- Department of Zoology, University of Granada, Granada, Spain
Human population growth is causing an expansion of urban areas, a phenomenon known to deeply impact on the Earth’s biodiversity. Therefore, it is key to understand how to conceal urban development with biodiversity conservation. In this context, university campuses can play an important role as they usually present a large array of different environments and green areas, crucial aspects for promoting urban biodiversity as well as human-nature interactions. Several studies have analyzed the biodiversity of university campuses, however, there are still important taxonomic (e.g., insects) and geographical biases (e.g., Mediterranean hotspot) in our current understanding of these urban areas. Insects are fundamental in many ecosystems as pollinators, prey, pest controllers or decomposers among others. This further increases the need to study this group in the urban context. In this study, we have investigated diurnal Lepidoptera and ground-dwelling Coleoptera in three university campuses and three non-campus areas of the city of Granada (Spain). We used spatial and temporal replicates for each area in order to explore whether university campuses hold higher levels of insect biodiversity (e.g., species richness or common species) than other nearby urban areas. In addition, we investigated the potential influence of several additional predictors on insect diversity such as type of land cover, vegetation origin, management intensity, and distance to the outskirts. Our results suggest that Lepidoptera species and Coleoptera families are more diverse in university campuses than in other urban areas, showing also a positive association with the proportion of bare soil and herbaceous cover. Furthermore, they also seem to be benefited from low vegetation management intensity whereas Coleoptera are favored by native vegetation providing clear management recommendations in order to promote such animal groups in cities. Our study indicates that university campuses are important urban areas to preserve insect biodiversity but also highlights the heterogeneity of response among insect groups.
Introduction
According to the last report of the United Nations, by mid-2020, the world’s population reached 7.8 billion, the result of unprecedented growth since the mid-twentieth century, a number that is expected to keep growing (United Nations, Department of Economic and Social Affairs, Population Division, 2022). Furthermore, more than 60% of the global population is located in urban areas (United Nations, Department of Economic and Social Affairs, Population Division, 2019), which occupy 3% of the Earth’s land surface (Balk et al., 2005), and keep expanding quickly due to a combination of four forces: population growth, rural exodus, massive migrations due to extreme events, and changes in administrative boundaries (McDonald et al., 2013; United Nations, Department of Economic and Social Affairs, Population Division, 2019). The urbanization process involves a rapid change in land cover, which has an enormous impact on the ecosystems of the planet (Gaston, 2010). Furthermore, there is growing evidence that urbanization has negative effects on biodiversity (Peng et al., 2020; Ren et al., 2022), mainly by habitat loss and fragmentation (Seto et al., 2012; Carpintero and Reyes-López, 2014; Elmqvist et al., 2015) although other forces such as water, air and soil pollution can also play an important role (Gaston, 2010).
In addition, non-native (sometimes introduced) species in urban areas (e.g., ornamental plants) could be determinant of an important biodiversity loss (Clarke et al., 2008; Nielsen et al., 2014; Threlfall et al., 2016). These species rise local biodiversity, but at the same time, reduce global biodiversity with the replacement of native with non-native taxa (McKinney and Lockwood, 1999) ultimately leading to the biotic homogenization of urban biodiversity caused by the presence of widely distributed and urban-tolerant non-native taxa (McKinney, 2006). This effect is especially relevant in regions with a high number of endemic species (biodiversity hotspots). In this sense, some authors draw attention to the Mediterranean area, which is a hotspot already diminished and severely fragmented, with soft habitat loss that may increase extinction rates (Cuttelod et al., 2009; Seto et al., 2012).
Historically, naturalists and ecologists have defended that urban areas are less valuable than wild or pristine environments, ignoring urban areas during the first half of the twentieth century (Grimm et al., 2008). This view has been changing since then, with the understanding that cultural and biological diversity together support resilience and sustainability (Berkes and Ross, 2013). Urbanization has multiple effects on the environment, but not all of them are negative. Human impacts diversify urban landscapes, modifying ecosystems and creating unique urban habitats (Gaston, 2010), which generates a mosaic of different, and often small land use types, that are the basic elements for generating research tools in urban ecology and urban biodiversity conservation (Breuste et al., 2008). In this sense, urban areas can still play an important role in conservation science, as there is evidence that these environments are sometimes the last hope for the conservation of several plant and animal species whose natural habitat is lost (Ives et al., 2016; Soanes and Lentini, 2019). The success of these programs in managing green patches and habitat corridors is necessary for the protection of area-sensitive species within urban areas (Vrezec et al., 2021), but the introduction of this scientific knowledge into city policy, governance, and design is still lacking (Gaston, 2010; Ikin et al., 2015). Furthermore, the study of urban biodiversity is key to conservation science and sustainability programs in urban landscapes, as it is referred to in the Sustainable Development Goals (goals 11 and 15, United Nations, 2015).
A well-designed urban environment can be fundamental in balancing human population growth with biodiversity conservation (Müller et al., 2010; Garrard et al., 2018). In this context, urban land cover types (Pauleit and Duhme, 2000) can be described according to their characteristic construction patterns and open spaces, that depend on the human activities that are developed within them, also determining the ecological characteristics of urban areas (Gaston, 2010). Some examples of land cover types are farmland, woodland, recreational areas, airports or schools (Gill et al., 2008). One particular type of the scholar type is the university campus, where students are not only trained in advanced studies but are also educated to increase their appreciation of local biodiversity (Zhang et al., 2014). In this sense, university campuses are part of the urban green space (Wang et al., 2021), that is associated to health and wellbeing (Adler and Tanner, 2013; Holt et al., 2019). As they are usually composed of a heterogeneous mosaic of habitats, they are also good targets for biodiversity conservation because they support breeding and foraging sites for different animals (Sattler et al., 2010; Threlfall et al., 2017). Despite this, there are few studies that compare different types of potentially valuable urban areas (e.g., urban parks vs restored prairies, see Lang et al., 2019) and especially, there is an important lack of studies about university campuses’ biodiversity worldwide, being most of the studies carried out in China and India, whereas the Mediterranean region presents very few studies on the topic and restricted to Turkish plants (Liu et al., 2021). Given that the Mediterranean hotspot is considered one of the most sensitive regions in the planet to the urbanization process (Seto et al., 2012; Carpio et al., 2017), it is very important to better understand the effect of urban areas in this region, and identify potential solutions or mitigation actions to minimize their impact.
In terms of biodiversity, insects are the most abundant animal taxon, with more than one million described species and constituting about 58–81.3% of the total depending of the author (see Foottit and Adler, 2009), but it is not the most studied group in urban ecology. According to the review by Rega-Brodsky et al. (2022), urban studies are mainly focused on plants (37.6%) and vertebrates (34.4%), while the high diversity of insects is underrepresented (23.7%). Specifically, the most studied insects are butterflies with 6.9% of urban papers, which is less than half the studies made on birds (18.6%). This and other studies strongly recommend overcoming this limitation of current urban ecology by performing more entomological research (Fenoglio et al., 2021). The importance of insects not only comes from their abundance, but also for playing a key role in the functioning of ecosystems as decomposers, parasites and parasitoids, pollinators, and prey for many other taxa (Bhullar and Majer, 2000), and they are not beyond the danger that urbanization presents to global biodiversity (Sánchez-Bayo and Wyckhuys, 2019). Therefore, understanding how the abundance and distribution of insects are affected in the urban landscape may help explain ecosystem functioning and the presence of other groups in cities. Some groups like birds should be directly affected by the presence of insects as they are crucial feeding resources for many bird species (Nyffeler et al., 2018). Furthermore, this information on urban insect communities could be crucial for the use of this group as bioindicator. Some insects can be used as rapid indicators of habitat quality (Carpintero and Reyes-López, 2014). For example, butterflies or beetles suffer sharp population declines with environmental changes because many species are seasonal and dependent of a particular habitat (Kunte, 1997; Ramírez-Restrepo and Halffter, 2016). This applies also to urban environments although not many studies have focused on this potential application of urban insects so far (e.g., Antony et al., 2016; Ramírez-Restrepo and Halffter, 2016; Paul and Sultana, 2020). Additional information on these groups (e.g., butterflies or beetles) in the urban context is a first needed step for their potential use as bioindicators helping in detecting and evaluating habitat quality and habitat changes in towns and cities.
Although we still need to considerably increase our knowledge on the effect of urbanization on insects and their distribution in the urban landscape (Paul and Sultana, 2020), some studies suggest mechanistic explanations for the intense reduction of insect diversity associated with urbanization. One of such mechanisms is the replacement of the native plant communities with non-native plants (Tallamy and Shriver, 2021), pointing that a higher percentage of native plants may increase butterfly, beetle, bee, and bug diversity (Tam and Bonebrake, 2016; Mata et al., 2017; Threlfall et al., 2017) in the urban environment. Also, management intensity could be an important factor affecting urban insects as there are several studies showing the positive effect of reducing the management intensity on insect diversity (Ade et al., 2012; Kricke et al., 2014; Wastian et al., 2016).
The present study aims to investigate the association between urban biodiversity and university campuses in the poorly known Mediterranean hotspot. We focused our study on diurnal Lepidoptera and ground-dwelling Coleoptera of the city of Granada (SE Spain). We chose these models as they are important taxonomic groups for biodiversity conservation, sensitive to the urbanization process (Ramírez-Restrepo and Halffter, 2016; Ramírez-Restrepo and MacGregor-Fors, 2017) as well as there are standardized and suitable sampling methods for each (see below). These two insect groups offer complementary information as they correspond with different life history traits (e.g., flying vs walking insects) and consequently could be differently affected by the urbanization process (e.g., Soga et al., 2014). Our methodological approach implied the comparison of different taxonomic diversity variables in several university campuses and nearby urban areas, thus standardizing for many environmental effects. Based on our previous argumentation (see above), we predict that university campuses will show higher species richness for both insect groups than other nearby urban areas from the same city. In addition, we explored the potential effect of several landscape variables (e.g., vegetation cover) or practices (e.g., management intensity) on insect diversity with the objective of providing more specific recommendations for city planners and managers willing to favor insects in Mediterranean cities.
Materials and methods
Field work and biodiversity measurements
The study was carried out in the city of Granada, located in SE Spain (37°11′ N, 3°36′ W) at about 700 m a.s.l. The city’s surface area is circa 22 km2 and its population is around 250,000 inhabitants. The climate is Mediterranean, which is characterized by a marked dry season in the warmer months (June to August). At a regional scale, the climate also presents strong daily and seasonal variations in temperature, and an annual rainfall of around 450 mm (Montávez et al., 2000). Sampling took place in three university campuses: Fuentenueva (FN), Cartuja (CA), and Parque Tecnológico de la Salud (PTS) and in three randomly selected urban areas separated by at least 1 km to secure the independence of sampling. Each campus was paired with a non-campus area (FN-U, CA-U, and PTS-U, respectively), so that they had the same perimeter (and thus total area). Six sampling points were selected within every study area, prioritizing different microhabitats potentially used by butterflies or ground-dwelling beetles and separated by a minimum distance of 100 m (Carpintero and Reyes-López, 2014).
Although Pollard transect is the most common standard method for butterfly surveys, there is evidence that purposive point counts can be more effective in small-scale landscape features (like public gardens and restored prairies in urban settings) because in these areas the vegetation resources are more limited and dispersed than in average natural areas (Lang et al., 2019). Furthermore, the line-transect method seems less efficient and difficult to implement in urban landscapes because of small, irregularly shaped, and widely spaced habitat patches (McFarland et al., 2017). Therefore, we conducted purposive point counts by documenting all butterflies within a circular area of 10 m radius over 15 min (Tam and Bonebrake, 2016; Ghosh and Saha, 2017). Survey data was registered in the eBMS (European Butterfly Monitoring Scheme)1 database. Each point count was sampled thrice, at the beginning (April), middle (May), and late spring season (June; Tzortzakaki et al., 2019; Paul and Sultana, 2020). According to Kõrösi et al. (2022) three samplings are enough to obtain sufficient data of the spring butterfly biodiversity. Surveys were performed in favorable weather conditions: no rain, soft wind (a maximum Beaufort scale of 4), and a minimum of 20°C (McFarland et al., 2017; Kõrösi et al., 2022) between 10:00 a.m. and 04:00 p.m. (Paul and Sultana, 2020). Butterflies were identified up to the species level during flight, feeding, basking, and mating activities. Butterflies were caught during the sampling using a sweeping net and kept in a plastic Petri dish, to avoid double-counting and to proceed with the correct identification. After the 15 min point count, individuals were released to minimize potential damage.
For ground-dwelling Coleoptera, we used pitfall traps, the standard protocol for sampling epigeal invertebrates with different daily activity rhythms (Woodcock, 2005; Carpintero and Reyes-López, 2014). Pitfall traps were placed six times in each sampling point (every 2 weeks during the spring season, from early April to late June) avoiding rainy days, so that they would not be filled with rainwater. Trap dimensions were 55 mm in diameter and 75 mm deep, and each one was half-filled with soapy water to break the superficial water tension (Carpintero and Reyes-López, 2014). The pitfall traps were visited after 48 h and the individuals caught were stored in alcohol for their identification in the laboratory up to the family level using dichotomous keys (e.g., Barrientos, 2004). Coleoptera were also classified into morphospecies, having into consideration external morphologic features such as body shape, color patterns or surface structures, which are sufficient to make a higher taxonomic degree approach (Derraik et al., 2002). As we are not expert taxonomists and there is an approx. 37% probability of failure in correct assignment to the morphospecies level (Derraik et al., 2002), we considered both taxon levels (family and morphospecies) for further analyses.
We calculated insect diversity using Hill diversity indices (Hill, 1973; Jost, 2007) based on Lepidoptera and Coleoptera data with the R package iNEXT (Hsieh et al., 2016). In the case of butterflies, we first obtained a single value for each sampled point by summing up the species identified for the three temporal replicates. Thus, this value provides species richness (q = 0) information from the whole sampling period (spring) for each of the 36 sampled points. In addition, we calculated the common species (q = 1) or Hill-Shannon diversity as it can respond strongly to rarity values that are both very high and to very low (Roswell et al., 2021). We followed a similar procedure for ground-dwelling beetles. In this case, we summed up morphospecies (or family) values for the five temporal replicates. This allowed us to obtain morphospecies (or family) richness for the whole sampling period for each sampled point. Following the same reasoning described above, we also calculated q = 1 for Coleoptera using either morphospecies or family data.
To investigate the effects of land cover on butterfly and beetle assemblages, land cover was measured in a 50 m circular area around the census point (Matteson and Langellotto, 2010; Delgado de la Flor et al., 2017), thus avoiding overlapping of circles from independent census points. We used the classification proposed by Cadenasso et al. (2007) which differentiates three basic elements of land cover: vegetation, impervious surfaces and buildings. Following this classification, we obtained proportional coverage data of each of the following land cover elements: woody vegetation (trees and shrubs), herbaceous vegetation (herbs and grasses), bare soil, pavement, and buildings. The typology of buildings was not indicated as the studied area was not big enough to differentiate this. The proportion of each land cover element was obtained from a manual selection with the ImageJ image processor2 from the most recent aerial photographs obtained from Google Earth Pro Version 7.3.3 Vegetation was also classified as native or exotic as there is evidence that some insects are more abundant in habitats dominated by native flora (Mata et al., 2017; Threlfall et al., 2017). Based on Cadenasso et al. (2007) we established five intervals to distinguish differences in low values of native plant proportion: (0) all vegetation is non-native, (1) less than 10% is native, (2) between 11--35% of the vegetation is native, (3) from 36 to 75% is native, and (4) more than 75% is native. The determination of the percentage of native vegetation was based on (i) the information provided by the gardening staff of the investigated areas, (ii) records for the sampled circles from two citizen science databases: GBIF4 and iNaturalist,5 and (iii) direct evaluation using plant identification guides (Cabezudo et al., 2009). In addition, we also considered the management intensity in every sampled circle, because in a landscape managed by humans, the type and intensity of this management can affect insect biodiversity (Wastian et al., 2016; Bonari et al., 2017). We contacted the personnel in charge of gardening in each sampled circle to assign it to one of five management level: (0) no maintenance, (1) basic cleaning maintenance, (2) annual weeding, (3) regular mowing, and (4) regular mowing and reseeding.
Finally, we also collected information of the distance to the outskirts of the city as some studies pointed this variable as an important determinant of insect diversity in the urban landscape (McIntyre, 2002). This was measured as the shortest Euclidean distance to the edge of the city (determined by the presence of cultivated land or peri-urban vegetation formations) using Google Earth Pro Version 7.3 (see text footnote 3).
Statistical analyses
We first checked for spatial autocorrelation by conducting Mantel tests to compare two matrices, one with the geographical distance and another with the Bray–Curtis dissimilarity between every Lepidoptera/Coleoptera assemblage in each sample (we tested families and morphospecies separately for beetles). These matrixes were compared using Spearman’s rank correlations. The significance of these tests was revised by permutation with 9,999 randomizations. The Mantel tests were performed using the R package “vegan” (Legendre and Legendre, 2012). The Lepidoptera species Bray–Curtis dissimilarity matrix had not a significant relationship with the geographic distance of the samples (Mantel statistic R = 0.01084, p-value = 0.4019), nor did the Coleoptera family Bray–Curtis dissimilarity matrix (Mantel statistic R = 0.03849, p-value = 0.2578) or the Coleoptera morphospecies Bray–Curtis dissimilarity matrix (Mantel statistic R = −0.06736, p-value = 0.8774). Therefore, there was no spatial autocorrelation, independently of their spatial separation and consequently no corrections were made in the following analyses.
Secondly, we estimated our sample coverage in order to know how complete the community of butterflies and ground-dwelling coleoptera was sampled in our study. By doing this, we can also estimate how comparable are our samples depending on the proportion of the diversity we could record (see Roswell et al., 2021). To do so we calculated the accumulation curves using the R package “iNEXT” (Hsieh et al., 2016).
Third, we explored taxonomic diversity with Hill numbers for Lepidoptera and Coleoptera, by fitting general linear mixed models (GLMMs) and obtained six models in total [i.e., two for butterflies species richness (q = 0)/common species (q = 1), two for ground-dwelling beetles family richness (q = 0)/common species (q = 1), and two more for ground-dwelling morphospecies richness (q = 0)/common species (q = 1)]. Each model included the following fixed predictors: the proportion of 50 m land cover elements (woody vegetation, herbaceous vegetation, bare soil, pavement, and buildings), the level of native flora in the sampled circle (0–4), management intensity (0–4), and distance to the outskirts (km). The type of urban area (Campus/No campus) was included as a fixed factor and as interaction with all additional fixed predictors mentioned above as we were also interested in identifying potential changes in the effect of predictors depending on whether the urban area is a university campus or not. Given that the sampling points were aggregated within specific study areas and not homogeneously distributed, we also incorporated the studied area ID as a random factor to our models. These models were performed using the R packages “lme4” (Bates et al., 2015) and “lmerTest” (Kuznetsova et al., 2017). We checked the normality with Shapiro–Wilk tests and the R package “stats” (Royston, 1995) whereas homocedasticity and multicollinearity were examined using the R package “car” (Fox et al., 2007).
After testing the independence of the variables, a backward selection process was used, so that the least significant terms were sequentially eliminated until only significant terms (P < 0.05) remained in the model (e.g., Ibáñez-Álamo et al., 2020). However, the type of urban area (Campus/No campus) was always kept in the models as it was our variable of interest. We also calculated the conditional (R2c = variance explained by the entire model) and marginal (R2m = variance explained by the fixed effects) coefficients with the R package “MuMIn” (Barton and Barton, 2015).
As there was a significant effect of the interaction between the type of urban area (Campus/No Campus) and the bare soil coverage ratio, we conducted a Mann–Whitney U test of bare soil coverage ratio means in both types of urban areas using the R package “stats” (Royston, 1995) to explore whether there are significant differences between campuses and non-campuses depending on this land cover type.
Finally, we performed an exploratory analysis by carrying out linear models (LMs) comparing each diversity variable calculated (same six variables described above) with the type of urban area (campus or non-campus), the studied area ID (Cartuja, Fuentenueva, and PTS), and the interaction between them, as fixed factors. We were particularly interested in the later interaction as it provides information on the potential differences in university campuses associations between paired areas (i.e., studied areas ID). Consequently, we conducted post hoc tests using a Tukey’s test in case the interaction was significant. Linear models and post hoc tests were performed with the R package “stats” (Royston, 1995).
Results
A total of 149 Lepidoptera and 492 Coleoptera specimens were captured in this study. We identified 15 different butterfly species (Supplementary Table 1), three of which were only found once, and none of them belonged to a threatened category according to the Red List of Spanish Threatened Invertebrates (Verdú et al., 2011). Among diurnal Lepidoptera, Pieris rapae was the most common species (48.3% of the individuals of Lepidoptera). Hesperiidae individuals were not found in this sampling. On the other hand, we identified 24 Coleoptera families, and among them, 105 morphospecies (Supplementary Table 2). In this sense, the most abundant and diverse family corresponded to Staphylinidae (34.6% of Coleoptera; 27 morphospecies).
The accumulation curves we obtained show a sample coverage above 75% for diurnal Lepidoptera, almost a 100% for Coleoptera families and above 70% for Coleoptera morphospecies (Supplementary Figure 1). In this sense, comparisons between Lepidoptera species and Coleoptera morphospecies would be quite balanced (Roswell et al., 2021).
In accordance with the model selection process (Table 1), we found a significant association between diurnal Lepidoptera species richness and the type of urban area (Figure 1). This effect seems to differ among paired studied areas as our simpler (exploratory) linear model showed a significant interaction between campus and studied area ID (Estimate ± SD = −2.00 ± 0.70, T = −2.87, p-value = 0.007; Supplementary Figure 2). However, we detected that the association between Lepidoptera species richness and the type of urban area (campus/non-campus) was mediated by the 50 m round bare soil coverage (Urban type × bare soil interaction; Table 1). The analyses on Lepidoptera common species (q = 1) showed a similar pattern with a significant general association regarding the type of urban area (Estimate ± SD = −0.72 ± 0.19, T = −3.72, p-value = 0.000716; Figure 1), but differing among paired areas (Supplementary Figure 2). This association is also affected by the bare soil coverage (Urban type × bare soil interaction; Table 1). For areas with low bare soil coverage, Granada university campuses are richer in Lepidoptera species and more diverse according to the common species than other urban areas, but these differences disappear as bare soil coverage increases (Figure 2). We found no significant difference between campus and non-campus areas in the bare soil coverage (W = 182, p-value = 0.54).
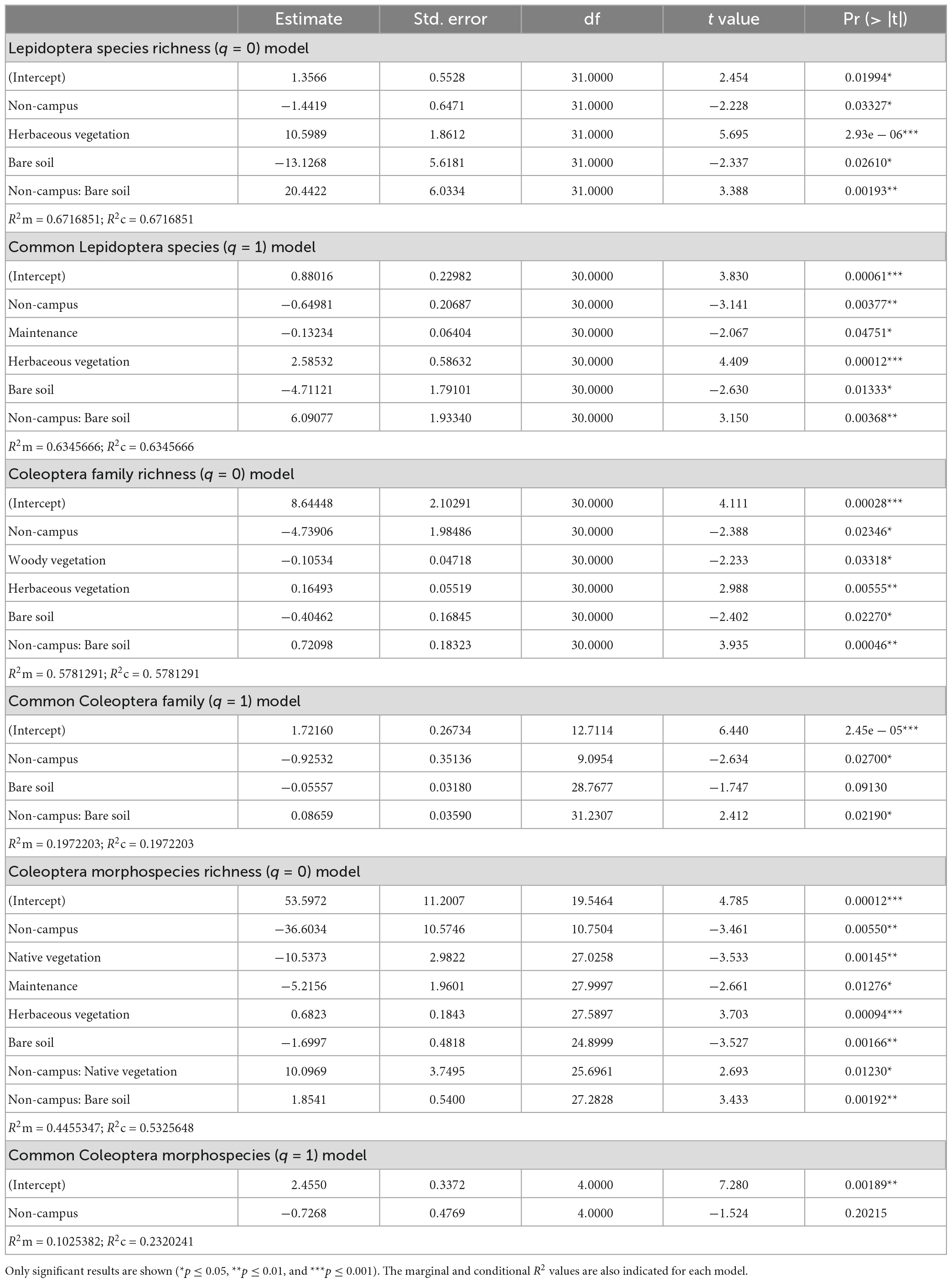
Table 1. Generalized linear mixed models (GLMMs) for species richness (q = 0) and the common species (q = 1) diversity index.
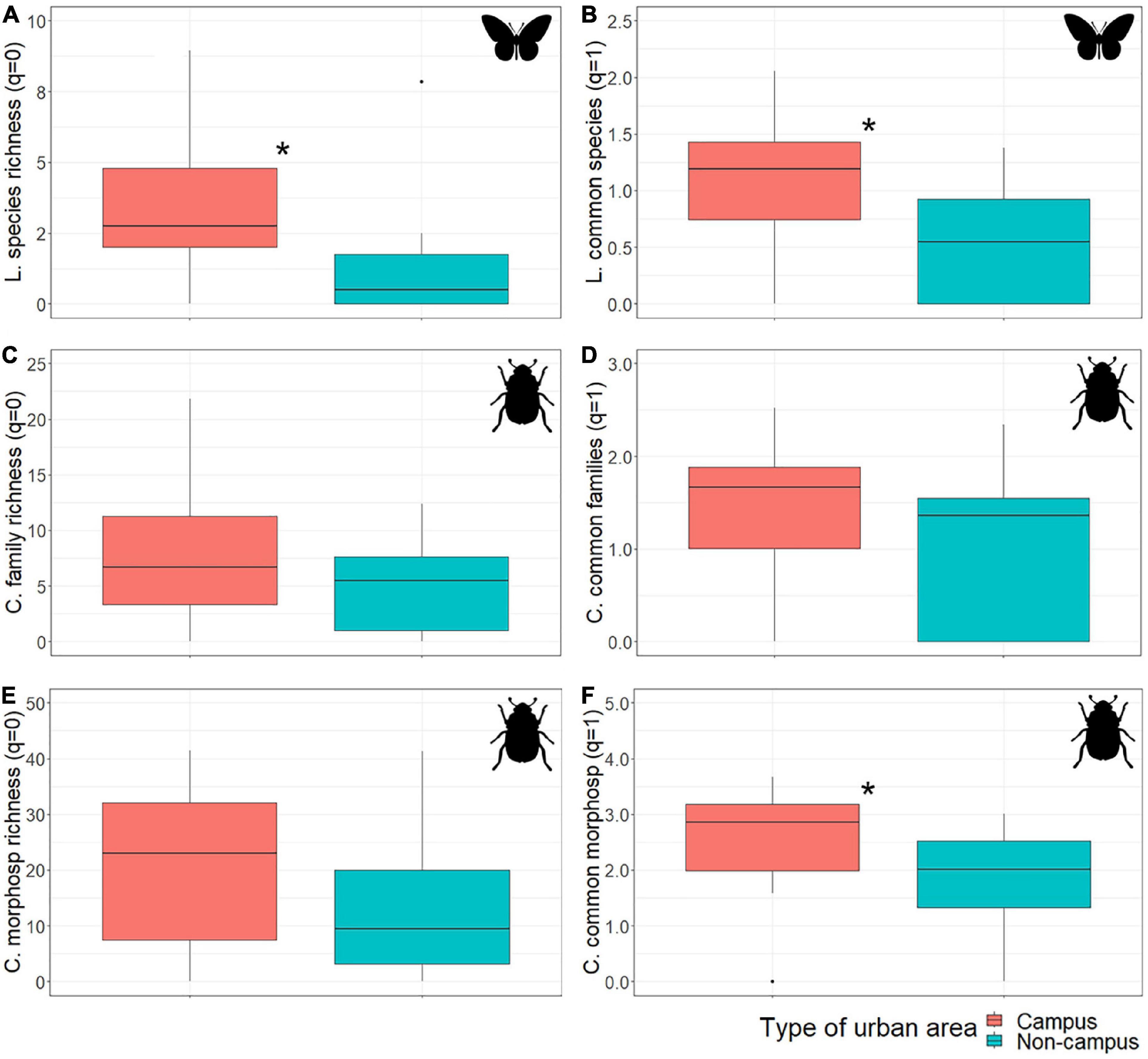
Figure 1. Taxonomic diversity comparison between campus/non-campus: (A) Lepidoptera species richness (q = 0), (B) Lepidoptera common species (q = 1), (C) Coleoptera family richness (q = 0), (D) Coleoptera common family (q = 1), (E) Coleoptera morphospecies richness (q = 0), and (F) Coleoptera common morphospecies (q = 1). The boxplots show the median (bar inside the rectangles), upper and lower quartiles and extreme values. Significantly different pairs are marked with an asterisk. Colored bands represent confidence intervals.
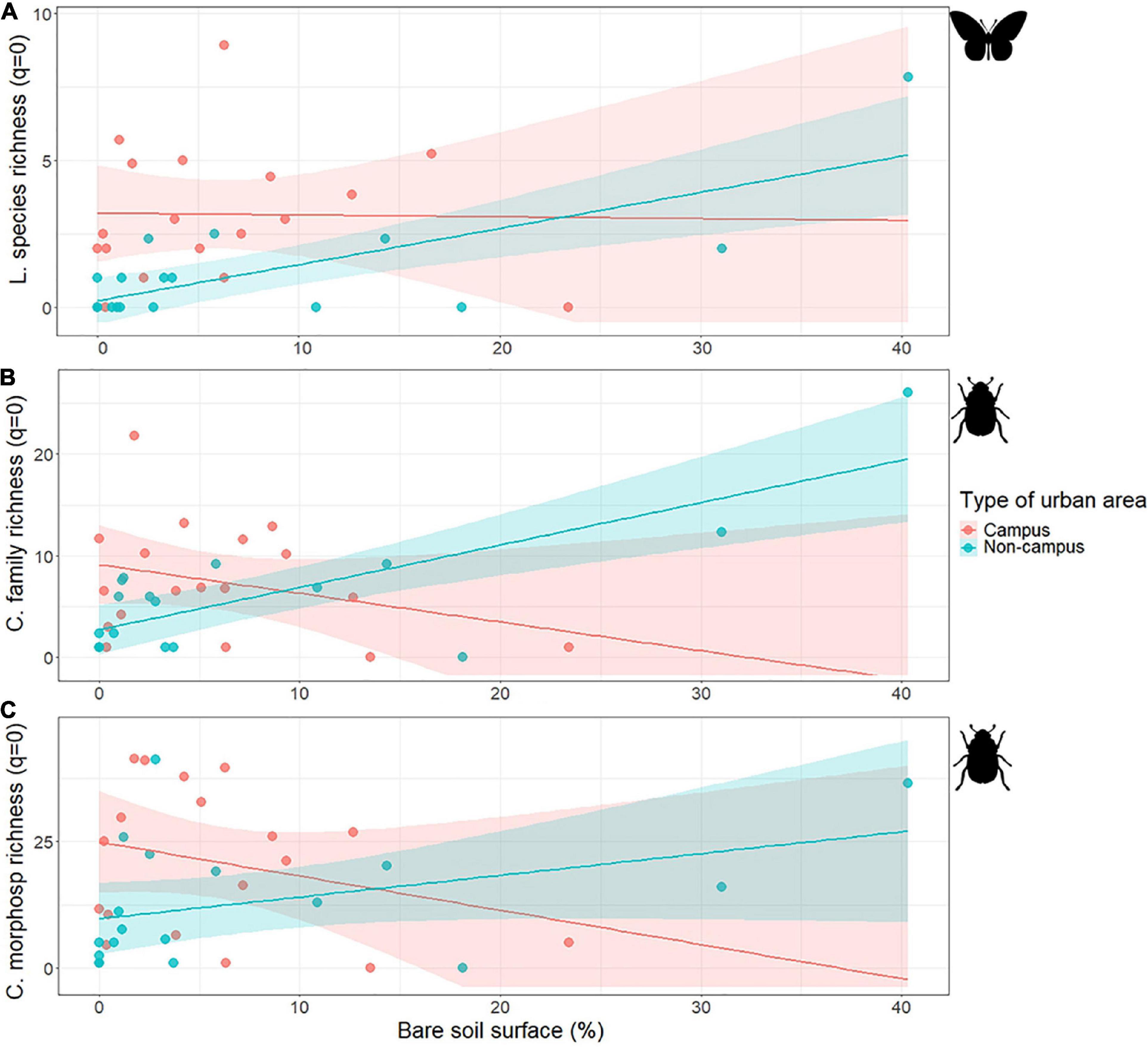
Figure 2. Association between the diurnal Lepidoptera species (A), the ground-dwelling Coleoptera family (B) and morphospecies (C) richness (q = 0) and the bare soil surface in combination with the type of urban area. Colored bands represent confidence intervals.
On the other hand, while ground-dwelling Coleoptera only showed a significant association with the type of urban area for common morphospecies (q = 1; Estimate ± SD = −0.73 ± 0.36, T = −2.04, p-value = 0.048; Figure 1), this association disappeared after analyzing paired areas (Supplementary Figure 2). In addition, we found a significant association with the type of urban area mediated by bare soil coverage (Urban type × bare soil interaction) and Coleoptera family richness, Coleoptera common families and Coleoptera morphospecies richness (see Table 1 and Figure 2).
Apart from the association with the type of urban area, the models showed other significant associations (Table 1). Diurnal Lepidoptera common species and Coleoptera morphospecies richness were negatively associated with the intensity of the management. In contrast, Coleoptera morphospecies richness was positively associated with the level of native vegetation, showing that areas with a larger proportion of native vegetation are more diverse than areas with exotic vegetation (Table 1). Furthermore, we also found a significant association between the type of urban area mediated by the native vegetation (Urban type × native vegetation interaction) and the Coleoptera morphospecies richness (see Table 1 and Figure 3). Also, diurnal Lepidoptera species richness and common species were significantly associated with the herbaceous coverage (Figure 4). Similarly, we found this significant positive association between herbaceous coverage and Coleoptera family richness as well as for morphospecies richness (Figure 4).
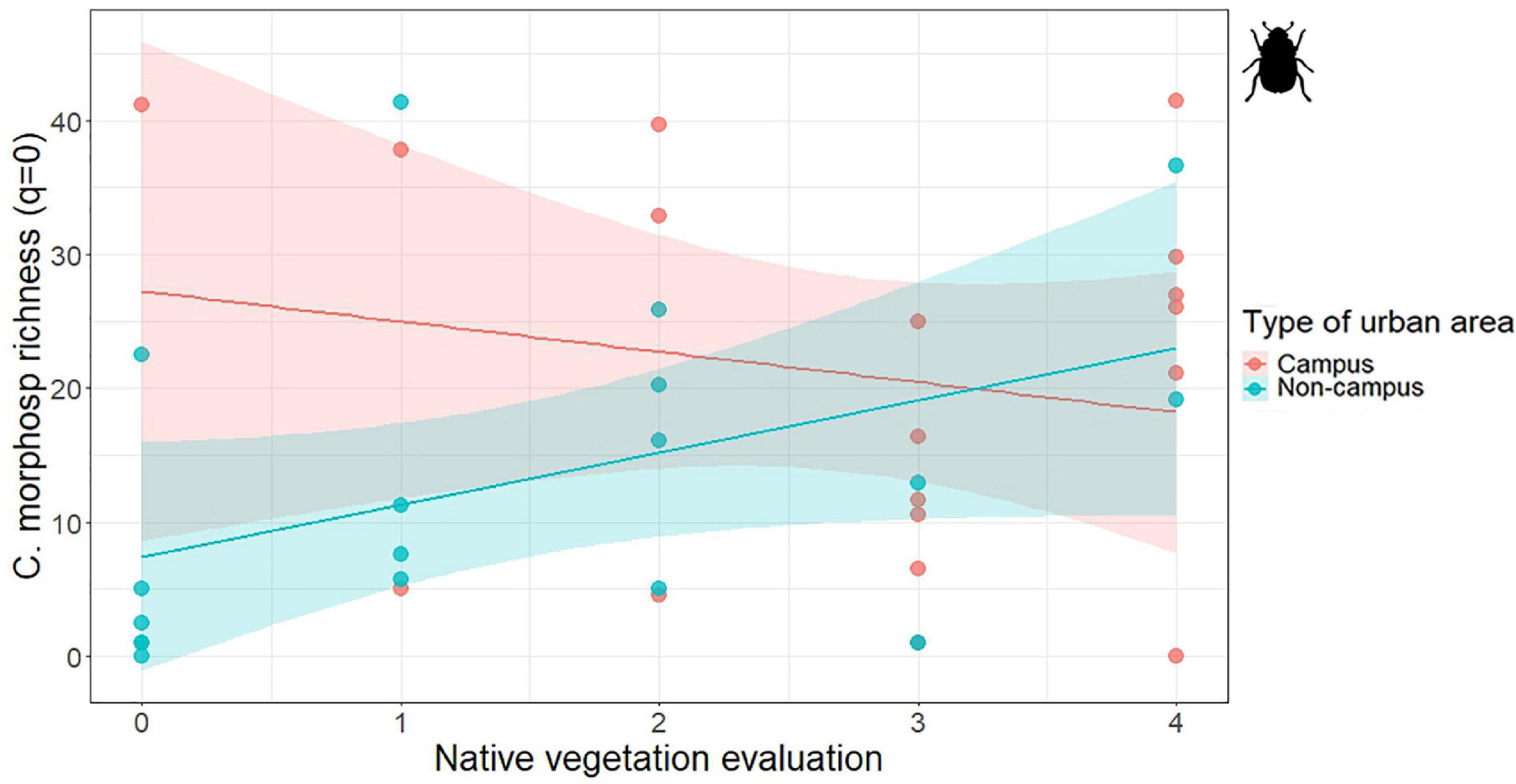
Figure 3. Association between the ground-dwelling Coleoptera morphospecies richness (q = 0) and the native vegetation in combination with the type of urban area. Colored bands represent confidence intervals.
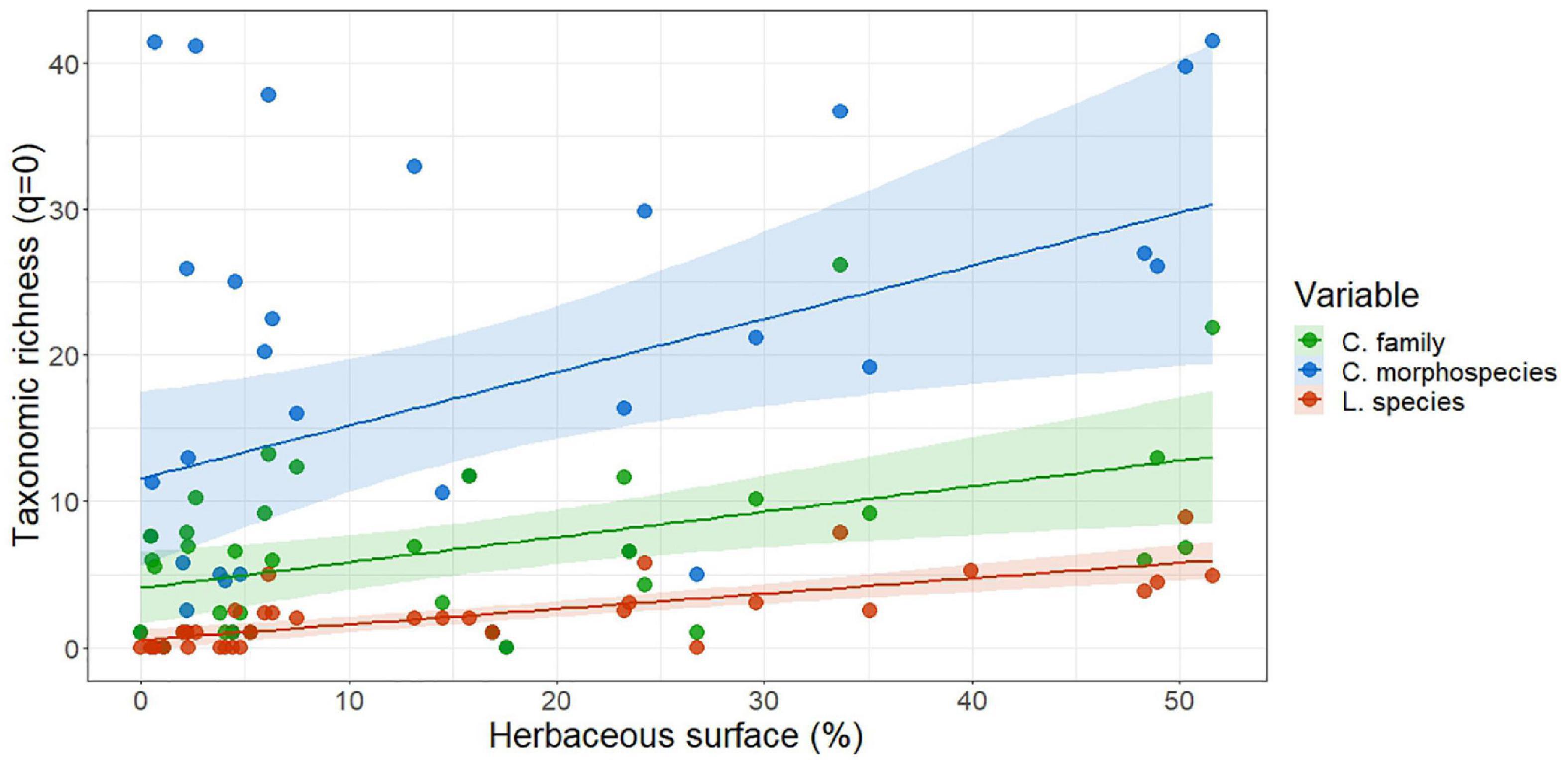
Figure 4. Association between the taxonomic (families and species)/morphological (morphospecies) richness (q = 0) of the two studied groups (ground-dwelling Coleoptera and diurnal Lepidoptera) and the herbaceous coverage. Colored bands represent confidence intervals.
The Lepidoptera models explained a good part of the variability found in the sampling (with a R2c higher than 0.63 in both models). But for Coleoptera, the models were less explanatory (with a R2c between 0.19 and 0.58). Coleoptera common species (q = 1) models had lower R2c values than the Coleoptera richness (q = 0) models, as they presented less significant variables after the model selection process (Table 1).
Discussion
To our knowledge, our study provides the first comparison of urban insect diversity between university campuses and non-campus urban areas in the Mediterranean region. Particularly, our investigation showed different results depending on the insect taxa analyzed (diurnal Lepidoptera or ground-dwelling Coleoptera). We found that university campuses yield higher diversity values for Lepidoptera but not for Coleoptera compared with other nearby urban areas. These differences seem to be mediated by bare soil cover for some of the diversity variables in both Lepidoptera and Coleoptera and thus, suggesting that these urban landscapes common in many Spanish cities could partially ameliorate the deep impact that urbanization can have on biodiversity (e.g., Deguines et al., 2016; Fenoglio et al., 2020). We also found a positive association of several diversity variables of butterflies and beetles with herbaceous coverage, a negative association of butterfly diversity with management intensity and a positive influence of native vegetation for beetle diversity. We discuss these findings in detail below although they already provide useful recommendations for urban managers and city planners to help them create more biodiversity-friendly cities in the Mediterranean area.
Lepidoptera diversity
Our data suggest that butterflies are favored in Granada university campuses, particularly in the PTS pair, which showed a significantly higher difference between the campus and the non-campus area for both, species richness (q = 0) and common species (q = 1). In order to understand this, we must revise the mixed models with the environmental variables. The models point that butterflies are favored in university campuses, but especially in those areas with low bare soil coverage. The proportion of bare soil cover is similar in both types of urban areas considered in this study (University campus and non-campus areas), which could suggest that the distribution, structure, and other characteristics of university campuses exert a corrective effect on the absence of bare soil, which would be, together with herbaceous vegetation, directly related to the existence and extension of open areas. Open areas are characterized by presenting higher rates of insolation and the possibility of flowering plants’ appearance, which some studies point as the major factors limiting pollinators diversity (Matteson and Langellotto, 2010). In fact, we did see a clear association between the herbaceous coverage and the increased presence of pollinators, specifically in Lepidoptera species richness and common species. For example, the highest value of species richness (five species) and common species (1.490) was found in an observation point within PTS campus. This area is dominated by a lawn garden with ornamental vegetation islands and some isolated trees (Celtis australis). This result is in accordance with previous studies that support the positive effect of early stages of vegetal succession (moistures) in butterfly abundance and species richness, while some species are disturbed directly by the increase of trees and shrubs (Öckinger et al., 2006). In addition, diurnal Lepidoptera seem to benefit from low maintenance activity, so that when the maintenance is more intense, butterfly diversity tends to decrease. This data is consistent with another study in a German urban landscape in which an intense maintenance area presented low values of both diurnal Lepidoptera species richness and common species (Kricke et al., 2014). In this sense, low maintenance areas would be more suitable habitats for butterflies, as they could find food sources and protection for every life stage, while in areas with continuous mowing events (values 3 and 4 in our study) these resources would be less available, and the individuals could be directly affected (Kricke et al., 2014; Tam and Bonebrake, 2016).
In the studied city (Granada), management intensity level 2 (annual weeding) must be carried out for fire prevention reasons according to the current legislation. However, one possible option to reduce management intensity would be to decrease mowing frequency, as suggested by Wastian et al. (2016). This action will favor entomophilous flowering plants, providing the necessary resource for nectar-feeding insects, like diurnal Lepidoptera (Kricke et al., 2014). In cases where management is necessary, Tam and Bonebrake (2016) propose that the plantation of native nectar plants should help butterflies adapt to the urban environment. Well-managed urban green areas can act as biodiversity islands, providing food and shelter for insects as well as ecosystem services to humans (Matthies et al., 2017). In this sense, native vegetation can hold a higher diversity of insects and better microhabitat conditions (Tallamy et al., 2010). However, diurnal Lepidoptera recorded do not seem to be benefited from higher values of native vegetation, this may be due to the fact that urban butterflies are more generalist than non-urban species for certain nutritious plants (Bergerot et al., 2011). Accordingly, there are records of non-native and invasive plants acting as prospective host plants for butterflies in urban sites (Mukherjee et al., 2015; Tallamy and Shriver, 2021). Furthermore, Matteson and Langellotto (2010) point out that rooftop gardens and terraces with southern exposures are good places to implement floral planting in order to benefit a greater diversity of pollinators. In Granada university campuses this could be implemented, improving the campus insect biodiversity and facilitating some field studies within, by using a place that nowadays is not used for any application. On the other hand, Granada urban area presents a high dominance of the species Pieris rapae (almost 50% of the observations), so it is not surprising that our results show lower butterfly common species (q = 1) values than other studies (Paul and Sultana, 2020). The huge dominance observed for P. rapae is consistent with some studies suggesting that this butterfly tends to increase in urban environments (Hardy and Dennis, 1999). In this sense, Pieridae was the most common family surveyed, followed by Nymphalidae (which showed the highest species richness), showing similar trends to other urban studies (e.g., Gandhi and Kumar, 2015; Prudic et al., 2018). The only diurnal Lepidoptera family that was not found in this study is Hesperiidae. This could be a real finding but could also be explained as a methodological bias because male individuals of this group rest in the vegetation and dart at passing objects in their search for females (Scott, 1973), and therefore they are not easily found in purposive point count sampling (Lang et al., 2019). For example, although Acharya and Vijayan (2015) found Hesperiidae individuals of six species using the point count method, they represented a lesser number of species sampled (3.7%).
Coleoptera diversity
For beetles, the university campuses of Granada are areas that seem to be favoring the diversity and richness of ground-dwelling beetles, but the effect is not visible in the paired areas as was for butterflies. Each of the beetle models showed a slightly different trend, hence the need to study this group at various taxonomic/morphological levels. Although we only detected a significant association between the Coleoptera common morphospecies and the type of urban area, there are no significant differences in the paired areas. However, we can observe an inverse tendency in FN and PTS compared with CA for Coleoptera family richness. Although we might think that CA campus could sustain higher values of Coleoptera diversity than the paired urban area, that is not happening, probably because of the importance of bare soil and herbaceous coverage, as it is explained as follows.
According to our results for ground-dwelling Coleoptera, university campuses could be mitigating the potential negative effect of the lack of bare soil in urban areas on the diversity of ground-dwelling beetle, just as it could be doing for butterflies. This may be due to the characteristics of the university campuses in Granada providing suitable sites for this group of beetles. As already shown in the meta-analysis of Méndez-Rojas et al. (2021), Staphylinidae (the most abundant Coleoptera family in our study) are not so negatively affected in the urban environment, because there are different microhabitats that they can use for shelter (fallen logs, stones, plant debris, etc.), which could be found in areas considered in the study as bare ground cover. In this sense, university campuses, although not having greater bare ground coverage, could be offering refuges that ground-dwelling Coleoptera use in bare soil areas. This association has been evidenced for all the Coleoptera models except for the common species. Based on both richness models (family and morphospecies), we can see that ground-dwelling beetles appear preferentially in areas with high herbaceous cover, just as butterflies do. And also similarly to butterfly species, Coleoptera morphospecies richness is positively associated with low values of maintenance intensity of vegetation. Since most of beetles are predators, scavengers, or detritivores, they may find the necessary resources, like plant debris, in this kind of habitat. These resources have been positively related to high values of diversity, as they act as an ideal habitat for the development of these insect larvae (Magura et al., 2013). In addition, humans themselves provide resources as domestic waste, which has been related to the increase of Staphylinidae diversity in urban areas (Yujie and Jindong, 2015). This type of waste is more unlikely to be removed from herbaceous cover areas (as opposed to paved areas that are cleaned assiduously). Furthermore, some studies indicate that there could be a selection of species from open spaces in fragmented habitats (Lange et al., 2014; Sánchez-Bayo and Wyckhuys, 2019), such as those found in urban areas, which can also be related to the positive associations found between Coleoptera richness variables and herbaceous cover.
The morphospecies richness model showed another association between beetles and vegetation: Coleoptera morphospecies are more diverse in areas with higher percentage of native vegetation. For example, the East area of the PTS campus showed the highest value of family (13 families) and morphospecies richness (18 morphospecies) as well as common family (2.072). This area is a wasteland dominated by native therophytes from the Asteraceae, Brassicaceae, and other families. The close positive relationship between Coleoptera diversity and the presence of native vegetation has been previously reported, resulting in similar findings (Mata et al., 2017; Threlfall et al., 2017; Correa et al., 2019), but as they are principally predators, they could not have a direct effect from the vegetation (Barratt et al., 2015). Furthermore, there is also another interesting interaction between native vegetation and the type of area: university campuses have higher Coleoptera morphospecies richness than other urban areas when the proportion of native vegetation is low. This result could reflect a buffer effect of university campuses maintaining beetle diversity despite hosting exotic vegetation. This is an example of an urban insect diversity predictor variable whose effect is still not very clear, and which needs further research in combination with other variables (Fenoglio et al., 2021).
To sum up, these results fit our initial hypothesis that Granada university campuses could sustain higher values of butterfly and beetle diversity. However, this effect varies depending on the urban variable analized and the taxonomical level considered. This reinforces the need for studies that not only contemplate multiple taxa, but also explain the individual effects of the different families of this diverse group of organisms. Our study opens a path for further insect studies, highlighting the value of university campuses as high diversity areas within the urban environment. In this sense, university campuses are not only important green spaces within the urban matrix, but also seem to have inner characteristics that favor the presence of insects, as has already been indicated for other taxa (Liu et al., 2021).
Data availability statement
The original contributions presented in this study are included in the article/Supplementary material, further inquiries can be directed to the corresponding author.
Ethics statement
Ethical review and approval were not required for the study on animals in accordance with the local legislation and institutional requirements.
Author contributions
JA: methodology, investigation, software, formal analysis, data curation, visualization, writing—original draft preparation, and writing—review and editing. JI-Á: conceptualization, methodology, formal analysis, writing—review and editing, and funding acquisition. OS: conceptualization, methodology, validation, resources, writing—original draft preparation, writing—review and editing, and funding acquisition. All authors contributed to the article and approved the submitted version.
Funding
This study was partially funded by a MSCA fellowship to OS (INSANE–101033024), a research initiation Santander Scholarship from the plan propio of Granada University to JA, and a FEDER/Junta de Andalucía-Consejería de Transformación Económica, Industria, Conocimiento y Universidades Project (A-RNM-618-UGR20) to JI-Á.
Acknowledgments
We are very grateful to F. S. Oi for his help in the field work and in Coleoptera identifications.
Conflict of interest
The authors declare that the research was conducted in the absence of any commercial or financial relationships that could be construed as a potential conflict of interest.
Publisher’s note
All claims expressed in this article are solely those of the authors and do not necessarily represent those of their affiliated organizations, or those of the publisher, the editors and the reviewers. Any product that may be evaluated in this article, or claim that may be made by its manufacturer, is not guaranteed or endorsed by the publisher.
Supplementary material
The Supplementary Material for this article can be found online at: https://www.frontiersin.org/articles/10.3389/fevo.2023.1130557/full#supplementary-material
Supplementary Figure 1 | Species accumulation curves of the sample coverage of the study for (A) diurnal Lepidoptera, (B) ground-dwelling Coleoptera families and (C) ground-dwelling Coleoptera morphospecies in university campuses and other urban areas. Colored bands represent confidence intervals.
Supplementary Figure 2 | Taxonomic diversity in each campus/non-campus pair: (A) Lepidoptera species richness (q = 0), (B) Lepidoptera common species (q = 1), (C) Coleoptera family richness (q = 0), (D) Coleoptera common family (q = 1), (E) Coleoptera morphospecies richness (q = 0), and (F) Coleoptera common morphospecies (q = 1). Significance was tested by Tukey’s Test (values sharing the same letter are not significantly different, 95% family-wise confidence level). The boxplots show the median (bar inside the rectangles), upper and lower quartiles and extreme values.
Footnotes
- ^ https://butterfly-monitoring.net/
- ^ https://imagej.nih.gov/ij/
- ^ www.google.es/earth/about/versions/#earth-pro
- ^ www.gbif.org/es/
- ^ www.inaturalist.org/
References
Acharya, B. K., and Vijayan, L. (2015). Butterfly diversity along the elevation gradient of eastern Himalaya, India. Ecol. Res. 30, 909–919.
Ade, J., Wolf-Schwenninger, K., and Betz, O. (2012). Auswirkungen der wiesenmahd auf verschiedene käferarten ausgewählter grünflächen im stadtgebiet tübingens. Jahresh. Ges. Naturkd. Württ. 168, 199–216.
Adler, F. R., and Tanner, C. J. (2013). Urban ecosystems: Ecological principles for the built environment. Cambridge: Cambridge University Press.
Antony, A. K., Prasad, G., and Kalesh, S. (2016). Diversity and abundance of butterflies of Kerala university campus, Kariavattom Thiruvananthapuram. J. Entomol. Zool. Stud. 4, 1074–1081.
Balk, D., Pozzi, F., Yetman, G., Deichmann, U., and Nelson, A. (2005). “The distribution of people and the dimension of place: Methodologies to improve the global estimation of urban extents,” in Proceedings of the international society for photogrammetry and remote sensing, urban remote sensing conference, Tempe, AZ.
Barratt, B. I. P., Dickinson, K. J. M., Freeman, C., Porter, S., Johnstone, P. D., Wing, J., et al. (2015). Biodiversity of coleoptera and other invertebrates in urban gardens: A case study in a New Zealand city. Insect Conserv. Divers. 8, 428–437. doi: 10.1111/icad.12120
Barrientos, J. A. (2004). Curso práctico de entomología. Barcelona: Universitat Autónoma de Barcelona.
Bates, D., Mächler, M., Bolker, B., and Walker, S. (2015). Fitting linear mixed-effects models using lme4. J. Stat. Softw. 67, 1–48. doi: 10.18637/jss.v067.i01
Bergerot, B., Fontaine, B., Julliard, R., and Baguette, M. (2011). Landscape variables impact the structure and composition of butterfly assemblages along an urbanization gradient. Lands. Ecol. 26, 83–94. doi: 10.1007/s10980-010-9537-3
Berkes, F., and Ross, H. (2013). Community resilience: Toward an integrated approach. Soc. Nat. Resour. 26, 5–20. doi: 10.1080/08941920.2012.736605
Bhullar, S., and Majer, J. (2000). Arthropods on street trees: A food resource for wildlife. Pac. Conserv. Biol. 6:171. doi: 10.1071/PC000171
Bonari, G., Fajmon, K., Malenovskı, I., Zelenı, D., Holuša, J., Jongepierová, I., et al. (2017). Management of semi-natural grasslands benefiting both plant and insect diversity: The importance of heterogeneity and tradition. Agric. Ecosyst. Environ. 246, 243–252. doi: 10.1016/j.agee.2017.06.010
Breuste, J., Niemelä, J., and Snep, R. P. H. (2008). Applying landscape ecological principles in urban environments. Lands. Ecol. 23, 1139–1142. doi: 10.1007/s10980-008-9273-0
Cabezudo, B., Cueto, M., Salazar, C., and Morales-Torres, C. (2009). Flora vascular de andalucía oriental. Sevilla: Consejería de Medio Ambiente, Junta de Andalucía.
Cadenasso, M. L., Pickett, S. T. A., and Schwarz, K. (2007). Spatial heterogeneity in urban ecosystems: Reconceptualizing land cover and a framework for classification. Front. Ecol. Environ. 5, 80–88. doi: 10.1890/1540-929520075[80:SHIUER]2.0.CO;2
Carpintero, S., and Reyes-López, J. (2014). Effect of park age, size, shape and isolation on ant assemblages in two cities of Southern Spain. Entomol. Sci. 17, 41–51. doi: 10.1111/ens.12027
Carpio, A. J., Barasona, J. A., Guerrero-Casado, J., Oteros, J., Tortosa, F. S., and Acevedo, P. (2017). An assessment of conflict areas between alien and native species richness of terrestrial vertebrates on a macro-ecological scale in a Mediterranean hotspot. Anim. Conserv. 20, 433–443. doi: 10.1111/acv.12330
Clarke, K. M., Fisher, B. L., and LeBuhn, G. (2008). The influence of urban park characteristics on ant (hymenoptera, formicidae) communities. Urban Ecosyst. 11, 317–334. doi: 10.1007/s11252-008-0065-8
Correa, C. M. A., Puker, A., Lara, M. A., Rosa, C. S., and Korasaki, V. (2019). Importance of urban parks in conserving biodiversity of flower chafer beetles (Coleoptera: Scarabaeoidea: Cetoniinae) in Brazilian Cerrado. Environ. Entomol. 48, 97–104. doi: 10.1093/ee/nvy176
Cuttelod, A., García, N., Malak, D. A., Temple, H. J., and Katariya, V. (2009). “The mediterranean: A biodiversity hotspot under threat,” in Wildlife in a changing world–an analysis of the 2008 IUCN red list of threatened species, eds J. Vié, C. Hilton-Taylor, and S. Stuart (Gland: IUCN).
Deguines, N., Julliard, R., de Flores, M., and Fontaine, C. (2016). Functional homogenization of flower visitor communities with urbanization. Ecol. Evol. 6, 1967–1976. doi: 10.1002/ece3.2009
Delgado de la Flor, Y. A., Burkman, C. E., Eldredge, T. K., and Gardiner, M. M. (2017). Patch and landscape-scale variables influence the taxonomic and functional composition of beetles in urban greenspaces. Ecosphere 8:e02007. doi: 10.1002/ecs2.2007
Derraik, J. G. B., Closs, G. P., Dickinson, K. J. M., Sirvid, P., Barratt, B. I. P., and Patrick, B. H. (2002). Arthropod morphospecies versus taxonomic species: A case study with araneae, coleoptera, and lepidoptera. Conserv. Biol. 16, 1015–1023. doi: 10.1046/j.1523-1739.2002.00358.x
Elmqvist, T., Zipperer, W. C., and Güneralp, B. (2015). “Urbanization, habitat loss and biodiversity decline: Solution pathways to break the cycle,” in The Routledge handbook of urbanization and global environmental change, eds K. Seto, W. Solecki, and C. Griffith (London: Routledge).
Fenoglio, M. S., Calviño, A., González, E., Salvo, A., and Videla, M. (2021). Urbanisation drivers and underlying mechanisms of terrestrial insect diversity loss in cities. Ecol. Entomol. 46, 757–771. doi: 10.1111/een.13041
Fenoglio, M. S., Rossetti, M. R., and Videla, M. (2020). Negative effects of urbanization on terrestrial arthropod communities: A meta-analysis. Glob. Ecol. Biogeogr. 29, 1412–1429. doi: 10.1111/geb.13107
Foottit, R. G., and Adler, P. H. (2009). Insect biodiversity: Science and society. Hoboken, NJ: John Wiley & Sons.
Fox, J., Friendly, G. G., Graves, S., Heiberger, R., Monette, G., and Nilsson, H. (2007). The car package. Vienna: R foundation for statistical computing.
Gandhi, S., and Kumar, D. (2015). Studies on butterfly diversity, abundance and utilization of plant resources in urban localities of Banyan city-Vadodara, Gujarat, India. J. Entomol. Zool. Stud. 3, 476–480.
Garrard, G. E., Williams, N. S. G., Mata, L., Thomas, J., and Bekessy, S. A. (2018). Biodiversity sensitive urban design. Conserv. Lett. 11:e12411. doi: 10.1111/conl.12411
Ghosh, S., and Saha, S. (2017). Ecobiological interaction between the pierides and their floral associates: An estimate of native environmental hygiene. J. Entomol. Zool. Stud. 5, 567–574.
Gill, S. E., Handley, J. F., Ennos, A. R., Pauleit, S., Theuray, N., and Lindley, S. J. (2008). Characterising the urban environment of UK cities and towns: A template for landscape planning. Lands. Urban Plan. 87, 210–222. doi: 10.1016/j.landurbplan.2008.06.008
Grimm, N. B., Faeth, S. H., Golubiewski, N. E., Redman, C. L., Wu, J., Bai, X., et al. (2008). Global change and the ecology of cities. Science 319, 756–760. doi: 10.1126/science.1150195
Hardy, P. B., and Dennis, R. L. H. (1999). The impact of urban development on butterflies within a city region. Biodivers. Conserv. 8, 1261–1279. doi: 10.1023/A:1008984905413
Hill, M. O. (1973). Diversity and evenness: A unifying notation and its consequences. Ecology 54, 427–432. doi: 10.2307/1934352
Holt, E., Lombard, Q., Best, N., Smiley-Smith, S., and Quinn, J. (2019). Active and passive use of green space, health, and well-being amongst university students. Int. J. Environ. Res. Public Health 16:424. doi: 10.3390/ijerph16030424
Hsieh, T. C., Ma, K. H., and Chao, A. (2016). iNEXT: An R package for rarefaction and extrapolation of species diversity (hill numbers). Methods Ecol. Evol. 7, 1451–1456. doi: 10.1111/2041-210X.12613
Ibáñez-Álamo, J. D., Morelli, F., Benedetti, Y., Rubio, E., Jokimäki, J., Pérez-Contreras, T., et al. (2020). Biodiversity within the city: Effects of land sharing and land sparing urban development on avian diversity. Sci. Total Environ. 707:135477. doi: 10.1016/j.scitotenv.2019.135477
Ikin, K., Le Roux, D. S., Rayner, L., Villaseñor, N. R., Eyles, K., Gibbons, P., et al. (2015). Key lessons for achieving biodiversity-sensitive cities and towns. Ecol. Manag. Restor. 16, 206–214. doi: 10.1111/emr.12180
Ives, C. D., Lentini, P. E., Threlfall, C. G., Ikin, K., Shanahan, D. F., Garrard, G. E., et al. (2016). Cities are hotspots for threatened species. Glob. Ecol. Biogeogr. 25, 117–126. doi: 10.1111/geb.12404
Jost, L. (2007). Partitioning diversity into independent alpha and beta components. Ecology 88, 2427–2439. doi: 10.1890/06-1736.1
Kõrösi, Á, Dolek, M., Nunner, A., Lang, A., and Theves, F. (2022). Pace of life and mobility as key factors to survive in farmland—relationships between functional traits of diurnal lepidoptera and landscape structure. Agric. Ecosyst. Environ. 334:107978. doi: 10.1016/j.agee.2022.107978
Kricke, C., Bahmann, T., and Betz, O. (2014). Einfluss städtischer mahdkonzepte auf die artenvielfalt der tagfalter. Naturschutz Landschaftsplan. 46, 52–58.
Kunte, K. J. (1997). Seasonal patterns in butterfly abundance and species diversity in four tropical habitats in northern Western Ghats. J. Biosci. 22, 593–603. doi: 10.1007/BF02703397
Kuznetsova, A., Brockhoff, P. B., and Christensen, R. H. B. (2017). lmerTest package: Tests in linear mixed effects models. J. Stat. Softw. 82, 1–26. doi: 10.18637/jss.v082.i13
Lang, B. J., Dixon, P. M., Klaver, R. W., Thompson, J. R., and Widrlechner, M. P. (2019). Characterizing urban butterfly populations: The case for purposive point-count surveys. Urban Ecosyst. 22, 1083–1096. doi: 10.1007/s11252-019-00880-8
Lange, M., Türke, M., Pašaliæ, E., Boch, S., Hessenmöller, D., Müller, J., et al. (2014). Effects of forest management on ground-dwelling beetles (coleoptera; carabidae, staphylinidae) in central Europe are mainly mediated by changes in forest structure. For. Ecol. Manage. 329, 166–176. doi: 10.1016/j.foreco.2014.06.012
Liu, J., Zhao, Y., Si, X., Feng, G., Slik, F., and Zhang, J. (2021). University campuses as valuable resources for urban biodiversity research and conservation. Urban For. Urban Green. 64:127255. doi: 10.1016/j.ufug.2021.127255
Magura, T., Nagy, D., and Tóthmérész, B. (2013). Rove beetles respond heterogeneously to urbanization. J. Insect Conserv. 17, 715–724. doi: 10.1007/s10841-013-9555-y
Mata, L., Threlfall, C. G., Williams, N. S. G., Hahs, A. K., Malipatil, M., Stork, N. E., et al. (2017). Conserving herbivorous and predatory insects in urban green spaces. Sci. Rep. 7:40970. doi: 10.1038/srep40970
Matteson, K. C., and Langellotto, G. A. (2010). Determinates of inner city butterfly and bee species richness. Urban Ecosyst. 13, 333–347. doi: 10.1007/s11252-010-0122-y
Matthies, S. A., Rüter, S., Schaarschmidt, F., and Prasse, R. (2017). Determinants of species richness within and across taxonomic groups in urban green spaces. Urban Ecosyst. 20, 897–909. doi: 10.1007/s11252-017-0642-9
McDonald, R. I., Marcotullio, P. J., and Güneral, B. (2013). “Urbanization and global trends in biodiversity and ecosystem services,” in Urbanization, biodiversity and ecosystem services: Challenges and opportunities, eds T. Elmqvist, W. Zipperer, and B. Güneralp (Cham: Springer), 31–52.
McFarland, K. P., Lloyd, J. D., and Hardy, S. P. (2017). Density and habitat relationships of the endemic white mountain fritillary (Boloria chariclea montinus) (lepidoptera: Nymphalidae). Insects 8:57. doi: 10.3390/insects8020057
McIntyre, N. E. (2002). Ecology of urban arthropods: A review and a call to action. Ecol. Popul. Biol. 93, 825–835. doi: 10.1603/0013
McKinney, M. L. (2006). Urbanization as a major cause of biotic homogenization. Biol. Conserv. 127, 247–260. doi: 10.1016/j.biocon.2005.09.005
McKinney, M. L., and Lockwood, J. L. (1999). Biotic homogenization: A few winners replacing many losers in the next mass extinction. Trends Ecol. Evo. 14, 450–453. doi: 10.1016/S0169-5347(99)01679-1
Méndez-Rojas, D. M., Cultid-Medina, C., and Escobar, F. (2021). Influence of land use change on rove beetle diversity: A systematic review and global meta-analysis of a mega-diverse insect group. Ecol. Indic. 122:107239. doi: 10.1016/j.ecolind.2020.107239
Montávez, J. P., Rodríguez, A., and Jiménez, J. I. (2000). A study of the urban heat island of Granada. J. R. Meteorol. Soc. 20, 899–911.
Mukherjee, S., Banerjee, S., Basu, P., Saha, G., and Aditya, G. (2015). Lantana camara and butterfly abundance in an urban landscape: Benefits for conservation or species invasion? Ekológia (Bratislava) 34, 309–328. doi: 10.1515/eko-2015-0029
Müller, N., Werner, P., and Kelcey, J. G. (2010). Urban biodiversity and design. Oxford: Wiley-Blackwell.
Nielsen, A. B., van den Bosch, M., Maruthaveeran, S., and van den Bosch, C. K. (2014). Species richness in urban parks and its drivers: A review of empirical evidence. Urban Ecosyst. 17, 305–327. doi: 10.1007/s11252-013-0316-1
Nyffeler, M., Şekercioğlu, Ç. H., and Whelan, C. J. (2018). Insectivorous birds consume an estimated 400–500 million tons of prey annually. Sci. Nat. 105:47. doi: 10.1007/s00114-018-1571-z
Öckinger, E., Eriksson, A. K., and Smith, H. G. (2006). Effects of grassland abandonment, restoration and management on butterflies and vascular plants. Biol. Conserv. 133, 291–300. doi: 10.1016/j.biocon.2006.06.009
Paul, M., and Sultana, A. (2020). Studies on butterfly (insecta: Lepidoptera) diversity across different urban landscapes of Delhi. India. Curr. Sci. 118:819. doi: 10.18520/cs/v118/i5/819-827
Pauleit, S., and Duhme, F. (2000). Assessing the environmental performance of land cover types for urban planning. Lands. Urban Plan. 52, 1–20. doi: 10.1016/S0169-2046(00)00109-2
Peng, M.-H., Hung, Y.-C., Liu, K.-L., and Neoh, K.-B. (2020). Landscape configuration and habitat complexity shape arthropod assemblage in urban parks. Sci. Rep. 10:16043. doi: 10.1038/s41598-020-73121-0
Prudic, K., Oliver, J., Brown, B., and Long, E. (2018). Comparisons of citizen science data-gathering approaches to evaluate urban butterfly diversity. Insects 9:186. doi: 10.3390/insects9040186
Ramírez-Restrepo, L., and Halffter, G. (2016). Copro-necrophagous beetles (coleoptera: Scarabaeinae) in urban areas: A global review. Urban Ecosyst. 19, 1179–1195. doi: 10.1007/s11252-016-0536-2
Ramírez-Restrepo, L., and MacGregor-Fors, I. (2017). Butterflies in the city: A review of urban diurnal Lepidoptera. Urban Ecosyst. 20, 171–182. doi: 10.1007/s11252-016-0579-4
Rega-Brodsky, C. C., Aronson, M. F. J., Piana, M. R., Carpenter, E.-S., Hahs, A. K., Herrera-Montes, A., et al. (2022). Urban biodiversity: State of the science and future directions. Urban Ecosyst. 25, 1083–1096. doi: 10.1007/s11252-022-01207-w
Ren, Q., He, C., Huang, Q., Shi, P., Zhang, D., and Güneralp, B. (2022). Impacts of urban expansion on natural habitats in global drylands. Nat. Sustain. 2022, 1–10. doi: 10.1038/s41893-022-00930-8
Roswell, M., Dushoff, J., and Winfree, R. (2021). A conceptual guide to measuring species diversity. Oikos 130, 321–338. doi: 10.1111/oik.07202
Royston, P. (1995). Remark AS R94: A remark on algorithm AS 181: The W-test for normality. Appl. Stat. 44:547. doi: 10.2307/2986146
Sánchez-Bayo, F., and Wyckhuys, K. A. G. (2019). Worldwide decline of the entomofauna: A review of its drivers. Biol. Conserv. 232, 8–27. doi: 10.1016/j.biocon.2019.01.020
Sattler, T., Duelli, P., Obrist, M. K., Arlettaz, R., and Moretti, M. (2010). Response of arthropod species richness and functional groups to urban habitat structure and management. Lands. Ecol. 25, 941–954. doi: 10.1007/s10980-010-9473-2
Scott, J. A. (1973). Convergence of population biology and adult behaviour in two sympatric butterflies, Neominois ridingsii (papilionoidea: Nymphalidae) and Amblyscirtes simius (hesperioidea: Hesperiidae). J. Anim. Ecol. 42, 663–372.
Seto, K. C., Güneralp, B., and Hutyra, L. R. (2012). Global forecasts of urban expansion to 2030 and direct impacts on biodiversity and carbon pools. Proc. Natl. Acad. Sci. U.S.A. 109, 16083–16088. doi: 10.1073/pnas.1211658109
Soanes, K., and Lentini, P. E. (2019). When cities are the last chance for saving species. Front. Ecol. Environ. 17, 225–231. doi: 10.1002/fee.2032
Soga, M., Yamaura, Y., Koike, S., and Gaston, K. J. (2014). Land sharing vs. Land sparing: Does the compact city reconcile urban development and biodiversity conservation? J. Appl. Ecol. 51, 1378–1386. doi: 10.1111/1365-2664.12280
Tallamy, D. W., and Shriver, W. G. (2021). Are declines in insects and insectivorous birds related? Ornithol. Appl. 123:duaa059. doi: 10.1093/ornithapp/duaa059
Tallamy, D. W., Ballard, M., and D’Amico, V. (2010). Can alien plants support generalist insect herbivores? Biol. Invasions 12, 2285–2292. doi: 10.1007/s10530-009-9639-5
Tam, K. C., and Bonebrake, T. C. (2016). Butterfly diversity, habitat and vegetation usage in Hong Kong urban parks. Urban Ecosyst. 19, 721–733. doi: 10.1007/s11252-015-0484-2
Threlfall, C. G., Mata, L., Mackie, J. A., Hahs, A. K., Stork, N. E., Williams, N. S. G., et al. (2017). Increasing biodiversity in urban green spaces through simple vegetation interventions. J. Appl. Ecol. 54, 1874–1883. doi: 10.1111/1365-2664.12876
Threlfall, C. G., Ossola, A., Hahs, A. K., Williams, N. S. G., Wilson, L., and Livesley, S. J. (2016). Variation in vegetation structure and composition across urban green space types. Front. Ecol. Evol. 4:66. doi: 10.3389/fevo.2016.00066
Tzortzakaki, O., Kati, V., Panitsa, M., Tzanatos, E., and Giokas, S. (2019). Butterfly diversity along the urbanization gradient in a densely-built Mediterranean city: Land cover is more decisive than resources in structuring communities. Lands. Urban Plan. 183, 79–87. doi: 10.1016/j.landurbplan.2018.11.007
United Nations (2015). Transforming our world: The 2030 agenda for sustainable development (A/RES/70/1). New York, NY: United Nations.
United Nations, Department of Economic and Social Affairs, Population Division (2019). World urbanization prospects: The 2018 revision. New York, NY: United Nations, Department of Economic and Social Affairs, Population Division.
United Nations, Department of Economic and Social Affairs, Population Division (2022). World population prospects 2022: Summary of results. New York, NY: United Nations, Department of Economic and Social Affairs, Population Division.
Verdú, J. R., Numa, C., and Galante, E. (Eds) (2011). “Atlas y libro rojo de los invertebrados amenazados de españa (especies vulnerables),” in Dirección general de medio natural y política forestal, (Madrid: Ministerio de Medio Ambiente, Medio Rural y Marino).
Vrezec, A., Bertoncelj, I., Kapla, A., and AmbrožIè Ergaver, Š. (2021). Urban population of the ground beetle Carabius variolosus nodulosus (coleoptera: Carabidae) in Ljubljana city (central Slovenia). Acta Entomol. Slov. 29, 133–147.
Wang, X., Svenning, J. C., Liu, J., Zhao, Z., Zhang, Z., Feng, G., et al. (2021). Regional effects of plant diversity and biotic homogenization in urban greenspace—the case of university campuses across China. Urban For. Urban Green. 62:127170. doi: 10.1016/j.ufug.2021.127170
Wastian, L., Unterweger, P. A., and Betz, O. (2016). Influence of the reduction of urban lawn mowing on wild bee diversity (hymenoptera, apoidea). J. Hymenopt. Res. 49, 51–63. doi: 10.3897/JHR.49.7929
Woodcock, B. A. (2005). “Pitfall trapping in ecological studies,” in Insect sampling in forest ecosystems, ed. S. R. Leather (Oxford: Blackwell publishing), 37–57.
Yujie, L., and Jindong, Z. (2015). Effect of human disturbance on species diversity of staphylidae. Plant Dis. Pests 6, 30–34.
Keywords: biodiversity, diurnal Lepidoptera, ground-dwelling Coleoptera, university campus, urbanization
Citation: Arjona JM, Ibáñez-Álamo JD and Sanllorente O (2023) Mediterranean university campuses enhance butterfly (Lepidoptera) and beetle (Coleoptera) diversity. Front. Ecol. Evol. 11:1130557. doi: 10.3389/fevo.2023.1130557
Received: 23 December 2022; Accepted: 20 February 2023;
Published: 06 March 2023.
Edited by:
Zoltan Elek, University of Szeged, HungaryReviewed by:
Paulo A. V. Borges, University of the Azores, PortugalJana Růžičková, ELKH-ELTE-MTM Integrative Ecology Research Group, Hungary
Copyright © 2023 Arjona, Ibáñez-Álamo and Sanllorente. This is an open-access article distributed under the terms of the Creative Commons Attribution License (CC BY). The use, distribution or reproduction in other forums is permitted, provided the original author(s) and the copyright owner(s) are credited and that the original publication in this journal is cited, in accordance with accepted academic practice. No use, distribution or reproduction is permitted which does not comply with these terms.
*Correspondence: Olivia Sanllorente, b2xpQHVnci5lcw==