- Illinois Natural History Survey, Prairie Research Institute, University of Illinois Urbana-Champaign, Champaign, IL, United States
Benthic macroinvertebrate protocols, in the United States, have been integral in the implementation of the Clean Water Act (33 U.S.C. §1251 et seq. (1972)). The methods in these protocols are designed to describe patterns of biological water quality and ecological condition relevant to legislative oversight, and require a tradeoff between taxonomic precision and protocol simplicity. The global similarity of ecological and evolutionary responses to the challenges of life in flowing waters, by stream organisms, has greatly facilitated the development and implementation of biological data from freshwater mussels, fishes and aquatic insects into streamlined regulatory frameworks. Unfortunately, the factors that expedite the use of benthic data into ecological descriptions sufficient for regulatory processes (taxonomic generalizations, standardization of sampling procedures to focus on a narrow subset of available habitats) also limit the utility of these methods in the conservation of benthic macroinvertebrate species (the large majority of which are insects). Using simple hypothetical simulations, some examples from a taxonomic and ecological database of one state in the USA (Illinois), and data from a large-scale inventory of aquatic insect species in a network of US National Parks, I illustrate how these methods are limited in their ability to effectively describe ecological patterns relevant to the conservation of aquatic insect species. I identify, challenge, and explore three basic assumptions implicit to the genus- and family-level identification methods widely used in freshwater benthic science, then discuss how the failure of these assumptions may create empirical, theoretical and philosophical obstacles to the interpretation of data and certainly hinder the conservation of species. I offer some hopeful suggestions for improving aquatic insect species conservation that are within the reach of all benthic freshwater ecologists.
Introduction
The biological integrity paradigm (Frey, 1975; Cairns, 1977; Karr et al., 1986; Davies and Jackson, 2006) is a revolutionary and successful scientific research program which synthesized widely disparate categories of ecological information into coherent, actionable policy directives. This research program has developed tools enabling regulators to prevent the continued degradation of the biological integrity of flowing waters (Kenney et al., 2009). Early investigations into what eventually became the study of “water quality” were focused in two general areas: species that serve as indicators of pollution, and general classification of water bodies by their legacy or experience of pollution (Cairns and Pratt, 1993). The evolutionary trajectories of modern freshwater ecology and biological assessment have assimilated these two perspectives, along with many other burgeoning ideas, into methods for evaluating the ecological condition of water bodies, in terms of the properties of the organisms inhabiting them, in a biogeographical and watershed position context (Frey, 1975; Minshall, 1988; Cairns and Pratt, 1993).
Freshwater systems are extraordinarily taxonomically rich, across spatial scales local to global. The taxonomic expertise required to accurately describe this diversity is frequently far greater than can be managed by individual researchers. Thus, by necessary design, many modern freshwater assessment methods reduce the complexity associated with large numbers of species by assuming a functional interchangeability within family or genus levels (Hilsenhoff, 1988). Generalizing across higher-level taxonomy (like “species within a genus” or “species within a family”; the genus or family generalization) enables the standardization of comparisons of biological water quality of streams across regions. These generalizations are useful, but may come with tradeoffs: loss of sensitivity to trends or patterns in the distribution or abundance of the species under monitoring (Cairns, 1975; Resh and Unzicker, 1975; Polhemus, 1993; Schmidt-Kloiber and Nijboer, 2004).
From the inception of state and federal biological monitoring programs, biologists have pointed out that generalized benthic assessments may not be sufficient to inform the conservation of many benthic macroinvertebrates species (Strayer, 2006). Biogeographic patterns relevant to both the conservation of species, and to inferring the underlying ecological and evolutionary processes that regulate species diversity or ecosystem function, may be more difficult to detect and describe using benthic methods that fail to tabulate data at the species level (Cranston, 1990; Robinson et al., 2016; Bried and Hinchliffe, 2019). Many practical taxonomic problems challenging the accurate species-level identification of immature aquatic insect species are unlikely to be soon resolved: within regional faunal assemblages the immature stages of many species remain unknown altogether, or are not sufficiently diagnosable from congeners (e.g., treatments in Morse et al., 2017). Even when taxonomic revisions (such as the description of new species or the synonymy of multiple species under existing names) or new associations of larval and adult forms and checklists of known species distributions are published, these revisions may not be rapidly incorporated into the working benthic monitoring protocols of state or federal agencies. Agency protocols, often with committee or working group oversight, may be revised on multi-year or decadal schedules and not immediately responsive to changes in taxonomy or the discovery of new species. Finally, the errors in lists of species expected to occur in a state or region may take several different forms; propagated in different ways that obscure the true underlying patterns in distributions or abundance that form the basis of data-driven conservation assessments.
I have used the benthic monitoring taxonomic and traits database from the Illinois Environmental Protection Agency [ILEPA] (2011a,b) to illustrate some of these issues. I use a simple simulation to demonstrate the risks of relying entirely upon data from biological water quality monitoring programs to inform the conservation of aquatic insect species. I analyze previously published data from a large-scale inventory of aquatic insects in national parks to illustrate how the loss of information associated with genus level identification can obscure patterns of faunal differentiation and gamma richness. Finally, I explore some assumptions at the foundation of research programs in benthic monitoring, in particular, and freshwater ecology more generally.
Materials and methods
To describe the current state of knowledge of the aquatic insect assemblages used for biological stream monitoring in the state of Illinois, I obtained the Illinois Environmental Protection Agency [ILEPA] (2011a,b) benthic macroinvertebrate tolerance and trophic habit database (Supplementary Appendix 1). This database is the working list of “expected” benthic taxa for the state of Illinois, and is occasionally revised following discussions between ILEPA and working taxonomists and experts in the many aquatic taxa covered by the database. From this database I selected all species taxa from four of the most common and species-rich families of aquatic insects [hydropsychid caddisflies (Trichoptera: Hydropsychidae), perlid stoneflies (Plecoptera: Perlidae), heptageniid mayflies (Ephemeroptera: Heptageniidae), and baetid mayflies (Ephemeroptera: Baetidae)]. This database primarily inherits tolerance and trophic values from Barbour et al. (1999), but has been modified by input from local and regional benthic workers (TetraTech Inc, 2004; Illinois Environmental Protection Agency [ILEPA], 2011a,b).
Illinois EPT species taxonomic coverage
To compare the taxonomic coverage of the ILEPA database to the most up to date list of species known to occur within Illinois, I used museum records (Illinois Natural History Survey Insect Collection, University of Illinois Urbana-Champaign; Dmitriev, 2015), published checklists, literature reviews and other accounts of species occurrences in Illinois (Korecki, 2006; Rasmussen and Morse, 2020; McCafferty and Jacobus, 2021; DeWalt et al., 2022; and references therein), and databases of valid taxonomic names (Morse, 2021; DeWalt et al., 2022) to compile a list of the valid species from these four taxonomic families that have been reliably reported from Illinois (Supplementary Appendix 2). I sought to classify the following: (1) species known to occur in IL and included in ILEPA database (accurate), (2) species not known to occur in IL but erroneously included in the ILEPA database (extralimital), (3) species occurring in IL but not included in the ILEPA database (omission), (4) invalid species names (junior synonyms) in the ILEPA database, and (5) species occurring in IL that are entered in the ILEPA database under the wrong genus (invalid combinations).
Illinois EPT species trophic diversity
These four families have many representative species in Illinois, and at least one species in each group occupies nearly any conceivable lotic habitat in the state. I used the ILEPA database to summarize the frequency of each trophic category in the species of four common families of aquatic insects in Illinois: Baetidae (Ephemeroptera), Heptageniidae (Ephemeroptera), Hydropsychidae (Trichoptera), and Perlidae (Plecoptera). I compared the frequency of trophic groups in the species pools in the Illinois Environmental Protection Agency [ILEPA] (2011a,b) database (as it is used; including all taxonomic and geographic errors) to the frequency in the “true pool” that I constructed from the published lists and descriptions of valid species occurring in Illinois (Supplementary Appendix 2). Specifically, I compared the frequency of trophic groups between these two species pools.
Simulation of biodiversity loss: measures of diversity
To simulate the effects of reducing taxonomic precision on measures of species diversity, I first conceptualized 10 replicate communities of 100 species: 20 genera, each with 5 species. For each individual replicate, I assigned each species a random number between 1 and 100 and then rank ordered all 100 species by this number. Next, I simulated increasing random species loss to each set by removing the lowest 30, 50, and 70 ranked species, then calculated the number of species and genera remaining in each of the simulated communities (10 replicates at each level of biodiversity loss). I used the presence-absence matrix of each community (identified to species or genus level) to calculate the true alpha, beta, and gamma diversities, with the d function in the vegetarian package in R (Jost, 2007; Charney and Record, 2012; R Core Team, 2018).
Does taxonomic information loss obscure patterns from EPT species inventories?
To illustrate how the effect of taxonomic information loss could influence observed patterns in real (not simulated) inventory data, I used lists of Ephemeroptera, Plecoptera, and Trichoptera species published in Robinson et al. (2016) (a multi-year, multi-season inventory of aquatic insects across a protected area network of 17 different US National Parks, in the highlands of the southeastern United States). I include from those results some undescribed (or later described) species. Robinson et al. (2016) discussed Rhyacophila sp. 2, this species was later described as Rhyacophila dandaganu Robinson and Parker, 2019. The formally undescribed species “Pycnopsyche sp. A” discussed in Wojtowicz (1982) and the formally undescribed species Rhyacophila sp. 1 also discussed in Robinson et al. (2016) do not currently have names but are believed to be valid species. I used the presence-absence matrix of EPT species to build a pair-wise distance matrix (metric = “jaccard”) of the parks, then used the metaMDS function in R package vegan (Oksanen et al., 2018) to find and project the best solution for each ordination. I then used Procrustes analysis functions procrustes, and protest in R package vegan (Oksanen et al., 2018), for asymmetric rotation and symmetric permutation tests, respectively. These analyses compared the change in position of sites between two non-metric multidimensional scaling (NMDS) ordinations of the aquatic insect assemblages of these parks: one constructed from species level identifications, one constructed from genus-level identifications.
Results
ILEPA database taxonomic coverage
The ILEPA database includes 1757 total entries encompassing 5 phyla, 9 classes, 33 orders, 140 families and 602 genera. Within this diversity are 223 genera with no entries for any species in the genus, effectively precluding the use of these terminal taxa for tracking taxonomic species. There are 945 species-level entries in the database; of these 680 entries are insects. A full review of the status of all the benthic macroinvertebrate species in the database is beyond the scope of this paper; our efforts to produce the “true pool” of species occurring in Illinois from museum specimens and literature records is restricted to the four insect families.
Illinois EPT species taxonomic coverage
I quantified the number of valid species, junior synonyms, invalid combinations and extralimital species in the ILEPA database. Table 1 details the mismatches between the 103 relevant species in the ILEPA taxonomic database used for water quality monitoring (Supplementary Appendix 1) and the current summary of the 117 species known from Illinois derived from museum specimens and published lists (Supplementary Appendix 2). All types of errors exist in the database, for these four insect families: 51 valid species known to occur in Illinois are listed in the database (accurate), 11 extralimital species are included in the database (included in ILEPA but no Illinois records are known), 30 valid species are known from Illinois but not included in ILEPA (omission), 13 species are listed in ILEPA that are junior synonyms of other valid taxa, and 22 valid species are listed in ILEPA in the incorrect genus-species combination. These results are broken down into greater detail in the Supplementary material.
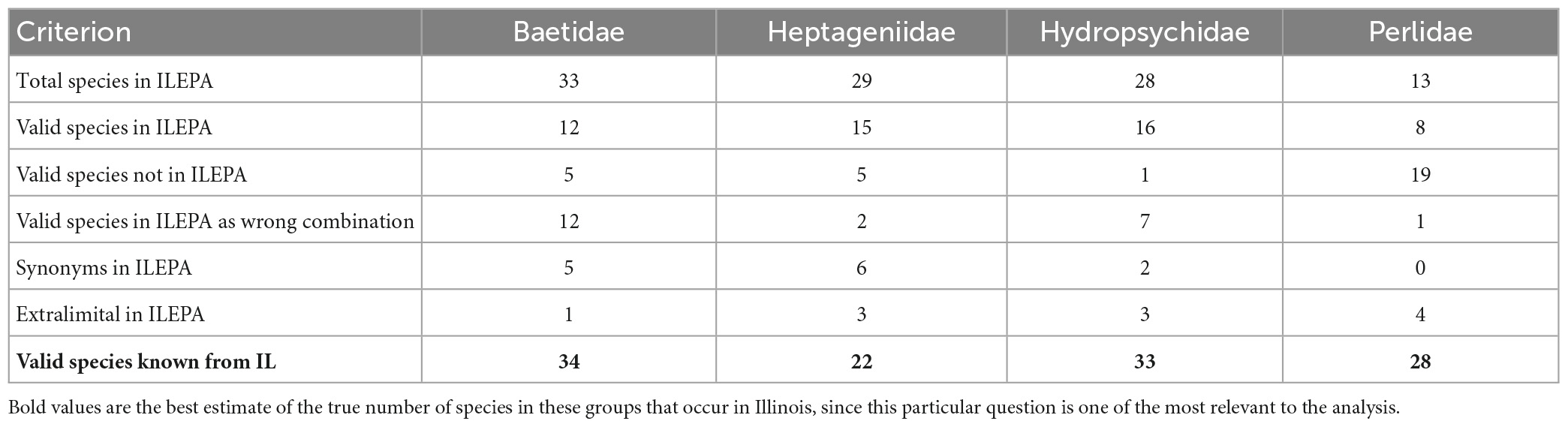
Table 1. Summary of species accounts from the Illinois Environmental Protection Agency [ILEPA] (2011a,b) database, and the “true pool” of valid species reliably reported as occurring in IL, constructed from museum collection records, literature records and databases of valid names.
Illinois EPT species taxonomic coverage
Figure 1 illustrates the proportion of taxonomic species in each group assigned, by ILEPA, to categories of collectors, predators, scrapers or unknown. In three families (Baetidae, Hydropsychidae, and Perlidae), the number of species assigned to the unknown (NA) trophic group is larger in the “true pool” of species occurring in Illinois than in the ILEPA database. One trophic group (hydropsychid predators) disappears altogether in the true pool. In all four EPT families, the benthic monitoring database (Illinois Environmental Protection Agency [ILEPA], 2011a,b) underestimates the number of species with unknown trophic affiliation.
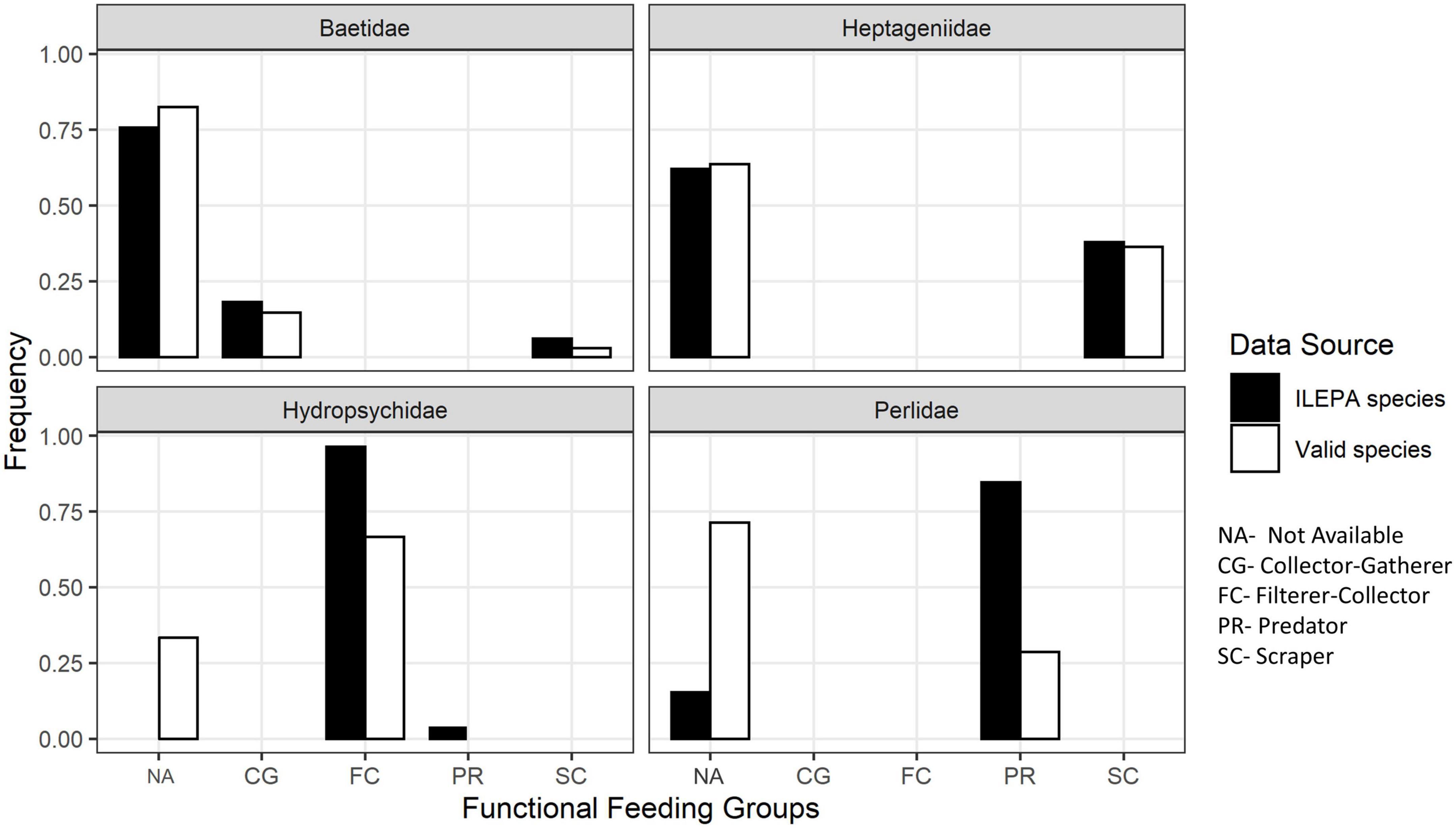
Figure 1. The frequency of functional feeding groups among the species in the Illinois Environmental Protection Agency [ILEPA] (2011a,b) benthic monitoring database, and the frequency among a list of valid species known to occur in Illinois compiled from museum specimens and literature records.
Simulation of biodiversity loss: measures of diversity
A simple example demonstrates the potential inability of genus-based methods to detect species loss in an unrealistic scenario. Consider some stream assemblage with 100 species, equally distributed among 20 genera, with 5 species per genus. It is possible for such a system to lose 80% of the species from the system (4 in each genus) prior to the loss of all species in a genus (Figure 2). A researcher using such a design (one that does not distinguish among the different species in the system, and instead relies on genus identifications) would not detect this loss.
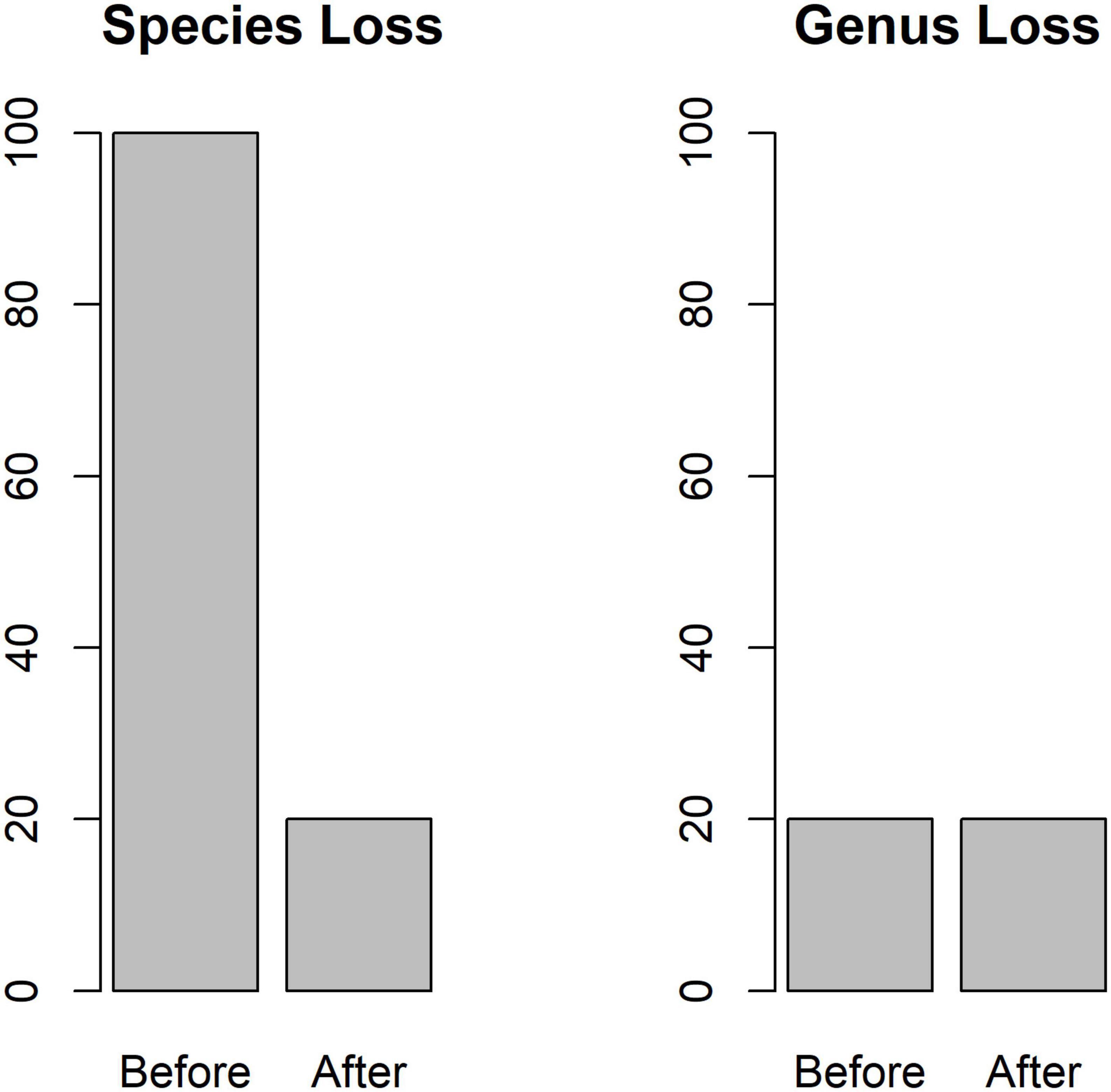
Figure 2. In a hypothetical stream system with 100 species equally distributed across 20 genera, up to 80% of the species could be extirpated before biodiversity loss is registered by genus level analyses.
A more realistic scenario may be one where species loss does not occur equitably across equally rich genera. I considered a model where all species are equally likely to go extinct, and species loss is a random draw from the same species pool (20 genera with 5 species each), replicated ten times for each category of taxonomic method (genus and species). When all species are equally likely to go locally extinct, genus-only methods may dramatically obscure the decline in biodiversity (Figure 3).
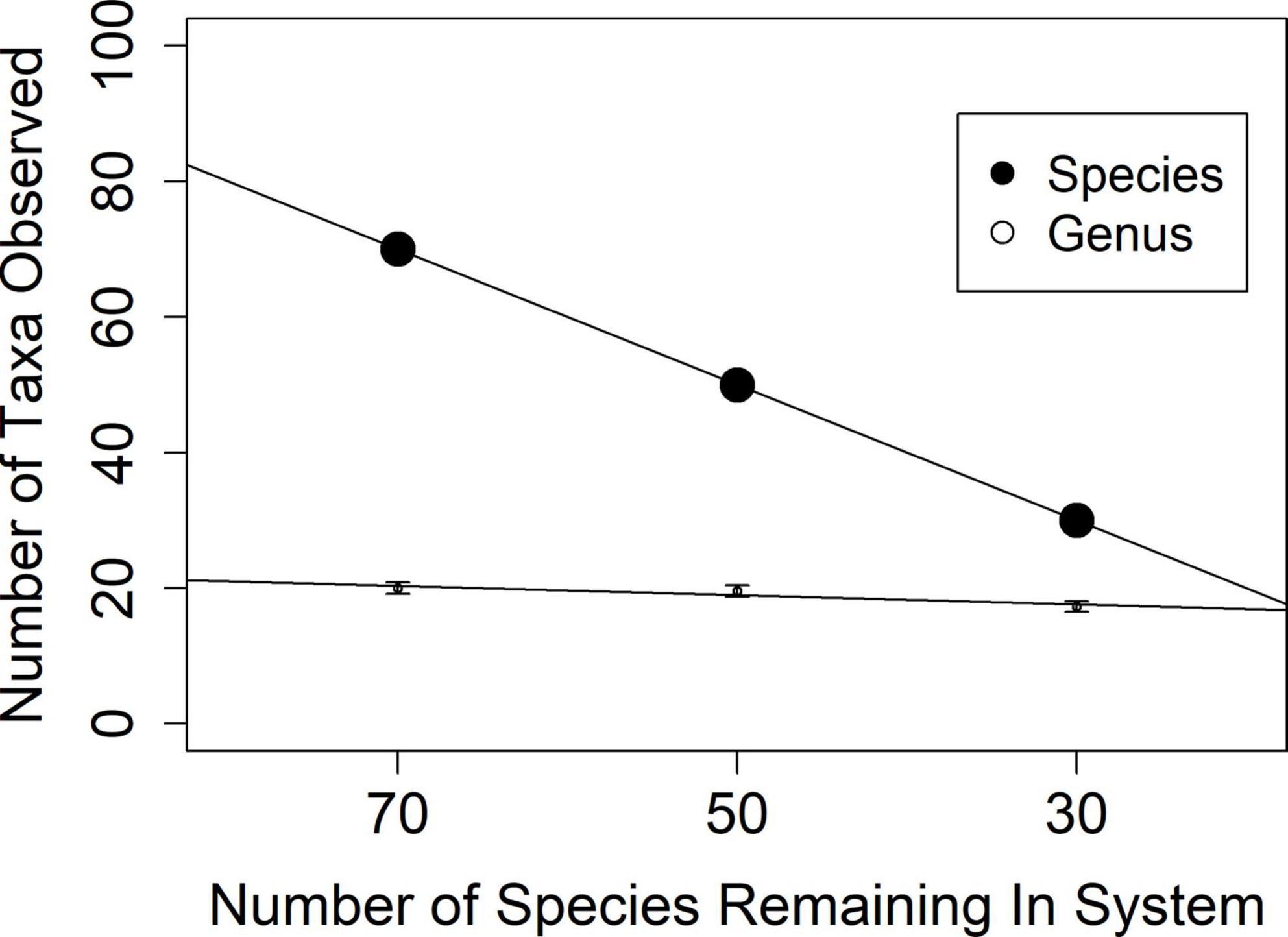
Figure 3. Genus and species richness in 10 simulated scenarios of increasing species loss from a hypothetical assemblage of 100 species in 20 genera. When species loss rates are equivalent across genera, genus level metrics fail to show taxonomic loss.
In our simulation, species turnover may be conceptualized as the effective number of distinct communities in the data. In other words, as simulated random species loss cumulatively eroded the assemblage (from 100 to 70, 50, and 30 species) across sites, estimates of the effective number of distinct communities from species data increased from one, to more than three, even as estimates from genus data did not vary (Figure 4). As long as one species in a genus persists at every site, there will appear to be no turnover.
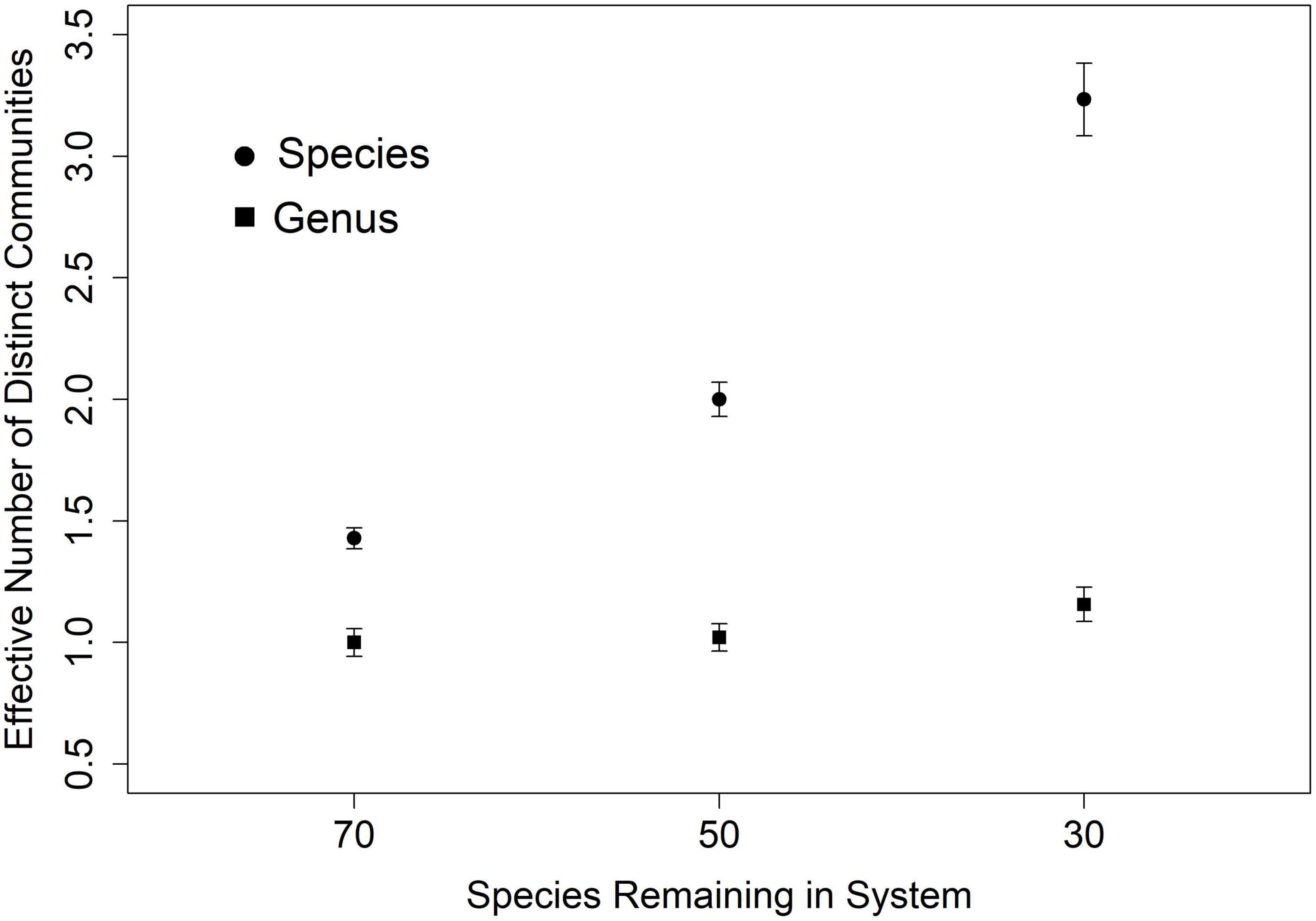
Figure 4. Genus level measure of beta diversity (effective number of distinct communities) in 10 simulated scenarios underestimates actual species turnover. This effect is larger as species assemblages are increasingly smaller draws from the regional pool of 100 species in 20 genera.
Does taxonomic information loss obscure patterns from EPT species inventories?
The biodiversity inventory described in Robinson et al. (2016) surveyed 17 national parks in 7 states, reporting 650 species of insects from 168 genera, in the orders Ephemeroptera, Plecoptera and Trichoptera, for an overall average of 3.9 species per genus. Caddisflies (Trichoptera) have an average of 4.8 species per genus in this inventory, stoneflies (Plecoptera) have 3.2 species per genus, and mayflies (Ephemeroptera) have 2.9 species per genus. Some genera have many species but most genera in each order have three or fewer species (Figure 5).
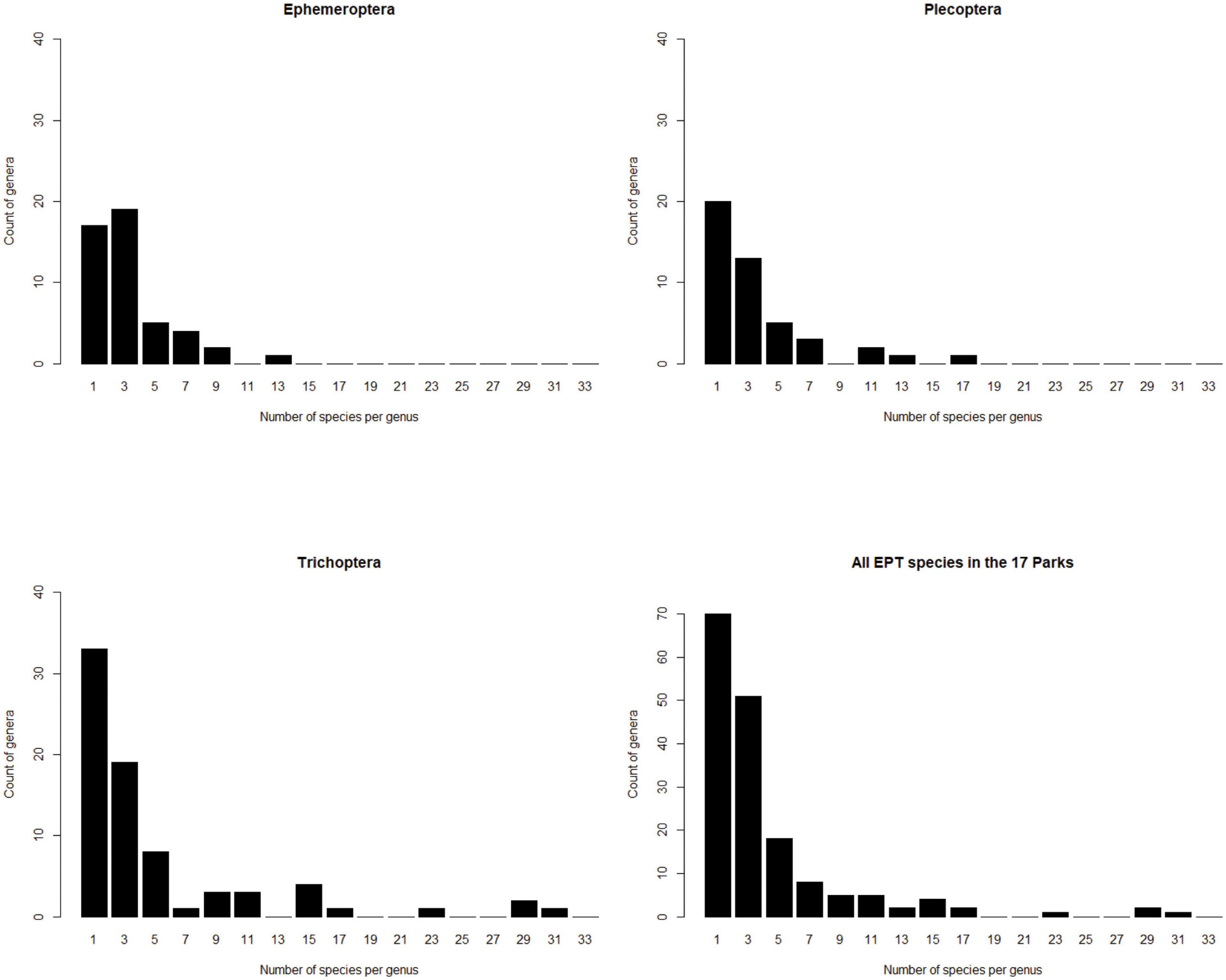
Figure 5. Species are not equitably distributed among genera in a survey of aquatic insects in 17 national parks in the southeastern United States (Robinson et al., 2016). The magnitude of this effect varies among insect orders.
Multivariate ordinations of aquatic insect assemblages in the national parks demonstrate that ordinations on genus-level identifications can obscure or even destroy patterns of similarity among the parks. Figure 6 is a plot of the Procrustes Error (change in position of sites) between NMDS plots built from jaccard distance matrixes of species and genus-level identifications. NMDS stress scores for each plot were high (species 0.280, genus 0.278) indicating that neither ordination was a particularly good explanation of the differences in species assemblages among parks. The Procrustes permutation test found low correlation between the ordinations (0.267) with no statistical significance (0.581).
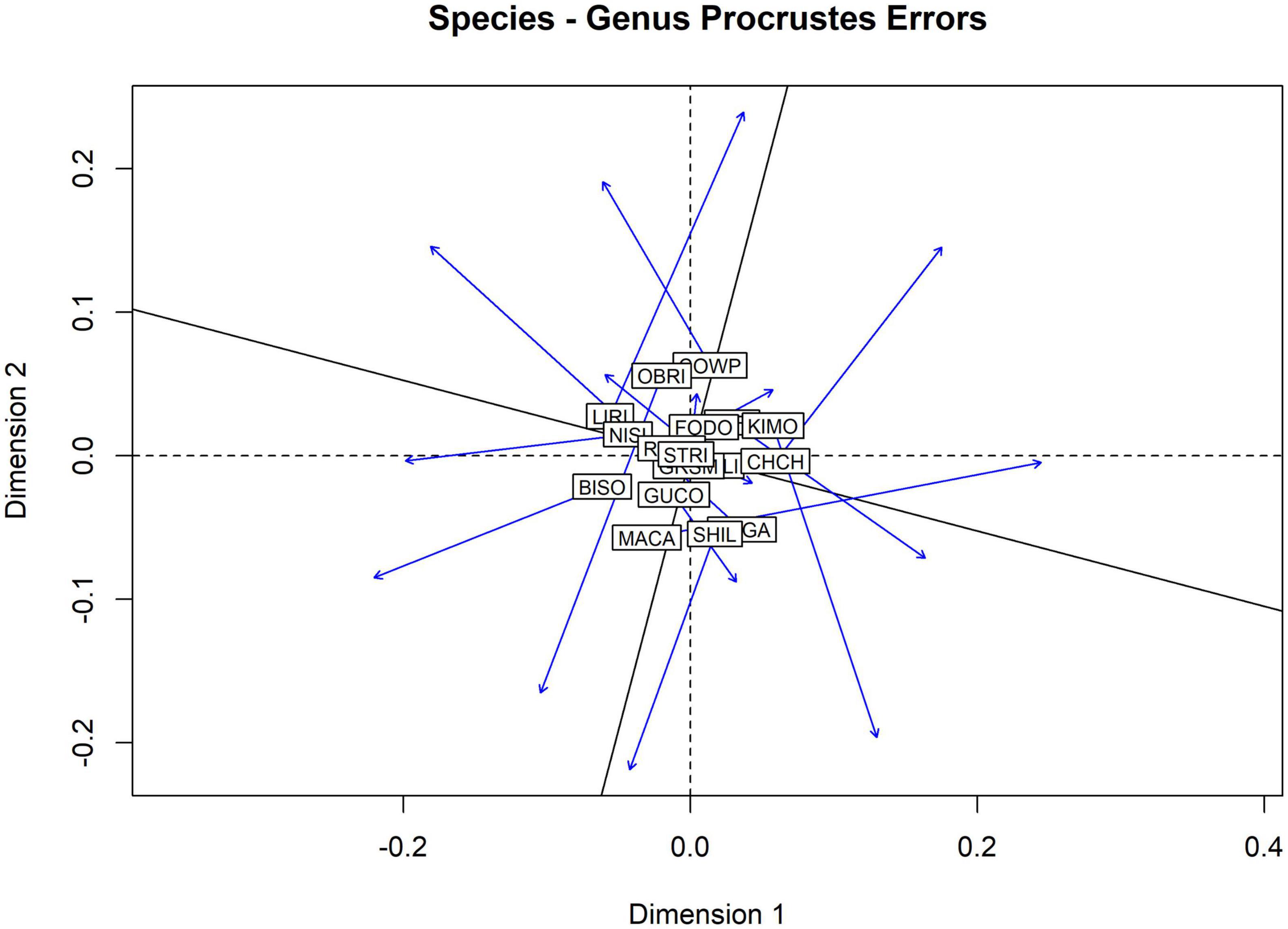
Figure 6. Procrustes analysis of non-metric multidimensional scaling (NMDS) ordinations on pairwise jaccard distances of the aquatic insect assemblages observed in17 national parks. Stress scores for NMDS ordinations were high (0.28 species, 0.278 genus) and low (0.267) statistically insignificant (0.588) correlation was observed between the two ordinations.
Discussion
Many published studies of diversity in aquatic systems have used genus or family level identifications of diatoms, insects and other aquatic organismal groups. The use of genus and family level information on these measures is a certain source of error when inferring patterns of species, but the magnitude (and direction) of the error likely will vary with the size and content of local and regional species pools, and with the sampling and/or analytical methods chosen by researchers. One result is clear: measures of changes in faunal diversity (richness, turnover, or properties of species pools) derived from genus-based methods are unlikely to be accurate. The implications of this flaw in the benthic research program reach more than just to measures of diversity, or to the conservation of species, and are worth considering briefly.
Ecological interchangeability of species in a genus
Worldwide, stream systems often perform similar functions in different landscapes (Vannote et al., 1980). It is likely no coincidence that there are general similarities in the ecological and evolutionary forces challenging the survival, dispersal and reproduction of organisms in aquatic systems across the planet (Forbes, 1887). On this view, higher phylogenetic groupings of aquatic insect species are essentially “packages” of shared (phylogenetic conserved) traits that are inherited adaptive responses to common environmental conditions. Genus or family based approaches utilize the first assumption in Table 2: species within these groupings are ecologically interchangeable.
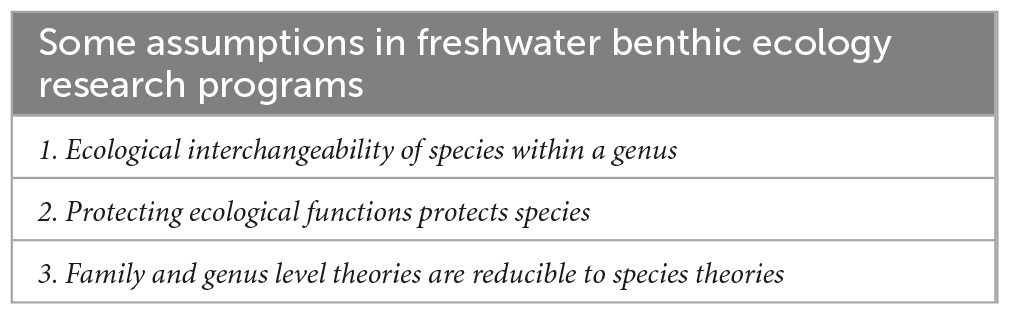
Table 2. These general assumptions underly many research questions utilizing benthic macroinvertebrates, particularly insects.
Although some organizational schemes designed to leverage the phylogenetic conservatism of aquatic insect ecological responses have been described as “species traits” (e.g., Poff, 1997), in practice immature forms of benthic insects may only infrequently be confidently diagnosed to actual species. In addition, many Hutchinsonian gaps (Cardoso et al., 2011) in the knowledge of the trophic and behavioral ecology of species persist. Because of this indeterminacy, benthic bioassessments have utilized higher taxonomic groupings (like genus or family) to ask questions in the vein of “What are organisms in these habitats doing?” rather than “What species occur in this habitat?” For example, changes in the relative abundance of some taxonomic groups may be construed evidence of certain types of pollution or disturbance (e.g., the disproportionate abundance of filter feeders in a system may be evidence of the organic enrichment and loss of riparian inputs). However, if the abundance or biomass of persisting species increase to compensate for the lost abundance of the other species, it can be the case that the biomass or abundance of some coarse taxonomic group does not change despite the loss of many species in each genus. Unpredictable ecosystem effects can attend from variation in the pattern of species loss or compensatory dynamics in aquatic systems (Thomsen et al., 2017).
In at least some cases, the generalization of “ecological interchangeability of lower taxa within a higher taxon” is more of an assumption than a demonstrated empirical fact. In my example from the ILEPA dataset, 28 of the 34 valid baetid mayfly species known from Illinois have unknown trophic categories. In taxonomic groups such as scraping heptageniid mayflies, filter-feeding hydropsychid caddisflies or predatory perlid stoneflies, assumptions of trophic function may be more likely to be accurate than in groups where there are fewer obvious morphological and behavioral adaptations “built into” the form of the animal. More frustrating is the complexity introduced to these classifications by the consideration of different instars or developmental stages of the immature insects. Direct observation or assessment of the ecological and trophic tendencies of species remain poorly known across ontogeny (e.g., I have seen 5th instars of ostensibly wood-shredding phryganeid caddisflies (Ptilostomis sp.) eating each other in my rearing chambers). These “Hutchinsonian” knowledge gaps, prevalent across many insect orders, should warrant caution around naïve assumptions of ecological interchangeability within taxonomic groups in any particular system. To the extent that the assumption of interchangeability does not hold in a local assemblage, this imprecision has the potential to introduce errors to the analysis of the frequency of traits in the species of that assemblage.
Meticulous studies of life history, habitat use, or careful rearing of individuals from different sites have provided an empirical basis of support for the assumption of interchangeability [see Brigham et al. (1982) for an excellent compendium of life history and autecological information for species of aquatic organisms in the southeastern United States]. Some analytical studies have professed support for the ecological interchangeability hypothesis. For a breadth of views as examples, see Martin et al. (2016) for a study that claims support for the ecological interchangeability of species (albeit, by using mtDNA barcodes instead of species level taxonomy), or Bowman and Bailey (1997) for an example that claims support for ecological interchangeability of genera within families, or Jones (2008) who points out (in a review of much of that literature) many conflicting results of studies on the level of ecological interchangeability within aquatic systems; for him these may be explained by variation in the goals and designs of studies, and the subjective preferences of authors in the optimal level of taxonomic detail.
I demonstrated the breadth of this knowledge gap in trophic status in the dataset used by the Illinois Environmental Protection Agency [ILEPA] (2011a,b) to characterize benthic macroinvertebrate communities in wadeable streams statewide (Figure 1), but this gap is not limited to Illinois or these four families of three common insect orders. In many instances (e.g., v ix B, Barbour et al., 1999) there can be substantial disagreement among estimates of taxon tolerance derived from workers in different regions of the United States. In nature, things vary, and the need to strike a truce between (1) taxonomic detail and (2) reliable inference of ecological function implicitly demands some loss of information. Benthic workers should endeavor to make the sorts of careful observational studies that characterized the early work toward standardized benthic monitoring in order to continue closing these knowledge gaps. Among some group, if some species are less ecologically interchangeable yet more imperiled (i.e., more intolerant to pollution, have smaller geographic range sizes or express narrower habitat preferences) then genus- or family-level biomonitoring may amplify gaps in conservation coverage.
Conservation of function does not implicitly facilitate the conservation of species
In Table 2, I suggest a second assumption that I believe underlies many regulatory and research programs: protecting ecological functions will protect species (Strayer, 2006). That the evolutionary conservatism of aquatic insect groups supports the first assumption (Table 2) also provides a reasoned basis for this argument, since species in a genus may have similar ecological functions or habitat preferences. However, to the extent that this generalization fails to hold (species within genus or family groupings are ecologically redundant), it may not be the case that biodiversity conservation protections afforded by conserving watershed conditions or stream function will not be equitably distributed among species in each genus. Further, metrics like “% shredders” do not have any substantive implications for the conservation of species: the reduction of a hypothetical species-rich community of shredding organisms to a population of one single species (attaining equal biomass and/or abundance to the former community) would not shift the metric, but has clear implications for loss of species diversity.
One strategy to approach this assumption from a regulatory angle has been to develop water quality criteria of species sensitivity distributions (SSDs) that (for benthic macroinvertebrates) rely on genus-level data to set acceptable levels of environmental insult that will protect some percentage of the taxa (e.g., Cormier and Suter, 2013). In this case, a criterion or benchmark is assumed to protect all species within a genus. However, as the example in Figure 2 demonstrates, these criteria may fail to protect the desired threshold of species in the system, if the more sensitive species are extirpated before the least sensitive species of the genus and the SSD is set for genera.
Again, the extent of this effect of this unmet assumption will likely vary across local assemblages and regional species pools, but the potential ramifications for the conservation of species are serious. When states occupy multiple biogeographic regions, i.e., there are many different local species pools for a similar habitat across a region, genus-based SSDs will fail to provide equal protection across taxa. The only way to reliably identify trends in the abundance or occurrence of species is to collect and evaluate information that can be associated to adult life history stages with certainty.
Family and genus level theories are reducible to species theories
A third assumption I believe to be embedded within modern freshwater benthic ecology is an assumption that family and genus level theories are reducible to species theories. This assumption may apply to other ecological research programs that have forsaken classical taxonomic procedures for the seductive apparent simplicity of DNA barcodes or morpho-taxa. Instead of grappling with the deeper metaphysical issues underlying the foundation of ecology and evolution, freshwater ecologists have largely ignored the philosophical issues raised by the introduction of non-cladistic theoretical units like DNA barcodes, “morphospecies” and other paraphyletic pseudotaxonomic units. The long-running debates on whether species are “real,” and if not “what are they?” (e.g., Mayr, 1996; Stamos, 2003) is a good place to begin to frame a broader review of some of the potential problems created by benthic monitoring programs.
A brief discussion of explanatory reduction and ecology
One general measure of the success or utility of a scientific theory is the capacity of a theory to be reducible to other theories, i.e., for the working parts of one theory to have a 1:1 relation to the working parts of another theory. Nagel (1961) put this another way: “theoretical notions employed in the basic assumptions of a theory may either not be associated with any experimental ideas whatever, or may be associated with experimental ideas that vary from context to context. The possibility of extending a theory to cover fresh subject matter depends in considerable measure upon this feature of theories” (Nagel, 1961, p. 105).
For Nagel, the generalization of empirical observations formed the basis of “experimental laws” that were linked to the “theoretical laws” by the correspondence of terms. In biological theory, species are the conceptual entities that connect empirical observations to predictions predicated on theoretical ecological and evolutionary mechanisms. Hennig (1966) thoroughly explored and justified that species (as clades) are one of many possible theoretical entities, but most family or genus level groupings of benthic macroinvertebrates are not clades, and thus observations of those entities cannot be satisfactorily reduced to observations of species or evolutionary units.
Darwin (1859) described his theory (evolution from natural selection on heritable characters) as an explanation for the origin of new species. Ecological and evolutionary theories explain many disparate empirical observations. Patterns of species richness, geographic distributions, habitat use, competitive exclusion, behavioral interactions, mate choice, population dynamics, dispersal and extinction are all examples of ecological phenomena (explananda) reducible to evolutionary theory by the use of “species” as an explanans (Hempel and Oppenheim, 1948). The correspondence of one such theory to another is a function of the ontological relations implied by “species”: common descent, reproductive compatibility, phenotypic cohesion, and so on (Mayr, 1996). For insects, in particular, these relations are signified by genitalic morphology (Shapiro and Porter, 1989) and not by morphological features borne by immature forms.
The question that must be faced is whether scientific research employing family or genus level identifications will ever be reducible to ecological or evolutionary theories on species [condition termed “incommensurability” by Feyerabend (1962)]. On consideration, it remains unclear what information that measures like “genus richness” or “family richness” are supposed to carry (or even could carry, in principle, except as totems of uncertainty). Since some genera contain many more species than other genera, the prospect of utilizing higher taxa as replicable or standardized units of evolutionary change has advanced very little since Hennig (1966) first took up this challenge (indeed, this problem is recognized by biologists of all backgrounds, see (Stevens (1985) or Round (1997) for the perspectives of a botanist and a diatomologist, respectively). In our case, in any particular and specific geographic area the species in a genus or a family are unlikely to form a clade (i.e., a group of species that are all the descendants of a single common ancestor). In some instances, a genus or family may only form a clade when considering all of the species on the globe. How can patterns of genus richness or other measures be meaningfully compared between two systems that have large differences in the frequency of species within genera, and the genera themselves are not evolutionary units?
The nature of the available tools shapes the questions that are asked
Molecular methods have been proposed as one potential solution to the problem of imprecise determination, and in the case of EPT insects have proven useful for distinguishing many species in taxonomic libraries (e.g., Zhou et al., 2011), associating larval forms with adults diagnosable to species (Ruiter et al., 2013), and as one of several integrated tools to illuminate phylogenetic structure (Zhou et al., 2016). Nonetheless, many of the applications of molecular barcoding remain firmly entrenched in the biomonitoring framework. Even if bar code methods were fully elaborated (all nominal species included and diagnosable from sequence alone), the purposes of biomonitoring (i.e., defining meaningful ecological metrics transferable across biogeographical boundaries and species pools) would remain at odds with species level biological inventories (demographic or biogeographic imperilment risk assessment of particular species at particular historic sites, habitats or systems).
Attempts to bridge the decline in taxonomic expertise with technological solutions suggest to some that the decline in the number of taxonomists has coincided with a decline in the general understanding among the broader community of ecological and evolutionary biologists of what taxonomy does and does not do (Engel et al., 2021). In some instances this has resurrected the ghosts of methods past: “The use of arbitrary ‘threshold values’ as measured by [DNA barcode] methodology to ‘distinguish species’ is nothing but a recent avatar of phenetic taxonomic methods that were dismissed long before the onset of DNA sequencing” (Engel et al., 2021, p. 385). In the case of aquatic insect species, we are fortunate to have species concepts that are predicated on the properties of adult genitalia, and a theoretical basis for justifying those concepts (Shapiro and Porter, 1989). The conservation of species requires us to make use of these tools.
What treacherous shoals lie downstream of these unmet assumptions?
The success of family and genus based methods in bioassessment (propelled by the “good enough for regulatory work” feature of genus and family level trophic relations) rapidly led to the use of these data to test other hypotheses. From the inception of this paradigm, biologists recognized that the use of higher-level taxonomic groupings (although a useful trade-off) came with consequences. For an account of the history of this discussion in relation to freshwater aquatic insects, along with a reasoned argument for a middle ground approach to water quality assessment, see Lenat and Resh (2001). I have used EPT orders as examples in this paper, due to my own familiarity with these groups, but a review of the literature in many other specific fields of interest will suggest how some problems I have identified here might follow from the use of imprecise taxonomy in other freshwater insect orders.
For example, taxonomic groups such as chironomid midges (Diptera) have many more species, and (in Illinois, at least, and relative to EPT) we have a poorer understanding of local and regional species pools (including rarity and imperilment risks). More sobering is the fact that there are few active workers competent at species-level diagnoses of adults in this group. Yet, paraphyletic higher taxa from this group are also commonly used for bioassessment, ecological and evolutionary studies. There are likely to be many more groups of aquatic crustaceans that could provide fertile ground for pointing out the misuse and abuse of paraphyletic taxonomic units. This issue is not entirely separate from the problem of how these practices create gaps in conservation protections. Without citing particular studies or authors, but just to provide a few examples, the inappropriate use of (paraphyletic) family and genus level methods have been used to test longitudinal patterns of diversity implied by the River Continuum Concept, compare patterns of richness among headwaters and larger order streams, test various mechanisms of community and metacommunity assembly, and to set priorities for freshwater conservation.
Is this really a problem, or is this pedantry? Nature is not uniform in her distribution of species to higher taxa, but neither are scientists in their categorization of those taxa. “Rank allocations of taxa are the products of a heterogeneous mixture of ideas through the last 250 years” (Bertrand et al., 2006, p. 156). The higher classification of living things reflects both historic evolutionary processes as well as the idiosyncrasies and historical vagaries of biologists. Except in the unrealistic edge case, when all genera are monotypic, it is trivially true that measures of aquatic insect species diversity estimated from genus or family-level identifications will underestimate species richness. The extent to which this is the case is the important question. Making sense of the inequitable distribution of species among genera is a classic problem of theoretical cladistics (Clayton, 1972), and has a long history of investigation in aquatic insects as well (Beck and Beck, 1968; Lindeberg, 1971). In most freshwater systems, some genera will have many species and some will be monotypic in that system (Figure 5). This provides a serious challenge to direct inference (of species level patterns) using genus or family level abundance data, since higher-level taxa richness may not function as a reliable proxy for species richness across all taxonomic levels, or across different spatial scales or areas of endemism (Gaston and Williams, 1993). Most importantly, genus or family level approaches do nothing to inform the conservation of species themselves.
This problem is far larger than benthic macroinvertebrate bioassessment, since these methods are used in ecology and evolutionary analyses, and simply forcing a finer taxonomic resolution onto benthic samples will not fill the gaps in conservation protection that aquatic insect species (increasingly) face. Neither is a glib solution like “let them learn to collect and identify adults”. The most important point here is to remind benthic workers that this problem (information gaps limiting conservation efforts) exists and is largely a consequence of misapplying bioassessment methods to conservation questions. To conserve aquatic insect species qua species, inventory and assessment protocols must be devised that seek to accurately and precisely detect species, account for variation in abundances and probability of detection, discover and elaborate habitat preferences, describe species pools, delimit phenological patterns and account for variation in observer efforts.
Evolutionary systematists have long recognized that common aquatic insect lineages are rife with pattern: rampant morphological and ecological symplesiomorphies and synapomorphies have provided the evidential basis for many phylogenetic hypotheses (Ross, 1956; Illies, 1965; Edmunds, 1972; McCafferty, 1991; Zwick, 2000). For over a century, these phyletic tendencies (toward similarity of form and function) have provided a scientific basis for using higher taxonomic levels of aquatic insects to assess the biological condition of flowing waters (Hynes, 1970; Cairns and Pratt, 1993; Cummins, 2016). Biological assessments, using family and genus-level benthic macroinvertebrate data serve a critical and unique regulatory need (Kenney et al., 2009), balancing specificity and generality. However, in order to conserve species we must observe species, and this may require biologists working with these groups to (among other things) familiarize themselves with (1) identification of multiple life history stages, (2) the composition of local and regional species pools, (3) associations of habitats, biogeographical regions at a finer scale than employed for monitoring, (4) lists of imperiled species, (5) museum collections and other specimen databases, (6) new literature libraries, (7) network with a larger sphere of workers.
Conclusion
I have demonstrated some ways that relying on genus-level identification in freshwater benthic ecological research programs can obscure or hinder patterns relevant to the conservation of species. In doing so, I identified three assumptions that I believe are endemic to a constellation of research programs in freshwater benthic ecology. I suggest that these assumptions are frequently unmet, and I have explored how real-world monitoring programs are likely to fail to meet these assumptions. I have offered some illustrations of how the loss of information resulting from using genus or family level data can obscure even strong ecological patterns. Finally, I have attempted to sketch why this problem has a much deeper significance to our science than a methodological quibble and offer some suggestions for the researcher.
Data availability statement
Publicly available datasets were analyzed in this study. This data can be found here: https://link.springer.com/article/10.1007/s10841-016-9889-3.
Author contributions
The author confirms being the sole contributor of this work and has approved it for publication.
Conflict of interest
The author declares that the research was conducted in the absence of any commercial or financial relationships that could be construed as a potential conflict of interest.
Publisher’s note
All claims expressed in this article are solely those of the authors and do not necessarily represent those of their affiliated organizations, or those of the publisher, the editors and the reviewers. Any product that may be evaluated in this article, or claim that may be made by its manufacturer, is not guaranteed or endorsed by the publisher.
Supplementary material
The Supplementary Material for this article can be found online at: https://www.frontiersin.org/articles/10.3389/fevo.2023.1148516/full#supplementary-material
References
Barbour, M. T., Gerritsen, J., Snyder, B. D., and Stribling, J. B. (1999). Rapid bioassessment protocols for use in streams and Wadeable Rivers: Periphyton, benthic macroinvertebrates and fish, 2nd Edn. Washington, DC: U.S. Environmental Protection Agency; Office of Water.
Beck, W. M. Jr., and Beck, E. C. (1968). The concept of genus in the family Chironomidae. Ann. Zool. Fennici 5, 14–16.
Bertrand, Y., Pleijel, F., and Rouse, G. W. (2006). Taxonomic surrogacy in biodiversity assessments, and the meaning of Linnaean ranks. Syst. Biodivers. 4, 149–159. doi: 10.1017/S1477200005001908
Bowman, M. F., and Bailey, R. C. (1997). Does taxonomic resolution affect the multivariate description of the structure of freshwater benthic macroinvertebrate communities? Can. J. Fish. Aqu. Sci. 54, 1802–1807. doi: 10.1139/f97-085
Bried, J. T., and Hinchliffe, R. P. (2019). Improving taxonomic resolution in large-scale freshwater biodiversity monitoring: An example using wetlands and Odonata. Insect Conserv. Divers. 12, 9–17. doi: 10.1111/icad.12323
Brigham, A. R., Brigham, W. U., and Gnilka, A. (1982). Aquatic insects and oligochaetes of North and South Carolina. Mahomet, IL: Midwest Aquatic Enterprises, 837.
Cairns, J. Jr. (1975). Critical species, including man, within the biosphere. Die Naturwissenschaften 62, 193–199. doi: 10.1007/BF00603163
Cairns, J. Jr. (1977). “Quantification of biological integrity,” in Proceedings of a symposium 10- 12 March 1975: The integrity of water, eds R. K. Ballentine and L. J. Gurriaia (Washington DC: U.S. Environmental Protection Agency, Office of Water and Hazardous Materials), 171–187.
Cairns, J. Jr., and Pratt, J. R. (1993). “A history of biological monitoring using benthic macroinvertebrates,” in Freshwater biomonitoring and benthic macroinvertebrates, eds D. M. Rosenberg and V. H. Resh (New York, NY: Chapman and Hall).
Cardoso, P., Erwin, T. L., Borges, P. A. V., and New, T. R. (2011). The seven impediments in invertebrate conservation and how to overcome them. Biol. Conserv. 144, 2647–2655. doi: 10.1016/j.biocon.2011.07.024
Charney, N., and Record, S. (2012). Vegetarian: Jost diversity measures for community data. R package version 1.2.
Clayton, W. D. (1972). Some aspects of the genus concept. Kew Bull. 27, 281–287. doi: 10.2307/4109454
Cormier, S. M., and Suter, G. W. II (2013). A method for deriving water-quality benchmarks using field data. Environ. Toxicol. Chem. 32, 255–262. doi: 10.1002/etc.2057
Cranston, P. S. (1990). Biomonitoring and invertebrate taxonomy. Biol. Assess. Impact 14, 265–273. doi: 10.1007/BF00677921
Cummins, K. W. (2016). Combining taxonomy and function in the study of stream macroinvertebrates. J. Limnol. 75(Suppl. 1) 235–241. doi: 10.4081/jlimnol.2016.1373
Darwin, C. (1859). On the origin of species by means of natural selection, or, The preservation of favoured races in the struggle for life. London: J. Murray. doi: 10.5962/bhl.title.82303
Davies, S. P., and Jackson, S. K. (2006). The biological condition gradient: A descriptive model for interpreting change in aquatic ecosystems. Ecol. Appl. 16, 1251–1266. doi: 10.1890/1051-0761(2006)016[1251:TBCGAD]2.0.CO;2
DeWalt, R. E., Maehr, M. D., Hopkins, H., Neu-Becker, U., and Stueber, G. (2022). Plecoptera species file. Version 5.0/5.0. Available online at: http://Plecoptera.SpeciesFile.org (accessed December 1, 2022).
Dmitriev, D. (2015). Illinois natural history survey insect collection. Champaign, IL: Illinois Natural History Survey.
Edmunds, G. F. (1972). Biogeography and evolution of Ephemeroptera. Annu. Rev. Ecol. Syst. 17, 21–42. doi: 10.1146/annurev.en.17.010172.000321
Engel, M. S., Ceriaco, L. M. P., Daniel, G. M., Dellape, P. M., Lobl, I., Marinov, M., et al. (2021). The taxonomic impediment: A shortage of taxonomists, not the lack of technical approaches. Zool. J. Linnean Soc. 193, 381–387. doi: 10.1093/zoolinnean/zlab072
Feyerabend, P. (1962). “Explanation, Reduction and empiricism,” in Scientific explanation, space and time: Minnesota studies in the philosophy of science, Vol. III, eds H. Feigl and G. Maxwell (Minneapolis, MN: University of Minneapolis Press), 28–97.
Forbes, S. A. (1887). The lake as a microcosm. Bull. Il. Nat. Hist. Surv. 15, 537–550. doi: 10.21900/j.inhs.v15.303
Frey, D. G. (1975). “Biological integrity, a historical approach,” in The integrity of water: Proceedings of a symposium 10–12 march 1975, eds R. K. Ballentine and L. J. Gurriaia (Washington DC: U.S. Environmental Protection Agency, Office of Water and Hazardous Materials), 127–140.
Gaston, K. J., and Williams, P. H. (1993). Mapping the world’s species- the higher taxon approach. Biodivers. Lett. 1, 2–8. doi: 10.2307/2999642
Hempel, C. G., and Oppenheim, P. (1948). Studies in the logic of explanation. Philos. Sci. 15, 135–175. doi: 10.1086/286983
Hilsenhoff, W. L. (1988). Rapid field assessment of organic pollution with a family-level biotic index. J. North Am. Benthol. Soc. 7, 65–68. doi: 10.2307/1467832
Illies, J. (1965). Phylogeny and zoogeography of the Plecoptera. Annu. Rev. Entomol. 10, 117–140. doi: 10.1146/annurev.en.10.010165.001001
Illinois Environmental Protection Agency [ILEPA] (2011a). Genus-List: Macroinvertebrate index of biotic integrity (m-IBI) tolerance list and functional feeding group classification. Illinois environmental protection agency bureau of water document control number 178. Springfield, IL: Illinois Environmental Protection Agency [ILEPA], 31.
Illinois Environmental Protection Agency [ILEPA] (2011b). Genus-List: Macroinvertebrate index of biotic integrity (m-IBI) tolerance list and functional feeding group classification. Illinois environmental protection agency bureau of water document control number 176. Springfield, IL: Illinois Environmental Protection Agency [ILEPA], 75.
Jones, C. F. (2008). Taxonomic sufficiency: The influence of taxonomic resolution on freshwater bioassessments using benthic macroinvertebrates. Environ. Rev. 16, 45–69. doi: 10.1139/A07-010
Jost, L. (2007). Partitioning diversity into independent alpha and beta components. Ecology 88, 2427–2439. doi: 10.1890/06-1736.1
Karr, J. R., Fausch, K. D., Angermeier, P. L., Yant, P. R., and Schlosser, I. J. (1986). Assessing biological integrity in running waters: A method and its rationale. Illinois natural history survey special publication 5. Champaign, IL: University of Illinois, 28.
Kenney, M. A., Sutton-Grier, A. E., Smith, R. F., and Gresens, S. E. (2009). Benthic macroinvertebrates as indicators of water quality: The intersection of science and policy. Terr. Arthropod Rev. 2, 99–128. doi: 10.1163/187498209X12525675906077
Korecki, J. (2006). Revision of the males of the Hydropsyche scalaris group in North America (Trichoptera: Hydropsychidae). M.S. thesis. Clemson, SC: Clemson University.
Lenat, D. R., and Resh, V. H. (2001). Taxonomy and stream ecology–the benefits of genus- and species-level identifications. J. North Am. Benthol. Soc. 20, 287–298. doi: 10.2307/1468323
Lindeberg, B. (1971). The delimitation of genera among the Chironomidae (Diptera). Can. Entomol. 103, 373–376. doi: 10.4039/Ent103373-3
Martin, G. K., Adamowicz, S. J., and Cottenie, K. (2016). Taxonomic resolution based on DNA barcoding affects environmental signal in metacommunity structure. Freshwater Sci. 35, 701–711. doi: 10.1086/686260
McCafferty, W. P. (1991). Toward a phylogenetic classification of the Ephemeroptera (Insecta): A commentary on systematics. Ann. Entomol. Soc. Am. 84, 343–360. doi: 10.1093/aesa/84.4.343
McCafferty, W. P., and Jacobus, L. M. (2021). Mayfly central North America species list. Available online at: https://www.entm.purdue.edu/mayfly/na-species-list.php (accessed February 1, 2021).
Minshall, W. G. (1988). Stream ecosystem theory: A global perspective. J. North Am. Benthol. Soc. 7, 263–288.
Morse, J. C., McCafferty, W. P., Stark, B. P., and Jacobus, L.M (editors) (2017). Larvae of the southeastern USA mayfly, stonefly, and caddisfly species, Vol. 9. Asheville, NC: South Carolina Agriculture and Forestry Research System.
Morse, J.C. (ed.) (2021). Trichoptera world checklist. Available online at: https://trichopt.app.clemson.edu/welcome.php (accessed February 1, 2021).
Nagel, E. (1961). The structure of science: Problems in the logic of scientific explanation. New York, NY: Harcourt, Brace & World, Inc, 618.
Oksanen, J., Blanchet, F. G., Friendly, M., Kindt, R., Legendre, P., McGlinn, D., et al. (2018). vegan: Community ecology package. R package version 2. 5-2. Available online at: https://CRAN.R-project.org/package=vegan
Poff, N. L. (1997). Landscape filters and species traits: towards mechanistic understanding and prediction in stream ecology. J. North Am. Benthol. Soc. 16, 391–409. doi: 10.2307/1468026
Polhemus, D. A. (1993). Conservation of aquatic insects: Worldwide crisis or localized threats? Am. Zool. 33, 588–598. doi: 10.1093/icb/33.6.588
R Core Team (2018). R: A language for statistical computing. Vienna: R Foundation for Statistical Computing.
Rasmussen, A. K., and Morse, J. C. (2020). Distributional checklist of nearctic Trichoptera. Tallahassee, FL: Florida A&M University, 517.
Resh, V. H., and Unzicker, J. D. (1975). Water quality monitoring and aquatic organisms: The importance of species identifications. J. Water Pollut. Control Fed. 47, 9–19.
Robinson, J. L., Fordyce, J. A., and Parker, C. R. (2016). Conservation of aquatic insect species across a protected area network: Null model reveals shortfalls of biogeographical knowledge. J. Insect Conserv. 20, 565–581. doi: 10.1007/s10841-016-9889-3
Robinson, J. L., and Parker, C. R. (2019). Rhyacophila dandaganu, a new caddisfly from the Southern Cumberland Plateau (USA) (Trichoptera: Rhyacophilidae). Zoosymposia 14, 261–272.
Ross, H. H. (1956). Evolution and classification of the mountain caddisflies. Urbana, IL: University of Illinois Press, 213.
Ruiter, D. E., Boyle, E. E., and Zhou, X. (2013). DNA barcoding facilitates associations and diagnoses for Trichoptera larvae of the Churchill (Manitoba, Canada) area. BMC Ecol. 13:5. doi: 10.1186/1472-6785-13-5
Schmidt-Kloiber, A., and Nijboer, R. C. (2004). The effect of taxonomic resolution on the assessment of ecological water classes. Hydrobiologia 516, 269–283. doi: 10.1023/B:HYDR.0000025270.10807.10
Shapiro, A. M., and Porter, A. H. (1989). The lock-and-key hypothesis: Evolutionary and biosystematics interpretation of insect genitalia. Annu. Rev. Entomol. 34, 231–245. doi: 10.1146/annurev.en.34.010189.001311
Stamos, D. L. (2003). The species problem: Biological species, ontology and the metaphysics of biology. Lanham, MD: Lexington Books, 381.
Stevens, P. F. (1985). The genus concept in practice: But for what practice? Kew Bull. 40, 457–465. doi: 10.2307/4109605
Strayer, D. L. (2006). Challenges for freshwater inveretebrate conservation. J. North Am. Benthol. Soc. 25, 271–287. doi: 10.1899/0887-3593(2006)25[271:CFFIC]2.0.CO;2
TetraTech Inc (2004). Illinois benthic macroinvertebrate collection method comparison and stream condition index revision. Pasadena, CA: TetraTech Inc, 122.
Thomsen, M. S., Garcia, C., Bolam, S. G., Parker, R., Godbold, J. A., and Solan, M. (2017). Consequences of biodiversity loss diverge from expectation due to post-extinction compensatory responses. Sci. Rep. 7:43695. doi: 10.1038/srep43695
Vannote, R. L., Minshall, G. W., Cummins, K. W., Sedell, J. R., and Cushing, C. E. (1980). The river continuum concept. Can. J. Fish. Aqu. Sci. 37, 130–137. doi: 10.1139/f80-017
Wojtowicz, J. A. (1982). A review of the adults and larvae of the genus Pycnopsyche (Trichoptera: Limnephilidae) with revision of the Pycnopsyche scabripennis (Rambur) and Pycnopsyche lepida (Hagen) complexes (Publication No. 8225372). Doctoral dissertation. Knoxville, TN: University of Tennessee.
Zhou, X., Frandsden, P. B., Holzenthal, R. W., Beet, C. R., Bennett, K. R., Blahnik, R. J., et al. (2016). The Trichoptera barcode initiative: A strategy for generating a species-level Tree of Life. Philos. Trans. R. Soc. B 371:20160025. doi: 10.1098/rstb.2016.0025
Zhou, X., Robinson, J. L., Geraci, C. J., Parker, C. R., Flint, O. S., Etnier, D. A., et al. (2011). Accelerated construction of a regional DNA-barcode reference library: Caddisflies (Trichoptera) in the great smoky Mountains national park. J. North Am. Benthol. Soc. 30, 131–162. doi: 10.1899/10-010.1
Keywords: benthic, macroinvertebrate, explanatory reduction, taxonomic precision, aquatic insect, species conservation, incommensurability
Citation: Robinson JL (2023) Genus-level macroinvertebrate methods limit the conservation coverage afforded aquatic species. Front. Ecol. Evol. 11:1148516. doi: 10.3389/fevo.2023.1148516
Received: 20 January 2023; Accepted: 30 March 2023;
Published: 02 May 2023.
Edited by:
Shannon J. McCauley, University of Toronto Mississauga, CanadaReviewed by:
Silvia Quadroni, University of Insubria, ItalyJennifer Gleason, Agriculture and Agri-Food Canada (AAFC), Canada
Copyright © 2023 Robinson. This is an open-access article distributed under the terms of the Creative Commons Attribution License (CC BY). The use, distribution or reproduction in other forums is permitted, provided the original author(s) and the copyright owner(s) are credited and that the original publication in this journal is cited, in accordance with accepted academic practice. No use, distribution or reproduction is permitted which does not comply with these terms.
*Correspondence: Jason L. Robinson, jrob@illinois.edu