- 1Laboratoire de Biométrie et Biologie Évolutive Unité Mixte de Recherche (UMR) 5558, Centre National de la Recherche Scientifique (CNRS), Université Lyon 1, Villeurbanne, France
- 2Office Français de la Biodiversité, Direction de la Recherche et de l’Appui Scientifique, Service Conservation et Gestion Durable des Espèces Exploitées, Châteauvillain, France
- 3Office Français de la Biodiversité, Direction de la Recherche et de l’Appui Scientifique, Service Conservation et Gestion des Espèces à Enjeux, Impasse de la Chapelle, Villeneuve-de-Rivière, France
- 4Comportement et Ecologie de la Faune Sauvage (CEFS), Institut National de Recherche pour l'Agriculture, l'Alimentation et l'Environnement (INRAe), Université de Toulouse, Castanet-Tolosan, France
It is now broadly admitted that female reproductive senescence – a decline in reproductive performance with increasing age – occurs in most species, at least among birds and mammals. Although information is more limited, male reproductive senescence has been regularly inferred from the decline in the size or performance of phenotypic traits that underly male reproductive success, particularly secondary sexual traits. However, the degree to which environmental conditions influence the pattern of senescence in sexual traits remains largely unknown. From the analysis of two long-term studies of populations of European roe deer (Capreolus capreolus) subjected to markedly different environmental contexts in the wild, we tested the hypothesis that harsh natal and/or current conditions should lead to earlier and/or stronger rates of senescence in the length of fully-grown antlers than good natal and/or current conditions. We found evidence of similar patterns of antler length senescence in both populations, with an onset of senescence around 7 years of age and a decrease of length by about 1–1.5 cm per additional year of life from 7 years of age onwards. We found that good early-life conditions delay senescence in antler length in roe deer. Our results also revealed that senescent males seem to be unable to allocate substantially to antler growth, confirming that antler size is, therefore, an honest signal of male individual quality. By modulating age-specific allocation to secondary sexual traits, natal and current conditions could influence female mate choice and male–male competition over mates, and as a result age-specific reproductive success, and should be accounted for when studying the dynamics of sexual selection.
Introduction
From an evolutionary viewpoint, the process of reproductive senescence (also called “reproductive ageing”) corresponds to a decline in reproductive performance with increasing age (Monaghan et al., 2008; Nussey et al., 2013; Gaillard and Lemaître, 2020). Given the wealth of recently accumulated empirical evidence, the widespread occurrence of female reproductive senescence in the wild is now unequivocal, at least for avian and mammalian species (Nussey et al., 2013; Lemaître et al., 2020a; Vágási et al., 2021). While various reproductive metrics have been used to evaluate female reproductive senescence, the number of viable daughters at birth produced by a female of age x (the “mx” value) has been the most frequently studied and has been shown to decrease with increasing age in most mammals (Lemaître et al., 2020a). However, several components of this quantity have also been shown to decline over the lifetime (Lemaître and Gaillard, 2017), including birth rate (e.g. red squirrels, Tamiasciurus hudsonicus, McAdam et al., 2007), pregnancy rate (e.g. moose, Alces alces, Ericsson et al., 2001), and litter/clutch size (e.g. barn swallows, Hirundo rustica, Balbontín et al., 2012).
Currently, the information on male reproductive performance throughout the life course is far more limited due to the difficulties of having large samples of known-age animals (e.g. in mammalian species with typical female-biased adult sex ratio) and of assigning paternities in the wild (Lemaître and Gaillard, 2017). In polygynous and promiscuous species, male reproductive success can only be reliably assessed using pedigree information, especially when multiple mating and paternity occur, and the lack of required genetic data for determining paternity likely explains the low number of studies on age-specific reproductive success in males (Lemaître and Gaillard, 2017). Despite this, a few recent studies of paternity in vertebrates indicate that male reproductive senescence is likely pervasive in the wild (e.g. Widdig et al., 2004; Dugdale et al., 2011 for case studies).
Male reproductive success depends on the allocation of energy to pre-copulatory traits that confer an advantage in terms of male–male competition and/or female mate choice (i.e. secondary sexual traits such as colouration in peacock, Pavo cristatus, Dakin and Montgomerie, 2013; antlers in white-tailed deer, Morina et al., 2018) and post-copulatory traits that confer an advantage in male–male competition to fertilise females (e.g. testes size and sperm concentration, Schulte-Hostedde and Millar, 2004; sperm quality, Gage et al., 2004). In polygynous species, where males compete intensely for access to females, allocation to pre-copulatory traits is a key determinant of reproductive success as they may signal male genetic quality and/or confer an advantage during physical contests among males (Clutton-Brock, 1982; Berglund et al, 1996). So far, studies that focused on the occurrence of male reproductive senescence in terms of a decline in the size and/or performance of secondary sexual traits with increasing age have provided contrasting results (see Lemaître and Gaillard, 2017 for a review). For instance, although senescence has been reported in tail length in barn swallow (Møller and De Lope, 1999); number of antler points in red deer, Cervus elaphus (Mysterud et al. 2005); antler size in moose, Alces alces (Stewart et al. 2000); horn growth in ibex, Capra ibex (Von Hardenberg et al., 2004) and pigmentation in kestrels, Falco tinnunculus (Lopez-Idiaquez et al., 2016), many other studies have failed to detect senescence in male reproductive traits (e.g. song parameters in reed warbler, Acrocephalus arundinaceus, Forstmeier et al., 2006; antler mass in red deer, Cervus elaphus, Kruuk et al., 2002). Moreover, a given secondary sexual trait may senesce in certain species, but not in other closely related species (Lemaître and Gaillard, 2017; see Table 1).
Empirical evidence also suggests that environmental conditions modulate patterns of senescence among populations from the same species. So far, these studies have largely focused on actuarial senescence (i.e. an increase of mortality rate with age) (Holand et al., 2016; Cayuela et al., 2021) or on female reproductive senescence (e.g. Svalbard reindeer, Rangifer tarandus platyrhynchus in Douhard et al., 2016; Asian elephant, Elephas maximus in Mumby et al., 2015). A meta-analysis based on 14 bird and mammal species reported that the rate of reproductive senescence in females is, on average, lower when the conditions experienced during post-natal development are favourable (Cooper and Kruuk, 2018). This relationship was not statistically significant in males, possibly because of a lack of statistical power (effects size only available for four species in this meta-analysis). Yet, evidence that both current and early-life environmental conditions influence male reproductive traits during adulthood is widespread in the literature (e.g. stalk-eyed fly, Cyrtodiopsis dalmanni in Cotton et al., 2004; red grouse, Lagopus lagopus scoticus in Vergara et al., 2012) and studies evaluating how environmental conditions modulate the age-related decline in secondary sexual traits, both in terms of the strength (rate) and timing (age at onset) of male reproductive senescence are now required.
To fill this knowledge gap, we investigated among- and between-population differences in senescence of antler length in two populations of European roe deer (Capreolus capreolus) that experience markedly different environmental contexts. The antlers of cervids are one of the most conspicuous secondary sexual traits in the living world (Gould, 1974; Clutton-Brock, 1982; Lemaître et al., 2014a) even if the relative antler length substantially differs among Cervids, which is largely explained by the species-specific intensity of sexual selection (Geist, 1987; Geist, 1998). Antlers are used in aggressive contests between males (Clutton-Brock, 1982; Hoem et al., 2007) and as an honest signal of phenotypic quality (see e.g. Vanpé et al., 2007; Morina et al., 2018). In the highly polygynous red deer (Cervus elaphus), antler size is correlated with testes size, as well as both ejaculate quantity and quality, confirming the hypothesis that antler size is a reliable indicator of male fertility (Malo et al. 2005). In this study, we used antler length as a measure of antler size, as antler mass or volume cannot been measured on living individuals. Moreover, antler length is known to be associated with mating success in males in red deer (e.g. Kruuk et al., 2002) and roe deer (Vanpé et al., 2010) and currently constitutes a commonly used measure in comparative studies (Clutton-Brock, 1982; Plard et al., 2011). Antlers are deciduous sexual traits that require a huge allocation of energy to grow each year (Goss, 1970; Baxter et al., 1999), which is likely to impact short-term survival (Lemaître et al., 2018). Senescence in antler traits, especially length, has already been documented in many deer species in natura (see Table 1), including roe deer (Vanpé et al., 2007). However, Vanpé et al. (2007) analysed age-dependence in antler size using age classes, precluding an accurate assessment of the onset and rate of reproductive senescence.
Based on the above previous findings, we first tested the hypothesis that hard (i.e. fully-grown) antler length decreases with increasing age in adult roe deer males. In addition, we expected that current environmental conditions should affect the pattern of senescence of antler length, with males living in a harsh environment showing faster/steeper senescence in antler size than those living in a favourable environment. Furthermore, since better environmental conditions in early life seem to be associated with slower rates of reproductive senescence, at least in females (Cooper and Kruuk, 2018), we expected males born in good cohorts, that experience a high-quality natal environment, should exhibit later and/or less steep senescence in antler length than males born in poor cohorts.
Material and methods
Study sites, population monitoring and data collection
The European roe deer is a weakly polygynous cervid species characterised by a slight size dimorphism, with males being ca.10% larger than females (e.g. Vanpé et al., 2008). During winter, males are non-territorial, whereas they defend exclusive territories from spring to late summer (e.g. Johansson, 1996). Males carry deciduous antlers that grow back each year starting from the 1st year of life. Antlers of yearlings and adults are cast after the summer rut, in October or November, and immediately grow back until they are cleaned of velvet around February–March, corresponding to their final hard state. In their first year of life, males can grow short antler (called “buttons”) atop their pedicles, before growing full antlers in following years (Sempéré, 1990). The photoperiod affects testosterone and luteinizing hormone (LH) concentration levels through a complex interaction, which in turn governs antler growth and maintenance, modulating the antler growth cycle (Sempéré et al., 1992).
We used data collected on roe deer from two enclosed forests located in France: Trois-Fontaines (Haute-Marne; 48°43′N, 4°55′E; 1,360 ha) and Chizé (Deux-Sèvres; 46°50′N, 0°25′W; 2,614 ha), both managed by the Office Français de la Biodiversité (OFB). These forests differ in terms of environmental conditions: rich soils and continental climate at Trois-Fontaines (i.e. highly productive environment) contrasting with the poor soils and oceanic climate at Chizé (i.e. weakly productive environment, Pettorelli et al., 2006). Roe deer at Trois-Fontaines and Chizé are intensively monitored by capture-mark-recapture (CMR) since 1975 and 1977, respectively, and for this study we used data from 1980 onwards at Trois-Fontaines and 1981 onwards at Chizé (because of the requirement for measurements of hard antlers on known-aged roe deer from 3 years of age onwards, see below). Winter capture sessions (from December until March) are organised each year by the OFB with the help of many volunteers, lasting for about 10 days spread over 7 or 8 weeks. During a capture session, about 2.5 km of nets are set up to encircle a few forest plots. Roe deer are then captured in these nets and transported to the handling site located in the centre of the forest. At each capture, sex, body mass (± 50 g) and antler length (± 0.5 cm) of males are recorded. Antler length is measured from the lower edge of the coronet to the tip of the longest antler beam. Each roe deer of known age (i.e. marked either in their birth spring at a few days of age or during winter capture when they still have a milk premolar tooth) is marked with ear tags and a collar (color/letter coded, VHF and/or GPS) before being released on site (Gaillard et al., 1993). More than two thirds of individuals are of known age in each of the two populations. Due to management restrictions in the two study areas, known-age individuals are rarely removed from the populations, but are right-censored when this occurs. Harvest is not selective in neither Chizé nor Trois-Fontaines, and is allowed only for population control.
Captures take place in winter, during the antler growth period, so that some males are in hard antler, whereas others are still in velvet when caught. A preliminary analysis of our data based on animals that were frequently recaptured during a given season suggested that antlers may remain in velvet for several weeks once they have attained their final size. Hence, because of the existence of individual variation in antler phenology (see also Clements et al., 2010), we only analysed measurements of hard (i.e. fully-grown) antlers to circumvent the need to control for antler growth. Also, preliminary analyses indicate that old males cast their antler later than young ones, which is beyond the scope of this study although it would be of particular interest for future investigations (see Figure S1, Tables S8, S9). There is, however, no reason to expect that the later shedding of velvet by older males would affect the outcomes of our analyses. We used the total sum length of the right and left antlers for a given individual as a measure of antler size. As broken antlers often result from human-related factors, they do not reflect any biological feature of the individuals. We thus attributed the measurement of intact antlers to broken or missing antlers (see also Lemaître et al., 2018). This implementation was justified by the very strong correlation between left and right antler lengths among males (Pearson coefficient of correlation, r = 0.88 [0.85;0.90], N = 325). Finally, since we focused on senescence, we only used antler measurements of males that were at least 3 years of age during the rut (captured during their third year), which corresponds to the age when most males sire fawns for the first time (Vanpé et al., 2009) and when most males have almost reached their full antler size (Vanpé et al., 2007).
Statistical analyses
We performed our analyses on both absolute and relative (to body mass) antler length. Indeed, as antler size is subject to strong positive allometry (Plard et al., 2011) like most ornaments (Kodric-Brown et al., 2006), we replicated all the analyses using antler length relative to body mass. We did not standardise adult male body mass for a certain date since we did not detect any change of mass with Julian date (slope of the linear regression between body mass and Julian date ± SE = −0.010 ± 0.15, t = 32.53, P = 0.486 and 5.884 e-4 ± 9.295 e-3, t = 0.063, P = 0.95 for Trois-Fontaines and Chizé, respectively, Figure S2), in line with the income breeder life history tactic displayed by roe deer (Andersen et al., 2000). For relative antler length, we used the same analytical procedure as described below, except that antler size was log-scaled and body mass was systematically included (on a log scale also) as a covariate in all models. For both absolute and relative antler length, we selected the best model describing age-specific changes in antler length through a comparison of linear mixed effect models, with antler length as the dependent variable, age as the covariate, and the individual identity and year as random effects on the intercept to account for repeated measures of a given individual and annual variations, respectively. We used the R package lme4 (Bates et al., 2015). We compared models that included no age effect (constant antler size), a linear effect of age, a quadratic effect of age, a full-age model (one parameter for each year of age) and a previously selected threshold age model (see below). To control for the confounding effect of the possible selective disappearance of males with small antlers, which could mask senescence at the population level, we sought to incorporate individual longevity as covariate in our models for hard-antlered males. Unfortunately, this information was not available for most males in our dataset and we thus used the age at last capture (corresponding to the age at which we last controlled a given individual) instead, as recommended by Nussey et al. (2008) (see also Van de Pol and Verhulst, 2006).
The threshold model included in the model selection procedure described above was previously identified by estimating the intercept (age of onset of senescence) and slope with their confidence intervals. Basically, we fitted threshold age models using three possible forms: (1) a constant antler length up to a threshold age after which antler length decreases with increasing age; (2) an increase in antler length up to a threshold age after which antler length remains constant; and (3) an increase in antler length up to a threshold age after which antler length decreases with increasing age. When the best model included a threshold age beyond which we did not have any data, we selected the second-best threshold model. Once the best threshold age was estimated, we used the R package OnAge (Jacob et al., 2017) to estimate the confidence interval on the onset of antler senescence.
To investigate the effect of current environmental conditions on antler length senescence, we first identified a population-specific senescence model and then estimated the onset and the rate of senescence (because the two populations face different ecological conditions, see above). Because the onset of senescence did not occur at the same age in both populations in the retained models (see Results), we performed a z test to compare regression coefficients and evaluate differences in the rate of senescence (see Paternoster et al., 1998). Given the limited support for senescence at one site, we also pooled data from the two populations to compare senescence rates (for both absolute and relative antler length) between populations using additive and interactive effects of population and age.
To test for the influence of natal conditions on antler length senescence, each individual was assigned a cohort quality value corresponding to the mean winter body mass of fawns (at 8 months of age) born in that individual birth year, corrected for sex (males taken as reference) and date of capture (standardised on 30th January). Fawn body mass (at 8 months of age) is impacted by climatic conditions and population density (Toïgo et al., 2006), thus serves as an integrative and suitable measure to assess the quality of the year and the influence of these variables on juvenile roe deer. Additionally, this metric is particularly reliable because of the overwhelming influence of cohort variation over current conditions on adult body mass in roe deer (Pettorelli et al., 2002).
In the following analyses, the quality of a given cohort was determined as being either low (i.e. mean fawn winter mass lower than the median among cohorts) or high (i.e. higher than the median among cohorts), with the median being determined separately for each population. Following the same logic as previously described, we evaluated the pattern of reproductive senescence for each cohort quality (i.e. low vs. high) to compare the onset and rate of reproductive senescence in relation to environmental conditions in early life.
Model selection was consistently based on the Akaike Information Criterion (AIC), using the correction for sample size (AICc) to avoid data overfitting (Burnham and Anderson, 2004). All analyses were performed using the R software (version 4.1.2; R Development Core Team, 2015) and all results are reported as parameter estimate ± SE. Coefficients of determination for the models were reported as conditional (providing the proportion of variance explained by both the fixed and random factors). All data and script for the analyses are available in supplementary information.
Results
Senescence of antler length
Our dataset contained fully-grown antler length measurements for 213 males (223 measurements of 142 males at Chizé and 111 measurements of 71 males at Trois-Fontaines). Antlers were on average 11.5% longer at Trois-Fontaines than at Chizé (sum of both antlers: mean of 417 ± 4.76 mm vs. 369 ± 3.43 mm; t224 = −8.208, P < 0.001). There were few data on very old individuals for either population in our dataset: for males 10 years of age or older, we obtained 11 measurements for 9 individuals at Chizé and 4 measurements for 4 individuals at Trois-Fontaines.
In Chizé, the selected model of age-specific changes in antler length was the threshold model (Tables S1–S6, Figures 1A, B). Absolute antler length started to decrease by about 1.4 cm each year from 7.28 [5.37; 8.97] years of age onwards (slope ± SE = −14.39 ± 4.02 mm, P < 0.001, R2 = 0.50) or by 1.35 cm when the allometric effect of body mass on antler length was accounted for (i.e. relative antler length), from 7.22 [5.12; 8.84] years of age onwards (slope ± SE = −0.04 ± 0.01 on a log scale, P < 0.001, R2 = 0.46).
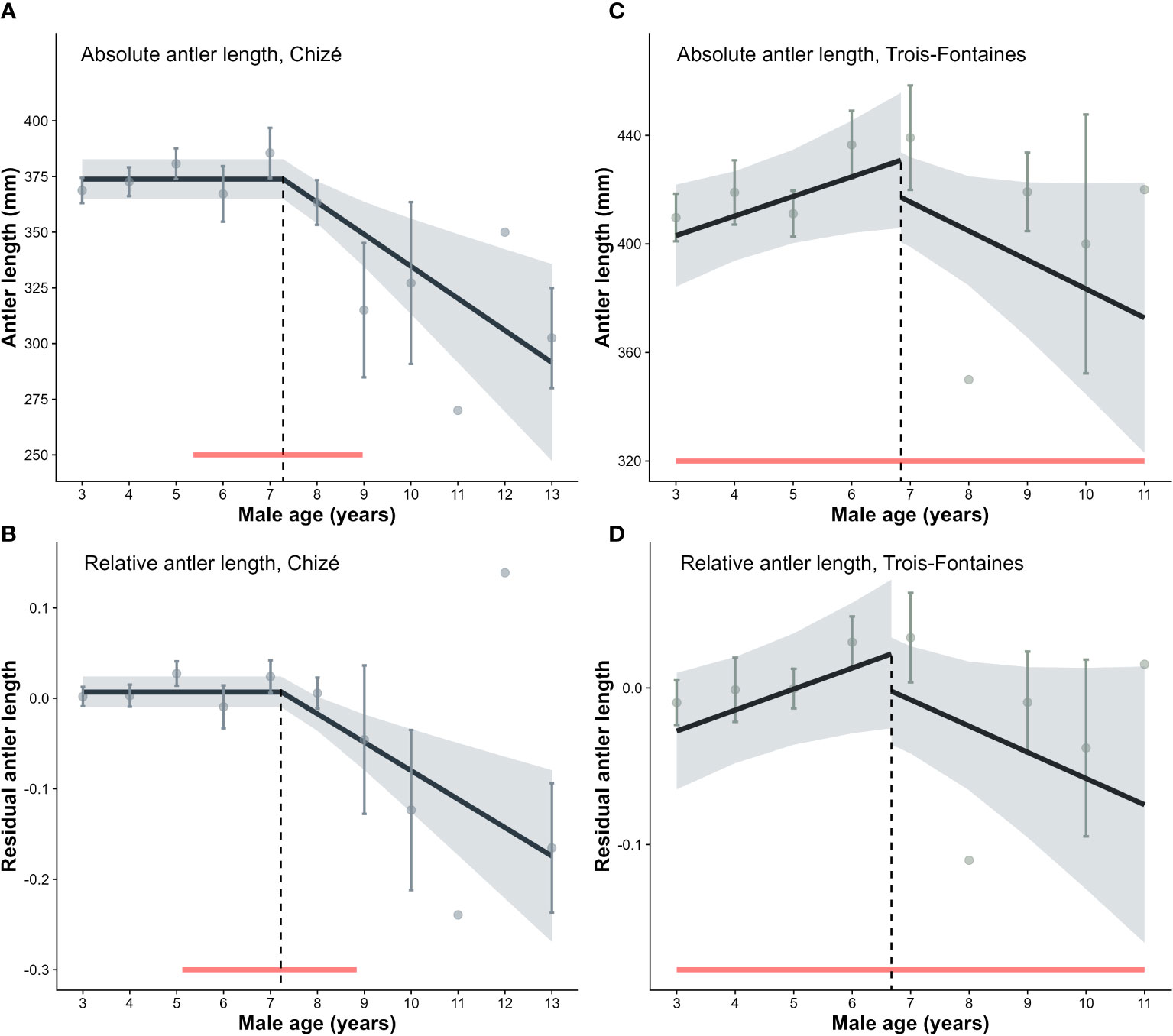
Figure 1 Patterns of age-specific changes in antlers in Chizé and Trois-Fontaines. (A) Absolute antler length in relation to age: age threshold set at 7.28 years old in Chizé (N = 223 measurements of 142 individuals). (B) Relative (to body mass) antler length in relation to age: age threshold set at 7.22 in Chizé. (C) Absolute antler length in relation to age: age threshold set at 6.84 in Trois-Fontaines (N = 110 measurements of 71 individuals). (D) Relative (to body mass) antler length in relation to age: age threshold set at 6.67 in Trois-Fontaines. Red bands correspond to the confidence intervals for the age at the onset of senescence. Light bands correspond to 95% confidence intervals. Residual antler length was obtained by extracting residuals from a regression model of antler length vs. body mass (both log-transformed).
In Trois-Fontaines, the baseline model (i.e. only including the age at last capture) was retained: no detectable diminution of antler length with increasing age was detected at first sight using either absolute or relative antler length (Tables S2–S6, Figures 1C, D). However, a threshold model allowing increased antler length throughout the prime adulthood provided the best fit (AICc = 1178.76 and −152.89 vs. 1179.09 and −152 for the constant model for absolute and relative antler length, respectively). The absolute antler length increased by approximately 0.7 cm each year from 3 to 6.84 [3; 11] years of age (slope before the threshold age ± SE = 7.23 ± 3.68 mm, P = 0.05), and then decreased by about 1 cm per additional year of age (slope beyond the threshold age = −10.67 ± 5.97, P = 0.08). When accounting for the allometric effect of body mass, antler length increased until 6.67 [3; 11] years of age, and then decreased by approximately 0.9 cm per additional year of age (slope before the threshold age ± SE = 0.02 ± 0.01 on a log scale P = 0.03; slope beyond the threshold age = −0.02 ± 0.01 P = 0.11, R2 = 0.71). Although the effect sizes of these models were not statistically different from 0, these models provided a satisfactory fit of the data (R2 = 0.63 and 0.71 for absolute and relative antler length, respectively), with a very similar explanatory power to what we reported at Chizé. The rate of decline of antler length tended to be lower in Trois-Fontaines than in Chizé although no significant differences were detected in neither absolute (1.07 ± 0.6 vs 1.44 ± 0.4 cm lower per year of age, z210 = − 0.51; P = 0.60) nor relative antler length analysis (−0.02 ± 0.01 vs −0.04 ± 0.01, z294 = −1.41; P = 0.16).
We did not find any statistical support for a difference in the age-dependent pattern of antler length between populations (i.e. no evidence of any interactive effect of age and population, Table S3). In both populations, antler length declined linearly from around 7 years of age onwards. We therefore conducted the following analyses by pooling the two populations and testing the additive effect of population in our model selection.
Natal environment and senescence of antler length
On average, fully-grown antler length of males from 3 years of age onwards did not differ between low- and high-quality cohorts (386 ± 4.07 mm vs. 382 ± 4.30 mm in high- and low-quality cohorts, respectively; t291 = 0.69; P = 0.49).
In high-quality cohorts (N = 117 measures on 79 individuals), a decrease in absolute antler length with increasing age was observed from 9.33 [5.23; 13.00] years of age, with a decrease of about 2.5 cm per additional year of age (slope ± SE = −2.5 ± 1.0, P = 0.02, R2 = 0.59, with an additive effect of population). The same pattern was observed once the allometric effect of body mass was included, with a diminution of antler length from 9.60 [5.70; 13.00] years of age and a decrease of 2.7 cm per additional year of age (slope ± SE = 0.08 ± 0.03, P = 0.01, R2 = 0.52; see Figure 2, Tables S5–S7).
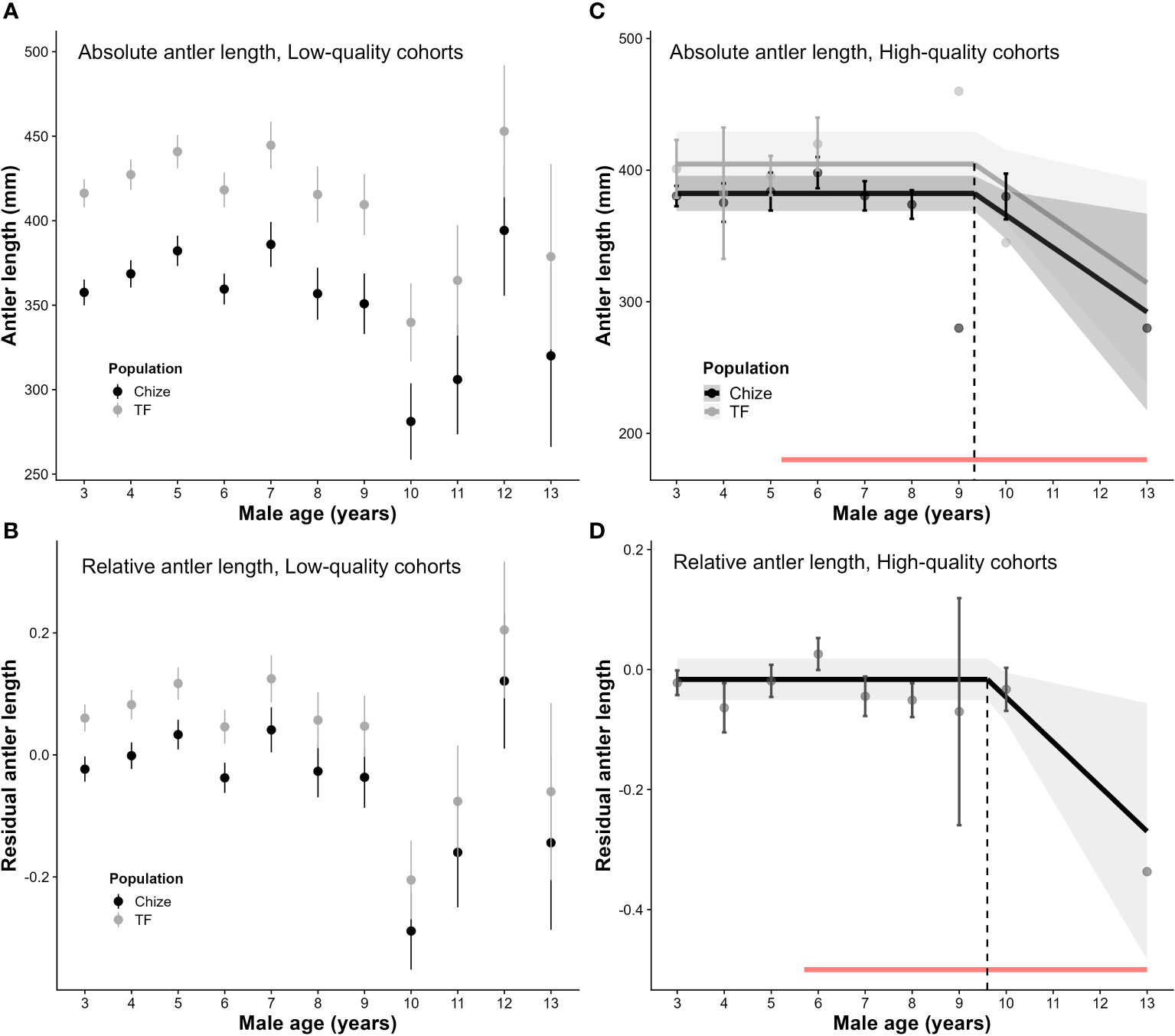
Figure 2 Patterns of age-specific changes in antlers in low- and high-quality cohorts. (A) Absolute total antler length with age in low-quality cohorts (i.e. with a mean yearling mass below the median over the study period): full-age model (N = 217 measures of 134 individuals). (B) Relative total antler length with age in low-quality cohorts: full-age model. (C) Absolute total antler length with age in high-quality cohorts (i.e. with a mean yearling mass above the median over the study period): threshold model with an onset of senescence at 9.33 years of age (N = 117 measures of 79 individuals). (D) Relative total antler length with age in high-quality cohorts: threshold model with an onset of senescence at 9.6 years of age. Red bands correspond to the confidence intervals for the age at the onset of senescence. Grey bands correspond to 95% confidence intervals. Residual antler length was obtained by extracting residuals from a regression model of antler length vs. body mass (both log-transformed).
In low-quality cohorts, however, the age threshold model was not selected for either absolute or relative antler length analyses (full-age model selected with additive effect of population, see Figure 2, Tables S4–S7). The age threshold model was close to the full age model (ΔAICc = 2.5) for absolute antler length, and showed the existence of a strong senescence for both absolute and relative antler length with a slope (± SE) of −12.2 (± 4.49, P = 0.007, R2 = 0.62) and of −0.03 (± 0.01, P = 0.01, R2 = 0.57), respectively. Antler length decreased from about 7 years of age, starting earlier in low- than high-quality cohorts. Slopes of both absolute and relative antler length in high-quality cohorts were strongly influenced by a single measurement (see Figures 2C, D).
Discussion
Senescence in antler length in populations facing different environmental contexts
Our study highlights the occurrence of senescence in the length of fully-grown antlers in roe deer, as evidenced by the decrease in both absolute and relative antler length with increasing age at Chizé and Trois Fontaines, starting at about 7 years of age (see Figure 1). Interestingly, while antler size was constant during the prime adulthood at Chizé, it increased with age until the onset of senescence at Trois-Fontaines. However, it is important to note that our dataset contains a low number of individuals older than 7 years of age, which may limit our ability to accurately quantify senescence patterns. Further data will be required to confirm this pattern. Our findings support Vanpé et al.’s (2007) study that reported evidence for age-specific variation in the length of velvet antler at a given date in both study populations, with a decrease between prime-aged (2–7 years old) and senescent males (8+ years old).
Antler senescence has previously been reported when studying fully grown antlers in more dimorphic and polygynous cervid species in natura, such as moose (Saether and Haagenrud, 1985), fallow deer (Dama dama, Smith et al., 2021) and red deer (Mysterud et al., 2005) (see also Table 1). In managed populations, “antler/trophy regression” (see e.g. Gauthier and Larsen, 1985) or “Zurücksetzen” (see e.g. Drechsler, 1980) or “ravalement” (Agence nationale des chasseurs de grand gibier, 2005) also has frequently been mentioned in roe deer, fallow deer and red deer, but it has never been firmly assessed by quantitative analyses based on detailed individual monitoring. Our results provide evidence that senescence in antler size might be widespread across deer species irrespective of the intensity of sexual selection. Thus, despite a modest sexual size dimorphism and a low level of polygyny, roe deer males cannot maintain the same allocation to antlers throughout their entire lifetime.
Our present analysis shows that the onset of antler length senescence takes place at about 7 years of age in roe deer, supporting Vanpé et al.’s study in which this onset was fixed a priori based on the onset of actuarial senescence. Our study thus indicates that senescence in antler size (this study) and actuarial senescence (Gaillard et al., 1993; Loison et al., 1999) are indeed synchronous in roe deer males, supporting the hypothesis that antler size is an honest signal of male phenotypic quality (Vanpé et al., 2007), at least when resources are limiting. In addition, given that male reproductive success declines with increasing age from 8 years onwards (Vanpé et al., 2009), our results suggest that senescence in antler size is one possible proximate mechanism driving impaired reproductive success among old males. Indeed, phenotypic traits such as the size of secondary characters might serve as an indicator of fertility (“phenotype-linked fertility hypothesis”, Sheldon, 1994), as observed in red deer where males with the longest antlers produce a higher quantity of sperm, as well as more rapid sperm (Malo et al., 2005). It would now be particularly interesting to analyse patterns of senescence in male fertility measured by various ejaculate-related traits to test the hypothesis that patterns of senescence for pre- and post-copulatory traits are aligned.
Interestingly, the allocation to antler size was substantially larger (>10%) at a given age at Trois Fontaines compared to Chizé. This pattern is likely explained by differences in the environmental context between populations. Environments with abundant resources, such as at Trois-Fontaines, should allow roe deer males to grow longer antlers (this study), develop higher body mass (Hewison et al., 2011) and attain better physiological performance (e.g. immunocompetence, Cheynel et al., 2017) compared to environments with more limited resources such as Chizé. Our results thus suggest that males adjust the life course of their antler size to available resources. In particular, old males in favourable environments allocate more resources in absolute terms to the development and maintenance of their secondary sexual traits than they do in less suitable environments. Whether environmental conditions might explain the lack of senescence in antler size in some populations of red deer (Nussey et al., 2009), of sika deer (Cervus nippon, Hayden et al., 1994) or of black-tailed deer (Odocoileus hemionus, Thalmann et al., 2015) is yet to be investigated, despite the potential confounding effect of the management practices (e.g., hunting selection and pressure) in some populations. Importantly, patterns of senescence may be asynchronous among antler traits (see Table 1, e.g. Smith et al., 2021).
Natal environmental conditions modulate senescence patterns in antler length
The impact of early-life conditions on male secondary sexual traits has previously been well documented, with good natal conditions generally improving the size of the trait during adulthood (e.g. in zebra finch, Taeniopygia castanotis: beak color and cheek patch size in Wilson et al., 2019; plumage ornaments in Naguib and Nemitz, 2007), but their impact on the senescence of secondary sexual traits had not yet been studied. Our results suggest that natal environmental conditions modulate patterns of senescence in antler length in both populations, although, notably, these senescence trajectories were mostly driven by the oldest males from Chizé. For poor-quality cohorts, a marked decrease in both absolute and relative (to body mass) antler length occurred from 7 years of age onwards (see Figure 2). That this pattern was not fully supported on statistical grounds comes from the low sample size of males older than 10 years of age, with only one individual at 12 and one at 13 years of age. In contrast, the decrease in both absolute and relative antler length with increasing age occurred somewhat later in high-quality cohorts, from 9.5 years onwards. Note, however, that this decrease was mostly driven by one old male with very small antlers at Chizé, leading the exact age of the onset of antler senescence of males born under favourable conditions to be difficult to determine. As in Trois-Fontaines, the very small sample size of animals older than 10 years of age prevented us to draw firm conclusions regarding the onset of antler length senescence in high-quality cohorts. According to a preliminary analysis (Tables S8, S9, Figure S1), the timing of velvet shedding seems to be delayed with age, while the opposite relationship has been documented in other cervids (e.g. Gómez et al., 2022 in red deer). In our study, this may partly explain the low number of old males (> 10 years of age) in our dataset. We cannot, therefore, conclude that senescence in antler length did actually occur for high-quality cohorts, although it appears that poor natal conditions lead to earlier age at onset of antler length senescence.
Natal conditions in roe deer strongly influence phenotypic traits such as body mass or jaw length (Hewison et al., 1996; Hewison et al., 2002; Pettorelli et al., 2002; Kjellander et al., 2006). The delayed senescence in antler length we report in males from high-quality cohorts suggests that favourable natal conditions also promote the expression of secondary sexual traits later in life, so that mature males are able to continue to allocate a lot to antler growth, even when old (i.e. > 7 years). Under the assumption that antler length reflects mating success (e.g. through the acquisition and maintenance of a high-quality territory) and fertilisation success (see above, Vanpé et al. 2010), the later onset of senescence in antler length for males born from high-quality cohorts might indicate a later onset of senescence in terms of reproductive success.
In contrast, males that experience limiting conditions in early life may suffer from earlier senescence in antler length as a result (see Figure 2), both in absolute and relative (to body mass) terms. The observed decrease in relative antler length with increasing age suggests that once senescence has started, males allocate less energy to antler growth for a given mass. As described above, this could either reflect the inability of old males to maintain their level of allocation to antler growth because of their poor physiological condition, or a tactic favouring the allocation of resources towards other traits in late life (e.g. gamete quality, somatic maintenance) (Maklakov and Immler, 2016). Finally, it would be valuable to study senescence in other antler characteristics (e.g. coronet circumference, casting date) which could potentially show a higher sensitivity to environmental conditions than antler length (Michel et al, 2016).
Conclusions
Our study demonstrates that even in a weakly dimorphic species such as roe deer, antlers remain honest signals of individual quality and are sensitive to early-life environmental conditions. Based on our results, adverse natal conditions induce earlier and stronger antler length senescence in roe deer, which could potentially influence female choice and male–male competition, and as a result reduce mating success among old males. Antler length is an honest signal of individual quality in roe deer (Vanpé et al., 2007), and a possible indicator of fertilisation capacity in adults, because antler size correlates with testes size and sperm velocity, as shown by Wahlström (1994) in yearling roe deer and by Malo et al. (2005) in adult red deer. Therefore, we suggest that females should prefer mating with males with longer antlers (as in white-tailed deer: Morina et al., 2018), and should mostly avoid mating with old males to limit the fitness costs of reproductive senescence in their partner. The results of Vanpé et al.’s (2019) study on female mating tactics in roe deer are in line with this assumption: old females seem to avoid mating with old males, contradicting the long-held view that old males should be preferred by females (see Johnson and Gemmell, 2012 for a review).
Data availability statement
The original contributions presented in the study are included in the article/Supplementary Material. Further inquiries can be directed to the corresponding author.
Ethics statement
The animal study was reviewed and approved by Director of Food, Agriculture and Forest. The protocol of capture and blood sampling under the authority of the Office Français de la Biodiversité (OFB) was approved by the Director of Food, Agriculture and Forest (Prefectoral order 2009–14 from Paris). The land manager of both sites, the Office National des Forêts (ONF), permitted the study of the populations (Partnership Convention ONCFS-ONF dated 2005-12-23). All experiments were performed in accordance with guidelines and regulations of the Ethical Committee of Lyon 1 University (project DR2014-09, June 5, 2014).
Author contributions
SC, J-MG, and J-FL designed the study with input from AJMH. SC, J-MG, CV, RG, FD, DD, MP and J-FL collected the data. SC analysed the data. SC wrote the first draft with inputs from J-MG, AJMH, and J-FL. All authors contributed to the article and approved the submitted version.
Funding
SC is supported by a grant from by the French Ministry of Education and Research. This research has been supported by ANR research programs DivInT and EVORA.
Acknowledgments
We thank all the staff from the Office Français de la Biodiversité (OFB), in particular Gilles Capron, Hervé Bidault, Claude Warnant, Stéphane Chabot and the field volunteers for organising the roe deer captures. We are also grateful to four reviewers for insightful comments on a previous draft of this work.
Conflict of interest
The authors declare that the research was conducted in the absence of any commercial or financial relationships that could be construed as a potential conflict of interest.
Publisher’s note
All claims expressed in this article are solely those of the authors and do not necessarily represent those of their affiliated organizations, or those of the publisher, the editors and the reviewers. Any product that may be evaluated in this article, or claim that may be made by its manufacturer, is not guaranteed or endorsed by the publisher.
Supplementary material
The Supplementary Material for this article can be found online at: https://www.frontiersin.org/articles/10.3389/fevo.2023.1139235/full#supplementary-material
References
Agence nationale des chasseurs de grand gibier (2005). Available at: https://www.ancgg.org/AD51/documents/rubd12_4116.pdf.
Andersen R., Gaillard J. M., Linnell J. D., Duncan P. (2000). Factors affecting maternal care in an income breeder, the European roe deer. J. Anim. Ecol. 69 (4), 672–682. doi: 10.1046/j.1365-2656.2000.00425.x
Balbontín J., Møller A. P., Hermosell I. G., Marzal A., Reviriego M., De Lope F. (2012). Geographical variation in reproductive ageing patterns and life-history strategy of a short-lived passerine bird. J. Evolutionary Biol. 25 (11), 2298–2309. doi: 10.1111/j.1420-9101.2012.02606.x
Bates D., Mächler M., Bolker B., Walker S. (2015). Fitting linear mixed-effects models using lme4. J. Stat. Softw. 67(1), 1–48. Available at: http://arxiv.org/abs/1406.5823.
Baxter B. J., Andrews R. N., Barrell G. K. (1999). Bone turnover associated with antler growth in red deer (Cervus elaphus). Anatomical Rec. 256 (1), 14–19. doi: 10.1002/(SICI)1097-0185(19990901)256:1<14::AID-AR3>3.0.CO;2-A
Berglund A., Bisazza A., Pilastro A. (1996). Armaments and ornaments: an evolutionary explanation of traits of dual utility. Biol. J. Linn. Soc. 58 (4), 385–399. doi: 10.1111/j.1095-8312.1996.tb01442.x
Bowyer R. T., Stewart K. M., Kie J. G., Gasaway W. C. (2001). Fluctuating asymmetry in antlers of alaskan moose: size matters. J. Mammalogy 82 (3), 814–824. doi: 10.1644/1545-1542(2001)082<0814:FAIAOA>2.0.CO;2
Burnham K. P., Anderson D. R. (2004). Multimodel inference: understanding AIC and BIC in model selection. Sociological Methods Res. 33 (2), 261–304. doi: 10.1177/0049124104268644
Cayuela H., Lemaître J. F., Muths E., McCaffery R. M., Frétey T., Le Garff B., et al. (2021). Thermal conditions predict intraspecific variation in senescence rate in frogs and toads. Proc. Natl. Acad. Sci. 118 (49), e2112235118. doi: 10.1073/pnas.2112235118
Cheynel L., Lemaître J. F., Gaillard J. M., Rey B., Bourgoin G., Ferté H., et al. (2017). Immunosenescence patterns differ between populations but not between sexes in a long-lived mammal. Sci. Rep. 7 (1), 1–11. doi: 10.1038/s41598-017-13686-5
Clements M. N., Clutton-Brock T. H., Albon S. D., Pemberton J. M., Kruuk L. E. B. (2010). Getting the timing right: antler growth phenology and sexual selection in a wild red deer population. Oecologia 164 (2), 357−368. doi: 10.1007/s00442-010-1656-7
Clutton-Brock T. H. (1982). The functions of antlers. Behaviour 79 (2/4), 108–125. doi: 10.1163/156853982X00201
Cooper E. B., Kruuk L. E. B. (2018). Ageing with a silver-spoon: a meta-analysis of the effect of developmental environment on senescence. Evol. Lett. 2 (5), 460–471. doi: 10.1002/evl3.79
Cotton S., Fowler K., Pomiankowski A. (2004). Condition dependence of sexual ornament size and variation in the stalk-eyed fly Cyrtodiopsis dalmanni (diptera: diopsidae). Evolution 58 (5), 1038–1046. doi: 10.1111/j.0014-3820.2004.tb00437.x
Dakin R., Montgomerie R. (2013). Eye for an eyespot: how iridescent plumage ocelli influence peacock mating success. Behav. Ecol. 24 (5), 1048–1057. doi: 10.1093/beheco/art045
Douhard M., Loe L. E., Stien A., Bonenfant C., Irvine R. J., Veiberg V., et al. (2016). The influence of weather conditions during gestation on life histories in a wild Arctic ungulate. Proc. R. Soc. B: Biol. Sci. 283 (1841), 20161760. doi: 10.1098/rspb.2016.1760
Drechsler H. (1980). Ueber die geweihbildung by rothirschen in rotwildring harz in den jahren 1959-1978. Z. für Jagdwissenschaft 26 (4), 207–219. doi: 10.1007/BF02241635
Dugdale H. L., Pope L. C., Newman C., Macdonald D. W., Burke T. (2011). Age-specific breeding success in a wild mammalian population: selection, constraint, restraint and senescence. Mol. Ecol. 20 (15), 3261−3274. doi: 10.1111/j.1365-294X.2011.05167.x
Ericsson G., Wallin K., Ball J. P., Broberg M. (2001). Age-related reproductive effort and senescence in free-ranging moose, alces alces. Ecology 82, 1613–1620. doi: 10.1890/0012-9658(2001)082[1613:ARREAS]2.0.CO;2
Forstmeier W., Hasselquist D., Bensch S., Leisler B. (2006). Does song reflect age and viability? a comparison between two populations of the great reed warbler Acrocephalus arundinaceus. Behav. Ecol. Sociobiology 59 (5), 634–643. doi: 10.1007/s00265-005-0090-z
Gage M. J., Macfarlane C. P., Yeates S., Ward R. G., Searle J. B., Parker G. A. (2004). Spermatozoal traits and sperm competition in atlantic salmon: relative sperm velocity is the primary determinant of fertilization success. Curr. Biol. 14 (1), 44–47. doi: 10.1016/j.cub.2003.12.028
Gaillard J. M., Delorme D., Jullien J. M. (1993). Effects of cohort, sex, and birth date on body development of roe deer (Capreolus capreolus) fawns. Oecologia 94 (1), 57–61. doi: 10.1007/BF00317301
Gaillard J.-M., Lemaître J.-F. (2020). An integrative view of senescence in nature. Funct. Ecol. 34 (1), 4–16. doi: 10.1111/1365-2435.13506
Gauthier D. A., Larsen D. G. (1985). Geographic variation in moose antler characteristics, Yukon. Alces: A J. Devoted to Biol. Manage. Moose 21, 91–101.
Geist V. (1987). “On the evolution of optical signals in deer: a preliminary analysis,” in Biology and management of the cervidae. Ed. Wemmer C. (Washington: Smithsonian Institution Press), pp 235–pp 255.
Geist V. (1998). Deer of the world: their evolution, behaviour, and ecology Vol. 272 (Mechanicsburg, Pennsylvania: Stackpole books).
Gómez J.Á., Pérez-Barbería J., García A. J., Cappelli J., Chonco L., Ceacero F., et al. (2022). Factors affecting antler growth period and casting date in red deer. J. Mammalogy 103 (1), 169–177. doi: 10.1093/jmammal/gyab097
Goss R. J. (1970). Section III basic sciences and pathology 24, problems of antlerogenesis. Clin. Orthopaedics Related Res. 69, 227–238. doi: 10.1097/00003086-197003000-00025
Gould S. J. (1974). The origin and function of'bizarre'structures: antler size and skull size in the'Irish Elk,'Megaloceros giganteus. Evolution 28 (2), 191–220. doi: 10.2307/2407322
Hayden T. J., Lynch J. M., O'Corry-Crowe G. (1994). Antler growth and morphology in a feral sika deer (Cervus nippon) population in killarney, Ireland. J. Zoology 232 (1), 21−35. doi: 10.1111/j.1469-7998.1994.tb01557
Hewison A. M., Gaillard J. M., Delorme D., Van Laere G., Amblard T., Klein F. (2011). Reproductive constraints, not environmental conditions, shape the ontogeny of sex-specific mass–size allometry in roe deer. Oikos 120, 1217–1226. doi: 10.1111/j.1600-0706.2011.19316.x
Hewison A. J. M., Vincent J. P., Bideau E., Angibault J. M., Putman R. J. (1996). Variation in cohort mandible size as an index of roe deer (Capredus capreolus) densities and population trends. J. Zoology 239 (3), 573–581. doi: 10.1111/j.1469-7998.1996.tb05943.x
Hewison A. J. M., Gaillard J. M., Angibault J. M., Van Laere G., Vincent J. P. (2002). The influence of density on post-weaning growth in roe deer Capreolus capreolus fawns. J. Zoology 257 (3), 303–309.
Hoem S. A., Melis C., Linnell J. D., Andersen R. (2007). Fighting behaviour in territorial male roe deer capreolus capreolus: the effects of antler size and residence. Eur. J. Wildlife Res. 53 (1), 1–8. doi: 10.1007/s10344-006-0053-3
Holand H. A., Kvalnes T., Gamelon M., Tufto J., Jensen H., Pärn H., et al. (2016). Spatial variation in senescence rates in a bird metapopulation. Oecologia 181 (3), 865–871. doi: 10.1007/s00442-016-3615-4
Huxley J. S. (1931). The relative size of antlers in deer. Proc. Zoological Soc. London 101 (3), 819–864. doi: 10.1111/j.1096-3642.1931.tb01047.x
Jacob L., Douhard F., Lemaıtre J. F., Gaillard J. M., Siberchicot A. (2017) OnAge: test of between-group differences in the onset of senescence. Available at: https://cran.biodisk.org/web/packages/OnAge.
Johansson A. (1996). Territory establishment and antler cycle in male roe deer. Ethology 102 (4), 549–559. doi: 10.1111/j.1439-0310.1996.tb01147.x
Johnson S. L., Gemmell N. J. (2012). Are old males still good males and can females tell the difference? do hidden advantages of mating with old males off-set costs related to fertility, or are we missing something else? Bioessays 34 (7), 609–619. doi: 10.1002/bies.201100157
Kjellander P., Gaillard J. M., Hewison A. M. (2006). Density-dependent responses of fawn cohort body mass in two contrasting roe deer populations. Oecologia 146, 521–530. doi: 10.1007/s00442-005-0188-z
Kodric-Brown A., Sibly R. M., Brown J. H. (2006). The allometry of ornaments and weapons. Proc. Natl. Acad. Sci. 103 (23), 8733–8738. doi: 10.1073/pnas.0602994103
Kruuk L. E., Slate J., Pemberton J. M., Brotherstone S., Guinness F., Clutton-Brock T. (2002). Antler size in red deer: heritability and selection but no evolution. Evolution 56 (8), 1683–1695. doi: 10.1111/j.0014-3820.2002.tb01480.x
Lemaître J. F., Cheynel L., Douhard F., Bourgoin G., Débias F., Ferté H., et al. (2018). The influence of early-life allocation to antlers on male performance during adulthood: evidence from contrasted populations of a large herbivore. J. Anim. Ecol. 87 (4), 921–932. doi: 10.1111/1365-2656.12833
Lemaître J.-F., Gaillard J.-M. (2017). Reproductive senescence: new perspectives in the wild. Biol. Rev. 92 (4), 2182–2199. doi: 10.1111/brv.12328
Lemaître J. F., Gaillard J. M., Pemberton J. M., Clutton-Brock T. H., Nussey D. H. (2014b). Early life expenditure in sexual competition is associated with increased reproductive senescence in male red deer. Proc. R. Soc. B: Biol. Sci. 281 (1792), 20140792. doi: 10.1098/rspb.2014.0792
Lemaître J.-F., Ronget V., Gaillard J.-M. (2020a). Female reproductive senescence across mammals: a high diversity of patterns modulated by life history and mating traits. Mech. Ageing Dev. 192, 111377. doi: 10.1016/j.mad.2020.111377
Lemaître J. F., Ronget V., Tidière M., Allainé D., Berger V., Cohas A., et al. (2020b). Sex differences in adult lifespan and aging rates of mortality across wild mammals. Proc. Natl. Acad. Sci. 117 (15), 8546–8553. doi: 10.1073/pnas.1911999117
Lemaître J. F., Vanpé C., Plard F., Gaillard J. M. (2014a). The allometry between secondary sexual traits and body size is nonlinear among cervids. Biol. Lett. 10 (3), 20130869. doi: 10.1098/rsbl.2013.0869
Loison A., Festa-Bianchet M., Gaillard J. M., Jorgenson J. T., Jullien J. M. (1999). Age-specific survival in five populations of ungulates: evidence of senescence. Ecology 80 (8), 2539−2554. doi: 10.1890/0012-9658(1999)080[2539:ASSIFP]2.0.CO;2
Lopez-Idiaquez D., Vergara P., Fargallo J. A., Martinez-Padilla J. (2016). Old males reduce melanin-pigmented traits and increase reproductive outcome under worse environmental conditions in common kestrels. Ecol. Evol. 6 (4), 1224–1235. doi: 10.1002/ece3.1910
Maklakov A. A., Immler S. (2016). The expensive germline and the evolution of ageing. Curr. Biol. 26 (13), R577–R586. doi: 10.1016/j.cub.2016.04.012
Malo A. F., Garde J. J., Soler A. J., García A. J., Gomendio M., Roldan E. R. (2005). Male Fertility in natural populations of red deer is determined by sperm velocity and the proportion of normal spermatozoa. Biol. Reprod. 72 (4), 822–829. doi: 10.1095/biolreprod.104.036368
Markussen S. S., Herfindal I., Loison A., Solberg E. J., Haanes H., Røed K. H., et al. (2019). Determinants of age at first reproduction and lifetime breeding success revealed by full paternity assignment in a male ungulate. Oikos 128 (3), 328–337. doi: 10.1111/oik.05494
Mattioli S., Ferretti F., Nicoloso S., Corlatti L. (2021). Spatial variation in antler investment of apennine red deer. Ecol. Evol. 11 (12), 7850–7864. doi: 10.1002/ece3.7617
McAdam A. G., Boutin S., Sykes A. K., Humphries M. M. (2007). Life histories of female red squirrels and their contributions to population growth and lifetime fitness. Ecoscience 14 (3), 362. doi: 10.2980/1195-6860(2007)14[362:LHOFRS]2.0.CO;2
McCorquodale S. M., Eberhardt L. E., Sargeant G. A. (1989). Antler characteristics in a colonizing elk population. J. Wildlife Manage. 53 (3), 618–621. doi: 10.2307/3809185
McElligott A. G., Altwegg R., Hayden T. J. (2002). Age-specific survival and reproductive probabilities: evidence for senescence in male fallow deer (Dama dama). Proc. R. Soc. London. Ser. B: Biol. Sci. 269 (1496), 1129–1137. doi: 10.1098/rspb.2002.1993
Michel E. S., Demarais S., Strickland B. K., Smith T., Dacus C. M. (2016). Antler characteristics are highly heritable but influenced by maternal factors. J. Wildlife Manage. 80 (8), 1420–1426. doi: 10.1002/jwmg.21138
Møller A. P., De Lope F. (1999). Senescence in a short-lived migratory bird: age-dependent morphology, migration, reproduction and parasitism. J. Anim. Ecol. 68 (1), 163–171. doi: 10.1046/j.1365-2656.1999.00274.x
Monaghan P., Charmantier A., Nussey D. H., Ricklefs R. E. (2008). The evolutionary ecology of senescence. Funct. Ecol. 22 (3), 371–378. doi: 10.1111/j.1365-2435.2008.01418.x
Morina D. L., Demarais S., Strickland B. K., Larson J. E. (2018). While males fight, females choose: male phenotypic quality informs female mate choice in mammals. Anim. Behav. 138, 69–74. doi: 10.1016/j.anbehav.2018.02.004
Mumby H. S., Mar K. U., Hayward A. D., Htut W., Htut-Aung Y., Lummaa V. (2015). Elephants born in the high stress season have faster reproductive ageing. Sci. Rep. 5 (1), 13946. doi: 10.1038/srep13946
Mysterud A., Meisingset E., Langvatn R., Yoccoz N. G., Stenseth N. C. (2005). Climate-dependent allocation of ressources to secondary sexual traits in red deer. Oikos 111, 245–252. doi: 10.1111/j.0030-1299.2005.14197.x
Naguib M., Nemitz A. (2007). Living with the past: nutritional stress in juvenile males has immediate effects on their plumage ornaments and on adult attractiveness in zebra finches. PloS One 2 (9), e901. doi: 10.1371/journal.pone.0000901
Nie H., Song Y., Zeng Z., Zhang Q. (2011). Life history pattern and fitness of an endangered hainan eld's deer population. Integr. Zoology 6 (1), 63–70. doi: 10.1111/j.1749-4877.2010.00232.x
Nussey D. H., Coulson T., Festa-Bianchet M., Gaillard J. M. (2013). Senescence in natural populations of animals: widespread evidence and its implications for bio-gerontology. Ageing Res. Rev. 12 (1), 214–225. doi: 10.1016/j.arr.2012.07.004
Nussey D. H., Coulson T., Festa-Bianchet M., Gaillard J. M.. (2008). Measuring senescence in wild animal populations: towards a longitudinal approach. Funct. Ecol. 22 (3), 393–406. doi: 10.1111/j.1365-2435.2008.01408.x
Nussey D. H., Kruuk L. E., Morris A., Clements M. N., Pemberton J. M., Clutton-Brock T. H. (2009). Inter-and intrasexual variation in aging patterns across reproductive traits in a wild red deer population. Am. Nat. 174 (3), 342–357. doi: 10.1086/603615
Paternoster R., Brame R., Mazerolle P., Piquero A. (1998). Using the correct statistical test for the equality of regression coefficients. Criminology 36 (4), 859–866. doi: 10.1111/j.1745-9125.1998.tb01268.x
Pettorelli N., Gaillard J. M., Mysterud A., Duncan P., Stenseth N., Delorme D., et al. (2006). Using a proxy of plant productivity (NDVI) to find key periods for animal performance: the case of roe deer. Oikos 112 (3), 565–572. doi: 10.1111/j.0030-1299.2006.14447.x
Pettorelli N., Gaillard J. M., Van Laere G., Duncan P., Kjellander P., Liberg O., et al. (2002). Variations in adult body mass in roe deer: the effects of population density at birth and of habitat quality. Proc. R. Soc. London. Ser. B: Biol. Sci. 269 (1492), 747−753. doi: 10.1098/rspb.2001.1791
Plard F., Bonenfant C., Gaillard J. M. (2011). Revisiting the allometry of antlers among deer species: male–male sexual competition as a driver. Oikos 120 (4), 601–606. doi: 10.1111/j.1600-0706.2010.18934.x
R Development Core Team (2015). R: a language and environment for statistical computing (Vienna: R Foundation for Statistical Computing). Available at: https://www.Rproject.org/.
Saether B.-E., Haagenrud H. (1985). Geographical variation in the antlers of norwegian moose in relation to age and size. J. Wildlife Manage. 49 (4), 983–986. doi: 10.2307/3801383
Schulte-Hostedde A. I., Millar J. S. (2004). Intraspecific variation of testis size and sperm length in the yellow-pine chipmunk (Tamias amoenus): implications for sperm competition and reproductive success. Behav. Ecol. Sociobiology 55 (3), 272–277. doi: 10.1007/s00265-003-0707-z
Sempéré A. J. (1990). “The annual antler cycle of the European roe deer (Capreolus capreolus) in relation to the reproductive cycle,” in Horns, pronghorns, and antlers. Eds. Bubenik G. A., Bubenik A. B. (New York: Springer), 396–415. doi: 10.1530/jrf.0.0950693
Sempéré A. J., Mauget R., Bubenik G. A. (1992). Influence of photoperiod on the seasonal pattern of secretion of luteinizing hormone and testosterone and on the antler cycle in roe deer (Capreolus capreolus). Reproduction 95 (3), 693–700.
Sheldon B. C. (1994). Male Phenotype, fertility, and the pursuit of extra-pair copulations by female birds. Proc. R. Soc B 257, 25–30. doi: 10.1098/rspb.1994.0089
Smith A. F., Bongi P., Ciuti S. (2021). Remote, non-invasive photogrammetry for measuring physical traits in wildlife. J. Zoology 313 (4), 250–262. doi: 10.1111/jzo.12858
Smolko P., Garaj P., Lebocký T., Bútora Ľ., Pataky T., Jaňáková Z., et al. (2022). Soil nutrients and deer density affect antler size of the Carpathian red deer. Mamm. Biol. 102, 119–130. doi: 10.1007/s42991-021-00203-5
Stewart K. M., Bowyer R. T., Kie J. G., Gasaway W. C. (2000). Antler size relative to body mass in moose: tradeoffs associated with reproduction. Alces: A J. Devoted to Biol. Manage. Moose 36, 77–83.
Taber R. D., Dasmann R. F. (1957). The dynamics of three natural populations of the deer odocoileus hemionus columbianus. Ecology 38 (2), 233–246. doi: 10.2307/1931682
Thalmann J. C., Bowyer R. T., Aho K. A., Weckerly F. W., McCullough D. R. (2015). Antler and body size in black-tailed deer: an analysis of cohort effects. Adv. Ecol. doi: 10.1155/2015/156041
Toïgo C., Gaillard J. M., Van Laere G., Hewison M., Morellet N. (2006). How does environmental variation influence body mass, body size, and body condition? Roe deer as Case study. Ecography 29 (3), 301–308. doi: 10.1111/j.2006.0906-7590.04394.x
Vágási C. I., Vincze O., Lemaître J. F., Pap P. L., Ronget V., Gaillard J. M. (2021). Is degree of sociality associated with reproductive senescence? a comparative analysis across birds and mammals. Philos. Trans. R. Soc. B 376 (1823). doi: 10.1098/rstb.2019.0744
Van de Pol M., Verhulst S. (2006). Age-dependent traits: a new statistical model to separate within-and between-individual effects. Am. Nat. 167 (5), 766–773. doi: 10.1086/503331
Vanpé C., Gaillard J. M., Hewison A. M., Quéméré E., Kjellander P., Pellerin M., et al. (2019). Old females rarely mate with old males in roe deer, Capreolus capreolus. Biol. J. Linn. Soc. 128 (3), 515–525. doi: 10.1093/biolinnean/blz110
Vanpé C., Gaillard J. M., Kjellander P., Mysterud A., Magnien P., Delorme D., et al. (2007). Antler size provides an honest signal of male phenotypic quality in roe deer. Am. Nat. 169 (4), 481–493. doi: 10.1086/512046
Vanpé C., Gaillard J. M., Kjellander P., Liberg O., Delorme D., Hewison A. M. (2010). Assessing the intensity of sexual selection on male body mass and antler length in roe deer capreolus capreolus: is bigger better in a weakly dimorphic species? Oikos 119 (9), 1484–1492. doi: 10.1111/j.1600-0706.2010.18312.x
Vanpé C., Gaillard J. M., Morellet N., Kjellander P., Liberg O., Delorme D., et al. (2009). Age-specific variation in male breeding success of a territorial ungulate species, the european roe deer. J. Mammalogy 90 (3), 661–665. doi: 10.1644/08-MAMM-A-137R.1
Vanpé C., Kjellander P., Galan M., Cosson J. F., Aulagnier S., Liberg O., et al. (2008). Mating system, sexual dimorphism, and the opportunity for sexual selection in a territorial ungulate. Behav. Ecol. 19 (2), 309–316. doi: 10.1093/beheco/arm132
Vergara P., Mougeot F., Martínez-Padilla J., Leckie F., Redpath S. M. (2012). The condition dependence of a secondary sexual trait is stronger under high parasite infection level. Behav. Ecol. 23 (3), 502–511. doi: 10.1093/beheco/arr216
Von Hardenberg A., Bassano B., Arranz M. D. P. Z., Bogliani G. (2004). Horn growth but not asymmetry heralds the onset of senescence in male alpine ibex (Capra ibex). J. Zoology 263 (4), 425–432. doi: 10.1017/S0952836904005485
Wahlström L. K. (1994). The significance of male-male aggression for yearling dispersal in roe deer (Capreolus capreolus). Behav. Ecol. Sociobiology 35 (6), 409–412. doi: 10.1007/BF00165843
Widdig A., Bercovitch F. B., Jürgen Streich W., Sauermann U., Nürnberg P., Krawczak M. (2004). A longitudinal analysis of reproductive skew in male rhesus macaques. Proc. R. Soc. London. Ser. B: Biol. Sci. 271 (1541), 819−826. doi: 10.1098/rspb.2003.2666
Keywords: Capreolus capreolus, reproductive ageing, secondary sexual traits, sexual selection, weapon
Citation: Cambreling S, Gaillard J-M, Pellerin M, Vanpé C, Débias F, Delorme D, Garcia R, Hewison AJM and Lemaître J-F (2023) Natal environmental conditions modulate senescence of antler length in roe deer. Front. Ecol. Evol. 11:1139235. doi: 10.3389/fevo.2023.1139235
Received: 06 January 2023; Accepted: 09 June 2023;
Published: 05 July 2023.
Edited by:
Ann Valerie Hedrick, University of California, Davis, United StatesReviewed by:
Adam Hayward, Moredun Research Institute, United KingdomStefano Mattioli, University of Siena, Italy
Aaron Foley, Texas A&M University Kingsville, United States
Copyright © 2023 Cambreling, Gaillard, Pellerin, Vanpé, Débias, Delorme, Garcia, Hewison and Lemaître. This is an open-access article distributed under the terms of the Creative Commons Attribution License (CC BY). The use, distribution or reproduction in other forums is permitted, provided the original author(s) and the copyright owner(s) are credited and that the original publication in this journal is cited, in accordance with accepted academic practice. No use, distribution or reproduction is permitted which does not comply with these terms.
*Correspondence: Solène Cambreling, c29sZW5lLmNhbWJyZWxpbmdAdW5pdi1seW9uMS5mcg==