- School of Biological Sciences, Washington State University Vancouver, Vancouver, WA, United States
The provision of habitat on private agricultural land is vital to the persistence of native species. This study aimed to understand how cattle grazing management strategies influence butterfly behavior. We conducted behavioral experiments with two species of common native butterflies, silvery blue butterflies (Glaucopsyche lygdamus columbia) and ochre ringlet butterflies (Coenonympha california eunomia), at two native prairies with no grazing; two pastures grazed according to “conventional” management; and two pastures grazed according to “conservation” management. We mapped butterfly flight paths to quantify behavioral response to grazing management to test the hypothesis that butterflies have lower diffusion rates in ungrazed and conservation grazed prairies. We used turning angles, step lengths, and flight time to calculate diffusion rates for each individual. We assessed butterfly movement parameters as a function of sex, management type, and their interaction using generalized linear mixed models and used partial least squares regression to assess the effects of resource availability (host plant volume and nectar inflorescence count) on butterfly diffusion rates. We observed the highest silvery blue female step lengths and diffusion rates in conventional grazing and lowest in native prairie. There was moderate evidence that female silvery blue diffusion rates were higher in conservation grazing than native prairie. Neither silvery blue nor ochre ringlet males differed in their movement parameters between management types. Silvery blue diffusion rates were closely associated with their primary host plants (Lupinus spp.). We conclude that there is potential for conservation grazing to contribute to butterfly habitat in the landscape if grazing management practices focus on supporting a diverse plant community with host plants for focal species.
Introduction
In December 2022, in the fifteenth meeting of the Conference of the Parties, the International Union for the Conservation of Nature called for at least 30% of global ecosystems to be conserved by 2030 (IUCN, 2022). However, protected conservation areas alone are inadequate to preserve native species due to poor management, lack of funding, spatial isolation, and containing only a small proportion of total biodiversity (Watson et al., 2014; Butchart et al., 2015; Kamal et al., 2015; Cortés Capano et al., 2019). The provision of habitat outside of reserves is vital, as approximately as only 21.7% of IUCN red list species have adequate protection in reserves worldwide (Maxwell et al., 2020).
The IUCN also called for the recognition of the importance of agriculture in contributions to biodiversity and habitat connectivity in the face of massive habitat loss and degradation (IUCN, 2022). For example, while the United States has lost 86% of its grassland habitat since European colonization due to fire suppression, urbanization, invasive species introduction, and conversion to agriculture (Samson et al., 2004), approximately 85% of remaining grassland and pastureland is privately owned (Bigelow and Borchers, 2017). In addition, 90% percent of listed species are found solely or in part on private land (Brook et al., 2003).
Livestock grazing occupies more than a third of all land in the US (Bigelow and Borchers, 2017), and it is often considered to be one of the largest threats to grassland habitat (Fleischner, 1994; Noss, 1994). Grazing can influence grassland systems in both positive and negative ways through herbivory, trampling, and defecation, depending how the livestock are managed (Fleischner, 1994). Grazing may cause water pollution, soil compaction, erosion, reduced plant and animal diversity, local extirpation of sensitive species, and invasion of nonnative species (Fleischner, 1994; Manley et al., 1997). Conversely, livestock grazing may also maintain plant community diversity, reduce competitive dominance of invasive grasses, lower vegetation height, and create heterogeneity on a pasture and regional scale, which benefits many different taxa (Weiss, 1999; Pöyry et al., 2004; Jerrentrup et al., 2014; Zakkak et al., 2014; Beck et al., 2015; WallisDeVries et al., 2016; Neilly et al., 2018; Davis et al., 2020). Studies attempting to quantify the effects of grazing on various taxa often use occupancy or abundance of the focal species as an indicator of habitat quality.
However, higher occupancy or abundance of the focal species is not always sufficient to indicate higher habitat preference or higher habitat quality (Van Horne, 1983; Bock and Jones, 2004), though it is a common assumption. Studies using movement or behavior data investigate habitat from the perspective of the focal species (Schultz et al., 2019). A review by Crone et al. (2019) of 78 studies across taxa (n = 18 vertebrates, 29 non-lepidopteran arthropods, 23 lepidopterans, and 8 “others”) that categorized movement behavior paired with an independent measure of habitat quality, such as resource density or abundance, showed that animals reduce their rate of movement through high quality habitat compared to low quality. This is because animals exhibit “area restricted search” when encountering high quality habitat, meaning that they will either take shorter steps between turns or larger turning angles, or both (Kareiva and Odell, 1987; Korösi et al., 2008; Crone et al., 2019; Dorfman et al., 2022). This will effectively slow their rate of movement, or diffusion rate (Kuefler et al., 2010; Brown et al., 2017; Schultz et al., 2017; Pugesek and Crone, 2022). For example, Kuefler et al. (2012) showed that rotifer diffusion rates were lower with increased food availability and higher with increased rotifer population density. Many studies have quantified movement behavior to describe some aspect of habitat, land cover, or resources animals encounter (e.g. Revilla et al., 2004; Stevens et al., 2004; de Knegt et al., 2007; Kuefler et al., 2010; Lebeau et al., 2015; Murphy and Boone, 2022). An animal’s movement parameters (i.e. step lengths, turning angles, and diffusion rates) in different habitats can indicate perceived habitat quality and provide more information about habitat and management effects on a species than abundance or occupancy estimates alone (Crone et al., 2019; Schultz et al., 2019).
Butterflies are good indicators of ecosystem health because they use different parts of the ecosystem in adult and larval stages and are sensitive to changes in their environment (Kerr et al., 2000). Prominent studies on the effects of grazing on grassland butterflies in Europe have found positive effects of low-intensity cattle grazing over large areas (often referred to as extensive grazing) for butterfly communities and species (Pöyry et al., 2004; Thomas et al., 2009; Jerrentrup et al., 2014; WallisDeVries et al., 2016; but see Kruess and Tscharntke, 2002). There is a consensus within the European literature that historical megafaunal grazing and current extensive cattle grazing has created shifting mosaics of semi-natural grassland. This shifting mosaic increases heterogeneity and niches available for butterflies on a pasture and regional scale, while preventing forest encroachment (Balmer and Erhardt, 2000; Nilsson et al., 2008; Konvička et al., 2021).
There is comparatively little research on butterfly responses to grazing in North America (Bussan, 2022). Most of the existing research is concentrated in the Midwest, with mixed results (Vogel et al., 2007; Debinski et al., 2011; Smith and Cherry, 2014; Delaney et al., 2016). In addition, the North American literature has less of a focus than the European literature on both satisfying conservation goals and providing a livelihood for the farmer or rancher (Bussan, 2022). The relative paucity of information on North American butterfly responses to grazing makes management recommendations and predictions difficult because differing evolutionary histories of grazing and grassland types mean that direct comparisons to European butterflies are not always appropriate.
We conducted an experiment using two common native butterfly species in western Washington prairies in a landscape with working cattle and dairy farms. We compared butterfly diffusion rates as an index of habitat quality in grazed pastures and in native prairie to determine factors that influence their movement through these environments. We quantified aspects of the habitat in which butterflies have lower diffusion rates, assuming that perceived higher quality habitat results in lower diffusion rates. Using butterfly movement parameters as an indicator, we tested how cattle grazing management influenced behavioral responses to grazed habitat. We expected that butterfly diffusion rates would be highest in conventional grazing, intermediate in conservation grazing, and lowest in native upland prairie. We also explored how nectar and host plants and vegetation structure are associated with butterfly movement, given different habitat management. We expected that butterfly diffusion rates would differ depending on the resources available to them along their flight paths. Diffusion rates would be lower when nectar and host plant density was high and diffusion rates would be higher when nectar and host plant density was low.
Materials and methods
Study area
Prior to European colonization, western Washington prairies covered approximately 72,843 ha, but 97% of the original extent of the prairies have been lost due to fire suppression, urbanization, and conversion to agriculture (Washington Department of Fish and Wildlife, 2022). Remnant prairies are restricted to a few isolated reserves, a military base (Joint Base Lewis-McChord), or located on working cattle ranches and dairy farms (Washington Department of Fish and Wildlife, 2022). Remnants are often heavily degraded and invaded by nonnative plants. Western Washington prairies are botanically distinct from other North American prairies, characterized by short bunchgrasses such as Roemer’s fescue (Festuca roemeri) (Bowcutt and Hamman, 2016, pg. 21). First peoples, including Nisqually, Puyallup, Duwamish, Steilacoom, Squaxin Island, Chehalis, Clatsop, Cowlitz, Chinook, and many others maintained the prairies through cultural burning and traditional harvesting practices for thousands of years (Leopold and Boyd, 1999; Noland and Carver, 2011, pg. 2; Hamman et al., 2011; Velasco, 2021). Unlike the Great Plains and tallgrass prairies of the Midwest, bison (Bison bison) were historically not present in western Washington (Zontek, 2007, pg. 29); thus the prairies were primarily grazed by elk (Cervus canidensis) (Noland and Carver, 2011, pg. 2). The climate is Mediterranean, with warm, dry summers and wet, cool winters (Western Regional Climate Center, 2022).
Focal species and study sites
From April to September 2019, we observed two common native butterfly species in different grazing management treatments, which allowed us to account for differing habitat needs and phenology (Figure 1). The first species, the silvery blue butterfly (Glaucopsyche lygdamus; Doubleday, 1841; family Lycaenidae), is distributed throughout the western US (Pelham, 2021); we worked with the subspecies G.l. columbia (Skinner 1917). Their host species are members of the Fabaceae family, specifically lupines and vetches. The adults fly from the end of April to early June in Western Washington prairies (James and Nunnallee, 2011, pg. 206). The second species, ochre ringlet (Coenonympha california, Westwood 1851; family Nymphalidae) is also distributed throughout the western US (Pelham, 2021). Note that C. california’s taxonomy was recently changed; it was formerly C. tullia (Zhang et al., 2020). We worked specifically with the subspecies C.c. eunomia (Dornfeld, 1967) which is concentrated mainly in the South Salish Sea region (also known as South Puget Sound). Ochre ringlets are hosted by various grass species and are bivoltine. The adults fly from early May to mid-July and from late July to September (James and Nunnallee, 2011, pg. 326).
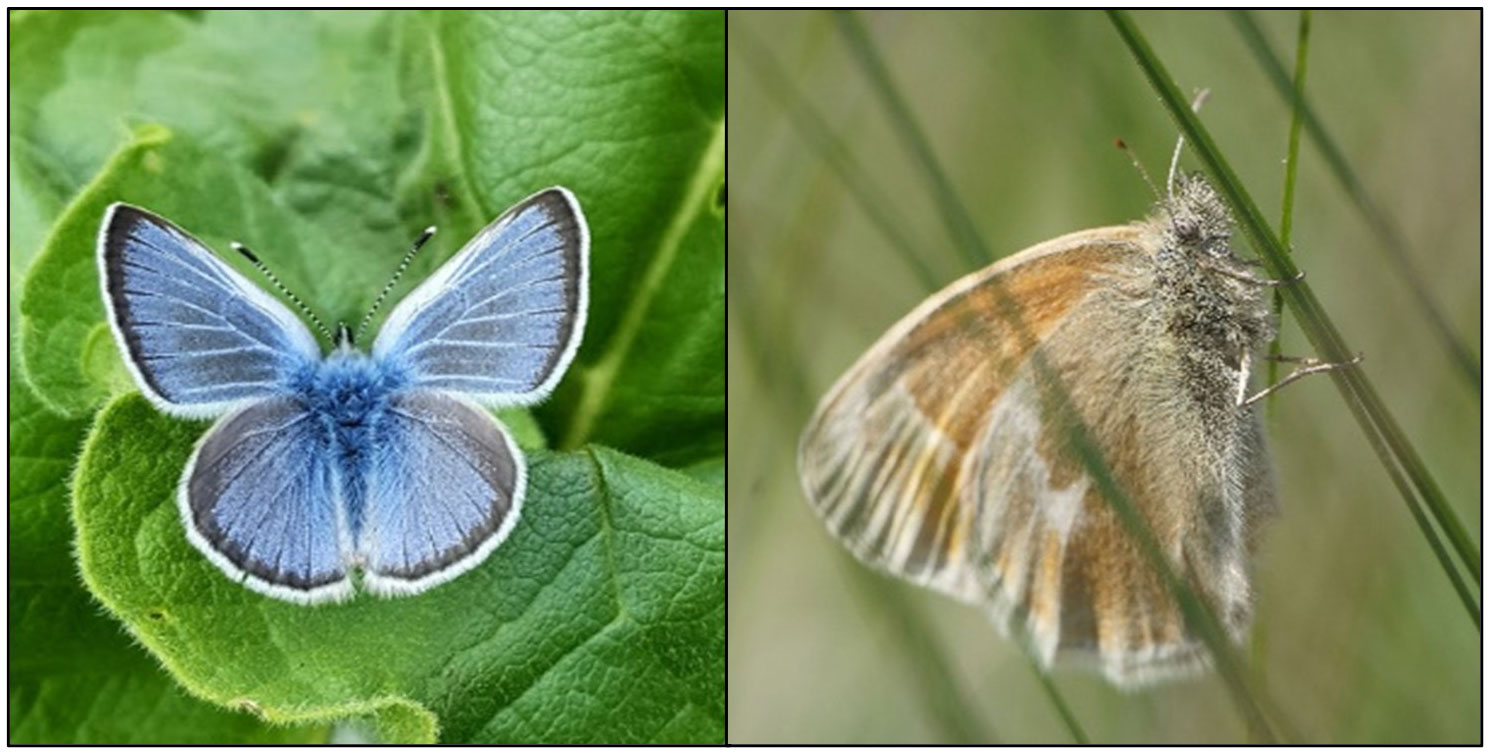
Figure 1 Silvery blue butterfly male (left; photo by Samantha Bussan) and ochre ringlet male (right; photo by Christopher Jason). Female silvery blue butterflies are brown. Ochre ringlets do not exhibit sexual dimorphism.
We chose six sites in western Washington (Table 1; Figure 2). Four are part of the South Salish Sea prairie ecosystem in Thurston County (Colvin Ranch, Riverbend Ranch, Johnson Prairie, and West Rocky Prairie) and two are part of the Boistfort prairie ecosystem in Lewis County (Maynard and Mary Mallonee’s farms). Four sites are grazed as part of active cattle and dairy farm operations. Of the grazed sites, two (Colvin Ranch and Mary Mallonee’s farm) are managed according to conservation grazing strategies, which involve rotational grazing with a spring deferment period (Farruggia et al., 2012; Ravetto Enri et al., 2017). The other two (Riverbend Ranch and Maynard Mallonee’s farm), are grazed according to conventional grazing strategies, i.e. continuous grazing with no spring deferment period. Rotational grazing is a grazing system that moves livestock regularly between paddocks to allow the plant community to recover between grazing periods. Continuous grazing is a grazing system that allows the livestock to have access to the entire pasture throughout the season (Blanchet et al., 2000). Both Maynard and Mary Mallonee’s farms fall under the umbrella of Mallonee Family Farms, which is a certified organic dairy farm, but they are located on different sites with different management regimes. The two native upland prairies (Johnson Prairie and West Rocky Prairie) are both part of the South Salish Sea prairie ecosystem and are managed with prescribed fire chemical and manual treatment of invasive plants, and native seeding. Johnson Prairie is located on Joint Base Lewis McChord and is owned by the US Department of Defense. West Rocky Prairie is owned by the Washington Department of Fish and Wildlife.
Butterfly behavior and movement observations
From April–September 2019, we quantified movement behavior at sites in different management categories (Table 1). As some of the grazed sites did not have native populations of the focal species, we collected individual butterflies from source sites (Supplementary Information) and transported them to the study site. Each individual was novel to the study site. To quantify movement behavior, following methods described by Schultz et al. (2012), we conducted observations by releasing an individual butterfly and following it for up to 15 distinct location points, for a maximum of 60 minutes. We chose the release point by haphazardly selecting a location within the study site as far from the site borders as possible, but within a resource patch if available. We recorded the individual’s behavior and marked its location with a pin flag every 15 seconds. If the butterfly remained in the same location for more than one 15 second interval, we recorded the number of intervals at that location, and waited to place the pin flag until the butterfly had moved from the location. An individual was considered to have changed locations once it moved more than 30 centimeters from the previous point, which was based on the accuracy of our GPS units (15–25 cm). All observers remained at least three meters away from the butterfly for silvery blues and four meters away for the more skittish ochre ringlets. After observation, each point on the individual’s flight path was recorded with a decimeter accuracy GPS unit (Thales ProMark™ 3 April through August 1st, Trimble© Geo 7X August 1st through the end of the season; decimeter accuracy was achieved in post processing of the GPS data).
Behavior types recorded included flying, sitting, basking, nectaring, ovipositing, plant walking, mud puddling, walking (on the ground), and mating (Schultz, 1998; Sei, 2009). Some behaviors are sex-specific; only males exhibit mud puddling behavior, and only females oviposit and plant walk. We recorded which plant species individuals chose for nectaring, ovipositing, or plant walking. We randomly selected four location points along each path using the app Random UX to measure habitat characteristics (host plants, nectar plants, and vegetation height). Within a meter’s radius of each point, we measured host plant volume (approximate width × length × height of each host plant “patch” within the plot) and counted flowering plant inflorescences. Following standard protocol for obtaining reliable measures of forage production, we used a Robel pole (Robel et al., 1970) placed on the center point to obtain an index of vegetation height. A Robel pole has alternating numbered bands for estimating the height of visual obstruction by the vegetation. A higher band number indicated taller vegetation.
Data analysis
We modeled butterfly movement as correlated random walks following the methods in Kareiva and Shigesada (1983) and Turchin (2015). We included only successful flight paths in our calculations; a flight path was considered successful if we recorded at least four distinct consecutive location points and we did not detect effects of the observer’s presence on butterfly behavior (e.g. angling their bodies to “hide” from the observer or evasive flight patterns). To analyze our flight path data, we first calculated the step lengths (distance between points within a path) and turning angles (deviation from the straight line) from our GPS data. Butterfly behavior can differ within habitat as compared to habitat boundaries; previous studies have shown that this change occurs within approximately 10–15 meters of habitat boundaries (Haddad, 1999; Schultz and Crone, 2001; Ross et al., 2005; Crone and Schultz, 2008; Schultz et al., 2012). As our goal was to assess within-habitat (i.e. within site or management type) behavior, we used ArcMap Pro version 2.9 to create a 10 m buffer around all pasture and prairie boundaries. All points and their associated step lengths and turning angles that intersected the buffer were removed from the following calculations, while retaining the path. We calculated the within-habitat expected net squared displacement and the diffusion rate (Supplementary Information) of each observed individual (Kareiva and Shigesada, 1983; Turchin, 2015, pgs. 102, 139). Diffusion rate is a metric that is derived from step lengths, turning angles, and time in flight (Turchin, 2015, pgs. 102, 139); it is important to consider both the direct measures of movement behavior and the diffusion coefficient because they may show different patterns of movement responses (e.g. Pugesek and Crone, 2022).
Effect of grazing management on butterfly movement behavior
We used similar methods for silvery blues and ochre ringlets. We fitted linear mixed models and generalized linear mixed models to examine differences in movement parameters by management type and sex. Preliminary data exploration showed potential side effects on silvery blue movement parameters. We log transformed move lengths to approximate normality and then used a linear mixed model (LMM) to evaluate them in relation to management type, sex, and an interaction between sex and management type. We also included a random effect of individual to account for pseudoreplication associated with repeated measurements of the same individual and a random effect of site. To assess turning angles as a measure of the tortuosity of the observation path (Turchin, 2015), we first calculated the cosine of the turning angles, which allowed us to assess how often individuals changed direction. More frequent direction reversals result in higher tortuosity (Turchin, 2015). Typical LMMs cannot be used with circular data (Schultz et al., 2012), so we scaled the cosine turning angles between zero (representing a 180° reversal in movement direction) and one (representing a completely straight line in movement direction) and logit transformed the resulting scaled cosines to approximate normality (Brown et al., 2017; Warchola et al., 2017). We used an LMM to assess the logit transformed scaled cosine of the turning angles in relation to management type, sex, and an interaction between sex and management type (Brown et al., 2017; Warchola et al., 2017). As above, we included random effects of individual and site. The step length and turning angle LMMs were fitted using restricted maximum likelihood (REML).
To evaluate diffusion rates, we used a generalized linear mixed model (GLMM), with diffusion rates as the response variable; sex, management type, and an interaction between sex and management type as fixed effects; and site as a random effect. We did not need to include a random effect of individual as diffusion rate is a path-level measurement and there was only one measurement of diffusion rate per individual. We used a gamma distribution with a log link for the response variable because the diffusion rates are bound by zero and infinity but have a greater mass towards zero. To assess whether there were differences in step lengths, turning angles, and diffusion rates across management types and between sexes, we compared estimated marginal means (Searle et al., 1980).
We followed similar procedures for ochre ringlet analysis. However, we were unable to include ochre ringlet females in the analysis due to low sample size per management type and site (Table 2). Therefore, the models used a similar framework to the silvery blues but used only male data and excluded female data. In addition, though ochre ringlets are bivoltine, we did not account for the two flights in the analysis due to low sample sizes in the second flight (Table 2).
Results are reported according to the framework in Muff et al. (2022), which describes relationships as having strong evidence, moderate evidence, or weak evidence according to the range of p-values as a “gradual language of evidence” rather than using an arbitrary cutoff value for statistical significance. In accordance with Muff et al. (2022), we report p-values between approximately 1 and 0.1 as having little or no evidence; p-values between 0.1 and 0.05 as weak evidence, between 0.05 and 0.01 as moderate evidence, between 0.01 and 0.001 as strong evidence, and <0.001 as very strong evidence.
Resource impacts on butterfly movement behavior
To assess the effects of habitat characteristics on butterfly movement behavior, we used partial least squares regression (PLSR; Wold, 1975). PLSR extracts latent factors that best explain, or maximize, the covariance between the explanatory and response variables (Chong and Jun, 2005; Carrascal et al., 2009). PLSR is more appropriate than traditional methods such as multiple regression or principal components analysis to assess multiple correlated explanatory variables in relation to the response variable (Chong and Jun, 2005; Carrascal et al., 2009; Scott and Crone, 2021). Several of our explanatory variables were correlated; Vicia sativa and Lupinus spp. can be both host and nectar plants for silvery blues, so host volume measurements and nectar inflorescence counts are correlated for those species.
For silvery blue butterflies, we fitted a PLSR model with diffusion rates as the response variable and potential nectar species, potential host species, site, and sex as explanatory variables. We selected potential nectar and host species for inclusion in the model based on whether we observed individual butterflies attempt nectaring or oviposition on that species at least once during the 2019 flight period. Of the three sites on which large perennial lupines were present, Lupinus oreganus was found only on one site (Mary Mallonee’s farm), while a related species, L. albicaulis, was found on both other sites. To account for this, we combined L. oreganus and L. albicaulis nectar counts and host volume measurements to model them as Lupinus spp. nectar and Lupinus spp. host volume. PLSR centers the data as part of the algorithm (Mevik and Wehrens, 2007). The predictor variables were scaled by dividing each variable by its standard deviation. We validated the model using leave-one-out (LOO) cross validation. We assessed statistical significance of the components based on the minimum value of the root mean square error in the projection (RMSEP), and on the percentage of variation in the diffusion rates explained by each component (Supplementary Information) (Chong and Jun, 2005; Carrascal et al., 2009).
We followed the same approach for ochre ringlets, with the following differences. First, instead of using individual grass species for host availability, we used the median Robel index number per path as a proxy for vegetation height and therefore amount of grass available. We were able to include female ringlet paths for this analysis, but we were unable to account for site due to the limited number of female paths on some sites (Table 2).
We assessed the effects of our predictor variables through two complementary methods: variable importance in the projection (VIP) and the regression coefficients (Chong and Jun, 2005). VIP qualitatively assesses the importance of each variable using the vector of loading weights on the components (Mehmood et al., 2012). Generally, a variable is considered important if the VIP value is above 1, though values above 0.8 may be marginally important (Mehmood et al., 2012). We used the regression coefficients to interpret the direction and magnitude of the effect of the predictor variables on butterfly diffusion rates.
Package list
All analyses were completed in R version 4.2.1 (R Core Team 2022). We used the following packages: moveHMM version 1.9 (Michelot et al., 2016) for calculating move lengths and turning angles; lme4 version 1.1-30 (Bates et al., 2015) for LMMs and GLMMs; emmeans version 1.8.1-1 (Lenth, 2022) for estimated marginal means; DHARMa version 0.4.6 (Hartig, 2022) for model diagnostics; pls version 2.8-1 (Liland et al., 2022) and plsVarSel version 0.9.8 (Mehmood et al., 2012) for partial least squares regression; and Tidyverse 1.3.2 (Wickham et al., 2019) for data processing and figure generation.
Results
We obtained 116 successful butterfly flight paths throughout the season, with 61 silvery blue and 55 ochre ringlet observations (Table 2). Our initial tally included 124 flight paths, but we excluded five male ochre ringlet paths where the GPS failed to record data; two male silvery blue paths that contained too many points in the border buffer zone to allow for diffusion rate calculations; and one silvery blue female whose field notes indicated that we had affected her behavior. Our observations lasted an average of 16.0 minutes for silvery blue females (ranged 1 min to 60 mins) and 14.2 minutes for silvery blue males (range: 1–58 min). We observed ochre ringlet females for an average of 36.8 minutes (range: 2.5–60 mins) and males for 12.4 minutes (range: 1.3–60 mins). Across all sites and management types, we calculated a median diffusion rate of 3.7 m2/s (range: 0.4–17.7 m2/s) for silvery blue males and 2.3 m2/s (range: 0.1–25.6 m2/s) for silvery blue females. We calculated a median diffusion rate of 3.9 m2/s (range: 0.2–32.8 m2/s) for ochre ringlet males and 2.4 m2/s (range: 0.2–14.4 m2/s) for ochre ringlet females.
Effect of grazing management on butterfly movement behavior
Silvery blue butterflies
Males and females differed in their responses to management types in terms of step lengths and diffusion rates (Table 3; Figures 3A, C; Supplementary Information Table 1). There was strong evidence that female diffusion rates were lower in native prairie than conventional grazing, moderate evidence that diffusion rates were lower in native prairie than in conservation grazing, and no evidence that diffusion rates differed between conservation and conventional grazing (Table 3; Figure 3C). There was moderate evidence that females took shorter steps in native upland prairie than conventional grazing, while there was weak evidence that their step lengths were shorter in conservation grazing than conventional grazing and no evidence that their step lengths differed between conservation grazing and native upland prairie (Table 3; Figure 3A). Males did not differ in their move lengths or diffusion rates between management types (Figures 3A, C). We did not observe differences between sex or management type in terms of the logit transformed cosine turning angles (Table 3; Figure 3B).
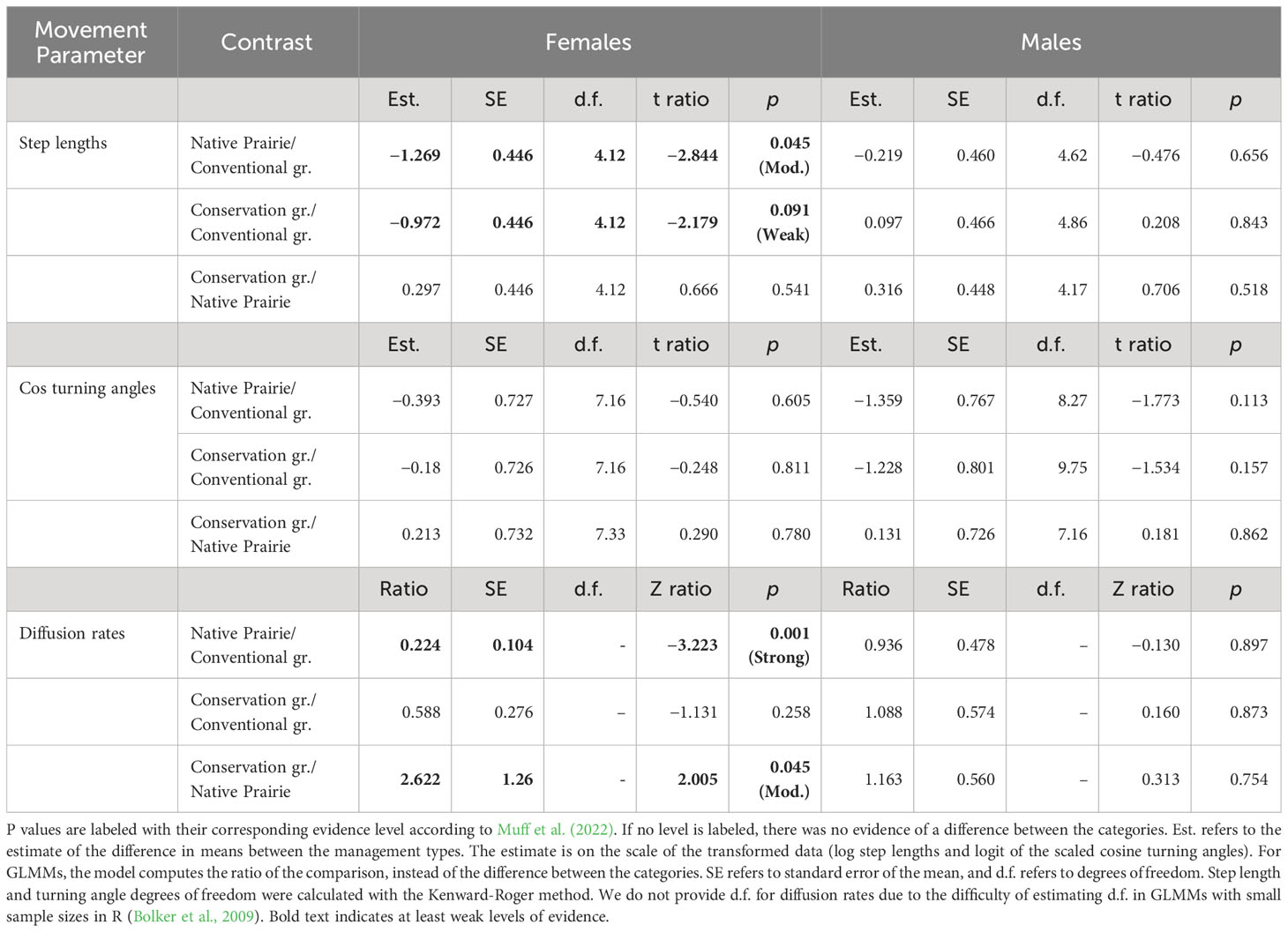
Table 3 Results from silvery blue estimated marginal means comparison of step lengths, turning angles, and diffusion rates.
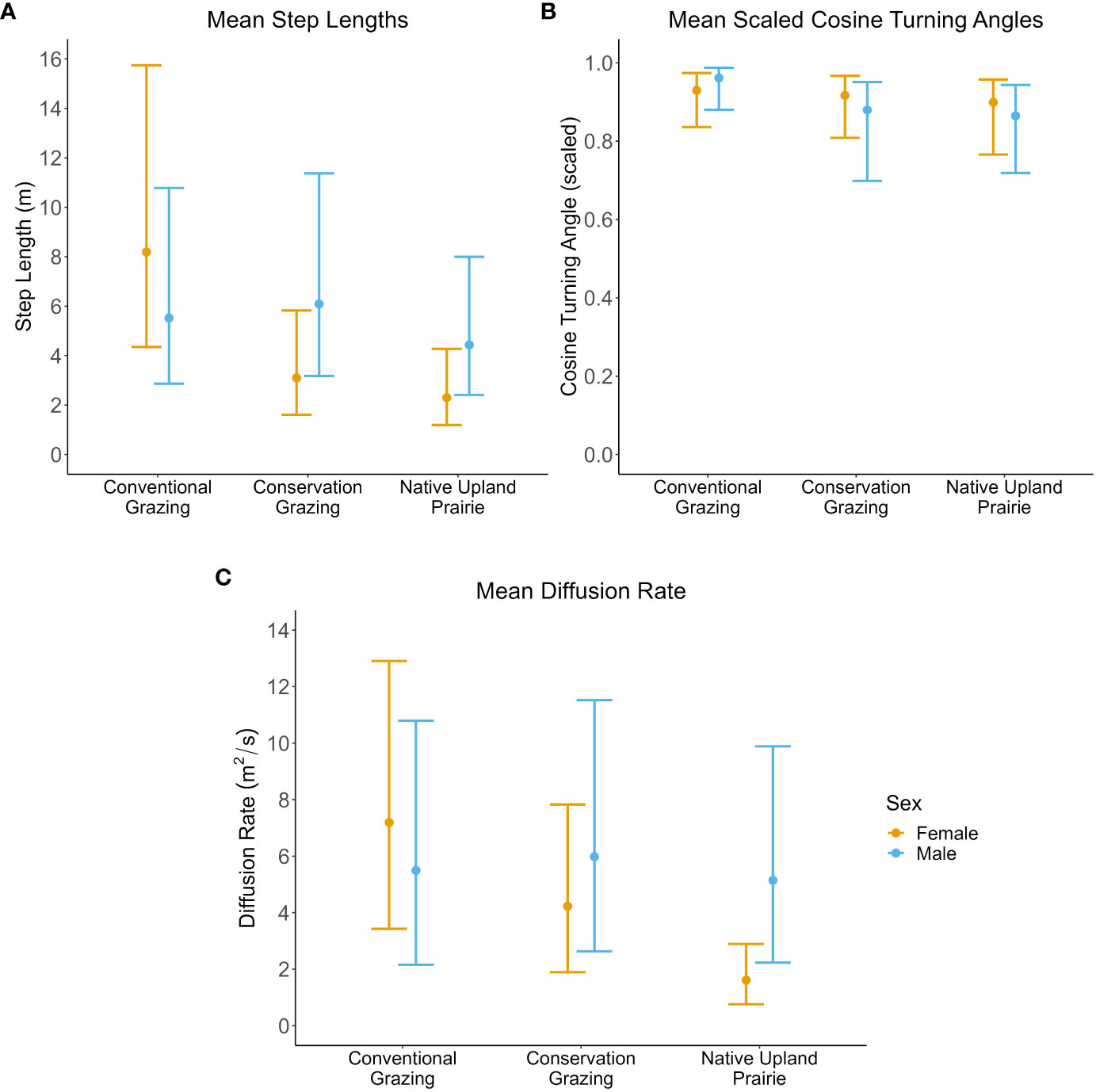
Figure 3 Silvery blue movement parameters by management type and sex. All error bars represent bootstrapped 95% prediction intervals. Female movement parameters are in yellow and male movement parameters are in blue. (A) Mean step length in meters. (B) Mean scaled cosine of the turning angles. Cosine turning angles were scaled between 0 (180° reversal) and 1 (straight line). (C) Mean diffusion rate in meters squared/second.
Ochre ringlets
There was no evidence of an effect of management type on ochre ringlet male step lengths, cosine turning angles, or diffusion rates (Table 4; Figures 4A–C; Supplementary Information Table 2). Their behavior was similar across all management types.
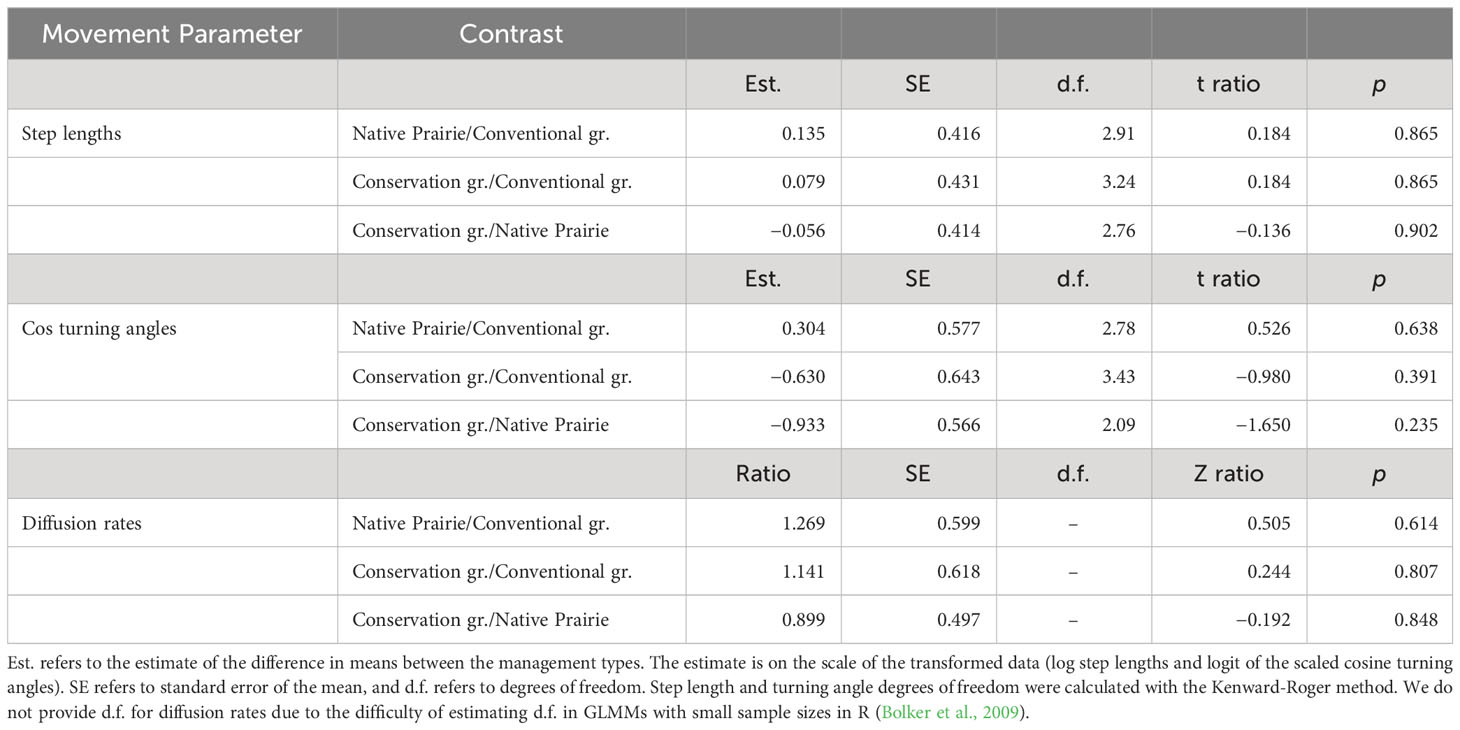
Table 4 Results from ochre ringlet male estimated marginal means comparison of step lengths, turning angles, and diffusion rates.
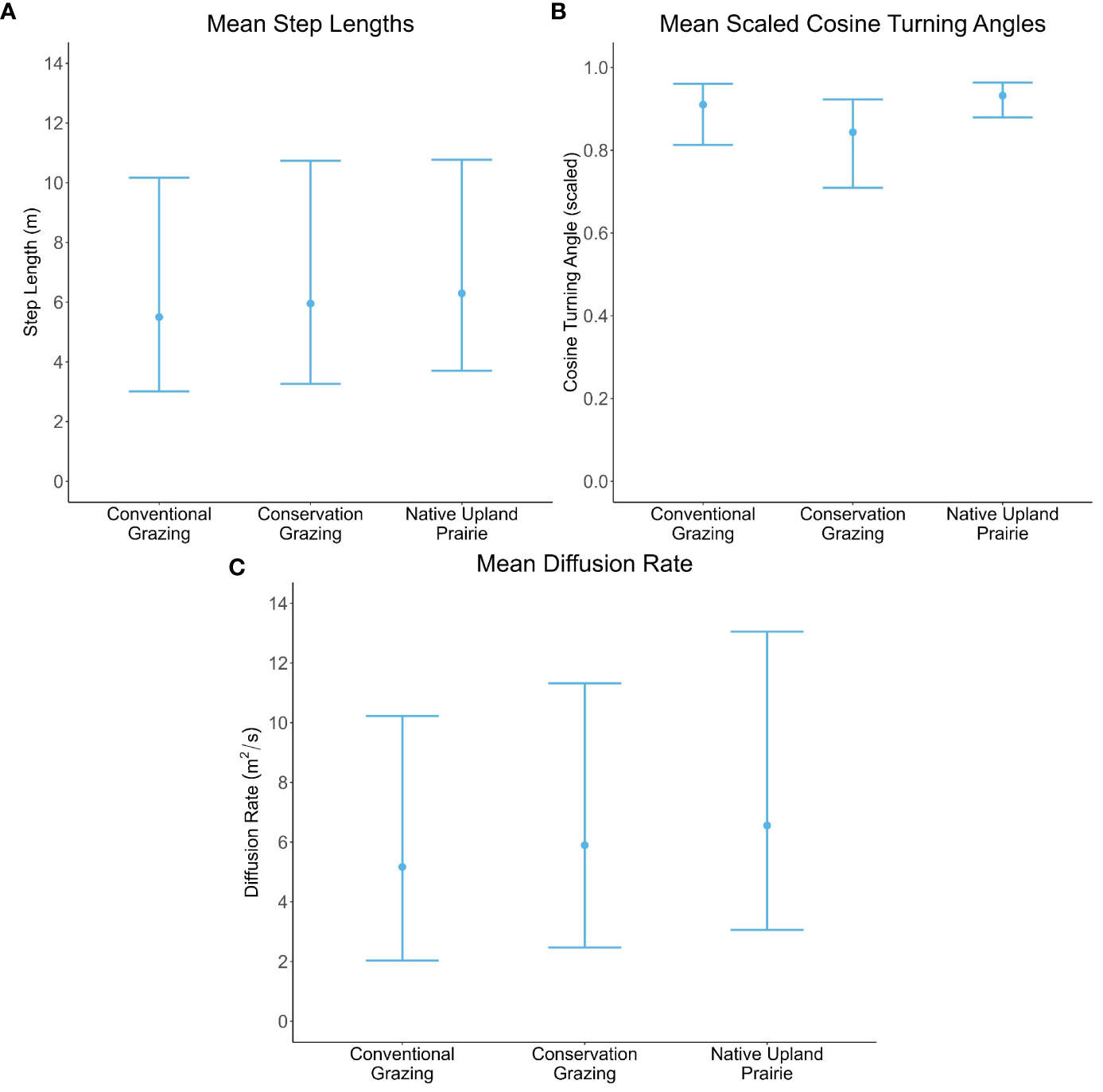
Figure 4 Male ochre ringlet movement parameters by management type. All error bars represent bootstrapped 95% prediction intervals. (A) Mean step length in meters. (B) Mean cosine of the turning angles. Cosine turning angles were scaled between 0 (180° reversal) and 1 (straight line). (C) Mean diffusion rate in meters squared/second.
Resource impacts on butterfly movement behavior
Silvery blue butterflies
We observed 40 flower species in bloom across the six sites throughout the silvery blue butterfly flight season (Supplementary Information Table 3). We observed silvery blues nectaring or attempting to nectar on thirteen species (Table 5; Figure 5A). We observed oviposition and plant walking behavior from a total of 11 individuals. Three females showed oviposition and plant walking behavior at Johnson Prairie; three females oviposited and plant walked and an additional two individuals plant walked at Mary Mallonee’s farm; and one individual oviposited, one oviposited and plant walked, and one individual plant walked at West Rocky Prairie. Host plant availability differed greatly across the six sites (Figure 5B). Though Vicia sativa is a potential host plant, all oviposition and plant walking behavior during our observations occurred on large perennial Lupinus spp. (L. oreganus and L. albicaulis). We saw only one instance of an individual exhibiting plant walking behavior on V. sativa, at Riverbend Ranch, which occurred after the observation had already ended. The individual left the plant without laying an egg.
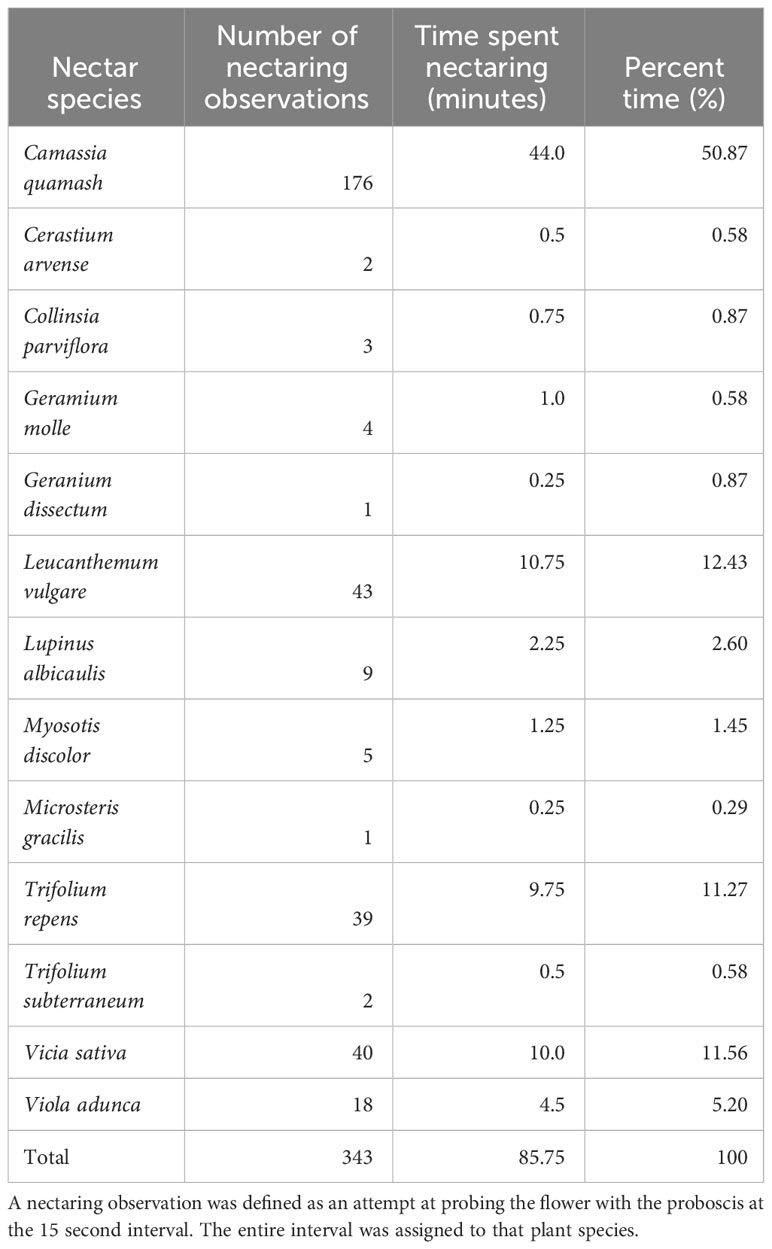
Table 5 Silvery blue nectaring observations, time spent, and percent time nectaring on each plant species.
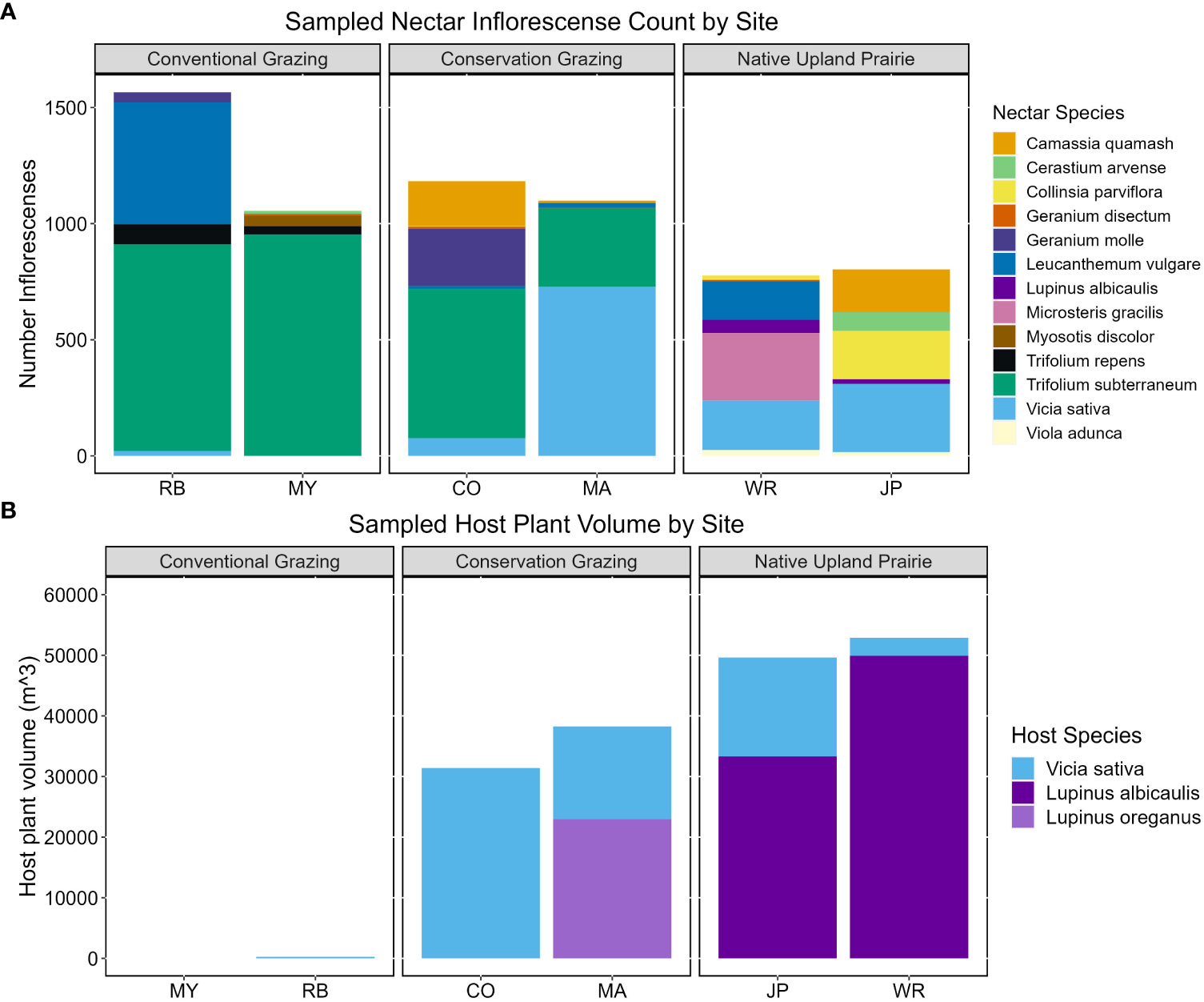
Figure 5 (A) Sampled silvery blue nectar species inflorescence count summed by site over the flight season. Nectar inflorescences were sampled on a path scale. (B) Sampled silvery blue host volume measurements summed by site in m3. Host volume was sampled on a path scale.
Our silvery blue PLSR results and model validation (Supplementary Information) indicated that the first two components were significant and cumulatively accounted for 54.25% of the variation in silvery blue diffusion rates (R2; 46.65% and 7.60% respectively). Our VIP results and regression coefficients indicated that the sites Riverbend Ranch (conventional grazing) and West Rocky Prairie (native upland prairie) had strong but opposite effects on silvery blue diffusion rates; diffusion rates were higher at Riverbend than West Rocky Prairie (Figures 6A–C). Riverbend had the highest VIP value and largest regression coefficients in the model (Figures 6A–C). Lupinus spp. volume and nectar count as well as V. sativa nectar count also had high VIP values and were associated with lower diffusion rates (Figures 6A–C). V. sativa host volume and most nectar species had little influence on silvery blue diffusion rates, with a few exceptions. C. parviflora and L. vulgare nectar counts both had high VIP values and were associated with higher diffusion rates (Figures 6A–C). Despite 50% of silvery blue nectaring behavior taking place on Camassia quamash (Table 5), C. quamash had little effect on diffusion rates (Figure 6C). V. sativa nectar and host measurements were less closely associated with each other than Lupinus spp. nectar and host measurements were (Figure 6D). Both components were loosely associated with a gradient of diffusion rates. Lupinus spp. host and Lupinus spp. nectar were associated with lower diffusion rates on both components. Variables such as site (Riverbend), which is a conventional grazing site, and L. vulgare were associated with higher diffusion rates on Component 1. On Component 2, site (Maynard Mallonee’s farm), which is the other conventional grazing site, was associated with higher diffusion rates (Figure 6D).
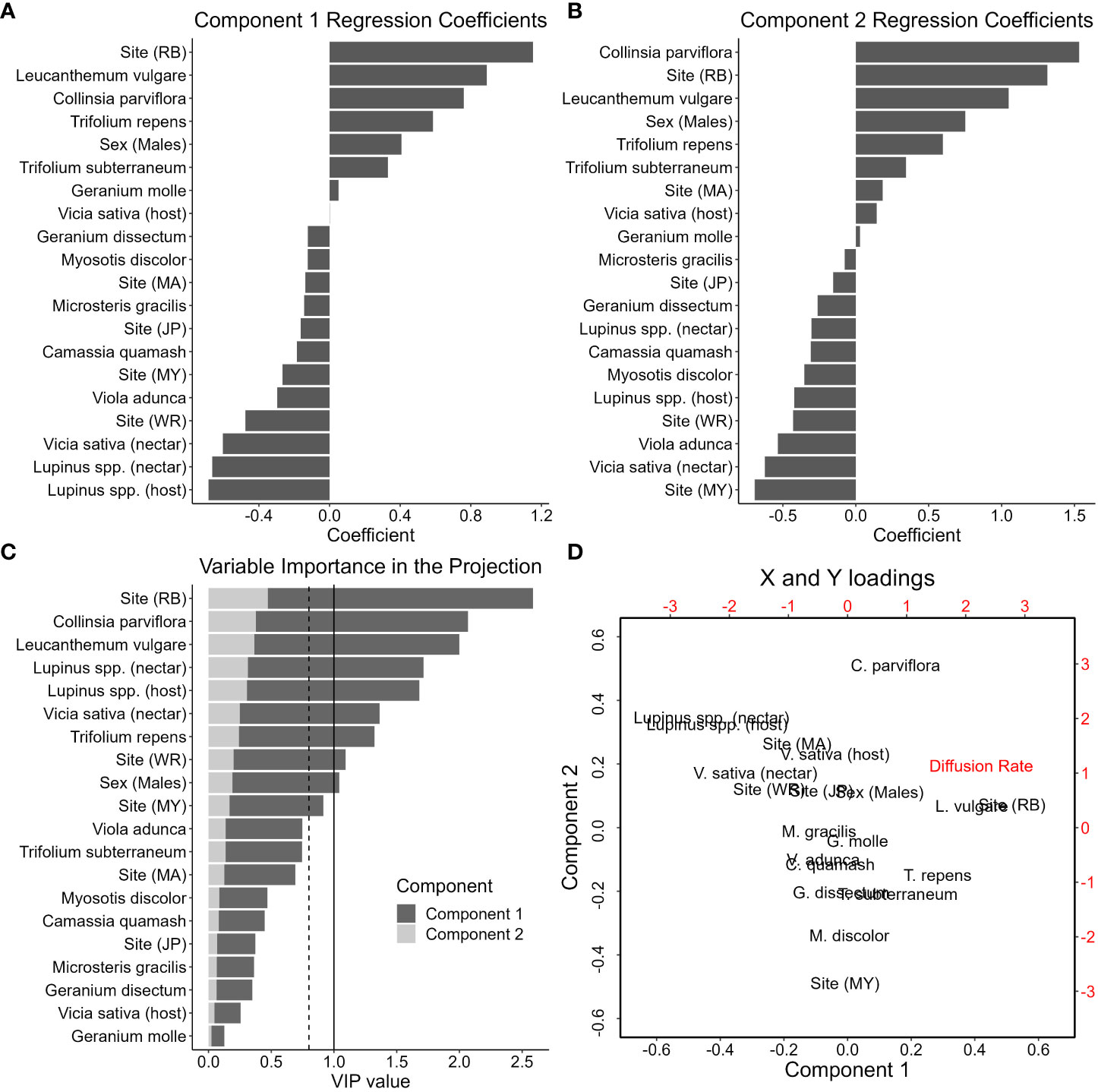
Figure 6 Results from the silvery blue PLSR model. (A) Regression coefficients from Component 1. (B) Regression coefficients from Component 2. (C) VIP loadings on Component 1 and Component 2. The solid line represents a VIP value of 1 (an important variable in the projection). The dotted line represents a VIP value of 0.8 (marginally important variable in the projection). (D) Biplot of predictor variable (X) and response variable (Y) loadings. X loadings are in black text and Y loadings are in red text.
Ochre ringlets
We observed 37 flower species in bloom across both ochre ringlet flight seasons (Supplementary Information Table 4). We observed ochre ringlets nectaring or attempting to nectar on 11 different species throughout both seasons (Table 6; Figure 7). L. vulgare and Daucus carota were the two most used nectar species (Table 6). We observed three females exhibiting oviposition behavior, one of which was on Carex inops, and the other two on thatch. We also observed plant walking behavior from four other individuals, all of which was spent crawling around in thatch near the base of plants. Ringlet female oviposition and plant walking behavior occurred in all three management types.
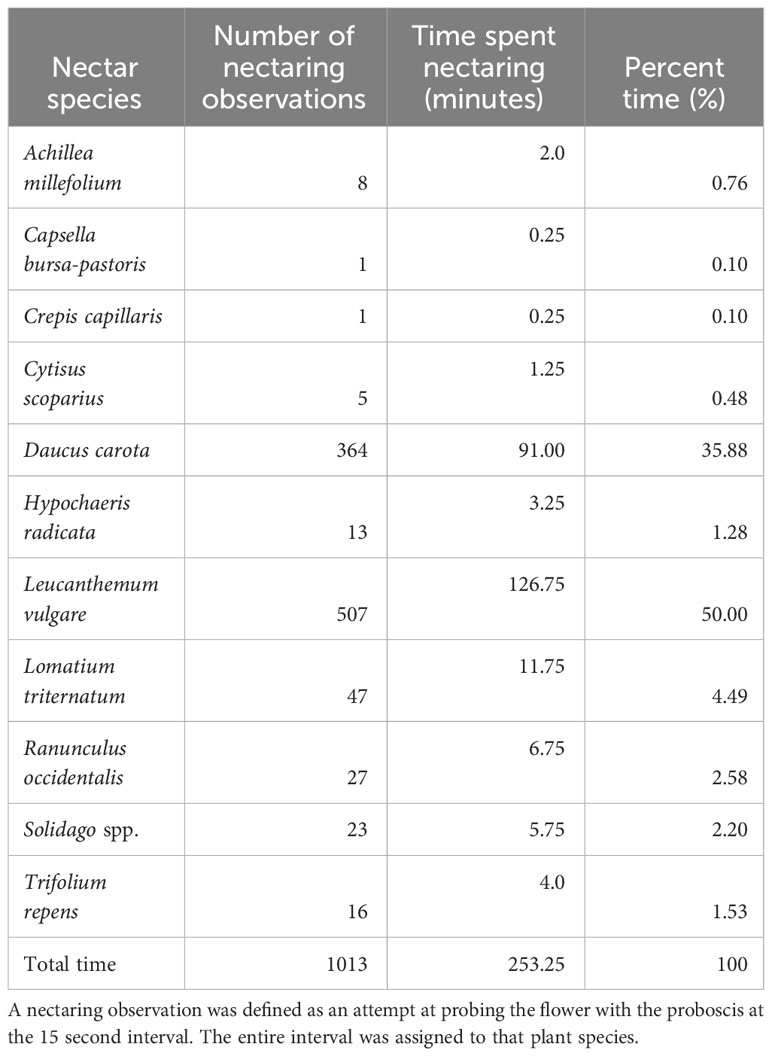
Table 6 Ochre ringlet nectaring observations, time spent, and percent time nectaring by plant species.
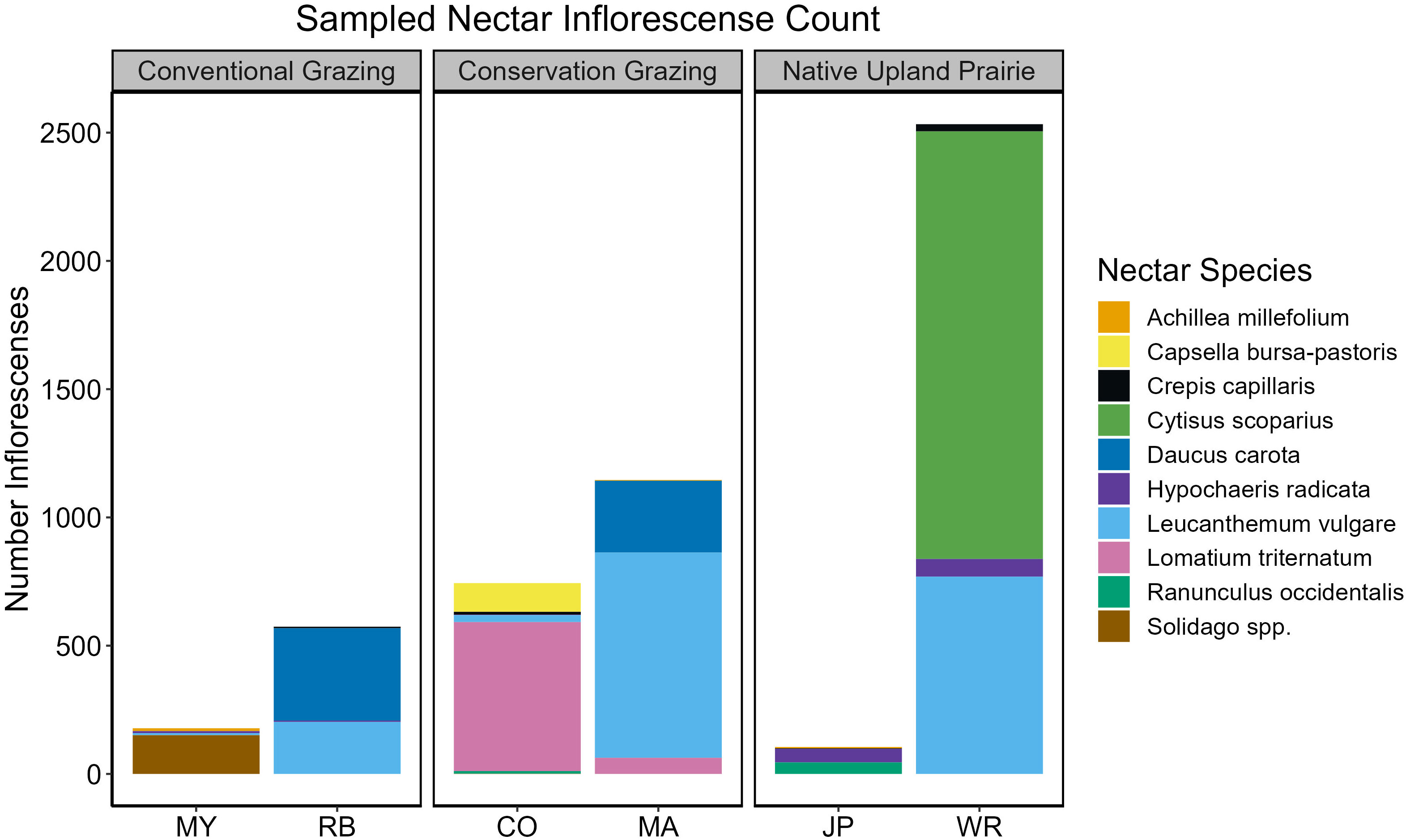
Figure 7 Sampled ringlet nectar species inflorescence count summed by site over both flight seasons. Nectar inflorescences were sampled on a path scale.
The ochre ringlet PLSR model was unstable because there was too little variation in the model; we were unable to use LOO to validate our model. Therefore we are unable to report the results of the ochre ringlet PLSR.
Discussion
Animal movement behavior has been used in many contexts to evaluate aspects of habitat or habitat quality (e.g. Dumont et al., 2007; Korösi et al., 2008; Dias et al., 2009; Dodge et al., 2014; Crone et al., 2019; Murphy and Boone, 2022; Pugesek and Crone, 2022); however, studies of butterfly movement have rarely been applied to evaluate habitat under grazing management (Bussan, 2022; but see Schtickzelle et al., 2007; Ehl et al., 2019). We quantified within-habitat diffusion rates to understand butterfly perceptions of habitat quality in conventional grazing, conservation grazing, and native upland prairie. We conclude that there is potential for conservation grazing to contribute to habitat in the landscape for native butterflies as long as pastures are managed to encourage diverse plant communities and important resources for focal species.
Effect of grazing management on butterfly movement behavior
Animals take shorter steps, larger turning angles, and have smaller diffusion rates in habitats that they perceive as high quality (Crone et al., 2019). This pattern has frequently been observed in butterflies; many studies have found evidence that butterflies have higher diffusion rates (Schultz, 1998; Fownes and Roland, 2002; Ovaskainen, 2004; Ross et al., 2005; Brown et al., 2017; Schultz et al., 2017; Warchola et al., 2017), longer steps (Stanton, 1982; Fownes and Roland, 2002; Ross et al., 2005; Brown et al., 2017; Schultz et al., 2017) and smaller turning angles in matrix or lower quality habitats than in high quality habitats (Brown et al., 2017; Schultz et al., 2017; Warchola et al., 2017). Our observed median diffusion rates for both species within habitat types were comparable to other similar studies. For example, Schultz et al. (2017) observed lycaenids of similar size to silvery blues (25–30 mm wingspan) to have diffusion rates approximately 0.1–6.9 m2/s in field margins with good nectar resources, 0.1–2.6 m2/s in seminatural habitats, and 1.4–15.1 m2/s in agricultural fields. The authors observed slightly higher diffusion rates for nymphalids than we observed for ochre ringlets, but the nymphalids in their study were slightly larger (> 40 mm wingspan compared to 35 mm ochre ringlets) and wing span is strongly correlated with diffusion rate (Schultz et al., 2017). They observed nymphalid species to have median diffusion rates approximately 6.7–48.3 m2/s in field margins, 1.5–14.3 m2/s in seminatural grasslands, and 2.77–12.95 m2/s in agricultural fields.
In our study, male and female silvery blues differed in their responses to management type; female diffusion rates and step lengths differed among management types, while males did not differ in any movement parameter between management types. Female silvery blue turning angles did not differ among habitat types, though they still exhibited area restricted search behavior through reduced step lengths and lower diffusion rates in native upland prairie (Dorfman et al., 2022). Female diffusion rates and step lengths were highest in conventional grazing and lowest in native upland prairie, suggesting that females exhibited area restricted search more often in native upland prairie (Dorfman et al., 2022; Pugesek and Crone, 2022) and perceived prairie as higher quality habitat than conventionally grazed pastures (Crone et al., 2019). This is likely because of the higher host plant availability in native prairie than conventional grazing (see Discussion: Resource impacts on butterfly movement behavior below). Many other studies have found that female butterflies have lower diffusion rates and step lengths in high quality habitat, which is often characterized by high host plant availability (Root and Kareiva, 1984; Schultz, 1998; Schultz et al., 2012; Brown et al., 2017; Warchola et al., 2017).
Female diffusion rates were higher in conservation grazing than native upland prairie, while female step lengths were lower in conservation grazing than conventional grazing. These results suggest that female silvery blues perceived the conservation grazing sites as intermediate quality between conventional grazing and native upland prairie (Crone et al., 2019; Schultz et al., 2019). In highly fragmented, human dominated landscapes, it may not be possible to provide enough high quality habitat on reserves to maintain species populations. Other studies have shown that intermediate quality habitat can still contribute to resource availability and increase animal populations in the landscape. For example, Kahara et al. (2022) found that each 100 ha increase in intermediate quality habitat over poor quality in California’s Central Valley resulted in 86 more mallards (Anas platyrhynchos) using the habitat. In addition, models accounting for the presence of intermediate habitat in addition to low and high quality performed better than models accounting for only low and high quality (Kahara et al., 2022). Our results provide support for the importance of intermediate quality habitat and the importance of providing this outside of reserves; female silvery blues still oviposited and took shorter step lengths in conservation grazing than conventional grazing, though this was heavily dependent on the resources present (see Resource impacts on butterfly movement behavior).
Both silvery blue males and ochre ringlet males showed little difference in any movement parameter between management types, which is unsurprising. Male butterflies are often less sensitive to habitat than females because of differing behavioral traits (Fischer et al., 1999). Males tend to focus on finding mates, while female butterflies must oviposit and are therefore usually more closely associated with their host plants (Rusterholz and Erhardt, 2000; Fischer and Fiedler, 2001; Schultz et al., 2012). We were unable to obtain enough female ochre ringlet observations to include them in the grazing management analysis, but their median diffusion rates across all sites were also lower than the median male diffusion rates. It is likely that if ochre ringlets do respond in terms of their movement behavior to differences in habitat quality between management types, the response would be driven by the females (Rusterholz and Erhardt, 2000; Fischer and Fiedler, 2001; Sei, 2009).
We tested butterfly diffusion rates under different types of grazing management. Rotational grazing is frequently recommended as a way to improve habitat heterogeneity and therefore butterfly community diversity in the landscape (e.g. Balmer and Erhardt, 2000; Pöyry et al., 2004; WallisDeVries et al., 2016), yet little attention has been paid in the literature to quantitatively testing the effects of continuous and rotational grazing on butterfly populations or communities (Bussan, 2022). Ravetto Enri et al. (2017) found “biodiversity-friendly” cattle grazing systems, or rotational grazing with a rest period for one paddock during the main flowering period, to have positive effects on butterfly abundance, richness, and flower cover. However, an important confounding factor is stocking rate. Farruggia et al. (2012) compared rotational grazing with a rest period for one paddock to continuous grazing at low and high stocking rates. At low stocking rates, there was little difference in butterfly species richness or abundance between management types, but at high stocking rates, richness and abundance was higher in rotational grazing than continuous. In our study, there was some evidence that silvery blue females did perceive rotational grazing with a spring rest period (conservation grazing) as higher quality than conventional grazing.
Both silvery blue butterflies and ochre ringlets are common, relatively generalist species. As a result, they may not react as strongly to differences between sites and management types as a more sensitive species (Murphy et al., 2011; Henry et al., 2019). Even our “conventional” grazing pastures did not cover the full range of grazing practices in the region, as producers willing to allow access to their land for butterfly experiments were already interested in conservation. Two grazed sites in the experiment were certified organic farms (Mary and Maynard Mallonee’s farms), and the other two, regardless of grazing strategy, use little to no pesticides, which is not the case at all farms and ranches in the region. All four grazed sites maintain a minimum stubble height of three inches (7.6 cm) in accordance with accepted sustainable grazing practices for the region (Fransen et al., 2017). It is possible that we may have seen more of a contrast in movement rates if we had access to pastures that were grazed more heavily or used pesticides.
Resource impacts on butterfly movement behavior
The impact of cattle grazing on butterflies is mediated by grazing effects on resources (host plants and nectar plants) (Kruess and Tscharntke, 2002; Schtickzelle et al., 2007; van Klink et al., 2015). Consistent with many previous studies on animal movement (e.g. Zalucki and Kitching, 1982; Kuefler and Haddad, 2006; Dumont et al., 2007; Dias et al., 2009; Avgar et al., 2013; Dodge et al., 2014; Murphy and Boone, 2022), we observed strong effects of resource availability on the path scale on butterfly diffusion rates. Here we discuss mainly silvery blue diffusion rate responses to resources, since we were unable to include the results of the ochre ringlet PLSR model due to poor model fit.
Host plant availability had strong effects on silvery blue diffusion rates. As in previous studies on lycaenid movement (e.g. Schultz, 1998; Schultz and Crone, 2001; Schultz et al., 2012; Warchola et al., 2017), silvery blue female diffusion rates were strongly associated with their host plants. Greater availability of their preferred host plants (large perennial Lupinus spp.) on a flight path resulted in slower silvery blue diffusion rates, indicating that when host plants are present, individuals perceive habitat as higher value (i.e. resource-rich). Despite being hosted by a variety of Fabaceae species throughout the Pacific Northwest (Pyle and LaBar, 2018, pg. 235), silvery blue females only oviposited on large Lupinus spp. in our observations. This clear preference for Lupinus spp. was reflected in the PLSR model, as V. sativa host measurements had a low VIP value, while Lupinus spp. host measurements had a high VIP value and were associated with lower diffusion rates. Both native upland prairie sites (lowest diffusion rates and step lengths) and one conservation grazing site (Mary Mallonee’s farm) had large perennial Lupinus spp. present, while both conventional grazing sites (highest diffusion rates and step lengths) and the other conservation grazing site (Colvin Ranch) did not. We observed oviposition and plant walking behavior by silvery blue females on both the native prairies and on Mary Mallonee’s farm, but not on the other sites. This suggests that management for butterflies under cattle grazing should focus on improving plant community diversity to provide host plants for a variety of species, as well as adding specific host plants for focal species.
Lupinus spp. plants are often considered to be undesirable by farmers and ranchers as they are toxic to cattle and may cause pregnant cows to abort their fetuses (Panter et al., 2002). However, cattle generally will not eat Lupinus spp. unless their other forage has been depleted (Lopez-Ortiz et al., 2007), and we observed cattle to remove individual grass leaves from under Lupinus plants without touching the plant itself (Bussan, pers. observation).
Adult butterflies are usually nectar generalists (Graves and Shapiro, 2003), though they may still exhibit preferences for some plant species over others (Thomas and Schultz, 2016). We observed silvery blues to frequently use the native plant C. quamash in our nectar observations, although this was not reflected in the diffusion rates in our PLSR model. Nonnative plants can provide valuable resources for butterflies in degraded landscapes (Graves and Shapiro, 2003; Hardy and Dennis, 2008). V. sativa, a nonnative plant, was associated with lower diffusion rates for silvery blue butterflies and therefore higher perceived habitat quality. V. sativa was often one of the few nectar species available on grazed sites. We observed ochre ringlets to nectar mainly on D. carota and L. vulgare. D. carota was one of the few late season nectar plants available on our sites, demonstrating the importance of nectar through the full butterfly flight season. There are relatively few late season nectar species available in the landscape in western Washington (Bowcutt and Hamman, 2016). Nectar is important for other ringlet species as well: Sei (2009) observed that maritime ringlet (Coenonympha nipisquit) females nectared more often in areas with a high larval survival rate, though it was unclear if the nectar was a causal factor in the larval survival rate. Silvery blues and ochre ringlets nectared on mostly different species, even in parts of their flight period that overlapped, highlighting both the importance of understanding the biological needs of individual species, and of providing a variety of resources through heterogeneous management in the landscape (Dennis, 2004; Dennis et al., 2006; Jerrentrup et al., 2014; Joubert-van der Merwe et al., 2019).
Grazed pastures may have potential to act as corridors between native prairie reserves. Butterflies are known to reach higher densities in habitat patches connected by corridors than patches that are unconnected (Haddad and Baum, 1999; Haddad and Tewksbury, 2005). Butterflies move faster in matrix habitat (Haddad and Tewksbury, 2005; Brown et al., 2017; Schultz et al., 2017; Crone et al., 2019) so providing a series of “stepping stones” with important resources between reserves could increase connectivity in fragmented landscapes (Schultz, 1998). We speculate that conservation grazing pastures, as potential providers of intermediate habitat quality, may act as both habitat and valuable stepping stones for migration between high quality native prairies in the highly fragmented landscape of western Washington.
Diffusion rates are an integrated method used in the current literature for comparing incidence of area restricted search across habitats or management types (Pugesek and Crone, 2022); lower diffusion rates indicate a higher incidence of area restricted search behavior, which in turn indicates perception of high habitat quality (Crone et al., 2019). The analysis of movement behavior can provide more reliable estimates of habitat quality than occupancy or abundance data (Winker et al., 1995). Movement parameters such as step lengths, turning angles, and diffusion rates are often used to discriminate between “habitat” and “non-habitat” (i.e. matrix habitat, with border habitat as a third option) (e.g. Schultz, 1998; Ross et al., 2005; Murphy and Boone, 2022). We applied diffusion rates as a more sensitive indicator of habitat quality on a fine scale and show that conservation grazing may be an important source of intermediate quality habitat in the landscape. However, it is important to note that our study measured only butterfly behavioral response to potential habitat quality, and makes the assumption that differences in movement parameters indicate differences in quality (Crone et al., 2019). In addition, our analysis assessed mainly external effects on butterfly movement, and did not take into account internal factors (see Nathan et al., 2008 for further discussion), such as butterfly age or body mass. We did classify wing wear as a proxy for age, but were unable to include it in the analysis due to limited sample size and the fact that it is confounded by changes in the plant community as the season progresses. Future studies should measure vital rates of butterflies under different grazing management strategies to directly measure habitat quality (Schultz et al., 2019). Demographic studies would indicate whether conservation grazing has the potential to become an ecological trap (Schlaepfer et al., 2002) by attracting females to lay eggs, only to face reduced larval survival due to trampling or other effects of cattle grazing.
Implications for conservation of native butterflies
We conclude that there is potential for grazed land to contribute to butterfly habitat in the landscape, though prairie refugia should be maintained for sensitive species. Grazed habitats may be able to contribute to butterfly habitat if important resources, especially host and nectar plants, are available. Therefore, to support a wide range of butterfly species, grazing practices that support the greatest diversity of host plants should be encouraged, such as rotational grazing with a spring rest period (Ravetto Enri et al., 2017). While the IUCN recommends that 30% of the world’s ecosystems be conserved by 2030 (IUCN, 2022), protected conservation areas alone are not enough to achieve this goal (Watson et al., 2014; Butchart et al., 2015). Incorporating conservation grazing into the agricultural landscape will be an important tool to conserve native species in light of accelerating habitat and biodiversity loss.
Data availability statement
Data will be made available in a data repository. The raw data supporting the conclusions of this article will be made available by the authors, without undue reservation. Code will be published on GitHub.
Ethics statement
This study was reviewed and permitted for scientific research by the Washington Department of Fish and Wildlife. No further ethical review was required for common invertebrates.
Author contributions
CS and SB developed the initial idea; CS obtained and provided grants and other resources; SB collected and analyzed data; SB wrote the manuscript; CS and SB edited the manuscript. Both authors contributed to the article and approved the submitted version.
Funding
This work was generously supported by Conservation Research Education and Outreach International (CREOi), Western Sustainable Agriculture Research and Education (WSARE; grant number 201207548), WSU CEREO, and Washington State University Vancouver.
Acknowledgments
We thank Sarah Hamman, Leslie New, Jonah Piovia-Scott, and the two reviewers, Jason Harmon and Adam Kőrösi, for helpful comments on previous versions of this manuscript. We also thank our collaborators and partnered organizations who facilitated our work: Stephen Bramwell, Sarah Hamman, Marty Chaney, Thurston County WSU Extension, Ecostudies Institute, and NRCS. We are deeply grateful to the farmers and ranchers who allowed us to conduct butterfly experiments on their land: Fred Colvin, Kevin Jensen, and Mary and Maynard Mallonee. We also thank the Washington Department of Fish and Wildlife and the Department of Defense (Joint Base Lewis-McChord) for access to the native prairies. Collin Edwards, Kelsey King, and Erica Henry provided invaluable statistics advice and coding assistance. We thank Christopher Jason for his instrumental help in the field. Rachael Bonoan, Kelsey King, Cassandra Doll, Chelsea Thomas, and Center for Natural Lands Management and Evergreen State College volunteers also provided field assistance. A previous version of this manuscript appeared in SB’s dissertation.
Conflict of interest
The authors declare that the research was conducted in the absence of any commercial or financial relationships that could be construed as a potential conflict of interest.
Publisher’s note
All claims expressed in this article are solely those of the authors and do not necessarily represent those of their affiliated organizations, or those of the publisher, the editors and the reviewers. Any product that may be evaluated in this article, or claim that may be made by its manufacturer, is not guaranteed or endorsed by the publisher.
Supplementary material
The Supplementary Material for this article can be found online at: https://www.frontiersin.org/articles/10.3389/fevo.2023.1162060/full#supplementary-material
References
Avgar T., Mosser A., Brown G. S., Fryxell J. M. (2013). Environmental and individual drivers of animal movement patterns across a wide geographical gradient. J. Anim. Ecol. 82, 96–106. doi: 10.1111/j.1365-2656.2012.02035.x
Balmer O., Erhardt A. (2000). Consequences of succession on extensively grazed grasslands for central European butterfly communities: rethinking conservation practices. Conserv. Biol. 14, 746–757. doi: 10.1046/j.1523-1739.2000.98612.x
Bates D., Maechler M., Bolker B., Walker S. (2015). Fitting linear mixed-Effects models using lme4. J. Stat. Software 67 (1), 1–48. doi: 10.18637/jss.v067.i01
Beck J. J., Hernández D. L., Pasari J. R., Zavaleta E. S. (2015). Grazing maintains native plant diversity and promotes community stability in an annual grassland. Ecol. Appl. 25, 1259–1270. doi: 10.1890/14-1093.1
Bigelow D. P., Borchers A. (2017). Major Uses of Land in the United States 2012. no. 178 (St. Paul, MN.: USDA Economic Research Service).
Blanchet K., Moechnig H., DeJong-Hughes J. (2000). Grazing Systems Planning Guide (University of Minnesota Extension Service).
Bock C. E., Jones Z. F. (2004). Avian habitat evaluation: should counting birds count? Front. Ecol. Environ. 2, 403–410. doi: 10.1890/1540-9295(2004)002[0403:AHESCB]2.0.CO;2
Bolker B. M., Brooks M. E., Clark C. J., Geange S. W., Poulsen J. R., Stevens M. H. H., et al. (2009). Generalized linear mixed models: a practical guide for ecology and evolution. Trends Ecol. Evol. 24, 127–135. doi: 10.1016/j.tree.2008.10.008
Bowcutt F., Hamman S. (2016). Vascular Plants of the South Sound Prairies. 1st edition (Olympia, Washington: The Evergreen State College Press).
Brook A., Zint M., De Young R. (2003). Landowners’ responses to an endangered species act listing and implications for encouraging conservation. Conserv. Biol. 17, 1638–1649. doi: 10.1111/j.1523-1739.2003.00258.x
Brown L. M., Fuda R. K., Schtickzelle N., Coffman H., Jost A., Kazberouk A., et al. (2017). Using animal movement behavior to categorize land cover and predict consequences for connectivity and patch residence times. Landscape Ecol. 32, 1657–1670. doi: 10.1007/s10980-017-0533-8
Bussan S. K. (2022). Can cattle grazing benefit grassland butterflies? J. Insect Conserv. 26, 359–374. doi: 10.1007/s10841-022-00373-8
Butchart S. H. M., Clarke M., Smith R. J., Sykes R. E., Scharlemann J. P. W., Harfoot M., et al. (2015). Shortfalls and solutions for meeting national and global conservation area targets. Conserv. Lett. 8, 329–337. doi: 10.1111/conl.12158
Carrascal L. M., Galván I., Gordo O. (2009). Partial least squares regression as an alternative to current regression methods used in ecology. Oikos 118, 681–690. doi: 10.1111/j.1600-0706.2008.16881.x
Chong I.-G., Jun C.-H. (2005). Performance of some variable selection methods when multicollinearity is present. Chemometrics Intelligent Lab. Syst. 78, 103–112. doi: 10.1016/j.chemolab.2004.12.011
Cortés Capano G., Toivonen T., Soutullo A., Di Minin E. (2019). The emergence of private land conservation in scientific literature: A review. Biol. Conserv. 237, 191–199. doi: 10.1016/j.biocon.2019.07.010
Crone E. E., Brown L. M., Hodgson J. A., Lutscher F., Schultz C. B. (2019). Faster movement in nonhabitat matrix promotes range shifts in heterogeneous landscapes. Ecology 100, e02701. doi: 10.1002/ecy.2701
Crone E. E., Schultz C. B. (2008). Old models explain new observations of butterfly movement at patch edges. Ecology 89, 2061–2067. doi: 10.1890/07-1173.1
Davis K. P., Augustine D. J., Monroe A. P., Derner J. D., Aldridge C. L. (2020). Adaptive rangeland management benefits grassland birds utilizing opposing vegetation structure in the shortgrass steppe. Ecol. Appl. 30, e02020. doi: 10.1002/eap.2020
Debinski D. M., Moranz R. A., Delaney J. T., Miller J. R., Engle D. M., Winkler L. B., et al. (2011). A cross-taxonomic comparison of insect responses to grassland management and land-use legacies. Ecosphere 2, art131. doi: 10.1890/ES11-00226.1
de Knegt H. J., Hengeveld G. M., van Langevelde F., de Boer W. F., Kirkman K. P. (2007). Patch density determines movement patterns and foraging efficiency of large herbivores. Behav. Ecol. 18, 1065–1072. doi: 10.1093/beheco/arm080
Delaney J. T., Moranz R. A., Debinski D. M., Engle D. M., Miller J. R. (2016). Exotic-dominated grasslands show signs of recovery with cattle grazing and fire. PloS One 11, e0165758. doi: 10.1371/journal.pone.0165758
Dennis R. L. H. (2004). Just how important are structural elements as habitat components? Indications from a declining lycaenid butterfly with priority conservation status. J. Insect Conserv. 8, 37–45. doi: 10.1023/B:JICO.0000027496.82631.4b
Dennis R. L. H., Shreeve T. G., Van Dyck H. (2006). Habitats and resources: the need for a resource-based definition to conserve butterflies. Biodiversity Conserv. 15, 1943–1966. doi: 10.1007/s10531-005-4314-3
Dias M. P., Granadeiro J. P., Palmeirim J. M. (2009). Searching behaviour of foraging waders: does feeding success influence their walking? Anim. Behav. 77, 1203–1209. doi: 10.1016/j.anbehav.2009.02.002
Dodge K. L., Galuardi B., Miller T. J., Lutcavage M. E. (2014). Leatherback turtle movements, dive behavior, and habitat characteristics in ecoregions of the Northwest Atlantic Ocean. PloS One 9, e91726. doi: 10.1371/journal.pone.0091726
Dorfman A., Hills T. T., Scharf I. (2022). A guide to area-restricted search: a foundational foraging behaviour. Biol. Rev. Camb. Philos. Soc 97, 2076–2089. doi: 10.1111/brv.12883
Dumont C. P., Himmelman J. H., Robinson S. M. C. (2007). Random movement pattern of the sea urchin Strongylocentrotus droebachiensis. J. Exp. Mar. Biol. Ecol. 340, 80–89. doi: 10.1016/j.jembe.2006.08.013
Ehl S., Böhm N., Wörner M., Rákosy L., Schmitt T. (2019). Dispersal and adaptation strategies of the high mountain butterfly Boloria pales in the Romanian Carpathians. Front. zoology 16, 1. doi: 10.1186/s12983-018-0298-1
Farruggia A., Dumont B., Scohier A., Leroy T., Pradel P., Garel J. P. (2012). An alternative rotational stocking management designed to favour butterflies in permanent grasslands. Grass Forage Sci. 67, 136–149. doi: 10.1111/j.1365-2494.2011.00829.x
Fischer K., Beinlich B., Plachter H. (1999). Population structure, mobility and habitat preferences of the violet copper Lycaena helle (Lepidoptera: Lycaenidae) in Western Germany: implications for conservation. J. Insect Conserv. 3, 43–52. doi: 10.1023/A:1009630506216
Fischer K., Fiedler K. (2001). Resource-based territoriality in the butterfly Lycaena hippothoe and environmentally induced behavioural shifts. Anim. Behav. 61, 723–732. doi: 10.1006/anbe.2000.1662
Fleischner T. L. (1994). Ecological costs of livestock grazing in western North America. Conserv. Biol. 8, 629–644. doi: 10.1046/j.1523-1739.1994.08030629.x
Fownes S., Roland J. (2002). Effects of meadow suitability on female behaviour in the alpine butterfly Parnassius smintheus. Ecol. Entomology 27, 457–466. doi: 10.1046/j.1365-2311.2002.00426.x
Fransen S., Pirelli G., Chaney M., Brewer L., Robbins S. (2017). The Western Oregon and Washington Pasture Calendar (Oregon State University, University of Idaho, Washington State University).
Graves S. D., Shapiro A. M. (2003). Exotics as host plants of the California butterfly fauna. Biol. Conserv. 110, 413–433. doi: 10.1016/S0006-3207(02)00233-1
Haddad N. M. (1999). Corridor use predicted from behaviors at habitat boundaries. Am. Nat. 153, 215–227. doi: 10.1086/303163
Haddad N., Baum K. A. (1999). An experimental test of corridor effects on butterfly densities. Ecol. Appl. 9, 623–633. doi: 10.1890/1051-0761(1999)009[0623:AETOCE]2.0.CO;2
Haddad N. M., Tewksbury J. J. (2005). Low-quality habitat corridors as movement conduits for two butterfly for two butterfly species. Ecol. Appl. 15, 250–257. doi: 10.1890/03-5327
Hamman S. T., Dunwiddie P. W., Nuckols J. L., McKinley M. (2011). Fire as a restoration tool in pacific northwest prairies and oak woodlands: challenges, successes, and future directions. Northwest Sci. 85, 317–328. doi: 10.3955/046.085.0218
Hardy P. B., Dennis R. L. H. (2008). Resources for British butterflies (Lepidoptera: Hesperioidea, Papilionoidea). The alien consumer component and its significance for butterfly habitats. Eur. J. entomology 105, 649–657. doi: 10.14411/eje.2008.089
Hartig F. (2022). “DHARMa: residual diagnostics for hierarchical (Multi-level/mixed) regression models,” in R package version 0.4.6. Available at: https://CRAN.R-project.org/package=DHARMa.
Henry E., Brammer-Robbins E., Aschehoug E., Haddad N. (2019). Do substitute species help or hinder endangered species management? Biol. Conserv. 232, 127–130.
IUCN (2022). “IUCN position paper on the post-2020 global biodiversity framework OEWG-5 and CBD COP15,” in Fifteenth meeting of the conference of the parties (COP15). Eds. Moreno S.Peña, Romero V. (Montreal, Canada: International Union for the Conservation of Nature).
James D., Nunnallee D. (2011). Life Histories of Cascadia Butterflies (Corvallis, OR: Oregon State University Press).
Jerrentrup J. S., Wrage-Mönnig N., Röver K.-U., Isselstein J. (2014). Grazing intensity affects insect diversity via sward structure and heterogeneity in a long-term experiment. J. Appl. Ecol. 51, 968–977. doi: 10.1111/1365-2664.12244
Joubert-van der Merwe L., Pryke J. S., Samways M. J. (2019). Well-managed grassland heterogeneity promotes butterfly conservation in a corridor network. J. Environ. Manage. 238, 382–395. doi: 10.1016/j.jenvman.2019.03.021
Kahara S. N., Skalos D., Madurapperuma B., Hernandez K. (2022). Habitat quality and drought effects on breeding mallard and other waterfowl populations in California, USA. J. Wildl. Manage. 86, e22133. doi: 10.1002/jwmg.22133
Kamal S., Grodzińska-Jurczak M., Brown G. (2015). Conservation on private land: a review of global strategies with a proposed classification system. J. Environ. Plann. Manage. 58, 576–597. doi: 10.1080/09640568.2013.875463
Kareiva P., Odell G. (1987). Swarms of predators exhibit “Preytaxis” if individual predators use area-restricted search. Am. Nat. 130, 233–270. doi: 10.1086/284707
Kareiva P. M., Shigesada N. (1983). Analyzing insect movement as a correlated random walk. Oecologia 56, 234–238. doi: 10.1007/BF00379695
Kerr J. T., Sugar A., Packer L. (2000). Indicator taxa, rapid biodiversity assessment, and nestedness in an endangered ecosystem. Conserv. Biol. 14, 1726–1734. doi: 10.1111/j.1523-1739.2000.99275.x
Konvička M., Ričl D., Vodičková V., Beneš J., Jirků M. (2021). Restoring a butterfly hot spot by large ungulates refaunation: the case of the Milovice military training range, Czech Republic. BMC Ecol. Evol. 21, 73. doi: 10.1186/s12862-021-01804-x
Korösi A., Orvössy N., Batáry P., Kövér S., Peregovits L. (2008). Restricted within-habitat movement and time-constrained egg laying of female Maculinea rebeli butterflies. Oecologia 156, 455–464. doi: 10.1007/s00442-008-0986-1
Kruess A., Tscharntke T. (2002). Grazing intensity and the diversity of grasshoppers, butterflies, and trap-nesting bees and wasps. Conserv. Biol. 16, 1570–1580. doi: 10.1046/j.1523-1739.2002.01334.x
Kuefler D., Avgar T., Fryxell J. M. (2012). Rotifer population spread in relation to food, density and predation risk in an experimental system. J. Anim. Ecol. 81, 323–329. doi: 10.1111/j.1365-2656.2011.01917.x
Kuefler D., Haddad N. M. (2006). Local versus landscape determinants of butterfly movement behaviors. Ecography 29, 549–560. doi: 10.1111/j.0906-7590.2006.04574.x
Kuefler D., Hudgens B., Haddad N. M., Morris W. F., Thurgate N. (2010). The conflicting role of matrix habitats as conduits and barriers for dispersal. Ecology 91, 944–950. doi: 10.1890/09-0614.1
Lebeau J., Wesselingh R. A., Van Dyck H. (2015). Butterfly density and behaviour in uncut hay meadow strips: behavioural ecological consequences of an agri-environmental scheme. PloS One 10, e0134945. doi: 10.1371/journal.pone.0134945
Lenth R. (2022). “emmeans: Estimated Marginal Means, aka Least-Squares Means,” in R package version 1.8.1-1. Available at: https://CRAN.R-project.org/package=emmeans.
Leopold E., Boyd R. (1999). “An ecological history of old prairie areas in southwestern washington,” in Indians, Fire, and the Land in the Pacific Northwest (Corvallis, OR: Oregon State University Press), 139–163.
Liland K., Mevik B., Wehrens R. (2022). “pls: partial least squares and principal component regression_,” in R package version 2.8-1. Available at: https://CRAN.R-project.org/package=pls.
Lopez-Ortiz S., Pfister J. A., Launchbaugh K. L., Gay C. C. (2007). Forage availability and body condition affect intake of lupine (Lupinus leucophyllus) by grazing cattle. Prof. Anim. Scientist 23, 459–466. doi: 10.1532/S1080-7446(15)31006-8
Manley W. A., Hart R. H., Samuel M. J., Smith M. A., Waggoner J. W., Manley J. T. (1997). Vegetation, cattle, and economic responses to grazing strategies and pressures. J. Range Management 50, 638.
Maxwell S. L., Cazalis V., Dudley N., Hoffmann M., Rodrigues A. S. L., Stolton S., et al. (2020). Area-based conservation in the twenty-first century. Nature 586, 217–227. doi: 10.1038/s41586-020-2773-z
Mehmood T., Liland K. H., Snipen L., Sæbø S. (2012). A review of variable selection methods in Partial Least Squares Regression. Chemometrics Intelligent Lab. Syst. 118, 62–69. doi: 10.1016/j.chemolab.2012.07.010
Mevik B.-H., Wehrens R. (2007). The pls package: principal component and partial least squares regression in R. J. Stat. Software 18. doi: 10.18637/jss.v018.i02
Michelot T., Langrock R., Patterson T. A. (2016). moveHMM: an R package for the statistical modelling of animal movement data using hidden Markov models. Methods Ecol. Evol. 7 (11), 1308–1315. doi: 10.1111/2041-210X.12578
Muff S., Nilsen E. B., O’Hara R. B., Nater C. R. (2022). Rewriting results sections in the language of evidence. Trends Ecol. Evol. 37, 203–210. doi: 10.1016/j.tree.2021.10.009
Murphy M., Boone M. (2022). Evaluating the role of body size and habitat type in movement behavior in human-dominated systems: A frog’s eye view. Ecol. Evol. 12, e9022. doi: 10.1002/ece3.9022
Murphy D. D., Weiland P. S., Cummins K. W. (2011). A critical assessment of the use of surrogate species in conservation planning in the Sacramento-San Joaquin Delta, California (U.S.A.). Conserv. Biol. 25, 873–878.
Nathan R., Getz W. M., Revilla E., Holyoak M., Kadmon R., Saltz D., et al. (2008). A movement ecology paradigm for unifying organismal movement research. Proc. Natl. Acad. Sci. United States America 105, 19052–19059. doi: 10.1073/pnas.0800375105
Neilly H., O’Reagain P., Vanderwal J., Schwarzkopf L. (2018). Profitable and sustainable cattle grazing strategies support reptiles in tropical savanna rangeland. Rangeland Ecol. Manage. 71, 205–212. doi: 10.1016/j.rama.2017.09.005
Nilsson S. G., Franzén M., Jönsson E. (2008). Long-term land-use changes and extinction of specialised butterflies. Insect Conserv. Diversity. 1, 197–207. doi: 10.1111/j.1752-4598.2008.00027.x
Noland S., Carver L. (2011). Prairie Landowner Guide for Western Washington (The Nature Conservancy), 2.
Noss R. F. (1994). Cows and conservation biology. Conserv. Biol. 8, 613–616. doi: 10.1046/j.1523-1739.1994.08030613.x
Ovaskainen O. (2004). Habitat-specific movement parameters estimated using mark–recapture data and a diffusion model. Ecology 85, 242–257. doi: 10.1890/02-0706
Panter K. E., James L. F., Gardner D. R., Society for Range Management (2002). Reproductive losses to poisonous plants: Influence of management strategies. Rev. Endocrinol. Metab. 55, 301–308. doi: 10.2458/azu_jrm_v55i3_panter
Pelham J. P. (2021). A catalogue of the butterflies of the United States and Canada. J. Res. Lepidoptera. Entomological news.
Pöyry J., Lindgren S., Salminen J., Kuussaari M. (2004). Restoration of butterfly and moth communities in semi-natural grasslands by cattle grazing. Ecol. Appl. 14, 1656–1670. doi: 10.1890/03-5151
Pugesek G., Crone E. E. (2022). Movement of nest-searching bumblebee queens reflects nesting habitat quality. Ecol. Entomology 47, 719–727. doi: 10.1111/een.13156
Pyle R. M., LaBar C. C. (2018). Butterflies of the Pacific Northwest (Portland, OR: Timber Press, inc.).
R Core Team. (2022). R: A language and environment for statistical computing. R Foundation for Statistical Computing, Vienna, Austria. Available at: https://www.R-project.org/. Version 4.4.2 (2022-10-31).
Ravetto Enri S., Probo M., Farruggia A., Lanore L., Blanchetete A., Dumont B. (2017). A biodiversity-friendly rotational grazing system enhancing flower-visiting insect assemblages while maintaining animal and grassland productivity. Agriculture Ecosyst. Environ. 241, 1–10. doi: 10.1016/j.agee.2017.02.030
Revilla E., Wiegand T., Palomares F., Ferreras P., Delibes M. (2004). Effects of matrix heterogeneity on animal dispersal: from individual behavior to metapopulation-level parameters. Am. Nat. 164, E130–E153. doi: 10.1086/424767
Robel R. J., Briggs J. N., Dayton A. D., Hulbert L. C. (1970). Relationships between visual obstruction measurements and weight of grassland vegetation. J. Range Manage. 23, 295. doi: 10.2307/3896225
Root R. B., Kareiva P. M. (1984). The search for resources by cabbage butterflies (Pieris rapae): ecological consequences and adaptive significance of markovian movements in a patchy environment. Ecology 65, 147–165. doi: 10.2307/1939467
Ross J. A., Matter S. F., Roland J. (2005). Edge avoidance and movement of the butterfly Parnassius smintheus in matrix and non-matrix habitat. Landscape Ecol. 20, 127–135. doi: 10.1007/s10980-004-1010-8
Rusterholz H.-P., Erhardt A. (2000). Can nectar properties explain sex-specific flower preferences in the Adonis Blue butterfly Lysandra bellargus? Ecol. Entomology 25, 81–90. doi: 10.1046/j.1365-2311.2000.00233.x
Samson F. B., Knopf F. L., Ostlie W. R. (2004). Great Plains ecosystems: past, present, and future. Wildlife Soc. Bull. 32, 6–15. doi: 10.2193/0091-7648(2004)32[6:GPEPPA]2.0.CO;2
Schlaepfer M. A., Runge M. C., Sherman P. W. (2002). Ecological and evolutionary traps. Trends Ecol. Evol. 17, 474–480. doi: 10.1016/S0169-5347(02)02580-6
Schtickzelle N., Turlure C., Baguette M. (2007). Grazing management impacts on the viability of the threatened bog fritillary butterfly Proclossiana eunomia. Biol. Conserv. 136, 651–660. doi: 10.1016/j.biocon.2007.01.012
Schultz C. B. (1998). Dispersal behavior and its implications for reserve design in a rare Oregon butterfly. Conserv. Biol. 12, 284–292. doi: 10.1111/j.1523-1739.1998.96266.x
Schultz C. B., Crone E. E. (2001). Edge-mediated dispersal behavior in a prairie butterfly. Ecology 82, 1879–1892. doi: 10.1890/0012-9658(2001)082[1879:EMDBIA]2.0.CO;2
Schultz C. B., Franco A. M. A., Crone E. E. (2012). Response of butterflies to structural and resource boundaries. J. Anim. Ecol. 81, 724–734. doi: 10.1111/j.1365-2656.2011.01947.x
Schultz C. B., Haddad N. M., Henry E. H., Crone E. E. (2019). Movement and demography of at-risk butterflies: building blocks for conservation. Annu. Rev. Entomology 64, 167–184. doi: 10.1146/annurev-ento-011118-112204
Schultz C. B., Pe’er B. G., Damiani C., Brown L., Crone E. E. (2017). Does movement behaviour predict population densities? A test with 25 butterfly species. J. Anim. Ecol. 86, 384–393. doi: 10.1111/1365-2656.12609
Scott E. R., Crone E. E. (2021). Using the right tool for the job: the difference between unsupervised and supervised analyses of multivariate ecological data. Oecologia 196, 13–25. doi: 10.1007/s00442-020-04848-w
Searle S. R., Speed F. M., Milliken G. A. (1980). Population marginal means in the linear model: An alternative to least squares means. Am. Statistician 34, 216–221. doi: 10.2307/2684063
Sei M. (2009). Flight and oviposition behavior of the adult maritime ringlet (Coenonympha nipisiquit mcDunnough) females in response to microhabitat. J. Insect Behav. 22, 87–100. doi: 10.1007/s10905-008-9156-x
Smith L. M., Cherry R. (2014). Effects of management techniques on grassland butterfly species composition and community structure. Am. midland Nat. 172, 227–235. doi: 10.1674/0003-0031-172.2.227
Stanton M. L. (1982). Searching in a patchy environment: foodplant selection by colis P. eriphyle butterflies. Ecology 63, 839–853. doi: 10.2307/1936803
Stevens V. M., Polus E., Wesselingh R. A., Schtickzelle N., Baguette M. (2004). Quantifying functional connectivity: experimental evidence for patch-specific resistance in the Natterjack toad (Bufo calamita). Landscape Ecol. 19, 829–842. doi: 10.1007/s10980-004-0166-6
Thomas R. C., Schultz C. B. (2016). Resource selection in an endangered butterfly: Females select native nectar species. J. Wildlife Manage. 80, 171–180. doi: 10.1002/jwmg.987
Thomas J. A., Simcox D. J., Clarke R. T. (2009). Successful conservation of a threatened Maculinea butterfly. Science 325, 80–83. doi: 10.1126/science.1175726
Turchin P. (2015). Quantitative analysis of movement: measuring and modeling population resdistribution in animals and plants (Beresta Books).
Van Horne B. (1983). Density as a misleading indicator of habitat quality. J. Wildlife Manage. 47, 893–901. doi: 10.2307/3808148
van Klink R., van der Plas F., van Noordwijk C. G. E. T., WallisDeVries M. F., Olff H. (2015). Effects of large herbivores on grassland arthropod diversity. Biol. Rev. Cambridge Philos. Soc. 90, 347–366. doi: 10.1111/brv.12113
Velasco J. (2021). Unsettling prairies: A critical reimagining of fire management in cities (Seattle, WA: University of Washington). Doctoral dissertation.
Vogel J. A., Debinski D. M., Koford R. R., Miller J. R. (2007). Butterfly responses to prairie restoration through fire and grazing. Biol. Conserv. 140, 78–90. doi: 10.1016/j.biocon.2007.07.027
WallisDeVries M. F., Noordijk J., Colijn E. O., Smit J. T., Veling K. (2016). Contrasting responses of insect communities to grazing intensity in lowland heathlands. Agriculture Ecosyst. Environ. 234, 72–80. doi: 10.1016/j.agee.2016.04.012
Warchola N., Crone E. E., Schultz C. B. (2017). Balancing ecological costs and benefits of fire for population viability of disturbance-dependent butterflies. J. Appl. Ecol. 55, 800–809. doi: 10.1111/1365-2664.12983
Washington Department of Fish and Wildlife. (2022). Westside prairie (Olympia, WA: Washington Department of Fish & Wildlife). Available at: https://wdfw.wa.gov/species-habitats/ecosystems/westside-prairie.
Watson J. E. M., Dudley N., Segan D. B., Hockings M. (2014). The performance and potential of protected areas. Nature 515, 67–73. doi: 10.1038/nature13947
Weiss S. B. (1999). Cars, cows, and checkerspot butterflies: nitrogen deposition and management of nutrient-poor grasslands for a threatened species. Conserv. Biol. 13, 1476–1486. doi: 10.1046/j.1523-1739.1999.98468.x
Western Regional Climate Center. (2022) WRCC: washington climate. Available at: https://wrcc.dri.edu/Climate/narrative_wa.php.
Wickham H., Averick M., Bryan J., Chang W., McGowan L. D., François R., et al. (2019). Welcome to the tidyverse. J. Open Source Software 4 (43), 1686. doi: 10.21105/joss.01686
Winker K., Rappole J. H., Ramos M. A. (1995). The use of movement data as an assay of habitat quality. Oecologia 101, 211–216.
Wold H. (1975). Soft modelling by latent variables: the non-linear iterative partial least squares (NIPALS) approach. J. Appl. probability 12, 117–142. doi: 10.1017/S0021900200047604
Zakkak S., Chatzaki M., Karamalis N., Kati V. (2014). Spiders in the context of agricultural land abandonment in Greek Mountains: species responses, community structure and the need to preserve traditional agricultural landscapes. J. Insect Conserv. 18, 599–611. doi: 10.1007/s10841-014-9663-3
Zalucki M. P., Kitching R. L. (1982). The analysis and description of movement in adult Danaus plexippus L. (lepidoptera: danainae). Behaviour 80, 174–197. doi: 10.1163/156853982X00346
Zhang J., Cong Q., Shen J., Opler P. A., Grishin N. V. (2020). Genomic evidence suggests further changes of butterfly names. Taxonomic Rep. Int. Lepidoptera Survey 8 (7), 1–41.
Keywords: butterflies, cattle grazing, conservation, movement, diffusion rates, agriculture, prairie
Citation: Bussan SK and Schultz CB (2023) Can cattle grazing contribute to butterfly habitat? Using butterfly behavior as an index of habitat quality in an agroecosystem. Front. Ecol. Evol. 11:1162060. doi: 10.3389/fevo.2023.1162060
Received: 09 February 2023; Accepted: 23 November 2023;
Published: 12 December 2023.
Edited by:
Piotr Nowicki, Jagiellonian University, PolandReviewed by:
Jason Harmon, North Dakota State University, United StatesAdam Kőrösi, Büro Geyer und Dolek, Germany
Copyright © 2023 Bussan and Schultz. This is an open-access article distributed under the terms of the Creative Commons Attribution License (CC BY). The use, distribution or reproduction in other forums is permitted, provided the original author(s) and the copyright owner(s) are credited and that the original publication in this journal is cited, in accordance with accepted academic practice. No use, distribution or reproduction is permitted which does not comply with these terms.
*Correspondence: Samantha K. Bussan, samantha.bussan@wsu.edu