- 1School of Life Sciences, Peking University, Beijing, China
- 2College of the Environment and Ecology, Xiamen University, Xiamen, China
Mangrove forests, which occur in the intertidal regions of tropical and sub-tropical zones, have high ecological and economic values. They have rapidly reduced over the past decades due to various reasons. Reforestation is a common strategy for the conservation of mangroves, but information on the renewal capacity and viability of these artificial mangrove populations is still lacking. Here, we estimated the effective population size (NE) of an artificial population of Kandelia obovata (Rhizophoraceae) and explored the effects of intrinsic and extrinsic factors on changes in NE to evaluate population viability and develop appropriate management strategies for mangrove forests. This population consisted of three ecologically and genetically differentiated groups that had experienced a major low-temperature event during the winter of 2015–2016 and varied in cold resistance. We first detected population bottlenecks and estimated contemporary values of NE for different groups using microsatellite data. Next, we performed paternity analyses for seedlings and propagules to explore variations in the reproductive success of individuals within the three groups before and after the low-temperature event. Lastly, we simulated four scenarios to characterize the effects of low temperature, mating system, and variance in reproductive success on changes in NE in a hypothetical metapopulation based on empirical estimates. Our results show that groups with moderate or poor cold resistance experienced local bottlenecks, and shifts in effective breeders occurred following the low-temperature event, which indicates that low temperature has an effect on not only population size but also reproductive success. Furthermore, our simulations revealed that changes in NE are jointly affected by reproductive success, mating system, and environmental conditions. These findings enhance our understanding of the multiple factors that affect NE, and provide key information that will aid the reforestation and management of mangrove forests, especially when they are introduced to high-latitude areas.
1. Introduction
Mangrove forests, which occur in the intertidal regions of tropical and sub-tropical zones, have high ecological and economic values because they provide refuges and breeding grounds for diverse organisms, attenuate waves, absorb pollutants, and sequester carbon (Alongi, 2012; Tomlinson, 2016). However, climate change, human activities, and stochastic events have continued to threaten the health of mangrove ecosystems over the past decades, leading to a steady global loss rate (1–2% per year) in mangrove forests (Field, 1995; Carugati et al., 2018; Bologna et al., 2019; Eddy et al., 2021; Lu et al., 2022). Reforestation is a common strategy for the conservation of mangroves in many countries. In China, because mangrove forests have experienced high rates of deforestation, reforestation has been widely conducted in recent years (Yang et al., 2017; Wang et al., 2020); an important strategy is to introduce mangrove plants, including their propagules and/or seedlings from multiple provenances at different latitudes to a nursery garden at a higher latitude (Zheng et al., 2010; Wang et al., 2020). Since the 1950s, a total of 1,700 ha of mangroves have been planted in Zhejiang Province; however, only 380 ha of mangroves currently remain, and merely 30.3 ha of mangrove forests possess a capacity for population renewal (Chen et al., 2019). The low temperature in the northern area is a key factor limiting such transplantation because mangroves are highly sensitive to low temperatures (Duke et al., 1998; Chen et al., 2010; Wu et al., 2018). Many studies have investigated the eco-physiological characteristics and adaptability of the artificial mangrove populations under low-temperature stress in Zhejiang Province (Song et al., 2009; Zheng et al., 2010, 2016; Wang et al., 2019; Lu et al., 2021). Nevertheless, it is still unknown to what extent low temperatures affect the renewal capacity and viability of these artificial mangrove populations.
Estimating the effective population size (NE), as well as identifying the factors that drive changes in NE, is important for evaluating population viability and developing appropriate management strategies for conservation biology (Frankham et al., 2014; Laikre et al., 2016; Trask et al., 2017). NE is a parameter of fundamental importance in population genetics and conservation biology, and it affects the magnitude of genetic drift and the efficacy of natural selection in populations (Araki et al., 2007; Charlesworth, 2009; Wang et al., 2016). Decreases in NE can have various deleterious effects, including the loss of genetic diversity and inbreeding depression of populations, which may increase extinction risk (Hare et al., 2011). NE can be affected by various intrinsic factors, including sex ratio, age structure, mating system, variation in reproductive success among individuals, fluctuations in the number of offspring, and spatial population structure (Schoen and Brown, 1991; Caballero and Hill, 1992; Frankham, 1995; Ruzzante et al., 2016; Trask et al., 2017). Extrinsic factors, such as anthropogenic activities, variation in climate, and stochastic events, may also induce changes in NE (Bucklin and Wiebe, 1998; Shrimpton and Heath, 2003; Araki et al., 2007; Okello et al., 2008; Espeland and Rice, 2010). For the artificial mangrove populations in the northern area, low temperature has a direct negative effect on NE by killing many seedlings and even adult trees; it can also influence population proliferation by causing immature flowers and fruits to prematurely detach from trees (Duke, 1990; Chen et al., 2010; Zheng et al., 2016; Lu et al., 2021). In addition, the variances of cold resistance ability of propagules and/or seedlings from different provenances may also affect NE of the artificial populations. Furthermore, the interaction between intrinsic and extrinsic factors may have a substantial effect on NE (Turner et al., 2006; Charlesworth, 2009), but it remains less understood. Therefore, more efforts are in need to examine both intrinsic and extrinsic factors and their joint effects on changes in NE of the artificial mangrove populations.
Estimating NE is of considerable difficulties empirically (Charlesworth, 2009; Robinson and Moyer, 2013; Jan et al., 2016; Wang et al., 2016). For the artificial mangrove population in Yueqing County, Zhejiang Province, however, we have a good opportunity to challenge the difficulty because individual plants in this population have clear transplanting and reforestation history. This is a population of Kandelia obovata (Rhizophoraceae) with plant individuals introduced from multiple provenances. K. obovata is a viviparous mangrove species that occurs widely across Southeast Asia, Southeastern China, and the Ryukyu Islands. It is a self-compatible species that depends on small insects for pollination (Tomlinson, 2016). K. obovata has been widely used for mangrove afforestation in China because of its ability to tolerate low temperatures (Lin et al., 1994; Yang and Lin, 1998; Chen et al., 2019). Since 1957, K. obovata has been introduced from multiple areas, including Hainan Province and Fujian Province, to the more northern area Yueqing County (YQ). Now YQ is newly defined as the northern range limit of this species in China (Li et al., 2001; Mu et al., 2005; Chen et al., 2019). Although the artificial population of K. obovata in YQ is adapted to low temperatures, extremely low temperatures still have substantial negative effects on its viability and proliferation (Lu et al., 2021). During the winter of 2015–2016, the YQ population was exposed to an exceptional low-temperature event in which the temperature fell to −5.1°C. The phenotypic responses of K. obovata in YQ population to such low temperatures included defoliation, bud withering, and death. Lu et al. (2021) reported three phenotypic groups of the YQ population according to the low-temperature tolerance of the individual plants: the SRC group with strong cold resistance, the MRC group with moderate resistance, and the PRC group with poor resistance. The authors further found that these phenotypic groups were genetically differentiated, which corresponds to the fact that they were transplanted from different source populations at different latitudes (Lu et al., 2021). The information provided by this previous study lays a foundation for an in-depth investigation of the intrinsic and extrinsic factors driving changes in NE so that we may predict the long-term viability of the population under chilling stress and adjust management strategies.
In the present study, we evaluated the influences of low temperature, mating system, and their interaction on changes in NE of the K. obovata population in YQ. We first analyzed genetic diversity and tested for population bottlenecks in the three phenotypic groups, SRC, MRC, and PRC, using ten nuclear and six plastid microsatellite loci. We then estimated mating system parameters and conducted paternity analyses for propagules and seedlings to determine the reproductive success of each phenotype group before and after the low-temperature event. On the bases of these analyses, we calculated NE and modeled changes in NE of the YQ population under different scenarios to characterize the joint effect of low temperature and mating system on the NE of this population. Our goals were to address the questions of to what extent low temperature, mating system, and their joint effect influence NE of the studied artificial population so that its viability and proliferation are influenced. The results of this study may provide guides for actions on reforestation management and transplantation of mangroves to higher-latitude areas.
2. Materials and methods
2.1. Study area and sample collection
Samples were collected from Yueqing County (YQ, 28°20′N, 121°10′E), Wenzhou City, Zhejiang Province, China. YQ experiences a tropical monsoon climate, with a mean annual temperature and precipitation of 17.7°C and 1,531.8 mm, respectively. At YQ, January is the coldest month (average temperature of 7.4°C). A total of 10 ha of mangroves had been planted in YQ since 1957; however, because of dam construction, anthropogenically induced damage, and poor management practices, the area of remaining mangrove forests is only 0.2 ha (Huang et al., 2009).
Two surveys were conducted to study the responses of K. obovata to extremely low temperatures and to collect samples from the YQ population from June to November 2016 (Figure 1). In the first survey, we collected leaves of all living adult individuals and seedlings. The coordinates of each individual sampled were taken using a Garmin eTrex30 (Garmin Ltd., Kansas, United States). All K. obovata individuals with propagules were used as mother trees, and all propagules from these mother trees were collected for paternity analysis. A second survey was conducted 5 months after the first survey. In this survey, we found that some ostensibly dead individuals in the first survey recovered, with leaves and branches sprouting from the base of the tree. We then collected leaf samples from all these individuals. A total of 183 individuals (including 35 seedlings) and nine propagules were analyzed for this study. Leaves and propagules collected were stored in plastic bags with silica gel until subsequent experiments.
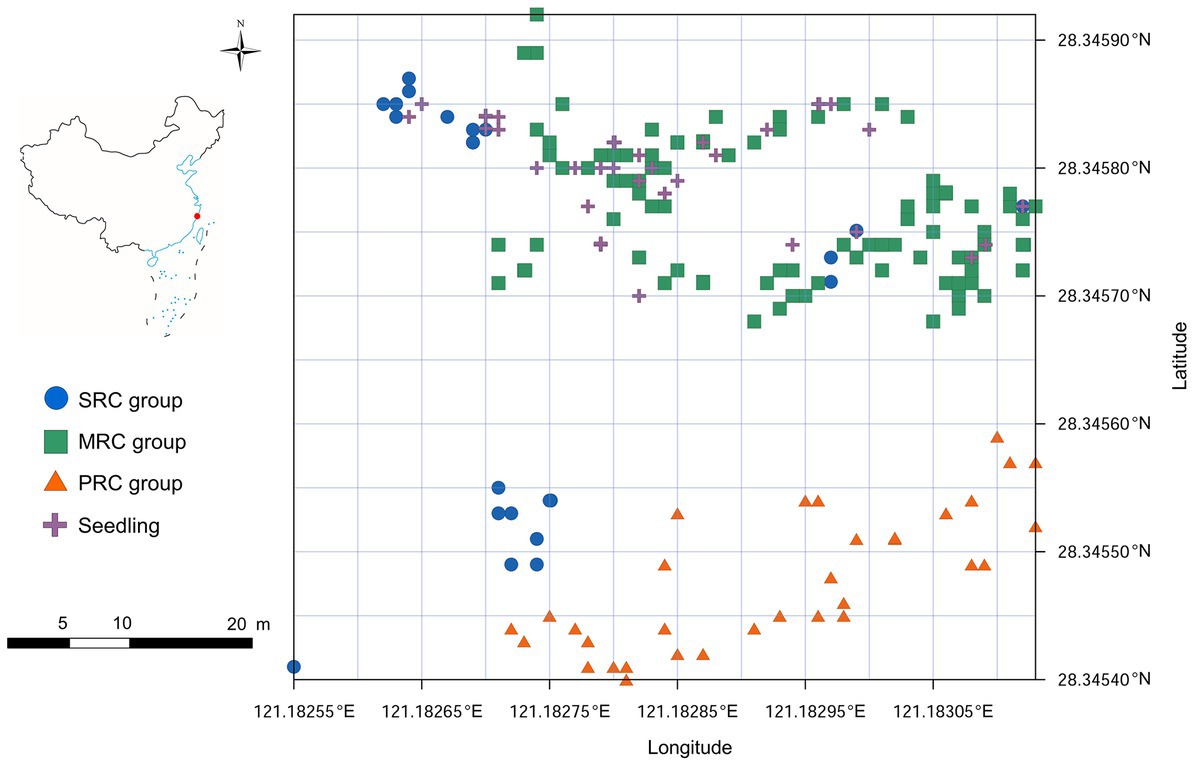
Figure 1. Location of the study area in China and distribution of Kandelia obovata individuals in YQ population. The horizontal axis represents longitude and the vertical axis represents latitude. SRC: individuals with strong resistance to cold; MRC: individuals with moderate resistance to cold; and PRC, individuals with poor resistance to cold.
2.2. DNA extraction and microsatellite screening
Total genomic DNA was extracted from the leaf samples of each seedling and from the propagule itself using a Plant Genomic DNA Kit DP305 (Tiangen Biotech, Beijing, China). The purity and concentration of the extracted DNA were determined using a NanoDrop 2000 spectrophotometer (Thermo Fisher Scientific, USA). Ten nuclear and six plastid microsatellite markers developed in previous studies were used for PCR amplification (Supplementary Table S1); thermal cycling conditions were based on those used in these previous studies (Sugaya et al., 2002; Islam et al., 2006, 2008). PCR products were screened using capillary electrophoresis by Sangon Biotech Co., Ltd. (Shanghai, China).
Based on the 10 nuclear and six plastid microsatellite loci, we screened 35 seedlings and nine propagules in the present study. Together with the 148 adult individuals that were genotyped in a previous study (Lu et al., 2021), we here evaluated the mating system, detected population bottlenecks, and estimated NE of the YQ population.
2.3. Genetic parameters
GENEPOP 3.4 was used to determine the linkage disequilibrium (LD), Hardy–Weinberg equilibrium (HWE), and null alleles for each nuclear microsatellite locus (Raymond and Rousset, 1995). Loci under selection were detected using Bayescan 2.0.1 Because the three phenotypic groups, SRC, MRC, and PRC, originated from different provenances at different latitudes, we calculated genetic parameters for the total population and for each of the three groups (regarded as sub-populations). GenAlEx 6.5 (Peakall and Smouse, 2006) was used to calculate genetic diversity parameters, including the number of alleles (Na), number of effective alleles (Ne), observed heterozygosity (Ho), expected heterozygosity (He), unbiased expected heterozygosity (uHe), and Shannon’s index of diversity (I). As genetic diversity estimates are affected by the number of individuals, ADZE 1.0 (Szpiech et al., 2008) was used to estimate the allelic richness (AR) and private allelic richness (PAR) for the SRC, MRC, and PRC groups.
Based on the plastid microsatellite data from adults, we calculated genetic diversity parameters Na, Ne, diversity (h), unbiased diversity (uh), and I for the whole population and for each of the three phenotype groups using GenAlEx 6.5 (Peakall and Smouse, 2006). We then analyzed the plastid haplotypes for adults and seedlings with Arlequin 3.5 (Excoffier and Lischer, 2010) and quantified divergence among the three groups and seedlings.
2.4. Mating system parameters and paternity analyses
The multilocus outcrossing rate (tm), single-locus outcrossing rate (ts), and biparental inbreeding rate (tm − ts) were determined using MLTR 3.2 (Ritland, 2002) with the nuclear microsatellite data. These parameters were calculated for both the entire population and each mother tree under the mixed-mating model with initial default parameter settings. Standard errors of the above parameters were evaluated using 1,000 bootstrap replications. GDA 1.12 was used to estimate the inbreeding coefficient (Fis) of each phenotypic group.
Because the development of a propagule starting from a flower bud takes approximately 11 months in K. obovata (Kamruzzaman et al., 2013), seedlings and developing propagules on the mother trees might separately reflect the proliferation of YQ population before and after exposure to low-temperature event during the winter of 2015–2016. The most likely pollen donor for each propagule was determined via maximum-likelihood assignment based on 10 nuclear loci in Cervus 3.0 (Slate et al., 2000). Prior to paternity analysis, 10,000 simulations were conducted using 150 individuals as candidate fathers, with 0.8 as the proportion of candidate fathers, 0.01 as the proportion of mistyped loci, 80% as the relaxed confidence level, and 95% as the strict confidence level. Next, complementary parent pairs of each seedling were detected using parent pair analysis (sex unknown) in Cervus 3.0 (Slate et al., 2000). Prior to the analysis, 10,000 simulations were conducted using the same parameter settings as described above in the propagule paternity analysis. Parentage assignment was then conducted following the methods described in previous studies (Geng et al., 2008; Lian et al., 2008). The logarithm of the odds score was used to identify the most likely parent when more than one individual was identified as a candidate parent. The sex of each candidate parent was determined using the maternally inherited plastid haplotype. The sex of the candidate parents could not be identified when each parent possessed identical plastid haplotypes.
2.5. Bottlenecks analysis and estimation of NE
To clarify the effect of extreme low temperatures on the three groups varying in cold resistance in the YQ population, we used BOTTLENECK 1.2.02 (Piry et al., 1999) to estimate the likelihood of population bottlenecks in the entire population and each of the three groups with nuclear microsatellite data. Analyses of bottlenecks were conducted using three models, the infinite allele model (IAA), the two-phase model (TPM), and the stepwise mutation model (SMM), with default settings. The significance of bottlenecks was estimated using the one-tailed Wilcoxon sign-rank test and sign test.
The LD method in NEEstimator 2.1 (Do et al., 2014) was used to determine the contemporary NE of the YQ population, and 95% confidence intervals for NE values were calculated using parametric and jackknife methods. Paternity and parent pair analysis revealed that the effective breeders were different before and after the low-temperature event. Therefore, NE was estimated for the whole population, for the SRC group + the MRC group, and for the SRC group separately, with the critical values determined based on the method of Waples and Do (2010).
2.6. Modeling changes in NE
Since the three phenotypic groups are introduced from different geographic provenances with observed population structures (Lu et al., 2021), the artificial YQ population can be regarded as a metapopulation. Changes in NE of the YQ population were simulated using GESP 1.01 (Olsson et al., 2017) to clarify the effects of intrinsic (mating system and reproductive success) and extrinsic factors (low temperature) on NE. The expected changes in NE of the metapopulation were modeled in a mathematical framework in GESP software. The values of NE and inbreeding coefficient of the subpopulations were set based on empirical estimates of NE of the possible source populations (Geng et al., 2021), as well as on our estimates for the three phenotypic groups. Changes in NE were modeled under four scenarios according to the results of analyses of population bottlenecks and paternity: Scenario I, the possible effects of low temperature on population size and variation in reproductive success are disregarded (Figure 2A); Scenario II, the PRC group is affected by the annual coldest air temperature, and its population size decreases steadily (Figure 2B). Notably, in this scenario, we considered two situations separately. One of them is the three subpopulations had the same reproductive contributions (w = 0.33), which was referred to as Scenario II in the subsequent result and discussion. The other one is the three subpopulations possessed different reproductive contributions, and we set different w for them (SRC = 0.2; MRC = 0.7; PRC = 0.1), which was called Scenario II different w; Scenario III, the MRC and PRC groups experience local bottlenecks caused by extreme low-temperature events between generations 20 and 30 (Figure 2C); and Scenario IV, some individuals with strong cold resistance are introduced from a higher-latitude population after extreme low-temperature events (Figure 2D). All scenarios were analyzed with initial inbreeding, and the number of migrants (m) was set to 2 to model gene flow between subpopulations. We simulated local bottleneck and introduction events by adjusting the sizes of the subpopulations in GESP software. We also set different subpopulation weights and inbreeding coefficients to explore the effects of reproductive success and mating system on changes in NE (Figure 2).
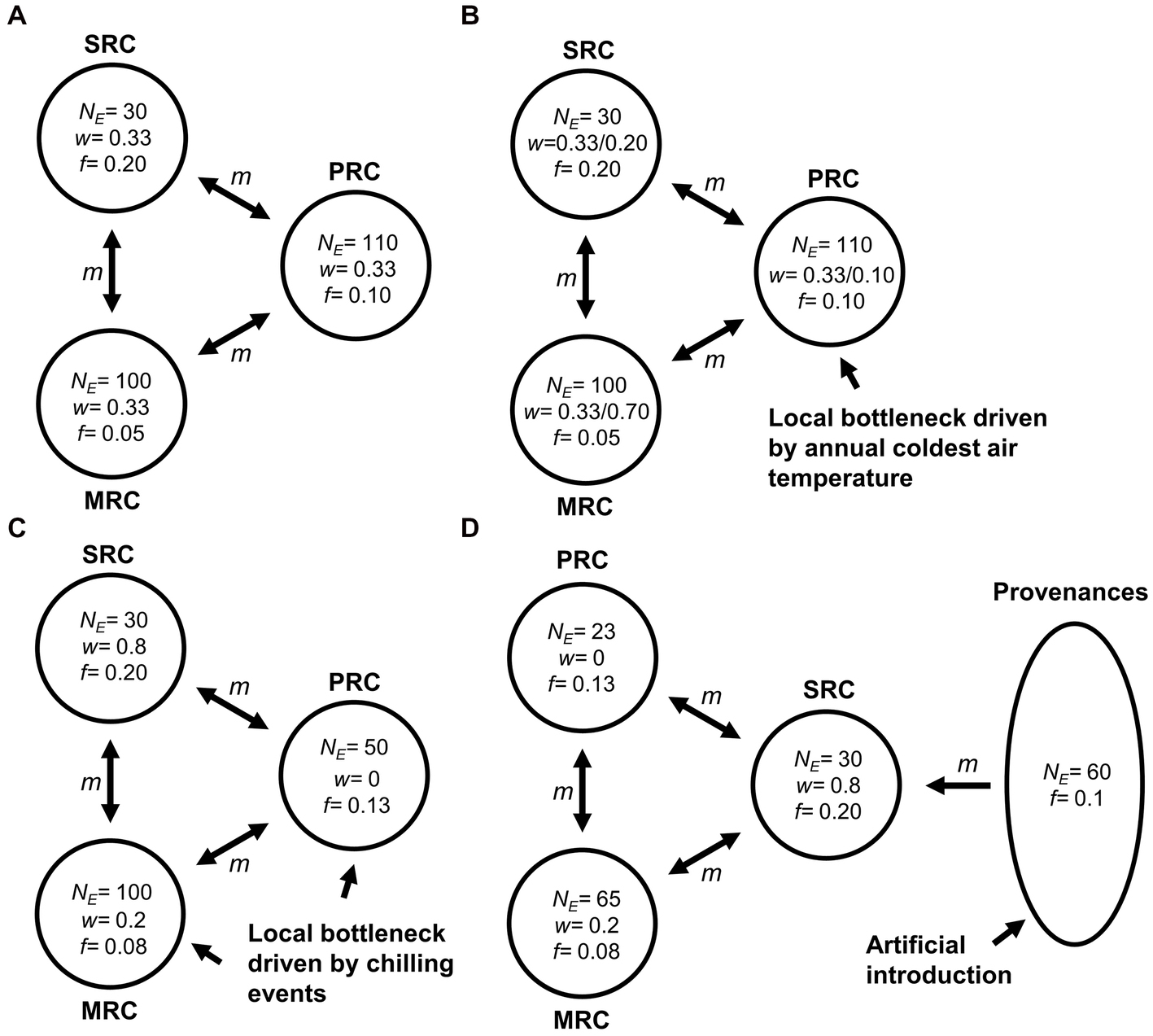
Figure 2. Schematic diagram of the Yueqing metapopulation of Kandelia obovata used for modeling changes in NE. We assume a metapopulation comprising three subpopulations and simulate four scenarios: (A) Scenario I: the effects of low temperature on each subpopulation are disregarded; (B) Scenario II: the subpopulation with poor cold resistance undergoes a local bottleneck induced by the annual coldest air temperature; (C) Scenario III: the subpopulations with moderate and poor cold resistance undergo local bottlenecks caused by extreme low-temperature events; and (D) Scenario IV: individuals from higher-latitude populations are introduced after extreme low-temperature events. SRC: group with strong resistance to cold; MRC: group with moderate resistance to cold; and PRC: group with poor resistance to cold. The values of NE and inbreeding levels (f) of each subpopulation under different scenarios are based on empirical estimates in Geng et al. (2021) and our results. Bidirectional gene flow between different subpopulations is indicated by two-headed arrows, and m indicates the strength of gene flow. Subpopulation weights (w) indicate the reproductive contributions of the different subpopulations, and subpopulation weight values are set based on the results of the parental analysis. In Scenario II, we consider two situations separately. One of them is the three subpopulations have the same reproductive contributions (w = 0.33), which is referred to as Scenario II in the subsequent result and discussion. The other one is the three subpopulations possess different reproductive contributions, and we set different w for them (SRC = 0.2; MRC = 0.7; PRC = 0.1), which is called Scenario II different w.
3. Results
3.1. Genetic diversity of the YQ artificial population
Genetic diversity was detected with the nuclear and plastid microsatellite markers separately. First, with the nuclear microsatellites, all 10 loci were identified by Bayescan as neutral for all individuals in the YQ population, and the average frequency of null alleles was 8.34% (0.51–31.37%, Supplementary Table S2). Significant deviations from HWE (heterozygote deficiency, p < 0.01) were observed for five loci (Kaca01, Kaca09, Kaca12, Kcan004, and Kcan034). Significant LD was observed for approximately a third of the pairwise comparisons (18 out of 45) in the YQ population (p < 0.01). A total of 107 alleles of the ten loci were detected in the total population, and the genetic diversity parameters of each locus are shown in Supplementary Table S3. Comparisons of genetic diversity parameters among the three groups that vary in cold resistance, and of the adult plants of the total YQ population are shown in Table 1: The lowest genetic diversity was found in the SRC group, and the highest in the MRC group; some parameters, e.g., Na (number of alleles) and PAR (private allelic richness) in the MRC group were nearly twice as high as those in the SRC group, and the highest PAR was found in the PRC group.
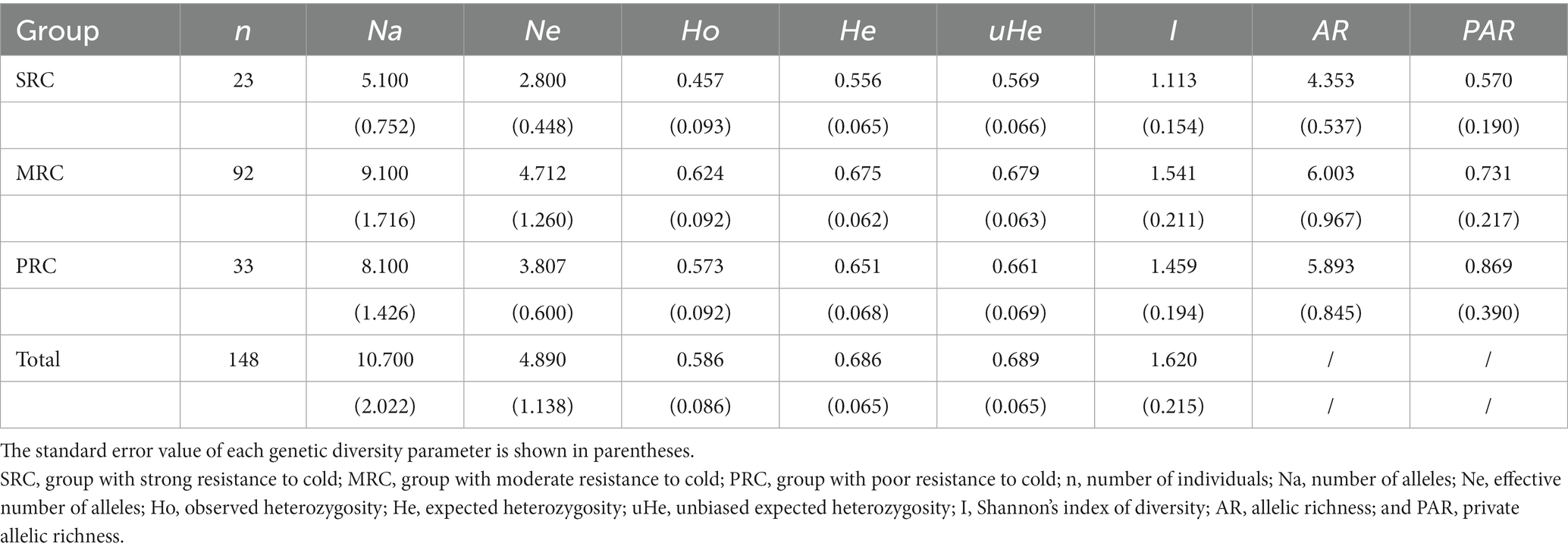
Table 1. Genetic diversity parameters within each of the phenotypic groups varying in cold resistance and the total population based on 10 nuclear microsatellite loci.
Second, with the plastid microsatellite markers, a total of 16 alleles were detected across six loci in the YQ population; the average number of alleles per locus was 2.667, with the minimum and the maximum number being one and seven, respectively (Supplementary Table S4). The genetic diversity of the plastid loci was inversely related to cold resistance. The highest polymorphism was found in the PRC group, and the lowest in the SRC group (Table 2). Two monomorphic loci (kacacp15 and kacacp16) were excluded from subsequent analyses. We detected 17 haplotypes from adults and seedlings in the YQ population across the four plastid loci (Supplementary Table S5). The frequent haplotypes are mostly specific to each of the three groups, and only one haplotype was shared between MRC and SRC. The two most frequent haplotypes of seedlings were also the most frequent in the MRC group (Figure 3A). Two haplotypes detected in seedlings were not present in adults.
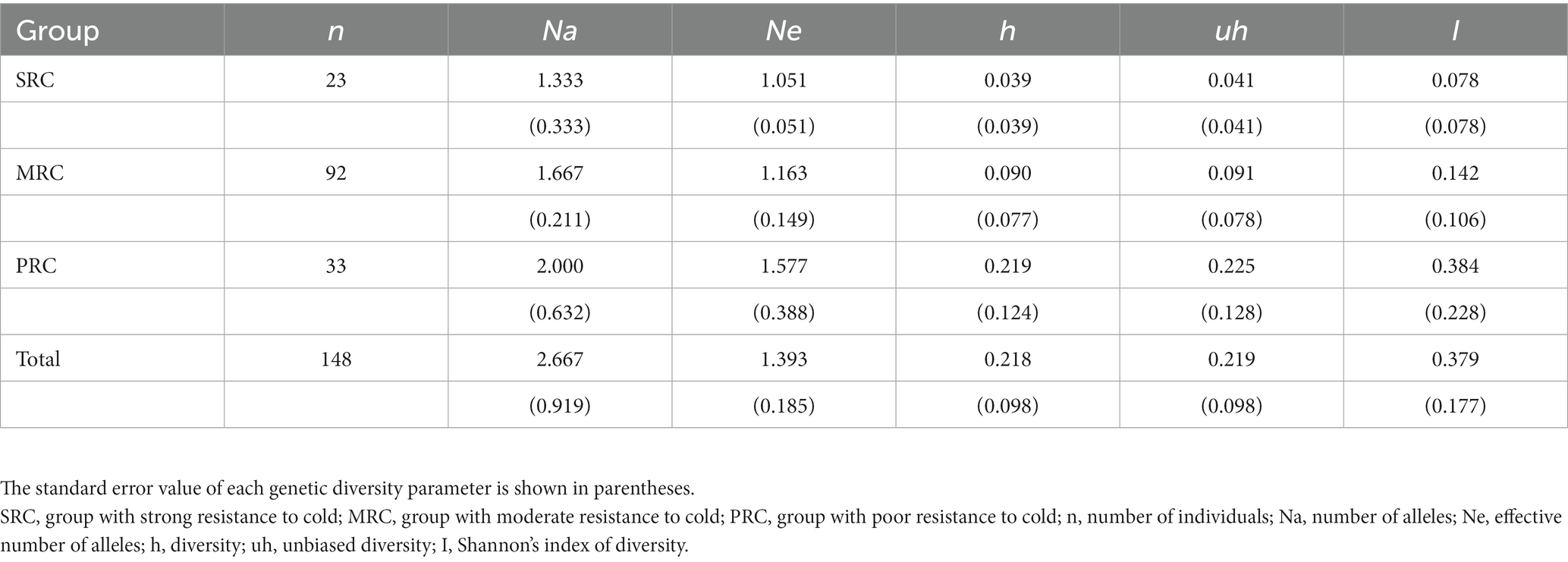
Table 2. Genetic diversity parameters within each of the phenotypic groups varying in cold resistance and the entire population based on six plastid microsatellite loci.
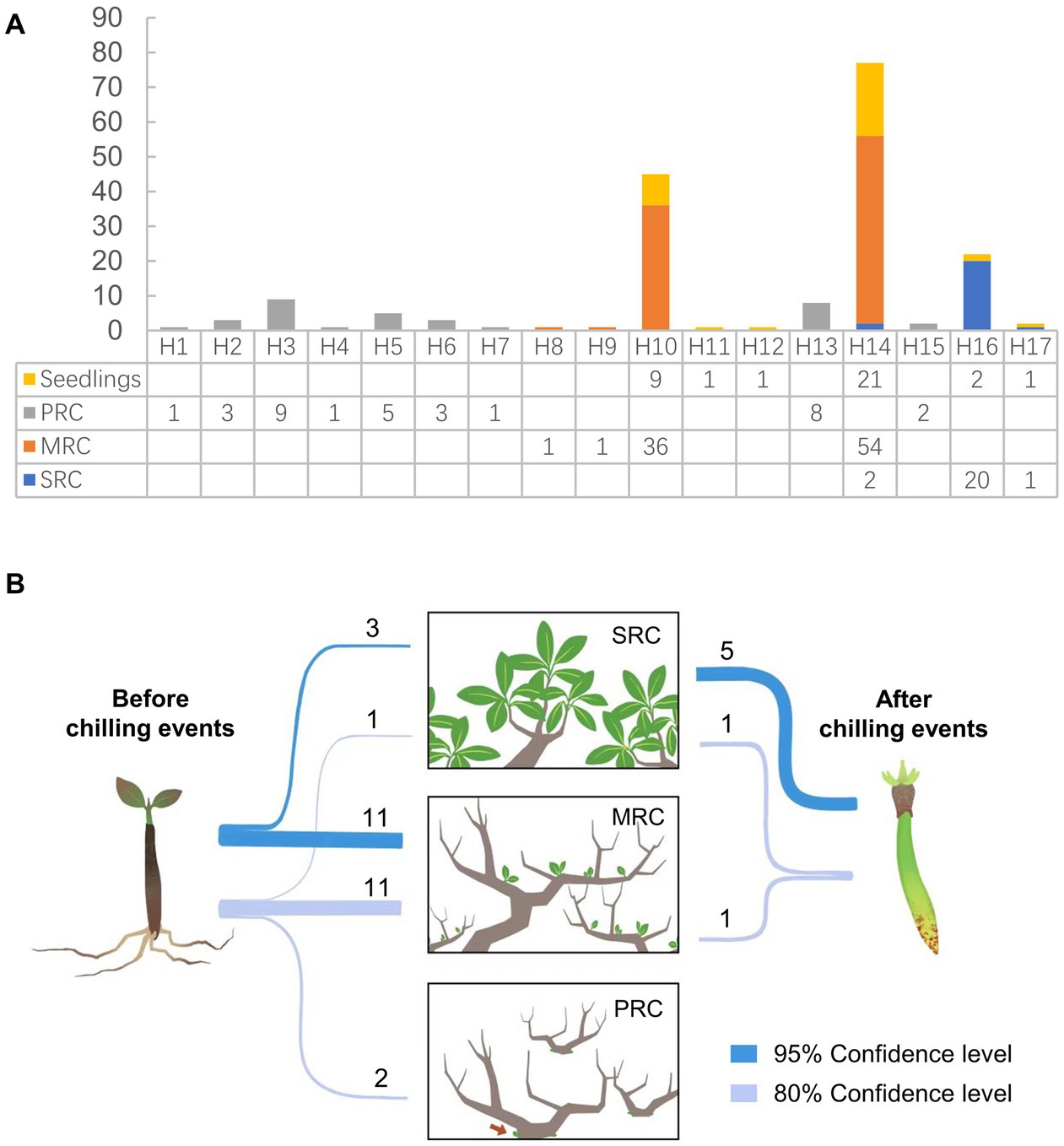
Figure 3. (A) Distribution of plastid haplotypes among seedlings and three phenotypic groups in the Yueqing population of Kandelia obovata. (B) Assignment of potential parents of seedlings and propagules among the three phenotypic groups. SRC: group with strong resistance to cold; MRC: group with moderate resistance to cold; and PRC: group with poor resistance to cold.
3.2. Mating system parameters, paternity analysis, and parentage analysis
The parameters tm, ts, and tm−ts in the YQ population were 1.200 (0.093), 1.000 (0.146), and 0.200 (0.122), respectively. Ranges of the tm and ts values of the mother trees were shown in Supplementary Table S6. Fis values were significantly greater than 0 in all three groups of the YQ population (SRC = 0.201, MRC = 0.081, and PRC = 0.135) and were especially high in the SRC and PRC groups.
Self-fertilization was rarely observed during this study. Pollen donors could be assigned for seven of the nine propagules analyzed, and five of the seven propagules were assigned at the strict (95%) confidence level (Figure 3B). Seven potential fathers fertilized these seven propagules; six of the most likely pollen donors were from the SRC group, and only one propagule was likely produced by a pollen donor from the MRC group. Parentage analysis revealed that the potential parents of 24 of the analyzed seedlings (68.6%) were among the adult plants sampled for this study (Figure 3B). Of these 24 seedlings, four were assigned with a pair of parents (at 80% confidence level), of which the paternal/maternal status could not be identified due to the shared plastid haplotype between the pair; for each of the other 20 seedlings, only one of the parent pair could be assigned, of the in total 20 candidate parents, nine were male and 11 were female. Approximately 78.6% of the potential parents of seedlings were assigned to the MRC group, and only 14.3% were assigned to the SRC group.
3.3. Bottlenecks and NE
Bottleneck analyses showed varying bottleneck effects among the three phenotypic groups and the entire population. Significant population bottlenecks were detected for the MRC and PRC groups, and for the entire population, but not for the SRC group. Significant heterozygote excess was observed in the MRC group under IAA and SMM models according to sign tests, and under the IAA model according to a one-tailed Wilcoxon sign-rank test. The PRC group showed a bottleneck effect under the SMM model (Table 3).
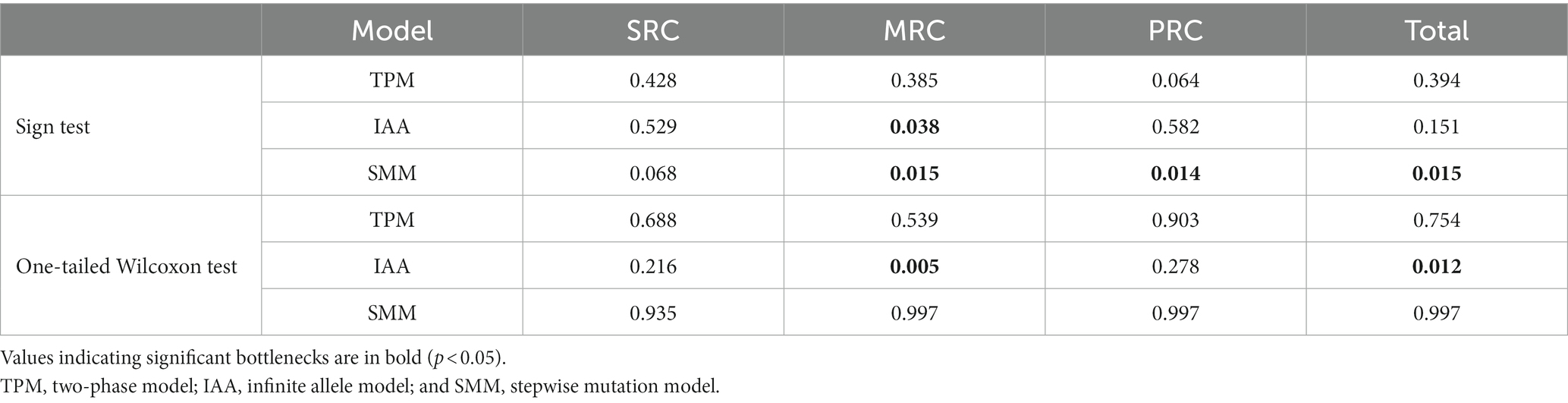
Table 3. The probability of population bottlenecks of the three phenotypic groups varying in cold resistance and the entire population based on 10 nuclear microsatellite loci under the three models.
The contemporary NE for the SRC group was 28.8, which was lower than that of the entire population (NE = 42.1). NE for the combined SRC plus MRC group (NE = 41.9) was slightly lower than that for the entire population. Changes in NE of the YQ population were modeled under four scenarios (Figure 2). NE of the metapopulation (entire population) was higher in Scenario I than in the other three scenarios (green line, Figure 4A). NE of the metapopulation decreased in Scenario II, and the equilibrium was achieved much more rapidly in Scenario II than in Scenario I (orange line, Figure 4A). The NE equilibrium of the metapopulation was achieved more rapidly in Scenario II when the subpopulation weights (w) were modified to simulate variation in reproductive success among groups (Scenario II different w, orange dotted line, Figure 4A). However, the values of NE of the metapopulation in Scenario II different w were similar with that of Scenario II (Figure 4A) no matter whether w was permitted to vary. Reductions in NE of the metapopulation were also observed in Scenario III. In Scenario IV, NE of the metapopulation decreased sharply to a value that was lower than the sum of NE of all the subpopulations (blue line, Figure 4A). Levels of inbreeding in the metapopulation decreased and then increased in all scenarios, and the magnitudes of the fluctuations varied among scenarios (Figure 4B).
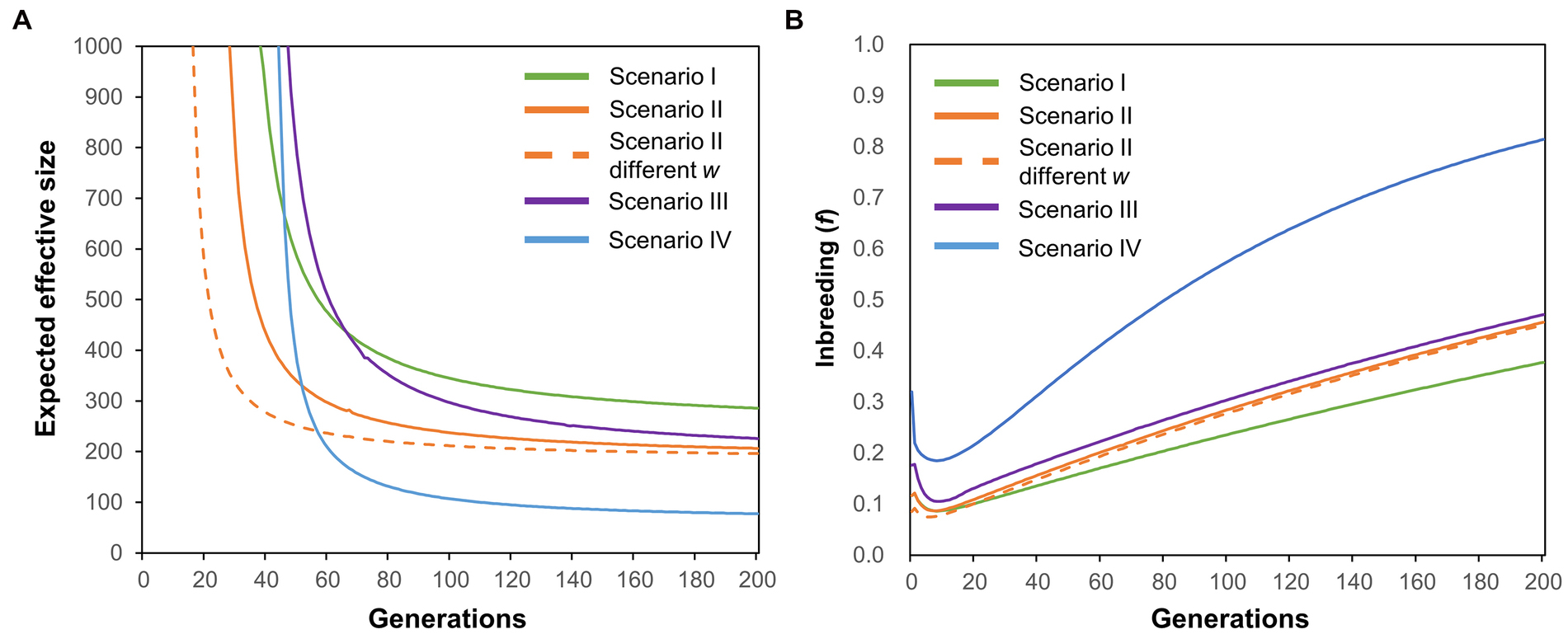
Figure 4. Expected effective size (A) and inbreeding levels (B) over 200 generations in a metapopulation under four scenarios. See Figure 2 and relevant descriptions in the main text for details regarding the four scenarios.
4. Discussion
Mangrove plants are sensitive to temperature, and they show a positive relationship between cold tolerance and the latitude of population localities (Duke et al., 1998; Yang and Lin, 1998; Chen et al., 2010; Wu et al., 2018). In the YQ population, the two phenotypic groups of which plants were introduced from low latitudes and displayed low cold resistance experienced bottlenecks following exposure to low temperatures. Bottleneck effects can result in changes in genetic composition of populations and loss of genetic diversity (Amos and Harwood, 1998; Bellinger et al., 2003), which often leads to inbreeding depression and endangers the genetic health, viability and recovery of populations (Keller et al., 2002; Frankham, 2005). Our data did reveal a negative correlation between genetic diversity (Na and Ne, Table 1) and the inbreeding coefficient (Fis) across different phenotypic groups in the YQ population. However, the lack of data before the low-temperature event prevents us from a direct assessment of the effect of bottlenecks on the inbreeding coefficient of this population.
Low temperatures may also affect the reproductive success of K. obovata. As the full development of a propagule needs approximately 11 months after fertilization (Kamruzzaman et al., 2013), the propagules we analyzed were those that survived the extreme low temperature in the winter of 2015–2016. Our results of the paternity analysis for those propagules indicated that their progenitors were mostly from the SRC group (Figure 3B), while parents of the seedlings produced before the low-temperature event were mostly from the MRC group, suggesting that the reproductive success of the three phenotypic groups varied under different environmental conditions. This may be the reason for the changes in contemporary NE values of the YQ population estimated by our genetic data of the effective breeders, which is in line with the findings of several previous studies that reported inter-individual variation in reproductive success being an important agent mediating decreases in NE (Araki et al., 2007; Ficetola et al., 2010; Trask et al., 2017). Nevertheless, the reductions in NE of the YQ population may be just temporary given that the viability of individuals in the MRC and PRC groups appeared well recovered after the low-temperature event (Lu et al., 2021), which needs further long-term investigations. Considering our data discussed above, we conclude that the effects of the interaction between low temperature and mating system on NE should be substantial for the YQ artificial population. In parallel, our simulation analysis revealed that environmental fluctuations, reproductive success, and the mating system could independently and jointly drive changes in NE of the metapopulation (Figure 4).
Temperature plays an important role in the survival and reproduction of mangrove species (Chen et al., 2010; de Lima Nadia et al., 2012; Tomlinson, 2016). For example, the reproductive success of Avicennia marina tends to be zero at higher latitudes (Duke, 1990), which means a severe reduction in effective population size according to the definition of NE (Wright, 1931). In the YQ artificial population of K. obovata, which is mixed with plant individuals introduced from natural populations at different latitudes, the limited reproductive contribution of individuals introduced from low-latitude may have played key roles in shaping the changes in NE of the metapopulation by affecting the level of inbreeding (Figure 4). Considering future forest management and conservation strategies of mangroves, we suggest that propagules from low-latitude regions, such as Hainan Province, not be used for transplantation to northern regions. Introduction of propagules from high and mid-latitude populations should be a more effective approach for mangrove reforestation, and interbreeding between individuals varying in resistance to cold may buffer fluctuations in NE if there exists “genetic compensation between cold resistance” (Araki et al., 2007). However, caution should be taken to avoid the introduction of individuals from source populations of low genetic diversity and NE so that to create a healthy transplanted population with good renewal capacity (Harmon and Braude, 2010).
In sum, the artificial mangrove populations in YQ with clear reforestation histories provided an excellent opportunity for us to study the diverse factors and their joint effects on changes in NE. We found that low-temperature events had a significant effect on the effective breeders of the YQ population because the reproductive success of its three ecologically and genetically differentiated groups became significantly different before and after the extreme low-temperature event. Nonetheless, this result needs supporting data from more studies as the sample size used to determine mating system parameters and to conduct parentage analyses in this study was relatively small, although we have collected all available samples from the YQ population. In addition, using genetic data to estimate variation in NE between generations and to identify the factors that drive changes in NE is often a considerable empirical challenge (Charlesworth, 2009; Robinson and Moyer, 2013; Jan et al., 2016; Wang et al., 2016). The mathematical framework for modeling changes in NE of a metapopulation provided by the GESP program is based on empirical or hypothetical estimates rather than on genetic data (Olsson et al., 2017). Therefore, the NE values of the simulated metapopulation might not reflect the “true” NE values of the YQ population, and our results only reflect estimates of NE dynamics of an artificial population under different scenarios. Anyhow, our findings provide insights into mangrove reforestation efforts, especially for introductions of mangroves to higher-latitude areas.
Data availability statement
The original contributions presented in the study are included in the article/Supplementary material, further inquiries can be directed to the corresponding author.
Author contributions
W-XL and S-CY conceived the idea, designed the experiments, collected the plant materials, and revised the manuscript. W-XL and B-HZ conducted the molecular work. W-XL performed the analyses and drafted the manuscript. All authors contributed to the study and approved the submitted version.
Funding
This research was supported by the National Key Research and Development Program of China [grant no. 2017YFC0506103]; Ocean Public Fund Research Project Grants of State Oceanic Administration [grant no. 201305021–2]; and the National Natural Science Foundation of China [grant no. 30972334].
Acknowledgments
The authors wish to thank Wan-Yu Zhu for her help with the illustrations. Additionally, the authors thank all reviewers for their comments and suggestions.
Conflict of interest
The authors declare that they have no known competing financial interests or personal relationships that could have appeared to influence the work reported in this paper.
Publisher’s note
All claims expressed in this article are solely those of the authors and do not necessarily represent those of their affiliated organizations, or those of the publisher, the editors and the reviewers. Any product that may be evaluated in this article, or claim that may be made by its manufacturer, is not guaranteed or endorsed by the publisher.
Supplementary material
The Supplementary material for this article can be found online at: https://www.frontiersin.org/articles/10.3389/fevo.2023.1160468/full#supplementary-material
Footnotes
References
Alongi, D. M. (2012). Carbon sequestration in mangrove forests. Carbon Manag. 3, 313–322. doi: 10.4155/cmt.12.20
Amos, W., and Harwood, J. (1998). Factors affecting levels of genetic diversity in natural populations. Philos. Trans. R. Soc. Lond. B Biol. Sci. 353, 177–186. doi: 10.1098/rstb.1998.0200
Araki, H., Waples, R. S., Ardren, W. R., Cooper, B., and Blouin, M. S. (2007). Effective population size of steelhead trout: influence of variance in reproductive success, hatchery programs, and genetic compensation between life-history forms. Mol. Ecol. 16, 953–966. doi: 10.1111/j.1365-294X.2006.03206.x
Bellinger, M. R., Johnson, J. A., Toepfer, J., and Dunn, P. (2003). Loss of genetic variation in greater prairie chickens following a population bottleneck in Wisconsin, U.S.A. Conserv. Biol. 17, 717–724. doi: 10.1046/j.1523-1739.2003.01581.x
Bologna, P. A., Campanella, J. J., Restaino, D. J., Fetske, Z. A., Lourenco, M., and Smalley, J. V. (2019). Lingering impacts of hurricane Hugo on Rhizophora mangle (red mangrove) population genetics on St. John, USVI. Diversity 11:65. doi: 10.3390/d11040065
Bucklin, A., and Wiebe, P. (1998). Low mitochondrial diversity and small effective population sizes of the copepods Calanus finmarchicus and Nannocalanus minor: possible impact of climatic variation during recent glaciation. J. Hered. 89, 383–392. doi: 10.1093/jhered/89.5.383
Caballero, A., and Hill, W. (1992). Effective size of nonrandom mating populations. Genetics 130, 909–916. doi: 10.1093/genetics/130.4.909
Carugati, L., Gatto, B., Rastelli, E., Lo Martire, M., Coral, C., Greco, S., et al. (2018). Impact of mangrove forests degradation on biodiversity and ecosystem functioning. Sci. Rep. 8, 1–11. doi: 10.1038/s41598-018-31683-0
Charlesworth, B. (2009). Effective population size and patterns of molecular evolution and variation. Nat. Rev. Genet. 10, 195–205. doi: 10.1038/nrg2526
Chen, L.-Z., Wang, W.-Q., Zhang, Y.-H., Huang, L., Zhao, C.-L., Yang, S.-C., et al. (2010). Damage to mangroves from extreme cold in early 2008 in southern China (in Chinese). Chin. J. Plant Ecol. 34, 186–194. doi: 10.3773/j.issn.1005-264x.2010.02.010
Chen, Q.-X., Yang, S., Wang, J.-W., Liu, X., Zheng, J., and Deng, R.-J. (2019). Development history and discussion of mangrove forest in Zhejiang Province (in Chinese). J. Zhejiang Agric. Sci. 60, 1177–1181. doi: 10.16178/j.issn.0528-9017.20190734
de Lima Nadia, T., Morellato, L. P. C., and Machado, I. C. (2012). Reproductive phenology of a northeast Brazilian mangrove community: environmental and biotic constraints. Flora Morphol. Distrib. Funct. Ecol. Plants 207, 682–692. doi: 10.1016/j.flora.2012.06.020
Do, C., Waples, R. S., Peel, D., Macbeth, G., Tillett, B. J., and Ovenden, J. R. (2014). NeEstimator v2: re-implementation of software for the estimation of contemporary effective population size (ne) from genetic data. Mol. Ecol. Resour. 14, 209–214. doi: 10.1111/1755-0998.12157
Duke, N. (1990). Phenological trends with latitude in the mangrove tree Avicennia marina. J. Ecol. 78, 113–133. doi: 10.2307/2261040
Duke, N., Ball, M., and Ellison, J. (1998). Factors influencing biodiversity and distributional gradients in mangroves. Glob. Ecol. Biogeogr. Lett. 7, 27–47. doi: 10.2307/2997695
Eddy, S., Milantara, N., Sasmito, S. D., Kajita, T., and Basyuni, M. (2021). Anthropogenic drivers of mangrove loss and associated carbon emissions in South Sumatra Indonesia. Forests 12:187. doi: 10.3390/f12020187
Espeland, E., and Rice, K. (2010). Ecological effects on estimates of effective population size in an annual plant. Biol. Conserv. 143, 946–951. doi: 10.1016/j.biocon.2010.01.003
Excoffier, L., and Lischer, H. E. (2010). Arlequin suite ver 3.5: a new series of programs to perform population genetics analyses under Linux and windows. Mol. Ecol. Resour. 10, 564–567. doi: 10.1111/j.1755-0998.2010.02847.x
Ficetola, G. F., Padoa-Schioppa, E., Wang, J., and Garner, T. W. (2010). Polygyny, census and effective population size in the threatened frog, Rana latastei. Anim. Conserv. 13, 82–89. doi: 10.1111/j.1469-1795.2009.00306.x
Field, C. D. (1995). “Impact of expected climate change on mangroves” in Asia-Pacific symposium on mangrove ecosystems. eds. Y. S. Wong and N. F. Y. Tam (Dordrecht: Springer), 75–81. doi: 10.1007/978-94-011-0289-6_10
Frankham, R. (1995). Effective population size/adult population size ratios in wildlife: a review. Genet. Res. 66, 95–107. doi: 10.1017/S0016672300034455
Frankham, R. (2005). Genetics and extinction. Biol. Conserv. 126, 131–140. doi: 10.1016/j.biocon.2005.05.002
Frankham, R., Bradshaw, C. J., and Brook, B. W. (2014). Genetics in conservation management: revised recommendations for the 50/500 rules, red list criteria and population viability analyses. Biol. Conserv. 170, 56–63. doi: 10.1016/j.biocon.2013.12.036
Geng, Q., Lian, C., Goto, S., Tao, J., Kimura, M., Islam, M. S., et al. (2008). Mating system, pollen and propagule dispersal, and spatial genetic structure in a high-density population of the mangrove tree Kandelia candel. Mol. Ecol. 17, 4724–4739. doi: 10.1111/j.1365-294X.2008.03948.x
Geng, Q., Wang, Z., Tao, J., Kimura, M. K., Liu, H., Hogetsu, T., et al. (2021). Ocean currents drove genetic structure of seven dominant mangrove species along the coastlines of southern China. Front. Genet. 12:615911. doi: 10.3389/fgene.2021.615911
Hare, M. P., Nunney, L., Schwartz, M. K., Ruzzante, D. E., Burford, M., and Waples, R. S. (2011). Understanding and estimating effective population size for practical application in marine species management. Conserv. Biol. 25, 438–449. doi: 10.1111/j.1523-1739.2010.01637.x
Harmon, L. J., and Braude, S. (2010). Conservation of small populations: effective population sizes, inbreeding, and the 50/500 rule. New Jersey: Princeton University Press.
Huang, X., Peng, X., Qiu, J., and Chen, S. (2009). Mangrove status and development prospects in southern Zhejiang Province (in Chinese). J. Zhejiang Forest. Coll. 26, 427–433.
Islam, M. S., Lian, C., Geng, Q., Kameyama, N., and Hogetsu, T. (2008). Chloroplast microsatellite markers for the mangrove tree species Bruguiera gymnorrhiza, Kandelia candel, and Rhizophora stylosa, and cross–amplification in other mangrove species. Conserv. Genet. 9, 989–993. doi: 10.1007/s10592-007-9424-x
Islam, M., Tao, J., Geng, Q., Lian, C., and Hogetsu, T. (2006). Isolation and characterization of eight compound microsatellite markers in a mangrove tree Kandelia candel (L.) Druce. Mol. Ecol. Notes 6, 1111–1113. doi: 10.1111/j.1471-8286.2006.01453.x
Jan, P. L., Gracianne, C., Fournet, S., Olivier, E., Arnaud, J. F., Porte, C., et al. (2016). Temporal sampling helps unravel the genetic structure of naturally occurring populations of a phytoparasitic nematode. 1. Insights from the estimation of effective population sizes. Evol. Appl. 9, 489–501. doi: 10.1111/eva.12352
Kamruzzaman, M., Sharma, S., and Hagihara, A. (2013). Vegetative and reproductive phenology of the mangrove Kandelia obovata plant species. Biology 28, 118–129. doi: 10.1111/j.1442-1984.2012.00367.x
Keller, L. F., Grant, P. R., Grant, B. R., and Petren, K. (2002). Environmental conditions affect the magnitude of inbreeding depression in survival of Darwin’s finches. Evolution 56, 1229–1239. doi: 10.1111/j.0014-3820.2002.tb01434.x
Laikre, L., Olsson, F., Jansson, E., Hössjer, O., and Ryman, N. (2016). Metapopulation effective size and conservation genetic goals for the Fennoscandian wolf (Canis lupus) population. Heredity 117, 279–289. doi: 10.1038/hdy.2016.44
Li, J.-Q., Xu, H.-F., Ye, Z.-L., Gu, J.-F., and Wang, R.-Q. (2001). Introduction andafforestation technique of Kandelia candel to the north (in Chinese). J. Zhejiang Forest. Sci. Technol. 21, 51–53.
Lian, C., Goto, S., Kubo, T., Takahashi, Y., Nakagawa, M., and Hogetsu, T. (2008). Nuclear and chloroplast microsatellite analysis of Abies sachalinensis regeneration on fallen logs in a subboreal forest in Hokkaido, Japan. Mol. Ecol. 17, 2948–2962. doi: 10.1111/j.1365-294X.2008.03802.x
Lin, P., Shen, R., and Lu, C. (1994). The characteristics of chilling-resistance on six mangrove plants (in Chinese). J. Xiamen Univ. 33, 249–252.
Lu, W.-X., Zhang, B.-H., Zhang, Y.-Y., and Yang, S.-C. (2021). Differentiation of cold tolerance in an artificial population of a mangrove species, Kandelia obovata, is associated with geographic origins. Front. Plant Sci. 12, –695746. doi: 10.3389/fpls.2021.695746
Lu, W.-X., Zou, Z., Hu, X.-Y., and Yang, S.-C. (2022). Genetic diversity and mating system of two mangrove species (Rhizophora apiculata and Avicennia marina) in a heavily disturbed area of China. Diversity 14:115. doi: 10.3390/d14020115
Mu, A.-Y., Liu, J.-J., Yang, J.-Q., Teng, Y.-P., and Zhou, C.-Q. (2005). Investigation of introduction mangroves of Kandelia candel Druce in the northest boundary of China (in Chinese). Protect. Forest Sci. Technol. 1, 6–8. doi: 10.3969/j.issn.1005-5215.2005.z1.004
Okello, J., Wittemyer, G., Rasmussen, H., Arctander, P., Nyakaana, S., Douglas-Hamilton, I., et al. (2008). Effective population size dynamics reveal impacts of historic climatic events and recent anthropogenic pressure in African elephants. Mol. Ecol. 17, 3788–3799. doi: 10.1111/j.1365-294X.2008.03871.x
Olsson, F., Laikre, L., Hössjer, O., and Ryman, N. (2017). GESP: a computer program for modelling genetic effective population size, inbreeding and divergence in substructured populations. Mol. Ecol. Resour. 17, 1378–1384. doi: 10.1111/1755-0998.12673
Peakall, R., and Smouse, P. E. (2006). GENALEX 6: genetic analysis in excel. population genetic software for teaching and research. Mol. Ecol. Notes 6, 288–295. doi: 10.1111/j.1471-8286.2005.01155.x
Piry, S., Luikart, G., and Cornuet, J. M. (1999). Computer note. BOTTLENECK: a computer program for detecting recent reductions in the effective size using allele frequency data. J. Hered. 90, 502–503. doi: 10.1093/jhered/90.4.502
Raymond, M., and Rousset, F. (1995). GENEPOP (version 1.2): population genetics software for exact tests and ecumenicism. J. Hered. 86, 248–249. doi: 10.1093/oxfordjournals.jhered.a111573
Ritland, K. (2002). Extensions of models for the estimation of mating systems using n independent loci. Heredity 88, 221–228. doi: 10.1038/sj.hdy.6800029
Robinson, J. D., and Moyer, G. R. (2013). Linkage disequilibrium and effective population size when generations overlap. Evol. Appl. 6, 290–302. doi: 10.1111/j.1752-4571.2012.00289.x
Ruzzante, D. E., McCracken, G. R., Parmelee, S., Hill, K., Corrigan, A., MacMillan, J., et al. (2016). Effective number of breeders, effective population size and their relationship with census size in an iteroparous species, Salvelinus fontinalis. Proc. R. Soc. B Biol. Sci. 283:20152601. doi: 10.1098/rspb.2015.2601
Schoen, D. J., and Brown, A. (1991). Intraspecific variation in population gene diversity and effective population size correlates with the mating system in plants. Proc. Natl. Acad. Sci. U. S. A. 88, 4494–4497. doi: 10.1073/pnas.88.10.4494
Shrimpton, J. M., and Heath, D. D. (2003). Census vs. effective population size in Chinook salmon: large-and small-scale environmental perturbation effects. Mol. Ecol. 12, 2571–2583. doi: 10.1046/j.1365-294X.2003.01932.x
Slate, J., Marshall, T., and Pemberton, J. (2000). A retrospective assessment of the accuracy of the paternity inference program CERVUS. Mol. Ecol. 9, 801–808. doi: 10.1046/j.1365-294x.2000.00930.x
Song, Y., Meng, Y., and Chen, S. (2009). Adaptation analysis of artificial introduction of mangrove in Wenzhou, China. In: 2009 3rd International Conference on Bioinformatics and Biomedical Engineering IEEE, 1–4. doi: 10.1109/ICBBE.2009.5162277
Sugaya, T., Takeuchi, T., Yoshimaru, H., and Katsuta, M. (2002). Development and polymorphism of simple sequence repeat DNA markers for Kandelia candel (L.) Druce. Mol. Ecol. Notes 2, 65–66. doi: 10.1046/j.1471-8286.2002.00151.x
Szpiech, Z. A., Jakobsson, M., and Rosenberg, N. A. (2008). ADZE: a rarefaction approach for counting alleles private to combinations of populations. Bioinformatics 24, 2498–2504. doi: 10.1093/bioinformatics/btn478
Trask, A. E., Bignal, E. M., McCracken, D. I., Piertney, S. B., and Reid, J. M. (2017). Estimating demographic contributions to effective population size in an age-structured wild population experiencing environmental and demographic stochasticity. J. Anim. Ecol. 86, 1082–1093. doi: 10.1111/1365-2656.12703
Turner, T. F., Osborne, M. J., Moyer, G. R., Benavides, M. A., and Alo, D. (2006). Life history and environmental variation interact to determine effective population to census size ratio. Proc. R. Soc. B Biol. Sci. 273, 3065–3073. doi: 10.1098/rspb.2006.3677
Wang, W., Fu, H., Lee, S. Y., Fan, H., and Wang, M. (2020). Can strict protection stop the decline of mangrove ecosystems in China? From rapid destruction to rampant degradation. Forests 11:55. doi: 10.3390/f11010055
Wang, J., Santiago, E., and Caballero, A. (2016). Prediction and estimation of effective population size. Heredity 117, 193–206. doi: 10.1038/hdy.2016.43
Wang, Z., Yu, D., Zheng, C., Wang, Y., Cai, L., Guo, J., et al. (2019). Ecophysiological analysis of mangrove seedlings Kandelia obovata exposed to natural low temperature at near 30°N. J. Mar. Sci. Eng. 7:292. doi: 10.3390/jmse7090292
Waples, R. S., and Do, C. H. I. (2010). Linkage disequilibrium estimates of contemporary ne using highly variable genetic markers: a largely untapped resource for applied conservation and evolution. Evol. Appl. 3, 244–262. doi: 10.1111/j.1752-4571.2009.00104.x
Wright, S. (1931). Evolution in Mendelian populations. Genetics 16, 97–159. doi: 10.1093/genetics/16.2.97
Wu, Y., Ricklefs, R. E., Huang, Z., Zan, Q., and Yu, S. (2018). Winter temperature structures mangrove species distributions and assemblage composition in China. Glob. Ecol. Biogeogr. 27, 1492–1506. doi: 10.1111/geb.12826
Yang, S., and Lin, P. (1998). Ecological studies on the resistance and adaptation to cold of some tidal mangrove species in China (in Chinese). Chin. J. Plant Ecol. 22, 60–67.
Yang, S.-C., Lu, W.-X., Zou, Z., and Li, S. (2017). Mangrove wetlands: distribution, species composition and protection in China (in Chinese). Subtrop. Plant Sci. 46, 301–310. doi: 10.3969/j.issn.1009-7791.2017.04.001
Zheng, J., Wang, J.-W., Chen, Q.-X., Xu, J.-Y., Li, X.-W., Lu, X., et al. (2010). Preliminary report on north–ward introduction experiment of several mangrove plants along the southern coast of Zhejiang Province (in Chinese). J. Southwest Forest. Univ. 30, 11–17.
Keywords: effective population size, low temperature, mating system, reproductive success, effective breeder, Kandelia obovata
Citation: Lu W-X, Zhang B-H and Yang S-C (2023) Survive the north: transplantation for conservation of mangrove forests requires consideration of influences of low temperature, mating system and their joint effects on effective size of the reforested populations. Front. Ecol. Evol. 11:1160468. doi: 10.3389/fevo.2023.1160468
Edited by:
Helen Regan, University of California, Riverside, United StatesReviewed by:
Paul Bologna, Montclair State University, United StatesTarcila Nadia, Federal University of Pernambuco, Brazil
Copyright © 2023 Lu, Zhang and Yang. This is an open-access article distributed under the terms of the Creative Commons Attribution License (CC BY). The use, distribution or reproduction in other forums is permitted, provided the original author(s) and the copyright owner(s) are credited and that the original publication in this journal is cited, in accordance with accepted academic practice. No use, distribution or reproduction is permitted which does not comply with these terms.
*Correspondence: Sheng-Chang Yang, c2N5YW5nQHhtdS5lZHUuY24=