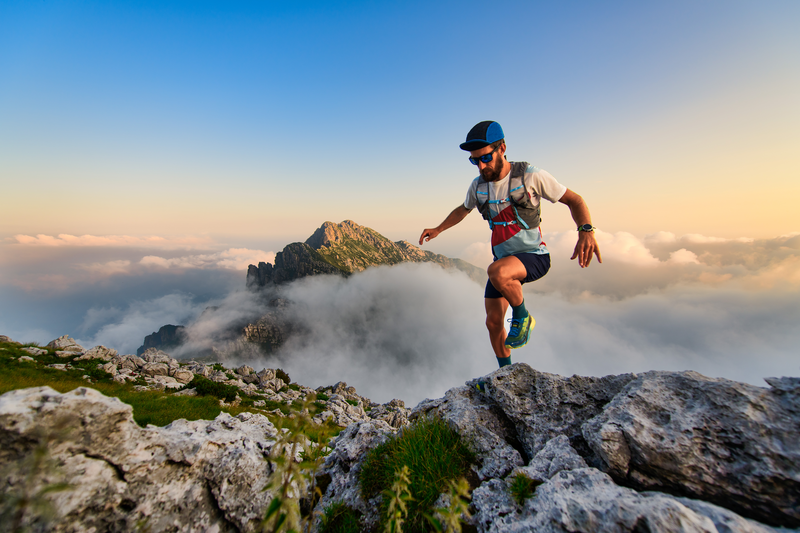
94% of researchers rate our articles as excellent or good
Learn more about the work of our research integrity team to safeguard the quality of each article we publish.
Find out more
ORIGINAL RESEARCH article
Front. Ecol. Evol. , 28 March 2023
Sec. Population, Community, and Ecosystem Dynamics
Volume 11 - 2023 | https://doi.org/10.3389/fevo.2023.1132248
This article is part of the Research Topic Forest Carbon Dynamics and Management Practices View all articles
Introduction: Increasing atmospheric N deposition and changes in precipitation patterns could profoundly impact forest community structure and ecosystem functions. However, most N and water (W) addition experiments have focused on direct N application to leaf litter or soil, neglecting canopy processes such as leaf evaporation and absorption.
Methods: In this study, we aimed to assess the effects of atmospheric N deposition and increased precipitation on the fine root biomass and morphology of plants in a temperate deciduous forest. To achieve this, we applied N and W above the forest canopy and quantified the seasonal dynamics (January, July, and October) of fine root biomass and morphology.
Results: Our results revealed that only canopy W addition significantly increased the biomass of fine roots in January compared to that in other seasons (p < 0.05). We observed no significant interaction effect of N and W on fine root biomass. However, we found that the different growth seasons had a significant impact on the fine root biomass (p < 0.001). The combined application of N and W significantly affected the root tip density (p = 0.002). Although canopy N addition was significantly positively correlated with available soil N (p < 0.05), we detected no significant association with fine root biomass or morphology.
Discussion: The findings of this study indicated that fine root biomass and morphology, are affected to a greater extent by the provision of W than by N application. These findings provide a new perspective and a more precise understanding of the effects of the actual N deposition and precipitation on the dynamics of plant fine roots in forest ecosystems.
Since the industrial revolution, the increase in industrial activities and widespread application of agricultural N fertilizers have considerably increased the N deposition on a global scale (Ackerman et al., 2019). Global climate models predict that global precipitation patterns will change in the future, including changes in the intensity, interval, spatial and temporal distribution, and total amounts of precipitation (Donat et al., 2016; Abbasi et al., 2020). These changes in the patterns of N deposition and precipitation are predicted to have a significant impact on the structure and function of some terrestrial ecosystems (Niu et al., 2008), leading to severe degradation in many areas.
Multiple drivers of global change affect the C cycle of forest ecosystems through complex interactions (Reich et al., 2018). N and water (W) are the main factors limiting plant growth and productivity in many terrestrial ecosystems (Zhang et al., 2020). N deposition can influence fine root (diameter ≤ 2 mm) biomass by altering forest ecosystem community composition and soil physicochemical properties. Although the effects of N deposition on fine root biomass have been extensively studied, no comprehensive consensus has been reached on the nature of these effects (Li et al., 2015; Peng et al., 2017; Geng et al., 2021). For example, an appropriate rate of N deposition can increase fine root biomass by altering forest plant community structure and composition (Yan et al., 2017), while excessive N input can lead to a loss of understory vegetation species (Walter et al., 2017), reduce species diversity (Payne et al., 2017), and result in a reduction in fine root biomass (Zeng et al., 2021). N deposition can lead to a reduction in soil pH (Shi et al., 2016), and an increase in the concentration of Al+ ions in acidified soil can inhibit fine root growth. Additionally, N input into the soil reduces the diversity of soil microorganisms (Wang et al., 2018), and soil microbial biomass is directly proportional to fine root biomass (Xu et al., 2022). Moreover, precipitation in the warm-temperate regions of China is expected to increase in the future (Yang et al., 2015). An increase in precipitation affects fine roots by increasing soil W content and reducing plant root respiration (Chen et al., 2019). For example, higher levels of precipitation can enhance root growth in the upper soil layer (Altinalmazis-Kondylis et al., 2020), and fine root biomass is positively correlated with N mineralization rate and soil W content (Singha et al., 2020). However, recent studies have shown that higher rainfall can reduce fine root biomass by reducing fine root respiration (Sun et al., 2020). To a certain extent, the levels of N deposition are associated with the amount of precipitation (Lim et al., 2015), and changes in precipitation patterns may substantially modify the effects of nitrogen deposition on the structure and function of ecosystems (Yang et al., 2011; Asaye and Zewdie, 2013). Given the non-additive effects of N and W addition in terrestrial ecosystems (Wang et al., 2019), it is important to simultaneously examine the impacts of these two factors on forest dynamics. However, few studies have assessed N and W interactions in temperate forests (Li X. et al., 2021). The seasonal dynamics of fine roots are also crucial, and therefore, seasonal differences in the response of highly active fine roots to N and W addition should be evaluated (Li W. et al., 2021).
It is estimated that the global fine root C storage exceeds atmospheric C storage by 5% (Bar-On et al., 2018). Fine root biomass accounts for 2–5% of total forest biomass (Veneklaas et al., 2012), and between 22–67% of annual terrestrial net primary productivity (Beidler and Pritchard, 2017). Fine roots play an essential role in the forest C cycle (Finér et al., 2011a), and their response to N deposition also affects the forest C cycle (Kou et al., 2018). Fine root dynamics have varying impacts on the C and N cycles. According to the resource economics theory, fine roots can increase their nutrient absorption and reduce C input by modifying their fineness, such as specific root length (SRL), specific root surface area (SRA), root tissue density (RTD), diameter, root tip, and root forks in response to changes in environmental resources (Eissenstat et al., 2000; Ostonen et al., 2007; Verburg et al., 2013; Kou et al., 2015; Li W. et al., 2021). Establishing the dynamic processes of fine roots is a fundamental requirement in the field of root ecology (Finér et al., 2011b). Moreover, to better understand the effects of fine root dynamics on nutrient cycles (Norby and Jackson, 2000; Pregitzer et al., 2002; Finér et al., 2011b), more experimental reports on the response of fine root biomass and morphology to global change are needed.
Although numerous studies have evaluated the effects of N and W addition on the fine root biomass and morphology of plants in forest ecosystems, most previous experimental work has focused solely on the addition of N and W to the soil or leaf litter, failing to consider canopy-associated ecological processes, such as evaporation, absorption by leaves, and microbial activity (Zhang et al., 2015; Shi et al., 2017). Therefore, little is known about the impact of N deposition and increased precipitation on the forest canopy and the structure of the forest ecosystem. For instance, foliar uptake of N and W may substitute or supplement root foraging, leading plants to alter their investment in fine roots (Zhang et al., 2015). In this study, we experimented in an area of broad-leaved mixed forest located within the Jigongshan (JGS) Nature Reserve, Central China. Based on the experimental addition of only N, only W, or both N and W to the canopy, we proposed three hypotheses. First, N element and W are the main limiting factors for plant growing. The addition of N and W may promote plant growth. So, we hypothesized that the effects of canopy N and W addition on the fine root biomass and morphology are consistent. Second, because the local rainfall is not scarce, we believe that the local forest ecosystem may be more deficient in N, followed by W. So, we hypothesized that N addition has a more significant effect on fine roots compared to W addition. Third, we hypothesized that there is an interaction effect between N and W on the fine root biomass and morphology.
The research site was situated in the JGS National Nature Reserve, located in central China (31°46′–31°52′N, 114°01′–114°06′E). The area experiences four distinct seasons, and its weather is typical of temperate forests. The mean annual temperature was 15.3°C, with a maximum of 27.5°C in July and a minimum of 1.9°C in January, based on the meteorological data recorded between 1951 to 2011. The mean annual rainfall was 1,102 mm, with 80% of the precipitation occurring between April and October, and the average annual air humidity was 79%. The soil type was Ferri-Udic Argosol (yellow-brown soil) with a pH of 5.0–6.0. The zonal vegetation was a deciduous broad-leaved mixed forest in which the dominant tree species were Quercus acutissima Carruth, Quercus variabilis Bl., and Liquidambar formosana Hance and common understory shrubs include Lindera glauca (Sieb. et Zucc.) Bl., Celtis sinensis Pers., and Rubus lambertianus Ser. The vegetation characteristics of the sample plots are listed in Table 1. The forest was 48 years old (thinned in 1970).
The experiment described in this study was conducted in 2012 using a completely random two-factor block design with four blocks. The blocks were selected to have more consistent vegetation, soil, and terrain to minimize errors. Each of the four treatments: control (CK, ambient conditions), canopy N addition (N, 25 kg ha−1 yr.−1), canopy W addition (W, 30% of the local precipitation), and canopy N and W addition (NW, addition of N at 25 kg ha−1 yr.−1 and W at 30% of the local precipitation) was randomly assigned to each block, resulting in a total of 16 sample plots. The N treatment received 25 kg ha−1 yr.−1, a rate expected to occur in the region in the near future. The magnitude of increased precipitation was within the range of model projections for future increases in Northern Hemisphere precipitation resulting from climate warming (Yang et al., 2015). The radius of each of the 16 sample plots had a radius of 17 m (907 m2 per plot). A buffer strip with a width of 20 m was placed between any two adjacent sample plots, and a PVC plate was inserted at a depth of 1 m in the buffer strip to reduce inter-plot root interference. A map of the experimental site can be found in Figure 1 of the study by Li X. et al. (2021).
Figure 1. Effects of the treatments on fine root biomass in July 2018, October 2018, and January 2019. CK, control; N, canopy N addition at 25 kg ha−1 yr.−1; W, canopy water (W) addition at 30% of the local precipitation; NW, canopy N addition at 25 kg ha−1 yr.−1 and W addition at 30% of the local precipitation. The data are presented as means ± standard errors.
Each of the 16 sample plots, except the control plots, had a 35 m tall tower, the upper parts of which projected 5 to 8 m above the canopy. A 360-degree rotating sprinkler was installed at the top of each tower to ensure uniform application of the N solution and water. The spraying distance of the sprinkler was 17 m (spraying area was 907 m2), and each spray delivered by the mounted sprinklers was equivalent to 2 mm of precipitation. Two large water storage tanks were set up near the experimental area, and the collected rainwater was used as a solution to dissolve N in the N treatment and as a source of rainwater for the W treatment. When the amount of rainwater collected was insufficient, the supply was replenished with water collected from a nearby lake. For the preparation of the N solution for the N treatment, the collected precipitation or lake W samples were analyzed each time, and the N concentration in the newly prepared solution was ensured to correspond to the specified treatment concentration by adding high-purity NH4NO3 (concentration 99.9%). Each spray delivered by the mounted sprinklers was equivalent to 2 mm of precipitation, which corresponded to less than 3% of the annual precipitation. For the W treatment, the additional water used in the experiment was sourced from the collected rainwater, which roughly represented the average chemical composition of rainfall in the area. Based on the precipitation data obtained between 1951 and 2011, the annual precipitation increase was estimated to be 336 mm. The increased amount of annual precipitation was evenly distributed during the growing season from April to October, which was equivalent to a 48 mm increase in precipitation per month. To ensure that the increased rainwater levels remained in the sample plot, monthly precipitation of 48 mm was increased every week (12 mm a week) to prevent rainwater from flowing out of the sample plot as a consequence of surface runoff. A sunny, windless morning or evening was selected for the application of each of the experimental treatments, thereby avoiding the confounding effects of local rainstorms and reduced evaporation. The treatments began in April 2013 and lasted for seven months each year and the timing of spraying was determined by the phenology of the JGS forest (Shi et al., 2017). The last treatment of the year was conducted in October. The test platform has been in operation since 2013.
Within each sample plot, we first determined the locations of the dominant species, including Q. acutissima, Q. variabilis, L. formosana, and common understory shrubs including L. glauca, C. sinensis, and R. lambertianus. We then selected a location in the sampling area that was relatively close to the dominant shrub species and not too far from the dominant tree species in the three sample plots. The terrain of the sampling area was relatively flat. To reduce errors, we used the same position in each sample plot, as the dominant species were widely distributed. We collected a soil block (30 × 30 × 30 cm length × width × height) from each plot using the block method (Liao et al., 2014). Three single samples were collected from each plot (16 × 3 samples in total) in mid-July 2018, mid-October 2018, and mid-January 2019 for a total of 144 clumps. To avoid damage to the internal root system, the soil blocks were maintained as intact as possible.
The soil blocks were transported to the laboratory and soaked in pure water to soften the soil. The intact root systems were then removed, and the remaining soil was passed through a 2-mm sieve to separate visible fine roots and remove large impurities. Finally, a 50-mesh sieve was used to completely separate the remaining fine roots. A Vernier caliper was used to measure the diameters of the extracted roots and collected fine roots with diameters of ≤2 (±0.5) mm. Finally, a scanner was used to determine the target fine roots (≤ 2 mm; 10000XL; Epson, Japan). The root samples were placed in self-sealing bags, numbered, and stored at −4°C until further analysis.
During the collection of fine root samples, three soil samples were also collected from each clump. The samples were then passed through a 2-mm sieve and combined to obtain a homogeneous composite sample, which served as the representative soil for each sub-batch. The composite samples were then divided into two sub-samples. One sub-sample was used as fresh material to measure available N (NO3-N and NH4-N), while the other sub-sample was used to measure soil moisture [(fresh weight -dry weight)/dry weight]. To measure NO3-N and NH4-N contents, a prepared soil sample (8 g) was extracted with 40 ml 2 M KCl (149 g L−1), shaken for 1 h, and filtered. NO3-N was directly measured from the filtrate using dual-wavelength colorimetry, whereas NH4-N was measured using the indophenol blue colorimetric method (chemical element analyzer; SMARTCHEM 200, Italy).
Morphology data, including length, surface area, volume, number of root tips, and number of root forks, were obtained using a double-lamp bed scanner (10000XL; Epson, Japan), and the scanned images were processed and analyzed using the WinRHIZO Pro 2007a software. To determine the biomass data, the fine roots were scanned and then dried in an oven at 65°C for 48 h until a constant weight was obtained. The SRL, SRA, and RTD were calculated using the following formulas:
where l, s, and v are the length, surface area, and volume of each fine root, respectively.
Previous studies have shown that N deposition has a significant impact on the forest community parameters (richness, stem density, mean diameter at breast height (DBH), and mean tree height) in a short time (Gundersen et al., 1998) unless the N concentration is high (more than 150 kg ha−1 yr.−1; Magill et al., 1997). Therefore, only one survey of vegetation was conducted in July 2018. All free-standing trees with a DBH of at least 1 cm (1.3 m above the ground) were tagged, measured, and identified to the species level. Vegetation height was measured using a height measuring rod (measuring range 0–40 m).
The R software (R v. 3.6.2; R Development Core Team) was used to calculate richness and stem density. Fine root data sorting and analysis were performed using the SPSS software (version 22.0; SPSS Inc., Chicago, IL, United States). A three-way ANOVA was used to examine the effects of N addition, W addition, sampling time (July, October, and January), and their interactions on fine root biomass and morphology. Redundancy analysis (RDA), carried out using Canoco 5, was used to analyze the relationship between environmental factors (soil properties and vegetation conditions) and fine root biomass and morphology. Structural equation modeling (SEM) was performed to assess how N and W affected the fine root biomass and morphology. Among them, morphological data used the axis with the highest interpretation rate in the RDA analysis. SEM was performed using Amos 26.0 (SPSS Inc. IL, United States). Graphics were produced using the Origin 2021 software.
Compared with the CK, the W treatment promoted a significant increase in fine root biomass in January (p < 0.05; Figure 1). Although the N and NW treatments had no significant effect on the fine root biomass in July, October, or January (Figure 1), we detected a significant effect of sampling time on fine root biomass, that is, fine root biomass increased in January and decreased in October (p < 0.001; Table 2).
Table 2. Effects of the addition of N, water (W), and sampling time (July 2018, October 2018, and January 2019); interactions between N and W addition, N addition and sampling time, and W addition and sampling time; and three-way interactions among N addition, W addition, and sampling time on fine root biomass, specific root length (SRL), specific root surface area (SRA), root tissue density (RTD), diameter, root tip density, root branching density, specific root tip density, and specific branching density.
Furthermore, the N and W treatments promoted a significant reduction in SRL in January (p < 0.05; Figure 2A), and the W treatment promoted a significant reduction in SRA in January (p < 0.05; Figure 2B). Compared with CK, in response to the W treatment, we detected a significant reduction in the RTD in October (p < 0.05; Figure 2C). Although the N treatment contributed to a reduction in the SRA and SRL, it promoted a significant increase in the RTD and diameter in January (p < 0.05; Figures 2C,D). Compared with the CK and NW treatments, the N treatment significantly increased the root tip density in July and January (p < 0.05; Figure 2E), We detected significant reductions in specific root tip density and specific branching density in response to the W treatment (p < 0.05; Figures 2G,H).
Figure 2. Effects of the treatments on specific root length (SRL); (A), specific root surface area (SRA); (B), root tissue density (RTD); (C), diameter (D), root tip density (E), root branching density (F), specific root tip density (G), and specific branching density (H) in July 2018, October 2018, and January 2019. CK, control; N, canopy N addition at 25 kg ha−1 yr.−1; W, canopy water (W) addition at 30% of the local precipitation; NW, canopy N addition at 25 kg ha−1 yr.−1 and W addition at 30% of the local precipitation. The data are presented as means ± standard errors.
The three-way interaction between the addition of N and W and sampling time was found to have a significant influence on SRL, SRA, RTD, root tip density, and root branching density (p = 0.008, p = 0.009, p = 0.017, p = 0.03, p = 0.032, respectively; Table 2). The interaction between the addition of N and W had a significant influence on root tip density (p = 0.02; Table 2). Furthermore, N addition had a significant effect on root diameter and root tip density (p = 0.04, p = 0.001, respectively; Table 2), whereas sampling time had a significant effect on fine root biomass and all morphological characteristics except for root tip density (p < 0.001; Table 2).
In response to the three treatments, we detected significant differences in the soil characteristics depending on the N addition levels (Table 3). The W treatment promoted a significant increase in soil moisture in July and October (p < 0.05), whereas the NW treatment promoted a significant increase in soil moisture in October (p < 0.05). In contrast, neither of these treatments had a significant impact on soil moisture in January. Furthermore, while the NW treatment significantly increased the soil content of NH4-N and NO3-N in July, October, and January (p < 0.05), the N treatment alone promoted a significant increase in NH4-N levels in October (p < 0.05, Table 3).
Table 3. Soil moisture content and available N (NH4-N, and NO3-N) content in July 2018, October 2018, and January 2019.
In July, species richness and soil moisture were positively correlated with fine root biomass but negatively correlated with fine root diameter (Figure 3A). Stem density, height, and DBH were positively correlated with RTD but negatively correlated with root branching density, SRL, specific branching density, specific root tip density, and SRA (Figure 3A). In October, soil moisture and NO3-N content were negatively correlated with root tip density and root branching density (Figure 3B). In January, in addition to soil NO3-N, other environmental factors were found to be positively correlated with fine root biomass and RTD but negatively correlated with SRL, SRA, specific root tip density, and specific branching density (Figure 3C).
Figure 3. Redundancy analysis of environmental factors (soil and vegetation) and fine root indices (biomass and morphology). Environmental factors (indicated by red lines) were used to explain the changes in fine root biomass and morphology (indicated by black lines). (A), July 2018; (B), October 2018; (C). January 2019; SRL, specific root length; SRA, specific root area; RTD, root tissue density; Tip/cm, root tip density; Forks/cm, root branching density; Tip/g, specific root tip density; forks/g, specific branching density. Vegetation index (DBH, height) refers to the average value of all species in the sample.
The canopy addition of N had significant direct positive effects on NH4-N (p < 0.001) and NO3-N (p < 0.001), no significant correlation with fine root biomass was found (Figure 4). W addition had a direct positive influence on plant morphology (p = 0.007; Figure 4) as well as indirect negative effects on plant morphology by increasing the soil moisture (Figure 4). Morphology was negatively correlated with fine root biomass (p = 0.001; Figure 4).
Figure 4. Structural-equation model (SEM) analysis of the influence pathways of canopy addition of N and water on fine root biomass and root fineness (include specific root length, specific root area, root tissue density, root tip density, root branching density, specific root tip density and specific branching density). The model fitting data are shown in the lower left corner of the pathway figure (a high p value associated with the χ2 test indicates a good fit of the model to the data, i.e., no significant discrepancies; DF, degrees of freedom; RMSEA, root mean squared error of approximation). The solid lines represent significant relationships (p < 0.05), and the dashed lines represent non-significant relationships (p > 0.05). The black arrows represent positive effects, and the red arrows represent negative effects. Values associated with the arrows and their width represent standardized path coefficients. R2 values associated with the response variables indicate the proportion of variation explained by relationships with other variables.
Our results revealed that the addition of N to the forest canopy had no significant effect on fine root biomass (Figure 1). This was not consistent with our first hypothesis, which we believe could be attributed to how N was applied. Compared with the traditional method of N addition to soils, we applied N at the level of the forest canopy, during which it can be assumed that part of the applied N was lost via natural processes such as evaporation, absorption by leaves and microorganisms, and adsorption by bark (Zhang et al., 2015). Our previous study demonstrated that 44–52% of N and W addition was intercepted by the canopy (Liu et al., 2020). Consequently, only a proportion of the originally applied N eventually enters the soil (Shi et al., 2016). Among the assessed response factors, we established that the N treatment only promoted a significant increase in NH4-N in October (p < 0.05, Table 3). Our SEM analysis revealed that although the addition of N had a direct positive effect on fine root biomass, this effect was not significant (Figure 4). Although the findings of some previous studies have indicated that the foliar uptake of N and W should increase the plant’s ability to assimilate C and may therefore increase the quantity of C allocated to fine root production (Berry et al., 2014; Nair et al., 2016), foliar uptake of N and W may substitute for or supplement root foraging, thereby causing plants to alter their investment in fine roots (Reich, 2014). Our results also showed that W addition to the forest canopy promoted a significant increase in fine root biomass in January (p < 0.05; Figure 1). This was inconsistent with our first and second hypotheses. The RDA revealed that soil moisture was positively correlated with fine root biomass in January (Figure 3C), and our SEM results also revealed a significant positive correlation between the addition of W and soil moisture (p < 0.001, Figure 4). Under a sufficient supply of soil nutrients, W becomes the only factor limiting fine root growth. The application of W to the canopy resulted in a significant increase in soil moisture (p < 0.05; Table 3) and promoted the growth of fine roots. Increasing W levels are known to enable vegetation to allocate larger amounts of photosynthetic products to fine root production (Bai et al., 2010).
In contrast to the addition of either N or W alone, their combined application was found to promote a reduction in the sum of fine root biomass over the three assessed months (Figure 1). This was consistent with our third hypothesis. The SEM revealed that the addition of N was positively correlated with NH4-N and NO3-N and that the addition of W positively correlated with NH4-N, which may be associated with an interaction between N and W (p < 0.05; Figure 4). Although some ecological processes in the forest canopy, such as evaporation and N uptake by leaves and trunks, can contribute to reducing the impact of canopy N addition on the soil N content, the simultaneous addition of N and W appeared to reduce the functioning of certain ecological processes in the forest canopy (for example, W addition can wash the N remaining in the canopy to the ground), thereby enabling larger amounts of N and W to reach the soil level (Table 3). The addition of W can also enhance soil W availability (Harpole et al., 2007), stimulate soil microbial activity, and modify soil N use efficiency (Wang et al., 2006). In wet soil, the most commonly present form of N is NO3-N, which is more easily used by plants than NH4-N. Our SEM findings also indicated a significant positive correlation between precipitation and soil NH4-N (p < 0.05; Figure 4). In this context, the theory of optimal partitioning allocation proposes that when the concentration of soil N exceeds the demands of plants, vegetation allocates fewer photosynthates to the belowground parts, resulting in a reduction in fine root biomass (Zhang and Wang, 2012).
Our experiment showed that the morphological changes in fine roots were not consistent with our previous two assumptions. Compared to the CK, we found that the application of W to the forest canopy was associated with a reduction in SRL and SRA in January (Figures 2A,B). An increase in SRL can effectively increase the contact area between the roots and soil, thereby increasing soil absorption of nutrients and reducing the C input of vegetation to fine roots (Eissenstat et al., 2000; Verburg et al., 2013; Kou et al., 2015; Li W. et al., 2021). The W treatment promoted an increase in soil moisture (p < 0.05; Table 3), and the RDA revealed that soil moisture was negatively correlated with SRL and SRA in January (Figure 3C). These findings indicated that although plants reduce SRL and SRA, an increase in biomass facilitates their nutrient uptake (Figure 1; Li W. et al., 2021). Compared with the W-only treatment, the combined application of W and N was found to contribute to a significant reduction in the RTD of fine roots in July (Figure 2C), whereas the W treatment promoted a significant reduction in RTD in October (Figure 2C). Given that low-density tissues can attain rapid relative growth rates and access to resources, plants can promptly expand their leaf, stem, or root systems, and their investment in dry matter is low (Huang et al., 2010). During the growing season, plant requirements for nutrients (such as available N and W) increase, and to obtain sufficient amounts of these nutrients, plants increase their SRL and SRA at the expense of RTD (Kramer-Walter et al., 2016). In the present study, the W treatment significantly decreased the RTD of fine roots (p < 0.05, Figure 2C), which may be because fine roots decreased the RTD by increasing SRL and SRA to meet W absorption. We found that all three additional treatments promoted increases in fine root RTD in January and that among these treatments, the N treatment had a significant effect (p < 0.05, Figure 2C). Notably, compared to those with slower growth and higher tissue density, fine roots with lower tissue density tend to have shorter lifespans and are generally more vulnerable to attacks by herbivores and pathogens (Wells et al., 2002). During the non-growing season, plants invest large amounts of resources in the RTD, thereby ensuring the survival of these roots. In addition, N addition reduces the soil pH value (Shi et al., 2017), thereby increasing the abundance of free Al3+ ions. These ions inhibit the growth of fine root tips and further increase the RTD (Li W. et al., 2021).
Furthermore, we found that the addition of N to the forest canopy significantly increased the fine root diameter in January (p < 0.05, Figure 2D). The RDA analysis revealed that NH4-N was positively correlated with root diameter in January (Figure 3C). The associated increase in soil N availability also alters the survival strategy of fine roots, and by promoting a thickening of fine root diameter and prolonging the life of these roots, facilitates the acquisition of nutrients (Wells et al., 2002). In terms of the cost–benefit theory, the benefit of prolonging fine root life (obtaining N) is greater than the cost of maintaining fine roots (consuming C) and may also be greater than the cost of producing new roots. Hence, the root growth strategy adopted by plants is based on selecting the strategy with the least cost and the most benefits (Eissenstat et al., 2013). Another explanation is that although N addition generally reduces mycorrhizal infections, the larger the diameter of the root and the thicker the cortex, the greater the chance of mycorrhizal infection. This leads to a cycle. For example, arbuscular mycorrhiza (AM) fungi infect the root cortex cells đ and form clumps or vesicles in the root cells, increasing the diameter of fine roots and cortex thickness (Kong et al., 2014).
It has been previously established that by increasing the number of root branches, plants can enhance their absorption of W and nutrients in the upper soil layers, whereas increasing the number of root tips can promote the absorption of W and nutrients in deeper soil layers (Børja et al., 2008). In the present study, we found that the N treatment significantly increased the tip density of fine roots in July and January (p < 0.05; Figure 2E). This effect can be ascribed to the fact that the addition of N promotes soil available N (Table 3), with NH4-N and NO3-N stimulating root branching and elongation, respectively (Remans et al., 2006).
Apart from the additional treatments per se, we found that, except for root tip density, sampling time was associated with significant differences in fine root biomass and morphology (three-way ANOVA, p < 0.001; Table 2). Consistent with the findings of numerous previous studies, we confirmed that root dynamics vary depending on the season (Pregitzer et al., 2000).
Based on the RDA, we detected associations between certain vegetation indicators and morphology. For example, DBH was found to be positively correlated with RTD in July (Figure 3A), but negatively correlated with root tip and root branching density in October (Figure 3B). We suspected that these differences may be a consequence of plant competition, which is necessary for plant growth and development (Rubio et al., 2001). A higher RTD indicates that the root system provides greater support to above-ground parts. However, an increase in RTD was obtained at the expense of inputs to fine roots with respect to other morphological trait indices, such as root tip density and root branching. Our SEM analysis revealed a significant negative correlation between morphology and biomass (p = 0.001; Figure 4). The increase in morphology (especially SRL and SRA) can compensate for the decrease in fine root biomass, which was consistent with the aforementioned minimum cost–maximum benefit strategy associated with root growth (Eissenstat et al., 2013). By increasing the length, surface area, and several root tips and forks, fine roots can increase the area of contact with soil, thereby enhancing their W and nutrient absorption capacities. We found that root morphology was negatively correlated with NH4-N and positively correlated with NO3-N (Figure 4). This may be because NO3-N, as the main N element in the soil rather than NH4-N, is better absorbed by fine roots. The absorption of NH4-N in fine roots may increase their respiration, thus increasing the consumption of C (Li W. et al., 2021). In addition, morphology was found to be more closely correlated with soil moisture than with available N, as indicated by the significant negative correlation between soil moisture and morphology (p < 0.05; Figure 4). This may indicate that morphology is particularly sensitive to soil moisture and, consequently, that soil moisture is the main factor influencing fine root dynamics in temperate forest ecosystems. This finding is contrary to our second hypothesis.
Because of the differences in plant height, vertical structure, and light conditions in a community (Poorter et al., 2005), the fine root morphology of trees and shrubs may also greatly differ. For example, trees can make more use of sunlight to synthesize organic matter, while shrubs can mainly derive nutrients from the soil. Therefore, canopy treatment first affects the trees, while the entry of N and W into the soil further affects the survival strategy of the roots of trees and shrubs (Reich, 2014). Previous studies have shown that the fine roots of shrubs have higher SRL and SRA and smaller diameters than those of trees (Jagodzinski and Kalucka, 2011). Therefore, the fine roots of shrubs are more sensitive to environmental changes. In this experiment, the morphological changes in the fine roots of shrubs may be more significant than those of trees, which requires further study in the future.
In the present study, we evaluated the effects of canopy addition of N, W, and N + W on the dynamics of fine root biomass and morphology in a temperate deciduous forest. Our findings indicated that canopy application of N alone or a combination of N and W had no significant effects on fine root biomass. The response of morphology to different canopy inputs tended to differ according to the season, although no clear patterns were identified. Collectively, however, our findings indicated that with respect to fine root dynamics, W, rather than N, is the predominant limiting factor in the study forest. To gain a more comprehensive understanding of the dynamics of fine roots in relation to soil C cycling, broader and more precise time scales should be considered, and an assessment of whole-plant data should be carried out.
The raw data supporting the conclusions of this article will be made available by the authors, without undue reservation.
WL, CW, HL, WW, RS, ML, YS, DZ, WD, LM, and SF contributed to the conception and design of this study. WL: conceptualization, methodology, software, validation, formal analysis, investigation, data curation, and writing - original draft. CW, HL, WW, RS, ML, and WD: investigation. YS: investigation and formal analysis. DZ: software, formal analysis, and data curation. LM: conceptualization, investigation, formal analysis, data curation, writing - review and editing, and project administration. SF: supervision, resources, and project administration. All authors contributed to the article and approved the submitted version.
This work was supported by the National Natural Science Foundation of China (grant numbers 41501568 and 31800438), the NSFC-Henan Joint Fund (grant number U1904204), the Innovation Scientists and Technicians Troop Construction Projects of Henan Province (grant number 182101510005), and the China Postdoctoral Science Foundation (2020 M672206).
The authors declare that the research was conducted in the absence of any commercial or financial relationships that could be construed as a potential conflict of interest.
All claims expressed in this article are solely those of the authors and do not necessarily represent those of their affiliated organizations, or those of the publisher, the editors and the reviewers. Any product that may be evaluated in this article, or claim that may be made by its manufacturer, is not guaranteed or endorsed by the publisher.
Abbasi, A. O., Salazar, A., Oh, Y., Reinsch, S., del Rosario Uribe, M., Li, J., et al. (2020). Reviews and syntheses: soil responses to manipulated precipitation changes–an assessment of meta-analyses. Biogeosciences 17, 3859–3873. doi: 10.5194/bg-17-3859-2020
Ackerman, D., Millet, D. B., and Chen, X. (2019). Global estimates of inorganic nitrogen deposition across four decades. Global Biogeochem. Cycles 33, 100–107. doi: 10.1029/2018GB005990
Altinalmazis-Kondylis, A., Muessig, K., Meredieu, C., Jactel, H., Augusto, L., Fanin, N., et al. (2020). Effect of tree mixtures and water availability on belowground complementarity of fine roots of birch and pine planted on sandy podzol. Plant Soil 457, 437–455. doi: 10.1007/s11104-020-04741-8
Asaye, Z., and Zewdie, S. (2013). Fine root dynamics and soil carbon accretion under thinned and un-thinned Cupressus lusitanica stands in, southern Ethiopia. Plant Soil 366, 261–271. doi: 10.1007/s11104-012-1420-3
Bai, W., Wan, S., Niu, S., Liu, W., Chen, Q., Wang, Q., et al. (2010). Increased temperature and precipitation interact to affect root production, mortality, and turnover in a temperate steppe: implications for ecosystem C cycling. Glob. Chang. Biol. 16, 1306–1316. doi: 10.1111/j.1365-2486.2009.02019.x
Bar-On, Y. M., Phillips, R., and Milo, R. (2018). The biomass distribution on earth. Proc. Natl. Acad. Sci. U. S. A. 115, 6506–6511. doi: 10.1073/pnas.1711842115
Beidler, K. V., and Pritchard, S. G. (2017). Maintaining connectivity: understanding the role of root order and mycelial networks in fine root decomposition of woody plants. Plant Soil 420, 19–36. doi: 10.1007/s11104-017-3393-8
Berry, Z. C., White, J. C., and Smith, W. K. (2014). Foliar uptake, carbon fluxes and water status are affected by the timing of daily fog in saplings from a threatened cloud forest. Tree Physiol. 34, 459–470. doi: 10.1093/treephys/tpu032
Børja, I., De Wit, H. A., Steffenrem, A., and Majdi, H. (2008). Stand age and fine root biomass, distribution and morphology in a Norway spruce chrono sequence in Southeast Norway. Tree Physiol. 28, 773–784. doi: 10.1093/treephys/28.5.773
Chen, F., Yan, G., Xing, Y., Zhang, J., Wang, Q., Wang, H., et al. (2019). Effects of N addition and precipitation reduction on soil respiration and its components in a temperate forest. Agri. For. Meteorol. 271, 336–345. doi: 10.1016/j.agrformet.2019.03.021
Donat, M. G., Lowry, A. L., Alexander, L. V., O’Gorman, P. A., and Maher, N. (2016). More extreme precipitation in the world’s dry and wet regions. Nat. Clim. Chang. 6, 508–513. doi: 10.1038/nclimate2941
Eissenstat, D. M., McCormack, M. L., and Du, Q. (2013). “Global change and root lifespan, plant roots” in The Hidden Half. 4th ed. eds. A. Eshel and T. Beeckman (Florida: CRC Press), 399–412.
Eissenstat, D. M., Wells, C. E., Yanai, R. D., and Whitbeck, J. L. (2000). Building roots in a changing environment: implications for root longevity. New Phytol. 147, 33–42. doi: 10.1046/j.1469-8137.2000.00686.x
Finér, L., Ohashi, M., Noguchi, K., and Hirano, Y. (2011a). Factors causing variation in fine root biomass in forest ecosystems. For. Ecol. Manag. 261, 265–277. doi: 10.1016/j.foreco.2010.10.016
Finér, L., Ohashi, M., Noguchi, K., and Hirano, Y. (2011b). Fine root production and turnover in forest ecosystems in relation to stand and environmental characteristics. For. Ecol. Manag. 262, 2008–2023. doi: 10.1016/j.foreco.2011.08.042
Geng, Q., Ma, X., Liao, J., Wu, W., Niu, S., Luo, Y., et al. (2021). Contrasting nutrient-mediated responses between surface and deep fine root biomass to N addition in poplar plantations on the east coast of China. For. Ecol. Manag. 490:119152. doi: 10.1016/j.foreco.2021.119152
Gundersen, P., Emmett, B. A., Kjønaas, O. J., Koopmans, C. J., and Tietema, A. (1998). Impact of nitrogen deposition on nitrogen cycling in forests: a synthesis of NITREX data. For. Ecol. Manag. 101, 37–55. doi: 10.1016/S0378-1127(97)00124-2
Harpole, W. S., Potts, D. L., and Suding, K. N. (2007). Ecosystem responses to water and nitrogen amendment in a California grassland. Glob. Chang. Biol. 13, 2341–2348. doi: 10.1111/j.1365-2486.2007.01447.x
Huang, G., Zhao, X. Y., Zhao, H. L., Huang, Y. X., and Zuo, X. A. (2010). Linking root morphology, longevity and function to root branch order: a case study in three shrubs. Plant Soil 336, 197–208. doi: 10.1007/s11104-010-0466-3
Jagodzinski, A. M., and Kalucka, I. (2011). Fine root biomass and morphology in an age-sequence of post-agricultural Pinus sylvestris L. stands. Dendrobiology 66, 71–84.
Kong, D., Ma, C., Zhang, Q., Li, L., Chen, X., Zeng, H., et al. (2014). Leading dimensions in absorptive root trait variation across 96 subtropical forest species. New Phytol. 203, 863–872. doi: 10.1111/nph.12842
Kou, L., Guo, D., Yang, H., Gao, W., and Li, S. (2015). Growth, morphological traits and mycorrhizal colonization of fine roots respond differently to nitrogen addition in a slash pine plantation in subtropical China. Plant Soil 391, 207–218. doi: 10.1007/s11104-015-2420-x
Kou, L., Jiang, L., Fu, X., Dai, X., Wang, H., and Li, S. (2018). Nitrogen deposition increases root production and turnover but slows root decomposition in Pinus elliottii plantations. New Phytol. 218, 1450–1461. doi: 10.1111/nph.15066
Kramer-Walter, K. R., Bellingham, P. J., Millar, T. R., Smissen, R. D., Richardson, S. J., and Laughlin, D. C. (2016). Root traits are multidimensional: specific root length is independent from root tissue density and the plant economic spectrum. J. Ecol. 104, 1299–1310. doi: 10.1111/1365-2745.12562
Li, W., Jin, C., Guan, D., Wang, Q., Wang, A., Yuan, F., et al. (2015). The effects of simulated nitrogen deposition on plant root traits: a meta-analysis. Soil Biol. Biochem. 82, 112–118. doi: 10.1016/j.soilbio.2015.01.001
Li, W., Shi, Y., Zhu, D., Wang, W., Liu, H., Li, J., et al. (2021). Fine root biomass and morphology in a temperate forest are influenced more by the nitrogen treatment approach than the rate. Ecol. Indic. 130:108031. doi: 10.1016/j.ecolind.2021.108031
Li, X., Zhang, C., Zhang, B., Wu, D., Zhu, D., Zhang, W., et al. (2021). Nitrogen deposition and increased precipitation interact to affect fine root production and biomass in a temperate forest: implications for carbon cycling. Sci. Total Env. 765:144497. doi: 10.1016/j.scitotenv.2020.144497
Liao, Y., McCormack, M. L., Fan, H., Wang, H., Wu, J., Tu, J., et al. (2014). Relation of fine root distribution to soil C in a Cunninghamia lanceolata plantation in subtropical China. Plant Soil 381, 225–234. doi: 10.1007/s11104-014-2114-9
Lim, H., Oren, R., Palmroth, S., Tor-ngern, P., Mörling, T., Näsholm, T., et al. (2015). Inter-annual variability of precipitation constrains the production response of boreal Pinus sylvestris to nitrogen fertilization. For. Ecol. Manag. 348, 31–45. doi: 10.1016/j.foreco.2015.03.029
Liu, Y., Tan, X., Wang, Y., Guo, Z., He, D., Fu, S., et al. (2020). Responses of litter, organic and mineral soil enzyme kinetics to 6 years of canopy and understory nitrogen additions in a temperate forest. Sci. Total. Environ 712:136383. doi: 10.1016/j.scitotenv.2019.136383
Magill, A. H., Aber, J. D., Hendricks, J. J., Bowden, R. D., Melillo, J. M., and Steudler, P. A. (1997). Biogeochemical response of forest ecosystems to simulated chronic nitrogen deposition. Ecol. Appl. 7, 402–415. doi: 10.1890/1051-0761(1997)007[0402:BROFET]2.0.CO;2
Nair, R. K., Perks, M. P., Weatherall, A., Baggs, E. M., and Mencuccini, M. (2016). Does canopy nitrogen uptake enhance carbon sequestration by trees? Glob. Chang. Biol. 22, 875–888. doi: 10.1111/gcb.13096
Niu, S., Wu, M., Han, Y., Xia, J., Li, L., and Wan, S. (2008). Water-mediated responses of ecosystem carbon fluxes to climatic change in a temperate steppe. New Phytol. 177, 209–219. doi: 10.1111/j.1469-8137.2007.02237.x
Norby, R. J., and Jackson, R. B. (2000). Root dynamics and global change: seeking an ecosystem perspective. New Phytol. 147, 3–12. doi: 10.1046/j.1469-8137.2000.00676.x
Ostonen, I., Püttsepp, Ü., Biel, C., Alberton, O., Bakker, M., Lõhmus, K., et al. (2007). Specific root length as an indicator of environmental change. Plant Biosys. 141, 426–442. doi: 10.1080/11263500701626069
Payne, R. J., Dise, N. B., Field, C. D., Dore, A. J., Caporn, S. J., and Stevens, C. J. (2017). Nitrogen deposition and plant biodiversity: past, present, and future. Front. Ecol. Env. 15, 431–436. doi: 10.1002/fee.1528
Peng, Y., Guo, D., and Yang, Y. (2017). Global patterns of root dynamics under nitrogen enrichment. Global Ecol. Biogeo. 26, 102–114. doi: 10.1111/geb.12508
Poorter, L., Bongers, F., Sterck, F. J., and Wöll, H. (2005). Beyond the regeneration phase: differentiation of height-light trajectories among tropical tree species. J. Ecol. 256–267. doi: 10.1111/j.1365-2745.2004.00956.x
Pregitzer, K. S., DeForest, J. L., Burton, A. J., Allen, M. F., Ruess, R. W., and Hendrick, R. L. (2002). Fine root architecture of nine north American trees. Ecol. Monogr. 72, 293–309. doi: 10.1890/0012-9615(2002)072[0293:FRAONN]2.0.CO;2
Pregitzer, K. S., King, J. S., Burton, A. J., and Brown, S. E. (2000). Responses of tree fine roots to temperature. New Phytol. 147, 105–115. doi: 10.1046/j.1469-8137.2000.00689.x
Reich, P. B. (2014). The world-wide ‘fast–slow’ plant economics spectrum: a traits manifesto. J. Ecol. 102, 275–301. doi: 10.1111/1365-2745.12211
Reich, P. B., Hobbie, S. E., Lee, T. D., and Pastore, M. A. (2018). Unexpected reversal of C3 versus C4 grass response to elevated CO2 during a 20-year field experiment. Science 360, 317–320. doi: 10.1126/science.aas9313
Remans, T., Nacry, P., Pervent, M., Filleur, S., Diatloff, E., Mounier, E., et al. (2006). The Arabidopsis NRT1. 1 transporter participates in the signaling pathway triggering root colonization of nitrate-rich patches. Proc. Natl. Acad. Sci. U. S. A. 103, 19206–19211. doi: 10.1073/pnas.0605275103
Rubio, G., Walk, T., Ge, Z., Yan, X., Liao, H., and Lynch, J. P. (2001). Root gravitropism and below-ground competition among neighbouring plants: a modelling approach. Ann. Bot. 88, 929–940. doi: 10.1006/anbo.2001.1530
Shi, L., Zhang, H., Liu, T., Mao, P., and Fu, S. (2017). An increase in precipitation exacerbates negative effects of nitrogen deposition on soil cations and soil microbial communities in a temperate forest. Env. Poll. 235, 293–301. doi: 10.1016/j.envpol.2017.12.083
Shi, L., Zhang, H., Liu, T., Zhang, W., Shao, Y., Ha, D., et al. (2016). Consistent effects of canopy vs. understory nitrogen addition on the soil exchangeable cations and microbial community in two contrasting forests. Sci. Total Env. 553, 349–357. doi: 10.1016/j.scitotenv.2016.02.100
Singha, D., Brearley, F. Q., and Tripathi, S. K. (2020). Fine root and soil nitrogen dynamics during stand development following shifting agriculture in Northeast India. Forests 11:1236. doi: 10.3390/f11121236
Sun, L., Hirano, T., Yazaki, T., Teramoto, M., and Liang, N. (2020). Fine root dynamics and partitioning of root respiration into growth and maintenance components in cool temperate deciduous and evergreen forests. Plant Soil 446, 471–486. doi: 10.1007/s11104-019-04343-z
Veneklaas, E. J., Lambers, H., Bragg, J., Finnegan, P. M., Lovelock, C. E., Plaxton, W. C., et al. (2012). Opportunities for improving phosphorus-use efficiency in crop plants. New Phytol. 195, 306–320. doi: 10.1111/j.1469-8137.2012.04190.x
Verburg, P. S., Young, A. C., Stevenson, B. A., Glanzmann, I., Arnone, J. A. III, Marion, G. M., et al. (2013). Do increased summer precipitation and N deposition alter fine root dynamics in a M ojave D esert ecosystem? Glob. Chang. Biol. 19, 948–956. doi: 10.1111/gcb.12082
Walter, C. A., Adams, M. B., Gilliam, F. S., and Peterjohn, W. T. (2017). Non-random species loss in a forest herbaceous layer following nitrogen addition. Ecology 98, 2322–2332. doi: 10.1002/ecy.1928
Wang, J., Hui, D., Lu, H., Wang, F., Liu, N., Sun, Z., et al. (2019). Main and interactive effects of increased precipitation and nitrogen addition on growth, morphology, and nutrition of Cinnamomum burmanni seedlings in a tropical forest. Global Ecol. Con. 20:e00734. doi: 10.1016/j.gecco.2019.e00734
Wang, C., Liu, D., and Bai, E. (2018). Decreasing soil microbial diversity is associated with decreasing microbial biomass under nitrogen addition. Soil Biol. Biochem. 120, 126–133. doi: 10.1016/j.soilbio.2018.02.003
Wang, C., Wan, S., Xing, X., Zhang, L., and Han, X. (2006). Temperature and soil moisture interactively affected soil net N mineralization in temperate grassland in northern China. Soil Biol. Biochem. 38, 1101–1110. doi: 10.1016/j.soilbio.2005.09.009
Wells, C. E., Glenn, D. M., and Eissenstat, D. M. (2002). Changes in the risk of fine-root mortality with age: a case study in peach, Prunus persica (Rosaceae). Am. J. Bot. 89, 79–87. doi: 10.3732/ajb.89.1.79
Xu, M. P., Wang, J. Y., Zhu, Y. F., Han, X. H., Ren, C. J., and Yang, G. H. (2022). Plant biomass and soil nutrients mainly explain the variation of soil microbial communities during secondary succession on the loess plateau. Microb. Ecol. 83, 114–126. doi: 10.1007/s00248-021-01740-9
Yan, G., Chen, F., Zhang, X., Wang, J., Han, S., Xing, Y., et al. (2017). Spatial and temporal effects of nitrogen addition on root morphology and growth in a boreal forest. Geoderma 303, 178–187. doi: 10.1016/j.geoderma.2017.05.030
Yang, S., Ding, Z., Li, Y., Wang, X., Jiang, W., and Huang, X. (2015). Warming-induced northwestward migration of the east Asian monsoon rain belt from the last glacial maximum to the mid-Holocene. Proc. Natl. Acad. Sci. U. S. A. 112, 13178–13183. doi: 10.1073/pnas.1504688112
Yang, H., Li, Y., Wu, M., Zhang, Z., Li, L., and Wan, S. (2011). Plant community responses to nitrogen addition and increased precipitation: the importance of water availability and species traits. Glob. Chang. Biol. 17, 2936–2944. doi: 10.1111/j.1365-2486.2011.02423.x
Zeng, W., Xiang, W., Zhou, B., Ouyang, S., Zeng, Y., Chen, L., et al. (2021). Positive tree diversity effect on fine root biomass: Via density dependence rather than spatial root partitioning. Oikos 130, 1–14. doi: 10.1111/oik.07777
Zhang, W., Shen, W., Zhu, S., Wan, S., Luo, Y., Yan, J., et al. (2015). Can canopy addition of nitrogen better illustrate the effect of atmospheric nitrogen deposition on forest ecosystem. Sci. Rep. 5:11245. doi: 10.1038/srep11245
Zhang, W., and Wang, S. (2012). Effects of NH4+ and NO3− on litter and soil organic carbon decomposition in a Chinese fir plantation forest in South China. Soil Biol. Biochem. 47, 116–122. doi: 10.1016/j.soilbio.2011.12.004
Keywords: canopy nitrogen addition, canopy water addition, fine root biomass, temperate forest, root morphology
Citation: Li W, Wang C, Liu H, Wang W, Sun R, Li M, Shi Y, Zhu D, Du W, Ma L and Fu S (2023) Fine root biomass and morphology in a temperate forest are influenced more by canopy water addition than by canopy nitrogen addition. Front. Ecol. Evol. 11:1132248. doi: 10.3389/fevo.2023.1132248
Received: 27 December 2022; Accepted: 08 March 2023;
Published: 28 March 2023.
Edited by:
Chunhua Zhang, Ludong University, ChinaCopyright © 2023 Li, Wang, Liu, Wang, Sun, Li, Shi, Zhu, Du, Ma and Fu. This is an open-access article distributed under the terms of the Creative Commons Attribution License (CC BY). The use, distribution or reproduction in other forums is permitted, provided the original author(s) and the copyright owner(s) are credited and that the original publication in this journal is cited, in accordance with accepted academic practice. No use, distribution or reproduction is permitted which does not comply with these terms.
*Correspondence: Lei Ma, cWRtYWxlaUBzaW5hLmNvbQ==; Shenglei Fu, ZnNsQGhlbnUuZWR1LmNu
Disclaimer: All claims expressed in this article are solely those of the authors and do not necessarily represent those of their affiliated organizations, or those of the publisher, the editors and the reviewers. Any product that may be evaluated in this article or claim that may be made by its manufacturer is not guaranteed or endorsed by the publisher.
Research integrity at Frontiers
Learn more about the work of our research integrity team to safeguard the quality of each article we publish.