- 1Key Laboratory of Agro-ecological Processes in Subtropical Region, Institute of Subtropical Agriculture, Chinese Academic of Sciences, Changsha, China
- 2Guangxi Key Laboratory of Karst Ecological Processes and Services, Huanjiang Observation and Research Station of Karst Ecosystem, Chinese Academic of Sciences, Huanjiang, China
- 3Hunan Research Academy of Environmental Sciences, Institute of Water Ecology and Environment, Changsha, China
- 4College of Resources & Environment, Hunan Agricultural University, Changsha, China
- 5Management Center for Guangxi Mulun National Nature Reserve, Huanjiang, China
The effects of habitat filtering on community assembly have been extensively researched, and topography has been identified as a critical factor influencing the spatial distribution of trees. In this study, a 25-ha plot was established in karst evergreen–deciduous broadleaf forests in southwestern China. Eight topographical factors were used to divide plots into four habitat types, i.e., hilltop, steep slope, gentle slope, and depression, using a multivariate regression tree. A total of 85 evergreen and deciduous tree species were recorded in these four habitats and classified into three life stages, the differentiation of which was assessed using torus-translation tests. A total of 65 species significantly positively associated with at least one habitat and 79 species significantly negatively associated with at least one habitat were identified. Most species, whether evergreen or deciduous, exhibited a positive correlation with steep slopes, whereas relatively few species were adapted to depressions. Moreover, the percentage of evergreen species positively associated with hilltops and steep slopes was higher than that of deciduous species. Both evergreen and deciduous species showed an increasing percentage of positive correlation with hilltops from the sapling stage to the mature stage. However, more evergreen species grew on steep slopes in the sapling stage, whereas deciduous species grew in the mature stage. Canonical correspondence was used to analyze the relationship between species and the eight topographical factors. Regardless of life form or life stage, results showed that species distribution was significantly affected by topography. Furthermore, the distribution of evergreen species on sapling-stage trees was found to be more influenced by topography, whereas deciduous species were more influenced by topography in the mature stage. Finally, elevation was identified as the most crucial topographical factor affecting species distribution.
1 Introduction
The coexistence of species has long been a central focus of community ecology research (Nakashizuka, 2001; Wright, 2002; Silvertown, 2004), with niche differentiation and species diffusion limitation theories being among the most important in this regard (Whitfield, 2002; Lai et al., 2009). According to the niche theory, community structure is affected by environmental heterogeneity and the interactions between species, with each species carving out unique niches to avoid competitive exclusion (Chesson, 2000; Potts et al., 2002). Therefore, environmental factors, such as topography and soil, can shape the distribution of species through their species-level associations with habitats (Whittaker, 1956). Thus, it is important to investigate species–habitat associations at the community scale.
Previous research has demonstrated that niche differentiation plays a critical role in community maintenance and species coexistence at various scales (Clark et al., 1999; Tuomisto et al., 2003; Potts et al., 2004). However, the association between a single species and its habitat is often not determined during the early stages of seed germination or seedling growth but rather undergoes developmental changes at different life stages (Webb & Peart, 2000; Yamada et al., 2006; Comita et al., 2007; Lai et al., 2009). Moreover, the adaptability of specific species to particular habitats may not be constant, as their response to extreme weather events and global changes can vary (Condit et al., 1995). In addition, topography has been identified as a crucial driver of habitat diversity and variations in soil moisture and nutrient levels (Harms et al., 2001; Daws et al., 2002; Sorensen et al., 2006; John et al., 2007), which are essential for plant growth and development. In this respect, karst areas, which are characterized by a complex topography consisting of cliffs, caves, and sinkholes, as well as variable climate conditions (Clements et al., 2006), offer a diverse range of ecological niches that promote high species diversity. As a result, the uniqueness of the karst ecosystem makes it an especially valuable reservoir of biodiversity (Schilthuizen et al., 2004).
The phenology of leaves is of great importance in community appearance, understory environment, litter decomposition, and ecosystem productivity, as leaves are the main site of plant photosynthesis (Quigley & Platt, 2003). Deciduous tree species can avoid damage and the effects of adverse environmental conditions, such as low temperatures and drought, on growth by shedding leaves (Reich et al., 2004; Poorter & Markesteijn, 2008). Previous studies have shown that plants with different life forms have differences in leaf nutrient uptake efficiencies (Liu et al., 2006). Typically, deciduous species have a higher rate of nitrogen mineralization than evergreen species (Malhi et al., 2010), which are often found in barren habitats and, thus, considered to have higher nutrient re-uptake efficiencies (Chapin & Kedrowski, 1983; Chapin & Moilanen, 1991).
Southwest China is one of the three largest karst regions in the world (Jiang et al., 2014). The area features a distinctive evergreen–deciduous broadleaf forest, which boasts a complex community structure, high species diversity, and unique habitat heterogeneity (Du et al., 2017). However, the mechanisms underlying the species’ coexistence in local-scale plots were unclear, and consequently, based on studies conducted on Barro Colorado Island, Panama, we surveyed a 25-ha forest plot in Mulun National Natural Reserve in 2014. Our hypotheses are that there are significant species–habitat associations in the karst evergreen–deciduous broadleaf forest and that topographical factors significantly affect species distribution. Furthermore, we assume that the percentage of species–habitat association ratio for evergreen species in slope habitats is lower than that of deciduous species. We anticipate that this result will be evident even in the mature stage. The results of this study will serve as a foundation for future research on the structure of tree communities in the highly heterogeneous, species-rich karst evergreen–deciduous broadleaf forest. This study will also aid in preserving the vulnerable ecosystem in the area.
2 Materials and methods
2.1 Study area
The study was conducted in the Mulun National Natural Reserve (MNNR) (25°07′01″–25°12′22″N, 107°54′01″–108°05′51″E), located in the northwest of Huanjiang County, Guangxi Province, Southwest China. The study area’s maximum elevation is 1,028.0 m. The region has an annual average temperature of 15.7°C, January has an average temperature of 10.1°C, July has an average temperature of 28°C, the minimum temperature is 5.2°C, the frost-free period is 290 days, the average annual sunshine is 1,451 h, the average relative humidity is 70%, the average evaporation is 1,571.1 mm, the and average annual rainfall is 1,389.1 mm, with precipitation from April to September accounting for 70% of the annual total (Du et al., 2017). The geomorphological type comprises depressions between the karst hills. The soil is mainly dark or brown calcareous soil developed over carbonate rocks, which is a non-zonal shallow soil with high rock exposure, and the pH ranges from 7.06 to 7.68 (Du et al., 2019).
In 2014, we established a 25-ha (500 m × 500 m) forest plot within the MNNR. We subdivided the plot into a grid of 625 cells, each of which was 20 m × 20 m in size. Within these grids, we measured plant characteristics and topographical factors, i.e., elevation, slope, and slope aspect, as previously described by Condit (1998). This plot belongs to the Chinese Forest Biodiversity Monitoring Network (CForBio) and is currently the largest forest plot in the karst region, with elevations ranging from 442.6 m to 651.4 m and slopes ranging from 0.12° to 66.97° (Du et al., 2017) (Figure 1).
2.2 Data collection
In the year of plot establishment, we mapped the individuals of all tree species with a diameter at breast height (DBH) ≥ 1 cm and tagged them following standard field procedures of the Center for Tropical Forest Science (CTFS). The first census documented 144,552 free-standing individual trees. The region is characterized by a subtropical mixed evergreen deciduous broadleaf forest, dominated by Cryptocarya microcarpa F. N. Wei and Lindera communis Hemsl. Other important species include Itoa orientalis Hemsl, Platycarya longipes Wu, Decaspermum gracilentum (Hance) Merr. et Perry, Brassaiopsis glomerulata (Bl.) Regel, Diospyros dumetorum W. W. Smith, and Clausena dunniana Levl. (Lu et al., 2021).
Trees were divided into the sapling, juvenile, and mature stages based on DBH (Bagchi et al., 2011). Within each species, trees were ranked by DBH, and the 99th percentile (DBH99) was determined. All trees with a DBH > DBH992/3 were classified as the mature stage, trees with a DBH < DBH991/2 were classified as saplings, and those with a DBH991/2 ≤DBH < DBH992/3 were classified as juveniles (Guo et al., 2017). The species–habitat associations were examined for those species with individual stems greater than 25 in the sapling, juvenile, and mature stages. In total, 85 species including 52 evergreen species and 33 deciduous species were included (Supplementary Table S1).
In terms of topographical factors, average elevation (ELE), slope (SLO), aspect (ASP), convexity (CON), soil thickness (STK), rock outcrop ratio (ROC), topographic wetness index (TWI), and altitude above channel (ACH) were used as eight major topographical factors. The first four factors were computed following the methods described by Du et al. (2017), and the remaining factors were assessed following Liu et al. (2020). TWI and ACH were used to quantify topographical control on hydrological processes, where the TWI was calculated as the ratio of the area upslope from any given point on the landscape to the local slope at that point, and the ACH was calculated as the vertical distance from the channel network (Punchi-Manage et al., 2013); these two indicators make up the lack of hydrology.
2.3 Statistical analysis
2.3.1 Multivariate regression tree analysis
For species–habitat association analysis, we used a multivariate regression tree (MRT) to classify habitats into four categories, i.e., hilltop, steep slope, gentle slope, and depression (Supplementary Figures S1, S2). We computed the MRT procedure using the “mvpart” package. The data are presented in Table 1.
2.3.2 Species–habitat association analysis
To determine the association between a species and a particular type of habitat, we used the torus-transformation test, which is the most commonly used method in this regard (Harms et al., 2001), with a slight modification as described by Comita et al. (2007). This test entails calculating the probability of the true distribution of a species in each habitat under the condition of random distribution and determining whether a species is significantly correlated with a certain type of habitat based on probability. Detailed descriptions of the test can be found in a paper published by Harms et al. (2001). Our field survey obtained a species abundance data matrix for 625 samples in three size classes. We computed the torus-transformation test using the tt_test function of the “fgeo” package. Using canonical correspondence analysis (CCA) in the “vegan” package (Oksanen et al., 2020), we assessed the influence of topographical factors, and we used the Monte Carlo permutation test to evaluate their significance. We used the hierarchical partitioning method to distinguish a single topographical factor’s contribution via the “rdacca.hp” package in R (Lai et al., 2022). We performed all the above analyses in R4.0.2 (Team, 2020).
3 Results
3.1 Topographical habitat types and their characteristics
The forest plot covers a topographically heterogeneous area that contains two small peaks and a complete depression. The cross-validation relative error (CVRE) value of the MRT analysis was approximately 0.716 (Supplementary Figure S1). Hilltop was exposed to limestones characterized by high rock exposure, received direct sunlight, and was dry. The steep slope was the largest of the assessed Mulun habitats, whereas the gentle slope was located at the foot of the hill and contained large amounts of rubble and a number of caves, and the depression was low-lying and moist. With respect to tree density, steep slopes and the depression were found to have the highest and lowest densities, respectively (Supplementary Figure S2). The Shannon–Wiener index values (species diversity per quadrat) ranged from 1.03 (depression habitats) to 1.68 (steep slope habitats), whereas the values of the Patrick index (species richness per quadrat) ranged from 20.82 to 35.06. Steep-slope habitats had both the highest species richness and diversity (Table 1).
3.2 Associations of species with the four habitat types
Based on the torus-translation test analysis, only Pistacia weinmannifolia J. Poisson ex Franch among the total 85 species was neither positively nor negatively associated with any of the four habitat types, whereas 65 (75.6%) species were positively associated with at least one habitat, and 79 (91.9%) species were negatively associated with at least one habitat. Furthermore, 44 species showed a positive association with steep slopes, whereas only C. microcarpa F. N was positively associated with depression. However, most species were negatively associated with depression habitats.
We conducted separate analyses for evergreen and deciduous species and found that 73.1% of evergreen species and 79.4% of deciduous species were positively associated with at least one habitat. Species in two life forms showed similar habitat association distributions, with a higher percentage found on slopes or hilltops and a lower percentage in depressions. However, evergreen species were found to have a higher percentage of growth on hilltops and steep slopes than deciduous species, whereas the percentage of evergreen species growing on gentle slopes was lower than that of deciduous species. There was little difference between the evergreen and deciduous species in terms of their habitat association with depressions.
The percentage of evergreen and deciduous species growing on hilltops increased from the sapling stage to the mature stage. Among all species and evergreen species in particular, the highest percentage of sapling stage trees was found on steep slopes, whereas deciduous species had the highest percentage on mature stage trees. Additionally, both evergreen and deciduous species had the highest number of mature-stage trees on gentle slopes and in depressions (Figure 2).
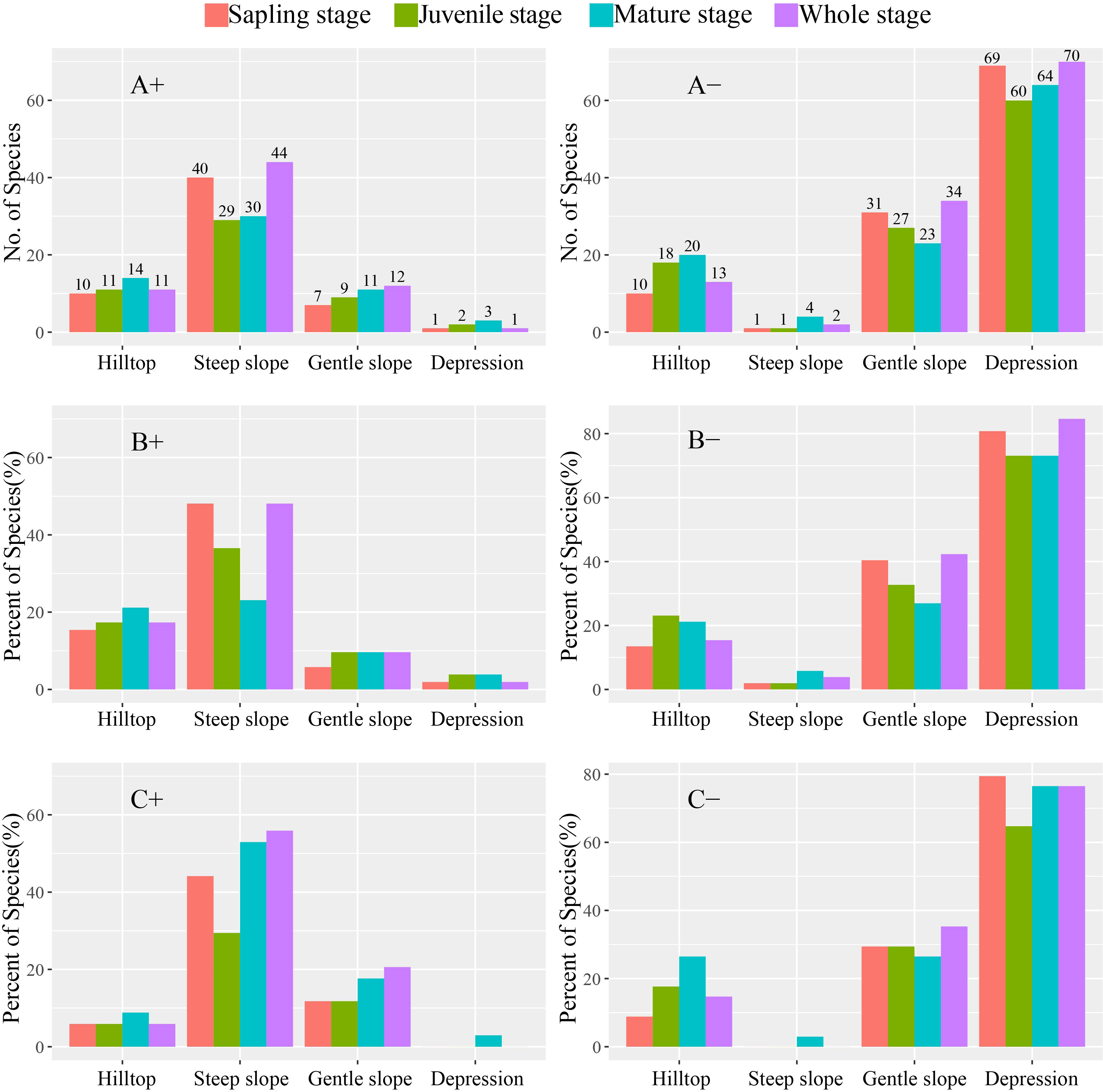
Figure 2 Number and percent of species in different stages positively and negatively associated with the four habitat types. (A) Whole, (B) evergreen, and (C) deciduous species. “+” and “−” indicate positive and negative, respectively.
3.3 The relationship between species distribution and topographical factors
Species distribution patterns as explained by CCA for all, evergreen, and deciduous species were similar. Regardless of life form or life stage, the permutation test showed that species distribution was significantly affected by topographic factors. When all, evergreen, and deciduous species were taken into consideration, topographical factors explained approximately 20.4%, 21.0%, and 18.2% of the variation, respectively (Table 2). The first axis explains most of the explainable parts across different stages (Figure 3) (Supplementary Figure S2). Among the eight topographic factors, ELE was the most important factor affecting species distribution in different life forms and stages, followed by SLO, CON, and ROC. Topographic factors explained the most variation in evergreen species at the sapling stage and deciduous species at the mature stage while explaining the least variation in evergreen and deciduous species at the juvenile stage (Table 2).
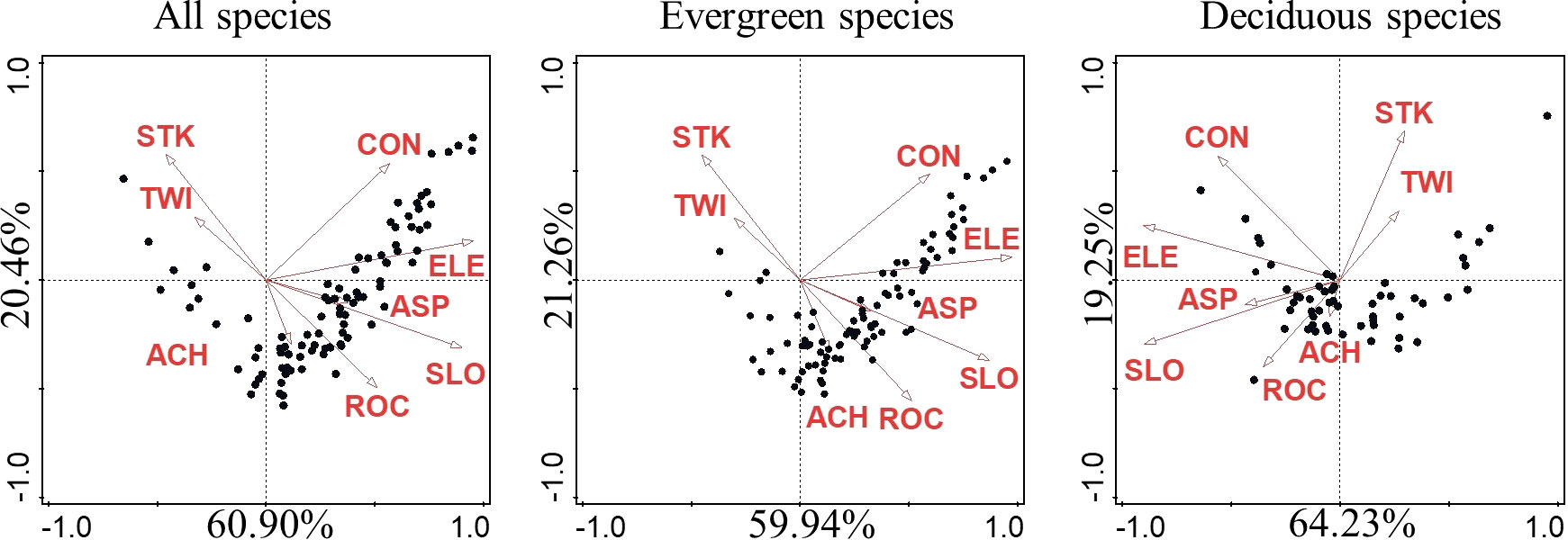
Figure 3 CCA diagram showing the relationship of species with the eight topographic factors. The black dots represent species. The percentage on the CCA axis represents the fraction of total explained variation. ELE, average elevation; SLO, slope; ASP, aspect; CON, convexity; STK, soil thick; ROC, rock outcrop ratio; TWI, topographic wetness index; ACH, altitude above channel; CCA, canonical correspondence analysis.
4 Discussion
4.1 Topographic habitat types and their characteristics
Karst, in southwest China, is characterized by large slopes and high rock exposure, which contribute to high habitat heterogeneity (Su et al., 2019). Although the habitat tends to be more homogeneous at the 20-m scale, a diverse range of niche types are widely distributed throughout the entire experiment plot because of the strong fragmentation of karst (Harms et al., 2001; Punchi-Manage et al., 2013; Guo et al., 2017). Along a hilltop-depression gradient within the MNNR, different habitat types occur. Our MRT analysis resulted in a CVRE value of 0.716, which is similar to values reported in previous studies (Kanagaraj et al., 2011; Punchi-Manage et al., 2013). Our study found that four habitat classifications covered most of the observed topographical variation. Given the spatial distribution of ecological factors (e.g., light, temperature, soil moisture, and soil nutrients), the differentiation of topography creates diverse habitats that contribute to distinct patterns of plant diversity and spatial distribution (Sorensen et al., 2006; John et al., 2007; Kanagaraj et al., 2011). On steep slopes, sunlight is more likely to penetrate the forest floor, thereby facilitating the regeneration and survival of a larger number and more diverse species. As a result, we found that steep slopes had the highest diversity index among the four habitat types assessed, whereas depressions tended to be more suitable for a small number of shade-tolerant plants (Table 1).
4.2 Habitat associations of species and the importance of topographical factors
We conducted torus-translation tests to examine the association between species with a DBH ≥ 1 cm and a density higher than 25 individuals in the 25-ha MNNR plot. The result showed that 84 (98.8%) species were significantly associated with at least one habitat type, with 65 (75.6%) and 79 (91.9%) species showing positive and negative associations, respectively. Values of 98.8% were compared with significant species–habitat association in the BCI, Sinharaja in the tropics, and Gutianshan in the subtropics at 33%, 79%, and 86%, respectively (Harms et al., 2001; Gunatilleke et al., 2006; Lai et al., 2009). However, our study found a lower percentage of positive associations (75.6%) when compared to Nonggang (85.1%), a tropical karst area in the same region (Guo et al., 2017). Only P. weinmannifolia J. Poisson ex Franch showed no clear habitat associations, likely due to its rapid growth and adaptability. Furthermore, we found that the distribution of 24 dominant species with importance > 1 was significantly correlated with habitat type (Du et al., 2017). These results suggest that species distribution in karst forests is strongly influenced by their responses to the environment, promoting strong habitat filtering. However, Baldeck et al. (2013) suggested that habitat filtering is stronger during the early stages of growth (DBH < 1 cm), leading to an underestimation of the percentages of species–habitat associations.
In this study, we found that most of the assessed species demonstrated a stronger species–habitat association on steep slopes, gentle slopes, and hilltops, whereas species growing in depressions were less common (Figure 2). Although the extent of the depression in the study plot was greater than that of hilltops (Table 1), the lack of sunlight in depressions limits plant survival, resulting in only a large number of shade-tolerant plants such as C. microcarpa F. N. Guo et al. (2017) suggested that the greater dependence of karst species on sunlight and their higher adaptability for higher elevations contribute to the higher abundance and diversity of species on slopes and hilltops. Consistent with this premise, we observed a greater number of species–habitat associations on steep slopes, which are characterized by greater sunlight exposure and a larger number of niches.
The number of species positively associated with hilltops increased from the sapling stage to the mature stage (Figure 2), indicating that while hilltops in karst areas are suitable for the growth and survival of trees, the greater abundance of pioneer species will be gradually replaced by other species during succession. Webb et al. (2002) and Wu et al. (2017) found that seed dispersal and seedling establishment are more likely to occur in moist habitats in a Bornean rainforest. In contrast, we recorded the highest number of species positively associated with steep slopes in the sapling stage, suggesting that species–habitat associations vary significantly according to different life stages (Battaglia et al., 2000; Dovciak et al., 2003). Negative density dependence in survival is incompatible with genetic shifts in resource requirements (Lusk, 2004; Comita et al., 2007). Our results show that the percentage of deciduous species at the mature stage was higher than that of evergreen species on steep slopes. This is because deciduous tree species have a greater requirement for sunlight, leading to higher tree height (Brando, 2018). Additionally, the crown coverage of dense species assemblages in depressions tends to create unfavorable conditions for deciduous tree growth (Malhi et al., 2010). Contrastingly, we found that both evergreen and deciduous species had the highest number in the mature stage on gentle slopes and depression, consistent with the result of the hilltops, which further shows that the germination of species was under strong environmental stress. These findings are generally consistent with those of previous studies (Comita et al., 2007; Bagchi et al., 2011; Hu et al., 2012), suggesting that species–habitat associations are largely influenced by the size class in a heterogeneous environment.
Based on our observations, there is little difference in the species–habitat associations of deciduous and evergreen species, except for a higher percentage of deciduous species growing on steep slopes at the mature stage (Figure 2). However, those observed differences may be attributed to the uniqueness of the karst study plot and strong niche differentiation (Guo et al., 2017). Furthermore, the results of CCA (Table 2) indicated that while topographical factors can explain the lower variation at juvenile trees, the distributions of the sapling, juvenile, and mature stages were relatively similar, further confirming the strong habitat filtering association with the karst ecosystem. Similar studies have suggested that seed dispersal may be the main driver of habitat change among different species (Comita et al., 2007; Kanagaraj et al., 2011). Therefore, in further studies, it is necessary to pay more attention to seed dispersal and seedling distribution. Furthermore, given the ecological fragility of karst areas and the fact that plants are primarily adapted to porous limestone bedrock and are highly sensitive to human activities, it is imperative to develop sound land-use regulations to protect biodiversity and prevent the further degradation of karst habitats.
5 Conclusion
Our study reveals that habitat heterogeneity plays an important role in maintaining diversity in the evergreen–deciduous broadleaf karst forests. The association between species and their habitats was found to be the strongest on slopes and hilltops and the weakest in depressions. Hilltops were suitable for tree growth and survival to maturity. Our results show that the proportion of evergreen species at the sapling stage was the highest on steep slopes, while deciduous species were dominant at the mature stage. Moreover, ELE emerged as the most important topographical factor affecting species distribution in the MNNR plot.
Data availability statement
The datasets generated during and/or analyzed during the current study are available from the corresponding author on reasonable request. Requests to access the datasets should be directed to hudu@isa.ac.cn.
Author contributions
HD, FZ, WP, and TS conceived and designed the experiments. HD, LS, LL, and ML conducted the experiments. LS, HD, and HW discussed the results. LS wrote the manuscript. The authors read and approved the final manuscript.
Funding
National Key Research and Development Program of China (2022YFF1300703), National Natural Science Foundation of China (42071073, 31971487), Youth Innovation Promotion Association of the Chinese Academy of Sciences (2021366), Guangxi Key Research and Development Program (AB17129009), and Hechi Distinguished Expert Program to FZ.
Acknowledgments
The authors thank two reviewers for helpful remarks on an earlier version of the paper.
Conflict of interest
The authors declare that the research was conducted in the absence of any commercial or financial relationships that could be construed as a potential conflict of interest.
Publisher’s note
All claims expressed in this article are solely those of the authors and do not necessarily represent those of their affiliated organizations, or those of the publisher, the editors and the reviewers. Any product that may be evaluated in this article, or claim that may be made by its manufacturer, is not guaranteed or endorsed by the publisher.
Supplementary material
The Supplementary Material for this article can be found online at: https://www.frontiersin.org/articles/10.3389/fevo.2023.1148910/full#supplementary-material
References
Bagchi R., Henrys P. A., Brown P. E., Burslem D. F. R. P., Diggle P. J., Gunatilleke C. V. S., et al. (2011). Spatial patterns reveal negative density dependence and habitat associations in tropical trees. Ecology 92, 1723–1729. doi: 10.1890/11-0335.1
Baldeck C. A., Harms K. E., Yavitt J. B., John R., Turner B. L., Valencia R., et al. (2013). Habitat filtering across tree life stages in tropical forest communities. Proc. R. Soc B 280, 20130548. doi: 10.1098/rspb.2013.0548
Battaglia L. L., Fore S. A., Sharitz R. R. (2000). Seedling emergence, survival and size in relation to light and water availability in two bottomland hardwood species. J. Ecol. 88, 1041–1050. doi: 10.1046/j.1365-2745.2000.00518.x
Chapin F. S., Kedrowski R. A. (1983). Seasonal changes in nitrogen and phosphorus fractions and autumn retranslocation in evergreen and deciduous Taiga trees. Ecology 64, 376–391. doi: 10.2307/1937083
Chapin F. S., Moilanen L. (1991). Nutritional controls over nitrogen and phosphorus resorption from Alaskan birch leaves. Ecology 72, 709–715. doi: 10.2307/2937210
Chesson P. (2000). Mechanisms of maintenance of species diversity. Annu. Rev. Ecol. Syst. 31, 343–366. doi: 10.1146/annurev.ecolsys.31.1.343
Clark D. B., Palmer M. W., Clark D. A. (1999). Edaphic factors and the landscape-scale distributions of tropical rain forest trees. Ecology 80, 2662–2675. doi: 10.1890/0012-9658(1999)080[2662:efatls]2.0.co;2
Clements R., Sodhi N. S., Schilthuizen M., Ng P. K. L. (2006). Limestone karsts of southeast Asia: Imperiled arks of biodiversity. Bioscience 56, 733–742. doi: 10.1641/0006-3568(2006)56[733:lkosai]2.0.co;2
Comita L. S., Condit R., Hubbell S. P. (2007). Developmental changes in habitat associations of tropical trees. J. Ecol. 95, 482–492. doi: 10.1111/j.1365-2745.2007.01229.x
Condit R. (1998). Tropical forest census plots: methods and results from Barro Colorado Island, Panama and a comparison with other plots (Berlin: Springer).
Condit R., Hubbell S. P., Foster R. B. (1995). Mortality-rates of 205 neotropical tree and shrub species and the impact of a severe drought. Ecol. Monogr. 65, 419–439. doi: 10.2307/2963497
Daws M. I., Mullins C. E., Burslem D., Paton S. R., Dalling J. W. (2002). Topographic position affects the water regime in a semideciduous tropical forest in Panama. Plant Soil 238, 79–90. doi: 10.1023/a:1014289930621
Dovciak M., Reich P. B., Frelich L. E. (2003). Seed rain, safe sites, competing vegetation, and soil resources spatially structure white pine regeneration and recruitment. Can. J. For. Res.-Rev. Can. Rech. For. 33, 1892–1904. doi: 10.1139/x03-115
Du H., Hu F., Zeng F., Wang K., Peng W., Zhang H., et al. (2017). Spatial distribution of tree species in evergreen-deciduous broadleaf karst forests in southwest China. Sci. Rep. 7, 15664. doi: 10.1038/s41598-017-15789-5
Du H., Liu L., Su L., Zeng F., Wang K., Peng W., et al. (2019). Seasonal changes and vertical distribution of fine root biomass during vegetation restoration in a karst area, Southwest China. Front. Plant Sci. 9. doi: 10.3389/fpls.2018.02001
Gunatilleke C. V. S., Gunatilleke I. A. U. N., Esufali S., Harms K. E., Ashton P. M. S., Burslem D. F. R. P., et al. (2006). Species-habitat associations in a Sri Lankan dipterocarp forest. J. Trop. Ecol. 22, 371–384. doi: 10.1017/s0266467406003282
Guo Y., Wang B., Mallik A. U., Huang F., Xiang W., Tao D., et al. (2017). Topographic species-habitat associations of tree species in a heterogeneous tropical karst seasonal rain forest, China. J. Plant Ecol. 10, 450–460. doi: 10.1093/jpe/rtw057
Harms K. E., Condit R., Hubbell S. P., Foster R. B. (2001). Habitat associations of trees and shrubs in a 50-ha neotropical forest plot. J. Ecol. 89, 947–959. doi: 10.1046/j.0022-0477.2001.00615.x
Hu Y. H., Lan G. Y., Sha L. Q., Cao M., Tang Y., Li Y. D., et al. (2012). Strong Neutral Spatial Effects Shape Tree Species Distributions across Life Stages at Multiple Scales. PloS One 7, e38247. doi: 10.1371/journal.pone.0038247
Jiang Z., Lian Y., Qin X. (2014). Rocky desertification in Southwest China: Impacts, causes, and restoration. Earth-Sci. Rev. 132, 1–12. doi: 10.1016/j.earscirev.2014.01.005
John R., Dalling J. W., Harms K. E., Yavitt J. B., Stallard R. F., Mirabello M., et al. (2007). Soil nutrients influence spatial distributions of tropical tree species. Proc. Natl. Acad. Sci. United States America 104, 864–869. doi: 10.1073/pnas.0604666104
Kanagaraj R., Wiegand T., Comita L. S., Huth A. (2011). Tropical tree species assemblages in topographical habitats change in time and with life stage. J. Ecol. 99, 1441–1452. doi: 10.1111/j.1365-2745.2011.01878.x
Lai J. S., Mi X. C., Ren H. B., Ma K. P. (2009). Species-habitat associations change in a subtropical forest of China. J. Veg. Sci. 20, 415–423. doi: 10.1111/j.1654-1103.2009.01065.x
Lai J. S., Zou Y., Zhang J. L., Peres-Neto P. R. (2022). Generalizing hierarchical and variation partitioning in multiple regression and canonical analyses using the rdacca.hp R package. Methods Ecol. Evol. 13, 782–788. doi: 10.1111/2041-210X.13800
Liu C., Berg B., Kutsch W., Westman C. J., Ilvesniemi H., Shen X., et al. (2006). Leaf litter nitrogen concentration as related to climatic factors in Eurasian forests. Glob. Ecol. Biogeogr. 15, 438–444. doi: 10.1111/j.1466-822x.2006.00251.x
Liu L., Zeng F. P., Song T. Q., Wang K. L., Du H. (2020). Stand structure and abiotic factors modulate karst forest biomass in Southwest China. Forests 11. doi: 10.3390/f11040443
Lu M. Z., Du H., Song T. Q., Peng W. X., Su L., Zhang H., et al. (2021). Drivers of tree survival in an evergreen-deciduous broadleaf karst forest in southwest China. For. Ecol. Manage. 499, 119598. doi: 10.1016/j.foreco.2021.119598
Lusk C. H. (2004). Leaf area and growth of juvenile temperate evergreens in low light: species of contrasting shade tolerance change rank during ontogeny. Funct. Ecol. 18, 820–828. doi: 10.1111/j.0269-8463.2004.00897.x
Malhi Y., Silman M., Salinas N., Bush M., Meir P., Saatchi S. (2010). Introduction: Elevation gradients in the tropics: laboratories for ecosystem ecology and global change research. Glob. Change Biol. 16, 3171–3175. doi: 10.1111/j.1365-2486.2010.02323.x
Nakashizuka T. (2001). Species coexistence in temperate, mixed deciduous forests. Trends Ecol. Evol. 16, 205–210. doi: 10.1016/s0169-5347(01)02117-6
Oksanen J., Blanchet F. G., Kindt R., Legendre P., Minchin P., O’hara R., et al. (2020). Community ecology package (R package version 2.5-7 2).
Poorter L., Markesteijn L. (2008). Seedling traits determine drought tolerance of tropical tree species. Biotropica 40, 321–331. doi: 10.1111/j.1744-7429.2007.00380.x
Potts M. D., Ashton P. S., Kaufman L. S., Plotkin J. B. (2002). Habitat patterns in tropical rain forests: A comparison of 105 plots in Northwest Borneo. Ecology 83, 2782–2797. doi: 10.2307/3072015
Potts M. D., Davies S. J., Bossert W. H., Tan S., Nur Supardi M. N. (2004). Habitat heterogeneity and niche structure of trees in two tropical rain forests. Oecologia 139, 446–453. doi: 10.1007/s00442-004-1525-3
Punchi-Manage R., Getzin S., Wiegand T., Kanagaraj R., Gunatilleke C. V. S., Gunatilleke I. A. U. N., et al. (2013). Effects of topography on structuring local species assemblages in a Sri Lankan mixed dipterocarp forest. J. Ecol. 101, 149–160. doi: 10.1111/1365-2745.12017
Quigley M. F., Platt W. J. (2003). Composition and structure of seasonally deciduous forests in the Americas. Ecol. Monogr. 73, 87–106. doi: 10.1890/0012-9615(2003)073[0087:casosd]2.0.co;2
Reich P. B., Uhl C., Walters M. B., Prugh L., Ellsworth D. S. (2004). Leaf demography and phenology in Amazonian rain forest: A census of 40000 leaves of 23 tree species. Ecol. Monogr. 74, 3–23. doi: 10.1890/02-4047
Schilthuizen M., Hoekstra R. F., Gittenberger E. (2004). Hybridization, rare alleles and adaptive radiation. Trends Ecol. Evol. 19, 404–405. doi: 10.1016/j.tree.2004.06.005
Silvertown J. (2004). Plant coexistence and the niche. Trends Ecol. Evol. 19, 605–611. doi: 10.1016/j.tree.2004.09.003
Sorensen R., Zinko U., Seibert J. (2006). On the calculation of the topographic wetness index: evaluation of different methods based on field observations. Hydrol. Earth Syst. Sci. 10, 101–112. doi: 10.5194/hess-10-101-2006
Su L., Du H., Zeng F., Peng W., Rizwan M., Nunez-Delgado A., et al. (2019). Soil and fine roots ecological stoichiometry in different vegetation restoration stages in a karst area, southwest China. J. Environ. Manage. 252, 109694. doi: 10.1016/j.jenvman.2019.109694
Tuomisto H., Ruokolainen K., Yli-Halla M. (2003). Dispersal, environment, and floristic variation of western Amazonian forests. Science 299, 241–244. doi: 10.1126/science.1078037
Webb C. O., Ackerly D. D., McPeek M. A., Donoghue M. J. (2002). Phylogenies and community ecology. Annu. Rev. Ecol. Syst. 33, 475–505. doi: 10.1146/annurev.ecolsys.33.010802.150448
Webb C. O., Peart D. R. (2000). Habitat associations of trees and seedlings in a Bornean rain forest. J. Ecol. 88, 464–478. doi: 10.1046/j.1365-2745.2000.00462.x
Whittaker R. H. (1956). Vegetation of the great smoky mountains. Ecol. Monogr. 26, 1–69. doi: 10.2307/1943577
Wright J. S. (2002). Plant diversity in tropical forests: a review of mechanisms of species coexistence. Oecologia 130, 1–14. doi: 10.1007/s004420100809
Wu H., Franklin S. B., Liu J. M., Lu Z. J. (2017). Relative importance of density dependence and topography on tree mortality in a subtropical mountain forest. For. Ecol. Manage. 384, 169–179. doi: 10.1016/j.foreco.2016.10.049
Keywords: environmental heterogeneity, habitat filtering, life form, species distribution, topography, karst forests
Citation: Su L, Du H, Zeng F, Wang H, Lu M, Luo L, Peng W and Song T (2023) Habitat associations of woody plant species in evergreen–deciduous broadleaf karst forests in southwest China. Front. Ecol. Evol. 11:1148910. doi: 10.3389/fevo.2023.1148910
Received: 20 January 2023; Accepted: 27 July 2023;
Published: 17 August 2023.
Edited by:
Cuicui Jiao, Sichuan University of Science and Engineering, ChinaReviewed by:
Nicolas Diaz-Kloch, Trent University, CanadaJuan-Jose Jiménez-Osornio, Universidad Autónoma de Yucatán, Mexico
Copyright © 2023 Su, Du, Zeng, Wang, Lu, Luo, Peng and Song. This is an open-access article distributed under the terms of the Creative Commons Attribution License (CC BY). The use, distribution or reproduction in other forums is permitted, provided the original author(s) and the copyright owner(s) are credited and that the original publication in this journal is cited, in accordance with accepted academic practice. No use, distribution or reproduction is permitted which does not comply with these terms.
*Correspondence: Hu Du, hudu@isa.ac.cn; Wanxia Peng, wxpeng@isa.ac.cn