- Department of Geography and Earth Sciences, University of North Carolina at Charlotte, Charlotte, NC, United States
Introduction: Plastics are found in ecosystems worldwide and can have widespread impacts on organisms and the environment. Cathartid vultures, including the black vulture (Coragyps atratus) and the turkey vulture (Cathartes aura), have adapted to urbanized environments, making frequent use of human-made structures and anthropogenic resources. Thus, urban vultures are likely exposed to more plastic materials than rural vultures, which they intentionally or unintentionally ingest when foraging or loafing.
Methods: Our objective was to determine the extent and type of plastic ingested by black and turkey vultures in an urban environment by (1) measuring the plastic content of regurgitated pellets collected along an urban-to-rural gradient, and (2) identifying the plastics within pellets. We dissected 1,087 pellets collected at eight vulture congregation sites in the Charlotte Metropolitan Area, United States between January 2021 and July 2022.
Results and Discussion: Sixty percent of pellets contained plastic materials, with an average plastic composition by weight of 2.66 ± 8.76%. Repeated measures linear mixed models of the proportion of pellets that were plastic suggested that black and turkey vultures are ingesting more plastic materials when congregation sites are surrounded by more developed landcover and a greater density of commercial food providers, such as food stores and restaurants, within 20km. Fourier transform infrared (FTIR) spectroscopy of a subset of pellets indicated that the most common types of plastic ingested by vultures were silicone rubber (used in tires and automobile/boat seals) and polyethylene (used in plastic bags and food packages). Future research should investigate the relative importance of plastic sources in vulture diets, vulture behavioral changes associated with plastic ingestion, and the consequences of plastic pollution on species health and urban ecosystem functioning.
1. Introduction
Plastics are widely used by humans and are found in ecosystems worldwide (Barnes et al., 2009). Common sources of environmental plastics include personal care products, plastic mulch, tires, and synthetic paints (Duis and Coors, 2016). In addition to urban areas, plastics have been found in remote locations such as the summit of Mount Everest (Napper et al., 2020) and the Mariana Trench (Jamieson et al., 2019). There is evidence of the cycling of plastics in each of Earth’s major subsystems (Dris et al., 2015, 2016; Wilcox et al., 2015; de Souza Machado et al., 2018) but the impact of pervasive plastic pollution on natural systems is not well understood.
Cathartid vultures (i.e., vultures from the Cathartidae family) have adapted to urbanized environments and are commonly considered pests in cities (Blackwell et al., 2007). Black vultures (Coragyps atratus) are a New World vulture species that use human-made structures and resources frequently. They regularly roost on transmission and cellular towers (Buckley, 1998; Avery et al., 2002; Seamans, 2004), nest in abandoned buildings (Stewart, 1974; Houston C. S. et al., 2007; Crowley et al., 2022), and forage regularly at street markets, dumpsters, and landfills in large numbers (Elías, 1987; Novaes and Cintra, 2015; Cunha et al., 2022; Hill et al., 2022). Turkey vultures (Cathartes aura), with a range that overlaps that of black vultures, may also use human-subsidized resources in urbanized environments, such as transmission towers in suburban and exurban areas for roosting, but they prefer rural and forested landscapes (Novaes and Cintra, 2015).
Plastic materials have been found in regurgitated black vulture pellets since at least the 1980s (Elías, 1987), showing evidence of plastic ingestion dating back decades. More recently, 30% of black or turkey vulture pellets in South Carolina (Hill et al., 2022) and 82% in the Falkland Islands contained plastics (Augé, 2017). In Patagonia, black and turkey vultures roosting near dumpsters and landfills had a high probability of ingesting plastic (Ballejo et al., 2021) and in Florida, the black vulture was the only raptor species found to ingest plastics (Carlin et al., 2020). This literature shows significant and widespread ingestion of plastic materials by black and turkey vultures across North and South America.
Vultures may be intentionally or unintentionally ingesting plastic materials by building up bulk to expel pellets (Houston D. C. et al., 2007), mistaking plastic materials as bone fragments, or ingesting plastics from carcasses and other food sources. Additionally, vultures may be ingesting plastic materials while loafing as they are known to regularly pick at materials such as boat seats, rubber seals, or roofing materials. Regardless of the cause, the impacts of plastic ingestion on vulture adults and juveniles are not well understood. Plastic ingestion could lead to gut blockages, internal injuries, and mortality (Houston D. C. et al., 2007) and plastics could contain pollutants and heavy metals (Borges-Ramírez et al., 2021)–black vultures that ingest more plastic materials have worse health than those that ingest less plastic (Cunha et al., 2022).
Although there is evidence of black and turkey vulture pellets containing plastics, to our knowledge, only a single study has investigated the ingestion of plastics by these species and their sources in an urban environment (Carlin et al., 2020). Given the potential impacts to vulture health, environmental plastic pollution, and ecosystem functioning, our objective was to determine the extent and type of plastic ingested by black and turkey vultures in an urban environment by (1) measuring the plastic content of regurgitated pellets collected along an urban-to-rural gradient, and (2) identifying the plastics within pellets. We predicted that the plastic content of pellets would increase with developed landcover, density of commercial food providers and density of livestock and game producers in landscapes surrounding pellet collection sites, and decreasing distance to the nearest landfill.
2. Materials and methods
2.1. Study area
The Charlotte Metropolitan Area (CMA) is an urban area in North Carolina and South Carolina composed of 12 counties surrounding the City of Charlotte. The CMA human population is estimated at 2.8 million with rapid growth rates over the last two decades (US Census Bureau, 2019). The population of Mecklenburg County, in which Charlotte is located, is expected to grow by over 570,000 people between 2010 and 2040, an annual rate of 2.3% (Future, 2019). Charlotte largely consists of developed landcover types, but development within the CMA is sprawling and dominated by developed open space and single-family housing.
Objective 1: Plastic content of regurgitated pellets along an urban-to-rural gradient
2.2. Pellet collections
In 2019 and 2020, for a related study, we identified 29 black and turkey vulture roosts within the CMA using eBird hotspots, expert reports, and personal observations of vulture movements (Partridge and Gagne, 2023). These 29 sites were active, overnight vulture roosts that usually hosted 20–500 individuals. As many roosts are inaccessible by foot, a total of eight vulture congregation sites were selected for this study (Figure 1). These eight sites were regularly occupied by vultures, accessible on foot, and occurred along an urban-to-rural gradient. In our study area, black and turkey vultures occasionally roost together in roughly equal numbers, but study sites were ~ 95–100% composed of black vultures. This research is likely more indicative of black vulture patterns than those of turkey vultures.
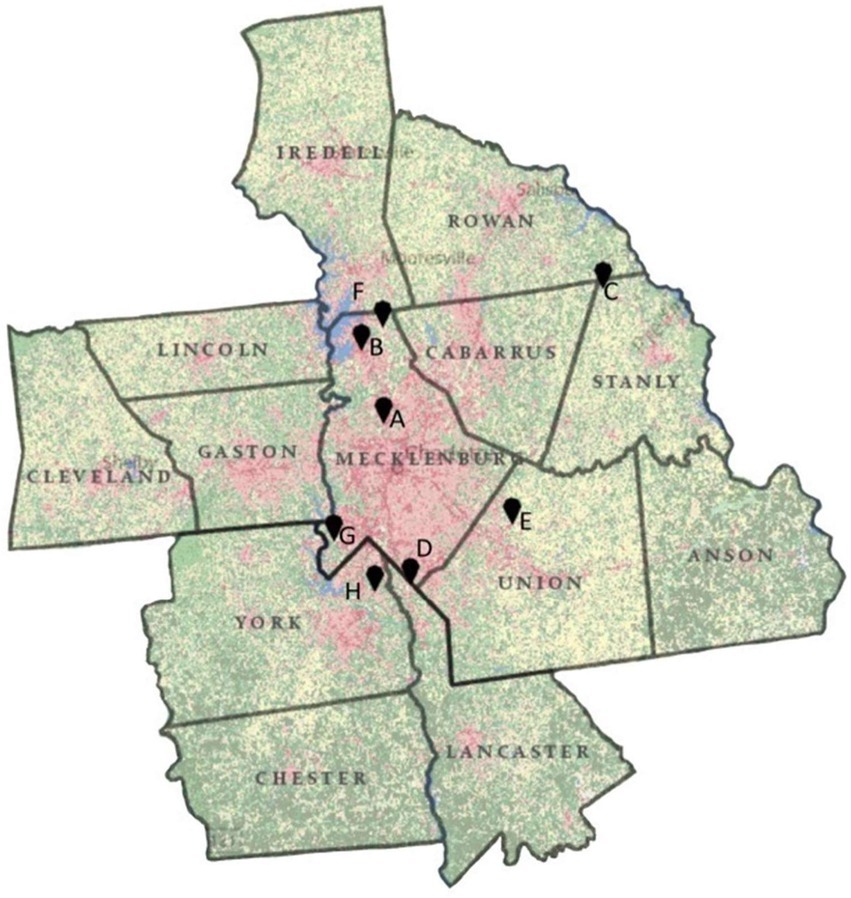
Figure 1. The Charlotte Metropolitan Area, USA with pellet collection sites identified by lettered markers and counties indicated by name. Developed land is shown in shades of red, open water is blue, forest types are in shades of green, and pasture, grassland, and cultivated crops are in shades of yellow (Dewitz and United States Geological Survey, 2021).
We collected structurally intact vulture pellets from the ground at each site, aiming for 10–15 pellets collected/visit/site. Each site was visited 15 times between January 2021 and July 2022, with visits occurring approximately every 2 weeks throughout each season.
2.3. Pellet dissections
Collected pellets were stored in a freezer at -21°C before dissection. We air-dried frozen pellets for 48 h to reduce moisture content (Yahner et al., 1986). Dried pellets were then weighed using a Mettler Toledo PL303 balance and dissected using a Nikon SMZ1000 stereomicroscope and a Parco compound microscope with magnifications of 4-100x. During dissection, we visually identified the natural materials (vegetation, dirt, rocks, and animal remains), plastic materials, and other anthropogenic materials (metal, fabric, paper, wood, and glass) in each pellet and weighed each material type for the proportion of each pellet composed of natural, plastic, or other anthropogenic material.
2.4. Explanatory variables
We measured four explanatory variables that could be important predictors of plastic ingestion by vultures (Campbell, 2014; Novaes and Cintra, 2015): commercial food provider density, livestock and game producer density, amount of developed landcover, and distance to the nearest landfill. Commercial food provider density, livestock and game producer density, and the amount of developed landcover were measured in circular landscapes centered on collection sites with 0.4, 0.5, 1, 2, 3, 4, 5, 10, 15, and 20 km radii, capturing variations in space use by black and turkey vultures (Houston et al., 2011; Holland et al., 2019).
We used Data Axle Reference Solutions databases to identify commercial food providers, livestock and game producers, and landfills (Axle, 2022). Database records were current as of June 6, 2022, when we accessed records. Commercial food providers included 9,105 “Retail Trade” records with the descriptors “Food Stores” and “Eating Places.” Livestock and game producers included 129 “Agriculture, Forestry, and Fishing” records with the descriptors “Agricultural Production - Livestock” or “Fishing, Hunting, and Trapping” and landfills included 29 “Public Administration” records with the descriptors “Air, Water, and Solid Waste Management.” We calculated the density of commercial food providers or livestock and game producers in landscapes as the number of businesses of each type divided by landscape area and measured the distance from each congregation site to the nearest landfill. Finally, we measured the amount of developed landcover in landscapes as the proportion composed of the National Land Cover Database developed classes (i.e., Developed Open Space, Developed Low Intensity, Developed Medium Intensity, and Developed High Intensity; Dewitz and United States Geological Survey, 2021). We used ArcGIS Pro 2.9.2 (ESRI Inc., 2021) and Google Earth Pro 7.3.4.8642 (Google, 2022) to calculate explanatory variables.
2.5. Analysis
We used repeated measures linear mixed models to test for the effects of commercial food provider density, livestock and game producer density, amount of developed landcover, and distance to the nearest landfill on the proportion of pellets composed of plastic materials, with a log transformation to address heteroscedasticity in the data. All models included a random site effect to account for non-independence of observations from the same collection site. We modeled each landscape scale separately to minimize collinearity among explanatory variables. For some scales, commercial food provider density, livestock and game producer density, and/or distance to the nearest landfill were nil because no businesses were located in landscapes. These variables were absent from models when this occurred.
Variance inflation factors (VIF) and pairwise correlation matrices were calculated to test for collinearity in explanatory variables, which we defined as VIF ≥5 or r ≥ 0.7 (Dormann et al., 2013). Due to high levels of collinearity between developed landcover, commercial food provider density, and livestock and game producer density (Supplementary Tables A1–A11), we created a total of 23 models (Table 1; Supplementary Tables A12–A37). With variables separated into these models, all VIF and pairwise correlation values were below specified thresholds.
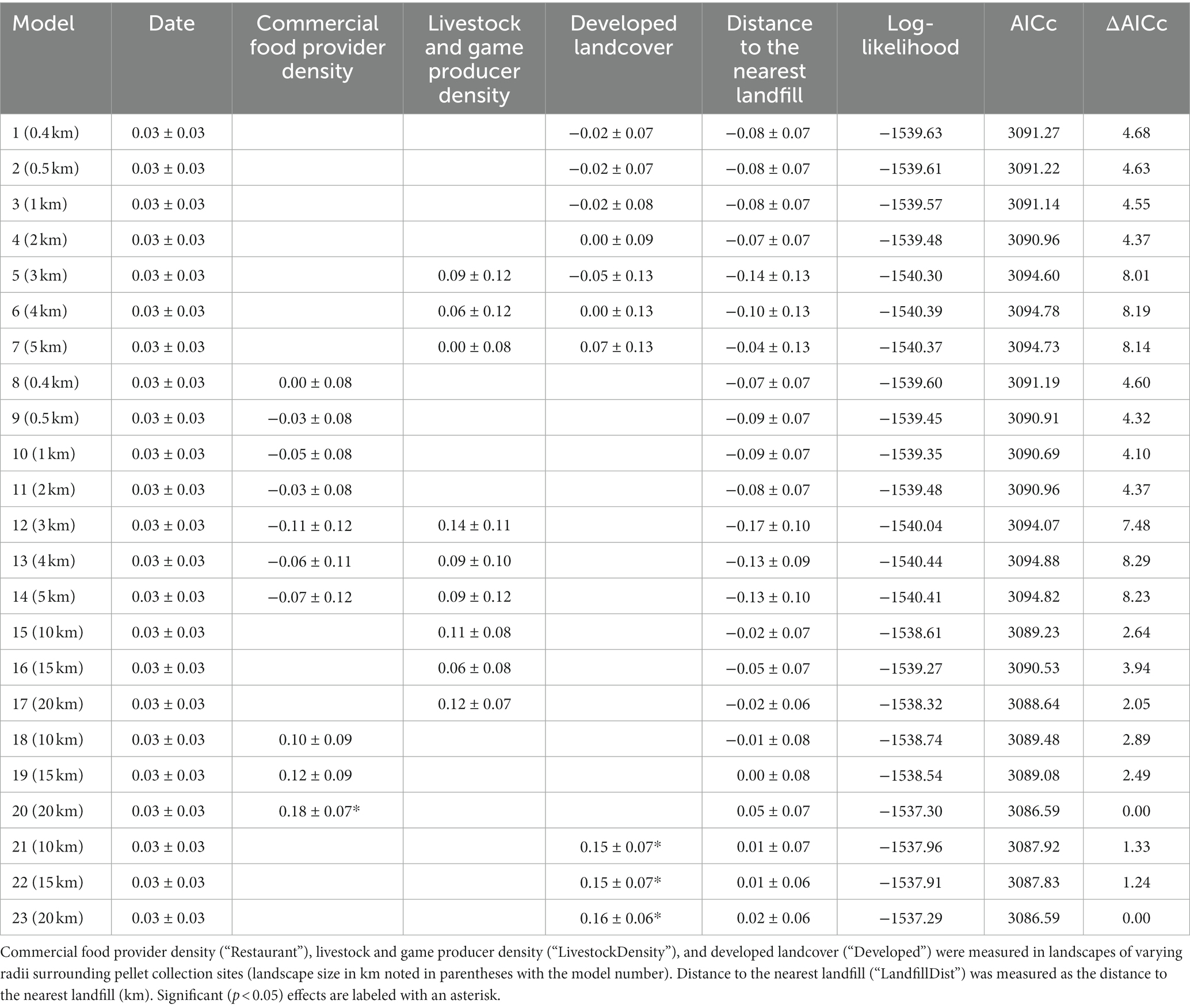
Table 1. Variable estimates (± SE) of models describing the plastic composition of black vulture (Coragyps atratus) and turkey vulture (Cathartes aura) pellets in the Charlotte Metropolitan Area, United States.
We tested for spatial autocorrelation in the residuals of each model using Moran’s I. Significant spatial autocorrelation did not occur in the residuals of any model (Supplementary Figures A1–A23). We inferred the effects of explanatory variables on pellet plastic composition using the model with the largest log-likelihood value. We used RStudio v2021.09.2 + 382 (R Core Team, 2022) and the ncf v1.3–2 (Bjornstad, 2020), regclass v1.6 (Petrie, 2020), spdep v1.2–4 (Bivand, 2022), stats v4.3.0 (R Core Team, 2022), and lme4 v1.1–30 (Bates et al., 2015) packages to carry out analyzes.
Objective 2: Identification of plastics in regurgitated pellets
Eighty-three pellets were randomly selected for plastic analysis with some stratification by site and date. Given time constraints, we sampled three out of four study seasons and eight out of eight study sites, resulting in 6.2 pellets/site analyzed in fall 2021, 6.5 pellets/site analyzed in spring 2022, and one pellet/site analyzed in summer 2022. For the selected pellets, we cleaned each plastic material and performed Fourier transform infrared (FTIR) spectroscopy using a PerkinElmer FT-IR Spectrometer Spectrum Two to produce infrared spectra of each material. The transmittance of plastics was analyzed by wavenumbers 450 cm−1 to 4,000 cm−1 with four scans and a resolution of 8 cm−1. We used Open Specy to match the infrared spectrum of each material to spectra within the Open Specy database, with intensity adjusted for transmittance, baseline correction, and using the full processed plot (Cowger et al., 2021). Database spectra correlated with sample spectra at Pearson’s r values of ≥0.75 were accepted as matches.
3. Results
Objective 1: Plastic content of regurgitated pellets along an urban-to-rural gradient
We dissected 1,087 pellets, 648 (60%) of which contained plastic material, with an average plastic composition by weight of 2.66 ± 8.76% (Supplementary Figure A24). Eleven pellets (1%) were more than 50% plastic material, and five pellets (0.5%) were more than 75% plastic material.
The best model describing the plastic content of vulture pellets included site, date [0.03 ± 0.03 (SE), p = 0.35], distance to the nearest landfill [0.02 ± 0.06 (SE), p > 0.05], and the amount of developed landcover [0.16 ± 0.06 (SE), p = 0.01] in 20 km landscapes. Another model that included site, date, distance to the nearest landfill and commercial food provider density in 20 km landscapes had a very similar log-likelihood value and variable estimates (Table 1; Figure 2). Overall, vulture pellets contained more plastic materials when congregation sites were located closer to landfills or were surrounded by more developed landcover within 20 km. There was minimal variation in pellet plastic composition across sites or dates with no noticeable trends in plastic ingestion over time.
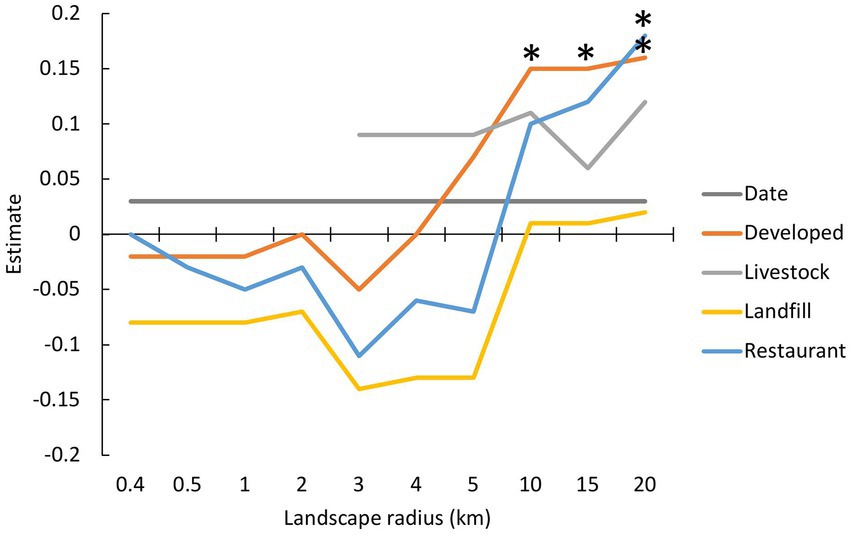
Figure 2. Effect sizes and significance of explanatory variables in models of the plastic content of black vulture (Coragyps atratus) and turkey vulture (Cathartes aura) pellets in the Charlotte Metropolitan Area, USA. Significant (p < 0.05) effects are labeled with an asterisk. Commercial food provider density (“Restaurant”), livestock and game producer density (“Livestock”), and amount of developed landcover (“Developed”) were measured in landscapes of varying radii surrounding pellet collection sites. Distance from pellet collection sites to the nearest landfill is labeled as “Landfill.” For variables that were included in more than one model at the same scale, the estimate from the best performing model was used. All models also included a site variable to account for the non-independence of pellets collected at the same site.
Objective 2: Identification of plastics in regurgitated pellets
We performed FTIR spectroscopy on 187 pieces of plastic, resulting in 171 matches with spectra in the Open Specy database. We report only the matches for each sample with the highest Pearson’s r value (Supplementary Table A23). Some samples were identified as different materials with the same Pearson’s r value and thus a total of 234 identification are presented. Of all matched samples, 54.7% were matched with plastic materials–the remaining 45.3% were matched with various natural or non-plastic materials (Figures 3A,B). The six most common plastic materials identified in pellets were silicone rubber (n = 14), high density polyethylene (n = 13), polyethylene (n = 12), silicate bio polyethylene (n = 10), polyethylene with silicate inorganic (n = 9), and low density polyethylene (n = 7; Figure 3C). There was no apparent variation in plastic identification across sites or dates.
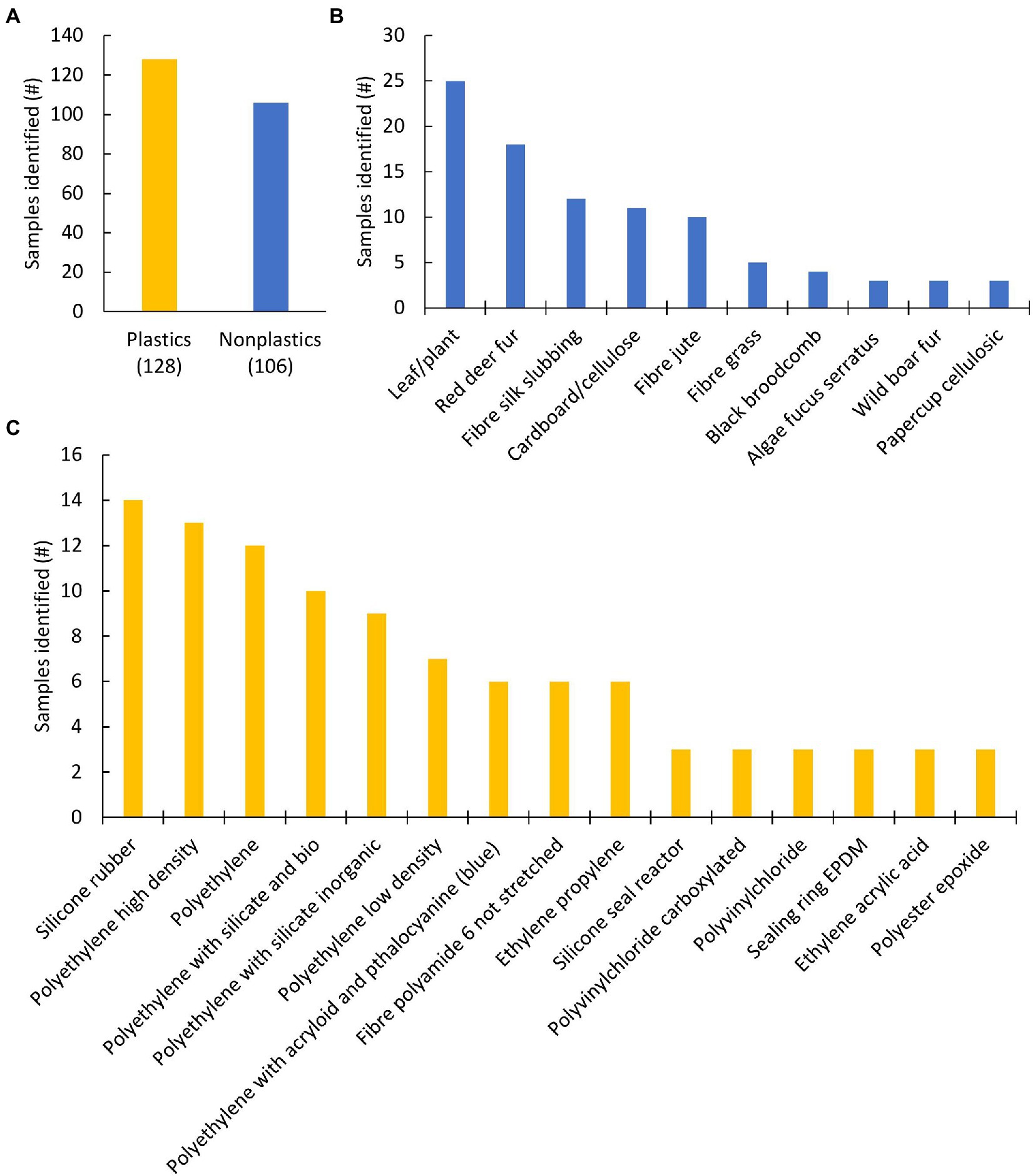
Figure 3. (A) Fourier transform infrared spectroscopy results for 187 pieces of plastic material from 83 black vulture (Coragyps atratus) and turkey vulture (Cathartes aura) pellets collected in the Charlotte Metropolitan Area, USA. The samples identified as different types of plastics or natural or other non-plastic anthropogenic materials coating or embedded in samples with the number of samples in parentheses There are a total of 234 sample identifications as some samples were identified as different materials with the same Pearson’s r value. (B) The number of samples identified as natural or nonplastic materials, identifications with less than three samples are omitted from this figure. (C) The number of samples identified as plastic materials, identifications with less than three samples are omitted from this figure.
4. Discussion
As we predicted, our results indicate that black and turkey vultures are ingesting more plastic materials when congregating in landscapes with more developed landcover and a greater density of commercial food providers. Developed landcover and commercial food provider density had the largest effects on pellet plastic proportion when we measured these variables in the largest landscapes we considered, those with radii of 20 km. Distance to the nearest landfill was also included in the most supported models, although it did not have a significant effect.
The positive effects of developed landcover and commercial food provider density that we report, and the presence of distance to the nearest landfill in the best models of pellet plastic content, are supported by evidence from the literature and our ongoing research in the study area. Black vultures are regularly observed foraging at dumpsters and landfills (Lowney, 1999; Kluever et al., 2020) where they would be more likely to ingest various plastic materials with food items. While loafing, black vultures are known to destroy property such as roofing, insulation, and vehicle/boat seats and seals in urban and rural landscapes (Tillman et al., 2002; Evans, 2013; Kluever et al., 2020). These patterns are also supported by ongoing research featuring interviews of researchers and practitioners working with nuisance black vulture populations worldwide. When not foraging, black vultures will loaf in various landscapes and pick at anthropogenic materials, often near a consistent food source.
The positive effect of developed landcover in our results suggests that plastic materials ingested by vultures in urban landscapes originate from a wide variety of sources, including commercial dumpsters and smaller waste containers, institutional and industrial waste, household waste, roadside waste and roadkill, and built structures themselves. The positive effect of commercial food provider density that we report suggests that, of these potential sources, food stores and restaurants may be particularly important and that foraging is a primary means by which plastic materials are ingested. However, future research is needed to test this hypothesis and to investigate in more detail the roles that other sources and vulture behaviors play in plastic ingestion.
A cosmopolitan nature to plastic ingestion by vultures in urban landscapes is supported by our plastic identification results. The most common plastics in our sample were silicone rubber and polyethylene, each commonly found in the environment due to human pollution and the breakdown of anthropogenic objects. Silicone rubber is used to make automobile tires, seals, baking pans, and food molds (Shit and Shah, 2013) and polyethylene (low-density and high-density) is used to make plastic bags, food containers, and liners for landfills and pools (Kumar et al., 2011).
Nearly half of the samples we analyzed using FTIR were matched with non-plastic materials such as plant material, fur, or fabric. It is likely that these were materials coating or embedded within the plastic samples and thus, may provide insight into what the vulture was eating when the plastic was ingested. Interestingly, the second most commonly matched non-plastic material was red deer (Cervus elaphus) fur, which is a frequently farmed deer species in the United States. While this finding requires additional investigation, it does provide some evidence that vultures are ingesting plastic in areas of livestock and game production where they may be preying on domestic animals. The other common non-plastic materials were silk slubbing fiber, which is used in clothing and bicycle tires (Madhu et al., 2022) and jute fiber, which is used in twines, clothing, and reusable bags (Aly-Hassan, 2015).
We observed the largest effects of developed landcover and commercial food provider density when we measured these variables in landscapes of 20 km radii, the largest we considered. This scale corresponds to the large home ranges of our study species – 30-60 km2 in the southeastern US, or possibly larger, as indicated by a 900 km2 estimate for turkey vultures near the northern limit of their range. The fact that the effect sizes of developed landcover and commercial food provider density were largest at the largest landscape scale we considered suggests that the scale of effect of these explanatory variables is likely to occur at even broader spatial scales, which future studies of vulture plastic ingestion should consider (Jackson and Fahrig, 2015).
5. Conclusion
We found widespread ingestion of plastic by vultures, with 60% of dissected pellets containing plastic materials. Vultures that congregated in landscapes with more developed land cover and a higher density of commercial food providers at broad spatial scales ingested more plastic, particularly silicone rubber and polyethylene. Vultures in urban landscapes may also be ingesting plastic at landfills and in areas of livestock and game production. Future research should seek to estimate the relative importance of sources of plastic in vulture diets, the vulture behaviors associated with plastic ingestion from different sources and of different types, and the physiological consequences of plastic pollution on species health and urban ecosystem functioning.
Data availability statement
The raw data supporting the conclusions of this article is available in the Supplementary material and PANGAEA depository.
Author contributions
HP and SG contributed to the conception and design of the study. HP, SB, JA, and JS conducted the field work. HP performed the statistical analysis. HP, SB, and JA wrote sections of the manuscript. All authors contributed to the manuscript revision, read, and approved the submitted version.
Funding
Grant funding for materials and field travel was provided by the Geological Society of America. Funding for SB and JA to complete this research during May 2022–August 2022 was provided by the Office of Undergraduate Research at the University of North Carolina at Charlotte.
Conflict of interest
The authors declare that the research was conducted in the absence of any commercial or financial relationships that could be construed as a potential conflict of interest.
Publisher’s note
All claims expressed in this article are solely those of the authors and do not necessarily represent those of their affiliated organizations, or those of the publisher, the editors and the reviewers. Any product that may be evaluated in this article, or claim that may be made by its manufacturer, is not guaranteed or endorsed by the publisher.
Supplementary material
The Supplementary material for this article can be found online at: https://www.frontiersin.org/articles/10.3389/fevo.2023.1158453/full#supplementary-material
References
Aly-Hassan, M. S. (2015). “A new perspective in multifunctional composite materials” in Multifunctionality of Polymer Composites (Amsterdam, Netherlands: Elsevier Inc.), 42–67.
Augé, A. A. (2017). Anthropogenic debris in the diet of Turkey vultures (Cathartes aura) in a remote and low-populated South Atlantic island. Polar Biol. 40, 799–805. doi: 10.1007/s00300-016-2004-0
Avery, M., Humphrey, J., Tillman, E., Phares, K. O., and Hatcher, J. E. (2002). Dispersing Vulture Roosts on Communication Towers. USDA Wildlife Services–Staff Publications. Available at: https://digitalcommons.unl.edu/icwdm_usdanwrc/456
Ballejo, F., Plaza, P., Speziale, K. L., Lambertucci, A. P., and Lambertucci, S. A. (2021). Plastic ingestion and dispersion by vultures may produce plastic islands in natural areas. Sci. Total Environ. 755:142421. doi: 10.1016/j.scitotenv.2020.142421
Barnes, D. K. A., Galgani, F., Thompson, R. C., and Barlaz, M. (2009). Accumulation and fragmentation of plastic debris in global environments. Philos. Trans. R. Soc. B Biol. Sci. 364, 1985–1998. doi: 10.1098/rstb.2008.0205
Bates, D., Maechler, M., Bolker, B., and Walker, S. (2015). Fitting linear mixed-effects models using lme4. J. Stat. Softw. 67, 1–48. doi: 10.18637/jss.v067.i01
Bivand, R. (2022). R packages for analyzing spatial data: A comparative case study with areal data. Geogr. Anal. 54, 488–518. doi: 10.1111/gean.12319
Bjornstad, O. N. (2020). Ncf: Spatial Covariance Functions. Available at: https://CRAN.R-project.org/package=ncf
Blackwell, B. F., Avery, M. L., Watts, B. D., and Lowney, M. S. (2007). Demographics of black vultures in North Carolina. J. Wildl. Manag. 71, 1976–1979. doi: 10.2193/2006-146
Borges-Ramírez, M. M., Escalona-Segura, G., Huerta-Lwanga, E., Iñigo-Elias, E., and Osten, J. R. (2021). Organochlorine pesticides, polycyclic aromatic hydrocarbons, metals and metalloids in microplastics found in regurgitated pellets of black vulture from Campeche, Mexico. Sci. Total Environ. 801:149674. doi: 10.1016/j.scitotenv.2021.149674
Buckley, N. J. (1998). Interspecific competition between vultures for preferred roost positions. Wilson Bull. 110, 122–125.
Campbell, M. O. (2014). The impact of urbanization and agricultural development on vultures in El Salvador. Vulture News 66, 16–28. doi: 10.4314/vulnew.v66i1
Carlin, J., Craig, C., Little, S., Donnelly, M., Fox, D., Zhai, L., et al. (2020). Microplastic accumulation in the gastrointestinal tracts in birds of prey in Central Florida, USA. Environ. Pollut. 264:114633. doi: 10.1016/j.envpol.2020.114633
Cowger, W., Steinmetz, Z., Gray, A., Munno, K., Lynch, J., Hapich, H., et al. (2021). Microplastic spectral classification needs an open source community: open Specy to the rescue! Anal. Chem. 93, 7543–7548. doi: 10.1021/acs.analchem.1c00123
Crowley, C. M., Tansley, K. F., and Buckley, N. J. (2022). First breeding record of the black vulture (Coragyps atratus) in Vermont. Northeast. Nat. 29, N1–N5. doi: 10.1656/045.029.0103
Cunha, W. A., Freitas, Í. N., Gomes, L. A. S., De Gonçalves, S. O., Montalvão, M. F., Ahmed, M. A. I., et al. (2022). From carrion-eaters to plastic material plunderers: toxicological impacts of plastic ingestion on black vultures, Coragyps atratus (Cathartiformes: Cathartidae). J. Hazard. Mater. 424:127753. doi: 10.1016/j.jhazmat.2021.127753
de Souza Machado, A. A., Lau, C. W., Till, J., Kloas, W., Lehmann, A., Becker, R., et al. (2018). Impacts of microplastics on the soil biophysical environment. Environ. Sci. Technol. 52, 9656–9665. doi: 10.1021/acs.est.8b02212
Dewitz, J., United States Geological Survey. (2021). National Land Cover Database (NLCD) 2019 Products, Version 2.0.
Dormann, C. F., Elith, J., Bacher, S., Buchmann, C., Carl, G., Carré, G., et al. (2013). Collinearity: a review of methods to deal with it and a simulation study evaluating their performance. Ecography 36, 27–46. doi: 10.1111/j.1600-0587.2012.07348.x
Dris, R., Gasperi, J., Saad, M., Mirande, C., and Tassin, B. (2016). Synthetic fibers in atmospheric fallout: a source of microplastics in the environment? Mar. Pollut. Bull. 104, 290–293. doi: 10.1016/j.marpolbul.2016.01.006
Dris, R., Imhof, H., Sanchez, W., Gasperi, J., Galgani, F., Tassin, B., et al. (2015). Beyond the ocean: contamination of freshwater ecosystems with (micro-)plastic particles. Environ. Chem. 12:539. doi: 10.1071/EN14172
Duis, K., and Coors, A. (2016). Microplastics in the aquatic and terrestrial environment: sources (with a specific focus on personal care products), fate and effects. Environ. Sci. Eur. 28:2. doi: 10.1186/s12302-015-0069-y
Elías, E. E. I. (1987). Feeding habits and ingestion of synthetic products in a black vulture population from Chiapas, Mexico. Acta Zool. Mex. 22, 1–15. doi: 10.21829/azm.1987.19222040
ESRI Inc. (2021). ArcGIS Pro (2.9.2). Available at: https://www.esri.com/en-us/arcgis/products/arcgis-pro/overview
Evans, B. A. (2013). Dynamics of a Problematic Vulture Roost in Southwest Florida and Responses of Vultures to Roost-Dispersal Management Efforts. Florida: Florida Gulf Coast University.
Future, C. (2019). 2040 Comprehensive Plan. Available at: http://ww.charmeck.org/Planning/CompPlan/Charlotte_Growth_Factors.pdf
Google (2022). Google Earth Pro 7.3.4.8642. Available at: https://earth.google.com/web/ (Accessed August 2, 2022).
Hill, J. E., Holland, A. E., Brohl, L. K., Kluever, B. M., Pfeiffer, M. B., DeVault, T. L., et al. (2022). Diets of black vultures and Turkey vultures in coastal South Carolina, USA with a review of species’ dietary information. Southeast. Nat. 21, 11–27. doi: 10.1656/058.021.0102
Holland, A. E., Byrne, M. E., Hepinstall-Cymerman, J., Bryan, A. L., DeVault, T. L., Rhodes, O. E., et al. (2019). Evidence of niche differentiation for two sympatric vulture species in the southeastern United States. Mov. Ecol. 7, 1–12. doi: 10.1186/s40462-019-0179-z
Houston, C. S., McLoughlin, P. D., Mandel, J. T., Bechard, M. J., Stoffel, M. J., Barber, D. R., et al. (2011). Breeding home ranges of migratory Turkey vultures near their northern limit. Wilson J. Ornithol. 123, 472–478. doi: 10.1676/10-090.1
Houston, D. C., Mee, A., and McGrady, M. (2007). Why do condors and vultures eat junk?: the implications for conservation. J. Rap. Res. 41, 235–238. doi: 10.3356/0892-1016(2007)41[235:WDCAVE]2.0.CO;2
Houston, C. S., Terry, B., Blom, M., and Stoffel, M. J. (2007). Turkey vulture nest success in abandoned houses in Saskatchewan. Wilson J. Ornithol. 119, 742–747. doi: 10.1676/06-055.1
Jackson, H. B., and Fahrig, L. (2015). Are ecologists conducting research at the optimal scale? Glob. Ecol. Biogeogr. 24, 52–63. doi: 10.1111/geb.12233
Jamieson, A. J., Brooks, L., Reid, W. D., Piertney, S., Narayanaswamy, B. E., and Linley, T. (2019). Microplastics and synthetic particles ingested by deep-sea amphipods in six of the deepest marine ecosystems on earth. R. Soc. Open Sci. 6:180667. doi: 10.1098/rsos.180667
Kluever, B. M., Pfeiffer, M. B., Barras, S. C., Dunlap, B. G., and Humberg, L. A. (2020). Black vulture conflict and management in the United States: damage trends, management overview, and research needs. Human-wildlife. Interactions 14:8. doi: 10.26077/9875-d38f
Kumar, S., Panda, A. K., and Singh, R. K. (2011). A review on tertiary recycling of high-density polyethylene to fuel. Resour. Conserv. Recycl. 55, 893–910. doi: 10.1016/j.resconrec.2011.05.005
Lowney, M. S. (1999). Damage by black and Turkey vultures in Virginia, 1990-1996. Wildlife Soc. Bull. 27, 715–719.
Madhu, P., Praveenkumara, J., Sanjay, M., Siengchin, S., and Gorbatyuk, S. (Eds.) (2022). “Introduction to bio-based fibers and their composites” in Advances in Bio-Based Fiber (Amsterdam, Netherlands: Elsevier), 1–20.
Napper, I. E., Davies, B. F., Clifford, H., Elvin, S., Koldewey, H. J., Mayewski, P. A., et al. (2020). Reaching new heights in plastic pollution–preliminary findings of microplastics on Mount Everest. One Earth 3, 621–630. doi: 10.1016/j.oneear.2020.10.020
Novaes, W. G., and Cintra, R. (2015). Anthropogenic features influencing occurrence of black vultures (Coragyps atratus) and Turkey vultures (Cathartes aura) in an urban area in central Amazonian Brazil. Condor 117, 650–659. doi: 10.1650/CONDOR-15-56.1
Partridge, H. C., and Gagne, S. A. (2023). Urban Vultures Preferentially Roost at Sites Surrounded by Landscapes with Fewer Edges between Forest and Urban Development and Near Water. Urban Ecosystems.
Petrie, A. (2020). Regclass: Tools for an Introductory Class in Regression and Modeling. Available at: https://CRAN.R-project.org/package=regclass
R Core Team (2022). R: A Language and Environment for Statistical Computing. Vienna, Austria: R Foundation for Statistical Computing. Available at: https://www.R-project.org/
Seamans, T. (2004). Response of Roosting Turkey Vultures to a Vulture Effigy. USDA Wildlife Services–Staff Publications. Available at: https://digitalcommons.unl.edu/icwdm_usdanwrc/385
Shit, S. C., and Shah, P. (2013). A review on silicone rubber. Nat. Acad. Sci. Lett. 36, 355–365. doi: 10.1007/s40009-013-0150-2
Tillman, E. A., Humphrey, J. S., and Avery, M. L. (2002). Use of Vulture Carcasses and Effigies to Reduce Vulture Damage to Property and Agriculture. Proceedings of the Vertebrate Pest Conference, No. 20.
US Census Bureau. (2019). ACS Demographic and Housing Estimates. Available at: https://data.census.gov/cedsci/table?q=population&g=330XX00US172&tid=ACSDP1Y2019.DP05&hidePreview=true
Wilcox, C., Van Sebille, E., and Hardesty, B. D. (2015). Threat of plastic pollution to seabirds is global, pervasive, and increasing. Proc. Natl. Acad. Sci. 112, 11899–11904. doi: 10.1073/pnas.1502108112
Keywords: black vulture, turkey vulture, plastic ingestion, urbanization, landscape, spectroscopy
Citation: Partridge HC, Barnett S, Amodeo J, Snyder J and Gagné SA (2023) Vultures in the southeastern United States ingest more plastic in landscapes with more developed landcover. Front. Ecol. Evol. 11:1158453. doi: 10.3389/fevo.2023.1158453
Edited by:
Franco Leandro de Souza, Federal University of Mato Grosso do Sul, BrazilReviewed by:
Augusto João Piratelli, Federal University of São Carlos, BrazilYang Wang, Hebei Normal University, China
Copyright © 2023 Partridge, Barnett, Amodeo, Snyder and Gagné. This is an open-access article distributed under the terms of the Creative Commons Attribution License (CC BY). The use, distribution or reproduction in other forums is permitted, provided the original author(s) and the copyright owner(s) are credited and that the original publication in this journal is cited, in accordance with accepted academic practice. No use, distribution or reproduction is permitted which does not comply with these terms.
*Correspondence: Hannah C. Partridge, aHBhcnRyaWRAdW5jYy5lZHU=
†ORCID: Hannah C. Partridge http://orcid.org/0000-0002-0025-4452