- 1Museum für Naturkunde, Berlin – Leibniz Institute for Evolution and Biodiversity Science, Berlin, Germany
- 2Berlin-Brandenburg Institute of Advanced Biodiversity Research, Berlin, Germany
- 3Leibniz Centre for Agricultural Landscape Research (ZALF), Müncheberg, Germany
Urbanization leads to drastic modifications of the terrestrial and aquatic environment. However, urban ponds may provide valuable habitats for different taxa, including aquatic insects and amphibians. We aim to understand how a set of biotic and abiotic factors influence aquatic insect diversity in 18 urban ponds in the German metropolis Berlin, one of the greenest whilst most densely populated European cities. Greenspace is important for the terrestrial stages of some aquatic insects and amphibians, providing crucial resources. Thus, greenspace was assumed to have positive effects on aquatic insect diversity, whereas built-up area was assumed to affect diversity negatively. Because some aquatic insects prey on tadpoles, their abundance and diversity were assumed to depend on tadpole abundance, which in turn, depends on other food (i.e., phytoplankton) availability in ponds. We visited the ponds twice a year, in spring and summer, and collected data on aquatic insects that are known to prey on tadpoles, tadpole abundance, phytoplankton biomass, the presence or absence of large insect predators, as well as physical–chemical parameters. We assumed higher total aquatic insect abundance, genera richness, alpha-diversity, and evenness, as well as abundance and genera richness of different aquatic insect taxonomic groups to be associated with high tadpole abundance in ponds surrounded by high amount of greenspace and low levels of built-up area. Accordingly, we expected aquatic insects to be modulated by phytoplankton biomass, the presence of newts and fish, and to be affected by ponds’ abiotic conditions. Our results showed that biological interactions and abiotic water conditions override urban effects in ponds’ terrestrial surroundings on aquatic insect diversity levels, whereas aquatic insects’ taxonomic groups responded differently on different land-use types around ponds. We explain our findings due to different dependences and demands towards terrestrial and/or aquatic habitats by different taxonomic groups of aquatic insects, and differences in their colonization behavior.
1. Introduction
Urban areas are defined as cultural landscapes where humans live at high densities, using constructed areas for housing, work and movement (Pickett et al., 2011; Wu, 2014). The process of urbanization drastically impacts the environment (Antrop, 2000; McIntyre, 2000). However, while former habitats within cities disappear, novel urban ecosystems are emerging (McIntyre, 2000; Hobbs et al., 2013), which have multifaceted negative and positive effects on therein living species (McKinney, 2008). On the one hand, urbanization is known to reduce species richness (McKinney, 2002, 2008) and biodiversity (Shochat et al., 2010), which is mainly caused by habitat loss and fragmentation (Goddard et al., 2010; Liu et al., 2016). On the other hand, urban ecosystems often offer higher habitat heterogeneity on small spatial scales compared to rural areas (Kühn et al., 2004). For instance, man-made urban greenspaces, such as parks, cemeteries, private and residential gardens provide valuable habitats for many species (Haaland and van den Bosch, 2015; Niemeier et al., 2020).
So far, most studies addressing biodiversity in urban ecosystems, focused on terrestrial and lotic habitats (Blair and Launer, 1997; Paul and Mayer, 2001; Blakely et al., 2006; Fontana et al., 2011). In particular, ponds, which are known to host high aquatic faunal and floral diversity, remained understudied (Biggs et al., 2005; Dudgeon et al., 2006; Goertzen and Suhling, 2013; Hassall, 2014). Ponds are defined as small, lentic, often temporary water bodies, usually with an area below two hectares (Biggs et al., 2005; De Meester et al., 2005). Urban ponds are known to provide valuable habitats for a number of rare and endangered species (Williams et al., 2003; Biggs et al., 2005; Colding et al., 2009; Goertzen and Suhling, 2013), such as amphibians (Brand and Snodgrass, 2009) and various aquatic insects (Goertzen and Suhling, 2013; Hill et al., 2015; Deacon et al., 2019). Especially aquatic insects have so far been mostly neglected in urban studies (but see Gledhill et al., 2008; Goertzen and Suhling, 2013; Briers, 2014; Noble and Hassall, 2014; Hassall and Anderson, 2015; Hill et al., 2015).
Aquatic insects however, have important ecological roles within the food-web of pond ecosystems, such as serving as prey for newts (Joly and Giacoma, 1992), and fish (Peckarsky, 1982; Vidotto-Magnoni and Carvalho, 2009; Glenn and Cormier, 2014), as well as being predators, e.g., on tadpoles (Cummins, 1973; Henrikson, 1990; see Wells, 2007 for review; Hanlon and Relyea, 2013; Müller and Brucker, 2015), but exhibit a wide food range by feeding additionally on phytoplankton, zooplankton, detritus, insect larvae, as well as eggs from newts and fish (Miaud, 1993; Yee, 2010; Culler et al., 2014; Hädicke et al., 2017).
Many aquatic insects can serve as reliable biological indicators for environmental change, like water pollution (Zapparoli, 1997; Muzón et al., 2019), and are known to be sensitive to ponds’ abiotic conditions, such as oxygen saturation, pH or water temperature (Winter et al., 2002; Simaika and Samways, 2011; Pallarés et al., 2017; Briggs et al., 2019; Jooste et al., 2020). As most aquatic insects have complex amphibiotic life cycles, with aquatic, semiaquatic, and terrestrial/aerial developmental stages, they are sensitive to environmental changes in the aquatic and the terrestrial habitat (Huryn et al., 2008; Smith and Lamp, 2008; Smith et al., 2009). In general, urban ponds are influenced by the effects of many human activities (Urban et al., 2006), such as habitat fragmentation (Johnson et al., 2013), modification and/or habitat loss (Gopal, 2013), or the establishment of invasive species. For instance, a study by Buria et al. (2007) showed that fish deliberately introduced in urban ponds have a direct negative impact on aquatic insect diversity and abundance.
Amphibians likewise have complex life cycles and are sensitive to environmental change (Catenazzi, 2015). Anuran larvae are predominantly feeding on periphyton and planktonic organisms, whereas newts and their larvae are carnivorous (Harrison, 1987; Griffiths, 1996). Holtmann et al. (2017) show that amphibians respond negatively to built-up areas in the vicinity of their breeding habitats. In particular, species richness decreased due to a loss in habitat connectivity. In contrast, a study of Niemeier et al. (2020) showed how cities might positively affect animals. These authors found that the European Common Frog (Rana temporaria) actually performed better in urban greenspaces with ponds compared to frogs in rural, agricultural areas.
In our study, we aimed to understand how urbanization affects aquatic insects on the levels of diversity and taxonomic groups. To assess the effects of biotic and abiotic urban pond conditions on aquatic insects, we examined 18 ponds, surrounded by different amounts of built-up area and greenspace, in the city of Berlin, Germany. We predicted aquatic insect abundance, richness, alpha-diversity, and evenness to be affected by ponds’ terrestrial surroundings.
Based on studies by Buchwald (1992), Gledhill et al. (2008), Briers (2014), and Hill et al. (2015), revealing higher macroinvertebrate diversity in park ponds than in other urban ponds, we assumed (i) aquatic insect abundance and diversity to increase with increasing amount of greenspace, and on the other hand, we predicted lower aquatic insect abundance and diversity in ponds surrounded by high percentage of built-up area (Heino et al., 2017).
We predicted (ii) the presence and abundance of amphibian larvae to have a positive relation with predatory aquatic insect abundance and diversity measures (Cummins, 1973; Henrikson, 1990; Wells, 2007; Hanlon and Relyea, 2013; Müller and Brucker, 2015). Because periphyton and planktonic organisms are food sources for tadpoles (Harrison, 1987; Griffiths, 1996), we assumed (iii) high chlorophyll-a concentration (indicating phytoplankton biomass), to influence aquatic insects positively due to high habitat quality for tadpoles as aquatic insect prey. In contrast, we anticipated (iv) large predators to have negative effects on aquatic insect abundance and diversity measures, because aquatic insects serve as prey for newts (Joly and Giacoma, 1992), and fish (Peckarsky, 1982; Vidotto-Magnoni and Carvalho, 2009; Glenn and Cormier, 2014).
Beside land-use effects and biotic interactions, we predicted (v) abiotic water conditions, such as oxygen saturation, pH, and water temperature to impact aquatic insect abundance and diversity measures (Fox and Taylor, 1955; Picot et al., 1993; Verberk and Bilton, 2013; Figure 1). For instance, high pH leads to toxic water conditions (Picot et al., 1993; Tadesse et al., 2004; Tucker and D’Abramo, 2008), and low oxygen saturation might result in aquatic insect mortality (Fox and Taylor, 1955).
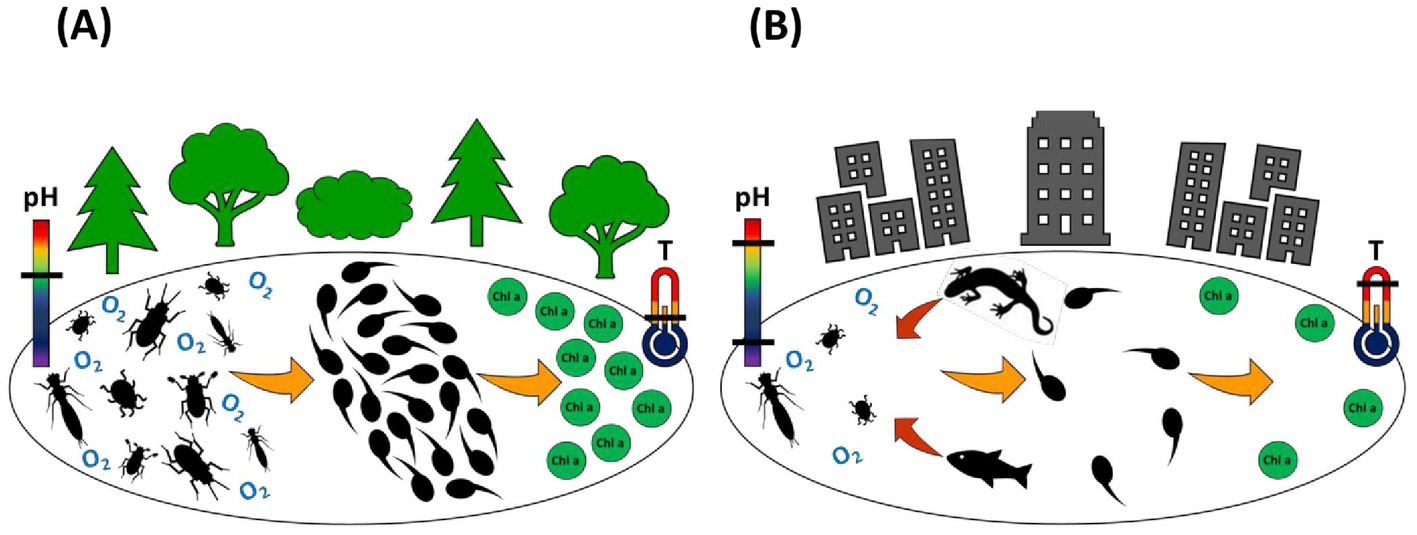
Figure 1. Predictions of biotic and abiotic factors, affecting aquatic insect abundance, genera richness, alpha-diversity, and evenness (A) positively: high amount of greenspace in ponds’ terrestrial surroundings, neutral pH, high oxygen saturation (O2), high tadpole abundance (as prey), high chlorophyll-a concentration (Chl-a, as tadpole food source), and moderate water temperatures (T, thermometer); (B) negatively: high amount of build-up area in ponds’ terrestrial surroundings, acidic or alkali pH, low oxygen saturation (O2), low tadpole abundance (as prey), low chlorophyll-a concentrations (Chl-a, as tadpole food source), high water temperatures (T, thermometer), and the presence of large predators on aquatic insects (newts and fish) (positive and negative feedbacks of biotic and abiotic factors are not considered).
Because aquatic insects are no functionally homogenous group, we assumed different taxonomic groups of aquatic insects, such as dragonfly larvae (Odonata), adults of aquatic beetles (Coleoptera), and bugs (Heteroptera) to react differently on ponds’ biotic and abiotic conditions, depending on their dominant life cycle in water or terrestrial habitats. Namely, we expected (vi) larvae to be more affected by water conditions because of their exclusively aquatic stages (Corbet, 1999), and (vii) volant adult stages to be more influenced by land-use around ponds, because most aquatic beetles and heteropterans exhibit high flight and dispersal abilities to colonize new habitats as uncolonized ponds (Bilton, 1994; Bilton et al., 2001; Bloechi et al., 2010).
2. Materials and methods
2.1. Study area
The German city of Berlin (52°31′N, 13°24′E) is covering an area of 891 km2 and supports nearly 3.7 million human inhabitants (Amt für Statistik Berlin-Brandenburg, 2020). Although Berlin is one of the most densely populated cities in Europe, it is simultaneously one of the greenest (Schewenius et al., 2014).
2.2. Study pond selection and urbanization measures
In late March/early April in 2019 and 2021, we selected ponds in which at least one of three anuran species, the European Common Frog Rana temporaria, the Moor Frog Rana arvalis, and/or the European Common Toad Bufo bufo were present. All three species are known to occur in Berlin (data received from the Stiftung Naturschutz, Berlin), and reproduce in March to April (Kühnel et al., 2017). Their tadpoles feed on periphyton and planktonic organisms (Harrison, 1987). The estimation of chlorophyll-a concentration (reflecting phytoplankton biomass) may thus be used as a proxy for tadpoles’ food availability (Minshall, 1978; Wood and Richardson, 2009).
We chose ponds surrounded by different amounts of built-up area and greenspace to assess land-use effects. All our ponds were at least partly covered by riparian vegetation like reed, hard stem bulrush, and sedge, and present a comparable hydroperiod, drying up only every few years after sustained precipitation deficit. Twelve of our 18 study ponds are natural, and six are artificial, created between the years 1900 and 1980 (see Table 1).
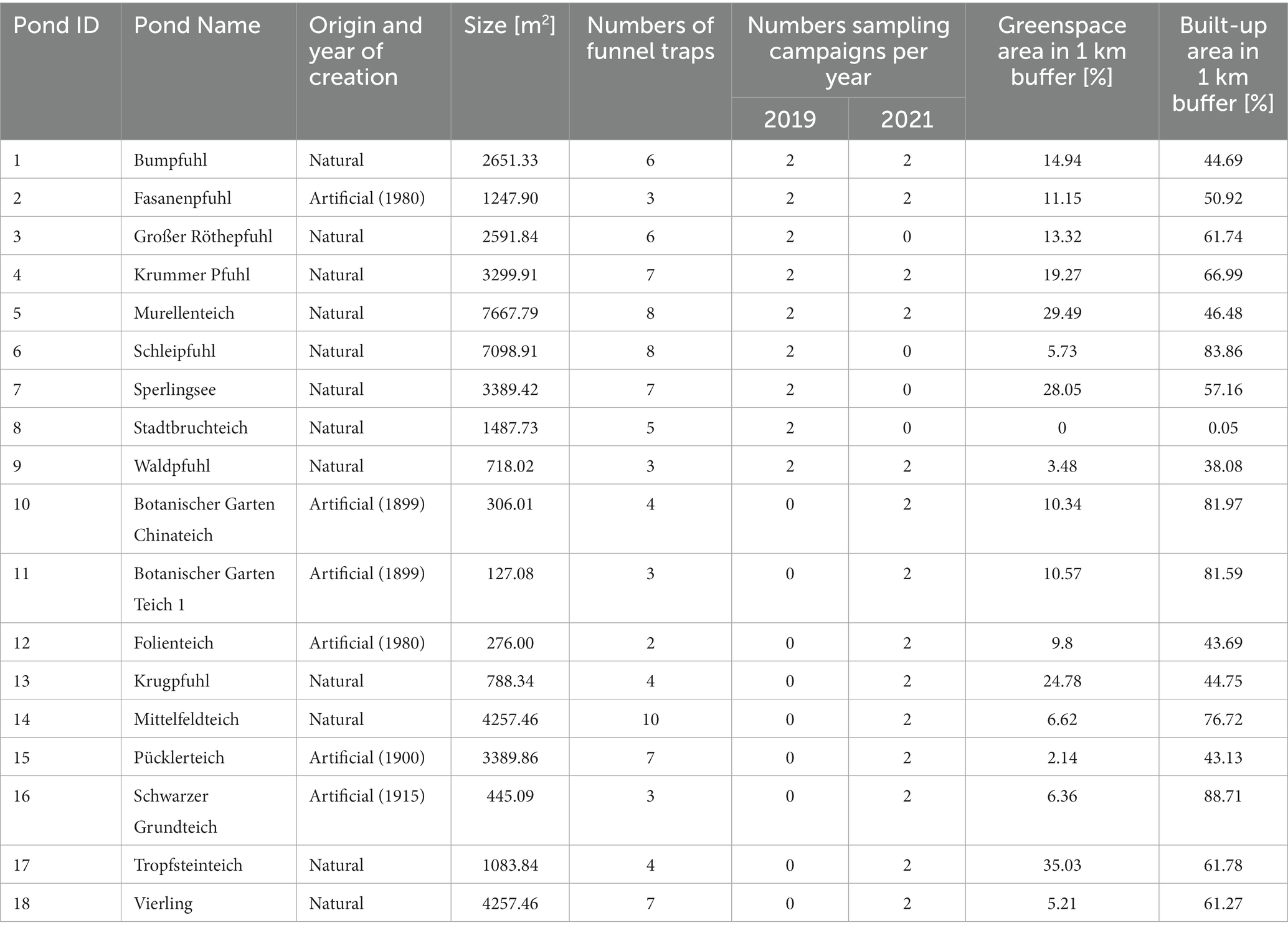
Table 1. List of study ponds (compare Figure 2), given are pond IDs, names, origin with approximate year of creation, pond size, the numbers of funnel traps per sampling campaign, numbers of sampling campaigns per year, and the calculated percentage of greenspace and built-up area in a 1 km buffer zone around the respective pond.
We investigated nine ponds in 2019 and 13 ponds in 2021, five ponds were investigated in both years (Figure 2; Table 1). Pond size was determined with QGIS (Version: 3.28; QGIS Development Team, 2021), using habitat type maps (Senatsverwaltung für Stadtentwicklung und Umwelt: Berlin, Germany, 2005). We calculated the percentage of built-up area and greenspace surrounding ponds in a radius of 1 km, by using digital habitat maps available from the city of Berlin (Senatsverwaltung für Stadtentwicklung und Umwelt: Berlin, Germany, 2005) with QGIS (Version: 3.28; QGIS Development Team, 2021). The 1 km buffer zone around ponds was chosen based on studies by Johnson et al. (2013), Dolný et al. (2014), and Niemeier et al. (2020), showing that the 1 km buffer was the most relevant zone for the studied organisms because it covers the approximate distribution of volant aquatic insects and amphibians in urban landscapes. Greenspace was defined as human modified areas, dominated by vegetation (non-sealed surfaces), like private and residential gardens, parks, cemeteries, etc. (Haaland and van den Bosch, 2015; Niemeier et al., 2020). Build-up areas comprised residual or industrial areas, as well as other infrastructure (streets, railways). The percentage of greenspace around ponds ranged from 0 to 35.03%. The percentage of built-up area in the 1 km circle around ponds ranged from 0.05 to 81.07% (Table 1).
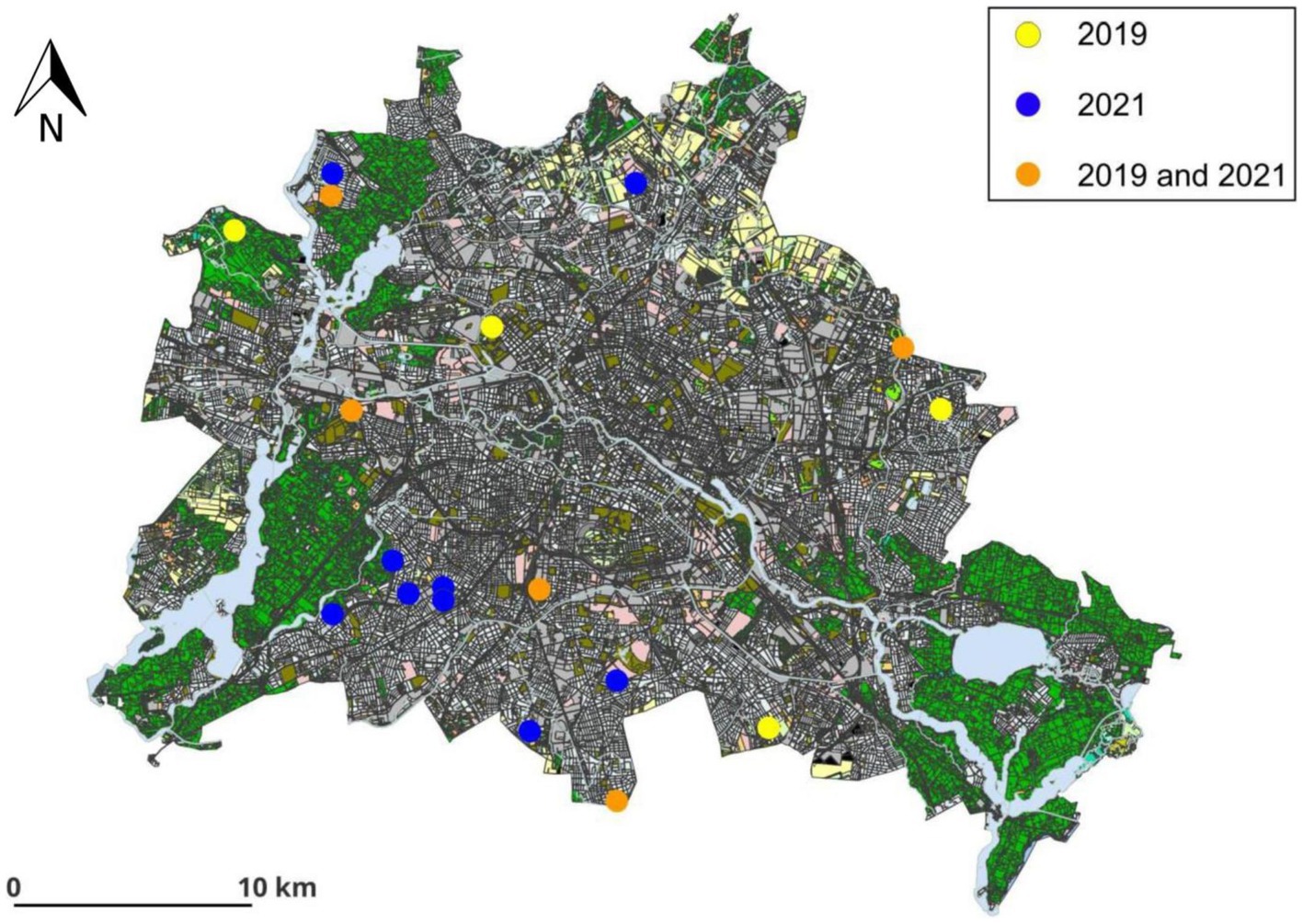
Figure 2. Locations of study ponds in Berlin, sampled in 2019 (yellow dots; n = 4), in 2021 (blue dots; 9), and in both years (orange dots; n = 5). North is indicated by a compass.
2.3. Abiotic data assessment
Abiotic parameters included oxygen saturation (%), pH, and water temperature (°C). These physical–chemical parameters were measured in situ in all sampling campaigns at a similar time of the day using portable electronic meters (Xylem Analytics Germany Sales GmbH, WTW, Weilheim, Germany; see Table 2 and Supplementary Table B for all measured biotic and abiotic parameters). We additionally assessed electric conductivity simultaneously to water abiotic parameters and found that all studied ponds fall within the freshwater range (Mean: 581.82 ± SD: 477.96 μs/cm).
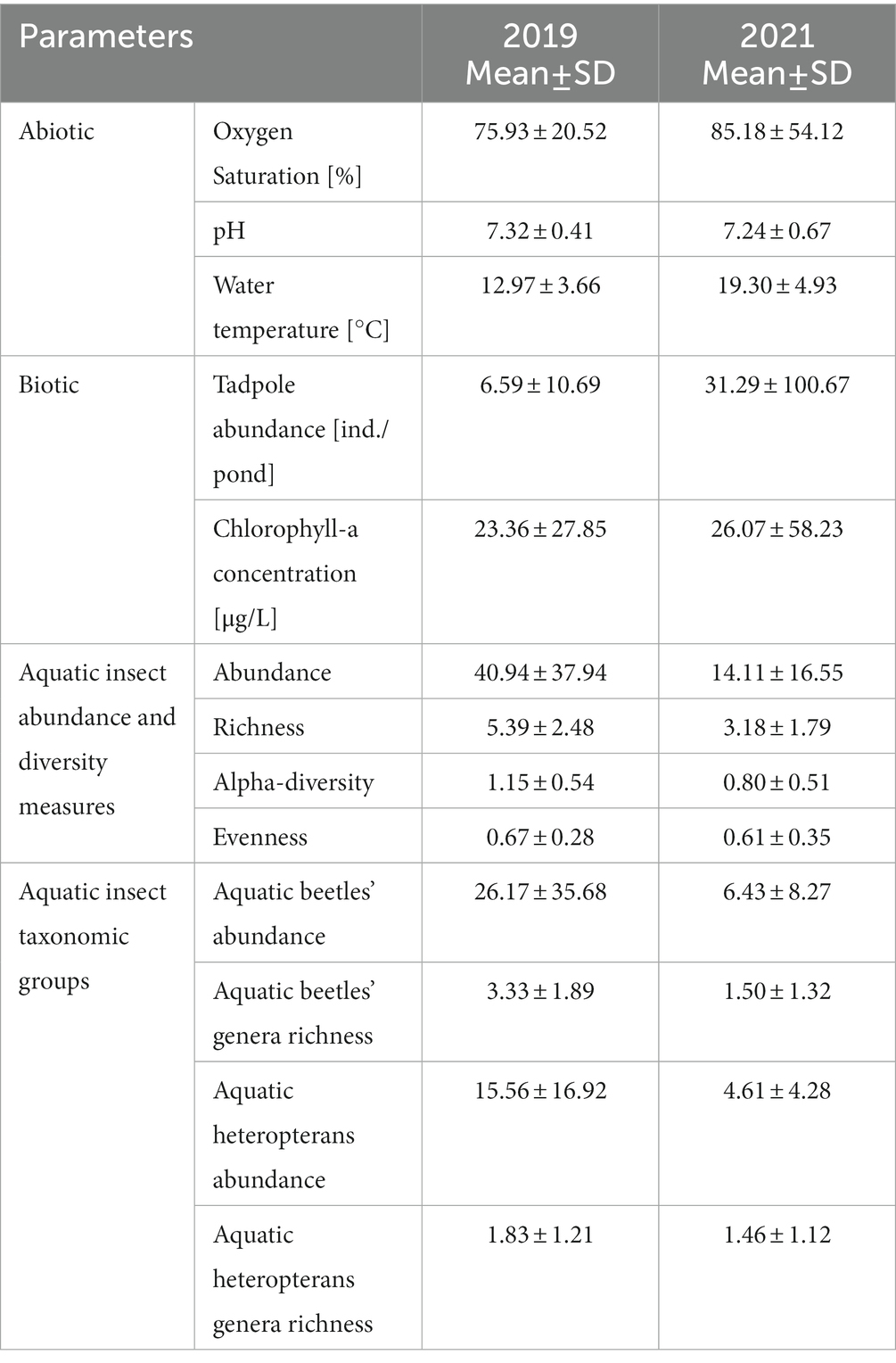
Table 2. Mean and standard deviation of abiotic and biotic parameters along with aquatic insect abundance and biodiversity measures (genera richness, alpha-diversity, evenness) and abundance and genera richness of aquatic insect taxonomic groups (aquatic beetles and heteropterans) in the studied ponds in 2019 (n = 9) and 2021 (n = 13).
2.4. Biotic data assessment
Biotic parameters included tadpole abundance, the presence or absence of fish and newts, and chlorophyll-a concentration. We recorded the presence of the anuran species Rana temporaria, Rana arvalis, and Bufo bufo by the presence of mating adults and/or spawn. In both years, we started a first sampling campaign in May, when all tadpoles reached a developmental stage in which they freely move and feed in the water. The second sampling campaign was conducted 3 weeks later, in June, prior to tadpoles’ metamorphosis.
In order to assess the presence, as well as the abundance of tadpoles, fish, newts and aquatic insects, we used commercial fish funnel traps (28 × 28 × 75 cm; Paladin, Angelplatz.de). We modified the funnel traps by narrowing the entrances with cut-off heads of PET bottle-necks. This makes it more difficult for animals, which entered the trap, to leave again. A former study has shown that modified traps are much more efficient in catching newts (Rödel et al., 2014). The number of funnel traps per pond was adjusted to pond size and pond heterogeneity (presence of different microhabitats; i.e. branches, stones, reeds, and other floating vegetation such as water lilies). In ponds ranging from 127 to 445 m2 surface area (N = 4) we installed two to four funnel traps; in ponds with 718–1,247 m2 (fewer microhabitats; N = 4) three to four funnel traps were used; ponds comprising 1,487–2,651 m2 (N = 3) were equipped with five to six traps; and the largest ponds (3,299–7,667 m2; N = 7) comprised seven to ten funnel traps (Table 1).
Funnel trapping extended 12 h, from early evening to the next morning. We equipped all traps with pieces of polystyrene, to ensure that one corner of the trap provided access to atmospheric oxygen. All captured animals were identified [amphibians (larvae and adults): species level; insects (larvae and adults): genus level], counted and released. We collected data of all aquatic insects that potentially feed on amphibian larvae [Coleoptera (larvae and adults): Dytiscidae, Hydrophilidae; Hemiptera (adults): Notonectidae, Corixidae, Naucoridae, Nepidae; Odonata (larvae): Aeshnidae, Corduliidae, Aeshnidae, and Gomphidae]. In addition to the funnel trap records we verified the presence or absence of fish and newts in the ponds by observations of surfacing individuals, and dip netting. For further analyses, we used tadpole abundance, calculated as the pooled number of tadpoles from all present anuran species (insect food availability), captured in all funnel traps per pond during a particular sampling campaign (May, June) and year (2019, 2021).
We determined the chlorophyll-a concentration per pond and sampling campaign as proxy for tadpole food availability (phytoplankton biomass). Water samples were prefiltered through a 100 μm mesh to remove detritus and organisms. These water samples were further filtered onto glassfiber filters (GF/F, Cytiva Europe GmbH, Freiburg, Germany), and then immediately placed inside a glass vessel and stored at −80°C in the dark until further processing. Chlorophyll-a was extracted with 96% ethanol and measured spectrophotometrically (DIN 38 412–16, 1985).
2.5. Aquatic insect abundance, diversity, and taxonomic groups
To assess aquatic insect abundance, we summed up numbers of all insect specimens, captured in all funnel traps per pond during each sampling campaign per year (N = 1,071). We calculated three measures of biodiversity: genera richness, alpha-diversity, and evenness. For the calculation of insect genera richness, we summed up all captured genera in all funnel traps per pond including both sampling campaign per year (N = 22). We applied Shannon-Wiener-Index (H’ ) Shannon and Weaver, 1949; Spellerberg and Fedor, 2003) for aquatic insect alpha-diversity calculations per pond including both sampling campaigns per year, using the formula:
Where is the number of individuals of each genus , and is the total number of genera for each pond.
For aquatic insect genera evenness analyses, we used the Pielou Evenness-Index (E’ ) Pielou, 1969; Hill, 1973) for each sampling campaign per year and pond, by using the formula:
Where H’ is the Shannon-Wiener-Index, and is the total numbers of genera.
To assess dragonfly larvae abundance, we summed up numbers of all dragonfly larvae, captured in all funnel traps per pond during each sampling campaign per year (N = 10). We calculated aquatic beetles’ abundance by summarize numbers of all water-and diving beetles captured in all funnel traps per pond during each sampling campaign per year (N = 652). To assess aquatic heteropteran abundance, we summed up numbers of all water heteropterans, captured in all funnel traps per pond during both sampling campaigns and years (N = 409). For aquatic beetle genera richness, and aquatic heteropteran genera richness calculations, we summed up numbers of all water-and diving beetle genera (N = 12), and the genera of aquatic heteropterans (N = 6), captured in all funnel traps during each sampling campaign per year, respectively. We did not calculate dragonfly larvae genera richness due to very low numbers of captured dragonfly larvae.
2.6. Statistical analyses
We used the R-project, version 4.1.3 (R Core Team, 2022) for all analyses, and ggplot2 (R package ‘ggplot2’; Wickham, 2016) for the visualization of model outputs. For testing normal distribution of our dependent variables (aquatic insect abundance, genera richness, alpha-diversity and evenness, dragonfly larvae abundance, aquatic beetle abundance, aquatic beetle genera richness, aquatic heteropteran abundance, aquatic heteropteran genera richness), we applied Shapiro Wilk tests and histograms. We further tested our response variables for equality of variance, by using the Levene-test. For our models we considered the percentage of built-up area, the percentage of greenspace, tadpole abundance, chlorophyll-a concentration, oxygen saturation, pH, and temperature as independent variables.
In a next step, we explored our data by testing our dependent (aquatic insect abundance and diversity measures) and independent (biotic and abiotic parameters) variables on outliers, non-linearity, and collinearity/multicollinearity. We found one true outlier in different ponds in the following variables: tadpole abundance, chlorophyll-a concentration, oxygen saturation, pH, and temperature. We chose to keep these outliers in our dataset as they represent natural variation. We thoroughly revised our data prior to statistically analyses and discarded measurement, counting or logging errors. We found no evidence on non-linearity between our dependent and independent variables when applying Ramsey’s RESET test (R package: lmtest; Ramsey, 1969; Krämer and Sonnberger, 1986). Further, we found no collinearity/multicollinearity between our independent variables when applying a correlation matrix.
The distribution of our dependent variables, representing count data (aquatic insect abundance and genera richness) was explored and tested on overdispersion for a Poisson distribution (Zuur et al., 2009). Because overdispersion was given for aquatic insect abundance, we applied generalized linear mixed-effects models (GLMMs; R package ‘lme4’; Bates et al., 2015) for negative binomial family (R package ‘MASS’; Venables and Ripley, 2002) to analyze biotic and/or abiotic effects on ponds’ aquatic insect abundance.
Therefore, we used ‘abundance’ as dependent variable and ran two models with ‘built-up area’ or ‘greenspace’ as fixed effects to avoid model overfitting, respectively. In addition, we included chlorophyll-a concentrations ‘chl-a’, ‘tadpole abundance’, the presence or absence of ‘fish’ and ‘newts’, oxygen saturation ‘O2’, ‘pH’, and ‘temperature’ as further fixed effects in both GLMMs. We included ‘sampling year’, ‘sampling campaign’, and ‘pond ID’ as random effects.
Because overdispersion for a Poisson distribution was not given for aquatic insect genera richness, we applied GLMMs for Poisson family (link function: sqrt) with ‘richness’ as dependent variable and again ‘built-up area’ or ‘greenspace’ as fixed effects for two separate models for analyzing biotic and/or abiotic effects on ponds’ aquatic insect genera richness. Again, we included ‘chl-a’, ‘tadpole abundance’, ‘fish’, ‘newts’, ‘O2’, pH’, and ‘temperature’ as fixed effects and ‘sampling year’, ‘sampling campaign’, and ‘pond ID’ as random effects in both models, respectively.
To analyze biotic and/or abiotic effects on ponds’ insect genera alpha-diversity and evenness, we ran GLMMs for Gaussian family (link function: identity). Dependent variables were ‘diversity’ and ‘evenness’. In each of the two models we used ‘built-up area’ or ‘greenspace’ as fixed effects, respectively. We included again ‘chl-a’, ‘tadpole abundance’, ‘fish’, ‘newts’, ‘O2’, ‘pH’, and ‘temperature’ as fixed effects and ‘sampling year’, ‘sampling campaign’, and ‘pond ID’ as random effects.
The distribution of dependent variables, representing count data for taxonomic groups (aquatic beetle and heteropteran abundance and genera richness) was explored and tested on overdispersion for a Poisson distribution (Zuur et al., 2009). Because overdispersion was given for aquatic beetle and heteropteran abundance, we applied generalized linear mixed-effects models (GLMMs; R package ‘lme4’; Bates et al., 2015) for negative binomial family (R package ‘MASS’; Venables and Ripley, 2002) to analyze biotic and/or abiotic effects on ponds’ aquatic insect abundance.
Therefore, we used ‘aquatic beetle abundance’ and ‘aquatic heteropteran abundance’ as dependent variables for the analyses of biotic and/or abiotic effects, respectively. For each dependent variable, we ran two models with ‘built-up area’ or ‘greenspace’ as fixed effects. Further we included again, ‘chl-a’, ‘tadpole abundance’, ‘fish’, ‘newts’, ‘O2’, pH’, and ‘temperature’ as fixed effects. ‘Sampling year’, ‘sampling campaign’, and ‘pond ID’ were included as random effects in both models, respectively.
Because overdispersion for a Poisson distribution was not given for aquatic beetle and heteropteran genera richness, we applied GLMMs for Poisson family (link function: sqrt) with ‘aquatic beetle genera richness’, and ‘aquatic heteropteran genera richness’ as response variables for the analyses of biotic and/or abiotic effects, respectively. For each dependent variable, we ran two models with ‘built-up area’ or ‘greenspace’ as fixed effects. Further we included ‘chl-a’, ‘tadpole abundance’, ‘fish’, ‘newts’, ‘O2’, pH’, and ‘temperature’ as fixed effects, and included ‘sampling year’, ‘sampling campaign’, and ‘pond ID’ as random effects in both models.
3. Results
We captured a total of 20 and 16 genera of predatory aquatic insects in 2019 and 2021, respectively. The genera belonged to the orders Coleoptera (Dytiscidae, Hydrophilidae); Hemiptera (Notonectidae, Corixidae, Naucoridae, Nepidae); and Odonata (Aeshnidae, Corduliidae, Aeshnidae, Gomphidae). Numbers of captured insects per pond and sampling campaign are provided in Supplementary Table B.
Abiotic and biotic parameters varied substantially across ponds and sampling years. Water temperature varied three to nearly fivefold, oxygen saturation up to fiftyfold, whereas pH showed small variations. The numbers of tadpoles, however, varied ten to hundredfold, and the concentration of chlorophyll-a varied up to nearly sixtyfold. Aquatic insect abundance and diversity measures, such as genera richness, alpha-diversity, and evenness, as well as abundance and genera richness of the taxonomic groups’ aquatic beetles and heteropterans showed huge variation in ponds, such as abundance of aquatic insects varied over thirtyfold (see Table 2).
When considering the random effects ‘sampling year’ (2019, 2021), ‘sampling campaign’ (May, June), and ‘pond ID’, the percentage of ‘built-up area’ surrounding ponds did not have significant associations with alpha-diversity and evenness of aquatic insect genera (Supplementary Tables A3, A4). In contrast, we revealed a trend to a negative effect on aquatic insect abundance and genera richness when ‘built-up area’ increased (Figure 3A; Supplementary Tables A1, A2). The amount of ‘greenspace’ had no significant relation with either aquatic insect genera richness, alpha-diversity or evenness. However, we found a trend to a positive association between increasing amount of ‘greenspace’ and increasing aquatic insect abundance (Figure 3B; Supplementary Tables A1–A4).
We found trends to positive effects of high ‘tadpole abundance’ on aquatic insect genera richness and alpha-diversity. This positive trend was visible in both GLMMs, run for ‘built-up area’ and ‘greenspace’, respectively (Supplementary Tables A2, A3). We did not find associations between ‘tadpole abundance’ and aquatic insect abundance and evenness in either model (Supplementary Tables A1, A4).
The concentration of ‘chl-a’ correlated negatively with aquatic insect abundance in the model run for ‘greenspace’, and a negative trend was visible in the model run for ‘built-up area’ (Figure 3C; Table 3; Supplementary Table A1). ‘Chl-a’ did not have effects on aquatic insect genera richness, alpha-diversity and evenness (Supplementary Tables A2–A4).
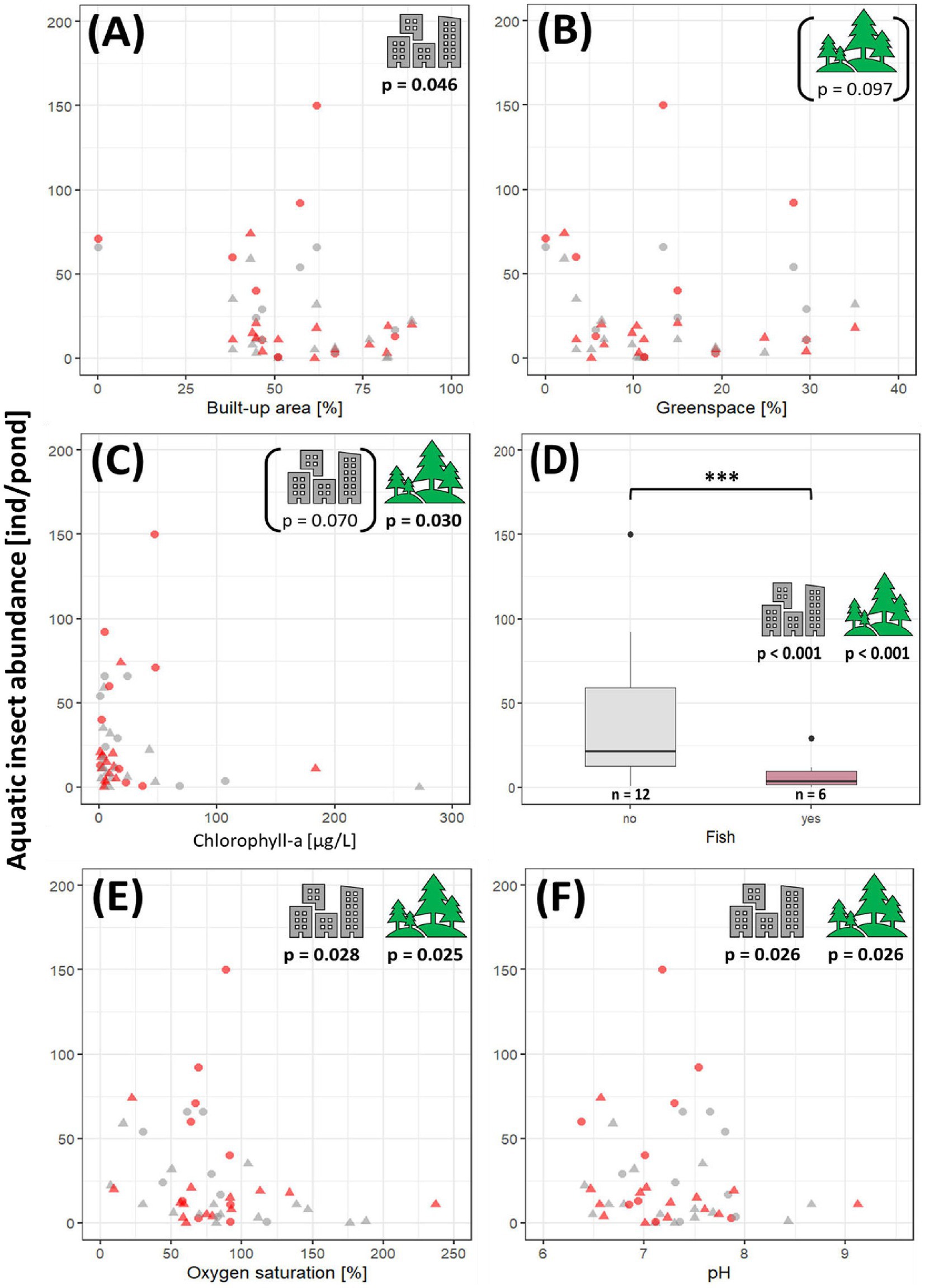
Figure 3. Aquatic insect abundance in individuals per pond influenced by (A) built-up area, (B) greenspace (C) chlorophyll-a concentration, (D) presence or absence of fish, (E) oxygen saturation, and (F) pH in ponds. Dot plots for count data are shown for the sampling years 2019 (dots; n = 9) and 2021 (triangles; n = 13), and sampling campaigns May (red color) and June (gray color). Boxplots show insect abundance in ponds without (gray) and with the presence of fish (purple). Numbers below boxplots show sample sizes. p-values are given for generalized linear mixed effect model results for built-up area and greenspace (Icons) with sampling year (2019, 2021), sampling campaign (May, June), and pond ID as random effects. Icons in brackets describe examined trends.
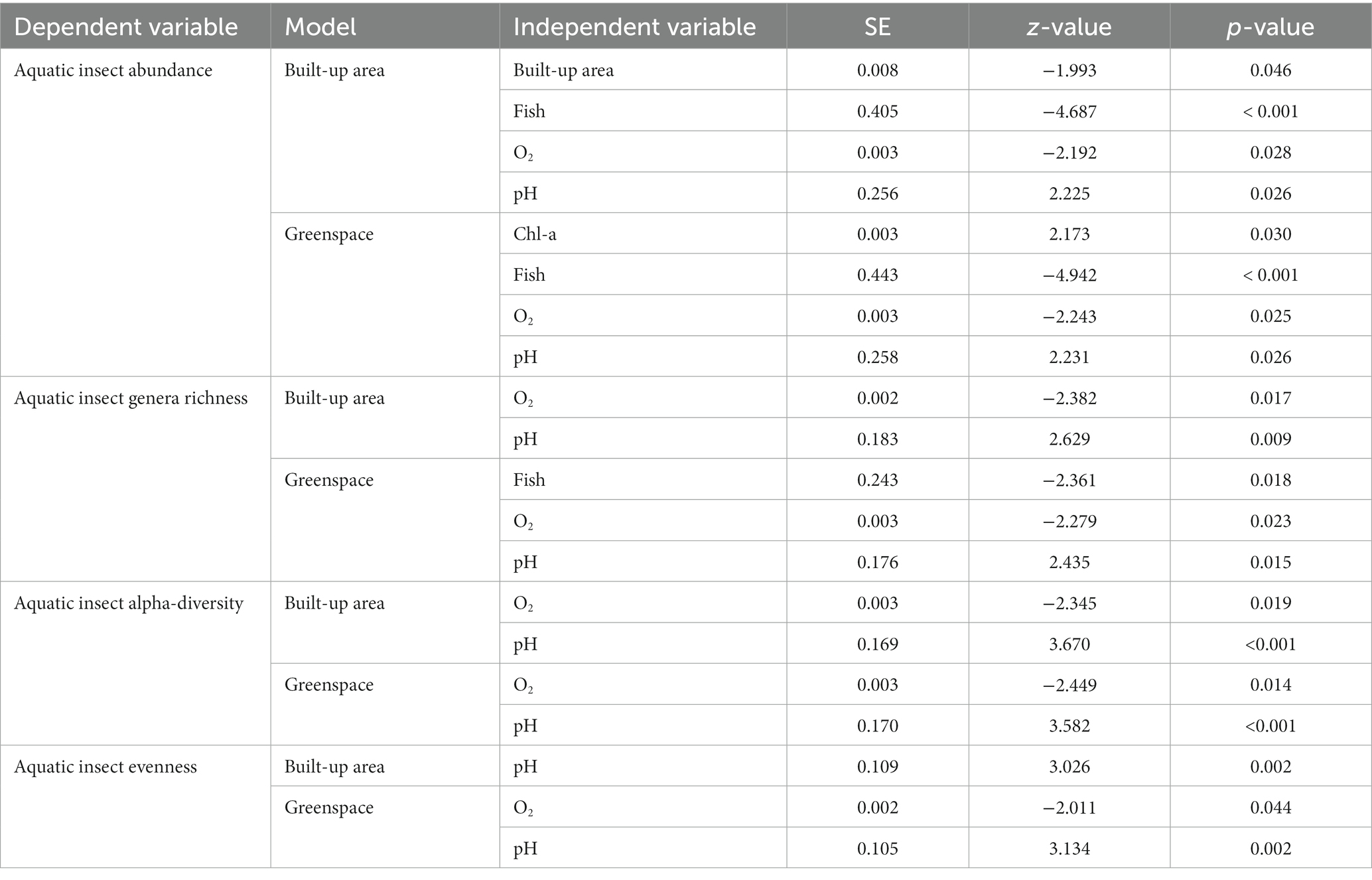
Table 3. Summary statistics of significant generalized linear mixed effects models testing the effect of fixed effects on aquatic insect abundance, genera richness, alpha-diversity and evenness (see non-significant model results in Supplementary Tables A1–A8 in Supplementary material).
We also found presence of ‘fish’ having negative effects on aquatic insect abundance in both GLMMs, run for ‘built-up area’ and ‘greenspace’, respectively (Figure 3D; Table 3; Supplementary Table A1). Aquatic insect genera richness was negatively affected by the presence of ‘fish’ in GLMM for ponds surrounded by ‘greenspace’, but only a trend to a negative association was visible when running the model with amount of ‘built-up area’ (Figure 4A; Table 3; Supplementary Table A2). ‘Fish’ did not correlate with aquatic insect alpha-diversity and evenness (Supplementary Tables A3, A4), neither did ‘newts’ affect aquatic insect abundance or any of our diversity measures (genera richness, alpha-diversity, and evenness; Supplementary Tables A1–A4).
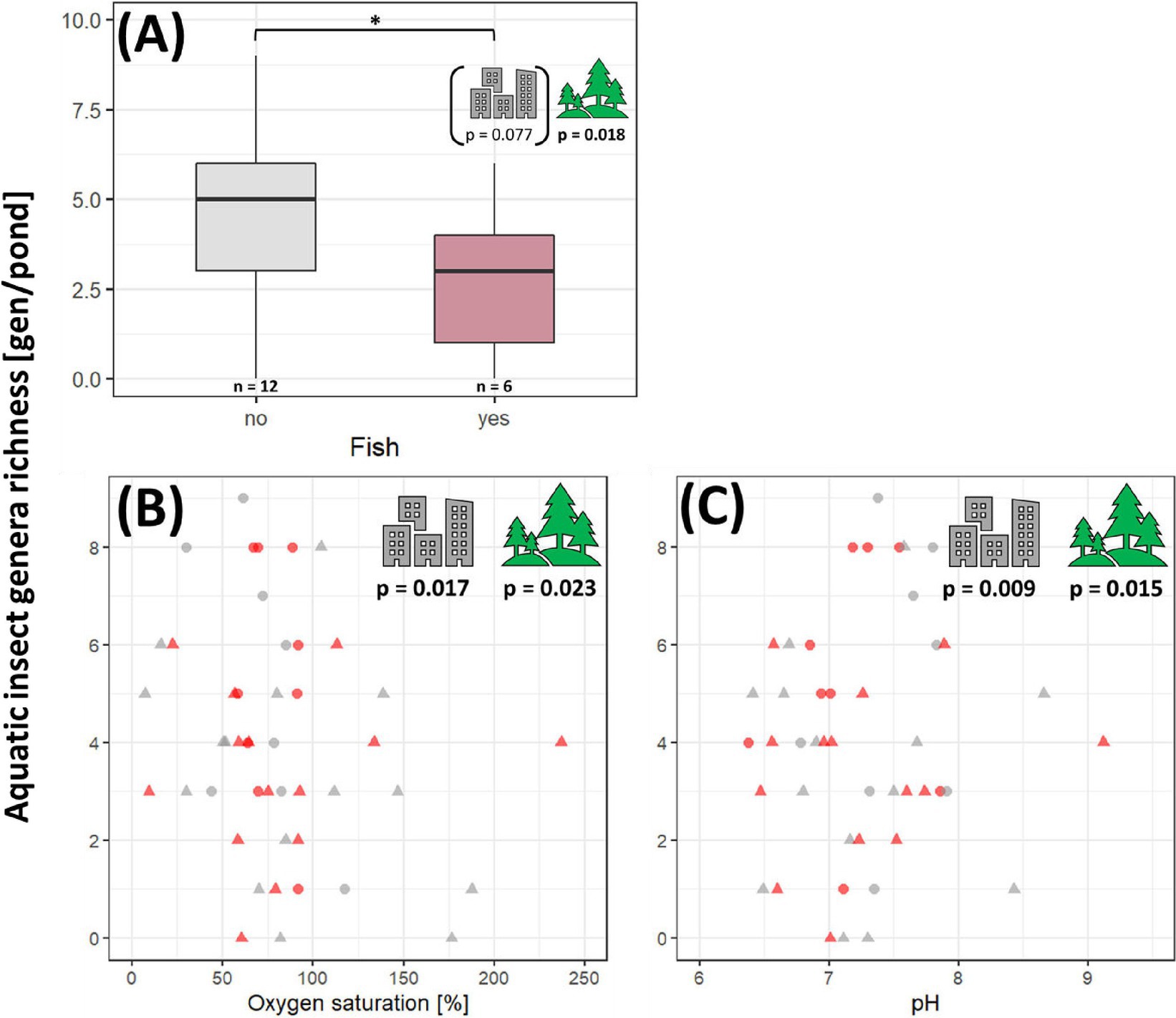
Figure 4. Aquatic insect genera richness in genera per pond influenced by (A) presence or absence of fish, (B) oxygen saturation (O2), and (C) pH in ponds. Boxplots show insect genera richness in ponds without (gray) and with the presence of fish (purple). Numbers below boxplots show sample sizes. Dot plots for count data are shown for the sampling years 2019 (dots; n = 9) and 2021 (triangles; n = 13), and sampling campaigns May (red color) and June (gray color). p-values are given for generalized linear mixed effect model results for built-up area and greenspace (Icons) with sampling year (2019, 2021), sampling campaign (May, June), and pond ID as random effects. Icons in brackets describe examined trends.
Oxygen saturation showed negative effects on aquatic insect abundance (Figure 3E), genera richness (Figure 4B), and alpha-diversity (Figure 5A) in both models, with ‘built-up area’ and ‘greenspace’ (Table 3; Supplementary Tables A1–A3). We further revealed a significant negative correlation between high ‘O2’ saturations and insect genera evenness in GLMM run for ‘greenspace’, and a trend towards a negative correlation in the model run for ‘built-up area’ (Figure 5C; Table 3; Supplementary Table A4).
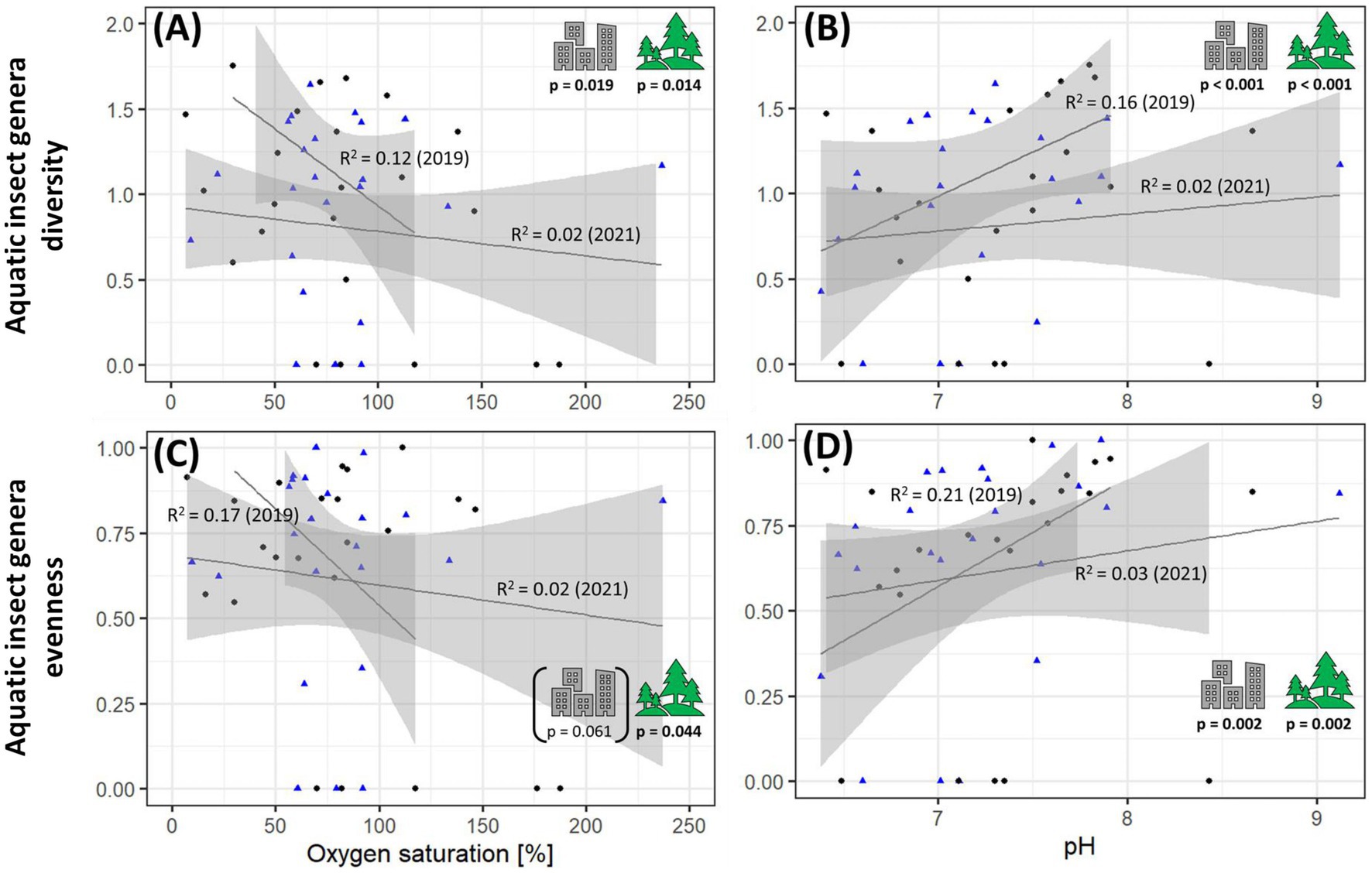
Figure 5. Aquatic insect genera alpha-diversity (A,B), and evenness (C,D) influenced by oxygen saturation (O2) (A,C), and pH (B,D). Smoothed regression lines are shown for the sampling years 2019 (n = 9) and 2021 (n = 13). Points show different sampling campaigns (blue triangles: May; black dots: June). p-values are given for generalized linear mixed effect model results for built-up area and greenspace (Icons) with sampling year (2019, 2021), sampling campaign (May, June), and pond ID as random effects. Icons in brackets describe examined trends.
High ‘pH’ values correlated negatively with aquatic insect abundance in both models (‘built-up area’ and ‘greenspace’; Figure 3F; Table 3; Supplementary Table A1). In contrast, insect genera richness, alpha-diversity, and evenness increased with increasing ‘pH’ values, independent of ‘built-up area’ and ‘greenspace’ (Figures 4C, 5B,D; Table 3; Supplementary Tables A2–A4). Our results revealed no significant effects of ‘temperature’ on aquatic insect abundance or any of the diversity measures in both models (Supplementary Tables A1–A4).
Contrary to our expectations, ponds’ terrestrial surroundings did only affect aquatic beetles’ abundance but not our diversity measures. However, diversity measures were affected by ponds biotic and abiotic conditions.
Because aquatic insects exhibit different life cycles, dominant in water or terrestrial habitats, in a second step, we examined aquatic insects regarding their taxonomic groups to understand their sensitivity to land-use and/or to biotic and abiotic water conditions. We examined aquatic beetles (Coleoptera) and bugs (Heteropteran), applying their abundance and genera richness. Due to the very low sample size of dragonfly larvae (Odonata; N = 10), captured in ponds during both sampling years, we excluded the analyses of dragonfly larvae abundance and genera richness.
When considering the random effects ‘sampling year’ (2019, 2021), ‘sampling campaign’ (May, June), and ‘pond ID’, we observed increasing aquatic beetle abundance (Figure 6B; Table 4; Supplementary Table A5) and genera richness (Figure 6D; Table 4; Supplementary Table A6) in ponds with increasing percentage of surrounded ‘greenspace’. We detected a trend towards a negative effect of increasing ‘built-up area’ on aquatic beetles’ abundance (Figure 6A; Supplementary Table A5), and a negative association with aquatic beetles’ genera richness (Figure 6C; Table 4; Supplementary Table A6). Aquatic heteropteran abundance and genera richness was neither affected by ‘greenspace’, nor ‘built-up area’ around ponds (Supplementary Tables A7, A8).
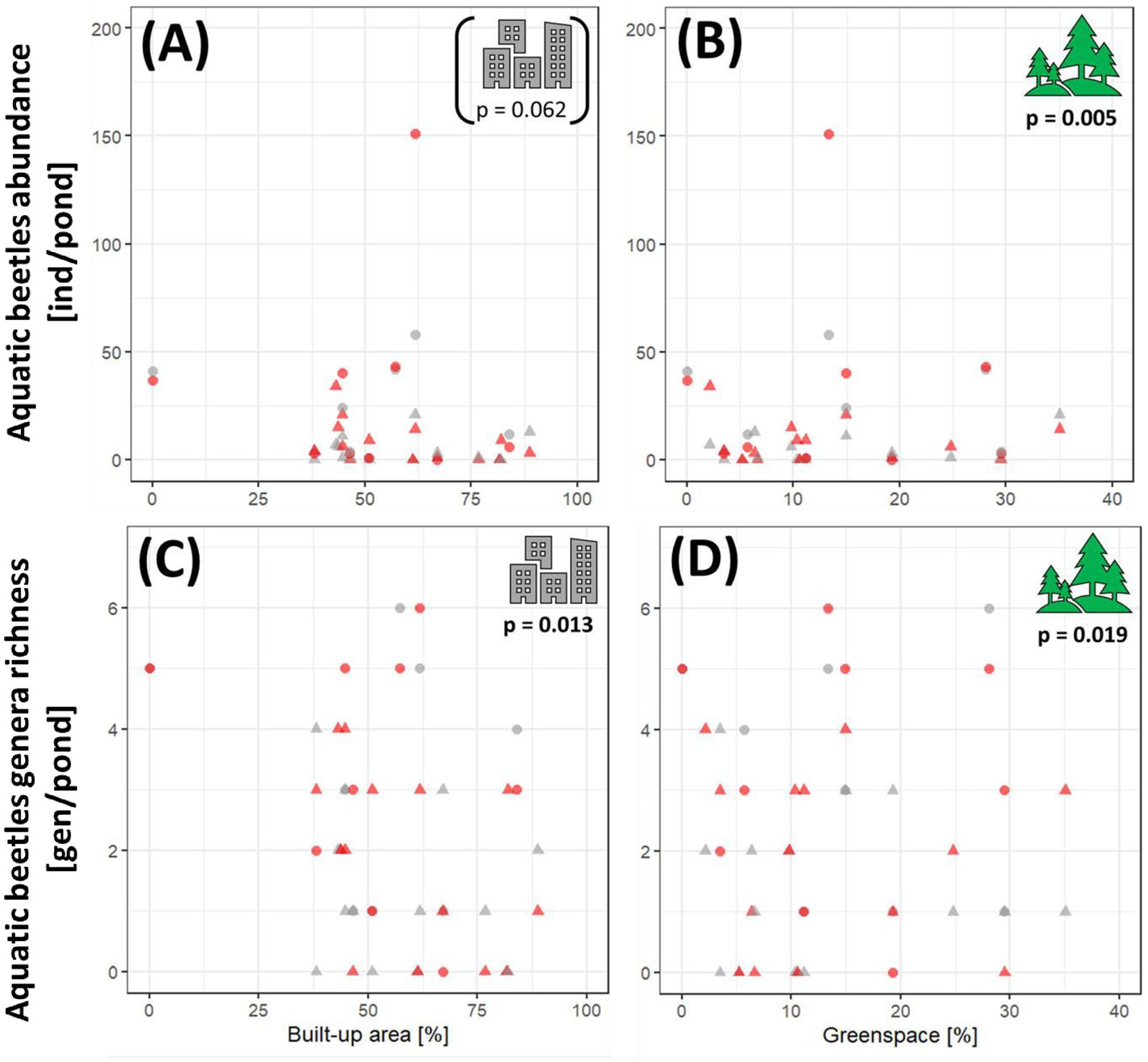
Figure 6. Aquatic beetles’ abundance in individuals per pond, influenced by the percentage of (A) built-up area and (B) greenspace around ponds, and aquatic beetles genera richness in genera per pond, influenced by (C) built-up area and (D) greenspace, 1 km around ponds, respectively. Dot plots for count data are shown for the sampling years 2019 (dots; n = 9) and 2021 (triangles; n = 13), and sampling campaigns May (red color) and June (gray color). p-values are given for generalized linear mixed effect model results for built-up area and greenspace (Icons) with sampling year (2019, 2021), sampling campaign (May, June), and pond ID as random effects. Icons in brackets describe examined trends.
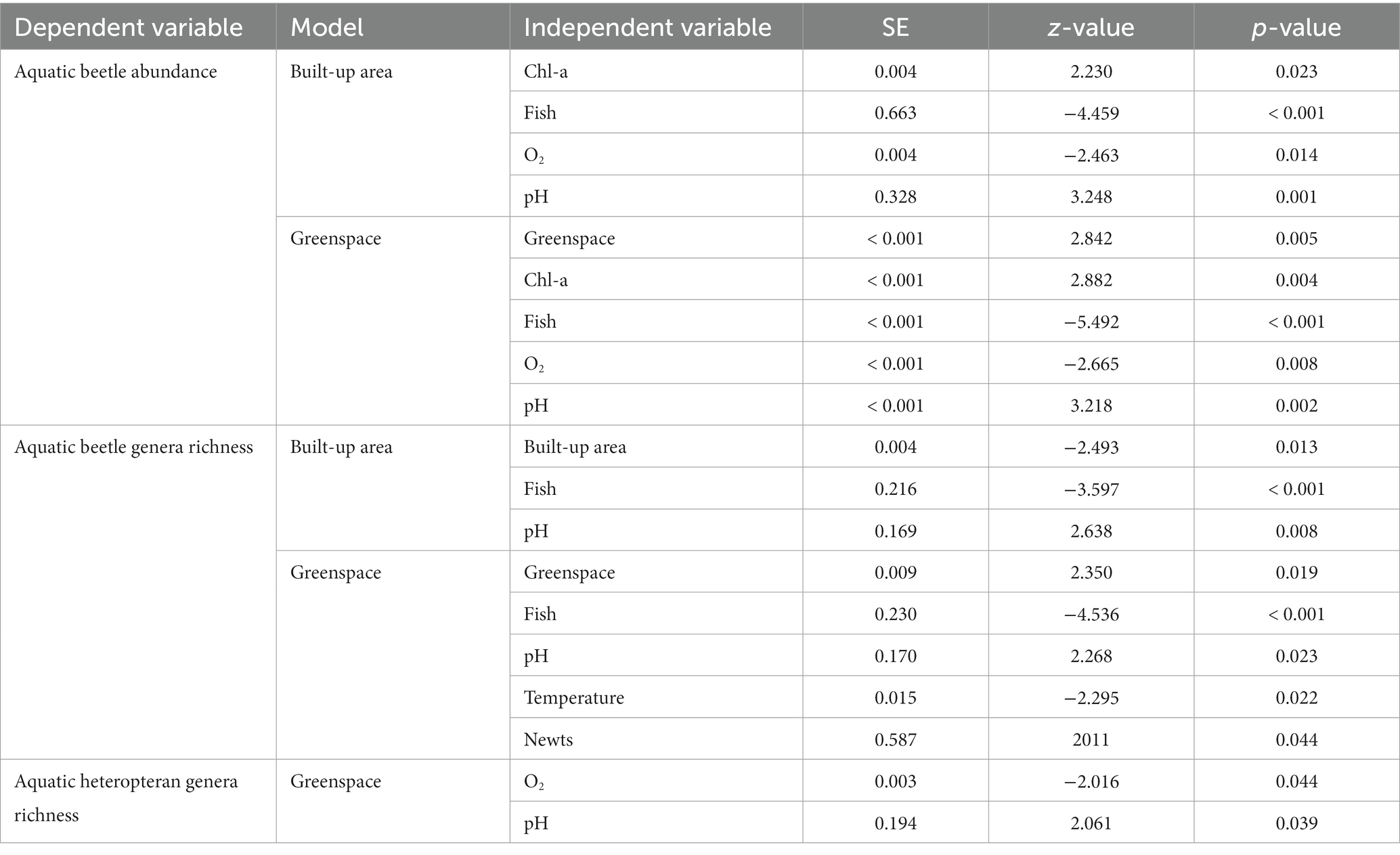
Table 4. Summary statistics of significant generalized linear mixed effects models testing the effect of fixed effects on aquatic beetle abundance and genera richness, and aquatic heteropterans abundance and genera richness (see non-significant model results in Supplementary Tables A1–A8 in Supplementary material).
‘Tadpole abundance’ had neither significant associations with aquatic beetle abundance and genera richness (Supplementary Tables A5, A6), nor with aquatic heteropteran abundance and genera richness (Supplementary Tables A7, A8). The lack of effects was visible in both GLMMs, run for ‘built-up area’ and ‘greenspace’, respectively.
In both models, with ‘built-up area’ and ‘greenspace’, we found decreasing beetles’ abundance with increasing concentration of ‘chl-a’ (Table 4; Supplementary Table A5), whereas we found no significant relation to aquatic beetles genera richness (Supplementary Table A6). ‘Chl-a’ concentrations had no significant effects on aquatic heteropteran abundance and genera richness, independent of ponds’ terrestrial surroundings (Supplementary Tables A7, A8).
In both models, with ‘built-up area’ and ‘greenspace’, the presence of ‘fish’ affected aquatic beetle abundance and genera richness negatively (Table 4; Supplementary Tables A5, A6), whereas we found no significant associations with aquatic heteropteran abundance and genera richness (Supplementary Tables A7, A8). The presence of ‘newts’ had neither effect on aquatic beetles’ abundance and genera richness (Supplementary Tables A5, A6), nor on heteropteran abundance and genera richness (Supplementary Tables A7, A8). The lack of effects was visible in both GLMMs, run for ‘built-up area’ and ‘greenspace’, respectively.
In both models, with ‘built-up area’ and ‘greenspace’, we found decreasing beetles’ abundance with increasing oxygen saturation ‘O2’ (Table 4; Supplementary Table A5), and trends to negative correlations on aquatic beetle genera richness (Supplementary Table A6). Oxygen saturation ‘O2’ was not significantly associated with aquatic heteropteran abundance, independent of ponds’ terrestrial surroundings (Supplementary Table A7). However, we revealed a negative correlation between increasing oxygen saturation ‘O2’ and heteropterans genera richness in ponds surrounded by ‘greenspace’ (Table 4; Supplementary Table A8), and a trend towards a negative correlation in ponds surrounded by ‘built-up area’ (Supplementary Table A8).
We found positive associations between increasing ‘pH’ and increasing aquatic beetles’ abundance and genera richness, independent on ponds terrestrial surroundings (Table 4; Supplementary Tables A5, A6). In contrast, ‘pH’ had no significant relation to aquatic heteropteran abundance in both models (Supplementary Table A7). However, we found a positive effect on heteropterans genera richness in ponds surrounded by ‘greenspace’ (Table 4; Supplementary Table A8), as well as a trend for a positive effect of increasing ‘pH’ on heteropteran genera richness in ponds surrounded by ‘built-up area’ (Supplementary Table A8).
We found no significant relation between water ‘temperature’ and aquatic beetle abundance in ponds surrounded by ‘built-up area’ and ‘greenspace’ (Supplementary Table A5). However, we found a negative effect of increasing ‘temperature’ on aquatic beetle genera richness in ponds surrounded by ‘greenspace’, but no significant association with ponds surrounded by ‘built-up area’ (Table 4; Supplementary Table A6). Ponds’ water ‘temperature’ had no significant effects on aquatic heteropteran abundance and genera richness, independent of ponds’ terrestrial surroundings (Supplementary Tables A7, A8). As expected, our results showed different aquatic insect taxonomic groups to be affected differently by ponds surrounded land-use, as well as biotic and abiotic conditions within ponds.
4. Discussion
Urbanization leads to modifications of the environment in order to serve humans as living space (Antrop, 2000; McIntyre, 2000). These drastic environmental changes also influence urban ponds (Urban et al., 2006; Gopal, 2013; Johnson et al., 2013). Due to their complex amphibiotic life cycle, aquatic insects are sensitive to aquatic and terrestrial environmental changes (Huryn et al., 2008; Smith and Lamp, 2008; Smith et al., 2009), and play important roles in pond food-webs (Cummins, 1973; Peckarsky, 1982; Joly and Giacoma, 1992; Vidotto-Magnoni and Carvalho, 2009; Glenn and Cormier, 2014). In our study, we aimed to know which biotic and abiotic conditions affect aquatic insect abundance and diversity measures, such as genera richness, alpha-diversity, and evenness in urban ponds surrounded by different amounts of greenspace and built-up area. Further, we aimed to understand if abundance and genera richness of different aquatic taxonomic groups (aquatic beetles, aquatic heteropterans, and dragonfly larvae) were affected differently by biotic and abiotic conditions.
We predicted high amount of built-up area around ponds to negatively affect different insect groups and their diversity, mainly due to unsuitability of the terrestrial habitats (Heino et al., 2017). In contrast, we assumed high amount of greenspace to have positive effects on aquatic insects, due to higher habitat quality for the terrestrial live stages (Buchwald, 1992; Gledhill et al., 2008; Briers, 2014; Hill et al., 2015). However, our results revealed that ponds’ terrestrial surroundings did not significantly influence aquatic insect diversity measures in our urban ponds. We found trends to negative effects of higher amount of built-up area around ponds on aquatic insect abundance and genera richness, as well as a trend to a positive effect on aquatic insect abundance in ponds surrounded by higher amounts of greenspace. This might hint on habitat loss and fragmentation, connected with a high percentage of built-up area, negatively impacting some aquatic insects in colonizing urban ponds (Smith et al., 2009), and/or that altered terrestrial urban habitats are less hospitable than unaltered ones for aquatic insects with terrestrial development stages. Terrestrial habitat alteration was shown to directly affect adult development, survival and mating success in aquatic insects (Smith et al., 2009), a finding that is further confirmed by studies of Gledhill et al. (2008), Briers (2014), and Hill et al. (2015). The latter studies showing lower macroinvertebrate diversity in ponds located in highly urbanized areas, compared to park ponds.
However, aquatic insects are not a functionally homogenous group. This particularly concerns the duration and the use of aquatic versus terrestrial environments throughout their life cycle. In a second step, we thus examined aquatic beetles, aquatic heteropterans, and dragonfly larvae separately. Unfortunately, the numbers of dragonfly larvae were too low to allow for in-depth analyses (but see below). Concerning the two other taxonomic groups, we found that land-use affected aquatic beetles and heteropterans differently. Our results showed that high amounts of built-up area around ponds tended to decrease aquatic beetles’ abundance (trend) and genera richness (significant), whereas more greenspace around ponds was positively associated with beetle abundance and genera richness. By contrast, aquatic heteropterans abundance and genera richness was affected neither by built-up area, nor by greenspace around ponds.
Our finding is an indication that aquatic beetles and heteropterans differ in their (terrestrial) habitat demands and/or flight and dispersal abilities. Most species in both taxonomic groups are known to have high flight and dispersal abilities as adults, an important trait to find and colonize new ponds (Bilton, 1994; Bilton et al., 2001; Bloechi et al., 2010). Biswas et al. (1995) and Bloechi et al. (2010) showed, that many aquatic beetles prefer small waterbodies with high amount of aquatic vegetation for egg laying and hiding, and that a (narrow) belt of vegetation around ponds was sufficient (Bloechi et al., 2010). Ponds in greenspaces have higher amounts of vegetation in their surroundings than ponds in areas with a high percentage of built-up area, and thus might provide more suitable habitats for aquatic beetles. Moreover, most aquatic beetles are known to prefer colonizing older over more recent ponds (Bloechi et al., 2010), further indicating the importance of aquatic and riparian vegetation. By contrast, many aquatic heteropterans are described to be ubiquitous (Hebauer, 1988; Bloechi et al., 2010), as pioneer species (Nilsson and Danell, 1981), preferring waterbodies with a high percentage of open water (Bloechi et al., 2010), a characteristic mainly shown by newly established ponds. Therefore, we assume that the colonizing process of ponds by heteropterans is less dependent on ponds’ terrestrial surroundings.
In contrast to the previous two insect groups, the abundance of dragonfly larvae in ponds is influenced by land-use of ponds surroundings (Goertzen and Suhling, 2013). This is not surprising as adult dragonflies, in contrast to aquatic beetles and heteropterans, spend more time in the terrestrial environment for, e.g., maturation of gonads, feeding and mating (Córdoba-Aguilar, 2008). However, based on the extremely low number of dragonfly larvae detected in our study, we found no effects of built-up area and greenspace around ponds. This low number per se however, is a clear indication that urban ponds are low quality habitats for dragonflies, as described in a study by Goertzen and Suhling (2013). We exclude the possibility that our trapping method was responsible for the low captures, as in the study by Hampton and Duggan (2003), funnel traps were successfully applied for capturing dragonfly larvae, and in other own studies (outside towns), we could catch huge numbers of dragonfly larvae in particular from species of the families Aeshnidae and Libellulidae (M.-O.-Rödel, unpubl. data).
In our study, we focused on macroinvertebrates that are known to predate on amphibian larvae (Henrikson, 1990; Wells, 2007; Hanlon and Relyea, 2013; Müller and Brucker, 2015), including on the toxic tadpoles of the common toad (Henrikson, 1990). We predicted a high availability on aquatic insect prey, such as high tadpole abundance, to have a positive effect on aquatic insect diversity measures and in all predatory taxa. We found that high tadpole abundance was not associated with high aquatic insect abundance, independent of ponds’ respective terrestrial surrounding. However, our results revealed trends towards positive effects of tadpole abundance on aquatic insect genera richness and alpha-diversity, in ponds surrounded by ‘built-up area’ and ‘greenspace’. Thus, there might be a relationship between high tadpole abundance and the numbers of predatory aquatic insect genera and their diversity. This relationship might be an indication that high tadpole abundance apparently contributes to a good/advantageous habitat for some predatory aquatic insects (Hanlon and Relyea, 2013; Müller and Brucker, 2015). By contrast, we found that aquatic beetles and heteropterans abundance and genera richness were not affected by high tadpole abundance in ponds, in neither land-use type, showing that our positive trends found in total aquatic genera richness and alpha-diversity were not reflected in our taxonomic groups. These might hint on, that tadpoles are not the predominant prey for many aquatic beetles and heteropterans and/or their different life stages. Although aquatic beetles and heteropterans are known to prey on tadpoles (Henrikson, 1990; Klecka and Boukal, 2012; Hanlon and Relyea, 2013; Müller and Brucker, 2015), the study by Klecka and Boukal (2012) described aquatic beetles to prefer cladocerans and dipteran larvae. Aquatic heteropterans, with sucking mouthparts and often using toxins, are known to additionally prey on other adult insects, insect larvae, amphibian and fish eggs, and some are also known to be omnivorous to feeding additionally on algae and detritus (Miaud, 1993; Yee, 2010; Culler et al., 2014; Hädicke et al., 2017). A further factor reducing predation rates of aquatic insects on tadpoles is aquatic vegetation (Klopp et al., 2006). Our study ponds were, at least partly, covered by riparian vegetation such as reed, hard stem bulrush, and sedge as well as emergent plants that might serve as refuges for tadpoles and prevent predation.
We predicted that the presence of large (vertebrate) predators, such as newts and fish, to negatively influence aquatic insect abundance, genera richness, alpha-diversity, and evenness, because species of both groups prey on aquatic insects (Peckarsky, 1982; Joly and Giacoma, 1992; Vidotto-Magnoni and Carvalho, 2009; Glenn and Cormier, 2014). Whereas newts are natural predators in ponds (Griffiths, 1996), most fish usually do not occur in stagnant and often temporary waters. They are however, deliberately released by humans, and negatively affect aquatic insect abundance and diversity (Buria et al., 2007). Our results confirmed this general knowledge. We verified the presence of fish in six of our 18 ponds (although all ponds were believed to be fish-free; data from the Stiftung Naturschutz, Berlin). A negative effect of the presence of fish on aquatic insect abundance was observed in ponds surrounded by built-up area and greenspace. Aquatic insect genera richness was negatively affected by fish in ponds surrounded by greenspace, and a similar trend was observed in ponds surrounded by built-up area. Thus, the presence of fish reduced the abundance and genera richness of aquatic insects in ponds, but did not have an influence on aquatic insect alpha-diversity and evenness. The reason why the presence of fish only impacted aquatic insect abundance and genera richness negatively, might be due to selective predation of fish on aquatic insects. Aquatic beetles (adults and larvae) and dragonfly larvae have strong mandibles (Peckarsky, 1982). Many heteropterans are able to sting with their proboscis (Peckarsky, 1982). This might lead fish to prey selectively on aquatic insects that are less well fortified. This assumption was supported by our findings when comparing the impact on beetles and heteropterans, respectively: fish negatively affected aquatic beetle abundance and genera richness, in both land-use types, but not aquatic heteropterans. Thus, fish indeed seem to prefer aquatic beetles over aquatic heteropterans (Peckarsky, 1982). The lack of effects of newts on aquatic insect abundance, diversity measures and on both taxonomic groups might as well be explained by defensive capabilities of our studied insect groups, but probably even more by a simple size relationship of predators to prey. Gape limitations would allow newts (mostly Smooth Newts, Lissotriton vulgaris) to only devour comparatively small insects.
The main food resource of tadpoles is periphyton and planktonic organisms (Harrison, 1987). Hence, we used in our study the concentration of chlorophyll-a as a proxy for phytoplankton biomass to make assertions on tadpoles’ food availability and habitat suitability in ponds (Minshall, 1978; Wood and Richardson, 2009). Therefore, we assumed higher tadpole abundance in ponds with higher chlorophyll-a concentrations. Because our study aquatic insects feed on tadpoles, we assumed higher tadpole abundance, in turn, to have positive effects on aquatic insects. However, we found high chlorophyll-a concentration to affect aquatic insect abundance, and aquatic beetles’ abundance negatively. Thus, abiotic parameters connected to algal growth, might override the potentially positive effects of high amounts of plankton on aquatic insects.
More algae increase photosynthetic activity in ponds (Picot et al., 1993; Ouarghi et al., 2000; Arbib et al., 2017). Our results showed that increasing oxygen saturation leads to decreasing aquatic insect abundance, richness, alpha-diversity, and evenness in both land-use types. This was also true if aquatic beetles (abundance) and heteropterans (genera richness) were examined separately. Eight of our 18 ponds showed, at least in one sampling campaign, oxygen saturation exceeding 100%, one pond even exceeded 200%. Such oversaturation can be explained by the very fast generation of oxygen through algae (Picot et al., 1993; Ouarghi et al., 2000; Arbib et al., 2017). Oxygen oversaturation however, leads to unbalanced pond conditions, which might result in oxygen toxicity for some aquatic insects (Fox and Taylor, 1955).
We also tested the impact of pH. Our results showed that increasingly basic water affected aquatic insect abundance negatively, whereas genera richness, alpha-diversity, and evenness were affected positively, independent on ponds terrestrial surroundings. Concerning beetles and heteropterans, increasing pH affected aquatic beetle abundance, genera richness and aquatic heteropterans genera richness positively, while heteropteran abundance was not affected. Ponds pH usually range from 6 to 8.5 pH, and if not influenced by high levels of biological activity, seldom reach pH exceeding 8.5 (Tucker and D’Abramo, 2008). In ponds with high levels of biological activity, such as high biomass of algae and/or fish, pH can exceed 8.5, resulting in chemical imbalance, what may create toxic conditions (Picot et al., 1993; Tadesse et al., 2004; Tucker and D’Abramo, 2008). In our ponds, pH ranged from 6.38 to 9.12, thus one pond exceeds a pH of 9. Our results might indicate that predatory aquatic insects in Berlin ponds might be dominated by species that are tolerant to slightly increased ponds’ pH.
As ectotherms, aquatic insects might benefit from warmer water that influence, e.g., metabolism and growth positively (Verberk and Bilton, 2013). However, water temperature did not have any effects on overall aquatic insect abundance and diversity measures, and likewise did not influence heteropterans. However, we found increasing temperature to negatively affect aquatic beetles’ genera richness in ponds surrounded by high amounts of greenspace. Our findings indicate that most aquatic insects might be tolerant to increasing water temperatures, independent on ponds’ surrounded areas, at least within the temperature ranges observed herein. However, some beetle genera showed low tolerances to increasing temperatures (Verberk and Bilton, 2013). The temperatures in our ponds across both sampling years ranged from 8.20 to 27.60°C.
In conclusion, our results showed that biological interactions and abiotic water conditions override urban effects of ponds’ terrestrial surroundings on aquatic insect diversity measures. However, when considering aquatic insects at the (functional) level of taxonomic groups, they respond differently to different land-use types around ponds, depending on their degree of ecological specialization and preferences.
Data availability statement
The original contributions presented in the study are included in the article/Supplementary material, further inquiries can be directed to the corresponding author.
Author contributions
M-OR, SK, GO, and FG contributed to conception and design of the study. SK, FG, and GO organized the database. SK performed the statistical analysis and wrote the first draft of the manuscript. GO wrote sections of the manuscript. All authors contributed to the article and approved the submitted version.
Funding
This work was funded by the German Federal Ministry of Education and Research BMBF within the Collaborative Project Bridging in Biodiversity Science—BIBS (funding number: 16LC1501F1).
Acknowledgments
We thank Stephanie Niemeier (Museum für Naturkunde, Berlin) for organization and support during fieldwork in 2019; Anne Hiller (Technische Universität, Berlin) for land-use calculations of the study ponds; Carlos Acame and Dorith Henning for logistic and technical support (ZALF) and Susanne Bengsch and Steven Lischke (Stiftung Naturschutz, Berlin) for providing information about amphibian occurrences at Berlin ponds. The permission for sampling invertebrates in Berlin was issued by Senatsverwaltung für Umwelt, Mobilität, Verbraucher-und Klimaschutz, City of Berlin.
Conflict of interest
The authors declare that the research was conducted in the absence of any commercial or financial relationships that could be construed as a potential conflict of interest.
Publisher’s note
All claims expressed in this article are solely those of the authors and do not necessarily represent those of their affiliated organizations, or those of the publisher, the editors and the reviewers. Any product that may be evaluated in this article, or claim that may be made by its manufacturer, is not guaranteed or endorsed by the publisher.
Supplementary material
The Supplementary material for this article can be found online at: https://www.frontiersin.org/articles/10.3389/fevo.2023.1121400/full#supplementary-material
References
Amt für Statistik Berlin-Brandenburg. Berlins Einwohnerzahl Stagniert; (2020). Available at: https://www.statistik-berlin-brandenburg.de/pms/2021/21-02-10.pdf. (Accessed February 27, 2021).
Antrop, M. (2000). Changing patterns in the urbanized countryside of Western Europe. Landsc. Ecol. 15, 257–270. doi: 10.1023/A:1008151109252
Arbib, Z., de Godos Crespo, I., and Corona, E. L. (2017). Understanding the biological activity of high rate algae ponds through the calculation of oxygen balances. Appl. Microbiol. Biotechnol. 101, 5189–5198. doi: 10.1007/s00253-017-8235-3
Bates, D., Mächler, M., Bolker, B., and Walker, S. (2015). Fitting linear mixed-effects models using lme4. J. Stat. Softw. 67, 1–48. doi: 10.18637/jss.v067.i01
Biggs, J. P., Williams, M., Whitfield, N. P., and Weatherby, A. (2005). 15 years of pond assessment in Britain: results and lessons learned from the work of pond conservation. Aquat. Conserv.: mar. Freshw. Ecosyst. 15, 693–714.
Bilton, D. (1994). The flight apparatus and flying ability of Hydroporus glabriusculus (Coleoptera, Dytiscidae), with a brief review of structural modifications in flightless beetles. Entomol. Tidskr. 115, 23–32.
Bilton, D. T., Freeland, J. R., and Okamura, B. (2001). Dispersal in freshwater invertebrates. Annu. Rev. Ecol. Syst. 32, 159–181. doi: 10.1146/annurev.ecolsys.32.081501.114016
Biswas, S., Mukhoupadhyay, P., and Saha, S. K. (1995). “Insecta: Coeloptera: Adephaga, family Dystiscidae”, in state fauna series 5: fauna of West Bengal, Z. S. I. CAL 5, 77–120.
Blair, R. B., and Launer, A. E. (1997). Butterfly diversity and human land use: species assemblages along an urban gradient. Biol. Conserv. 80, 113–125. doi: 10.1016/S0006-3207(96)00056-0
Blakely, T. J., Harding, J. S., Mcintosh, A. R., and Winterbourn, M. J. (2006). Barriers to the recovery of aquatic insect communities in urban streams. Freshw. Biol. 51, 1634–1645. doi: 10.1111/j.1365-2427.2006.01601.x
Bloechi, A., Koenemann, S., Philippi, B., and Melber, A. (2010). Abundance, diversity and succession of aquatic Coleoptera and Heteroptera in a cluster of artificial ponds in the north German lowlands. Limnologica 40, 215–225. doi: 10.1016/j.limno.2009.08.001
Brand, A. B., and Snodgrass, W. J. (2009). Value of artificial habitats for amphibian reproduction in altered landscapes. Biol. Conserv. 24, 295–301. doi: 10.1111/j.1523-1739.2009.01301.x
Briers, R. A. (2014). Invertebrate communities and environmental conditions in a series of urban drainage ponds in eastern Scotland: implications for biodiversity and conservation value of SUDS. Clean–Soil Air Water 42, 193–200. doi: 10.1002/clen.201300162
Briggs, J. A., Pryke, S. J., Samways, J. M., and Conlong, E. D. (2019). Complementary among dragonflies across a pondscape in a rural landscape mosaic. Insect Conserv. Divers. 12, 241–250. doi: 10.1111/icad.12339
Buchwald, R. (1992). Vegetation and dragonfly fauna characteristics and examples of biocenological field studies. Vegetatio 10, 99–107. doi: 10.1007/BF00033195
Buria, L., Albariño, R., and Villanueva, V. D. (2007). Impact of exotic rainbow trout on the benthic macroinvertebrate community from Andean-Patagonian headwater streams. Fundam. Appl. Limnol. 168, 145–154. doi: 10.1127/1863-9135/2007/0168-0145
Catenazzi, A. (2015). State of the world’s amphibians. Annu. Rev. Environ. Resour. 40, 91–119. doi: 10.1146/annurev-environ-102014-021358
Colding, J., Lundberg, J., Lundberg, S., and Andersson, E. (2009). Golf courses and wetland fauna. Ecol. Appl. 19, 1481–1491. doi: 10.1890/07-2092.1
Córdoba-Aguilar, A. (2008). Dragonflies and Damselflies. Model Organisms for Ecological and Evolutionary Research. Oxford: Oxford University Press.
Culler, L. E., Ohba, S., and Crumrine, P. (2014). “Predator-prey interactions of Dytiscids” in Ecology, Systematics, and the Natural History of Predaceous Diving Beetles (Coleoptera: Dytiscidae). ed. D. A. Yee (Dordrecht: Springer), 363–386.
De Meester, L., Declerck, S., Stoks, R., Louette, G., Van De Meutter, F., De Bie, T., et al. (2005). Ponds and pools as model systems in conservation biology, ecology and evolutionary biology. Aquat. Conserv: Mar. Freshw. Ecosyst. 15, 715–725. doi: 10.1002/aqc.748
Deacon, C., Samways, J. M., and Pryke, S. J. (2019). Aquatic insects decline in abundance and occupy low-quality artificial habitats to survive hydrological droughts. Freshw. Biol. 64, 1643–1654. doi: 10.1111/fwb.13360
DIN 38 412–16 (1985). German Standard Methods for the Examination of Water, Waste Water and Sludge; Test Methods Using Water Organisms (Group L); Determination of Chlorophyll-a in Surface Water (L 16). Deutsches Institut für Normung, Beuth Verlag GmbH, Berlin, p. 8.
Dolný, A., Harabiš, F., and Mižičová, H. (2014). Home range, movement, and distribution patterns of the threatened dragonfly Sympetrum depressiusculum (Odonata: Libellulidae): a thousand times greater territory to protect? PLoS One 9:e100408. doi: 10.1371/journal.pone.0100408
Dudgeon, D., Arthington, H. A., Gessner, O. M., Kawabata, Z.-I., Knowler, J. D., Lévêque, C., et al. (2006). Freshwater biodiversity: importance, threats, status and conservation challenges. Biol. Rev. 81, 163–182. doi: 10.1017/S1464793105006950
Fontana, S., Sattler, T., Bontadina, F., and Moretti, M. (2011). How to manage the urban green to improve bird diversity and community structure. Landsc. Urban Plan. 101, 278–285. doi: 10.1016/j.landurbplan.2011.02.033
Fox, H. M., and Taylor, R. E. A. (1955). The tolerance of oxygen by aquatic invertebrates. Proc. Royal Soc. B. 143, 214–225. doi: 10.1098/rspb.1955.0006
Gee, J. H. R., Smith, B. D., Lee, K. M., and Griffiths, S. W. (1997). The ecological basis of freshwater pond management for biodiversity. Aquat. Conserv.: mar. Freshw. Ecos. 7, 91–104. doi: 10.1002/(SICI)1099-0755(199706)7:2<91::AID-AQC221>3.0.CO;2-O
Gledhill, D. G., James, P., and Davies, H. D. (2008). Pond density as a determinant of aquatic species richness in an urban landscape. Landsc. Ecol. 23, 1219–1230. doi: 10.1007/s10980-008-9292-x
Glenn, W. S., and Cormier, S. M. (2014). Why care about aquatic insects: uses, benefits, and services. Integr. Environ. Assess. Manag. 11, 188–194. doi: 10.1002/ieam.1600
Goddard, M. A., Dougill, J. A., and Benton, G. T. (2010). Scaling up from gardens: biodiversity conservation in urban environments. Trends Ecol. Evol. 25, 90–98. doi: 10.1016/j.tree.2009.07.016
Goertzen, D., and Suhling, F. (2013). Promoting dragonfly diversity in cities: major determinants and implications for urban pond design. J. Insect Conserv. 17, 399–409. doi: 10.1007/s10841-012-9522-z
Gopal, B. (2013). Future of wetlands in tropical and subtropical Asia, especially in the face of climate change. Aquat. Sci. 75, 39–61. doi: 10.1007/s00027-011-0247-y
Haaland, C., and van den Bosch, C. K. (2015). Challenges and strategies for urban green-space planning in cities undergoing densification: a review. Urban For. Urban Green. 14:760771. doi: 10.1016/j.ufug.2015.07.009
Hädicke, C. W., Rédei, D., and Kment, P. (2017). The diversity of feeding habits recorded for water boatman (Heteroptera: Corixidae) world-wide with implications for evaluating information on the diet of aquatic insects. Eur. J. Entomol. 114, 147–159. doi: 10.14411/eje.2017.020
Hampton, E. S., and Duggan, C. (2003). Diel habitat shifts of macrofauna in a fishless pond. Mar. Freshw. Res. 54, 797–805. doi: 10.1071/MF02165
Hanlon, M. S., and Relyea, R. (2013). Sublethal effects of pesticides on predator–prey interactions in amphibians. ASIH. 4, 691–698. doi: 10.1643/CE-13-019
Harrison, D. J. (1987). Food and feeding relations of common frog and common toad tadpoles (Rana temporaria and Bufo bufo) at a pond in mid-Wales. Herpetol. J. 1, 141–143.
Hassall, C. (2014). The ecology and biodiversity of urban ponds. Wiley Interdiscip. Rev. Water 1, 187–206. doi: 10.1002/wat2.1014
Hassall, C., and Anderson, S. (2015). Stormwater ponds can contain comparable biodiversity to unmanaged wetlands in urban areas. Hydrobiologia 745, 137–149. doi: 10.1007/s10750-014-2100-5
Hebauer, F. (1988). Gesichtspunkte der ökologischen Zuordnung aquatischer Insekten zu den Sukzessionsstufen der Gewässer. Berichte der ANL 12, 229–239.
Heino, J., Bini, M. L., Andersson, J., Bergsten, J., Bjelke, U., and Johansson, F. (2017). Unravelling the correlates of species richness and ecological uniqueness in a metacommunity of urban pond insects. Ecol. Indic. 73, 422–431. doi: 10.1016/j.ecolind.2016.10.006
Henrikson, B.-I. (1990). Predation on amphibian eggs and tadpoles by common predators in acidified lakes. Ecography 13, 201–206. doi: 10.1111/j.1600-0587.1990.tb00609.x
Hill, O. M. (1973). Diversity and evenness: a unifying notation and its consequences. Ecology 54, 427–432. doi: 10.2307/1934352
Hill, J. M., Mathers, L. M., and Wood, J. P. (2015). The aquatic macroinvertebrate biodiversity of urban ponds in a medium-sized European town (Loughborough, UK). Hydrobiologia 760, 225–238. doi: 10.1007/s10750-015-2328-8
Hobbs, R. J., Higgs, E. S., and Hall, C. M. (2013). Novel Ecosystems. Intervening in a New Ecological World Order. Wiley-Blackwell, New Jersey.
Holtmann, L., Philipp, K., Becke, C., and Fartmann, T. (2017). Effects of habitat and landscape quality on amphibian assemblages of urban stormwater ponds. Urban Ecosyst. 20, 1249–1259. doi: 10.1007/s11252-017-0677-y
Huryn, A. D., Wallace, B. J., and Anderson, H. N. (2008). “Habitat, life history, secondary production, and behavioural adaptations of aquatic insects” in An Introduction to the Aquatic Insects of North America. eds. R. W. Merritt, K. W. Cummins, and M. B. Berg. 4th ed (Dubuque, Iowa: Kendall/Hunt Publishing Company), 55–103.
Johnson, P. T. J., Hoverman, T. J., McKenzie, J. V., Blaustein, R. A., and Richgels, D. L. K. (2013). Urbanization and wetland communities: applying metacommunity theory to understand the local and landscape effects. J. Appl. Ecol. 50, 34–42. doi: 10.1111/1365-2664.12022
Joly, P., and Giacoma, C. (1992). Limitation of similarity and feeding habits in three syntopic species of newts (Triturus, Amphibia). Ecography 15, 401–411. doi: 10.1111/j.1600-0587.1992.tb00050.x
Jooste, L. M., Samways, J. M., and Deacon, C. (2020). Fluctuating pond water levels and aquatic insect persistence in a drought-prone Mediterranean-type climate. Hydrobiologia 847, 1315–1326. doi: 10.1007/s10750-020-04186-1
Klecka, J., and Boukal, S. D. (2012). Who eats whom in a pool? A comparative study of prey selectivity by predatory aquatic insects. PLoS One 7:e37741. doi: 10.1371/journal.pone.0037741
Klopp, K., Wachlevski, M., and Eterovick, P. C. (2006). Environment complexity reduces tadpole predation by water bugs. Can. J. Zool. 84, 136–140. doi: 10.1139/Z05-186
Kühn, I., Brandl, R., and Klotz, S. (2004). The flora of German cities is naturally species rich. Evol. Ecol. Res. 6, 749–764.
Kühnel, K.-D., Charon, J., Kitzmann, B., and Schonert, B. (2017). “Rote Liste und Gesamtartenliste der Lurche (Amphibia) von Berlin,” in DER LANDESBEAUFTRAGTE FÜR NATURSCHUTZ UND LANDSCHAFTSPFLEGE /SENATSVERWALTUNG FÜR UMWELT, VERKEHR UND KLIMASCHUTZ (Hrsg.): Rote Listen der gefährdeten Pflanzen, Pilze und Tiere von Berlin, 23 S.
Liu, Z., He, C., and Wu, J. (2016). The relationship between habitat loss and fragmentation during urbanization: an empirical evaluation from 16 world cities. PLoS One 11:e0154613. doi: 10.1371/journal.pone.0154613
McIntyre, N. E. (2000). Ecology of urban arthropods: a review and a call to action. Ann. Entomol. Soc. Am. 93, 825–835. doi: 10.1603/0013-8746(2000)093[0825:EOUAAR]2.0.CO;2
McKinney, M. L. (2002). Urbanization, biodiversity, and conservation: the impacts of urbanization on native species are poorly studied, but educating a highly urbanized human population about these impacts can greatly improve species conservation in all ecosystems. Bio Science 52, 883–890. doi: 10.1641/0006-3568(2002)052[0883:UBAC]2.0.CO;2
McKinney, M. L. (2008). Effects of urbanisation on species richness: a review of plants and animals. Urban Ecosyst. 11, 161–176. doi: 10.1007/s11252-007-0045-4
Miaud, C. (1993). Predation on newts eggs (Triturus alpestris and T. helveticus): identification of predators and protective role of oviposition behaviour. J. Zool. Lond. 231, 575–582. doi: 10.1111/j.1469-7998.1993.tb01939.x
Minshall, G. W. (1978). Autotrophy in stream ecosystems. Bioscience 28, 767–771. doi: 10.2307/1307250
Müller, H., and Brucker, C. A. (2015). Predation on a Discoglossus pictus (anura: discoglossidae) tadpole by the larva of a water beetle (Dytiscidae: Dytiscinae: Dytiscus sp.) in Tunisia. Herpetol. Notes 8, 453–454.
Muzón, J., Ramos, L. S., and del Palacio, A. (2019). “Urban aquatic insects” in Aquatic Insects. eds. K. Del-Claro and R. Guillermo (Cham: Springer), 349–364.
Niemeier, S., Müller, J., Struck, U., and Rödel, M.-O. (2020). Superfrogs in the city: 150 year impact of urbanization and agriculture on the European Comon frog. Glob. Change Biol. 26, 6729–6741. doi: 10.1111/gcb.15337
Nilsson, A. N., and Danell, K. (1981). The annual and seasonal succession of larvae and imagines of water beetles in a shallow, man-made lake in northern Sweden. Aquat. Insect. 3, 233–243. doi: 10.1080/01650428109361068
Noble, A., and Hassall, C. (2014). Poor ecological quality of urban ponds in northern England: causes and consequences. Urban Ecosyst. 18, 649–662. doi: 10.1007/s11252-014-0422-8
Ouarghi, H., El Boumansour, E. B., Dufayt, O., El Hamouri, B., and Vasel, L. J. (2000). Hydrodynamics and oxygen balance in a high-rate algal pond. Water Sci. Technol. 42, 349–356. doi: 10.2166/wst.2000.0678
Pallarés, S., Botella-Cruz, M., Arribas, P., Millán, A., and Velasco, J. (2017). Aquatic insects in a multistress environment: cross-tolerance to salinity and desiccation. J. Exp. Biol. 220, 1277–1286. doi: 10.1242/jeb.152108
Paul, M. J., and Mayer, L. J. (2001). Streams in the urban landscape. Annu. Rev. Ecol. Evol. Syst. 32, 333–365. doi: 10.1007/978-0-387-7312-5_12
Peckarsky, B. L. (1982). Aquatic insect predator-prey relations. Oxford Unive Press Am Inst Biol Sci 32, 261–266. doi: 10.2307/1308532
Pickett, S. T. A., Cadenasso, M. L., Grove, J. M., Boone, C. G., Groffman, P. M., Irwin, E., et al. (2011). Urban ecological systems: scientific foundations and a decade of progress. J. Environ. Manag. 92, 331–362. doi: 10.1016/j.jenvman.2010.08.022
Picot, B., Moersidik, S., Casellas, C., and Bontoux, J. (1993). Using diurnal variations in a high rate algae pond for management pattern. Water Sci. Technol. 28, 169–175. doi: 10.2166/wst.1993.0227
QGIS Development Team (2021). QGIS Geographic Information System. Open Source Geospatial Foundation Project. Available at: http://qgis.osgeo.org (Accessed August 1, 2021).
R Core Team (2022). R: A Language and Environment for Statistical Computing. R Foundation for Statistical Computing, Vienna, Austria.
Ramsey, J. B. (1969). Tests for specification errors in classical linear least-squares regression analysis. J. R. Stat. Soc. Series B. Stat. Methodol. 31, 350–371. doi: 10.1111/j.2517-6161.1969.tb00796.x
Rödel, M.-O., Demtröder, S., Fuchs, C., Petrich, D., Pfisterer, F., Richter, A., et al. (2014). Modifizierte Kleinfischreusen zur verbesserten Fängigkeit adulter Molche. Z. Feldherpetol. 21, 75–82.
Schewenius, M., McPhearson, T., and Elmqvist, T. (2014). Opportunities for increasing resilience and sustainability of urban social-ecological systems: insights from the URBES and the cities and biodiversity outlook projects. Ambio 43, 434–444. doi: 10.1007/s13280-014-0505-z
Senatsverwaltung für Stadtentwicklung und Umwelt: Berlin, Germany (2005). Gelände-und Luftbild-Kartierungen der Biotoptypen; Umweltatlas Stadtstruktur, Grünflächen-, Friedhofs-und Kleingartenkataster, Gewässerkarte. Available at: https://fbinter.stadt-berlin.de/fb/index.jsp (Accessed August 02, 2021).
Shannon, C. E., and Weaver, W. (1949). The Mathematical Theory of Communication. Urbana: University of Illinois Press.
Shochat, E., Lerman, B. S., Anderies, M. J., Warren, S. P., Faeth, H. S., and Nilon, H. C. (2010). Invasion, competition, and biodiversity loss in urban ecosystems. Bio Science 60, 199–208. doi: 10.1525/bio.2010.60.3.6
Simaika, J. P., and Samways, M. J. (2011). Comparative assessment of indices of freshwater habitat conditions using different invertebrate taxon sets. Ecol. Indic. 11, 370–378. doi: 10.1016/j.ecolind.2010.06.005
Smith, R. F., Alexander, L. C., and Lamp, W. O. (2009). Dispersal by terrestrial stages of stream insects in urban watersheds: a synthesis of current knowledge. J. North Am. Benthol. Soc. 28, 1022–1037. doi: 10.1899/08-176.1
Smith, R. F., and Lamp, W. O. (2008). Comparison of insect communities between adjacent headwater and main-stem streams in urban and rural watersheds. J. North Am. Benthol. Soc. 27, 161–175. doi: 10.1899/07-071.1
Spellerberg, I. F., and Fedor, P. J. (2003). A tribute to Claude Shannon (1916/2001) and a plea for more rigorous use of species richness, species diversity and the ‘Shannon/wiener’ index. Glob. Ecol. Biogeogr. 12, 177–179. doi: 10.1046/j.1466-822X.2003.00015.x
Tadesse, I., Green, B. F., and Puhakka, A. J. (2004). Seasonal and diurnal variations of temperature, pH and dissolved oxygen in advanced integrated wastewater pond system® treating tannery effluent. Water Res. 38, 645–654. doi: 10.1016/j.watres.2003.10.006
Tucker, C. S., and D’Abramo, L. R. (2008). Managing High pH in Freshwater Ponds. Stoneville: Southern Regional Aquaculture Centre, 1–5.
Urban, M. C., Skelly, K. D., Burchsted, D., Price, W., and Lowry, S. (2006). Stream communities across a rural-urban landscape gradient. Divers. Distrib. 12, 337–350. doi: 10.1111/j.1366-9516.2005.00226.x
Verberk, P. E. C. W., and Bilton, T. D. (2013). Respiratory control in aquatic insects dictates their vulnerability to global warming. Biol. Lett. 9:20130473. doi: 10.1098/rsbl.2013.0473
Vidotto-Magnoni, P. A., and Carvalho, D. C. (2009). Aquatic insects as the main food resource of fish the community in a Neotropical reservoir. Neotrop. Ichthyol. 7, 701–708. doi: 10.1590/S1679-62252009000400020
Wickham, H. (2016). Ggplot2: Elegant Graphics for Data Analysis [R Package Version 4.1.3]. New York: Springer-Verlag.
Williams, P., Whitfield, M., Biggs, J., Bray, S., Fox, G., Nicolet, P., et al. (2003). Comparative biodiversity of rivers, streams, ditches and ponds in an agricultural landscape in southern England. Biol. Conserv. 115, 329–341. doi: 10.1016/S0006-3207(03)00153-8
Winter, G. J., Somers, M. K., Dillon, J. P., Paterson, C., and Reid, A. R. (2002). Impacts on golf ourses on macroinvertebrate community structure in precambrian shield streams. J. Environ. Qual. 31, 2015–2025. doi: 10.2134/jeq2002.2015
Wood, R. L. S., and Richardson, S. J. (2009). Impact of sediment and nutrient inputs on growth and survival of tadpoles of the Western toad. Freshw. Biol. 54, 1120–1134. doi: 10.1111/j.1365-2427.2008.02139.x
Wu, J. (2014). Urban ecology and sustainability: the state-of-the-science and future directions. Landsc. Urban Plan. 125, 209–221. doi: 10.1016/j.landurbplan.2014.01.018
Yee, D. A. (2010). Behavior and aquatic plants as factors affecting predation by three species of larval predaceous diving beetles (Coleoptera: Dytiscidae). Hydrobiologia 637, 33–43. doi: 10.1007/s10750-009-9982-7
Zapparoli, M. (1997). Urban development and insect biodiversity of the Rome area. Italy. Landsc. Urban Plan. 38, 77–86. doi: 10.1016/S0169-2046(97)00020-0
Keywords: amphibians, built-up area, chlorophyll-a, greenspace, macroinvertebrates, plankton, temperature, water chemistry
Citation: Keinath S, Onandia G, Griesbaum F and Rödel M-O (2023) Effects of urbanization, biotic and abiotic factors on aquatic insect diversity in urban ponds. Front. Ecol. Evol. 11:1121400. doi: 10.3389/fevo.2023.1121400
Edited by:
Olivia Sanllorente, University of Granada, SpainReviewed by:
Boudjéma Samraoui, University of Annaba, AlgeriaTiffany A. Schriever, Western Michigan University, United States
Copyright © 2023 Keinath, Onandia, Griesbaum and Rödel. This is an open-access article distributed under the terms of the Creative Commons Attribution License (CC BY). The use, distribution or reproduction in other forums is permitted, provided the original author(s) and the copyright owner(s) are credited and that the original publication in this journal is cited, in accordance with accepted academic practice. No use, distribution or reproduction is permitted which does not comply with these terms.
*Correspondence: Keinath Silvia, c2lsdmlhLmtlaW5hdGhAbWZuLmJlcmxpbg==