- 1College of Forestry, Fujian Agriculture and Forestry University, Fuzhou, China
- 2Chinese Fir Engineering Technology Research Center of the State Forestry and Grassland Administration, Fujian Agriculture and Forestry University, Fuzhou, Fujian, China
- 3Fujian Shanghang Baisha Forestry Farm, Shanghang, Fujian, China
Introduction: The longitudinal section cell morphology of Chinese fir roots was studied to better understand the relationship between root structure and root function.
Materials: In this study, the adjusted microwave paraffin section method and the selected two sample transparency methods were used to process the Chinese fir roots and combined with the laser scanning confocal microscopy (LSCM) technique, the morphology of Chinese fir roots longitudinal section can be clearly observed in a short time. At the same time, the observation effect of the longitudinal section cell morphology of the LSCM image of the thick section of the Chinese fir roots and the ordinary optical imaging of the thin section was analyzed and compared.
Results and Discussion: The results showed that: (1) There were apparent differences in the observation effect of cell morphology in longitudinal sections of Chinese fir roots obtained using various treatment methods. Under LSCM, the section with a thickness of 20 μm generated by the microwave paraffin section technique displayed complete cell morphology and clear structure in the root cap, meristem zone, and elongation zone. The overall imaging effect was good; the thickness was 0.42–1.01, 0.64–1.57, and 0.95–2.71 mm, respectively. The cell arrangement in maturation zone cells was more regular. (2) Compared to the ordinary optical imaging of thin sections, the thick sections of roots made by the microwave paraffin section method shortened the time to obtain high-quality sections to ensure the observation effect. Therefore, adopting the microwave paraffin cutting approach to produce thicker root sections under LSCM allows for rapid observation of the cell morphology in longitudinal sections of Chinese fir roots. The current study provides the efficient operation procedure for the microscopic observation technology of the longitudinal section of Chinese fir roots, which is not only beneficial to reveal the relationship between the root structure and function from the microscopic point of view but also provides a technical reference for the anatomical study of other organs and the observation of the longitudinal section cell morphology of plant roots with similar structural characteristics.
1. Introduction
Roots are critical functional organs for plants to uptake, transport, and store soil moisture and nutrients. It can respond to the combined effects of genetic characteristics and external environmental factors by regulating or changing its structural characteristics and morphological plasticity (Cabrera et al., 2022). The root cell morphology is the direct reflection of its development level. Its morphological structure can induce the tropism of root growth and extension and play an essential role in the growth and development of new roots (Hayashi et al., 2013). In addition, microstructural changes such as the thickening of the endodermis cell wall and suberification of the radial wall in the maturation zone of roots had significant effects on the selection of nutrients absorbed by roots (Scacchi et al., 2010; Yao et al., 2020).
At present, free-hand and paraffin sections are the widely used methods to observe the root anatomical structure. The hand-cut cross-section method is simple to operate, does not require fixation, dehydration, paraffin-immersion, and embedding, and is not treated with chemical reagents, which can significantly maintain the natural color and shape of the cells. However, when it is used to make the anatomical structure of longitudinal sections of the root system, the thickness is not well controlled, and the incision level cannot be guaranteed. This can easily damage the cell tissue structure (Wu et al., 2018). On the other hand, the paraffin section method uses paraffin to support cell tissue and turn it into a clear sheet, making it easy to observe changes in cell morphological characteristics (Mrak et al., 2021). However, there are often different degrees of bending in the development of longitudinal sections of the anatomical structure of the root system, particularly the root system of trees. The paraffin section technique makes it difficult to maintain cell alignment in longitudinal sections, leading to incomplete cell tissue structure (Wang et al., 2019). Additionally, the production procedure for this method is time-consuming, and the chemical reagents utilized in the sample processing and dyeing process can be dangerous to human health.
Researchers have optimized and improved every aspect of the paraffin section process, from selecting sectioning tools to the reagents used. Currently, the microwave paraffin section method, which uses microwave radiation heating to make polar molecules move at high speed to increase cell permeability and solvent molecule penetration, has become an effective method to shorten the pre-treatment time of paraffin section samples (Schichnes et al., 2001). The leaf anatomical structures of Setaria italica (Zhang et al., 2015), Arabidopsis thaliana (Inada and Wildermuth, 2005) and other plants produced by this method observed clear cell structures. Although the microwave paraffin section method can significantly shorten the pre-treatment time of samples, the later dyeing procedure is the same as the traditional paraffin section method. This process is cumbersome and has a low yield of excellent sections (Inada and Wildermuth, 2005; Wei et al., 2011).
The laser scanning confocal microscope (LSCM) is widely used in plant cytology, development, and histochemistry studies. It is based on fluorescence microscope imaging with a laser scanning device connected to a computer and related application software (Hasegawa, 2006; Wang et al., 2021). It has been used to observe the cell morphology of the roots’ longitudinal section of plants such as Pisum sativum (Ropitaux et al., 2019) and Camellia sinensis (Sun et al., 2020). This provides a solution for optimizing the microwave paraffin section dyeing process. However, when the roots samples’ thickness and hardness are more, light is challenging to penetrate the inside of the roots.
Consequently, the cell image on the computer will not be clear, the internal organizational structure will be blurred, and the complete cell morphology cannot be fully displayed. Due to lignin deposition, unequal hardness of each section of the tissue, and a high degree of lignification, it is not easy to detect a strong enough fluorescence signal inside the root system, particularly in trees (Barros-Rios et al., 2015). This has made it extremely difficult to clearly observe tree root cell morphology clearly. To meet the observation requirements under the LSCM, it is essential to appropriately transparent the tree root samples or to reduce their thickness.
Chinese fir [Cunninghamia lanceolata (Lamb) Hook] is an important timber tree species in southern China. Its roots have the characteristics of high lignification, large and uneven hardness in various parts. This study aimed to establish a method for precise and clearer observation of microscopic cell morphology in the longitudinal section of Chinese fir roots in a short time. This method is of great significance for improving the production efficiency of the longitudinal section anatomical structure of Chinese fir root samples and further understanding its growth characteristics. We hypothesized that the observed effect of Chinese fir root samples obtained by different treatment methods would differ. And compared to the transparent treatment of Chinese fir root samples directly, the effect of making root slices under the microscope would be more apparent and complete.
2. Materials and methods
2.1. Plant materials, experimental reagents, and apparatus
The plant seedlings of Chinese fir were from the 1-year-old No. 25 clone cultivated by the Chinese Fir Engineering Technology Research Center of the State Forestry and Grassland Administration. Late in July 2021, 60 young Chinese fir roots 5–7 cm long, well-grown, and free of pests and diseases were randomly selected and stored in formalin-acetic acid-absolute ethyl alcohol (FAA) for later use (Figure 1).
Experimental reagents include FAA (Formalin: Acetic acid: 70% Absolute ethanol = 1:1:18, v/v/v), PBS (0.1 mol·L−1 Phosphate Buffer Solution, pH = 7.2), PI (0.05 mg·L−1 Propidium Iodide, 0.1 mol·L−1 PBS was prepared), Safranine O-Fast green, TBA (Tert butyl alcohol), Benzyl benzoate, Benzyl alcohol, Xylene, Chloroform, and Absolute ethanol. Experimental apparatus includes (1) ultra-high resolution and sensitivity laser confocal microscope (Carl Zeiss LSM 880, Oberkochen, Germany), (2) upright biological microscope (Nikon ECLIPSE E100, Tokyo, Japan), (3) full automatic rotary microtome (Leica RM 2265, Hessian, Germany), (4) Microwave [Galanz P70F23P-G5 (S0), Foshan, China], and (5) Diaphragm vacuum pump (Yuhua YH-500, Gongyi, China).
2.2. Methods for treatment of Chinese fir roots sample
The methods used in treating Chinese fir root samples are shown in Table 1, and the process flow chart is shown in Figure 2. The Chinese fir root samples were cut into 0.5 ± 0.1 cm long segments with a sharp blade and placed in a 10 ml centrifuge tube filled with FAA. Complete six segments for each method following the steps, ① fixed: centrifugal tubes containing Chinese fir root samples and FAA placed in a vacuum pump, vacuumized three times (pressure than 0.08 MPa), 10 min at a time, each time with a new fixative, after vacuuming, the fixative was replaced again and kept at room temperature for 12 h to fully fixation the material; ② dehydrated: After the fixation was completed, the fixative was sucked out, and gradient dehydration was performed according to the alcohol concentration of 70, 80, 90, 95, and 100%. Each gradient was treated for 45–60 min, and transparent treatment was performed after dehydration. Due to the different hardness and thickness of different plant tissue materials, the selection of transparent reagents and the duration needed for treatment had to be adjusted. This study made different transparent treatment schemes for Chinese fir root samples based on their lignification, hardness, and thickness. These schemes were based on traditional transparent treatment methods (Table 1, methods A, B, C, and D) to filter out the transparent treatment methods suitable for Chinese fir root samples. The dyeing was done following the transparency technique.
Method E treats Chinese fir root samples by microwave paraffin section after adjustment. The root samples were put in a centrifuge tube with FAA and pumped with a vacuum pump for 20 min. The fixative was then placed in a beaker with distilled water, set in a microwave, and set to heat preservation when the water temperature reached 70°C. The root samples were then placed in FAA and microwaved 3–4 times, each for 15 min. For dehydration, root samples were placed in 60% absolute ethanol, left at room temperature for 10 min, then placed in a mixture of absolute ethanol and TBA (1:1, v/v) and microwaved for 15 min. For transparency, root samples were placed in pure TBA and treated twice using a microwave for 15 min at each trial. For paraffin immersion, the root samples were placed in TBA: paraffin (1: 1, v/v) and microwaved once for 15 min. Following that, the new pure paraffin was replaced. The microwave treatment was repeated four times, each time for 15 min, to ensure that the paraffin was well absorbed in the root samples. The entire microwave treatment process maintained the water temperature at 70°C. Finally, it was embedded in paraffin. The rotary microtome was used in sections with different thicknesses (10 m, 20 m) to compare the imaging effect of thick sections under LSCM and conventional thin sections under an ordinary optical microscope. After sectioning, the paraffin tape was wrapped, dewaxed, and rehydrated (Table 2). Finally, the dyeing treatment was applied to the material.
2.3. Dyeing treatment
The dyeing procedures for PI and the other three conventional reagents (Safranine O-Fast green, Toluidine blue, and Hematoxylin) are shown in Table 3. In this study, the PI fluorescent dye was utilized for staining by the characteristics of the fluorescence signal of the tissue sample received by the LSCM. The prepared root samples and sections (20 μm) were placed in PI for staining at room temperature in the dark. The remaining PI was washed away by rinsing them thoroughly in PBS. Safranine O-fast green was used to dye the thickness sections (10 m) simultaneously.
2.4. Observation and photography
The stained root samples and sections (20 μm) were observed and photographed by LSCM. The excitation light wavelength was 561 nm. Chinese fir root samples were scanned layer by layer at a thickness of 4 m until the entire root sample was scanned. Simultaneously, the ScopeImage 9.0 (Nanjing, China) software was used to measure the cell morphological parameters of the functional areas (root cap, meristem zone, and elongation zone) of the longitudinal section of the Chinese fir root. The thickness sections (10 μm) were observed and photographed using an upright biological microscope.
3. Results
3.1. Observed effect of LSCM
The conventional transparent treatment methods are difficult to apply to the root tissue samples of Chinese fir. In this experiment, the transparent treatment scheme of Chinese fir root tissue samples was screened according to the characteristics of tree roots. The results showed that the transparency effect of method A (Table 1) was poor when the transparent treatment time was only 180 min. This was because tissue samples were not utterly transparent because of the short transparent treatment time. Longer treatment times with absolute ethanol and xylene and chloroform combined displacement (methods C and D in Table 1) resulted in severe hardening of Chinese fir root tissue and even shrinkage deformation. Finally, the root samples of Chinese fir treated with Method A (Treatment time 240 min) and Method B transparent treatment, which had better maintenance of original root morphology, were subjected to PI dyeing (Table 1).
The test samples were treated with the above two transparent methods A (Treatment time 240 min) and B. The results significantly differed when the LSCM was used (Figure 3). When the fixation and dehydrated steps were the same, after treatment with benzyl benzoate and benzyl alcohol as transparent reagents (Table 1, Method A, Treatment time 240 min), the fluorescence brightness of the root surface edge of Chinese fir was more potent than that of the root middle area. Still, no clear cell morphology was observed (Figures 3a1,a4). As the number of scanning layers increased by 3–5 layers, the apical and non-apical portions moved closer to the root center area, and the fluorescence intensity darkening range gradually increased (Figures 3a1–a7). Still, the clear cell morphology of the root’s longitudinal section was not observed, indicating that the transparency of Chinese fir root samples failed to meet the observation requirements of LSCM.
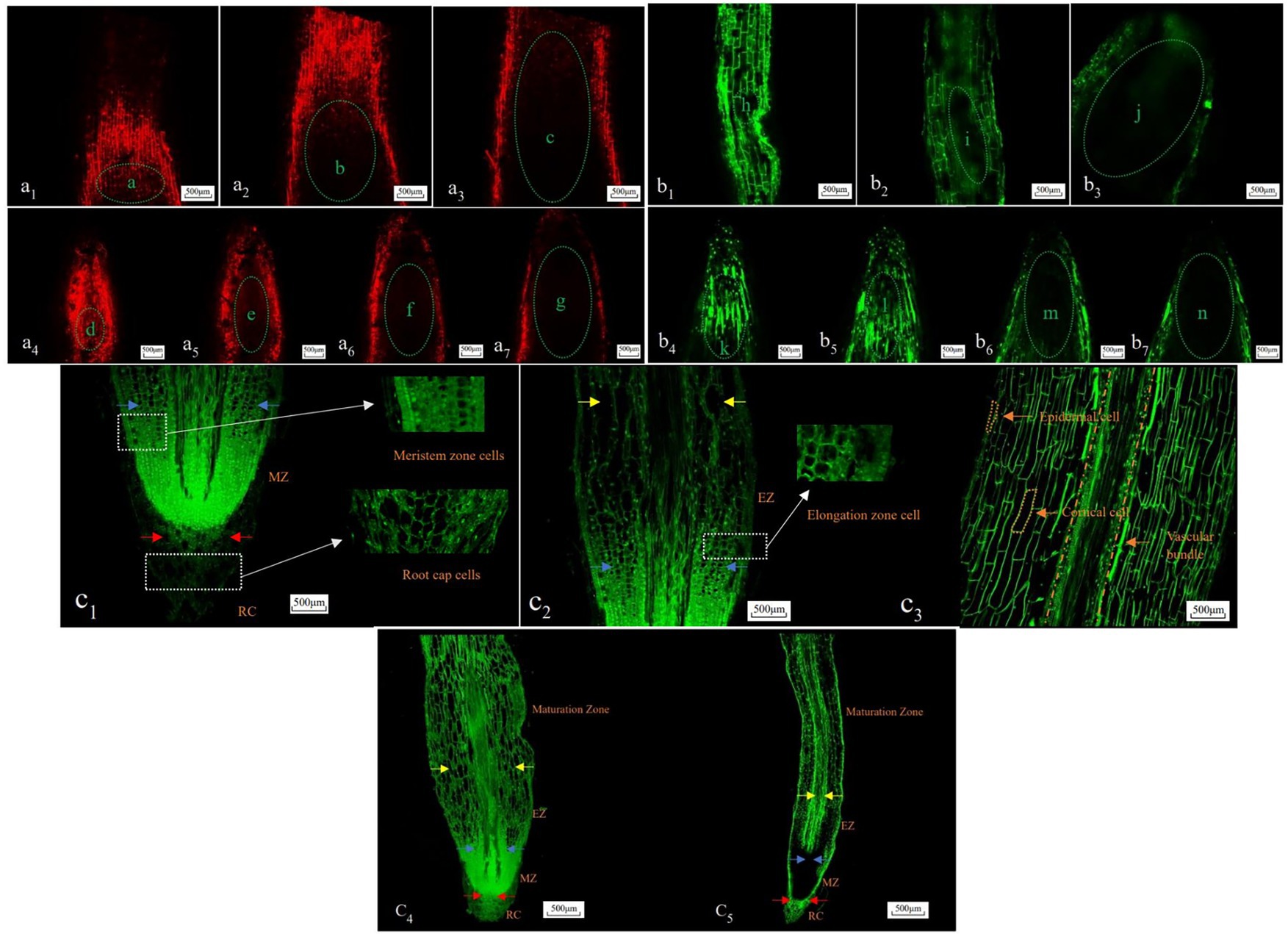
Figure 3. Longitudinal section cell morphology of Chinese fir roots under laser scanning confocal microscopy (LSCM) technique. a1–a7, b1–b7, c1–c5: Bar = 500 μm. a1–a3 and b1–b3: non-apical portion, a4–a7 and b4–b7 apical portion, a–n: middle root zone, c1–c3: longitudinal section anatomical structure of Chinese fir roots in microwave paraffin sections method, c4,c5: Vertical and bending longitudinal section anatomical structure of Chinese fir roots in microwave paraffin sections method (×10). RC, root cap; MZ, meristem zone; EZ, elongation zone; c3 and above the elongation zone is the maturation zone, the red arrow represents the upper boundary of the root cap, the blue arrow represents the upper boundary of the dividing zone, and the yellow arrow represents the upper boundary of the elongation zone.
When absolute ethanol and xylene were used as transparent reagents (Table 1, Method B), it was observed that under the same excitation light source conditions, compared with the apical portion surface, the longitudinal rectangular cell morphology in the edge area of the non-apical portion surface was clearly visible. Still, the complete and clear cell morphology was not observed in the middle area (Figures 3b1,b4). Similarly, when 3–5 layers increased the number of scanning layers, the fluorescence intensity of both the apical and non-apical portions gradually decreased, and the darkening range gradually increased (Figures 3b1–b7). This indicates that the Chinese fir root samples treated with this transparent reagent could not achieve the transparency observed under the LSCM.
Compared with the above two transparent processing methods, the anatomical structure made by the adjusted microwave paraffin section method (Table 1, Method E) can show the clear cell morphology of the longitudinal section of the Chinese fir roots under the LSCM (Figures 3c1–c5). The root’s functional areas, such as the root cap, meristem zone, elongation zone, and maturation zone, were clearly distinguishable. The size of the root cap cells was conical, the overall shape was irregular, and the arrangement was loose. The thickness of this functional area was 0.42–1.01 mm. A dome-shaped boundary distinguishes the root cap and meristem zone. The thickness of the meristem was 0.64–1.57 mm. The elongation zone moved to the maturation zone, the larger the individual cells were in rapid elongation, and the longitudinal size gradually increased (Figures 3c1,c2). This functional zone was between 0.95 and 2.71 mm thick. The maturation zone was above the elongation zone. The cells in the maturation zone were regular rectangles; the individual cells were alike and can be seen in the epidermal and cortical structures of the tissue (Figures 3c3). In summary, this method can more clearly observe the cell morphology of the longitudinal section of Chinese fir roots than the transparent treatment of the sample.
3.2. Comparison of LSCM image and ordinary optical microscopy image
There are differences in the imaging effect of different thickness sections made by the microwave paraffin section method (Figure 4). The sections (10 μm thick) were better observed under an upright biological microscope. The cell morphology of the root cap, meristem zone, elongation zone, and maturation zone was identified (Figures 4d1,d2). The sections (20 μm thick) had intact morphology and high identification of apical and non-apical portion cells under LSCM (Figures 4e1,e2), and the functional areas of cells were clearly visible. As a result, clear cell morphology can be observed by LSCM and ordinary optical microscope for the longitudinal anatomical structure of Chinese fir roots with section thicknesses of 20 and 10 μm, respectively, in terms of imaging effect.
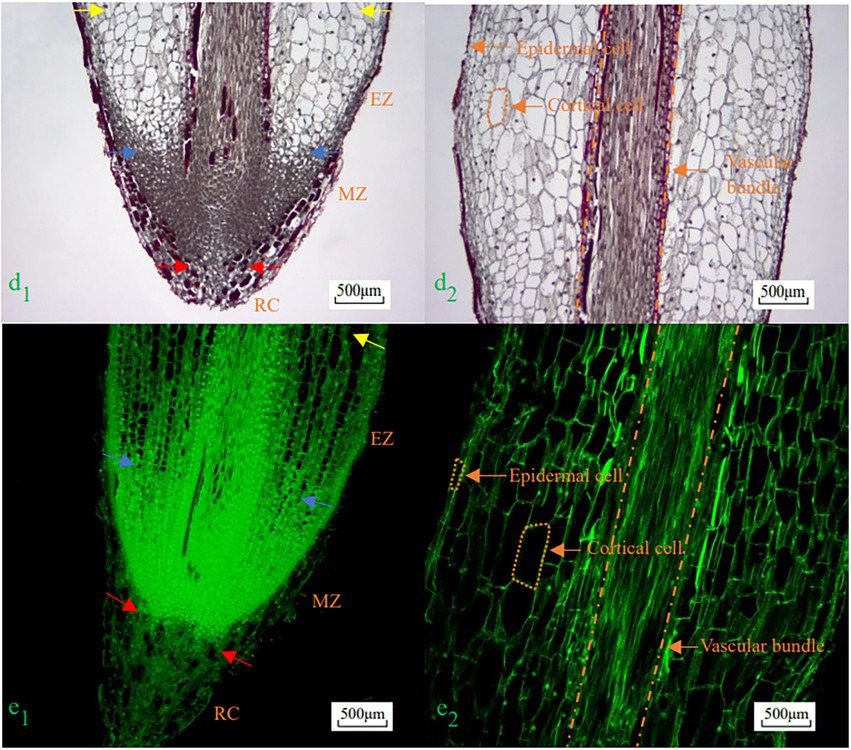
Figure 4. Morphological imaging effect of Chinese fir roots longitudinal section cells with different slice thickness. d1,d2, e1,e2: Bar = 500 μm, d1,e1: apical portion, d2,e2: non-apical portion, d1,d2: upright biological microscope, e1,e2: LSCM (×10). RC, root cap; MZ, meristem zone; EZ, elongation zone; d2,e2 respectively is the maturation zone, the red arrow represents the upper boundary of the root cap, the blue arrow represents the upper boundary of the dividing zone, and the yellow arrow represents the upper boundary of the elongation zone.
3.3. Comparison of treatment methods of Chinese fir roots sample
Significant differences exist in the processing time and operation steps of the cell morphology observation of different Chinese fir root’s longitudinal sections (Table 4). The two transparent treatment procedures (Table 4, Methods A and B) go through four processes in 20 ~ 22 h for the same fixation and dehydration steps. Combined with the reagent treatment method of root samples in Table 1, it can be seen that Method A (Treatment time 240 min) has a less specific operation process than method B, however, the observation effect of method B is more ideal (Table 4). When the root samples were treated with the microwave paraffin section method (Table 4, Method E), the number of procedures was increased to 9. The complete and clear cell morphology of the longitudinal section of Chinese fir roots was observed in the anatomical structures of different thickness sections. However, under the same observation effect, the production of roots anatomical structure with a thickness of 20 μm combined with LSCM has fewer steps. It takes the least time, only 4–5 h, significantly improving production efficiency.
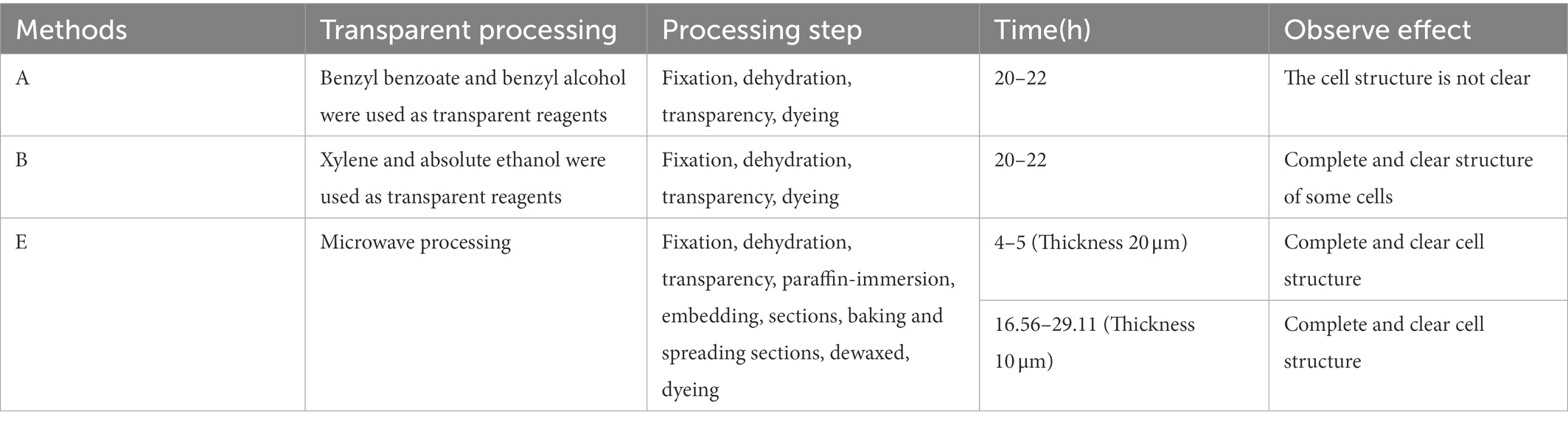
Table 4. Comparison of the observation preparation methods and effects of longitudinal section cell morphology of Chinese fir roots.
4. Discussion
The radial thickening growth stage of tree roots accumulates a considerable amount of lignin, and the degree of lignification is becoming increasingly evident; its cell wall is more solid than crop roots. The conventional paraffin section method requires the plant tissue to be softened for an extended period to resolve the sample’s high density, hardness, and lignin content. However, the long-term softening treatment can easily cause the tissue to become brittle, and the cells appear empty or broken after sectioning (Moreno-Sanz et al., 2020). On the other hand, microwave paraffin sectioning can drastically reduce sample processing time. However, different plant organs perform different functions in the environment, the degree of lignification varies, and the penetration rate and effect of extracellular solution vary. For example, under low-temperature stress, the content of soluble phenols in wheat (Triticum aestivum L.) roots decreased while the content of lignin increased, and the opposite trend was observed in leaf organs (Olenichenko and Zagoskina, 2005). Due to this, the microwave paraffin section method’s final observation results were somewhat highly unclear. To achieve this, LSCM can directly monitor the samples with fluorescent labelling and sufficient transparency without a prolonged softening treatment, considerably reducing the risk of sample damage.
In the current study, Chinese fir root samples were made transparent using benzyl benzoate and benzyl alcohol, absolute ethanol and xylene, absolute ethanol, and chloroform as transparent reagents for different amounts of time. Methods A (Treatment time 240 min) and B did not show root hardening and deformation after treatment. However, under the LSCM, as the number of scanning layers increased, the root fluorescence signal intensity gradually decreased, and the internal cell morphology could not be clearly shown. This may be due to the natural growth and development of the roots of Chinese fir after the completion of primary growth. The oxidative polymerization of lignin monomers in the secondary xylem forms lignin and deposits on the cell wall. The lignification degree of most fine roots was also very high, and it gets firmer as it moves from the meristem zone to the root axis (Barros-Rios et al., 2015; Song et al., 2019). Even though the clear reagent could get through some of the surface cell tissue, it could not get through the more woody cells inside the root system. Finally, the Chinese fir’s root system wasn’t transparent enough, which led to pigment absorption and light scattering by cytoplasmic components. The LSCM did not detect a strong enough fluorescence signal throughout the observation; hence a clear cell structure was not observed (Donaldson et al., 2001). It can be seen that the directly transparent treatment of Chinese fir roots with high lignification and hardness makes it difficult to clealry observe root cell morphology under the LSCM. The types of transparent reagents and treatment time can be further explored.
Our study used the microwave paraffin section method to process tissue samples quickly and the laser scanning confocal microscope to scan and analyze samples point by point and layer by layer. As a result of extensive experimentation, a production method was devised that provided clear observation of cell morphology in a longitudinal section of the root system of the Chinese fir. Under a laser scanning confocal microscope, the sections produced with this technique generate clear and structurally complete images of cell morphology. This study used sections with a thickness of about 20 μm. The laser scanning confocal microscope was used to observe the optical “sections.” To a certain extent, this study avoids the problem of aligning the longitudinal sections of partially curved roots after making them. This can effectively replace the mechanical sections and clearly observe the complete cell morphology of the longitudinal sections of Chinese fir roots.
Moreover, the anatomical structure of Chinese fir roots with a 10 μm thick section provided an excellent optical microscopic imaging effect and a complete structure. Clear structures of different functional areas of Chinese fir roots were observed under a microscope. However, it is easy to cause tissue material damage and force the original cell morphology to change by moving back and forth often when using this method to make sections (Barbosa et al., 2010). The serious lignification of Chinese fir roots greatly affected the light penetration and had a certain effect on the good color of the late dyeing solution (Barros-Rios et al., 2015). Furthermore, the dyeing process for safranine O-fast green dyeing solution and other conventional dyeing reagents requires multiple replacement solutions and moving sections, which can easily lead to tissue material shedding. An increase in xylene treatment steps on the final observation effect also has a certain impact. As a result, while creating the anatomical structure of the Chinese fir root system, utilizing a thicker slice and PI staining combined with the characteristics of LSCM can considerably reduce the possible damage of conventional paraffin sections during the sectioning and dyeing process. This method can ensure the observation effect and significantly shorten the production time. Moreover, conventional paraffin sectioning frequently uses safranine O-fast green, toluidine blue, and hematoxylin sections. However, these procedures are laborious and often include the use of chemical reagents, particularly xylene odor stimulation, a suspected carcinogen that is volatile. As a result, the integrity of the cell structure can easily be damaged (Premalatha et al., 2013).
As an ethidium bromide analogue, PI emits red fluorescence after being incorporated into double-stranded DNA. Depending on the excitation light source, it can dye both living and dead cells simultaneously. It is a regularly used cell fluorescent dye. It is frequently employed in the observation and study of the life activities of plant tissues and organs, such as herbaceous plant root hairs and pollen tubes. It can cause the observed samples to have strong fluorescence properties (Rounds et al., 2011; Kirchhoff and Cypionka, 2017). In this study, Chinese fir root samples were dyed with PI. Compared to the conventional paraffin section dyeing method, the process was reduced to four steps and only 16 min of processing time. The use of toxic chemical reagents was effectively reduced and decreased the harmful effect on the operators. The results also indicated that PI could be used as a good fluorescent dye in the morphological observation of Chinese fir root cells. Also, the PI dyeing process does not require the slice to be moved back and forth many times, which reduces the risk of tissue material shedding to some degree. At the same time, the combination of absolute ethanol and TBA was used as a dehydrating agent in the process of dehydrating root samples by microwave paraffin sectioning. TBA is a widely used dehydrating agent. It has a clear effect and does not make it easy to shrink or harden cell tissue (Baskin et al., 2014). After the treatment, the paraffin-immersion treatment can be performed directly. The process not only reduces the need for organic reagents but also makes the final tissue sample clearer.
Although Chinese fir roots have high lignification and large hardness compared with herbs such as rice (Oryza sativa L.) and A. thaliana, it is not conducive to observing their cell morphology clearly and completely using a single microwave paraffin section method or LSCM. However, combining the two technical characteristics can comprehensively observe the cell morphology in large quantities of Chinese fir roots in a short time. Plant cell research increasingly focuses on three-dimensional structure analysis of plant organs based on optical sections using LSCM three-dimensional reconstruction technology. For example, Hasegawa et al. (2016) used 200 optical sections to construct the three-dimensional structure of A. thaliana flowers and stems. Buda et al. (2016) reconstructed the cuticle of tomato (Solanum lycopersicum cv. M82) fruit using LSCM based on sequential optical sections and performed three-dimensional modeling. However, these techniques are rarely used in studying root growth and development of Chinese fir. The method described in the current study can be used to obtain root slices quickly. It is expected to reconstruct the three-dimensional configuration of the Chinese fir root in space using laser confocal technology to image its cell morphological structure as a whole, allowing for an in-depth study of the interaction between functional areas-environment or structure–function of Chinese fir roots.
In addition, LSCM technology is widely used in the study of plant life activities due to its improvement and perfection in the observation of ion (including Ca2+, pH) changes in plant cells, organelles, and the cytoskeleton, as well as the process of plant growth and development and a particular protein signal transduction pathway (Pozhvanov, 2018). For example, Wang et al. (2016) used LSCM to find that the NO regulates the signal network, including Ca2+, reactive oxygen species, and pH, during pollen tube development of C. sinensis under low-temperature stress. Therefore, compared to other microscopes, the benefits of LSCM in the research of microscopic plant graphics show its vast application potential in investigating the development mechanism of trees’ underground roots. LSCM can be used to learn more about the biology of tree roots. For example, specific fluorescent probes can be used to label root-related hormones that are released when the tree is under stress. LSCM on a computer screen shows clearly how the hormone moves through the root (Li et al., 2016). When combined with related gene technology, the vital mechanism of tree roots’ resistance to stress was also shown.
5. Conclusion
The microwave paraffin section method combined with LSCM can achieve clear observation of the cell morphology of the longitudinal section of Chinese fir roots in a short period of time. The anatomical structure of the longitudinal section of Chinese fir roots with a thickness of 20 μm was made by fixation, dehydration, transparency, and paraffin immersion. After PI dyeing, the tissue structure’s complete and clear cell morphology was observed under an LSCM. To ensure the quality of observation, the production time and staining steps were significantly shortened and reduced. This method can also provide some technical reference for the observation and study of cell morphology of other tree roots longitudinal sections. Still, there are differences in hardness, water content, and maturity of different plants and parts of the same plant. Moreover, the time of microwave treatment needs to be adjusted accordingly to make the tissue complete and not deformed to meet the needs of scientific experiments.
Data availability statement
The original contributions presented in the study are included in the article/supplementary material, further inquiries can be directed to the corresponding author.
Author contributions
PW, XM, JL, XZ, and LL conceived and designed the experiment. LL, TZ, and XL conducted the study. LL and PW wrote the manuscript. PW, XM, and JL reviewed the manuscript and contributed to the discussion. All authors have read and agreed to the published version of the manuscript.
Funding
The study was funded by the National Natural Science Foundation of China (31870614), the Key Program of Natural Science of Fujian Province, China (grant number 2020J01519), and the Science and Technology Project of Fuzhou Science and Technology Bureau, Fujian Province, China (grant number 2021-P-035).
Acknowledgments
We are very grateful to the editors, reviewers and experts who made suggestions for the manuscript.
Conflict of interest
The authors declare that the research was conducted in the absence of any commercial or financial relationships that could be construed as a potential conflict of interest.
Publisher’s note
All claims expressed in this article are solely those of the authors and do not necessarily represent those of their affiliated organizations, or those of the publisher, the editors and the reviewers. Any product that may be evaluated in this article, or claim that may be made by its manufacturer, is not guaranteed or endorsed by the publisher.
References
Barbosa, A. C. F., Pace, M. R., Witovisk, L., and Angyalossy, V. (2010). A new method to obtain good anatomical slides of heterogeneous plant parts. IAWA J. 31, 373–383. doi: 10.1163/22941932-90000030
Barros-Rios, J., Serk, H., Granlund, I., and Pesquet, E. (2015). The cell biology of lignification in higher plants. Ann. Bot. 115, 1053–1074. doi: 10.1093/aob/mcv046
Baskin, T. I., Orr, T. J., Jercinovic, M., and Yoshida, M. (2014). Sample preparation for scanning electron microscopy: the surprising case of freeze drying from tertiary butanol. Microsc. Today 22, 36–39. doi: 10.1017/S1551929514000522
Buda, G. J., Isaacson, T., Matas, A. J., Paolillo, D. J., and Rose, J. K. C. (2016). Three-dimensional imaging of plant cuticle architecture using confocal scanning laser microscopy. Plant J. Cell Mol. Biol. 60, 378–385. doi: 10.1111/j.1365-313X.2009.03960.x
Cabrera, J., Conesa, C. M., and Del Pozo, J. C. (2022). May the dark be with roots: a perspective on how root illumination may bias in vitro research on plant-environment interactions. New Phytol. 233, 1988–1997. doi: 10.1111/nph.17936
Chaffey, N. J. (2000). Plant microtechnique and microscopy. New Phytol. 148, 57–58. doi: 10.1046/j.1469-8137.2000.00735.x
Donaldson, L., Hague, J., and Snell, R. (2001). Lignin distribution in coppice poplar, linseed and wheat straw. Holzforschung 55, 379–385. doi: 10.1515/HF.2001.063
Gao, D., Bao, W. W., Chen, Y. Y., Pan, J. S., and Zhang, W. W. (2021). Study on paraffin section technology of fruit tumor tissues in Cucumber (Cucumis sativus L.). Biosci. Methods 12. doi: 10.5376/BM.2021.12.0001
Hasegawa, H. (2006). Laser scanning confocal microscopy. Kobunshi 55, 961–965. doi: 10.1295/kobunshi.55.961
Hasegawa, J., Sakamoto, Y., Nakagami, S., Aida, M., Sawa, S., and Matsunage, S. (2016). Three-dimensional imaging of plant organs using a simple and rapid transparency technique. Plant Cell. Physiol. 57, 462–472. doi: 10.1093/pcp/pcw027
Hayashi, K., Hasegawa, J., and Matsunaga, S. (2013). The boundary of the meristematic and elongation zones in roots: endoreduplication precedes rapid cell expansion. Sci. Rep. 3, 1–8. doi: 10.1038/srep02723
Inada, N., and Wildermuth, M. C. (2005). Novel tissue preparation method and cell-specific marker for laser microdissection of Arabidopsis mature leaf. Planta 221, 9–16. doi: 10.1007/s00425-004-1427-y
Kirchhoff, C., and Cypionka, H. (2017). Propidium ion enters viable cells with high membrane potential during live-dead staining. J. Microbiol. Meth. 142, 79–82. doi: 10.1016/j.mimet.2017.09.011
Li, X. W., Li, Y. L., Qu, M., Xiao, H. D., Feng, Y. M., Liu, J. Y., et al. (2016). Cell wall pectin and its methyl-esterification in transition zone determine Al resistance in cultivars of pea (Pisum sativum). Front. Plant Sci. 7:39. doi: 10.3389/fpls.2016.00039
Lopez-Bucio, J. S., Salmeron-Barrera, G. J., Ravelo-Ortega, G., Raya-Gonzalez, J., Leon, P., Cruz, H. R., et al. (2019). Mitogen-activated protein kinase 6 integrates phosphate and iron responses for indeterminate root growth in Arabidopsis thaliana. Planta 250, 1177–1189. doi: 10.1007/s00425-019-03212-4
Moreno-Sanz, P., Amato, E. D., Nebish, A., Costantini, L., and Grando, M. S. (2020). An optimized histological proceeding to study the female gametophyte development in grapevine. Plant Methods 16, 61–76. doi: 10.1186/s13007-020-00604-6
Mrak, T., Dovč, N., Gričar, J., Hoshika, Y., Paoletti, E., and Kraigher, H. (2021). Poplar root anatomy after exposure to elevated O3 in combination with nitrogen and phosphorus. Trees 35, 1233–1245. doi: 10.1007/s00468-021-02111-0
Olenichenko, N. A., and Zagoskina, N. A. (2005). Response of winter wheat to cold: production of phenolic compounds and L-phenylalanine ammonia lyase activity. Appl. Biochem. Micro. 41, 600–603. doi: 10.1007/s10438-005-0109-2
Pozhvanov, G. (2018). Visualization and analysis of actin cytoskeleton organization in plants. Biol. Commun. 63, 59–69. doi: 10.21638/SPBU03.2018.107
Premalatha, B. R., Patil, S., Rao, R. S., and Indu, M. (2013). Mineral oil-a biofriendly substitute for xylene in deparaffinization: a novel method. J. Contemp. Dent. Pract. 14, 281–286. doi: 10.5005/jp-journals-10024-1314
Ropitaux, M., Bernard, S., Follet-Gueye, M. L., Vicre, M., Boulogne, L., and Driouich, A. (2019). Xyloglucan and cellulose form molecular cross-bridges connecting root border cells in pea (Pisum sativum). Plant Physiol. Bioch. 139, 191–196. doi: 10.1016/j.plaphy.2019.03.023
Rounds, C. M., Lubeck, E., Hepler, P. K., and Winship, L. J. (2011). Propidium iodide competes with Ca2+ to label pectin in pollen tubes and Arabidopsis root hairs. Plant Physiol. 157, 175–187. doi: 10.1104/pp.111.182196
Scacchi, E., Salinas, P., Gujas, B., and Hardtke, C. S. (2010). Spatio-temporal sequence of cross-regulatory events in root meristem growth. Proc. Natl. Acad. Sci. U. S. A. 107, 22734–22739. doi: 10.1073/pnas.1014716108
Schichnes, D., Nemson, J. A., and Ruzin, S. E. (2001). “Microwave paraffin techniques for botanical tissues” in Microwave Techniques and Protocols (Totowa: Humana Press), 181–189.
Song, C. W., Shen, W. W., Du, L., Wen, J. L., Lin, J. X., and Li, R. L. (2019). Development and chemical characterization of Casparian strips in the roots of Chinese fir (Cunninghamia lanceolata). Trees 33, 827–836. doi: 10.1007/s00468-019-01820-x
Sun, C. Q., Chen, F. D., Teng, N. J., Liu, Z. L., Fang, W. M., and Hou, X. L. (2010). Factors affecting seed set in the crosses between Dendranthema grandiflorum (Ramat.) Kitamura and its wild species. Euphytica 171, 181–192. doi: 10.1007/s10681-009-0005-6
Sun, L. L., Zhang, M. S., Liu, X. M., Mao, X. Z., Shi, C., Kochian, L. V., et al. (2020). Aluminium is essential for root growth and development of tea plants (Camellia sinensis). J. Integr. Plant Biol. 62, 984–997. doi: 10.1111/jipb.12942
Wang, X., Lu, X. C., and Huang, C. J. (2021). Advanced label-free laser scanning microscopy and its biological imaging application. Appl. Sci. 11:1002. doi: 10.3390/app11031002
Wang, W. D., Sheng, X. Y., Shu, Z. F., Li, D. Q., Pan, J. T., Ye, X. L., et al. (2016). Combined cytological and transcriptomic analysis reveals a nitric oxide signaling pathway involved in cold-inhibited Camellia sinensis pollen tube growth. Front. Plant Sci. 7:456. doi: 10.3389/fpls.2016.00456
Wang, H. F., Wang, Z. Q., and Dong, X. Y. (2019). Anatomical structures of fine roots of 91 vascular plant species from four groups in a temperate forest in Northeast China. PLoS One 14:e0215126. doi: 10.1371/journal.pone.0215126
Wei, X. Y., Su, Y., Yang, J., Lin, L., and Li, C. Y. (2011). Microwave paraffin section in rice leaf tissue. J. Yunnan Agric. Univ. 26, 454–457. doi: 10.3969/j.issn.1004-390X(n)
Wu, P. F., Lai, H. Y., Tigabu, M., Wu, W. J., Wang, P., Wang, G. Y., et al. (2018). Does phosphorus deficiency induce formation of root cortical aerenchyma maintaining growth of Cunninghamia lanceolata. Trees 32, 1633–1642. doi: 10.1007/s00468-018-1739-3
Yao, S. C., Luo, S. L., Pan, C. L., Xiong, W. J., Xiao, D., Wang, A. Q., et al. (2020). Metacaspase MC1 enhances aluminum-induced programmed cell death of root tip cells in Peanut. Plant Soil 448, 479–494. doi: 10.1007/s11104-020-04448-w
Zhang, R. Q., Fu, K. Y., Li, C., Zu, S. C., Li, C. Y., and Li, C. (2017). Changes of micro-structural characteristics of starch granules and the mechanisms under different phosphorus application rates in wheat (Triticum aestivum L.). Sci. Geol. Sin. 50, 4235–4246. doi: 10.3864/j.issn.0578-1752.2017.22.001
Zhang, S. M., Yang, S. J., Liu, H. Y., and Liu, Z. (2015). Optimization of microwave paraffin techniques for clear observation of cell structures of millet (Setaria italica) young leaves. Genomics Appl. Biol. 34, 669–673. doi: 10.13417/j.gab.034.000669
Zhao, J. N., Wang, P. C., Jia, X. W., Xu, S. B., and Wang, X. M. (2021). Observation and analysis of wheat root cytology. J. Triticeae Crop. 41, 1161–1170. (in Chinese with English abstract). doi: 10.7606/j.issn.1009-1041.2021.09.13
Keywords: Cunninghamia lanceolata, root tissue, cell morphology, microwave paraffin sections, laser scanning confocal microscope
Citation: Li L, Zhang T, Lin J, Lian X, Zou X, Ma X and Wu P (2023) Longitudinal section cell morphology of Chinese fir roots and the relationship between root structure and function. Front. Ecol. Evol. 11:1122860. doi: 10.3389/fevo.2023.1122860
Edited by:
Wen Xing Long, Hainan University, ChinaReviewed by:
Aitezaz Ali Asad Shahani, Yanbian University, ChinaAmmarah Shabbir, Institute of Biochemistry and Biophysics (PAN), Poland
Bamisope Steve Bamisile, South China Agricultural University, China
Copyright © 2023 Li, Zhang, Lin, Lian, Zou, Ma and Wu. This is an open-access article distributed under the terms of the Creative Commons Attribution License (CC BY). The use, distribution or reproduction in other forums is permitted, provided the original author(s) and the copyright owner(s) are credited and that the original publication in this journal is cited, in accordance with accepted academic practice. No use, distribution or reproduction is permitted which does not comply with these terms.
*Correspondence: Pengfei Wu, ✉ fjwupengfei@126.com