- 1School of Geography and Ecotourism, Southwest Forestry University, Kunming, Yunnan, China
- 2Southwest Research Center for Eco-civilization, National Forestry and Grassland Administration, Kunming, Yunnan, China
- 3College of Biodiversity Conservation and Utilization, Southwest Forestry University, Kunming, Yunnan, China
Alpine grassland ecosystem supports high diversity of terrestrial flora and fauna species. Despite the ecological importance and economic potential of this unique ecosystem type, it experiences increasing anthropogenic disturbances such as trampling, which impose negative impact on the health and integrity of alpine grasslands. Previous studies of trampling impact on alpine vegetation mainly focus on changes in vegetation cover and taxonomic diversity after trampling disturbance, but rarely test community-level responses of alpine vegetation to trampling from a functional trait perspective. Through the lens of vegetation functional traits, the present study evaluates the impacts of simulated trampling on typical alpine grasslands in Shangri-la, China. The results showed that although increased trampling intensity did not always lead to changes in functional diversity across all three experimental sites, characteristics of community-weighted mean trait values had consistently changed toward plant species with shorter height, reduced leaf area and lower leaf dry matter content, and such strong shifts in functional attributes may further affect ecosystem goods and services provided by alpine grasslands. Therefore, a functional trait approach can help us better understand the mechanisms that drive trait changes, function shifts and vegetation stability following anthropogenic disturbances.
1. Introduction
With the continuous development of human societies, people’s quality of life has been greatly improved. However, accelerating pace of life and rapid changes in lifestyle also affects our physical and mental health in a negative way. Meanwhile, industrialization and urbanization has caused severe environmental problems, and threatened human health and wellbeing (Farhud, 2015). Therefore, people crave a close contact with nature through recreational and tourism activities, and nature-based tourism is booming worldwide.
Recreational and tourism activities, however, are causing disturbances in natural ecosystems (Pickering and Hill, 2007; Memoli et al., 2019), such as the pollution of air, water, and soil (Zeng et al., 2021; Xiong et al., 2022), the loss of biodiversity (Habibullah et al., 2016), and the degradation of ecosystem functions and services (Pueyo-Ros, 2018). In particular, enormous visitation and tourist activities pose an urgent threat to alpine grasslands, which are known for spectacular scenes, high endemic diversity, irreplaceable social and cultural values, and extreme vulnerability (Körner, 1999; Argenti et al., 2020).
In recent years, measuring the impacts of anthropogenic activities on grassland ecosystems has received increasing attention, and a large number of studies are conducted in temperate grasslands, which focus on the vulnerability of plants using measures of resistance and resilience (Pickering and Hill, 2007; Prescott and Steward, 2022). However, we know less about the impacts of anthropogenic activities on alpine grasslands. Particularly, studies that examine the responses of alpine vegetation to disturbance from a functional trait perspective are very limited. Plant functional traits are defined as the characteristics of plants that affect overall plant fitness via their effects on plant growth, reproduction and survival, and varying driving forces cause functional trait differences (Violle et al., 2007; Carmona et al., 2016). For example, competitive pressure selects for functional traits associated with competitiveness, whereas environmental stresses filter out species with low resistance traits (Reich, 2014; Díaz et al., 2016). Therefore, an analysis of changes in characteristics of disturbed communities can help us better understand the relationship between plant functional traits and disturbances, and offer a mechanistic explanation of disturbance processes and impacts.
Actually, by assessing the links between plant functional traits and disturbances, we can not only capture the response signals of plant communities to anthropogenic disturbances in a timely manner, but also quantify functional diversity, which reflects the value, range, and distribution of functional traits of organisms within a given community (Mouchet et al., 2010; Mouillot et al., 2013). However, such community-level changes may not be reflected in some conventional indexes used to characterize ecological communities, such as the total number of species within a community (specie richness), despite the fact that this community has undergone functional changes after disturbance (Li et al., 2021; Smith et al., 2022). Beside the obvious advantage of using functional diversity to reflect the breadth of the functional space occupied by species in a community, functional diversity provides a more effective way to describe community diversity (Mason et al., 2005), establishes a link between the community and environment (Laureto et al., 2015), and influences the provisioning of ecosystem functions and services (Dı́az and Cabido, 2001; Suding and Goldstein, 2008). Therefore, the use of functional diversity can help facilitate our better understanding of the impacts of anthropogenic activities on plant communities at different organizational levels (e.g., individual, population, community and ecosystem levels), and reveal the complex nature of changes in disturbed communities (Vandewalle et al., 2010; Mouillot et al., 2013).
Located on the southeastern edge of the Tibetan Plateau, Shangri-La, Yunnan Province, China, is dotted with vast alpine grasslands. With high esthetic values, these alpine grasslands have special appeal to urban residents. Each year, tourists travel across the country to enjoy Shangri-La’s beautiful and spectacular grassland scenery. As an important natural resource, alpine grasslands exert an increasingly important role in promoting Shangri-La’s socio-economic development. However, the negative effects brought by tourism are hard to miss. For example, as tourists are admiring the lush and charming alpine grasslands, their hiking behavior inevitably causes disturbance, or even damage to the alpine vegetation. In recent years, although local government and relevant organizational authorities have started to direct more attention to the conservation of alpine grasslands, studies that address the impacts of anthropogenic disturbance on alpine vegetation are still very limited. Particularly, little information is available regarding the impacts of trampling disturbance on alpine grasslands from a functional trait perspective.
Through the lens of vegetation functional traits, the present study evaluates the impacts of simulated trampling on typical alpine grasslands in Shangri-La. Also, we examine the conditions of soil moisture, one type of habitat heterogeneity, across three experimental sites as trampling impacts over vegetation may vary with soil moisture conditions. Our study provides an assessment of trampling disturbance on plant traits and functional biodiversity of alpine vegetation, which complement previous studies that focus on the responses of temperate grasslands to disturbances using measures of resistance and resilience, or solely monitor changes in taxonomic diversity metrics (e.g., species richness) that may only capture part of the mechanisms of disturbance. With the aim of providing some scientific evidence for the conservation and management of alpine grasslands in Shangri-La, we specifically address the following questions: (1) How would functional traits of alpine grasslands change after disturbance? (2) How would functional diversity of alpine grassland change after disturbance? (3) Whether the impact of trampling on alpine vegetation is also affected by soil moisture conditions? We hypothesized that trampling disturbance would significantly affect the functional traits and diversity of alpine vegetation, and high soil moisture would increase the magnitude of trampling impact in addition to high trampling intensity.
2. Methods
Within the territory of Yunnan Province, high-altitude alpine grasslands are mainly distributed in Shangri-La, one of the most important biodiversity conservation areas in China. Shangri-La experiences typical plateau climate. Average annual precipitation is 606 mm, and average monthly temperature ranges from −3.7°C in January to 13.2°C in July (Tian, 2010). We conducted our study at three experimental sites, namely, Bitahai site (27°48′N, 99°59′E, 3560 m a.s.l), Lanyuegu site (27°48′N, 99°39′E, 3270 m a.s.l) and Pudacuo site (27°45′N, 99°56′E, 3460 m a.s.l; Figure 1). Within each site, alpine vegetation is generally distributed in homogeneous landscapes, and initial vegetation cover in the experimental area is close to 100%.
We conducted simulated trampling experiment following the standardized protocol developed by Cole and Bayfield (1993) to quantify the impacts of trampling disturbance on functional traits and diversity of alpine vegetation. Four experimental blocks were established in the uniform vegetation at each site. Each experimental block consisted of five trampling plots (0.5 m wide and 2.0 m long) separated by 0.5 m wide buffer zones. Following the standardized experimental design by Cole, Bayfield and other researchers (Cole and Bayfield, 1993; Monz, 2002; Roovers et al., 2004; Dumitraşcu et al., 2017), different treatments (25, 75, 250, and 500 passes) were randomly assigned to four plots, leaving one plot as an undisturbed control plot that receives no pedestrian pressure. The trampling experiment was conducted once on a typical sunny summer day. As the weight of the five research participants varied between 60 and 78 kg, each of them completed 5, 15, 50, and 100 passes, respectively, corresponding to treatment lanes assigned to 25, 75, 250, and 500 passes. Each research participant walked at a natural gait, with a completion of 2-m walk counted as one pass.
The first field measurement was performed immediately before the trampling experiment was conducted. Within each plot, species identity, species density, and vegetation coverage were measured and recorded. Same measurement procedures were repeated half a month later after trampling disturbance. Due to the fact that some plant species with small population size are distributed sporadically, we only made measurements of plants that occurred in at least three replicate plots that received the same trampling treatment. Also, a soil profile of 20 cm deep was dug to measure soil moisture conditions at each site, with five representative soil samples randomly collected at this depth. The three experimental sites differed considerably in regard to soil gravimetric water content, among which Bitahai site and Lanyuegu site had the highest and lowest water content, respectively (Figure 2).
To have a clear understanding of the impacts of trampling disturbance on functional traits and diversity of alpine vegetation, we measured plant height, leaf area and leaf dry matter content following the standardized protocols (Garnier et al., 2001; Cornelissen et al., 2003). These traits can reflect the sensitivity (e.g., plant height and leaf area) and resistance (e.g., leaf dry matter content) of plants to physical disturbance (Table 1), and their measurements are relatively independent (Lavorel and Garnier, 2002; Mason et al., 2003; Suding et al., 2008). Plant height was measured directly using a ruler, and four individuals of each species were used to measure plant height. For the measurement of foliar traits, we selected young, intact leaves without the appearance of mechanical damage, as the traits of these leaves can reflect the capacity of alpine plants for acclimation in response to trampling disturbance. For each plant species, 3–5 young, intact but fully expanded leaves were collected to measure leaf area and leaf dry matter content. To avoid dehydration, collected leaf samples were wrapped into damp paper towels, sealed in labeled plastic bags, stored in a heat-insulated container with ice packs, and transported to the laboratory for further analysis. Fresh leaves were first rehydrated for 6 h, then dried to remove surface water and weighted immediately to determine their saturated fresh mass using a sensitive balance. Their dry mass was measured after being oven-dried at 60°C for 72 h. The fully expanded leaf was scanned to measure leaf area, and leaf dry matter content was quantified as the ratio of leaf dry mass to leaf saturated fresh mass. Wet mass of soil samples were determined by the difference between the moist soil and the soil dried at 105°C for 24 h, and gravimetric water content is quantified as the mass of water per mass of dry soil.
The community-weighted mean trait values for each trait were calculated for every sample using species mean trait values and species relative abundance, or, in other words, community-weighted mean (CWM) trait values are plot-level trait values weighted by species abundance (Garnier et al., 2004). As to functional trait-based diversity metrics, three functional diversity metrics, including functional richness, functional evenness and functional divergence, were used to collectively quantify the range, distribution and relative abundance of functional traits within a community (Mason et al., 2003; Villeger et al., 2008). Functional richness represents the total amount of functional space filled by the community, and is calculated as the minimum convex hull volume that includes all the species in the community. Functional evenness represents the evenness of species abundance distributions in functional trait space, and is calculated as the average branch length of the minimum-spanning tree that connects all the species in trait space, weighted by each species’ relative abundance. Functional divergence measures how species are distributed within trait space and is calculated as an individual species’ deviation from the mean distance of all species to the centroid of the convex hull of the community (Kuebbing et al., 2018). We also calculated percentage changes (%) in functional traits and diversity of alpine vegetation due to trampling disturbance as changes in functional values of plots receiving trampling treatment plot divided by functional values of the control plots and then multiplied by 100. We speculated that the impact of trampling disturbance on functional traits and diversity of alpine vegetation was stronger in sites with higher soil moisture content, so were percentage changes in these functional values.
All statistical analyses were conducted in the R statistics 3.4.0 platform (R Core Team, 2017). One-way analysis of variance (ANOVA) was used to analyze the impact of trampling disturbance on functional traits and diversity of alpine vegetation, and multiple comparisons were performed using Tukey’s HSD test. To correct for variance heterogeneity and provide heteroscedasticity-consistent estimations of the covariance matrix, a sandwich estimator was applied (Hothorn et al., 2008). FD package was used to calculate functional diversity metrics (Laliberté et al., 2014).
3. Results
Trampling disturbance imposed significant influence over CWM trait values of alpine vegetation at Bitahai site. With an increase in trampling intensity, CWM trait values of plant height (F4,15 = 114.5, p < 0.001), leaf area (F4,15 = 15.83, p < 0.001) and leaf dry matter content (F4,15 = 6.5, p = 0.003) all decreased (Figure 3 and Table 2), among which CWM trait values of plant height and leaf area were significantly lower in plots receiving 250 or 500 passes than other plots, and CWM trait values of leaf dry matter content were significantly lower in plots receiving 500 passes than other plots. Trampling disturbance also imposed significant influence over functional diversity of alpine vegetation at Bitahai site, although its impacts on different functional diversity metrics varied. With an increase in trampling intensity, functional richness (F4,15 = 8.59, p < 0.001) decreased, with the highest and lowest functional richness occurring in plots receiving 0 and 500 passes, respectively (Figure 4 and Table 3). By contrast, functional evenness (F4,15 = 13.99, p < 0.001) and functional divergence (F4,15 = 10.25, p < 0.001) increased with increasing trampling intensity, among which functional evenness was significantly lower in control plots than other plots, and functional divergence was significantly higher in plots receiving 250 or 500 passes than other plots.
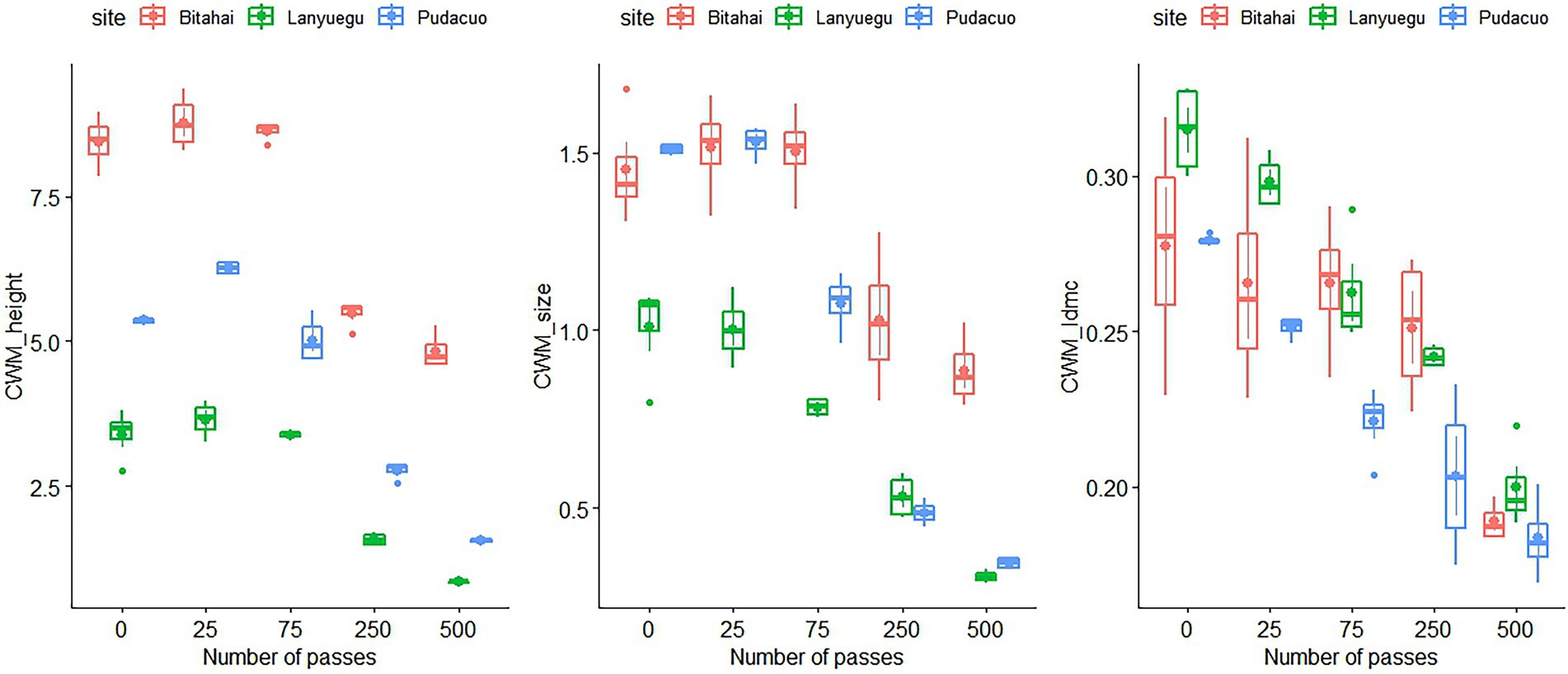
Figure 3. The impacts of trampling disturbance on functional traits of alpine vegetation at three experimental sites. CWM_height, community weighted mean of plant height; CWM_size, community weighted mean of leaf size; CWM_ldmc, community weighted mean of leaf dry matter content.
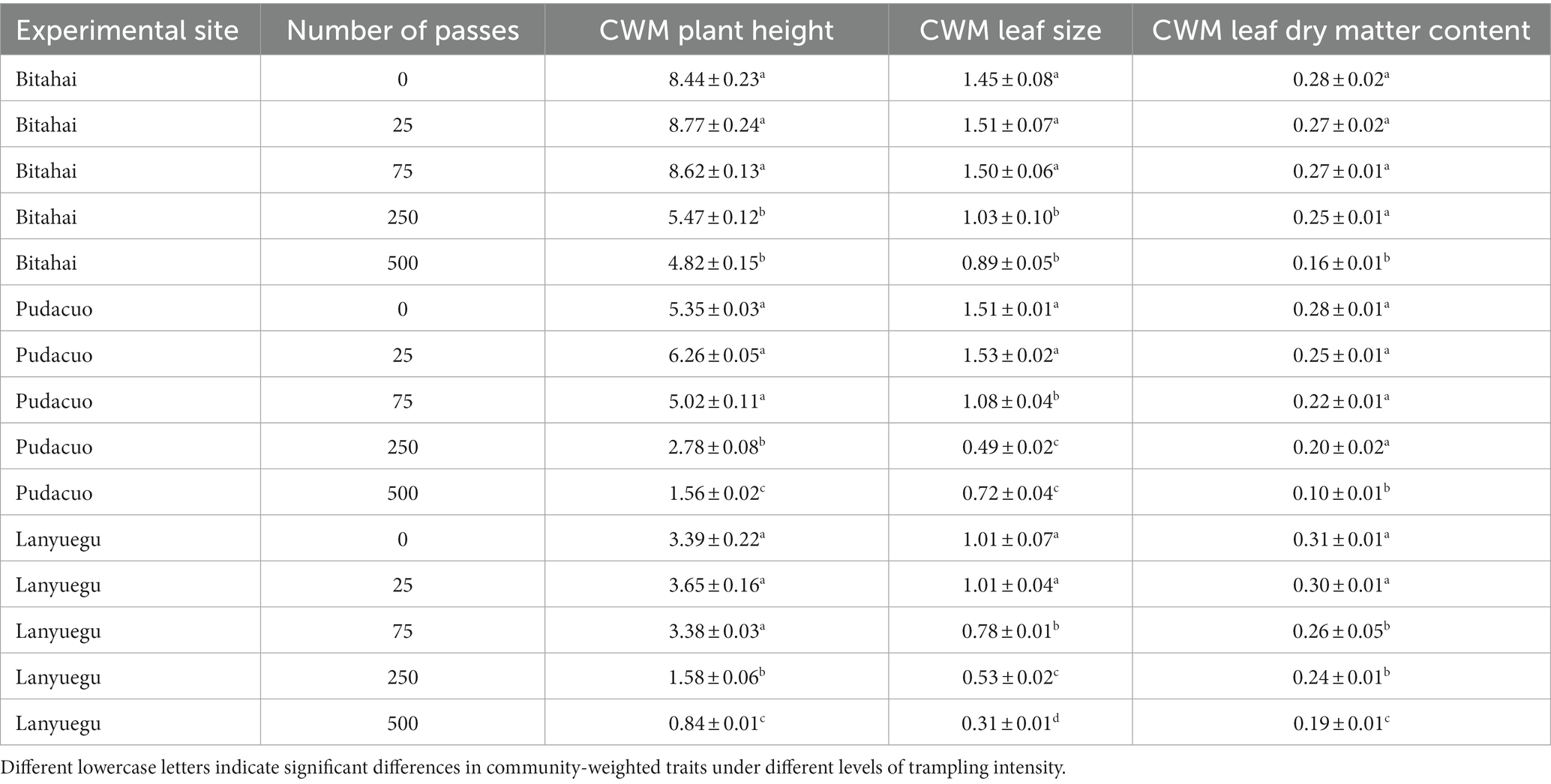
Table 2. The impacts of trampling disturbance on community-weighted traits of alpine vegetation at three experimental sites.
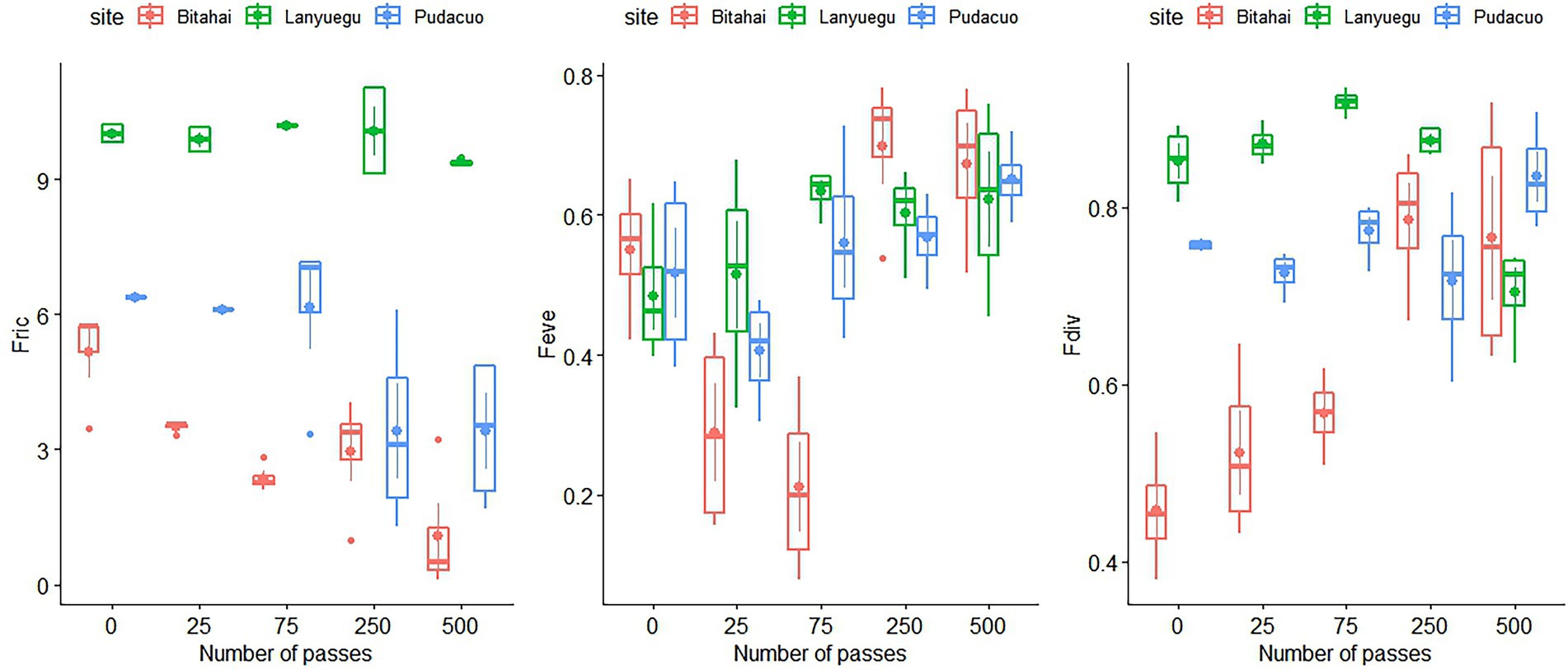
Figure 4. The impacts of trampling disturbance on functional diversity metrics of alpine vegetation at three experimental sites. Fric, functional richness; Feve, functional evenness; Fdiv; functional divergence.
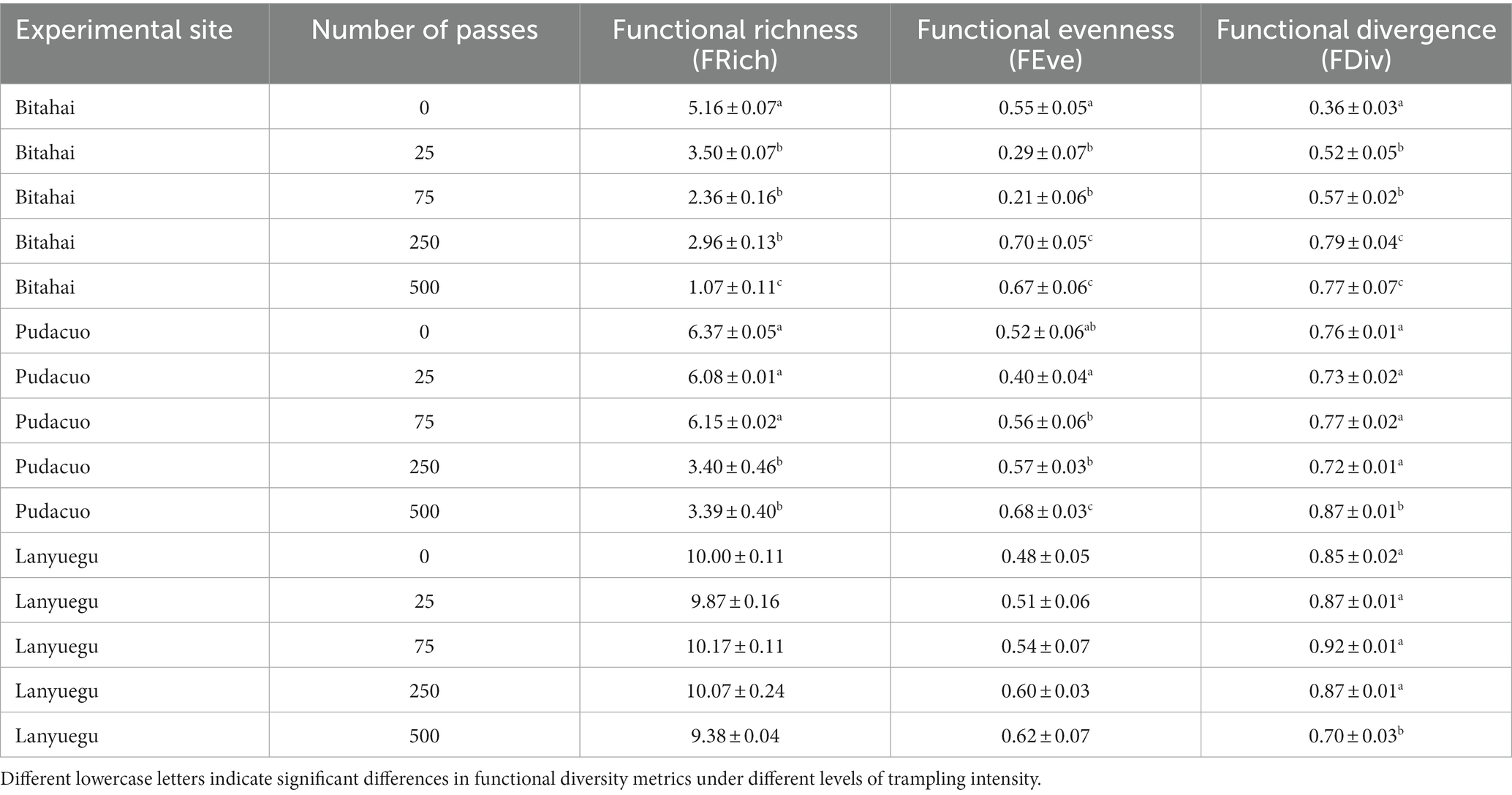
Table 3. The impacts of trampling disturbance on functional diversity metrics of alpine vegetation at three experimental sites.
Trampling disturbance imposed significant influence over CWM trait values of alpine vegetation at Pudacuo site. With an increase in trampling intensity, CWM trait values of plant height (F4,15 = 374.8, p < 0.001), leaf area (F4,15 = 585.8, p < 0.001) and leaf dry matter content (F4,15 = 29.13, p < 0.001) all decreased (Figure 3 and Table 2), among which CWM trait values were significantly lower in plots receiving 500 passes than other plots. Trampling disturbance also imposed significant influence over functional diversity of alpine vegetation at Pudacuo site, although its impacts on different functional diversity metrics varied. With an increase in trampling intensity, functional richness (F4,15 = 4.32, p = 0.02) decreased, and plots receiving 250 or 500 passes had the lowest functional richness (Figure 4 and Table 3). By contrast, functional evenness (F4,15 = 16.17, p < 0.001) and functional divergence (F4,15 = 3.48, p < 0.001) increased with increasing trampling intensity, among which both functional evenness and divergence was significantly higher in plots receiving 500 passes than other plots.
Trampling disturbance imposed significant influence over CWM trait values of alpine vegetation at Lanyuegu site. With an increase in trampling intensity, CWM trait values of plant height (F4,15 = 103.7, p < 0.001), leaf area (F4,15 = 55.07, p < 0.001) and leaf dry matter content (F4,15 = 50.63, p < 0.001) all decreased (Figure 3 and Table 2), among which CWM trait values were significantly lower in plots receiving 500 passes than other plots. For Lanyuegu site, neither functional richness (F4,15 = 1.41, p = 0.28) nor functional evenness (F4,15 = 1.61, p = 0.22) differed significantly among treatment plots. By contrast, trampling disturbance significantly affected functional divergence (F4,15 = 24.59, p < 0.001), and plots receiving 500 passes had lower functional divergence than other plots (Figure 4 and Table 3).
Percentage changes in functional diversity metrics were statistically significant among three sites (for changes in functional richness, F2,57 = 10.05, p < 0.001; for changes in functional evenness, F2,57 = 4.03, p = 0.02; for changes in functional divergence, F2,57 = 15.02, p < 0.001), with the highest and the lowest percentage changes in functional diversity metrics occurring in Bitahai site (with the highest soil moisture content) and Lanyuegu site (with the lowest soil moisture content), respectively (Figure 5). By contrast, percentage changes in functional traits did not differ significantly among three experimental sites.
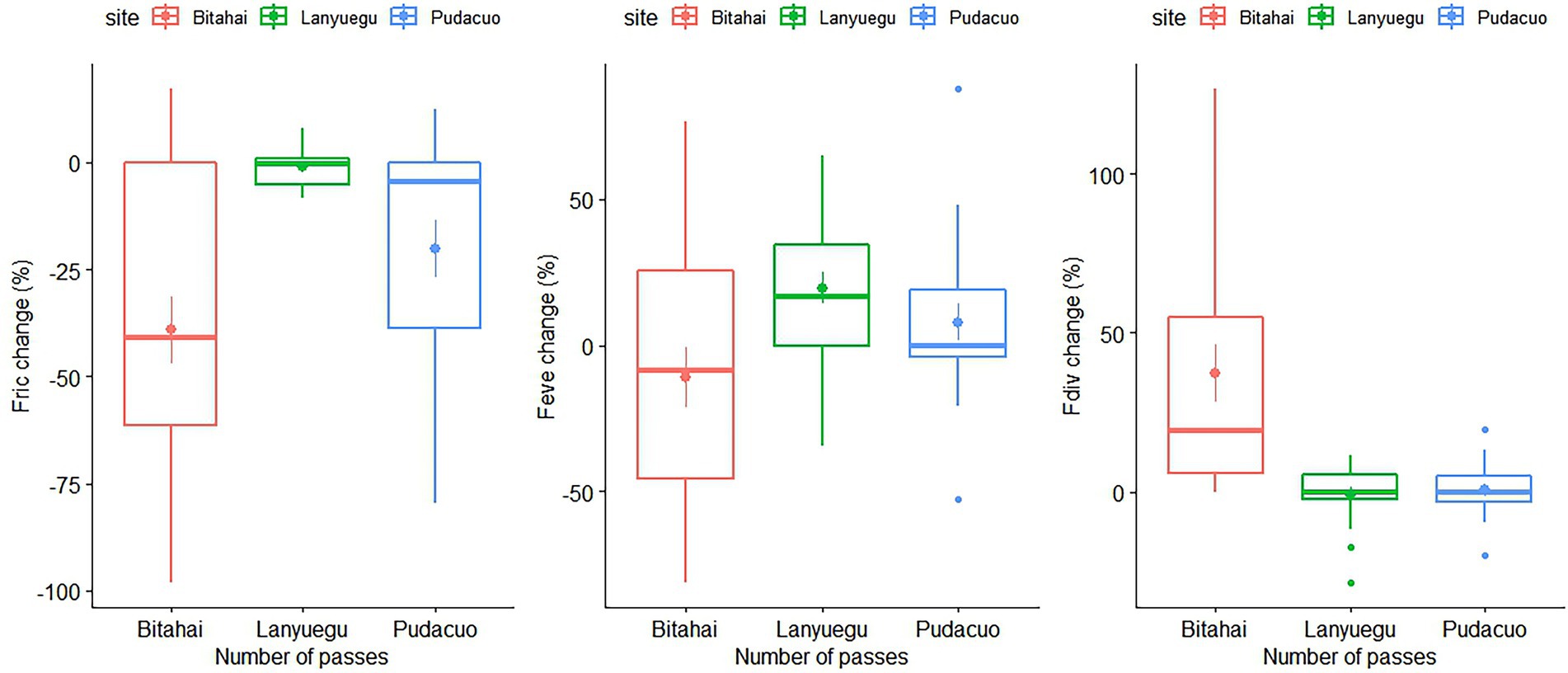
Figure 5. Percentage changes in functional diversity metrics of alpine vegetation at three experimental sites, among which Bitahai site had the highest water content, Lanyuegu site had the lowest water content, and that of Pudacuo site was in between.
4. Discussion
It can be seen from our findings that trampling disturbance significantly affected the functional traits of alpine vegetation. For example, trait characteristics measured in three experimental sites, including plant height, leaf area and leaf dry matter content, all changed after trampling disturbance. In other words, disturbed vegetation consistently exhibited shortened height, smaller leaf area and lower leaf dry matter content. The reduction in plant height and leaf size reflects the effect of mechanical damage to plant tissues by trampling, which compromises photosynthesis efficiency and community productivity. Meanwhile, the decrease in leaf dry matter content suggests that alpine plants might have put low investment in foliar defense after disturbance in order to facilitate recovery. Obviously, changes in functional traits of alpine vegetation reflect the filtering effects of trampling disturbance, which mainly select disturbance-resistance traits, and our findings are in line with several other studies (Pickering and Hill, 2007; Prescott and Stewart, 2022; Smith et al., 2022).
Trampling disturbance also significantly affected functional diversity metrics of alpine communities, although disturbance impacts on different attributes of functional diversity varied. For example, both functional evenness and divergence increased with trampling intensity in Bitahai and Pudacuo experimental sites. An increase in functional evenness after disturbance suggests that the functional distances between species became more even in disturbed plots, probably because disturbance had interrupted resource monopolization by dominant species (Mason et al., 2005). Similarly, an increase in functional divergence after disturbance indicates that competitive pressure is temporarily reduced, and a high degree of niche differentiation exists between species (Eler et al., 2018). By contrast, functional divergence decreased with trampling intensity in Lanyuegu site, suggesting an increase in functional redundancy, or functional homogenization after disturbance (Smith et al., 2022).
In the present study, soil moisture conditions may help explain the varying impacts of trampling disturbance on alpine vegetation across three experimental sites, reflected by a higher degree of percentage changes in functional diversity at Bitahai site, which has a relatively high level of soil water content among all three experimental sites, whereas Lanyuegu site has the lowest soil moisture among these three. Therefore, trampling disturbance might have imposed stronger mechanical stress on plants living at Bitahai site with damp soil environment than plants living at Lanyuegu site with drier soil conditions. One likely explanation is that under wet soil conditions, a pedestrian’s feet tend to sink into the soil, and consequently the structural damage of the footstep to plant tissues is considerably greater than on more compact, dry soils (Gremmen et al., 2003; Conradi et al., 2015). However, percentage changes in functional traits were not associated with soil moisture conditions. This is probably because sources of variation in functional traits are complex, such as species composition, genetic diversity, phenotypic plasticity, geographical and environmental variation (Fyllas et al., 2020), which may mask changes in functional traits caused by trampling disturbance. By contrast, changes in functional diversity may reflect changes of communities in response to disturbance in a more persistent and nuanced manner (Mouillot et al., 2013; Smith et al., 2022). Given that current studies that explicitly test how functional traits and local environment co-determine vegetation responses to disturbances are still very limited, this is a promising area of future research that merits more attention.
Although alpine grasslands exhibit certain resistance to trampling disturbance, excessive levels of trampling can lead to the reduction of vegetation cover, the loss of sensitive endemic species, and the degradation of goods and ecosystems services (Li et al., 2018; Berauer et al., 2019). For example, endemic species with small population size and low resistance are particularly vulnerable to trampling, and thus face the highest risk of extinction (Nomoto and Alexander, 2021). Therefore, anthropogenic disturbances such as extensive trampling can impoverish biological diversity of alpine grasslands, and further dismantle important ecosystem functions (e.g., carbon sequestration, nutrient cycling regulation, soil fertility maintenance), and lead to the collapse of essential ecosystem services (e.g., water quality improvement, food and energy supply) that sustain human society (Verrall and Pickering, 2020). It is worth noting that alpine grasslands are known for high esthetic values, one key component of cultural ecosystem service. However, dramatic reduction in vegetation cover due to trampling disturbance can severely degrade alpine grasslands valued for their esthetic qualities, and increase the risk of loss of touristic attractiveness (Mameno et al., 2022).
The negative impacts of trampling disturbance on alpine grasslands often occur in a short time frame, but the recovery of vegetation after disturbance can take a long time due to the harsh environmental conditions, short growing seasons, and slow rate of biological processes that characterize these high-altitude areas (Willard et al., 2007; Vloon et al., 2022). Therefore, we need to adopt an active protection and management approach, because preventive measures to avoid or mitigate the negative impacts of tourism-related disturbance on alpine vegetation are far more effective than ecological restoration that aims to assist the recovery of alpine ecosystems that have been degraded, damaged, or destroyed. Only on the basis of scientific management can people maintain the health and integrity of alpine grasslands, and utilize alpine natural resources in a reasonable and sustainable way.
In conclusion, trampling disturbance lead to changes in functional traits and diversity of alpine vegetation, and shifts in the functional trait space of the disturbed communities could further alter the provisioning of ecosystem services by alpine grasslands. Therefore, the conservation of high-altitude alpine grasslands with high sensitivity and vulnerability is urgent, especially as multiple environmental and climatic stressors nowadays are bringing unprecedented pressure on them.
Data availability statement
The original contributions presented in the study are included in the article/supplementary material, further inquiries can be directed to the corresponding author.
Author contributions
WL: conceptualization. WL, XPC, and SQH: methodology. WL and TDZ: validation. WL and SQH: formal analysis. WL and XPC: resources. WL, TDZ, and SQH: writing – original draft preparation. WL and SQH: writing – review and editing. All authors have read and agreed to the published version of the manuscript.
Funding
This study was supported by the National Natural Science Foundation of China (31460158 and 31760175).
Acknowledgments
We would like to thank the editor and two reviewers for their constructive comments and suggestions, which helped to improve the quality of the manuscript.
Conflict of interest
The authors declare that the research was conducted in the absence of any commercial or financial relationships that could be construed as a potential conflict of interest.
Publisher’s note
All claims expressed in this article are solely those of the authors and do not necessarily represent those of their affiliated organizations, or those of the publisher, the editors and the reviewers. Any product that may be evaluated in this article, or claim that may be made by its manufacturer, is not guaranteed or endorsed by the publisher.
References
Argenti, G., Stagliano, N., and Bellini, E. (2020). Environmental and management drivers of alpine grassland vegetation types. Ital. J. Agron. 15, 156–164. doi: 10.4081/ija.2020.1600
Berauer, B., Wilfahrt, P., Arfin-Khan, M., Eibes, P., Von Heßberg, A., Ingrisch, J., et al. (2019). Low resistance of montane and alpine grasslands to abrupt changes in temperature and precipitation regimes. Arct. Antarct. Alp. Res. 51, 215–231. doi: 10.1080/15230430.2019.1618116
Carmona, C., De Bello, F., and Mason, N. (2016). Traits without borders: integrating functional diversity across scales. Trends Ecol. Evol. 31, 382–394. doi: 10.1016/j.tree.2016.02.003
Cole, D. N., and Bayfield, N. G. (1993). Recreational trampling of vegetation: standard experimental procedures. Biol. Conserv. 63, 209–215. doi: 10.1016/0006-3207(93)90714-C
Conradi, T., Strobl, K., and Wurfer, A. (2015). Impacts of visitor trampling on the taxonomic and functional community structure of calcareous grassland. Appl. Veg. Sci. 18, 359–367. doi: 10.1111/avsc.12164
Cornelissen, J., Lavorel, S., and Garnier, E. (2003). A handbook of protocols for standardized and easy measurement of plant functional traits worldwide. Aust. J. Bot. 51, 335–380. doi: 10.1071/BT02124
Díaz, S., Kattge, J., Cornelissen, J. H., Wright, I. J., Lavorel, S., and Dray, S. (2016). The global spectrum of plant form and function. Nature 529, 167–171. doi: 10.1038/nature16489
Dı́az, S., and Cabido, M. (2001). Vive la différence: plant functional diversity matters to ecosystem processes. Trends Ecol. Evol. 16, 646–655. doi: 10.1016/S0169-5347(01)02283-2
Dumitraşcu, M., Elena, P., Țîbîrnac, M., Andrei, M., and Vădineanu, A. (2017). Trampling effects on vegetation composition in Romanian LTSER sites. Environ. Eng. Manage. J. 16, 2451–2459. doi: 10.30638/eemj.2017.253
Eler, K., Kermavnar, J., Marinsek, A., and Kutnar, L. (2018). Short-term changes in plant functional traits and understory functional diversity after logging of different intensities: a temperate fir-beech forest experiment. Ann. For. Res. 61, 223–241. doi: 10.15287/afr.2018.1192
Fyllas, N., Michelaki, C., and Galanidis, A. (2020). Functional trait variation among and within species and plant functional types in mountainous Mediterranean forests. Front. Plant Sci. 11:212. doi: 10.3389/fpls.2020.00212
Garnier, E., Cortez, J., Billès, G., Navas, M., and Roumet, C. (2004). Plant functional markers capture ecosystem properties during secondary succession. Ecology 85, 2630–2637. doi: 10.1890/03-0799
Garnier, E., Shipley, B., and Roumet, B. (2001). Standardized protocol for the determination of specific leaf size and leaf dry matter content. Funct. Ecol. 15, 688–695. doi: 10.1046/j.0269-8463.2001.00563.x
Gremmen, J., Smith, V., and van Tongeren, O. (2003). Impact of trampling on the vegetation of subantarctic Marion island. Arct. Antarct. Alp. Res. 35, 442–446. doi: 10.1657/1523-0430(2003)035[0442:IOTOTV]2.0.CO;2
Habibullah, M., Din, B., Chong, C., and Radam, A. (2016). Tourism and biodiversity loss: implications for business sustainability. Procedia Econ. 35, 166–172. doi: 10.1016/S2212-5671(16)00021-6
Hothorn, T., Bretz, F., and Westfall, P. (2008). Simultaneous inference in general parametric models. Biom. J. 50, 346–363. doi: 10.1002/bimj.200810425
Körner, C. (1999). Alpine plant life: Functional plant ecology of high mountain ecosystems. Springer, Berlin
Kuebbing, S., Maynard, D., and Bradford, M. (2018). Linking functional diversity and ecosystem processes: a framework for using functional diversity metrics to predict the ecosystem impact of functionally unique species. J. Ecol. 106, 687–698. doi: 10.1111/1365-2745.12835
Laliberté, E., Legendre, P., and Shipley, B. (2014). FD: measuring functional diversity from multiple traits, and other tools for functional ecology. R package version 1.0–12.
Laureto, L., Cianciaruso, M., and Samia, D. (2015). Functional diversity: an overview of its history and applicability. Nat. Conserv. 13, 112–116. doi: 10.1016/j.ncon.2015.11.001
Lavorel, S., and Garnier, E. (2002). Predicting changes in community composition and ecosystem functioning from plant traits: revisiting the holy grail. Funct. Ecol. 16, 545–556. doi: 10.1046/j.1365-2435.2002.00664.x
Li, W., He, S., Cheng, X., and Zhang, M. (2021). Functional diversity outperforms taxonomic diversity in revealing short-term trampling effects. Sci. Rep. 11:18889. doi: 10.1038/s41598-021-98372-3
Li, D., Wu, S., Liu, L., Zhang, Y., and Li, S. (2018). Vulnerability of the global terrestrial ecosystems to climate change. Glob. Chang. Biol. 24, 4095–4106. doi: 10.1111/gcb.14327
Mameno, K., Kubo, T., and Oguma, H. (2022). Decline in the alpine landscape aesthetic value in a national park under climate change. Clim. Chang. 170:35. doi: 10.1007/s10584-022-03322-1
Mason, N., MacGillivray, K., and Steel, J. (2003). An index of functional diversity. J. Veg. Sci. 14, 571–578. doi: 10.1111/j.1654-1103.2003.tb02184.x
Mason, N., Mouillot, D., Lee, W., and Wilson, J. (2005). Functional richness, functional evenness and functional divergence: the primary components of functional diversity. Oikos 111, 112–118. doi: 10.1111/j.0030-1299.2005.13886.x
Memoli, V., Esposito, F., Panico, S., De Marco, A., Barile, R., and Maisto, G. (2019). Evaluation of tourism impact on soil metal accumulation through single and integrated indices. Sci. Total Environ. 682, 685–691. doi: 10.1016/j.scitotenv.2019.05.211
Monz, C. (2002). The response of two arctic tundra plant communities to human trampling disturbance. J. Environ. Manag. 64, 207–217. doi: 10.1006/jema.2001.0524
Mouchet, M., Villeger, S., Mason, N., and Mouillot, D. (2010). Functional diversity measures: an overview of their redundancy and their ability to discriminate community assembly rules. Funct. Ecol. 24, 867–876. doi: 10.1111/j.1365-2435.2010.01695.x
Mouillot, D., Graham, N., Villéger, S., and Mason, N. (2013). A functional approach reveals community responses to disturbances. Trends Ecol. Evol. 28, 167–177. doi: 10.1016/j.tree.2012.10.004
Nomoto, H., and Alexander, J. (2021). Drivers of local extinction risk in alpine plants under warming climate. Ecol. Lett. 24, 1157–1166. doi: 10.1111/ele.13727
Pickering, C. M., and Hill, W. (2007). Impacts of recreation and tourism on plant biodiversity and vegetation in protected areas in Australia. J. Environ. Manage. 85, 791–800. doi: 10.1016/j.jenvman.2006.11.021
Prescott, O., and Stewart, G. (2022). Assessing the impacts of human trampling on vegetation: a systematic review and meta-analysis of experimental evidence. PeerJ 2:e360. doi: 10.7717/peerj.360
Pueyo-Ros, J. (2018). The role of tourism in the ecosystem services framework. Land 7:111. doi: 10.3390/land7030111
R Core Team. (2017). R: A Language and Environment for Statistical Computing. Available at: https://www.R-project.org/
Reich, P. (2014). The world-wide ‘fast-slow’ plant economics spectrum: a traits manifesto. J. Ecol. 102, 275–301. doi: 10.1111/1365-2745.12211
Roovers, P., Verheyen, K., Hermy, M., and Gulinck, H. (2004). Experimental trampling and vegetation recovery in some forest and heathland communities. Appl. Veg. Sci. 7, 111–118. doi: 10.1111/j.1654-109X.2004.tb00601.x
Smith, E., Holden, E., Brown, C., and Cahill, J. (2022). Disturbance has lasting effects on functional traits and diversity of grassland plant communities. PeerJ. 10:e13179. doi: 10.7717/peerj.13179
Suding, K., and Goldstein, L. (2008). Testing the holy grail framework: using functional traits to predict ecosystem change. New Phytol. 180, 559–562. doi: 10.1111/j.1469-8137.2008.02650.x
Suding, K., Lavorel, F. S., and Chapin, I. I. I. (2008). Scaling environmental change through the community-level: a trait-based response-and-effect framework for plants. Glob. Chang. Biol. 14, 1125–1140. doi: 10.1111/j.1365-2486.2008.01557.x
Tian, K. (2010). “Plateau wetlands: functions, values, and environmental deterioration.” in The China Environment Yearbook, Volume 4. doi: 10.1163/9789004190351_006
Vandewalle, M., de Bello, F., and Berg, M. P. (2010). Functional traits as indicators of biodiversity response to land use changes across ecosystems and organisms. Biodivers. Conserv. 19, 2921–2947. doi: 10.1007/s10531-010-9798-9
Verrall, B., and Pickering, C. (2020). Alpine vegetation in the context of climate change: a global review of past research and future directions. Sci. Total Environ. 748:141344. doi: 10.1016/j.scitotenv.2020.141344
Villeger, S., Mason, N., and Mouillot, D. (2008). New multidimensional functional diversity indices for a multifaceted framework in functional ecology. Ecology 89, 2290–2301. doi: 10.1890/07-1206.1
Violle, C., Navas, M. L., Vile, D., and Kazakou, E. (2007). Let the concept of trait be functional! Oikos 116, 882–892. doi: 10.1111/j.0030-1299.2007.15559.x
Vloon, C., Evju, M., and Klanderud, K. (2022). Alpine restoration: planting and seeding of native species facilitate vegetation recovery. Restor. Ecol. 30:e1347. doi: 10.1111/rec.13479
Willard, B., Cooper, D., and Forbes, B. (2007). Natural regeneration of alpine tundra vegetation after human trampling: a 42-year data set from Rocky Mountain National Park, Colorado. USA. Arct. Antarct. Alp. Res. 39, 177–183. doi: 10.1657/1523-0430(2007)39[177:NROATV]2.0.CO;2
Xiong, C., Khan, A., Bibi, S., Hayat, H., and Jiang, S. P. (2022). Tourism subindustry level environmental impacts in the US. Curr. Issues Tour. 10, 1–19. doi: 10.1080/13683500.2022.2043835
Keywords: alpine grasslands, trampling disturbance, functional traits, functional diversity, Shangri-La
Citation: Li W, Zheng TD, Cheng XP and He SQ (2023) Changes in functional traits and diversity of typical alpine grasslands after a short-term trampling disturbance. Front. Ecol. Evol. 11:1154911. doi: 10.3389/fevo.2023.1154911
Edited by:
Hongbo Li, Chinese Academy of Agricultural Sciences, ChinaReviewed by:
Xiao Guo, Qingdao Agricultural University, ChinaCongcong Liu, Institute of Geography Science and Natural Resources (CAS), China
Copyright © 2023 Li, Zheng, Cheng and He. This is an open-access article distributed under the terms of the Creative Commons Attribution License (CC BY). The use, distribution or reproduction in other forums is permitted, provided the original author(s) and the copyright owner(s) are credited and that the original publication in this journal is cited, in accordance with accepted academic practice. No use, distribution or reproduction is permitted which does not comply with these terms.
*Correspondence: Wei Li, d3cwNTkyQGdtYWlsLmNvbQ==