- 1Departamento de Ciencias Naturales y Matemáticas, Pontificia Universidad Javeriana Seccional Cali, Cali, Colombia
- 2Posgrado en Ciencias-Biología, Universidad del Valle, Cali, Colombia
- 3Department of Biology, University of Puerto Rico–Humacao Campus, Humacao, PR, United States
The tropical Andean landscape has been dramatically transformed over the last century with remaining native forest limited to small fragments within a heterogeneous matrix of crops, cattle pastures, and urban environments. We aimed to explore the impact of habitat transformation on the population dynamics in an endemic twig epiphytic orchid located within the undisturbed forest and within modified matrix habitat in two regions with contrasting landscape structures: with a dominant shade coffee matrix and a dominant grassland matrix. Over 2 years, we surveyed 4,650 individuals of the Colombian endemic orchid, Rodriguezia granadensis. We undertook four post-breeding censuses in three sites in each region in both native forest and pasture sub-sites (12 sub-sites; 48 censuses in total), and constructed demographic transition matrices (n = 36). The transition probabilities were calculated using a Bayesian approach and population grow rates were evaluated using asymptotic models and elasticities using transient dynamics. Between regions, higher population growth rate and inertia (defined as the largest or smallest long-term population density with the same initial density distribution) was seen in the shade coffee-dominated landscape. Additionally, population growth rate and damping ratio was higher in forest compared with pasture, with lower convergence time for the forest subsites. These demographic patterns reveal the contrasting levels of population resilience of this orchid in different landscape structures with the more connected shade-coffee dominated landscape permitting some healthier populations with greater population growth and survival in forest than pasture. This study highlights that twig epiphyte colonization of isolated phorophytes in pastures should not be interpreted as a sign of a healthy population but as a temporal transitory period.
Introduction
Habitat fragmentation threatens the survival of populations and species in two main ways. Firstly, smaller, isolated populations in habitats with high fragmentation are more vulnerable to stochastic events (Fischer and Lindenmayer, 2007). These may be the result of environmental catastrophes, particularly in the context of increasingly extreme climatic events, random genetic processes, with the loss of evolutionary potential through genetic drift (Lienert, 2004; Honnay and Jacquemyn, 2007) or demographic processes, with year-to-year variability in reproductive success (Tomimatsu and Ohara, 2010; Jacquemyn et al., 2012). Secondly, with the reduction in area of natural habitat, the abiotic conditions of the surrounding landscape change, with consequent negative impact on plant reproduction (Aguilar et al., 2006; Aguilar et al., 2019) and biotic interactions (Brosi, 2009; Briggs et al., 2013).
The impact of habitat transformation (Ritchie and Roser, 2013; Winkler et al., 2021) on ecological characteristics of species has been well-studied from diverse perspectives, such as life histories characteristics (Kolb and Diekmann, 2005; Bruna et al., 2009), extinction probabilities (Fréville et al., 2007), plant animal interactions (Benítez-Malvido and Arroyo-Rodríguez, 2008; Benitez-Malvido et al., 2016) and reproductive success (Brudvig et al., 2015; Vellend et al., 2017).
Following the theory of island biogeography, fragments of native habitat can be considered as islands within a “sea” of transformed terrain. In a mosaic of patches of different land use, the dominant, usually non-native background in a transformed landscape is known as the matrix (Fischer and Lindenmayer, 2007). Spatial matrix types, such as cattle pasture, different agricultural systems, or urbanization, have differential impacts on the connectivity between native habitat fragments, altering resource availability, as well as the activity of pollinators, seed dispersers, and herbivores (Jules and Shahani, 2003; Debinski, 2006).
For example, fragmentation and loss of habitat quality affect pollinator communities, including the so-called “orchid bees” (Apidae: Euglossini), impacting home ranges (Brosi, 2009) and reproductive success (Newman et al., 2013). Small, isolated plant populations are expected to have lower reproductive success when dependent on non-resident pollinators (Murren, 2002).
The tropical Andes represent a hotspot of biodiversity (Myers et al., 2000; Liang et al., 2022) and endemism (Gentry, 1982; Olson and Dinerstein, 1998) and at the same time is one of the geographical areas with the highest rate of anthropogenic habitat transformation (Etter and van Wyngaarden, 2000; Etter et al., 2006). These anthropogenic changes can influence demographic dynamics (Rodríguez-Echeverry and Leiton, 2021), survivorship or persistence of populations (Philpott et al., 2008). Nonetheless, our understanding of the impact of habitat transformation in this biodiverse region is limited (Hoang and Kanemoto, 2021; Winkler et al., 2021). In the neotropics, the influence of modified landscape mosaics on the diversity of birds, bats (Harvey and González Villalobos, 2007), insects (Vandermeer et al., 2019), and trees (Philpott et al., 2008) have been documented, however there are only a few studies focused on epiphytes (Richards et al., 2020), including epiphytic orchids (García-González and Riverón-Giró, 2013; Raventós et al., 2018), with only one study in the Andes (Parra Sánchez et al., 2016).
Epiphytic plants grow on the trunk, branches, twigs, and even the leaves (Alvarenga and Pôrto, 2007) of a plant host, the phorophyte, enabling growth in higher light conditions. Vascular epiphytes are one of the most dominant guilds of species in the tropics and are potentially highly impacted and endangered by habitat transformation (Hernández-Pérez and Solano, 2015; Osie et al., 2022). The distribution and survival of epiphytes is influenced by the landscape structure, phorophyte diversity, the age of the forest and tree size (Hietz, 1999).
The main plant families with epiphytic species are Bromeliaceae and Orchidaceae (Zotz, 2013). Some epiphytic species can also be rupiculous, growing on rock substrate, while others may also be terrestrial. Those species that are exclusively epiphytic are often limited to a particular ecological niche in a restricted zone of the architecture of the tree (Catling et al., 1986; Medeiros, 2010). The so-called twig epiphytes use as their substrate the smallest branch size, most often located in the outer fringe of the tree canopy (Ventre-Lespiaucq et al., 2017). Obligate twig epiphytes often are characterized by their accelerated life cycle, psigmoid or terete leaves, and thickened seed testa (Chase, 1987; Zotz, 2007).
Orchids specialized as twig epiphytes, while numerous, are phylogenetically restricted to Oncidiinae and Vandeae clade (Chase, 1987; Gravendeel et al., 2004). A limited amount of information of the life history of these species is available [Tolumnia variegata (Sw.) Braem, Calvo and Horvitz, 1990; Ackerman et al., 1996; Erycina crista-galli (Rchb.f.) N.H.Williams and M.W.Chase, Mondragón et al., 2007; Ionopsis utricularioides (Sw.) Lindl., García-González and Riverón-Giró, 2013] and most of the supposed advantages of being a twig epiphyte are circumstantial. It is commonly assumed that the advantage in being in the outer rim of the canopy twig epiphytes is the higher availability of light. However, this advantage may have tradeoffs (Ventre-Lespiaucq et al., 2017) including a greater risk of dehydration (Chase, 1987). A study in T. variegata found that plants located on twigs at the canopy edge had a reproductive disadvantage compared with those located within the tree canopy (Tremblay et al., 2010). Increased light availability may result in higher reproductive potential (flower production) but could also result in lower survival of the smaller individuals (for example because of desiccation), resulting in an overall decrease in the long-term persistence of the population.
Twig epiphytic orchids are often transitory pioneer species and frequently colonize phorophytes in transformed habitats. Given the tolerance of twig epiphytes to higher light intensities (Ventre-Lespiaucq et al., 2017), such populations on trees in transformed, open environment may be perceived to be as healthy as those in undisturbed forest habitat. However, this perspective may be misleading as the number of individuals may be temporary.
Our study aims to evaluate the impact of habitat transformation on the twig epiphytic species, Rodriguezia granadensis (Lindl) Rchb.f. This orchid is commonly distributed across Andean premontane and montane forests. Although endemic to Colombia, its natural tendency to colonize isolated phorophytes in open pastures is a major contributing factor to its classification of least concern (LC) in national red-list evaluations (Calderón-Sáenz, 2007; López-Gallego and Morales, 2021).
We used an approach which includes the complete life history of the species, following individuals in a mark-recapture approach and population projection matrices (PPM), comparing the demographic structure and dynamics in native forest fragments and on isolated phorophytes in pastures across two contrasting landscapes. We aim to understand the potential different demographic responses in each landscape and land use, thereby drawing inferences on the dynamics of orchid twig epiphyte populations in varying anthropogenically modified environments. We hope our findings may inform landscape management practices to promote orchid conservation in this biodiverse region.
As a null model, we would expect no differences in population dynamics between the two landscapes, a matrix dominated by either coffee crop or sugar cane and cattle grassland, nor between native forest and pastureland cover populations. If the main driver for the niche occupancy of twig epiphytes in the outer tree canopy is to maximize exposure to light, it could be expected that populations on isolated trees in an open environment such as pastures would present more favorable demographic parameters, with a higher population growth rate and stability. Specifically, we aim to determine whether population dynamics of twig epiphytes are similar in native forest and pastures in the two regions across 2 years of survey considering the following parameters (1) deterministic population growth rate, (2) transient dynamics and (3) non-linear elasticity (transfer function) of the different life stages, (4) reproductive potential (fruit set), and (5) recruitment.
Materials and methods
Study species
Rodriguezia granadensis (Lindl.) Rchb.f. is widely distributed at mid-elevation (700–1,900 m.a.s.l.) in Andean Forest. This orchid frequently colonizes coffee or fruit tree plantations. The species is common and widely distributed and consequently an excellent model species to study the impact of changing landscapes in the northern Andes on epiphytic population dynamics (Ventre-Lespiaucq et al., 2017).
Rodriguezia granadensis has two flowering seasons a year (March–April and October–November), which coincide with bimodal peaks of rainfall (Calderón-Sáenz, 2007). It is pollinated by euglossine bee species–Eulaema meriana Oliver, E. cingulata Fabricius, and Exaerete frontalis Guérin-Méneville–that forage for nectar in a melitophilly syndrome behavior (Ospina-Calderón et al., 2015).
Study sites
Populations of R. granadensis were studied in three field sites each in two regions of the tropical Andes in Colombia, in the departments of Cauca, and Valle del Cauca (from here on “Valle”). These two regions, separated by approximately 150 km, are located at the same elevation (approximately 1,700 m.a.s.l.) on the eastern slope of western cordillera of the Andes (Figure 1).
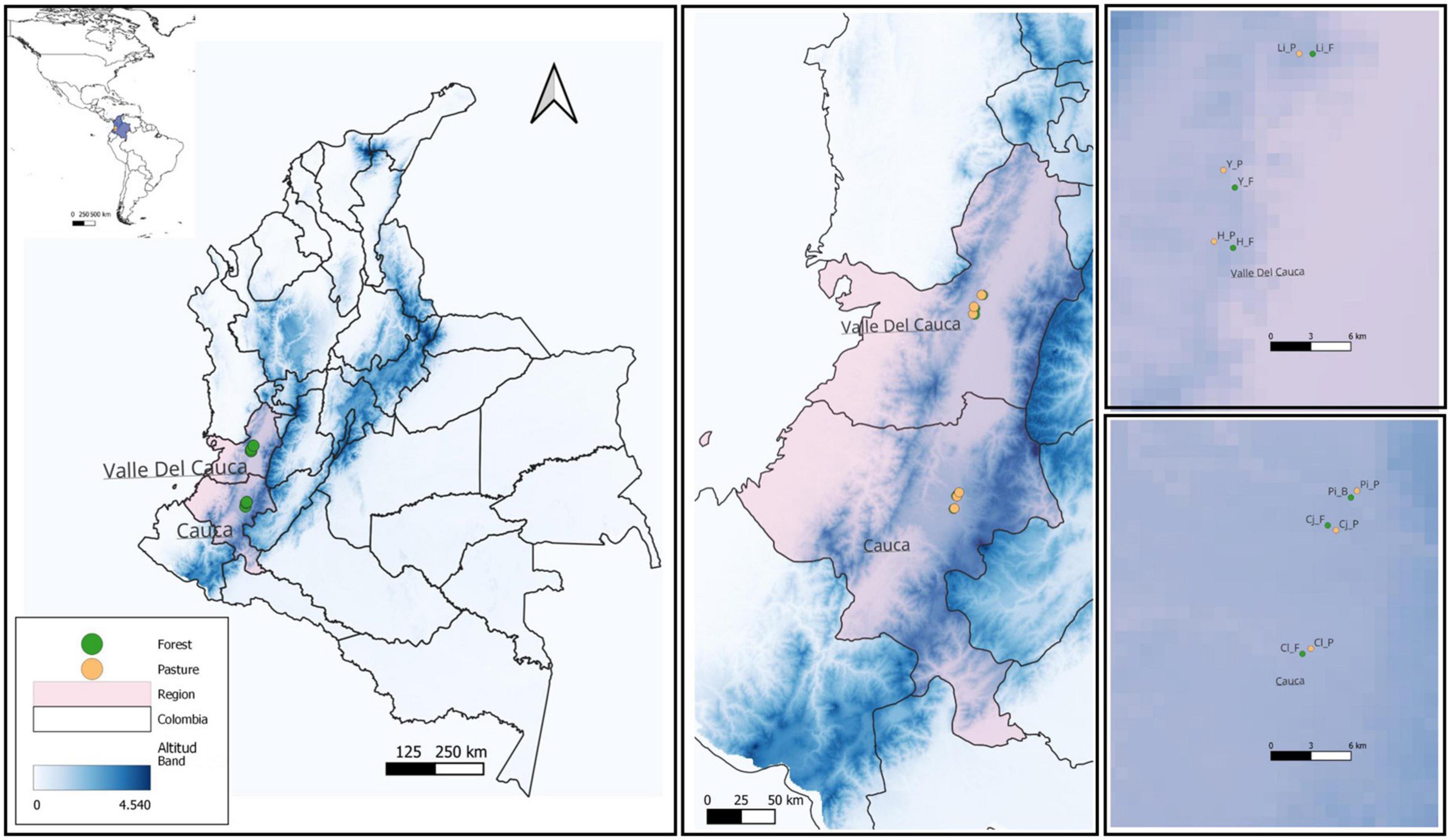
Figure 1. Geographical location study sites and experimental design showing sampling organization over three scales, Region: Cauca and Valle. Site: Cl, Calibío; Cj, Cajibío; Pi, Piendamó; H, Hondonada; Li, Lilas; Y, Yotoco. Sub-site: F, forest; P, pasture.
The Cauca region is in the Colombian massif of the Popayán plateau with sun and shade coffee crops in an agroforestry mosaic, with mixed and forestry crops, small fragments of forest and riparian forests (Criollo and Bastidas, 2011; Arenas-Clavijo and Armbrecht, 2018). Although the coffee landscape is increasing in agricultural intensity, to the detriment of biodiversity (Armbrecht, 2003; Philpott et al., 2008; Harvey et al., 2021), it continues to host more diversity as an agroecosystem (Letourneau et al., 2011) than extensive monocultures such as the sugar cane and cattle ranching model in the Valle del Cauca department to the north (Marull et al., 2018; Sardi et al., 2018). In the latter, we find a few isolated forests in a predominantly pasture matrix, where the landscape and biodiversity has been dramatically affected (Torres et al., 2012; Vélez-Torres et al., 2019).
The southern region in Cauca, has a mean annual precipitation of ± 2,120 mm, and temperature of 15°C (IDEAM, 2010; Puertas-Orozco et al., 2011). The three field sites in this region were: (1) Calibío (Cl) (2° 37.446′ N, 76° 33.525′ W); (2) Cajibío (Cj) (2° 38.888′ N, 76° 32.328′ W); and (3) Piendamó (Pi) (2° 41.126′ N, 76° 33.710′ W). The region to the north, in Valle del Cauca, has a mean annual precipitation ± 1,480 mm and temperature of 18°C (IDEAM, 2010; Puertas-Orozco et al., 2011). Our three field sites were (1) Hondonada (H) (3° 49.896′ N, 76° 26.043′ W), (2) Lilas (L) (3° 50.986′ N, 76° 26.344′ W) and (3) the National Forest Reserve of Yotoco (Y) (3° 52.712′ N, 76° 26.291′ W). The three field sites within each region had pairwise geographic distances between 5 and 15 km (Figure 1).
Survey
At each of the six field sites we surveyed plants of R. granadensis in two sub-sites of contrasting land cover: native forest (continuous canopy) and pasture (grassland with isolated trees), for a total of 12 sampled sub-sites. From here on, we refer to three different analysis levels: Region comparing Cauca with Valle, Site Calibío (Cl), Cajibío (Cj), Piendamó (Pi) in Cauca, and Hondonada (H), Lilas (Li), and Yotoco (Y) in Valle and Sub-site, contrasting landcover, forest or pasture. Within sub-sites all individual orchids present in each phorophyte (host tree) were marked and counted until reaching 300 at the first census. The position of each phorophyte sampled was registered with a GPS Global Positional System (Garmin Oregon 750), and the minimum convex polygon for each sub-site was calculated to report phorophyte distance and density (QGIS 3.26). Individual orchid plants were marked with permanent Dymo tags for monitoring over consecutive censuses.
While density per meter square is a common metric used to describe the dispersion pattern of many plant species, it is not always an adequate description of the dispersion pattern of epiphytic orchids (Tremblay, 1997). Because epiphytic orchids are dependent on the presence of the host tree, measuring density per host tree is a more realistic index, and we took both of these variables into account.
We surveyed plants at four different times, resulting in three transition matrices between consecutive post-breeding censuses for each site. In the first census in March 2017, we tagged the first plants in each sub-site. For the subsequent three censuses (Oct. 2017, March 2018, and Oct. 2018) when additional individuals were detected, these were tagged too and included in the population analyses. Thus, an additional 30 to 50 plants were registered per sub-site per survey for a total of between 348 and 440 plants per sub-site and a grand total of 4,650 unique individual plants in the study (Supplementary Table 1).
For each plant we registered the number of live pseudobulbs, inflorescences, flowers, and fruits as an index of reproductive potential in addition to survival among time periods. The reproductive potential for a specific stage was estimated as the number of fruits/number of flowers in the time period (Sabat and Ackerman, 1996). The expected number of recruits at time t is assumed to be proportional to the fruit set at time t-1, consequently, recruitment does not include the seed stage or dormancy of seeds (Tremblay and Hutchings, 2002).
Data analysis
We reviewed the distribution of all the demographic and reproductive variables per region, site, and subsite. After conducting assumption tests with Shapiro Wilks and without transformation, we ran an analysis of variance ANOVA to test for differences in demography and reproductive variables between region and site. For subsites, we ran a paired t-test.
For the population projection matrices (PPM), we applied the life cycle structure previously determined for this species, based on pseudobulb and inflorescence number (Ospina-Calderón, 2009), and the methodology for PPM developed for orchids, following Tremblay and Hutchings (2002) and Martorell et al. (2022). Our simplified life history of R. granadensis is based on four size classes describing the life stages: (1) Seedling (S), individuals lacking pseudobulbs; (2) Juvenile (J) with 1–2 pseudobulbs; (3) Small adults–stage 1 (A1), possessing 3–6 pseudobulbs and no more than one inflorescence; and 4. Large adults–stage 2 (A2) for plants possessing more than 7 pseudobulbs with one or more inflorescences. Transitions between life stages from one census to the next were recorded as growth (G); fecundity (Fe), stasis within the same life stage (L); and reversal (R) (Figure 2).
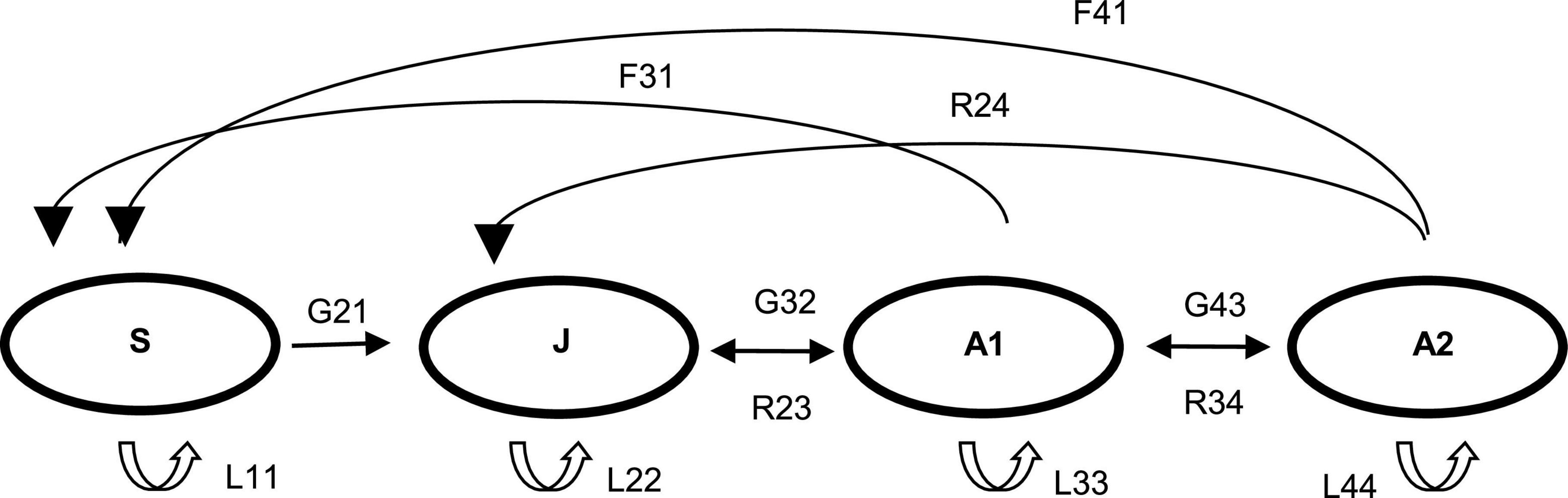
Figure 2. Life cycle diagram of Rodriguezia granadensis. S, seedling; J, juvenile; A1, adult, stage 1; A2, adult, stage 2. Transitions: G, growth; F, fecundity; L, stasis in the same stage; R, reverse.
Estimating transition probabilities
From the data registered during the four censuses (March and October, 2017 and 2018) we calculated parameters for 36 transition matrices (time period x sub-sites). Each matrix corresponds to a time period: Time 1–March to October 2017; Time 2–October 2017 to March 2018; Time 3–March to October 2018. Thus, three matrices for each of the 12 sub-sites were constructed. The transition probabilities were estimated using a Bayesian approach (Tremblay et al., 2021). This analysis is more appropriate for the current dataset for two reasons. Firstly, it resolves issues for estimating the parameters of some transitions with small sample sizes (for example, seedlings were scarce or not detected in some populations). Secondly, the parameter estimates (transitions, survival, death, and stasis) follow the required beta distribution and the credible intervals are bounded between 0 and 1. With this Bayesian approach infrequent transitions can be estimated while avoiding improbable values that may be generated with small sample sizes or few observed transitions for some of the specific stages (Tremblay et al., 2021). For the Bayesian analysis, prior data for the matrix (Table 1) were selected from a previous census undertaken in the population of Yotoco in 2008 (Ospina-Calderón, 2009) with an effective sample size of n = 1. Consequently, this weak prior has little impact on the transition probabilities when sample sizes are large. This choice of an effective sample size yields posterior parameters that are dominated by the data.
Population growth rates
We employed population projection matrix (PPM) analysis to evaluate the asymptotic populations growth rate, lambda. When lambda is equal to one (including the credible intervals, CrI) populations are considered to be stable, while lambda values either smaller or larger than one (with the CrI) indicate a decreasing or increasing population size, respectively. The median population growth rate and the CrI were calculated with 15,000 simulations corresponding to the posterior lambda values and the CrI (Tremblay et al., 2021).
Transient dynamics, transfer function
Transient dynamics analysis and the indices described by Stott et al. (2012a) are mathematical approaches to study the short-term effect of ecological disturbances or perturbation on the population structure of a species in addition to understanding the impact of population structure not at equilibrium (Stott et al., 2011). This innovative approach to understanding short–term dynamics has been applied broadly in plants (McDonald et al., 2016), for example, plant invasions (Iles et al., 2016) and orchids (Raventós et al., 2015; Ortiz-Rodríguez et al., 2020). Transient dynamics PPM models are time-invariant, however by varying the starting demographic distribution, and modeling demographic stochasticity whether of biotic, abiotic, and anthropogenic origin, transient dynamics may result in a stage distribution that differs from the stable stage distribution.
The different starting scenarios lead to either a short-term increase in population size and density (amplifications) or a short-term decrease (attenuation). If no other perturbations or disturbances are present, then the transient dynamics models are expected to stabilize to the stable stage distribution. The time to reach the stable stage is the transient period (Stott et al., 2011). One of the most useful measurements of transient population density and growth are reactivity and inertia. These indices and their bounds describe the majority of variation in transient population density with biological interpretations because they describe shorts term changes (Stott et al., 2011). In general, orchid populations are not at stable stage distribution (Schödelbauerová et al., 2010; Tremblay et al., 2015), however a comprehensive review is still lacking.
Transfer function is an approach for evaluating the non-linear effect of perturbation on population dynamics. The traditional approach has been to evaluate the elasticities of the parameters of the matrix (Caswell, 2000), with the limitation that elasticities are assumed to be linear and consequently are usually more applicable when perturbation is small (Stott et al., 2012a). The advantage of transfer function analysis is that it can elucidate the possible impact across a wider range of perturbation without assuming that the response is linear.
Software
All the analysis was performed in the R 4.2.0 environment. The PPM parameters based on a Bayesian approach were evaluated using the raretrans package (Tremblay et al., 2021). The asymptotic population growth rates, transient dynamics, and transfer function were attained using the popdemo R package (Stott et al., 2012b) using the posterior matrices. Data were visualized, contrasted, and wrangled using the ggplot2 and tidyverse packages (Wickham, 2016; Wickham et al., 2019).
Results
We surveyed a total of 4,650 plants, in 12 sub-sites of both native forest and pasture land cover sub-sites in three sites each in two regions with differing landscape composition: shade-coffee dominated, and pasture-dominated (Supplementary Table 1). A total of 1,636 individuals died across the survey period (Supplementary Table 2).
Spatial distribution and fruit set
The spatial distribution of R. granadensis plants and phorophytes varied among landcover sub-sites in a similar way in both regions. In the native forest populations, plants were found over areas from 1,830 to 6,218 m2, while the number of phorophytes varied from nine to 96. The number of orchids per phorophyte varied between four and 10 in each forest sub-site. In the pasture sub-sites in both regions, the distribution areas were half to five times less (582 to 1,127 m2) with a range of nine to 35 phorophytes and between 10 and 46 individuals per phorophyte (Supplementary Table 1). Thus, population density was 2 ind./m2 (sd = 0.51) in forest sub-sites, and 10 ind./m2 (sd = 5.16) in pasture sub-sites. A more aggregated distribution in the isolated phorophytes in the pasture matrix was observed. The number of plants per phorophyte were significantly lower in forest sub-sites with fewer plants per tree in forest (6.93, ANOVA sd = 2.22, p = 0.01) than in pastures (25.67, sd = 13.95).
The average fruit set was 0.055 fruits/flowers (sd = 0.035) for all sub-sites and seasons (Supplementary Table 3). However, over both regions, fruit set was significantly greater for forest sub-sites compared with pasture sub-sites (Forest mean = 0.067, sd = 0.041; Pasture mean = 0.040, ANOVA sd = 0.023, p = 0.01) (Table 2). The exception was in the Hondonada pasture (Valle), which presented a greater number of fruits than the forest (Supplementary Table 4).
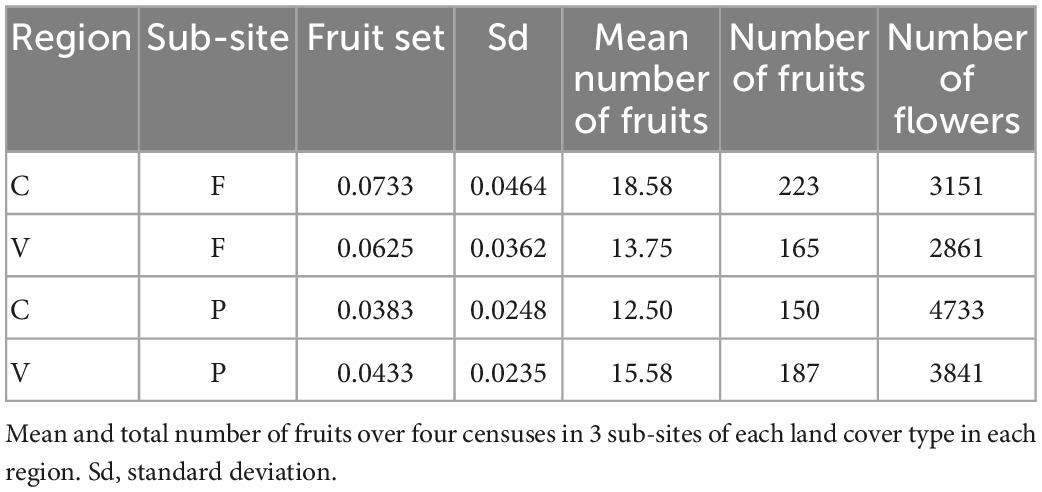
Table 2. Summary of Rodriguezia granadensis fruit set for forest (F) and pasture (P) sub-sites in two regions, Cauca (C) and Valle (V), Colombia.
Population projection matrices (PPM), asymptotic population grow rate
A total of 36 transition matrices representing three time periods for each of the 12 subsites were constructed: three subsites each of forest or pasture within each of the two regions Cauca and Valle. The most common transition detected for all stages was for stasis (L), with plants remaining in the same stage through at least two consecutive censuses (Figure 2). Over both regions, in forest sub-sites the most common transition was for the Adult 1 stasis, L33, and in pasture sub-sites for Adult 2 stasis, L44 (Supplementary Table 2).
Overall, the intrinsic population growth rates (lambda) in all 36 matrices ranged from a minimum of 0.742 to a maximum of 1.268 (Figure 3; Supplementary Table 5). A striking difference was seen between the forest and pasture subsites over both regions. In the forest sub-sites 12 of the 18 PPM yielded a lambda greater than one (increasing population), with two less than one. In contrast, in the pasture subsites, 12 PPM yielded a lambda less than one, with two being greater than one. The distribution of population reduction, stability and growth was not equal among the forest sub-sites (Fisher’s exact test = 12.42, df = 2, p = 0.002), however it was independent of regions, although forest populations may be of slightly better health in both Cauca and Valle.
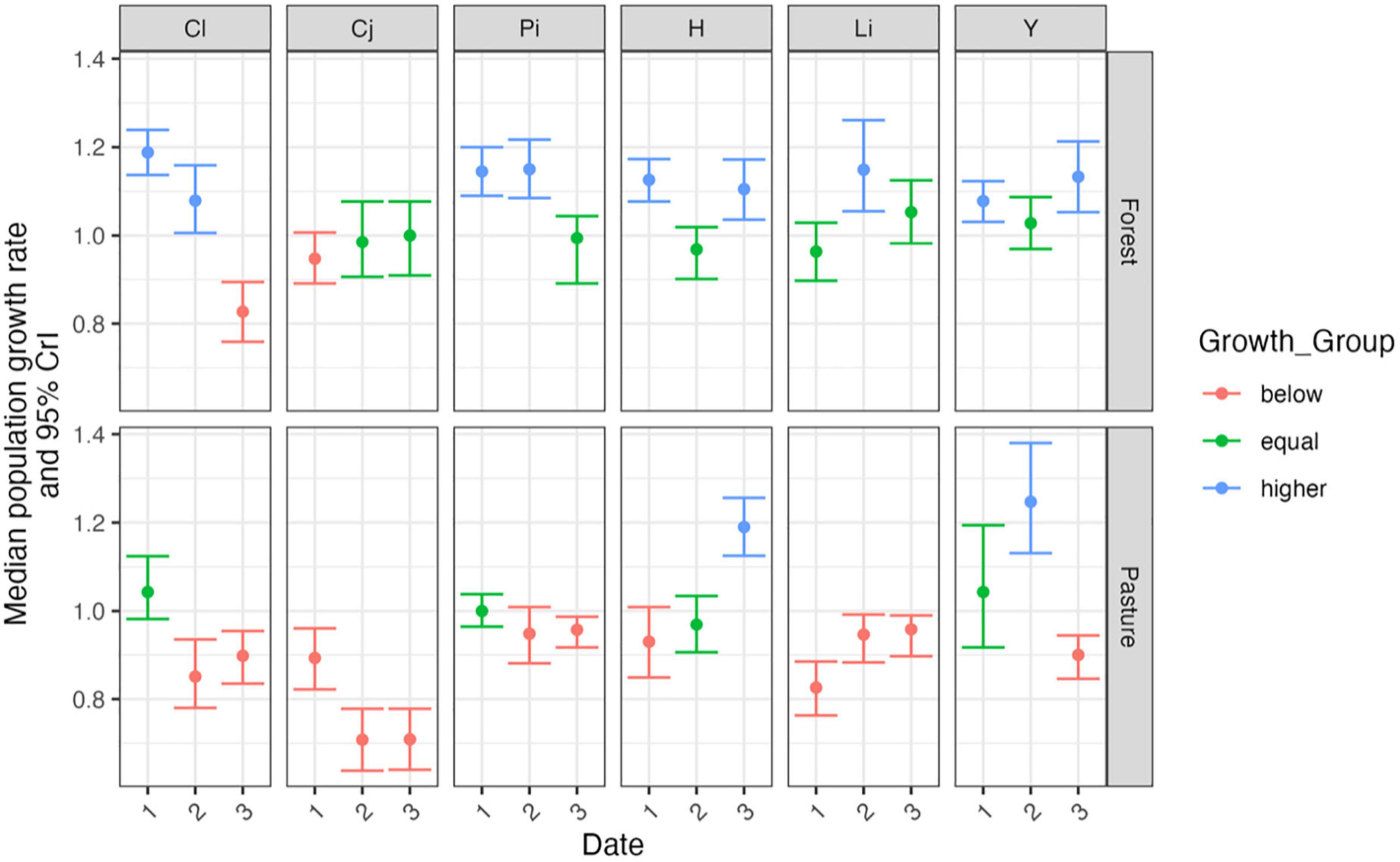
Figure 3. Median posterior asymptotic population growth rate and 95% credible intervals estimated for Rodriguezia granadensis population at two subsites, Forest and Pasture in six Sites (Cauca sites: Cl, Calibío; Cj, Cajibío; Pi, Piendamó. Valle sites: H, Hondonada; Li, Lilas; Y, Yotoco), over three time periods; Time 1, Mar.–Oct. 2017; Time 2, Mar. 2017–Oct. 2018; Time 3, Mar.–Oct. 2018. Red are populations/time periods where lambda was significantly smaller than 1, green for lambda equal to one (not significantly different from stability), and blue for lambda significantly larger than one.
Among sub-sites and time periods, variation was seen in the population growth rates, from reductions of close to 30% to increases of 25%. The sub-site with the largest population reduction was at Cajibío pasture, in Cauca (Time 2, lambda 0.742; 95% CrI 0.667–0.819). The two sub-sites which had the largest increase were in forest, in Valle, Hondonada (Time 1, lambda 1.268, 95%CrI 1.187–1.349) and at Cauca, Calibío (Time 1, lambda 1.199; 95%CrI 1.147–1.253).
Transient dynamics
The transient dynamics indices revealed that the convergence times to stable structure tended to be smaller in forest than in pasture sub-sites for all sites, with the exception of Cajibío in the coffee-dominated landscape in Cauca. The shadow diagram confirms that Cj Forest is more likely to grow than pasture, with darker zones showing decline and tendency to extinction in 5 to 10 years (Figure 4; Supplementary Figure 1). Additionally, higher values for inertia, reactivity and damping ratio indicate greater resilience for forest than pasture sub-sites (Supplementary Table 5).
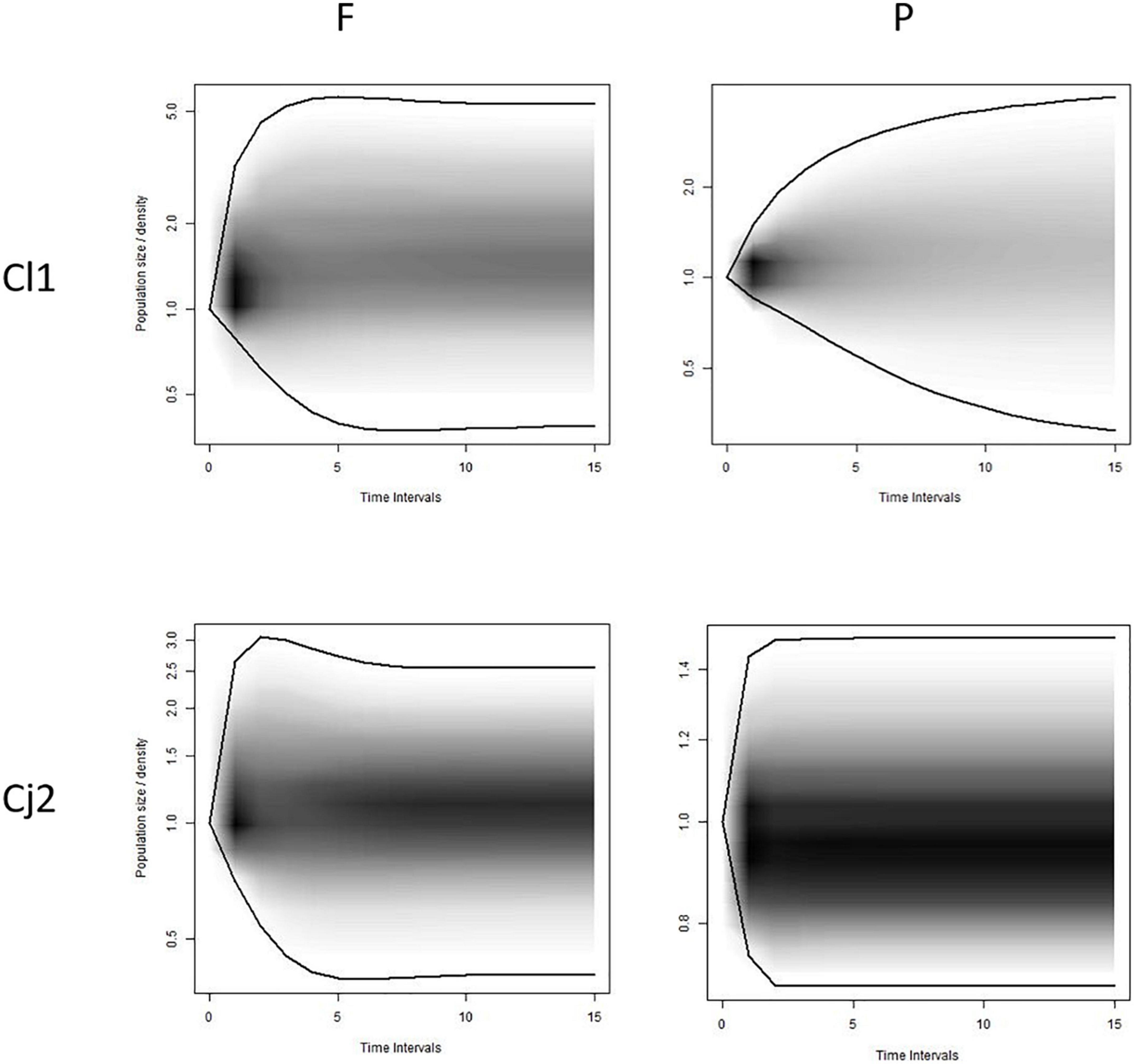
Figure 4. Visualizations of transient dynamics for four selected sub-sites at different time periods showing the diversity of plausible transient responses. Data from; Cl1, Calibío Time period 1; Cj2, Cajibío Time period 2, (F) Forest, (P) Pasture. Decreasing Cj2P, stable, Cl1F and increasing Cj2F. y Cl1P the figure represents the diversity of transient dynamics as a function of the initial starting vector (the number of distribution of individuals at each stage). Darker shading indicates a higher probability of the population size in that range.
The upper value for inertia upper was for the forest subsites in the sites Calibío, Cl (Cauca) and Hondonada, H (Valle) and for inertia low, the lowest was Calibío pasture and Lilas, Li (Valle) pasture. Reactivity confirms this pattern of more resilient plants in the forest sub-sites, with a higher register for Calibío forest and lower for Lilas (Valle) pasture (Supplementary Table 5).
Transient population dynamics simulation for 50 flowering seasons (25 years) revealed a greater tendency for populations to decline and possible extinction in 5 to 10 years in pasture compared with forest populations (Figure 5; Supplementary Figure 2). In four of the six pasture sub-sites over both regions, simulations indicated probable population decline tending to extinction in 5 to 10 years (Supplementary Figure 2). In contrast, for the forest sub-sites, these simulations suggested population growth for four of the six sub-sites, with only Cajibío (Cauca) and Lilas (Valle) indicating a decrease in population size.
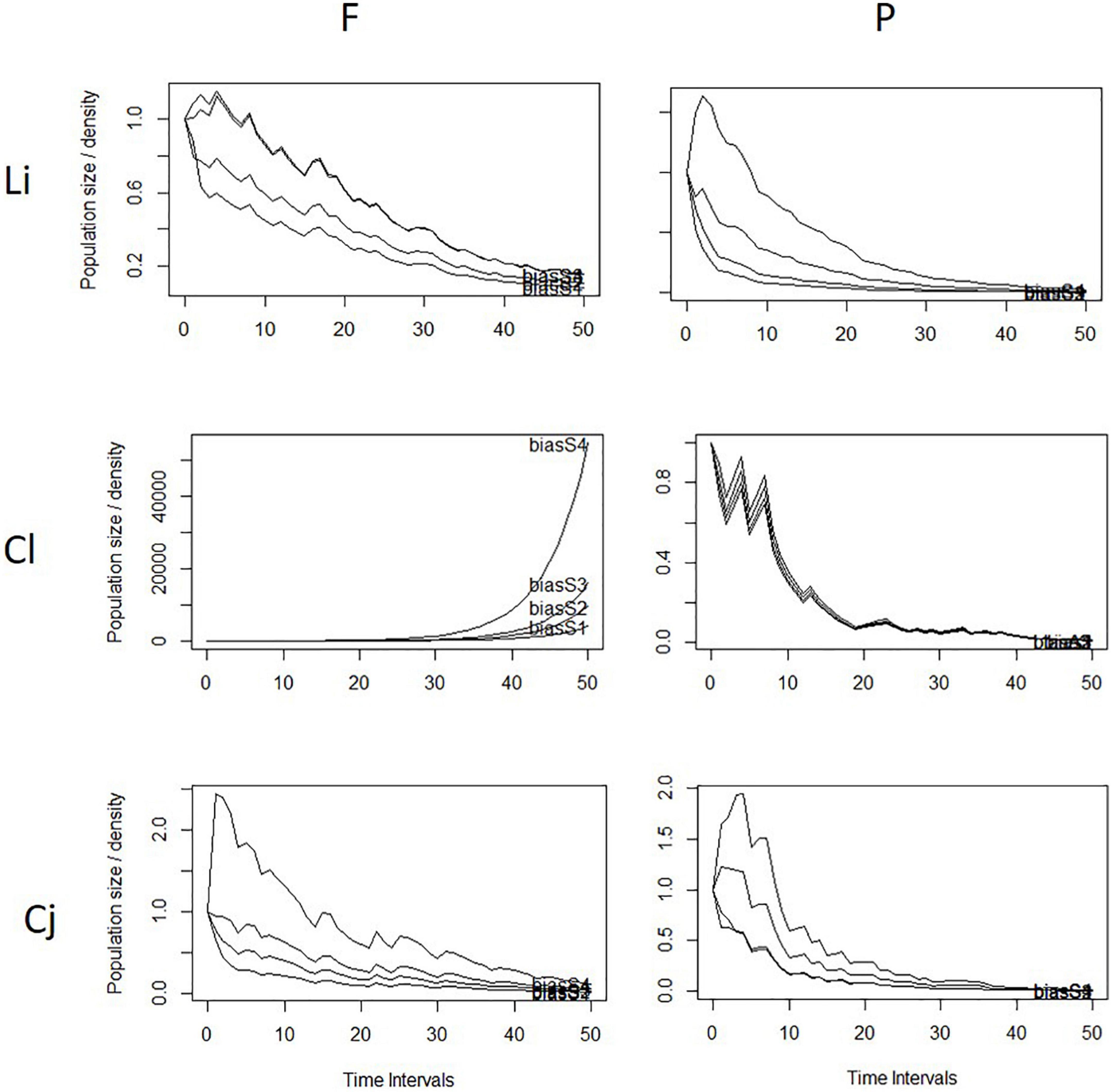
Figure 5. Rodriguezia granadensis transient dynamic simulation for 50 seasons, 25 years for tree of six study sites, Li, Lilas; Cl, Calibío; Cj, Cajibío. Subsites: F, forest; P, pasture. Bias S1, S2, S3, S4, stage-biased demographic vectors.
Perturbation analysis
Perturbation analysis using the non-linear elasticities approach, transfer function, revealed a non-linear relation on relative importance of the influence of perturbation for each stage (Supplementary Figure 3). It is evident that perturbation results in non-linear response of population growth rate as a function of the amount of perturbation in almost all of the parameters. This is most evident in the stasis stage (the diagonal of the matrix) where most have a narrow peak with a rapid decrease and increase around an optimum. Increases in reproductive success (fruits/flowers) show a near linear response in almost all cases. While transitions to the next life stage results in a “U” shape response in some cases, the pattern is inconsistent across sites and time periods showing how the population is likely to respond if there is an increase or decrease in the parameters and how that would affect growth rate.
Discussion
This is the first study to compare the demographic patterns of a twig epiphytic orchid between populations in native and transformed habitat matrices in the tropical Andes. Our findings of less favorable population dynamics for R. granadensis in transformed compared with forest land covers, and between two contrasting landscape structures has important implications for the evaluation of the conservation status of this species and will inform landscape management practices to promote conservation of other similar twig epiphytic orchids in this biodiverse region.
Previous studies of tropical epiphytic communities have shown that species diversity and abundance decrease over gradients of increasing human impact (Larrea and Werner, 2010; Hylander and Nemomissa, 2017). However, in the present study, the populations of R. granadensis colonizing isolated fruit or shade trees within a transformed pasture matrix had a higher density of plants per phorophyte compared with the forest sub-sites (Supplement Table 1). This may be partly explained as isolated trees in open pastures tend to grow larger and wider crowns (Elias et al., 2021).
In Andean human-transformed landscape, Köster et al. (2011) found that tree traits explain 60% of the epiphytic community composition in an Ecuadorian cloud forest, where the isolated trees act as steppingstones that permit some persistence of epiphytes outside of the forest in a changing landscape mosaic (Köster et al., 2009; Elias et al., 2021). In these circumstances some species become denser and more abundant outside of the forest, possibly as a response to scarce available of phorophytes (Larrea and Werner, 2010), as well as changing abiotic conditions.
Nonetheless, studies in other epiphytic species have shown that in transformed habitat, population density initially increases quickly, only to later decrease, often leading to extinction, depending on time and distance to the forest source of seeds (Pellegrino et al., 2015; Hylander and Nemomissa, 2017). Thus, the high-density populations of R. granadensis in open pasture found in this study may be of a transient nature. Continued population monitoring over a longer period is needed to gauge this temporal effect.
The abundance of reproductive adults and fruit set was greater in the forest environment (Supplementary Table 3). Native forests likely comprise a more suitable ecological niche and adequate pollinator community compared to isolated trees within a pasture matrix. With increasing isolation of phorophytes from the native forests, a reduction in the number of Euglossine pollinators visiting these isolated patches has been observed (Briggs et al., 2013).
Our study reveals that the demographic health of orchid twig epiphytes is negatively influenced in transformed environments; hence, in both landscape structures, the forest sub-sites showed higher asymptotic population growth rate with greater resilience (inertia, reactivity) and a lower short-term population decline. Population growth simulation across multiple time periods suggests that the forest populations are less likely to go extinct as compared to pasture sub-sites. The likelihood of extinction of forest sites within a 20-year period is 20% (lambda mean 1.021), while pasture sites have a 45% (lambda mean 0.938) probability of extinction (Figure 2; Supplementary Table 5). According to Criterion C for the IUCN Red List evaluations, a species may be categorized as vulnerable with less than 1,000 mature individuals in each subpopulation and/or a probability of extinction of 10% in 10 years. While it is likely that R. granadensis has more than a total of 10,000 individuals across its range (the central aspect of criterion C), our findings indicate that the likelihood of subpopulation extinction is high even in the forest environment.
Only a small number of multi-period censuses of orchid population dynamics have been undertaken in the tropics, and these have similarly found a negative impact on orchid populations in landscapes with anthropogenic activity. In a study of three epiphytic species growing on coffee trees, Oncidium poikilostalix (Kraenzl.) M.W.Chase and N.H.Williams, Lepanthes acuminata Schltr. and Telipogon helleri (L.O.Williams) N.H.Williams and Dressler in Chiapas México, lambda was greater in populations in unmanaged coffee plantations compared with managed plantations (García-González et al., 2017; Raventós et al., 2018). In the terrestrial tropical invasive species, Oeceoclades maculata (Lindl.) Lindl. a higher population growth rate was noted within a Mexican forest than in a managed coffee plantation (Riverón-Giró et al., 2019). While in Phaius flavus (Blume) Lindl. in southeast China, Li et al. (2022) found that populations tended to decrease, and this change was attributed to the low germination rate in the wild and the loss of adult individuals caused by anthropogenic disturbances. In this current study, individual plants tended to remain in the same stage from one census to the next (Supplementary Table 2). Such stasis as the predominant life history process has also been registered in other neotropical epiphytic orchids (Tremblay and Hutchings, 2002; Crain et al., 2019). In general, in iteroparous forest plants with long lifespans, multi-year reproductive adult stages, and generation overlap, populations often consist of a preponderance of adults of varying sizes that remain in the same stage and contribute to population recruitment through the reproductive (Fe) stage (Silvertown et al., 1996). In contrast, iteroparous plants in open habitat plants typically exhibit populations with predominantly growth (G) and reproductive (Fe) transitions (Silvertown et al., 1993; Franco and Silvertown, 2004). Our data show that R. granadensis populations in an open habitat retain the forest strategy, with persistence of adults, lower generational turnover, fewer seedlings and juveniles that survive to adulthood, slower growth rates, all leading to declining populations.
Population convergence time to a stable state distribution was lower for forest sites than in pastures, which suggest that forest habitat may be beneficial for population stability and promoting higher population resiliency (Supplementary Table 5). Furthermore, values for inertia and reactivity, amplification and attenuation had wider intervals for forest populations, and so greater resilience in the face of changing environmental circumstances, including habitat transformations or climate change. Rapid fluctuations in the population size through time (Figure 4) could be advantageous if a population can increase rapidly after a size reduction due to stochastic phenomena. However, it may also suggest vulnerability if the fluctuation results in a rapid decrease in population size, as noted in Lepanthes caritensis Tremblay and Ackerman, Dendrophylax lindenii (Lindl.) Benth. ex Rolfe, Broughtonia cubensis Cogn (Raventós et al., 2015,b; Tremblay et al., 2015; Crain et al., 2019).
Most of the transient dynamics simulations for R. granadensis reflect the tendency for rapid reduction and high probability of extinction in about five to 10 years. Although some subsites showed population growth (Cl, Pi, H, Y forest and Pi, H pastures), the remaining subsites were near “equilibrium” without increased tendency to growth for more than 10 years. Even though populations are near equilibrium this does not necessarily guarantee that these populations will persist. A number of studies have shown that even when population sizes fluctuate, they are vulnerable to extinction when stochastic events are common (Raventós et al., 2015b; Crain et al., 2019). Twig epiphytes may be highly vulnerable to stochastic events, as loss of small branches as a consequence of the architectural growth of trees and competition with surrounding trees may result in reduced niche availability for these obligate small branch epiphytes.
Inherent fluctuations of epiphytic and twig epiphytic habit represent important constrictions for population growth, structure and distribution of R. granadensis. Transient dynamics are highly influenced by the initial vector and therefore linked to explosion or extinction and stochastic phenomena (García-González et al., 2017; Raventós et al., 2018). The pattern and intensity of fluctuation in population size may be exacerbated by natural phenomena. For example, in Central American and Caribbean orchid populations, the growth rate and high intensity fluctuations are mediated by stochastic disturbance due to large storms or hurricanes (Crain et al., 2019; Ortiz-Rodríguez et al., 2020; Raventós et al., 2021).
Perturbation analysis allows us to identify the effects of probable changes in each transition on the growth rate (Stott et al., 2011). In R. granadensis the stasis stages may be the most elastic as small changes the parameters could result in large, non-linear changes in population growth rates, most often showing a pattern close to a narrow inverted “U.”
Our analyses show that R. granadensis populations have lower survival probability when colonizing phorophytes dispersed in a pasture matrix as compared to forest sites. The diminished persistence of this orchid in a modified landscape can likely be considered an extinction debt. Colonization of isolated trees may prevent extinction in the short term, but the persistence of these sites may depend on the dynamics of the sink-source and the distance from a more suitable forest fragment (Pellegrino et al., 2015; Hylander and Nemomissa, 2017). While orchid populations within the forest and pasture landscape may function as a sink-source dynamic, the importance of the source vs. sink processes are presently little understood. Apposite questions include: how important is this process for the persistence of pasture populations, and is the direction of the seed source always from forest to pastures sites? Moreover, stochastic processes linked with the natural population dynamics of the species are drivers of epiphyte presence and persistence, and subsequently their interaction in land transformation and habitat fragmentation need to be considered (Armbrecht, 2003; Rivera-Pedroza et al., 2019; Zewdie et al., 2022).
Conclusion
The endemic twig epiphyte, R. granadensis, is present in anthropogenically-transformed land covers, but analysis of asymptotic and transitory dynamics indicates that these populations have lower viability than those in native forest fragments. Populations on isolated trees have lower generational turnover, fewer seedlings and juveniles that survive to adulthood, slower growth rates, and, in general, declining populations. Our data suggests that the demographic dynamics of epiphytic orchids are of a fluctuating nature, which makes them more vulnerable to disturbances and stochastic events. Since R. granadensis is a species categorized as of least concern (LC) according to IUCN Red List criteria, a more hopeful pattern in its population dynamics was expected, especially since it is found more or less frequently in disturbed landscapes. This contradiction in the health of a species when comparing observed long-term population growth rates and IUCN criteria may in part be that IUCN criteria used are those which do not explicitly include the ecology and long-term dynamics of the species but a snapshot of the population based on multiple assumptions which may not be predictors of the future health for some species.
Data availability statement
The original contributions presented in this study are included in this article/Supplementary material, further inquiries can be directed to the corresponding author.
Author contributions
NO-C collected the data and ran the analysis. All authors contributed equally on writing and approved the submitted version.
Funding
This study was supported by Internal call project Pontificia Universidad Javeriana Seccional Cali (PUJ) project no. 020100486 and Doctorate in Sciences-Biology Universidad del Valle, National Doctorates Scholarship, COLCIENCIAS call 647.
Acknowledgments
This work collects partial data from the Doctorate in Biology Sciences thesis of the Universidad del Valle-Cali, with honors (Res. 154 Nov. 2020), with the support of UNAL Palmira and RNFBY, Payanesa Orchids Association, Popayán, Julia Calderón and Luis Fernando Ospina, Cristian Delgado, Ana Isabel Parra, Constanza Álvarez, María Mercedes Valencia Falla, Adriana Villalba, Fabián and Luis Paz, Valentín Hidalgo, Beatriz Vásquez, Álvaro José Botero, and García Ayala family.
Conflict of interest
The authors declare that the research was conducted in the absence of any commercial or financial relationships that could be construed as a potential conflict of interest.
Publisher’s note
All claims expressed in this article are solely those of the authors and do not necessarily represent those of their affiliated organizations, or those of the publisher, the editors and the reviewers. Any product that may be evaluated in this article, or claim that may be made by its manufacturer, is not guaranteed or endorsed by the publisher.
Supplementary material
The Supplementary Material for this article can be found online at: https://www.frontiersin.org/articles/10.3389/fevo.2023.1135316/full#supplementary-material
References
Ackerman, J. D., Sabat, A., and Zimmerman, J. K. (1996). Seedling establishment in an epiphytic orchid: an experimental study of seed limitation. Oecologia 106, 192–198. doi: 10.1007/BF00328598
Aguilar, R., Ashworth, L., Galetto, L., and Aizen, M. A. (2006). Plant reproductive susceptibility to habitat fragmentation: review and synthesis through a meta-analysis. Ecol. Lett. 9, 968–980. doi: 10.1111/j.1461-0248.2006.00927.x
Aguilar, R., Cristóbal-Pérez, E. J., Balvino-Olvera, F. J., de Jesús Aguilar-Aguilar, M., Aguirre-Acosta, N., Ashworth, J. A., et al. (2019). Habitat fragmentation reduces plant progeny quality: a global synthesis. Ecol. Lett. 22, 1163–1173. doi: 10.1111/ele.13272
Alvarenga, L. D. P., and Pôrto, K. C. (2007). Patch size and isolation effects on epiphytic and epiphyllous bryophytes in the fragmented Brazilian Atlantic forest. Biol. Conservat. 134, 415–427. doi: 10.1016/j.biocon.2006.08.031
Arenas-Clavijo, A., and Armbrecht, I. (2018). Gremios y diversidad de hormigas (Hymenoptera: Formicidae) en tres usos del suelo de un paisaje cafetero del Cauca-Colombia. Rev. Biol. Trop. 66, 48–57. doi: 10.15517/rbt.v66i1.30269
Armbrecht, I. (2003). Habitat changes in Colombian coffee farms under increasing management intensification. Endang. Spec. Update 20, 163–180.
Benítez-Malvido, J., and Arroyo-Rodríguez, V. (2008). Habitat fragmentation, edge effects and biological corridors in tropical ecosystems. Oxford: Eolss Publishers.
Benitez-Malvido, J., Dattilo, W., Martinez-Falcon, A. P., Duran-Barron, C., Valenzuela, J., Lopez, S., et al. (2016). The multiple impacts of tropical forest fragmentation on arthropod biodiversity and on their patterns of interactions with host plants. PLoS One 11:e0146461. doi: 10.1371/journal.pone.0146461
Briggs, H. M., Perfecto, I., and Brosi, B. J. (2013). The role of the agricultural matrix: coffee management and euglossine bee (Hymenoptera: Apidae: Euglossini) communities in southern Mexico. Environ. Entomol. 42, 1210–1217. doi: 10.1603/EN13087
Brosi, B. J. (2009). The effects of forest fragmentation on euglossine bee communities (Hymenoptera: Apidae: Euglossini). Biol. Conservat. 142, 414–423. doi: 10.1016/j.biocon.2008.11.003
Brudvig, L. A., Damschen, E. I., Haddad, N. M., Levey, D. J., and Tewksbury, J. J. (2015). The influence of habitat fragmentation on multiple plant–animal interactions and plant reproduction. Ecology 96, 2669–2678. doi: 10.1890/14-2275.1
Bruna, E. M., Fiske, I. J., and Trager, M. D. (2009). Habitat fragmentation and plant populations: Is what we know demographically irrelevant? J. Veg. Sci. 20, 569–576.
Calderón-Sáenz, E. (2007). Libro rojo de plantas de Colombia. Vol. 6. Orquídeas, primera parte. Serie Libros Rojos de Especies Amenazadas de Colombia. Bogotá: Instituto Alexander von Humboldt-Ministerio de Ambiente, Vivienda y Desarrollo Territorial.
Calvo, R. N., and Horvitz, C. C. (1990). Pollinator limitation, cost of reproduction, and fitness in plants: a transition-matrix demographic approach. Am. Natur. 136, 499–516.
Catling, P. M., Brownell, V. R., and Lefkovitch, L. P. (1986). Epiphytic orchids in a Belizean grapefruit orchard: distribution, colonization and association. Lindleyana 1, 194–202.
Chase, M. W. (1987). Obligate twig epiphytism in the Oncidiinae and other neotropical orchids. Selbyana 10, 24–30.
Crain, B. J., Tremblay, R. L., and Ferguson, J. M. (2019). Sheltered from the storm? Population viability analysis of a rare endemic under periodic catastrophe regimes. Populat. Ecol. 61, 74–92. doi: 10.1002/1438-390X.1002
Criollo, Y., and Bastidas, S. (2011). Análisis del Paisaje y de su Relación con Características Florísticas de los Bosques Naturales de la Compañía Smurfit Kappa Cartón de Colombia, en el Núcleo Meseta, Municipios de Popayan y Cajibio, Departamento del Cauca. Doctoral thesis. Popayán: Universidad del Cauca.
Debinski, D. M. (2006). Forest fragmentation and matrix effects: the matrix does matter. J. Biogeogr. 33, 1791–1792. doi: 10.1111/j.1365-2699.2006.01596.x
Elias, J. P., Mortara, S. R., Nunes-Freitas, A. F., van den Berg, E., and Ramos, F. N. (2021). Host tree traits in pasture areas affect forest and pasture specialist epiphyte species differently. Am. J. Bot. 108, 598–606. doi: 10.1002/ajb2.1634
Etter, A., McAlpine, C., Wilson, K., Phinn, S., and Possingham, H. (2006). Regional patterns of agricultural land use and deforestation in Colombia. Agric. Ecosyst. Environ. 114, 369–386. doi: 10.1016/j.agee.2005.11.013
Etter, A., and van Wyngaarden, W. (2000). Patterns of landscape transformation in Colombia, with emphasis in the Andean region. Ambio 29, 432–439.
Fischer, J., and Lindenmayer, D. B. (2007). Landscape modification and habitat fragmentation: a synthesis. Glob Ecol. Biogeogr. 16, 265–280. doi: 10.1111/j.1466-8238.2007.00287.x
Franco, M., and Silvertown, J. (2004). A comparative demography of plants based upon elasticities of vital rates. Ecology 85, 531–538.
Fréville, H., McConway, K., Dodd, M., and Silvertown, J. (2007). Prediction of extinction in plants: Interaction of extrinsic threats and life history traits. Ecology 88, 2662–2672
García-González, A., Damon, A., Raventós, J., Riverón-Giró, F. B., Mújica, E., and Solís-Montero, L. (2017). Impact of different shade coffee management scenarios, on a population of Oncidium poikilostalix (Orchidaceae), in Soconusco, Chiapas, Mexico. Plant Ecol. Div. 10, 185–196. doi: 10.1080/17550874.2017.1315840
García-González, A., and Riverón-Giró, F. B. (2013). Organización espacial y estructura de una población de Ionopsis utricularioides (Orchidaceae) en un área suburbana de Pinar del Río, Cuba. Lankesteriana Int. J. Orchidol. 13, 419–427.
Gentry, A. H. (1982). Neotropical floristic diversity: phytogeographical connections between Central and South America, Pleistocene climatic fluctuations, or an accident of the Andean orogeny? Ann. Missouri Bot. Gard. 69, 557–593.
Gravendeel, B., Smithson, A., Slik, F. J., and Schuiteman, A. (2004). Epiphytism and pollinator specialization: drivers for orchid diversity? Philos. Trans. R. Soc. Lond B Biol. Sci. 359, 1523–1535. doi: 10.1098/rstb.2004.1529
Harvey, C. A., and González Villalobos, J. A. (2007). Agroforestry systems conserve species-rich but modified assemblages of tropical birds and bats. Biodiv. Conserv. 16, 2257–2292.
Harvey, C. A., Pritts, A. A., Zwetsloot, M. J., Jansen, K., Pulleman, M. M., Armbrecht, I., et al. (2021). Transformation of coffee-growing landscapes across Latin America. A review. Agron. Sustain. Dev. 41, 1–19. doi: 10.1007/s13593-021-00712-0
Hernández-Pérez, E., and Solano, E. (2015). Effects of habitat fragmentation on the diversity of epiphytic orchids from a montane forest of southern Mexico. J. Trop. Ecol. 31, 103–115. doi: 10.1017/S0266467414000662
Hietz, P. (1999). Diversity and conservation of epiphytes in a changing environment. Pure Appl. Chem. 70, 1–11.
Hoang, N. T., and Kanemoto, K. (2021). Mapping the deforestation footprint of nations reveals growing threat to tropical forests. Nat. Ecol. Evol. 5, 845–853. doi: 10.1038/s41559-021-01417-z
Honnay, O., and Jacquemyn, H. (2007). Susceptibility of common and rare plant species to the genetic consequences of habitat fragmentation. Conserv. Biol. 21, 823–831. doi: 10.1111/j.1523-1739.2006.00646.x
Hylander, K., and Nemomissa, S. (2017). Waiving the extinction debt: Can shade from coffee prevent extinctions of epiphytic plants from isolated trees? Div. Distribut. 23, 888–897. doi: 10.1111/ddi.12579
IDEAM (2010). Promedios Climatológicos 1981-2010. Available online at: http://www.ideam.gov.co/web/tiempo-y-clima/clima (accessed October 27, 2022).
Iles, D. T., Salguero-Gómez, R., Adler, P. B., and Koons, D. N. (2016). Linking transient dynamics and life history to biological invasion success. J. Ecol. 104, 399–408.
Jacquemyn, H., De Meester, L., Jongejans, E., and Honnay, O. (2012). Evolutionary changes in plant reproductive traits following habitat fragmentation and their consequences for population fitness. J. Ecol. 100, 76–87. doi: 10.1111/j.1365-2745.2011.01919.x
Jules, E. S., and Shahani, P. (2003). A broader ecological context to habitat fragmentation: why matrix habitat is more important than we thought. J. Veg. Sci. 14, 459–464. doi: 10.1111/j.1654-1103.2003.tb02172.x
Kolb, A., and Diekmann, M. (2005). Effects of life-history traits on responses of plant species to forest fragmentation. Conserv. Biol. 19, 929–938.
Köster, N., Friedrich, K., Nieder, J., and Barthlott, W. (2009). Conservation of epiphyte diversity in an Andean landscape transformed by human land use. Conserv. Biol. 23, 911–919. doi: 10.1111/j.1523-1739.2008.01164.x
Köster, N., Nieder, J., and Barthlott, W. (2011). Effect of host tree traits on epiphyte diversity in natural and anthropogenic habitats in Ecuador. Biotropica 43, 685–694. doi: 10.1111/j.1744-7429.2011.00759.x
Larrea, M. L., and Werner, F. A. (2010). Response of vascular epiphyte diversity to different land-use intensities in a neotropical montane wet forest. For. Ecol. Manage. 260, 1950–1955. doi: 10.1016/j.foreco.2010.08.029
Letourneau, D. K., Armbrecht, I., Salguero Rivera, B., Montoya Lerma, J., Jiménez Carmona, B., Daza, M. C., et al. (2011). Does plant diversity benefit agroecosystems? A synthetic review. Ecol. Appl. 21, 9–21.
Li, Z., Wang, Y., and Mu, L. (2022). How does deforestation affect the growth of cypripedium (Orchidaceae) species? A simulation experiment in Northeast China. Forests 13:166. doi: 10.3390/f13020166
Liang, J., Gamarra, J. G., Picard, N., Zhou, M., Pijanowski, B., Jacobs, D. F., et al. (2022). Co-limitation towards lower latitudes shapes global forest diversity gradients. Nat. Ecol. Evol. 6, 1423–1437. doi: 10.1038/s41559-022-01831-x
Lienert, J. (2004). Habitat fragmentation effects on fitness of plant populations–a review. J. Nat. Conserv. 12, 53–72. doi: 10.1016/j.jnc.2003.07.002
López-Gallego, C., and Morales, M. P. A. (2021). Rodriguezia Granadensis. The IUCN Red List of Threatened Species 2021: e.T118267887A203443808. Available online at: https://dx.doi.org/10.2305/IUCN.UK.2021-3.RLTS.T118267887A203443808.es (accessed October 26, 2022).
Martorell, C., Flores-Martínez, A., and Franco, M. (2022). Conceptual and methodological issues in structured population models of plants. Bot. Sci. 100, 110–136.
Marull, J., Delgadillo, O., Cattaneo, C., La Rota, M. J., and Krausmann, F. (2018). Socioecological transition in the Cauca river valley, Colombia (1943–2010): towards an energy–landscape integrated analysis. Reg. Environ. Change 18, 1073–1087. doi: 10.1007/s10113-017-1128-2
McDonald, J. L., Stott, I., Townley, S., and Hodgson, D. J. (2016). Transients drive the demographic dynamics of plant populations in variable environments. J. Ecol. 104, 306–314. doi: 10.1111/1365-2745.12528
Medeiros, T. D. S. (2010). Distribuição Vertical de Orquídeas Epífitas e Relação com os Forófitos na apa Ilha do Combú, Belém, Pará, Brasil. Brasil: Universidade Federal Rural da Amazônia.
Mondragón, D., Maldonado, C., and Aguilar-Santelises, R. (2007). Life history and demography of a twig epiphyte: a case study of Erycina crista-galli (Orchidaceae). Selbyana 28, 137–144.
Murren, C. J. (2002). Effects of habitat fragmentation on pollination: pollinators, pollinia viability and reproductive success. J. Ecol. 90, 100–107.
Myers, N., Mittermeier, R. A., Mittermeier, C. G., Da Fonseca, G. A., and Kent, J. (2000). Biodiversity hotspots for conservation priorities. Nature 403, 853–858.
Newman, B. J., Ladd, P., Brundrett, M., and Dixon, K. W. (2013). Effects of habitat fragmentation on plant reproductive success and population viability at the landscape and habitat scale. Biol. Conserv. 159, 16–23. doi: 10.1016/j.biocon.2012.10.009
Olson, D. M., and Dinerstein, E. (1998). The Global 200: a representation approach to conserving the Earth’s most biologically valuable ecoregions. Conserv. Biol. 12, 502–515. doi: 10.1046/j.1523-1739.1998.012003502.x
Ortiz-Rodríguez, I. A., Raventós, J., Mújica, E., González-Hernández, E., Vega-Peña, E., Ortega-Larrocea, P., et al. (2020). Spatiotemporal effects of Hurricane Ivan on an endemic epiphytic orchid: 10 years of follow-up. Plant Ecol. Div. 13, 29–45. doi: 10.1080/17550874.2019.1673495
Osie, M., Shibru, S., Dalle, G., and Nemomissa, S. (2022). Habitat fragmentation effects on vascular epiphytes diversity in Kafa biosphere reserve and nearby coffee Agroecosystem, Southwestern Ethiopia. Trop. Ecol. 63, 1–11. doi: 10.1007/s42965-022-00223-3
Ospina-Calderón, N. H. (2009). Selección de Rasgos Florales en Rodriguezia Granadensis (Lindl.) Rchb.f. (Orchidaceae): Estudio de la Eficacia Biológica en Una Especie Polimórfica. Bogotá: Universidad Nacional de Colombia.
Ospina-Calderón, N. H., Duque-Buitrago, C. A., Tremblay, R. L., and Tupac Otero, J. (2015). Pollination ecology of Rodriguezia granadensis (Orchidaceae). Lankesteriana 15, 129–139. doi: 10.15517/lank.v15i2.20745
Parra Sánchez, E., Armenteras, D., and Retana, J. (2016). Edge influence on diversity of orchids in Andean cloud forests. Forests 7:63.
Pellegrino, G., Bellusci, F., and Palermo, A. M. (2015). Effects of population structure on pollen flow, clonality rates and reproductive success in fragmented Serapias lingua populations. BMC Plant Biol. 15:222. doi: 10.1186/s12870-015-0600-8
Philpott, S. M., Arendt, W. J., Armbrecht, I., Bichier, P., Diestch, T. V., Gordon, C., et al. (2008). Biodiversity loss in Latin American coffee landscapes: review of the evidence on ants, birds, and trees. Conserv. Biol. 22, 1093–1105. doi: 10.1111/j.1523-1739.2008.01029.x
Puertas-Orozco, O. L., Carvajal Escobar, Y., and Quintero Angel, M. (2011). Estudio de tendencias de la precipitación mensual en la cuenca alta-media del río Cauca, Colombia. Dyna 78, 112–120.
Raventós, J., García-González, A., Riverón-Giró, F. B., and Damon, A. (2018). Comparison of transient and asymptotic perturbation analyses of three epiphytic orchid species growing in coffee plantations in Mexico: effect on conservation decisions. Plant Ecol. Div. 11, 133–145. doi: 10.1080/17550874.2018.1444110
Raventós, J., González, E., Mújica, E., and Bonet, A. (2015). Transient population dynamics of two epiphytic orchid species after Hurricane Ivan: implications for management. Biotropica 47, 441–448. doi: 10.1111/btp.12231
Raventós, J., González, E., Mújica, E., and Doak, D. F. (2015b). Population viability analysis of the epiphytic ghost orchid (Dendrophylax lindenii) in Cuba. Biotropica 47, 179–189. doi: 10.1111/btp.12202
Raventós, J., Mújica, E., Gonzalez, E., Bonet, A., and Ortega-Larrocea, M. P. (2021). The effects of hurricanes on the stochastic population growth of the endemic epiphytic orchid Broughtonia cubensis living in Cuba. Populat. Ecol. 63, 302–312. doi: 10.1002/1438-390X.12098
Richards, J. H., Luna, I. M. T., and Waller, D. M. (2020). Tree longevity drives conservation value of shade coffee farms for vascular epiphytes. Agric. Ecosyst. Environ. 301:107025. doi: 10.1016/j.agee.2020.107025
Ritchie, H., and Roser, M. (2013). “Land Use”. Published Online at OurWorldInData.org. Available online at: ‘https://ourworldindata.org/land-use’ [Online Resource] (accessed September 9, 2013).
Rivera-Pedroza, L. F., Escobar, F., Philpott, S. M., and Armbrecht, I. (2019). The role of natural vegetation strips in sugarcane monocultures: ant and bird functional diversity responses. Agric. Ecosyst. Environ. 284:106603. doi: 10.1016/j.agee.2019.106603
Riverón-Giró, F. B., Raventós, J., Damon, A., García-González, A., and Mújica, E. (2019). Spatio-temporal dynamics of the invasive orchid Oeceoclades maculata (Orchidaceae), in four different habitats in southeast Chiapas, Mexico. Biol. Invasions 21, 1905–1919. doi: 10.1007/s10530-019-01945-7
Rodríguez-Echeverry, J., and Leiton, M. (2021). State of the landscape and dynamics of loss and fragmentation of forest critically endangered in the tropical andes hotspot: implications for conservation planning. J. Landsc. Ecol. 14, 73–91. doi: 10.2478/jlecol-2021-0005
Sabat, A. M., and Ackerman, J. D. (1996). Fruit set in a deceptive orchid: the effect of flowering phenology, display size, and local floral abundance. Am. J. Bot. 83, 1181–1186.
Sardi, A., Torres, A. M., and Corredor, G. (2018). Diversidad florística en un paisaje rural del piedemonte de los Farallones de Cali, Colombia. Colomb. For. 21, 142–160. doi: 10.14483/2256201x.10866
Schödelbauerová, I., Tremblay, R. L., and Kindlmann, P. (2010). Prediction vs. reality: Can a PVA model predict population persistence 13 years later? Biodiv. Conserv. 19, 637–650. doi: 10.1007/s10531-009-9724-1
Silvertown, J., Franco, M., and Menges, E. (1996). Interpretation of elasticity matrices as an aid to the management of plant populations for conservation. Conserv. Biol. 10, 591–597. doi: 10.1046/j.1523-1739.1996.10020591.x
Silvertown, J., Franco, M., Pisanty, I., and Mendoza, A. N. A. (1993). Comparative plant demography–relative importance of life-cycle components to the finite rate of increase in woody and herbaceous perennials. J. Ecol. 81, 465–476.
Stott, I., Hodgson, D. J., and Townley, S. (2012a). Beyond sensitivity: nonlinear perturbation analysis of transient dynamics. Methods Ecol. Evol. 3, 673–684. doi: 10.1111/j.2041-210X.2012.00199.x
Stott, I., Hodgson, D. J., and Townley, S. (2012b). Popdemo: an R package for population demography using projection matrix analysis. Methods Ecol. Evol. 3, 797–802. doi: 10.1111/j.2041-210X.2012.00222.x
Stott, I., Townley, S., and Hodgson, D. J. (2011). A framework for studying transient dynamics of population projection matrix models. Ecol. Lett. 14, 959–970. doi: 10.1111/j.1461-0248.2011.01659.x
Tomimatsu, H., and Ohara, M. (2010). Demographic response of plant populations to habitat fragmentation and temporal environmental variability. Oecologia 162, 903–911. doi: 10.1007/s00442-009-1505-8
Torres, A. M., Adarve, J. B., Cárdenas, M., Vargas, J. A., Londoño, V., Rivera, K., et al. (2012). Dinámica sucesional de un fragmento de bosque seco tropical del Valle del Cauca, Colombia. Biota Colomb. 13, 66–85.
Tremblay, R. L. (1997). Distribution and dispersion patterns of individuals in nine species of Lepanfhes (Orchidaceae) 1. Biotropica 29, 38–45.
Tremblay, R. L., Ackerman, J. D., and Pérez, M. E. (2010). Riding across the selection landscape: Fitness consequences of annual variation in reproductive characteristics. Philos. Trans. R. Soc. B 365, 491–498.
Tremblay, R. L., and Hutchings, M. J. (2002). Population Dynamics in Orchid Conservation: a Review of Analytical Methods Based on the Rare Species Lepanthes eltoroensis. Orchid conservation. Kota Kinabalu: Natural History Publications (Borneo), 183–204.
Tremblay, R. L., Raventos, J., and Ackerman, J. D. (2015). When stable-stage equilibrium is unlikely: integrating transient population dynamics improves asymptotic methods. Ann. Bot. 116, 381–390. doi: 10.1093/aob/mcv031
Tremblay, R. L., Tyre, A. J., Pérez, M. E., and Ackerman, J. D. (2021). Population projections from holey matrices: using prior information to estimate rare transition events. Ecol. Model. 447:109526. doi: 10.1016/j.ecolmodel.2021.109526
Vandermeer, J., Armbrecht, I., De la Mora, A., Ennis, K. K., Fitch, G., Gonthier, D. J., et al. (2019). The community ecology of herbivore regulation in an agroecosystem: lessons from complex systems. BioScience 69, 974–996. doi: 10.1093/biosci/biz127
Vélez-Torres, I., Varela, D., Cobo-Medina, V., and Hurtado, D. (2019). Beyond property: rural politics and land-use change in the Colombian sugarcane landscape. J. Agrarian Change 19, 690–710. doi: 10.1111/joac.12332
Vellend, M., Baeten, L., Becker-Scarpitta, A., Boucher-Lalonde, V., McCune, J. L., Messier, J., et al. (2017). Plant biodiversity change across scales during the Anthropocene. Annu. Rev. Plant Biol. 68, 563–586. doi: 10.1146/annurev-arplant-042916-040949
Ventre-Lespiaucq, A. B., Delgado, J. A., Ospina-Calderón, N. H., Otero, J. T., Escudero, A., Sánchez, M. A., et al. (2017). A tropical epiphytic orchid uses a low-light interception strategy in a spatially heterogeneous light environment. Biotropica 49, 318–327. doi: 10.1111/btp.12425
Wickham, H., Averick, M., Bryan, J., Chang, W., McGowan, L., François, R., et al. (2019). Welcome to the tidyverse. J. Open Source Softw. 4:1686. doi: 10.21105/joss.01686
Winkler, K., Fuchs, R., Rounsevell, M., and Herold, M. (2021). Global land use changes are four times greater than previously estimated. Nat. Commun. 12, 1–10. doi: 10.1038/s41467-021-22702-2
Zewdie, B., Tack, A. J., Ayalew, B., Wondafrash, M., Nemomissa, S., and Hylander, K. (2022). Plant biodiversity declines with increasing coffee yield in Ethiopia’s coffee agroforests. J. Appl. Ecol. 59, 1198–1208. doi: 10.1111/1365-2664.14130
Zotz, G. (2007). Johansson revisited: the spatial structure of epiphyte assemblages. J. Veg. Sci. 18, 123–130. doi: 10.1111/j.1654-1103.2007.tb02522.x
Keywords: demography, landscape, matrix models, resiliency, Rodriguezia granadensis, tropical Andes, reproductive success, PPM
Citation: Ospina-Calderón NH, Tremblay RL, Torres AM and Flanagan NS (2023) The effect of habitat transformation on a twig epiphytic orchid: Evidence from population dynamics. Front. Ecol. Evol. 11:1135316. doi: 10.3389/fevo.2023.1135316
Received: 31 December 2022; Accepted: 27 January 2023;
Published: 14 February 2023.
Edited by:
Dennis Whigham, Smithsonian Institution, United StatesReviewed by:
Vladan Djordjević, University of Belgrade, SerbiaJonas Morales-Linares, Benemérita Universidad Autónoma de Puebla, Mexico
Copyright © 2023 Ospina-Calderón, Tremblay, Torres and Flanagan. This is an open-access article distributed under the terms of the Creative Commons Attribution License (CC BY). The use, distribution or reproduction in other forums is permitted, provided the original author(s) and the copyright owner(s) are credited and that the original publication in this journal is cited, in accordance with accepted academic practice. No use, distribution or reproduction is permitted which does not comply with these terms.
*Correspondence: Nhora Helena Ospina-Calderón, bmhvcmEub3NwaW5hQGNvcnJlb3VuaXZhbGxlLmVkdS5jbw==
†ORCID: Nhora Helena Ospina-Calderón, orcid.org/0000-0002-2433-8132; Raymond L. Tremblay, orcid.org/0000-0002-8588-4372; Alba Marina Torres, orcid.org/0000-0002-3010-2505; Nicola S. Flanagan, orcid.org/0000-0002-4909-8710