- 1Université Grenoble Alpes, INRAE, LESSEM, Saint-Martin-d'Hères, France
- 2IMBE, Avignon University, CNRS, IRD, Aix Marseille University, IUT, Avignon, France
Introduction: Semi-natural dry grasslands are among the species-richest and most diverse habitats in Europe especially in the Mediterranean Basin. They evolved with long-term severe environmental constraints, either biotic (e.g., grazing or mowing) or abiotic (e.g., dryness or oligotrophy). Their recovery after sever anthropogenic disturbance, such as cultivation is often very slow and incomplete. A deeper understanding of the processes driving the recovery would be of great benefit to better restore or conserve these grasslands. We used the filter model as a framework to assess the relative importance of dispersion, abiotic and biotic filters as drivers of this recovery.
Methods: We studied the medium-term dynamics of 37 Mediterranean dry grassland plant communities after an episode of cultivation in the plain of La Crau (Southern France) by two census: after 25 years of abandonment, and revisited 10 years later (35 years of abandonment). Our aims were to characterize the temporal medium-term dynamics of these communities, to measure their taxonomic resilience and to identify the drivers. We performed structural equation modeling to measure the importance of the various drivers, with cover of remnant dry grasslands surrounding each studied community as a proxy for the dispersion filter, soil pH and phosphorus content as proxies for the abiotic filter and cover of the most dominant species as a proxy for the biotic filter.
Results and Discussion: Our results show that former arable field vegetation do not recover after more than 40 years. The three filters drive the recovery of dry grassland vegetation: former arable fields that have lower phosphorus content (abiotic filter) have a lower cover of dominant species (biotic filter), a higher species richness and a higher similarity with remnant dry grassland plant communities, especially if the former arable fields are surrounded by remnant dry grasslands (dispersion filter).
1. Introduction
Impacts of land-use changes, notably abandonment of intensive agriculture, are a major topic in conservation biology (Stoate et al., 2009). The conversion from intensive cultivation to extensive grasslands often raises the issue of ecosystem ability to recover their pre-cultivation state of semi-natural species-rich grasslands (Cramer and Hobbs, 2012). This is an important topic as ecosystems recovering after cultivation will cover more than 4 billion ha in the next decades (including both grasslands and forests; Hurtt et al., 2011). Secondary succession is an essential concern in plant ecology (Cramer et al., 2008; Cramer and Hobbs, 2012), not only for theoretically-driven studies (Connell and Slatyer, 1977; Prach and Walker, 2011; Seabloom et al., 2020), but also for conservation and restoration perspectives (Luken, 1990; Walker et al., 2007; Suding, 2011; Rapson, 2023).
Species-rich grasslands are rarely resilient to severe anthropogenic disturbances (Helm et al., 2019; Nerlekar and Veldman, 2020; Buisson et al., 2022). They are composed with species that developed strategies to survive in moderately stressed and/or disturbed environment (i.e., xericity, grazing, mowing, combined or not) but not to recover after severe disturbance (Herben et al., 2018), such as intensive arable cultivation which involve soil disturbance as well as nutrient, herbicide and pesticide additions. In order to restore these degraded ecosystems it would be useful to better understand the drivers of recovery (i.e., their relative importance, their magnitude and directions). These drivers could then be used as levers that practitioners can modify.
Processes have been linked to theoretical models in order to summarize how communities assemble (Keddy, 1992; Lortie et al., 2004; Vellend, 2016). Most of these models are structured by filters that constrain community composition starting from a regional species pool. The first filter is dispersion: species have to be able to disperse and reach the community. This ability depends both on the landscape matrix and on the dispersability of species found in adjacent areas (Gibson and Brown, 1991; Pärtel and Zobel, 1999; Lindborg and Eriksson, 2004; Herault and Thoen, 2009). The second is the abiotic filter: species have to be able to germinate, grow and reproduce under given environmental conditions, and this ability depends on each species physiological capability (Kraft et al., 2015). The last one is the biotic filter: the occurrence and nature of biotic interactions alter the first two filters either by enhancing their mesh (sensu White and Jentsch, 2004), e.g., by increasing dispersion or by facilitating growth or establishment in limiting environmental conditions; or by reducing their mesh, e.g., by competitive exclusion (Bruno et al., 2003; Lortie et al., 2004). The aim of this paper is to disentangle the effect of dispersion, abiotic and biotic filters in driving the recovery of species-rich dry grassland plant communities after decades of intensive cultivation abandonment.
In this study, we measured diversity and composition recoveries in 35 and 45 year-old former arable fields in Mediterranean semi-natural species-rich dry grasslands and we have a hypothesis for each filter. The amount of remnant ecosystem in the surrounding of the former arable fields was used as a proxy of the dispersion filter as it provides a seed source for species occurring in the dry grassland. Our hypothesis was that its importance was rather low in driving plant communities. It is indeed widely recognized that most of the species from dry grasslands do not produce a permanent seed bank or seed that disperse on large distances (Graham and Hutchings, 1988; Buisson et al., 2006; Török et al., 2018; Arruda et al., 2021). Considering the size of the former arable fields (>100,000 m2) and the fact that most of the species do not colonize more than a few meters after decades (Buisson and Dutoit, 2004, 2006), these species are not expected to disperse to the whole area of each former arable fields, whatever the amount of remnant ecosystem in the surroundings. Two soil variables that were modified during former cultivation episode were used as proxies for the abiotic filter: one is related to fertility (phosphorus content) and the other is not directly related to soil fertility (soil pH). Phosphorus is one of the most important abiotic drivers of grassland community assembly (Yang et al., 2022). It is also known to remain in soil decades after fertilized cultivation, contrary to potassium or nitrogen (Smits et al., 2008; Jaunatre et al., 2016; Saatkamp et al., 2021). Ploughing which scraped the underlying calcareous conglomerate (Römermann et al., 2005), changed soil chemistry, increasing CaO, CaCO3 and pH. We thus selected pH as a proxy of these changes, as they may prevent the plant community to recover the composition of remnant dry grasslands [e.g., pH contributes to explain plant species distribution (Dubuis et al., 2013)]. Our hypotheses were that soil pH would influence plant community composition as soil pH is also a soil physical-parameter integrative of others changes than chemical fertility (i.e., increase of Ca and CaCO3 contents) while phosphorus content would decrease diversity through an increase in competition (Janssens et al., 1998; Palpurina et al., 2019). The biotic filter may depend on the abiotic characteristics (Belyea, 2004). During former arable field recovery, plant species have to face dense cover of ruderals or opportunistic species (Baeten et al., 2009; Öster et al., 2009). Hence, we used the dominant species cover as an indicator of potential competition in former arable fields and thus as a proxy of the biotic filter. Our hypothesis was that phosphorus content would drive the dominant species cover which itself would drive the plant community diversity. We also hypothesized that high composition recovery would only occur when species richness has recovered. The composition of the community could indeed be different from that of the remnant dry grasslands if the number of species is lower.
We monitored vegetation on 37 former arable field plots and 10 remnant dry grassland plots (in 2009 and in 2019), respectively 35 and 45 years after cultivation abandonment. We also characterized soil physico-chemical parameters on each plot and the landscape in the surroundings of each plot. In order to link the different drivers of plant community assembly we performed a structural equation modeling for each date were filter proxies were explanatory variables and vegetation diversity and composition were response variables.
2. Materials and methods
2.1. Site description
The La Crau area is a large dry grassland in southeastern France (ca. 10,000 ha; c. 43°33′ N, 4°52′ E; Figure 1) that has been shaped by (i) a Mediterranean climate with mean annual temperature of 15°C, a variable annual sum of precipitation between 400 and 600 mm concentrated in autumn, 4 months of summer drought, and more than 110 days with a >50 km.h-1 wind; (ii) 40 cm deep soil composed with about 50% of silicaceous stones overlaying a calcareous conglomerate layer, making the alluvial water table unreachable by the roots of plants and (iii) itinerant sheep that have grazed the area over the past several 1000 years (Devaux et al., 1983; Buisson and Dutoit, 2006). This has led to a unique and species-rich plant community composed mainly by a high number of annuals yet dominated in biomass by perennials, such as Brachypodium retusum Pers. and Thymus vulgaris L. Most of the species occurring in La Crau area are species adapted to extensive grazing, xeric and oligotrophic conditions. This community characterizes the remnant ecosystem in this study (i.e., that has been grazed for millennia but that has not undergone any disturbance due to cultivation) and will henceforth be referred to as dry grasslands. The 60,000 ha of original dry grasslands was characterized by relatively homogeneous vegetation composed only of different micro-patterns due to particular soil conditions and difference in sheep grazing regimes (Martin et al., 2022), before being fragmented by various severe anthropogenic disturbances, in particular, cultivation (Devaux et al., 1983; Buisson and Dutoit, 2006). Melon cultivation occurred in the La Crau area between 1971 and 1988. This cultivation implied soil disturbance, plant mortality and fertilizers application. Most of the fields have been subsequently abandoned; they now contain various plant communities dominated by Anisantha species (Römermann et al., 2005).
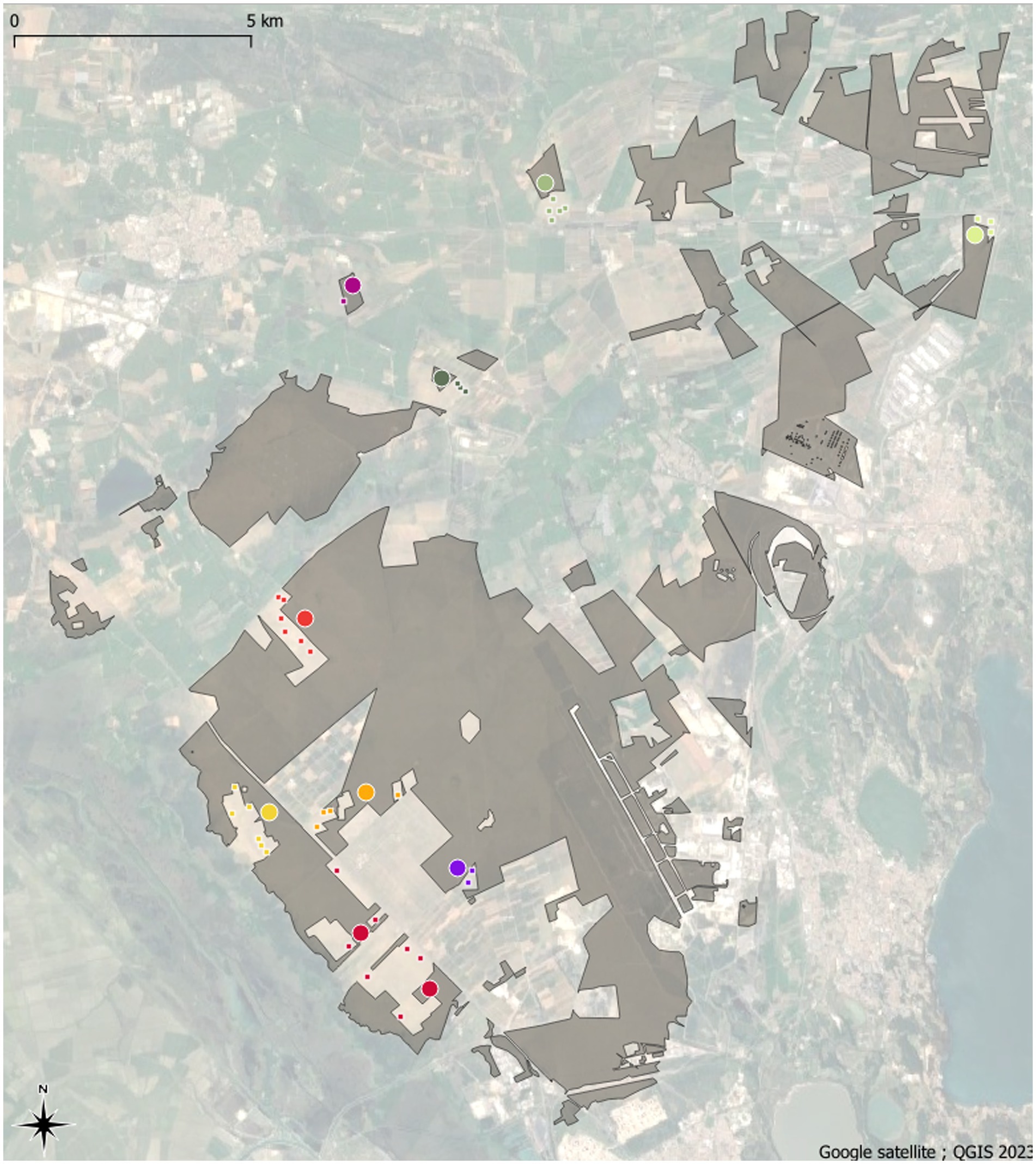
Figure 1. Map of the La Crau area in 2023. Remnant dry grasslands habitats are shown in grey. Colored large circles are remnant dry grasslands plots whereas colored small squares are former arable field plots. The different geographical areas are discriminated by different colors that are used in the ordination plot (Figure 4).
2.2. Site selection
Mapping based on aerial photographs enabled us to identify more than 220 areas that (i) appear as dry grasslands on the earliest photographs available (1947); (ii) show evidence of cultivation in later photographs (1955–1971–1975–1978–1979–1984–1988); and (iii) were abandoned between 1978 and 1988. After abandonment, all these areas were grazed again with the same intensity as the remnant dry grasslands. To avoid the introduction of an additional source of variability, we focused only on former open-field melon cultivation and not on large plastic tunnel fields that left less-disturbed trackways between the tunnels (Römermann et al., 2005). Thirty-seven of these Former Arable Fields, hereafter called FAF, were chosen and are spatially randomly distributed. The distance between any two sampled sites varies between 100 m and 20.9 km (Figure 1).
2.3. Vegetation sampling
Vegetation sampling was carried out at the center of each of the 37 FAF and of 10 dry grassland areas from all geographical zones of the La Crau area (Figure 1) in 2009 and in 2019, by the same team of botanists. On each FAF and remnant dry grassland areas, we monitored the vegetation in three 4 m2 quadrats at the tips of an 11 m side triangle. A Braun-Blanquet coefficient (Braun-Blanquet et al., 1952) was given to all plant species recorded and then transformed into percentage according to Guinochet (1973; 5: 87.5%, 4: 62.5%, 3: 37.5%, 2: 17.5%, 1: 5% and +: 0.1%). To avoid biases in abundance attributions, we transformed these percentages into relative percentage to the total vegetation cover. By doing so, we keep abundance hierarchy but we lever the potential overlap between species cover by constraining the sum of abundances into the total vegetation cover. We used species richness (alpha diversity) and similarity to the remnant dry grassland communities (composition) as response variables. The similarity to the remnant dry grassland communities was calculated as 1 – the minimum Bray-Curtis distance (Bray and Curtis, 1957) with the remnant dry grassland plot communities. We used the minimum Bray-Curtis distance instead of the average value in order to reduce the effect of the heterogeneity of remnant dry grassland vegetation. This similarity index with remnant dry grassland communities was thereafter referred as composition.
2.4. Explanatory variables
2.4.1. Remnant dry grassland cover
We used remnant dry grassland percent cover within a 900 m radius circle around the centre of each field as a proxy of dispersion potential. Map of remnant dry grassland areas was provided by the national reserve environmental managers (Réserve Nationale des Coussouls de Crau, Wolff et al., 2015). The 900 m radius was chosen based on preliminary analyses showing a tradeoff between (i) a smaller radius that would have caused the landscape factors to be especially influenced by the area of each field, and (ii) a larger radius that would have made the landscape factors of two fields close together almost identical. Cover were calculated using Geographical Information System software: Quantum GIS 2.18 “Las Palmas” (QGIS Development Team, 2016).
2.4.2. Soil analyses
We used soil analyses as a proxy of abiotic filter. For soil analyses, in 2009 and in 2019, a subsample of 70 g of soil was gathered in each of the three vegetation quadrats in the topsoil layer (0-10 cm) before being pooled to obtain one 210 g soil sample for each FAF. Samples were then sieved with a 2 mm mesh sieve. Analyses were carried out by the INRAE (Institut National de Recherche pour l’Agriculture l’Alimentation et l’Environnement). Phosphorus content (P2O5; Olsen et al., 1954) and water pH were measured according to the standard methods described in Baize (2018).
2.4.3. Dominance
We used the dominance as a proxy of the biotic filter. Dominance was calculated as the sum of abundances of the four most abundant species in each quadrat, which represented in average 45% of the sum of all species abundances (Appendix 1).
2.5. Statistical analyses
All the analyses were conducted using R 4.2.2 (R Core Team, 2022).
The alpha diversity of remnant dry grasslands and FAF were compared using a generalized linear mixed model performed with LME4 package (Bates et al., 2015). The kind of plant community (remnant dry grasslands or FAF) and monitoring years were included as fixed factors and the monitoring years and plot interaction as random factor to take into account the repeated nature of data.
In order to describe the vegetation of dry grasslands and FAF together, a Non-metric Multidimensional Scaling (NMDS) ordination was performed on the vegetation matrix to ordinate quadrats according to their plant community characteristics (Borcard et al., 2011) with VEGAN package (Oksanen et al., 2022). We performed a permutational analysis of variance using Bray-Curtis distance matrices (McArdle and Anderson, 2001), with the VEGAN::ADONIS2 function. This analysis measures the difference in the position of the centroid by groups, but may confound location and dispersion effects (Warton et al., 2012), we therefore also performed a test of homogeneity of group dispersion (Anderson, 2006) with the VEGAN::BETADISPER function.
In order to understand how the variation of landscape context, soil parameters and cover of dominant species influence the diversity and composition (i.e., similarity to remnant dry grassland areas) of FAF, we built a structural equation model (SEM) with PIECEWISESEM package (Lefcheck, 2016) for each monitoring year (2009 and 2019). This analysis, based on pathway dependence between variables, makes it possible to identify hypothesized relationships between selected variables (Lefcheck, 2016). We included dominance, alpha diversity and composition as response variables and remnant dry grassland cover, phosphorus content, pH and dominance as predictor variables for each plot (Figure 2). We fitted the SEMs with mixed models using the predictor variables as fixed factors and plots as random factor. For dominance, we used a linear model, with LMERTEST package (Kuznetsova et al., 2017), after square root transformation in order to fulfill parametric conditions. For alpha diversity we used a generalized linear model, with LME4 package (Bates et al., 2015), with poisson family and log link function. For composition, we used linear model, with LMERTEST package (Kuznetsova et al., 2017). Once fitted, we added missing pathways if they had been highlighted by Fisher d-separation tests (Shipley, 2013). We chose the best SEM model as the one with no significant missing pathways and with the lowest Akaike information criterion by at least 2 units (AIC; Akaike, 1974; Burnham and Anderson, 2004; Appendix 1).
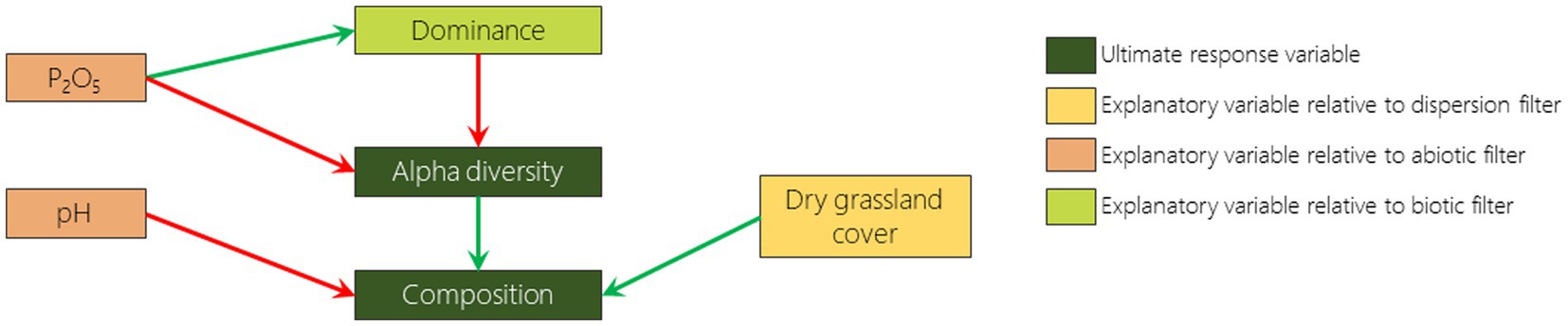
Figure 2. Pathway diagram representing the hypotheses tested in the structural equation modelling. Each arrow represents an expected effect, positive in green and negative in red.
3. Results
In total, 172 plant species were recorded: 156 in 222 FAF quadrats (37 × 3 × 2 quadrats) and 127 in 60 remnant dry grassland quadrats (10 × 3 × 2 quadrats). Despite the high number of species in common between remnant dry grasslands and FAF plots, mean species richness was significantly higher in remnant dry grassland areas than in FAFs (38.6 ± 0.9 vs. 31.1 ± 0.5; z = −2.49, p = 0.013; Figure 3). There was however neither an effect of monitoring year (z = 0.292; p = 0.771) nor an effect of interaction between year and location (z = −0.011; 0.992).
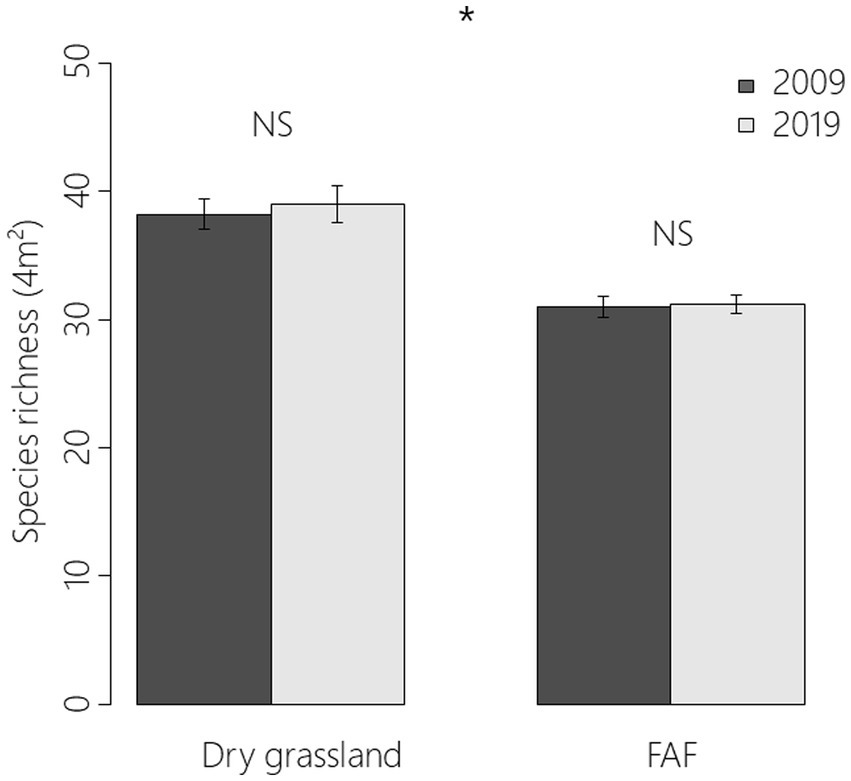
Figure 3. Means and standard errors of species-richness (4 m2) for 2009 and 2019 in remnant dry grasslands and former arable field plots (GLMM; z = −2.49, p = 0.013). “NS” indicated that the effect was not significant and “*” that it was with a p value between 0.05 and 0.01 according to a generalized linear mixed model.
There were no clear tendencies for the composition trajectories between 2009 and 2019 (Figure 4), although there is a significant year effect on the position of the centroid (F1 = 15.06; p < 0.001) with no difference of dispersion (F1 = 0.16; p = 0.686). On the contrary, remnant dry grasslands and FAF have a significantly different position of centroids (F1 = 24.25; p < 0.001) associated with a significant difference of dispersion (F1 = 88.98; p < 0.001). Some FAFs are indeed embedded within remnant dry grassland vegetation and characterized by species, such as Brachypodium retusum P. Beauv., Thymus vulgaris L., Medicago spp. and Trifolium spp. while others are still discriminated from the remnant dry grasslands and are characterized by the dominance of more ruderal type species for the Crau area, such as Lobularia maritima (L.) Desv., Anisantha madritensis (L.) Nevski or Clinopodium nepeta (L.) Kuntze.
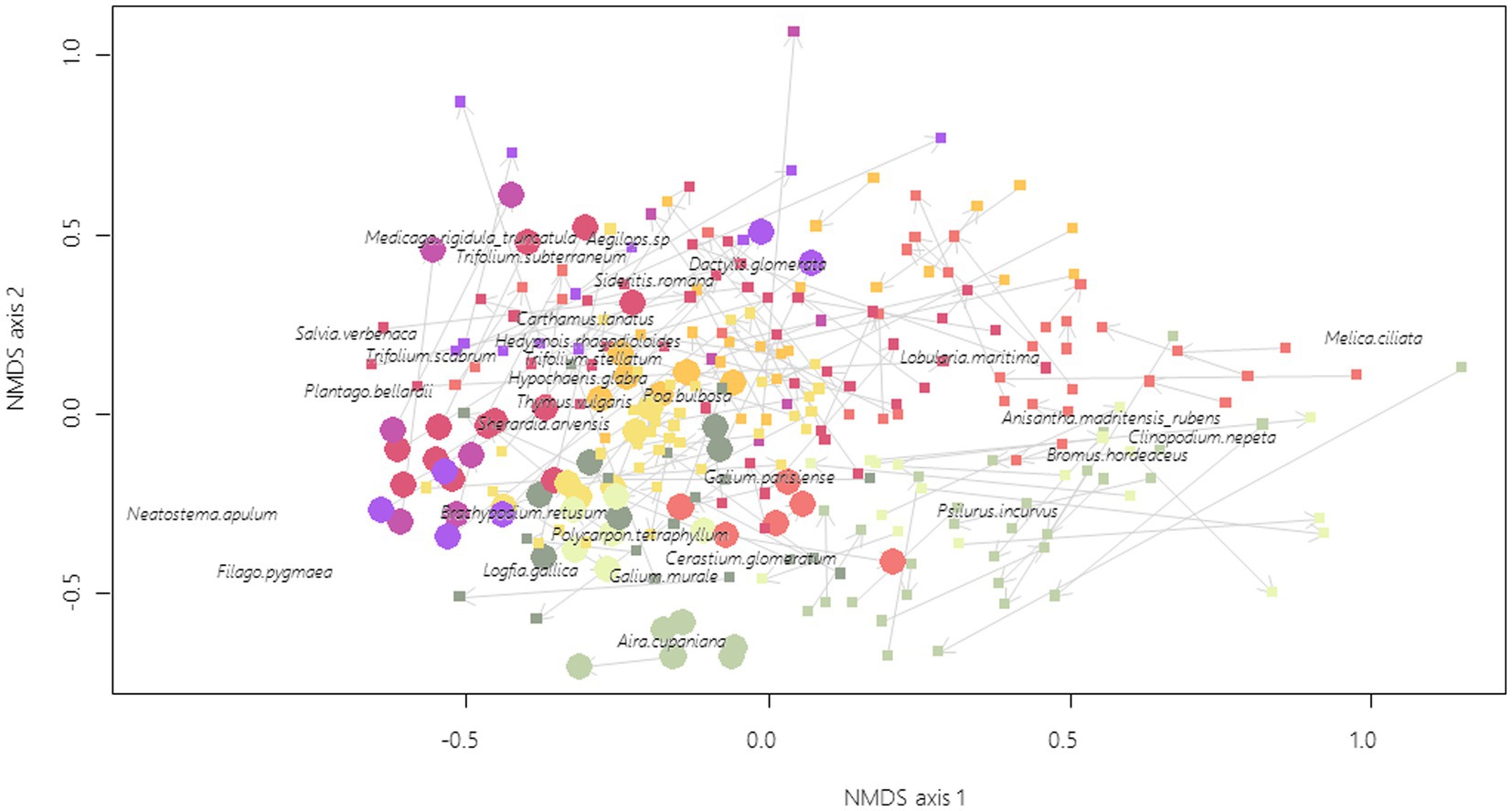
Figure 4. Remnant dry grasslands (filled circles, 10 sites × 3 plots × 2 years) and FAF (filled squares, n = 37 FAF × 3 plots × 2 years) ordination based on NMDS of plant community composition, final stress = 0.28. For clarity purpose, only the 30 most correlated species are shown (out of 172). Arrows link 2009 plots to 2019 plots. Points with the same colors are located within the same geographical zones (Figure 1).
According to the best fitted SEM, 2 out of 6 hypothesized relationships were confirmed for 2009 and 2019: the negative relationship between dominance and alpha diversity and the positive relationship between alpha diversity and composition (Figures 5, 6). The hypothesized negative relationship between pH and composition was confirmed in none of the models. Other relationships depended on the monitoring year. Phosphorus content was positively correlated (although non significantly) with dominance in 2009 and negatively correlated with alpha diversity in 2019. The remnant dry grassland cover had a positive relationship with composition in 2019 only. Surprisingly, a negative relationship that was not included in our a priori hypotheses was identified as missing pathways by Fisher d-separation tests (Shipley, 2013) between remnant dry grassland cover and dominance in 2009 (Figure 5).
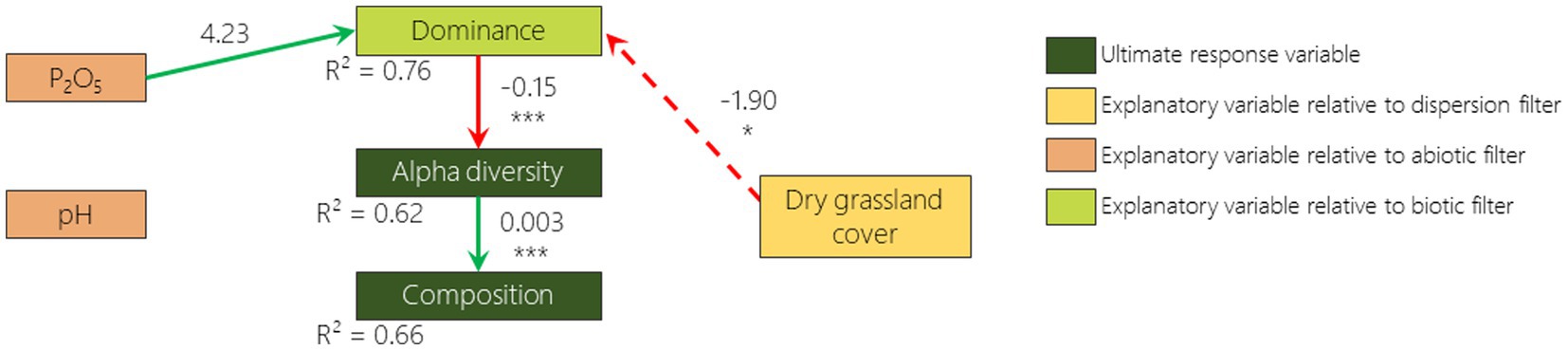
Figure 5. 2009 survey pathway diagram of the best fitted SEM illustrating how dominance, alpha diversity and composition are influenced by remnant dry grasslands cover, phosphorus content, pH and dominance. Boxes represent responses and predictor variables. Only included links in this model are drawn with arrows (green if the relationship is positive and red if negative). Dotted arrows represent links that were not included in our a priori hypotheses but were added after looking for missing pathways by Fisher d-separation tests (Shipley, 2013). Numbers on arrows are the slope estimates, accompanied or not with stars (*p < 0.05; **p < 0.01; ***p < 0.001).
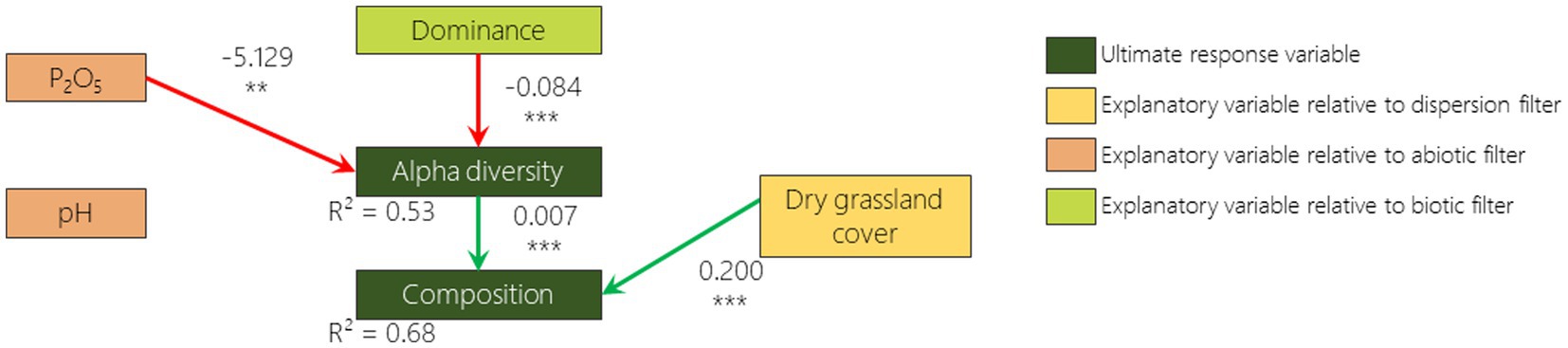
Figure 6. 2019 survey pathway diagram of the best fitted SEM illustrating how dominance, alpha diversity and composition are influenced by remnant dry grasslands cover, phosphorus content, pH, and dominance. Boxes represent responses and predictor variables. Only included links in this model are drawn with arrows (green if the relationship is positive and red if negative). Numbers on arrows are the slope estimates, accompanied or not with stars (*p < 0.05; **p < 0.01; ***p < 0.001).
4. Discussion
As reported in many studies that have focused on plant community resilience (Tomanek et al., 1955; Bonet and Pausas, 2004; Jaunatre et al., 2016; Helm et al., 2019; Isbell et al., 2019), even more than 30 years after disturbance, the species richness of the plant communities that colonized the disturbed sites are still lower than, and the composition is still considerably different from, those from the remnant dry grasslands. When we disentangle the different drivers of vegetation, we first notice that in 2009 phosphorus content and remnant dry grassland cover in the surroundings increase the abundance of the most dominant species in the FAF (e.g., Anisantha spp.). This dominance appears to reduce alpha diversity which itself drives the composition (i.e., a lower species richness leads to a lower similarity to the remnant dry grasslands). We then note that in 2019 while alpha diversity is reduced by dominance, it is also reduced by phosphorus content. Finally, we measured that while composition is driven by alpha diversity, it is also driven by the cover of dry grasslands in the surroundings (i.e., a higher cover of remnant dry grasslands in the surrounding leads to a higher similarity with these dry grasslands). It is therefore interesting to stress that the three filters play an important role in driving the vegetation recovery, but not with the same processes.
Surprisingly, the dispersion proxy (remnant dry grassland cover) switched from being negatively correlated with dominance in 2009 to being positively correlated with composition in 2019. A possible explanation of this result, is that when remnant dry grassland cover is low, cultivated area or former arable field covers are high. Differential dispersal limitation can cause wide species-specific deviations in the probability of finding a species from the landscape species pool in the community (Grace, 2001). Fields surrounded by cultivated areas or former arable fields may therefore exhibit a higher abundance of anemochorous ruderal species like Anisantha spp. that are known to exhibit higher dispersion abilities (Gaba et al., 2017). Ten years later, the presence of remnant dry grasslands in the vicinity promotes a composition more similar to the dry grassland. It is well known that presence of remnant patches in the vicinity promotes the dispersal of remnant dry grassland species to the community (Tansley and Adamson, 1925) and that this effect increases with time (Cook et al., 2005). We had not hypothesized a high importance of remnant patches as most of the species from dry grasslands do not disperse on large distances due to the low quantity of seeds from remnant communities in the seed bank and the seed rain (Graham and Hutchings, 1988; Buisson and Dutoit, 2004; Török et al., 2018; Arruda et al., 2021). Nevertheless, on the long term (over 30 years), long distance dispersion events (i.e., hundreds of meters) with low probability (i.e., which was not detected by sampling design) can occur (Higgins and Richardson, 1999) and may be favored by the presence of remnant patches in the vicinity. This is supported by the fact that FAF tend to differ from each other in patterns similar to those of the dry grasslands. Such patterns are also observed on secondary succession in abandoned mining sites in Central Europe (Jírová et al., 2012). However, the design of our study does not allow us to differentiate whether this pattern is a result of the dispersion or the abiotic filters. A more in depth spatial characterization of the remnant dry grasslands would be required to disentangle the effect of the species-pool it provides and the abiotic spatial organization. In order to thoroughly tackle the dispersion filter, numerous census of occurring species would be required as well as the correspondence with dispersal traits of these species (Lewis et al., 2016).
The pH does not appear to exert an effect on composition, which was not expected by our hypotheses. We expected higher changes due to the calcareous conglomerate that was broken during the cultivation phase (Buisson and Dutoit, 2004) and therefore lower composition recovery of plant community compared to remnant dry grasslands that contains some acidophilous species (Devaux et al., 1983). The range of variation of pH was however rather low: 6.9 and 7.2 being, respectively, the first and third quartiles. If relationship between pH and composition should occur, a higher range of variation would be require to detect it. Phosphorus content has first a positive relationship with dominance and then with species richness. Higher fertility leads to higher biomass of annual grasses (higher dominance) that should have increased competition (Michalet et al., 2023). Nutrient enrichment does not prevent remnant dry grassland species development in itself but enhances competition of more generalist species due to their dense cover (Huenneke et al., 1990; Buisson et al., 2015). Sowing typical remnant dry grassland species in FAF abiotic conditions or in remnant dry grassland abiotic conditions does not influence their establishment (Coiffait-Gombault et al., 2012). Our results show that species richness is limited both by biotic (dominance) and abiotic (phosphorus content) filters that interact together. This is in accordance with previous studies that show that dominance by more competitive and ruderal species on soil with higher nutrient contents can lead to a decline of remnant community species (Yurkonis and Meiners, 2004; Seabloom et al., 2020). Apart from the important feedback with the abiotic filter, the biotic filter is complicated to take into account. In our study, we only focused on the dominance of the above ground vegetation. The competition between plants may also be tackled by measurement of functional trait patterns, but inferring competition processes from these patterns may sometimes be misleading (Münkemüller et al., 2020). Besides intra guild competition, there is also below ground interactions (Kiær et al., 2013), facilitative interactions (Brooker et al., 2007), soil microfauna (Sun et al., 2023), microbial communities (Kaisermann et al., 2017), etc. We are currently able to measure the importance of these trophic network in the driving of ecosystem functioning (Martinez-Almoyna et al., 2022), but it is still very difficult to disentangle all the direct and indirect interactions that could lead to the favoring or (disfavoring) of specific set of species.
We studied the recovery on the vegetation composition having as reference, remnant patches of remnant dry grassland vegetation (i.e., extensively grazed vegetation). Considering the vegetation dynamics that we measured after several decades of recovery, it is unlikely that these plant communities will converge toward remnant dry grassland vegetation on the medium term. As was shown in previous studies, dozens of decades after abandonment, the composition is still different from the remnant ecosystems (Jaunatre et al., 2016, for species-rich dry grassland; Nerlekar and Veldman, 2020 for various grasslands). For such non-resilient species-rich grasslands, reconstructive restoration (i.e., ending degradation, active remediation of substrate condition and reintroduction of major proportion of target biota sensu Atkinson and Bonser, 2020) would be needed to ensure a potential complete recovery of plant communities. Our result confirm the fact that the restoration of abiotic conditions prior to forcing the dispersion of target species would be a key for successful restoration.
Our results show that FAF vegetation did not recover after more than 30 years. The three filters drive the recovery of remnant dry grassland vegetation: FAF that have lower phosphorus content (abiotic filter) have a lower cover of dominant species (biotic filter), a higher species richness and a higher similarity with remnant dry grassland plant communities, especially if the FAF are surrounded by remnant dry grasslands (dispersion filter). Based on these results we therefore recommend that the restoration of species-rich semi-natural remnant dry grasslands is primarily focused on the recovery of suitable abiotic conditions [i.e., by decreasing fertility, for instance with expensive topsoil removal (Hölzel and Otte, 2003; Jaunatre et al., 2014), repeated carbon addition (Corbin and D’Antonio, 2004; Ossanna and Gornish, 2023) or long-term phosphorus mining (Schelfhout et al., 2015)] and on increasing propagule pressure of the typical remnant dry grassland species in a second phase. In order to be able to use SEM, we only used a few variables that we attributed to the three filters. These variables were chosen regarding the specific characteristics of the degradation and of the remnant ecosystem. Our study is a piece into the comprehension of the relative importance of each filters that would benefit from both similar analyses in other ecosystems but also more in depth measure of how the propagule pressure and the abiotic characteristics drive interaction between species and therefore plant community recovery.
Data availability statement
The original contributions presented in the study are included in the article/Supplementary material, further inquiries can be directed to the corresponding author. Code and data are also publicly available at https://forgemia.inra.fr/renaud.jaunatre/friches_frontiers.
Author contributions
RJ, EB, and TD conceived the design of this study. RJ performed the data analysis and wrote the first draft of the manuscript. All authors contributed to floristic surveys and approved the final version.
Funding
This study was supported by the CDC Biodiversité, CEN PACA, the Réserve Naturelle des Coussouls de Crau, the Conseil Régional de Provence Alpes Côtes d’Azur, and CNRS RTP Ingecotech funding.
Acknowledgments
The authors would like to thank Jennifer Matwyschuk and Daniel Pavon (IMBE) for help with fieldwork.
Conflict of interest
The authors declare that the research was conducted in the absence of any commercial or financial relationships that could be construed as a potential conflict of interest.
Publisher’s note
All claims expressed in this article are solely those of the authors and do not necessarily represent those of their affiliated organizations, or those of the publisher, the editors and the reviewers. Any product that may be evaluated in this article, or claim that may be made by its manufacturer, is not guaranteed or endorsed by the publisher.
Supplementary material
The Supplementary material for this article can be found online at: https://www.frontiersin.org/articles/10.3389/fevo.2023.1148226/full#supplementary-material
References
Akaike, H. (1974). A new look at the statistical model identification. IEEE Trans. Automat. Contr. 19, 716–723. doi: 10.1109/TAC.1974.1100705
Anderson, M. J. (2006). Distance-based tests for homogeneity of multivariate dispersions. Biometrics 62, 245–253. doi: 10.1111/j.1541-0420.2005.00440.x
Arruda, A. J., Junqueira, P. A., Rodrigues, H. T. S., Yvanez, F., Poschlod, P., Silveira, F. A. O., et al. (2021). Limited seed dispersability in a megadiverse OCBIL grassland. Biol. J. Linn. Soc. 133, 499–511. doi: 10.1093/biolinnean/blaa145
Atkinson, J., and Bonser, S. P. (2020). “Active” and “passive” ecological restoration strategies in meta-analysis. Restor. Ecol. 28, 1032–1035. doi: 10.1111/rec.13229
Baeten, L., Jacquemyn, H., Van Calster, H., Van Beek, E., Devlaeminck, R., Verheyen, K., et al. (2009). Low recruitment across life stages partly accounts for the slow colonization of forest herbs. J. Ecol. 97, 109–117. doi: 10.1111/j.1365-2745.2008.01455.x
Baize, D. (2018). Guide des analyses en pédologie. 3e éd. revue et augmentée Versailles: Éditions Quae.
Bates, D., Mächler, M., Bolker, B., and Walker, S. (2015). Fitting linear mixed-effects models using lme4. J. Stat. Soft. 67, 1–48. doi: 10.18637/jss.v067.i01
Belyea, L. R. (2004). “Beyond ecological filters: feedback networks in the assembly and restoration of community structure” in Assembly rules and restoration ecology: bridging the gap between theory and practice. eds. V. M. Temperton, R. J. Hobbs, T. Nuttle, and S. Halle, (Washington, DC: Island Press), 115–131.
Bonet, A., and Pausas, J. G. (2004). Species richness and cover along a 60-year chronosequence in old-fields of southeastern Spain. Plant Ecol. 174, 257–270. doi: 10.1023/B:VEGE.0000049106.96330.9c
Braun-Blanquet, J., Roussine, N., and Nègre, R. (1952). Groupements végétaux de la France méditerranéenne. CNRS. Montpellier.
Bray, J. R., and Curtis, J. T. (1957). An ordination of the upland forest communities of southern Wisconsin. Ecol. Monogr. 27, 325–349. doi: 10.2307/1942268
Brooker, R. W., Maestre, F. T., Callaway, R. M., Lortie, C. L., Cavieres, L. A., Kunstler, G., et al. (2007). Facilitation in plant communities: the past, the present, and the future. J. Ecol. 96, 18–34. doi: 10.1111/j.1365-2745.2007.01295.x
Bruno, J. F., Stachowicz, J. J., and Bertness, M. D. (2003). Inclusion of facilitation into ecological theory. Trends Ecol. Evol. 18, 119–125. doi: 10.1016/S0169-5347(02)00045-9
Buisson, E., Archibald, S., Fidelis, A., and Suding, K. N. (2022). Ancient grasslands guide ambitious goals in grassland restoration. Science 377, 594–598. doi: 10.1126/science.abo4605
Buisson, E., Corcket, E., and Dutoit, T. (2015). Limiting processes for perennial plant reintroduction to restore dry grasslands: perennial plant reintroduction in dry grasslands. Restor. Ecol. 23, 947–954. doi: 10.1111/rec.12255
Buisson, E., and Dutoit, T. (2004). Colonisation by native species of abandoned farmland adjacent to a remnant patch of Mediterranean steppe. Plant Ecol. 174, 371–384. doi: 10.1007/BF03543067
Buisson, E., and Dutoit, T. (2006). Creation of the natural reserve of La Crau: implications for the creation and management of protected areas. J. Environ. Manag. 80, 318–326. doi: 10.1016/j.jenvman.2005.09.013
Buisson, E., Holl, K. D., Anderson, S., Corcket, E., Hayes, G. F., Torre, F., et al. (2006). Effect of seed source, topsoil removal, and plant neighbor removal on restoring California coastal prairies. Restor. Ecol. 14, 569–577. doi: 10.1111/j.1526-100X.2006.00168.x
Burnham, K. P., and Anderson, D. R. eds. (2004). Model selection and multimodel inference. New York, NY: Springer.
Coiffait-Gombault, C., Buisson, E., and Dutoit, T. (2012). Using a two-phase sowing approach in restoration: sowing foundation species to restore, and subordinate species to evaluate restoration success. Appl. Veg. Sci. 15, 277–289. doi: 10.1111/j.1654-109X.2012.01182.x
Connell, J. H., and Slatyer, R. O. (1977). Mechanisms of succession in natural communities and their role in community stability and organization. Am. Nat. 111, 1119–1144. doi: 10.1086/283241
Cook, W. M., Yao, J., Foster, B. L., Holt, R. D., and Patrick, L. B. (2005). Secondary succession in an experimentally fragmented landscape: community patterns across space and time. Ecology 86, 1267–1279. doi: 10.1890/04-0320
Corbin, J. D., and D’Antonio, C. M. (2004). Can carbon addition increase competitiveness of native grasses? A case study from California. Restor. Ecol. 12, 36–43. doi: 10.1111/j.1061-2971.2004.00299.x
Cramer, V. A., and Hobbs, R. (2012). Old fields: dynamics and restoration of abandoned farmland. Washington, DC: Island Press.
Cramer, V., Hobbs, R., and Standish, R. (2008). What’s new about old fields? Land abandonment and ecosystem assembly. Trends Ecol. Evol. 23, 104–112. doi: 10.1016/j.tree.2007.10.005
Devaux, J. P., Archiloque, A., Borel, L., Bourrelly, M., and Louis-Palluel, J. (1983). Notice de la carte phyto-écologique de la Crau (Bouches du Rhône). Biologie et écologie méditerranéenne 10, 5–54.
Dubuis, A., Rossier, L., Pottier, J., Pellissier, L., Vittoz, P., and Guisan, A. (2013). Predicting current and future spatial community patterns of plant functional traits. Ecography 36, 1158–1168. doi: 10.1111/j.1600-0587.2013.00237.x
Gaba, S., Perronne, R., Fried, G., Gardarin, A., Bretagnolle, F., Biju-Duval, L., et al. (2017). Response and effect traits of arable weeds in agro-ecosystems: a review of current knowledge. Weed Res. 57, 123–147. doi: 10.1111/wre.12245
Gibson, C. W. D., and Brown, V. K. (1991). The nature and rate of development of calcareous grassland in southern Britain. Biol. Conserv. 58, 297–316. doi: 10.1016/0006-3207(91)90097-S
Grace, J. B. (2001). Difficulties with estimating and interpreting species pools and the implications for understanding patterns of diversity. Folia Geobot. 36, 71–83. doi: 10.1007/BF02803140
Graham, D. J., and Hutchings, M. J. (1988). Estimation of the seed bank of a chalk grassland ley established on former arable land. J. Appl. Ecol. 25, 241–252. doi: 10.2307/2403622
Helm, J., Dutoit, T., Saatkamp, A., Bucher, S. F., Leiterer, M., and Römermann, C. (2019). Recovery of Mediterranean steppe vegetation after cultivation: legacy effects on plant composition, soil properties and functional traits. Appl. Veg. Sci. 22, 71–84. doi: 10.1111/avsc.12415
Herault, B., and Thoen, D. (2009). How habitat area, local and regional factors shape plant assemblages in isolated closed depressions. Acta Oecol. 35, 385–392. doi: 10.1016/j.actao.2009.02.002
Herben, T., Klimešová, J., and Chytrý, M. (2018). Philip Grime’s fourth corner: are there plant species adapted to high disturbance and low productivity? Oikos 127, 1125–1131. doi: 10.1111/oik.05090
Higgins, S. I., and Richardson, D. M. (1999). Predicting plant migration rates in a changing world: the role of long-distance dispersal. Am. Nat. 153, 464–475. doi: 10.1086/303193
Hölzel, N., and Otte, A. (2003). Restoration of a species-rich flood meadow by topsoil removal and diaspore transfer with plant material. Appl. Veg. Sci. 6, 131–140. doi: 10.1111/j.1654-109X.2003.tb00573.x
Huenneke, L. F., Hamburg, S. P., Koide, R., Mooney, H. A., and Vitousek, P. M. (1990). Effects of soil resources on plant invasion and community structure in Californian serpentine grassland. Ecology 71, 478–491. doi: 10.2307/1940302
Hurtt, G. C., Chini, L. P., Frolking, S., Betts, R. A., Feddema, J., Fischer, G., et al. (2011). Harmonization of land-use scenarios for the period 1500–2100: 600 years of global gridded annual land-use transitions, wood harvest, and resulting secondary lands. Clim. Chang. 109, 117–161. doi: 10.1007/s10584-011-0153-2
Isbell, F., Tilman, D., Reich, P. B., and Clark, A. T. (2019). Deficits of biodiversity and productivity linger a century after agricultural abandonment. Nat. Ecol. Evol. 3, 1533–1538. doi: 10.1038/s41559-019-1012-1
Janssens, F., Peeters, A., Tallowin, J. R. B., Bakker, J. P., Bekker, R. M., Fillat, F., et al. (1998). Relationship between soil chemical factors and grassland diversity. Plant Soil 202, 69–78. doi: 10.1023/A:1004389614865
Jaunatre, R., Buisson, E., and Dutoit, T. (2014). Topsoil removal improves various restoration treatments of a Mediterranean steppe (La Crau, Southeast France). Appl. Veg. Sci. 17, 236–245. doi: 10.1111/avsc.12063
Jaunatre, R., Fonvieille, N., Spiegelberger, T., Buisson, E., and Dutoit, T. (2016). Recovery of arbuscular mycorrhizal fungi root colonization after severe anthropogenic disturbance: four species assessed in old-growth Mediterranean grassland. Folia Geobot. 51, 319–332. doi: 10.1007/s12224-016-9254-z
Jírová, A., Klaudisová, A., and Prach, K. (2012). Spontaneous restoration of target vegetation in old-fields in a central European landscape: a repeated analysis after three decades. Appl. Veg. Sci. 15, 245–252. doi: 10.1111/j.1654-109X.2011.01165.x
Kaisermann, A., de Vries, F. T., Griffiths, R. I., and Bardgett, R. D. (2017). Legacy effects of drought on plant–soil feedbacks and plant–plant interactions. New Phytol. 215, 1413–1424. doi: 10.1111/nph.14661
Keddy, P. A. (1992). Assembly and response rules: two goals for predictive community ecology. J. Veg. Sci. 3, 157–164. doi: 10.2307/3235676
Kiær, L. P., Weisbach, A. N., and Weiner, J. (2013). Root and shoot competition: a meta-analysis. J. Ecol. 101, 1298–1312. doi: 10.1111/1365-2745.12129
Kraft, N. J. B., Adler, P. B., Godoy, O., James, E. C., Fuller, S., and Levine, J. M. (2015). Community assembly, coexistence and the environmental filtering metaphor. Funct. Ecol. 29, 592–599. doi: 10.1111/1365-2435.12345
Kuznetsova, A., Brockhoff, P. B., and Christensen, R. H. B. (2017). lmerTest package: tests in linear mixed effects models. J. Stat. Soft. 82, 1–26. doi: 10.18637/jss.v082.i13
Lefcheck, J. S. (2016). piecewiseSEM: piecewise structural equation modelling in R for ecology, evolution, and systematics. Methods Ecol. Evol. 7, 573–579. doi: 10.1111/2041-210X.12512
Lewis, R. J., Szava-Kovats, R., and Pärtel, M. (2016). Estimating dark diversity and species pools: an empirical assessment of two methods. Methods Ecol. Evol. 7, 104–113. doi: 10.1111/2041-210X.12443
Lindborg, R., and Eriksson, O. (2004). Historical landscape connectivity affects present plant species diversity. Ecology 85, 1840–1845. doi: 10.1890/04-0367
Lortie, C. J., Brooker, R. W., Choler, P., Kikvidze, Z., Michalet, R., Pugnaire, F. I., et al. (2004). Rethinking plant community theory. Oikos 107, 433–438. doi: 10.1111/j.0030-1299.2004.13250.x
Martin, G., Courtial, A., Génin, A., Ramone, H., and Dutoit, T. (2022). Why grazing and soil matter for dry grassland diversity: new insights from multigroup structural equation modeling of micro-patterns. Front. Ecol. Evol. 10:879060. doi: 10.3389/fevo.2022.879060
Martinez-Almoyna, C., Saillard, A., Zinger, L., Lionnet, C., Arnoldi, C., Foulquier, A., et al. (2022). Differential effects of soil trophic networks on microbial decomposition activity in mountain ecosystems. Soil Biol. Biochem. 172:108771. doi: 10.1016/j.soilbio.2022.108771
McArdle, B. H., and Anderson, M. J. (2001). Fitting multivariate models to community data: a comment on distance-based redundancy analysis. Ecology 82, 290–297. doi: 10.1890/0012-9658(2001)082[0290:FMMTCD]2.0.CO;2
Michalet, R., Delerue, F., and Liancourt, P. (2023). Disentangling the effects of biomass and productivity in plant competition. Ecology 104:e3851. doi: 10.1002/ecy.3851
Münkemüller, T., Gallien, L., Pollock, L. J., Barros, C., Carboni, M., Chalmandrier, L., et al. (2020). Dos and don’ts when inferring assembly rules from diversity patterns. Glob. Ecol. Biogeogr. 29, 1212–1229. doi: 10.1111/geb.13098
Nerlekar, A. N., and Veldman, J. W. (2020). High plant diversity and slow assembly of old-growth grasslands. Proc. Natl. Acad. Sci. U. S. A. 117, 18550–18556. doi: 10.1073/pnas.1922266117
Oksanen, J., Simpson, G. L., Blanchet, F. G., Kindt, R., Legendre, P., Minchin, P. R., et al. (2022). Vegan: community ecology package. Available at: https://CRAN.R-project.org/package=vegan ()
Olsen, S. R., Cole, C. V., Watanabe, F. S., and Dean, L. A. (1954). Estimation of available phosphorus in soils by extraction with sodium bicarbonate. United States Department of Agriculture, 1–18.
Ossanna, L. Q. R., and Gornish, E. S. (2023). Efficacy of labile carbon addition to reduce fast-growing, invasive non-native plants: a review and meta-analysis. J. Appl. Ecol. 60, 218–228. doi: 10.1111/1365-2664.14324
Öster, M., Ask, K., Römermann, C., Tackenberg, O., and Eriksson, O. (2009). Plant colonization of ex-arable fields from adjacent species-rich grasslands: the importance of dispersal vs. recruitment ability. Agric. Ecosyst. Environ. 130, 93–99. doi: 10.1016/j.agee.2008.12.005
Palpurina, S., Chytrý, M., Hölzel, N., Tichý, L., Wagner, V., Horsák, M., et al. (2019). The type of nutrient limitation affects the plant species richness–productivity relationship: evidence from dry grasslands across Eurasia. J. Ecol. 107, 1038–1050. doi: 10.1111/1365-2745.13084
Pärtel, M., and Zobel, M. (1999). Small-scale plant species richness in calcareous grasslands determined by the species pool, community age and shoot density. Ecography 22, 153–159. doi: 10.1111/j.1600-0587.1999.tb00463.x
Prach, K., and Walker, L. R. (2011). Four opportunities for studies of ecological succession. Trends Ecol. Evol. 26, 119–123. doi: 10.1016/j.tree.2010.12.007
QGIS Development Team (2016). QGIS geographic information system. Available at: https://www.qgis.org
R Core Team (2022). R: A language and environment for statistical computing. Available at: https://www.R-project.org/
Rapson, G. L. (2023). Tertiary succession: a new concept to help vegetation restoration. Restor. Ecol. 31:e13683. doi: 10.1111/rec.13683
Römermann, C., Dutoit, T., Poschlod, P., and Buisson, E. (2005). Influence of former cultivation on the unique Mediterranean steppe of France and consequences for conservation management. Biol. Conserv. 121, 21–33. doi: 10.1016/j.biocon.2004.04.006
Saatkamp, A., Henry, F., and Dutoit, T. (2021). Romans shape today’s vegetation and soils: two millennia of land-use legacy dynamics in Mediterranean grasslands. Ecosystems 24, 1268–1280. doi: 10.1007/s10021-020-00581-w
Schelfhout, S., De Schrijver, A., De Bolle, S., De Gelder, L., Demey, A., Du Pré, T., et al. (2015). Phosphorus mining for ecological restoration on former agricultural land. Restor. Ecol. 23, 842–851. doi: 10.1111/rec.12264
Seabloom, E. W., Borer, E. T., and Tilman, D. (2020). Grassland ecosystem recovery after soil disturbance depends on nutrient supply rate. Ecol. Lett. 23, 1756–1765. doi: 10.1111/ele.13591
Shipley, B. (2013). The AIC model selection method applied to path analytic models compared using a d-separation test. Ecology 94, 560–564. doi: 10.1890/12-0976.1
Smits, N. A. C., Willems, J. H., and Bobbink, R. (2008). Long-term after-effects of fertilisation on the restoration of calcareous grasslands. Appl. Veg. Sci. 11, 279–286. doi: 10.3170/2008-7-18417
Stoate, C., Báldi, A., Beja, P., Boatman, N. D., Herzon, I., van Doorn, A., et al. (2009). Ecological impacts of early 21st century agricultural change in Europe – a review. J. Environ. Manag. 91, 22–46. doi: 10.1016/j.jenvman.2009.07.005
Suding, K. N. (2011). Toward an era of restoration in ecology: successes, failures, and opportunities ahead. Annu. Rev. Ecol. Evol. Syst. 42, 465–487. doi: 10.1146/annurev-ecolsys-102710-145115
Sun, D., Mudrák, O., Ardestani, M. M., and Frouz, J. (2023). Unravelling the role of soil microflora from micro and macro aggregates in plant growth during primary and secondary successions. Catena 220:106655. doi: 10.1016/j.catena.2022.106655
Tansley, A. G., and Adamson, R. S. (1925). Studies of the vegetation of the English chalk: III. The chalk grasslands of Hampshire-Sussex border. J. Ecol. 13, 177–223. doi: 10.2307/2255283
Tomanek, G. W., Albertson, F. W., and Riegel, A. (1955). Natural revegetation on a field abandoned for thirty-three years in Central Kansas. Ecology 36, 407–412. doi: 10.2307/1929575
Török, P., Helm, A., Kiehl, K., Buisson, E., and Valkó, O. (2018). Beyond the species pool: modification of species dispersal, establishment, and assembly by habitat restoration. Restor. Ecol. 26, S65–S72. doi: 10.1111/rec.12825
Walker, L. R., Walker, J., and Hobbs, R. J. (2007). Linking restoration and ecological succession. New York, NY: Springer.
Warton, D. I., Wright, S. T., and Wang, Y. (2012). Distance-based multivariate analyses confound location and dispersion effects. Methods Ecol. Evol. 3, 89–101. doi: 10.1111/j.2041-210X.2011.00127.x
White, P. S., and Jentsch, A. (2004). “Disturbance, succession and community assembly in terrestrial plant communities” in Assembly rules and restoration ecology: bridging the gap between theory and practice (Washington, DC: Island Press), 342–346.
Wolff, A., Tatin, L., and Sauguet, F. (2015). La Réserve Naturelle des Coussouls de Crau. Plan de gestion 2015–2024. Section A: Diagnostic et vision. CEN-PACA, Saint-Martin de Crau, France. Available at: https://www.paca.developpement-durable.gouv.fr/IMG/pdf/1_plan_gestion_RNCC_2015-2024_section_A.pdf.
Yang, J., Su, P., Zhou, Z., Shi, R., and Ding, X. (2022). Environmental filtering rather than dispersal limitation dominated plant community assembly in the Zoige plateau. Ecol. Evol. 12:e9117. doi: 10.1002/ece3.9117
Keywords: old field, secondary succession, resilience, plant community, dry grassland
Citation: Jaunatre R, Buisson E, Leborgne E and Dutoit T (2023) Soil fertility and landscape surrounding former arable fields drive the ecological resilience of Mediterranean dry grassland plant communities. Front. Ecol. Evol. 11:1148226. doi: 10.3389/fevo.2023.1148226
Edited by:
Orsolya Valkó, Hungarian Academy of Sciences, HungaryReviewed by:
José Antonio Molina, Complutense University of Madrid, SpainPanayiotis G. Dimitrakopoulos, University of the Aegean, Greece
Florian Delerue, Institut Polytechnique de Bordeaux, France
Copyright © 2023 Jaunatre, Buisson, Leborgne and Dutoit. This is an open-access article distributed under the terms of the Creative Commons Attribution License (CC BY). The use, distribution or reproduction in other forums is permitted, provided the original author(s) and the copyright owner(s) are credited and that the original publication in this journal is cited, in accordance with accepted academic practice. No use, distribution or reproduction is permitted which does not comply with these terms.
*Correspondence: Renaud Jaunatre, renaud.jaunatre@inrae.fr