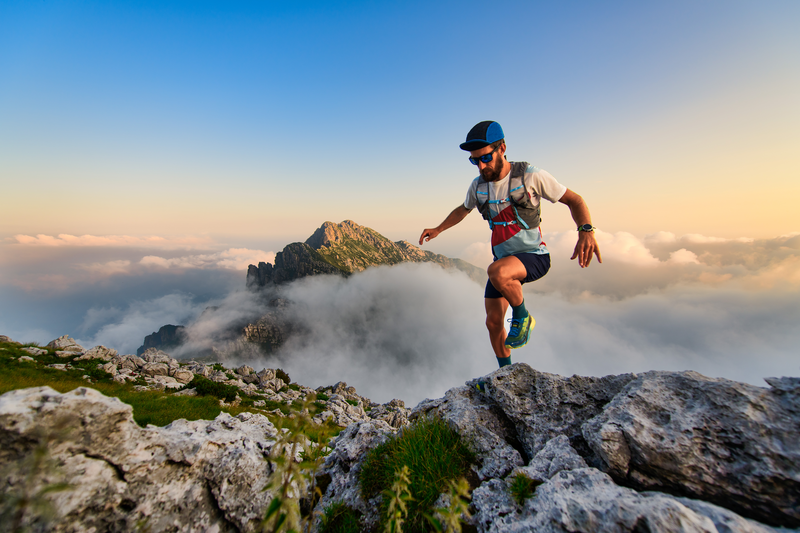
94% of researchers rate our articles as excellent or good
Learn more about the work of our research integrity team to safeguard the quality of each article we publish.
Find out more
ORIGINAL RESEARCH article
Front. Ecol. Evol. , 09 March 2023
Sec. Conservation and Restoration Ecology
Volume 11 - 2023 | https://doi.org/10.3389/fevo.2023.1145979
This article is part of the Research Topic Recent Advances in Restoration, Preservation and Eco-Morphophysiology of Plants Under Integrated Management Approaches and Current Climate Change View all 11 articles
Iodine deficiency disorder (IDDs) is one of the most prevailing and common health issues in mountainous communities. An effective way to control the prevalence and emergence of IDDs in remote areas is to use iodized salt. However, recent studies indicated that iodized salt is mostly lost during the cooking process. The current study of iodine biofortification differed from the previous studies in two main aspects: it involved exogenous organic iodine (OI), and inorganic iodine such as potassium iodide (KI), added in the amended soils, which previous studies did not consider. Moreover, the translocation, transformation, and distribution of iodine from soil to plants are poorly understood in amended soil. Thus, identifying an effective management option to enhance iodine (I) bioavailability in nutrient-deficient soils is currently a significant challenge. Therefore, a greenhouse study was conducted to investigate the effects of organic and inorganic soil amendments on the uptake of different iodine sources in coriander crops. Results showed that applying an inorganic iodine source significantly enhanced the iodine edible part of the crop compared to the control (p < 0.05). The application of soil amendments relatively improved iodine uptake by the coriander crop compared to the control. The highest iodine was found in crop tissues grown in wood ash-amended soil supplemented with KI (291.97 μg kg−1). The KI uptake was significantly higher than the OI (p < 0.05). Compared to OI, a higher translocation factor (0.96) and distribution coefficient (3.51) were found for plants treated with KI. Thus, this study indicates that a suitable soil amendment can be a better option for iodine biofortification and that it can serve as an alternative to iodized salt in preventing IDDs.
Iodine (I) is an essential constituent of thyroid hormones and critical for the metabolism and normal functioning of the human body (Ujowundu et al., 2011). Iodine deficiency disorders (IDDs) are prevalent in many countries worldwide, affecting between 80 million to 2 billion people around the globe (Dasgupta et al., 2008; Szybinski et al., 2010). The deficiency symptoms can lead to endemic goiter, cretinism, and fetal abnormalities (Smoleń et al., 2016; Ligowe et al., 2021). The recommended daily allowance (RDA) of iodine for adults is µg d-1 with a tolerable upper limit of µg d-1 (WHO, 2007a,b; Patrick, 2008). I deficiency has increased more than fourfold over the past few years (Szybinski et al., 2010; Verduzco-Gallo et al., 2014; Lawson et al., 2015; Zia et al., 2015).
The most common method for mitigating I deficiency is iodized salt, a common supplement used to eliminate iodine deficiency (Vejbjerg et al., 2007). Despite the broad application of iodized salt, pathological symptoms related to inadequate I intake are diagnosed in about 38% of the world’s population (De Benoist et al., 2008; White and Broadley, 2009). This is because the concentration of I in salt is highly variable and dependent on the level of iodization (Kalimbira et al., 2005). Losses of up to 20% can occur during production, processing, packaging, and transportation while cooking processes can contribute to an additional loss of 20% (Winger et al., 2008; Lawson et al., 2015). Adults are also advised to lower their daily salt intake to reduce risks of kidney damage and cardiovascular diseases (Piccone, 2011; Zimmermann, 2011).
One way to counter IDD is the optimization of I into the edible portion of crops through different crop and soil management strategies (Chilimba et al., 2012; Manzeke et al., 2014). Among these management approaches, biofortification emerged as a practical crop-based approach dealing effectively with mineral malnutrition by enriching crops and food products with bioavailable micronutrients (Lawson et al., 2015). Iodine uptake via edible crops has been reported as a cost-effective measure to combat IDDs in humans (Zhu et al., 2003). Crop plants can increase iodine absorption and accumulation in their edible parts when subjected to exogenous applications of different iodine sources (Comandini et al., 2013; Weng et al., 2014). Tonacchera et al. (2013) reported a noticeable increase of iodine in the urine samples of people who consumed biofortified vegetables. Moreover, 80% of the iodine in human and animal bodies was derived from the edible part of the crop/vegetable under natural conditions (Welch and Graham, 2005). In contrast, the bioavailability of iodine from food sources can easily be enhanced by up to 99% (Weng et al., 2014).
Soil geochemistry plays a vital role in iodine retention and conversion, as it primarily depends on the transfer of iodine from the atmosphere via precipitation. Scientists are interested in studying this atmospheric transport of iodine and the soil’s ability to absorb it. Moreover, soils of mountainous areas are more prone to iodine deficiency due to topographical and soil characteristics (Von Oettingen et al., 2017). Edible crops grown in iodine-deficient soils are usually low in iodine concentration, and humans and animals consuming those crops face multiple IDDs. Plants can uptake and translocate the organic and inorganic iodine species (iodide and iodate) from the soil (Takeda et al., 2019). Applying iodine-rich organic (alfalfa, dulse) or inorganic (potassium iodide-KI) sources may enhance the concentration and retention of iodine in the soil.
Coriander (Coriandrum sativum L.) is a culinary, herbal, and medicinal plant of the Apiaceae family and is an important spice crop (Mandal and Mandal, 2015). It is commonly used as an ingredient in daily food preparation (Fahad et al., 2020). Coriander is primarily grown in Pakistan, Bangladesh, India, Russia, Morocco, Central Europe, and China (Sahib et al., 2013). Different parts of the plant are associated with various health and biological activities. Coriander has been used remedially for several gastrointestinal disorders (Sahib et al., 2013). Seeds of the coriander plant are used in several food items (Bhuiyan et al., 2009; Anwar et al., 2011). It has been reported that the concentration of iodine in edible parts of fresh coriander may reach a level of 2.28 mg kg−1 (Weng et al., 2013).
Previous studies analyzed the fate, accumulation, and transformation of iodine as a function of various organic and inorganic treatments for crops (Zhu et al., 2003). However, research reports on the uptake of iodine by edible crops have been poorly documented. Moreover, the bioavailability of iodine from soils after applying the selected amendment has not been well established. Therefore, the objective of this study was to investigate iodine uptake by coriander (Coriandrum sativum L.) applied with organic and inorganic forms of iodine after adding soil amendments, namely, sawdust, rice husk, charcoal, wood ash, and gypsum.
In the current study, two exogenous iodine sources were used to evaluate their bioavailability to plants grown in amended soils. One source was an inorganic conventional iodine source, i.e., potassium iodide (KI), and the second was derived from seaweed and was organic in nature. The organic source of iodine was extracted from alfalfa (Medicago sativa) and dulse (Rhodymenia palmata) and was purchased commercially. According to Hou et al. (1997), the seaweeds contained 2–3% water-soluble iodine.
The experiment was carried out from April to June 2020, in a 350 m2 (7 × 50 m) greenhouse, at the Commission on Science and Technology for Sustainable Development in the South (COMSATS) University Islamabad, Abbottabad (34°11′57.19″N, 73°14′50.36″E). The greenhouse had a bow-roof shed design structure covered with 100 μm thick transparent polyethylene film. The air temperature ranged between 30.7 and 38.4°C, while the humidity was between 21 and 28%.
The soil used in the experiments was collected from the old vegetable production area of the Abbottabad district. The soil was sieved through a 3 mm mesh sieve, and its main agrochemical properties are listed in (Table 1). The methods for soil analysis have been reported previously (Mohiuddin et al., 2019a). The soil was sieved through a 3 mm sieve and packed into a pot. The soil had a background iodine level of 0.6 mg kg−1. Vegetables planted without iodine were used as control groups for comparison. Sawdust, rice husk, charcoal, wood ash, and gypsum were applied to the soil as amendments at the rate of 10 t ha−1 (based on 2 million kg soil per plow layer in ha). Amendments were mixed in the column’s topsoil (0–20 cm). Iodine was applied using an inorganic form, i.e., potassium iodide (KI), and an organic source.
The organic source of iodine [extracted from alfalfa (Medicago sativa) and dulse (Rhodymenia palmata)] was commercially purchased and applied to the soil. The iodine sources were fertilized in the soil at 50 mg kg−1. I dose of more than 50 mg kg−1 was reported as phytotoxic by Humphrey et al. (2019). The experiment was a factorial (6 × 2) (6 soil amendments × 2 sources of iodine) resulting in 12 experimental units and arranged into a randomized complete block design (RCBD) replicated three times under greenhouse conditions. The base fertilizer was applied, and the soil moisture was maintained at 50% of the maximum field water capacity. During the experimental period, deionized water was added regularly to keep the moisture stable. Seeds of coriander were germinated on wet filters for 2 days in the dark at 25°C after sterilization. To develop seedlings, the germinated seeds were subsequently transferred into quartz sand and cultivated on a nutritional solution (Table 2). After 8 weeks of development, the seedlings were transferred into pots with a homogeneous growth condition. Each treatment was performed in triplicates. Vegetable tissues from the root, stem, leaf, and fruit were separately collected after harvest. They were then dried with absorbent paper after being washed with deionized water. The fresh weight of each part was measured before slicing it into smaller pieces. These were then dried at 50°C, and pulverized in a stainless steel mill. The tissue samples were homogenized after sieving through a 60-mesh sieve and then kept at 4°C until analysis.
Iodine in soil and plant was measured by methods used by Kesari et al. (1998). For the experiment, 0.5 g of sieved soil r was mixed with 15 ml of deionized water, shaken for 30 min, and filtered via filter paper Whatman 42. The filtrate was centrifuged for 15 min at 5000 rpm, and the supernatant was taken. Then, 1 ml of 5% EDTA solution and 0.5 ml of bromine water were added to the supernatant and mixed thoroughly. Formic acid was added drop by drop to remove excess brominated water. Then, 1 ml of 0.1% KI and 1 ml of leuco violet crystal solution were added. The pH of the solution was kept between 4.4–5.4 by using 0.5 M NaOH and formic acid. The mixture was diluted to 25 ml and left for 30 min for color development. Iodine contents were determined calorimetrically using a UV spectrophotometer (Model: LI-UV-7000) at 591 nm. For plant samples, a similar abovementioned procedure was adopted. All the chemicals used in the current study were analytical grade and purchased from Sigma Aldrich.
A subsample from each plot was secured to analyze zinc (Zn), iron (Fe), phosphorous (P), calcium (Ca), magnesium (Mg), sodium (Na), and potassium (K) content. Shoot samples were washed rapidly with tap water and deionized water and then dried at approximately 45°C in a forced-draft oven to constant weight. The dried grains were ground to fine flour using an agate mill (Pulverisette 9, Fritsch GmbH, Germany) and digested with HNO3 + H2O2 in a microwave digester. Ca, Mg, Na, K, Fe, and Zn were analyzed through atomic absorptive (AAS-), while P was determined using the blue method via UV spectrophotometer (Model: LI-UV-7000) at 700 nm.
The iodine concentrations in leaves and roots were calculated on a fresh weight basis. The transfer factor was calculated by using Equation (1):
Whereas the distribution constant was determined by using Equation (2):
Effects of different treatments on the dependent iodine uptake, biomass production, and mineral composition were determined using two-way ANOVA at 0.05 level of least significant difference (LSD) SPSS. Figures were prepared using the ORIGIN software version 2020.
The application of amendments had a significant effect on both shoot and root biomass irrespective of the iodine treatments (p < 0.05) (Figure 1). The application of wood ash amended soil fertigated with KI treatment significantly increased the shoot (40.3 g m−2) and root biomass (12.1 g m−2) (p < 0.05). Soil amendments differed for coriander biomass (shoot) in the order of wood ash (52.4 g m−2) > charcoal (50.8 g m−2) > sawdust (47.4 g m−2) > gypsum (45.1 g m−2) > rice husk (42.5 g m−2). The coriander biomass was enhanced by 42% in the wood ash mixed soil supplemented with KI salt compared to the sole control.
Figure 1. Shoot and root biomass yield of coriander plants after soil amendments as affected by (A) potassium iodide (KI) and (B) organic iodine (OI).
Iodine accumulation in roots of coriander plants significantly varied (p ≤ 0.005) among iodine sources (IS) (F = 1688.38, p = 0.01), and soil amendments (SA) (F = 5589.72, p < 0.01) (Table 3). A significantly higher I concentration was found in the roots of coriander plants that were grown in gypsum-amended soil fertigated with organic iodine (240.4 ± 1.29 μg kg−1). However, the lowest iodine content in the roots was found in a plant grown in control settings (without amendment) fertigated with the organic iodine (OI) (26.89 ± 1.17 μg kg−1). All plants grown in amended soils retained significantly higher iodine content irrespective of the source compared to respective controls. All the possible interactions between IS × SA had a significant effect (F = 1196.34, p ≤ 0.005) on iodine uptake by the coriander grown in amended soil. The interactive effect of IS × SA revealed that the KI treatments significantly differed (p ≤ 0.05) from OI regarding iodine uptake in the roots of the coriander plant (Figure 1). Maximum iodine was accumulated (230 μg kg−1) in roots of coriander grown in gypsum-amended soil fertigated with KI when compared with the control (Figure 2).
Table 3. Effect of different iodine sources (IS) and soil amendments (SA) on iodine uptake in roots of coriander crop.
Figure 2. Iodine concentrations in the shoots and roots of coriander plants after soil amendments as affected by (A) potassium iodide (KI) and (B) organic iodine (OI).
Coriander is usually utilized as a fresh shoot. Iodine accumulation in shoots of coriander plants significantly varied (p ≤ 0.005) among I sources (IS) (F = 1246.32, p = 0.01) and soil amendments (SA) (F = 5023.77, p < 0.01) (Table 3). A significantly higher I concentration was found in coriander plant shoots grown in wood ash-amended soil fertigated with KI (291.97 ± 1.5 μg kg−1). The combination IS × SA revealed a significant effect on the biofortification of iodine in the shoot (F = 476.94, p < 0.01) (Table 4). Iodine uptake by plants may be associated with soil properties that have modified the coriander’s chemical composition and growth after applying soil amendments. The incorporation of wood ash showed a remarkable increase in iodine concentration in plants over control. Iodine concentration in the plant shoot was significantly higher with KI grown in wood ash amended soil (291.97 μg kg−1), while the lowest iodine was retained by a plant grown in gypsum amended soil fertigated irrespective of the source of iodine (134.65 μg kg−1) (Figure 2). The iodine retention in soil fertigated with KI differed as wood ash (291.97 μg kg−1) > rice hulk (237.66 μg kg−1) > sawdust (217.10 μg kg−1) > charcoal (190.53 μg kg−1) > gypsum (134.28 μg kg−1).
Table 4. Effect of different iodine sources (IS) and soil amendments (SA) on iodine uptake in shoots of coriander crop.
Soil-to-coriander iodine transfer factor values (TF) are shown in Figure 3. The translocation factor of coriander plants added with KI was significantly higher than the organic form of iodine. For plants treated with KI, the TF increased from 0.45 to 0.96 when the soil was amended with wood ash. The translocation factor declined with the application of organic iodine. A lower TF was found in the gypsum-amended soils either supplemented with inorganic or organic iodine. These values were slightly lowered in plants added with organic iodine. The distribution coefficient (DC) values of iodine between the shoot and root are given in Figure 3. The DC values for plants treated with KI increased significantly compared to the control. Wood ash application gave higher DC values (3.51, p < 0.001); while for plants treated with organic iodine, the sawdust treatment exhibited a higher DC value (1.56, p < 0.01) than the control. The effects of iodine sources, soil amendment, and their interaction significantly enhanced the concentration of iodine in coriander plants.
Figure 3. Iodine transfer factors (TF) and distribution coefficients (DC) of coriander plants after soil amendments as affected by (A) potassium iodide (KI) and (B) organic iodine (OI).
Soil amendment increased shoot concentrations of essential nutrients, irrespective of treatments (Table 5). The concentrations of nutrients in the coriander shoot varied in the order of K > P > Mg > Ca > Na > Fe > Zn. K concentrations in coriander shoots supplemented with KI increased by 9.2, 18.9, 28.6, 111.8, and 55.2% in the sawdust, rice husk, charcoal, wood ash, and gypsum treatments, respectively. Ca concentrations in coriander shoots increased by 30.2, 27.6, 14.5, 34.8, and 63.2% in the sawdust, rice husk, charcoal, wood ash, and gypsum treatments, respectively. Sawdust could not enhance Ca in coriander plants. Compared with the control, the amendment application improved P concentrations in coriander from 20.1 mg kg−1 (control) to 29.3 mg kg−1 in rice husk, 23.6 mg kg−1 in wood ash, and 20.3 mg kg−1 in gypsum treatment. Organic sources of iodine enhanced the concentration of nutrients in coriander plants. Gypsum treatment could not enhance the concentration of P in the coriander shoot. Changes in the mineral composition of coriander plants could be associated with the physicochemical variations that occurred within the soil due to the soil amendment.
Although some studies have demonstrated that different iodine types have different absorption or translocation rates by plants (Zhu et al., 2003), there is a lack of any concrete proof of a connection between soil solution iodine and plant uptake. The current study’s findings showed that iodine uptake by coriander was closely connected to varied iodine levels in the soil. KI had a considerably higher capacity for iodine enrichment in coriander plants than OI. Iodine availability in soils, primarily controlled by adsorption–desorption processes, is a prerequisite for iodine uptake by plants growing in soils. The results showed that OI has a significantly higher affinity for the soil utilized in this experiment than KI; hence its availability in soils was lower than iodide. Therefore, the difference in iodine concentrations in soil solution, which is immediately available for root uptake, might account for the difference in iodine uptake by coriander plants supplied with KI and OI. Low iodine concentrations in soil solutions after OI treatments may be caused by significant iodine volatilization. According to Fuge (1996), the global iodine cycle and the transfer of iodine to the biosphere are significantly influenced by the volatilization of iodine from soils. Iodine in the soil was discovered to be volatilized from the soil–plant system into the atmosphere as organic iodine (Muramatsu et al., 1995; Kumar and Hemantaranjan, 2017). Organoiodides, volatile iodine molecules, can escape terrestrial ecosystems, including peat bogs and rice fields (Redeker et al., 2000; Dimmer et al., 2001), and the concentrations of iodide in soils eventually determine how much volatile organoiodides may be produced from soil (Keppler et al., 2003).
Soil amendment significantly affected the plant biomass and nutritional value. The application of charcoal and wood ash produced higher biomass than other soil amendments. This might have resulted from adding soil amendments that affected soil properties and crop growth by improving soil pH, organic matter, and several nutrient concentrations, as well as crop emergence and yield relative to control treatment (Mohiuddin et al., 2019a). Wood ash, charcoal, and rice husk increased the iodine concentration in both root and shoot. The TF and DC of iodine between shoot and root were relatively higher for plants treated with KI. Similarly, wood ash showed a higher DC ratio than other soil amendments. The lower levels of iodine in the growth environment (0.02–0.2 mg kg−1) benefit several plant species (Zhu et al., 2003).
The addition of soil amendment significantly enhanced the biomass of plants. This could be due to the provision of essential nutrients to plants. Phytofortification of iodine was higher with the addition of an iodide form of iodine compared to the organic source. The higher iodine accumulation in plant tissue with KI was also reported by (Zhu et al., 2003; Voogt et al., 2010; Humphrey et al., 2019). Mohiuddin et al. (2019b) reported enhanced bioaccumulation of iodine after adding different soil amendments. Applying organic amendments retained more iodine in the soil and reduced its losses via leaching.
Previous reports have shown that iodine was not phloem-mobile; therefore, iodine accumulation in grains was lower (Mackowiak and Grossl, 1999). For leafy vegetables, iodine accumulation in the edible parts is dependent mainly on the xylem transport. Therefore, one of the goals of the present study was to demonstrate whether iodine can be accumulated in coriander shoots for supplementary iodine nutrition. This study showed a higher iodine TF than reported for rice crops (Mackowiak and Grossl, 1999) and wheat grains (Shinonaga et al., 2001). It was observed that plants grown in gypsum-amended soil accumulated a lower amount of iodine content in plant shoots when compared with other soil amendments. Applying gypsum resulted in higher Ca content, lower iodine retention in soil (Fuge, 2013), and lesser iodine bioavailability (Hu et al., 2012). Ca-containing amendment increased soil pH and CEC and affected the soil’s ability for iodine retention at higher pH (Fuge, 2013). Instead, the organic amendments enhanced the soil’s organic carbon, which substantially enhanced the soil’s capacity to retain iodine contents (Johnson, 2003). The wood ash amendment showed an improvement in iodine bioavailability from the soil.
The amount of iodine accumulation in the shoots was higher than in the roots of coriander plants. Soil properties such as cation exchange capacity, organic matter, and clay minerals have affected iodine adsorption and bioavailability (Yoshida et al., 1992). Changes in soil properties due to the soil amendment could be attributed to the enhanced mineral contents in coriander plants. Mohiuddin et al. (2021) reported the relationship of iodine with soil properties and predicted the substantial effects on the extractability of iodine contents from soils. This study confirms that the availability of iodine to coriander can be improved using a suitable soil amendment. Coriander edible shoots and leaves can absorb and store iodine when exogenous iodine fertilizer is used. After using the exogenous iodine fertilizers, KI, and seaweed composite, a clear difference was shown in the amount of iodine that plants could absorb.
It is concluded that the KI-fertigated soils accumulate higher iodine than the OI-fertigated soils. Soil amended with wood ash increased the iodine concentration in plant tissues. Higher values of TF and DC were recorded for plants treated with KI. Plants absorb more iodine from KI than from the seaweed composite. Favorable iodine addition can speed up the development of vegetables; however, too much iodine might be detrimental to them. Therefore, a suitable soil amendment can enhance iodine availability in soil. It is necessary to investigate iodine accumulation in vegetables under different soil amendments concerning soil attributes under field conditions.
The original contributions presented in the study are included in the article/supplementary material, further inquiries can be directed to the corresponding author/s.
YT and AmF: conceptualization. YT, MM, and AmF: methodology. MM and AsF: fieldwork and chemical analysis. YT, AA, and NG: data analysis. YT and MM: writing – original draft preparation. AA, AH, and MI: validation and writing – review and editing. AA: supervision. All authors contributed to the article and approved the submitted version.
This research was supported by the Key Research and Development Program of Hunan Province (2020NK2022), Joint Funds of the National Natural Science Foundation of China (grant number: U21A20187), the Scientific Research Fund of Hunan Provincial Education Department (18A150), and National Key R&D Program (grant number: 2020YFA0608100).
We acknowledge the editor and reviewers for their comments and suggestions to improve our manuscript.
MM was employed by Environmental Management Consultant (EMC) Pvt Ltd, Pakistan.
The remaining authors declare that the research was conducted in the absence of any commercial or financial relationships that could be construed as a potential conflict of interest.
All claims expressed in this article are solely those of the authors and do not necessarily represent those of their affiliated organizations, or those of the publisher, the editors and the reviewers. Any product that may be evaluated in this article, or claim that may be made by its manufacturer, is not guaranteed or endorsed by the publisher.
Anwar, F., Sulman, M., Hussain, A. I., Saari, N., Iqbal, S., and Rashid, U. (2011). Physicochemical composition of hydro-distilled essential oil from coriander (Coriandrum sativum L.) seeds cultivated in Pakistan. J. Med. Plants Res. 5, 3537–3544.
Bhuiyan, M. N. I., Begum, J., and Sultana, M. (2009). Chemical composition of leaf and seed essential oil of Coriandrum sativum L. from Bangladesh. Bangladesh J. Pharmacol. 4, 150–153.
Chilimba, A. D. C., Young, S. D., Black, C. R., Meacham, M. C., Lammel, J., and Broadley, M. R. (2012). Agronomic biofortification of maize with selenium (Se) in Malawi. Field Crop Res. 125, 118–128. doi: 10.1016/j.fcr.2011.08.014
Comandini, P., Cerretani, L., Rinaldi, M., Cichelli, A., and Chiavaro, E. (2013). Stability of Iodine during cooking: investigation on biofortified and not fortified vegetables. Int. J. Food Sci. Nutr. 64, 857–861. doi: 10.3109/09637486.2013.798270
Dasgupta, P. K., Liu, Y., and Dyke, J. V. (2008). I nutrition: I content of iodized salt in the United States. Environ. Sci. Technol. 42, 1315–1323. doi: 10.1021/es0719071
De Benoist, B., McLean, E., Andersson, M., and Rogers, L. (2008). Iodine deficiency in 2007: global progress since 2003. Food Nutr. Bull. 29, 195–202. doi: 10.1177/156482650802900305
Dimmer, C. H., Simmonds, P. G., Nickless, G., and Bassford, M. R. (2001). Biogenic fluxes of halomethanes from Irish peatland ecosystems. Atmos. Environ. 35, 321–330. doi: 10.1016/S1352-2310(00)00151-5
Fahad, B. A., Agheem, M. H., Memon, S. A., Baloch, A. R., Tunio, A., and Gabole, A. A. (2020). Efficient mitigation of cadmium and lead toxicity in coriander plant utilizing magnetite (Fe3O4) nanofertilizer as growth regulator and antimicrobial agent. Int. J. Environ. Anal. Chem. 102, 3868–3879. doi: 10.1080/03067319.2020.1776861
Fuge, R. (1996). Geochemistry of Iodine in relation to iodine deficiency diseases. Geol. Soc. Lond., Spec. Publ. 113, 201–211. doi: 10.1144/GSL.SP.1996.113.01.16
Fuge, R. (2013). “Soils and iodine deficiency” in Essentials of Medical Geology. ed. O. Selinus (Dordrecht: Springer), 417–432.
Hou, X., Chai, C., Qian, Q., Liu, G., Zhang, Y., and Wang, K. (1997). The study of Iodine in Chinese total diets. Sci. Total Environ. 193, 161–167. doi: 10.1016/S0048-9697(96)05323-5
Hu, Q. H., Moran, J. E., and Gan, J. Y. (2012). Sorption, degradation, and transport of methyl iodide and other iodine species in geologic media. Appl. Geochem. 27, 774–781. doi: 10.1016/j.apgeochem.2011.12.022
Humphrey, O. S., Young, S. D., Bailey, E. H., Crout, N. M. J., Ander, E. L., Hamilton, E. M., et al. (2019). Iodine uptake, storage and translocation mechanisms in spinach (Spinacia oleracea L.). Environ. Geochem. Health 41, 2145–2156. doi: 10.1007/s10653-019-00272-z
Johnson, C. C. (2003). The Geochemistry of Iodine and Its Application to Environmental Strategies for Reducing the Risk from Iodine Deficiency Disorders (IDD). Report Number: CR/03/057N Affiliation: British Geological Survey Commissioned Report.
Kalimbira, A. A., Chilima, D. M., Mtimuni, B., and Mvula, N. (2005). Knowledge and practices related to use of iodised salt among rural Malawian households Bunda. J. Agric. Environ. Sci. Technol. 3, 73–82.
Keppler, F., Borchers, R., Elsner, P., Fahimi, I., Pracht, J., and Schöler, H. F. (2003). Formation of volatile iodinated alkanes in soil: results from laboratory studies. Chemosphere 52, 477–483. doi: 10.1016/S0045-6535(03)00198-X
Kesari, R., Rastogi, R., and Gupta, V. K. (1998). A simple and sensitive spectrophotometric method for the determination of Iodine in environmental samples. Chem. Anal. 43, 201–207.
Kumar, P., and Hemantaranjan, A. (2017). Iodine: a unique element with special reference to soil-plant-air system. Adv. Plant Physiol. 17:314.
Lawson, P. G., Daum, D., Czaudema, R., Meuser, H., and Hartling, J. W. (2015). Soil versus foliar I fertilization as a biofortification strategy for field-grown vegetables Frontiers in Plant. Science 6:450.
Ligowe, I. S., Bailey, E. H., Young, S. D., Ander, E. L., Kabambe, V., Chilimba, A. D., et al. (2021). Agronomic iodine biofortification of leafy vegetables grown in Vertisols, Oxisols and Alfisols. Environ Geochem Health 43, 361–374. doi: 10.1007/s10653-020-00714-z
Mackowiak, C. L., and Grossl, P. R. (1999). Iodate and iodide effects on iodine uptake and partitioning in rice (Oryza sativa L.) grown in solution culture. Plant Soil 212, 133–141. doi: 10.1023/A:1004666607330
Mandal, S., and Mandal, M. (2015). Coriander (Coriandrum sativum L.) Essential oil: chemistry and biological activity. Asian Pac. J. Trop. Biomed. 5, 421–428. doi: 10.1016/j.apjtb.2015.04.001
Manzeke, G. M., Mtambanengwe, F., Ne Zomba, H., and Mapfumo, P. (2014). Zinc fertilization influence on maize productivity and grain nutritional quality under integrated soil fertility management in Zimbabwe. Field Crop Res. 166, 128–136. doi: 10.1016/j.fcr.2014.05.019
Mohiuddin, M., Irshad, M., Farig, M., and Shahzad, M. (2021). Extractability of Iodine from soils using different methods in relation to soil properties. Arab. J. Geosci. 14:374. doi: 10.1007/s12517-021-06782-4
Mohiuddin, M., Irshad, M., Hussain, Z., An, P., and Tahir, A. A. (2019b). Leachability of Iodine from soils of different land uses as affected by selected amendments. Environ. Eng. Manag. J. 18, 2095–2103.
Mohiuddin, M., Irshad, M., Ping, A., Hussain, Z., and Shahzad, M. (2019a). Bioavailability of Iodine to mint from soil applied with selected amendments. Environ. Pollutants Bioavailability 31, 138–144. doi: 10.1080/26395940.2019.1588077
Muramatsu, Y., Yoshida, S., and Ban-Nai, T. (1995). Tracer experiments on the behavior of radioiodine in the soil-plant-atmosphere system. J. Radioanal. Nucl. Chem. 194, 303–310. doi: 10.1007/BF02038428
Patrick, L. (2008). Iodine: deficiency and therapeutic considerations. Altern. Med. Rev. 2, 116–127.
Piccone, N. (2011). The Silent Epidemic of I Deficiency. Life Extension Magazine. Available at: https://www.lifeextension.com/magazine/2011/10/. (Accessed November 16, 2020).
Redeker, K. R., Wang, N. Y., Low, J. C., McMillan, A., Tyler, S. C., and Cicerone, R. J. (2000). Emissions of methyl halides and methane from rice paddies. Science 290, 966–969. doi: 10.1126/science.290.5493.966
Sahib, N. G., Anwar, F., Gilani, A. H., Hamid, A. A., Saari, N., and Alkharfy, K. M. (2013). Coriander (Coriandrum sativum L.): a potential source of high-value components for functional foods and nutraceuticals-A Review. Phytother. Res. 27, 1439–1456. doi: 10.1002/ptr.4897
Shinonaga, T., Gerzabek, M. H., Strebl, F., and Muramatsu, Y. (2001). Transfer of Iodine from soil to cereal grains in agricultural areas of Austria. Sci. Total Environ. 267, 33–40. doi: 10.1016/S0048-9697(00)00764-6
Smoleń, S., Ledwożyw-Smoleń, I., and Sady, W. (2016). The role of exogenous humic and fulvic acids in iodine biofortification in spinach (Spinacia oleracea L.). Plant Soil 402, 129–143. doi: 10.1007/s11104-015-2785-x
Szybinski, Z., Jarosz, M., Hubalewska-Dydejczyk, A., Stolarz- Skrzypek, K., Kawecka-Jaszcz, K., Traczyk, I., et al. (2010). I-deficiency prophylaxis and the restriction of salt consumption - a 21st century challenge. Endokrynol. Pol. 1, 135–140.
Takeda, A., Unno, Y., Tsukada, H., Takaku, Y., and Hisamatsu, S. (2019). Speciation of Iodine in soil solution in forest and grassland soils in Rokkasho, Japan. Radiat. Prot. Dosimetry 184, 368–371. doi: 10.1093/rpd/ncz103
Tonacchera, M., Dimida, A., De Servi, M., Frigeri, M., Ferrarini, E., De Marco, G., et al. (2013). Iodine fortification of vegetables improves human iodine nutrition: In vivo evidence for a new model of iodine prophylaxis. J. Clin. Endocrinol. Metab. 98, E694–E697. doi: 10.1210/jc.2012-3509
Ujowundu, C. O., Kalu, F. N., Nwosunjoku, E. C., Nwaoguikpe, R. N., Okechukwu, R. I., and Igwe, K. O. (2011). Iodine and inorganic mineral contents of some vegetables, spices and grains consumed in Southeastern Nigeria. Afr. J. Biochem. Res. 2, 57–64.
Vejbjerg, P., Knudsen, N., Perrild, H., Carle, A., Laurberg, P., Pedersen, I. B., et al. (2007). Effect of a mandatory iodization program on thyroid gland volume based on individuals' age, gender, and preceding severity of dietary iodine deficiency: A prospective, population-based study. J. Clin. Endocrinol. Metab. 92, 1397–1401. doi: 10.1210/jc.2006-2580
Verduzco-Gallo, I., Ecker, O., and Pauw, K. (2014). “Changes in food and nutrition security in Malawi: Analysis of recent survey evidence” in Working Paper 06 (Washington DC, USA: International Food Policy Research Institute)
Von Oettingen, J. E., Brathwaite, T. D., Carpenter, C., Bonnell, R., He, X., Braverman, L. E., et al. (2017). Population survey of iodine deficiency and environmental disruptors of thyroid function in young children in Haiti. J. Clin. Endocrinol. Metab. 102, 644–651. doi: 10.1210/jc.2016-2630
Voogt, W., Holwerda, H. T., and Khodabaks, R. (2010). Biofortification of lettuce (Lactuca sativa L.) with Iodine: the effect of iodine form and concentration in the nutrient solution on growth, development and Iodine uptake of lettuce grown in water culture. J. Sci. Food Agric. 90, 906–913. doi: 10.1002/jsfa.3902
Welch, R. M., and Graham, R. D. (2005). Agriculture: the real nexus for enhancing bioavailable micronutrients in food crops. J. Trace Elem. Med. Biol. 18, 299–307. doi: 10.1016/j.jtemb.2005.03.001
Weng, H., Hong, C., Xia, T., Bao, L., Liu, H., and Li, D. (2013). Iodine biofortification of vegetable plants- an innovative method for iodine supplementation. Chin. Sci. Bull. 58, 2066–2072. doi: 10.1007/s11434-013-5709-2
Weng, H. X., Liu, H. P., Li, D. W., Ye, M., Pan, L., and Xia, T. H. (2014). An innovative approach for iodine supplementation using iodine-rich phytogenic food. Environ. Geochem. Health 36, 815–828. doi: 10.1007/s10653-014-9597-4
White, P. J., and Broadley, M. R. (2009). Biofortification of crops with seven mineral elements often lacking in human diets–iron, zinc, copper, calcium, magnesium, selenium and Iodine. New Phytol. 182, 49–84. doi: 10.1111/j.1469-8137.2008.02738.x
WHO (2007a). Assessment of Iodine Deficiency Disorders and Monitoring their Elimination: A Guide for Programme Managers. 3rd edn.
WHO (2007b). Iodine deficiency in Europe: A continuing public health problem. In M. Andersson, B. Benoistde, I. Darnton- Hill, and F. Delange (Eds.), WHO Published Jointly with Unicef. Iodine – Deficiency. Deficiency Diseases –Prevention and Control. Europe: Andersson Maria, World Health Organization, UNICEF.
Winger, R. J., Konig, J., House, D. A., Konig, J., and House, D. H. (2008). Technological issues associated with iodine fortification of foods. Trends Food Sci. Technol. 19, 94–101. doi: 10.1016/j.tifs.2007.08.002
Yoshida, S., Muramatsu, Y., and Uchida, S. (1992). Studies on the sorption of I-(iodide) and IO3-(iodate) onto Andosols. Water Air Soil Pollut. 63, 321–329. doi: 10.1007/BF00475499
Zhu, Y. G., Huang, Y. Z., Hu, Y., and Liu, Y. X. (2003). Iodine uptake by spinach (Spinacia oleracea L.) plants grown in solution culture: effects of iodine species and solution concentrations. Environ. Int. 29, 33–37. doi: 10.1016/S0160-4120(02)00129-0
Zia, M. H., Watts, M. J., Gardner, A., and Chenery, S. R. (2015). Iodine status of soils, grain crops, and irrigation waters in Pakistan. Environ. Earth Sci. 73, 7995–8008. doi: 10.1007/s12665-014-3952-8
Keywords: iodine deficiency disorders, iodine uptake, amended soils, micronutrients, coriander
Citation: Tianyi Y, Farooq A, Mohiuddin M, Farooq A, Gonzalez NCT, Abbasi A, Hina A and Irshad M (2023) Role of different organic and inorganic amendments in the biofortification of iodine in Coriandrum sativum crop. Front. Ecol. Evol. 11:1145979. doi: 10.3389/fevo.2023.1145979
Received: 16 January 2023; Accepted: 14 February 2023;
Published: 09 March 2023.
Edited by:
Awais Shakoor, Universitat de Lleida, SpainReviewed by:
Ghulam Yasin, Bahauddin Zakariya University, PakistanCopyright © 2023 Tianyi, Farooq, Mohiuddin, Farooq, Gonzalez, Abbasi, Hina and Irshad. This is an open-access article distributed under the terms of the Creative Commons Attribution License (CC BY). The use, distribution or reproduction in other forums is permitted, provided the original author(s) and the copyright owner(s) are credited and that the original publication in this journal is cited, in accordance with accepted academic practice. No use, distribution or reproduction is permitted which does not comply with these terms.
*Correspondence: Yan Tianyi, eWFudGlhbnlpMUAxNjMuY29t; Muhammad Mohiuddin, bWQubW9oaXVkZGluODdAZ21haWwuY29t
Disclaimer: All claims expressed in this article are solely those of the authors and do not necessarily represent those of their affiliated organizations, or those of the publisher, the editors and the reviewers. Any product that may be evaluated in this article or claim that may be made by its manufacturer is not guaranteed or endorsed by the publisher.
Research integrity at Frontiers
Learn more about the work of our research integrity team to safeguard the quality of each article we publish.