- 1Jilin Provincial Key Laboratory of Animal Resource Conservation and Utilization, Northeast Normal University, Changchun, China
- 2Key Laboratory of Vegetation Ecology of Education Ministry, Institute of Grassland Science, Northeast Normal University, Changchun, China
- 3College of Life Science, Jilin Agricultural University, Changchun, China
- 4Jilin Songnen Grassland Ecosystem National Observation and Research Station, Northeast Normal University, Changchun, China
Predation is a powerful selection pressure that shapes predator–prey interactions. Due to long-term interactions, moths have developed hearing to detect the echolocation calls of bats. This allows bats to impose the non-consumptive effects of predation on moths. However, information on the changes in the growth, development, reproduction, and hormones of moths that are vulnerable to bat predation is limited. In this study, we used Spodoptera litura (Lepidoptera: Noctuidae) to determine the effects of the predation risks of bats on their growth, development, reproduction, and hormone titers. Our results showed that the larvae of S. litura increased food intake, accelerated the development of the larval stages, increased mortality and metamorphosis failure, and had a smaller body size under the risk of predation by bats. Additionally, the reproductive activity and fecundity decreased in the adults of S. litura, but the juvenile hormone titers and 20-Ecdydysone hormone titers increased. These results suggested an adaptive response in S. litura under bat predation risks at the cost of lower survival and reproduction. Finally, we found that S. litura moths showed different responses to different predation risk cues, which suggested that they might assess the magnitude of different predation risks to make the most suitable decision for survival and reproduction. Our results highlight the importance of the predation risk imposed by bats to negatively regulate the population dynamics of moths. Our findings indicated that the biological control of bugs using bats is a promising strategy.
Introduction
The risk of predation strongly influences predator–prey interactions (Carpenter et al., 2001; Frank, 2008) and leads to changes in population dynamics, community structure, and ecosystem function (Krebs et al., 1995; Zanette et al., 2011). Predator–prey interactions are generally quantified by comparing the changes in the abundance of prey populations due to the direct consumption effects (CEs) of predators (Sih et al., 1985; Sheriff et al., 2020). However, researchers have realized that predation risks can also exert strong non-consumptive effects (NCEs) on prey by changing prey traits (e.g., behavior, physiology, and morphology) and development through multiple signals (e.g., auditory, olfactory, and visual signals) (MacLeod et al., 2018; DeWitt et al., 2019) which in turn can negatively affect the adaptations and fitness of prey (Sheriff et al., 2020). Several studies have shown that NCEs associated with predator–prey interactions might be equally or even more important than CEs on the prey population and community dynamics (Peckarsky et al., 2008; Peacor et al., 2020). Several studies on NCEs have been conducted with insects (Bauman et al., 2019), fishes (Hughes et al., 2014; Mitchell and Harborne, 2020; Benti et al., 2021), amphibians (Zamzow et al., 2010), birds (Malone et al., 2017), and mammals (Abom and Schwarzkopf, 2016; Fauteux et al., 2018). For example, Melanoplus femurrubrum (Orthoptera: Acrididae) increases the mass-specific metabolic rate by 32%, with a concomitant increase in carbohydrate intake by 40% when they perceive the risk of predation by spiders (Hawlena and Schmitz, 2010). The presence of fish predators that cannot attack also might increase the failure rate of metamorphosis and larval mortality in Leucorrhinia intacta (Odonata: Libelulidae) (McCauley et al., 2011). Different ultrasound frequencies can negatively affect juvenile hormone titers in Monochamus alternatus (Coleoptera: Cerambycidae), which might further disrupt sexual maturation (Zha et al., 2021). However, these studies mainly focused on the changes in the traits of the prey (i.e., vigilance, body size, habitat selection), but studies on the effects of the changes in traits on fitness components or the abundance of the prey remains unclear (Peacor et al., 2022).
There are more than 1,400 species of bats, most of which occupy a unique nocturnal ecological niche (Kunz et al., 2011). Insectivorous bats with precise echolocation systems mainly hunt nocturnal insects (Wilson and Reeder, 2005). The interaction between bats and moths is a fascinating evolutionary “arms race” (Rubin et al., 2018). During coevolution, echolocating bats influenced the evolution of numerous characteristics of nocturnal insects, and many insects evolved ultrasound-sensitive ears, evasive behaviors, or other survival strategies in response to the selection pressure imposed by bats. Thus, even in the absence of predation, bats can still pose a strong predation risk to moths. However, the NCEs of the predation risk of bats on moths remain unclear.
The echolocation calls of bats vary among species and can be classified into three types: constant frequency (CF), frequency modulation (FM), and quasi-constant frequency (QCF) (Schnitzler et al., 2003). CF bats use CF acoustic signals for echolocation, and the duration of the acoustic signal is long, i.e., up to 30–60 ms per signal. FM bats use FM acoustic signals for echolocation, and the duration of the acoustic signal is short, about 0.5–5 ms per signal (Jones and Teeling, 2006). Exposure to the risk cues (e.g., ultrasound) of predators can have strong indirect effects on the physiology of the exposed moths and also the performance of the offspring, which indicates promising results for biological control strategies based on the ecology of fear (Cinel et al., 2020). For example, exposure of Spodoptera litura (Lepidoptera: Noctuidae) and S. exigua to pulsed ultrasound white noise elicited a flight-stopping response in moths with no or little auditory adaptations, which indicated that the moths tended to escape from the ultrasound (Nakano et al., 2022). In another study, ultrasonic treatment had strong negative effects on the biological parameters, such as longevity, body mass, and fecundity, of the immature life stages of Sesamia cretica (Lepidoptera: Noctuidae) (Agah-Manesh et al., 2021). These studies suggested that bat ultrasound might be used to effectively control agricultural pests. However, many studies have used synthetic ultrasound signals to recreate a stimulus similar to the calls of bats (Lalita and McNeil, 1998; Huang and Subramanyam, 2004). These synthetic ultrasounds often lack the biological attributes of real bat ultrasounds, and thus, they may not be suitable for assessing the magnitude of bat NCEs on prey. Additionally, prey can detect predators using visual, tactile, chemical, physical, and other cues (Hermann and Thaler, 2014). As most studies have only focused on the acoustic cue of bats, it remains unclear whether the multimodal cues related to the risk of predation can cause a greater degree of negative effects on the prey.
Spodoptera litura (Lepidoptera: Noctuidae) is a polyphagous and important agricultural pest found worldwide (Xiao et al., 2021). It has evolved the ultrasound-sensitive tympanal organ in response to predation by echolocating bats. Thus, the predation risk of bats, especially using ultrasound stimuli, might be used for controlling this pest. Previously, we discovered that the CF-FM bat Rhinolophus sinicus (Chiroptera: Rhinolophidae) and the FM bat Miniopterus fuliginosus (Chiroptera: Vespertilionidae) could prey on a large number of S. litura (unpublished data), and the two bats have different foraging habitats, physiological ecology, and ultrasonic parameters. Though it is not clear if the bats prefer to eat this species or whether the prey cannot effectively evade predation, it still provides a good opportunity to study the NCEs of the predation risk of bats on the changes in the traits of these moths and the consequences of these changes on their fitness.
For prey, there is usually a trade-off between predator avoidance and growth (survival and reproduction) (Thaler et al., 2012). An increase in the survival of prey is associated with a reduction in foraging activity and an increase in vigilance when exposed to high predation risk (Lalita and McNeil, 1998; MacLeod et al., 2018). Conversely, prey allocates more energy to reproduction and adaptive changes in behavior, physiology, and life history traits (Sheriff et al., 2020). In this study, we used R. sinicus and M. fuliginosus and their echolocation calls related to foraging as predation risk cues to investigate the effects of different predation risks imposed by bats on the growth, development, reproduction, and hormone levels of S. litura. We hypothesized that the predation risks of bats would negatively affect the growth, development, reproduction, and physiology of S. litura. We tested the following predictions: (1) bat predation risk might be associated with changes in the growth and development of S. litura, including food intake, body mass, survival rate, death rate, pupation rate, and eclosion rate; (2) bat predation risk might be associated with a decrease in reproductive behavior and fecundity in S. litura; (3) the levels of the JH and 20-E hormone of S. litura might change after exposure to the risk of predation by bats.
Materials and methods
Capture and housing of bats
We collected 10 individuals of R. sinicus from Jiumen Cave, Lengshuijiang City, Hunan Province, in May 2021. We also collected 10 individuals of M. fuliginosus from Feilong Cave, Jingnan Town, Xingyi City, Guizhou Province, in June 2021. We placed mist nets at the entrance of the caves in the early morning to capture the bats when they returned from foraging (Gong et al., 2022). Each individual was placed in a sterile cloth bag and brought back to the laboratory (5 m × 10 m × 3 m) for rearing at Northeast Normal University. In the laboratory, the temperature and relative humidity were set at 21–22°C and 40%, respectively, and a 12-h/12-h light/dark cycle (light conditions of 07:00–19:00 h) was maintained to mimic the natural environment inside caves (Zhang et al., 2022). All bats were provided ad libitum freshwater and larvae of Zophobas morio (Coleoptera: Tenebrionidae) enriched with vitamins and minerals. Bats were kept healthy during the acclimation or experimental periods. All bats were released in good health at the location where they were captured, after completion of the experiments.
Recording of echolocation calls during foraging
Before the experiments, we recorded the foraging echolocation calls from each bat to use as acoustic stimuli in our experiment. In total, 20 wild-caught S. litura were periodically released in an acoustics laboratory (3 m × 5 m × 3 m), and we also hung 10 S. litura larvae with a thin wire (0.7 mm in diameter) 1 m from the top of the room to ensure that preys were available for bats. Before the experiment, the bats were fed 5 Z. morio to reach a semi-starved state to motivate the bats to feed on the S. litura. During each experiment, we brought one bat into the laboratory to record echolocation calls, from search-phase calls to feeding buzzes. The interactions were captured using two infrared cameras (FDR-AX60; Sony Corp., Tokyo, Japan) placed opposite to each other and two single-channel ultrasound recording devices (Ultra-Sound Gate 116, Avisoft Bioacoustics, Berlin, Germany). The sampling frequency was 375 kHz with a 16-bit resolution (Sun et al., 2018). The experiments were performed when the foraging activity was the highest (Appel et al., 2021) (between 20:00 and 06:00 h), and the calls of each bat were recorded for 1 h. After the calls were recorded, the bats were returned to their large flight cage in the husbandry room and given food as a reward.
Synthesis and editing of playback files
Each playback file was created by randomly mixing the echolocation calls from one individual. The echolocation call sequences (including searching, approaching, and feeding buzz phases) with a good signal-to-noise ratio were selected for editing. These calls were randomly stitched together using the Avisoft-SASLab Pro 5.2 (Avisoft Bioacoustics, Berlin, Germany) software to construct playback files of 1 min. Each playback file consisted of 14 echolocation call sequences with random silent intervals of 5–8 s between sequences to mimic the natural intervals of echolocation calls. The playback files were normalized, with the peak amplitude of the weakest call set at about −30 dB. Each group of playback files was randomly arranged based on the 14 echolocation call sequences to generate 10 playback files. All playback files were high-pass filtered at 2 kHz to minimize the effect of background noise. We constructed a white noise (0 ~ 100 kHz) stimulus to determine whether the response of the moths to bat echolocation calls was similar to that of white noise. The individuals of S. litura in each treatment group were stimulated with a group of playback files every night during the experimental period.
Collection and maintenance of S. litura
In total, 1,000 eggs of S. litura were purchased from Baiyun Industrial Co., Ltd. in Henan Province and housed in polypropylene plastic rearing boxes (20 cm × 14 cm × 8 cm) in an artificial climate chamber (PRX-450C, NingboSaifu, Ningbo, China) at 27 ± 1°C, 65 ± 5% relative humidity, and a 14-h/10-h light/dark photoperiod. All the newly-hatched larvae in the experiment were reared together on an artificial diet (Guanghong et al., 1998). They were isolated individually into finger tubes to prevent the effect of density on their growth and development. After pupation in the test tubes, the pupae were transferred to polypropylene plastic boxes (20 cm × 14 cm × 8 cm) individually and moisturized by spraying water every day until they emerged as adults. The adults were placed in a cage (40 cm × 40 cm × 40 cm) made of 120-mesh gauze and with a 10% honey solution applied on a ball of cotton in a glass dish for mating and laying eggs. The eggs on the wall of the cage of S. litura were collected on disinfected plastic sheets and disinfected with sodium hypochlorite solution (340.0–460.0 μg/L effective chlorine content) for 30 min to prevent pathogen infection (Xiong et al., 2015). Then, the eggs were rinsed with water for 3 min, air dried, placed in a rearing box as mentioned above, and incubated in an artificial climate chamber. The individuals of S. litura were reared for a generation under predator-free conditions for subsequent experiments.
The playback experiment
All experiments were performed in artificial climate chambers under the same physical conditions. To assess the differences between two acoustic bat cues and the NCEs of white noise on the S. litura, and to determine whether bats have only acoustic cues that can cause effects on S. litura, six treatment groups were established, including the control group (Control), white noise playback group (Whitenoise), CF echolocation call playback group (CF-call), R. sinicus exposure group (CF-exp), FM echolocation call playback group (FM-call), and M. fuliginosus exposure group (FM-exp). The Whitenoise, CF-call, and FM-call groups were the echolocation call playback groups, and the CF-exp and FM-exp groups were bat the exposure groups. We provided chronic stimulation throughout the life history stages of S. litura to determine the sustained effects of chronic stimulation. For the echolocation call playback groups, the microphone, and the loudspeaker were connected to an ultrasound recording system and an ultrasound playback interface (UltraSoundGate player 116), respectively. The speaker was set on a tripod 50 cm above the individuals of S. litura. These speakers provide an acoustic cue to S. litura. For the bat exposure groups, each group consisted of five adult bats in a cage (50 cm × 50 cm × 50 cm) 50 cm away from the S. litura moths to create an environment where the moths were exposed to bat predators. These bats might provide acoustic, visual, and olfactory cues to S. litura. All stimulation treatments were provided from 10:00 p.m. to 8:00 a.m. and stopped for the rest of the day, whereas the larvae in the control group were placed in the same environment in the absence of stimuli.
Development and food intake of S. litura larvae under the risk of predation
Newly hatched larvae of S. litura were randomly assigned to one of the six above-mentioned treatment groups. We chose 300 newly hatched larvae from each group and divided them into six replicates of 50 larvae each. The larvae were reared individually in sterilized glass test tubes (20 mm × 150 mm) and fed an artificial diet. Each replicate had 50 test tubes in one test tube rack, counting a total of six test tube racks per group. The test tubes were sealed with sterilized gauze to ensure air permeability, and the tubes were placed in plastic containers (22 cm × 9 cm × 15 cm). The development progress of S. litura was recorded, specifically, the developmental stages, number of pupae, and number of emerging adults. After the third instar of the S. litura larvae, randomly 100 larvae in each treatment group were weighed daily using an electronic balance (BSA124S 120 g/0.1 mg, Sartorius Ltd., Germany) till they started to develop into pre-pupae. At this time point, larvae stopped feeding and made pupal chambers. The weight of the pupae in all treatment groups was recorded 3 days after pupation. The condition of the moths and wing abnormalities were recorded every day until all moths died. For each treatment type, we selected additional 100 third instar larvae and reared them individually in single tubes containing enough fresh artificial diet to satisfy their food intake when exposed to different stimuli. The remaining artificial feed was weighed each day and replaced with a sufficient amount of fresh artificial diet. The experiment was completed after all larvae entered the prepupal stage; the amount of food consumed was calculated for the larvae in each treatment group.
The reproductive behavior of S. litura under the risk of predation
The behavior of insects is complex and variable. We selected three main behaviors to determine the behavioral responses of moths to different types of treatments, which included movement, mating, and courtship (Svensson et al., 2003; Zhou et al., 2010). We selected moths of similar body condition that emerged on the same day from each of the treatment groups and placed them in plastic cups (10 cm × 10 cm × 10 cm) with 10% fresh honey solution applied on cotton balls to provide supplementary nutrients. Ten pairs of moths were assigned to each treatment group (Sandhyarani and Rani, 2013). The behaviors of the moths were recorded using an infrared camera (HDR-CX 760E; Sony Corp., Japan) every day within the duration in which the stimuli were provided (Wu et al., 2018). We recorded three nights total because the peak of S. litura movement, mating, and courtship occurred within 3 days of eclosion (Li et al., 2012). We used QvodPlayer (Version 5.0.80, ShenzhenQvod Technology Co., Ltd., Guangdong, China) to analyze the recorded video files of the moths in the six treatment groups and quantify their behavior. Based on the recorded data, the percentage of time spent performing each behavior was calculated. Following the methods described above, we selected another 10 pairs of moths to determine the effects of the different types of treatments on the oviposition of female moths (Wu et al., 2018). The eggs were collected and counted daily. From them, 100 eggs laid on the same day by females of the same age were randomly selected to calculate the hatching rate, and 10 replicates were used for each treatment (Wu et al., 2018).
The titers of JH and 20-E of S. litura under predation risk
To determine the effects of different types of treatments on the hormone levels of S. litura, we selected two hormones that are critical for insect growth and development and analyzed their changes. From the six treatment groups, the late fourth, late fifth, and late sixth instar larvae, as well as, 1-, 3-, and 5-day-old female and male moths, were selected to determine the titers of JH and 20-E. Furthermore, because the JH titer was generally low in pupae and the 20-E titer did not differ significantly (Plantevin et al., 1984), we chose 1-, 3-, and 5-day-old pupae without distinguishing between male and female to only determine the 20-E titers. Three biological replicates were set up for each treatment (Zha et al., 2021). The double-antibody sandwich enzyme-linked immunosorbent assay (ELISA) was performed to evaluate the levels of JH and 20-E. Insect JH and 20E ELISA kits (detection range of 6.25–200 pg./mL) (Shanghai Enzyme-Linked Biotechnology Co., Ltd., China) were used to determine JH and 20E levels, following the manufacturer’s instructions. Each analysis phase was performed separately three times and averaged.
Data analysis
The Kolmogorov–Smirnov test was conducted to test the normality of the data, and parametric tests were performed to analyze normally distributed data. Non-parametric tests were performed to analyze the data that were not normally distributed. The data on development duration, mortality, adult longevity, pupation rate, eclosion rate, larval food intake, maximum body mass of last instar larvae, 3-day-old pupal weight, total egg production, hatching rate, titers of JH, and titers of 20-E were normally distributed in each treatment group, and the differences among the different treatment groups were analyzed by performing the one-way analysis of variance (ANOVA) with Tukey’s multiple comparisons. The percentage of time spent moving (adults), courting, and mating was not normally distributed. For these three parameters, the Kruskal-Wallis and Wilcoxon rank sum tests were performed to compare the differences among/between different treatment groups. All statistical analyses were performed using SPSS version 22.0 (IBM, Armonk, NY, United States).
Results
Effects of bat predation risks on the growth and development of S. litura
The food intake of S. litura larvae exposed to different predation risks and white noise was significantly higher than that of the larvae in the Control group (F5, 449 = 28.976, p < 0.001, Figure 1A), and the larvae in the CF-call, CF-exp, and FM-exp groups had the highest food intake, followed by those in the FM-call and Whitenoise groups. Additionally, larvae exposed to predation risk showed faster development of the larval stages compared to those in the Control group (Figure 1B), whereas their maximum fresh weight was not significantly different from those in the Control group (Figure 1C). However, the weight of pupae was significantly lower for those that were exposed to different predation risks compared to the weight of pupae in the Control group (F5, 436 = 11.017, p < 0.001, Figure 1D). The weight of pupae was significantly lower in the FM-exp and Whitenoise groups (p < 0.001, Figure 1D), and although it was also lower in the CF-exp, CF-call, and FM-call groups, the differences were not significant.
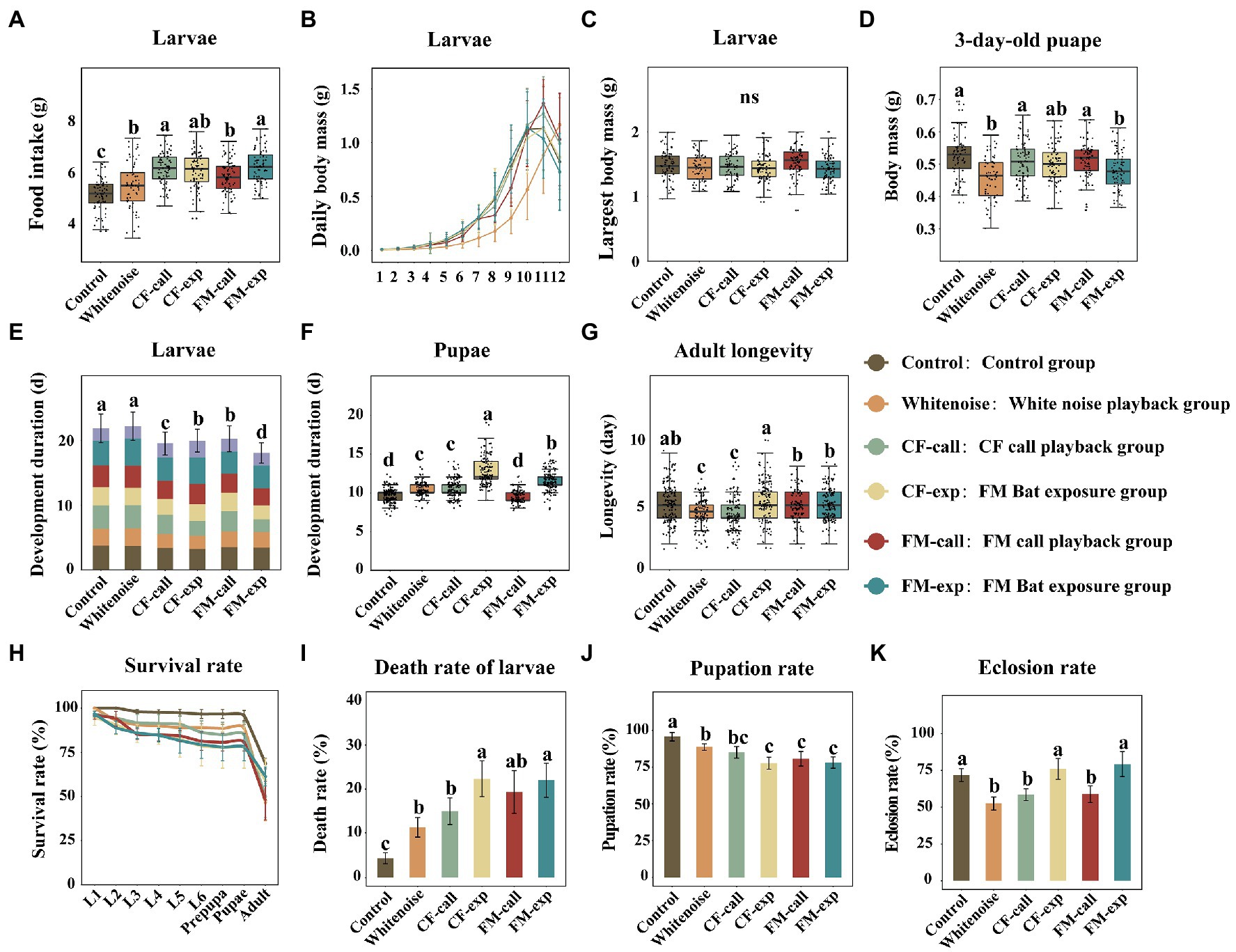
Figure 1. Effects of different treatments on the growth and development of S. litura. (A) Food intake of larvae in different treatment groups, (B) diurnal variation in the body mass of the larvae, (C) maximum fresh body mass of the larvae, (D) body mass of 3-day-old pupae, (E) the duration of larval development, (F) the duration of pupal development, (G) longevity of moths, (H) the survival rate at different life stages, (I) the death rate of larvae, (J) the pupation rate of larvae, and (K) the eclosion rate of pupae. The data are expressed as the mean ± SD. Different colors represent data from different treatment groups. The different letters on the bar and box plots indicate significant differences between treatment groups (Tukey’s test, p < 0.05); ns indicates no significant difference between treatments.
The bat and echolocation playback call treatments significantly accelerated larval growth (Figure 1E) and decreased larval developmental duration (F5, 1,514 = 18.630, p < 0.001, Figure 1E), but the growth of larvae was not significantly different between the Whitenoise and Control groups (Figure 1E). The duration of the pupal period also showed differences between treatments (F5, 733 = 18.630, p < 0.001; Figure 1F). The pupae in the CF-exp group had the longest pupal period (12.62 ± 1.63 day), followed by those in the FM-exp (11.63 ± 1.19 day), CF-call (10.56 ± 1.19 day), and Whitenoise (10.27 ± 0.97 day) groups. The pupal period of the FM-call group was 9.57 ± 0.71 day, which was not significantly different from that of the pupae in the Control group. The longevity of S. litura adults was significantly lower for those in the CF-call and Whitenoise groups compared to the longevity of those in the Control group (F5, 733 = 16.285, p < 0.001, Figure 1G). However, although the longevity of adults was lower for those in the FM-exp, FM-call, and CF-exp groups relative to those in the Control group, the differences were not significant (Figure 1G).
For the S. litura individuals exposed to different predation risks and white noise, their survival rate was significantly lower (Figure 1H), larval mortality was significantly higher (F5, 30 = 11.017, p < 0.001, Figure 1I), and pupation rate was significantly lower (F5, 30 = 17.575, p < 0.001, Figure 1J) than those of the individuals in the Control group. Overall, the moths exposed to the two bat playback treatments had the highest larval mortality (p < 0.001, Figure 1I), followed by those in the FM-call, CF-call, and Whitenoise groups (p = 0.032, Figure 1I). Similarly, the moths in the CF-exp and FM-exp groups had the lowest pupation rate (p < 0.001, Figure 1J), followed by those in the FM-call and CF-call groups (p = 0.001, Figure 1J), but no significant difference was found between the moths in the Whitenoise and Control groups. The eclosion rate was significantly lower (F5, 30 = 17.084, p < 0.001, Figure 1K) only for pupae exposed to CF echolocation calls (p = 0.014, Figure 1K), FM echolocation calls (p = 0.019, Figure 1K), and white noise (p < 0.001, Figure 1K) relative to that of the pupae in the Control group, however, exposure to the two bat playback treatments had no significant effect on the eclosion rate of the pupae.
Effects of bat predation risks on the reproductive behavior and fecundity of S. litura
The percentage of time spent moving was significantly lower for S. litura individuals exposed to different bat predation risks and white noise (H5, 30 = 20.268, p = 0.001, Figure 2A) than for the individuals in the Control group. The percentage of time spent moving for individuals in the FM-exp group was significantly lower than that for the individuals in the Control group (p = 0.001, Figure 2A). The proportion of mating behavior of S. litura individuals in all treatment groups was similar (H5, 30 = 6.792, p = 0.237, Figure 2B). However, among all groups of individuals exposed to bats and bat echolocation playback calls, mating behavior was only observed once in the CF-call, FM-call, and FM-exp groups. The percentage of time spent courting for individuals in all treatment groups was similar to that for individuals in the Control group, but it was significantly lower for individuals in the FM-call group than that for individuals in the CF-call group (p = 0.001, Figure 2C).
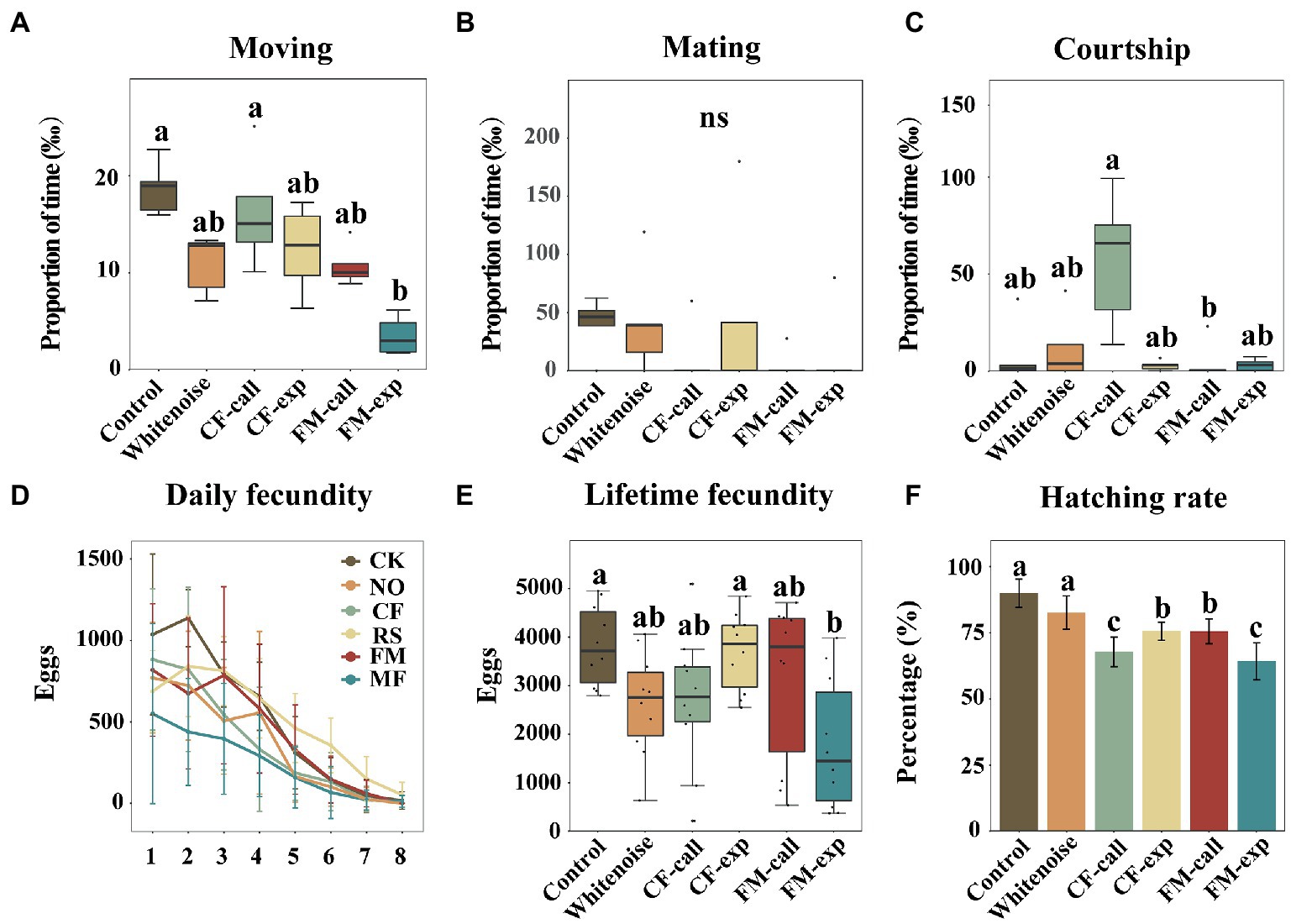
Figure 2. Reproductive behavior and fecundity of S. litura moths under different types of predation risk. (A) The proportion of time spent moving, (B) the proportion of time spent mating, (C) the proportion of time spent courting, (D) daily fecundity, (E) lifetime fecundity, and (F) the hatching rate. The data are expressed as the mean ± SD. Different letters on the bars or box plots indicate significant differences between treatments (Tukey’s test, p < 0.05); ns indicates no significant difference between treatments.
The peak fecundity period of S. litura was the third day after laying, and then, the fecundity of the moths decreased, except for those in the CF-call group, where the daily fecundity was higher than that of the moths in the Control group on the fourth day after laying (Figure 2D). Overall, the total fecundity of S. litura decreased when exposed to different predation risks and white noise (F5, 54 = 3.855, p = 0.005, Figure 2E). The moths in the FM-exp group produced the lowest number of eggs (1,935 ± 1,465), which was significantly lower than the number of eggs laid by moths of the other groups (p < 0.001, Figure 2E), i.e., the Whitenoise group (2,847 ± 1,149), the CF-call group (2,912 ± 1,507), and the FM-call group (3,397 ± 1,798). The number of eggs laid by moths in the CF-exp group (4,008 ± 864) was lower than that laid by moths in the Control group (4,138 ± 892), but the differences were not significant. The hatching rate of the moths exposed to different predation risks and white noise was significantly lower than that of the moths in the Control group (F5, 54 = 29.333, p < 0.001, Figure 2F), with the lowest hatching rate (64.20%) recorded for moths in the FM-exp group (p < 0.001, Figure 2E), followed by those in the CF-call (67.80%), FM-call (75.50%), CF-exp (75.6%), Whitenoise (82.60%), and Control groups (89.90%).
Effects of the predation risk of bats on the titers of JH and 20-E in S. litura
Except for the late fifth instar in the Whitenoise group, the titers of JH in S. litura larvae under different predation risks and white noise were significantly higher at the late fourth instar (F5, 12 = 63.152, p < 0.001, Figure 3A), late fifth instar (F5, 12 = 92.645, p < 0.001, Figure 3A), and late sixth instar (F5, 12 = 92.645, p < 0.001, Figure 3A) compared to that in the larvae of the Control group. The larvae in the FM-call group had the highest JH titers at the late fourth instar, but no significant differences were observed between the larvae in different predation risk groups at the late fifth instar. The highest titers of JH were found in the larvae of the FM-exp group (p < 0.001, Figure 3A) at the late sixth instar. The JH titers were significantly higher in the larvae of the Whitenoise group than that in the larvae of the Control group at both the late fourth and late sixth instars (p < 0.001, Figure 3A) but not in the late fifth instar.
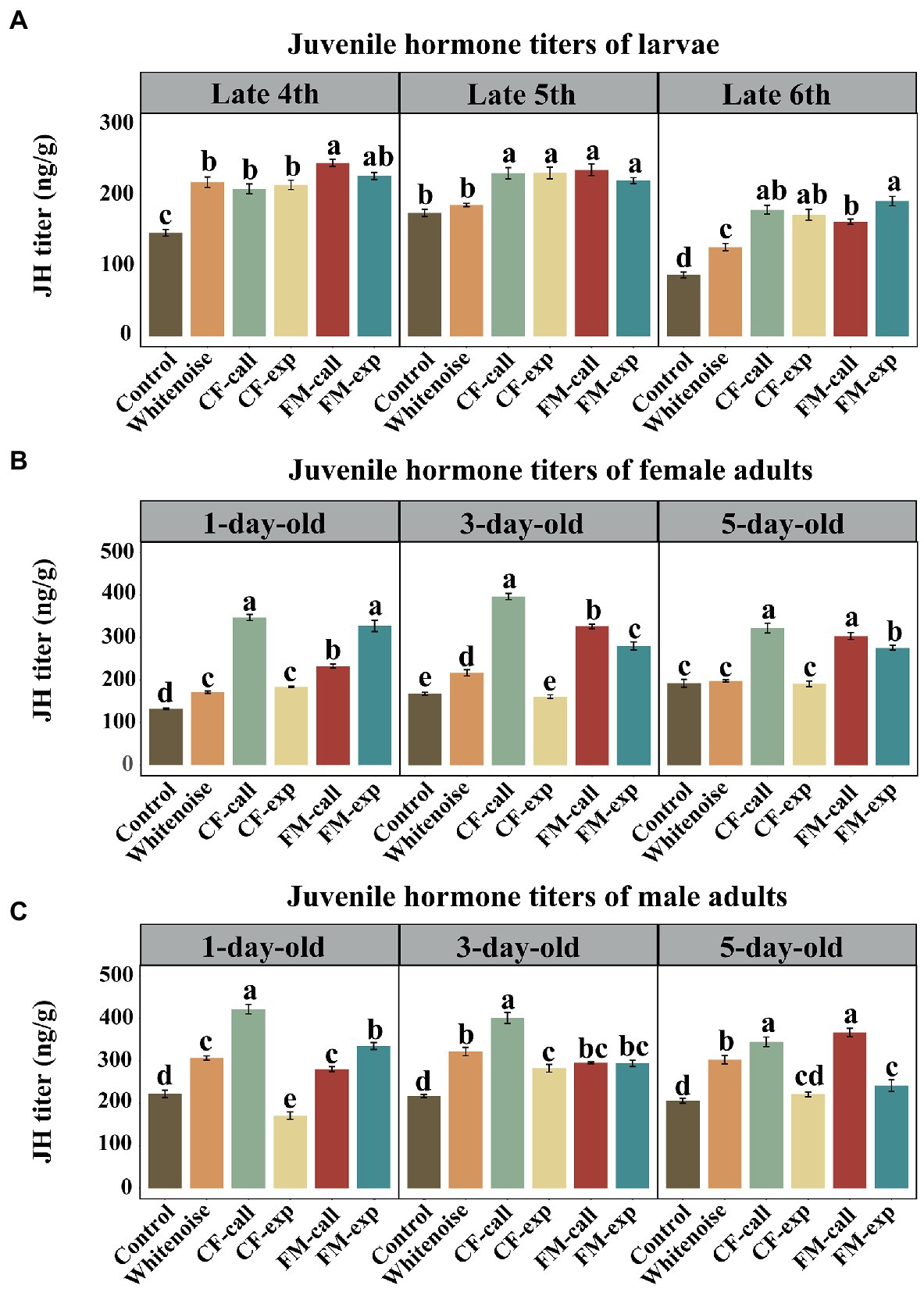
Figure 3. The JH titers of S. litura under different types of predation risk. The JH titers of (A) larvae of different ages, (B) female adult moths, and (C) male adult moths. The data are expressed as the mean ± SD. Different letters on the bars indicate significant differences between treatments (Tukey’s test, p < 0.05).
Except for the 3- and 5-day-old female moths in the CF-exp group and the 5-day-old female moths in the Whitenoise group, the 1-day-old (F5, 12 = 344.102, p < 0.001, Figure 3B), 3-day-old (F5, 12 = 406.822, p < 0.001, Figure 3B), and 5-day-old (F5, 12 = 119.925, p < 0.001, Figure 3B) female moths under the predation risk of bats and white noise treatment had significantly higher JH titers than those in the Control group. The highest JH titers were recorded in the 1-day-old female moths of the CF-call (p < 0.001, Figure 3B) and FM-exp groups (p < 0.001, Figure 3B). Additionally, the highest JH titers were also observed in the 3-day-old female adults of the CF-call group (p < 0.001, Figure 3B) and in the 5-day-old female moths of the CF-call and FM-call groups (p < 0.001, Figure 3B).
Except for the 1- and 5-day-old male moths in the CF-exp group, the 1-day-old (F5, 12 = 344.102, p < 0.001, Figure 3C), 3-day-old (F5, 12 = 406.822, p < 0.001, Figure 3C), and 5-day-old (F5, 12 = 119.925, p < 0.001, Figure 3C) adult male moths exposed to bat predation risk or white noise treatment had significantly higher JH titers than those in the Control group. The highest titers of JH were found in the moths of the CF-call group for both 1-day-old (p < 0.001, Figure 3C) and 3-day-old (p < 0.001, Figure 3C) male moths, but the highest titers of JH was observed in the 5-day-old moths of the FM-call and CF-call groups (p < 0.001 Figure 3C).
Effects of bat predation risks on the 20-E titers of S. litura
Except for the late fifth instar in the CF-exp group, the 20-E titers of larvae exposed to the predation risk of bats and white noise treatment were significantly higher than those in the larvae of the Control group at the late fourth instar (F5, 12 = 105.267, p < 0.001, Figure 4A), late fifth instar (F5, 12 = 79.6340, p < 0.001, Figure 4A) and late sixth instar (F5, 12 = 28.492, p < 0.001, Figure 4A). The highest 20-E titers were observed in the larvae of the CF-call group (p < 0.001, Figure 4A) at the late fourth instar, and in the larvae of the CF-call and FM-exp groups at the late fifth instar and late sixth instar (p < 0.001, Figure 4A).
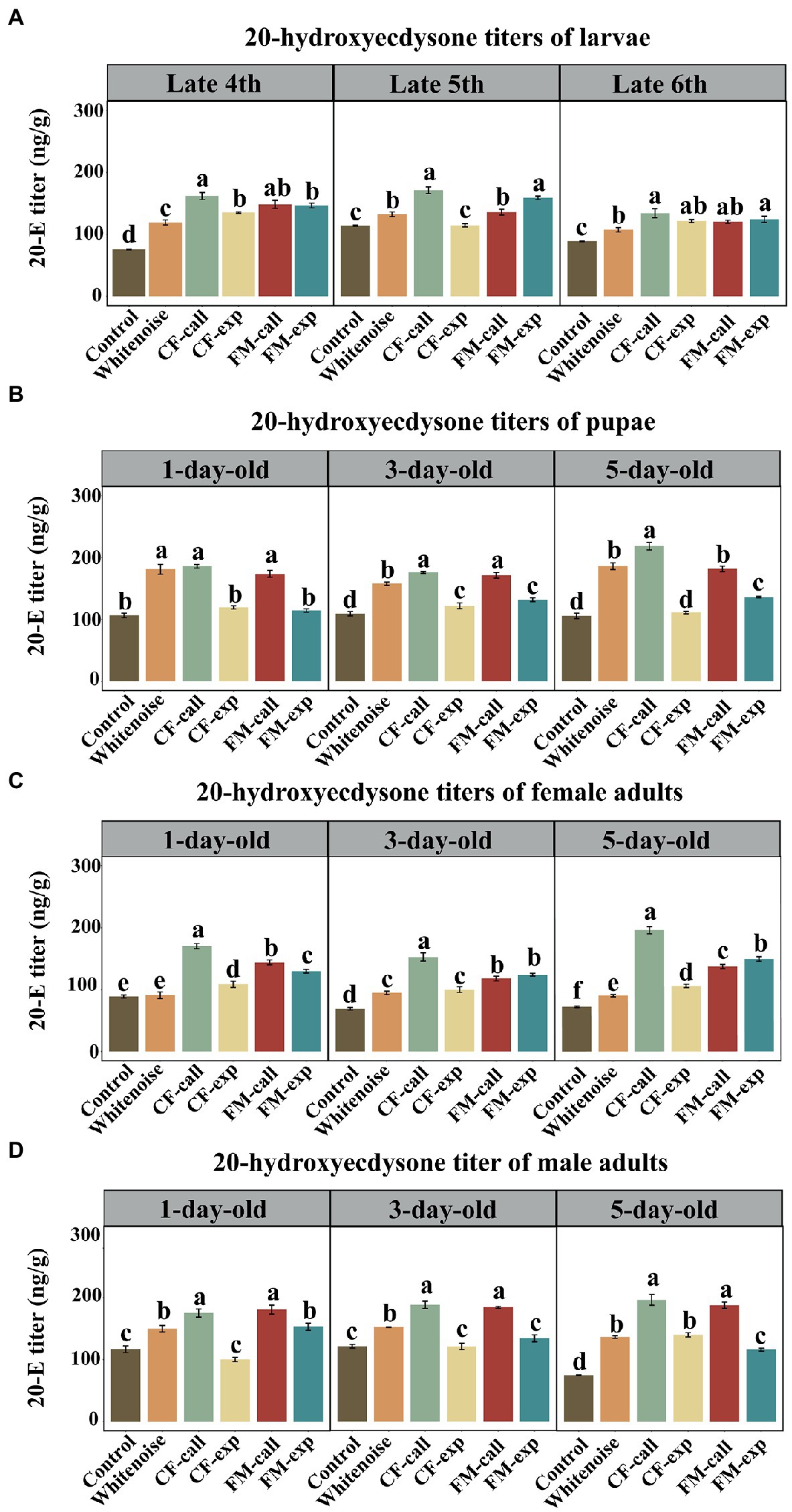
Figure 4. The 20-E titers in the different life stages of S. litura under different types of predation risk. The 20-E titers of (A) larvae, (B) pupae, (C) female adults, and (D) male adults. The data are expressed as the mean ± SD. Different letters on the bars indicate significant differences between treatments (Tukey’s test, p < 0.05).
The 20-E titers of 1-day-old (F5, 12 = 128.045, p < 0.001, Figure 4B), 3-day-old (F5, 12 = 122.559, p < 0.001, Figure 4B), and 5-day-old (F5, 12 = 218.364, p < 0.001, Figure 4B) pupae in the CF-call, FM-call, and Whitenoise groups were significantly higher than that in the pupae of the Control group. The 20-E titers among the three acoustic groups for the 1-day-old pupae were not significantly different (Figure 4B). The highest 20-E titers for 3-day-old pupae were recorded in the CF-call (p < 0.001, Figure 4B) and FM-call groups (p < 0.001, Figure 4B) and in the 5-day-old pupae of the CF-call group (p < 0.001, Figure 4B).
Except for the 1-day-old female adults in the Whitenoise group, the 1-day-old (F5, 12 = 118.900, p < 0.001, Figure 4C), 3-day-old (F5, 12 = 108.990, p < 0.001, Figure 4C), and 5-day-old (F5, 12 = 336.080, p < 0.001, Figure 4C) adult females exposed to predation by bats and white noise treatment had higher 20-E titers than the females in the Control group. The highest titers of 20-E were found in the 1-day-old (p < 0.001, Figure 4C), 3-day-old (p < 0.001, Figure 4C), and 5-day-old (p < 0.001, Figure 4C) female adults of the CF-call group.
Except for the 1- and 3-day-old male adults of S. litura in the CF-exp group and the 3-day-old male adults in the FM-exp group, the 1-day-old (F5, 12 = 61.749, p < 0.001, Figure 4D), 3-day-old (F5, 12 = 104.083, p < 0.001, Figure 4D), and 5-day-old (F5, 12 = 202.927, p < 0.001, Figure 4D) adult male moths exposed to predation risk of bats and white noise treatment had significantly higher 20-E titers than those in the Control group. The highest titers of 20-E were found in the 1-day-old (p < 0.001; Figure 4C), 3-day-old (p < 0.001; Figure 4C), and 5-day-old (p < 0.001; Figure 4C) adult males of the CF-call and FM-call groups.
Discussion
Our results showed that the larvae of S. litura responded to predation risks by increasing their food intake and accelerating their development, but their body mass did not increase. The duration of larval development in the presence of predation risk was shorter than that without predation risk, and the duration of pupal development was longer. In addition, mortality and the rate of metamorphosis failure, which included pupation and eclosion failure, were higher at all stages for the moths exposed to predation risk. These results supported our first prediction that bat predation risk might be associated with changes in the growth and development of S. litura, including food intake, body mass, survival rate, death rate, pupation rate, and eclosion rate. The predation risk decreased the reproductive behavior, fecundity, and adult longevity of S. litura, which supported our second prediction that bat predation risk might be associated with a decrease in reproductive behavior and fecundity in S. litura. Finally, larvae and pupae of S. litura exposed to predation risk cues showed significantly higher titers of JH and 20-E, which supported our third prediction that the levels of the JH and 20-E hormone of S. litura might change after exposure to the risk of predation by bats.
Predation risk can affect prey growth and development (Higginson and Ruxton, 2010; Moore et al., 2018). For example, in Manduca sexta, individuals exposed to predators decreased their food intake by 30–40% but developed faster and gained the same weight as the individuals with no predation risk (Thaler et al., 2012). Individuals of Helicoverpa armigera accelerate their development, enter the pupal and adult stages earlier, and have a bigger body size as adults when exposed to the risk of predation by Harmonia axyridis (Xiong et al., 2015). Individuals of Aedes notoscriptus have slower development and a smaller size as adults when exposed to the risk of predation by fish (van Uitregt et al., 2012). In this study, the predation risk of bats resulted in faster development, smaller size of adults, higher mortality and metamorphosis failure rates, and lower adult longevity of S. litura moths compared to the corresponding changes in the moths of the control group. Predation risk-induced rapid development in prey demands more energy for the maintenance of physiological functions, which in turn requires them to obtain more resources (Barton, 2010). In general, the large size of the body is, in many cases, positively correlated with fecundity and survival probability (Brodin and Johansson, 2004). However, our results were contrary to this pattern. Predation risk can increase stress in prey, decrease antioxidant defenses, and result in oxidative damage (Zha and Lei, 2012; Janssens and Stoks, 2013, 2014; Roux et al., 2021; Venkanna et al., 2021). Oxidative damage is a major determinant of the life histories of animals and can influence organisms over a long period in many ways, e.g., decrease fertility, accelerate aging (Monaghan et al., 2009), and increase mortality and metamorphosis failure rates (Baranowski and Preisser, 2018). Predation risk also induces faster metabolism and increases consumption rates in prey (Schmitz et al., 2016), which might explain the increased food intake but smaller body size of S. litura moths exposed to predation risk. Additionally, some studies have found a trade-off between prey stress and immune function under predation risk (Duong and McCauley, 2016; Schwenke et al., 2016). When exposed to predation risk, the energy allocated to immunity decreases in prey, which decreases the immune function and increases mortality. In this study, the larvae of S. litura were vulnerable to the environment, and the adults were more susceptible to predation by bats. This caused individuals to increase their food intake, accelerate larval development, and extend the pupal stage, as an adaptive response to the risk of predation by bats. The increase in the mortality and metamorphosis failure rates were associated with the adaptive response of S. litura.
Predation risk affects prey reproduction by decreasing foraging behavior or by adversely affecting physiological effects, which in turn decreases their fecundity and the fitness of their offspring (Peckarsky et al., 1993; Mukherjee et al., 2014; Villalobos-Sambucaro et al., 2020). Usually, the resources accumulated by lepidopterans in the larval stage are used for reproduction (Fox and Czesak, 2000). An increase in body size increases fitness, which might be positively related to higher fecundity, probability of survival, and mating success (Brodin and Johansson, 2004). For example, bigger males of S. litura have greater chances of mating (Fox and Czesak, 2000; Okuzaki, 2021). A smaller body might decrease reproductive behavior and reproductive success. A decrease in the fecundity of S. litura individuals under predation risk might be related to a decrease in the reproductive success of S. litura. Furthermore, all of S. litura’s activities took place at night (Li et al., 2012). In our study, no mating behavior was observed in the S. litura exposed to the risk of predation by bats, but egg production was observed, which could be the S. litura started to lay eggs at one point, whether they were mated or not, and thus these eggs were unfertilized, which may explain why the predation risk reduces the hatching rate of the egg of the S. litura. Additionally, a trade-off might occur between reproduction and immunity in insects (Schwenke et al., 2016; Guo et al., 2022). Mating promotes reproductive activity, and an increase in reproductive activity might decrease immune function (Gao et al., 2020). For example, delayed or no mating in adult S. litura is associated with longer life, probably due to lower energy investment in reproduction; this effect is especially prominent among females, as they allocate more energy to reproduction (Wu et al., 2018). Additionally, individuals of Helicoverpa armigera (Xiong et al., 2015), Mamestra brassicae (Huang et al., 2003) and Plodia interpunctella (Huang and Subramanyam, 2004) also had lower fecundity when exposed to ultrasound. The predation risk of bats decreased reproductive behavior, fecundity, and adult longevity in S. litura, which was similar to the findings of previous studies. We speculated that S. litura exposed to bat predation risk might be under chronic stress; oxidative stress might lead to a smaller body size and an increase in energy requirements allocated to survival. This, in turn, might cause lesser energy to be allocated to immunity and reproduction. However, there is a trade-off between reproduction and immunity in S. litura, where energy is preferentially allocated to immunity; this leads to a decrease in reproductive activity and fecundity.
Besides morphological and reproductive changes, physiological changes might occur in prey exposed to predation risk (Duong and McCauley, 2016). For example, the antioxidant metabolism and oxidoreductase activity increased in Spodoptera frugiperda after exposure to bat ultrasound (Cinel and Taylor, 2019). In our study, larvae, pupae, and adults of S. litura exposed to predation risk showed an increase in the levels of JH and 20-E to different degrees. Hormones are regulatory signaling factors and coordinate multiple developmental and physiological processes in insects (Cherbas et al., 1989; Flatt et al., 2005). JH and 20-E synergistically regulate insect growth and development, metamorphosis, reproduction, and different behaviors (Kim et al., 2019; Santos et al., 2019). The 20-E hormone can initiate and regulate molting and metamorphosis, while JH can regulate the direction of metamorphosis (Truman and Riddiford, 2002). High levels of JH not only promote oocyte maturation (Fleig, 1995) and the expression of the yolk protein genes but also suppress stress resistance and immune function (Parthasarathy et al., 2010; Süren-Castillo et al., 2012). High levels of 20-E, however, increase the expression of immune-related genes and lead to egg resorption of immature yolk (Soller et al., 1999; Schwenke et al., 2016). Therefore, maintaining the balance between JH and 20-E levels is essential for insect metamorphosis, reproduction, and immunity. In our study, exposure to the predation risk of bats increased larval 20-E titers, which accelerated development by advancing the molting time of S. litura. However, an increase in JH titers ensured that S. litura could maintain its larval form under predation risk. Exposure to white noise, CF-call, and FM-call treatments significantly increased the 20-E titers relative to that after exposure to the control and bat treatments; the eclosion rate increased significantly. We speculated that the increase in the 20-E titers in the pupal stage might be responsible for the decrease in the eclosion rate. An increase in the JH and 20-E titers in the adult stage was closely related to the reproductive and immune activities of adults. An increase in the JH titers under predation risk can promote the reproductive activities of adults, while an increase in 20-E titers can promote the immune activities of adults. However, although individuals showed physiological adaptation to predation risk, those in different life stages still experienced high mortality, higher failure of metamorphosis, and lower fecundity and longevity.
The strength of NCEs depends on the ability of the prey to perceive predators, and prey perceive predators through various sense organs (Hermann and Thaler, 2014). They might use multiple sensory systems individually or simultaneously (Rosier and Langkilde, 2011) and assess the magnitude of predation risk through multiple sensory systems (Gonzálvez and Rodríguez-Gironés, 2013). The FM-bat exposure group developed the fastest and had the lowest reproduction ability in this study, followed by the CF-bat exposure group, FM-call playback group, CF-call playback group, and White noise playback group. We discovered that exposure to bat predators had a greater negative impact than a single acoustic cue, with FM calls and FM bat predators having a greater negative impact than CF calls and CF bat predators. Previous research has shown that moth behavior and physiology vary with predation risk (Lalita and McNeil, 1998). M. fuliginosus prefers to forage in open farmland (Alberdi et al., 2020), R. sinicus prefers to forage in complex habitats (Aldridge and Rautenbach, 1987), and the former has a broader range of calls. The tympanic membranes of nocturnal moths can detect ultrasound frequencies from 10 to 100 kHz, but they are better at detecting frequencies between 20 and 50 kHz (Zha and Lei, 2012). The bandwidth of the echolocation calls of M. fuliginosus was larger than that of the CF bat R. sinicus. Thus, FM calls might pose a higher predation risk than CF calls. Noise is also a common abiotic environmental stress factor affecting organisms, which has a significant negative impact on the physiological ecology of organisms (Zha and Lei, 2012). In our study, the white noise frequency was 0–100 kHz which was wider than the bandwidth of two bats echolocation calls. However, the effects were lesser than those associated with bat predators and bat echolocation calls. Additionally, exposure to bats might be perceived as a greater predation risk by individuals of S. litura because they might detect chemical and visual cues of bats through smell or vision. We also found that acoustic cues had a greater effect on the pupae, which might be because the pupal shell prevented the detection of some of the chemical components, but acoustic signals could still be detected by S. litura through physical vibrations. Additionally, the effects of exposure to bats during the larval and adult stages were greater than the effects of exposure to a single acoustic cue, suggesting that S. litura might also perceive predation risk via vision and smell. These findings imply that S. litura can recognize various predation risks, assess the magnitude of predation risk, and devise appropriate defense strategies.
In conclusion, our results indicated that S. litura moths exposed to bat predation risk were in a state of chronic oxidative stress and suffered oxidative damage. Although the individuals showed varying degrees of adaptive responses to stress, they still had lower survival and reproduction. We found that the presence of bats might impose a greater risk of predation on S. litura than the presence of only their ultrasound calls. Our study showed that the NCEs associated with the risk of predation strongly influenced the size of the S. litura population in the laboratory, which might provide a novel approach to the biological control of S. litura in the field.
However, the echolocation calls used in this study were recorded while the bats were semi-starved, and the bats in the exposed group were also semi-starved, and it is unclear whether the hungry bats made the moths more terrified, which would require further investigation. The effects of bats on the population of S. litura moths in the field might be greater, which needs to be confirmed through field trials. Additionally, nocturnal predation by bats can significantly decrease agricultural pests on farmland (Rodríguez-San Pedro et al., 2020; Charbonnier et al., 2021), and the combination of direct and indirect effects of bats might be more effective in controlling pest populations than each effect in isolation. Field experiments will be conducted in the future to assess the effects of bat CEs and NCEs on moths, as well as the ability of bat biological control and the potential economic benefits.
Data availability statement
The original contributions presented in the study are included in the article/Supplementary material, further inquiries can be directed to the corresponding authors.
Ethics statement
The animal study was reviewed and approved by Bat capture methods and experiments conformed to the Northeast Normal University guidelines for animal behavior research. All husbandry and experimental procedures adhered to with the ASAB/ABS Guidelines for the Treatment of Animals in Behavioral Research and were approved by the Wildlife Conservation Office of Jilin Forestry Department, China.
Author contributions
TJ, WZ, and YL conceived the ideas and designed methodology. WZ and TL collected the samples and data. WZ analyzed the data. WZ and YL wrote the manuscript. TJ and JF revised the original draft. All authors contributed critically to the drafts and gave final approval for publication.
Funding
This research was supported by the National Natural Science Foundation of China (Grant Nos. 31922050, 31872680, and 32071492) and the Fund of the Jilin Province Science and Technology Development Project (Grant No. 20220101273JC).
Acknowledgments
We sincerely thank YinLi Hu and Jiqian Li for their great contributions to the recording of foraging echolocation calls. We are also grateful to Yang Gen and Yinli Hu for their contributions to larval and bat rearing.
Conflict of interest
The authors declare that the research was conducted in the absence of any commercial or financial relationships that could be construed as a potential conflict of interest.
Publisher’s note
All claims expressed in this article are solely those of the authors and do not necessarily represent those of their affiliated organizations, or those of the publisher, the editors and the reviewers. Any product that may be evaluated in this article, or claim that may be made by its manufacturer, is not guaranteed or endorsed by the publisher.
References
Abom, R., and Schwarzkopf, L. (2016). Differential behavioural flexibility in response to predation risk in native and introduced tropical savannah rodents. Anim. Behav. 122, 117–124. doi: 10.1016/j.anbehav.2016.10.005
Agah-Manesh, H., Rajabpour, A., Yarahmadi, F., and Farsi, A. (2021). Potential of ultrasound to control Sesamia cretica (Lepidoptera: Noctuidae). Environ. Entomol. 50, 1393–1399. doi: 10.1093/ee/nvab103
Alberdi, A., Razgour, O., Aizpurua, O., Novella-Fernandez, R., Aihartza, J., Budinski, I., et al. (2020). DNA metabarcoding and spatial modelling link diet diversification with distribution homogeneity in European bats. Nat. Commun. 11:1154. doi: 10.1038/s41467-020-14961-2
Aldridge, H., and Rautenbach, I. (1987). Morphology, echolocation and resource partitioning in insectivorous bats. J. Anim. Ecol. 56, 763–778. doi: 10.2307/4947
Appel, G., López-Baucells, A., Rocha, R., Meyer, C. F., and Bobrowiec, P. E. D. (2021). Habitat disturbance trumps moonlight effects on the activity of tropical insectivorous bats. Anim. Conserv. 24, 1046–1058. doi: 10.1111/acv.12706
Baranowski, A. K., and Preisser, E. L. (2018). Predator cues increase silkmoth mortality. Front. Ecol. Evol. 6:220. doi: 10.3389/fevo.2018.00220
Barton, B. T. (2010). Climate warming and predation risk during herbivore ontogeny. Ecology 91, 2811–2818. doi: 10.1890/09-2278.1
Bauman, A. G., Seah, J. C. L., Januchowski-Hartley, F. A., Hoey, A. S., Fong, J., and Todd, P. A. (2019). Fear effects associated with predator presence and habitat structure interact to alter herbivory on coral reefs. Biol. Lett. 15:20190409. doi: 10.1098/rsbl.2019.0409
Benti, B., Miller, P. J. O., Biuw, M., and Curé, C. (2021). Indication that the behavioural responses of humpback whales to killer whale sounds are influenced by trophic relationships. Mar. Ecol. Prog. Ser. 660, 217–232. doi: 10.3354/meps13592
Brodin, T., and Johansson, F. (2004). Conflicting selection pressures on the growth/predation-risk trade-off in a damselfly. Ecology 85, 2927–2932. doi: 10.1890/03-3120
Carpenter, S. R., Cole, J. J., Hodgson, J. R., Kitchell, J. F., Pace, M. L., Bade, D., et al. (2001). Trophic cascades, nutrients, and lake productivity: Whole-lake experiments. Ecol. Monogr. 71, 163–186. doi: 10.1890/0012-9615(2001)071[0163:Tcnalp]2.0.Co;2
Charbonnier, Y., Papura, D., Touzot, O., Rhouy, N., Sentenac, G., and Rusch, A. (2021). Pest control services provided by bats in vineyard landscapes. Agric. Ecosyst. Environ. 306:107207. doi: 10.1016/j.agee.2020.107207
Cherbas, L., Koehler, M. M. D., and Cherbas, P. (1989). Effects of juvenile hormone on the ecdysone response of drosophila Kc cells. Dev. Genet. 10, 177–188. doi: 10.1002/dvg.1020100307
Cinel, S. D., Hahn, D. A., and Kawahara, A. Y. (2020). Predator-induced stress responses in insects: A review. J. Insect Physiol. 122:104039. doi: 10.1016/j.jinsphys.2020.104039
Cinel, S. D., and Taylor, S. J. (2019). Prolonged bat call exposure induces a broad transcriptional response in the male fall armyworm (Spodoptera frugiperda; Lepidoptera: Noctuidae) brain. Front. Behav. Neurosci. 13:36. doi: 10.3389/fnbeh.2019.00036
DeWitt, P. D., Visscher, D. R., Schuler, M. S., and Thiel, R. P. (2019). Predation risks suppress lifetime fitness in a wild mammal. Oikos 128, 790–797. doi: 10.1111/oik.05935
Duong, T. M., and McCauley, S. J. (2016). Predation risk increases immune response in a larval dragonfly (Leucorrhinia intacta). Ecology 97, 1605–1610. doi: 10.1890/15-1964.1
Fauteux, D., Gauthier, G., Berteaux, D., Palme, R., and Boonstra, R. (2018). High Arctic lemmings remain reproductively active under predator-induced elevated stress. Oecologia 187, 657–666. doi: 10.1007/s00442-018-4140-4
Flatt, T., Tu, M. P., and Tatar, M. (2005). Hormonal pleiotropy and the juvenile hormone regulation of drosophila development and life history. BioEssays 27, 999–1010. doi: 10.1002/bies.20290
Fleig, R. (1995). Role of the follicle cells for yolk uptake in ovarian follicles of the honey bee Apis mellifera L. (Hymenoptera: Apidae). Int. J. Insect Morphol. Embryol. 24, 427–433. doi: 10.1016/0020-7322(95)98841-Z
Fox, C. W., and Czesak, M. E. (2000). Evolutionary ecology of progeny size in arthropods. Ann. Rev. Entom. 45, 341–369. doi: 10.1146/annurev.ento.45.1.341
Frank, D. A. (2008). Evidence for top predator control of a grazing ecosystem. Oikos 117, 1718–1724. doi: 10.1111/j.1600-0706.2008.16846.x
Gao, B., Song, X. Q., Yu, H., Fu, D. Y., Xu, J., and Ye, H. (2020). Mating-induced differential expression in genes related to reproduction and immunity in Spodoptera litura (Lepidoptera: Noctuidae) female moths. J. Insect Sci. 20, 1–10. doi: 10.1093/jisesa/ieaa003
Gong, L., Geng, Y., Wang, Z., Lin, A., Wu, H., Feng, L., et al. (2022). Behavioral innovation and genomic novelty are associated with the exploitation of a challenging dietary opportunity by an avivorous bat. iScience 25:104973. doi: 10.1016/j.isci.2022.104973
Gonzálvez, F. G., and Rodríguez-Gironés, M. A. (2013). Seeing is believing: Information content and behavioural response to visual and chemical cues. Proc. R. Soc. B Biol. Sci. 280:20130886. doi: 10.1098/rspb.2013.0886
Guanghong, L., Qijin, C., and Yi, P. (1998). Studies of artificial diets for the beet armyworm, Spodoptera exigua. Acta Sci. Nat. Univ. Sunyatseni 37, 1–5.
Guo, P., Yu, H., Xu, J., Li, Y. H., and Ye, H. (2022). Flight muscle structure and flight capacity of females of the long-distance migratory armyworm, Spodoptera frugiperda: Effect of aging and reproduction, and trade-offs between flight and fecundity. Entomol. Exp. Appl. 1–11. doi: 10.1111/eea.13248
Hawlena, D., and Schmitz, O. J. (2010). Herbivore physiological response to predation risk and implications for ecosystem nutrient dynamics. Proc. Natl. Acad. Sci. U. S. A. 107, 15503–15507. doi: 10.1073/pnas.1009300107
Hermann, S. L., and Thaler, J. S. (2014). Prey perception of predation risk: Volatile chemical cues mediate non-consumptive effects of a predator on a herbivorous insect. Oecologia 176, 669–676. doi: 10.1007/s00442-014-3069-5
Higginson, A., and Ruxton, G. (2010). Adaptive changes in size and age at metamorphosis can qualitatively vary with predator type and available defenses. Ecology 91, 2756–2768. doi: 10.1890/08-2269.1
Huang, F., and Subramanyam, B. (2004). Behavioral and reproductive effects of ultrasound on the Indian meal moth, Plodia interpunctella. Entomol. Exp. Appl. 113, 157–164. doi: 10.1111/j.0013-8703.2004.00217.x
Huang, F., Subramanyam, B., and Taylor, R. (2003). Ultrasound affects spermatophore transfer, larval numbers, and larval weight of Plodia interpunctella (Hübner) (Lepidoptera: Pyralidae). J. Stored Prod. Res. 39, 413–422. doi: 10.1016/s0022-474x(02)00035-8
Hughes, A. R., Mann, D. A., and Kimbro, D. L. (2014). Predatory fish sounds can alter crab foraging behaviour and influence bivalve abundance. Proc. Biol. Sci. 281:20140715. doi: 10.1098/rspb.2014.0715
Janssens, L., and Stoks, R. (2013). Predation risk causes oxidative damage in prey. Biol. Lett. 9:20130350. doi: 10.1098/rsbl.2013.0350
Janssens, L., and Stoks, R. (2014). Chronic predation risk reduces escape speed by increasing oxidative damage: A deadly cost of an adaptive antipredator response. PLoS One 9:e101273. doi: 10.1371/journal.pone.0101273
Jones, G., and Teeling, E. C. (2006). The evolution of echolocation in bats. Trends Ecol. Evol. 21, 149–156. doi: 10.1016/j.tree.2006.01.001
Kim, K. N., Sin, U. C., Jo, Y. C., Huang, Z. J., Hassan, A., Huang, Q. Y., et al. (2019). Influence of green light at night on juvenile hormone in the oriental armyworm Mythimna separata (Lepidoptera: Noctuidae). Physiol. Entomol. 44, 245–251. doi: 10.1111/phen.12300
Krebs, C. J., Boutin, S., Boonstra, R., Sinclair, A. R. E., Smith, J. N. M., Dale, M. R. T., et al. (1995). Impact of food and predation on the snowshoe hare cycle. Science 269, 1112–1115. doi: 10.1126/science.269.5227.1112
Kunz, T. H., Braun de Torrez, E., Bauer, D., Lobova, T., and Fleming, T. H. (2011). Ecosystem services provided by bats. Ann. N. Y. Acad. Sci. 1223, 1–38. doi: 10.1111/j.1749-6632.2011.06004.x
Lalita, A., and McNeil, J. N. (1998). Predation risk and mating behavior: The responses of moths to bat-like ultrasound. Behav. Ecol. 9, 552–558. doi: 10.1093/beheco/9.6.552
Li, C., Yu, J.-F., Xu, J., Liu, J.-H., and Ye, H. (2012). Reproductive rhythms of the tobacco cutworm, Spodoptera litura (Lepidoptera: Noctuidae). GSTF J. Biosci. 2, 25–29. doi: 10.5176/2251-3140_2.1.18
MacLeod, K. J., Krebs, C. J., Boonstra, R., and Sheriff, M. J. (2018). Fear and lethality in snowshoe hares: The deadly effects of non-consumptive predation risk. Oikos 127, 375–380. doi: 10.1111/oik.04890
Malone, K. M., Powell, A. C., Hua, F., and Sieving, K. E. (2017). Bluebirds perceive prey switching by Cooper’s hawks across an urban gradient and adjust reproductive effort. Écoscience 24, 21–31. doi: 10.1080/11956860.2017.1346449
McCauley, S. J., Rowe, L., and Fortin, M.-J. (2011). The deadly effects of “nonlethal” predators. Ecology 92, 2043–2048. doi: 10.1890/11-0455.1
Mitchell, M. D., and Harborne, A. R. (2020). Non-consumptive effects in fish predator–prey interactions on coral reefs. Coral Reefs 39, 867–884. doi: 10.1007/s00338-020-01920-y
Monaghan, P., Metcalfe, N. B., and Torres, R. (2009). Oxidative stress as a mediator of life history trade-offs: Mechanisms, measurements and interpretation. Ecol. Lett. 12, 75–92. doi: 10.1111/j.1461-0248.2008.01258.x
Moore, M. P., Lis, C., and Martin, R. A. (2018). Larval body condition regulates predator-induced life-history variation in a dragonfly. Ecology 99, 224–230. doi: 10.1002/ecy.2056
Mukherjee, S., Heithaus, M. R., Trexler, J. C., Ray-Mukherjee, J., and Vaudo, J. (2014). Perceived risk of predation affects reproductive life-history traits in Gambusia holbrooki, but not in Heterandria formosa. PLoS One 9:e88832. doi: 10.1371/journal.pone.0088832
Nakano, R., Ito, A., and Tokumaru, S. (2022). Sustainable pest control inspired by prey-predator ultrasound interactions. Proc. Natl. Acad. Sci. U. S. A. 119:e2211007119. doi: 10.1073/pnas.2211007119
Okuzaki, Y. (2021). Effects of body size divergence on male mating tactics in the ground beetle Carabus japonicus. Evolution 75, 2269–2285. doi: 10.1111/evo.14302
Parthasarathy, R., Sun, Z., Bai, H., and Palli, S. R. (2010). Juvenile hormone regulation of vitellogenin synthesis in the red flour beetle, Tribolium castaneum. Insect. Biochem. Mol. Biol. 40, 405–414. doi: 10.1016/j.ibmb.2010.03.006
Peacor, S. D., Barton, B. T., Kimbro, D. L., Sih, A., and Sheriff, M. J. (2020). A framework and standardized terminology to facilitate the study of predation-risk effects. Ecology 101:e03152. doi: 10.1002/ecy.3152
Peacor, S. D., Dorn, N. J., Smith, J. A., Peckham, N. E., Cherry, M. J., Sheriff, M. J., et al. (2022). A skewed literature: Few studies evaluate the contribution of predation-risk effects to natural field patterns. Ecol. Lett. 25, 2048–2061. doi: 10.1111/ele.14075
Peckarsky, B. L., Abrams, P. A., Bolnick, D. I., Dill, L. M., Grabowski, J. H., Luttbeg, B., et al. (2008). Revisiting the classics: Considering nonconsumptive effects in textbook examples of predator-prey interactions. Ecology 89, 2416–2425. doi: 10.1890/07-1131.1
Peckarsky, B. L., Cowan, C. A., Penton, M. A., and Anderson, C. (1993). Sublethal consequences of stream-dwelling predatory stoneflies on mayfly growth and fecundity. Ecology 74, 1836–1846. doi: 10.2307/1939941
Plantevin, G., De Reggi, M., and Nardon, C. (1984). Changes in ecdysteroid and juvenile hormone titers in the hemolymph of Galleria mellonella larvae and pupae. Gen. Comp. Endocrinol. 56, 218–230. doi: 10.1016/0016-6480(84)90034-0
Rodríguez-San Pedro, A., Allendes, J. L., Beltrán, C. A., Chaperon, P. N., Saldarriaga-Córdoba, M. M., Silva, A. X., et al. (2020). Quantifying ecological and economic value of pest control services provided by bats in a vineyard landscape of Central Chile. Agric. Ecosyst. Environ. 302:107063. doi: 10.1016/j.agee.2020.107063
Rosier, R., and Langkilde, T. (2011). Behavior under risk: How animals avoid becoming dinner. Nat. Educ. Knowl. 2:8.
Roux, O., Renault, D., Mouline, K., Diabaté, A., and Simard, F. (2021). Living with predators at the larval stage has differential long-lasting effects on adult life history and physiological traits in two anopheline mosquito species. J. Insect Physiol. 131:104234. doi: 10.1016/j.jinsphys.2021.104234
Rubin, J. J., Hamilton, C. A., McClure, C. J., Chadwell, B. A., Kawahara, A. Y., and Barber, J. R. (2018). The evolution of anti-bat sensory illusions in moths. Sci. Adv. 4:eaar7428. doi: 10.1126/sciadv.aar7428
Sandhyarani, K., and Rani, P. U. (2013). Morphometric and developmental consequences in Spodoptera litura due to feeding on three varieties of sweet potato plants (Ipomoea batata). Phytoparasitica 41, 317–325. doi: 10.1007/s12600-013-0292-2
Santos, C. G., Humann, F. C., and Hartfelder, K. (2019). Juvenile hormone signaling in insect oogenesis. Curr. Opin. Insect. Sci. 31, 43–48. doi: 10.1016/j.cois.2018.07.010
Schmitz, O. J., Rosenblatt, A. E., and Smylie, M. (2016). Temperature dependence of predation stress and the nutritional ecology of a generalist herbivore. Ecology 97, 3119–3130. doi: 10.1002/ecy.1524
Schnitzler, H. U., Moss, C. F., and Denzinger, A. (2003). From spatial orientation to food acquisition in echolocating bats. Trends Ecol. Evol. 18, 386–394. doi: 10.1016/S0169-5347(03)00185-X
Schwenke, R. A., Lazzaro, B. P., and Wolfner, M. F. (2016). Reproduction-immunity trade-offs in insects. Annu. Rev. Entomol. 61, 239–256. doi: 10.1146/annurev-ento-010715-023924
Sheriff, M. J., Peacor, S. D., Hawlena, D., and Thaker, M. (2020). Non-consumptive predator effects on prey population size: A dearth of evidence. J. Anim. Ecol. 89, 1302–1316. doi: 10.1111/1365-2656.13213
Sih, A., Crowley, P., McPeek, M., Petranka, J., and Strohmeier, K. (1985). Predation, competition, and prey communities: A review of field experiments. Annu. Rev. Ecol. Syst. 16, 269–311. doi: 10.1146/annurev.es.16.110185.001413
Soller, M., Bownes, M., and Kubli, E. (1999). Control of oocyte maturation in sexually mature drosophila females. Dev. Biol. 208, 337–351. doi: 10.1006/dbio.1999.9210
Sun, C., Jiang, T., Kanwal, J. S., Guo, X., Luo, B., Lin, A., et al. (2018). Great Himalayan leaf-nosed bats modify vocalizations to communicate threat escalation during agonistic interactions. Behav. Process. 157, 180–187. doi: 10.1016/j.beproc.2018.09.013
Süren-Castillo, S., Abrisqueta, M., and Maestro, J. L. (2012). FoxO inhibits juvenile hormone biosynthesis and vitellogenin production in the German cockroach. Insect Biochem. Mol. Biol. 42, 491–498. doi: 10.1016/j.ibmb.2012.03.006
Svensson, G. P., Skals, N., and Lofstedt, C. (2003). Disruption of the odour-mediated mating behaviour of Plodia interpunctella using high-frequency sound. Entomol. Exp. Appl. 106, 187–192. doi: 10.1046/j.1570-7458.2003.00022.x
Thaler, J. S., McArt, S. H., and Kaplan, I. (2012). Compensatory mechanisms for ameliorating the fundamental trade-off between predator avoidance and foraging. Proc. Natl. Acad. Sci. U. S. A. 109, 12075–12080. doi: 10.1073/pnas.1208070109
Truman, J. W., and Riddiford, L. M. (2002). Endocrine insights into the evolution of metamorphosis in insects. Annu. Rev. Entomol. 47, 467–500. doi: 10.1146/annurev.ento.47.091201.145230
van Uitregt, V. O., Hurst, T. P., and Wilson, R. S. (2012). Reduced size and starvation resistance in adult mosquitoes, Aedes notoscriptus, exposed to predation cues as larvae. J. Anim. Ecol. 81, 108–115. doi: 10.1111/j.1365-2656.2011.01880.x
Venkanna, Y., Suroshe, S. S., and Dahuja, A. (2021). Non-consumptive effects of the zigzag ladybird beetle, Cheilomenes sexmaculata (fab.) on its prey, the cotton aphid, Aphis gossypii glover. Biocontrol Sci. Tech. 31, 1204–1219. doi: 10.1080/09583157.2021.1937521
Villalobos-Sambucaro, M. J., Nouzova, M., Ramirez, C. E., Eugenia Alzugaray, M., Fernandez-Lima, F., Ronderos, J. R., et al. (2020). The juvenile hormone described in Rhodnius prolixus by Wigglesworth is juvenile hormone III skipped bisepoxide. Sci. Rep. 10:3091. doi: 10.1038/s41598-020-59495-1
Wilson, D. E., and Reeder, D. M. (2005). Mammal species of the world: A taxonomic and geographic reference. Maryland, USA: JHU Press.
Wu, C. X., Liu, J. F., Di, X. Y., and Yang, M. F. (2018). Delay in mating reduces Reproductivity but increases life span in tobacco cutworm, Spodoptera litura Fabricius (Lepidoptera: Noctuidae). J. Econ. Entomol. 111, 1650–1657. doi: 10.1093/jee/toy108
Xiao, H.-Y., Li, G.-C., Wang, Z.-Q., Guo, Y.-R., and Liu, N.-Y. (2021). Combined transcriptomic, proteomic and genomic analysis identifies reproductive-related proteins and potential modulators of female behaviors in Spodoptera litura. Genomics 113, 1876–1894. doi: 10.1016/j.ygeno.2021.04.006
Xiong, X., Michaud, J. P., Li, Z., Wu, P., Chu, Y., Zhang, Q., et al. (2015). Chronic, predator-induced stress alters development and reproductive performance of the cotton bollworm, Helicoverpa armigera. BioControl 60, 827–837. doi: 10.1007/s10526-015-9689-9
Zamzow, J. P., Amsler, C. D., McClintock, J. B., and Baker, B. J. (2010). Habitat choice and predator avoidance by Antarctic amphipods: The roles of algal chemistry and morphology. Mar. Ecol. Prog. Ser. 400, 155–163. doi: 10.3354/meps08399
Zanette, L. Y., White, A. F., Allen, M. C., and Clinchy, M. (2011). Perceived predation risk reduces the number of offspring songbirds produce per year. Science 334, 1398–1401. doi: 10.1126/science.1210908
Zha, Y.-P., and Lei, C.-L. (2012). Effects of ultrasound-stress on antioxidant enzyme activities of Helicoverpa Armigera (Hübner) (Lepidoptera: Noctuidae). J. Agric. Urban Entomol. 28, 34–41. doi: 10.3954/1523-5475-28.1.34
Zha, Y. P., Wu, X. L., Zhang, Z. Y., Chen, J. Y., and Chen, Q. C. (2021). Influence of ultrasound on juvenile hormone titers in Monochamus alternatus Hope (Coleoptera: Cerambycidae). Sci. Rep. 11:1450. doi: 10.1038/s41598-021-81227-2
Zhang, C. M., Sun, C. N., Lucas, J. R., Feng, J., and Jiang, T. L. (2022). Acoustic signal dominance in the multimodal communication of a nocturnal mammal. Curr. Zool. 68, 592–599. doi: 10.1093/cz/zoab089
Keywords: predation risks, bats, moths, non-consumptive effects, biological control
Citation: Zhang W, Liu Y, Wang Z, Lin T, Feng J and Jiang T (2023) Effects of predation risks of bats on the growth, development, reproduction, and hormone levels of Spodoptera litura. Front. Ecol. Evol. 11:1126253. doi: 10.3389/fevo.2023.1126253
Edited by:
Ann Valerie Hedrick, University of California, Davis, United StatesReviewed by:
Astrid T. Groot, University of Amsterdam, NetherlandsAlex Baranowski, University of Rhode Island, United States
Copyright © 2023 Zhang, Liu, Wang, Lin, Feng and Jiang. This is an open-access article distributed under the terms of the Creative Commons Attribution License (CC BY). The use, distribution or reproduction in other forums is permitted, provided the original author(s) and the copyright owner(s) are credited and that the original publication in this journal is cited, in accordance with accepted academic practice. No use, distribution or reproduction is permitted which does not comply with these terms.
*Correspondence: Jiang Feng, fengj@nenu.edu.cn; Tinglei Jiang, jiangtl730@nenu.edu.cn