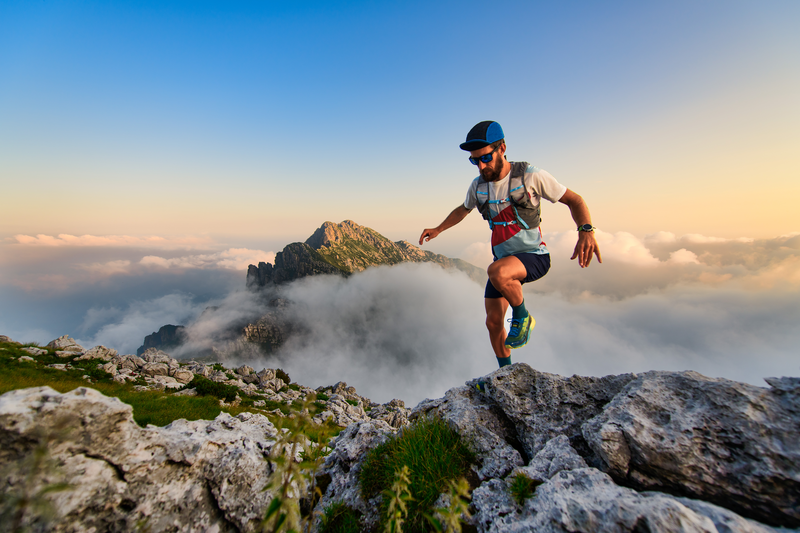
94% of researchers rate our articles as excellent or good
Learn more about the work of our research integrity team to safeguard the quality of each article we publish.
Find out more
ORIGINAL RESEARCH article
Front. Ecol. Evol. , 30 March 2023
Sec. Behavioral and Evolutionary Ecology
Volume 11 - 2023 | https://doi.org/10.3389/fevo.2023.1135924
This article is part of the Research Topic Aquatic Insect Ecology in a Changing World View all 6 articles
Freshwater salinity varies in natural systems and plays a role in species distribution. Anthropogenic alterations to freshwater salinity regimes include sea level rise and subsequent intrusion of saline waters to inland habitats. While mayflies are generalized to be sensitive to increasing salinity, we still know remarkably little about the physiological processes (and their plasticity) that determine the performance of species in a changing world. Here, we explored life-history outcomes and physiological plasticity in a population of Callibaetis floridanus (Ephemeroptera: Baetidae) from a coastal pond that routinely experiences saltwater intrusion. We reared naiads from egg hatch to adulthood across a gradient of increasing salinities (113, 5,020, 9,921 μS/cm). Radiotracer flux studies (22Na, 35SO4, and 45Ca) were conducted in naiads reared at each salinity, revealing a positive association between ionic concentration and uptake rates. However, the influence of rearing history on ionic influx rates was apparent when naiads were transferred from their respective rearing water to the other experimental conditions. For example, we observed that naiads reared in the low salinity treatment (113 μS/cm) had 10.8-fold higher Na uptake rates than naiads reared at 9,921 μS/cm and transferred to 113 μS/cm. Additionally, naiads acclimated to the higher salinity water exhibited reduced uptake in ion-rich water relative to those reared in more dilute conditions (e.g., in 9,921 μS/cm water, 113 and 5,020 μS/cm acclimated naiads had 1.5- and 1.1-fold higher Na uptake rates than 9,921 μS/cm acclimated naiads, respectively). We found no significant changes in survival (80 ± 4.4%, mean ± s.e.m.) or naiad development time (24 ± 0.3 days, mean ± s.e.m.) across these treatments but did observe a 27% decrease in subimago female body weight in the most dilute condition. This reduction in female weight was associated with higher oxygen consumption rates in naiads relative to the other rearing conditions. Collectively, these data suggests that saline adapted C. floridanus may be more energetically challenged in dilute conditions, which differs from previous observations in other mayfly species.
Freshwater salinity varies greatly globally (Cañedo-Argüelles et al., 2013; Iglesias, 2020) and plays a role in determining where species can survive (Cormier and Suter, 2013; Olson and Hawkins, 2017). As anthropogenic activities (e.g., road de-icing and mountaintop coal mining) lead to further salinization, there are growing concerns about the impact on biodiversity in these systems (Pond et al., 2008; Entrekin et al., 2011; Kunz et al., 2013; Jackson and Funk, 2019). Saltwater intrusion is specifically a concern in costal freshwater systems, where climate change is contributing to sea-level rise (Barlow and Reichard, 2010; Mastrocicco et al., 2019). Sensitive species such as aquatic insects can be impacted by such changes (Pond et al., 2008; Beermann et al., 2018; Kefford, 2019), as the vast majority of aquatic insects occur in relatively dilute freshwater habitats, and are relatively rare in more saline habitats (Maddrell, 1998; Bradley, 2013).
Aquatic insects generally, and mayflies more specifically, can be sensitive to increases in salinity (Pond et al., 2008; Beermann et al., 2018; Kefford, 2019). However, we still know remarkably little about the physiological processes that determine the performance of species in a changing world. In nature, mayfly species have been extirpated from salinized streams associated with mountaintop coal mining (Pond et al., 2008). However, a few aquatic insect species have overcome the apparent physiological barriers to invading saline habitats. Examples of this include Halobates sp. Eschscholtz, 1822 (Sekimoto et al., 2014), brine flies (Shimizu and Bradley, 1994; Herbst, 1999, 2001), certain species of water boatmen (Scudder, 1976; Bradley et al., 2022), and mosquitoes (Bradley, 1987), and the euryhaline caddisfly Limnephilus affinis Curtis, 1834 (Sutcliffe, 1960). There is further evolutionary evidence for the successful invasion of mosquitoes of more saline habitats by freshwater-adapted ancestors (Bradley, 2013). It appears that more holometabolous lineages have been successful in invading and adapting to saline environments relative to hemimetabolous species, though we are not aware that this has been specifically addressed. Salt tolerance in hemimetabolous groups such as mayflies is exceedingly rare.
Previous work has demonstrated some physiological plasticity in the stenohaline mayfly, Neocloeon triangulifer McDunnough, 1931 (Orr et al., 2021; Cochran and Buchwalter, 2022). Naiads reared in water with elevated major ion concentrations were able to decrease sodium and sulfate uptake rates relative to naive naiads (Orr et al., 2021). This suggests that acclimated naiads can evade excessive ion uptake in saltier waters, which may limit their energetic costs of osmoregulation. Further, naiads reared across a gradient of decreasing Na concentrations had no significant changes in survival, growth, development time and whole-body Na content across these treatments (Cochran and Buchwalter, 2022). Naiads acclimated to their dilute exposures by increasing their rates of Na uptake and were able to maintain a relatively narrow range of uptake rates across all treatments (contrasting the concentration-dependent Na uptake rates observed in naive naiads). Comparing these two studies suggests that N. triangulifer has a physiological preference for dilute environments, relative to ion-rich environments (Cochran and Buchwalter, 2022). However, it is unknown how broadly these observations apply to other taxa, especially more euryhaline species.
The baetid mayfly Callibaetis floridanus Banks, 1900 is known to tolerate a wide range of water conditions, including dissolved oxygen ranging from 0.7 to 5.25 ppm and pH from 4 to 10 (Trost and Berner, 1963). Further, C. floridanus has been observed to be relatively salt tolerant and has been found in brackish water (Berner, 1954; Trost and Berner, 1963; Goetsch and Palmer, 1997). C. floridanus naiads are also frequently found in ditches or temporary ponds, which are subject to drying and great changes in physical and chemical conditions (Lahr, 1997). In these fluctuating environments, it is possible that temporal changes in salinity could require more physiological plasticity to tolerate these conditions. However, little is known about what physiological traits allow C. floridanus to survive in these conditions.
Here, we reared C. floridanus naiads (in a population from a coastal pond that routinely experiences saltwater intrusion) from egg hatch to adulthood across a gradient of increasing salinities (113, 5,020, 9,921 μS/cm), then assessed life history outcomes and physiological plasticity. We hypothesized that naiads would demonstrate a physiological affinity for dilute conditions, as observed in previous studies with N. triangulifer. We hypothesized survival, naiad development, and subimago weight would be negatively impacted by more saline conditions. Further, we expected physiological features, such as ion uptake rates, to be relatively plastic and demonstrate lower energetic costs in more dilute conditions. Because earlier work has shown that aquatic insects tightly regulate whole body salts (Buchwalter et al., 2018), we did not expect that whole-body salt content (measured via ICP-MS) would be significantly impacted by rearing conditions.
Callibaetis floridanus naiads used in this study were F2 progeny from a wild collection of 41 individuals made in October 2021 at Andelot Pond in Worton, Maryland (39.33342, −76.150896), which is located about 800 meters inland from the Still Pond Bay in the upper portion of the Chesapeake Bay. Andelot pond is a natural formation with historically common saltwater intrusion. Conductivity at the time of collection was 3.47 mS/cm and had ranged from 7.5 to 12.7 mS/cm at the time of previous collections in Fall 2013 and Fall 2015 (Dave Funk, personal observation). To obtain more detailed information about the salinity variation at the collection site, a HOBO conductivity logger was deployed at approximately 1 foot (at low tide) from May 30-September 3, 2022, in Still Pond Bay and from September 3-October 1, 2022, in Andelot Pond (see Figure 1).
Figure 1. Conductivity logger data from Still Pond Bay and Andelot pond, MD from May 30-October 1, 2022. (A) Data from HOBO conductivity logger deployed in Still Pond Bay (black dots) May 30-Sept. 3, 2022, and then moved to Andelot Pond (blue triangles) Sept. 3-Oct. 1, 2022. There is no data for August 20-28, 2022 in Still Bay Pond when the logger was out of the water. (B) Map of the area where C. floridanus was originally collected from and the conductivity logger was deployed.
Naiads were reared at Stroud Water Research Center (SWRC) in natural stream water (conductivity ~240 μS/cm). Adult copulation was induced manually in the laboratory. Callibaetis species are ovoviviparous and C. floridanus females gestate for ~9 days (at 22°C) before laying eggs that hatch immediately. Gravid adult females were transferred to NC State University where fresh hatchlings were obtained.
Callibaetis floridanus naiads were reared in 200-mL glass Pyrex® dishes (South Greencastle, Pennsylvania) at room temperature (21–23°C) and a 14:10 h light: dark photoperiod. Two replicate glass dishes (with about 150 C. floridanus naiads each) for each salinity condition were set up and randomly spaced out on the bench top. All pans were gently aerated for the entire experiment to maintain oxygen saturation. Food was provided as periphyton grown on an acrylic plate at the SWRC. Periphyton plates were grown by allowing fresh stream water from White Clay Creek, PA to flow over the plates continuously for 2–4 weeks (as previously described by Conley et al., 2009; Xie et al., 2010). Five periphyton plates were used per pan for the duration of development (about 21 days).
To create waters with different salinities, Crystal Sea® Marine Mix was added to reverse-osmosis deionized water to create the desired concentration (see Table 1). Conductivity was measured directly after the waters were made, then waters were allowed to equilibrate overnight before conductivity was measured again. Conductivities presented represent the average conductivity between those two measurements (see table 1).
Callibaetis floridanus naiads were reared in 1.8 l glass jars at room temperature (21–23°C) and a 14:10 h light: dark photoperiod. Three replicate jars (with 10 naiads each) for each condition were set up and randomly spaced out on the bench top. Food was provided as periphyton grown on an acrylic plate (as described above). Two periphyton plates were immediately added to each treatment water, and an additional supplementary plate was added 2 weeks into rearing for the lowest conductivity treatment as food consumption in this treatment was visibly faster than in the other treatments. Deionized water was added as needed to offset any evaporation. All jars were gently aerated for the entire experiment to maintain oxygen saturation. Larval development time ranged from 21 to 29 days, with subimagoes emerging over an 8-day period for all conditions. As they emerged in the late afternoon, subimagoes were collected into a mesh-lined collection lid. Subimagoes were immediately collected, placed in clean, labeled 1.5 ml microcentrifuge tubes, and stored frozen (−20°C) before wet weights were obtained. All data were analyzed for normality. Life history traits (% survival, subimago weight, and development time) were compared among treatments as the mean value of each response variable from each jar using a one-way ANOVA with Tukey’s multiple comparisons test using GraphPad Prism (v6, GraphPad Software, La Jolla, CA, United States).
Our whole-body salt content protocol has been previously described in detail (Cochran and Buchwalter, 2022), but here we give a summary. Subimagoes were dried overnight at 60°C and subsequently weighed before being microwave digested (CEM MARSXpress) in 1.5 ml Omnitrace Ultra High Purity Nitric Acid (EMD Chemicals, Darmstadt, Germany). NC State University’s Environmental and Agriculture Testing Services Lab analyzed samples via ICP-OES (Department of Soil Science, North Carolina State University, Raleigh, NC, United States) to determine the whole-body concentration of sodium. Quality control blanks were below Na/Ca/SO4 detection limits. Measurements were compared among groups using a one-way ANOVA with Tukey’s multiple comparisons test using GraphPad Prism. All data were also analyzed for normality.
Methods for measuring glycogen content were derived from Lee (2019) and are briefly described here. C. floridanus naiads (n = 8) were collected, weighed, and placed into sterile microcentrifuge tubes before being stored at −80°C. Each 1.5 ml microcentrifuge tube then received 13 μL 2% sodium sulfate before a micropestle was used to crush the naiad. 113 μL of chloroform methanol (1:2 v/v) was used to rinse each micropestle after use. The microcentrifuge tubes were then vortexed to mix the contents and centrifuged for 2–3 min at 13,000 rpm. The microcentrifuge tube was then inverted (keeping the pellet intact) and the solution was poured off. 240 μl of Anthrone reagent was then added to the microcentrifuge tube with the pellet and was vortexed until the pellet was mixed. The tube was then heated at 90°C for 3–5 min before being cooled on ice for 3 min. The microcentrifuge tubes were then vortexed and 200 μL of the sample was pipetted onto a well-plate before being read at 630 nm on a Thermo Scientific Multiskan FC. Standards (10, 25, 50, 75, 100, 150 μg) were prepared using rabbit liver.
We used an 8-channel, fiber-optic, intermittent flow respirometry system (Loligo Systems, Tjele, Denmark) with AutoResp (version 2.1.0; Loligo Systems, Tjele, Denmark) software to obtain SMR measurements. Chambers (1.3 ± 0.1 ml) were fitted with a glass spacer ring and stainless-steel mesh, which kept naiads separated from the continuously stirring magnet glass stir bar. Each chamber and corresponding fiber-optic channel recorded measurements of oxygen consumption for one naiad. Chambers were submerged in an aerated, temperature-controlled water bath (approx. 4 L). Water bath temperature was controlled using a programmable heater/chiller (12108–30; Cole Palmer, Vernon Hills, IL, United States), which cycled water between the respirometry bath, and a heat exchange coil submerged in the heater/chiller reservoir for the entire experiment. For each experimental run (in which up to 8 naiads were evaluated), background oxygen consumption measurements (n = 3–4) cycles were first taken from blank respirometry chambers using the intermittent respirometry setting in AutoResp 2.0. A Gilson Minipuls peristaltic pump (Gilson Inc., Middleton, WI, United States) refreshed the chamber medium with surrounding bath at the initiation of each 15-min cycle (300 s flush, 350 s wait, 250 s measure). Then, an individual naiad was introduced to each of 8 chambers and intermittent flow respirometry was performed for 2–3 cycles (30–45 min). Individuals underwent no prior acclimatization or fasting. The number of serial MO2 measurements obtained for each individual naiad ranged from 14 to 17. After completion, naiads were blotted dry, and weights were determined. We used the (q0.2) approach to estimate the standard metabolic rate (SMR) (Reemeyer and Rees, 2019).
Naiads were 19 days old for all 22Na, 45Ca, and 35SO4 uptake experiments. Radioactive experimental waters were made with each treatment water, spiked with 45CaCl2 or dual-labeled with 22NaCl and Na235SO4 (PerkinElmer, Billerica, MA, United States). Exposure activities ranged from 130 to 220 Bq mL−1. Exposures were measured with PerkinElmer Wallac Wizard 1,480 Automatic Gamma Counter (Shelton, CT, United States) or Beckman LS6500 Multipurpose Scintillation Counter (Beckman Coulter, Brea, CA, United States) immediately before the experiments began.
Hundred ml high-density polyethylene beakers with 15 ml of radioactive exposure water were gently aerated, sealed with ParaFilm™ and spatially randomized. Each treatment had 8 replicates per time point (3, 6, and 9-h for dual-labeled with 22NaCl and Na235SO4 and 12, 24, and 36-h for 45CaCl2). At each time point, naiads were removed from the radioactive exposure waters by gently pipetting them into a mesh strainer (collecting any residual radioactive water in a waste container) and gently blotting dry. The larvae were then rinsed in two consecutive water baths of the corresponding unlabeled exposure water to remove loosely adsorbed ions from the exoskeleton. For 45Ca experiments, naiads were also rinsed with 0.05 M EDTA and 0.1 M L-ascorbic acid sodium salt due to the adsorptive nature of Ca on insect exoskeletons (Poteat and Buchwalter, 2014a). After rinsing, naiads were blotted dry, weighed, and digested in 500 μl of Soluene 350 (PerkinElmer) in a 20 ml glass vial at 28°C. After 48 h, they were neutralized with 500 μl of glacial acetic acid and 12 ml of scintillation cocktail (PerkinElmer Ultima Gold uLLT).
Uptake rates were calculated using the slopes of linear regression analysis using GraphPad Prism. Mass-specific calculations were based on wet weights. We applied appropriate corrections for spill-over and quench, and only measurements with lumex values <5% and error rates <10% were used in analyses. Flux rates were compared among treatments using a one-way ANOVA with Tukey’s multiple comparisons test using GraphPad Prism. Data were also analyzed for normality.
Mean percent survival 80 ± 4.4% (mean ± s.e.m.) and days to emergence 24 ± 0.3 (mean ± s.e.m.) (Table 2) were not significantly different among salinity treatments. Mean subimago wet weight was not significantly different among treatments for males (Table 2). However, females in the 113 μS cm−1 treatment had 27% lower mean subimago wet weight compared to the 5,020 μS cm−1 treatment group (Table 2) (p = 0.0004). Female subimagoes reared in 113 μS cm−1 had a dry weight of 2.7 ± 0.2 mg, female subimagoes reared in 5,020 μS cm−1 had a dry weight of 3.7 ± 0.1 mg, and female subimagoes reared in 9,921 μS cm−1 had a dry weight of 3.5 ± 0.3 mg (mean ± s.e.m.).
Table 2. Summary of life-history outcomes (including mean days to emergence, mean percent survival and mean subimago weights (dry weight) of males and females) (mean ± s.e.m.).
113 μS cm−1 reared naiads were observed to have markedly higher grazing rates relative to naiads reared in higher salinity treatments (Supplementary Figure S1).
Calcium content 1.7 ± 0.1 (mean ± s.e.m.) was not significantly impacted by salinity (Table 3). However, the 113 μS cm−1 treatment group had significantly less Mg (p = 0.0468), K (p = 0.0087), Na (p = 0.0005), P (p = 0.0093), and S (p = 0.0142) relative to the 9,921 μS cm−1 treatment group and K (p = 0.0062), P (p = 0.0092), and S (p = 0.0142) relative to the 5,020 μS cm−1 treatment group.
Naiad glycogen content was not statistically different between rearing conditions, but there was a 13% decrease in glycogen in the lowest treatment group, compared to the highest treatment group (Figure 2A). A one-way ANOVA and Tukey’s multiple comparisons test found no significant differences between males and females from any given rearing condition, so all runs were combined for each rearing condition. Glycogen content was 9.3 ± 0.5 in 113 μS cm−1 reared naiads, 10.4 ± 0.9 in 5,020 μS cm−1 reared naiads and 10.8 ± 0.8 in 9,921 μS cm−1 reared naiads (mean ± s.e.m.).
Figure 2. (A) Glycogen content of nymphs reared in either 113, 5,020 or 9,921uS/cm waters. (B) C. floridanus metabolic rates (MO2) across three experimental waters. The x-axis indicates the exposure condition for each major ion of interest whereas the color of the bar indicates the rearing condition. Values are mean ± 1 standard error of the mean.
We found that in 113 μS cm−1 reared naiads, MO2 decreased with increased salinity (Figure 2B). For example, 113 μS cm−1 reared naiads had a metabolic rate of 804 ± 57.3 μg O2 g−1 h−1 (mean ± s.e.m.) in their rearing water and 568 ± 51.5 μg O2 g−1 h−1 in 9,921 μS cm−1 water (p ≤ 0.0001). Naiads reared in 5,020 μS cm−1 water had a higher metabolic rate in 5,020 μS cm−1 water (676 ± 42.5 μg O2 g−1 h−1), with decreased MO2 in 113 μS cm−1 water (584 ± 83.7 μg O2 g−1 h−1, p = 0.019) and the lowest MO2 in 9,921 μS cm−1 water (389 ± 40.4 μg O2 g−1 h−1, p ≤ 0.0001). In 9,921 μS cm−1 reared naiads, MO2 decreased with decreasing salinity. For example, naiads reared in 9,921 μS cm−1 water had a metabolic rate of 648 ± 88.7 μg O2 g−1 h−1 in their rearing water and 442 ± 93.9 μg O2 g−1 h−1 in 113 μS cm−1 water (p ≤ 0.0001).
Ion uptake rates of naiads in their rearing water were positively associated with ionic concentration (Figure 3). For example, in their respective rearing waters, 113 μS cm−1 reared naiads had a sodium uptake rate of 104 ± 7.8 μg Na g−1 h−1 (mean ± s.e.m.), 5,020 μS cm−1 reared naiads had a sodium uptake rate of 170 ± 20 μg Na g−1 h−1 and 9,921 μS cm−1 reared naiads had a sodium uptake rate of 199 ± 20 μg Na g−1 h−1 (p ≤ 0.001) (Figure 3A). The only exception was Ca uptake of 113 and 5,020 μS cm−1 reared individuals in their respective waters (2.6 ± 0.2 and 3.3 ± 0.2 3 μg Ca g−1 h−1 respectively, p = 0.0526) (Figure 3C).
Figure 3. Ion uptake rates in C. floridanus for (A) Sodium, (B) Sulfate and (C) Calcium. The x-axis indicates the exposure condition for each major ion of interest whereas the color of the bar indicates the rearing condition. Each column represents a rate of uptake from a time-course study. Values are mean ± 1 standard error of the mean. Wet weight is abbreviated as ww.
When naiads were transferred from their respective rearing water to the other experimental conditions, all rearing groups had ion uptake rates that were increased with exposure ionic concentration. For example, 113 μS cm−1 reared naiads had a Ca uptake rate of 2.6 ± 0.3 μg Ca g−1 h−1 in 113 μS cm−1, 4.7 ± 0.3 μg Ca g−1 h−1 in 5,020 μS cm−1 water and 15.0 ± 0.9 μg Ca g−1 h−1 in 9,921 μS cm−1 water (Figure 3C).
Across all ions and exposure waters, 113 μS cm−1 reared individuals had the highest ion uptake rates (Figure 3). For Na and SO4, 9,921 μS cm−1 reared individuals had the lowest uptake rates (except in 113 μS cm−1, where 9,921 and 5,020 μS cm−1 reared individuals are not statistically different). For Ca, 5,020 and 9,921 μS cm−1 reared individuals were not statistically different from each other but were both significantly lower than 113 μS cm−1 reared individuals in all waters (Figure 3C). For example, in 9,921 μS cm−1 water, 113, 5,020 and 9,921 μS cm−1 reared naiads had SO4 uptake rate of 18.3 ± 2.5, 14.2 ± 1.9, and 9.1 ± 1.1 μg SO4 g−1 h−1, respectively (p = <0.0001) (Figure 3B).
Aquatic insects, such as C. floridanus, are considered secondarily aquatic, as phylogenomic analyses show that there is no clear common aquatic ancestor, but rather that multiple invasions of water occurred over millions of years (Wootton and Clark, 1972; Wootton, 1988; Misof et al., 2014). While most freshwater taxa are thought to have marine origins, aquatic insects have terrestrial ancestors (Wootton and Clark, 1972; Wootton, 1988; Misof et al., 2014; Miller and Román-Palacios, 2019). Aquatic insects dominate most freshwater ecosystems (Resh and Jackson, 1993; Hawkins et al., 2000; Funk et al., 2006; Hawkins, 2006) and are more abundant in relatively dilute freshwater habitats, relative to more saline habitats (Maddrell, 1998; Bradley, 2013). We hypothesized that C. floridanus naiads (of a population from a coastal pond that routinely experiences saltwater intrusion) reared across a gradient of increasing salinities (113, 5,020, 9,921 μS/cm) would demonstrate physiological affinity for dilute conditions, as observed in previous studies with N. triangulifer (Cochran and Buchwalter, 2022). However, our results collectively show a lower energetic cost for naiads in higher salinities.
We provide several lines of evidence suggesting C. floridanus may be more energetically challenged in dilute conditions, relative to saltier conditions. While there was no significant impact of rearing condition on survival or development time, subimago female body weight was 27% lower in the dilute rearing condition. As adult weight is closely associated with fecundity, this decrease in female weight could be associated with decreased reproductive success (Peckarsky et al., 1993). In N. triangulifer, there was no significant impact of rearing condition on survival, development time nor adult weight even when individuals were reared in 1 μS cm−1 water (Cochran and Buchwalter, 2022). Further, exposure to elevated salts has been observed to delay development in N. triangulifer (Johnson et al., 2015; Soucek and Dickinson, 2015; Buchwalter et al., 2018). However, this differs from other studies in damselflies (Ischnura heterosticta Burmeister, 1842) (Kefford et al., 2006), snails (Physella acuta Draparnaud, 1805) (Kefford and Nugegoda, 2005), mosquitoes (Clark et al., 2004) and fish (Bœuf and Payan, 2001) which have found negative impacts on growth and survival rates in lower salinity waters. Further, we found that C. floridanus naiads do not tightly control whole body salts across a range of conditions. This is not commensurate with studies in N. triangulifer, where sulfur and sodium content were regulated across a wide range of salinities (Scheibener et al., 2016; Buchwalter et al., 2018; Cochran and Buchwalter, 2022). Further, studies in Hydropsyche sparna Ross, 1938 and Maccaffertium sp. Bednarik, 1979 showed that Na was regulated across a range of Na concentrations (Scheibener et al., 2016). Similarly, studies in Aedes aegypti Linnaeus, 1762 and Culex quinquefasciatus Say, 1823 (Patrick et al., 2002) and Austrophlebioides pusillus Harker, 1954 (Dowse et al., 2017) have shown maintenance of haemolymph NaCl concentrations despite being reared in dilute water for multiple generations.
We observed markedly increased grazing rates by naiads reared in 113 μS cm−1, relative to naiads reared in the higher salinity treatments (Supplementary Figure S1). Yet there were no differences in the final subimago body weights or in naiad development times. We propose a few potential explanations (not mutually exclusive) that could explain this unexpected result. One possibility is that this increase in food consumption may be an attempt to increase acquisition of salts via periphyton. Salt is almost exclusively obtained through the diet in terrestrial insects, with evidence suggesting that some animals, such as cervids, specifically consume sodium rich aquatic plants to meet their sodium needs (Ceacero et al., 2014). Further, studies suggest that salt content of Locusta migratoria Linnaeus, 1758 depends on the salt concentration of their food (Trumper and Simpson, 1993; Niewalda et al., 2008). Other studies in terrestrial insects show changes in food consumption to meet salt demands (Kaspari et al., 2008; Kaspari, 2020, 2021). However, it is currently unclear what role diet plays in aquatic insects’ acquisition of salts from the environment. It is known that the dietary acquisition of trace elements is tremendously important in aquatic insects (Xie et al., 2010; Cain et al., 2011; Kim et al., 2012; Poteat and Buchwalter, 2014b; Buchwalter et al., 2017). Our findings suggest that changes in food consumption may have been caused by the different water salinities, with lowest salinity water requiring a greater intake of salts and/or energy via food consumption. Alternatively, there may be some additional energetic cost associated with removing excess body water, with the rates of passive water influxes being potentially commensurate with the concentration gradients between hemolymph and surrounding water (Cochran and Buchwalter, 2022).
Further, that glycogen content was marginally lower in naiads reared in lowest salinity treatment suggests that there is some energetic cost to surviving in dilute water (Figure 2A). This is evidenced by the high metabolic rate of naiads in the low salinity treatment (Figure 2B). Presumably, as salts become more readily available in the more saline waters, less energy is expended to osmoregulate. This trend is commensurate with studies in Gammarus Fabricius, 1775 (Sutcliffe, 1984), snails (McMahon and Russell-Hunter, 1978; Paolucci and Thuesen, 2020), cutthroat trout (Oncorhynchus clarkii Richardson, 1836) (Morgan and Iwama, 1999), and common carp (Cyprinus carpio Linnaeus, 1758) (de Boeck et al., 2000). However, decreasing energetic costs with increasing salinity was not observed in naiads reared in 5,020 and 9,921 μS cm−1 waters. In 9,921 μS cm−1 reared naiads, the lowest metabolic rate is observed in the lowest salinity exposure and increased with salinity (Figure 2B). This pattern is more consistent with observations in euryhaline or marine species such as caridean shrimp (Palaemon peringueyi Stebbing, 1915) (Allan et al., 2006), Magadi tilapia (Alcolapia grahami Boulenger, 1912) (Wood et al., 2002) and white leg shrimp (Litopenaeus vannamei Boone, 1931) (Ding et al., 2014) where metabolic rate increases with increased salinity. Taken together, the patterns in glycogen and metabolic rate suggest that there are differences in the energetic response to salinity based on the rearing condition.
Callibaetis floridanus naiads reared in more dilute salinity treatments also had higher uptake rates of salts compared to naiads reared in more salty conditions when exposed to similar conditions (Figure 3). Other studies have found that acclimation to dilute water increases Ca2+ uptake in Daphnia Müller, 1785 (Durant et al., 2018) and Na uptake in the mangrove crab Ucides cordatus Linnaeus, 1763 (Harris and Santos, 1993). Further, other studies have found that increased salinity decreases sulfate uptake in freshwater mussels (Dietz et al., 2000) and calcium uptake in rainbow trout (Salmo gairdneri Richardson, 1836) and tilapia (Oreochromis mossambicus W. K. H. Peters, 1852) (Perry and Wood, 1985). However, our results do not match those of Nguyen and Donini (2010), who showed that long-term exposure to ion poor water resulted in no changes to ion uptake in midges (Chironomus riparius Meigen, 1804) (despite increased anal papillae size). Previous observations in N. triangulifer have shown that exposure to elevated major ion concentrations led to a marked decrease in ion uptake rates, relative to naïve naiads (Orr et al., 2021). Further, exposure to a gradient of dilute waters led to a marked increase in ion uptake relative to naïve naiads (Cochran and Buchwalter, 2022). Dilute-acclimated N. triangulifer naiads also maintained a relatively narrow range of uptake rates in all treatments (contrasting the concentration-dependent Na uptake rates observed in naive naiads). In contrast, our findings suggest that C. floridanus does not maintain such a narrow range in ion uptake rates.
Collectively, our findings suggest that unlike most other aquatic insects in general, and mayflies in particular, C. floridanus naiads are remarkably plastic in their ability to live across a huge gradient of salinity. To our surprise, individuals from this population appear physiologically challenged in dilute conditions relative to saltier conditions, even though the two previous generations of this cohort were reared under dilute conditions and their presumed ancestral habitats were likely dilute. This contrasts with the general observation that mayflies are typically challenged by more ion rich conditions (Pond et al., 2008; Orr et al., 2021; Cochran and Buchwalter, 2022). While it is unclear what specifically makes C. floridanus’ salinity tolerances so broad relative to other mayflies, we posit that their remarkable habitat variability with respect to environmental salinity (Figure 1A) has shaped a great deal of physiological plasticity.
The raw data supporting the conclusions of this article will be made available by the authors, without undue reservation.
JC performed the research and data analysis and wrote the manuscript. DF discovered the population, cultured the mayflies, and provided the editorial support. DB oversaw the experimental design and provided the editorial support. All authors contributed to the article and approved the submitted version.
This research was supported by a grant from the National Science foundation (IOS 1754884).
The authors declare that the research was conducted in the absence of any commercial or financial relationships that could be construed as a potential conflict of interest.
All claims expressed in this article are solely those of the authors and do not necessarily represent those of their affiliated organizations, or those of the publisher, the editors and the reviewers. Any product that may be evaluated in this article, or claim that may be made by its manufacturer, is not guaranteed or endorsed by the publisher.
The Supplementary material for this article can be found online at: https://www.frontiersin.org/articles/10.3389/fevo.2023.1135924/full#supplementary-material
SUPPLEMENTARY FIGURE 1 | Photographs of periphyton plates after 21 days of nymphal development/feeding. There is very little periphyton remaining in the 113 μS cm-1 treatment (A) compared to the 5,020 and 9,921 μS cm-1 treatments (B,C, respectively).
Allan, E. L., Froneman, P. W., and Hodgson, A. N. (2006). Effects of temperature and salinity on the standard metabolic rate (SMR) of the caridean shrimp Palaemon peringueyi. J. Exp. Mar. Biol. Ecol. 337, 103–108. doi: 10.1016/j.jembe.2006.06.006
Barlow, P. M., and Reichard, E. G. (2010). Saltwater intrusion in coastal regions of North America. Hydrogeol. J. 18, 247–260. doi: 10.1007/s10040-009-0514-3
Beermann, A. J., Elbrecht, V., Karnatz, S., Ma, L., Matthaei, C. D., Piggott, J. J., et al. (2018). Multiple-stressor effects on stream macroinvertebrate communities: a mesocosm experiment manipulating salinity, fine sediment and flow velocity. Sci. Total Environ. 610–611, 961–971. doi: 10.1016/j.scitotenv.2017.08.084
Berner, L. (1954). The occurrence of a mayfly nymph in brackish water. Ecology 35:98. doi: 10.2307/1931414
Bœuf, G., and Payan, P. (2001). How should salinity influence fish growth? Comp. Biochem. Physiol. Part C Toxicol. Pharmacol. 130, 411–423. doi: 10.1016/S1532-0456(01)00268-X
Bradley, T. J. (1987). Physiology of osmoregulation in mosquitoes. Annu. Rev. Entomol. 32, 439–462. doi: 10.1146/annurev.en.32.010187.002255
Bradley, T. J. (2013) ‘Saline-water insects: ecology, physiology and evolution’, in J. Lancaster and R. A. Briars (Eds) Aquatic insects: Chalenges to populations: Proceedings of the Royal Entomological Society’s 24th symposium. Oxfordshire: Royal Entomological Society of London, 20–35.
Bradley, T., Ajami, H., and Porter, W. (2022). Ecological transitions at the Salton Sea: past, present and future. Calif. Agric. 76, 8–15. doi: 10.3733/ca.2022a0004
Buchwalter, D. B., Clements, W. H., and Luoma, S. N. (2017). Modernizing water quality criteria in the United States: a need to expand the definition of acceptable data. Environ. Toxicol. Chem. 36, 285–291. doi: 10.1002/etc.3654
Buchwalter, D., Scheibener, S., Chou, H., Soucek, D., and Elphick, J. (2018). Are sulfate effects in the mayfly Neocloeon triangulifer driven by the cost of ion regulation? Philos. Trans. R. Soc. Lond. B Biol. Sci. 374:20180013. doi: 10.1098/rstb.2018.0013
Cain, D. J., Crotrau, M.-N., and Luoma, S. N. (2011). Bioaccumulation dynamics and exposure routes of cd and cu among species of aquatic mayflies. Environ. Toxicol. Chem. 30, 2532–2541. doi: 10.1002/etc.663
Cañedo-Argüelles, M., Kefford, B. J., Piscart, C., Prat, N., Schäfer, R. B., and Schulz, C. J. (2013). Salinisation of rivers: an urgent ecological issue. Environ. Pollut. 173, 157–167. doi: 10.1016/j.envpol.2012.10.011
Ceacero, F., Landete-Castillejos, T., Miranda, M., García, A. J., Martínez, A., and Gallego, L. (2014). Why do cervids feed on aquatic vegetation? Behav. Process. 103, 28–34. doi: 10.1016/j.beproc.2013.10.008
Clark, T. M., Flis, B. J., and Remold, S. K. (2004). Differences in the effects of salinity on larval growth and developmental programs of a freshwater and a euryhaline mosquito species (Insecta: Diptera, Culicidae). J. Exp. Biol. 207, 2289–2295. doi: 10.1242/jeb.01018
Cochran, J. K., and Buchwalter, D. B. (2022). The acclimatory response of the mayfly Neocloeon triangulifer to dilute conditions is linked to the plasticity of sodium transport. Proc. R. Soc. B Biol. Sci. 289:20220529. doi: 10.1098/rspb.2022.0529
Conley, J. M., Funk, D. H., and Buchwalter, D. B. (2009). Selenium bioaccumulation and maternal transfer in the mayfly Centroptilum triangulifer in a life-cycle, periphyton-biofilm trophic assay. Environ. Sci. Tech. 43, 7952–7957. doi: 10.1021/es9016377
Cormier, S. M., and Suter, G. W. (2013). A method for assessing causation of field exposure-response relationships. Environ. Toxicol. Chem. 32, 272–276. doi: 10.1002/etc.2056
de Boeck, G., Vlaeminck, A., van der Linden, A., and Blust, R. (2000). The energy metabolism of common carp (Cyprinus carpio) when exposed to salt stress: an increase in energy expenditure or effects of starvation? Physiol Biochem. Zool. 73, 102–111. doi: 10.1086/316717
Dietz, T., Udoetok, A. S., Cherry, J. S., Silverman, H., and Byrne, R. A. (2000). Kidney function and sulfate uptake and loss in the freshwater bivalve Toxolasma texasensis. Biol. Bull. 199, 14–20. doi: 10.2307/1542702
Ding, S., Wang, F., Dong, S., and Li, Y. (2014). Comparison of the respiratory metabolism of juvenile Litopenaeus vannamei cultured in seawater and freshwater. J. Ocean Univ. China 13, 331–337. doi: 10.1007/s11802-014-2134-3
Dowse, R., Palmer, C. G., Hills, K., Torpy, F., and Kefford, B. J. (2017). The mayfly nymph Austrophlebioides pusillus Harker defies common osmoregulatory assumptions. R. Soc. Open. Sci. 4:160520. doi: 10.1098/rsos.160520
Durant, A. C., Celis-Salgado, M. P., Ezatollahpour, S., Yan, N. D., Arnott, S. E., and Donini, A. (2018). Ca2+ levels in Daphnia hemolymph may explain occurrences of daphniid species along recent ca gradients in Canadian soft-water lakes. Comp. Biochem. Physiol. A Mol. Integr. Physiol. 218, 8–15. doi: 10.1016/j.cbpa.2018.01.009
Entrekin, S., Evans-White, M., and Hagenbuch, E. (2011). Rapid expansion of natural gas development poses a threat to surface waters. Front. Ecol. Environ. 9, 503–511. doi: 10.1890/110053
Funk, D. H., Jackson, J. K., and Sweeney, B. W. (2006). Taxonomy and genetics of the parthenogenetic mayfly Centroptilum triangulifer and its sexual sister Centroptilum alamance (Ephemeroptera:Baetidae). J. N. Am. Benthol. Soc. 25, 417–429. doi: 10.1899/0887-3593(2006)25[417:TAGOTP]2.0.CO;2
Goetsch, P. A., and Palmer, C. G. (1997). Salinity tolerances of selected macroinvertebrates of the Sabie River, Kruger National Park, South Africa. Arch. Environ. Contam. Toxicol. 32, 32–41. doi: 10.1007/s002449900152
Harris, R. R., and Santos, F. (1993). Sodium uptake and transport (Na+ + K+)ATPase changes following Na+ depletion and low salinity acclimation in the mangrove crab Ucides cordatus (L.). Comp. Biochem. Physiol. A Physiol. 105, 35–42. doi: 10.1016/0300-9629(93)90170-9
Hawkins, C. P. (2006). Quantifying biological integrity by taxonomic completeness: its utility in regional and global assessments. Ecol. Appl. 16, 1277–1294. doi: 10.1890/1051-0761(2006)016[1277:QBIBTC]2.0.CO;2
Hawkins, C. P., Norris, R. H., Hogue, J. N., and Feminella, J. W. (2000). Development and evaluation of predictive models for measuring the biological integrity of streams. Ecol. Appl. 10, 1456–1477. doi: 10.1890/1051-0761(2000)010[1456:DAEOPM]2.0.CO;2
Herbst, D. B. (1999). Biogeography and physiological adaptations of the brine fly genus Ephydra (Diptera: Ephydridae) in saline waters of the Great Basin. Great Basin Nat. 59, 127–135. doi: 10.2307/4171309
Herbst, D. B. (2001). Gradients of salinity stress, environmental stability and water chemistry as a templet for defining habitat types and physiological strategies in inland salt waters. Hydrobiologia 466, 209–219. doi: 10.1023/A:1014508026349
Iglesias, M. C.-A. (2020). A review of recent advances and future challenges in freshwater salinization. Limnetica 39, 185–211. doi: 10.23818/limn.39.13
Jackson, J. K., and Funk, D. H. (2019). Temperature affects acute mayfly responses to elevated salinity: implications for toxicity of road de-icing salts. Philos Trans R Soc B Biol Sci 374:20180081. doi: 10.1098/rstb.2018.0081
Johnson, B. R., Weaver, P. C., Nietch, C. T., Lazorchak, J. M., Struewing, K. A., and Funk, D. H. (2015). Elevated major ion concentrations inhibit larval mayfly growth and development. Environ. Toxicol. Chem. 34, 167–172. doi: 10.1002/etc.2777
Kaspari, M. (2020). The seventh macronutrient: how sodium shortfall ramifies through populations, food webs and ecosystems. Ecol. Lett. 23, 1153–1168. doi: 10.1111/ele.13517
Kaspari, M. (2021). The invisible hand of the periodic table: how micronutrients shape ecology. Annu. Rev. Ecol. Evol. Syst. 52, 199–219. doi: 10.1146/annurev-ecolsys-012021-090118
Kaspari, M., Yanoviak, S. P., and Dudley, R. (2008). On the biogeography of salt limitation: a study of ant communities. Proc. Natl. Acad. Sci. 105, 17848–17851. doi: 10.1073/pnas.0804528105
Kefford, B. J. (2019). Why are mayflies (Ephemeroptera) lost following small increases in salinity? Three conceptual osmophysiological hypotheses. Philos. Trans. R. Soc. Lond B Biol. Sci. 374:20180021. doi: 10.1098/rstb.2018.0021
Kefford, B. J., and Nugegoda, D. (2005). No evidence for a critical salinity threshold for growth and reproduction in the freshwater snail Physa acuta. Environ. Pollut. 134, 377–383. doi: 10.1016/j.envpol.2004.09.018
Kefford, B. J., Zalizniak, L., and Nugegoda, D. (2006). Growth of the damselfly Ischnura heterosticta is better in saline water than freshwater. Environ. Pollut. 141, 409–419. doi: 10.1016/j.envpol.2005.08.064
Kim, K. S., Funk, D. H., and Buchwalter, D. B. (2012). Dietary (periphyton) and aqueous Zn bioaccumulation dynamics in the mayfly Centroptilum triangulifer. Ecotoxicology 21, 2288–2296. doi: 10.1007/s10646-012-0985-1
Kunz, J. L., Conley, J. M., Buchwalter, D. B., Norberg-King, T. J., Kemble, N. E., Wang, N., et al. (2013). Use of reconstituted waters to evaluate effects of elevated major ions associated with mountaintop coal mining on freshwater invertebrates. Environ. Toxicol. Chem. 32, 2826–2835. doi: 10.1002/etc.2391
Lahr, J. (1997). Ecotoxicology of organisms adapted to life in temporary freshwater ponds in arid and semi-arid regions. Arch. Environ. Contam. Toxicol. 32, 50–57. doi: 10.1007/s002449900154
Lee, J. C. (2019). What we can learn from the energetic levels of insects: a guide and review. Ann. Entomol. Soc. Am. 112, 220–226. doi: 10.1093/aesa/say051
Maddrell, S. H. (1998). Why are there no insects in the open sea? J. Exp. Biol. 201, 2461–2464. doi: 10.1242/jeb.201.17.2461
Mastrocicco, M., Busico, G., Colombani, N., Vigliotti, M., and Ruberti, D. (2019). Modelling actual and future seawater intrusion in the Variconi coastal wetland (Italy) due to climate and landscape changes. Water 11:1502. doi: 10.3390/w11071502
McMahon, R. F., and Russell-Hunter, W. (1978). Respiratory responses to low oxygen stress in marine littoral and sublittoral snails. Physiol. Zool. 51, 408–424. doi: 10.1086/physzool.51.4.30160965
Miller, E., and Román-Palacios, C. (2019). Evolutionary time explains the global distribution of freshwater fish diversity. bioRxiv 10:668079. doi: 10.1101/668079
Misof, B., Liu, S., Meusemann, K., Peters, R. S., Donath, A., Mayer, C., et al. (2014). Phylogenomics resolves the timing and pattern of insect evolution. Science 346, 763–767. doi: 10.1126/science.1257570
Morgan, J. D., and Iwama, G. K. (1999). Energy cost of NaCl transport in isolated gills of cutthroat trout. Am. J. Physiol. 277, R631–R639.
Nguyen, H., and Donini, A. (2010). Larvae of the midge Chironomus riparius possess two distinct mechanisms for ionoregulation in response to ion-poor conditions. Am. J. Phys. Regul. Integr. Comp. Phys. 299, R762–R773. doi: 10.1152/ajpregu.00745.2009
Niewalda, T., Singhal, N., Fiala, A., Saumweber, T., Wegener, S., and Gerber, B. (2008). Salt processing in larval drosophila: choice, feeding, and learning shift from appetitive to aversive in a concentration-dependent way. Chem. Senses 33, 685–692. doi: 10.1093/chemse/bjn037
Olson, J. R., and Hawkins, C. P. (2017). Effects of total dissolved solids on growth and mortality predict distributions of stream macroinvertebrates. Freshw. Biol. 62, 779–791. doi: 10.1111/fwb.12901
Orr, S. E., Negrão Watanabe, T. T., and Buchwalter, D. B. (2021). Physiological plasticity and acclimatory responses to salinity stress are ion-specific in the mayfly, Neocloeon triangulifer. Environ. Pollut. 286:117221. doi: 10.1016/j.envpol.2021.117221
Paolucci, E. M., and Thuesen, E. V. (2020). Effects of osmotic and thermal shock on the invasive aquatic mudsnail Potamopyrgus antipodarum: mortality and physiology under stressful conditions. NeoBiota 54, 1–22. doi: 10.3897/neobiota.54.39465
Patrick, M. L., Ferreira, R. L., Gonzalez, R. J., Wood, C. M., Wilson, R. W., Bradley, T. J., et al. (2002). The characterization of ion regulation in Amazonian mosquito larvae: evidence of phenotypic plasticity, population based disparity, and novel mechanisms of ion uptake. Physiol. Biochem. Zool. 75, 215–222. doi: 10.1086/342004
Peckarsky, B. L., Cowan, C. A., Penton, M. A., and Anderson, C. (1993). Sublethal consequences of stream-dwelling predatory stoneflies on mayfly growth and fecundity. Ecology 74, 1836–1846. doi: 10.2307/1939941
Perry, S. F., and Wood, C. M. (1985). Kinetics of Branchial calcium uptake in the rainbow trout: effects of acclimation to various external calcium levels. J. Exp. Biol. 116, 411–433. doi: 10.1242/jeb.116.1.411
Pond, G. J., Passmore, M. E., Borsuk, F. A., Reynolds, L., and Rose, C. J. (2008). Downstream effects of mountaintop coal mining: comparing biological conditions using family and genus-level macroinvertebrate bioassessment tools. J. N. Am. Benthol. Soc. 27, 717–737. doi: 10.1899/08-015.1
Poteat, M. D., and Buchwalter, D. B. (2014a). Calcium uptake in aquatic insects: influences of phylogeny and metals (cd and Zn). J. Exp. Biol. 217, 1180–1186. doi: 10.1242/jeb.097261
Poteat, M. D., and Buchwalter, D. B. (2014b). Four reasons why traditional metal toxicity testing with aquatic insects is irrelevant. Environ. Sci. Technol. 48, 887–888. doi: 10.1021/es405529n
Reemeyer, J. E., and Rees, B. B. (2019). Standardizing the determination and interpretation of Pcrit in fishes. J. Exp. Biol. 222:jeb210633. doi: 10.1242/jeb.210633
Resh, V. H., and Jackson, J. K. (1993). “Rapid assessment approaches to biomonitoring using benthic macroinvertebrates” in Freshwater biomonitoring and benthic macroinvertebrates. eds. D. Rosenberg, M. Vincent, and H. Resh (New York: Chapman & Hall), 195–233.
Scheibener, S. A., Richardi, V. S., and Buchwalter, D. B. (2016). Comparative sodium transport patterns provide clues for understanding salinity and metal responses in aquatic insects. Aquat. Toxicol 171, 20–29. doi: 10.1016/j.aquatox.2015.12.006
Scudder, G. G. (1976). Water-boatmen of saline waters (Hemiptera: Corixidae). Marine Insects, 263–289.
Sekimoto, T., Osumi, Y., Shiraki, T., Kobayashi, A., Emi, K., Nakajo, M., et al. (2014). Comparative study of salinity tolerance an oceanic sea skater, Halobates micans and its closely related fresh water species, Metrocoris histrio. Nat. Sci. 06, 1141–1148. doi: 10.4236/ns.2014.614102
Shimizu, M., and Bradley, T. J. (1994). Concentrated excretion of sulfate by the anterior colon of the brine fly Ephydra hians. Physiol. Zool. 67, 54–67. doi: 10.1086/physzool.67.1.30163834
Soucek, D. J., and Dickinson, A. (2015). Full-life chronic toxicity of sodium salts to the mayfly Neocloeon triangulifer in tests with laboratory cultured food. Environ. Toxicol. Chem. 34, 2126–2137. doi: 10.1002/etc.3038
Sutcliffe, D. (1960) Observations on the salinity tolerance and habits of a Euryhaline caddis larva. Limsepiillus Affinis Curtis (Trichoptera: Limnephilidae). In Proceedings of the Royal Entomological Society of London. Series A, General Entomology, Wiley Online Library, 156–162, 35
Sutcliffe, D. (1984). Quantitative aspects of oxygen uptake by Gammarus (Crustacea, Amphipoda): a critical review. Freshw. Biol. 14, 443–489. doi: 10.1111/j.1365-2427.1984.tb00168.x
Trost, L. M., and Berner, L. (1963). The biology of Callibaetis floridanus banks (Ephemeroptera: Baetidae). Fla. Entomol. 46, 285–299. doi: 10.2307/3493578
Trumper, S., and Simpson, S. (1993). Regulation of salt intake by nymphs of Locusta migratoria. J. Insect Physiol. 39, 857–864. doi: 10.1016/0022-1910(93)90118-B
Wood, C. M., Wilson, P., Bergman, H. L., Bergman, A. N., Laurent, P., Otiang’a-Owiti, G., et al. (2002). Obligatory urea production and the cost of living in the Magadi tilapia revealed by acclimation to reduced salinity and alkalinity. Physiol. Biochem. Zool. 75, 111–122. doi: 10.1086/340626
Wootton, R. J. (1988). The historical ecology of aquatic insects: an overview. Palaeogeogr. Palaeoclimatol. Palaeoecol. 62, 477–492. doi: 10.1016/0031-0182(88)90068-5
Wootton, R. J., and Clark, R. B. (1972) Essays in hydrobiology. Available at: http://agris.fao.org/agris-search/search.do?recordID=US201300489917 (Accessed April 8, 2020).
Keywords: mayfly, saline adaptation, ion fluxes, saltwater intrusion, plasticity
Citation: Cochran JK, Funk DH and Buchwalter DB (2023) Physiological and life history responses in a mayfly (Callibaetis floridanus) inhabiting ponds with saltwater intrusion. Front. Ecol. Evol. 11:1135924. doi: 10.3389/fevo.2023.1135924
Received: 02 January 2023; Accepted: 13 March 2023;
Published: 30 March 2023.
Edited by:
Jason L. Robinson, Illinois Natural History Survey (INHS), United StatesReviewed by:
William Wyatt Hoback, Oklahoma State University, United StatesCopyright © 2023 Cochran, Funk and Buchwalter. This is an open-access article distributed under the terms of the Creative Commons Attribution License (CC BY). The use, distribution or reproduction in other forums is permitted, provided the original author(s) and the copyright owner(s) are credited and that the original publication in this journal is cited, in accordance with accepted academic practice. No use, distribution or reproduction is permitted which does not comply with these terms.
*Correspondence: David B. Buchwalter, RGF2aWRfYnVjaHdhbHRlckBuY3N1LmVkdQ==
Disclaimer: All claims expressed in this article are solely those of the authors and do not necessarily represent those of their affiliated organizations, or those of the publisher, the editors and the reviewers. Any product that may be evaluated in this article or claim that may be made by its manufacturer is not guaranteed or endorsed by the publisher.
Research integrity at Frontiers
Learn more about the work of our research integrity team to safeguard the quality of each article we publish.