- 1Vetsuisse and Medical Faculty, National Centre for Vector Entomology, Institute of Parasitology, University of Zürich, Zürich, Switzerland
- 2Research Institute of Organic Agriculture FiBL, Frick, Switzerland
- 3Department of Biological Sciences and Biomolecular Sciences Institute, Florida International University, Miami, FL, United States
- 4Department of Environmental System Sciences, ETH Zürich, Zürich, Switzerland
The human skin bacteria play an important role in the production of volatiles that attract mosquitoes. Using some of the most abundant human skin bacterial species, we created in vitro community models to assess whether increased microbial biodiversity could reduce human attractiveness to females of the dengue fever mosquito Aedes aegypti and whether co-culturing bacterial commensals affects overall attraction. More complex bacterial models were less attractive to female mosquitoes than the simplest models. For instance, the triple bacterial community model was approximately three times less attractive than Staphylococcus epidermidis alone. Our data show, for instance, that an in vitro community model mimicking the skin composition of a highly attractive individual to the anthropophilic Anopheles gambiae was also more attractive to anthropophilic Ae. aegypti than a community model mimicking the skin composition of a poorly attractive individual to An. gambiae. In line with these results, volatile analyses of the blends emitted by the different in vitro community models showed that the more complex models had lower emission overall. Effects on mosquito responses differed sharply when the different bacteria species were sharing the same resources used for growth, showing that either competition or commensalism may influence their relative growth, and that this consequently can influence mosquito responses. We conclude that studies on mosquito responses to skin volatiles need to take the microbial community into account.
Introduction
Skin emissions are part of human natural odor playing an important role in mosquito attraction and may explain why some people are bitten more often than others (Logan et al., 2010; Verhulst et al., 2013). These emissions are largely composed of odors produced by skin bacteria (Verhulst et al., 2010, 2011; Jha, 2017). The human skin microbiota plays an essential role in the protection against pathogens, but their odor emission is likely to be also exploited by mosquitoes to locate humans (Byrd et al., 2018; Callewaert et al., 2021).
Mosquitoes do not only cause annoyance and discomfort, but through their bites they can transmit a wide range of pathogens to humans, which can lead to diseases such as malaria and dengue fever (Takken and Verhulst, 2017). Aedes aegypti, Ae. albopictus, and Anopheles gambiae are among the most important vectors of such diseases (Takken and Knols, 1999; McBride et al., 2014). In 2020, the World Health Organization (WHO) estimated 241 million cases of malaria and 627 thousand deaths. Cases of dengue fever have been increasing over the years, and 5.2 million cases were reported by the WHO in 2019. Vector control is one of the most important measures to combat the spread of such diseases and this can involve strategies to reduce mosquito populations and/or personal protection approaches to prevent mosquito bites (Takken and Verhulst, 2017).
Data on microbial species and odors emitted by the human skin bacteria explain differential attraction among people, and this knowledge can be exploited to protect humans from mosquito bites. Manipulation of the bacterial commensals on human skin can offer a valuable option for the creation of a microbial-based (Lucas-Barbosa et al., 2022) topical probiotic that can reduce mosquito attraction. An advantage of using skin bacteria over DEET or any other commercially available repellent is the potential for dramatically extending the duration of protection (DeGennaro, 2015; Mapossa et al., 2021; Lucas-Barbosa et al., 2022). Such a microbial-based topical probiotic would continuously release odors produced by living organisms that either repel or make individuals less attractive, while the commercially available synthetic repellents evaporate within a few hours and must be frequently reapplied (DeGennaro, 2015; Mapossa et al., 2021).
The role of bacteria on mosquito attraction was first established when it was demonstrated that An. gambiae mosquitoes were attracted to sweat incubated with skin bacteria (Meijerink et al., 2000). Follow-up studies have revealed that volatiles produced by skin bacteria are attractive to An. gambiae (Verhulst et al., 2010, 2011, 2013), and that the attraction levels depend on the bacteria species (Verhulst et al., 2010; Showering et al., 2022). Interestingly, An. gambiae mosquitoes were more attracted to people with a lower microbial diversity and with higher levels of Staphylococcus spp., while they were less attracted to people with higher levels of Pseudomonas spp. (Verhulst et al., 2011). The skin bacteria species and the ratio in which the bacterial species are present on the human skin determine how attractive an individual is, though attractiveness may also be dependent on the mosquito species (Busula et al., 2017; Lucas-Barbosa et al., 2022). Thus, the bacterial taxa that are characteristic of the skin of poorly attractive individuals may indicate odors that are important in reducing human attractiveness to mosquitoes (Verhulst et al., 2011, 2013). Studies which had characterized volatile organic compounds (VOCs) which play a role in mosquito attraction illustrate that many of these volatile compounds are of skin microbial origin (Wooding et al., 2020; Dormont et al., 2021; Lucas-Barbosa et al., 2022). Data recently reviewed highlights the volatile compounds most exploited by some of mosquito species which are important vector of diseases (Lucas-Barbosa et al., 2022). Volatile emission may depend on the bacterial community characteristic of the skin and on the available resources for bacterial growth. Thus, questions remain as to whether these bacteria compete for available nutrients in the human skin environment, and whether this competition among skin commensals influences volatile emission and mosquito attraction (Zhang et al., 2015; Lucas-Barbosa et al., 2022).
Our goal was to use in vitro community models to study the impact of human skin bacteria on mosquito attraction, in scenarios in which the different bacteria species must share or cannot share the resources available for growth, and what the consequences are for behavior and odor emission. Using four of the most abundant human skin bacterial species, we created in vitro community models to assess whether and how microbial biodiversity and competition for resources influence the behavior of Ae. aegypti. In vitro community models reflecting the skin composition of highly and poorly attractive individuals based on attraction to An. gambiae (Verhulst et al., 2011, 2013) were created and used to test whether and how such blends influence the behavior of Ae. aegypti. We also collected volatiles from the headspace of the in vitro community models to correlate odor emission with behavioral responses to the different bacterial species and communities.
Materials and methods
Study system
Mosquitoes
A laboratory colony of Ae. aegypti (obtained originally from New Caledonia, Institut Pasteur de Nouvelle Calédonie) was used in the experiments. The laboratory colony was maintained in cages (30 × 30 × 30 cm) in a climate room (27°C ± 2°C, 75–85% r.h., 16L:8D) and reared as previously described (Verhulst et al., 2020; Ziegler et al., 2022). In short, adult mosquitoes had continuous access to 10% sucrose solution and were provided with EDTA anticoagulated cow blood three times a week using a Hemotek feeding system. Larvae were provided with ground Tetramin fish food (Tetra, Blacksburg, VA, United States). Female Ae. Aegypti used for the bioassays were 6–11 days old, had not received a blood meal, and had access to 10% sucrose solution except in the 24 h prior to the bioassay when they only had access to water. Behavioral experiments were carried out in a climate-controlled room (24°C ± 3°C, 60–75% r.h.) and under artificial light (6,500 K).
Bacteria cultivation
Sweat medium and tryptic soy broth (TSB) medium were used to cultivate skin bacteria. Sweat media was prepared by adding 20.9 g L−1 MOPS (Thermo Fisher, BP308500), 1 g L−1 yeast extract (BP1422-2), 2 g L−1 NaCl (BP3581), 0.65 mg L−1 cod methyl ester fatty acids (Sigma-Aldrich, C5650-5G), 0.1 g L−1 Tween 80 (Thermo Fisher, BP338-500), and 7.5 g L−1 of TSB (Sigma-Aldrich, 22,092-500G) to purified water while stirring, and then media was autoclaved for 15 min at 121° C. TSB was prepared by suspending 30 g in 1 L of purified water, followed by sterilization for 15 min at 121°C. In both cases, we used 1.5% of Bactor Agar (Sigma-Aldrich, 05039-500G) when preparing solid media.
Stocks of Staphylococcus epidermidis (Deutsche Sammlung von Mikroorganismen und Zellkulturen GmbH, DSMZ, Braunschweig, Germany; 1798; ATCC 12228), Pseudomonas aeruginosa (DSMZ 1128), Corynebacterium minutissimum (DSMZ 20651), and Brevibacterium epidermidis (DSMZ 20660) were prepared according to the DSMZ protocol. TSB medium was used to prepare bacteria stocks. To determine when the stationary phase for each isolate was reached, we estimated bacterial growth over time. Hundred microliter of bacteria stock was pipetted in 2 mL of liquid sweat media or TSB in the case of B. epidermidis, as this species does not grow well in liquid sweat media. Then, 200 μL inocula for each bacteria species was transferred to the wells of a sterile 96-wells plate, and absorbance measured using the Infinite 200 PRO (Tecan Trading AG, Switzerland). Measurement settings consisted of 10-min intervals with 8.5 min of incubation time under continuous shaking, 10 s of vigorous shaking and absorbance measured at 600 nm. This was performed at 37°C for a total experimental runtime of 48 h. The average of three technical replicates was used to construct the growth curves.
Monocultures of each of the bacteria species were cultured in liquid sweat media at 37°C, shaking at 220 rpm for 24 h, or in TSB in the case of B. epidermidis. For the bioassays, single species previously cultivated as monocultures were added to a sweat media agar plate to form the different human skin in vitro bacterial communities (Figure 1). We had six in vitro community models in total composed of up to four bacteria species that were tested in what we call competitive and non-competitive environments. For the competitive models, the different bacteria species were co-cultured in a regular single chamber, 9 cm Petri-dish. For the non-competitive environment, we used Petri dishes (9 cm) with 1, 2, 3, and 4 chambers (Roth AG, Switzerland) and had each bacteria species grown in a separate chamber so that they could not consume/compete for the same resources, i.e., the medium. Staphylococcus epidermidis was the reference control grown as a single model. Double was composed of S. epidermidis and P. aeruginosa in 1:1 ratio; Triple was composed of S. epidermidis, P. aeruginosa, and C. minutissimum in 1:1:1 ratio; quadruple was composed of S. epidermidis, P. aeruginosa, C. minutissimum, and B. epidermidis in 1:1:1:1 ratio; the Highly Attractive (HA) community was composed of S. epidermidis, P. aeruginosa, C. minutissimum, and B. epidermidis in 3:3:2:8 ratio and the Poorly Attractive (PA) community was composed of the same four species but in 1:6:1:8 ratio, both HA and PA were based on the ratios of skin bacteria determined for individuals that were highly or poorly attractive to An. gambiae (Verhulst et al., 2011). A total of 300 μL of the bacterial inoculum was applied on sweat media agar plates and incubated at 37°C for 24 h prior to the bioassay.
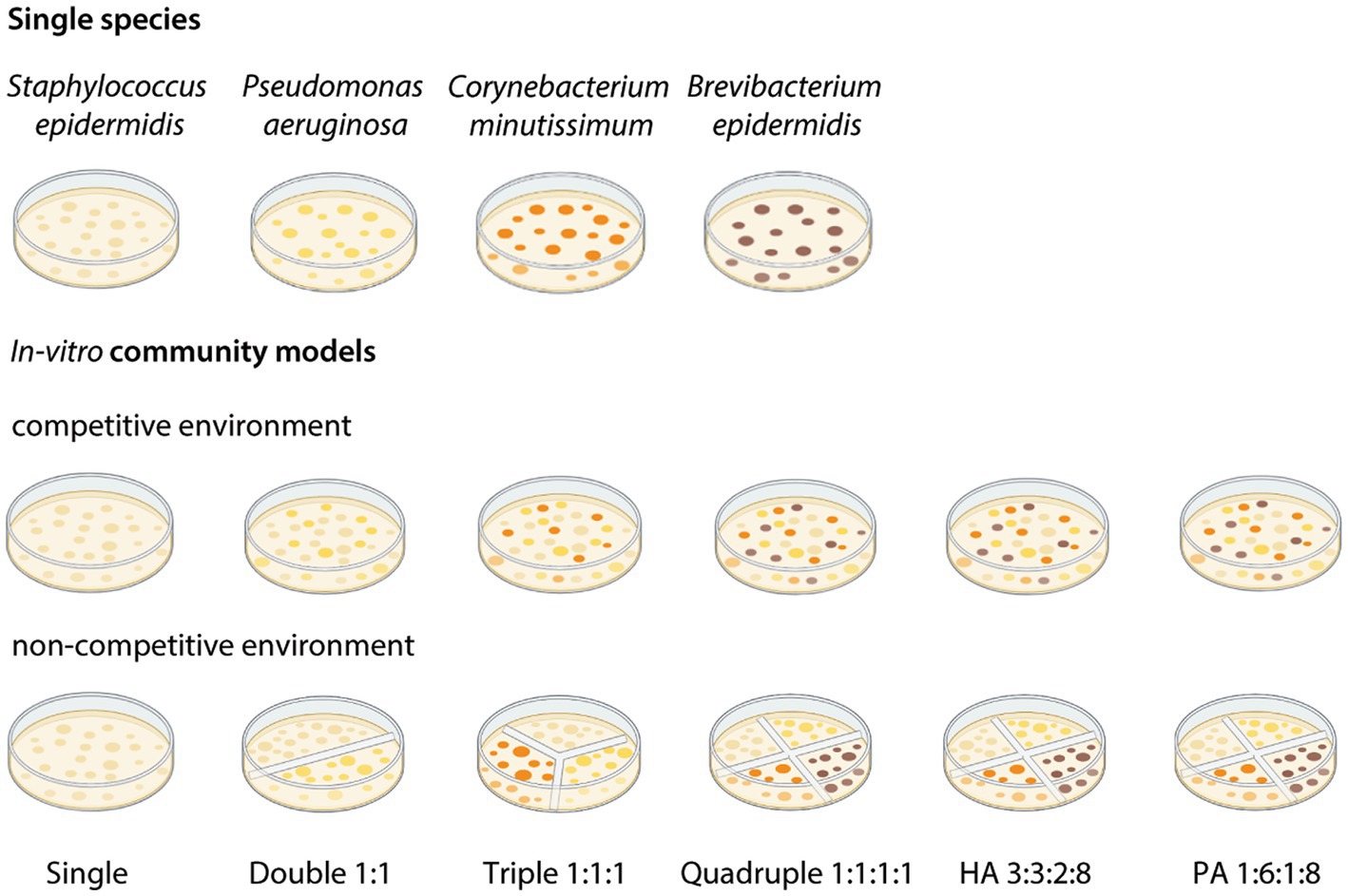
Figure 1. Schematic representation of the single bacteria species and of the in vitro community models used. In vitro community models were composed of up to 4 bacteria species, in competitive and non-competitive environments. Staphylococcus epidermidis was the reference control grown as a single model. Double was composed of S. epidermidis, Pseudomonas aeruginosa in a 1:1 ratio; Triple was composed of S. epidermidis, P. aeruginosa, Corynebacterium minutissimum in a 1:1:1 ratio; Quadruple was composed of S. epidermidis, P. aeruginosa, C. minutissimum, Brevibacterium epidermidis in a 1:1:1:1 ratio; Highly Attractive (HA) community was composed of S. epidermidis, P. aeruginosa, C. minutissimum, B. epidermidis in a 3:3:2:8 ratio and the Poorly Attractive (PA) community was composed of S. epidermidis, P. aeruginosa, C. minutissimum, B. epidermidis in a 1:6:1:8 ratio.
Behavioral assays
In the behavioral assays we recorded responses of Ae. aegypti to the different in vitro skin bacteria community models vs. controls that was either the sweat medium used to grow the bacteria or an S. epidermidis culture. In vitro skin bacteria community models were tested in competitive and in non-competitive environments (Figure 1). We recorded the number of landings and time spent in the arena using the Observer TM software (Noldus, Netherlands). The set-up consisted of a Petri dish containing the bacterial communities and placed inside a cage (30 × 30 × 30 cm) on the top of a heating plate (36°C) (Cosori mug warmer, Arovast Corporation, United States). Twenty female mosquitoes were released at a time, and the number of landings (attraction component of the behavior observed) and time spent per individual (arrestment component of the behavior observed) in the arena (Petri dish) were recorded for 8 min. We report average per replicate in each case. If the same individual flew and landed again in the arena, we scored that as a new landing. CO2 was released at a rate of 260 mL min−1 through a tube that rested on top of the BugDorm cage to imitate the human breathing rate. A Plexiglas shield was used to separate the observer’s odors from the set-up. Every experimental condition was replicated eight times.
Profiling of skin bacterial volatiles
To quantify and identify VOCs emanating from skin bacteria, we collected volatiles from the headspace of bacterial isolates and communities. Bacterial volatiles were collected for 1.5 h onto Tenax GR (GL Sciences, Eindhoven, Netherlands) adsorbent filters by pumping charcoal-purified air into the top of the glass dome at a rate of 400 mL min−1 and simultaneously applying a vacuum of 200 mL min−1 to the back of the glass thermal desorption tubes filled with 200 mg of Tenax GR that was positioned in one of the glass dome side outlets. The Petri dishes containing a bacteria culture or media were put on Teflon plates under the glass dome. The volatile collection was replicated eight times for every treatment, in four experimental batches. Domes were cleaned with hexane and acetone 24 h before each collection.
Skin bacterial volatile profiles were characterized by gas-chromatography-mass-spectrometry with a thermodesorption unit (TD-GC/MS, Leco Coorporation, St. Joseph, United States). VOCs were desorbed from the tubes for 10 min at 250°C and trapped in a liquid nitrogen cooled sorbent trap at −110°C. Compounds were desorbed from this trap during the secondary desorption at a heating rate of 40°C s−1 and kept at 280°C for 10 min, and were transferred to a non-polar gas-chromatography (GC) column (RXI-5ms 30 m × 0.25 mm × 1.00 μm, GL Sciences, Eindhoven, Netherlands) in split ratio resulting in ¼ of the total amount. The chromatographic process was carried out at a flow rate of the carrier gas of 1 mL min−1. The GC oven temperature was programmed from 40°C (5 min hold time) to 280°C (8 min hold time) at 5°C min−1. The MS transfer line was set to 280°C. The energy of the electron beam was set to 70 eV, and the temperature of the ion source was set to 250°C. The mass spectrometer scanned m/z 35–400 at a rate of 4.7 scans s−1. Helium gas was used for desorption and chromatographic analyses. A standard mixture of linear alkanes was also analyzed by GC–MS for the determination of the arithmetic retention index (AI) values of the VOCs. The concentration of each alkane in the working solution was approximately 10 μg mL−1. An aliquot of 1 μL of this working solution was added to a TD tube using a 10 μL-glass syringe. Analysis of the alkane mixture was performed in similar conditions than those described above for the samples. AI values above 800 were determined for all VOCs of the samples based on their retention times and those of the linear alkanes. GC–MS data were processed using the MetAlign–MSClust software pipeline (Lommen, 2009; Tikunov et al., 2012). In brief, MetAlign corrects the baseline and eliminates the noise of each GC–MS output file. Subsequently, it aligns the individual mass peaks in all chromatograms (Lommen, 2009). MSClust then clusters the aligned mass peaks so that mass spectra of putative compounds are reconstructed (Tikunov et al., 2012). Only mass peaks with a retention time within 5–25 min and in the 55–400 m/z range were further processed. VOCs were tentatively annotated by comparing their mass spectra and their experimentally determined AI values with those of the reference Wageningen Mass Spectral Database of Natural Products and NIST library (National Institute of Standards and Technology). An individual mass peak (single ion identification) was selected for each putatively identified VOC, with its intensity across samples relating to the abundance of the compound. Only VOCs from the samples whose peak intensity above the background were accounted as bacterial headspace VOCs and further processed. Compounds origins were checked in the CAS database SciFinder.
Statistical analyses
For count data such as the number of landings, we used generalized linear (mixed) models (GLM or GLMM) with a Poisson distribution and a log link function or negative binomial distribution with a log link function to correct for overdispersion. For continuous data such as time spent in the arena, we used generalized linear (mixed) models with a Gamma distribution with a log link function if the data did not follow a normal distribution. In both cases, the treatment was included in the model as a fixed factor. For post hoc analysis, we used Bonferroni or Tukey’s post-hoc tests. Random factors were selected using a backwards approach; all random factors such as environmental variables like temperature or relative humidity were initially added to the model and removed if not significant (P > 0.05). We analyzed volatile blend composition with cluster analysis using the median per treatment. Data were then log transformed, and range scaled. Clustering was done using Euclidian distances as distance measure and Ward’s clustering criterion as clustering method. Heatmaps were produced with MetaboAnalyst 4.0 with default settings, except we did not use standardization.
Results
Growth curves
Cultures of S. epidermidis, P. aeruginosa, C. minutissimum, and B. epidermidis reached the stationary phase within 24 h (Supplementary Figure S1).
Single species assays
Females of Ae. aegypti landed three times more frequently on the S. epidermidis plate than on sweat medium alone (Figure 2, GLM, χ2 84.541, df 1, P < 0.001). Female mosquitoes also spent nearly four times more time on the bacteria than on the medium (Figure 2, GLM, χ2 8.443, df 1, P = 0.004). There was no effect of the different bacteria species on the number of landings (Figure 3, GLM, χ2 8.443, df 3, P = 0.210). In contrast, the time female mosquitoes spent resting on the arena varied with the treatment (Figure 3, GLMM, χ2 15.285, df 3, P = 0.002). Pairwise comparisons with Tukey’s post-hoc test revealed that females of Ae. aegypti spent less time resting on plates where S. epidermidis grew than on plates with B. epidermidis (GLMM, P = 0.031) and C. minutissimum (GLMM, P = 0.007). Mosquitoes rested equally long on P. aeruginosa plates as they did on B. epidermidis, S. epidermidis, and on C. minutissimum plates (GLMM, P > 0.05).
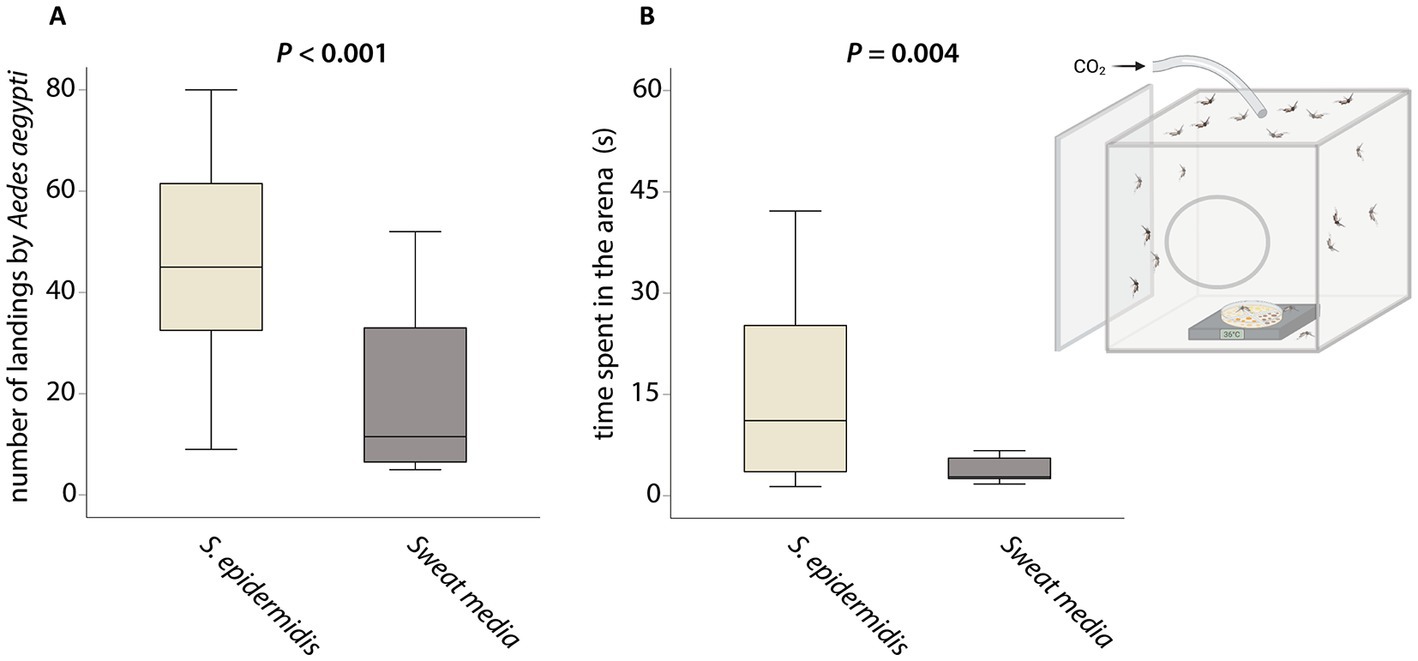
Figure 2. Behavioral responses of Aedes aegypti to Staphylococcus epidermidis versus the sweat media used to grow the bacteria. (A) Number of landings in the arena by Ae. aegypti. (B) Time spent in the arena per individual (s). Behavioral data was analyzed by generalized linear model with a negative binomial distribution, using likelihood function and chi-square test (A) or by generalized linear model with gamma distribution using likelihood function and chi-square test (B). We had eight biological replicates per treatment.
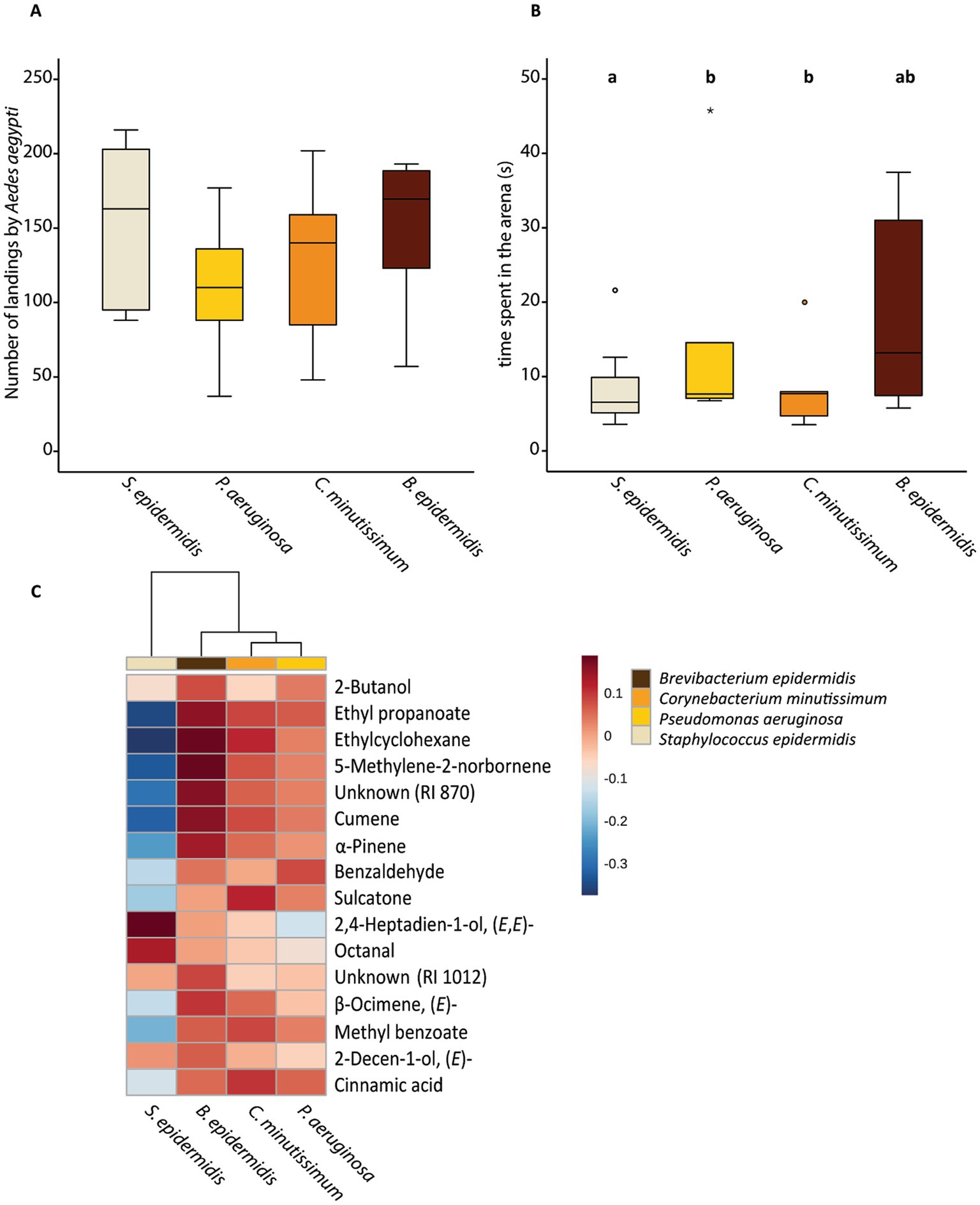
Figure 3. Behavioral responses of Aedes aegypti females to the different bacteria species and emitted volatile compounds per bacteria species (Staphylococcus epidermidis, Pseudomonas aeruginosa, Corynebacterium minutissimum, and Brevibacterium epidermidis). (A) Number of landings in the arena by Ae. aegypti. (B) Time spent resting in the arena per individual (s). (C) Dendrogram and heatmap of the different volatiles emitted by the different bacteria species. Volatile organic compounds appear in the order of elution. Blue shades should be read as traces or absent. Behavioral data was analyzed by generalized linear model with a negative binomial distribution, using likelihood function and chi-square test (A) or by generalized linear mixed model with gamma distribution using likelihood function and chi-square test (B). Tukey’s post-hoc test was used for pairwise comparisons at the 0.05 significance level. Lowercase letters indicate significant differences between treatments at the 0.05 level. Outliers are represented by circles (out) and stars (far out). Dendrogram clustering was performed using Ward’s clustering algorithm with Euclidean distances (C). We had 8 biological replicates per treatment.
Based on clustering analyses, the volatile blends produced by P. aeruginosa and C. minutissimum were the most similar to each other (Figure 3). The volatile profile emitted by S. epidermidis was more distinct from the other bacteria species, particularly from the odor profile of B. epidermidis. Data suggest, for instance, that the volatile compounds 2,4-heptadien-1-ol and octanal were produced in larger amounts by S. epidermidis than by the other species. Sulcatone and benzaldehyde are, on average, produced in lower amounts by S. epidermidis when compared with the other species.
In vitro community models—competitive environment assays
Using four of the most abundant human skin bacterial species, we created in vitro community models to assess whether increased microbial biodiversity could reduce human attractiveness to Ae. aegypti females. More complex bacterial models were generally less attractive to female mosquitoes than the simpler models (Figure 4, GLMM, χ2 277.075, df 5, P < 0.001), but the composition of the bacterial community could increase or decrease mosquito attraction. For instance, female mosquitoes landed three times more often on S. epidermidis alone than on the triple community model (Figure 4, GLMM, P < 0.001). Data demonstrated that the in vitro community model reflecting the skin composition of a highly attractive individual to An. gambiae was indeed more attractive to Ae. aegypti mosquitoes than the community model reflecting the skin composition of a poorly attractive individual to An. gambiae (Figure 4, GLMM, P < 0.001). We did not detect differences between treatments with respect to the time spent resting in the arena (Figure 4, GLMM, χ2 9.636, df 5, P = 0.086).
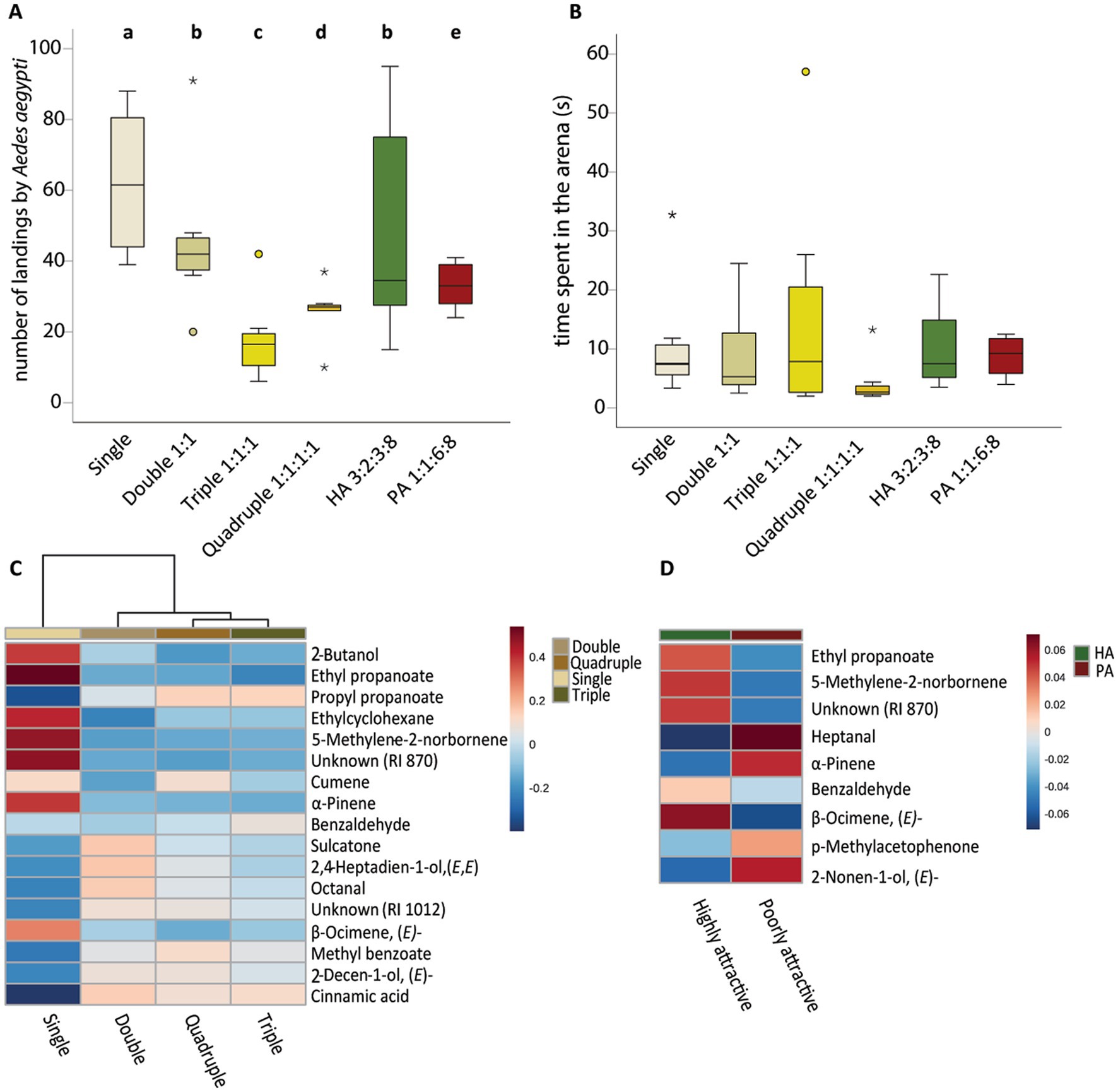
Figure 4. Behavioral responses of Aedes aegypti females to the different in vitro community models and emitted volatile compounds by the different community models, in competitive environments. (A) Number of landings in the arena by Ae. aegypti. (B) Time spent in the arena per individual (s). (C) Dendrogram and heatmap of the different volatiles emitted by the community models with increased complexity. (D) Dendrogram and heatmap of the different volatiles emitted by the community models reflecting the skin bacterial composition of highly and poorly attractive individuals. Volatile organic compounds appear in the order of elution. Blue shades should be read as traces or absent. Staphylococcus epidermidis was the reference control grown as a single model. Double model was composed of S. epidermidis, Pseudomonas aeruginosa in a 1:1 ratio; Triple was composed of S. epidermidis, P. aeruginosa, Corynebacterium minutissimum in a 1:1:1 ratio; Quadruple was composed of S. epidermidis, P. aeruginosa, C. minutissimum, Brevibacterium epidermidis in a 1:1:1:1 ratio; Highly Attractive (HA) community was composed of S. epidermidis, P. aeruginosa, C. minutissimum, B. epidermidis in a 3:3:2:8 ratio and the Poorly Attractive community was composed of S. epidermidis, P. aeruginosa, C. minutissimum, B. epidermidis in a 1:6:1:8 ratio. Behavioral data was analyzed by general linear model with a negative binomial distribution, using likelihood function and chi-square test (A) or by generalized linear model with gamma distribution using likelihood function and chi-square test (B). Tukey’s post-hoc test was used for pairwise comparisons at the 0.05 significance level. Lowercase letters indicate significant differences between treatments at the 0.05 level. Outliers are represented by circles (out) and stars (far out). Dendrogram clustering was performed using Ward’s clustering algorithm with Euclidean distances (C,D). We had 8 biological replicates per treatment.
Based on clustering analyses, the volatile blends produced by the triple and quadruple in vitro community models were the most similar to each other. The volatile profile emitted by S. epidermidis was more distinct from the other models. Blends emitted by the different in vitro community models showed that the more complex models had overall a lower emission. When comparing the volatile blends emitted by highly and poorly attractive individuals, the monoterpenes α-pinene and (E)-β-ocimene were among the compounds contributing to the differences.
In vitro community models—non-competitive environment assays
The number of landings (Figure 5, GLMM, χ2 15.685, df 5, P = 0.008) and time spent resting in the arena of Ae. aegypti females (Figure 5, GLMM, χ2 43.269, df 5, P < 0.001) varied depending on the in vitro community model tested. Pairwise comparisons with Tukey’s post-hoc test show that the mosquitoes landed less often on the control (single S. epidermidis) than on the double (GLMM, P = 0.006), triple (GLMM, P = 0.032) and quadruple (GLMM, P = 0.037) in vitro community models. No difference was detected between the number of landings on plates where the in vitro community models simulated highly and poorly attractive individuals (GLMM, P = 1.000) when no competition between the bacteria was possible.
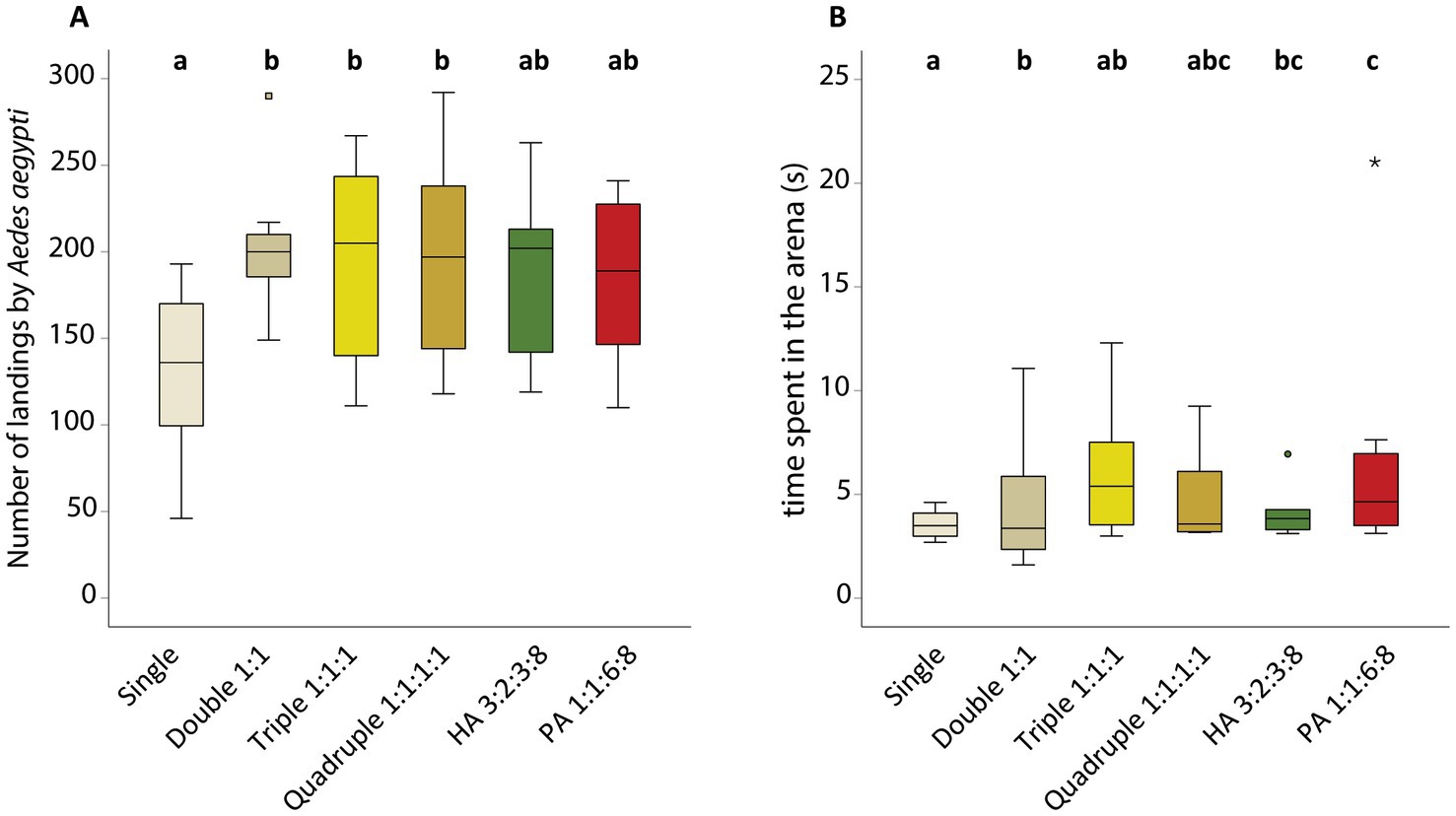
Figure 5. Behavioral responses of Aedes aegypti females to the different in vitro community models and volatile compounds emitted by the different community models, in non-competitive environments. (A) Number of landings in the arena by Ae. aegypti. (B) Time spent in the arena per individual (s). Staphylococcus epidermidis was the reference control grown as a single model. Double was composed of S. epidermidis and Pseudomonas aeruginosa in a 1:1 ratio; Triple was composed of S. epidermidis, P. aeruginosa, and Corynebacterium minutissimum in a 1:1:1 ratio; Quadruple was composed of S. epidermidis, P. aeruginosa, C. minutissimum, and Brevibacterium epidermidis in a 1:1:1:1 ratio; Highly Attractive (HA) community was composed of S. epidermidis, P. aeruginosa, C. minutissimum, and B. epidermidis in a 3:3:2:8 ratio and the Poorly Attractive community was composed of S. epidermidis, P. aeruginosa, C. minutissimum, and B. epidermidis in a 1:6:1:8 ratio. Behavioral data was analyzed by generalized linear mixed model with a negative binomial distribution, using likelihood function and chi-square test (A) or by generalized linear mixed model with gamma distribution using likelihood function and chi-square test (B). Tukey’s post-hoc test was used for pairwise comparisons at the 0.05 significance level. Lowercase letters indicate significant differences between treatments at the 0.05 level. Outliers are represented by circles (out) and stars (far out). We had 8 biological replicates per treatment.
Pairwise comparisons between the different in vitro models showed that the mosquitoes spent more time resting on plates of the poorly attractive community model than on the single (GLMM, P < 0.001), double (GLMM, P = 0.004), and triple (GLMM, P = 0.002) community models. Aedes aegypti females spent less time resting on plates of the single in vitro community models than on the double (GLMM, P = 0.047) and highly attractive (GLMM, P = 0.018) community models. For this parameter, no difference was indicated between the highly and poorly attractive in vitro community model neither (GLMM, P = 0.842).
Discussion
We used four of the most abundant human skin bacterial species to create in vitro community models and to study how microbial biodiversity and competition among skin bacteria species may affect human attractiveness to mosquitoes. Our data reveal that more complex skin bacterial models were up to three times less attractive to Ae. aegypti than the simplest model. Data also suggests that an individual who is highly attractive to An. gambiae (Verhulst et al., 2011, 2013) is likely to be also attractive to Ae. Aegypti given that these in vitro bacterial models were constructed based on the attraction rate of An. gambiae. Effects on mosquito responses greatly differed when the different bacteria species were not co-cultured in a way that they could consume the same resources. This shows that competition for the same resources may matter. Analyses of the volatile blends emitted by the different in vitro community models showed that the more complex models had overall lower emission.
Mosquito responses to the in vitro community models in the so-called competitive environment showed that models with increased complexity were less attractive to Ae. aegypti than the single model with only S. epidermidis. This corresponds with previous findings which demonstrated that people with a higher diversity of bacteria were less attractive to An. gambiae than people with a lower diversity of skin bacteria (Verhulst et al., 2011). Complexity alone cannot explain all our results as even a complex community can be poorly attractive as shown by the comparison of our highly attractive and poorly attractive models. We speculate that the distinct volatile profiles and the difference in attraction between the competitive and non-competitive community models can be explained by competition or commensalism among a diverse range of bacteria species, and also within species (Zhang et al., 2015), which can result in the in-vitro community emitting a different volatile blend. Interestingly, mosquito responses were different when skin bacterial communities were presented in a non-competitive scenario. Females of Ae. aegypti landed more often on the double, triple and quadruple community models than on the control with S. epidermidis as single bacteria species which is the opposite of what was observed in the competitive scenario. Our results show that when bacteria were co-cultured in such a way that they were consuming the same resources, mosquito responses were different than from scenario where such potential for competition for resources was excluded. Mosquitoes were also arrested by odors emitted by the in-vitro communities and the time they spent on the arena was influenced by the bacterial community. In our opinion, such competitive models provide a more realistic view of what happens on the human skin, meaning that skin bacteria coexist with many different species in a heterogeneous environment (Grice and Segre, 2011; Callewaert et al., 2021). Our goal with the so-called non-competitive models was to infer if the different bacteria species were co-cultured and could consume or not the same resources, i.e., the media ingredients, and whether this could alter mosquito responses to the odor blend the community emits. We recognize that we did not completely prevent competition between the bacterial species, but we only prevented potential competition for the main resources (i.e., nutrients in medium) used for growth. The airspace between the bacteria species was shared in the non-competitive environment, and volatiles alone can affect the growth of neighboring microbial species (Tyc et al., 2015; Cordovez et al., 2017; Moisan et al., 2019). Whether via competition or commensalism, our data clearly demonstrates that mosquito responses are different in each of the two scenarios and depending on the microbial community composition.
Our results show that an individual who is highly attractive to An. gambiae is likely to be also attractive to Ae. aegypti. This difference was, however, lost in the non-competitive scenario, and we did not detect differences in mosquito attraction to the communities that mimicked the skin composition of highly and of poorly attractive individuals when potential competition was reduced. Taken together, our results suggest that microbial interactions on the human skin result in cues that mosquitoes use to find us and that these cues may be conserved in two evolutionary distant anthropophilic mosquitoes.
Our results showed that females of Ae. aegypti landed three times more frequently on the bacteria S. epidermidis than on sweat media alone emphasizing that this species is very attractive to Ae. aegypti. Our data also suggests that the highly attractive S. epidermidis is outcompeted, and the volatiles emitted by this species would play a key role in determining attraction of Ae. aegypti and An. gambiae, as showed in previous studies (Verhulst et al., 2011, 2013; Michalet et al., 2019; Martinez et al., 2021; Showering et al., 2022). Co-occurrence by the different bacteria species influences the attraction of Ae. aegypti perhaps by influencing the volatile blend emitted. Indeed, in the present study, volatiles that were produced in larger amounts by S. epidermidis were detected on average in lower amounts by the other more complex models. Our results also showed that females of Ae. aegypti landed three times more frequently on the bacteria S. epidermidis than on sweat media we used to grow the bacteria emphasizing that this species on itself is very attractive to Ae. aegypti.
Ae. aegypti females landed frequently on the four single skin bacteria species tested, and no differences were detected in terms of attraction by any of the single species. Difference in attraction to different species of human skin bacteria have been associated with the mosquito species and their feeding behavior, and authors have considered that the more anthropophilic species would be expected to respond to cues specifically emitted by the human skin (Busula et al., 2015; Michalet et al., 2019). Our results primarily suggest that the human skin bacteria community needs to be considered, and that efficacy of candidate mosquito attractants and repellents should be tested against attractive cues that mimic the mosquito host and include human skin microbes (Menger et al., 2014).
Skin bacteria affect mosquito attraction, here measured with the number of individuals landing on the arena, but also impact the mosquito’s decision whether to remain on the attractive source that mimics a human host, here measured as time spent in the arena. Landing assays with single skin bacteria species also showed that Ae. aegypti were arrested, i.e., spent more time on the surface of S. epidermidis plates than on plates with C. minutissimum or P. aeruginosa. Volatile blends emitted by these bacteria showed that C. minutissimum and P. aeruginosa were most similar, in line with the behavioral responses by the mosquitoes. Sulcatone and benzaldehyde are produced in higher amounts by C. minutissimum and P. aeruginosa than by S. epidermidis, making them candidates to be tested as repellents because those bacterial species are most abundance on the skin of poorly attractive individuals (Verhulst et al., 2011). Indeed, sulcatone, or 6-methyl-5-hepten-2-one, can repel An. gambiae (Logan et al., 2008; Menger et al., 2014), Culex quinquefasciatus (Logan et al., 2010), and Ae. aegypti (Logan et al., 2008, 2010) whereas it seems to be attractive to Cx. pipiens pallens (Tian et al., 2018) and increased the number of Ae. aegypti mosquitoes caught in a trap (Tchouassi et al., 2019). Interestingly, the sulcatone-responsive odorant receptor, Or4, has been linked to the evolution of anthropophily in Ae. aegypti mosquitoes, although the addition of sulcatone alone to non-preferred guinea-pig odor did not increase its attractiveness to Ae. aegypti mosquitoes (McBride et al., 2014). Understanding how sulcatone levels alter mosquito attraction to humans warrants further exploration.
Using four of the most abundant human skin bacterial species, we successfully created in vitro community models, and our results suggest that both microbial biodiversity and potential competition or commensalism between species play a role in determining human attractiveness to mosquitoes. We suggest that higher skin microbial diversity can reduce mosquito attraction to humans; our data reveals that the more complex in vitro community models were up to three times less attractive to Ae. aegypti than the single species model. We argue that by enhancing the relative abundance of such a skin bacterial species on the human skin we could render such individuals less attractive to mosquitoes. Thus, altering the relative composition of human skin microbial commensals likely would reduce mosquito attractiveness to humans. In our view, the main advantage of a probiotic (a topic product based on microbes) is that the odors produced by such bacteria will be continuously produced, offering relatively longer protection to the individual, whereas all the currently available repellents evaporate within hours, requiring frequent reapplication. Such skin bacteria could be potentially incorporated in to traps but methodology would need to be developed to secure that media remains moist, while volatiles can still be released. VOCs produced by bacteria and that are important in determining mosquito attraction could be incorporated into synthetic blends that are used in traps. The outlook of our study is to temporarily alter the skin microbial community to lower the emission of attractive odors emanating from the host. In addition, it is important to consider that some bacteria may produce natural repellents and increasing their relative abundance on human skin would further reduce mosquito attraction to humans. Furthermore, the use of skin bacterial models may reduce the use of human and animal subjects. For example, the efficacy of candidate mosquito repellents could be tested against the background of an attractive source like our community models that mimic the mosquito host. In addition, the models may be used to further understand the attraction of insect vectors to their host and to develop skin probiotics that can lower a person’s attractiveness to insect vectors.
Data availability statement
The raw data supporting the conclusions of this article will be made available by the authors, without undue reservation.
Author contributions
DL-B, NV, AB, JC, AC-d-S, and MD contributed to the study conception and design. Material preparation, data collection and analysis were performed by DL-B and CB. DL-B wrote the first draft of the manuscript. All authors commented on previous versions of the manuscript.
Funding
This material was based upon work supported by the Defense Advanced Research Projects Agency (DARPA) Biological Technologies Office (BTO) (Contract No. HR0011-20-C-0073) and funding by the Swiss National Science Foundation (grant no. 212671).
Acknowledgments
We thank Raffael Stegmayer for all the help with the rearing of Ae. aegypti. We also appreciate the suggestions Alexander Mathis and Kaylee Marrero provided for the manuscript. We also highly appreciate the Swiss Federal Food Safety and Veterinary Office as sponsor of the Swiss National Centre for Vector Entomology.
Conflict of interest
The authors declare that the research was conducted in the absence of any commercial or financial relationships that could be construed as a potential conflict of interest.
Publisher’s note
All claims expressed in this article are solely those of the authors and do not necessarily represent those of their affiliated organizations, or those of the publisher, the editors and the reviewers. Any product that may be evaluated in this article, or claim that may be made by its manufacturer, is not guaranteed or endorsed by the publisher.
Supplementary material
The Supplementary material for this article can be found online at: https://www.frontiersin.org/articles/10.3389/fevo.2023.1156311/full#supplementary-material
References
Busula, A. O., Takken, W., Loy, D. E., Hahn, B. H., Mukabana, W. R., and Verhulst, N. O. (2015). Mosquito host preferences affect their response to synthetic and natural odour blends. Malar. J. 14:133. doi: 10.1186/s12936-015-0635-1
Busula, A. O., Verhulst, N. O., Bousema, T., Takken, W., and de Boer, J. G. (2017). Mechanisms of plasmodium-enhanced attraction of mosquito vectors. Trends Parasitol. 33, 961–973. doi: 10.1016/j.pt.2017.08.010
Byrd, A. L., Belkaid, Y., and Segre, J. A. (2018). The human skin microbiome. Nat. Rev. Microbiol. 16, 143–155. doi: 10.1038/nrmicro.2017.157
Callewaert, C., Knödlseder, N., Karoglan, A., Güell, M., and Paetzold, B. (2021). Skin microbiome transplantation and manipulation: current state of the art. Comput. Struct. Biotechnol. J. 19, 624–631. doi: 10.1016/j.csbj.2021.01.001
Cordovez, V., Mommer, L., Moisan, K., Lucas-Barbosa, D., Pierik, R., Mumm, R., et al. (2017). Plant phenotypic and transcriptional changes induced by volatiles from the fungal root pathogen Rhizoctonia solani. Front. Plant Sci. 8:1262. doi: 10.3389/fpls.2017.01262
DeGennaro, M. (2015). The mysterious multi-modal repellency of DEET. Fly 9, 45–51. doi: 10.1080/19336934.2015.1079360
Dormont, L., Mulatier, M., Carrasco, D., and Cohuet, A. (2021). Mosquito attractants. J. Chem. Ecol. 47, 351–393. doi: 10.1007/s10886-021-01261-2
Grice, E. A., and Segre, J. A. (2011). The skin microbiome. Nat. Rev. Microbiol. 9, 244–253. doi: 10.1038/nrmicro2537
Jha, S. K. (2017). “Characterization of human body odor and identification of aldehydes using chemical sensor,” Reviews in Analytical Chemistry. 36:20160028. doi: 10.1515/revac-2016-0028
Logan, J. G., Birkett, M. A., Clark, S. J., Powers, S., Seal, N. J., Wadhams, L. J., et al. (2008). Identification of human-derived volatile chemicals that interfere with attraction of Aedes aegypti mosquitoes. J. Chem. Ecol. 34, 308–322. doi: 10.1007/s10886-008-9436-0
Logan, J. G., Stanczyk, N. M., Hassanali, A., Kemei, J., Santana, A. E. G., Ribeiro, K. A. L., et al. (2010). Arm-in-cage testing of natural human-derived mosquito repellents. Malar. J. 9:239. doi: 10.1186/1475-2875-9-239
Lommen, A. (2009). MetAlign: interface-driven, versatile metabolomics tool for hyphenated full-scan mass spectrometry data preprocessing. Anal. Chem. 81, 3079–3086. doi: 10.1021/ac900036d
Lucas-Barbosa, D., DeGennaro, M., Mathis, A., and Verhulst, N. O. (2022). Skin bacterial volatiles: propelling the future of vector control. Trends Parasitol. 38, 15–22. doi: 10.1016/j.pt.2021.08.010
Mapossa, A. B., Focke, W. W., Tewo, R. K., Androsch, R., and Kruger, T. (2021). Mosquito-repellent controlled-release formulations for fighting infectious diseases. Malar. J. 20:165. doi: 10.1186/s12936-021-03681-7
Martinez, J., Showering, A., Oke, C., Jones, R. T., and Logan, J. G. (2021). Differential attraction in mosquito-human interactions and implications for disease control. Philos. Trans. R. Soc. B Biol. Sc. 376:20190811. doi: 10.1098/rstb.2019.0811
McBride, C. S., Baier, F., Omondi, A. B., Spitzer, S. A., Lutomiah, J., Sang, R., et al. (2014). Evolution of mosquito preference for humans linked to an odorant receptor. Nature 515, 222–227. doi: 10.1038/nature13964
Meijerink, J., Braks, M. A. H., Brack, A. A., Adam, W., Dekker, T., Posthumus, M. A., et al. (2000). Identification of olfactory stimulants for Anopheles gambiae from human sweat samples. J. Chem. Ecol. 26, 1367–1382. doi: 10.1023/A:1005475422978
Menger, D. J., Van Loon, J. J. A., and Takken, W. (2014). Assessing the efficacy of candidate mosquito repellents against the background of an attractive source that mimics a human host. Med. Vet. Entomol. 28, 407–413. doi: 10.1111/mve.12061
Michalet, S., Minard, G., Chevalier, W., Meiffren, G., Saucereau, Y., Tran Van, V., et al. (2019). Identification of human skin bacteria attractive to the Asian Tiger mosquito. Environ. Microbiol. 21, 4662–4674. doi: 10.1111/1462-2920.14793
Moisan, K., Cordovez, V., van de Zande, E. M., Raaijmakers, J. M., Dicke, M., and Lucas-Barbosa, D. (2019). Volatiles of pathogenic and non-pathogenic soil-borne fungi affect plant development and resistance to insects. Oecologia 190, 589–604. doi: 10.1007/s00442-019-04433-w
Showering, A., Martinez, J., Benavente, E. D., Gezan, S. A., Jones, R. T., Oke, C., et al. (2022). Skin microbiome alters attractiveness to Anopheles mosquitoes. BMC Microbiol. 22:98. doi: 10.1186/s12866-022-02502-4
Takken, W., and Knols, B. G. (1999). Odor-mediated behavior of Afrotropical malaria mosquitoes. Annu. Rev. Entomol. 44, 131–157. doi: 10.1146/annurev.ento.44.1.131
Takken, W., and Verhulst, N. O. (2017). Chemical signaling in mosquito–host interactions: the role of human skin microbiota. Curr. Opin. Insect Sci. 20, 68–74. doi: 10.1016/j.cois.2017.03.011
Tchouassi, D. P., Jacob, J. W., Ogola, E. O., Sang, R., and Torto, B. (2019). Aedes vector-host olfactory interactions in sylvatic and domestic dengue transmission environments. Proc. Biol. Sci. 286:20192136. doi: 10.1098/rspb.2019.2136
Tian, J., Mao, J., Yu, B., Fouad, H., Ga'al, H., Mao, G., et al. (2018). Laboratory and field evaluation of multiple compound attractants to Culex pipiens pallens. J. Med. Entomol. 55, 787–794. doi: 10.1093/jme/tjy015
Tikunov, Y. M., Laptenok, S., Hall, R. D., Bovy, A., and de Vos, R. C. (2012). MSClust: a tool for unsupervised mass spectra extraction of chromatography-mass spectrometry ion-wise aligned data. Metabolomics 8, 714–718. doi: 10.1007/s11306-011-0368-2
Tyc, O., Zweers, H., de Boer, W., and Garbeva, P. (2015). Volatiles in inter-specific bacterial interactions. Front. Virol. 6:1412. doi: 10.3389/fmicb.2015.01412
Verhulst, N. O., Andriessen, R., Groenhagen, U., Bukovinszkiné Kiss, G., Schulz, S., Takken, W., et al. (2010). Differential attraction of malaria mosquitoes to volatile blends produced by human skin bacteria. PLoS One 5:e15829. doi: 10.1371/journal.pone.0015829
Verhulst, N. O., Beijleveld, H., Qiu, Y. T., Maliepaard, C., Verduyn, W., Haasnoot, G. W., et al. (2013). Relation between HLA genes, human skin volatiles and attractiveness of humans to malaria mosquitoes. Infect. Genet. Evol. 18, 87–93. doi: 10.1016/j.meegid.2013.05.009
Verhulst, N. O., Brendle, A., Blanckenhorn, W. U., and Mathis, A. (2020). Thermal preferences of subtropical Aedes aegypti and temperate Ae. japonicus mosquitoes. J. Therm. Biol. 91:102637. doi: 10.1016/j.jtherbio.2020.102637
Verhulst, N. O., Qiu, Y. T., Beijleveld, H., Maliepaard, C., Knights, D., Schulz, S., et al. (2011). Composition of human skin microbiota affects attractiveness to malaria mosquitoes. PLoS One 6:e28991. doi: 10.1371/journal.pone.0028991
Wooding, M., Naude, Y., Rohwer, E., and Bouwer, M. (2020). Controlling mosquitoes with semiochemicals: a review. Parasit. Vectors 13:80. doi: 10.1186/s13071-020-3960-3
Zhang, X. Y., Crippen, T. L., Coates, C. J., Wood, T. K., and Tomberlin, J. K. (2015). Effect of quorum sensing by Staphylococcus epidermidis on the attraction response of female adult yellow fever mosquitoes, Aedes aegypti (Linnaeus) (Diptera: Culicidae), to a blood-feeding source. PLoS One 10:e0143950. doi: 10.1371/journal.pone.0143950
Keywords: human skin bacteria, Aedes (Ae.) aegypti, in vitro communities, volatiles, mosquito
Citation: Lucas-Barbosa D, Balvers C, Bellantuono AJ, Castillo JS, Costa-da-Silva AL, De Moraes CM, DeGennaro M and Verhulst NO (2023) Competition matters: using in vitro community models to study the impact of human skin bacteria on mosquito attraction. Front. Ecol. Evol. 11:1156311. doi: 10.3389/fevo.2023.1156311
Edited by:
Panagiotis Milonas, Benaki Phytopathological Institute, GreeceReviewed by:
Spyros Zographos, National Hellenic Research Foundation, GreeceStefan Schulz, Technical University of Braunschweig, Germany
Copyright © 2023 Lucas-Barbosa, Balvers, Bellantuono, Castillo, Costa-da-Silva, De Moraes, DeGennaro and Verhulst. This is an open-access article distributed under the terms of the Creative Commons Attribution License (CC BY). The use, distribution or reproduction in other forums is permitted, provided the original author(s) and the copyright owner(s) are credited and that the original publication in this journal is cited, in accordance with accepted academic practice. No use, distribution or reproduction is permitted which does not comply with these terms.
*Correspondence: Dani Lucas-Barbosa, ZGFuaS5sdWNhcy1iYXJib3NhQHZldHBhcmFzLnV6aC5jaA==; ZGFuaS5sdWNhcy1iYXJib3NhQGZpYmwub3Jn
†ORCID: Dani Lucas-Barbosa https://orcid.org/0000-0003-3440-1040
Consuelo M. De Moraes https://orcid.org/0000-0001-6737-9842
Niels O. Verhulst https://orcid.org/0000-0002-1106-9711