- 1Department of Ethology and Companion Animal Science, Faculty of Agrobiology, Food and Natural Resources, Czech University of Life Sciences Prague, Prague, Czechia
- 2Department of Ethology, Institute of Animal Science, Prague, Czechia
- 3Department of Game Management and Wildlife Biology, Faculty of Forestry and Wood Sciences, Czech University of Life Sciences Prague, Prague, Czechia
- 4Department of Ecology and Environmental Sciences, Faculty of Natural Sciences and Informatics, Constantine the Philosopher University in Nitra, Nitra, Slovakia
- 5Laboratory of Anaerobic Microbiology, Institute of Animal Physiology and Genetics, Czech Academy of Sciences, Prague, Czechia
- 6Independent Researcher, Tampere, Finland
- 7Section of Ecology, Department of Biology, University of Turku, Turku, Finland
The knowledge about the mortality rate of offspring is crucial for estimating bird population dynamics and conserving species with declining populations. Parents of predatory birds provide food for their offspring during the post-fledging dependency period, which is frequently described as essential due to inexpert flying skills. Using radio telemetry, we studied fledglings’ probability of dying by starvation and predation in Tengmalms’ owls (Aegolius funereus). Nestlings (21 and 39) from 10 and 14 broods in 2019 and 2021, respectively, were equipped with leg-mounted tags and monitored throughout the post-fledging dependency period in west-central Finland. In total, 28 out of 60 fledglings did not survive the post-fledging dependency period (12 died due to starvation, and 16 were predated). The fledglings’ probability of dying by starvation and predation was 3.7 and 2.4 times higher, respectively, in the decreasing (2019) than during the increasing (2021) abundance of main foods (voles), showing that prey availability is essential for survival during the post-fledging dependency period. The probability of starvation increased with augmenting agricultural lands in the home range and increasing precipitation after fledging, which indicated that parent owls avoided hunting in open areas and during rainy nights. The predation rate during the post-fledging dependency period increased with augmenting cover of old-growth forests in the home range. This result suggested that coverage of old-growth forests is nowadays so small in the study area that they act as ecological traps for newly-fledged owlets. The reason is that the main avian enemies of Tengmalm’s owls’ (goshawks and Ural owls) also prefer old-growth forests for breeding and hunting.
1. Introduction
Understanding the causes of mortality and the survival probability of offspring is essential for estimating bird population dynamics and recruitment probability (Lack, 1966; Clutton-Brock, 1988; Newton, 1989). It is also critical in conserving species with declining populations (Vormwald et al., 2011). Low survival soon after fledging is common across bird species (e.g., Newton et al., 1982; Naef-Daenzer et al., 2001; Sunde, 2005; Adams et al., 2006; Tome, 2011). Furthermore, offspring sex, the timing of birth within the breeding season, age at dispersal, and body mass often influence variation in offspring survival (Tarwater et al., 2011; Kouba et al., 2021a). Sex differences in survival commonly arise when the sexes differ in size or dispersal behavior (Green and Cockburn, 2001). Generally, offspring that disperse at older ages have higher survival compared to early dispersers (Griesser and Barnaby, 2010).
Most survival data in birds were previously gathered by ringing studies and have found the recruitment probability of fledglings to vary with environmental and individual variables (e.g., Korpimäki and Lagerström, 1988; Nilsson, 1999). In addition, survival data of offspring have already been collected for three decades using conventional VHF telemetry or, more recently, satellite UHF telemetry (Kenward, 2001).
The post-fledging dependence period (PFDP) is one of birds’ most sensitive life history stages (Newton, 1979; Newton et al., 1982; Weathers and Sullivan, 1989). Fledglings of birds of prey entirely depend on their parents for food during PFDP and stay within the natal area until the initiation of natal dispersal (Kenward et al., 1993). This period is frequently described as crucial due to incomplete feather growth and inexpert flying skills (McFadzen and Marzluff, 1996; Todd et al., 2003; Sunde, 2005) and ranges in different species from a few weeks to several months (Newton, 1979).
Published studies identifying fledgling mortality of different owl and diurnal raptor species have considered predation and starvation the most frequent cause of death (e.g., Sunde, 2005; Wiens et al., 2006). Infections, drowning, electrocution, collisions with vehicles and other or unknown cases were less frequent (e.g., Sunde, 2005; Larrat et al., 2012; Kouba et al., 2021b). Significantly higher mortality was observed in years with low prey availability compared to years with high prey availability (e.g., Todd et al., 2003; Sunde, 2005), indicating the validity of the alternative prey hypothesis (Lack, 1954; Korpimäki et al., 1990). It predicts that the search intensity of potential predators is possibly reduced in years with high availability of main prey and vice versa. Thus, the abundance of voles likely benefited juvenile owls in two ways: directly, by serving as an abundant food resource; and indirectly, as an alternative food source for their predators (Rohner and Hunter, 1996). The mortality risk is highest immediately after leaving the nest, mainly due to mammalian predators (Sunde, 2005). The increased mortality after fledging was confirmed by many studies (e.g., McFadzen and Marzluff, 1996; Tome, 2011).
The Tengmalm’s owl (Aegolius funereus) is a small, nocturnal, cavity-nesting owl living in coniferous forests in the boreal zone and alpine forests further south in Eurasia (Korpimäki and Hakkarainen, 2012). Hatching occurs at approximately two-day intervals (Korpimäki and Hakkarainen, 2012). The young stay in the nest for 27–38 days after hatching (Korpimäki, 1981; Kouba et al., 2015), thus fledging at different times, and reach independence 5 to 9 weeks after fledging (Kouba et al., 2013). Survival of nestlings and fledgling production of Tengmalm’s owls in the decreasing phase of the vole cycle declined steeply with an increasing proportion of agricultural fields in the home range (Hakkarainen et al., 2003). In contrast, clutch size was not significantly related to landscape composition (Hakkarainen et al., 2003). A positive effect of old-growth forests on over-winter survival of male owls was found during the 1980s and 1990s (Hakkarainen et al., 2008), whereas the area of old-growth forests in the study area has gradually decreased thereafter (Korpimäki and Hakkarainen, 2012; Korpimäki, 2020).
The main objective of this study was to determine the probability of death in Tengmalm’s owl fledglings during the PFDP and to find out how relationships between environmental variables (e.g., the abundance of main prey, weather conditions, habitat composition and/or the number of siblings) and individual characteristics (e.g., laying date of the first egg, date and order of hatching and fledging, age at fledging, sex and/or body condition) affect different causes of death, i.e., mortality caused by (1) starvation (which is usually accompanied by infections; Larrat et al., 2012, Kouba, unpublished data) and (2) predation throughout the PFDP.
We predicted that fledglings’ probability of dying by starvation would be (i) essentially lower in the increase phase than in the decrease phase of the vole cycle (Korpimäki and Lagerström, 1988; Korpimäki and Hakkarainen, 2012), (ii) higher in areas with larger agricultural fields in the home range (Hakkarainen et al., 2003), and (iii) increasing with advancing hatching date. Early breeders usually are the fittest and most productive individuals in the population (e.g., Korpimäki and Hakkarainen, 1991; Sergio, 2003). Regarding the fledglings’ probability of dying by predation, we predicted that (iv) predation events would occur more frequently in the season with decreasing abundance of main prey (voles) as a consequence of food shortage. Further, predation will be (v) lower in areas with an increasing cover of old-growth forests around the nest box, as it was similarly found in male parents of owls (Hakkarainen et al., 2008). The main predators of Tengmalms’ owls, such as goshawks (Accipiter gentilis) and Ural owls (Strix uralensis), also prefer old-growth forests. Therefore, one might alternatively expect the old-growth forest patches to act as ecological traps (sensu Schlaepfer et al., 2002) for owl offspring during the PFDP. Finally, predation will be (vi) increasing with the advancing hatching date, as found in tawny owls (Strix aluco; Sunde, 2005).
2. Materials and methods
2.1. Study area
The study was carried out during two breeding seasons, 2019 and 2021, in the Kauhava region of west-central Finland (ca 63° N, 23° E). The study area is 30–120 m above sea level, and approximately 61% of the study area is forested: in the early 1990s, ca. 39% was formed by young, 11% by middle-aged (30–80 years) and 11% by old-growth forests (> 80 years; Hakkarainen et al., 2003). Almost all the woods in the study area are managed; firstly, harvested by thinning around 30–40 years, and secondly, undergo clear-cutting at 60–80 years. Clear-cut areas are usually managed by planting Norway spruce (Picea abies) or Scots pine (Pinus sylvestris) saplings or, less often, seeded. Consequently, old-growth forests comprise nowadays around 1% of the study area. Clear-cut and sapling areas form about 6% of the study area, agricultural land (mainly crop fields and pastures) cover ca. 25%, peatland bogs 2%, other (settlements, roads etc.) 3%, and water (lakes, rivers, streams) 2% of the area (Korpimäki and Hakkarainen, 2012; Korpimäki, 2020).
In the study area, long-term studies of different birds of prey are carried out (e.g., Korpimäki and Hakkarainen, 2012), so the locations of avian predator nests are quite well known. The local densities of Tengmalm’s owls’ main predators are as follows. There are ca. two territories of Ural owls and one of goshawks per 100 square kilometers (Korpimäki and Hänninen, unpublished data).
2.2. Environmental variables
For this study, we divided habitat composition around each involved nest box into three classes (with three forest subclasses, see below) according to the methodology described by Morosinotto et al. (2017) and Baroni et al. (2021) as we worked in the same study area. We calculated areas of (1) forest stands and individual areas of young, middle-aged, and old-growth forests, (2) clear-cuts, and (3) agricultural lands (Table 1). The habitat types (variables 1–3) were identified using landscape maps based on the SLICE dataset (Mikkola et al., 1999; Morosinotto et al., 2017; Baroni et al., 2021) and further processed in ArcGIS software (version 10.5; Esri, United States). Areas newly clear-cut in 2021 were identified personally during the field season and confirmed by comparing older and current satellite landscape images. We evaluated areas of each habitat variable around involved nest boxes in the distances from 250 to 4,000 m in a step of 250 m (i.e., in 16 radii in total) following an approach suggested by Jackson and Fahrig (2015) as we were interested which radius with its habitat composition is the best to predict the fledglings’ mortality. We found the given radii sufficient as the mean hunting home range size of breeding Tengmalm’s owl males was found to be 1–3 square kilometers (Jacobsen and Sonerud, 1987; Santangeli et al., 2012; Kouba et al., 2017), and maximum recorded distance of hunting male is about 4 km from the nest (Hakkarainen et al., 2003).
From the Finnish Meteorological Institute, we obtained meteorological data from the weather station at Kauhava airport, situated approximately in the middle of the study area. The data sets included mean daily temperature (°C; an average of hourly measurements) and daily precipitation (mm).
2.3. Field procedures
In the study area, there are currently 450 wooden nest boxes (18–20 × 18–20 cm base, 50–60 cm height and 8–10 cm diameter entrance hole) suitable for the breeding of Tengmalm’s owls in the area covering about 1,100 square km (Korpimäki and Hakkarainen, 2012; Korpimäki, 2020). Tengmalm’s owls readily accept artificial nest boxes because the density of natural cavities made by black woodpeckers (Dryocopus martius) is low.
We visited the nest boxes in the study area twice a year (late March to late April and mid-May to early June) to find nests (Korpimäki and Hakkarainen, 1991, 2012). We calculated the laying date of the first egg mainly by back-dating from hatching dates using 29 days as the incubation period for the first-laid egg (Korpimäki, 1981). Nests were inspected sufficiently often to record the final number of eggs and hatchlings and determine the hatching date (± 1 day). The nestlings’ age and hatching order were based on the recorded hatching date. All nestlings were ringed, and from the age of about 25 days after hatching of the first chick (i.e., shortly before the time when chicks might be expected to start to leave the nest), the nest boxes were checked at two-day intervals. All individuals were repeatedly weighed, and their wing length was measured to estimate the appropriate time for tagging. The details of primary breeding data are listed in Table 2.
Further, following Hipkiss and Hörnfeldt (2004), we collected a 50 μl blood sample from each nestling by brachial vein puncture under the wing, ca. 14 days after hatching, for molecular sexing as in our previous study (Kouba et al., 2020a). Sex determination of nestlings relied on polymerase chain reaction (PCR) amplification of one intron from the sex chromosome-linked CHD1 gene, which in birds differs in size between the Z and W chromosomes (Fridolfsson and Ellegren, 1999). Males showed only the shorter Z-fragment, while females displayed both a 1.2 kb W-specific and a 0.7 kb Z-specific fragment (Fridolfsson and Ellegren, 1999).
Nestlings from 10 nest boxes in 2019 (n = 21) and 14 nest boxes in 2021 (n = 39) were equipped with leg-mount transmitter type PIP4 (Biotrack Ltd., United Kingdom) about 5 days before fledging. In both years, we tagged and radio-tracked whole sibling groups (i.e., each fledged individual) from all 24 nest boxes. In 2019 we tracked all fledglings produced by the entire Tengmalm’s owl population in our study area; in 2021, we followed 40% of all produced fledglings (39 out of 97 inds, Table 2). Leg-mount transmitters weighed ca. 3 g in 2019 and ca. 3.3 g in 2021 (lifespan >75 days in both years), which followed welfare recommendations not to exceed 3% of the body mass of tagged individuals (e.g., Withey et al., 2001). Specifically, transmitters attached to the nestlings were 2.3% in 2019 and 2.6% in 2021 of their body mass on average (weighing was performed 3 days before fledging on average).
After tagging, we visited nest boxes at 12–hour intervals following the methods of Kouba et al. (2013, 2014) during the night (23:00–03:00) and during daylight (8:00–21:00) until all siblings fledged and we could determine the exact date of nest box departure. After fledging, we located the young at 12–hour intervals once every night and every day by the ‘homing-in’ method (Kenward, 2001) until they became independent (i.e., we followed the signal to a particular tree or until we saw the individual), were found dead or disappeared. We defined the end of the PFDP with the first rapid and abrupt movement away from habitual locations, which may correspond with the cessation of begging for food, as shown in our previous studies (Kouba et al., 2013, 2014). Thus, we could detect individuals’ death or disappearance events within about 12 h. We received radio signals using MVT-9000 receivers (Yupiteru Industries Co. Ltd., Japan) and 3-element Yagi antennas. Fledglings’ positions, including places of death (2,670 locations in total), were recorded using the GPS receivers (GPSmap 60CSx or Astro 230, Garmin Ltd., United States).
We trapped 22 out of 24 parent males and all females at the 24 nests where we tagged and radio-tracked offspring. We trapped the parents during the middle of the nestling period, ringed (if not re-trapped), aged by checking the moult of primary and secondary feathers (Korpimäki and Hakkarainen, 2012), and measured their wing length and body mass (for trapping methods and measurement details, see Korpimäki and Hakkarainen, 2012). Further, we calculated the “scaled mass index” of parent owls following Peig and Green (2009) to quantify the body mass relative to the body size of the owls that allows adjusting the weight of all individuals to that which they would have if they had the same body size (Kouba et al., 2020, 2021a).
All applicable international, national and institutional guidelines for the care and use of animals were followed in this study. Parent owls and fledglings were captured and ringed under the ringing licence of the Finish Museum of Natural History (licence no. 524 to EK). The owl nestlings were blood sampled under the approval of the Animal Experiment Committee of the State Provincial Office (Etelä-Suomen aluehallintovirasto ESAVI; permit number ESAVI/3021/04.10.07/2017). The owl nestlings were tagged and radio-tracked under the approval of the Centre for Economic Development, Transport and the Environment (Varsinais-Suomen Elinkeino-, Liikenne- ja Ympäristökeskus: permit number VARELY/1389/2018).
2.4. Prey availability
We estimated abundances of the main prey of Tengmalm’s owls (bank voles Myodes glareolus, field voles Microtus agrestis and sibling voles M. rossiaemeridioinalis) by snap-trapping in both study years in early May (i.e., the early breeding season of owls) and mid-September (shortly after PFDP of owls) in both western and central parts of the study area. Sampling was carried out in the four main habitat types (i.e., cultivated and abandoned fields, spruce and pine forests). Fifty-to-sixty baited Finnish metal mouse snap traps were set at 10 m intervals in vole runways on each sample plot and were checked daily for three consecutive days. The sample plot area was 0.5–0.6 ha, and the pooled trapping effort was ca. 600 trap-nights in both western and central parts of the study area in May and September in the two study years. The number of voles captured was standardised to the number of animals caught per 100 trap-nights to obtain the vole abundance index (Korpimäki et al., 2005). As found earlier (Huitu et al., 2003; Korpimäki et al., 2005), densities of bank voles and Microtus voles fluctuate in the study area in three-year cycles, and the regional synchrony of vole population cycles extends up to 80 km (i.e., to the whole study area). In 2019 there was a declining phase of the vole cycle when abundance indices of voles increased only slightly from May to September (from 0.4 to 0.6 voles per 100 trap-nights). Whereas 2021 was an increasing phase of the vole cycle when abundance indices of voles more than doubled from May to September (from 7.8 to 18.1 voles per 100 trap-nights). Thus, the abundance of main prey in May 2021 was 18.6 times higher than in May 2019 and 28.7 times higher in September 2021 than in September 2019.
2.5. Statistical analyses
We analysed the data using SAS System version 9.4 (SAS Institute Inc.) in three steps. First, the analyses checking for intercorrelation and multicollinearity were done separately for the data of Tengmalm’s owl study population, weather, and habitat composition. We subsequently made a judgment of the extent of collinearity by checking related statistics, such as Tolerance value or Variance Inflation factor (VIF), Eigenvalue, and Condition Number following the approach of Schreiber-Gregory and Jackson (2017) and using TOL, VIF and COLLIN options of the MODEL statement in the SAS REG procedure. Correlations are generally considered “strong” above 0.8 (Schreiber-Gregory and Jackson, 2017) or at least 0.5 (Zuur et al., 2007). In further analyses, no variables found to be intercorrelated were used in the same model together in any case.
Second, we applied model selection based on the information-theoretic paradigm using Akaike’s Information Criterion – IT-AIC (Burnham and Anderson, 1998). We prepared a priori multiple hypotheses based on the remaining biologically relevant variables after testing for collinearity (each model/hypothesis tested, including biological explanations of applied fixed effects, are listed in the Supplementary Tables S1, S2).
Since the introduction of Akaike’s Information Criterion (AIC, Akaike, 1973), more information criteria have been developed with different mathematical properties and philosophies of model selection in mind (Christensen, 2018). After Christensen (2018), we used expanded information criteria AICC, AIC (Hurvich and Tsai, 1989), BIC (Schwarz, 1978), CAIC (Bozdogan, 1987), and HQIC (Hannan and Quinn, 1979) to select a true model of the seasonal or social. Then we compared the candidate models by ranking them based on the information criteria used (PROC RANK). The model with the lowest value (i.e., closest to zero) is considered to be the “best” model (Burnham and Anderson, 2002; Christensen, 2018).
We calculated Δi (the differences between the Fit statistic values, the smallest values indicating the best-fitting model), wi (the weight, interpreted as the probability that Mi is the best model), and wmin/wj (for estimating the strength of evidence in favor of one model over the other – AIC or AICC, BIC, CAIC, and HQIC Odds; Burnham and Anderson, 2002) for all five fit criteria (AIC, AICC, BIC, CAIC, and HQIC; Christensen, 2018). Models with Δi values less than 2 were considered to be essentially as good as the best model, and “models with Δi up to 6 should probably not be discounted” (Richards, 2005). Since there was a similar or identical rank order across these criteria and Δi, wi, and wmin/wj gave similar results, here we present the values for AICC only [a generally appropriate small-sample version of AIC (e.g., Hurvich and Tsai, 1989; Anderson et al., 2001)] as an illustration of the results (Table 3 and Supplementary Table S3).
To find out whether the best model has merit, we compared our best model to the null model for all dependent variables using delta AICC (for AICC, AICC null – AICC best model; the same was also calculated for AIC, BIC, CAIC, and HQIC; Table 3 and Supplementary Table S3) and a relative information loss [for AICC exp.((AICC_null − AICCi_best)/2); the same for AIC, BIC, CAIC, and HQIC], an approach adapted from Burnham and Anderson (2002).
Third, using two different models (a, b; Table 3) analyzed by General Linear Mixed Model procedure (PROC GLIMMIX for binary distribution) as a fixed effects model, with associations between fledglings’ probability of dying by starvation (predictions i – iii) and fledglings’ probability of dying by predation (predictions iv – vi) as dependent variables.
Before the final analysis, we estimated which radius (16 radii, 250–4,000 m, see above and Supplementary Table S4) around nest boxes was the most important regarding habitat composition variables in explaining the two dependent variables tested (a, b). We found that the 500 m radius was the most appropriate and omitted all the other radii from the further analyses (the procedure is not presented). We further analysed which interaction of fledglings’ survival age and the mean daily precipitation was the most important in explaining the two dependent variables tested (a, b). We checked the interaction for 1, 5, 8, 16, and 18 days after leaving the nest (Supplementary Table S5). The “survival age” was defined as the number of days between fledging and death for individuals who have died. For individuals who survived until independence, a period of 18 days was taken because none of the fledglings died later. The “mean precipitation” was defined as the average precipitation for the number of days between fledging and death for individuals who did not survive the PFDP. The means of 1, 5, 8, 16 and 18 days were calculated for individuals who survived until independence. The interaction for the 18 days appeared the best, and we omitted the others (not presented). As a part of the preliminary analysis, we also tested the effect of scaled body mass indices of parent owl females and males on the tested dependent variables. However, they did not appear to be influential (not presented).
Thus, fixed effects used in the final analyses were: year (spring prey availability), the number of fledglings, laying or hatching date, sex of individuals, nestlings’ wing length approximated to the age of 30 days after hatching, individual duration of nestling period (days), mean precipitation (mm), survival age (days) and areas of total forest stands, separate areas of young, middle-aged, and old-growth forests, clear-cuts or agricultural lands within 500 m radius of involved nest boxes. The combination of fixed effects in alternative models tested was dependent on the formulation of a priori hypotheses (Supplementary Tables S1, S2).
Finally, having the best composition of all models according to IT-AIC, we calculated these using GLIMMIX. We calculated each model’s coefficient estimates, standard errors, and 95% confidence intervals (Supplementary Table S6). For the graphs, we estimated associations between the dependent variables and fixed effects by fitting a random coefficient model using PROC MIXED as described by Tao et al. (2002).
3. Results
In total, we monitored 24 nests and 60 fledglings during two breeding seasons, during which we recorded 137 hatchlings in all monitored nests (Table 2). There were 53 and 84 hatchlings and 21 and 39 fledglings in the 10 and 14 study nest boxes, and the nestlings’ mortality mostly caused by starvation was 60.4 and 53.6% in 2019 and 2021, respectively. In total, 32 out of 60 fledglings survived throughout the PFDP (its duration ranged between 28 and 54 days) and dispersed. Out of 28 dead fledglings, 12 died of starvation, and 16 were predated. In 2019, 81% (17 out of 21) of fledglings died during the PFDP (eight starved and nine predated), whereas 28% (11 out of 39) of fledglings died in 2021 (four starved and seven predated). In both seasons, the main predators were goshawks and Ural owls.
The 2019 breeding season (nestling phase and PFDP) turned out to be overall colder and poorer in rainfall compared to 2021 (temperature monthly means: April 5.6–3.1, May 8.9–8.5, June 15.2–17.4, and July 15.4–19.1; monthly precipitation means: April 0.3–1.0, May 2.0–2.1, June 1.4–1.1, and July 0.9–1.4; respectively). However, the differences between the two study seasons were not significant, neither for mean daily temperature in April – July (Mann–Whitney U test: U = 6,792, p = 0.24, n = 122 days) nor for daily precipitation in April – July (Mann–Whitney U test: U = 7,328, p = 0.84, n = 122 days).
Supplementary Table S7 (model a) and S8 (model b) show the five best candidate models ranked using the five information criteria. They all ranked the same five GLIMMIX models as the best; therefore, only AICC’s results are presented here. The five best candidate models for the two dependent variables (models a, b) tested, accordings to the AICC information criteria, including Δ AICC, Akaike weights, and AICC Odds sorted by AICC (from the lowest to the highest value), are presented in Table 3. Information criteria and rankings for the five best candidate models (“best” model first) and Null models are shown in Table 3. Uniform rankings across the five information criteria make a convincing argument that the models with cross-level interactions are the five best models. Relative information loss resulting from the comparison between Null and best models confirms that the best and other top-ranked models have merit (Table 3). The estimate, standard error and 95% confidence interval of the fixed effects in the best and equivalent models for the two dependent variables tested are shown in Supplementary Table S6.
3.1. Fledglings’ probability of dying by starvation (a)
For the dependent variable, the fledglings’ probability of dying by starvation, the Δ values of AICC (the other information criteria used, too; see Supplementary Table S7) nominated three best-fitting models covering a similar combination of fixed effects (Table 3). According to AICC, the probability of being the correct model was ambiguous for these three combinations (50, 22, and 18%). Therefore, we could not choose the best model; thus, we considered all three best models, which included six fixed effects repeatedly (Supplementary Table S6).
The three best models explaining the fledglings’ probability of dying by starvation included study year, duration of stay in the nest for a given individual, area of agricultural land within a 500 m radius around the nest box, number of fledglings in the brood and interaction between the mean amount of precipitation and fledglings’ survival age (Table 3). The fledglings’ probability of dying by starvation was 3.7 times higher in 2019 than in 2021 (Figure 1A; Model a1, Supplementary Table S6), decreased with a longer duration of stay in the nest (Figure 1B; Model a1, Supplementary Table S6), increased with augmenting area of agricultural land within a 500 m radius around the nest box (Figure 1C; Model a2, Supplementary Table S6), and declined with an increasing number of fledged individuals in the brood (Figure 1D; Model a3, Supplementary Table S6). The probability of starvation also increased with a higher mean amount of precipitation that occurred after fledging a given individual and decreased with advancing time from leaving the nest (Figure 2A; Model a1, Supplementary Table S6).
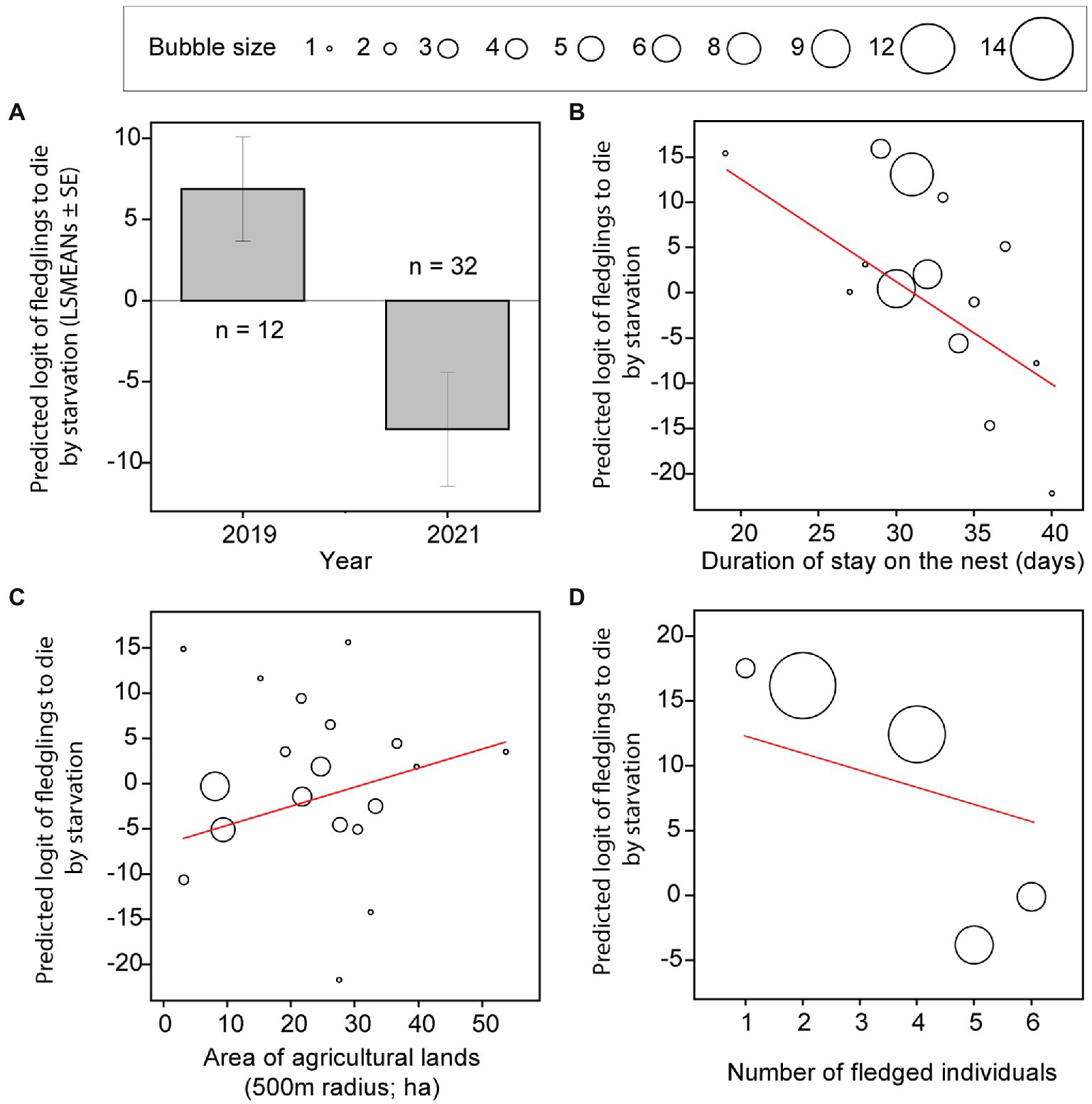
Figure 1. Fledglings’ probability of dying by starvation. Least square means (A) and bubble graphs (B–D) of predicted logit values of fledglings’ probability of dying by starvation of Tengmalm’s owl offspring during two post-fledging dependency periods (2019, 2021) (A; LSMEANs ± SE), and plotted against individual nestlings’ duration of stay in the nest (B), the area of agricultural lands within a 500 m radius from a given nest (C), and the number of fledged individuals in the brood (D) with regression line (red). The bubble size corresponds to the number of predicted (overlapping) cases between 1 and 14.
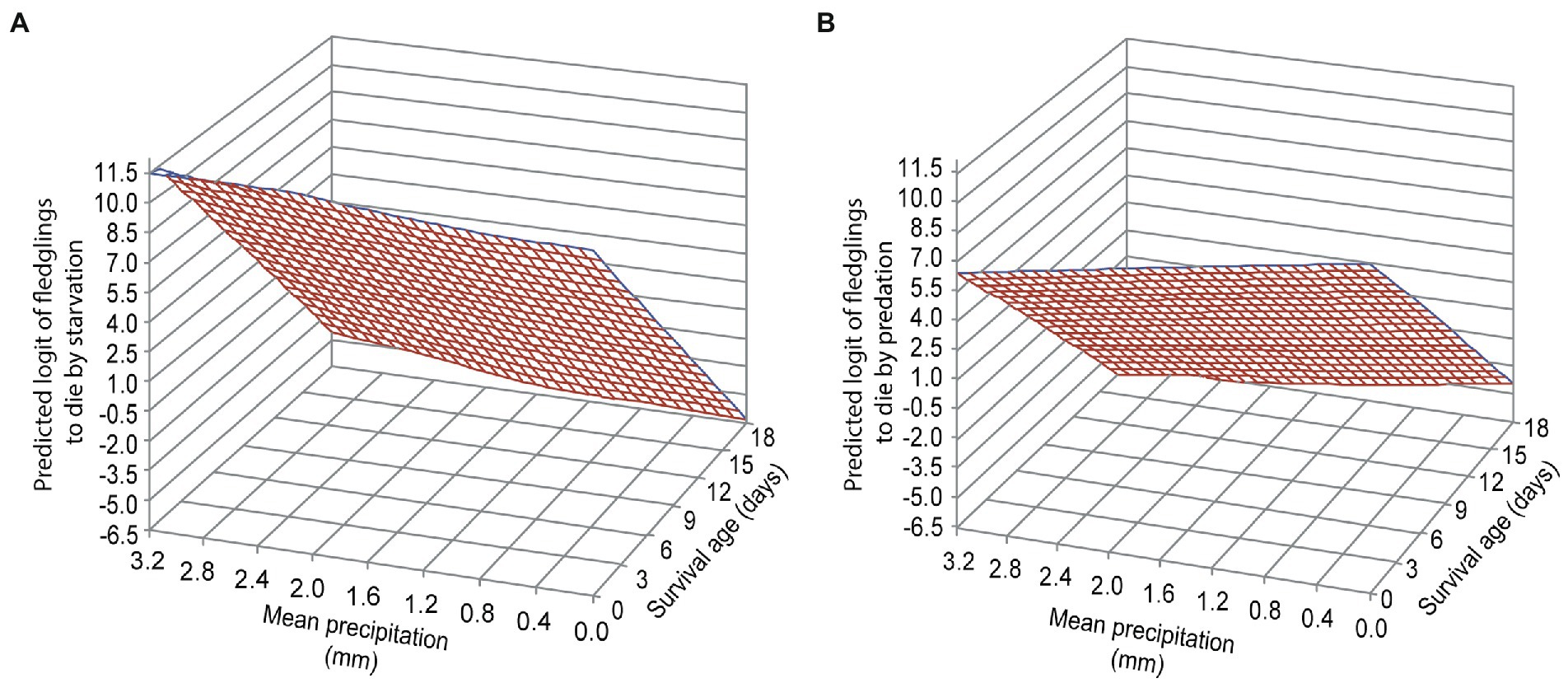
Figure 2. Fledglings’ probability of dying. Predicted logit values of fledglings’ probability of dying by starvation (A) and predation (B) of Tengmalm’s owl offspring during two post-fledging dependency periods (2019, 2021) plotted against the interaction of mean amount of precipitation and fledglings’ survival age.
3.2. Fledglings’ probability of dying by predation (b)
For the dependent variable, the fledglings’ probability of dying by predation, the Δ values of AICC (and all the other selection criteria; see Supplementary Table S8) conclusively nominated one best-fitting model with the probability of 77% that it is the best model (Table 3). The model with the second lowest AICC value had odds 4.61 times against it being the best model compared to the best model in the candidate set (Table 3). Therefore, the second and all the subsequent models did not need to be considered.
The best model explaining the fledglings’ probability of dying by predation included study year, hatching date, area of old-growth forests within a 500 m radius around the nest box and interaction between the mean amount of precipitation and fledglings’ survival age (Table 3). The fledglings’ probability of dying by predation was 2.4 times higher in 2019 than in 2021 (Figure 3A), decreased with advancing hatching of a given individual (Figure 3B), and increased with augmenting area of old-growth forests within a 500 m radius around the nest box (Figure 3C). The predation probability also decreased with a higher mean amount of precipitation that occurred after fledging a given individual and advancing time from leaving the nest (Figure 2B).
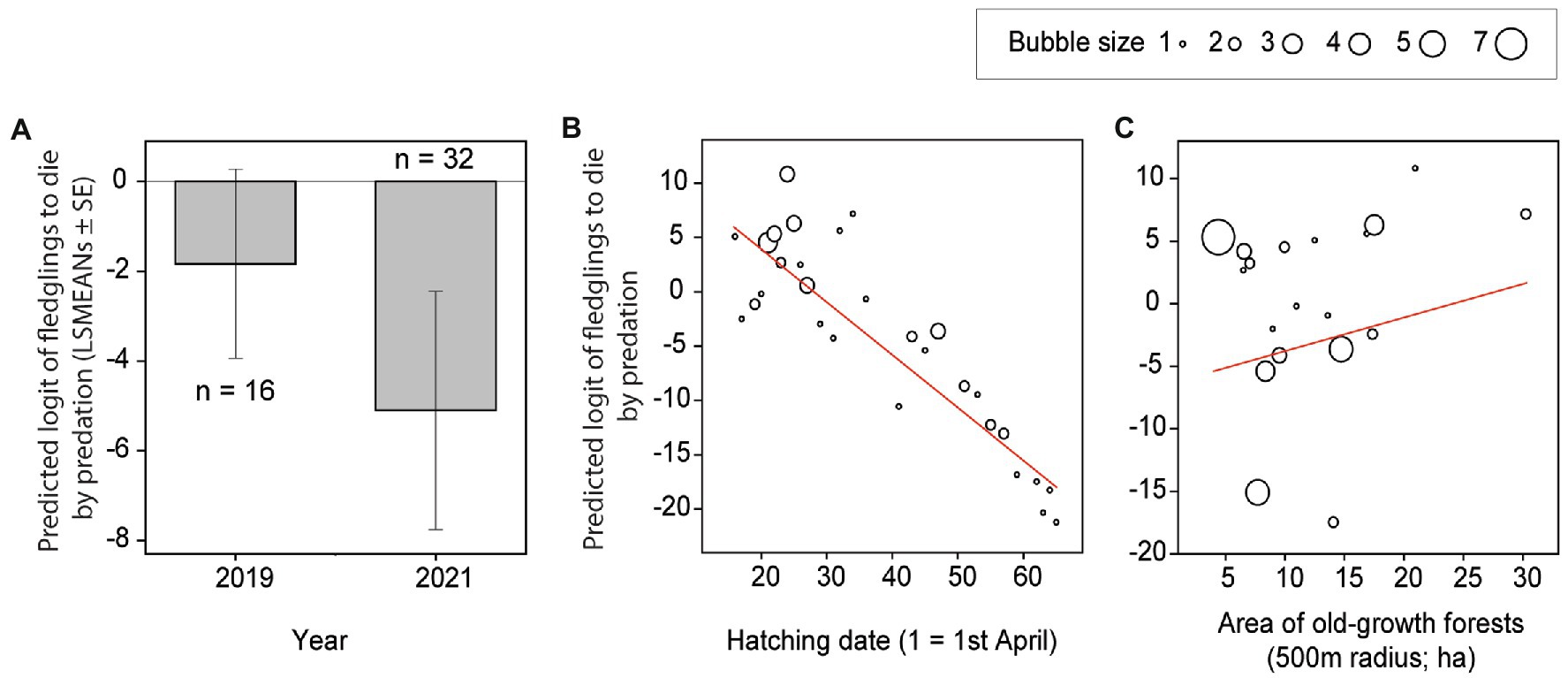
Figure 3. Fledglings’ probability of dying by predation. Least square means (A) and bubble graphs (B, C) of predicted logit values of fledglings’ probability of dying by predation of Tengmalm’s owl offspring during two post-fledging dependency periods (2019, 2021) (A; LSMEANs ± SE), and plotted against individual nestlings’ hatching date (B), and the area of old-growth forests within a 500 m radius from a given nest (C) with regression line (red). The bubble size corresponds to the number of predicted (overlapping) cases between 1 and 7.
4. Discussion
The first main finding of this study was more than three times higher mortality of fledglings caused by both starvation and predation during the decreasing phase of the vole cycle in 2019 compared to the increasing phase in 2021 (predictions i and iv). This result is consistent with the finding that first-year survival of Tengmalm’s owl offspring hatched in the increase phase of the vole cycle is three times as high as in those hatched in the decrease phase of the cycle (Korpimäki and Lagerström, 1988; Korpimäki and Hakkarainen, 2012). Therefore, the abundance of main prey (voles) during the PFDP and later when parents stop food-provision to their offspring and the young become independent is the primary determinant of the first-year survival of Tengmalms’ owl offspring. When main food resources are abundant, the probability of starvation essentially declined. In addition, satiated owl offspring do not beg for food from their parents as much as hungry owlets (Kouba et al., 2014). Silent owl offspring are thus much safer and cannot be located and captured by predators relying on auditory cues. Similar results stressing the importance of abundant main foods for fledglings’ survival throughout the PFDP were also reported in other birds of prey, for instance, in tawny owls (Overskaug et al., 1999; Sunde, 2005), goshawks (Wiens et al., 2006), great horned owls (Bubo virginianus; Rohner and Hunter, 1996), and burrowing owls (Athene cunicularia; Todd et al., 2003). Our findings regarding fledglings’ probability of death are thus in general agreement with these studies.
Our result (prediction iv) further supports the alternative prey hypothesis (Lack, 1954; Angelstam et al., 1984) reported for various bird and mammal species (e.g., Pienkowski, 1983; Korpimäki et al., 1990; Wilson and Bromley, 2001). Overall, the fledglings’ mortality throughout the PFDP was unexpectedly high, particularly in the decreasing phase of the vole cycle, regardless of whether caused by starvation or predation. High post-fledging mortality may also partly explain previous results about the long-term decline of the local Tengmalms’ owl population (Korpimäki and Hakkarainen, 2012; Korpimäki, 2020). In addition, nationwide Tengmalm’s owl population has declined by an annual rate of 2–3% from the 1980s to the 2010s (Korpimäki and Hakkarainen, 2012; Honkala et al., 2022).
The second main finding was a negative impact of agricultural lands on fledglings’ survival throughout the PFDP. Thus, we confirmed the prediction ii. The increasing probability of starvation in areas with larger agricultural fields was consistent with previous results that nestlings’ survival and fledgling production decreased steeply with the increasing proportion of farmland in home ranges (Hakkarainen et al., 2003). A possible explanation could be that Tengmalms’ owl males avoid hunting in open areas (Korpimäki and Hakkarainen, 2012). Thus, their hunting range in such locations is probably limited, and their prey delivery rate might be limited, too. Alternatively, because most starvation cases appeared during the declining phase of the vole cycle, the explanation may match the one mentioned by Hakkarainen et al. (2003). The sudden summer decline of vole populations begins earlier on agricultural lands than in forests, probably because the numbers of vole-eating predators, and hence their impact on vole populations is higher in farmland areas than in forested hinterlands (Korpimäki and Norrdahl, 1991; Hakkarainen et al., 2003). Thus, our result also supports the „spill-over “hypothesis stating that predators and their exploitation tends to ‘spill over’ from luxuriant habitats to barren habitats (Oksanen, 1990).
We rejected hypothesis v that fledglings’ survival throughout the PFDP increases with the cover of old-growth forests around the nest box. The increasing probability of predation with augmenting areas of old-growth forests around the nest box was in direct contrast to the finding that the over-winter survival of adult Tengmalms’ owl males increased with the cover of old forest stands, though the extent of old forests within their home ranges was small (mean 12%, range 2–37%; Hakkarainen et al., 2008). The representation of old-growth forests around the nests where we radio-tracked the fledglings was similarly small (mean ± SD 15 ± 7%, range 6–27%) and, therefore, well comparable. On the one hand, the old-growth forests provide ideal habitat for the over-winter survival of experienced male owls because they sustain high populations of small mammals and passerines (forming essential food sources) and serve as refuges against large avian predators (Hakkarainen et al., 2008). On the other hand, we suggest that the relatively small old forest patches in our study area may act as an ecological trap (e.g., Gates and Gysel, 1978; Schlaepfer et al., 2002; Hale and Swearer, 2016) for naive and inexperienced fledglings, and particularly at the very beginning of the PFDP. It should be so because, across different forest habitats, larger mature forest stands are also preferred for breeding and hunting by the main predators of Tengmalms’ owls, such as Ural owls (e.g., Lohmus, 2003; Bylicka et al., 2010) and goshawks (e.g., Beier and Drennan, 1997; Widen, 1997; Greenwald et al., 2005; Selas et al., 2008; Garcia-Salgado et al., 2018). Thus, possibly making newly fledged owlets highly vulnerable to avian predation. This explanation further supports our result that the predation probability declined steeply with advancing time from fledging. We are aware that the validity of the suggested „trap hypothesis “needs to be further tested, for instance, by following the survival of fledglings in areas with known numbers of potential avian predators and areas without them.
The third main finding of the current study regarded the interaction between the mean amount of precipitation and the fledglings’ survival age. Rainfall had the opposite effect on the probability of starvation and predation. The starvation probability increased with the increasing precipitation, whereas the predation probability declined with the rising rainfall. It is an expected result because it was previously shown that Tengmalms’ owl males probably could not efficiently hunt during rainy weather (Klaus et al., 1975; Kouba et al., 2017). Thus, the probability that their offspring will starve during such harsh weather periods is higher. It was also described earlier that the frequency of begging calls by fledglings decreased with increasing precipitation (Kouba et al., 2014), which further supports the above explanation.
On the contrary, one could expect that the predation probability will be higher during rainless periods because the hunting efficiency and success of larger birds of prey might also be hampered by rainfall, as in the case of Tengmalms’ owl males (Klaus et al., 1975; Kouba et al., 2017). In agreement with this, it was found that foraging activity and success declined with precipitation, for instance, in the black kite (Milvus migrans; Sergio, 2003). Our above explanation further supports previous results showing that predation risk for small mammals decreased during rainy nights (Vickery and Bider, 1981; Orrock et al., 2004). The starvation and predation probability further steeply declined with the advancing time after the owlets left the nest. The majority of both starvation and predation cases occurred very soon after fledging: during the first day after leaving the nest died ca. 33% of all individuals; between the second and the fifth day died ca. 30%; between the sixth and the eighth day died ca. 22%, and later the rest ca. 15% of monitored fledglings. We suggest that the stated numbers reflect both the fledglings’ rapidly improving flying skills along with the ability to escape predators and that the males are later better able to provide their offspring with enough prey until the end of the PFDP after some of the siblings have already died.
We have recorded mammalian predation (pine marten Martes martes, domestic cat Felis catus) only within the first few days after fledging, which is in line with studies on post-fledging mortality of tawny owls (Overskaug et al., 1999; Sunde, 2005). Contrary to our prediction (vi), we did not find that the mortality risk increased with the hatching and/or fledging date due to increased raptor predation risk, as it was found by Sunde (2005) in tawny owls. Conversely, we have detected most predation events by goshawks and Ural owls during the beginning of both breeding seasons. We can only speculate why fledglings’ mortality decreased with the advancing hatching date. The unexpected result might be explained by the following. The fledging period for all nests in 2019 was 11 days long only, while it was 47 days in 2021. The young started to fledge in both years on almost the exact date on 19 and 22 May, respectively. Nine predation events in 2019 took place at the very beginning of the PFDP, and though the seven cases in 2021 were more spread throughout the PFDP, most of them happened early in the season, too. Another possible explanation, and not mutually exclusive, could be that the prey availability continuously increased during the breeding season of 2021. It could cause more frequent predation of the monitored fledglings early in the season, according to the alternative prey hypothesis (Lack, 1954; Korpimäki et al., 1990).
The starvation probability was further affected by the duration of the nestling period. The longer the nestlings stayed in the nest, the lower the probability of starving to death throughout the PFDP. It seems beneficial for the Tengmalms’ owl young to remain in the nest longer, if possible, fledge with grown feathers and in better condition. We suggest that such fledglings can better cope with an unknown environment outside the nest box. We have, in contrast, previously found (Kouba et al., 2015) that the duration of the nestling period depends on the wing length [as a measure of body condition and individual quality (Granadeiro et al., 2000; Risely et al., 2013)] supporting the threshold size hypothesis (Johnson et al., 2004) that fledging begins when nestlings reach a specific developmental stage (Radersma et al., 2011; Johnson et al., 2013). We suggest that our result in the current study could have been affected by the fact that there were more than four fledglings in three out of 24 nests only. Thus, there was for the young no need to fledge soon after reaching the wing length threshold, for instance, because of limited space in the nest box. We can also speculate that Tengmalm’s owl parents do not tend to prefer feeding fledglings over the nestlings as described in passerines (Nilsson and Svensson, 1993; Radersma et al., 2011). This could be supported by multiple findings of up to several uneaten (and later rotten) prey items in the nest boxes from which all siblings have fledged (Kouba and Korpimäki, unpublished data).
Decreasing probability of dying by starvation with the increasing number of fledged siblings was connected with the fact that in the increasing year of the vole cycle (2021), abundances of main prey more than doubled from the early breeding season to the PFDP and independence period of offspring, whereas in the decline phase of the vole cycle (2019) abundance of main prey only slightly increased from May to September. It coincided with the known facts that under good food conditions, Tengmalm’s owls start to lay eggs earlier, have larger clutches, and more hatchlings and fledglings (Korpimäki and Hakkarainen, 1991, 2012). In principle, it can be said that during both study seasons, one or two siblings of the whole-fledged group did not survive the PFDP, which means that in the poor food year 2019, only one or no individual gained independence. Still, in the rich food year 2021, up to five individuals gained independence. This can explain why the fledglings’ probability of starving decreased with the increasing number of fledged individuals.
We found a positive relationship between the area of agricultural fields around the nest box and fledglings’ probability of starvation: the more agricultural areas, the higher mortality. This result shows the importance of preferably unfragmented forests for the long-term survival of Tengmalms’ owls. From this point of view, the conversion of forests to agricultural fields, which is still happening in our study area and elsewhere in Finland, is rather detrimental for forest-dwelling owls and probably other forest-dwelling species. Even more important was the finding that the fledglings’ probability of predation was higher in areas with more extensive patches of old-growth forests around the nest box. We conclude that in west-central Finland, mature forests nowadays cover only so limited areas that they act as an ecological trap (sensu Schlaepfer et al., 2002) for newly fledged owlets because remaining old-growth forest patches are also preferred by local large avian predators of Tengmalm’s owls. Future research should be directed to investigate how climate and weather interact with land use and habitat changes to modify trophic interactions, predator foraging behavior, and demography of different species, particularly in rapidly changing boreal ecosystems.
In the meantime, the evidence that populations of many forest-dwelling specialists inhabiting boreal forests are currently declining primarily due to clear-cutting inducing forest habitat loss, fragmentation and degradation is gradually increasing (e.g., Laaksonen and Lehikoinen, 2013; Venier et al., 2014; Fraixedas et al., 2015; Virkkala, 2016). This is also true for Tengmalms’ owls (Korpimäki and Hakkarainen, 2012; Kouba et al., 2020, 2021a). To conclude, we stress that forest management strategies designed to keep more and larger parts of boreal forests for natural and long-term development, including limitation in growing Scots pine plantations instead of Norway spruce ones and avoidance of forest clear-cutting, are necessary to reverse the gloomy prospects of forest-dwelling specialists and biodiversity loss in boreal forest areas while there is still time.
Data availability statement
The raw data supporting the conclusions of this article will be made available by the authors, without undue reservation.
Ethics statement
The animal study was reviewed and approved by Finish Museum of Natural History (licence no. 524 to EK), Animal Experiment Committee of the State Provincial Office (Etelä-Suomen aluehallintovirasto ESAVI; permit number ESAVI/3021/04.10.07/2017), and Centre for Economic Development, Transport and the Environment (Varsinais-Suomen Elinkeino-, Liikenne- ja Ympäristökeskus: permit number VARELY/1389/2018).
Author contributions
MK and EK are the principal contributors and conceivers of this study. MK, TB, SS, FT, MJ, and PK collected the field data. MŠ, MK, and FT prepared and processed the habitat data. SS did the laboratory sexing of the nestlings. MK, EK, and LB wrote the main manuscript text. MK and LB prepared all the figures. JB prepared scripts for statistical analysis. LB and MK did the statistical analysis. All authors contributed to the article and approved the submitted version.
Funding
The research project was financially supported by two grants provided to MK by the Regional Fund of the South Ostrobothnia of the Finnish Cultural Foundation (Business ID 0116947–3 and 10201775). LB and JB were supported by the Ministry of Agriculture of the Czechia (No. MZE-RO0723).
Acknowledgments
We thank Jorma Nurmi for his help in the field and Lubomír Peške for technical assistance with radiotelemetry and manufacturing Yagi antennas. Next, we would like to thank Charles Krebs and Gianpasquale Chiatante for their comments and suggestions to the earlier draft of the manuscript.
Conflict of interest
The authors declare that the research was conducted in the absence of any commercial or financial relationships that could be construed as a potential conflict of interest.
Publisher’s note
All claims expressed in this article are solely those of the authors and do not necessarily represent those of their affiliated organizations, or those of the publisher, the editors and the reviewers. Any product that may be evaluated in this article, or claim that may be made by its manufacturer, is not guaranteed or endorsed by the publisher.
Supplementary material
The Supplementary material for this article can be found online at: https://www.frontiersin.org/articles/10.3389/fevo.2023.1151622/full#supplementary-material
References
Adams, A. A. Y., Skagen, S. K., and Savidge, J. A. (2006). Modeling post-fledging survival of lark buntings in response to ecological and biological factors. Ecology 87, 178–188. doi: 10.1890/04-1922
Akaike, H. (1973). “Information theory and an extension of the maximum likelihood principle” in Proceeding the 2nd international symposium on information theory. eds. B. N. Petrov and F. Csaki (Budapest: Akademia Kiado), 267–281.
Anderson, D. R., Link, W. A., Johnson, D. H., and Burnham, K. P. (2001). Suggestions for presenting the results of data analyses. J. Wildl. Manag. 65, 373–378. doi: 10.2307/3803088
Angelstam, P., Lindström, E., and Widén, P. (1984). Role of predation in short-term population fluctuations of some birds and mammals in Fennoscandia. Oecologia 62, 199–208. doi: 10.1007/bf00379014
Baroni, D., Masoero, G., Korpimäki, E., Morosinotto, C., and Laaksonen, T. (2021). Habitat choice of a secondary cavity user indicates higher avoidance of disturbed habitat during breeding than during food-hoarding. For. Ecol. Manag. 483:118925. doi: 10.1016/j.foreco.2021.118925
Beier, P., and Drennan, J. E. (1997). Forest structure and prey abundance in foraging areas of northern goshawks. Ecol. Appl. 7, 564–571. doi: 10.1890/1051-0761(1997)007[0564:FSAPAI]2.0.CO;2
Bozdogan, H. (1987). Model selection and Akaike information criterion (AIC)–the general-theory and its analytical extensions. Psychometrika 52, 345–370. doi: 10.1007/BF02294361
Burnham, K. P., and Anderson, D. R. (1998). Model selection and inference: A practical information-theoretical approach. New York: Springer-Verlag, doi: 10.1007/978-1-4757-2917-7.
Burnham, K. P., and Anderson, D. R. (2002). Model selection and multimodel inference: A practical information-theoretic approach. Second edition. New York, Berlin, Heidelberg: Springer-Verlag.
Bylicka, M., Kajtoch, L., and Figarski, T. (2010). Habitat and landscape characteristics affecting the occurrence of Ural owls Strix uralensis in an agroforestry mosaic. Acta Ornithol. 45, 33–42. doi: 10.3161/000164510x516065
Christensen, W. (2018). A Greeting to disagree: using SAS to make reasoned decisions when information criteria select different models. SAS Conference Proceedings: Western Users of SAS Software 2018. September 5–7, 2018, Sacramento, California.
Fraixedas, S., Linden, A., and Lehikoinen, A. (2015). Population trends of common breeding forest birds in southern Finland are consistent with trends in forest management and climate change. Ornis Fenn. 92, 187–203.
Fridolfsson, A. K., and Ellegren, H. (1999). A simple and universal method for molecular sexing of non-ratite birds. J. Avian Biol. 30, 116–121. doi: 10.2307/3677252
Garcia-Salgado, G., Rebollo, S., Perez-Camacho, L., Martinez-Hesterkamp, S., De la Montana, E., Domingo-Munoz, R., et al. (2018). Breeding habitat preferences and reproductive success of northern goshawk (Accipiter gentilis) in exotic eucalyptus plantations in southwestern Europe. For. Ecol. Manag. 409, 817–825. doi: 10.1016/j.foreco.2017.12.020
Gates, J. E., and Gysel, L. W. (1978). Avian nest dispersion and fledging success in field-forest ecotones. Ecology 59, 871–883. doi: 10.2307/1938540
Granadeiro, J. P., Bolton, M., Silva, M. C., Nunes, M., and Furness, R. W. (2000). Responses of breeding Cory's shearwater Calonectris diomedea to experimental manipulation of chick condition. Behav. Ecol. 11, 274–281. doi: 10.1093/beheco/11.3.274
Green, D. J., and Cockburn, A. (2001). Post-fledging care, philopatry and recruitment in brown thornbills. J. Anim. Ecol. 70, 505–514. doi: 10.1046/j.1365-2656.2001.00503.x
Greenwald, D. N., Crocker-Bedford, D. C., Broberg, L., Suckling, K. F., and Tibbitts, T. (2005). A review of northern goshawk habitat selection in the home range and implications for forest management in the western United States. Wildl. Soc. Bull. 33, 120–128. doi: 10.2193/0091-7648(2005)33[120:Arongh]2.0.Co;2
Griesser, M., and Barnaby, J. (2010). “Families: a place of loving care and violent conflicts. The role of nepotism, cooperation and competition for the evolution of avian families” in New research in behavioral and chemical ecology. eds. W. Zhang and H. Liu (New York: Nova Science Publishers), 47–90.
Hakkarainen, H., Korpimäki, E., Laaksonen, T., Nikula, A., and Suorsa, P. (2008). Survival of male Tengmalm's owls increases with cover of old forest in their territory. Oecologia 155, 479–486. doi: 10.1007/s00442-007-0929-2
Hakkarainen, H., Mykra, S., Kurki, S., Korpimäki, E., Nikula, A., and Koivunen, V. (2003). Habitat composition as a determinant of reproductive success of Tengmalm's owls under fluctuating food conditions. Oikos 100, 162–171. doi: 10.1034/j.1600-0706.2003.11906.x
Hale, R., and Swearer, S. E. (2016). Ecological traps: current evidence and future directions. Proc. R. Soc. B-Biol. Sci. 283:20152647. doi: 10.1098/rspb.2015.2647
Hannan, E. J., and Quinn, B. G. (1979). Determination of the order of an autoregression. J. R. Stat. Soc. Ser. B-Methodol. 41, 190–1195. doi: 10.1111/j.2517-6161.1979.tb01072.x
Hipkiss, T., and Hörnfeldt, B. (2004). High interannual variation in the hatching sex ratio of Tengmalm's owl broods during a vole cycle. Popul. Ecol. 46, 263–268. doi: 10.1007/s10144-004-0195-7
Honkala, J., Lehikoinen, P., Saurola, P., and Valkama, J. (2022). Breeding and population trends of common raptors and owls in Finland in 2021 (in Finnish with English summary). Linnut-vuosikirja 2021, 62–77.
Huitu, O., Norrdahl, K., and Korpimäki, E. (2003). Landscape effects on temporal and spatial properties of vole population fluctuations. Oecologia 135, 209–220. doi: 10.1007/s00442-002-1171-6
Hurvich, C. M., and Tsai, C. L. (1989). Regression and time-series model selection in small samples. Biometrika 76, 297–307. doi: 10.2307/2336663
Jackson, H. B., and Fahrig, L. (2015). Are ecologists conducting research at the optimal scale? Glob. Ecol. Biogeogr. 24, 52–63. doi: 10.1111/geb.12233
Jacobsen, B. V., and Sonerud, G. A. (1987). Home range of Tengmalm's owl: a comparison between nocturnal hunting and diurnal roosting. USDA Serv. Gen. Tech. Rep. RM 142, 189–192.
Johnson, L. S., Hebert, R. M., Napolillo, F. M., and Allen, A. (2013). The process of fledging in the mountain bluebird. J. Field Ornithol. 84, 367–376. doi: 10.1111/jofo.12036
Johnson, L. S., Rauch, R. L., and Dellone, S. N. (2004). The process and causes of fledging in a cavity-nesting passerine bird, the house wren (Troglodytes aedon). Ethology 110, 693–705. doi: 10.1111/j.1439-0310.2004.01001.x
Kenward, R. E., Marcström, V., and Karlbom, M. (1993). Post-nestling behavior in goshawks, Accipiter gentilis: 1 The causes of dispersal. Anim. Behav. 46, 365–370. doi: 10.1006/anbe.1993.1198
Klaus, S., Mikkola, H., and Wiesner, J. (1975). Aktivität und Ernährung des Rauhfusskauzes Aegolius funeres (L.) während der Fortpflanzungsperiode. Zool. Jb. Syst. 102, 485–507.
Korpimäki, E. (1981). On the ecology and biology of Tengmalm's owl (Aegolius funereus) in southern Ostrobothnia and Soumenselkä, western Finland. Acta Univ Oul A 118, 1–84.
Korpimäki, E. (2020). Highlights from a long-term study of Tengmalm’s owls: cyclic fluctuations in vole abundance govern mating systems, population dynamics and demography. Brit. Birds 113, 316–333.
Korpimäki, E., and Hakkarainen, H. (1991). Fluctuating food supply affects the cluch size of Tengmalm’s owl independent of laying date. Oecologia 85, 543–552. doi: 10.1007/BF00323767
Korpimäki, E., and Hakkarainen, H. (2012). The boreal owl: Ecology, behavior and conservation of a forest-dwelling predator. Cambridge: Cambridge University Press, doi: 10.1017/CBO9780511844164.
Korpimäki, E., Huhtala, K., and Sulkava, S. (1990). Does the year-to-year variation in the diet of eagle and Ural owls support the alternative prey hypothesis. Oikos 58, 47–54. doi: 10.2307/3565359
Korpimäki, E., and Lagerström, M. (1988). Survival and natal dispersal of fledglings of Tengmalm's owl in relation to fluctuating food conditions and hatching date. J. Anim. Ecol. 57, 433–441. doi: 10.2307/4915
Korpimäki, E., and Norrdahl, K. (1991). Numerical and functional-responses of kestrels, short-eared owls, and long-eared owls to vole densities. Ecology 72, 814–826. doi: 10.2307/1940584
Korpimäki, E., Norrdahl, K., Huitu, O., and Klemola, T. (2005). Predator-induced synchrony in population oscillations of coexisting small mammal species. Proc. R. Soc. B-Biol. Sci. 272, 193–202. doi: 10.1098/rspb.2004.2860
Kouba, M., Bartoš, L., Bartošová, J., Hongisto, K., and Korpimäki, E. (2020). Interactive influences of fluctuations of main food resources and climate change on long-term population decline of Tengmalm’s owls in the boreal forest. Sci. Rep. 10:20429. doi: 10.1038/s41598-41020-77531-y
Kouba, M., Bartoš, L., Bartošová, J., Hongisto, K., and Korpimäki, E. (2021a). Long-term trends in the body condition of parents and offspring of Tengmalm's owls under fluctuating food conditions and climate change. Sci. Rep. 11:18893. doi: 10.1038/s41598-41021-98447-41591
Kouba, M., Bartoš, L., Korpimäki, E., and Zárybnická, M. (2015). Factors affecting the duration of nestling period and fledging order in Tengmalm's owl (Aegolius funereus): effect of wing length and hatching sequence. PLoS One 10:e0121641. doi: 10.1371/journal.pone.0121641
Kouba, M., Bartoš, L., and Šťastný, K. (2013). Differential movement patterns of juvenile Tengmalm's owls (Aegolius funereus) during the post-fledging dependence period in two years with contrasting prey abundance. PLoS One 8:e67034. doi: 10.61371/journal.pone.0067034
Kouba, M., Bartoš, L., and Šťastný, K. (2014). Factors affecting vocalization in Tengmalm's owl (Aegolius funereus) fledglings during post-fledging dependence period: scramble competition or honest signaling of need? PLoS One 9:e95594. doi: 10.91371/journal.pone.0095594
Kouba, M., Bartoš, L., Tomášek, V., Popelková, A., Šťastný, K., and Zárybnická, M. (2017). Home range size of Tengmalm's owl during breeding in Central Europe is determined by prey abundance. PLoS One 12:e0177314. doi: 10.0171371/journal.pone.0177314
Kouba, M., Dušek, A., Bartoš, L., Bušina, T., Hanel, J., Menclová, P., et al. (2020a). Low food abundance prior to breeding results in female-biased sex allocation in Tengmalm's owl (Aegolius funereus). J. Ornithol. 161, 159–170. doi: 10.1007/s10336-019-01707-1
Kouba, M., Slobodník, R., and Chavko, J. (2021b). Post-fledging dependence period, dispersal movements and temporary settlement areas in saker falcons (Falco cherrug). Raptor J. 15, 75–87. doi: 10.2478/srj-2021-0005
Laaksonen, T., and Lehikoinen, A. (2013). Population trends in boreal birds: continuing declines in agricultural, northern, and long-distance migrant species. Biol. Conserv. 168, 99–107. doi: 10.1016/j.biocon.2013.09.007
Larrat, S., Dallaire, A. D., and Lair, S. (2012). Emaciation and larval filarioid nematode infection in boreal owls (Aegolius funereus). Avian Pathol. 41, 345–349. doi: 10.1080/03079457.2012.688940
Lohmus, A. (2003). Do Ural owls (Strix uralensis) suffer from the lack of nest sites in managed forests? Biol. Conserv. 110, 1–9. doi: 10.1016/s0006-3207(02)00167-2
McFadzen, M. E., and Marzluff, J. M. (1996). Mortality of prairie falcons during the fledging-dependence period. Condor 98, 791–800. doi: 10.2307/1369859
Mikkola, A., Jaakkola, O., and Sucksdorff, Y. (1999). The slices- project: national classification of land use, land cover and soil, and the production of databases. Finnish Environ. 342, 1–86.
Morosinotto, C., Villers, A., Thomson, R. L., Varjonen, R., and Korpimäki, E. (2017). Competitors and predators alter settlement patterns and reproductive success of an intraguild prey. Ecol. Monogr. 87, 4–20. doi: 10.1002/ecm.1238
Naef-Daenzer, B., Widmer, F., and Nuber, M. (2001). Differential post-fledging survival of great and coal tits in relation to their condition and fledging date. J. Anim. Ecol. 70, 730–738. doi: 10.1046/j.0021-8790.2001.00533.x
Newton, I., Bell, A. A., and Wyllie, I. (1982). Mortality of sparrowhawks and kestrels. Brit. Birds 75, 195–204.
Nilsson, J.-Å. (1999). “fitness consequences of timing of reproductive” in Proceeding of 22nd International Ornithological Congress, Durban. eds. N. J. Adams and R. H. Slotow (Johannesburg: Birdlife South Africa), 234–247.
Nilsson, J.-Å., and Svensson, M. (1993). Fledging in altricial birds–parental manipulation or sibling competition. Anim. Behav. 46, 379–386. doi: 10.1006/anbe.1993.1200
Oksanen, T. (1990). Exploitation ecosystems in heterogeneous habitat complexes. Evol. Ecol. 4, 220–234. doi: 10.1007/bf02214331
Orrock, J. L., Danielson, B. J., and Brinkerhoff, R. J. (2004). Rodent foraging is affected by indirect, but not by direct, cues of predation risk. Behav. Ecol. 15, 433–437. doi: 10.1093/beheco/arh031
Overskaug, K., Bolstad, J. P., Sunde, P., and Øien, I. J. (1999). Fledgling behavior and survival in northern tawny owls. Condor 101, 169–174. doi: 10.2307/1370460
Peig, J., and Green, A. J. (2009). New perspectives for estimating body condition from mass/length data: the scaled mass index as an alternative method. Oikos 118, 1883–1891. doi: 10.1111/j.1600-0706.2009.17643.x
Pienkowski, M. W. (1983). A poor breeding season in 1983 for some waders on the Taymyr peninsula. Wader Study Group Bull. 39:34.
Radersma, R., Tinbergen, J. M., and Komdeur, J. (2011). Do brood sex ratio, nestling development and sex affect fledging timing and order? An experimental study on great tits. Anim. Behav. 81, 69–75. doi: 10.1016/j.anbehav.2010.09.007
Richards, S. A. (2005). Testing ecological theory using the information-theoretic approach: examples and cautionary results. Ecology 86, 2805–2814. doi: 10.1890/05-0074
Risely, A., Nightingale, J., Richardson, D. S., and Barr, I. (2013). Wing length and age, but not tarsus or mass, independently determine spring arrival at breeding territories in a long-distance migrant the common whitethroat Sylvia communis. Bird Study 60, 539–546. doi: 10.1080/00063657.2013.846293
Rohner, C., and Hunter, D. B. (1996). First-year survival of great horned owls during a peak and decline of the snowshoe hare cycle. Can. J. Zool. Rev. Can. Zool. 74, 1092–1097. doi: 10.1139/z96-121
Santangeli, A., Hakkarainen, H., Laaksonen, T., and Korpimäki, E. (2012). Home range size is determined by habitat composition but feeding rate by food availability in male Tengmalm's owls. Anim. Behav. 83, 1115–1123. doi: 10.1016/j.anbehav.2012.02.002
Schlaepfer, M. A., Runge, M. C., and Sherman, P. W. (2002). Ecological and evolutionary traps. Trends Ecol. Evol. 17, 474–480. doi: 10.1016/s0169-5347(02)02580-6
Schreiber-Gregory, D.N., and Jackson, H.M. (2017). Multicollinearity: What is it, why should we care, and how can it be controlled. Proceedings of the SAS R Global Forum 2017, Conference Paper 1404. Orlando, FL, USA: SAS.
Schwarz, G. (1978). Estimating the dimension of a model. Ann. Stat. 6, 461–464. doi: 10.1214/aos/1176344136
Selas, V., Steen, O. F., and Johnsen, J. T. (2008). Goshawk breeding densities in relation to mature forest in southeastern Norway. For. Ecol. Manag. 256, 446–451. doi: 10.1016/j.foreco.2008.04.047
Sergio, F. (2003). From individual behavior to population pattern: weather-dependent foraging and breeding performance in black kites. Anim. Behav. 66, 1109–1117. doi: 10.1006/anbe.2003.2303
Sunde, P. (2005). Predators control post-fledging mortality in tawny owls. Strix aluco. Oikos 110, 461–472. doi: 10.1111/j.0030-1299.2005.14069.x
Tao, J., Littel, R., Patetta, M., Truxillo, C., and Wolfinger, R. (2002). Mixed model analyses using the SAS system course notes. Carry, NC, United States: SAS Institute Inc.
Tarwater, C. E., Ricklefs, R. E., Maddox, J. D., and Brawn, J. D. (2011). Pre-reproductive survival in a tropical bird and its implications for avian life histories. Ecology 92, 1271–1281. doi: 10.1890/10-1386.1
Todd, L. D., Poulin, R. G., Wellicome, T. I., and Brigham, R. M. (2003). Post-fledging survival of burrowing owls in Saskatchewan. J. Wildl. Manag. 67, 512–519. doi: 10.2307/3802709
Tome, D. (2011). Post-fledging survival and dynamics of dispersal in long-eared owls Asio otus. Bird Study 58, 193–199. doi: 10.1080/00063657.2011.559531
Venier, L. A., Thompson, I. D., Fleming, R., Malcolm, J., Aubin, I., Trofymow, J. A., et al. (2014). Effects of natural resource development on the terrestrial biodiversity of Canadian boreal forests. Environ. Rev. 22, 457–490. doi: 10.1139/er-2013-0075
Vickery, W. L., and Bider, J. R. (1981). The influence of weather on rodent activity. J. Mammal. 62, 140–145. doi: 10.2307/1380484
Virkkala, R. (2016). Long-term decline of southern boreal forest birds: consequence of habitat alteration or climate change? Biodivers. Conserv. 25, 151–167. doi: 10.1007/s10531-015-1043-0
Vormwald, L. M., Morrison, M. L., Mathewson, H. A., Cocimano, M. C., and Collier, B. A. (2011). Survival and movements of fledgling willow and dusky flycatchers. Condor 113, 834–842. doi: 10.1525/cond.2011.110009
Weathers, W. W., and Sullivan, K. A. (1989). Juvenile foraging proficiency, parental effort, and avian reproductive success. Ecol. Monogr. 59, 223–246. doi: 10.2307/1942600
Widen, P. (1997). How, and why, is the goshawk (Accipiter gentilis) affected by modern forest management in Fennoscandia? J. Raptor Res. 31, 107–113.
Wiens, J. D., Noon, B. R., and Reynolds, R. T. (2006). Post-fledging survival of northern goshawks: the importance of prey abundance, weather, and dispersal. Ecol. Appl. 16, 406–418. doi: 10.1890/04-1915
Wilson, D. J., and Bromley, R. G. (2001). Functional and numerical responses of predators to cyclic lemming abundance: effects on loss of goose nests. Can. J. Zool.-Rev. Can. Zool. 79, 525–532. doi: 10.1139/cjz-79-3-525
Withey, J. C., Bloxton, T. D., and Marzluff, J. M. (2001). “Effects of tagging and location error in wildlife radiotelemetry studies” in Radio tracking and animal populations. eds. J. J. Millspaugh and J. M. Marzluff (San Diego: Academic Press), 43–70. doi: 10.1016/B978-012497781-5/50004-9
Keywords: birds of prey, mortality rate, post-fledging dependence period, predation, radio telemetry, small mammal abundance, starvation, weather variables
Citation: Kouba M, Bartoš L, Tulis F, Ševčík M, Sovadinová S, Bušina T, Janouš M, Kouba P, Bartošová J, Hongisto K and Korpimäki E (2023) Post-fledging survival of Tengmalm’s owl offspring in boreal forests: Interactive effects of varying dynamics of main prey and habitat composition. Front. Ecol. Evol. 11:1151622. doi: 10.3389/fevo.2023.1151622
Reviewed by:
Charles Krebs, University of British Columbia, CanadaGianpasquale Chiatante, University of Florence, Italy
Copyright © 2023 Kouba, Bartoš, Tulis, Ševčík, Sovadinová, Bušina, Janouš, Kouba, Bartošová, Hongisto and Korpimäki. This is an open-access article distributed under the terms of the Creative Commons Attribution License (CC BY). The use, distribution or reproduction in other forums is permitted, provided the original author(s) and the copyright owner(s) are credited and that the original publication in this journal is cited, in accordance with accepted academic practice. No use, distribution or reproduction is permitted which does not comply with these terms.
*Correspondence: Marek Kouba, bWFyZWtrb3ViYThAZ21haWwuY29t
†ORCID: Marek Kouba https://orcid.org/0000-0003-2262-5733%0D
Luděk Bartoš https://orcid.org/0000-0003-2667-5930
Filip Tulis https://orcid.org/0000-0003-2673-5630
Michal Ševčík https://orcid.org/0000-0003-4533-9887
Tomáš Bušina https://orcid.org/0000-0003-0301-5171
Erkki Korpimäki https://orcid.org/0000-0001-7596-1955