- Key Laboratory of Genetics and Germplasm Innovation of Tropical Special Forest Trees and Ornamental Plants, Ministry of Education, College of Forestry, Hainan University, Haikou, China
Nitrogen and phosphorus are important nutrient elements for plants and underground organisms. The nematode is an important part of the soil food web. Although many studies have explored the effects of fertilization on soil nematode community structure, little is known about the response mechanism of the nematode community to fertilization. In this study, we investigated the diversity and functional diversity of soil nematode communities, as well as soil physicochemical properties, root functional traits, and plant richness. We explored the response mechanism of soil nematode communities to nitrogen and phosphorus fertilizer. Nitrogen fertilizer increased the abundance and richness of bacterivorous nematodes, while phosphorus fertilizer decreased the total abundance of bacterivorous nematodes. Meanwhile, the diversity of the nematode community was significantly affected by soil physicochemical properties and plant root functional traits. Therefore, our study revealed the effects of nitrogen and phosphorus fertilizer on soil nematode community diversity and functional diversity. Exploring the response mechanism of soil nematode communities to fertilization interference provides further evidence for the role of nematodes in maintaining the function of subsurface ecosystems.
Introduction
Nematodes are pseudocoelomata belonging to the phylum Nematoda, the most abundant phylum in the kingdom Animalia (Lorenzen, 1994; van den Hoogen et al., 2019). Nematodes are widespread in terrestrial ecosystems, where they are noted for their diversity and hardiness (Yeates et al., 1993; Wu, 1999). These organisms are the most important component of the soil food web, and monitoring changes to nematode community composition is a useful tool for assessing soil quality (Liu et al., 2018). Soil nematodes are classified according to their feeding habits as bacterivores, fungivores, plant-feeders, or omnivore-predators (Wu, 1999). In recent years, atmospheric nitrogen deposition has emerged as an important global change factor (Vitousek et al., 1997; Reay et al., 2008). In many natural or semi-natural plant communities, nitrogen is often the main factor limiting plant growth and development, and phosphorus is an essential nutrient for plant growth (Bardgett et al., 1998). Nitrogen addition can mitigate this limitation, inducing changes in the productivity of above-ground vegetation communities and preferentially promoting the growth of particular species, resulting in a loss of diversity (Bobbink et al., 2010). Moreover, nitrogen addition can also change plant community structure (Reich et al., 2006; Yang H. et al., 2012) and productivity, and affect plant root exudates and soil physicochemical properties (DeForest et al., 2004; Niu et al., 2010; Contosta et al., 2011). Human agricultural management has greatly altered the amount of nitrogen and phosphorus found in the soil (Villenave et al., 2010; Liu et al., 2012; Yang Y. et al., 2012).
Recently, the focus has shifted to the effects of fertilization on soil faunal communities (Qi et al., 2010; Zhang et al., 2022), especially on soil nematode communities. Fertilizer application is not only known to affect the structure and composition of vegetation but also belowground organisms and the soil environment has potential effects (Bardgett et al., 1998) and may alter nematode community structure. In grassland ecosystems, the long-term addition of nitrogen fertilizer reduces the number of plant-feeders (Ruan et al., 2012); the application of nitrogenous fertilizers is associated with a decline in the size of fungivore, plant-feeder, and omnivore-predator populations and an increase in the size of bacterivore populations (Murray et al., 2006; Zhang et al., 2022). Nitrogen fertilization is also associated with soil acidification, which may directly contribute to nematode population declines (Wei et al., 2012). Scientists have observed a negative correlation between plant-feeder and fungivore population sizes and levels of ammonium and nitrate after fertilization (Sarathchandra et al., 2001; Pey et al., 2014), suggesting that nitrogen fertilization has a direct effect on soil nematodes. The addition of phosphorus fertilizer increases the number of bacterivores (Qi et al., 2010) and overall soil nematode population, while at the same time altering organic matter decomposition pathways and reducing the number of rare species (Chen et al., 2014). In tropical rainforest, phosphorus supplementation significantly inhibited the total density of soil nematodes and the density of omnivore-predators nematodes (Zhao et al., 2014). In contrast, Rovira and Simon (1985) found that the addition of phosphorus fertilizer significantly increased the number of nematodes. There are few studies on the effects of mixed application of N and P fertilizer on soil nematode community. It has been found that in a long-term fertilization experiment for agricultural ecosystems in Heilongjiang Province, China, mixed application of N and P fertilizer increased the abundance of bacterivores nematodes (Pan et al., 2015).
The health of soil nematode populations is coupled to interactions with other animals, fungi, and bacteria, as well as to soil physicochemical properties and inter-root resource inputs from plants (Bongers and Ferris, 1999). Plants promote soil nematode diversity (Liu et al., 2012) by increasing the diversity of nematode food sources (Hooper et al., 2000; De Deyn et al., 2004). Changes in the soil environment or vegetation composition can directly or indirectly affect soil nematode communities (Wang et al., 2019; Chen et al., 2021). An increase in soil water content is associated with an increase in the number of plant-feeders (Ruan et al., 2012) and a decrease in the number of bacterivores and omnivore-predators (Chen et al., 2021); changes in the biomass of dominant plants in an ecosystem indirectly affects soil nematode abundance (Yeates, 1999; Wang et al., 2019).
With increasing scientific interest in soil nematodes and the development of functional ecology (Violle et al., 2007), researchers have begun to quantify the functional attributes of soil nematodes using traits such as individual size. The objective of these efforts is to understand how nematode functional diversity changes in response to environmental changes and their associated effects on ecosystem function (Tita et al., 1999; Pey et al., 2014). One metric for assessing functional attributes is community-weight mean (CWM), which is calculated as the weighted average of individual size and relative abundance of each genus. Wang et al. (2002) found that soil organic carbon, microbial biomass, and plant tissues provided food resources for soil nematodes, contributing to nematode growth and overall size. Recent work found that increasing soil nutrient concentrations by applying fertilizers increased the length of individual nematodes (Liu et al., 2015). When soil organic matter and total nitrogen content are higher, food resources for larger nematodes increase, thereby increasing CWM (Liu et al., 2015; Andriuzzi and Wall, 2018). In contrast, phosphorus limitation favors the survival of smaller soil animals and, as such, phosphorus content is positively correlated with community level size (Mulder and Elser, 2009). Consistent with this finding, researchers found that small individual nematodes have a better survival rate in gully bottoms with lower phosphorus levels in alpine meadow ecosystems (Wang and Niu, 2020).
The Qinghai-Tibet Plateau alpine meadow is an important grassland ecosystem in China (Du et al., 2003), and numerous studies have investigated the effects of fertilization on plant communities and soil factors in this ecosystem (Yang Z. et al., 2012). A recent experiment in alpine meadows on the Tibetan plateau did not find significant changes to nematode communities resulting from long-term nitrogen and phosphorus fertilization (Andriuzzi and Wall, 2018). However, little research has been conducted on the effects of fertilizer application on the functional diversity of soil nematode communities in this ecosystem. It is unclear whether the effect of fertilization on the nematode community is due to changes in the soil microenvironment or plant community composition, and there are few studies on the interaction between plants and nematodes. In this study, we conducted an experiment in alpine meadows on the Tibetan Plateau were continuously fertilized for 4 years to investigate the effects of nitrogen and phosphorus addition on soil nematode diversity. CWM was used as an indicator of soil nematode response to fertilization. We also investigated the effects of aboveground vegetation, soil physicochemical properties, and plant root characteristics on soil nematode communities and the mechanisms of fertilization on nematode communities. We hypothesized that (1) fertilization reduces soil nematode abundance and the species richness of aboveground vegetation; (2) fertilization increases CWM values of the individual size of soil nematode communities; and (3) fertilization affects soil nematode communities by changing plant community structure.
Materials and methods
Study site and experimental design
The study was conducted at the Haibei Alpine Meadow Ecosystem Open Experimental Station of the Chinese Academy of Sciences in Menyuan County, Qinghai Province, in the northeastern part of the Tibetan Plateau (101°12′–101°23′, 37°29′–37°45′). The area has a plateau continental climate with damp and rainy summers and cold and dry winters, with an average altitude of 3,215 m, an average annual temperature of −1.2°C, and annual precipitation of 489 mm. Precipitation occurs mainly between May and September (Wang et al., 2012). The plant community in this typical alpine meadow is dominated mainly by Poaceae, Asteraceae, and members of the buttercup family, such as silver lotus (Anemone obtusiloba), sheep grass (Elymus nutans), big leaf gentian (Gentiana macrophylla), yellow flower Poaannua, fern (Potentilla bifurca), and black snowdrop (Saussurea nigrescens) (Liu et al., 2020). The soils are thin with high organic matter content but low effective phosphorus and nitrogen content; thus, plant communities in this area are limited by both nitrogen and phosphorus (Niu et al., 2016).
Fertilization experiments began in 2018 in sample plots in the study area. Four fertilization treatments were randomly distributed among six blocks arranged across the sample plots, with six replicates of each treatment and a 3 m buffer interval between each block. Each sample square was 3 × 3 m in size, with a 2 m distance separating each square. A 0.5 m × 0.5 m fixed sample square in the middle of each 3x3m square was used to assess plant cover and abundance. There were four treatments in this study: blank control (CK), phosphorus fertilizer (P, added amount: 10 g m−2 year−1), nitrogen fertilizer (N, added amount: 10 g m−2 year−1), and nitrogen and phosphorus fertilizer applied together (NP, N: 10 g m−2 year−1, P: 10 g m−2 year−1) (Liu et al., 2020). Fertilization treatments were applied every June.
Sample collection
Sampling was conducted at the end of August 2021 after 4 years of fertilization treatments. Plants were at the time of sampling. A 0.5 m × 0.5 m area was randomly selected within the lower left 1/4 of the sample plot for plant species identification and counting. Above-ground plant parts were cut, sorted, and taken to the laboratory, where they were dried at 65°C before weighing.
Soil and root samples were collected and analyzed in early September 2021. Soil samples were taken from each 3 m × 3 m treatment sample square with a 4-cm diameter soil auger, using the five-point method to bore five holes 15 cm deep. Each set of five samples was mixed into a single sample and placed into a self-sealing bag, after which it was labeled and stored at 4°C during transit to the laboratory. In the laboratory, a 2 mm soil sieve was used to remove debris and sort out plant roots in the soil. Samples were divided into 2 parts: one portion was stored at 4°C for 1 day until soil nematodes could be extracted, and the other one was used to characterize soil physicochemical properties.
Measurement of plant root functional traits
Sorted roots were gently washed with deionized water to remove attached soil and other residues, wrapped in plastic wrap and tin foil and refrigerated at 1°C. Root samples were scanned and imaged (Winrhizo, Regent Instruments, Inc. Nepean, Ontario, Canada) to determine total root length (Length), total root volume (Root Volume), and total root surface area (Surf Area). Specific root area (SRA: root length per unit weight), root tissue density (RTD), total root biomass (Root biomass), specific root length (SRL), and root length density (RLD) were calculated from root dry weights (Freschet et al., 2021).
Physical and chemical soil properties
10 g of soil was placed in an aluminum box and dried at 105°C for 72 h to determine soil moisture content (SM). Soil pH was determined using a pH analyzer (meter-s210 Seven Compact™, Switzerland) with a 1:2 ratio of soil to water. Soil total nitrogen (TN) was determined by the semi-Kaeschner method (Sparks et al., 1996), soil total phosphorus (TP) by the sulfuric acid-perchloric acid digestion-molybdenum antimony anti-colorimetric method, soil available phosphorus (P) by the sodium bicarbonate leaching-molybdenum antimony anti-colorimetric method, and soil organic carbon (SOC) by the walker-black method (Nelson and Sommers, 1982). Soil ammonium (NH4 +) and soil nitrate (NO3−) were determined by the indophenol blue colorimetric method (Dorich and Nelson, 1983) and salicylate colorimetric method (Yang et al., 1998), respectively.
Isolation, identification, and measurement of soil nematodes
Soil nematodes were isolated using a modified Bellman funnel method (Liu et al., 2015). The soil was stored in a refrigerator at 4°C, after which a 50 g sample was taken and isolated for 48 h before being fixed and filmed with glycerol. Head and tail morphology were used to sort nematodes into genera, after which nematodes were counted and photographed under a compound microscope (Olympus BX53 microscope at 10–1,000×). Nematodes were identified using the Soil Fauna of China Retrieval Atlas (Yin, 1998) and the Study of Freshwater and Soil Nematodes in China (Wu, 1999).
The number of individual nematodes was converted to bars/100 g of dry soil. When the number of isolated nematodes was less than 100, all nematodes were identified; if the number was greater than 100, 100 individuals were randomly selected. The nematodes were classified into four groups according to their feeding characteristics: bacterivores, fungivores, plant-feeders, and omnivore-predators (Bongers and Bongers, 1998).
For each sample, the overall size was calculated by summing the sizes of all identified nematodes. The relative abundance of a genus was calculated as the ratio of the abundance of a genus to the total abundance of nematodes in a sample. Community-weighted mean (CWM) was calculated by weighting the average body size to relative diversity. The calculation of the cluster individual size weighted mean is based on the equation presented by Liu et al. (2015):
where N represents the number of nematode genera, Xi represents the mean body size value for genus i; Pi represents the relative abundance of genus i.
The body type characteristics of soil nematodes were determined by Digimizer software. The nematode body size (V) was calculated using the following equation:
where V represents the volume of each nematode; L represents the body length of each nematode; D represents the maximum body width of each nematode, and 1.7 is a constant.
Statistical analysis
Here, we assess how soil nematode diversity is influenced by changes in soil physicochemical properties and plant communities occurring in response to nitrogen and phosphorus fertilization. Species richness was calculated from plant community data; Soil physicochemical properties (e.g., soil pH, soil organic carbon, total nitrogen, total phosphorus, ammonium nitrogen, nitrate nitrogen, and available phosphorus) were measured from soil samples; Plant root traits were determined from subsurface sections.
At the community level, nematode abundance, functional group abundance, genus richness, and Shannon diversity were calculated. CWM was calculated using individual body length and body width data. This index was used to better explain the response of nematode communities to nitrogen and phosphorus additions. Through the analysis of different functional groups of nematodes, the changes in the structure and function of the soil food web were studied, to evaluate the response of the soil food web to environmental disturbance.
A linear mixed-effects model and two-factor ANOVA were used to analyze changes to soil physicochemical factors, root functional traits, plant species richness, soil nematode diversity, and each nematode functional group. Principal component analysis (PCA) was used to standardize the soil physicochemically and root functional trait variables, and the respective first axis principal component data were extracted and recorded as “SoilPC1” and “RootPC1.” SoilPC1 and RootPC1 were then used to analyze the effects of soil physicochemical properties and root functional traits on soil nematode diversity (i.e., total abundance, abundance, Shannon diversity, Simpson diversity, and evenness) and functional diversity (i.e., CWM values dispersion based on body size). Simple linear regression analysis was used to investigate the correlation between soil nematode diversity and functional diversity and “SoilPC1,” “RootPC1,” and plant species richness using the “lm” function in R. Finally, structural equation modeling (SEM) was used to explore the pathways by which fertilizer application influences soil nematode communities, with “SoilPC1,” “RootPC1,” and plant species richness as potential variables.
All data were analyzed using R software version 4.1.2. The “FD” package was used to calculate CWM and FDis based on nematode size (Laliberté et al., 2014). The “vegan” package was used to calculate Shannon diversity, Simpson diversity, and evenness metrics for nematodes. PCA was also conducted using the “vegan” package (Oksanen, 2016). The “lme” function in the “lme4” package was used to calculate linear mixed effects (Bates et al., 2015). Finally, the “ggplot2” package was used for plotting, and the “piecewiseSEM” package was used for SEM (Lefcheck, 2016).
Results
The influence of fertilizer application on nematode and plant diversity
Fertilizer application had different effects on soil nematode diversity and functional diversity. Shannon diversity, Simpson diversity, and evenness increased significantly when only nitrogen (N) fertilizer was applied. When only phosphorus (P) fertilizer was added, the individual abundance of soil nematodes was reduced significantly and the functional richness index (FRic) of nematode communities increased significantly. Nematode abundance, Shannon diversity, Simpson diversity, community functional dispersion (FDis), and Rao’s quadratic entropy index (RaoQ) decreased significantly with the co-application of nitrogen and phosphorus (NP) fertilizers. Moreover, the co-application of NP fertilizer significantly reduced above-ground plant species richness (Table 1). This indicates that the addition of different chemical fertilizers has different effects on the soil nematode community. Soil nematode diversity was greater in all cases of N-only fertilization compared to P-only fertilization, suggesting that phosphorus deposition may be a key factor affecting nematode communities.
The influence of fertilizer application on soil physicochemical properties and root functional traits
Fertilization treatments were observed to affect the subsurface soil environment and plant roots. Under N addition, soil pH decreased significantly and nitrate (NO3−) content increased significantly, but the effect of N addition on root traits was not significant (Table 2). Under P addition, soil effective phosphorus content and total soil phosphorus (TP) content increased significantly; fine root dry weight (FM) and root length density (RLD) values of the underground root fraction decreased significantly (Table 2). However, the effect of fertilization on soil physicochemical factors and root traits was not significant under the NP treatment (Table 2). These changes indicate that soil physicochemical factors were more sensitive to the addition of either N or P fertilizers compared to NP treatment. Changes to root system functional properties were stronger for P treatment than for N or NP.
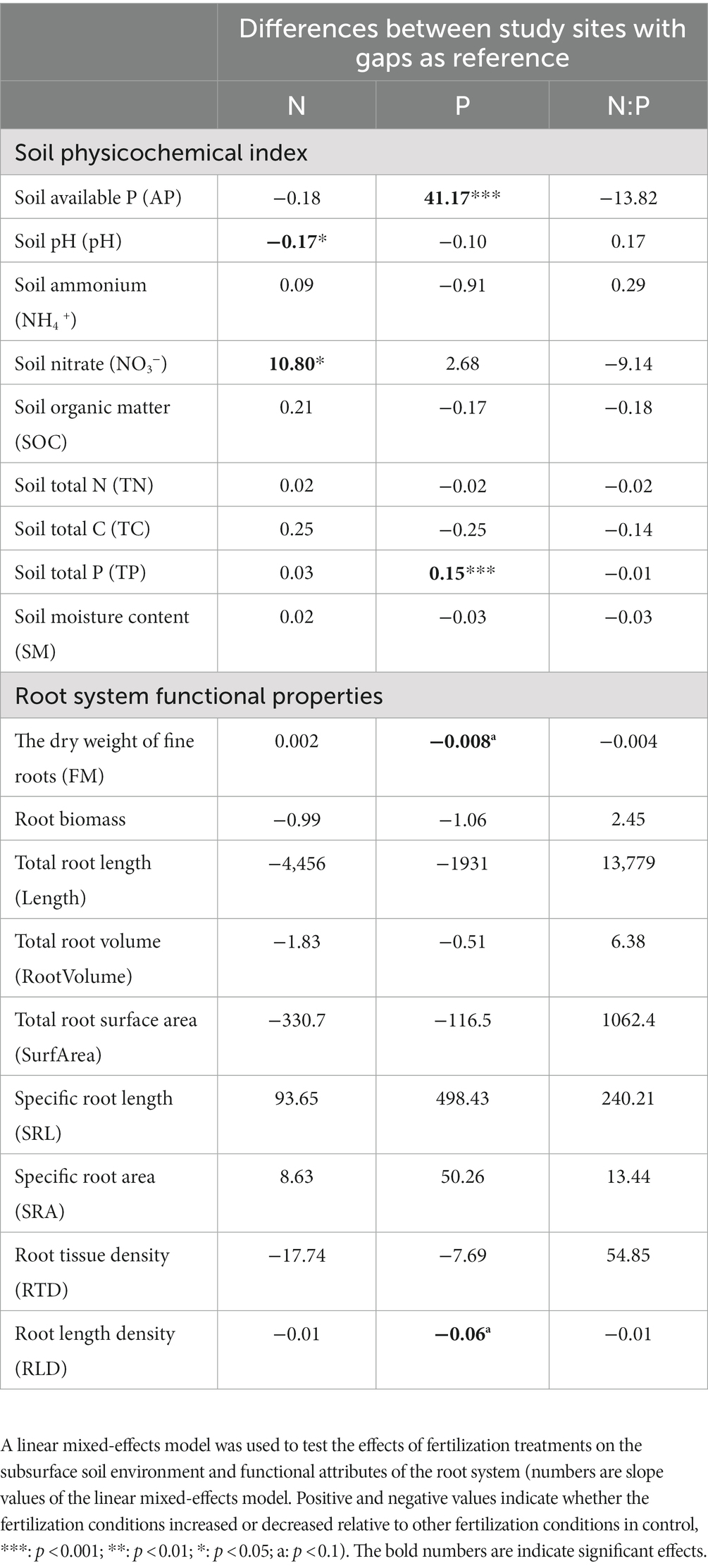
Table 2. Effect of N and P fertilizer addition and their interaction on subsurface soil environmental factors and functional attributes of the root system.
Effect of fertilizer application on nematode functional groups
Fertilizer application also affected soil nematodes of different functional groups. Bacterivore richness and abundance increased significantly under the N application and decreased significantly under the NP application (Table 3). In the P treatment, only the abundance of plant-feeder nematodes was reduced significantly (Table 3). The abundance and richness of omnivore-predator nematodes decreased significantly in the NP treatment (Table 3). We found that fertilization did not significantly affect soil nematode volume in any group. This indicates that N fertilization favored the growth of bacterivore nematode communities, P fertilization inhibited the survival of plant-feeder nematodes, and NP fertilization was determinantal to the development of soil nematode communities (Table 3).
Effects of soil physicochemical properties and root traits on soil nematode diversity
Principal component analysis (PCA) was performed on soil physicochemical properties and root characteristics. Results showed a significant positive correlation between soil physicochemical properties, nematode species richness, Shannon diversity, Simpson diversity, and FDis (Figure 1A; Supplementary Figure S1). CWM and nematode community evenness (E) were not correlated to soil physicochemical properties (Figure 1A). There was a significant negative correlation between root characteristics, Shannon diversity, and Simpson diversity (Figure 1B; Supplementary Figure S2). Plant species richness was negatively correlated to E and root characteristics (Supplementary Figure S3).
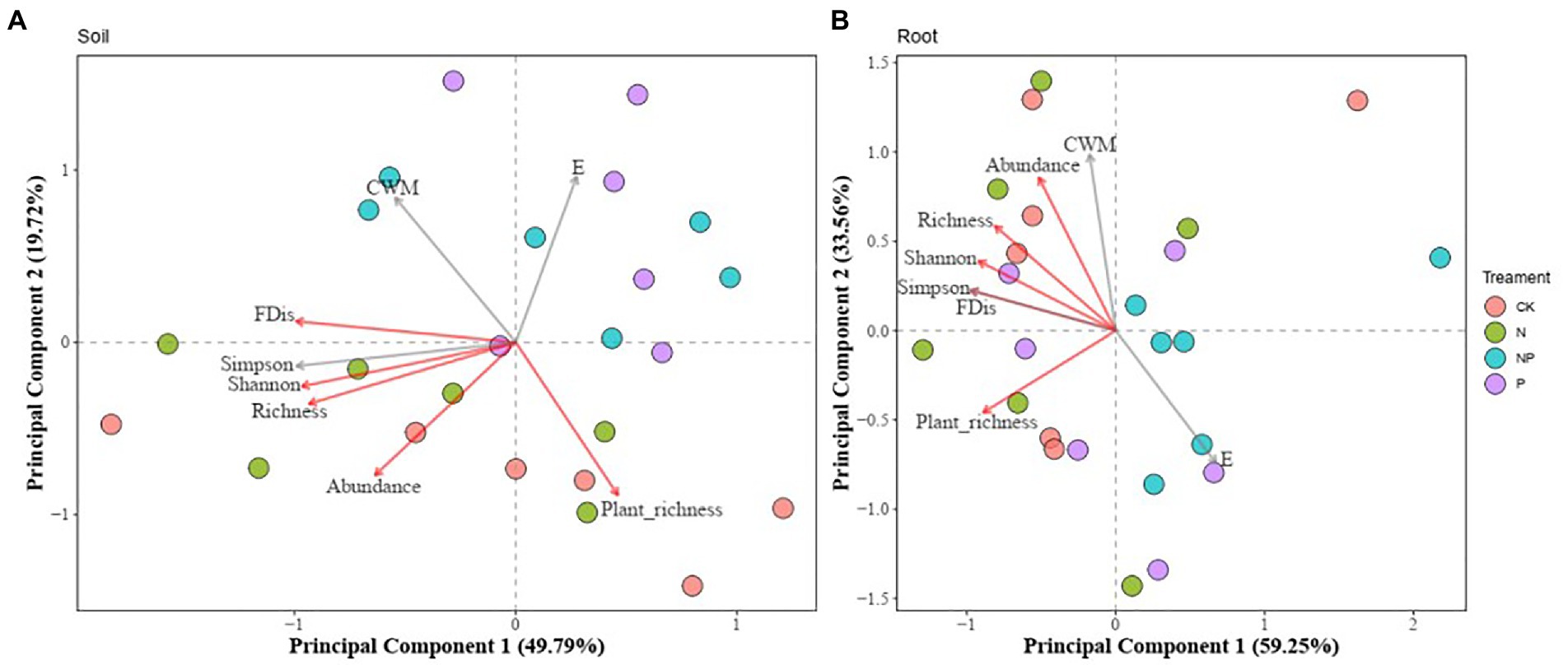
Figure 1. Principal component analysis (PCA). Relationship between soil nematode diversity and soil physicochemical properties (A) and plant root functional traits (B) under fertilization treatments. CWM, community means based on nematode body size; FDis, Functional dispersion index; Abundance, Abundance of soil nematodes in 100 g of dry soil; Richness, Soil nematode richness; E, Community evenness; Plant_ richness, Plant species richness. Soil physical and chemical properties and root functional traits were extracted separately from the first principal component (PC1) after dimensionality reduction by principal component analysis. The indicators measured for root functional traits were RTD, Root tissue density; RLD, Root length density; FM, Fine root dry weight; Length, Total root length; RootVolume, Total root volume; SRL, Specific root length; SRA, Specific root area; SurfArea, Total root surface area. Soil physical and chemical properties were measured as follows: SOC, Soil organic carbon; TN, Total soil nitrogen; TC, Total soil carbon; TP, Total soil phosphorus; P, Soil available phosphorus; pH, Soil pH; NH4+, Soil ammonium; NO3−, Soil nitrate. Variables corresponding to the red arrows indicate that the effect on soil or root traits is significant. Gray arrows indicate that the effects are not significant.
Structural equation modeling (SEM) analysis was performed to illuminate the relative importance of relationships between measured variables. The model included plant community richness, root functional attributes, and soil nematode community variables. NP fertilization significantly reduced plant community richness, and soil physicochemical properties; N and P treatments had a direct effect on soil physicochemical properties (Figures 2A,B). P fertilization directly resulted in lower nematode abundance (Figure 2A). NP treatment indirectly affected nematode species richness by altering soil physicochemical properties (Figure 2B). Therefore, we concluded that P fertilization exerts a direct effect on soil nematode communities. N and P fertilization had no significant effect on CWM (Table 1). In summary, we found that P fertilization had a direct effect on soil nematode abundance and that NP fertilization had an indirect effect on nematode species richness.
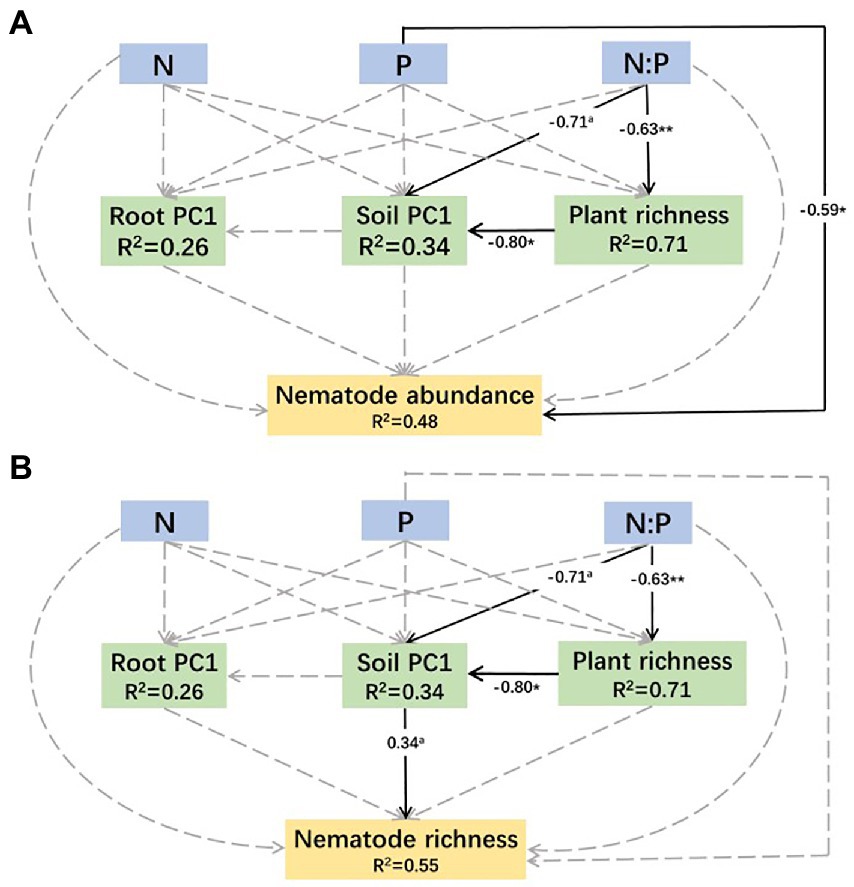
Figure 2. SEM analysis showed the interaction between plant and soil and its effects on (A) soil nematode abundance (AIC = 60, Fisher’s C = 0, p = 1) and (B) richness (AIC = 60, Fisher’s C = 0, p = 1). Roots and soils are represented by principal component analysis (PCA) of the reduced dimensional first principal component, respectively. Root functional property variables include all indices measured, and soil physicochemical property variables include so-measured soil physicochemical indicators. Boxes in the model represent individual variables; solid arrows represent significant effects (p < 0.1); dashed arrows represent non-significant effects (p > 0.1); numbers on the arrows represent path coefficients. R2 values represent the variance of response variables explained by other variables. ***: p < 0.001; **: p < 0.01; *: p < 0.05; a: p < 0.1.
Discussion
The influence of fertilization on soil nematode diversity
Fertilization significantly altered the soil nitrogen and phosphorus levels. This experiment found that the addition of N significantly altered soil nitrate N content and pH; P treatment significantly altered soil effective P and total soil P, while the mixed addition of N and P fertilizer had no effect on soil physical and chemical properties. The effects of the treatment type on soil nematodes were different from their effects on physicochemical properties. N fertilization significantly increased Shannon diversity, Simpson diversity, and E, which is consistent with previous work conducted in Inner Mongolia (Wang et al., 2019).
These results corroborated our first hypothesis and found that P fertilization greatly reduced soil nematode abundance and that the interaction of N and P fertilizers significantly reduced soil nematode richness. The addition of P fertilizer significantly decreased overall nematode abundance, consistent with work done in a low-lying paddy field located on the Ivory Coast of Africa and a tropical forest (Zhao et al., 2014; Čerevková et al., 2017). However, other studies have shown that fertilization can have a positive effect on nematodes and improve overall species richness (Sarathchandra et al., 2001). One of the reasons for this inconsistency is likely due to the amount of fertilizer applied. In the study by Sarathchandra et al., the amount of fertilizer applied was 20 g per m 2 N and 20 g per m 2 P, and our application amount of N and P fertilizer was only 10 g per m 2. Most of the fertilizer was probably taken up by the plants, and the remaining amount was insufficient to change the soil nematode community. Moreover, the effects of N and P fertilization on soil physicochemical properties are also important. N application lowers soil pH and increases soil nitrate N content, and P application increases the content of effective and total phosphorus; the decrease in soil pH leads to soil acidification, which contributes to the decreasing nematode populations because soil acidification destroys optimal growth conditions, and some soil nematodes are sensitive to changes in the soil environment (Li et al., 2013), especially large nematodes (Liang et al., 2009). Furthermore, it has been shown that P fertilization is toxic to nematodes and can restrict their reproductive ability (Hu et al., 2017). Here, we found that N fertilization increased the abundance and richness of bacterivores, similar to findings by other researchers (Hu et al., 2017). Hu et al. found that soil microbial biomass, C and N increased significantly, increasing the amount of food available for bacterivore nematodes. Consistent with these findings, PCA revealed that soil N and organic matter content increased, positively impacting bacterivores (Figure 1A).
In contrast, a study conducted in a New Zealand pasture found that P additions significantly increased fungivore abundance (Chen et al., 2014), while other work found that P fertilization resulted in slight increases in bacterivore and omnivore-predator populations as well as soil nematode species richness (Sarathchandra et al., 2001). Bottom-up control mechanisms are widely present in subsurface ecosystems (Neher, 2010), and P input changes the composition and functional characteristics of the soil microbial community by promoting the rapid growth of vegetation (Leff et al., 2015). In this study, structural equation modeling revealed that P fertilization had a direct negative effect on soil nematode abundance (Figure 2A), which was inconsistent with hypothesis 3. Other nematode studies found that the addition of P fertilizer under N-enriched conditions significantly increased the functional variables in soil nematode communities, which attenuated the positive effect of P (Zhao et al., 2014). In contrast, our experiments found that NP application significantly reduced nematode richness and functional diversity, and P attenuated the positive effect of N on soil nematode communities.
At the same time, fertilization reduces plant species richness. Numerous studies have shown that fertilizer application increases above-ground plant biomass, increasing canopy cover. This results in decreased light for non-grass vegetation and legumes, ultimately leading to the loss of these species (Qi, 2013). Previous studies have found that fertilization alters soil nematode community structure by decreasing plant species diversity. However, our results differ in that fertilization did not affect the functional traits of plant roots (Figure 2). The reduction in nematode abundance under P fertilization was mostly due to a decrease in the number of plant-feeders (Table 3); this is probably because plant-feeders subsist on root secretions, and P fertilization reduced fine root dry weight and root tissue density and had an indirect negative effect on root functional attributes by reducing plant species diversity. Thus, changes in root functional attributes may affect root exudation, thereby affecting the population of plant-feeders. An additional explanation may be that the loss of plant species that results from fertilization causes changes in root biomass (Cortois et al., 2017), which in turn reduces plant-feeder nematode populations.
Roots play a vital role in ecosystems and provide habitats for many underground organisms (Bardgett and van der Putten, 2014). Thin roots provide more space for herbivores and predators to survive in the soil (Legay et al., 2020). The greater the root length, the more beneficial it is to microbial life, and thus to the microfauna living there (Picot et al., 2019). Second, there is a strong selectivity of root secretions to rhizosphere microorganisms, and the addition of nitrogen has a significant effect on the rhizosphere bacterial community (Wang et al., 2022). Although we did not find any significant effect of fertilization on the root functional traits, research based on root characteristics can help us to better understand the soil nematode community.
The influence of fertilization on plant functional diversity
To improve investigation into the relationship between soil nematode communities and environmental changes and ecosystem functions, nematode body size indicators are increasingly used as a measure of functional diversity in soil systems (Mulder et al., 2009). Indicators such as CWM, which are based on body size, are more reliable than those based on abundance to explain the response of soil nematode communities to fertilization (Liu et al., 2015). Of the large variation in body size among different genera of nematodes, CWM was introduced to facilitate the description of soil nematode communities (Niu et al., 2014). Work in rice fields found that CWM values of soil nematode communities were positively correlated with soil nutrients, and could serve as a sensitive indicator of nematode community response to fertilizer application (Liu et al., 2015). Research by Christian et al. found that low P soils were more favorable for the survival of small-bodied soil animals, and high P soils supported larger-bodied soil animals at higher trophic levels; this was because increased P content improved the quality of food for small soil animals, who were previously limited by their food’s stoichiometric ratio and also improved the quality of organic matter in the soil, Together, these factors increased the nutrient transfer rate among soil animals (Mulder and Elser, 2009). However, some work suggests that in areas with high P content, small nematodes such as bacterivores, fungivores, and plant-feeders can survive better due to higher microbial diversity (Zhao et al., 2020). Other studies have also found that functional diversity improves as soil N and soil organic matter increases (van den Hoogen et al., 2019) and results in increasing size of individual nematodes, indicating that nitrogen fertilization favors the survival of large-bodied nematodes (Liu et al., 2015; Andriuzzi and Wall, 2018).
Here, we found that the CWM of soil nematode communities did not respond significantly to fertilization, which was not consistent with hypothesis 2. Changes in nematode communities take place over long periods, and 4 years of fertilization may not be long enough to induce substantial changes in nematode community structure. As such, we need longer-term fertilization experiments to understand soil nematode community dynamics under such conditions. Additionally, further investigation is needed to determine how fertilization affects soil nematodes at different trophic levels.
Conclusion
In alpine meadow ecosystems of the Tibetan Plateau, although N fertilization increased the abundance and richness of bacterivore nematodes, the abundance and richness of both bacterivore and omnivore-predator nematodes decreased under NP application, suggesting that P fertilization has a greater impact on soil nematode communities than N fertilization does. Here, we use soil physicochemical properties and root functional traits to understand how fertilization alters nematode communities; however, we did not investigate how changes in plant root secretions and soil microorganisms resulting from fertilization influence nematode communities. Longer-term studies examining how fertilization alters subsurface biological communities and abiotic factors are needed. Moreover, the effects of fertilization, the soil environment, and plant root systems are intertwined. We must further investigate how interactions between these factors alter soil nematode communities in fertilized soils to provide a scientific basis for the management of alpine meadows in the Tibetan Plateau.
Data availability statement
The raw data supporting the conclusions of this article will be made available by the authors, without undue reservation.
Author contributions
SZ designed the experiment. SZ and YQ conceived the research. YQ, XS, XT, and SP collected the data. YQ and XT analyzed the data. YQ wrote the manuscript and all authors contributed to revisions. All authors approved the final manuscript.
Funding
This study was supported by the National Natural Science Foundation of China (grant no. 31830009) and the Fundamental Research Funds in Hainan University (KYQD (ZR)-20081).
Conflict of interest
The authors declare that the research was conducted in the absence of any commercial or financial relationships that could be construed as a potential conflict of interest.
Publisher’s note
All claims expressed in this article are solely those of the authors and do not necessarily represent those of their affiliated organizations, or those of the publisher, the editors and the reviewers. Any product that may be evaluated in this article, or claim that may be made by its manufacturer, is not guaranteed or endorsed by the publisher.
Supplementary material
The Supplementary material for this article can be found online at: https://www.frontiersin.org/articles/10.3389/fevo.2023.1122505/full#supplementary-material
References
Andriuzzi, W. S., and Wall, D. H. (2018). Grazing and resource availability control soil nematode body size and abundance-mass relationship in semi-arid grassland. J. Anim. Ecol. 87, 1407–1417. doi: 10.1111/1365-2656.12858
Bardgett, R. D., and Van Der Putten, W. H. (2014). Belowground biodiversity and ecosystem functioning. Nature 515, 505–511. doi: 10.1038/nature13855
Bardgett, R. D., Wardle, D. A., and Yeates, G. W. (1998). Linking above-ground and below-ground interactions: how plant responses to foliar herbivory influence soil organisms. Soil Biol. Biochem. 30, 1867–1878. doi: 10.1016/S0038-0717(98)00069-8
Bates, D., Mächler, M., Bolker, B., and Walker, S. (2015). Fitting linear mixed-effects models using lme4. J. Stat. Softw. 67, 1–48. doi: 10.18637/jss.v067.i01
Bobbink, R., Hicks, K., Galloway, J., Spranger, T., Alkemade, R., Ashmore, M., et al. (2010). Global assessment of nitrogen deposition effects on terrestrial plant diversity: a synthesis. Ecol. Appl. 20, 30–59. doi: 10.1890/08-1140.1
Bongers, T., and Bongers, M. (1998). Functional diversity of nematodes. Appl. Soil Ecol. 10, 239–251. doi: 10.1016/S0929-1393(98)00123-1
Bongers, T., and Ferris, H. (1999). Nematode community structure as a bioindicator in environmental monitoring. Trends Ecol. Evol. 14, 224–228. doi: 10.1016/S0169-5347(98)01583-3
Čerevková, A., Miklisová, D., and Cagáň, Ľ. (2017). Effects of experimental insecticide applications and season on soil nematode communities in a maize field. Crop Prot. 92, 1–15. doi: 10.1016/j.cropro.2016.10.007
Chen, X., Daniell, T. J., Neilson, R., O’Flaherty, V., and Griffiths, B. S. (2014). Microbial and microfaunal communities in phosphorus limited, grazed grassland change composition but maintain homeostatic nutrient stoichiometry. Soil Biol. Biochem. 75, 94–101. doi: 10.1016/j.soilbio.2014.03.024
Chen, H., Luo, S., Li, G., Jiang, W., Qi, W., Hu, J., et al. (2021). Large-scale patterns of soil nematodes across grasslands on the Tibetan plateau: relationships with climate, soil and plants. Diversity 13:369. doi: 10.3390/d13080369
Contosta, A. R., Frey, S. D., and Cooper, A. B. (2011). Seasonal dynamics of soil respiration and N mineralization in chronically warmed and fertilized soils. Ecosphere 2:art36. doi: 10.1890/ES10-00133.1
Cortois, R., Veen, G. F. C., Duyts, H., Abbas, M., Strecker, T., Kostenko, O., et al. (2017). Possible mechanisms underlying abundance and diversity responses of nematode communities to plant diversity. Ecosphere 8:e01719. doi: 10.1002/ecs2.1719
De Deyn, G. B., Raaijmakers, C. E., Van Ruijven, J., Berendse, F., and Van Der Putten, W. H. (2004). Plant species identity and diversity effects on different trophic levels of nematodes in the soil food web. Oikos 106, 576–586. doi: 10.1111/j.0030-1299.2004.13265.x
DeForest, J. L., Zak, D. R., Pregitzer, K. S., and Burton, A. J. (2004). Atmospheric nitrate deposition, microbial community composition, and enzyme activity in northern hardwood forests. Soil Sci. Soc. Am. J. 68, 132–138. doi: 10.2136/sssaj2004.1320
Dorich, R. A., and Nelson, D. W. (1983). Direct colorimetric measurement of ammonium in potassium chloride extracts of soils. Soil Sci. Soc. Am. J. 47, 833–836. doi: 10.2136/sssaj1983.03615995004700040042x
Du, G.-Z., Qin, G.-L., Li, Z.-Z., Liu, Z.-H., and Dong, G.-S. (2003). Relationship between species richness and productivity in an alpine Meadow Plant community. Chin. J. Plant Ecol. 27, 125–132. doi: 10.17521/cjpe.2003.0019
Freschet, G. T., Pagès, L., Iversen, C. M., Comas, L. H., Rewald, B., Roumet, C., et al. (2021). A starting guide to root ecology: strengthening ecological concepts and standardising root classification, sampling, processing and trait measurements. New Phytol. 232, 973–1122. doi: 10.1111/nph.17572
Hooper, D. U., Bignell, D. E., Brown, V. K., Brussard, L., Mark Dangerfield, J., Wall, D. H., et al. (2000). Interactions between aboveground and belowground biodiversity in terrestrial ecosystems: patterns, mechanisms, and feedbacks. Bioscience 50:1049. doi: 10.1641/00063568(2000)050[1049:IBAABB]2.0.CO;2
Hu, J., Chen, G., Hassan, W. M., Chen, H., Li, J., and Du, G. (2017). Fertilization influences the nematode community through changing the plant community in the Tibetan plateau. Eur. J. Soil Biol. 78, 7–16. doi: 10.1016/j.ejsobi.2016.11.001
Laliberté, E., Legendre, P., and Shipley, B. (2014). FD: Measuring functional diversity (FD) from multiple traits, and other tools for functional ecology. R package version 1.0-12.1, 1-12.
Lefcheck, J. S. (2016). piecewiseSEM: piecewise structural equation modelling in r for ecology, evolution, and systematics. Methods Ecol. Evol. 7, 573–579. doi: 10.1111/2041-210X.12512
Leff, J. W., Jones, S. E., Prober, S. M., Barberán, A., Borer, E. T., Firn, J. L., et al. (2015). Consistent responses of soil microbial communities to elevated nutrient inputs in grasslands across the globe. Proc. Natl. Acad. Sci. 112, 10967–10972. doi: 10.1073/pnas.1508382112
Legay, N., Clément, J. C., Grassein, F., Lavorel, S., Lemauviel-Lavenant, S., Personeni, E., et al. (2020). Plant growth drives soil nitrogen cycling and N-related microbial activity through changing root traits. Fungal Ecol. 44:100910. doi: 10.1016/j.funeco.2019.100910
Li, Q., Bai, H., Liang, W., Xia, J., Wan, S., and van der Putten, W. H. (2013). Nitrogen addition and warming independently influence the belowground micro-food web in a temperate steppe. PLoS One 8:e60441. doi: 10.1371/journal.pone.0060441
Liang, W., Lou, Y., Li, Q., Zhong, S., Zhang, X., and Wang, J. (2009). Nematode faunal response to long-term application of nitrogen fertilizer and organic manure in Northeast China. Soil Biol. Biochem. 41, 883–890. doi: 10.1016/j.soilbio.2008.06.018
Liu, T., Guo, R., Ran, W., Whalen, J. K., and Li, H. (2015). Body size is a sensitive trait-based indicator of soil nematode community response to fertilization in rice and wheat agroecosystems. Soil Biol. Biochem. 88, 275–281. doi: 10.1016/j.soilbio.2015.05.027
Liu, X., Lu, Y., Zhang, Z., and Zhou, S. (2020). Foliar fungal diseases respond differently to nitrogen and phosphorus additions in Tibetan alpine meadows. Ecol. Res. 35, 162–169. doi: 10.1111/1440-1703.12064
Liu, Y., Shi, G., Mao, L., Cheng, G., Jiang, S., Ma, X., et al. (2012). Direct and indirect influences of 8 yr of nitrogen and phosphorus fertilization on Glomeromycota in an alpine meadow ecosystem. New Phytol. 194, 523–535. doi: 10.1111/j.1469-8137.2012.04050.x
Liu, A., Yang, T., Xu, W., Shangguan, Z., Wang, J., Liu, H., et al. (2018). Status, issues and prospects of belowground biodiversity on the Tibetan alpine grassland. Biodivers. Sci. 26, 972–987. doi: 10.17520/biods.2018119
Lorenzen, S. (1994). The phylogenetic systematics of free-living nematodes[M]. London: Ray Society, 1–383.
Mulder, C., den Hollander, H. A., Vonk, J. A., Rossberg, A. G., Jagers op Akkerhuis, G. A. J. M., and Yeates, G. W. (2009). Soil resource supply influences faunal size–specific distributions in natural food webs. Naturwissenschaften 96, 813–826. doi: 10.1007/s00114-009-0539-4
Mulder, C., and Elser, J. J. (2009). Soil acidity, ecological stoichiometry and allometric scaling in grassland food webs: Soil pH, Stoichiometry And Allometric Scaling. Glob. Chang. Biol. 15, 2730–2738. doi: 10.1111/j.1365-2486.2009.01899.x
Murray, P. J., Cook, R., Currie, A. F., Dawson, L. A., Gange, A. C., Grayston, S. J., et al. (2006). Interactions between fertilizer addition, plants and the soil environment: implications for soil faunal structure and diversity. Appl. Soil Ecol. 33, 199–207. doi: 10.1016/j.apsoil.2005.11.004
Neher, D. A. (2010). Ecology of plant and free-living nematodes in natural and agricultural soil. Annu. Rev. Phytopathol. 48, 371–394. doi: 10.1146/annurev-phyto-073009-114439
Nelson, D. W., and Sommers, L. E. (1982). “Total carbon, organic carbon and organic matter” in Methods of soil analysis, part 2. eds. A. L. Page, R. H. Miller, and D. R. Keenay (Madison: ASA), 539–580.
Niu, K., Choler, P., de Bello, F., Mirotchnick, N., Du, G., and Sun, S. (2014). Fertilization decreases species diversity but increases functional diversity: a three-year experiment in a Tibetan alpine meadow. Agric. Ecosyst. Environ. 182, 106–112. doi: 10.1016/j.agee.2013.07.015
Niu, K., He, J.-S., and Lechowicz, M. J. (2016). Foliar phosphorus content predicts species relative abundance in P-limited Tibetan alpine meadows. Perspect. Plant Ecol. Evol. Syst. 22, 47–54. doi: 10.1016/j.ppees.2016.08.002
Niu, S., Wu, M., Han, Y., Xia, J., Zhang, Z., Yang, H., et al. (2010). Nitrogen effects on net ecosystem carbon exchange in a temperate steppe: Carbon Flux Responses To Nitrogen Enrichment. Glob. Chang. Biol. 16, 144–155. doi: 10.1111/j.1365-2486.2009.01894.x
Oksanen, J. (2016). Design decisions and implementation details in vegan. The vignette of the package is vegan. R package version, 2–4.
Pan, F., Han, X., Mclaughlin, N. B., Li, C., and Xu, Y. (2015). Effect of long-term fertilization on free-living nematode community structure in Mollisols. J. Soil Sci. Plant Nutr. 15, 129–141. doi: 10.4067/S0718-95162015005000011
Pey, B., Nahmani, J., Auclerc, A., Capowiez, Y., Cluzeau, D., Cortet, J., et al. (2014). Current use of and future needs for soil invertebrate functional traits in community ecology. Basic Appl. Ecol. 15, 194–206. doi: 10.1016/j.baae.2014.03.007
Picot, A., Monnin, T., and Loeuille, N. (2019). From apparent competition to facilitation: impacts of consumer niche construction on the coexistence and stability of consumer-resource communities. Funct. Ecol. 33, 1746–1757. doi: 10.1111/1365-2435.13378
Qi, R. Response of plant community to nitrogen and phosphorous additions in sub-alpine meadows of the Qinghai Tibetan plateau[D]. Diss. Lanzhou University, Lanzhou, (2013).
Qi, S., Zhao, X., Zheng, H. X., and Lin, Q. (2010). Changes of soil biodiversity in Inner Mongolia steppe after 5 years of N and P fertilizer applications. Acta Ecol. Sin. 30, 5518–5526.
Reay, D. S., Dentener, F., Smith, P., Grace, J., and Feely, R. A. (2008). Global nitrogen deposition and carbon sinks. Nat. Geosci. 1, 430–437. doi: 10.1038/ngeo230
Reich, P. B., Hobbie, S. E., Lee, T., Ellsworth, D. S., West, J. B., Tilman, D., et al. (2006). Nitrogen limitation constrains sustainability of ecosystem response to CO2. Nature 440, 922–925. doi: 10.1038/nature04486
Rovira, A. D., and Simon, A. (1985). Growth, nutrition and yield of wheat in calcareous sandy loams of South Australia: effects of soil fumigation, fungicide, nematicide and nitrogen fertilizers. Soil Biol. Biochem. 17, 279–284. doi: 10.1016/0038-0717(85)90061-6
Ruan, W., Sang, Y., Chen, Q., Zhu, X., Lin, S., and Gao, Y. (2012). The response of soil nematode community to nitrogen, water, and grazing history in the inner Mongolian steppe, China. Ecosystems 15, 1121–1133. doi: 10.1007/s10021-012-9570-y
Sarathchandra, S. U., Ghani, A., Yeates, G. W., Burch, G., and Cox, N. R. (2001). Effect of nitrogen and phosphate fertilisers on microbial and nematode diversity in pasture soils. Soil Biol. Biochem. 33, 953–964. doi: 10.1016/S0038-0717(00)00245-5
Sparks, D. L., Page, A., Helmke, P., Loeppert, R., Soltanpour, P., Tabatabai, M., et al. (1996). Methods of soil analysis, part 3, Chemical Methods. Madison, WI: Soil Science Society of America, Madison.
Tita, G., Vincx, M., and Desrosiers, G. (1999). Size spectra, body width and morphotypes of intertidal nematodes: An ecological interpretation. J. Mar. Biol. Assoc. 79, 1007–1015. doi: 10.1017/S0025315499001241
van den Hoogen, J., Geisen, S., Routh, D., Ferris, H., Traunspurger, W., Wardle, D. A., et al. (2019). Soil nematode abundance and functional group composition at a global scale. Nature 572, 194–198. doi: 10.1038/s41586-019-1418-6
Villenave, C., Saj, S., Pablo, A.-L., Sall, S., Djigal, D., Chotte, J.-L., et al. (2010). Influence of long-term organic and mineral fertilization on soil nematofauna when growing Sorghum bicolor in Burkina Faso. Biol. Fertil. Soils 46, 659–670. doi: 10.1007/s00374-010-0471-y
Violle, C., Navas, M.-L., Vile, D., Kazakou, E., Fortunel, C., Hummel, I., et al. (2007). Let the concept of trait be functional! Oikos 116, 882–892. doi: 10.1111/j.0030-1299.2007.15559.x
Vitousek, P. M., Aber, J. D., Howarth, R. W., Likens, G. E., Matson, P. A., Schindler, D. W., et al. (1997). Human alteration of the global nitrogen cycle: sources and consequences. Ecol. Appl. 7, 737–750. doi: 10.1890/1051-0761(1997)007[0737:HAOTGN]2.0.CO;2
Wang, J., Liao, L., Ye, Z., Liu, H., Zhang, C., Zhang, L., et al. (2022). Different bacterial co-occurrence patterns and community assembly between rhizosphere and bulk soils under N addition in the plant–soil system. Plant and Soil 471, 697–713. doi: 10.1007/s11104-021-05214-2
Wang, W., Ma, Y., Xu, J., Wang, H., Zhu, J., and Zhou, H. (2012). The uptake diversity of soil nitrogen nutrients by main plant species in Kobresia humilis alpine meadow on the Qinghai-Tibet plateau. Sci. China Earth Sci. 55, 1688–1695. doi: 10.1007/s11430-012-4461-9
Wang, Y., and Niu, K. (2020). Effect of soil environment on the functional diversity of soil nematodes in Tibetan alpine meadows. Biodivers. Sci. 28, 707–717. doi: 10.17520/biods.2020042
Wang, K., Sipes, B. S., and Schmitt, D. P. (2002). Crotalaria as a cover CROP for nematode management: a review. Nematropica 32, 35–57. doi: 10.1046/j.1365-2907.2002.00105.x
Wang, X., Xiao, S., Yang, X., Liu, Z., Zhou, X., Du, G., et al. (2019). Dominant plant species influence nematode richness by moderating understory diversity and microbial assemblages. Soil Biol. Biochem. 137:107566. doi: 10.1016/j.soilbio.2019.107566
Wei, C., Zheng, H., Li, Q., Lü, X., Yu, Q., Zhang, H., et al. (2012). Nitrogen addition regulates soil nematode community composition through ammonium suppression. PLoS One 7:e43384. doi: 10.1371/journal.pone.0043384
Wu, J. H. (1999). Studies on freshwater and soil nematodes in China. Institute of Hydrobiology, Chinese Academy of Science.
Yang, Y., Ji, C., Ma, W., Wang, S., Wang, S., Han, W., et al. (2012). Significant soil acidification across northern China’s grasslands during 1980s-2000s. Glob. Chang. Biol. 18, 2292–2300. doi: 10.1111/j.1365-2486.2012.02694.x
Yang, H., Jiang, L., Li, L., Li, A., Wu, M., and Wan, S. (2012). Diversity-dependent stability under mowing and nutrient addition: evidence from a 7-year grassland experiment: diversity-dependent stability in steppe. Ecol. Lett. 15, 619–626. doi: 10.1111/j.1461-0248.2012.01778.x
Yang, J. E., Kim, J. J., Skogley, E. O., and Schaff, B. E. (1998). A simple spectrophotometric determination of nitrate in water, resin, and soil extracts. Soil Sci. Soc. Am. J. 62, 1108–1115. doi: 10.2136/sssaj1998.03615995006200040036x
Yang, Z., Powell, J. R., Zhang, C., and Du, G. (2012). The effect of environmental and phylogenetic drivers on community assembly in an alpine meadow community. Ecology 93, 2321–2328. doi: 10.1890/11-2212.1
Yeates, G. (1999). Effects Of Plants On Nematode Community Structure. Annu. Rev. Phytopathol. 37, 127–149. doi: 10.1146/annurev.phyto.37.1.127
Yeates, G. W., Bongers, T., De Goede, R. G., Freckman, D. W., and Georgieva, S. S. (1993). Feeding habits in soil nematode families and genera-an outline for soil ecologists. J. Nematol. 25, 315–331.
Zhang, Z.-W., Li, Q., Hu, Y.-Y., Wei, H.-W., Hou, S.-L., Yin, J.-X., et al. (2022). Nitrogen and phosphorus additions interactively affected composition and carbon budget of soil nematode community in a temperate steppe. Plant and Soil 473, 109–121. doi: 10.1007/s11104-021-05145-y
Zhao, J., Wang, F., Li, J., Zou, B., Wang, X., Li, Z., et al. (2014). Effects of experimental nitrogen and/or phosphorus additions on soil nematode communities in a secondary tropical forest. Soil Biol. Biochem. 75, 1–10. doi: 10.1016/j.soilbio.2014.03.019
Keywords: alpine meadow, soil nematode, fertilization, functional diversity, species diversity, root traits
Citation: Qi Y, Sun X, Peng S, Tan X and Zhou S (2023) Effects of fertilization on soil nematode communities in an alpine meadow of Qinghai-Tibet plateau. Front. Ecol. Evol. 11:1122505. doi: 10.3389/fevo.2023.1122505
Edited by:
Xiang Liu, Lanzhou University, ChinaCopyright © 2023 Qi, Sun, Peng, Tan and Zhou. This is an open-access article distributed under the terms of the Creative Commons Attribution License (CC BY). The use, distribution or reproduction in other forums is permitted, provided the original author(s) and the copyright owner(s) are credited and that the original publication in this journal is cited, in accordance with accepted academic practice. No use, distribution or reproduction is permitted which does not comply with these terms.
*Correspondence: Xiaodan Tan, dGFueGlhb2RhbjExNDE0M0AxNjMuY29t; Shurong Zhou, emhzaHJvbmdAaGFpbmFudS5lZHUuY24=