- 1Institute of Sedimentary Geology, Chengdu University of Technology, Chengdu, China
- 2Department of Earth and Planetary Sciences, Kyushu University, Fukuoka, Japan
- 3Department of Geosciences, University of Padova, Padova, Italy
It is generally accepted that conodonts went extinct at the end of the Triassic, but younger conodont fossils have been reported, and it is becoming clear that conodont extinction occurred asynchronously across different regions. Although some reports of post-Triassic conodonts have been disproven, Lower Jurassic conodonts have been found in the Buda area of Hungary and the Inuyama area of Japan. Here, we report the discovery of more conodonts Misikella posthernsteini above the first occurrence of the typical Jurassic radiolarian Pantanellium tanuense, which is not know from the Triassic, in the Kastuyama section, Inuyama area, reaffirming the authenticity of the Lower Jurassic conodonts. The conodonts survived into the Jurassic in the Inuyama area might be due to their remote locations relative to the Central Atlantic Magmatic Province, which buffered them from hypoxia and ocean acidification. Although conodont survived into the Lower Jurassic, they failed to recover and quickly went extinct in post-extinction ecosystems. The “dead clades walking” (DCWs) of conodont may have been due to protracted ocean acidification in the earliest Jurassic. Food scarcity and competition with other organisms may have led to the eventual extinction of conodonts.
Introduction
Conodonts are important index fossils in marine strata (Rigo et al., 2018; Tong et al., 2021). Although it is generally accepted that conodonts appeared in the Cambrian and went extinct at the end of the Triassic (Clark, 1983), some post-Triassic conodonts have been reported. For instance, Diebel (1956) reported “Upper Cretaceous” conodonts from West Africa; Nohda and Setoguchi (1967) reported “Upper Jurassic” conodonts from Japan. Although these two examples were later shown to be of Triassic age (Müller and Mosher, 1970), the reports had already attracted attention. Nonetheless, Kozur and Mock (1991) identified a new ramiform conodont Neohindeodella detrei in the Várhegy section (Buda, Hungary), and subsequent studies confirmed that its highest appearance in the Várhegy and nearby Csővár sections is above Lower Jurassic radiolarians and ammonoids (Kozur, 1993; Pálfy et al., 2007; Götz et al., 2009; Korte and Kozur, 2011). Thus, N. detrei became the first confirmed ramiform conodont to have survived into the Early Jurassic. In addition, Hori (1997) found some conodonts in Toarcian (Lower Jurassic) chert beds from southwestern Japan, but these post-Triassic conodonts are considered reworked. Recently, Golding (2020) reported a single conodont Neohindeodella sp. at the Grotto Creek section in southeast Alaska originated from the same bed as the first occurrence (FO) of the Hettangian ammonoid Psiloceras sp., but the age of Neohindeodella sp. has been assigned to Triassic-Jurassic boundary transition in Caruthers et al. (2022). Du et al. (2020) identified conodont species of the genus Misikella that appear above the FO of the Lower Jurassic radiolarian species, including Pantanellium tanuense, Archaeocenosphaera laseekensis, in the Katsuyama section, Inuyama, Japan.
The extinction of the conodonts is one of the most important events in the end-Triassic mass extinction (ETE). Studying the Lower Jurassic conodonts is thus important to both refining stratigraphy and understanding the extinction pattern of conodonts, and more broadly the ETE. Here, we review well-known reports of post-Triassic conodonts around the world and incorporate the newly discovered Lower Jurassic conodonts in the Katsuyama section to discuss the temporal pattern and mechanism of conodont extinction.
Geological setting
The Katsuyama section (35°25′22”N, 136°58′16″E) is in the Inuyama area, central Honshu Island, Japan (Figures 1B,C). The entire section is continuous, about 32 m thick, and comprises the Upper Triassic to the Lower Jurassic rhythmically layered siliceous rocks and shales (Hori, 1990). Single layers are typically 1–10 cm thick and exhibit different colors, including black, green, red, and purple (Hori, 1992). Upper Triassic strata are dominated by dark red siliceous rocks that transition into purple cherts and shales at the Triassic-Jurassic (T-J) boundary; the Lower Jurassic strata are red again (Figure 2). The layers of the T-J boundary in the Katsuyama section are about 3.5 m thick (Figure 1D).
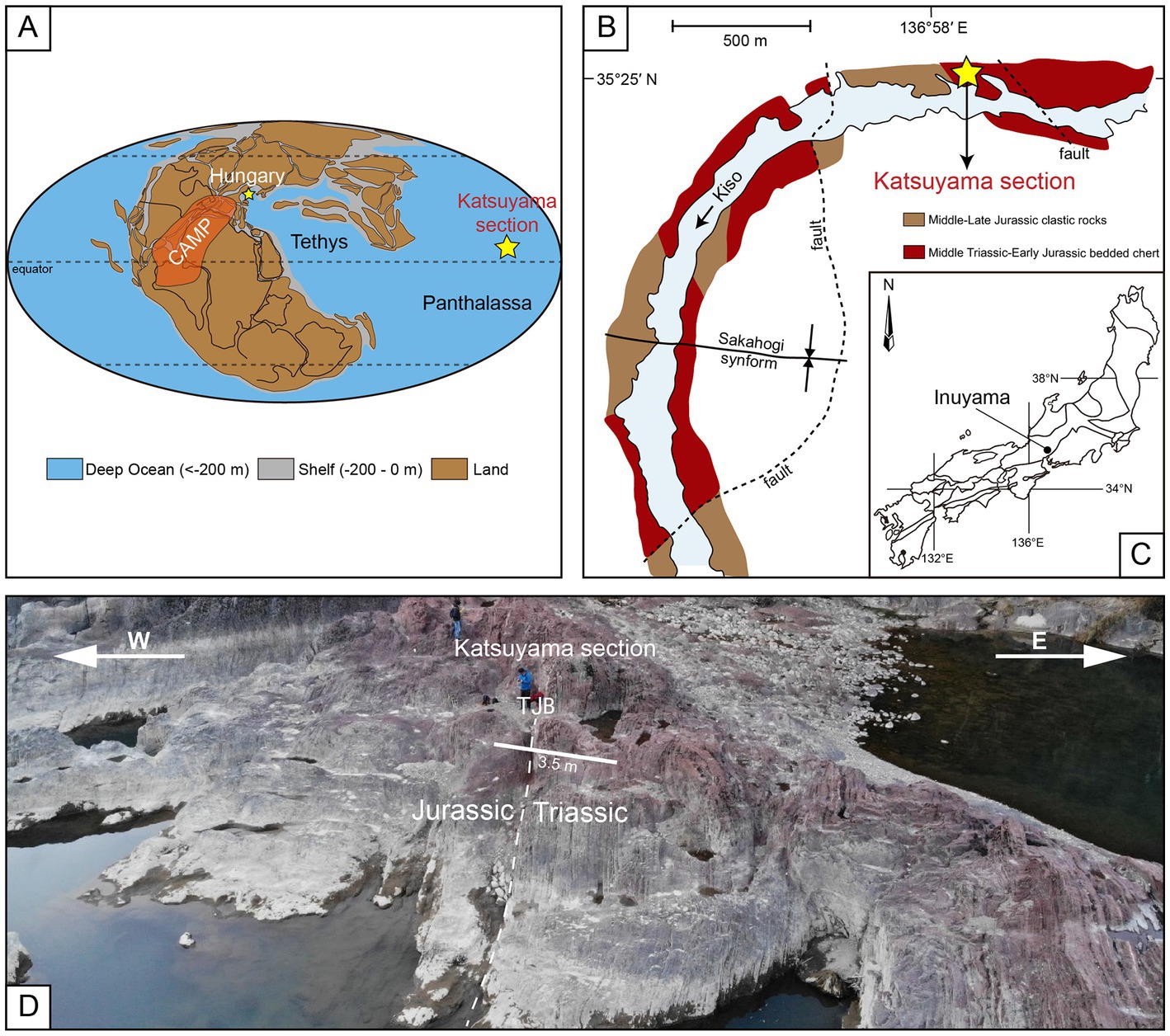
Figure 1. (A) Global paleogeographic map of the end Triassic (modified after Du et al., 2020); CAMP, Central Atlantic Magmatic Province. (B) Geological map of the Inuyama area (modified after Fujisaki et al., 2018). (C) Location of the Inuyama area in central Japan. (D) Annotated photograph of an outcrop of the Katsuyama section; TJB, Triassic-Jurassic boundary.
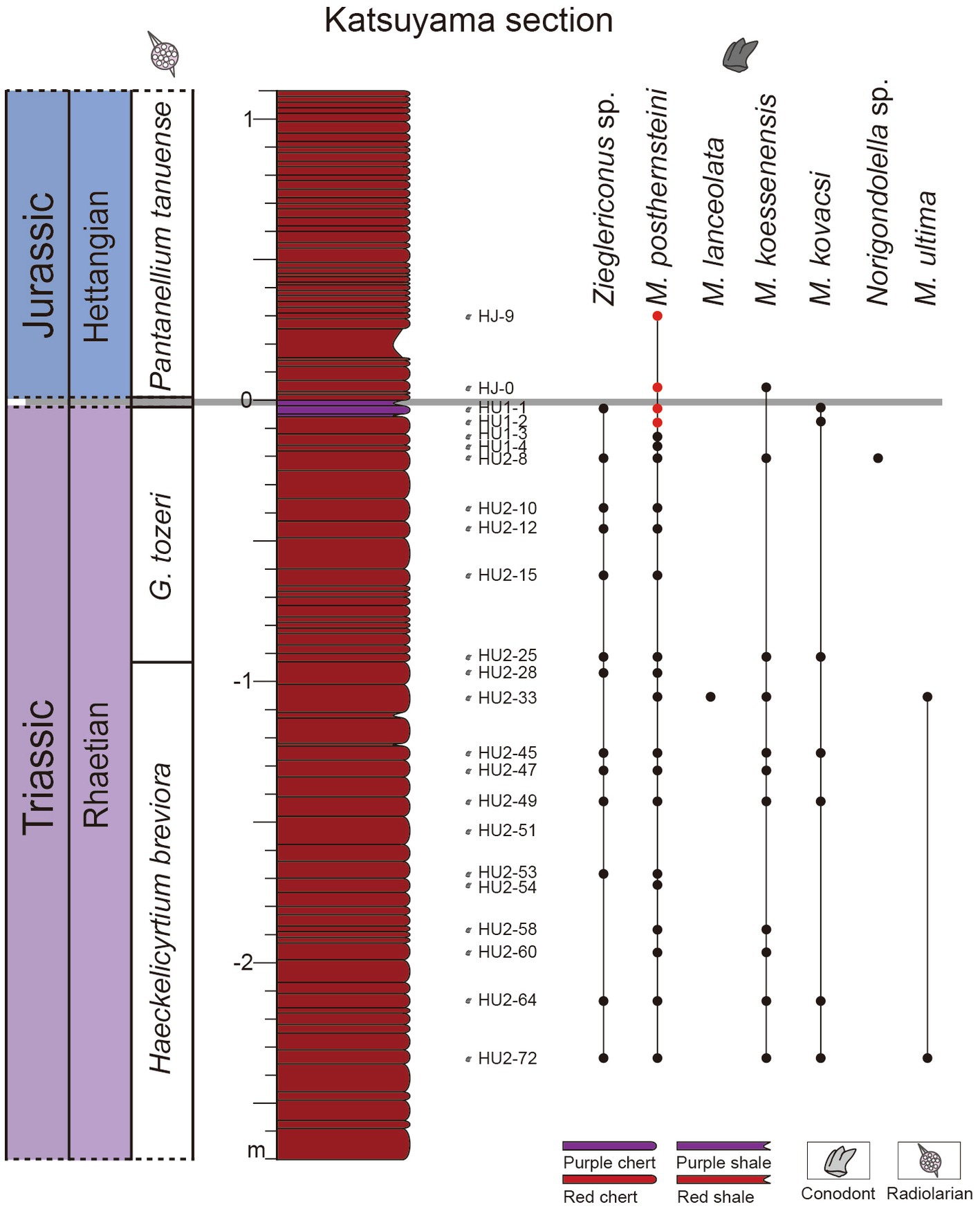
Figure 2. Lithology of the Katsuyama section. Radiolarian biozones are from Carter and Hori (2005), the conodont distribution is from Du et al. (2020). Samples used in this study are HJ-9, HJ-0, HU 1–1, and HU 1-2 (red dots).
During the Late Triassic to Early Jurassic, Inuyama was in a low-paleolatitude pelagic area of Panthalassa (Figure 1A; Ando et al., 2001; Uno et al., 2015), the deposition rate was very slow (1–7 mm/kyr), and the siliceous rocks were deposited below the carbonate compensation depth (i.e., at least 4,000 M depth; Matsuda and Isozaki, 1991).
Hori (1992) and Carter and Hori (2005) established globally comparable radiolarian biozones in the Katsuyama section: the Globolaxtorum tozeri Zone is the last Upper Triassic radiolarian zone and the T-J boundary is defined by their last appearance of this index species (Carter and Hori, 2005), although younger than the T-J boundary as defined by the FO of ammonoid Psiloceras spelae tirolicum. The first Lower Jurassic radiolarian zone is the Pantanellium tanuense Zone. Ikeda and Tada (2014) established an astronomical time scale (ATS) in the Inuyama area, and the Inuyama-ATS anchored at the end-Triassic radiolarian extinction event as 201.4 ± 0.2 Ma, the age was revised in Bôle et al. (2022) as 201.57 ± 0.2 Ma. Du et al. (2020) identified pectiniform conodonts Zieglericonus sp., Norigondolella sp., Misikella posthernsteini, M. lanceolata, M. koessenensis, M. kovacsi, and M. ultima in the Katsuyama section, as well as some ramiform conodont elements. Some M. posthernsteini and M. koessenensis are found above the FO of the Lower Jurassic radiolarian P. tanuense (Figure 2).
Organic carbon isotope profiles have been established in the Inuyama area (Kuroda et al., 2010; Okada et al., 2015; Fujisaki et al., 2018). Okada et al. (2015) and Fujisaki et al. (2018) obtained the organic carbon isotope data from cherts and mudstones respectively, but the results of them are obviously different. The values in Fujisaki et al. (2018) are more scattered, from −29.07‰ to −21.10‰. They identified three prominent negative carbon isotopic excursions (NCIEs) in the Katsuyama section and correlated these three NCIEs, respectively, with the precursor, initial, and main CIEs in the Tethys. However, the δ13Corg values obtained by Okada et al. (2015) from cherts are relatively stable, ranges from −25.7‰ to −24.1‰. The reasons for this difference still need further studies and discussions.
Materials and methods
The new conodonts reported herein were found in chert samples HJ-9, HJ-0, HU 1-1, and HU 1-2 collected from the Katsuyama section in 2019 (Figure 2). About 100 grams of cherts per sample were dissolved in hydrofluoric acid at Kyushu University, Japan, following the method described by Yamashita et al. (2018). Conodonts were picked from the sorted residue under binocular stereoscope. The conodonts are now preserved in the Laboratory of Paleoceanography, Paleoclimate and Paleontology, Institute of Sedimentary Geology, Chengdu University of Technology.
Results
Seventeen conodonts were collected from the Jurassic samples. Most of the conodonts are not particularly well preserved, but some can be identified at species level and consist in Misikella posthernsteini and M. posthernsteini? from samples HJ-9, HJ-0, HU 1-1, and HU 1-2 (Figure 3).
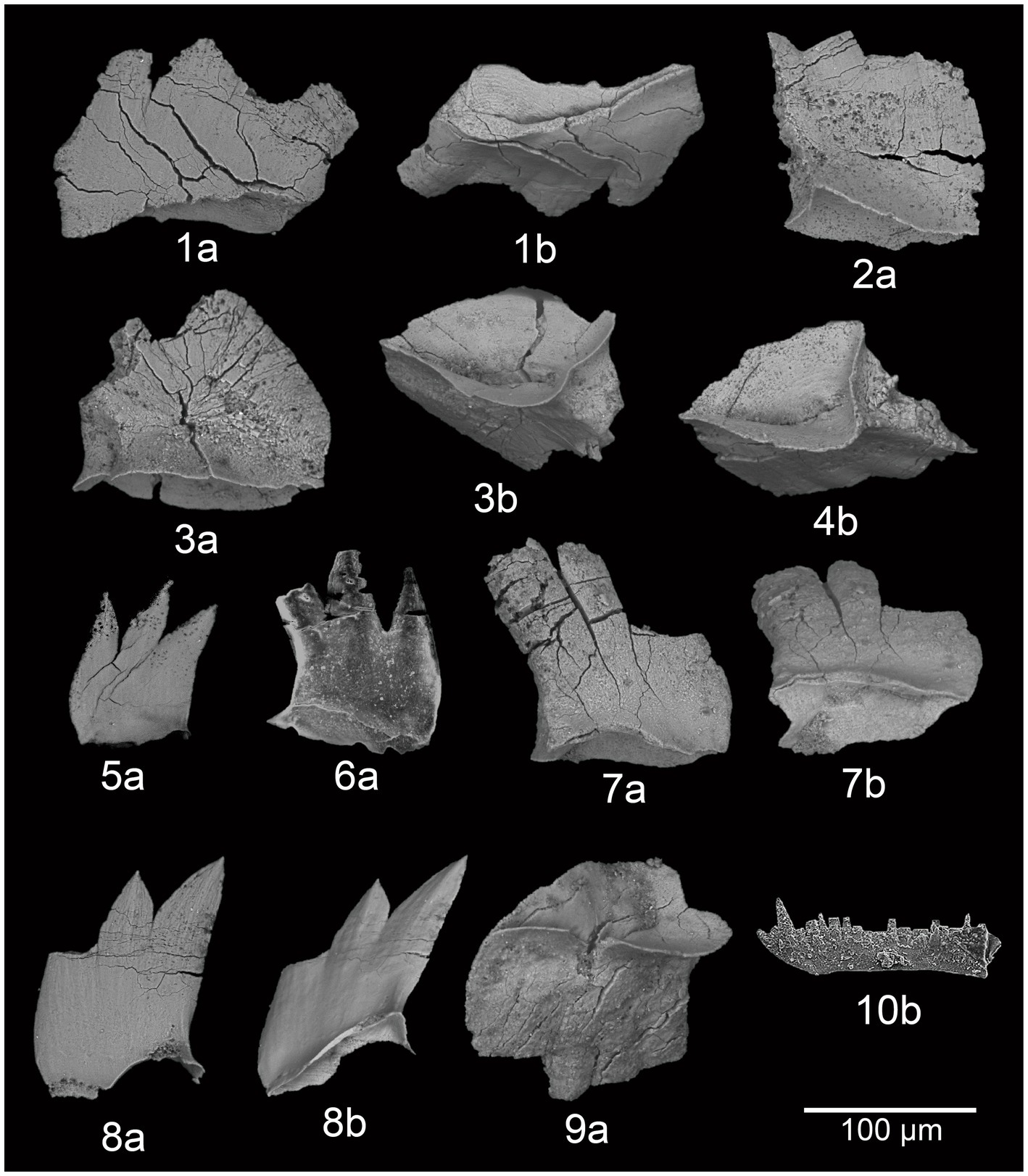
Figure 3. SEM photos of conodonts from the Kastuyama and Csővár sections; a and b indicate lateral and lower views, respectively. (1, 3) Misikella posthernsteini?, HJ-0; (2, 4) Misikella posthernsteini, HJ-0; (5) Misikella posthernsteini, HU 1-2; (6) Misikella posthernsteini, HU 1-1; (7) Misikella posthernsteini, HJ-9; (8, 9) Misikella posthernsteini from Du et al. (2020); (10) Neohindeodella detrei from Pálfy et al. (2007).
Conodonts in samples HJ-9 and HJ-0 were stratigraphically above the FO of the Lower Jurassic radiolarian Pantanellium tanuense (Figure 2). The positions of sample HJ-0 and HJ-9 are about 6 cm and 33 cm higher than the FO of P. tanuense, respectively. Du et al. (2020) had already described Lower Jurassic conodonts M. posthernsteini and M. koessenensis at the levels of HJ-9 and HJ-0. Here, we report the discovery of more conodonts above the P. tanuense from the Kastuyama section, it is a supplement to the study of Jurassic conodonts in the Inuyama area, also reaffirming the authenticity of the Lower Jurassic conodonts in the study area.
Discussion
Reported post-Triassic conodonts
“Post-Triassic” conodonts from West Africa and Japan
Diebel (1956) reported conodonts from West Africa to be of Late Cretaceous in age based on an individual ammonoid fossil found with them. However, the most abundant species they discovered were “Polygnathus mungoensis” and Enantiognathus ziegleri. Mosher (1968) reclassified “P. mungoensis” into the genus Epigondolella, which is abundant in Ladinian (Middle Triassic) rocks in some areas of the United States and Canada. Currently, mungoensis has again been reclassified into the genus Budurovignathus, and it is a typical representative of the Ladinian to lower Carnian. Enantiognathus ziegleri was thought to be a ramiform conodont that appeared throughout the Triassic. However, Koike (2004) suggested that E. ziegleri may be the S1 element of B. mungoensis. Therefore, conodonts from West Africa generally do not belong to the Upper Cretaceous, but the Middle Triassic to lower part of Upper Triassic.
Nohda and Setoguchi (1967) reported the Upper Jurassic conodonts in Japan, but they were proven to be the Triassic conodont Epigondolella abneptis. Epigondolella abneptis was originally discovered in Anisian (Middle Triassic) to Norian (Upper Triassic) strata (Huckriede, 1958), but the original description was insufficiently detailed and many conodonts with distinct morphological characteristics were misclassified as E. abneptis (Karádi, 2018). Therefore, Moix et al. (2007) described E. abneptis in more detail, and now it has a confirmed age range limited to the Norian, in the Upper Triassic (Karádi, 2018). Nohda and Setoguchi (1967) found these Triassic conodonts from a limestone block in the Jurassic accretionary complex (Chichibu Belt) because they did not realize that the limestone is included as an extraneous block in the melange complex.
Furthermore, conodonts, especially pectiniform elements, evolved rapidly, so it highly unlikely that morphological characteristics could have remained unchanged from the Triassic to the Upper Jurassic, much less the Upper Cretaceous (Müller and Mosher, 1970). In addition, determinations that conodonts are post-Triassic age must be confirmed by other coeval fossils and/or reliable chronological markers.
Lower Jurassic conodonts from Hungary
Kozur and Mock (1991) established two important Upper Triassic conodont species in the Várhegy section. The first, Misikella ultima (‘the last Misikella’), is an important conodont element of middle–late Rhaetian age that roughly corresponds to the Upper Triassic ammonoid Choristoceras marshi Zone. The second is the ramiform Neohindeodella detrei, the highest occurrence of which is above the uppermost Triassic ammonoid Choristoceras. Accordingly, Kozur and Mock (1991) defined the age of N. detrei as late Rhaetian to early Hettangian (Early Jurassic), and Kozur (1993) subsequently identified other N. detrei above Lower Jurassic radiolarians, indicating that some N. detrei survived into the Hettangian (Figure 4C). Continued research in the Várhegy and Csővár sections found that the highest occurrence of N. detrei is above the Lower Jurassic ammonoid Psiloceratids indet. and radiolarian Canoptum merum (Kozur, 1993; Pálfy et al., 2007; Götz et al., 2009; Korte and Kozur, 2011). Neohindeodella detrei is the first conodont to have been extant into the Lower Jurassic before the discovery of the Lower Jurassic conodonts in the Katsuyama section (Japan).
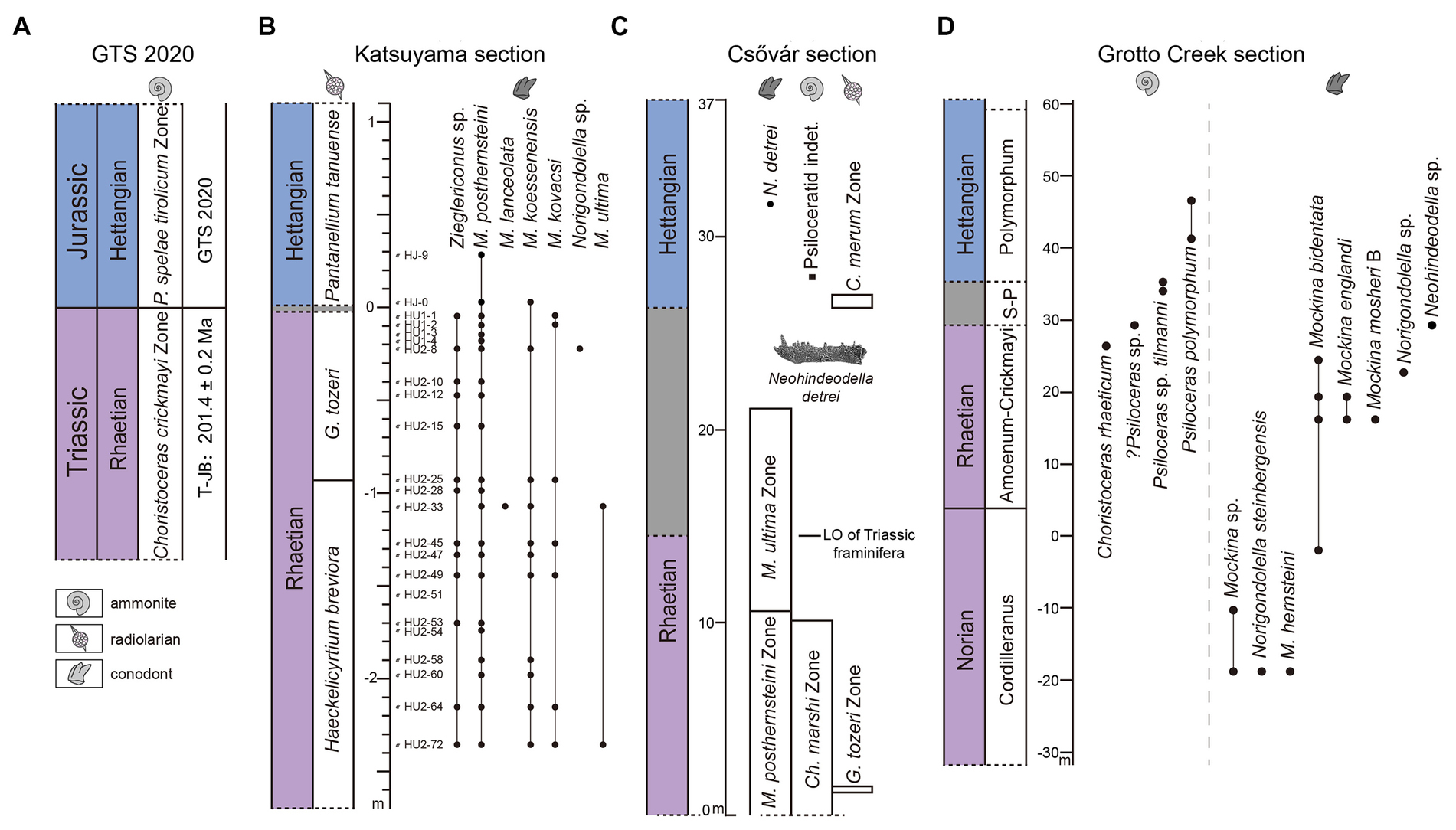
Figure 4. Comprehensive correlation chart. (A) The Global Boundary Stratotype Section and Points for the Hettangian (Jurassic) is identified by the FO of the Lower Jurassic ammonoid Psiloceras spelae tirolicum (Hillebrandt et al., 2013), and the age of the T-J boundary, 201.4 ± 0.2 Ma, is from GTS 2020 (Hesselbo et al., 2020). (B) In the Katsuyama section, conodont samples HJ-9 and HJ-0 are above the FO of the Lower Jurassic radiolarian. (C) In the Csővár section, Hungary, conodont Neohindeodella detrei appears above the Lower Jurassic ammonoid Psiloceratids indet. and radiolarian Canoptum merum. The image of N. detrei is from Pálfy et al. (2007). (D) In the Grotto Creek section, Alaska, conodont Neohindeodella sp. occurs with ammonoid? Psiloceras sp. (Caruthers et al., 2022).
However, the certainty of the Jurassic age of the conodonts N. detrei has always been controversial. First, the age of radiolarian C. merum is not considered exclusively Jurassic, Tekin et al. (2020) presents a range of Rhaetian to lower Sinemurian for this radiolarian in southern Turkey. Second, poor preservation hinders firm identification of ammonoids (Pálfy et al., 2007). Third, the presence of slump structures in the section cast doubt on whether N. detrei truly extended into the Lower Jurassic (Pálfy et al., 2007).
Lower Jurassic conodonts from Alaska
Golding (2020) reported conodonts from the Norian to Hettangian in the Grotto Creek section in southeast Alaska. The highest conodont sample corresponds to the same bed where the Hettangian ammonoid Psiloceras sp. first occurs, and included a single conodont specimen referred to Neohindeodella sp. The discovery of an additional specimen of the same genus Neohindeodella as in Hungary from Jurassic rocks at a different location likely suggested that conodonts did survive into at least the earliest Hettangian. However, Caruthers et al. (2022) thinks the classification of ammonoid Psiloceras sp. is dubious, they reclassified it as Psiloceras sp. Therefore, the Jurassic age of the ammonoid Psiloceras sp. and conodont Neohindeodella sp. originated from the same bed as the ammonoid Psiloceras sp. is uncertain. In the Grotto Creek section, there is a stratigraphically continuous T-J boundary transition interval from meter 29.42 to 35.46 between the bed with the ammonoid Psiloceras sp. along with conodont Neohindeodella sp., and the ammonoid Psiloceras cf. tilmanni (Figure 4D). The reclassification of Psiloceras sp. makes the Jurassic age of Neohindeodella sp. uncertain, but does not rule out the possibility that Neohindeodella sp. belongs to the Lower Jurassic.
Lower Jurassic conodonts in the Inuyama area of Japan
In the Katsuyama section, the T-J boundary is generally defined by radiolarians due to the lack of ammonoid fossils. However, the radiolarian-defined T-J boundary is inconsistent with the standard T-J boundary based on ammonoids. Therefore, although it can be confirmed that some pectiniform conodont elements in the Katsuyama section are Lower Jurassic based on their occurrence relative to Hettangian radiolarians, it is necessary to discuss the radiolarian-defined T-J boundary in the Katsuyama section.
When the International Subcommittee on Jurassic Stratigraphy decided the primary marker of the T-J boundary, they included the ammonoid Psiloceras spelae (Ferguson Hill section, United States, and Kuhjoch East section, Northern Calcareous Alps, Austria), the ammonoid Psiloceras planorbis (St. Audrie’s Bay section, England, and Waterloo Bay section, Northern Ireland), a major evolutionary replacement of radiolarian fauna (Kunga Island section, Queen Charlotte Islands, Canada), and a carbon isotopic excursion (Ferguson Hill section). Psiloceras spelae from the Kuhjoch East section was eventually selected as the primary marker (Hillebrandt et al., 2013). Therefore, the Global Boundary Stratotype Section and Point (GSSP) for the Hettangian is now the FO of primary marker ammonoid Psiloceras spelae tirolicum in the Kuhjoch East section (Figure 4A; Hillebrandt et al., 2013). However, a numerical age has not been obtained in the Kuhjoch East section to constrain the age of the T-J boundary.
Numerous radiometric ages have been reported from North American tuff layers produced by the Central Atlantic Magmatic Province (CAMP), along with biostratigraphic age constraints. For example, Schoene et al. (2010) measured a zircon age of 201.33 ± 0.13 Ma in a tuff layer 1.5 m above the FO of P. spelae in the New York Canyon section and they also estimated the age of the T-J boundary in the Pucara section, Peru, to be 201.31 ± 0.18 Ma based on radiometric ages above and below the FO of ammonoid P. spelae. Guex et al. (2012) also obtained a zircon U–Pb age of 201.45 ± 0.14 Ma in a tuff layer 4.5 m below the FO of P. spelae in the Pucara section. Hillebrandt et al. (2013) estimated the mean age of the T-J boundary to be 201.3 Ma based on previous studies. Subsequently, Hesselbo et al. (2020) combined multiple datasets to estimate the age of the T-J boundary to be 201.4 ± 0.2 Ma in the Geologic Timescale (GTS) 2020 (Figure 4A).
Although not selected as the primary marker of the T-J boundary, the evolutionary turnover of radiolarian fauna remains a landmark event across the T-J boundary transition. Indeed, changes in radiolarian fauna have been used to define the T-J boundary in regions where ammonoids are absent. The Kunga Island section was a candidate section for the Hettangian GSSP based on the major evolutionary replacement of radiolarian fauna at the T-J boundary (Hillebrandt et al., 2013). There, the change of radiolarian fauna is represented by the disappearance of the Upper Triassic radiolarian Globolaxtorum tozeri. However, the ammonoid-defined T-J boundary is higher than the highest occurrence of G. tozeri. There is thus a brief interval between the disappearance of G. tozeri and the FO of the Lower Jurassic radiolarians Canoptum merum and Pantanellium tanuense in the Kunga Island section, and the exact position of the T-J boundary could not be determined there due to the absence of ammonoid P. spelae.
In the Katsuyama section, the T-J boundary was also defined based on radiolarians due to the absence of ammonoids since the basin was deposited below the CCD. Previous studies have established high-precision radiolarian zones in the Inuyama area (Hori, 1988, 1992; Carter and Hori, 2005; Hori et al., 2007), including G. tozeri Zone for the upper Rhaetian and P. tanuense Zone for the lower Hettangian (Carter and Hori, 2005). Carter and Hori (2005) correlated radiolarian assemblages in strata near the T-J boundary between Queen Charlotte Islands and Inuyama. Although the environments and depositional rates of the two regions differ, the evolutionary patterns of radiolarian species are quite similar, and radiolarian zones are well correlated: the Rhaetian G. tozeri Zone in the two regions correspond directly, and the Hettangian C. merum Zone in Queen Charlotte Islands corresponds to the Hettangian P. tanuense Zone in the Inuyama area (Carter and Hori, 2005). Ikeda and Tada (2014) established an astronomical time scale (ATS) in the Inuyama area, and the Inuyama-ATS anchored at the end-Triassic radiolarian extinction event as 201.4 ± 0.2 Ma, and recently this age has been revised as 201.57 ± 0.2 Ma (Bôle et al., 2022). In the Katsuyama section, there was also a brief period of transition between the disappearance of G. tozeri and the first appearance of the Lower Jurassic radiolarian P. tanuense. The ammonoid-defined T-J boundary might be later than the last appearance of G. tozeri and predate the FO of P. tanuense in the Katsuyama section.
In general, the radiolarian zones on either side of the T-J boundary in the Katsuyama section are well correlated to coeval radiolarian zones of the Kunga Island section (Carter and Hori, 2005). More importantly, the conodonts found in the Katsuyama section not only occurred above the radiolarian-defined T-J boundary, but also above the base of the P. tanuense Zone. The conodonts occurred beyond the first appearance of Hettangian radiolarians, such as P. tanuense, Archaeocenosphaera laseekensis, Amuria impensa, Praehexasaturnalis tetraradiatus, Spongotrochus? sp. sensu Carter (1994) and some primitive species of Charlottea, Parashuum, Udalia in the Katsuyama section.
Du et al. (2020) collected two elements of the genus Misikella (i.e., M. posthernsteini and M. koessenensis) in Lower Jurassic strata of the Katsuyama section, at positions HJ-9 and HJ-0, respectively. Herein, we collected more M. posthernsteini from samples HJ-9 and HJ-0, and the highest appearance of M. posthernsteini was about 0.33 m above the FO of the Hettangian radiolarian P. tanuense (Figure 4B). In the western Tethys, Rigo et al. (2018) identified the FO of M. posthernsteini as a marker for the base of the Rhaetian stage. In North America, M. posthernsteini apparently occurs in the middle–late Rhaetian (Orchard et al., 2007). Misikella posthernsteini is generally believed to have survived until the end of the Triassic, for instance in the Inuyama area of Japan, British Columbia of Canada and Steinbergkogel of Austria (Krystyn et al., 2007; Orchard et al., 2007; Ikeda and Tada, 2014). Actually, the duration of this conodont is longer than previously known. The records in the Katsuyama section indicate that some M. posthernsteini survived also into the Lower Jurassic.
It is important to mention that Hori (1997) first reported conodonts that occasionally occurred in Toarcian (Jurassic) bedded chert from the Inuyama areas in Japan. The reported conodonts are very similar to the Triassic forms according to Hori (1997). However, the Toarcian radiolarian biostratigraphy reveals that the conodonts could be reworked, because it is unlikely that Triassic conodonts suddenly appeared in Toarcian strata. Nevertheless, the strata of the studied T-J boundary section are continuous and without a clear depositional discontinuity, and any intraclast grains have been not found in the samples yet. Significantly, the conodonts found in the Katsuyama section occurred continuously from the Upper Triassic to the Lower Jurassic strata and the radiolarian biostratigraphy of the T-J boundary Katsuyama section is well-correlated with other regions, highlighting that there is no evidence that the Lower Jurassic (Hettangian) conodonts might have been reworked.
Implications for conodont extinction
During the ETE, one of the five Phanerozoic mass extinction events (Hallam, 1996), a large number of marine and terrestrial organisms were affected or went extinct (McElwain et al., 1999, 2009). The extinction of the conodonts might be the most remarkable event of the ETE, and there are three outstanding questions regarding their extinction. What was the main reason for their extinction, and when and how (i.e., by what mechanism) did it occur?
As one of the most remarkable events of the ETE, the cause of conodont extinction remains debated. The most widely accepted hypothesis is that multiple pulses of the CAMP caused the larger ETE (Marzoli et al., 1999; Schlische et al., 2003) and directly led to the extinction of the conodonts (Fujisaki et al., 2018).
Most of the mass extinctions and some of the biotic turnover events are associated with perturbations of the carbon cycle (Fox et al., 2020). It has also been recorded during the T-J boundary transition. The prevailing view is that the massive exogenous isotopically light carbon from the emplacement of CAMP resulted the profound perturbations in the carbon cycle (Hesselbo et al., 2002; Pálfy et al., 2007; Thibodeau et al., 2016; Fujisaki et al., 2018; Du et al., 2020). Three NCIEs occurred in both the Tethys and Panthalassa and they are considered to have resulted from global carbon perturbations due to CAMP volcanism (e.g., Hesselbo et al., 2002; Thibodeau et al., 2016; Fujisaki et al., 2018; Du et al., 2020). Fujisaki et al. (2018) identified three NCIEs, NCIE1, NCIE2, and NCIE3, in the Katsuyama section, and proposed that the three NCIEs correspond to the precursor, initial, and main CIEs in the Tethys, respectively. Based on global well-correlation of these three NCIEs, Du et al. (2020) considered that the three NCIEs were synchronous in the Tethys, eastern-Panthalassa and mid-Panthalassa. By using these three NCIEs as a calibration to establish the temporal pattern of conodont extinction event, conodonts disappeared at the end of the Upper Triassic in most areas (i.e., below the T-J boundary), but survived into the Lower Jurassic in the Buda and Inuyama areas (Du et al., 2020). However, although the three well-known global NCIEs are accepted by most people, another view considers that the NCIEs, especially for the initial-NCIE is not necessarily global (Fox et al., 2020; Bôle et al., 2022). Fox et al. (2020) used biomarker and compound specific carbon isotopic data in the Bristol Channel Basin (United Kingdom) where the iconic initial-NCIE was first observed demonstrated that the initial-NCIE is a record of microbial-mat development and other ecological changes driven by regional sea-level change, not because of the input of massive exogenous isotopically light carbon. Similarly, Okada et al. (2015) studied the carbon isotope from cherts in the Inuyama area show a different result compared with Fujisaki et al. (2018). Although there is debate of organic carbon isotope studying, it does not affect the conclusion that the extinction of conodont was asynchronous since the calibration is not only based on the organic carbon isotope curves, but also supported by integrated biostratigraphy of ammonoids, radiolarians, and palynomorphs (Du et al., 2020).
The mechanism by which CAMP volcanism caused conodont extinction remains debated. One view is that the large amounts of greenhouse gases (e.g., CO2) released by the CAMP resulted in global warming that ultimately led to the extinction of conodonts and other organisms (McElwain et al., 2005; Korte et al., 2009; Schaller et al., 2012; Blackburn et al., 2013). In this context, conodonts in shallow environments or near the eruptions should have been the first affected, whereas those in deep waters, benthic habitats, or environments far from the CAMP would only have been affected after some delay. However, the degree to which the release of greenhouse gases by CAMP volcanism affected global temperatures remains to be demonstrated (Du et al., 2020). The second possibility is that a widespread perturbation of the oceanic nitrogen cycle resulted in reduced nitrogen availability, limiting productivity throughout the oceans. However, existing evidence indicates that nitrogen cycle disturbances at the end of the Triassic were only regional (Schoepfer et al., 2016). Therefore, unless a global nitrogen cycle perturbation can be demonstrated, it is unlikely that regional nitrogen cycle perturbations could have led to the global extinction of conodonts. The third possible mechanism is ocean hypoxia. Biomarker tests indicate that some regions of the western Tethys and Panthalassa became hypoxic during the Late Triassic (Richoz et al., 2012; Jaraula et al., 2013; Kasprak et al., 2015; Schoepfer et al., 2016; Fox et al., 2022). However, major, trace, and rare earth element analyses have confirmed that the middle of Panthalassa remained oxic during the late Rhaetian to the T-J boundary transition (Fujisaki et al., 2018). Therefore, the living conditions there should have been suitable for conodont survival. Furthermore, most Upper Triassic conodonts, including Misikella, have been proved to be surface dwellers (Rigo and Joachimski, 2010; Rigo et al., 2012; Trotter et al., 2015) and it is unlikely that reducing conditions might have set on the upper zone of the column water. Another hypothesis is that ocean acidification caused conodont extinction. The large amount of CO2 released by CAMP volcanism may have led to seawater acidification in some areas of the western Tethys and Panthalassa (Hautmann, 2004; Ikeda et al., 2015), and the ensuing unfavorable conditions, e.g., food scarcity, may have propagated the impacts to conodonts worldwide. Fujisaki et al. (2018) proposed that the ocean acidification and recurring shallow-marine anoxia events which caused by protracted CAMP volcanisms may place environmental stresses on the biosphere and eventually resulted in the end-Triassic biotic crisis. Fox et al. (2022) used high-resolution biomarker and compound-specific isotope revealed that the intensive euxinia and acidification driven by CAMP formed a two-pronged kill mechanism at the ETE. This also could be the mechanism of conodont extinction.
However, why some conodonts survived into the Lower Jurassic in the Buda and Inuyama areas remains unclear. In the Buda area, N. detrei was collected above the FO of a typical Jurassic psiloceratid ammonoid in the Csővár section (Pálfy et al., 2001). Unfortunately, those N. detrei specimens are uniquely S elements of an unknown conodont apparatus and are never found associated with P and M elements, so very little information is available about the conodont’s lifestyle. Despite the lack of sufficient knowledge about N. detrei, one possible reason for their survival into the Jurassic in the Buda area is that they lived in a restricted basin environment near the Dachstein carbonate platform (Haas et al., 1995; Pálfy et al., 2007) and were thus less affected by global events. Nonetheless, some outstanding questions about N. detrei remain. For example, why have they only been identified in the Buda area, and why have no P and/or M elements been found?
The Inuyama area was in the middle of Panthalassa during the Late Triassic. Although shallow marine areas were anoxic during the T-J boundary transition, the seawater remained oxic in pelagic areas such as Inuyama (Ikeda et al., 2015; Fujisaki et al., 2018). Nonetheless, the effects of ocean acidification were inevitable. The combination of ocean acidification and marine anoxia events can explain the biotic crisis at the end of Triassic, but cannot explain why conodonts in the Inuyama area survived longer than other places in the same environment. In addition, Misikella were surface-water dwellers (Trotter et al., 2015) and would have been affected by both ocean acidification and hypoxia. The explanation may be that, because the Inuyama area was in an open sea environment far from the CAMP, hypoxia and ocean acidification were less severe than areas closer to the CAMP. This relatively less harsh environment may have sustained these conodonts into the Lower Jurassic.
The “dead clades walking” of conodont
Based on the Lower Jurassic conodonts discovered so far, both Neohindeodella and Misikella were extant from the Upper Triassic to the Lower Jurassic. Yet, no exclusively Jurassic conodonts have been found. Thus, another outstanding question is why those conodonts went extinct instead of slowly recovering like conodonts that survived the end-Permian mass extinction. The phenomenon of clades survives major extinction event, but fail to recover and very quickly goes extinct in post-extinction ecosystems is called “dead clades walking” (DCWs), this term coined by Jablonski (2002). The DCWs of conodont is an issue worthy of further study. Conodonts suffered several extinctions and faunal turnovers from the Carnian (Upper Triassic) to the end of Triassic before their final extinction (Rigo et al., 2018; Du et al., 2021). One of the main events was in the late Julian (early Carnian), during which only few conodonts survived beyond that event (Kozur, 1989; Rigo et al., 2007). Afterwards, the diversity of conodonts began to recover, more than ten genera of pectiniform conodont (e.g., Mazzaella, Carnepigondolella, Metapolygnathus, Mockina, Ancyrogondolella, Epigondolella, Orchardella, Zieglericonus, Parvigondolella, Norigondolella, Misikella) appeared. However, only three genera (Zieglericonus, Norigondolella, Misikella) of them appeared at the end of the Triassic, and finally only Misikella survived the ETE to the lowermost Jurassic, but it also became extinct soon. Compared with Zieglericonus and Norigondolella in the same environment, the advantage of Misikella was that the diversity of species was more abundant (Figure 2), whether it was in the Inuyama area or other regions. Therefore, it had a greater advantage in the competition for survival, allowing it to survive until the Lower Jurassic. The eventual extinction of conodont survivors in the Early Jurassic may have been due to protracted ocean acidification from the end Triassic to the earliest Jurassic (Fujisaki et al., 2018). Bôle et al. (2022) observed two negative silicon isotope excursions (NSIEs) within the end-Triassic radiolarian turnover interval, and it could reflect the drops of radiolarian productivity. In fact, the main anomaly in these NSIEs occurred within ~1 mm interval, implying <kyr or up to decadal scale drastic environmental perturbation across the T-J extinction interval. This may also be one of the factors resulting in the extinction of conodonts.
In general, there were various adverse environmental factors in the Inuyama area during T-J boundary transition that may have led to the eventual extinction of conodonts or the disappearance of other organisms. However, its specific mechanism remains to be clarified.
Conclusion
We reported the Upper Triassic and Lower Jurassic conodonts Misikella posthernsteini in four siliceous rock samples from the Katsuyama section (Inuyama area, Japan). The conodonts in samples HJ-9 and HJ-0 occur above the radiolarian-defined T-J boundary and the FO of the typical Lower Jurassic radiolarian Pantanellium tanuense, especially conodonts in the sample HJ-9 are about 33 cm above the P. tanuense. Therefore, these conodonts must have been extant in the Lower Jurassic. Although we did not find Lower Jurassic M. koessenensis like Du et al. (2020), these Lower Jurassic M. posthernsteini reaffirm the existence of Hettangian conodonts in the Inuyama area. The reason why some conodonts survived into the Lower Jurassic might be that the remote locations of the Inuyama area relative to the CAMP buffered them against hypoxia and ocean acidification resulting from protracted volcanism, allowing them to survive beyond the T-J boundary. The DCWs of conodont may have been due to protracted ocean acidification, food scarcity and competition with other organisms may have led to the eventual extinction of conodonts.
Author contributions
YD conceived and designed the project. TO, YT, QW, and MR wrote the manuscript. All authors contributed to the article and approved the submitted version.
Funding
This work was supported by the Scientific Research Foundation of Chengdu University of Technology (10912-KYQD2021_09147), the Japan Society for the Promotion of Science (Grants JP19H00711 and JP20H00203 to TO) and the University of Padova, project DOR2281100 to MR.
Conflict of interest
The authors declare that the research was conducted in the absence of any commercial or financial relationships that could be construed as a potential conflict of interest.
Publisher’s note
All claims expressed in this article are solely those of the authors and do not necessarily represent those of their affiliated organizations, or those of the publisher, the editors and the reviewers. Any product that may be evaluated in this article, or claim that may be made by its manufacturer, is not guaranteed or endorsed by the publisher.
References
Ando, A., Kodama, K., and Kojima, S. (2001). Low-latitude and southern hemisphere origin of Anisian (Triassic) bedded chert in the Inuyama area, Mino terrane, Central Japan. J Geophys Res Solid Earth 106, 1973–1986. doi: 10.1029/2000JB900305
Blackburn, T. J., Olsen, P. E., Bowring, S. A., McLean, N. M., Kent, D. V., Puffer, J., et al. (2013). Zircon U-Pb geochronology links the end-Triassic extinction with the Central Atlantic Magmatic Province. Science 340, 941–945. doi: 10.1126/science.1234204
Bôle, M., Ushikubo, T., Hori, R. S., Baumgartner, P. O., Nakai, Y., and Ikeda, M. (2022). Si isotope ratio of radiolaria across Triassic–Jurassic transition in a pelagic deep-sea bedded chert (Inuyama, Japan). Glob. Planet. Chang. 215:103882. doi: 10.1016/j.gloplacha.2022.103882
Carter, E. S. (1994). “Evolutionary trends of latest Triassic (upper Norian) and earliest Jurassic (Hettangian) Radiolaria” in 3rd International Symposium on Jurassic Stratigraphy, Poitiers, France, 1991. eds. E. Cariou and P. Hantzperque, vol. 17 (Geobios, France: Mémoire Spécial), 111–119.
Carter, E. S., and Hori, R. S. (2005). Global correlation of the radiolarian faunal change across the Triassic–Jurassic boundary. Can. J. Earth Sci. 42, 777–790. doi: 10.1139/e05-020
Caruthers, A. H., Marroquín, S. M., Gröcke, D. R., Golding, M. L., Aberhan, M., Them, T. R. II, et al. (2022). New evidence for a long Rhaetian from a Panthalassan succession (Wrangell Mountains, Alaska) and regional differences in carbon cycle perturbations at the Triassic-Jurassic transition. Earth Planet. Sci. Lett. 577:117262. doi: 10.1016/j.epsl.2021.117262
Du, Y., Chiari, M., Karádi, V., Nicora, A., Onoue, T., Pálfy, J., et al. (2020). The asynchronous disappearance of conodonts: new constraints from Triassic-Jurassic boundary sections in the Tethys and Panthalassa. Earth-Sci. Rev. 203:103176. doi: 10.1016/j.earscirev.2020.103176
Du, Y., Onoue, T., Karádi, V., Williams, I. S., and Rigo, M. (2021). Evolutionary process from Mockina bidentata to Parvigondolella andrusovi: evidence from the Pizzo Mondello section, Sicily, Italy. J Earth Sci 32, 667–676. doi: 10.1007/s12583-020-1362-2
Fox, C. P., Cui, X., Whiteside, J. H., Olsen, P. E., Summons, R. E., and Grice, K. (2020). Molecular and isotopic evidence reveals the end-Triassic carbon isotope excursion is not from massive exogenous light carbon. Proc. Natl. Acad. Sci. 117, 30171–30178. doi: 10.1073/pnas.1917661117
Fox, C. P., Whiteside, J. H., Olsen, P. E., Cui, X., Summons, R. E., Idiz, E., et al. (2022). Two-pronged kill mechanism at the end-Triassic mass extinction. Geology 50, 448–453. doi: 10.1130/G49560.1
Fujisaki, W., Matsui, Y., Asanuma, H., Sawaki, Y., Suzuki, K., and Maruyama, S. (2018). Global perturbations of carbon cycle during the Triassic–Jurassic transition recorded in the mid-Panthalassa. Earth Planet. Sci. Lett. 500, 105–116. doi: 10.1016/j.epsl.2018.07.026
Golding, M. L. (2020). Conodonts from the Norian to Hettangian of Southeast Alaska, and a Review of Jurassic Conodont Occurrences [Conference Presentation Abstract]. Applications of Conodonts to Integrated Studies of Biostratigraphy, Chemostratigraphy and Sequence Stratigraphy—North American Pander Society Annual Meeting. Available at: https://gsa.confex.com/gsa/2020AM/webprogram/Paper354808.html
Götz, A. E., Ruckwied, K., Pálfy, J., and Haas, J. (2009). Palynological evidence of synchronous changes within the terrestrial and marine realm at the Triassic/Jurassic boundary (Csővár section, Hungary). Rev. Palaeobot. Palynol. 156, 401–409. doi: 10.1016/j.revpalbo.2009.04.002
Guex, J., Schoene, B., Bartolini, A., Spangenberg, J., Schaltegger, U., O'Dogherty, L., et al. (2012). Geochronological on post-extinction constraints recovery of the ammonoids and carbon cycle perturbations during the early Jurassic. Palaeogeogr. Palaeoclimatol. Palaeoecol. 346-347, 1–11. doi: 10.1016/j.palaeo.2012.04.030
Haas, J., Kovács, S., Krystyn, L., and Lein, R. (1995). Significance of late Permian-Triassic facies zones in terrane reconstructions in the alpine-north Pannonian domain. Tectonophysics 242, 19–40. doi: 10.1016/0040-1951(94)00157-5
Hallam, A. (1996). “Major bio-events in the Triassic and Jurassic” in Global Events and Event Stratigraphy in the Phanerozoic. ed. O. H. Walliser (Berlin, Heidelberg: Springer), 265–283.
Hautmann, M. (2004). Effect of end-Triassic CO2 maximum on carbonate sedimentation and marine mass extinction. Facies 50, 257–261. doi: 10.1007/s10347-004-0020-y
Hesselbo, S. P., Ogg, J. G., Ruhl, M., Hinnov, L. A., and Huang, C. J. (2020). “The Jurassic period” in Geologic Time Scale 2020. eds. F. M. Gradstein, J. G. Ogg, M. D. Schmitz, and G. M. Ogg (Amsterdam: Elsevier), 955–1021.
Hesselbo, S. P., Robinson, S. A., Surlyk, F., and Piasecki, S. (2002). Terrestrial and marine extinction at the Triassic-Jurassic boundary synchronized with major carbon-cycle perturbation: a link to initiation of massive volcanism? Geology 30, 251–254. doi: 10.1130/0091-7613(2002)030<0251:TAMEAT>2.0.CO;2
Hillebrandt, A. V., Krystyn, L., Kürschner, W. M., Bonis, N. R., Ruhl, M., Richoz, S., et al. (2013). The global stratotype sections and point (GSSP) for the base of the Jurassic system at Kuhjoch (Karwendel Mountains, northern calcareous Alps, Tyrol, Austria). Episodes 36, 162–198. doi: 10.18814/epiiugs/2013/v36i3/001
Hori, R. S. (1988). Some characteristic radiolarians from Lower Jurassic bedded cherts of the Inuyama area, Southwest Japan. In transactions and proceedings of the paleontological Society of Japan. New Ser 151, 543–563.
Hori, R. S. (1990). Lower Jurassic radiolarian zones of SW Japan. Transactions and proceedings of the paleontological Society of Japan. New Ser 159, 562–586.
Hori, R. S. (1992). Radiolarian biostratigraphy at the Triassic/Jurassic period boundary in bedded cherts from the Inuyama area, Central Japan. J. Geosci. Osaka City Univ. 35, 53–66.
Hori, R. S. (1997). The Toarcian radiolarian event in bedded cherts from southwestern Japan. Mar. Micropaleontol. 30, 159–169. doi: 10.1016/S0377-8398(96)00024-2
Hori, R. S., Fujiki, T., Inoue, E., and Kimura, J. I. (2007). Platinum group element anomalies and bioevents in the Triassic–Jurassic deep-sea sediments of Panthalassa. Palaeogeogr. Palaeoclimatol. Palaeoecol. 244, 391–406. doi: 10.1016/j.palaeo.2006.06.038
Huckriede, R. (1958). Die Conodonten der mediterranen Trias und ihr stratigraphischer Wert. Paläontol. Z. 32, 141–175. doi: 10.1007/BF02989028
Ikeda, M., Hori, R. S., Okada, Y., and Nakada, R. (2015). Volcanism and deep-ocean acidification across the end-Triassic extinction event. Palaeogeogr. Palaeoclimatol. Palaeoecol. 440, 725–733. doi: 10.1016/j.palaeo.2015.09.046
Ikeda, M., and Tada, R. (2014). A 70 million year astronomical time scale for the deep-sea bedded chert sequence (Inuyama, Japan): implications for Triassic–Jurassic geochronology. Earth Planet. Sci. Lett. 399, 30–43. doi: 10.1016/j.epsl.2014.04.031
Jablonski, D. (2002). Survival without recovery after mass extinctions. Proc. Natl. Acad. Sci. 99, 8139–8144. doi: 10.1073/pnas.102163299
Jaraula, C. M., Grice, K., Twitchett, R. J., Böttcher, M. E., LeMetayer, P., Dastidar, A. G., et al. (2013). Elevated pCO2 leading to late Triassic extinction, persistent photic zone euxinia, and rising sea levels. Geology 41, 955–958. doi: 10.1130/G34183.1
Karádi, V. (2018). Middle Norian conodonts from the Buda Hills, Hungary: an exceptional record from the western Tethys. J. Iber. Geol. 44, 155–174. doi: 10.1007/s41513-017-0009-3
Kasprak, A. H., Sepúlveda, J., Price-Waldman, R., Williford, K. H., Schoepfer, S. D., Haggart, J. W., et al. (2015). Episodic photic zone euxinia in the northeastern panthalassic Ocean during the end-Triassic extinction. Geology 43, 307–310. doi: 10.1130/G36371.1
Koike, T. (2004). Early Triassic Neospathodus (Conodonta) apparatuses from the Taho formation, Southwest Japan. Paleontol Res 8, 129–140. doi: 10.2517/prpsj.8.129
Korte, C., Hesselbo, S. P., Jenkyns, H. C., Rickaby, R. E., and Spötl, C. (2009). Palaeoenvironmental significance of carbon-and oxygen-isotope stratigraphy of marine Triassic–Jurassic boundary sections in SW Britain. J. Geol. Soc. 166, 431–445. doi: 10.1144/0016-76492007-177
Korte, C., and Kozur, H. W. (2011). Bio-and chemostratigraphic assessment of carbon isotope records across the Triassic–Jurassic boundary at Csővár quarry (Hungary) and Kendlbachgraben (Austria) and implications for global correlations. Bull. Geol. Soc. Den. 59, 100–115. doi: 10.37570/bgsd-2011-59-10
Kozur, H. (1989). The taxonomy of the Gondolellid conodonts in the Permian and Triassic. Cour. Forschungsinst. Senck. 117, 409–469.
Kozur, H. (1993). First evidence of Liassic in the vicinity of Csővár (Hungary), and its paleogeographic and paleotectonic significance. Jahrb. Geol. Bundesanst. 136, 89–98.
Kozur, H., and Mock, R. (1991). New middle Carnian and Rhaetian conodonts from Hungary and the Alps. Stratigraphic importance and tectonic implications for the Buda Mountains and adjacent areas. Jahrb. Geol. Bundesanst. 134, 271–297.
Krystyn, L., Richoz, S., Gallet, Y., Bouquerel, H., Kürschner, W. M., and Spötl, C. (2007). Updated bio-and magnetostratigraphy from Steinbergkogel (Austria), candidate GSSP for the base of the Rhaetian stage. Albertiana 36, 164–173.
Kuroda, J., Hori, R. S., Suzuki, K., Gröcke, D. R., and Ohkouchi, N. (2010). Marine osmium isotope record across the Triassic-Jurassic boundary from a pacific pelagic site. Geology 38, 1095–1098. doi: 10.1130/G31223.1
Marzoli, A., Renne, P. R., Piccirillo, E. M., Ernesto, M., Bellieni, G., and De Min, A. (1999). Extensive 200-millionyear-old continental flood basalts of the Central Atlantic Magmatic Province. Science 284, 616–618. doi: 10.1126/science.284.5414.616
Matsuda, T., and Isozaki, Y. (1991). Well-documented travel history of Mesozoic pelagic chert in Japan: from remote ocean to subduction zone. Tectonics 10, 475–499. doi: 10.1029/90TC02134
McElwain, J. C., Beerling, D. J., and Woodward, F. I. (1999). Fossil plants and global warming at the Triassic-Jurassic boundary. Science 285, 1386–1390. doi: 10.1126/science.285.5432.1386
McElwain, J. C., Wade-Murphy, J., and Hesselbo, S. P. (2005). Changes in carbon dioxide during an oceanic anoxic event linked to intrusion into Gondwana coals. Nature 435, 479–482. doi: 10.1038/nature03618
McElwain, J. C., Wagner, P. J., and Hesselbo, S. P. (2009). Fossil plant relative abundances indicate sudden loss of late Triassic biodiversity in East Greenland. Science 324, 1554–1556. doi: 10.1126/science.1171706
Moix, P., Kozur, H. W., Stampfli, G. M., Mostler, H., Lucas, S. G., and Spielmann, J. A. (2007). New paleontological, biostratigraphical and paleogeographic results from the Triassic of the Mersin Mélange, SE Turkey. The global Triassic. N M Mus Nat Hist Sci Bull 41, 282–311.
Mosher, L. C. (1968). Triassic conodonts from western North America and Europe and their correlation. J. Paleontol. 42, 895–946.
Müller, K. J., and Mosher, L. C. (1970). Post-Triassic conodonts. Geol. Soc. Am. Mem. 127, 467–470. doi: 10.1130/MEM127-p467
Nohda, S., and Setoguchi, T. (1967). An occurrence of Jurassic conodonts from Japan. Mem Coll Sci Kyoto Univ Ser B 33, 227–239.
Okada, Y., Hori, R. S., Ikeda, M., and Ikehara, M. (2015). Geochemical study of Panthalassa deepsea sedimentary rocks across the Triassic–Jurassic boundary. News Osaka Micropaleontol Spec 15, 219–232.
Orchard, M. J., Whalen, P. A., Carter, E. S., and Taylor, H. J. (2007). Latest Triassic conodonts and radiolarian-bearing successions in Baja California Sur. The global Triassic. N M Mus Nat Hist Sci Bull 41, 355–365.
Pálfy, J., Demény, A., Haas, J., Carter, E. S., Görög, Á., Halász, D., et al. (2007). Triassic-Jurassic boundary events inferred from integrated stratigraphy of the Csővár section, Hungary. Palaeogeogr. Palaeoclimatol. Palaeoecol. 244, 11–33. doi: 10.1016/j.palaeo.2006.06.021
Pálfy, J., Demény, A., Haas, J., Hetényi, M., Orchard, M. J., and Vető, I. (2001). Carbon isotope anomaly and other geochemical changes at the Triassic-Jurassic boundary from a marine section in Hungary. Geology 29, 1047–1050. doi: 10.1130/0091-7613(2001)029<1047:CIAAOG>2.0.CO;2
Richoz, S., Van De Schootbrugge, B., Pross, J., Püttmann, W., Quan, T. M., Lindström, S., et al. (2012). Hydrogen sulphide poisoning of shallow seas following the end-Triassic extinction. Nat. Geosci. 5, 662–667. doi: 10.1038/ngeo1539
Rigo, M., and Joachimski, M. M. (2010). Palaeoecology of late Triassic conodonts: constraints from oxygen isotopes in biogenic apatite. Acta Palaeontol. Pol. 55, 471–478. doi: 10.4202/app.2009.0100
Rigo, M., Mazza, M., Karádi, V., and Nicora, A. (2018). “New Upper Triassic conodont biozonation of the Tethyan realm” in The Late Triassic World. ed. L. H. Tanner (Cham: Springer), 189–235.
Rigo, M., Preto, N., Roghi, G., Tateo, F., and Mietto, P. (2007). A rise in the carbonate compensation depth of western Tethys in the Carnian (late Triassic): deep-water evidence for the Carnian pluvial event. Palaeogeogr. Palaeoclimatol. Palaeoecol. 246, 188–205. doi: 10.1016/j.palaeo.2006.09.013
Rigo, M., Trotter, J. A., Preto, N., and Williams, I. S. (2012). Oxygen isotopic evidence for late Triassic monsoonal upwelling in the northwestern Tethys. Geology 40, 515–518. doi: 10.1130/G32792.1
Schaller, M. F., Wright, J. D., Kent, D. V., and Olsen, P. E. (2012). Rapid emplacement of the Central Atlantic Magmatic Province as a net sink for CO2. Earth Planet. Sci. Lett. 323-324, 27–39. doi: 10.1016/j.epsl.2011.12.028
Schlische, R. W., Withjack, M. O., Olsen, P. E., and Hames, W. E. (2003). Relative timing of CAMP, rifting, continental breakup, and basin inversion: tectonic significance. Geophys Monogr Am Geophys Union 136, 33–60. doi: 10.1029/136GM03
Schoene, B., Guex, J., Bartolini, A., Schaltegger, U., and Blackburn, T. J. (2010). Correlating the end-Triassic mass extinction and flood basalt volcanism at the 100ka level. Geology 38, 387–390. doi: 10.1130/G30683.1
Schoepfer, S. D., Algeo, T. J., Ward, P. D., Williford, K. H., and Haggart, J. W. (2016). Testing the limits in a greenhouse ocean: did low nitrogen availability limit marine productivity during the end-Triassic mass extinction? Earth Planet. Sci. Lett. 451, 138–148. doi: 10.1016/j.epsl.2016.06.050
Tekin, U. K., Krystyn, L., Okuyucu, C., Bedi, Y., and Sayit, K. (2020). Late Triassic to early Jurassic radiolarian, conodont and ammonite assemblages from the Tavuscayiri block, Mersin Mélange, southern Turkey: time constraints for the T/J boundary and sedimentary evolution of the southern margin of the northern Neotethys. Geodiversitas 42, 493–537. doi: 10.5252/geodiversitas2020v42a27
Thibodeau, A. M., Ritterbush, K., Yager, J. A., West, A. J., Ibarra, Y., Bottjer, D. J., et al. (2016). Mercury anomalies and the timing of biotic recovery following the end-Triassic mass extinction. Nat. Commun. 7:11147. doi: 10.1038/ncomms11147
Tong, J., Chu, D., Miao, X., Shu, W., Guo, W., Wu, Y., et al. (2021). Lithostratigraphic subdivision and correlation of the Triassic in China. J. Stratigr. 45, 340–363. doi: 10.19839/j.cnki.dcxzz.2021.0028
Trotter, J. A., Williams, I. S., Nicora, A., Mazza, M., and Rigo, M. (2015). Long-term cycles of Triassic climate change: a new δ18O record from conodont apatite. Earth Planet. Sci. Lett. 415, 165–174. doi: 10.1016/j.epsl.2015.01.038
Uno, K., Yamashita, D., Onoue, T., and Uehara, D. (2015). Paleomagnetism of Triassic bedded chert from Japan for determining the age of an impact ejecta layer deposited on peri-equatorial latitudes of the paleo-Pacific Ocean: a preliminary analysis. Phys. Earth Planet. Inter. 249, 59–67. doi: 10.1016/j.pepi.2015.10.004
Keywords: mass extinction, post-Triassic, T-J boundary, Lower Jurassic, conodonts
Citation: Du Y, Onoue T, Tomimatsu Y, Wu Q and Rigo M (2023) Lower Jurassic conodonts from the Inuyama area of Japan: implications for conodont extinction. Front. Ecol. Evol. 11:1135789. doi: 10.3389/fevo.2023.1135789
Edited by:
Hepin Wu, Nanjing Normal University, ChinaReviewed by:
Andrej Spiridonov, Vilnius University, LithuaniaLawrence H. Tanner, Le Moyne College, United States
Copyright © 2023 Du, Onoue, Tomimatsu, Wu and Rigo. This is an open-access article distributed under the terms of the Creative Commons Attribution License (CC BY). The use, distribution or reproduction in other forums is permitted, provided the original author(s) and the copyright owner(s) are credited and that the original publication in this journal is cited, in accordance with accepted academic practice. No use, distribution or reproduction is permitted which does not comply with these terms.
*Correspondence: Manuel Rigo, manuel.rigo@unipd.it