Lomariopsis), or by once-pinnate blades with sori arranged in one to several rows on each side of the costa that is covered by peltate indusia (Cyclopeltis, Dryopolystichum) (Tryon and Tryon, 1982; Kramer, 1990d; Kramer et al., 1990a; Mickel and Smith, 2004; Chen et al., 2017).
Affinities of the fossil to the family Pteridryaceae can be also discarded. Members of this family possess a characteristic lobe in the sinus between segments, containing a veinlet (Kramer et al., 1990a; Liu et al., 2014; Zhou et al., 2018), which is absent in Cretacifilix. Likewise, only a few members of the Arthropteridaceae produce pinnate-pinnatifid blades with sori covered by reniform indusia resembling Cretacifilix. In these cases, the lateral veins end in hydathodes close to the margin (Kramer, 1990a; Liu et al., 2013), showing a different pattern compared to the fossil.
Among the Tectariaceae, free veins are only present in Hypoderris p.p., Tectaria p.p., and Triplophyllum (Holttum, 1983; Zhang et al., 2016; Zhou et al., 2018). The ultimate segments are asymmetric at their bases in the Neotropical Triplophyllum (Kramer et al., 1990a; Prado and Moran, 2008), which allows for excluding this genus. Within the neotropical genus Hypoderris, only H. brauniana shares pinnatifid blades with reniform indusia and lophate spores with the fossil but differs in having veins ending close to the margins. The members of Tectaria with free veins usually have an arrangement of venation with the basal posterior vein commonly arising from the costae and not from the main veins of the segments (Kramer et al., 1990a; Ding et al., 2014; Zhang et al., 2016); the blade surface is covered with ctenitoid hairs (Holttum, 1983; Kramer et al., 1990a; Smith et al., 2006; Zhou et al., 2018), and the perine ornamentation generally bears spines or spinules (Zhou et al., 2018). Although those characteristics are not exclusive to free-veined Tectaria species, this combination of characters allows us to recognize them among other members of Tectariaceae, but none of them were observed in the fossil fragments.
Although Hypodematiaceae was suggested by the ancestral state reconstruction as one of the families with closer morphological similarities to Cretacifilix, extant members of this family can be distinguished from the fossil by having coarsely tuberculate or verrucate spores (Kramer et al., 1990a; Zhang et al., 2013a). Hypodematium differs from Cretacifilix in its blades covered with acicular and/or glandular hairs, its veins reaching lobe margins, and its sori growing in a medial position on the veins (Fan et al., 2022). The genus Leucostegia can be also distinguished from the fossil by having lateral veins ending in submarginal orbicular hydathodes (Fan et al., 2022).
The species-rich family Dryopteridaceae lacks morphological or cytological synapomorphies (Labiak et al., 2014), but nearly all extant members of the family possess petiole vasculature with two enlarged adaxial vascular bundles and several smaller abaxial ones (Kramer et al., 1990a), as well as monolete spores with loosely folded perines (Tryon and Lugardon, 1991). Otherwise, the family is well-known for its heterogeneous morphology. Our ancestral state reconstructions suggest a possible morphological affinity of Cretacifilix to two genera belonging to this family: Dryopteris and Arachniodes (Figure 7 and Supplementary Figures SM14–SM23, Supplementary Table 1). These genera share all the evaluated character states with the fossil, i.e., leaf pinnule or segments with adaxially grooved axes, free simple pinnate venation, sporangia arranged in orbicular sori, terminal on veins, situated in a medial position between the margin and the mid-vein, covered by reniform lateral, entire-margined indusia, and monolete spores with a prominent lophate perine.
All other genera of the Dryopteridaceae can be distinguished from the fossil morphology based on several combinations of characters. For example, Bolbitis, Cyclodium p.p. Lomagramma, Mickelia, and Pleocnemia differ from Cretacifilix by having veins anastomosing or at least forming a series of narrow costal areoles (Moran et al., 2010; Bohn et al., 2020, 2021); Lastreopsis, Parapolystichum, Polystichopsis, and Trichoneuron possess axes with abundant pluricellular hairs, whereas the fossils have glabrous axes (Labiak et al., 2014, 2015; Moran and Labiak, 2015; Liu et al., 2016; Prado and Moran, 2016).
Other genera within this family have morphological features different from the fossil, e.g., blade axes not grooved in Megalastrum (Moran et al., 2009; Labiak et al., 2014); blades covered with ctenitoid hairs in Ctenitis, Pleocnemia, and Dryopteris p.p. (Holttum, 1974, 1983, 1985; Zhang et al., 2013b); fertile leaves with strongly reduced laminae in Maxonia (Moran and Labiak, 2015); dimorphic leaves with acrostichoid sporangia in fertile leaves in Bolbitis, Elaphoglossum, and Mickelia (Moran et al., 2010); round sori frequently confluent and covering the segment beneath in Olfersia, Polybotrya, Arthrobotrya, and Teratophyllum (Kramer et al., 1990a; Labiak et al., 2014; Moran and Labiak, 2015); indusia generally peltate in Cyclodium p.p., Cyrtomium, Phanerophlebia, Polystichum, and Rumohra (Kramer et al., 1990a; Bohn et al., 2020, 2021); or exindusiate sori in Stigmatopteris (Moran and Labiak, 2016).
Establishing the generic limits between Arachniodes and Dryopteris has been historically difficult due to the morphological similarity between species of both genera. The genus Arachniodes is only monophyletic when two species, A. macrostegia (Hook.) R. M. Tryon and D. S. Conant and A. ochropteroides (Baker) Lellinger, are excluded from the phylogeny (Lu et al., 2019). These authors argued that the stem branch of Arachniodes s.s. is short, which could be related to the lack of morphological synapomorphies (Lu et al., 2019). They followed the morphological definition of Zhang et al. (2013b) in which Arachniodes can be distinguished within the family by frequent long and creeping or ascending rhizomes with adaxially lustrous blades and anadromous blade segments. In contrast, members of Dryopteris have short and erect or ascending rhizomes, blades dull or lustrous, adaxially and blade segments usually catadromous (except for secondary segments of basal most pair of pinnae of bipinnate fronds) (Zhang et al., 2013b). Most of these characters cannot be evaluated in the currently available leaf fragments of Cretacifilix which renders a clear separation of this fossil species from the extant genera Arachniodes and Dryopteris impossible. Cretacifilix should consequently be considered a member of the stem group of the clade that includes Arachniodes and Dryopteris.
4.4. Exploring the morphological characters
The lack of phylogenetic signals found for nearly all the examined characters at family and genus ranks in both suborders Aspleniineae and Polypodiineae is arguably caused by several evolutionary processes which include fluctuations in the rate of evolution over time (Pagel, 1999), strong stabilizing selection, regular divergent selection (Revell et al., 2008), adaptive radiations in which close relatives rapidly diversify to fill new niches (Kamilar and Cooper, 2013), or high frequency of homoplasy (Voyta et al., 2022; Yassin et al., 2022). Consistently with a multi-factor hypothesis, a previous study utilizing a smaller dataset of eupolypod II (Sundue and Rothfels, 2014) retrieved moderate to low values of homoplasy for most of the characters studied. Although there are slight differences in character scoring between this and our study, we expected similar levels of homoplasy.
In this context, it is crucial to consider that the uncertainty of character reconstruction is exacerbated by the decline of phylogenetic informativeness (Borges et al., 2019). Given the low phylogenetic signal detected across the examined characters, the uncertainty of assessed values for nodes is expected to be high. However, despite these limitations, the retrieved uncertainties of ancestral states were notably low (Supplementary Table 1, Supplementary Figures SM2–SM23).
5. Conclusion
Although ancestral character state reconstructions are sensitive to changes in evolutionary rates and processes along the tree through time, homoplasy, character correlation, or methodological issues in sampling strategies, trait coding, or model selection, we consider our approach as being useful to assess taxonomic affiliations of fossil taxa by exploring the phylogenetic history of the morphological characters documented in extant taxa. The combinations of reconstructed ancestral states, regardless of whether these traits are diagnostic or not, ultimately serve as predictions that are contrasted with the fossil evidence. Based on the new morphological evidence presented here, we were able to confirm the placement of Cretacifilix fungiformis within the eupolypod ferns, as suggested by Poinar and Buckley (2008). Our analysis through a character state reconstruction of extant eupolypod families and genera reveals the morphological affinity of Cretacifilix to the closely related genera Arachniodes and Dryopteris and thus supports the assignment of this fossil genus to the family Dryopteridaceae within Polypodiineae.
Data availability statement
The original contributions presented in the study are included in the article/Supplementary material, further inquiries can be directed to the corresponding author.
Author contributions
LR and ARS conceived the study. PM provided the fossil specimens and conducted initial preparation. LR, HS, PM, and ARS examined the fossils. LR designed and performed the analyses and wrote the first draft of the manuscript. ARS prepared and photographed the fossils. All authors reviewed the results, commented on the manuscript, and approved the final version.
Funding
The authors acknowledge the support by the Deutsche Forschungsgemeinschaft (DFG, project 450754641 to LR) and the Open Access Publication Funds of Göttingen University.
Acknowledgments
The authors thank the two reviewers for their helpful and supportive comments and suggestions, and appreciate the help of Leyla J. Seyfullah (Vienna) for kindly making linguistic improvements to the manuscript. We remember our fruitful discussions of some of the fossils with our late colleague Jochen Heinrichs (1969–2018). With reference to the current conflicts in Kachin State of Myanmar that have been aggravating living conditions since 2017 (e.g., Sokol, 2019), we chose to limit our research to only those amber specimens purchased before 2017 and follow the recommendations by Haug et al. (2020) and Peretti (2021). The authors hope that publishing research on specimens collected before the recent hostilities in Myanmar arose will serve to raise and maintain awareness for the existence of unresolved severe conflicts that need increasing attention from the international community while showcasing, rather than dismissing, the unique natural heritage of Myanmar.
Conflict of interest
The authors declare that the research was conducted in the absence of any commercial or financial relationships that could be construed as a potential conflict of interest.
Publisher's note
All claims expressed in this article are solely those of the authors and do not necessarily represent those of their affiliated organizations, or those of the publisher, the editors and the reviewers. Any product that may be evaluated in this article, or claim that may be made by its manufacturer, is not guaranteed or endorsed by the publisher.
Supplementary material
The Supplementary Material for this article can be found online at: https://www.frontiersin.org/articles/10.3389/fevo.2023.1162577/full#supplementary-material
References
Bohn, A., Matos, F. B., and Labiak, P. H. (2020). Taxonomy, distribution and conservation status of the fern genus Cyclodium (Dryopteridaceae). Willdenowia 50, 279–304. doi: 10.3372/wi.50.50213
Bohn, A., Smith, A. R., Matos, F. B., Moran, R. C., and Labiak, P. H. (2021). Phylogeny and character evolution of the neotropical fern Genus Cyclodium (Dryopteridaceae). Syst. Bot. 46, 916–928. doi: 10.1600/036364421X16370109698605
Bollback, J. P. (2006). SIMMAP: stochastic character mapping of discrete traits on phylogenies. BMC Bioinform. 7, 88. doi: 10.1186/1471-2105-7-88
Borges, R., Machado, J. P., Gomes, C., Rocha, A. P., and Antunes, A. (2019). Measuring phylogenetic signal between categorical traits and phylogenies. Bioinformatics 35, 1862–1869. doi: 10.1093/bioinformatics/bty800
Chen, C. W., Sundue, M., Kuo, L. Y., Teng, W. C., and Huang, Y. M. (2017). Phylogenetic analyses place the monotypic Dryopolystichum within Lomariopsidaceae. PhytoKeys 78, 83–107. doi: 10.3897/phytokeys.78.12040
Chen, F., Deng, S. H., and Sun, K. Q. (1997). Early Cretaceous Athyrium Roth from northeastern China. Palaeobotanist 46, 117–133. doi: 10.54991/jop.1997.1356
Crabtree, D. R. (1987). The early Campanian flora of the Two Medicine Formation, northcentral Montana. Missoula, MT: University of Montana.
Cruickshank, R. D., and Ko, K. (2003). Geology of an amber locality in the Hukawng valley, northern Myanmar. J. Asian Earth Sci. 21, 441–445. doi: 10.1016/S1367-9120(02)00044-5
Ding, H. H., Chao, Y. S., Callado, J. R., and Dong, S. Y. (2014). Phylogeny and character evolution of the fern genus Tectaria (Tectariaceae) in the Old World inferred from chloroplast DNA sequences. Mol. Phylogenet. Evol. 80, 66–78. doi: 10.1016/j.ympev.2014.06.004
Du, X., Lu, J., Zhang, L., Wen, J., Kuo, L., Mynssen, C. M., et al. (2021). Simultaneous diversification of Polypodiales and angiosperms in the Mesozoic. Cladistics 37, 518–539. doi: 10.1111/cla.12457
Fan, X. P., Lu, N. T., Li, C. X., Knapp, R., He, H., Zhou, X. M., et al. (2022). Phylogeny, biogeography, and character evolution in the fern family Hypodematiaceae. Mol. Phylogenet. Evol. 166, 107340. doi: 10.1016/j.ympev.2021.107340
Fawcett, S., and Smith, A. (2021). A Generic Classification of the Thelypteridaceae. Fort Worth, TX: Botanical Research Institute of Texas Press.
Grimaldi, D. A., Engel, M. S., and Nascimbene, P. C. (2002). Fossiliferous Cretaceous amber from Myanmar (Burma): its rediscovery, biotic diversity, and paleontological significance. Amer. Mus. Novit. 3361, 1–71. doi: 10.1206/0003-0082(2002)361<0001:FCAFMB>2.0.CO;2
Harmon, L. J., Weir, J. T., Brock, C. D., Glor, R. E., and Challenger, W. (2008). GEIGER: investigating evolutionary radiations. Bioinformatics 24, 129–131. doi: 10.1093/bioinformatics/btm538
Haug, J. T., Azar, D., Ross, A., Szwedo, J., Wang, B., Arillo, A., et al. (2020). Comment on the letter of the Society of Vertebrate Paleontology (SVP) dated April 21, 2020 regarding “Fossils from conflict zones and reproducibility of fossil-based scientific data”: Myanmar amber. Paläontol. Z. 94, 431–437. doi: 10.1007/s12542-020-00524-9
Holttum, R. E. (1983). The fern-genera Tectaria, Heterogonium and Ctenitis in the Mascarene Islands. Kew Bull. 18, 107–130. doi: 10.2307/4107974
Holttum, R. E. (1985). Studies in the fern genera allied to Tectaria Cav. IV. The genus Ctenitis in Asia, Malesia and the Western Pacific. Blumea 31, 1–38. doi: 10.2307/4103042
Kamilar, J. M., and Cooper, N. (2013). Phylogenetic signal in primate behaviour, ecology and life history. Philos. Trans. R. Soc. B: Biol. Sci., 368, 20120341. doi: 10.1098/rstb.2012.0341
Kettunen, E., Sadowski, E.-M., Seyfullah, L. J., Dörfelt, H., Rikkinen, J., and Schmidt, A. R. (2019). Caspary's fungi from Baltic amber: historic specimens and new evidence. Pap. Palaeontol. 5, 365–389. doi: 10.1002/spp2.1238
Kramer, K. U. (1990a). “Oleandraceae”, in The Families and Genera of Vascular Plants I, Pteridophytes and Gymnosperms, eds K. Kubitzki, and P. S. Green (Berlin: Springer-Verlag), 190–193. doi: 10.1007/978-3-662-02604-5_37
Kramer, K. U. (1990b). “Nephrolepidaceae”, in The Families and Genera of Vascular Plants I, Pteridophytes and Gymnosperms, eds K. Kubitzki, and P. S. Green (Berlin: Springer-Verlag), 188–190. doi: 10.1007/978-3-662-02604-5_36
Kramer, K. U. (1990c). “Davalliaceae”, in The Families and Genera of Vascular Plants I, Pteridophytes and Gymnosperms, eds K. Kubitzki and P. S. Green (Berlin: Springer-Verlag), 74–80. doi: 10.1007/978-3-662-02604-5_19
Kramer, K. U. (1990d). “Lomariopsidaceae”, in The families and genera of vascular plants I, Pteridophytes and gymnosperms, eds K. Kubitzki and P. S. Green (Berlin: Springer-Verlag), 164–170. doi: 10.1007/978-3-662-02604-5_28
Kramer, K. U., Chambers, T. C., and Hennipman, E. (1990b). “Blechnaceae”, in The Families and Genera of Vascular Plants I, Pteridophytes and Gymnosperms, eds K. Kubitzki, and P. S. Green (Berlin: Springer-Verlag), 60–68. doi: 10.1007/978-3-662-02604-5_16
Kramer, K. U., and Green, P. S. (1990). “Pteridophytes and gymnosperms,” in The Families and Genera of Plants I, ed K. Kubitzki (Berlin: Springer-Verlag), 1–404.
Kramer, K. U., Holttum, R. E., Moran, R. C., and Smith, A. R. (1990a). “Dryopteridaceae”, in The Families and Genera of Vascular Plants I, Pteridophytes and Gymnosperms, eds K. Kubitzki, and P. S. Green (Berlin: Springer-Verlag), 101–144. doi: 10.1007/978-3-662-02604-5_23
Kramer, K. U., and Viane, R. (1990). “Aspleniaceae”, in The Families and Genera of Vascular Plants I, Pteridophytes and Gymnosperms, eds K. Kubitzki, and P. S. Green (Berlin, Springer-Verlag), 52–57. doi: 10.1007/978-3-662-02604-5_14
Labiak, P. H., Sundue, M., Rouhan, G., Hanks, J. G., Mickel, J. T., and Moran, R. C. (2014). Phylogeny and historical biogeography of the lastreopsid ferns (Dryopteridaceae). Am. J. Bot. 101, 1207–1228. doi: 10.3732/ajb.1400071
Labiak, P. H., Sundue, M., Rouhan, G., and Moran, R. C. (2015). New combinations in Lastreopsis and Parapolystichum (Dryopteridaceae). Brittonia 67, 79–86. doi: 10.1007/s12228-014-9351-3
Lehtonen, S., Silvestro, D., Karger, D. N., Scotese, C., Tuomisto, H., Kessler, M., et al. (2017). Environmentally driven extinction and opportunistic origination explain fern diversification patterns. Sci. Rep. 7, 4831. doi: 10.1038/s41598-017-05263-7
Lellinger, D. B. (2002). A modern multilingual glossary for taxonomic pteridology. Pteridologia 3, 1–263. doi: 10.5962/bhl.title.124209
Li, C.-X., Ma, J. Y., Hao, J. S., and Yang, Q. (2022). On the systematic position of the early Cretaceous fern genus “Athyrium”. Palaeoworld. 32, 116–123. doi: 10.1016/j.palwor.2022.07.003
Li, C.-X., Moran, R. C., Ma, J.-Y., Wang, B., and Hao, J.-S. (2020). A new fossil record of Lindsaeaceae (Polypodiales) from the mid-Cretaceous amber of Myanmar. Cretac. Res. 105, 104040. doi: 10.1016/j.cretres.2018.12.010
Liu, H.-M., Zhang, X.-C., Wang, M.-P., Shang, H., Zhou, S.-L., Yan, Y.-H., et al. (2016). Phylogenetic placement of the enigmatic fern genus Trichoneuron informs on the infra-familial relationship of Dryopteridaceae. Plant Syst. Evol. 302, 319–332. doi: 10.1007/s00606-015-1265-3
Liu, H. M., He, L. J., and Schneider, H. (2014). Towards the natural classification of tectarioid ferns: confirming the phylogenetic relationships of Pleocnemia and Pteridrys (eupolypods I). J. Syst. Evol. 52, 161–174. doi: 10.1111/jse.12073
Liu, H. M., Jiang, R.-H., Guo, J., Hovenkamp, P., Perrie, L. R., Shepherd, L., et al. (2013). Towards a phylogenetic classification of the climbing fern genus Arthropteris. Taxon 62, 688–700. doi: 10.12705/624.26
Long, X., Peng, Y., Feng, Q., Engel, M. S., Shi, C., and Wang, S. (2023a). A new fossil fern of the Dryopteridaceae (Polypodiales) from the mid-Cretaceous Kachin amber. Palaeobio. Palaeoenv. doi: 10.1007/s12549-023-00572-4
Long, X., Peng, Y., Zhang, H., Fan, Y., Shi, C., and Wang, S. (2023b). Microlepia burmasia sp. nov., a new fern species from mid-Cretaceous Kachin amber of norther Myanmar (Dennstaedtiaceae, Polypodiales). Cretaceous. Res. 143, 105417. doi: 10.1016/j.cretres.2022.105417
Lu, N. T., Ebihara, A., He, H., Zhang, L., Zhou, X. M., Knapp, R., et al. (2019). A plastid phylogeny of the fern genus Arachniodes (Dryopteridaceae). Mol. Phylogenet. Evol. 133, 214–235. doi: 10.1016/j.ympev.2018.12.013
Mickel, J. T., and Smith, A. R. (2004). The pteridophytes of Mexico. Mem. N. Y. Bot. Gard. 88, 1–1054.
Moran, R. C., and Labiak, P. H. (2015). Phylogeny of the polybotryoid fern clade (Dryopteridaceae). Int. J. Plant Sci. 176, 880–891. doi: 10.1086/683393
Moran, R. C., and Labiak, P. H. (2016). Phylogeny and character evolution of the Neotropical fern genus Stigmatopteris (Dryopteridaceae). Brittonia 68, 476–488. doi: 10.1007/s12228-016-9437-1
Moran, R. C., Labiak, P. H., and Sundue, M. (2010). Phylogeny and character evolution of the bolbitidoid ferns (Dryopteridaceae). Int. J. Plant Sci. 171, 547–559. doi: 10.1086/652191
Moran, R. C., Prado, J., and Labiak, P. H. (2009). Megalastrum (Dryopteridaceae) in the West Indies. Brittonia 61, 273–292. doi: 10.1007/s12228-009-9081-0
Mynssen, C. M., Vasco, A., Moran, R. C., Sylvestre, L. S., and Rouhan, G. (2016). Desmophlebiaceae and Desmophlebium: a new family and genus of eupolypod II ferns. Taxon 65, 19–34. doi: 10.12705/651.2
Nitta, J., Schuettpelz, E., Ramírez-Barahona, S., and Iwasaki, W. (2022). An open and continuously updated fern tree of life. Front. Plant Sci. 13, 909768. doi: 10.3389/fpls.2022.909768
Pagel, M. (1999). Inferring the historical patterns of biological evolution. Nature 401, 877–884. doi: 10.1038/44766
Peretti, A. (2021). An alternative perspective for acquisitions of amber from Myanmar including recommendations of the United Nations Human Rights Council. J. Int. Humanit. Action 6, 12. doi: 10.1186/s41018-021-00101-y
Poinar, G. O. Jr. (2021). A new fern, Cladarastega burmanica gen. et sp. nov. (Dennstaedtiaceae: Polypodiales) in mid-Cretaceous Burmese amber. Palaeodiversity 14, 153–160. doi: 10.18476/pale.v14.a7
Poinar, G. O. Jr., and Buckley, R. (2008). Cretacifilix fungiformis gen. and sp. nov., an eupolypod fern (Polypodiales) in Early Cretaceous Burmese amber. J. Bot. Res. Inst. Texas 2, 1175–1182.
PPG I (2016). A community-derived classification for extant lycophytes and ferns. J. Syst. Evol. 54, 563–603. doi: 10.1111/jse.12229
Prado, J., and Moran, R. C. (2008). Revision of the neotropical species of Triplophyllum (Tectariaceae). Brittonia 60, 103–130. doi: 10.1007/s12228-008-9024-1
Prado, J., and Moran, R. C. (2016). Monograph of the West Indian fern genus Polystichopsis (Dryopteridaceae). Brittonia 68, 1–24. doi: 10.1007/s12228-015-9387-z
Punt, W., Hoen, P. P., Blackmore, S., Nilsson, S, and Le Thomas, A. (2007). Glossary of pollen and spore terminology. Rev. Palaeobot. Palynol. 143, 1–81. doi: 10.1016/j.revpalbo.2006.06.008
Regalado, L., Schmidt, A. R., Appelhans, M., Ilsemann, B., Schneider, H., Krings, M., and Heinrichs, J. (2017a). A fossil species of the enigmatic early polypod fern genus Cystodium (Cystodiaceae) in Cretaceous amber from Myanmar. Sci. Rep. 7, 14615. doi: 10.1038/s41598-017-14985-7
Regalado, L., Schmidt, A. R., Krings, M., Bechteler, J., Schneider, H., and Heinrichs, J. (2018). Fossil evidence of eupolypod ferns in the mid-Cretaceous of Myanmar. Plant Syst. Evol. 304, 1–13. doi: 10.1007/s00606-017-1439-2
Regalado, L., Schmidt, A. R., Müller, P., Kobbert, M. J., Schneider, H., and Heinrichs, J. (2017b). The first fossil of Lindsaeaceae (Polypodiales) from the Cretaceous amber forest of Myanmar. Cretaceous. Res. 72, 8–12. doi: 10.1016/j.cretres.2016.12.003
Regalado, L., Schmidt, A. R., Müller, P., Niedermeier, L., Krings, M., and Schneider, H. (2019). Heinrichsia cheilanthoides gen. et sp. nov., a fossil fern in the family Pteridaceae (Polypodiales) from the Cretaceous amber forests of Myanmar. J. Syst. Evol. 57, 329–338. doi: 10.1111/jse.12514
Ren, W., Wu, G., Han, L., Hua, Y., and Sun, B. (2022). New species of fossil Dryopterites from the Lower Cretaceous in the Zhongkouzi Basin, Beishan area, Northwest China, and its geological significance. Hist. Biol. 35, 84–91. doi: 10.1080/08912963.2021.2022135
Revell, L. J. (2012). phytools: an R package for phylogenetic comparative biology (and other things). Methods Ecol. Evol. 3, 217–223. doi: 10.1111/j.2041-210X.2011.00169.x
Revell, L. J., Harmon, L. J., and Collar, D. C. (2008). Phylogenetic signal, evolutionary process, and rate. Syst. Biol. 57, 591–601. doi: 10.1080/10635150802302427
Rothfels, C. J., Li, F.-W., Sigel, E. M., Huiet, L., Larsson, A., Burge, D. O., et al. (2015). The evolutionary history of ferns inferred from 25 low-copy nuclear genes. Am. J. Bot. 102, 1–19. doi: 10.3732/ajb.1500089
Rothfels, C. J., Sundue, M. A., Kuo, L. Y., Larsson, A., Kato, M., Schuettpelz, E., and Pryer, K. M. (2012). A revised family–level classification for eupolypod II ferns (Polypodiidae: Polypodiales). Taxon 61, 515–533. doi: 10.1002/tax.613003
Sadowski, E.-M., Schmidt, A. R., Seyfullah, L. J., Solórzano-Kraemer, M. M., Neumann, C., Perrichot, V., et al. (2021). Conservation, preparation and imaging of diverse ambers and their inclusions. Earth-Sci. Rev. 220, 103653. doi: 10.1016/j.earscirev.2021.103653
Schmidt, A. R., Korall, P., Krings, M., Weststrand, S., Bergschneider, L., Sadowski, E.-M., et al. (2022). Selaginella in Cretaceous amber from Myanmar. Willdenowia 52, 179–245. doi: 10.3372/wi.52.52203
Schneider, H., Schmidt, A. R., and Heinrichs, J. (2016). Burmese amber fossils bridge the gap in the Cretaceous record of polypod ferns. Perspect. Plant Ecol. Evol. Syst. 18, 70–78. doi: 10.1016/j.ppees.2016.01.003
Schneider, H., Schuettpelz, E., Pryer, K. M., Cranfill, R., Magallón, S., and Lupia, R. (2004). Ferns diversified in the shadow of angiosperms. Nature 428, 553–557. doi: 10.1038/nature02361
Schneider, H., Smith, A. R., and Pryer, K. M. (2009). Is morphology really at odds with molecules in estimating fern phylogeny? Syst. Bot. 34, 455–475. doi: 10.1600/036364409789271209
Schuettpelz, E., and Pryer, K. M. (2007). Fern phylogeny inferred from 400 leptosporangiate species and three plastid genes. Taxon 56, 1037–1050. doi: 10.2307/25065903
Schuettpelz, E., and Pryer, K. M. (2009). Evidence for a Cenozoic radiation of ferns in an angiosperm-dominated canopy. Proc. Natl. Acad. Sci. U.S.A. 106, 11200–11205. doi: 10.1073/pnas.0811136106
Shi, G.-H., Grimaldi, D. A., Harlow, G. E., Wang, J., Wang, J., Yang, M.-C., et al. (2012). Age constraint on Burmese amber based on U–Pb dating of zircons. Cretaceous. Res. 37, 155–163. doi: 10.1016/j.cretres.2012.03.014
Shmakov, A. I. (2015). The new system of family Woodsiaceae. Turczaninowia 18, 11–16. doi: 10.14258/turczaninowia.18.2.2
Smith, A. R. (1990). “Thelypteridaceae”, in The Families and Genera of Vascular Plants I, Pteridophytes and Gymnosperms, eds K. Kubitzki, and P. S. Green (Berlin: Springer-Verlag), 263–272. doi: 10.1007/978-3-662-02604-5_45
Smith, A. R., Pryer, K. M., Schuettpelz, E., Korall, P., Schneider, H., and Wolf, P. G. (2006). A classification for extant ferns. Taxon 55, 705–731. doi: 10.2307/25065646
Sundue, M. A., and Rothfels, C. J. (2014). Stasis and convergence characterize morphological evolution in eupolypod II ferns. Ann. Bot. 113, 35–54. doi: 10.1093/aob/mct247
Testo, W., and Sundue, M. (2016). A 4000-species dataset provides new insight into the evolution of ferns. Mol. Phylogenet. Evol. 105, 200–211. doi: 10.1016/j.ympev.2016.09.003
Tryon, A. F., and Lugardon, B. (1991). The Spores of Pteridophyta. Berlin: Springer. doi: 10.1007/978-1-4613-8991-0
Tryon, R. M., and Tryon, A. F. (1982). Ferns and Allied Plants with Special Reference to Tropical America. New York, NY: Springer Verlag. doi: 10.1007/978-1-4613-8162-4
Upchurch, G. R. Jr, and Mack, G. H. (1998). “Latest Cretaceous leaf megafloras from the Jose Creek Member, McRae Formation of New Mexico,” in Las Cruces Country II, eds G. H. Mack, G. S. Austin, and J. M. Barker (Socorro: New Mexico Geological Society Guidebook, 49th Field Conference), 209–222. doi: 10.56577/FFC-49.209
Voyta, L. L., Abramov, A. V., Lavrenchenko, L. A., Nicolas, V., Petrova, E. A., and Kryuchkova, L. Y. (2022). Dental polymorphisms in Crocidura (Soricomorpha: Soricidae) and evolutionary diversification of crocidurine shrew dentition. Zool. J. Linn. Soc. 196, 1069–1093. doi: 10.1093/zoolinnean/zlab103
Wang, Z. R., He, Z. R., and Kato, M. (2013). “Athyriaceae”, in Flora of China. 2–3 (Lycopodiaceae through Polypodiaceae), eds Z. Wu, P. H. Raven, and D. Hong (Beijing: Science Press; St. Louis: Missouri Botanical Garden Press), 418–534.
Yassin, A., Gidaszewski, N., Debat, V., and David, J. R. (2022). Long-term evolution of quantitative traits in the Drosophila melanogaster species subgroup Genetica 150, 343–353. doi: 10.1007/s10709-022-00171-9
Zhang, G. M., Xing, F. W., Wang, F. G., Iwatsuki, K., and Nooteboom, H. P. (2013a). “Hypodematiaceae”, in Flora of China. 2–3 (Lycopodiaceae through Polypodiaceae), eds Z. Wu, P. H. Raven, and D. Hong (Beijing: Science Press; St. Louis: Missouri Botanical Garden Press), 535–540.
Zhang, L., Schuettpelz, E., Rothfels, C. J., Zhou, X.-M., Gao, X.-F., and Zhang, L.-B. (2016). Circumscription and phylogeny of the fern family Tectariaceae based on plastid and nuclear markers, with the description of two new genera: Draconopteris and Malaifilix (Tectariaceae). Taxon 65, 723–738. doi: 10.12705/654.3
Zhang, L.-B., Wu, S., Xiang, J., Xing, F., He, H., Wang, F., et al. (2013b). “Dryopteridaceae”, in Flora of China. 2–3 (Lycopodiaceae through Polypodiaceae), eds Z. Wu, P. H. Raven, and D. Hong (Beijing: Science Press; St. Louis: Missouri Botanical Garden Press), 541–724.
Zhang, L.-B., and Zhang, L. (2015). Didymochlaenaceae: a new fern family of eupolypods I (Polypodiales). Taxon 64, 27–38. doi: 10.12705/641.4
Keywords: Cretaceous, Kachin amber, Polypodiales, Polypodiineae, phylogenetic signal, Burmese amber
Citation: Regalado L, Schneider H, Müller P and Schmidt AR (2023) Character evolution of modern eupolypods supports the assignment of the fossil fern Cretacifilix fungiformis to Dryopteridaceae. Front. Ecol. Evol. 11:1162577. doi: 10.3389/fevo.2023.1162577
Received: 09 February 2023; Accepted: 17 March 2023;
Published: 17 May 2023.
Edited by:
Mario Coiro, University of Vienna, AustriaReviewed by:
Candela Blanco Moreno, Autonomous University of Madrid, SpainMichael A. Sundue, University of Vermont, United States
Copyright © 2023 Regalado, Schneider, Müller and Schmidt. This is an open-access article distributed under the terms of the Creative Commons Attribution License (CC BY). The use, distribution or reproduction in other forums is permitted, provided the original author(s) and the copyright owner(s) are credited and that the original publication in this journal is cited, in accordance with accepted academic practice. No use, distribution or reproduction is permitted which does not comply with these terms.
*Correspondence: Ledis Regalado, bGVkaXNyZWdhbGFkbzc1JiN4MDAwNDA7Z21haWwuY29t