- 1College of Life Sciences and Technology, Harbin Normal University, Harbin, China
- 2Key Laboratory of Biodiversity of Aquatic Organisms, Harbin Normal University, Harbin, China
- 3Modern Educational Technology and Experiment Center, Harbin Normal University, Harbin, China
In this study, we explored the driving factors behind plankton community structure. Due to the rapid development of cities, the occupation and development of wetland resources have increased lately, making the urban wetland ecosystems unstable and degrading the ecological functions gradually. Understanding the driving factors behind plankton community structure has certain theoretical and guiding significance for the protection, sustainable development, and ecological restoration of aquatic biodiversity in urban wetland ecosystems. We set up 12 sampling points in the Hulanhe Wetland, with the continuous monitoring of plankton from April to August and October 2021. The eco-environmental factors, plankton community structure, biodiversity index, resource use efficiency (RUE), and Bray–Curtis community turnover value were analyzed. A total of 209 species of 91 genera, 42 families, 11 classes, 22 orders of phytoplankton, and 90 species of four classes of zooplankton were identified. The community structure was mainly composed of Bacillariophyta, Chlorophyta, Cyanophyta, Protozoa, and Rotifera. To explore the correlation between phytoplankton and zooplankton, a correlation study was performed. We found a stable feeding preference between phytoplankton and zooplankton. The key influencing factors were identified by ordinary least squares regression, and the main driving factors of plankton community structure were discussed. The results showed that the stability of the Increased biodiversity and resource utilization efficiency have led to more stable plankton communities. This stability pattern is also strongly affected by water temperature, pH and total nitrogen in the external environment. This study will be helpful in the restoration of damaged wetlands, which would be beneficial for the protection of urban wetland ecosystems.
1. Introduction
Plankton is an important part of the aquatic ecosystem (MacArthur, 1955; Liu et al., 2021). It has a short life cycle and responds quickly to anthropogenic effects and natural changes. They are also considered good biological indicators for assessing wetland ecological health (Seilheimer et al., 2009; Easson and Lopez, 2019; Jonkers et al., 2019; Liu et al., 2021). Therefore, studying the plankton community structure is beneficial for wetland ecological environmental protection.
Community stability refers to the ability of a community to maintain its original structural and functional state, resist disturbance, or return to its original state after disturbance (Holling, 1973; Boucot, 1985; Sennhauser, 1991). It is an important manifestation of ecosystem function (Tilman, 1999; Pennekamp et al., 2018). Community structure is affected by the combined effects of biotic and abiotic factors, such as resource use efficiency (RUE) (Tian et al., 2017), biodiversity (Zhang H. et al., 2016), and water environmental factors (Schaum et al., 2016; Tian et al., 2016; Amorim and Moura, 2021). In the same natural aquatic ecosystem, different habitats often cause differences in plankton community structure partly due to different nutrient concentrations (Zheng et al., 2020). The application of RUE can eliminate community differences caused by different nutrient concentrations; therefore, it is widely used in the study of plankton community structure. Anthropogenic disturbance can destroy ecosystem function and brings challenges to ecological restoration. The correlation between RUE and community stability depends on the degree of disturbance to the ecosystem. In other words, with the aggravation of human disturbance, such as water pollution and fishery, RUE will decrease with the decrease in plankton species abundance (Norberg et al., 2001; Filstrup et al., 2014). In recent years, the correlation between plankton diversity and community stability has become one of the ecological hotspots in conservation biology. Communities with more species or complex interspecies associations are more stable than single-species communities (Mougi, 2022). Communities with high biodiversity have less competition among species, which makes the community more stable and the ecological function guarantee insure higher (MacArthur, 1955; Elton, 2020). In addition, prior studies have shown that total phosphorus (TP), total nitrogen (TN), water temperature (WT), and dissolved oxygen (DO) have also been confirmed to relate to plankton community stability (Tian et al., 2016; He et al., 2021).
Global climate changes and anthropogenic activities lead to a rapid decrease in urban wetland areas and threaten the habitat integrity and stability of the urban wetland ecosystems (Matchett and Fleskes, 2017; Zhang et al., 2020). The overall ecological condition of urban wetlands has shown a declining pattern over the years (Hui et al., 2011). The Hulanhe Wetland Reserve is located on the north bank of Songhua River, Heilongjiang Province. It is the largest natural urban wetland in China (Xing, 2016). The community structure of diverse animals (Gao et al., 2015), diverse plants (Cheng, 2013), hydrozoan (Liu, 2012), and phytoplankton in the Hulanhe Wetland and surrounding waters has received much attention(Hui et al., 2011; Lu et al., 2014; Jia et al., 2021). To date, most research on the water ecology of the Hulanhe Wetland has mainly focused on the effects of plankton, plant community composition, abundance changes, and environmental factors (Hui et al., 2011; Lu et al., 2014; Jia et al., 2021). However, the research on the stability of plankton community is still insufficient.
Our study focused on the Hulanhe Wetland Reserve and the Hulan River, a tributary of the Songhua River that flows through the wetland, in 2021. We aimed to describe the plankton community structures and the correlations between them in the Hulanhe Wetland, to screen and identify the main factors driving plankton community structure, and to provide a theoretical basis for the protection of wetland biodiversity in the Hulanhe Estuary for the restoration of the damaged wetland ecosystems. Therefore, we put forward the following hypotheses: (1) there was a specific corresponding tendency to a predatory relationship between phytoplankton and zooplankton in the Hulanhe Wetland and (2) the stability of the plankton community in the Hulanhe Wetland is driven and regulated by both biotic and abiotic factors. The validation of these hypotheses would provide a theoretical basis for the water environment management of the Hulanhe Wetland from the perspective of plankton stability, which is beneficial for local water quality management and makes specific contributions to the further research and development of the restoration of other damaged wetland ecosystems.
2. Materials and methods
2.1. Research area, sampling point layout, and sampling time
The Hulan River, a tributary of the Songhua River, is located in the central part of Heilongjiang Province, between N 45°09′-46°10′, E 126°76′-127°31′. The reserve extends in the east–west belt along the north bank of the Songhua River. The length of the reserve is 63.5 km from east to west, 21.3 km from north to south, and 179.5 km in circumference. According to the habitat characteristics of the Hulanhe Wetland, it is divided into 12 sampling points: water inlet areas (S1, S2, S3, and S12), water outlet areas (S9, S10, and S11), wetland protection areas (S4, S5, S6, S7, and S8), an area affected by agriculture (S2), and an area affected by fisheries (S10) (Figure 1). In this study, phytoplankton and zooplankton specimens were collected in spring (April, May), summer (June, July, August), and autumn (October) in 2021, and the physicochemical indicators of the water body were analyzed. The longitude and latitude data of the sampling points were collected via GPS, and ArcGIS 10.2 (ESRI, Redlands, CA, United States) was used to draw the sampling points map.
2.2. Environmental data collection and sampling
2.2.1. Environmental variables
At each sampling site, surface water samples (0–0.5 m) were collected in triplicate for physicochemical analyses. The collection of water quality samples and plankton samples from the Hulanhe Wetland was carried out simultaneously. The WT, pH, conductivity (Cond.), DO, and turbidity (Tur) were measured with a multi-parameter water quality analyzer (YSI ProPlus, YSI, United States). TP, TN, chemical oxygen demand (CODMn), and biochemical oxygen demand (BOD5) were the environmental factors analyzed in the laboratory within 24 h of sampling (GB 3838–2002).
2.2.2. Plankton sampling
Plankton samples and water samples were collected simultaneously in the Hulanhe Wetland. Phytoplankton (5 L) and zooplankton (5 L) samples were collected at 1 m depth from each sampling site. Phytoplankton samples were stored in acidified Lugol’s solution, and zooplankton samples were stored in formaldehyde (4%). All samples were stored for 24 h before analysis and concentrated to 50 mL (Zhang and Hang, 1991).
Phytoplankton and protozoa were examined at ×400 magnification (×10 eyepiece and ×40 objective) using an Olympus microscope (Optec B302, Chongqing, China) with a 0.1 mL plankton counting chamber. Rotifera, cladocera, and copepods were examined at ×200 magnification (×10 eyepiece and ×20 objective) using an Olympus microscope with a 1 mL plankton counting chamber. Majority of the phytoplankton samples were identified at the species level, and their abundance was expressed as cells L−1 (Zhang and Hang, 1991).
Enumerating plankton cells, usually requires settling of samples in order to concentrate the cells (Claessens and Prast, 2007). Precipitation and concentration are carried out in a cylindrical funnel. Fix the funnel on a shelf and place it on a stable table. The water sample was poured into the funnel, and the plankton was naturally precipitated for 60 h. The supernatant was sucked with a siphon and the sample was concentrated to 50 mL (Zhang and Hang, 1991).
The publications ‘Freshwater algae in China: systems, classification and ecology’ (Hu and Wei, 2006), ‘Freshwater Microorganisms and Benthic Animals Atlas’ (Zhou and Chen, 2011), ‘China Freshwater Rotifer’ (Wang, 1961), the Rotifer World Catalog1, International Commission on Zoological Nomenclature2, and ‘Chinese Fauna Freshwater Cladocera’ (Board, 1979) were used to identify the plankton organisms.
2.3. Statistical analysis
The dominant species of plankton were determined based on the dominance value Y for each species, and plankton diversity was represented by the Shannon–Wiener (H′) (Magnussen and Boyle, 1995), Margalef (H) (Margalef, 1969), Pielou evenness (J) (Pielou, 1966), and Simpson indices(D) (Simpson, 1949), as follows:
Shannon–Wiener index:
Margalef index:
Pielou evenness index:
Simpson index:
where and are the number of individuals of species and the total number of individuals of all species within a given area in the whole year, respectively; is the relative proportion of species , which is calculated by ; is the number of species within a given sample; and is the occurrence frequency of species , which is calculated by the ratio of the number of samples with species to the total number of samples within the given area in the whole year. If the dominance value of a species was greater than 0.02, it was considered dominant.
2.4. Plankton RUE
In this experiment, the mass per unit volume was used to represent the biomass of plankton, and the calculation formula was: biomass = density×volume×abundance×10−6. It is assumed that the density of plankton is 1 g/L, the volume unit is μm3, the abundance unit is cells/L, and the biomass unit is μg/L.
RUE represents the amount of standing stock biomass per unit resource. We evaluated the RUE of phytoplankton (RUEpp) and zooplankton (RUEzp) communities, which quantifies the ratio of realized to potential productivity. RUEpp was calculated as phytoplankton biomass per unit TP (Davidson and Howarth, 2007; Filstrup et al., 2014). RUEzp was calculated as zooplankton biomass per unit of phytoplankton biomass. As we were interested in calculating community biomass per unit resource directly available to each trophic level, RUEzp was calculated in relation to phytoplankton biomass, whereas RUEpp was calculated in relation to TP (Filstrup et al., 2014).
Here, is phytoplankton biomass; is zooplankton biomass; and is total phosphorus.
Plankton community turnover was determined by Bray–Curtis dissimilarity index between the two samples; the smaller the community turnover, the stronger the community stability (Jeppesen et al., 2011; Filstrup et al., 2014). Bray–Curtis dissimilarity index was calculated as:
Here, BC is the Bray–Curtis dissimilarity index between samples 1 and 2; and are the biomasses of plankton species in the two samples, respectively; and is the total number of plankton species in both the samples.
2.5. Data analysis
Analysis of variance (ANOVA) and Post-hoc test were conducted to evaluate differences in environmental data and was performed using SPSS 22.0 for Windows. Pearson correlation coefficient between community stability and RUE, biodiversity, and water environment factors were determined using SPSS 22.0 for Windows. Then, Origin 2022 was used for ordinary least squares regression analysis. The ‘vegan’, ‘ggpubr’, ‘ggplot2’, and ‘corrplot’ packages in R 4.0.3 were used for the difference tests and heat maps.
3. Results
3.1. Physicochemical characteristics of water samples
There were significant differences in WT (ANOVA, p < 0.001), pH (ANOVA, p < 0.001) and TN (ANOVA, p < 0.001) (Tables 1, 2). The variation range of WT was 8.10–27.80°C and an average of 18.07°C. The variation pattern of Tur, DO, and BOD5 was to increase gradually with the change of season, with a variation range of 1.70–186.80 NTU (Tur), 4.47–14.11 mg/L (DO), and 0.05–10.04 mg/L (BOD5), The mean values were 54.65 NTU (Tur), 9.96 mg/L (DO), and 5.53 mg/L (BOD5). TN decreased gradually with the change of spring, summer, and autumn, with a variation range of 0.15–0.78 mg/L and an average of 0.46 mg/L. The pH, CODMn, TP, and Cond. values were the greatest in summer, followed by in spring, and the lowest in autumn, ranging from 6.47–9.06 (pH), 2.47–15.80 mg/L (CODMn), 0.03–1.03 mg/L (TP), and 160.90–1123.00 μS/cm (Cond.), with an average of 7.72 (pH), 6.46 mg/L (CODMn), 0.30 mg/L (TP), and 359.03 μS/cm (Cond.).
3.2. Plankton community structure
3.2.1. Composition of plankton community
The species composition and dominant species of the plankton community in the Hulanhe Wetland are shown in Figures 2, 3 and Tables 3, 4. The identified phytoplankton in the Hulanhe Wetland belonged to eight phyla, 11 classes, 22 orders, 42 families, 91 genera, and 209 species. Among them, Bacillariophyta had the most number of species (74 species), followed by Chlorophycophyta (73 species), Cyanophyta (32 species), Euglenophyta (16 species), Pyrrophyta (6 species), Cryptophyta (4 species), Chrysophyta (3 species), and Xanthophyta (1 species), respectively.
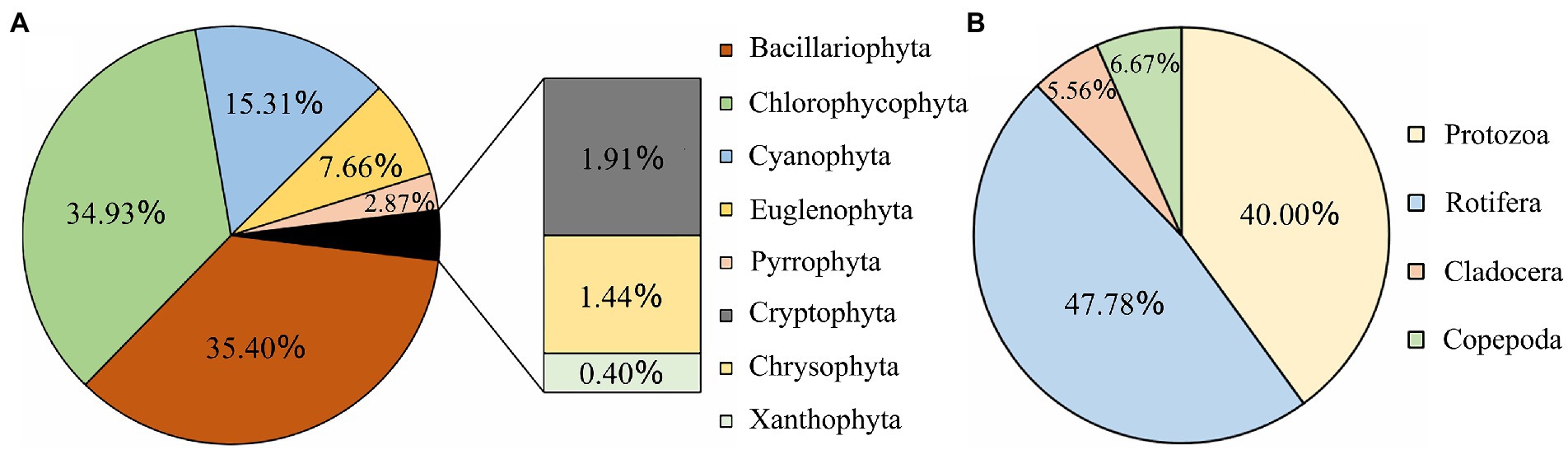
Figure 2. Schematic diagram of plankton species distribution in Hulanhe Wetland. (A) Phytoplankton. (B) Zooplankton.
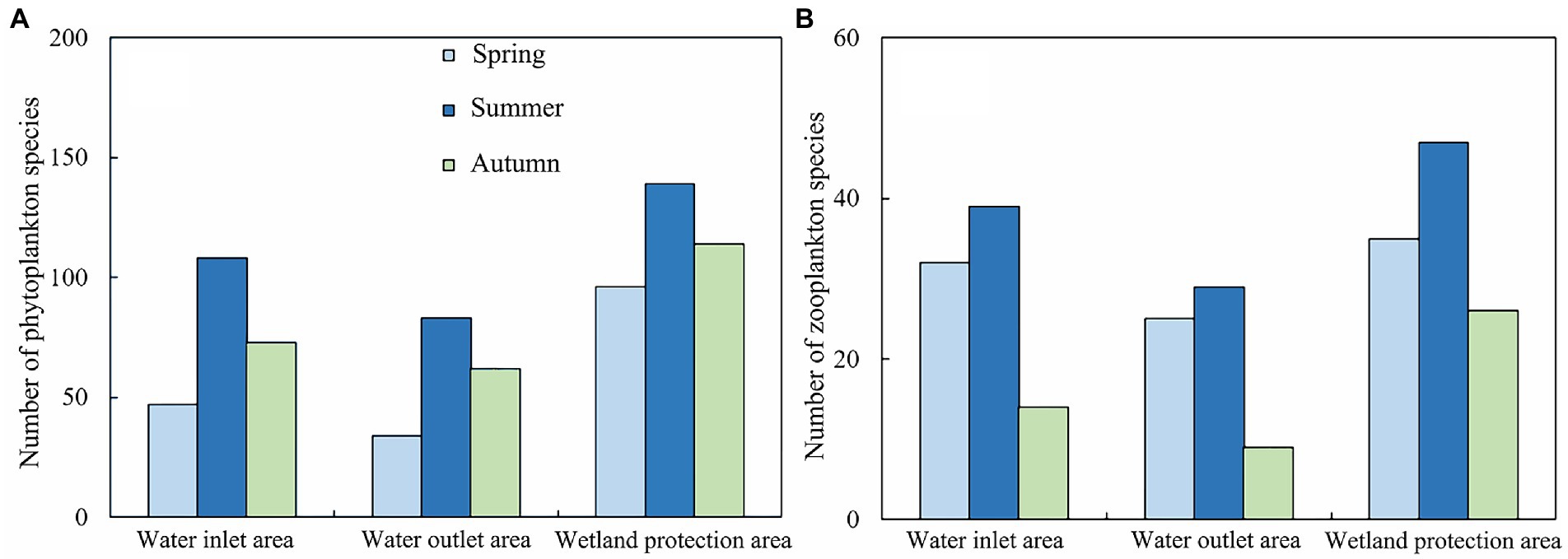
Figure 3. Schematic diagram of plankton species distribution in Hulanhe Wetland. (A) Phytoplankton. (B) Zooplankton.
A total of four categories and 90 species of zooplankton were identified in the Hulanhe Wetland, including 43 species of rotifers, 36 species of protozoa, 5 species of copepods, and 6 species of cladocerans. There were 18 dominant species of zooplankton. The dominant species of zooplankton in spring and summer were mainly protozoa and rotifers. The dominant species in autumn were less, mainly copepods. Figure 3 shows the spatio-temporal distribution of plankton species in the Hulanhe Wetland. In terms of time distribution, the number of phytoplankton species was in the order of summer>autumn>spring and the number of zooplankton species was in the order of summer>spring>autumn.
There were 39 dominant species of phytoplankton, with a significant seasonal distribution. In spring, the dominant species were mainly Bacillariophyta and Cryptophyta, such as Cyclotella meneghiniana and Chroomonas acuta. The dominant species in summer were Chlorophyta, Cyanophyta, and Chrysophyta, among which Ankistrodesmus angustus, Arthrospira platensis, and Dinobryon cylindricum were the most dominant. Bacillariophyta and some cyanobacteria were the dominant species in autumn, and Cyclotella meneghiniana and Chroomonas acuta were the dominant species in spring. The spatial distribution also had certain differences. The proportions of the dominant species (cyanobacteria) in the water outlet areas were higher than those in the water inlet areas, while the proportions of other categories were lower than those in the water inlet areas. The dominant species in the wetland protection area were evenly distributed and much higher than those in the water inlet and outlet areas. Melosira granulata had the highest dominance in the Hulanhe Wetland. There were eight dominant species of protozoa, among which Strombidium viride had the highest dominance in spring, while Difflugia acuminate had absolute dominance in summer. There were seven dominant species of rotifers, Polyarthra euryptera in spring and Polyarthra euryptera and Keratella valga in summer. There were three dominant species of copepods. Nauplius was mainly dominant in summer, and the dominance of Neutrodiaptomus incongruens was the highest in autumn. Cladocera was the least dominant with only one species. From the perspective of spatial variation, the dominant species in the water inlet areas were mainly rotifers (such as Anuiaeopsis fissa and Polyarthra euryptera), while that at the water outlet areas were protozoa (such as Difflugia acuminate). The dominant species in the wetland reserve were generally higher than those in the water inlet and outlet areas. Rotifers, protozoa, copepods, and cladocera were mainly distributed, among which Neutrodiaptomus incongruens and Polyarthra euryptera had the highest dominance in the entire region.
3.2.2. Plankton abundance
The variation range of phytoplankton abundance in the three seasons in 2021 was 0.53–16.61 × 106 cells/L. The average abundance of phytoplankton in the time scale was in the order summer>autumn>spring, and the variation range was 0.99–16.61 × 106 cells/L (summer), 0.85–14.96 × 106 cells/L (autumn), and 0.53–13.55 × 106 cells/L (spring) (Figure 4A). The zooplankton community structure also showed temporal distribution differences, and the community abundance was mainly dominated by protozoa and rotifers. The abundance of zooplankton in the three seasons in 2021 was in the range 0.15–8.60 × 104 cells/L, and the abundance of zooplankton species was in the order summer>autumn>spring, with a range of 0.20–8.60 × 104 cells/L (summer), 0.15–3.25 × 104 cells/L (autumn), and 0.20–4.08 × 104 cells/L (spring) (Figure 4C).
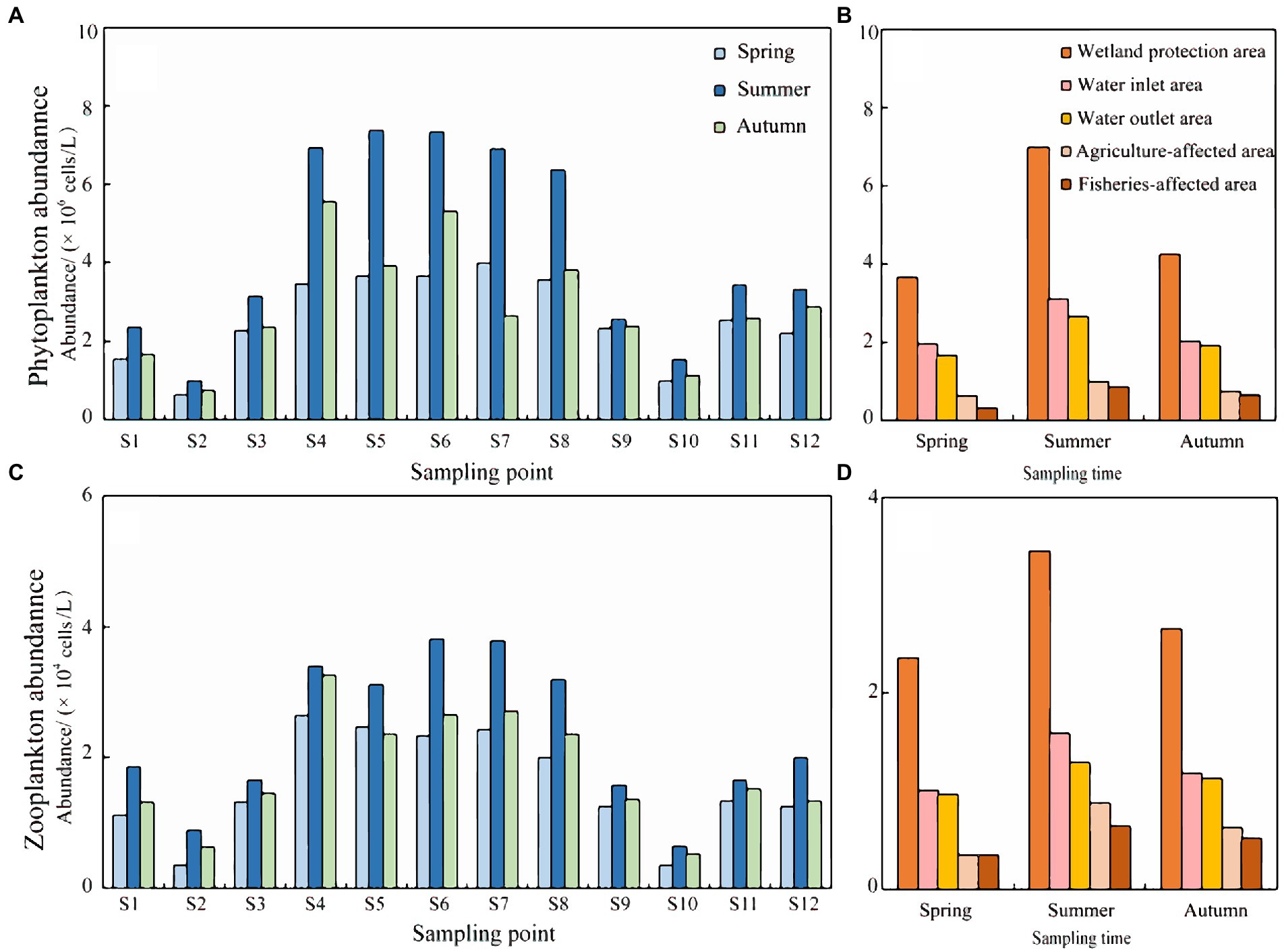
Figure 4. Schematic diagram of plankton abundance in Hulanhe Wetland. (A) Temporal distribution of phytoplankton abundance. (B) Spatial distribution map of phytoplankton abundance. (C) Time distribution map of zooplankton abundance. (D) Spatial distribution of zooplankton abundance.
The variation in phytoplankton abundance on a spatial scale was in the order wetland protection area > water inlet area > water outlet area > agriculture-affected area > fisheries-affected area. The wetland protection area had the highest abundance, with a range of 0.53–15.75 × 106 cells/L; the abundance in the water inlet area was the second, with a range of 0.85–8.67 × 106 cells/L; the abundance of the water outlet area ranged from 1.92–9.43 × 106 cells/L; the abundance was the lowest in the agriculture-affected area and fisheries-affected area, with a variation range of 0.05–4.28 × 106 cells/L and 0.22–5.61 × 106 cells/L, respectively (Figure 4B). The overall spatial variation of zooplankton was in the order wetland protection area > water inlet area > water outlet area > agriculture-affected area > fisheries-affected area. The wetland protection area had the highest abundance, with a range of 2.25–8.60 × 104 cells/L; the abundance in the water inlet area was second, with a range of 0.20–7.25 × 104 cells/L; the variation ranges were 0.20–2.25 × 104 cells/L, 0.35–3.00 × 104 cells/L, and 0.20–0.95 × 104 cells/L for water outlet area, agriculture-affected area, and fisheries-affected area, respectively (Figure 4D).
3.2.3. Correlation between phytoplankton and zooplankton
Predation and competition are the main driving forces for regulating the structure of plankton communities in freshwater ecosystems. Plankton can be classified into phytoplankton and zooplankton according to the trophic relationship (Bottom-up/Top-down effect) of the food web, and there is a long-term predation and predation relationship between them. In this study, the correlations between phytoplankton (eight phyla) and zooplankton (four categories) were analyzed by correlation heatmap (Figure 5). As shown in the diagram, rotifers showed a significant correlation between Chlorophyta and Cryptophyta (p < 0.01); there was a significant correlation between Protozoa and Bacillariophyta (p < 0.05). However, compared with Chlorophyta, Cyanophyta was not significantly correlated with zooplankton although it was the dominant phylum. It may be because Cyanophyta clusters often have gum sheaths and cyanobacterial toxins, resulting in discomfort and rarely preyed by zooplankton.
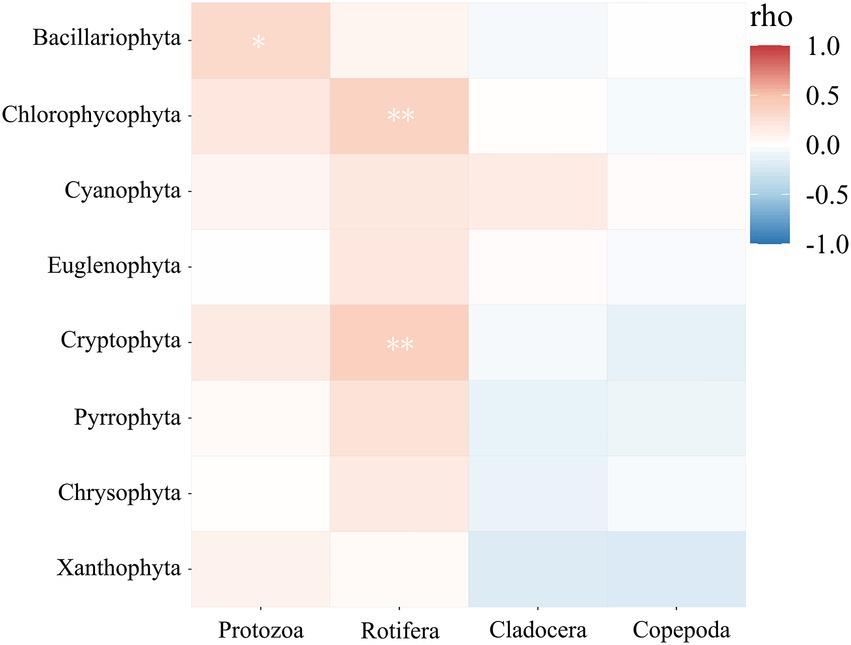
Figure 5. Heat map of correlation between phytoplankton and zooplankton in Hulanhe Wetland. **represents extremely significant correlation between groups (p<0.01); *Significant correlation between representative groups (p<0.05).
3.3. Factors affecting plankton community stability
3.3.1. Resource utilization and plankton community stability
As shown in Figures 6A,B the utilization rate of phytoplankton resources (RUEpp) and zooplankton resources (RUEzp) in the Hulanhe Wetland showed that they were much larger in summer than those in autumn and spring. As shown in Figures 6C,D, both RUEpp and RUEzp showed that they much larger in the wetland protected areas (S4–S8) than those in other regional samples, while RUEpp and RUEzp, in the agriculture-affected area (S2) and fisheries-affected area (S10) were the lowest. The community turnover (BC) of the plankton community showed an opposite trend to the community stability. The larger the BC, the easier the community to produce species turnover (that is, the worse the community stability). The ordinary least squares regression curve between plankton community stability and RUE in Hulanhe Wetland is shown in Figure 6E. There was a significant correlation between stability and RUE (p < 0.01), indicating that plankton communities with high resource utilization often have higher stability.
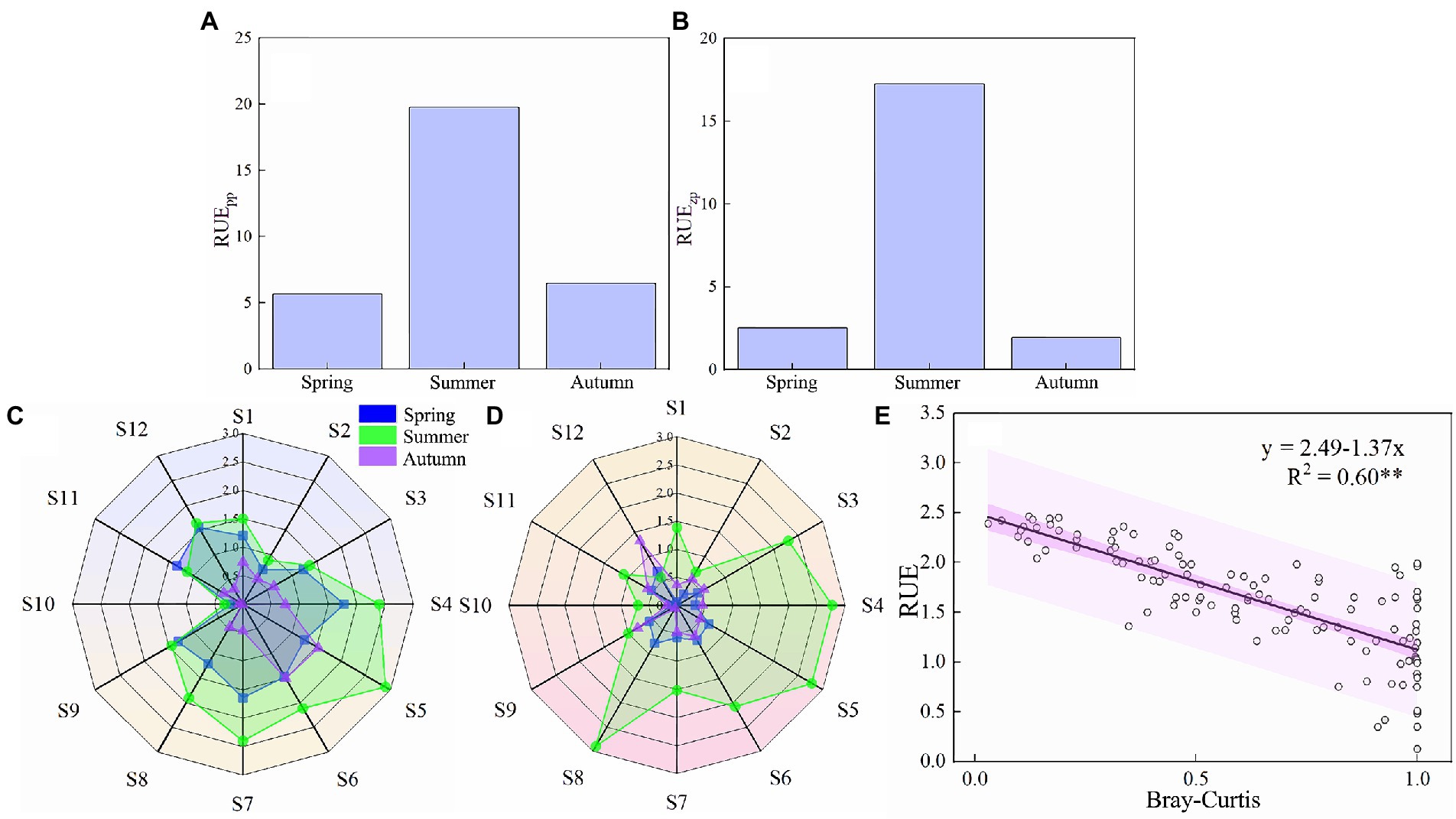
Figure 6. Relationship between the utilization rate of plankton community resources and community turnover in Hulanhe Wetland. (A) Average RUEpp in different seasons. (B) Average RUEzp in different seasons. (C) Radar map of sample points of RUEpp. (D) Radar map of RUEzp. (E) Ordinary least regression curve between RUE and community turnover.
3.3.2. Species diversity and plankton community stability
As shown in Figure 7, the Margalef diversity (H), Pielou evenness (J), Shannon-Wiener diversity (H′), and Simpson ecological dominance indices (D) of plankton in the Hulanhe Wetland in three seasons were calculated. The variation ranges were 1.36–3.89 (H), 0.39–0.81 (J), 1.43–3.54 (H′), and 0.43–0.88 (D). The diversity of plankton communities was generally higher in summer than that in spring and autumn. The diversity of sampling sites (S4–S8) in wetland reserves was higher than that in other sites, and the areas affected by agriculture and fishery (S2, S10) were generally lower than other sites. The linear relationship between plankton community stability and species diversity is shown in Figure 8. BC had a significant positive correlation with H and J, and there was no significant linear relationship with H′ and D. This shows that with the increase in species diversity and evenness, the community structure of plankton in the Hulanhe Wetland tended to be stable.
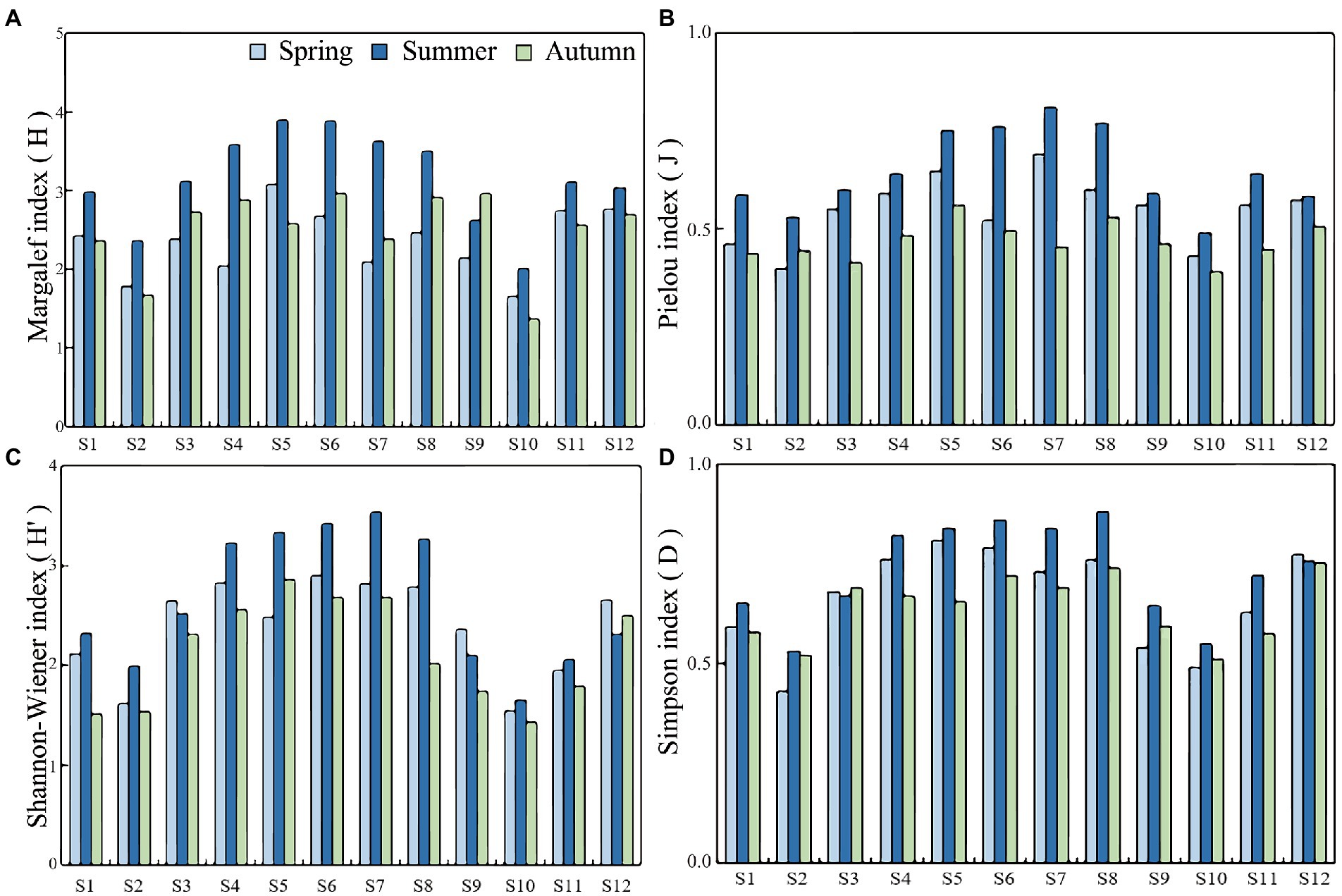
Figure 7. Species diversity index of plankton at various site of Hulanhe Wetland in different seasons. (A) Margalef diversity index. (B) Pielou evenness index. (C) Shannon-Wienner index. (D) Simpson’s index.
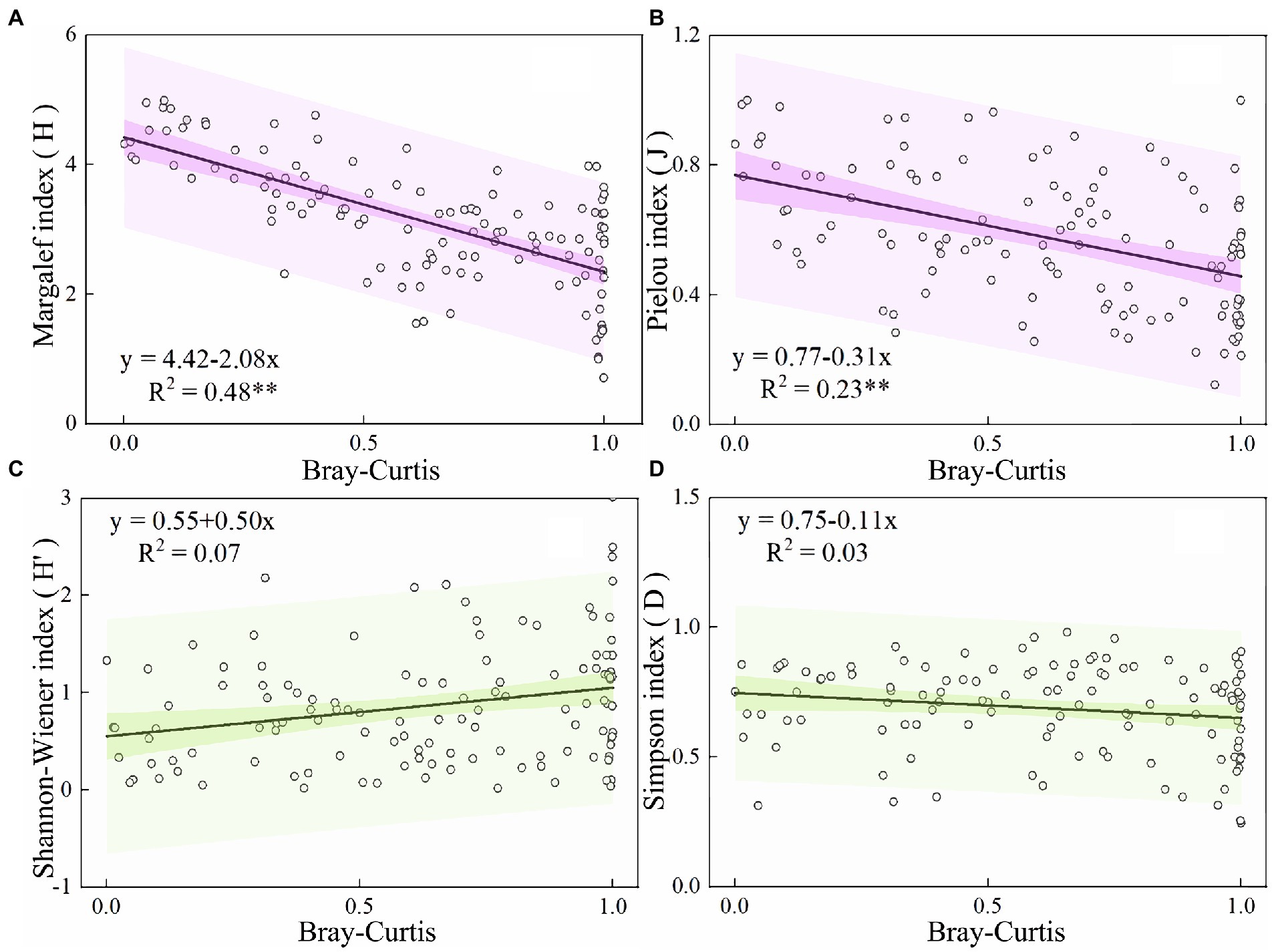
Figure 8. Relationship between biodiversity index and community turnover of phytoplankton community in Hulanhe Wetland. (A) Ordinary least squares regression curve between Margelef diversity index and community turnover. (B) Pielou evenness index. (C) Shannon-Wiener index. (D) Simpson’s index.
3.3.3. Environmental factors and plankton community stability
The ordinary least squares equation of the stability of the plankton community and environmental factors in the Hulanhe Wetland is shown in Figures 9, 10. There was a significant negative correlation between the stability of the phytoplankton community and WT and pH (p < 0.01), and there was a significant negative correlation between the stability of the zooplankton community and WT, pH, and TN (p < 0.01). There was no significant linear relationship between other environmental factors, indicating that only some water environmental factors can increase the stability of the plankton community.
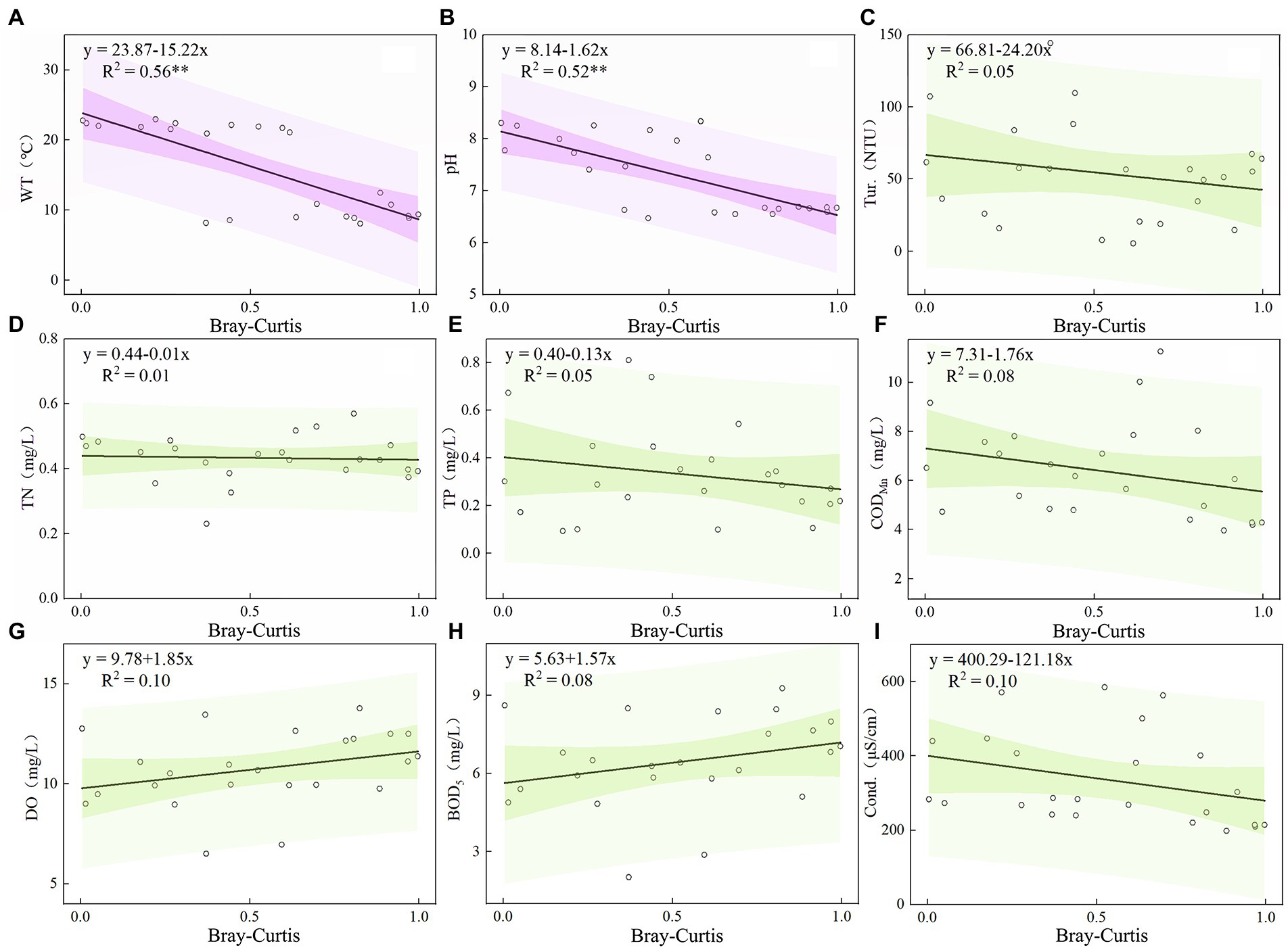
Figure 9. Relationship between Environmental Factors and Community Turnover of Phytoplankton Community in Hulanhe Wetland. (A) Water temperature and community turnover. (B) pH. (C) Turbidity. (D) Total nitrogen. (E) Total phosphorus. (F) Permanganate index. (G) Dissolved oxygen. (H) Bio-chemical oxygen demand. (I) Conductivity.
4. Discussion
4.1. Plankton community structure
4.1.1. Spatio-temporal distribution characteristics of plankton community
During the study period, the plankton community structure in the Hulanhe Wetland showed some heterogeneity at the spatial and temporal scales (Tables 2, 3 and Figures 3, 4). The Hulanhe Wetland was affected by the temperate monsoon climate, and the physicochemical factors of the waterbody changed between seasons. In particular, the change in WT can directly affect the community composition and succession direction of phytoplankton in the Hulanhe Wetland. In spring and autumn, when the Hulanhe Wetland was just released or was about to enter the frozen period, the WT was low, and the dominant species of phytoplankton were mostly cold-tolerant Bacillariophyta, such as Melosira varians, Cyclotella meneghiniana. However, after entering summer, the WT and light intensity increased rapidly, and cyanobacteria and Chlorophycophyta that prefer warm water began to multiply and gradually occupy a dominant position (Zhu et al., 2022), such as Arthrospira platensis, Anabaenopsis circularis, and Ankistrodesmus falcatus. The results of this experiment are similar to the results of phytoplankton community characteristics and succession rules of Hui et al. (2011), Lu et al. (2014), and Jia et al. (2021) in 2013, 2018 and 2019, respectively. WT is an important environmental factor affecting the phytoplankton community, which further indicates that the composition and succession direction of phytoplankton community in the Hulanhe Wetland were relatively stable in the past 10 years.
From the perspective of the zooplankton community structure, high similarity in species composition for three seasons in 2021 and the temporal variation was not obvious. The proportion of species and abundance of small zooplankton (protozoa, rotifers) was higher than that of large zooplankton (cladocerans, copepods). The community structure of zooplankton showed a trend of miniaturization, such as Centorpyxis aculeata, Tintinnidium fluviatile, and Polyarthra trigla, which was similar to the results of other wetland studies on the distribution of zooplankton (Chen et al., 2014; An et al., 2017; Wang et al., 2017). The reason may be that protozoa and rotifers have small size, short cycle, and rapid development (Arndt, 1993; Li et al., 2017). They can quickly adapt to changes in hydrological conditions and physicochemical environment in the water body; therefore, they often occupy a dominant position in wetland habitats. This result is consistent with the research results of Zhang et al. (2018) showing a trend of miniaturization of zooplankton community structure.
Anthropogenic activities and urban sewage are the reasons behind the nutrient content of the Hulan River being at a relatively high-level (Xing, 2016), and wetlands can restore the water quality of polluted rivers (Zheng et al., 2014). As the largest urban wetland in China, Hulanhe Wetland has achieved great results in ecological restoration in recent years. From the spatial scale analysis, phytoplankton and zooplankton species abundance changes are consistent. The species number and average abundance of plankton in the Hulanhe Wetland protection area were much larger than those in the Hulanhe Wetland water outlet and inlet areas, which may be because the wetland had the function of purifying water and depositing nutrients, and the slow water flow rate in the wetland caused the deposition of nutrients (Kobayashi et al., 2014), which was also conducive to the growth and reproduction of plankton, such as Cyclotella meneghiniana, Arthrospira platensis, and Tintinnidium fluviatile. Compared with the area outside the wetland, the species number and abundance of plankton in the water inlet area were larger than those in the water outlet area. The richness and abundance of plankton in the water inlet area were higher than those in the water outlet area. Water outlet area through the wetland sediment filtration and biological filtration effect resulted in reduced nutrients (Aziz and Van Cappellen, 2021). The richness and abundance of plankton in the water outlet area decreased with the decrease in nutrients. Most of the plankton were Anabaena circinalis, Ankistrodesmus angustus, and Polyarthra euryptera, indicating that the Hulanhe Wetland had a certain effect of nutrient salt deposition. This led to certain spatial differences in plankton communities.
The composition of the plankton community is a strong indicator of the water environment (Jagadeesan et al., 2019). As one of the key wetlands in Harbin, the Hulanhe Wetland has achieved great results in ecological restoration in recent years. During the investigation period, the dominant species of plankton communities in the Hulanhe Wetland in the three seasons were mainly pollution-indicator organism species of clean water and oligo-sewage bodies. Zooplankton, such as Polyarthra euryptera (Chen et al., 2011), Strombidium virid (Ju et al., 2017), and Difflugia acuminate (Ju et al., 2017) were dominant. Phytoplankton, such as Asterionella formosa (Shan et al., 2022), Dinobryon cylindricum (Zhang and Li, 2016), Ulnaria ulna (Zhu et al., 2017), and Navicula radiosa (Zhang N. et al., 2016), which prefer clean water, also appeared as dominant communities, which are highly similar to the results of plankton surveys in the Macquarie wetland (Chaparro et al., 2018), which is also a temperate area and has been ecologically restored. The results show that in recent years, with the restoration of the Hulanhe Wetland, the ecological function gradually improved, the water quality was cleaner, and the damage to water ecosystem health was reduced to a controllable range (Hui et al., 2011).
4.1.2. Correlation between phytoplankton and zooplankton
Phytoplankton and zooplankton are important components of the food web in aquatic ecosystems, and there is a very close competition between them and the relationship between predation and predation (Mcqueen et al., 1989). Some studies have confirmed that the predatory grazing behavior of zooplankton is the main reason for the change in the phytoplankton communities (Mcqueen et al., 1989; Vanni and Temte, 1990; Figure 5).
In this study, protozoa and diatoms showed a significant correlation. Protozoa are eukaryotic unicellular animals that belong to heterotrophic organisms and are preyed by large zooplankton (such as copepods and cladocerans) (Burns and Schallenberg, 2001; Turner et al., 2001). Protozoa constitute a complete food chain by preying on diatoms (Min et al., 2019). Many experiments have shown that (Liu and Dagg, 2003; Stelfox-Widdicombe et al., 2004; Kim et al., 2007; Hu et al., 2015) large zooplankton cannot directly ingest diatoms, probably because of the shell of diatoms or because diatoms release harmful substances (such as unsaturated aldehydes) when they reach a certain high concentration to inhibit the growth and reproduction of large zooplankton. Therefore, large zooplankton will directly choose to feed on protozoa and then transfer the primary productivity of diatom sources upwards in the food chain, thus playing a role in the transmission of diatoms in the food chain.
During the study period, rotifers had a strong correlation with Chlorophycophyta, Euglenophyta, and cryptophytes. Rotifer is a small filter-feeding zooplankton. The efficiency of filtering phytoplankton is determined by the size of the phytoplankton. The palatable food range of most filter-feeding rotifers is between 1 and 15 μm (Zhang and Hang, 1991). Chlorophyta and some Cryptophyta (such as Chroomonas acuta), as the dominant species in the Hulanhe Wetland, have a fast reproduction rate and a palatable volume. They mostly exist in the filter-feeding range of Rotifers and are easy for rotifers to prey on. Some previous studies have shown that cryptophytes are often used as high-quality bait for zooplankton because of their cell wall digestibility, non-toxicity and suitable biochemical composition (Porter, 1973). Chlorophycophyta and cryptophytes can be successfully ingested by rotifers and can meet the corresponding nutritional needs; therefore, rotifers tend to prey on Chlorophyta and Cryptophyta (Wang et al., 2012). The predation of rotifers on green algae is also one of the reasons that affect the phytoplankton community structure, with Chlorophycophyta as the main dominant species (Yang, l., 2012).
Cyanophyta, Bacillariophyta, and Chlorophyta were the dominant species of phytoplankton in the Hulanhe Wetland, but there was no significant correlation between Cyanophyta and zooplankton. The reason may be that zooplankton does not prefer ingesting cyanobacteria. Studies have shown that cyanobacteria do not have zooplankton palatability, and the lack of highly unsaturated fatty acids has a negative impact on the growth rate of zooplankton (Juliana et al., 2016); simultaneously, the life form of cyanobacteria is mostly gathered into filaments or surrounded by gelatinous sheaths, which are too large and not easy to be fed (Tan and Ransangan, 2017); some species of zooplankton may also be sensitive to toxins produced by cyanobacteria (De Silva and Jang, 2017).
In conclusion, we found that the abundance of phytoplankton in water was closely related to the growth and reproduction of zooplankton. Phytoplankton is an important food source for zooplankton. High-density phytoplankton ensures the supply of zooplankton food and promotes the reproduction and growth of zooplankton. However, the feeding of zooplankton on phytoplankton is also selective. Zooplankton is more inclined to be easily ingested and palatable than phytoplankton, such as Chlorophyta and Cryptophyta.
4.2. Driving factors of plankton stability
4.2.1. RUE
RUE can reflect the nutrient cycle and transfer process of the ecosystem (He et al., 2021). Communities with high abundance of plankton often have higher RUE, and species in high-abundance communities have a high occupancy rate of trophic niches, increasing the overall RUE (Tian, 2017). In this study, the spatio-temporal differences in RUE and plankton abundance showed the same pattern (Figures 6A,B). The WT of the Hulanhe Wetland increased gradually with the increase of months, which was conducive to the growth and reproduction of plankton. Summer was the season with the most plankton species. At the same time, studies have shown that communities with diatoms, Chlorophycophyta, and protozoa as dominant species can use resources more efficiently (Tillmanns, 2006; Filstrup et al., 2014). Cyanobacteria, Chlorophycophyta, and Protozoa were the dominant phyla of plankton in the Hulanhe Wetland in summer. They have a wide ecological range and strong adaptability to the environment, which improves the RUE of plankton in summer; therefore, the RUE in summer was much greater than that in other seasons.
Some studies have found that the increase in biodiversity will increase RUE and community stability (Filstrup et al., 2014; Zhang et al., 2021), which is similar to the results of this study (Figure 6E). This study found that the gradually abundant plankton species community can use resources more effectively in the ecosystem, and the species community with high diversity has a large number of species and uniform distribution, which makes the community more stable. The abundance and diversity of plankton in the Hulanhe Wetland showed the same pattern (Figures 4, 7). The level of biodiversity increased with the increase in abundance, which enhanced the niche complementarity of plankton in the aquatic ecosystem to a certain extent. The ecological complementarity effect will cause overproduction (Hillebrand et al., 2008), which will promote the utilization efficiency of ecosystem resources.
High concentrations of nutrients are also one of the reasons for the correlation between RUE and the stability of plankton communities (Zhang et al., 2021). Higher concentrations of nutrients can promote the rapid growth and reproduction of plankton. The wetland ecosystem deposits nutrients and purifies water, which is one of the reasons for the high abundance of plankton in the Hulanhe Wetland protection area. Therefore, the RUE in the reserve was much higher than that in other areas (Figures 6C,D). In this study, the RUE in the agriculture-affected area (S2) and fisheries-affected area (S10) was the lowest of all samples (Figures 6C,D). The long-term effects of field herbicides on macrophytes may result in delayed, indirect responses to zooplankton and phytoplankton communities, and pollution may result in single species and a low abundance of plankton (Relyea, 2005). Due to the obvious agricultural disturbance, the RUE of the agriculture-affected area (S2) of the Hulan River inlet was low, indicating that anthropogenic disturbance destroyed the ecological function systems, resulting in low resource utilization (Wang et al., 2019). S10 was an area greatly affected by the fishery. The disturbance caused by the fishing process inhibited the growth of plankton. At the same time, the fish school carries out a large number of downward effect control on plankton, resulting in low species number and abundance (Shen and Wang, 1991), which leads to a low RUE. Therefore, to maintain the stability and sustainability of the regional ecological security pattern, the regional ecological function system affected by anthropogenic disturbance needs further restoration.
4.2.2. Species diversity
Species diversity is an important measure of community stability. There is a certain coupling relationship between plankton species diversity and community stability (Tilman, 1999). Exploring the correlation between plankton diversity and community stability is of great significance for explaining plankton community structure and diversity. In aquatic ecosystems, the increase in plankton species diversity makes the community more stable (Ptacnik et al., 2007), which is the same as the results of this study (Figure 8A).
In this study, with the increase in plankton community diversity, the community became more stable. It is speculated that the food chain and food web of communities with high diversity tend to be more complex, and there are more ways for energy flow within the community. If a certain way is blocked by interference, there may be other routes to compensate (Cao and Zhan, 2015). Therefore, a community with high diversity has less fluctuation among species, which makes the community more stable. A structurally stable plankton community has a strong feedback system, which can buffer environmental changes or fluctuations in the population from within the community and is less susceptible to external disturbances (MacArthur, 1955; Cao and Zhan, 2015).
The evenness index reflects the uniformity of the individual number distribution of each species. The higher the evenness, the more uniform the species distribution. This study showed high Pielou evenness, which implies high community stability (Figure 8B). Many studies have shown that the higher the species’ evenness, the stronger the ecosystem function conversion, making the plankton community more stable (Wittebolle et al., 2009; Allan et al., 2011; Filstrup et al., 2014). The results of this study also reached the same conclusion. The community with high plankton evenness had a more uniform distribution of species resources, and the anti-interference ability of the community also increased, which is conducive for the better adaptation of the plankton community to the aquatic ecological environment (Tian, 2017; Zheng, 2019).
4.2.3. Environmental factors
Through ordinary least squares analysis, it was found that the physical and chemical environmental factors of the waterbody had a certain driving effect on the stability of the phytoplankton community, and the environmental factors affecting phytoplankton and zooplankton were similar. WT (p < 0.01, p < 0.01) and pH (p < 0.01, p < 0.01) were both the driving factors of phytoplankton and zooplankton community stability, while TN (p < 0.01) was only the driving factor of zooplankton community stability.
Plankton community stability will increase with the increase of WT (Figures 9A, 10A). WT can affect the metabolic intensity, growth and development, and reproduction cycle of plankton, and then affect its abundance and biomass changes and the distribution of community structure, and ultimately affect the material circulation and energy flow of the ecosystem. The average temperature of the Hulanhe Wetland in summer is 22.0°C, while cyanobacteria, Chlorophycophyta, and rotifers are more suitable for growing in warm water. The average temperature in spring and autumn is slightly lower than 16.3°C and 9.1°C, which is more suitable for the growth and reproduction of diatoms, Chrysophyta, and Protozoa, and is more dominant in the plankton community. In addition, the predation rate of zooplankton is similar to the growth rate of zooplankton, which increases the grazing frequency and achieves rapid reproduction of zooplankton as the water temperature increases (Pulsifer and Laws, 2021). This further proves that the stability of plankton community will be more stable with the increase of WT.
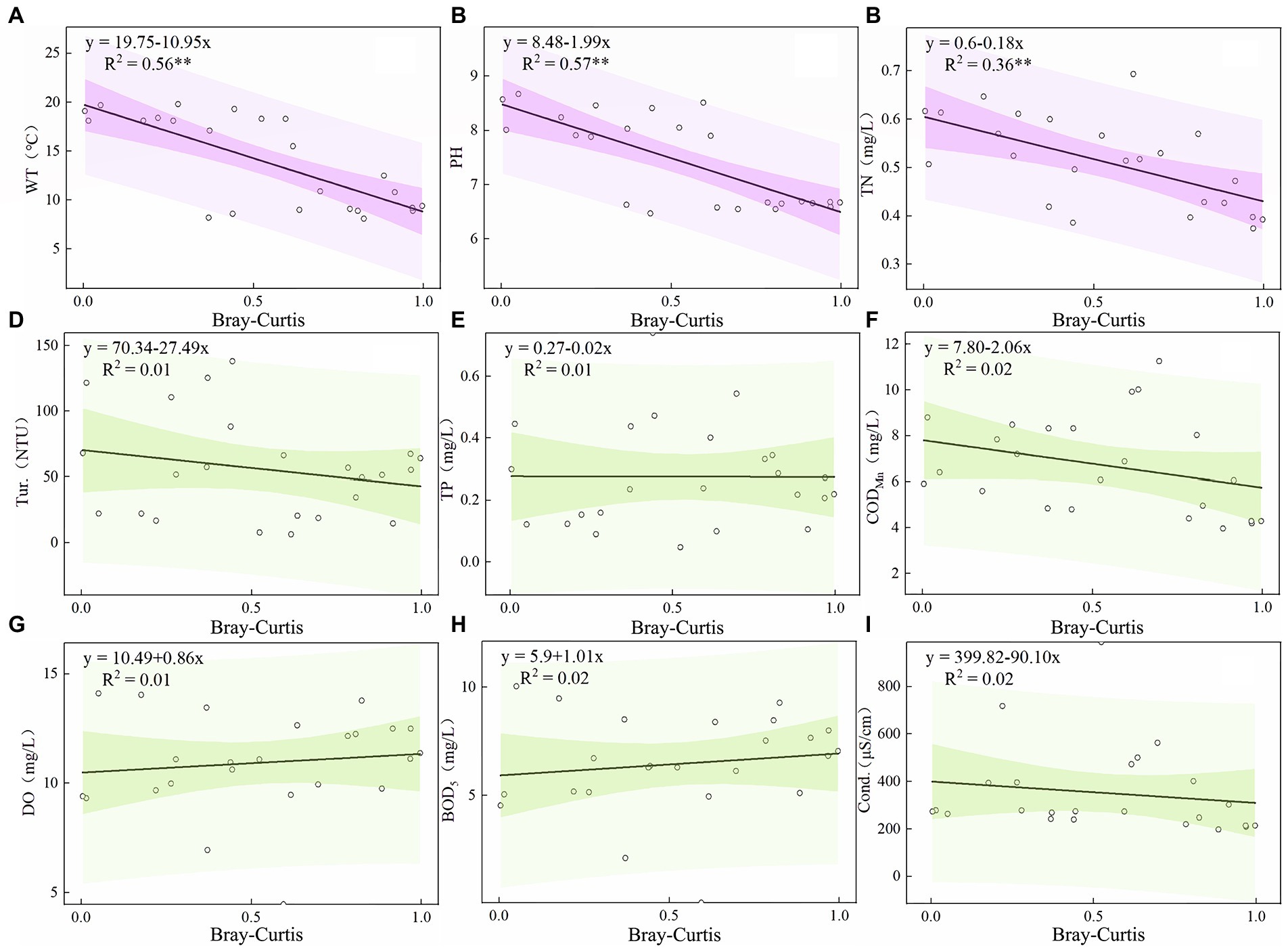
Figure 10. Relationship between Environmental Factors and Community Turnover of Zooplankton Community in Hulanhe Wetland. (A) Water temperature and community turnover. (B) pH. (C) Total nitrogen. (D) Turbidity. (E) Total phosphorus. (F) Permanganate index. (G) Dissolved oxygen. (H) Bio-chemical oxygen demand. (I) Conductivity.
pH is an important water environmental factor driving the stability of phytoplankton and zooplankton communities. The results showed that there was a significant negative correlation between pH and the turnover of zooplankton and phytoplankton communities (Figures 9B, 10B). Phytoplankton communities were more stable in alkaline water. The pH affects the absorption of nutrients by affecting the activity of some enzymes in plankton cells or transmembrane proton gradient. In the season with high WT, the dissolved CO2 in water decreases, increasing the pH value, and the Hulanhe Wetland is weakly alkaline (7.51 ± 0.38), which promotes the growth and reproduction of plankton, thus affecting its community structure (Zhang et al., 2021). The alkaline environment is conducive to phytoplankton absorbing CO2 for photosynthesis. In alkaline water, phytoplankton has high primary productivity, which ensures the food source of zooplankton. The abundance of zooplankton also increases, and the phytoplankton community gradually stabilizes (Jakobsen et al., 2015).
TN is an essential element for the synthesis and energy transfer of aquatic organisms and also an important nutrient source for zooplankton. The variation in TN concentration can affect the food source of zooplankton and the dynamic pattern of community (Shen et al., 2021). TN indirectly affects the composition of zooplankton by affecting the community structure of phytoplankton (Guo et al., 2017). At the same time, changes in the ratio of nitrogen to phosphorus in the water affects the competition between zooplankton with different growth characteristics and the change in population structure (Yang et al., 2021). During the study period, the average nitrogen-phosphorus ratio of the Hulanhe Wetland was 1.52 (N/p < 16:1), which was a nitrogen limitation. The average TN of the Hulanhe Wetland in spring, summer, and autumn was at a relatively high level (0.46 mg/L). High concentrations of TN made the zooplankton community more stable (Figure 10C). Bhavya et al. (2020)have shown that the total uptake of nitrogen by phytoplankton is in the range of 10.24–59.36% and 30.21–68.55%. Therefore, high concentration of TN promotes the reproduction of phytoplankton (Liu et al., 2022). Phytoplankton photosynthesis in water not only provides sufficient food for zooplankton but also creates an oxygen-rich environment for zooplankton, which makes zooplankton species more abundant and increases community stability.
Overall, the stability of the plankton community is a very complex problem. The stability of the plankton community is not only affected by the single factor of community structure characteristics but also has a certain correlation with RUE, biodiversity, and water environment factors.
For future studies, we suggest observing the effects of microorganisms on plankton (Pearce and Butler, 2002; Rana et al., 2021), the predation of plankton by fish (Villéger et al., 2019; Xia et al., 2020), and the variation in plankton stability under special conditions (e.g., heavy rain, freezing, and advection; Manna et al., 2019; Liu et al., 2021; Lu et al., 2022), which will help to improve our mechanistic understanding of the maintenance and stability of plankton community structure.
5. Conclusion
Specific predation relationship was observed between phytoplankton and zooplankton in the Hulanhe Wetland. Protozoa tend to prey on diatoms, rotifers prefer to ingest Chlorophycophyta and cryptophytes, but cyanobacteria are rarely preyed on by zooplankton because of their own cyanobacterial toxins and mismatched caliber. The stability of plankton community in the Hulanhe Wetland is controlled by biotic and abiotic factors. The stability of the plankton community had a significant linear relationship with the Margalef diversity index, Pielou evenness index, and RUE. The key factors affecting phytoplankton community stability in the Hulanhe Wetland were WT and pH, while zooplankton community stability was mainly driven by WT, pH, and TN.
Data availability statement
The raw data supporting the conclusions of this article will be made available by the authors, without undue reservation.
Author contributions
XL conceived the original concept. XL and YF developed experimental strategies and sampling design. TT carried out the collection of plankton samples and the determination of water physic-chemical indicators under the guidance of XL. TT and HW carried out the counting and identification of plankton specimens and contributed in sampling collection, data analysis, and manuscript writing. TT, HW, and XN performed all data analysis and integration with help from XL and YF. TT prepared the manuscript with significant input from HW, XN, NZ, XL, YL, and YF. All authors contributed experimental assistance and intellectual input to this study.
Acknowledgments
We thank Yimin Zhu, Zhenxiang Li, Tao Shan, Tian Lou, Chao Ma, Anlong Yuan, Hongying Xin, Jinyan Liang, and Xiaoyi Chen for sample collection.
Conflict of interest
The authors declare that the research was conducted in the absence of any commercial or financial relationships that could be construed as a potential conflict of interest.
Publisher’s note
All claims expressed in this article are solely those of the authors and do not necessarily represent those of their affiliated organizations, or those of the publisher, the editors and the reviewers. Any product that may be evaluated in this article, or claim that may be made by its manufacturer, is not guaranteed or endorsed by the publisher.
Footnotes
References
Allan, E., Weisser, W., Weigelt, A., Roscher, C., Fischer, M., and Hillebrand, H. (2011). More diverse plant communities have higher functioning over time due to turnover in complementary dominant species. Proc. Natl. Acad. Sci. U. S. A. 108, 17034–17039. doi: 10.1073/pnas.1104015108
Amorim, C. A., and Moura, A. D. N. (2021). Ecological impacts of freshwater algal blooms on water quality, plankton biodiversity, structure, and ecosystem functioning. Sci. Total Environ. 758:143605. doi: 10.1016/j.scitotenv.2020.143605
An, R., Wang, F. Y., Yu, H. X., and Ma, C. X. (2017). Seasonal dynamics of zooplankton functional groups and their relationships with environmental factors in the Sanhuanpao wetland reserve. Acta Ecol. Sin. 37, 1851–1860. doi: 10.5846/stxb201510292184
Arndt, H. (1993). Rotifers as predators on components of the microbial web (bacteria, heterotrophic flagellates, ciliates) - a review. Hydrobiologia 255-256, 231–246. doi: 10.1007/bf00025844
Aziz, T., and Van Cappellen, P. (2021). Economic valuation of suspended sediment and phosphorus filtration services by four different wetland types: A preliminary assessment for southern Ontario, Canada. Hydrol. Process. 35, 1–15. doi: 10.1002/hyp.14442
Bhavya, P. S., Kang, J. J., Jang, H. K., Joo, H., Lee, J. H., Lee, J. H., et al. (2020). The contribution of small phytoplankton communities to the total dissolved inorganic nitrogen assimilation rates in the east/Japan Sea: An experimental evaluation. J. Mar. Sci. Eng. 8:854. doi: 10.3390/jmse8110854
Boucot, A. J. (1985). The complexity and stability of ecosystems. Nature 315, 635–636. doi: 10.1038/315635c0
Burns, C. W., and Schallenberg, M. (2001). Calanoid copepods versus cladocerans: Consumer effects on protozoa in lakes of different trophic status. Limnol. Oceanogr. 46, 1558–1565. doi: 10.4319/lo.2001.46.6.1558
Chaparro, G., Horvath, Z., O'Farrell, I., Ptacnik, R., and Hein, T. (2018). Plankton metacommunities in floodplain wetlands under contrasting hydrological conditions. Freshw. Biol. 63, 380–391. doi: 10.1111/fwb.13076
Chen, Q., He, W., Liu, Y., and Huang, J. (2014). Characteristics of the Zooplankton communities in four typical wetlands of Macao. J. Hydroecol. 35, 24–30. doi: 10.15928/j.1674-3075.2014.06.004
Chen, L., Liu, Q., Peng, Z., Hu, Z., Xue, J., and Wang, W. (2011). The variation and analysis of rotifer community structure in and out of crab-net-pen closing in the Yangcheng Lake. J. Fish. China 35, 1247–1257. doi: 10.3724/SP.J.1231.2011.17365
Cheng, X. (2013). Flora of Hulan estuary wetlands nature reserve. Prot. For. Sci. Technol. 8, 14–17+19. doi: 10.13601/j.issn.1005-5215.2013.08.002
Claessens, M., and Prast, M. (2007). Concentration of fixed plankton samples via settling: How long is long enough? J. Plankton Res. 30, 57–64. doi: 10.1093/plankt/fbm095
Davidson, E. A., and Howarth, R. W. (2007). Environmental science: Nutrients in synergy. Ecol. Lett. 10, 1135–1142. doi: 10.1111/j.1461-0248.2007.01113.x
De Silva, M., and Jang, S. R. J. (2017). Dynamical behavior of systems of two phytoplankton and one zooplankton populations with toxin producing phytoplankton. Math. Methods Appl. Sci. 40, 4295–4309. doi: 10.1002/mma.4305
Easson, C. G., and Lopez, J. V. (2019). Depth-dependent environmental drivers of microbial plankton community structure in the Northern Gulf of Mexico. Front. Microbiol. 9:3175. doi: 10.3389/fmicb.2018.03175
Elton, C. S. (2020). The ecology of invasions by animals and plants. Berlin. Germany: Springer Nature.
Filstrup, C. T., Hillebrand, H., Heathcote, A. J., Harpole, W. S., and Downing, J. A. (2014). Cyanobacteria dominance influences resource use efficiency and community turnover in phytoplankton and zooplankton communities. Ecol. Lett. 17, 464–474. doi: 10.1111/ele.12246
Gao, Z., Xiao, R., Ren, A., and Zhao, W. (2015). Community structure and diversity of summer birds in Hulan River estuary wetland nature reserve, Heilongjiang Province. Sichuan J. Zool. 34, 285–289. doi: 10.3969/j.issn.1000-7083.2015.02.021
Guo, K., Peng, T., Luo, J., Yang, D., He, Y., and Chai, Y. (2017). Community structure of zooplankton and the driving physicochemical factors in Changhu Lake. Oceanol. Limnol. Sin. 48, 40–49. doi: 10.11693/hyhz20160700152
He, S., Ouyang, T., Zhao, L., Ji, L., Yang, S., Shi, J., et al. (2021). Analysis of phytoplankton community stability and influencing factors in a tributary of the three gorges reservoir. Environ. Sci. 42, 3242–3252. doi: 10.13227/j.hjkx.202012096
Hillebrand, H., Bennett, D. M., and Cadotte, M. W. (2008). Consequences of dominance: A review of evenness effects on local and regional ecosystem processes. Ecology 89, 1510–1520. doi: 10.1890/07-1053.1
Holling, S. C. (1973). Resilience and stability of ecological systems. Annu. Rev. Ecol. Syst. 4, 1–23. doi: 10.2307/2096802
Hu, S., Guo, Z., Li, T., Xu, C., Huang, H., Liu, S., et al. (2015). Molecular analysis of in situ diets of coral reef copepods: Evidence of terrestrial plant detritus as a food source in Sanya Bay, China. J. Plankton Res. 37, 363–371. doi: 10.1093/plankt/fbv014
Hu, H., and Wei, Y. (2006). Freshwater Algae in China—System, Classification and Ecology. Beijing, China: Science Press.
Hui, H., Ma, Y., and Fan, Y. (2011). Algae community structure and distribution in Hulanhe Wetland, Heilongjiang Province. J. Lake Sci. 23, 949–954. doi: 10.18307/2011.0618
Jagadeesan, L., Kumar, G. S., Rao, D. N., Babu, N. S., and And Srinivas, T. N. R. (2019). Role of eddies in structuring the mesozooplankton composition in coastal waters of the western Bay of Bengal. Ecol. Indic. 105, 137–155. doi: 10.1016/j.ecolind.2019.05.068
Jakobsen, H. H., Blanda, E., Staehr, P. A., Højgård, J. K., Rayner, T. A., Pedersen, M. F., et al. (2015). Development of phytoplankton communities: Implications of nutrient injections on phytoplankton composition, pH and ecosystem production. J. Exp. Mar. Biol. Ecol. 473, 81–89. doi: 10.1016/j.jembe.2015.08.011
Jeppesen, E., Nõges, P., Davidson, T. A., Haberman, J., Nõges, T., Blank, K., et al. (2011). Zooplankton as indicators in lakes: A scientific-based plea for including zooplankton in the ecological quality assessment of lakes according to the European Water Framework Directive (WFD). Hydrobiologia 676, 279–297. doi: 10.1007/s10750-011-0831-0
Jia, P., Fan, Y., and Lu, X. (2021). Analysis of phytoplankton structure based on different functional groups in Hulanhe Wetland. Acta Ecol. Sin. 41, 1042–1054. doi: 10.5846/stxb201911152444
Jonkers, L., Hillebrand, H., and Kucera, M. (2019). Global change drives modern plankton communities away from the pre-industrial state. Nature 570, 372–375. doi: 10.1038/s41586-019-1230-3
Ju, Y., Yu, H., Yu, T., Chai, F., Yao, Y., and Yu, P. (2017). Zooplankton community structure characters and water quality assessment in Harbin section of Songhua River. J. Lake Sci. 29, 646–653. doi: 10.18307/2017.0313
Juliana, D. S. S., Lúcia, D. S. A.-M. V., Enaide, M. D. M.-M., Maria, D. C. B.-O., and And Ariadne, D. N. M. (2016). Effects of zooplankton and nutrients on phytoplankton: An experimental analysis in a eutrophic tropical reservoir. Mar. Freshw. Res. 68, 1061–1069. doi: 10.1071/mf15393
Kim, S., Park, M. G., Moon, C., Shin, K., and Chang, M. (2007). Seasonal variations in phytoplankton growth and microzooplankton grazing in a temperate coastal embayment, Korea. Estuar. Coast. Shelf Sci. 71, 159–169. doi: 10.1016/j.ecss.2006.07.011
Kobayashi, T., Ralph, T. J., Ryder, D. S., Hunter, S. J., Shiel, R. J., and Segers, H. (2014). Spatial dissimilarities in plankton structure and function during flood pulses in a semi-arid floodplain wetland system. Hydrobiologia 747, 19–31. doi: 10.1007/s10750-014-2119-7
Li, Y., Chen, Y., Wang, L., Yao, L., Pan, X., and Lee, D. (2017). Pollution tolerant protozoa in polluted wetland. Bioresour. Technol. 240, 115–122. doi: 10.1016/j.biortech.2017.02.051
Liu, M. (2012). The ecological monitoring and health assessment based on aquatic animals in Hulan estuary natural reserves and the surrounding waters. Harbin: Northeast Forestry University.
Liu, H., and Dagg, M. (2003). Interactions between nutrients, phytoplankton growth, and micro- and mesozooplankton grazing in the plume of the Mississippi River. Mar. Ecol. Prog. Ser. 258, 31–42. doi: 10.3354/meps258031
Liu, Z., Liu, S., Tian, D., and Wang, D. (2021). Stability analysis of the plankton community with advection. Chaos Solitons Fractals 146:110836. doi: 10.1016/j.chaos.2021.110836
Liu, Q., Liu, Z., Wang, J., Liao, C., Li, J., Guo, C., et al. (2022). Spatial-temporal characteristics of zooplankton community structure and analysis for the impact factors in the Shanmei Reservoir, Fujian Province. J. Lake Sci. 34, 1–22. doi: 10.18307/2022.0619
Lu, X., Liu, Y., and Fan, Y. (2014). Relationships between environmental variables and seasonal succession in phytoplankton functional groups in the Hulan River Wetland. Acta Ecol. Sin. 34, 1264–1273. doi: 10.5846/stxb201306061369
Lu, X., Zhang, Y., Liu, Y., and Fan, Y. (2022). Differences in planktonic and benthic diatoms reflect water quality during a rainstorm event in the Songhua River Basin of Northeast China. Ecol. Indic. 144:109547. doi: 10.1016/j.ecolind.2022.109547
MacArthur, R. (1955). Fluctuations of animal populations and a measure of community stability. Ecology 36, 533–536. doi: 10.2307/1929601
Magnussen, S., and Boyle, T. (1995). Estimating sample size for inference about the Shannon-weaver and the Simpson indices of species diversity. For. Ecol. Manag. 78, 71–84. doi: 10.1016/0378-1127(95)03596-1
Manna, V., Fabbro, C., Cerino, F., Bazzaro, M., Del Negro, P., and Celussi, M. (2019). Effect of an extreme cold event on the metabolism of planktonic microbes in the northernmost basin of the Mediterranean Sea. Estuar. Coast. Shelf Sci. 225:106252. doi: 10.1016/j.ecss.2019.106252
Margalef, R. (1969). Abnormal expression of normal transferrin alleles in cattle. Biochem. Genet. 2, 371–382. doi: 10.1007/BF01458497
Matchett, E. L., and Fleskes, J. P. (2017). Projected impacts of climate, urbanization, water management, and wetland restoration on Waterbird habitat in California's Central Valley. PLoS One 12:e0169780. doi: 10.1371/journal.pone.0169780
Mcqueen, D. J., Johannes, M. R. S., and Post, J. R. (1989). Bottom-up and top-down impacts on freshwater pelagic community structure. Ecology 59, 289–309. doi: 10.2307/1942603
Min, W., Xiang, Y., Wang, X., Wang, W., Wang, X., Zeng, S., et al. (2019). Community structure and diversity of plankton in Wujiang River basin in autumn. Guizhou Agric. Sci. 47, 70–74. doi: 10.3969/j.issn.1001-3601.2019.07.016
Mougi, A. (2022). Predator interference and complexity-stability in food webs. Sci. Rep. 12:2464. doi: 10.1038/s41598-022-06524-w
Norberg, J., Swaney, D. P., Dushoff, J., Lin, J., Casagrandi, R., Levin, S. A., et al. (2001). Phenotypic diversity and ecosystem functioning in changing environments: A theoretical framework. Proc. Natl. Acad. Sci. U. S. A. 98, 11376–11381. doi: 10.1073/pnas.171315998
Pearce, D., and Butler, H. (2002). Short-term stability of the microbial community structure in a maritime Antarctic lake. Polar Biol. 25, 479–487. doi: 10.1007/s00300-002-0370-2
Pennekamp, F., Pontarp, M., Tabi, A., Altermatt, F., Alther, R., Choffat, Y., et al. (2018). Biodiversity increases and decreases ecosystem stability. Nature 563, 109–112. doi: 10.1038/s41586-018-0627-8
Pielou, E. C. (1966). The measurement of diversity in different types of biological collections. J. Theor. Biol. 13, 131–144. doi: 10.1016/0022-5193(66)90013-0
Porter, K. R. R. (1973). Selective grazing and differential digestion of algae by zooplankton. Nature 244, 179–180. doi: 10.1038/244179a0
Ptacnik, R., Solimini, A. G., Andersen, T., Tamminen, T., Brettum, P., Lepistö, L., et al. (2007). Diversity predicts stability and resource use efficiency in natural phytoplankton communities. Proc. Natl. Acad. Sci. U. S. A. 105, 5134–5138. doi: 10.1073/pnas.0708328105
Pulsifer, J., and Laws, E. (2021). Temperature dependence of freshwater phytoplankton growth rates and zooplankton grazing rates. Water 13:1591. doi: 10.3390/w13111591
Rana, A., Kobayashi, T., and Ralph, T. J. (2021). Planktonic metabolism and microbial carbon substrate utilization in response to inundation in semi-arid floodplain wetlands. J. Geophys. Res. Biogeosci. 126:e2019JG005571. doi: 10.1029/2019jg005571
Relyea, R. A. (2005). The impact of insecticides and herbicides on the biodiversity and productivity of aquatic communities. Ecol. Appl. 15, 618–627. doi: 10.1890/03-5342
Schaum, C. E., Rost, B., and Collins, S. (2016). Environmental stability affects phenotypic evolution in a globally distributed marine picoplankton. ISME J. 10, 75–84. doi: 10.1038/ismej.2015.102
Seilheimer, T. S., Mahoney, T. P., and Chow-Fraser, P. (2009). Comparative study of ecological indices for assessing human-induced disturbance in coastal wetlands of the Laurentian Great Lakes. Ecol. Indic. 9, 81–91. doi: 10.1016/j.ecolind.2008.02.001
Sennhauser, E. B. (1991). The concept of stability in connection with thegallery forests of the Chaco region. Vegetatio 94, 1–13. doi: 10.1007/bf00044911
Shan, T., Yuan, A., Huang, Z., Zhou, J., Lu, X., and Fan, Y. (2022). Characteristics of benthic diatom community structure and water ecological health evaluation in the Lalin River basin. Environ. Sci. 44, 266–275. doi: 10.13227/j.hjkx.202205168
Shen, J., Qin, G., Yu, R., Zhao, Y., Yang, J., An, S., et al. (2021). Urbanization has changed the distribution pattern of zooplankton species diversity and the structure of functional groups. Ecol. Indic. 120:106944. doi: 10.1016/j.ecolind.2020.106944
Shen, B., and Wang, S. (1991). A model of catching food in fishery ecology. J. Dalian Ocean Univ. 6s, 66–70. doi: 10.16535/j.cnki.dlhyxb.1991.01.011
Stelfox-Widdicombe, C. E., Archer, S. D., Burkill, P. H., and Stefels, J. (2004). Microzooplankton grazing in Phaeocystis and diatom-dominated waters in the southern North Sea in spring. J. Sea Res. 51, 37–51. doi: 10.1016/j.seares.2003.04.004
Tan, K. S., and Ransangan, J. (2017). Effects of nutrients and zooplankton on the phytoplankton community structure in Marudu Bay. Estuar. Coast. Shelf Sci. 194, 16–29. doi: 10.1016/j.ecss.2017.05.008
Tian, W. (2017). Response mechanisms of Lake plankton community to environment and biodiversity. Beijing, China: North China Electric Power University.
Tian, W., Zhang, H., Zhang, J., Zhao, L., Miao, M., and Huang, H. (2017). Biodiversity effects on resource use efficiency and community turnover of plankton in Lake Nansihu, China. Environ. Sci. Pollut. Res. Int. 24, 11279–11288. doi: 10.1007/s11356-017-8758-2
Tian, W., Zhang, H., Zhao, L., Xiong, Y., and Huang, H. (2016). Effects of environmental factors on the temporal stability of phytoplankton biomass in a eutrophic man-made Lake. Water 8:582. doi: 10.3390/w8120582
Tillmanns, A. E. W. O. S. A. R. (2006). Effects of cyanobacterial toxicity and morphology on the population growth of freshwater zooplankton: Meta-analyses of laboratory experiments. Limnol. Oceanogr. 51, 1915–1924. doi: 10.4319/lo.2006.51.4.1915
Tilman, D. (1999). The ecological consequences of changes in biodiversity: A search for general principles. Ecology 80, 1455–1474. doi: 10.1890/0012-9658(1999)080[1455:tecoci]2.0.co;2
Turner, J. T., Levinsen, H., Nielsen, T. G., and Hansen, B. (2001). Zooplankton feeding ecology: Grazing on phytoplankton and predation on protozoans by copepod and barnacle nauplii in Disko Bay, West Greenland. Mar. Ecol. Prog. Ser. 221, 209–219. doi: 10.3354/meps221209
Vanni, M. J., and Temte, J. (1990). Seasonal patterns of grazing and nutrient limitation of phytoplankton in a eutrophic lake. Limnol. Oceanogr. 35, 697–709. doi: 10.4319/lo.1990.35.3.0697
Villéger, S., Fouilland, E., Argenty, J., Bouvier, C., Carré, C., and Bouvier, T. (2019). Interspecific differences in the effect of fish on marine microbial plankton. Aquat. Microb. Ecol. 82, 289–298. doi: 10.3354/ame01897
Wang, H., Hou, W., Ma, C., Yu, H., Gao, J., Bai, R., et al. (2017). Distribution of Zooplankton in Genheyuan National Wetland Park. Wetland Sci. 15, 99–106. doi: 10.13248/j.cnki.wetlandsci.2017.01.015
Wang, H., Huang, X., Wu, J., Du, X., Wang, Q., Song, D., et al. (2019). Effect of agricultural non-point source pollution on plankton and Zoobenthos communities in a reservoir in Morin Dawa Daur autonomous banner. Chinese J. Fish. 32, 55–62.
Wang, Y., Qiu, G., Chen, S., and Hu, S. (2012). Research on plankton community in three gorges reservoir during its trial impoundment. Yangtze River 43, 4–9. doi: 10.16232/j.cnki.1001-4179.2012.12.027
Wittebolle, L., Marzorati, M., Clement, L., Balloi, A., Daffonchio, D., Heylen, K., et al. (2009). Initial community evenness favours functionality under selective stress. Nature 458, 623–626. doi: 10.1038/nature07840
Xia, Y. P., Zhou, W. S., and Yang, Z. C. (2020). Global analysis and optimal harvesting for a hybrid stochastic phytoplankton-zooplankton-fish model with distributed delays. Math. Biosci. Eng. 17, 6149–6180. doi: 10.3934/mbe.2020326
Xing, C. (2016). Protection strategy of Hulan Estuary National Wetland Park in Harbin. J. Green Sci. Technol. 2, 33–34. doi: 10.16663/j.cnki.lskj.2016.02.013
Yang, L. (2012). Zooplankton community structure and its relation to environment factors in Qiandao Lake. Shanghai: Shanghai Ocean University.
Yang, X., Ma, J., Zhang, H., and Zhou, Q. (2021). Community structure and the water quality during different hydrological periods in poyang lake. Acta Hydrobiol. Sin. 45, 1093–1103. doi: 10.7541/2021.2020.148
Zhang, Z., and Hang, X. (1991). Freshwater plankton research methods. Beijing, China: Science Press.
Zhang, Y., Jin, R., Zhu, W., Zhang, D., and Zhang, X. (2020). Impacts of land use changes on wetland ecosystem services in the Tumen River Basin. Sustainability 12:9821. doi: 10.3390/su12239821
Zhang, X., and Li, C. (2016). Analysis of phytoplankton community characteristics and its influencing factors in Tanghe reservoir. Environ. Sci. Technol. 39, 394–401. doi: 10.3969/j.issn.1003-6504.2016.S2.078
Zhang, X., Liu, K., Wan, A., Chen, M., Liu, Z., Lian, Y., et al. (2018). Community structure of zooplankton and its relationship with survivability of the yangtze finless porpoise in xijiang oxbow, Anqing city. Acta Hydrobiol. Sin. 42, 392–399. doi: 10.7541/2018.050
Zhang, N., Liu, Y., and Zang, S. (2016). Relationships between phytoplankton community in different functional regions and environmental factors in Zhalong Wetland, Heilongjiang Province. J. Lake Sci. 28, 554–565. doi: 10.18307/2016.0311
Zhang, M., Shi, X., Chen, F., Yang, Z., and Yu, Y. (2021). The underlying causes and effects of phytoplankton seasonal turnover on resource use efficiency in freshwater lakes. Ecol. Evol. 11, 8897–8909. doi: 10.1002/ece3.7724
Zhang, H., Zhao, L., Tian, W., and Huang, H. (2016). Stability of food webs to biodiversity loss: Comparing the roles of biomass and node degree. Ecol. Indic. 67, 723–729. doi: 10.1016/j.ecolind.2016.03.045
Zheng, Y. (2019). Research on the effects of plankton species diversity on community productivity and stability. Beijing, China: North China Electric Power University.
Zheng, B., Chen, Z., Li, Y., Fohrer, N., Zhang, Y., Wu, D., et al. (2020). Structural characteristics and driving factors of the planktonic eukaryotic community in the Danjiangkou Reservoir, China. Water 12:3499. doi: 10.3390/w12123499
Zheng, Y., Wang, X., Xiong, J., Liu, Y., and Zhao, Y. (2014). Hybrid constructed wetlands for highly polluted river water treatment and comparison of surface- and subsurface-flow cells. J. Environ. Sci. 26, 749–756. doi: 10.1016/s1001-0742(13)60482-9
Zhou, F., and Chen, J. (2011). Freshwater microorganisms and Zoobenthos atlas. 2nd Edn. Beijing, China: Chemical Industry Publishing House.
Zhu, W., Pang, W., You, Q., and Wang, Q. (2017). Phytoplankton community structure and the evaluation of water quality in spring, Huaihe River Basin. J. Lake Sci. 29, 637–645. doi: 10.18307/2017.0312
Keywords: Hulanhe Wetland, plankton, grazing, community stability, resource use efficiency, diversity indicators
Citation: Tao T, Wang H, Na X, Liu Y, Zhang N, Lu X and Fan Y (2023) Temperate urban wetland plankton community stability driven by environmental variables, biodiversity, and resource use efficiency: A case of Hulanhe Wetland. Front. Ecol. Evol. 11:1148580. doi: 10.3389/fevo.2023.1148580
Edited by:
Xiaodong Zhang, Chinese Academy of Forestry, ChinaCopyright © 2023 Tao, Wang, Na, Liu, Zhang, Lu and Fan. This is an open-access article distributed under the terms of the Creative Commons Attribution License (CC BY). The use, distribution or reproduction in other forums is permitted, provided the original author(s) and the copyright owner(s) are credited and that the original publication in this journal is cited, in accordance with accepted academic practice. No use, distribution or reproduction is permitted which does not comply with these terms.
*Correspondence: Xinxin Lu, luxinxinchina@163.com; Yawen Fan, fanyaw_hrbnu@163.com
†These authors have contributed equally to this work