- 1Department of Biological Sciences, University of Alberta, Edmonton, AB, Canada
- 2Department of Applied Zoology and Wildlife Management, Technical University in Zvolen, Zvolen, Slovakia
Chronic wasting disease (CWD) is a fatal, prion disease of cervids that was first detected in Alberta in 2005. Transmission of CWD by direct contact with infected individuals plays a major role in the early phases of an outbreak. Mule deer (Odocoileus hemionus) comprise 85% of CWD-infected animals in the province, and we investigated the seasonal effects of grouping patterns and landscape heterogeneity on direct, pair-wise contacts (distance of 3 m) within and between sex-specific (same or mixed sex) groups of mule deer in east-central Alberta. We determined seasonal contacts of mule deer based on proximity loggers that alter GPS schedules to record contact locations. We modeled the relative risk of contact between sex-specific dyads both within and between social groups based on landscape characteristics at the location of contact. We then assessed the support for 5 hypotheses that linked locations of seasonal contacts to occurrence of CWD on the landscape. Disease occurrence on the landscape was derived by comparing locations of CWD-infected and CWD-uninfected deer collected as part of the Alberta’s CWD hunter-harvest surveillance program. We found that contacts in winter occurred in areas where deer use was concentrated, whereas in summer, contact locations were less constrained in space where patterns of landscape characteristics at contact locations varied between sex-specific dyads. Contact probabilities of within and between-group male dyads in winter and between-group female dyads in summer were the best predictors of CWD risk in east-central Alberta. Our results relate habitat specific, social behaviors between conspecific mule deer to potential routes of CWD transmission and contribute to CWD research that guides management strategies for an emergent wildlife disease.
1. Introduction
Identifying routes of disease transmission among hosts is one of the primary challenges associated with managing and controlling wildlife diseases (Lloyd-Smith et al., 2005). Patterns in transmission dynamics are disease-specific and are largely dependent on the ecology of host-pathogen systems. When diseases are transmitted by direct contact between infected and susceptible individuals, social interactions become the basis for disease spread. Spatial patterns in host prevalence across heterogeneous landscapes suggest that environmental factors influencing host density, space use, and sociality dictate the locations of infectious contacts (Conner and Miller, 2004; Ostfeld et al., 2005; Paull et al., 2012). For example, habitat quality and configuration can alter disease transmission by increasing local host density (Joly et al., 2006; Habib et al., 2011; Ehrmann et al., 2018) while connectivity between suitable habitats can affect the spread and persistence of disease between infected and susceptible subpopulations (Page et al., 2001; Nobert et al., 2016; Miller et al., 2020). Further, human land use affects patterns of wildlife disease by artificially aggregating hosts and by altering host movements and space use (Becker et al., 2018; Fountain-Jones et al., 2021; Janousek et al., 2021). Understanding what landscape features influence direct contacts among conspecifics and how this differs among segments of the population may help explain patterns of disease prevalence on the landscape to help focus surveillance and management of wildlife diseases.
Chronic wasting disease (CWD) is a fatal, prion encephalopathy in free-ranging cervid populations that has been spreading across landscapes in North America and Scandinavia (Mysterud and Edmunds, 2019; Ågren et al., 2021). Prions, the infectious agents of CWD, are shed in bodily fluids of hosts and can also persist in the environment for many years, which allows the disease to spread through direct contact and via contaminated environments (Miller et al., 2000, 2004). All cervids are susceptible to CWD, but there is a higher prevalence in adult age classes and in males for both white-tailed deer (Odocoileus virginianus) and mule deer (O. hemionus; Conner and Miller, 2004; Heisey et al., 2010). There is evidence that the demographic patterns of CWD prevalence reflect behavioral differences between host classes, which suggests that early dynamics of CWD transmission are driven by direct rather than environmental contacts (Grear et al., 2010; Ketz et al., 2019). Spatial patterns in CWD are heterogeneous and likely reflect social dynamics and attraction to habitats. In the agriculturally dominated landscapes of Wisconsin and Illinois, high proportions of deciduous forest and edge density that represent high-quality deer habitat were associated with increased prevalence of CWD in white-tailed deer (Joly et al., 2006; Storm et al., 2013). In the Northeastern United States, where forested landscapes were homogenous, the risk of harvesting a deer infected with CWD was greatest in areas with relatively small amounts of forest cover resulting from greater dispersal distances by deer in low cover areas (Evans et al., 2016). In Canadian prairie-dominated landscapes, risk of a deer being CWD infected increased near agricultural areas that were far from streams and comprised isolated patches of woody cover due to this relatively high-quality habitat facilitating aggregations of deer and increasing infection risk (Rees et al., 2012; Smolko et al., 2021). Further, Nobert et al. (2016) also found that areas facilitating deer movement between patches had a higher probability of being CWD-infected, suggesting habitat connectivity is a key driver of patterns of CWD on the landscape.
In this paper, we build on previous research that documents spatial patterns of CWD and demonstrates linkages between landscape features and disease risk in mule deer in the prairie-parklands of Alberta, Canada (Smolko et al., 2021). We hypothesized that spatial patterns of CWD prevalence were associated with areas that had high probabilities of direct contact between mule deer during the initial 14 years of disease progression. We proposed five non-mutually exclusive hypotheses predicting which type of contact would have the strongest association with risk of a deer harvested from a specific location being CWD infected (Table 1). To assess hypotheses, we first defined seasonal contact locations for female–female, male–male, and mixed-sex deer dyads belonging to the same (within) or different (between) social groups. Second, we measured which landscape features were associated with a high relative contact probability (RCP) value for each contact type. Third, we assessed the relationship between seasonal, group, and sex-specific RCP with patterns of CWD risk on the landscape to evaluate the relative support for each hypothesis.
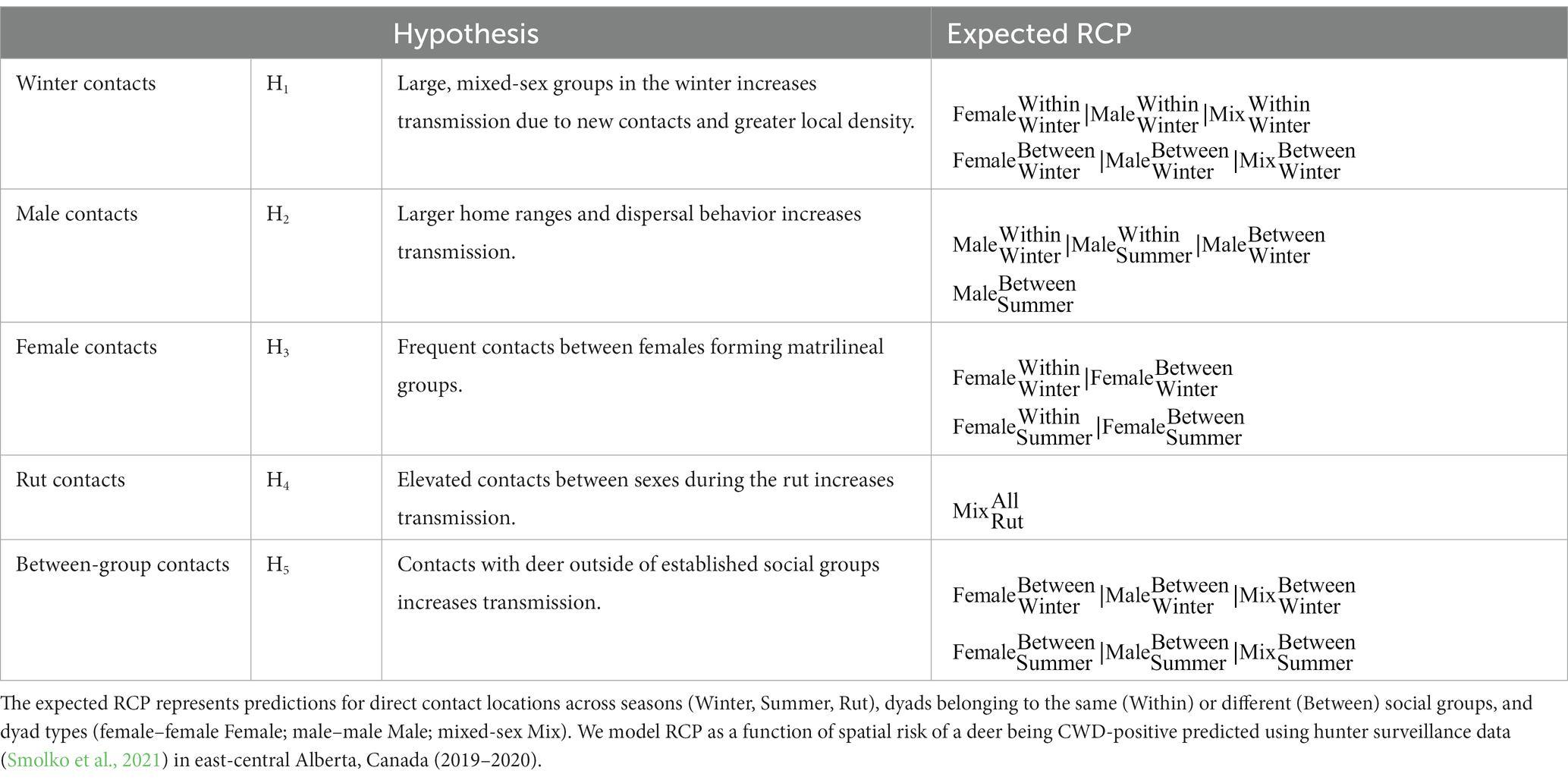
Table 1. Five non-mutually exclusive hypotheses outlining the mechanisms in which direct contact between mule deer influence transmission of chronic wasting disease and the relative contact probabilities (RCP) associated with each hypothesis.
Specifically, we hypothesized that winter contact locations (H1) would be most associated with where the disease was found because, in winter, higher overlap between mule deer home ranges and increased group sizes relative to other seasons due to the snow restricting available habitat has been documented (Wood et al., 1989; Lingle, 2003). In contrast, we hypothesized locations of contacts with males across seasons would have the greatest association with the risk of deer being CWD infected (H2) because male deer have the highest prevalence and exhibit greater home range size and longer dispersal distances (Robinette, 1966; Walter et al., 2018; Smolko et al., 2021). Alternatively, we hypothesized that locations where the probability of a female contact was high (H3) would be most closely associated with areas of high CWD risk because of the higher social interactions among females, especially within groups (Schauber et al., 2007; Grear et al., 2010). Because males have a high prevalence along with elevated mixed-sex contacts due to polygynous mating structure (Bowyer and Kie, 2004; Mejía-Salazar et al., 2017), we also hypothesized a close association between locations of rut contacts and where the risk of the disease is high (H4). Finally, we hypothesized that locations of a contact made by a deer with an individual from a different group (i.e., between-group contact locations) regardless of sex would increase CWD exposure and would be associated with where the risk of CWD was highest (H5). We also assessed a global model because we expected all these mechanisms could contribute to disease distribution and a null model (Hnull) because direct contacts may be too variable or other factors may dominant disease distribution. We used the magnitude and direction of the coefficients to explain the importance of the mechanisms to spatial patterns in CWD risk.
2. Materials and methods
2.1. Study area
The study area (1,440 km2) was in the prairie-parklands of east-central Alberta, approximately 4 km southeast of Edgerton, AB (Figure 1). It includes rolling hills (546 to 782 m) with a landscape of a matrix of agricultural fields, pastures, and native grasslands interspersed with woody cover. Land cover in the study area was dominated by agriculture cropland (48%), followed by grassland (19%), deciduous cover (20%), human development (1%), wetland (7%), water (3%), and exposed land (1%). Croplands were commonly planted with annual crops such as canola (Brassica spp.), wheat (Triticum spp.) and alfalfa (Medicago spp.), or perennial crops and tame grasses for pasture. Native grasslands are made up of drought-tolerant forbs and grasses including (Stipa spp., Bouteloua spp., Calamovilfa spp., and Artemisia spp). We defined woody cover to include deciduous tree stands (Populus spp.) and tall shrubland (Elaeagnus commutata, Salix spp., Prunus spp., and Amelancier alnifolia).
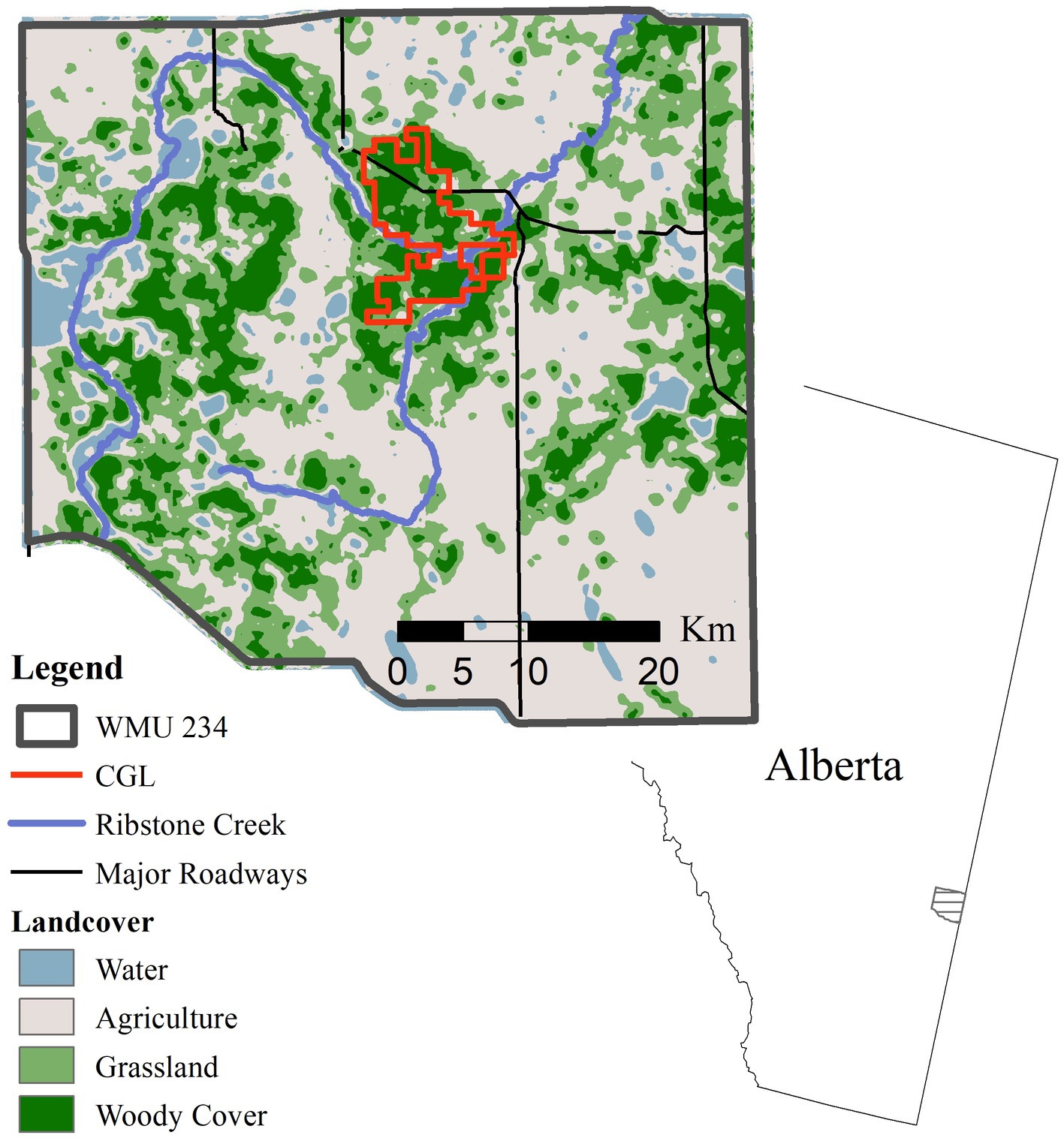
Figure 1. Study area located in east-central Alberta, Canada within wildlife management unit (WMU) 234. Telemetry data recorded from collared mule deer (2019–2020) and focused around the Cresthill Grazing Lease (CGL).
Human development includes paved and gravel roads and clearings for oil and gas, development of seismic lines, pipelines, access roads, OHV and well-sites. Included is a Heritage Rangeland Natural Area (76 km2) that is grazed by cattle 1 June – 31 October. Mule deer, white- tailed and moose are subject to hunting from 1 November to 30 November, and elk from 1 November to 20 January. The area is within the CWD Zone where hunters have been required to submit the heads harvested from deer for CWD testing following the first recorded case in free-ranging deer in 2005. Mule deer density in 2021 was 2.15 deer/km2 (Government of Alberta 2021). Previous research in the study area reported that 23% of mule deer migrated >2 km were a majority of distances between winter and summer ranges were 5–10 km apart (Merrill et al., unpublished data). The coyote (Canis latrans) is the primary predator of deer within the study area, with possible, but rare, predation by black bears (Ursus americanus) and cougars (Puma concolor).
2.2. Deer capture, collaring, and monitoring
We used movement data of collared mule deer collected in 2019–2020. Deer were captured by helicopter using a net gun in January each year. Deer were sedated using xylazine or butorphanol-azaperone-medetomidine (BAM) upon capture and equipped with Lotek Litetrack 420 collars (Lotek Wireless Inc., Newmarket, ON) with global positioning unit (GPS) and proximity logger (PL) devices were programmed to transmit VHF signals at 20 bpm, allowing PL to record the presence of another collar every 3 s. The received signal strength indicator (RSSI) threshold was set to −100 dBm, which corresponds to distance between devices and that we determined to equate to approximately 3 meters. GPS locations were scheduled to record locations at 2-h intervals or, if the PL begins to record a contact event, the schedule changed and collars began recording GPS locations at 15-min intervals on the hour (i.e., 0, 15, 30, and 45 min; Figure 2). We determined mean GPS error to be 6.2 ± 1.0 m throughout our study area (Dobbin, 2022). The protocol for deer collaring in this study was reviewed and received research ethics approval from the University of Alberta Research Ethics Board (AUP00001369).
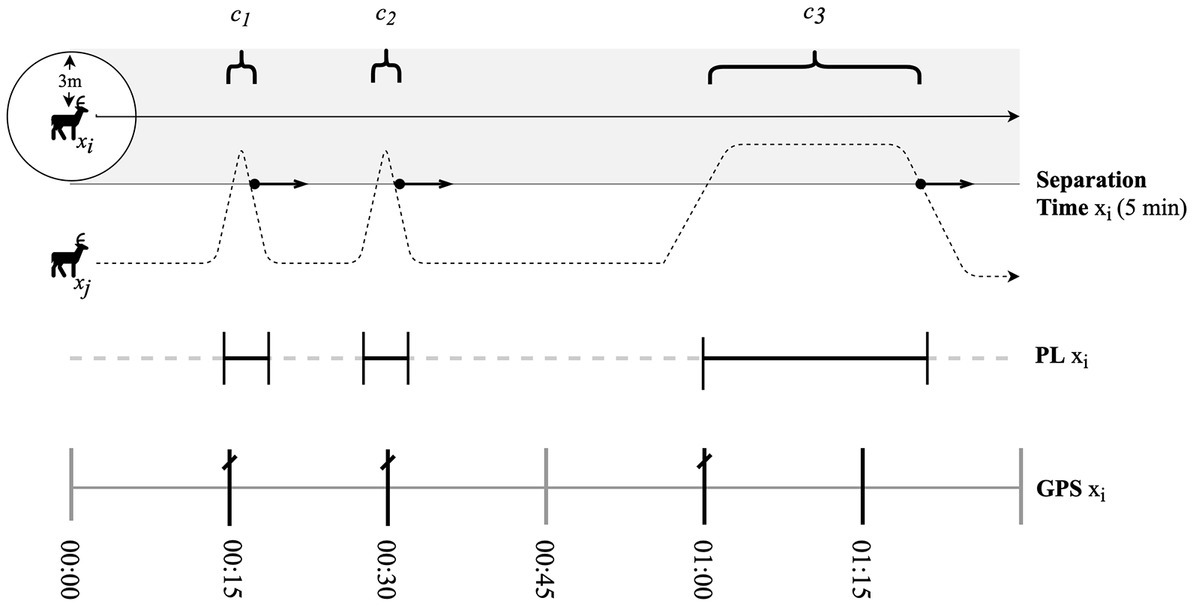
Figure 2. Diagram of collar functioning in relation to separation time, defined as the time elapsed that allows a proximity event to be recorded as a new contact. Dotted line leading from deer xj represents its path as it overlaps with deer xi. The shaded grey area represents the threshold at which collars will begin recording contact events (cn). Bolded arrow represents separation time. Dashed line (PL xi) denotes times recorded by PL depending on whether a contact has been detected. The demarcated line (GPS xi) denotes the altered 15-min GPS schedule where bolded sections are fixes recorded depending on whether a contact has been detected. Crossed lines represent points used in the relative contact probability analysis. If separation time is surpassed, the end time of contact event will be recorded at the time collared exited the contact threshold (3 m).
2.3. Seasonal within and between group contacts
We defined three biological seasons that were based on changes in pairwise, nearest neighbor distance of collared mule deer, to reflect dynamics in social grouping and the potential for distinct seasonal contact rates. The start date of a season corresponding to the maximum changed in the mean daily pairwise distance between individuals in a dyad for each sex-specific dyad type (female–female, male–male and mixed-sex) modeled as a function of Julian day (for more details see Dobbin, 2022). We delineated summer (10 May – 12 November), winter (16 December – 9 May), and rut (13 November – 15 December). We defined a contact event as a time period during which two deer were within 3 m of each other and not separated for more than 5 min. To avoid unequal representation of locations from long contact events we used only the first location recorded once the PL began recording a contact event (Figure 2). Each dyad was categorized as either within-group or between-group at the time of contact by first quantifying the seasonal space use overlap and then the cohesion in the movement paths of all deer pairs. In every season, we determined a threshold to delineate within and between-group dyads based on both pairwise metrics (see Dobbin, 2022 for more details).
2.4. Spatial modeling of RCP
We compared resource characteristics at known contact locations to available resources within areas of shared space use to assess the RCP between two mule deer at a location within the study area. This design is comparable to resource selection modeling (Manly et al., 2002; Lele et al., 2013) whereby contact locations of dyads represent “used” locations and random locations within the area of shared space use represent “available” locations. We defined overlap areas by calculating the seasonal 95% kernel UDs using GPS locations taken at 2-h intervals from the individual deer that comprised each dyad and created a polygon surrounding the areas in which the two distributions overlapped using ArcMap software (ESRI, 2008). To model RCP, we measured landscape characteristics at known contact locations (1) and at random locations (0), where the total number of random locations that were generated within the dyad-specific overlap areas were sampled at a 1:15 ratio in each season, group, and dyad type. Next, we used a logistic regression to obtain the parameters of an exponential model to predict the RCP including dyad ID as a random effect (Gillies et al., 2006) using the TMB package in the program R (Kristensen et al., 2016; R Core Team, 2021). For each category (season, group, and dyad type) we developed a series of candidate models with varying combinations of landscape covariates that best predicted the relative probability of contact in a given location. To evaluate top models, we used model selection based on Akaike’s Information Criterion (AIC) using a threshold ∆AIC > 2 and parsimony to identify the best-supported model (Burnham and Anderson, 2002).
Landscape covariates included in the models were percent woody cover, percent agricultural cover, open-woody cover edge density, distance to roads, distance to wells, distance to streams and terrain ruggedness (Table 2). Because deer use of an area has previously been associated with intermediate levels of woody cover (Serrouya and D’Eon, 2008; Morano et al., 2019), we included both woody cover and square-transformed woody cover. In an initial univariate analysis, we first assessed the relationship between contact probability and mean values for edge density, woody cover, and agricultural cover in 3 buffer sizes (250 m, 500 m, and 1 km) and used the most supported seasonal buffer size thereafter (Table 2; Supplementary Table S1). We used ArcMap software to measure the means of landscape covariates within variable buffer sizes, calculate terrain ruggedness indices, and to calculate Euclidean distance layers (ESRI, 2008). Prior to modeling, we tested for collinearity among variables (r > |0.6|) and did not enter correlated variables into the same model.
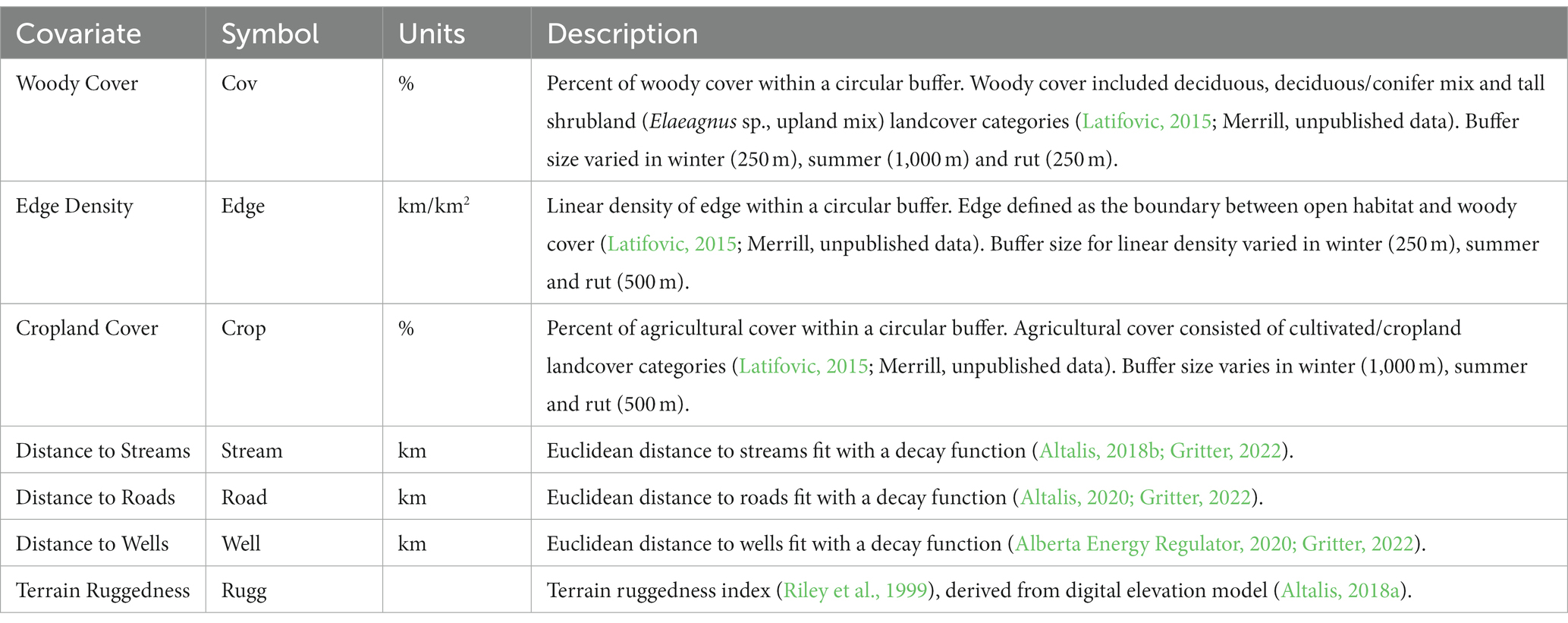
Table 2. Description of landscape covariates used in spatial modeling of relative contact probability of collared mule deer in 2019–2020 in east-central Alberta, Canada.
2.4.1. Assessing RCP predictions
To evaluate the model, we predicted the RCP for each 100-m2 cell across the study area and scaled the predicted RCP values at each location across seasons, groups, and dyad types by dividing by the maximum value. We compared the predicted value at all known contact locations used for each RCP model (n = 114–23,451) and at an equal number of randomly generated locations. We compared the mean values using a t-test assuming equal variance at α = 0.05.
2.4.2. Comparing contact locations with deer use
We also evaluated whether landscape features at deer contact locations differed from those used by deer to determine whether contacts were merely a reflection of deer use. To quantify deer use, we measured landscape covariates at all 2-h GPS locations collared deer that were not contact locations. We included only locations from individuals that comprised a dyad and occurred within the areas of overlap for that same dyad. For each season and group we used Kolmogorov–Smirnov (KS) tests to assess the differences between sex-specific empirical cumulative distribution functions (ECDF) of landscape covariates measured at contact and use locations in the program R (R Core Team, 2021). In the case of mixed-sex contact locations, we compared them with distributions from both male and female use locations.
2.5. Relating RCP to CWD risk
We evaluated the support for the five hypotheses relating types of RCP to the probability of disease occurrence at a location (hereafter, CWD risk) in two steps. First, because the predicted RCP values for a location were seasonally correlated (Supplementary Figure S1), we used univariate analysis to model CWD risk as a function of all the related seasonal, dyad-specific RCP for each hypothesis (Table 1) and retained the covariate from the best supported model. In step two, we used the representative RCP covariates determined in step one to create a set of candidate models and used Bayesian Information Criterion (BIC) model selection to compare the support between the candidate models and a null model (no variables) based on ∆BIC > 2 (Burnham and Anderson, 2002). For steps 1 and 2, we used generalized linear models (GLMs) using the R package lme4 to relate mean values of CWD risk with standardized RCP at 5000 randomly generated locations across the study area (Bates et al., 2015). We standardized predictions by subtracting the mean from each value then dividing by the standard deviation of all RCP values. We measured mean risk and RCP values within a 5-km2 circular buffer to represent the minimum mean home range size for mule deer in our study area across seasons and sexes. We used Bayesian Information Criterion (BIC) model selection instead of AIC in previous modeling due to the large sample size (n = 5,000) and used Pearson correlation to relate spatial predictions from the final global model with sex-weighted predictions of CWD risk across the 5,000 randomly generated locations in the study area.
CWD risk was derived in Smolko et al. (2021), where disease risk was defined as the probability of a hunter-harvested deer being CWD positive (1) or negative (0) using data collected during the Alberta surveillance program (2005–2019). Spatial risk of CWD was modeled as a function of characteristics of the deer (sex, species), time since first detection of CWD in an area, Euclidean distance to nearest positive case in the previous year and environmental characteristics of the location of where a hunter removed the deer using rare-event logistic regression. Environmental variables included terrain ruggedness, soil type, distance to rivers, streams, wells, and urban development (see Smolko et al., 2021 for details). Because the predicted CWD risk values from the disease occurrence models were year and sex-specific, we compared RCP values to a values predicted in 2019 (when contact data were collected) weighted by the male (30%) and female (70%) predictions to reflect assumed sex-ratio in our study area (Freeman et al., 2014). We compared the seasonal and dyad-specific RCP values to the weighted CWD risk values at 5,000 random locations across the study area.
3. Results
3.1. RCP models
We used movement data from 68 deer (n = 19 males, 49 females) which resulted in contacts from 56,674 total contacts from 244 deer dyads across seasons (Table 3). In general, we found more contacts (x̅ ± SD) occurred among female dyads within the same social group in summer (1,818 ± 1,734) then in winter (533 ± 810). Meanwhile, the fewest occurred among mixed-sex dyads in different social groups (between-group) in winter (9.0 ± 35) then in summer (11 ± 32). In summer, we did not record any contacts among mixed-sex dyads belonging to the same social group. Further, we did not delineate deer groups during the rut and assumed that all mixed-sex contacts were the result of mating behaviors between males and females during the breeding season. Because there was a limited number of same-sex dyads monitored consistently during the rut (male = 0, female = 4), we were unable to produce RCP models.
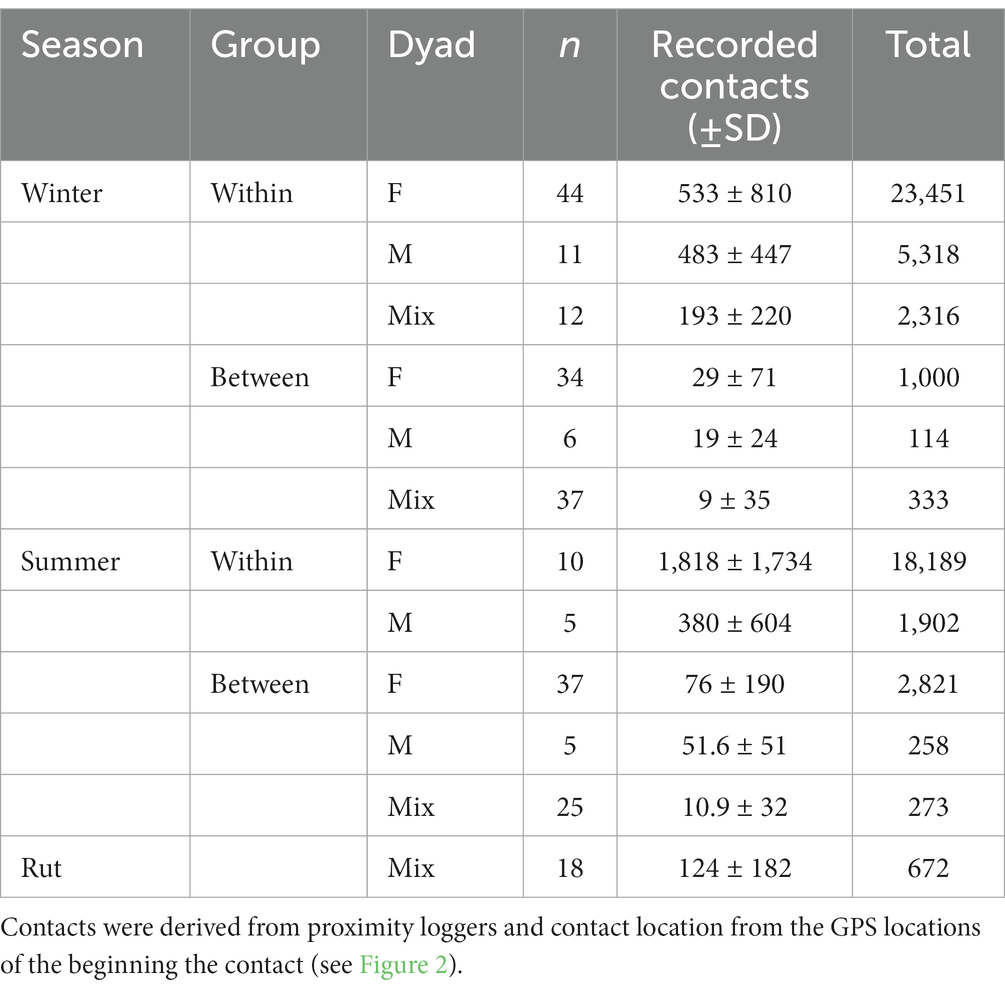
Table 3. Number of contact locations among 68 (n = 19 males, 49 females) mule deer collared in central easter Alberta (2019–2020) summarized by season, group type and dyad type (F, female–female; M, male–male; Mix, mixed sex).
In modeling the RCP in a location, the top competitive models for each season, group and sex-specific dyad had AIC weights >0.5 with one exception, winter within-group male (w = 0.49). There was more similarity in the factors associated with contact locations among sex-specific dyad types in winter than in summer, particularly for female and mixed-sex dyads (Table 4; Supplementary Table S2). In winter, rugged terrain was the most consistent factor influencing the probability of contact across all combinations of deer, with a higher probability of contact in rugged areas. Factors influencing female and mixed-sex dyads in winter also were consistent in that a contact was more likely to occur in areas low in croplands and far from streams. Generally, contacts were less likely to occur far from roads in winter except for those among between-group, mixed-sex dyads. Woody cover influenced RCP differently among group and dyad types in winter, with the RCP of female dyads both within and between-group types showing the highest RCP at intermediate levels of cover, whereas RCP of males both within and between groups decreased with edge density.
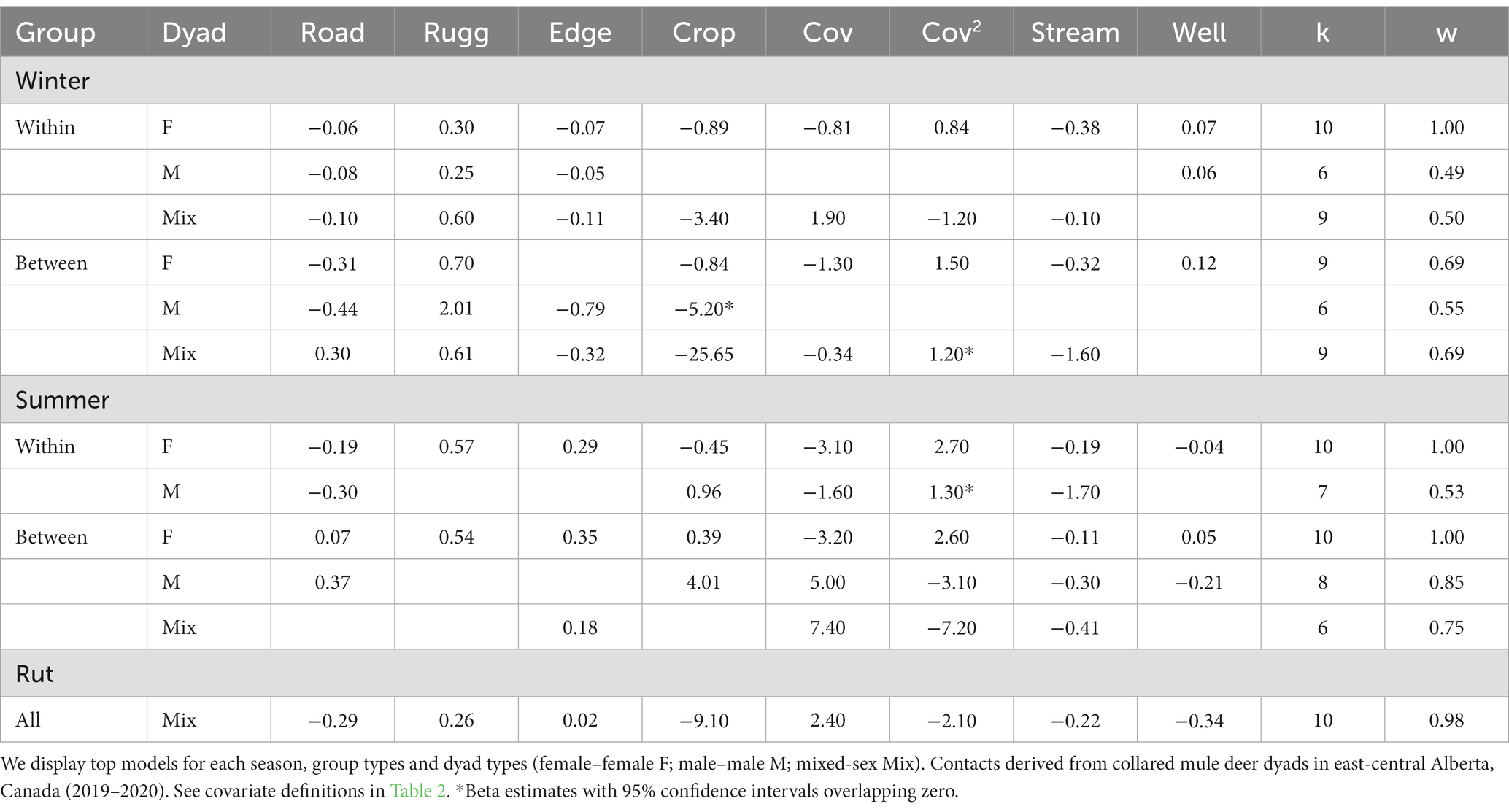
Table 4. Model coefficients from the logistic regressions used to derive the exponential function when relating contact locations compared to randomly generate points within areas of space use overlap based on covariates, number of model parameters (k), and model weights calculated from AIC model selection (w).
In summer, the greatest similarities in the types of landscape features influencing probability of a contact were between same-sex dyads. Female dyads had a greater probability of contacting each other in rugged areas with high densities of edge habitat and in high-cover areas. The contact probabilities for female dyads differed among group types in areas near roads, well pads and agricultural areas whereby within group dyads had a greater probability of contact far from roads, well pads in areas low in croplands. Meanwhile, between-group female dyads showed the opposite pattern and contacted each other when nearby roads and well sites and had more contacts in areas with high cropland cover. There were fewer similarities among male dyad types in summer. Within-group males in summer had higher RCP values when dyads were far from roads and in low woody cover areas while between-group males contacted each other nearby roads in areas of intermediate woody cover. Like between-group males, mixed-sex dyads in summer had higher contact probabilities in areas of intermediate woody cover but differed by having higher RCP values when edge was high (Table 4).
3.1.1. Assessing RCP predictions
In evaluating the RCP predictions, for 8 of the 11 RCP models, we found mean values predicted by the RCP model were higher (p < 0.05) at known contact locations than at random points (Table 5). Two exceptions were when RCP values were greater at random points than at known contact locations for the models predicting winter, within-group male contacts (p < 0.001, tstat = 26.1), and summer between-group female contacts (p < 0.001, tstat = 11.6). Further, RCP values at known contact locations were only marginally higher than random points (p = 0.07, tstat = 0.77) in the models predicting contact probabilities among between-group male dyads in summer.
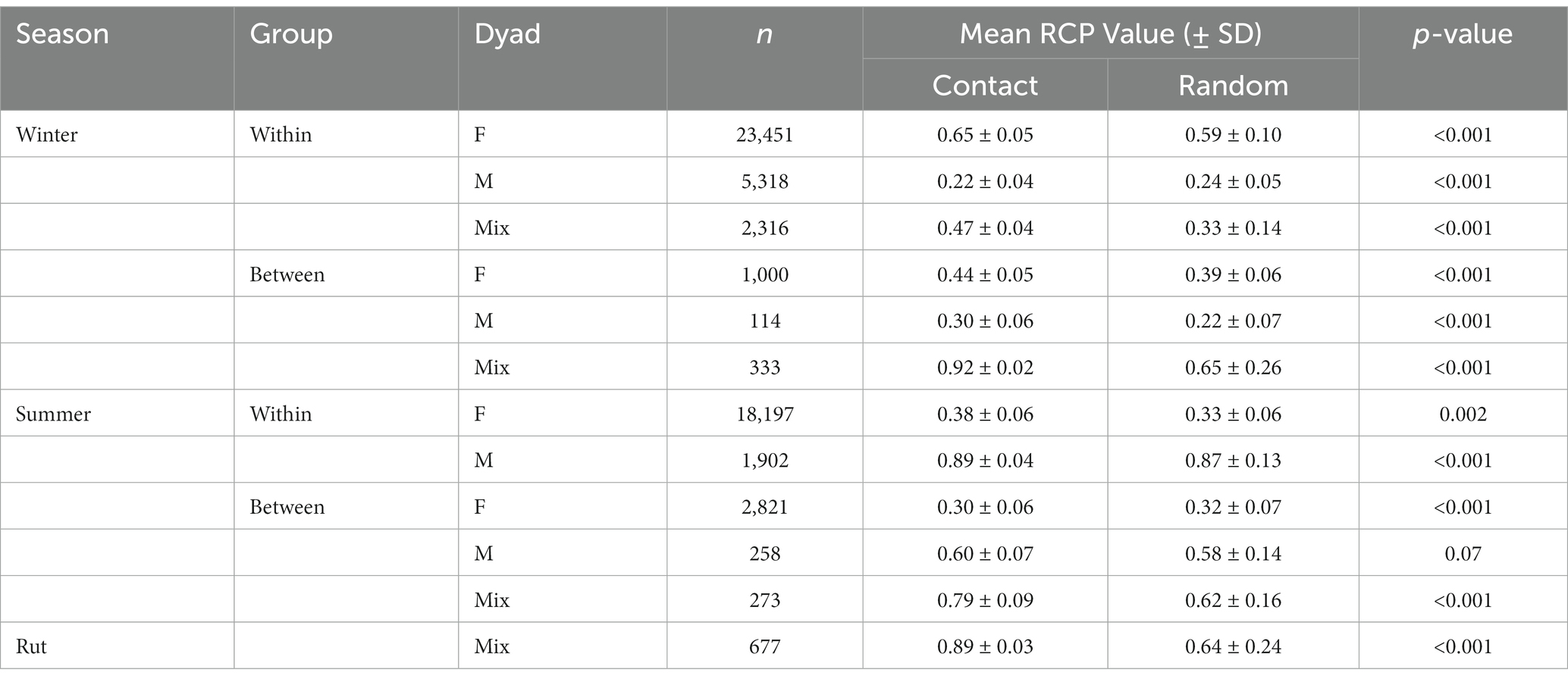
Table 5. Evaluation of relative contact probability models (RCP) using a t-test to compare differences in the mean, scaled (between 0 and 1) RCP values at known contact locations (n) for each season, group type, and dyad type (female–female F; male–male M; mixed-sex Mix) to those at randomly generated locations (n) in east-central Alberta, Canada (2019–2020).
3.1.2. Comparing contact locations with deer use
We used 870 ± 216 (x̅ ± SD) male and 864 ± 227 female locations in winter, 10,151 ± 443 male and 1,165 ± 456 female locations in summer, and 107 ± 58 male and 134 ± 63 female locations during the rut to compare the distribution of locations used by deer to locations where deer contacts occurred along landscape gradients. We found contact distributions significantly differed from used distributions along the same landscape gradients in 94.3% of the 105 comparisons. The exceptions were for deer in summer, where we found no differences in the distributions of percent cropland cover among locations of mixed sex contacts and male (D = 0.06, p = 0.26) and female use (D = 0.05, p = 56) and in the distributions of ruggedness values among male (D = 0.07, p = 0.19) and mixed-sex dyads (D = 0.08, p = 0.08) with male and female use, respectively. In the rut, where we only recorded mixed-sex contacts, there were no differences in contacts locations and the distributions of edge density values and female use (D = 0.05, p = 0.16) or in ruggedness values and male use (D = 0.05, p = 0.34).
We visually compared frequency distributions of the ECDF that were determined to be significantly different by the KS test and we found the distributions of landscape covariate values were more similar among contact locations and known within-group contact locations except that contacts among within-group dyads in summer were more likely to occur in lower cover areas relative to use (Figure 3). Similarly, between-group contact locations we comparable to used locations for values of cropland cover, terrain ruggedness, and distance to roads, wells, and streams (Supplementary Figures S2–S5). However, in winter, between-group dyads contacted each other high woody cover areas relative to their use, particularly for male and mixed-sex dyads. Further, same-sex dyads in winter were more likely to contact each other in low edge habitat relative to their use (Figure 3). Meanwhile, in summer, mixed-sex dyads had a higher probability of contact in high degrees of edge density when compared to individual use. During the rut, contact locations reflected used locations for both sexes (Supplementary Figure S6).
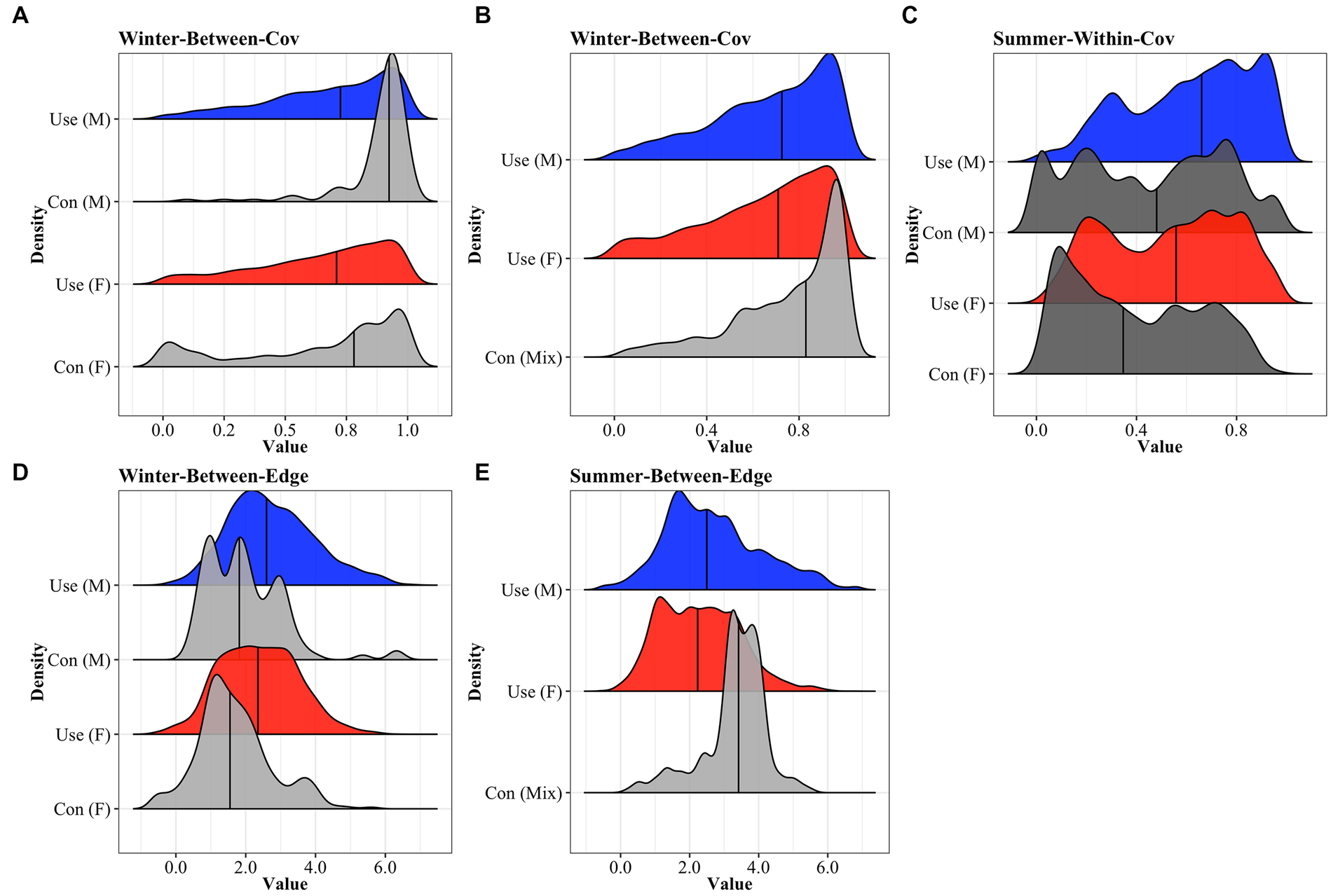
Figure 3. Comparison between density distributions of extent woody cover (top panels) and edge density (bottom panels) values measured at locations used by individual female (red) and male (blue) mule deer and contact locations among (A) between-group male and female contact locations in winter; (B) between-group mixed-sex dyads in winter; (C) within-group male and female dyads in summer; (D) between-group male and female dyads in winter and (E) between-group male and female dyads in summer. Use is defined by GPS locations along 2-h intervals within areas of overlap between the same deer that comprise seasonal, sex-specific dyads. Used and contact locations were recorded from collared mule deer captured within east-central Alberta, Canada (2019–2020). Horizontal black lines represent medians.
3.2. Relating RCP to CWD risk
For each hypothesis, there was clear support for the relationship between CWD risk and predictions from a single season, group type and dyad-specific contact model (Table 6). The relationship between within-group male contacts had the most support for both H1 and H2, indicating that CWD risk increased where the within-group male contacts were high in winter. In contrast, predictions of contacts among between-group female dyads in summer were best supported for H3 and contacts among between-group males best supported H5, indicating that between-group contacts also may played a key role in CWD transmission, but the season may differ. Finally, while the model for mixed-sex dyads in rut best supported H4 and outcompeted the null model, we found a negative relationship between disease risk and the probability of contact.
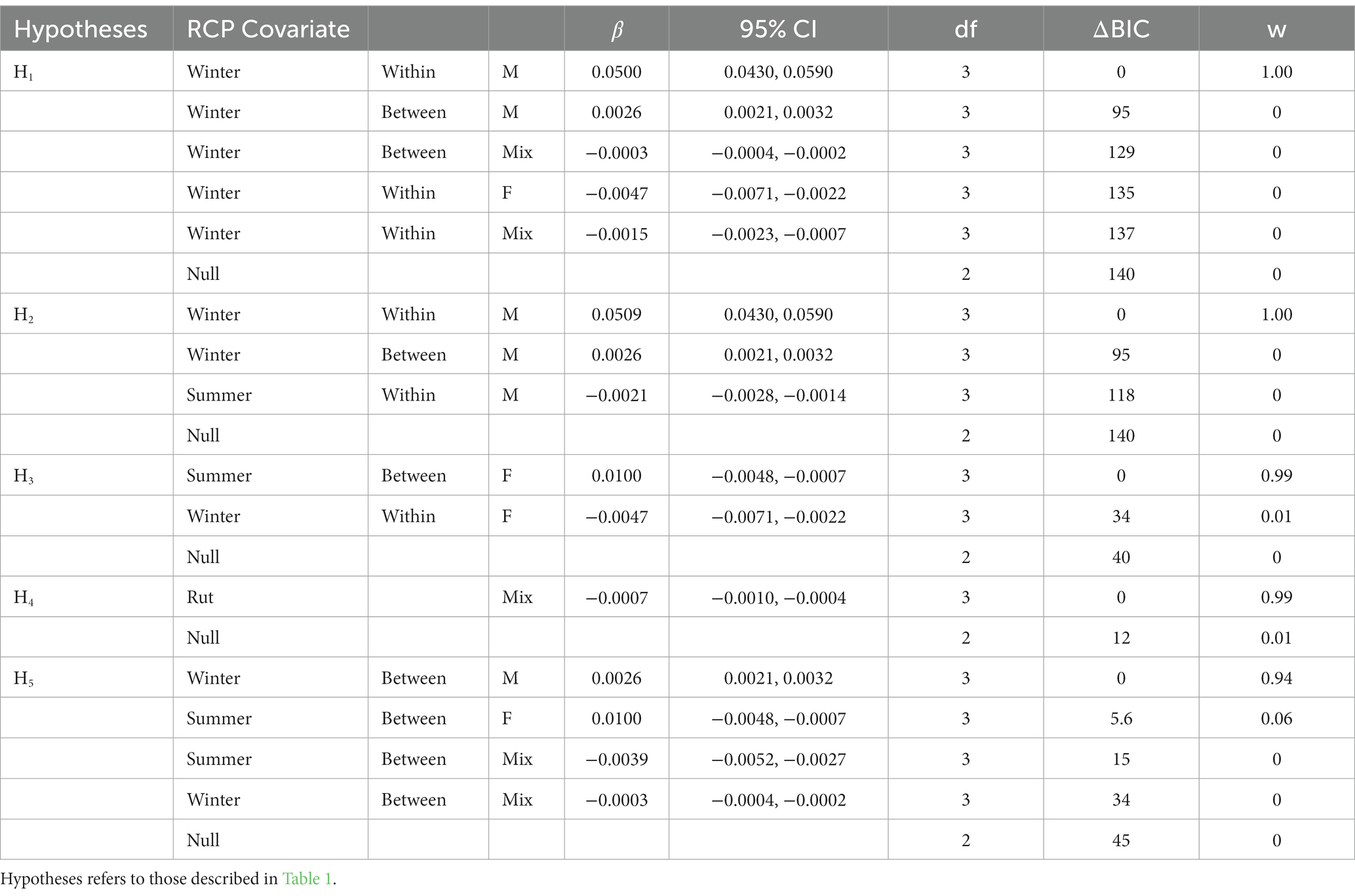
Table 6. Results of model selection using Bayesian Information Criterion (BIC) for univariate, general linear models outcompeting the null model relating predicted risk of CWD occurrence in mule deer to the season, group, and dyad-specific predictions of relative contact probabilities at 5,000 random points within the study in east-central Alberta, Canada.
In the global model using results from the univariate analysis, the best-supported model included the covariates representing between and within-group males in the winter and between-groups females in summer (Table 7; Supplementary Figure S7). The top model was 25 BIC points above second-best supported model and 185 points above the null model. All the covariates in the top model were positive and significant although, according to the beta estimate, the effect size of within-group winter males was largest. Spatial predictions from the global RCP model were correlated to sex-weighted predictions of CWD risk across the 5,000 randomly located sample units corresponding to minimum deer home range areas (Figure 4; Supplementary Figure S8; r = 0.31, p < 0.001).
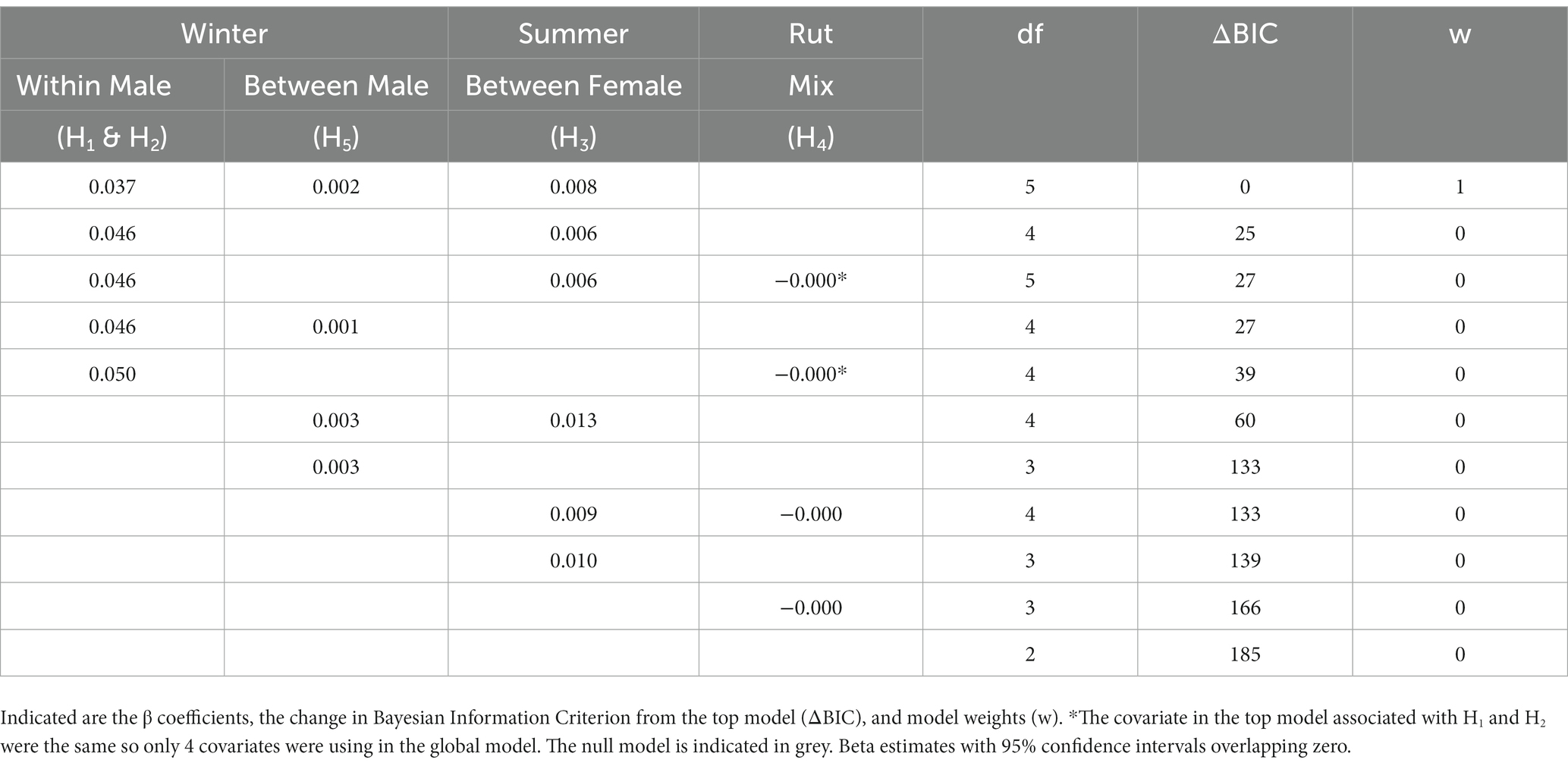
Table 7. Model selection results for the multivariable general linear model that included the covariates from each top univariate model (Table 6) associated with the 5 hypotheses relating chronic wasting disease risk to the season, group and dyad-specific relative contact probability at 5,000 random points within the study in east-central Alberta, Canada.
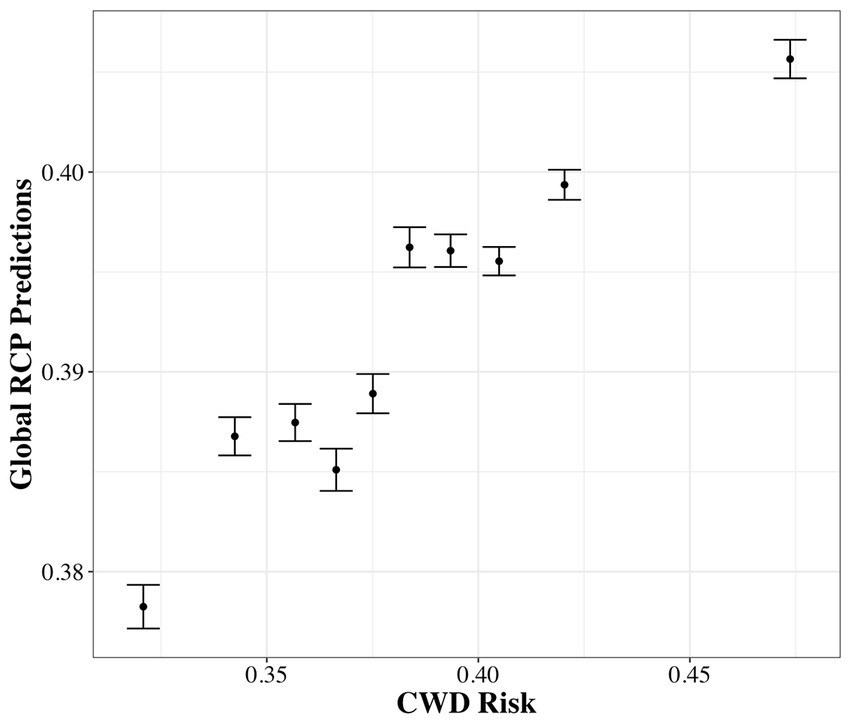
Figure 4. Relationship between the relative contact probability (RCP) predicted from Table 7 and the sex-weighted predictions of CWD risk (Smolko et al., 2021). Shown are the mean (±SE) values derived for randomly located 5-km2 areas averaged within 10 equally sized bins (n = 500/bin). Sample unit size represents the minimum mean home range size of a mule deer across seasons and sexes in the study area.
4. Discussion
Understanding where direct contacts occur across heterogeneous landscapes is key to modeling disease dynamics in a spatial context. Previous studies have used landscape features as surrogates for host density (Joly et al., 2006; Heisey et al., 2010) because of the assumed influence of density on total number of contacts and disease transmission in wildlife. In this study, we quantified the relative probability of where direct, pairwise contacts of mule deer were likely to occur, and found it corresponds to the spatial patterns of CWD-infection in deer on the landscape. We found locations of contacts varied by season and among sex-specific dyad types depending on whether they were within the same or different social groups. We expected the spatial patterns in contacts to reflect areas that deer generally used on the landscape. Indeed, this has been a common assumption in predicting the spatial risk of disease occurring across a region (Farnsworth et al., 2005; Storm et al., 2013; Rosatte et al., 2014). For example, models predicting where CWD would be detected in Montana, USA and west-central Manitoba, Canada, were based primarily on host distributions as predicted by resource selection functions (Dugal et al., 2013; Russell et al., 2015). However, in observing where deer contacts occurred relative to their use of landscape features, we found mixed results.
Where contacts occurred were more consistent among within- and between-group dyads in winter than in summer for both male and female dyads. Mule deer form large aggregations in winter, especially where habitat is limited by snow cover and because deer are no longer sexually segregated (Wood et al., 1989; Lingle, 2003). In winter, deer contacts were more likely to occur in rugged areas far from agriculture and less likely to occur near human activities. Mule deer select rugged areas as escape terrain from predators and because they are associated with shallow snow in winters (Anderson et al., 2012; Webb et al., 2013; Coe et al., 2018). Typically deer also avoid roads and well pads because they are associated with high human activity and noise (Webb et al., 2011; Northrup et al., 2021). We found most between-group contacts were more likely to occur in areas of high woody cover relative to their use. Mule deer selection for woody cover in winter has been attributed to the provision of thermal cover, reduced snow depth, and camouflage from predators (Connolly, 1981; Nixon et al., 1991; McClure et al., 2005). The combination of deer attraction to woody cover and the insular and fragmented nature of forest stands in the aspen parklands (Shorthouse, 2010; Nobert, 2012) may crowd deer from different groups into these habitats similar areas.
In summer, contact locations of within and between-group dyads and those across same-sex dyad types were more distinct than in winter. The seasonal differences between sex-specific dyads likely reflect shifts in sex-specific, seasonal habitat selection due to reduced constraint of snow on movements, wider distribution of available forage, and segregation of the sexes (Bowyer and Kie, 2004; Pierce et al., 2004). For example, same sex between-group dyads, particularly males, were more likely to occur in agricultural areas that provide summer forage (Kjær et al., 2008; Nixon et al., 2008). Further, seasonal increases in forage alongside roadsides provide opportunities that increase contact among same sex dyads in differing social groups (Bellis and Graves, 1971; Waring et al., 1991). Mixed-sex contacts did not show similar increases when near croplands or roadsides, which may reflect either that the different sexes forage in distinct areas or foraging activities occur at different times the same areas (Ruckstuhl and Neuhaus, 2000; Bowyer and Kie, 2004; Biggerstaff et al., 2017). In summer as in winter, woody cover attracts mule deer and we found it influenced contact locations across all groups (Habib et al., 2011). For female dyads, contact locations in rugged terrain and edge habitats may reflect key antipredator and nutritional requirements of post-parturient females with fawns (Mysterud and Østbye, 1999; Kie et al., 2002; D’Eon and Serrouya, 2005; Lingle et al., 2005; Horncastle et al., 2013).
We expected patterns of where contacts occurred to be related to the types of areas that deer generally used on the landscape. In particular, we expected that locations of within-group contacts of deer dyads would closely reflect habitat use by deer due to the cohesiveness of group members (Lingle, 2003; Bowyer and Kie, 2004), and we found this to be generally true. The exception was that contacts of same-sex dyads during summer in both males and females were less common when the extent of woody cover in an area was low (i.e., more open-canopied areas) than indicated by their use of these types of areas. Although deer show strong selection for woody cover in summer (Silbernagel et al., 2011; Nobert et al., 2016), this may reflect that animals even within the same group are less spatially aggregated reducing contacts when foraging in summer when resources are abundant.
Although we also found general correspondence between what deer used on the landscape and where between-group contacts occurred, it was less consistent than that of within-group contacts locations. Distribution of deer GPS locations and locations of contacts were similar relative to agricultural crops, rugged terrain, proximity to streams and industrial well sites. In contrast, there were differences in the distribution of between-group contact locations of deer relative to their use when considering the extent of woody cover and edge habitat in an area. In winter, contacts between groups of same-sex dyads (both males and females) were higher in areas of extensive cover and low edge habitat relative to what they used, whereas in summer between-group contacts of mixed-sex dyads increased where edge density was high relative to what they used. The study area is a heterogeneous mix of woody cover, open prairie, and croplands, where the covariation in these landscape components reflects the fragmentation of woodlands into patches. We suspect that in winter, when deer groups are concentrated on limited winter ranges, over time individual deer may be drawn to the remaining high-quality patchy of food resources associated within woody cover (Pierce et al., 2004; Habib et al., 2011; Anderson et al., 2012) increasing contacts with other deer similar when deer are attracted to feeding sites (Thompson et al., 2008; Sorensen, 2014). In summer, between-group contacts in areas of high-edge density (small, fragmented woody cover patches) may increase the probability of a contact between mixed-sex dyads potentially due behavioral changes and social attraction. For example, mule deer in Idaho were more vigilant in forest-edge habitats relative to open or forest alone (Altendorf et al., 2001). If high vigilance in these habitats increases detection of conspecifics, attraction to the opposite sex due either to familiar ties, social hierarchy, or curiosity, this may increase contacts rates.
Based on our results, we propose that using selected deer habitat is a useful approximation of where disease transmission is likely to occur when devising risk assessment and surveillance strategies in areas without recorded cases of CWD positive animals. Nonetheless, the correspondence will depend on the degree of variation in deer preferences that lead to overlapping use of landscape features, and the extent that additional behaviors alter contact probability. Host distribution also may be a reasonable first approximation to environmental transmission, but similarly it may depend on host activity in the areas when assessing its reliability in predicting CWD-infected hosts on the landscape. We suggest that an understanding of spatial contacts in disease is like that proposed for understanding kill sites in predation, where the probability of a predator making a kill at a location depends on the probabilities of encounter, followed by the probability of attack, as well as other environmental variables influencing the vulnerability of the prey (Hebblewhite et al., 2005; McPhee et al., 2012). Importantly, we assume that the patterns reflected here are the result of direct contacts being the primary route of transmission in the study area. Although there is evidence that the demographic patterns in CWD prevalence arise from the influence of direct contacts in early stages of an outbreak (Potapov et al., 2013; Storm et al., 2013; Ketz et al., 2019) there is a possibility that environmental contacts are influencing the relationship between disease risk and landscape feature in this study area. We did find support that areas of standard deer use varied from those associated with direct contacts, but more work is needed to partition the relative effects of direct and environmental contacts on disease dynamics.
One caveat of our results is that we stratified RCP models by season to account for changes in deer social behavior between the winter, summer, and rut. Thus, season-specific behavioral differences such as migration should be reflected in our models. However, migratory behaviors could affect patterns of disease if infected animals are traveling large distances between winter and summer ranges. In our study area, 23% of mule deer migrated between winter and summer ranges, where ~80% of the distances moved were < 10 km (Merrill et al., unpublished data). Because mule deer in this area are not prone to large migratory movements, we assume that deer behavior represented by CWD risk models are the reflections of behaviors by local animals. This may not be the case in other ecosystems where mule deer migrations are more than 66 km (e.g., Wyoming; Berger, 2004). Despite this, we provide some of the first evidence that where contacts occur between hosts, we also expect to find a high probability of CWD infection. We found most support for the combined effects of within (H1 and H2) and between-group (H5) male contacts in winter and between-group females in summer (H3) being most directly associated with disease occurrence. However, we cannot rule out the importance of other types of contacts because the RCP of male dyads was correlated with contacts of other group and dyad types in winter. Nevertheless, concentration of deer on winter ranges has often been proposed as a mechanism for increased CWD prevalence because of the greater overlap between groups (Habib et al., 2011; Silbernagel et al., 2011; Garlick et al., 2014). We did not find the same support for the relationship between disease risk and contacts among between-group females during winter as in males despite our predictions of CWD risk being weighted more with predictions from female deer (70%) relative to males (30%) to account for generalized sex ratios in the area (Freeman et al., 2014). It is not surprising that male contact rates in winter are more closely related to disease occurrence in mule deer because they have apparent prevalence that is ~2–3 times higher than female mule deer (Smolko et al., 2021). A similar difference in prevalence between the sexes also has been reported for other jurisdictions at the beginning of a CWD epidemic (Miller and Conner, 2005; Osnas et al., 2009; Rees et al., 2012). There is little evidence to support physiological or immunological differences between the sexes in terms of CWD susceptibility suggesting behavioral differences likely apply (Grear et al., 2006; Mawdsley, 2020; Winter and Escobar, 2020). For example, Potapov et al. (2013) found that the 2:1 ratio in male to female prevalence did not exist when their models did not include strong seasonal sexual segregation, which they attributed to high contacts in summer bachelor groups. However, our findings suggest a stronger link between contacts occurring in winter than in summer when sexual segregation is most common (Bailey, 1960; Bowyer and Kie, 2004).
We also found some support that contacts between groups by female deer in summer influenced disease risk (H3). In socially structured populations, between-group contacts can facilitate population-wide transmission by spreading disease to uninfected groups (Keeling and Eames, 2005; Sah et al., 2018). For example, disease simulations derived from contact networks of GPS-collared badgers (Meles meles) demonstrated that populations with a greater degree of social structure (i.e., fewer between-group connections) resulted in smaller epidemics and lower peak prevalence of infection when compared to populations with more fluid social connections (Rozins et al., 2018). Spread of CWD between related female deer have been attributed to matrilineal social structures where related individuals establish themselves nearby in a so-called “rose-petal” social structure (Hawkins and Klimstra, 1970; Mathews and Porter, 1993). Indeed, studies demonstrate that females highly related to infected deer are also more likely to be CWD-positive (Grear et al., 2010; Cullingham et al., 2011). Thus, contacts occurring between related female deer that are segregated during the fawning season is a plausible linkage between disease occurrence and contact probabilities among females. At the beginning of a disease outbreak, males may play key role in disease transmission, but over the progression of the disease as prevalence increases, females may play a greater role in disease transmission (Rees et al., 2012; Smolko et al., 2021). For example, in Wyoming, where CWD has been endemic since the 1970’s, female prevalence estimates were greater than in males among populations of white-tailed deer (Edmunds et al., 2016), indicating that later stages of epidemics may become more dependent on transmission dynamics of female deer. Previous research also suggests that males in mixed-sex contacts during the rut are key in influencing in the spread of CWD and are a possible mechanism of elevated CWD prevalence in male deer (Grear et al., 2006; Potapov et al., 2013; Storm et al., 2013; Keon et al., 2017). We did not find support for the relationship between spatial risk of CWD and contact locations during the rut; however, this could be due low number of collared male mule during deer breeding season. Alternatively, human activity during hunting season could impact social behaviors at the end of summer and during the rut. For example, in Oregon, habitat selection by female mule deer was unaffected by hunting season while movement rates increased (Brown et al., 2020). In the context of this study, these behavioral changes could alter the frequency of contacts and timing of direct contact, but the locations of contacts would remain consistent.
Devising strategies to combat the spread of CWD is a major focus of most wildlife agencies in North American and now Europe. Our results support intervention strategies currently proposed to target the removal of males broadly in a population and female social groups on a landscape where CWD is an emerging disease (Western Association of Fish and Wildlife Agencies, 2018) because disease risk on the landscape was most closely associated contact locations of these groups. Further, removal of deer prior to when they concentrate on winter range may also slow rates of transmission. Our work also supports targeting infected female groups to reduce transmission as indicated by the long-term management of mule deer in Colorado (Miller et al., 2020), and white-tailed deer in Illinois (Manjerovic et al., 2014) where sustained government culling has maintained relatively stable CWD prevalence numbers.
Data availability statement
The raw data supporting the conclusions of this article will be made available by the authors, without undue reservation.
Ethics statement
The animal study was reviewed and approved by University of Alberta Research Ethics Board (AUP00001369).
Author contributions
EM and MD contributed to conception and design of the study. PS and LP performed the statistical analysis for risk of chronic wasting disease. MD wrote the first draft of the manuscript. All authors contributed to manuscript revision, read, and approved the submitted version.
Funding
The authors would like to acknowledge our gratitude for the funding received from the Government of Alberta, National Science and Engineering Research Council (NSERC; RGPIN-2016-04733), Rocky Mountain Elk Foundation (NA190034), Boone and Crockett Club (BCC WISCGP), Wildlife Management Institute, Alberta Prion Research Institute (AB innovat APRIRTP 201700001M; AB Innovat APRIEP 201800002 Me), University of Alberta, Innovation Canada, Alberta Conservation Association (ACA GECF 030-00-90-228), Northern Alberta Chapter of Safari Club International Hunting Heritage Fund, and Alberta Fish and Game Association (MSL 2020 MD 04, MSL 2019 MD 03) and the Alberta Conservation Association Grants in Biodiversity program.
Acknowledgments
The authors thank the Merrill Lab and members of the CWD project at the University of Alberta for valuable discussion and feedback. Additionally, we thank the technicians whose work contributed to the project including Johanna Thalmann, Liam Horne, Greg Melvin, Gary Elaschuk, and Alia Schamehorn.
Conflict of interest
The authors declare that the research was conducted in the absence of any commercial or financial relationships that could be construed as a potential conflict of interest.
Publisher’s note
All claims expressed in this article are solely those of the authors and do not necessarily represent those of their affiliated organizations, or those of the publisher, the editors and the reviewers. Any product that may be evaluated in this article, or claim that may be made by its manufacturer, is not guaranteed or endorsed by the publisher.
Supplementary material
The Supplementary material for this article can be found online at: https://www.frontiersin.org/articles/10.3389/fevo.2023.1156853/full#supplementary-material
References
Ågren, E. O., Sörén, K., Gavier-Widén, D., Benestad, S. L., Tran, L., Wall, K., et al. (2021). First detection of chronic wasting disease in moose (Alces alces) in Sweden. J. Wildl. Dis. 57, 461–463. doi: 10.7589/JWD-D-20-00141
Alberta Energy Regulator (2020). ST37: List of Wells in Alberta. Available at: https://www.aer.ca/providing-information/data-and-reports/statistical-reports/st37 (Accessed July 24, 2020).
Altalis (2018a). 25m Raster DEM. Available at: https://www.altalis.com/map;id=150 (Accessed December 14, 2020).
Altalis (2018b). Hydrography. Available at: https://www.altalis.com/map;id=116 (Accessed July 31, 2020).
Altalis (2020). Access. Available at: https://www.altalis.com/map;id=201 (Accessed July 23, 2020).
Altendorf, K. B., Laundré, J. W., López González, C. A., and Brown, J. S. (2001). Assessing effects of predation risk on foraging behavior of mule deer. J. Mammal. 82, 430–439. doi: 10.1644/1545-1542(2001)082<0430:AEOPRO>2.0.CO;2
Anderson, E. D., Long, R. A., Atwood, M. P., Kie, J. G., Thomas, T. R., Zager, P., et al. (2012). Winter resource selection by female mule deer Odocoileus hemionus: functional response to spatio-temporal changes in habitat. Wildlife Biol. 18, 153–163. doi: 10.2981/11-048
Bailey, E. D. (1960). Behavior of the rattlesnake mule deer on their winter range. Available at: https://scholarworks.umt.edu/cgi/viewcontent.cgi?article=7507&context=etd (Accessed August 25, 2022).
Bates, D., Mächler, M., Bolker, B., and Walker, S. (2015). Fitting linear mixed-effects models using lme4. J. Stat. Softw. 67, 1–48. doi: 10.18637/jss.v067.i01
Becker, D. J., Streicker, D. G., and Altizer, S. (2018). Using host species traits to understand the consequences of resource provisioning for host–parasite interactions. J. Anim. Ecol. 87, 511–525. doi: 10.1111/1365-2656.12765
Bellis, E. D., and Graves, H. B. (1971). Deer mortality on a Pennsylvania interstate highway. J. Wildl. Manag. 35:232. doi: 10.2307/3799596
Berger, J. (2004). The last mile: how to sustain long-distance migration in mammals. Conserv. Biol. 18, 320–331. doi: 10.1111/j.1523-1739.2004.00548.x
Biggerstaff, M. T., Lashley, M. A., Chitwood, M. C., Moorman, C. E., and DePerno, C. S. (2017). Sexual segregation of forage patch use: support for the social-factors and predation hypotheses. Behav. Process. 136, 36–42. doi: 10.1016/j.beproc.2017.01.003
Bowyer, R. T., and Kie, J. G. (2004). Effects of foraging activity on sexual segregation in mule deer. J. Mammal. 85, 498–504. doi: 10.1644/BOS-115
Brown, C. L., Smith, J. B., Wisdom, M. J., Rowland, M. M., Spitz, D. B., and Clark, D. A. (2020). Evaluating indirect effects of hunting on mule deer spatial behavior. J. Wildl. Manag. 84, 1246–1255. doi: 10.1002/jwmg.21916
Coe, P. K., Clark, D. A., Nielson, R. M., Gregory, S. C., Cupples, J. B., Hedrick, M. J., et al. (2018). Multiscale models of habitat use by mule deer in winter. J. Wildl. Manag. 82, 1285–1299. doi: 10.1002/jwmg.21484
Conner, M. M., and Miller, M. W. (2004). Movement patterns and spatial epidemiology of a prion disease in mule deer population units. Ecol. Appl. 14, 1870–1881. doi: 10.1890/03-5309
Connolly, G. E. (1981). Trends in populations and harvests. Nebraska Press, Lincoln: Nebraska, 225–243.
Cullingham, C. I., Nakada, S. M., Merrill, E. H., Bollinger, T. K., Pybus, M. J., and Coltman, D. W. (2011). Multiscale population genetic analysis of mule deer (Odocoileus hemionus hemionus) in western Canada sheds new light on the spread of chronic wasting disease. Can. J. Zool. 89, 134–147. doi: 10.1139/Z10-104
D’Eon, R. G., and Serrouya, R. (2005). Mule deer seasonal movements and multiscale resource selection using global positioning system radiotelemetry. J. Mammal. 86, 736–744. doi: 10.1644/1545-1542(2005)086[0736:MDSMAM]2.0.CO;2
Dobbin, M. A. (2022). Implications of direct contacts between mule deer (Odocoileus hemionus) on transmission of chronic wasting disease. Department of Biological Science at the University of Alberta. doi: 10.7939/r3-b45g-tv43
Dugal, C. J., van Beest, F. M., Vander Wal, E., and Brook, R. K. (2013). Targeting hunter distribution based on host resource selection and kill sites to manage disease risk. Ecol. Evol. 3, 4265–4277. doi: 10.1002/ece3.788
Edmunds, D. R., Kauffman, M. J., Schumaker, B. A., Lindzey, F. G., Cook, W. E., Kreeger, T. J., et al. (2016). Chronic wasting disease drives population decline of white-tailed deer. PLoS One 11, 1–19. doi: 10.1371/journal.pone.0161127
Ehrmann, S., Ruyts, S. C., Scherer-Lorenzen, M., Bauhus, J., Brunet, J., Cousins, S. A. O., et al. (2018). Habitat properties are key drivers of Borrelia burgdorferi (s.l.) prevalence in Ixodes ricinus populations of deciduous forest fragments. Parasites Vectors 11, 23–15. doi: 10.1186/s13071-017-2590-x
Evans, T. S., Kirchgessner, M. S., Eyler, B., Ryan, C. W., and Walter, W. D. (2016). Habitat influences distribution of chronic wasting disease in white-tailed deer. J. Wildl. Manag. 80, 284–291. doi: 10.1002/jwmg.1004
Farnsworth, M. L., Wolfe, L. L., Hobbs, N. T., Burnham, K. P., Williams, E. S., Theobald, D. M., et al. (2005). Human land use influences chronic wasting disease prevalence in mule deer. Ecol. Appl. 15, 119–126. doi: 10.1890/04-0194
Fountain-Jones, N. M., Kraberger, S., Gagne, R. B., Trumbo, D. R., Salerno, P. E., Chris Funk, W., et al. (2021). Host relatedness and landscape connectivity shape pathogen spread in the puma, a large secretive carnivore. Commun. Biol. 4:12. doi: 10.1038/s42003-020-01548-2
Freeman, E. D., Larsen, R. T., Peterson, M. E., Anderson, C. R., Hersey, K. R., and McMillan, B. R. (2014). Effects of male-biased harvest on mule deer: implications for rates of pregnancy, synchrony, and timing of parturition. Wildl. Soc. Bull. 38, 806–811. doi: 10.1002/wsb.450
Garlick, M. J., Powell, J. A., Hooten, M. B., and MacFarlane, L. R. (2014). Homogenization, sex, and differential motility predict spread of chronic wasting disease in mule deer in southern Utah. J. Math. Biol. 69, 369–399. doi: 10.1007/s00285-013-0709-z
Gillies, C. S., Hebblewhite, M., Nielsen, S. E., Krawchuk, M. A., Aldridge, C. L., Frair, J. L., et al. (2006). Application of random effects to the study of resource selection by animals. J. Anim. Ecol. 75, 887–898. doi: 10.1111/j.1365-2656.2006.01106.x
Grear, D. A., Samuel, M. D., Langenberg, J. A., and Keane, D. (2006). Demographic patterns and harvest vulnerability of chronic wasting disease infected white-tailed deer in Wisconsin. J. Wildl. Manag. 70, 546–553. doi: 10.2193/0022-541X(2006)70[546:DPAHVO]2.0.CO;2
Grear, D. A., Samuel, M. D., Scribner, K. T., Weckworth, B. V., and Langenberg, J. A. (2010). Influence of genetic relatedness and spatial proximity on chronic wasting disease infection among female white-tailed deer. J. Appl. Ecol. 47, 532–540. doi: 10.1111/j.1365-2664.2010.01813.x
Gritter, K. (2022). Individual-based movement model of mule deer (Odocoileus hemionus) contacts and application to artificial attractants. Department of Biological Science at the University of Alberta. doi: 10.7939/r3-6rah-cm59
Habib, T. J., Merrill, E. H., Pybus, M. J., and Coltman, D. W. (2011). Modelling landscape effects on density-contact rate relationships of deer in eastern Alberta: implications for chronic wasting disease. Ecol. Model. 222, 2722–2732. doi: 10.1016/j.ecolmodel.2011.05.007
Hawkins, R. E., and Klimstra, W. D. (1970). A preliminary study of social organization of white-tailed deer. J. Wildl. Manag. 34:407. doi: 10.2307/3799027
Hebblewhite, M., Merrill, E. H., and McDonald, T. L. (2005). Spatial decomposition of predation risk using resource selection functions: an example in a wolf–elk predator–prey system. Oikos 111, 101–111. doi: 10.1111/j.0030-1299.2005.13858.x
Heisey, D. M., Osnas, E. E., Cross, P. C., Joly, D. O., Langenberg, J. A., and Miller, M. W. (2010). Linking process to pattern: estimating spatiotemporal dynamics of a wildlife epidemic from cross-sectional data. Ecol. Monogr. 80, 221–240. doi: 10.1890/09-0052.1
Horncastle, V. J., Fenner Yarborough, R., Dickson, B. G., and Rosenstock, S. S. (2013). Summer habitat use by adult female mule deer in a restoration-treated ponderosa pine forest. Wildl. Soc. Bull. 37, 707–713. doi: 10.1002/wsb.301
Janousek, W. M., Graves, T. A., Berman, E. E., Chong, G. W., Cole, E. K., Dewey, S. R., et al. (2021). Human activities and weather drive contact rates of wintering elk. J. Appl. Ecol. 58, 667–676. doi: 10.1111/1365-2664.13818
Joly, D. O., Samuel, M. D., Langenberg, J. A., Blanchong, J. A., Batha, C. A., Rolley, R. E., et al. (2006). Spatial epidemiology of chronic wasting disease in Wisconsin white-tailed deer. J. Wildl. Dis. 42, 578–588. doi: 10.7589/0090-3558-42.3.578
Keeling, M. J., and Eames, K. T. D. (2005). Networks and epidemic models. J. R. Soc. Interface 2, 295–307. doi: 10.1098/rsif.2005.0051
Keon, E. L., Tosa, M. I., Nielsen, C. K., and Schauber, E. M. (2017). Does landscape connectivity shape local and global social network structure in white-tailed deer? PLoS One 12:e0173570. doi: 10.1371/journal.pone.0173570
Ketz, A. C., Storm, D. J., and Samuel, M. (2019). Chronic wasting disease and implications for cervid populations. CAB Rev. 2019, 1–15. doi: 10.1079/PAVSNNR201914038
Kie, J. G., Terry Bowyer, R., Nicholson, M. C., Boroski, B. B., and Loft, E. R. (2002). Landscape heterogeneity at differing scales: effects on spatial distribution of mule deer. Ecology 83, 530–544. doi: 10.1890/0012-9658(2002)083[0530:LHADSE]2.0.CO;2
Kjær, L. J., Schauber, E. M., and Nielsen, C. K. (2008). Spatial and temporal analysis of contact rates in female white-tailed deer. J. Wildl. Manag. 72, 1819–1825. doi: 10.2193/2007-489
Kristensen, K., Nielsen, A., Berg, C. W., Skaug, H., and Bell, B. M. (2016). TMB: automatic differentiation and Laplace approximation. J. Stat. Softw. 70, 1–21. doi: 10.18637/jss.v070.i05
Latifovic, R. (2015). 2015 Land Cover of Canada. Available at: https://open.canada.ca/data/en/dataset/4e615eae-b90c-420b-adee-2ca35896caf6 (Accessed November 1, 2022).
Lele, S. R., Merrill, E. H., Keim, J., and Boyce, M. S. (2013). Selection, use, choice and occupancy: clarifying concepts in resource selection studies. J. Anim. Ecol. 82, 1183–1191. doi: 10.1111/1365-2656.12141
Lingle, S. (2003). Group composition and cohesion in sympatric white-tailed deer and mule deer. Can. J. Zool. 81, 1119–1130. doi: 10.1139/z03-097
Lingle, S. E., Pellis, S. M., and Wilson, W. F. (2005). Interspecific variation in antipredator behaviour leads to differential vulnerability of mule deer and white tailed deer fawns early in life. J. Anim. Ecol. 74, 1140–1149. doi: 10.1111/j.1365-2656.2005.01014.x
Lloyd-Smith, J. O., Schreiber, S. J., Kopp, P. E., and Getz, W. M. (2005). Superspreading and the effect of individual variation on disease emergence. Nature 438, 355–359. doi: 10.1038/nature04153
Manjerovic, M. B., Green, M. L., Mateus-Pinilla, N., and Novakofski, J. (2014). The importance of localized culling in stabilizing chronic wasting disease prevalence in white-tailed deer populations. Prev. Vet. Med. 113, 139–145. doi: 10.1016/j.prevetmed.2013.09.011
Manly, B., Mcdonald, L., Thomas, D., Mcdonald, T., and Erickson, W. (2002). Resource Selection by Animals: Statistical Design and Analysis for Field Studies. J. Anim. Ecol. 63. doi: 10.2307/5247
Mathews, N. E., and Porter, W. F. (1993). Effect of social structure on genetic structure of free-ranging white-tailed deer in the Adirondack Mountains. J. Mammal. 74, 33–43. doi: 10.2307/1381903
Mawdsley, J. R. (2020). Phylogenetic patterns suggest broad susceptibility to chronic wasting disease across Cervidae. Wildl. Soc. Bull. 44, 152–155. doi: 10.1002/wsb.1059
McClure, M. F., Bissonette, J. A., and Conover, M. R. (2005). Migratory strategies, fawn recruitment, and winter habitat use by urban and rural mule deer (Odocoileus hemionus). Eur. J. Wildl. Res. 51, 170–177. doi: 10.1007/s10344-005-0086-z
McPhee, H. M., Webb, N. F., and Merrill, E. H. (2012). Hierarchical predation: wolf (Canis lupus) selection along hunt paths and at kill sites. Can. J. Zool. 90, 555–563. doi: 10.1139/z2012-021
Mejía-Salazar, M. F., Goldizen, A. W., Menz, C. S., Dwyer, R. G., Blomberg, S. P., Waldner, C. L., et al. (2017). Mule deer spatial association patterns and potential implications for transmission of an epizootic disease. PLoS One 12, e0175385–e0175322. doi: 10.1371/journal.pone.0175385
Miller, M. W., and Conner, M. M. (2005). Epidemiology of chronic wasting disease in free-ranging mule deer: spatial, temporal, and demographic influences on observed prevalence patterns. J. Wildl. Dis. 41, 275–290. doi: 10.7589/0090-3558-41.2.275
Miller, W. L., Miller-Butterworth, C. M., Diefenbach, D. R., and Walter, W. D. (2020). Assessment of spatial genetic structure to identify populations at risk for infection of an emerging epizootic disease. Ecol. Evol. 10, 3977–3990. doi: 10.1002/ece3.6161
Miller, M. W., Williams, E. S., Hobbs, N. T., and Wolfe, L. L. (2004). Environmental sources of prion transmission in mule deer. Emerg. Infect. Dis. 10, 1003–1006. doi: 10.3201/eid1006.040010
Miller, M. W., Williams, E. S., McCarty, C. W., Spraker, T. R., Kreeger, T. J., Larsen, C. T., et al. (2000). Epizootiology of chronic wasting disease in free-ranging Cervids in Colorado and Wyoming. J. Wildl. Dis. 36, 676–690. doi: 10.7589/0090-3558-36.4.676
Morano, S., Stewart, K. M., Dilts, T., Ellsworth, A., and Bleich, V. C. (2019). Resource selection of mule deer in a shrub-steppe ecosystem: influence of woodland distribution and animal behavior. Ecosphere 10:2811. doi: 10.1002/ecs2.2811
Mysterud, A., and Edmunds, D. R. (2019). A review of chronic wasting disease in North America with implications for Europe. Eur. J. Wildl. Res. 65:1260. doi: 10.1007/s10344-019-1260-z
Mysterud, A., and Østbye, E. (1999). Cover as a habitat element for temperate ungulates: effects on habitat selection and demography. Wildl. Soc. Bull. 27, 385–394.
Nixon, C. M., Hansen, L. P., Brewer, P. A., and Chelsvig, J. E. (1991). Ecology of white-tailed deer in an intensively farmed region of Illinois. Wildl. Monogr. 3–77.
Nixon, C. M., Mankin, P. C., Etter, D. R., Hansen, L. P., Brewer, P. A., Chelsvig, J. E., et al. (2008). Migration behavior among female white-tailed deer in central and northern Illinois. Am. Midl. Nat. 160, 178–190. doi: 10.1674/0003-0031(2008)160[178:MBAFWD]2.0.CO;2
Nobert, B. R. (2012). Landscape ecology of mule deer (Odocoileus hemionus) and white-tailed deer (O. virginianus) with implications for chronic wasting disease. Department of Biological Sciences at the University of Alberta. doi: 10.7939/R3BC95
Nobert, B. R., Merrill, E. H., Pybus, M. J., Bollinger, T. K., and Hwang, Y. T. (2016). Landscape connectivity predicts chronic wasting disease risk in Canada. J. Appl. Ecol. 53, 1450–1459. doi: 10.1111/1365-2664.12677
Northrup, J. M., Anderson, C. R. Jr., Gerber, B. D., and Wittemyer, G. (2021). Behavioral and demographic responses of mule deer to energy development on Winter range. Wildl. Monogr. 208, 1–37. doi: 10.1002/wmon.1060
Osnas, E. E., Heisey, D. M., Rolley, R. E., and Samuel, M. D. (2009). Spatial and temporal patterns of chronic wasting disease: fine-scale mapping of a wildlife epidemic in Wisconsin. Ecol. Appl. 19, 1311–1322. doi: 10.1890/08-0578.1
Ostfeld, R. S., Glass, G. E., and Keesing, F. (2005). Spatial epidemiology: an emerging (or re-emerging) discipline. Trends Ecol. Evol. 20, 328–336. doi: 10.1016/j.tree.2005.03.009
Page, L. K., Swihart, R. K., Kazacos, K. R., Page, L. K., Swihart, R. K., and Kazacos, K. R. (2001). Nordic Society Oikos Changes in Transmission of Baylisascaris procyonis to Intermediate Hosts as a Function of Spatial Scale. Oikos 93, 213–220.
Paull, S. H., Song, S., McClure, K. M., Sackett, L. C., Kilpatrick, A. M., and Johnson, P. T. J. (2012). From super spreaders to disease hotspots: linking transmission across hosts and space. Front. Ecol. Environ. 10, 75–82. doi: 10.1890/110111
Pierce, B. M., Bowyer, R. T., and Bleich, V. C. (2004). Habitat selection by mule deer: forage benefits or risk of predation? J. Wildl. Manag. 68, 533–541. doi: 10.2193/0022-541X(2004)068[0533:HSBMDF]2.0.CO;2
Potapov, A., Merrill, E., Pybus, M., Coltman, D., and Lewis, M. A. (2013). Chronic wasting disease: possible transmission mechanisms in deer. Ecol. Model. 250, 244–257. doi: 10.1016/j.ecolmodel.2012.11.012
R Core Team (2021). R: A language and environment for statistical computing. Vienna, Austria: R Foundation for Statistical Computing Available at: https://www.r-project.org/
Rees, E. E., Merrill, E. H., Bollinger, T. K., Hwang, Y.Ten, Pybus, M. J., and Coltman, D. W. (2012). Targeting the detection of chronic wasting disease using the hunter harvest during early phases of an outbreak in Saskatchewan, Canada. Prev. Vet. Med. 104, 149–159. doi: 10.1016/j.prevetmed.2011.10.016
Riley, S. J., DeGloria, S. D., and Elliot, R. (1999). A terrain ruggedness index that quantifies topographic heterogeneity. Int. J. Sci. 5, 23–27.
Robinette, W. L. (1966). Mule Deer Home Range and Dispersal in Utah. J. Wildl. Manag. 30, 335–349. doi: 10.2307/3797822
Rosatte, R., Buchanan, T., Davies, C., Middel, K., Patterson, B., Bruce, L., et al. (2014). Chronic wasting disease surveillance program and proactive response plan for Ontario, Canada. Can. Wildl. Biol. Manag. 3:52.
Rozins, C., Silk, M. J., Croft, D. P., Delahay, R. J., Hodgson, D. J., McDonald, R. A., et al. (2018). Social structure contains epidemics and regulates individual roles in disease transmission in a group-living mammal. Ecol. Evol. 8, 12044–12055. doi: 10.1002/ece3.4664
Ruckstuhl, K. E., and Neuhaus, P. (2000). Sexual segregation in ungulates: a new approach. Behaviour 137, 361–377. doi: 10.1163/156853900502123
Russell, R. E., Gude, J., Anderson, N. J., and Ramsey, J. M. (2015). Identifying priority chronic wasting disease surveillance areas for mule deer in Montana. J. Wildl. Manag. 79, 989–997. doi: 10.1002/jwmg.914
Sah, P., Mann, J., and Bansal, S. (2018). Disease implications of animal social network structure: a synthesis across social systems. J. Anim. Ecol. 87, 546–558. doi: 10.1111/1365-2656.12786
Schauber, E. M., Storm, D. J., and Nielsen, C. K. (2007). Effects of joint space use and group membership on contact rates among white-tailed deer. J. Wildl. Manag. 71, 155–163. doi: 10.2193/2005-546
Serrouya, R., and D’Eon, R. G. (2008). The influence of forest cover on mule deer habitat selection, diet, and nutrition during winter in a deep-snow ecosystem. For. Ecol. Manag. 256, 452–461. doi: 10.1016/j.foreco.2008.04.048
Shorthouse, J. D. (2010). “Ecoregions of Canada’s prairie grasslands,”in Arthropods of Canadian Grasslands (Volume 1): Ecology and Interactions in Grassland Habitats. eds. J. D. Shorthouse and K. D. Floate (Biological Survey of Canada), 53–81.
Silbernagel, E. R., Skelton, N. K., Waldner, C. L., and Bollinger, T. K. (2011). Interaction among deer in a chronic wasting disease endemic zone. J. Wildl. Manag. 75, 1453–1461. doi: 10.1002/jwmg.172
Smolko, P., Seidel, D., Pybus, M., Hubbs, A., Ball, M., and Merrill, E. (2021). Spatio-temporal changes in chronic wasting disease risk in wild deer during 14 years of surveillance in Alberta, Canada. Prev. Vet. Med. 197:105512. doi: 10.1016/j.prevetmed.2021.105512
Sorensen, A. A. (2014). Habitat selection by sympatric ungulates in an agricultural landscape: Implications for disease transmission and human-wildlife conflict. Department of Animal and Poultry Science at the University of Saskatchewan. Available at: http://hdl.handle.net/10388/ETD-2013-01-1390
Storm, D. J., Samuel, M. D., Rolley, R. E., Shelton, P., Keuler, N. S., Richards, B. J., et al. (2013). Deer density and disease prevalence influence transmission of chronic wasting disease in white-tailed deer. Ecosphere 4:art10. doi: 10.1890/ES12-00141.1
Thompson, A. K., Samuel, M. D., and VanDeelen, T. R. (2008). Alternative feeding strategies and potential disease transmission in Wisconsin white-tailed deer. J. Wildl. Manag. 72, 416–421. doi: 10.2193/2006-543
Walter, W. D., Evans, T. S., Stainbrook, D., Wallingford, B. D., Rosenberry, C. S., and Diefenbach, D. R. (2018). Heterogeneity of a landscape influences size of home range in a north American cervid. Sci. Rep. 8:14667. doi: 10.1038/s41598-018-32937-7
Waring, G. H., Griffis, J. L., and Vaughn, M. E. (1991). White-tailed deer roadside behavior, wildlife warning reflectors, and highway mortality. Appl. Anim. Behav. Sci. 29, 215–223. doi: 10.1016/0168-1591(91)90249-W
Webb, S. L., Dzialak, M. R., Kosciuch, K. L., and Winstead, J. B. (2013). Winter resource selection by mule deer on the Wyoming-Colorado border prior to wind energy development. Rangel Ecol. Manag. 419–427. doi: 10.2111/REM-D-12-00065.1
Webb, S. L., Dzialak, M. R., Osborn, R. G., Harju, S. M., Wondzell, J. J., Hayden-Wing, L. D., et al. (2011). Using pellet groups to assess response of elk and deer to roads and energy development. Wildl. Biol. Pract. 7, 32–40. doi: 10.2461/wbp.2011.7.3
Western Association of Fish and Wildlife Agencies . (2018). Recommendations for adaptive management of chronic wasting disease in the West. Western Association of Fish and Wildlife Agencies, Edmonton, Alberta, Canada and Fort Collins, Colorado.
Winter, S. N., and Escobar, L. E. (2020). Chronic wasting disease modeling: an overview. J. Wildl. Dis. 56, 741–758. doi: 10.7589/2019-08-213
Wood, A. K., Hamlin, K. L., Mackie, R. J., Richard, J., and Division, M. W. (1989). Ecology of sympatric populations of mule deer and white-tailed deer in a prairie environment. [S.l.]: Wildlife Division, Montana Dept. of Fish, Wildlife & Parks Available at: https://www.biodiversitylibrary.org/item/137531
Keywords: mule deer, chronic wasting disease, contacts, epidemiology, landscape
Citation: Dobbin MA, Smolko P, Put L and Merrill EH (2023) Risky business: relating probability of direct contact to risk of chronic wasting disease. Front. Ecol. Evol. 11:1156853. doi: 10.3389/fevo.2023.1156853
Edited by:
Ruscena P. Wiederholt, Everglades Foundation, United StatesReviewed by:
W. David Walter, United States Department of the Interior, United StatesNohra Mateus-Pinilla, University of Illinois Urbana-Champaign (UIUC), United States
Brian Dugovich, University of Georgia, United States
Copyright © 2023 Dobbin, Smolko, Put and Merrill. This is an open-access article distributed under the terms of the Creative Commons Attribution License (CC BY). The use, distribution or reproduction in other forums is permitted, provided the original author(s) and the copyright owner(s) are credited and that the original publication in this journal is cited, in accordance with accepted academic practice. No use, distribution or reproduction is permitted which does not comply with these terms.
*Correspondence: Maria A. Dobbin, bWRvYmJpbkB1YWxiZXJ0YS5jYQ==