- 1School of Environmental Sciences, University of Hull, Hull, United Kingdom
- 2Department of Zoology, University of Cambridge, Cambridge, United Kingdom
- 3Froglife Trust, Peterborough, United Kingdom
Underpasses or road tunnels are increasingly installed to reconnect habitats and ensure safe wildlife passage, thus preventing habitat fragmentation caused by roads and mortality from collisions with vehicles. In the UK, such underpasses are regularly implemented for amphibians and especially the protected great crested newt, Triturus cristatus. However, roads are also a key source of environmental pollutants including trace metals, road salt, petroleum and diesel hydrocarbons and these might impact road mitigation structures where amphibians are funnelled to, yet the extent and implications of such pollutants are almost never quantified in relation to this aspect, despite the recognised sensitivity of amphibians to chemical pollutants. Sediments were analysed from four amphibian road mitigation tunnel sites across the UK and compared to natural soil formations at local reference sites to determine whether contaminants were indeed accumulating within the tunnels. Three potential contaminants (copper, lead, and total petroleum hydrocarbons) were found in greater concentrations in the underpass sediments than respective reference sites at three of four locations, while one (zinc) was found in greater concentrations at all four studied underpasses compared to reference sites. Aggregated sediment pH value was significantly greater in the underpass sediment than the respective reference sites at all four study sites and in several instances the contaminants reached values that exceeded the thresholds of environmental concern. Despite the large geographic area covered and the significant site differences the absolute values of potential pollutants in tunnels were similar across sites, thus suggesting similar pollution sources and pathways. These results suggest road tunnels installed for ecological mitigation could be a significant pathway for pollutants from road surfaces to amphibians and it is recommended that focussed monitoring and maintenance of the underpasses is enacted given that their short or long-term impacts on amphibians are currently unknown. Potential management options could include regular jet-washing of the underpasses, or alternatively, pre- or post-implementation modifications of mitigation designs should aim to minimise the pollution pathway from road surfaces.
1. Introduction
Roads are well understood to cause complex and substantial negative impacts on global biodiversity and the research related to these impacts, often termed “road ecology,” has developed significantly in the past decades in terms of documenting and understanding the consequences of wildlife traffic mortality and fragmentation of habitats, but also indirect impacts linked to noise, artificial light, and chemical pollution for a variety of taxa (Forman et al., 2003; Van Der Ree et al., 2015). Amphibians are often the focus of such studies as they can be relatively easy to study compared to other taxa but are also considered to be exceptionally at risk from road impacts including from direct road mortality due to their need to travel between terrestrial and aquatic habitats but also their vulnerability to pollution due to their more permeable skin (Beebee, 2013). Roads can create “road-effect zones,” where their impact extends as much as 1 km beyond their physical boundaries and influences the abundance and distribution of amphibian taxa (Eigenbrod et al., 2009) due to a combination of mortality, fragmentation and indirect impacts of disturbance. Chemical pollution from road surfaces for instance impacts amphibians in multiple ways and creates substantial road zones in some areas due to road salt and can create behavioural, physiological and demographic consequences for amphibians through wetland salinisation affecting different life stages (Sanzo and Hecnar, 2006; Karraker et al., 2008; Hintz and Relyea, 2019). However, little comparative research focus has been directed towards understanding the impacts of other road pollutants in relation to amphibians, such as specific trace metals, probably due to the multifactorial nature of these pollutants (e.g., acting in combinations of substances, pH levels, terrestrial or aquatic, etc.) as well as the logistical complexity of many of the required analyses. Given the continuous global expansion of road networks and traffic and the increasing available volume of research and evidence from practical mitigation implementation, it is important to identify outstanding knowledge gaps that might hamper the overall aim of reducing the negative impacts of transport infrastructure on amphibians, including for road pollutant substances for which, in some cases, even basic availability data remains scarce.
In the UK, the great crested newt (Triturus cristatus) is the largest species of newt and a strictly protected amphibian (Langton et al., 2001) yet, despite undergoing substantial declines in the latter half of the 20th century due to habitat loss (especially ponds) and fragmentation from urbanisation and agricultural intensification, the species remains relatively widespread and can be locally abundant (Langton et al., 2001). The species was listed on Annex IV of the EC Habitats and Species Directive (English Nature, 2001; Griffiths et al., 2010) and legislation protects the species at all life stages, and their habitats, from reckless and intentional damage and destruction (English Nature, 2001; Langton et al., 2001; Natural England, 2015). In areas considered potentially suitable, it is therefore a legal requirement to check for T. cristatus populations before construction of any new developments to prevent adverse impacts on the species that might be sublethal or lethal and, if a population is discovered, then mitigate the anticipated adverse impacts. The level of mitigation required depends on the size and type of anticipated impact, and the size and importance of the population affected but may include changes in timings of construction works, translocating the population to an area of newly created habitat, or exclusion from the area of works (English Nature, 2001). Given that development impacts often mean newt habitat becomes fragmented by a road, one specific mitigation option is the installation of tunnels beneath roads, which enable the species to migrate safely from one side of a road to another, thereby preventing habitat and metapopulation fragmentation and direct road mortality caused by crossing roads (English Nature, 2001; Schmidt and Zumbach, 2008; Lesbarrères and Fahrig, 2012; Matos et al., 2017). Such tunnels or underpasses have been shown to be effective solutions in some contexts (Lesbarrères et al., 2004; Jarvis et al., 2019) and come in a variety of technical specifications, from simple concrete tubes and various dimensions of concrete box culverts, to polymer concrete surface tunnels (ACO-type), where the top part is embedded in the road surface and provisioned with small grid openings that allow temperature and humidity to balance between the outside environment and the inner part of the tunnel, something directly relevant as temperate amphibians are reluctant to use cold, dry and entirely dark tunnels, especially over distances exceeding 15–20 m in length (Schmidt and Zumbach, 2008; Matos et al., 2019). However, White et al. (2017) highlighted concern that road pollutants may be entering and accumulating in sediments inside these tunnel structures, potentially exposing migrating newts or other amphibians to the plethora of pollutants associated with roads and traffic. Broadly, these typically consist of total petroleum and diesel hydrocarbons, trace metals, de-icing salts, and plastic/rubber particulates from vehicle wear and tear (Ward, 1990; Bäckström et al., 2004; Ziajahromi et al., 2020). There are 11 elements that are strongly correlated with roads which show an increase in concentration with mean traffic density (Ward, 1990). Several of these elements are sourced from general vehicle wear; copper (Cu), cadmium (Cd), and zinc (Zn) are known to disperse from brake linings, brake pads and tyre wear, and Zn may additionally be sourced from the corrosion of galvanised safety barriers and similar structures (Ward, 1990; Legret and Pagotto, 1999). Some multielement contamination may also stem from fuel drips and spillages; as well as typical hydrocarbons, petroleum and diesel can also contain a range of different trace metals including Cu, Pb, vanadium (V), and Zn (Chu-Van et al., 2020). Additional diffuse sources of some elements include vehicle emissions, drips of crankcase oil, and wear of asphalt road surfaces (Brown and Peake, 2006). Furthermore, White et al. (2017) found evidence of pollution in mitigation tunnels associated with the tunnel structures themselves, with highly alkaline waters (pH >10) leaching from the cement used as mortar to link together the polymer concrete sections of the tunnels. At such high pH, mobility of oxyanionic elements such as chromium (Cr), can also pose environmental risks alongside the extreme pH itself (e.g., Gomes et al., 2016). However, this was based on survey data from multiple tunnels but within a single mitigation site in Northern England and additional data is required to understand the broader relevance and implications of these initial findings.
Due to their permeable epidermis and typically biphasic aquatic and terrestrial life stages, many amphibians are highly sensitive to environmental changes, and environmental pollution is a regularly documented cause of global amphibian declines (Hayes et al., 2010). If road pollutants are accumulating in the tunnel sediment which migrating newts are frequently in close contact with, it is possible that they could be exposed to sublethal or even lethal effects in an environment which was placed to protect them from harm (White et al., 2017). Exposure of amphibians to road pollutants via mitigation is important to verify given that amphibians are funnelled by people towards and into such structures using barrier fences, therefore concentrating numerous or most individuals from an area into narrow engineering structures. Ensuring such structures are safe to use by amphibians, both in terms of lethal and non-lethal impacts, should be an important element of road ecology and ecological mitigation yet, in practice such studies are virtually non-existent to our knowledge in relation to road pollutants.
This study undertakes a multi-site assessment to investigate whether mitigation underpasses are preferential sinks for road-based pollutants compared to nearby reference sites in the UK and to create a first stage assessment of the potential problems and impacts as well as opportunities for solutions and future prioritised research.
2. Methods
2.1. Study sites
Road mitigation tunnels at four study sites across the UK were chosen for sediment analysis. Three of the four sites (Site 1, Site 2, and Site 3: Figure 1) host known T. cristatus populations of medium – large size, based on the Great Crested Newt Mitigation Guidelines (English Nature, 2001) as well as other amphibians and other threatened vertebrates. Site 4 has several substantial amphibian populations including common frog, Rana temporaria, common toad, Bufo bufo and palmate newt Lissotriton helveticus, though no T. cristatus populations, and all of which are known to use the road mitigation tunnels (Hill et al., 2019).
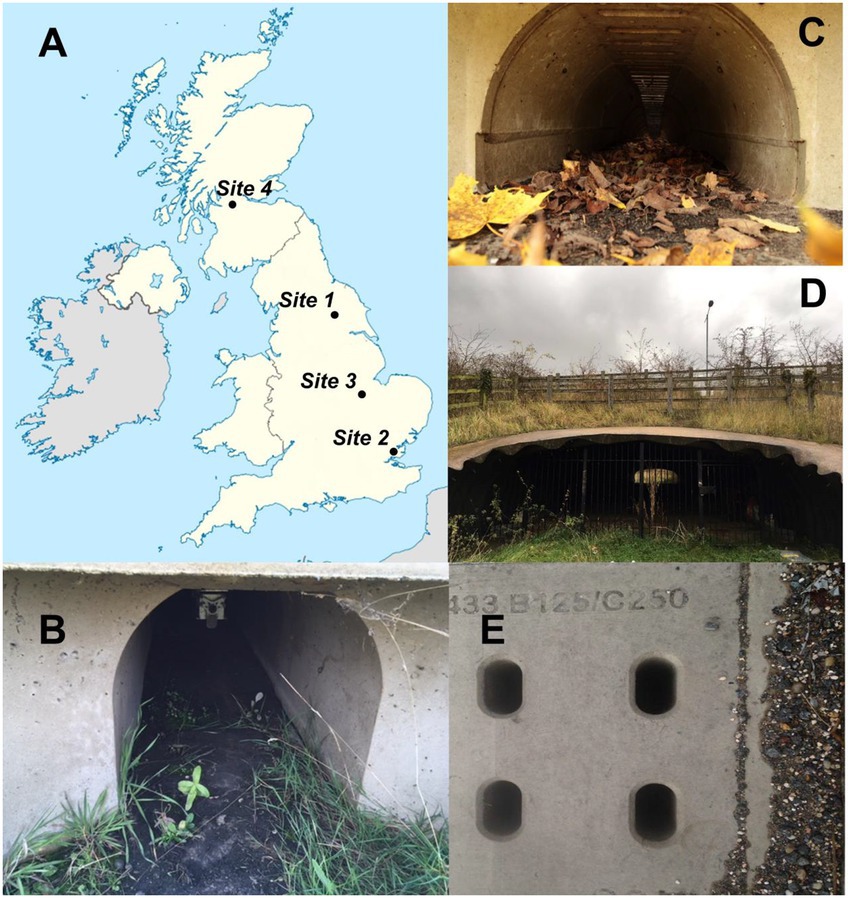
Figure 1. Site location (A) and example images of sample locations. (B) sediment build up in ACO tunnel entrance at Site 1; (C) ACO tunnel entrance showing grid at the top providing opening to the road surface above (Site 1); (D) larger concrete culverts – one of the two types of underpass installed at Site 3; (E) Road surface at Site 1 showing dust build up (right of image) and apertures in tunnel to provide light (and unintentional pollutant pathway).
Widely used polymer-concrete amphibian climate tunnels (also called surface tunnels or ACO-type tunnels) were installed at all four sites to enable individuals to safely navigate between ponds and terrestrial habitat to forage, breed and overwinter. The tunnels are approx. 0.5 m × 0.5 m and have open 6 × 3 cm slots along the road surface which lead down into the tunnels to enable light penetration during the day and humidity circulation inside the structures, to make the tunnels more attractive for individuals to use (Figure 1).
2.2. Site 1
The tunnels at Site 1 are situated beneath a main access road to a retail complex. The site consists of two pairs of polymer-concrete tunnels approximately 24 m in length, installed in 2013 (KT 500 climate tunnel with climate slots; KST 700 stilt tunnel sections and KP 1000 entrances, produced by ACO Germany). Traffic mostly comprises cars and buses. The site sits on Sherwood sandstone group bedrock and Alne glaciolacustrine superficial formation of clay and silt deposits [British Geological Survey (BGS), 2017].
2.3. Site 2
The four tunnels at Site 2 are situated beneath a main access road to an industrial park and were installed in 2013. Together, the tunnels and their respective fences cover a 350 m stretch of road, each tunnel approximately 20 m in length. Traffic largely comprises heavy goods vehicles (HGVs) and cars. The site sits on a Lambeth Group bedrock formation of clay, silt and sand and tidal flats from the Paleocene – Eocene epoch, and superficial deposits from the Holocene epoch, consisting of clay and silt [British Geological Survey (BGS), 2017].
2.4. Site 3
The three tunnels at Site 3 are situated beneath a busy arterial (‘A’) road traversing a designated protected site (Hampton Nature Reserve or Orton Pit SSSI) and were installed in 2006 to reconnect the two areas of habitat which is inhabited by an exceptional T. cristatus population of approximately 30,000 individuals; considered to be among the largest populations of the species in Europe (Matos et al., 2017). The tunnels include a very long, 30 m ACO surface tunnel as well two metal sheet and concrete large culverts (5.5 m wide × 2 m high, 40 m long; Matos et al., 2017, 2019; Figure 1). The site is situated on Oxford clay formation of Callovian age – Oxfordian age [British Geological Survey (BGS), 2017].
2.5. Site 4
Site 4 is situated below a residential access road which is estimated to experience a lower rate of daily traffic than Sites 1–3, mostly comprising cars, vans and non-motorised users, e.g., cyclists and pedestrians. The tunnels, three ACOs were installed in 2010 and are each approximately 13 m in length and together cover approximately 100 m stretch of road. The local area was historically industrial, with fired clay brick works and quarries. The site sits on a Passage formation clay bedrock of Arnsbergian sub-age and Langsettian sub-age and Lacustrine silt and clay superficial deposits from the Quaternary period [British Geological Survey (BGS), 2017].
2.6 Reference sites
Reference sites for all four study sites were selected in the local environs to the tunnels in scrub and pond habitat used by amphibians and chosen based on the following criteria: (1) they were at least 200 m from road influences and the tunnel locations (i.e., away from contemporary pollution sources and any drains from roads), and (2) they were located on the same superficial and bedrock geology as the tunnels are situated on at each site, with similar topography (White et al., 2017).
2.7. Field sampling
All four study sites were visited in Autumn (Fall) 2016 prior to application of de-icing salt to obtain a baseline of sediment quality (prior to potential variability associated with de-icing operations in cold months) with six sediment samples of approximately 30 g dry weight taken from the underpass entrances on both sides of the road and from the soil surface at the reference sites, less than 20 cm depth. Samples were collected with a stainless steel trowel, wiped clean between replicates to prevent cross-contamination, and sealed in air-tight plastic sample bags. Smaller quantities of sediment (approximately 20 g dry weight) were also sealed in-situ in amber glass jars which were to be taken for external analysis of Total Petroleum Hydrocarbons (TPH) content at Socotec Ltd. (UK). At some of the more recently installed tunnel sites, accumulation of sediment in the tunnels was relatively modest so the number of samples taken per site was inherently volume-limited. As such, the six samples from each tunnel site and associated reference site represented the balance between maximum number of replicate samples and the logistical constraints of sampling where sediment build-up was modest.
2.8. Laboratory analysis
Before analysis, all samples were air-dried in petri dishes in the lab for 5 days, until completely dry. Samples were individually homogenised using a pestle and mortar and separated with a <2 mm sieve. The fine fraction was used for subsequent analyses. For soil pH and electrical conductivity, sub-samples of fine (<2 mm) sediment were mixed with distilled water at a ratio of 5:1 (25 ml distilled water to 5 g dry sediment). Sediment solutions were then mixed using magnetic stirrers for 30 min in line with standard soil paste methods outlined by Johnsson et al. (2005) and ASTM D4972-13 (2013). The pH and electrical conductivity values of the solution were measured using a Myron L Company Ultrameter (6Psi), calibrated on the day of operation.
Organic matter content was measured on 5 g of each sample (after moisture removed at 105oC) at 450°C until a constant weight was achieved. Trace element content on sediments was undertaken on disaggregated fine fraction material which were encapsulated in cassettes topped with 6.0 μ Mylar X-Ray film (TF-160-255). These were analysed with a Thermo Niton XL3t XRF Analyser in soils mode for Ca, Ti, K, Fe, Mn, Zn, Cu, Cr, Zr, and Pb, which are widely used for screening elemental composition of soils and sediments (McComb et al., 2014; Rouillon and Taylor, 2016). Matrix-matched sediment Certified Reference Materials (CRM) were also used throughout this stage of the analysis for quality control and showed consistent accuracy within the range of values apparent in samples (Supplementary Table S1). For analysis of TPH, amber glass jars filled with sediment at source were sent to Socotec Ltd. (Bretby, South Yorkshire, UK) for analysis using gas chromatography with flame ionisation detector (GC-FID) within 2 days of sampling.
2.9. Data analysis
Significant differences in average elemental and major physico-chemical parameter composition between tunnel samples and adjacent reference samples were tested at each site using either Mann Whitney U tests given the small sample sizes (6 samples per site). Principal Component Analysis was used to assess the broader geochemical fingerprint of sediments across the sites and to create a visual representation of the chemical results in relation to their composition and survey site as is often undertaken in such initial studies (Emmerson et al., 1997; Álvarez-Iglesias et al., 2003; Reid and Spencer, 2009). Data were normalised using centre log ratio with values below detection limit recorded at half the detection limit given these accounted for less than 4% of data points (Reimann et al., 2011). Data were analysed in Minitab 17 (for comparisons between tunnels and reference sites) or R (PCA: R Core Team, 2022).
3. Results
3.1. Site 1
Sediment pH was significantly greater in the underpass sediment (Mann Whitney U, p = <0.001, median 8.3, range 8.1–8.6) than at the reference site (median = 6.4, range = 6.3–7.1; Figure 2). Neither electrical conductivity or organic content were significantly different between the underpass and reference sites (p values 0.0678 and 0.114 respectively). Most (10 of the 11 tested) parameters showed significant differences in concentration between the underpasses and reference sediments (Supplementary Table S2). Of these, six (Ca, Cu, Pb, Sr, Zn, and TPH) were in elevated concentrations in the underpass sediments (p-values between <0.001 and 0.026; Figure 3). The remaining four (Cr, Fe, K, and Ti) were elevated at the reference site (p-values between <0.001 to 0.049, Figure 3; Supplementary Figure S1). Mn was present in similar concentrations across the two sites (p values 0.743).
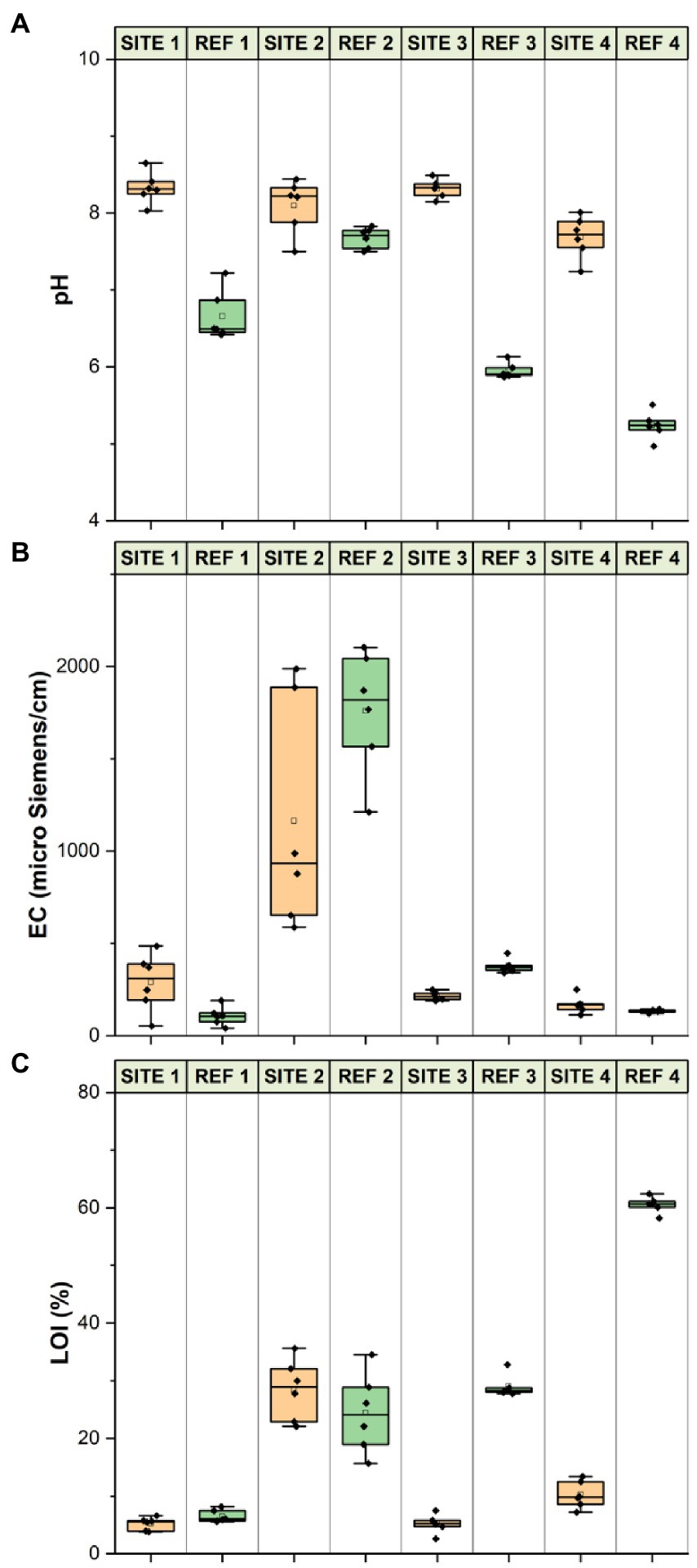
Figure 2. Distribution of sediment pH (A), sediment electrical conductivity (B) and sediment Loss on Ignition (C) between underpass sites (orange) and respective reference sites (green). Data show raw data points as dots, with 95th percentile, interquartile range and median shown by boxplots.
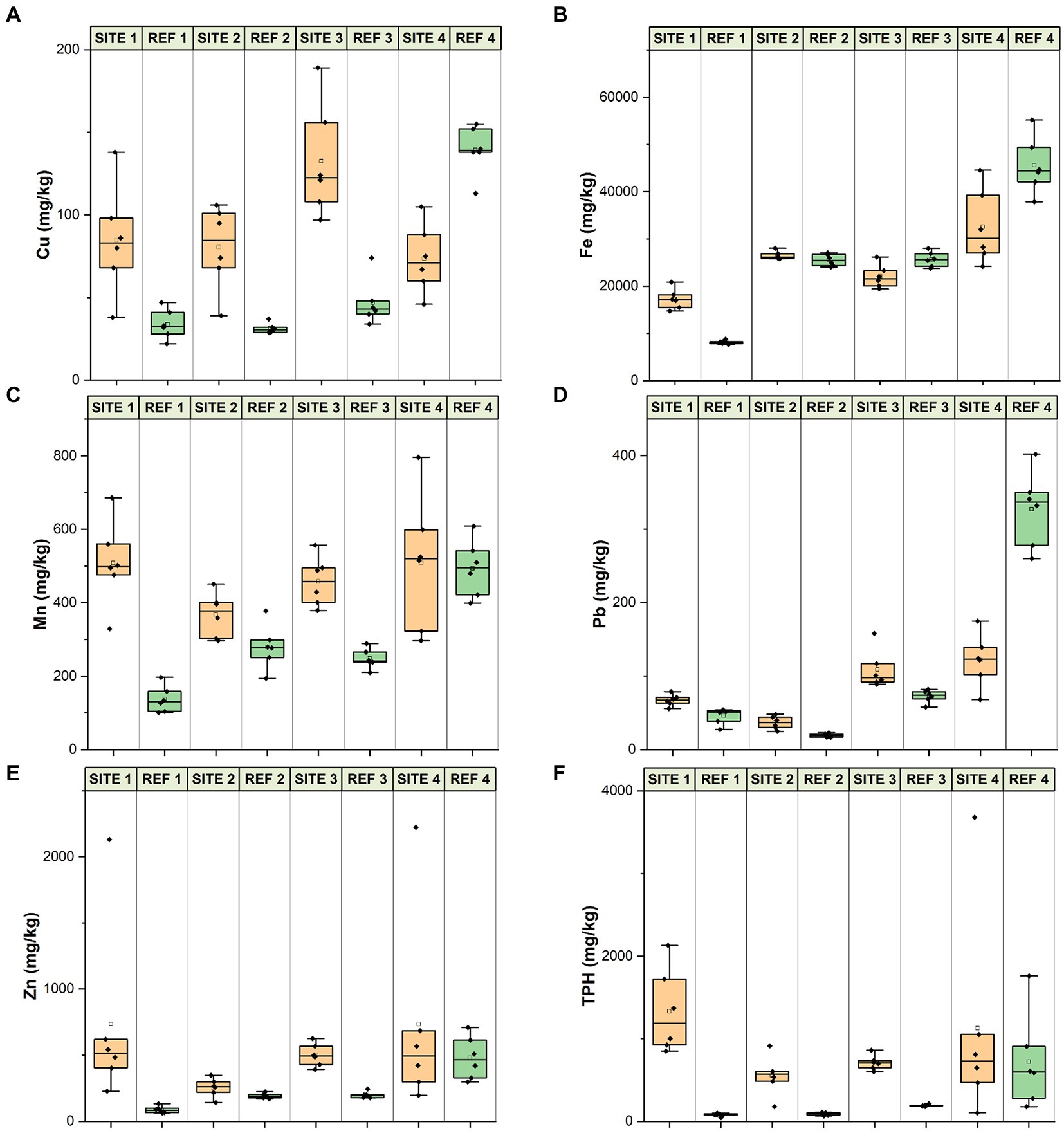
Figure 3. Concentrations of a range of potential pollutants between underpass sites (orange) and respective reference sites (green). (A) Copper; (B) Iron; (C) Manganese; (D) Lead; (E) Zinc; (F) Total Petroleum Hydrocarbons. All values in mg/kg dry weight sediment. Data show raw data points as dots, with 95th percentile, interquartile range and median shown by boxplots.
3.2. Site 2
Sediment pH was significantly higher in the underpasses compared to the reference site (Mann Whitney U, p = 0.002; Figure 2). Electrical conductivity was significantly lower in underpass sediments than reference samples (Mann Whitney U, p = <0.001). The median conductivity values of the underpass and reference replicates were 244 and 1154μS cm−1, respectively, (Figure 2). Sediment taken from the underpass had a significantly higher organic content than the reference site (Mann Whitney U, p = <0.001; Figure 2). Seven of the 10 tested parameters (Ca, Cu, Mn, Pb, Sr, Zn, and TPH) were in significantly greater concentrations in the underpass than at the reference site (Mann Whitney, p values between <0.001 and 0.0142; Figure 3; Supplementary Figure S1). Three elements (Cr, K, and Ti) were in greater concentrations at the reference site (p values between <0.001 and 0.002). Iron and zirconium were in similar concentrations across the two sample locations and no significant difference was observed (Mann Whitney, p = 0.301 and 0.309 respectively).
3.3. Site 3
All three major physicochemical parameters (pH, EC, Loss on Ignition) were significantly different between underpass and reference sites (Mann Whitney, all p-values < 0.001). Median pH of the underpass sediment was 8.3, which was much greater than the median pH of the reference sediment which was 5.9 (Figure 2; Supplementary Table 2). Conversely, electrical conductivity and organic content were both significantly greater at the reference site than the underpasses; median conductivity readings were 358.7 and 199.8μS cm−1 respectively, and organic matter content 28.8 and 5.7% (Figure 2). Organic content was almost 5× greater at the reference site. Seven of the 11 other tested parameters (Ca, Cu, Mn, Pb, Sr, Zn, and TPH) were in significantly higher concentrations in the underpass sediment than at the reference site (Figure 3; Supplementary Figure S1). Fe, K, and Ti were all in higher concentrations at the reference site (p values all <0.001; Figure 3; Supplementary Figure S1). There was no significant difference in chromium concentrations between tunnel and reference sites (Mann Whitney, p = 0.909).
3.4. Site 4
Electrical conductivity and pH were both significantly greater in the underpass sediment than the reference sediment (Mann Whitney U test: p-values 0.005 and <0.001 respectively). Median pH in the underpasses was relatively neutral at pH 7.68 but was only 5.14 at the reference site (Figure 2). Organic matter content was 7.5 times greater at the reference site than inside the underpasses; 60% compared to 8% (Mann Whitney U-test, p = <0.001; Figure 2), which is ascribable to the peat-rich soils in the habitat around the tunnels. Ca, Ti, Zn, and TPH were all found in similar concentrations at the underpass and reference sites and any differences were not statistically significant (p-values between 0.852 and 0.6171). Five elements (Cr, Cu, Fe, Mn, and Pb) were abundant in greater concentrations at the reference site (p-values between <0.001 and 0.023), and three (K, Sr, and Zr) were in greater concentrations in the underpasses (p-values between <0.001 and 0.017: Figure 3; Supplementary Figure S1).
3.5. Multisite comparison
3.5.1. pH and electrical conductivity
When sediment pH values were aggregated across sites the resulting value was significantly greater in the underpass sediment than the respective reference sites at all four study sites (p values between <0.001 and 0.01; see Supplementary Figure S2). No significant differences were evident in electrical conductivity or organic content of the sediments when aggregated and analysed together across the four sites (see Supplementary Figure S2).
3.5.2. Major and trace element patterns across sites
Very similar trends were observed between Site 1, Site 2 and Site 3; Ca, Cu, Pb, Zn, and TPH were all in significantly greater concentrations in the underpasses at three sites (p values all between <0.001 and 0.026), while K and Ti were all significantly greater at their respective reference sites (see Supplementary Table S2). Site 4 was only similar to the other three sites with concentrations of Sr and Zn, which were both significantly greater in the underpasses. This site departed from the trends of the other three for every other potential variable (Figure 3).
The Principal Component Analysis performed on the underpass sediment across all four sites and their respective reference sites shows where sites were similar (sites plot close together) or dissimilar (sites plot far apart) in chemical composition (Figure 4). Three significant components accounted for more than 10% of the variance individually (PCA 1 = 0.33.4, PCA 2 = 0.265, PCA 3 = 0.153). Cumulative of the 3 PCAs = 0.739 (75.2%). There is significant clustering of underpass sites in the centre to upper right of Figure 4 characterised by elevated Ca, Sr, TPH, Mn, Cu, and Zn, as well as elevated pH compared to most reference sites. The reference sites typically plot in the lower left (Sites 1–3) and typically poor in concentrations of those parameters, and lower in pH, but with relative enrichment of K, Ti, and organic matter (LOI). The Site 4 reference samples are a clear outlier from both underpass sites and other reference sites, being enriched in organic matter (LOI), Pb, Fe, and Ti in particular (Figure 4).
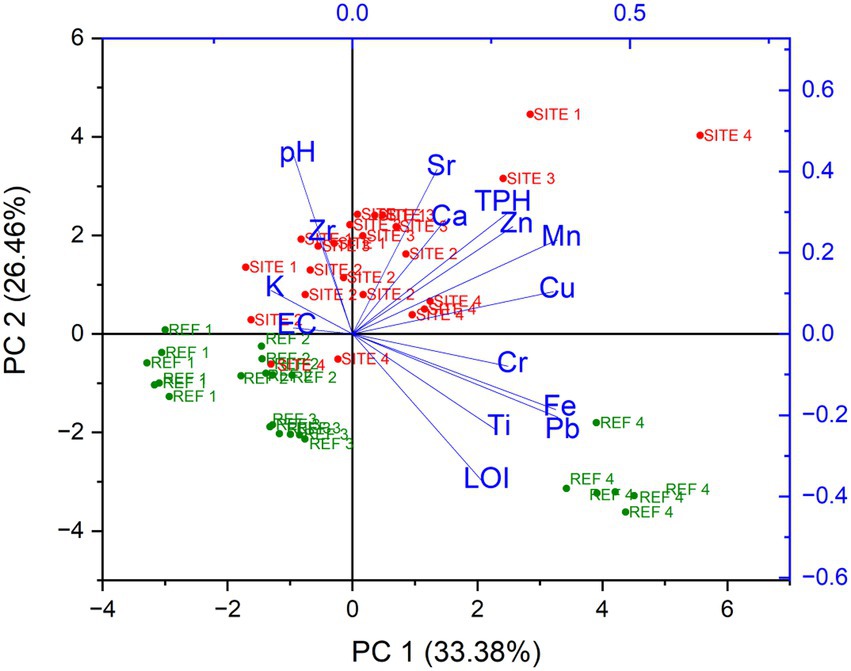
Figure 4. Principal Component Analysis of sediment chemical composition across the four study sites. Individual samples shown as points (red points: underpasses; green points: reference sites). Eigenvectors in blue show key characteristics of sample stations and inter-relationships between variables (where they plot in a similar direction and extent, variables are positively correlated). Based on scree analysis, there are three significant components accounting for a cumulative variance of 75.2% in the data.
4. Discussion
The data presented provide the first multisite assessment of potential pollutant build up in sediments of amphibian mitigation underpasses in the UK. There was significant elevation of Zn, Cu, Pb, and Total Petroleum Hydrocarbons (TPH) at three of the four study sites above adjacent reference site values. A comparison with regulatory sediment quality guidance (see Table 1 for definitions) shows concentrations of Zn, Cu, and Pb all exceed the lower screening values with Zn concentrations above the more precautionary Probable Effects Level (PEL) threshold at three sites. Even at Site 4, where tunnel sediment metal concentrations (Cu, Pb, and Zn) were either significantly lower or showed no difference from reference site values, the absolute metal concentrations are still in a range considered potentially problematic according to sediment quality screening criteria (Environment Agency, 2004; Buchmann, 2008). The unusually high metal concentrations in reference soils at Site 4 were despite the sample locations showing no link with contemporary or historical sources of water pollution. However, the lowland raised bog site lies downwind of what was historically a major centre for coal mining and iron and steel production (Riley et al., 2020) and as such the soils and sediments may reflect regional metal-enrichment from historical atmospheric deposition. Crucially, the absolute values of potential pollutants in tunnels are similar across all sites suggesting similar pollution sources and pathways between road surfaces and underpasses.
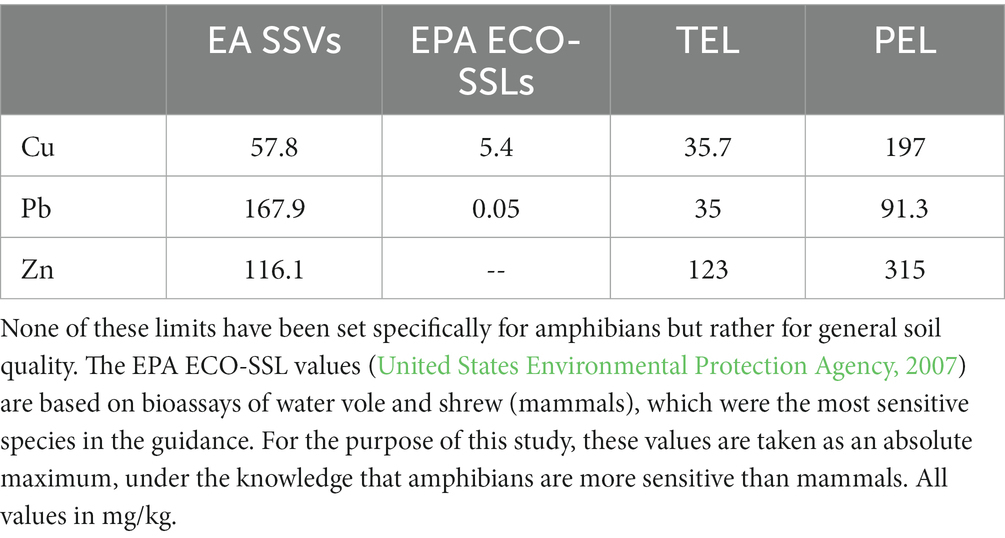
Table 1. Guidance values for good soil and sediment quality, including the UK Environment Agency’s non-statutory Soil Screening Values (SSVs: Environment Agency, 2017), the United States Ecological Soil Screening Levels (ECO-SSLs), and Threshold Effects Levels (TEL) and Probable Effects Levels (PEL – 50% adverse effects occur above this) taken from NOAA Buchman Screening Quick Reference Tables (2008).
Zn concentrations ranged between 258 and 567 mg/kg in the underpasses (Figure 3), which exceeded the Environment Agency Soil Screening Values (EA SSVs: Environment Agency, 2017) and Threshold Effects Levels (TEL: Environment Agency, 2004) at all sites. Site 1, 2, and 4, all exceeded the PEL of 315 mg/kg, though Site 3 did not. Zinc has been singled out as a particularly common hazard occurring in highways dusts in high concentrations (Świetlik et al., 2015) and is associated with tyre wear, corrosion of road barriers and brake disc wear (Blok, 2005). The data presented here suggest modest to high Zn concentrations are ubiquitous in amphibian underpasses at the sites sampled in concentrations of potential ecological significance.
Mean underpass concentrations of Cu across the four study sites ranged between 67 and 124 mg/kg (Figure 3), which were all greater than the lower soil guidance values at all study sites, but lower than the Probable Effects Level (PEL). The only standard that no sites were greater than was the more precautionary PEL (197 mg/kg), which Site 2 was closest to exceeding (average of 124 mg/kg). Cu concentrations in road dust have been shown to be more variable than Zn, being driven largely by wear and tear from braking, with higher concentrations around road junctions and intersections (Bäckström et al., 2004).
Mean concentrations of Pb across the four underpass sites (range 40–124 mg/kg; Figure 3) exceeded the US ECO-SSL (United States Environmental Protection Agency, 2007) and TEL at all sites, and at sites 2 and 4 concentrations additionally exceeded the PEL. Since the phasing out of lead additives in automobile fuel, Pb release associated with vehicles has fallen dramatically (Hwang et al., 2016). However, residual sources of Pb can be associated with tyre and brake wear as well as the loss and subsequent pulverisation of wheel weights (Świetlik et al., 2015).
Manganese (Mn) is present in underpass sediments in concentrations that are significantly elevated above reference sites at three of the four sites (Figure 2). Although there remains uncertainty about direct sources of Mn in highway dusts, Mn is used in anti-knocking fuel additives and can be released from brake wear (Fujiwara et al., 2011), and has been found in elevated concentrations in road dust (Świetlik et al., 2013). There are no formal UK soil or sediment screening guidance to compare absolute Mn values, but along with Ca, Cu and Zn, Mn is a key indicator of underpass sediment composition (Figure 4).
There are no formal standards or screening values for TPH values, but the data show significant elevations of TPH in all underpass sediments compared to reference conditions (Figure 3). The TPH method does not categorically show the hydrocarbons present are of petroleum origin (as analysis is subject to interference from organic matter and chlorinated solvents: Villalobos et al., 2008), but the generally lower organic matter concentrations in the underpass sediments are likely to limit such interference compared to reference sites (Supplementary Figure S2). As such the data suggest a potential pathway of exposure from petroleum hydrocarbons spilled on the road surface to the amphibian underpasses and should be the focus of more detailed analyses and attention.
The broader matrix of the tunnel sediments is generally consistent across the sites, being more mineral-rich (notably Ca and Fe) and displaying lower concentrations and clay-forming elements (e.g., K and Ti) than adjacent reference sites which typically have clay-rich superficial deposits (Figures 3, 4). Only at Site 3, where the larger culverts had a local soil substrate imported does the broader matrix reflect reference conditions. The generally higher inorganic content of the underpass sediments is reflected in the circum-neutral to alkaline pH reflecting the mineral-rich highways dusts as well as the weathering of tunnel construction materials and road surfaces (Gomes et al., 2016; White et al., 2017). Under this pH range, the mobility of most metals should be relatively restricted (Langmuir, 1997), which is tentatively positive. Furthermore, studies elsewhere of road dust metal mobility typically demonstrate metals present in hard-to-leach phases of low mobility (Świetlik et al., 2015). None of the sediments displayed highly alkaline conditions that have been previously documented in the drainage waters at one of the study sites (Site 1) and associated with leaching from cement in the tunnel walls (White et al., 2017).
Highway de-icing operations are a potential key threat to amphibians and widely documented as a pollutant pressure in many studies (e.g., Karraker and Ruthig, 2009; Denoël et al., 2010). The underpass sediment electrical conductivity (EC) values collected here did not show consistent elevation above reference sites (only Site 4 did: Figure 2), suggesting the accumulation and release of soluble salts from de-icing operations are of limited importance at the time of sampling (Autumn/Fall), but seasonal sampling suggests these underpass sediment EC can be variable during the course of the year (White, 2019) and should be a focus of future research.
These data provide a first multisite assessment of potentially common highways-derived pollutants in amphibian mitigation tunnels and highlight a pathway of potential movement of contaminants from road surfaces to tunnels. However, further research is needed to assess the subsequent risk associated with these pollutants (as well as other highways-derived pollutants such as microplastics: Kole et al., 2017) which should focus on potential bioavailability and likelihood of remobilisation into the water column during flood events (e.g., into adjacent ponds used by amphibians). More refined analyses of sediments to assess specific organic pollutants present and analyses that establish whether tunnel sediments reflect weathering products of the polymer-concrete tunnels themselves (e.g., through sediment carbonate analysis: e.g. Heiri et al., 2001) would be beneficial. Furthermore, seasonal assessment of changes in both sediment and water quality would be beneficial to understand and identify any short-term deterioration of water quality in receiving tunnels and adjacent habitat (e.g., rainfall events after de-icing salt application: Gallagher et al., 2011) and how these compare with critical times of year for amphibian movement. A degree of caution is required in the interpretation of these results as the current study was limited by funding for chemical analyses and aimed to cover a wide range of potential pollutants given that it is an initial investigation. Future studies should focus on specific elements included here and extend the surveys to more sites and multiple seasons, which would also allow a more robust analysis.
5. Management implications
It is clear from the literature on underpass effectiveness that although knowledge gaps remain for numerous species and types of mitigation designs, this form of mitigation can be successful at reducing habitat fragmentation and direct mortality via collisions with vehicles for amphibians (Schmidt and Zumbach, 2008; Hamer et al., 2015; Helldin and Petrovan, 2019; Jarvis et al., 2019; Schmidt et al., 2020). However, pollution remains very poorly quantified and the findings of this and previous studies (White et al., 2017) caution for the need for further consideration of highway-derived pollutants in the designing, installation and maintenance of such underpasses. The design of systems should endeavour to break any potential pathway between surface runoff on highways and the tunnels, for example by having road drains placed immediately up-gradient from tunnel vents and indeed and this is sometimes considered for ACO-type tunnels, which are positioned so that rainfall runoff is not directed towards the open grid at the top instead of normal water drainage. However, such aspects should be better clarified in technical guidance documents and then followed up during post-implementation monitoring. Importantly, it is relevant that while most of the underpasses we included were >20 m long, the road surface itself was much smaller and sometimes only represented a third of the tunnel length, with the rest taken by pedestrian access areas, cycle tracks and raised grass verges. This indicates that there might be simple compromises where the open grid tunnel sections would only be installed in the non-traffic areas and the part actually under the road could be kept without such open grids, thus reducing the opportunity for road pollutants to enter and accumulate in the tunnel sediment. However, any such design modifications should be implemented cautiously and include careful testing given that they might negatively impact on the acceptance of the structure for amphibians, including the great crested newt, which already show a propensity for U-turns and abandoning the tunnel crossing (Jarvis et al., 2019; Matos et al., 2019).
In addition, the variety of mitigation solutions used mean there is a need to target additional sites of different construction type for specific pollutant monitoring, both in the underpass and alongside the barrier fencing in order to create a clearer image on this topic. There is also a need to include other types of pollution and especially microplastic from tyre wear, which has been shown to be a significant issue in road dust (Leads and Weinstein, 2019). The impact of microplastics on amphibians of different life stages are very poorly understood but might be significant (Boyero et al., 2020; da Costa Araújo et al., 2020).
For existing tunnels and underpasses where highways-derived pollution might be an obvious problem (e.g., due to heavy vehicle or high volume traffic), potentially the most effective solution for maintenance may be to periodically jet-wash the inside of the underpasses to clear them of any contaminants from the road surface. Whilst sediment accumulation is attractive to amphibians and may increase usage rates compared to bare concrete surface inside the underpass (Lesbarrères et al., 2004), it is counter-productive if that sediment contains potentially harmful elements for amphibians. Tunnel jet-washing is already common practice in some European countries, e.g., Germany and is typically carried out at the end of winter, in order to remove potential road salt but also to clear the underpass from accumulated leaf litter which can be a significant issue and can prevent usage by amphibians by blocking the underpass. Issues of longer-term maintenance responsibility however need to be considered and clarified during planning (i.e., would the developer be responsible for long-term management of the underpasses or would local councils or even volunteers from conservation organisations). While the costs of such maintenance are usually low, in practice this can be a serious issue if there is no specific agreement in place and thus the entire mitigation structure might in fact be abandoned after the required monitoring period which is typically 5 years for protected species such as T. cristatus in the UK (Matos et al., 2017).
6. Conclusion
From the results of this study, it could be concluded that highway-derived pollutants are accumulating in road underpasses installed primarily for Triturus cristatus. Four potential contaminants (Cu, Zn, Pb, and TPH) were accumulating in the underpasses at three out of four study sites in levels above potential soil and sediment quality thresholds, suggesting that contamination is not an isolated occurrence at one development site yet the potential impacts of this remain unknown. The contaminated sediments could provide a secondary source of water pollution after rainfall events that may be of significance to amphibians using the tunnels. Further research is recommended, to include more refined analyses of contaminant form, to incorporate investigations of further sites, and consider bioavailabilty of potential contaminants. Ecotoxicological studies of T. cristatus and other amphibians utilising the tunnels to determine whether contamination is affecting their overall health and population size would also be desirable.
Data availability statement
The original contributions presented in the study are included in the article/Supplementary material, further inquiries can be directed to the corresponding author.
Author contributions
KW collected and analysed the samples. KW, SP, and WM contributed to the experiment design, interpretation, and manuscript writing. All authors contributed to the article and approved the submitted version.
Funding
The researchers are grateful for funding at Froglife Trust by the Esmée Fairbairn Foundation under grant NR 14-0534.
Acknowledgments
We thank the three reviewers for their comments and suggestions on the manuscript.
Conflict of interest
The authors declare that the research was conducted in the absence of any commercial or financial relationships that could be construed as a potential conflict of interest.
Publisher’s note
All claims expressed in this article are solely those of the authors and do not necessarily represent those of their affiliated organizations, or those of the publisher, the editors and the reviewers. Any product that may be evaluated in this article, or claim that may be made by its manufacturer, is not guaranteed or endorsed by the publisher.
Supplementary material
The Supplementary material for this article can be found online at: https://www.frontiersin.org/articles/10.3389/fevo.2023.1133253/full#supplementary-material
References
Álvarez-Iglesias, P., Rubio, B., and Vilas, F. (2003). Pollution in intertidal sediments of San Simon Bay (Inner Ria de Vigo, NW of Spain): total heavy metal concentrations and speciation. Mar. Pollut. Bull. 46, 491–503. doi: 10.1016/S0025-326X(03)00004-3
ASTM D4972-13 (2013). Standard test method for pH of soils, ASTM International, West Conshohocken, PA.
Bäckström, M., Karlsson, S., Bäckman, L., Folkeson, L., and Lind, B. (2004). Mobilisation of heavy metals by deicing salts in a roadside environment. Water Res. 38, 720–732. doi: 10.1016/j.watres.2003.11.006
Beebee, T. J. (2013). Effects of road mortality and mitigation measures on amphibian populations. Conserv. Biol. 27, 657–668. doi: 10.1111/cobi.12063
Blok, J. (2005). Environmental exposure of road borders to zinc. Sci. Total Environ. 348, 173–190. doi: 10.1016/j.scitotenv.2004.12.073
Boyero, L., López-Rojo, N., Bosch, J., Alonso, A., Correa-Araneda, F., and Pérez, J. (2020). Microplastics impair amphibian survival, body condition and function. Chemosphere 244:125500. doi: 10.1016/j.chemosphere.2019.125500
British Geological Survey (BGS) (2017). Geology of Britain viewer. Available at: http://mapapps.bgs.ac.uk/geologyofbritain/home.html (Accessed August 27, 2018).
Brown, J. N., and Peake, B. M. (2006). Sources of heavy metals and polycyclic aromatic hydrocarbons in urban stormwater runoff. Sci. Total Environ. 359, 145–155. doi: 10.1016/j.scitotenv.2005.05.016
Buchmann, M. F. (2008) NOAA screening quick reference tables. Available at: https://repository.library.noaa.gov/view/noaa/9327 (Accessed December 20, 2022).
Chu-van, T., Surawski, N., Ristovski, Z., Yuan, C. S., Stevanovic, S., Ashrafur Rahman, S. M., et al. (2020). The effect of diesel fuel sulphur and vanadium on engine performance and emissions. Fuel 261:116437. doi: 10.1016/j.fuel.2019.116437
da Costa Araújo, A. P., de Melo, N. F. S., de Oliveira Junior, A. G., Rodrigues, F. P., Fernandes, T., de Andrade Vieira, J. E., et al. (2020). How much are microplastics harmful to the health of amphibians? A study with pristine polyethylene microplastics and Physalaemus cuvieri. J. Hazard. Mater. 382:121066. doi: 10.1016/j.jhazmat.2019.121066
Denoël, M., Bichot, M., Ficetola, G. F., Delcourt, J., Ylieff, M., Kestemont, P., et al. (2010). Cumulative effects of road de-icing salt on amphibian behavior. Aquat. Toxicol. 99, 275–280. doi: 10.1016/j.aquatox.2010.05.007
Eigenbrod, F., Hecnar, S. J., and Fahrig, L. (2009). Quantifying the road-effect zone: threshold effects of a motorway on anuran populations in Ontario, Canada. Ecol. Soc. 14.
Emmerson, R. H. C., O’Reilly-Wiese, S. B., Macleod, C. L., and Lester, J. N. (1997). A multivariate assessment of metal distribution in inter-tidal sediments of the Blackwater Estuary, UK. Mar. Pollut. Bull. 34, 960–968. doi: 10.1016/S0025-326X(97)00067-2
Environment Agency (2004). Assessment of sediment contaminants in estuaries. Report for habitats directive technical advisory group on water quality by the sediment subgroup. Environment Agency of England and Wales. Report number WQTAG078K.
Environment Agency (2017). Soil screening values for assessing ecological risk. Available at: https://www.gov.uk/government/publications/soil-screening-values-for-assessing-ecological-risk [Accessed December 20, 2022].
Forman, R. T., Sperling, D., Bissonette, J. A., Clevenger, A. P., Cutshall, C. D., Dale, V. H., et al. (2003). Road ecology: Science and solutions. Washington: Island Press.
Fujiwara, F., Rebagliati, R. J., Dawidowski, L., Gómez, D., Polla, G., Pereyra, V., et al. (2011). Spatial and chemical patterns of size fractionated road dust collected in a megacitiy. Atmos. Environ. 45, 1497–1505. doi: 10.1016/j.atmosenv.2010.12.053
Gallagher, M. T., Snodgrass, J. W., Ownby, D. R., Brand, A. B., Casey, R. E., and Lev, S. (2011). Watershed-scale analysis of pollutant distributions in stormwater management ponds. Urban Ecosyst. 14, 469–484. doi: 10.1007/s11252-011-0162-y
Gomes, H. I., Mayes, W. M., Rogerson, M., Stewart, D. I., and Burke, I. T. (2016). Alkaline residues and the environment: a review of impacts, management practices and opportunities. J. Clean. Prod. 112, 3571–3582. doi: 10.1016/j.jclepro.2015.09.111
Griffiths, R. A., Sewell, D., and McCrea, R. S. (2010). Dynamics of a declining amphibian metapopulation: survival, dispersal and the impact of climate. Biol. Conserv. 143, 485–491. doi: 10.1016/j.biocon.2009.11.017
Hamer, A. J., Langton, T. E., and Lesbarrères, D. (2015). “Making a safe leap forward: mitigating road impacts on amphibians” in Handbook of road ecology. (Chichester, UK: Wiley), 261–270.
Hayes, T. B., Falso, P., Gallipeau, S., and Stice, M. (2010). The cause of global amphibian declines: a developmental endocrinologist’s perspective. J. Exp. Biol. 213, 921–933. doi: 10.1242/jeb.040865
Heiri, O., Lotter, A. F., and Lemcke, G. (2001). Loss on ignition as a method for estimating organic and carbonate content in sediments: reproducibility and comparability of results. J. Paleolimnol. 25, 101–110. doi: 10.1023/A:1008119611481
Helldin, J. O., and Petrovan, S. O. (2019). Effectiveness of small road tunnels and fences in reducing amphibian roadkill and barrier effects at retrofitted roads in Sweden. PeerJ 7:e7518. doi: 10.7717/peerj.7518
Hill, I. D. C., Rossi, C. A., Petrovan, S. O., Hartup, M., Clark, F., and Downie, J. R. (2019) in Mitigating the effects of a road on amphibian migrations: a Scottish case study of road tunnels vol. 27. eds. C. J. McInerny and I. Wilkie (Glasgow, UK: Glasgow Natural Histry Society), 25.
Hintz, W. D., and Relyea, R. A. (2019). A review of the species, community, and ecosystem impacts of road salt salinisation in fresh waters. Freshwater Biol. 64, 1081–1097. doi: 10.1111/fwb.13286
Hwang, H. M., Fiala, M. J., Park, D., and Wade, T. L. (2016). Review of pollutants in urban road dust and stormwater runoff: part 1. Heavy metals released from vehicles. Int. J. Urban Sci. 20, 334–360. doi: 10.1080/12265934.2016.1193041
Jarvis, L. E., Hartup, M., and Petrovan, S. O. (2019). Road mitigation using tunnels and fences promotes site connectivity and population expansion for a protected amphibian. Europ. J. Wildlife Res. 65:27. doi: 10.1007/s10344-019-1263-9
Johnsson, L., Nilsson, S. I., and Jennische, P. (2005). Desk study to assess the feasibility of a draft horizontal standard for electrical conductivity. Literature review.
Karraker, N. E., Gibbs, J. P., and Vonesh, J. R. (2008). Impacts of road deicing salt on the demography of vernal pool-breeding amphibians. Ecol. Appl. 18, 724–734. doi: 10.1890/07-1644.1
Karraker, N. E., and Ruthig, G. R. (2009). Effect of road deicing salt on the susceptibility of amphibian embryos to infection by water molds. Environ. Res. 109, 40–45. doi: 10.1016/j.envres.2008.09.001
Kole, P. J., Löhr, A. J., Van Belleghem, F. G., and Ragas, A. M. (2017). Wear and tear of tyres: a stealthy source of microplastics in the environment. Int. J. Environ. Res. Public Health 14:1265. doi: 10.3390/ijerph14101265
Langmuir, D. (1997). Aqueous environmental geochemistry. Prentice Hall: Upper Saddle River, NJ, 600.
Langton, T., Beckett, C., and Foster, J. (2001). Great crested newt conservation handbook. Suffolk, UK: Froglife.
Leads, R. R., and Weinstein, J. E. (2019). Occurrence of tire wear particles and other microplastics within the tributaries of the Charleston Harbor Estuary, South Carolina, USA. Mar. Pollut. Bull. 145, 569–582. doi: 10.1016/j.marpolbul.2019.06.061
Legret, M., and Pagotto, C. (1999). Evaluation of pollutant loadings in the runoff waters from a major rural highway. Sci. Total Environ. 235, 143–150. doi: 10.1016/S0048-9697(99)00207-7
Lesbarrères, D., and Fahrig, L. (2012). Measures to reduce population fragmentation by roads: what has worked and how do we know? Trends Ecol. Evol. 27, 374–380. doi: 10.1016/j.tree.2012.01.015
Lesbarrères, D., Lodé, T., and Merilä, J. (2004). What type of amphibian tunnel could reduce road kills? Oryx 38, 220–223. doi: 10.1017/S0030605304000389
Matos, C., Petrovan, S., Ward, A. I., and Wheeler, P. (2017). Facilitating permeability of landscapes impacted by roads for protected amphibians: patterns of movement for the great crested newt. PeerJ 5:e2922. doi: 10.7717/peerj.2922
Matos, C., Petrovan, S. O., Wheeler, P. M., and Ward, A. I. (2019). Short‐term movements and behaviour govern the use of road mitigation measures by a protected amphibian. Animal Conserv. 22, 285–296. doi: 10.1111/acv.12467
McComb, J. Q., Rogers, C., Han, F. X., and Tchounwou, P. B. (2014). Rapid screening of heavy metals and trace elements in environmental samples using portable X-ray fluorescence spectrometer, a comparative study. Water Air Soil Pollut. 225, 1–10. doi: 10.1007/s11270-014-2169-5
Natural England (2015) Great crested newts: protection and licensing. Available at: https://www.gov.uk/guidance/great-crested-newts-protection-surveys-and-licences [Accessed August 27, 2018].
R Core Team (2022). R: A language and environment for statistical computing. Vienna, Austria. Available at: https://www.R-project.org/ [Accessed December 19, 2022].
Reid, M. K., and Spencer, K. L. (2009). Use of principal components analysis (PCA) on estuarine sediment datasets: the effect of data pre-treatment. Environ. Pollut. 157, 2275–2281. doi: 10.1016/j.envpol.2009.03.033
Reimann, C., Filzmoser, P., Garrett, R., and Dutter, R. (2011). Statistical data analysis explained: Applied environmental statistics with R John Wiley & Sons.
Riley, A. L., MacDonald, J. M., Burke, I. T., Renforth, P., Jarvis, A. P., Hudson-Edwards, K. A., et al. (2020). Legacy iron and steel wastes in the UK: extent, resource potential, and management futures. J. Geochem. Explor. 219:106630. doi: 10.1016/j.gexplo.2020.106630
Rouillon, M., and Taylor, M. P. (2016). Can field portable X-ray fluorescence (pXRF) produce high quality data for application in environmental contamination research? Environ. Pollut. 214, 255–264. doi: 10.1016/j.envpol.2016.03.055
Sanzo, D., and Hecnar, S. J. (2006). Effects of road de-icing salt (NaCl) on larval wood frogs (Rana sylvatica). Environ. Pollut. 140, 247–256. doi: 10.1016/j.envpol.2005.07.013
Schmidt, B. R., Brenneisen, S., and Zumbach, S. (2020). Evidence-based amphibian conservation: a case study on toad tunnels. Herpetologica 76, 228–239. doi: 10.1655/0018-0831-76.2.228
Schmidt, B. R., and Zumbach, S. (2008). Amphibian road mortality and how to prevent it: a review. Urban herpetology. Herpetol. Conserv. 3, 157–167. doi: 10.5167/uzh-10142
Świetlik, R., Strzelecka, M., and Trojanowska, M. (2013). Evaluation of traffic-related heavy metals emissions using noise barrier road dust analysis. Pol. J. Environ. Stud. 22, 561–567.
Świetlik, R., Trojanowska, M., Strzelecka, M., and Bocho-Janiszewska, A. (2015). Fractionation and mobility of Cu, Fe, Mn, Pb and Zn in the road dust retained on noise barriers along expressway–a potential tool for determining the effects of driving conditions on speciation of emitted particulate metals. Environ. Pollut. 196, 404–413. doi: 10.1016/j.envpol.2014.10.018
United States Environmental Protection Agency (2007). Ecological soil screening level (eco-SSL) guidance and documents. Available at: https://www.epa.gov/risk/ecological-soil-screening-level-eco-ssl-guidance-and-documents (Accessed December 20, 2022).
Villalobos, M., Avila-Forcada, A. P., and Gutierrez-Ruiz, M. E. (2008). An improved gravimetric method to determine total petroleum hydrocarbons in contaminated soils. Water Air Soil Pollut. 194, 151–161. doi: 10.1007/s11270-008-9704-1
Ward, N. I. (1990). Multielement contamination of British motorway environments. Sci. Total Environ. 93, 393–401. doi: 10.1016/0048-9697(90)90130-M
White, K. J., (2019). Potential for accumulation of highway pollutants in road underpasses installed for the great crested newt (Triturus cristatus). MSc thesis. University of Hull, UK.
White, K. J., Mayes, W. M., and Petrovan, S. O. (2017). Identifying pathways of exposure to highway pollutants in great crested newt (Triturus cristatus) road mitigation tunnels. Water Environ. J. 31, 310–316. doi: 10.1111/wej.12244
Keywords: road mitigation, urban pollutants, road runoff, trace metals, de-icing salts, Triturus cristatus
Citation: White KJ, Petrovan SO and Mayes WM (2023) Pollutant accumulation in road mitigation tunnels for amphibians: A multisite comparison on an ignored but important issue. Front. Ecol. Evol. 11:1133253. doi: 10.3389/fevo.2023.1133253
Edited by:
Tibor Hartel, Babeș-Bolyai University, RomaniaReviewed by:
Andreea Zsigmond, Sapientia Hungarian University of Transylvania, RomaniaNándor Erős, Babeș-Bolyai University, Romania
Mikołaj Kaczmarski, Poznan University of Life Sciences, Poland
Copyright © 2023 White, Petrovan and Mayes. This is an open-access article distributed under the terms of the Creative Commons Attribution License (CC BY). The use, distribution or reproduction in other forums is permitted, provided the original author(s) and the copyright owner(s) are credited and that the original publication in this journal is cited, in accordance with accepted academic practice. No use, distribution or reproduction is permitted which does not comply with these terms.
*Correspondence: Silviu O. Petrovan, c29wMjFAY2FtLmFjLnVr