- 1Negaunee Integrative Research Center, Field Museum, Chicago, IL, United States
- 2Department of History, University of Chicago, Chicago, IL, United States
The living fossil concept continues to foster development of integrative research, as it has done since Darwin’s use of the term in 1859. It has been applied to a vast menagerie of biological entities across the kingdoms of life and at many levels in the biological hierarchy. Correspondingly, a number of primary living fossil criteria emerged and persisted all the while as disciplines, theories and practices of biology transformed. In this paper we attempt the first comprehensive analysis of the use of the term ‘living fossil’ from 1860 to the present. Employing a compilation of 719 publications and 853 unique entities, we examine: (1) patterns of change in the phylogenetic diversity of living fossils, their taxonomic ranks and groups, (2) the diverse subject areas in which living fossils play a role, and (3) the primary criteria used to designate living fossil status. Our analyses reveal how the menagerie has extended and diversified alongside technological developments in biological research. The average number of living fossil criteria applied per entity has escalated even more rapidly than the menagerie has expanded. We argue that the demonstrated persistence, ever-expanding scientific and public interest, and future utilization of living fossils reflects increasingly complex challenges to deep-seated expectations at the heart of a living fossil research agenda: omnipresent evolutionary change and inevitable disappearance or extinction.
Introduction
The centrifugal pull of a capacious concept could threaten its survival. Several concepts in the history of science that have been stretched to include subsequent definitions and societal uses eventually fracture in practice or in subsequent historical analyses (Latour, 2000)—a phenomenon that can be used to trace discipline formation over time. Investigations of the concept ‘organism,’ for example, demonstrate that it morphed and refined in meaning, paralleling the emergence of technical biological disciplines beginning in the nineteenth century (Cheung, 2006; Wolfe, 2014). Conversely, in exceptional cases, ever-expanding senses of a concept emerge and persist, retaining a core that proliferates across decades, disciplines, and discrete entities (Daston, 2000; Love, 2015). ‘Living fossil’ is one such concept. Persistent use of an important concept over a decade, much less a generation or a century, does not indicate that concept’s meaning is truly invariable (Brigandt, 2010; Abrams, 2012; Wolfe, 2014). Different meanings of concepts such as ‘gene,’ ‘homology’ and ‘evolutionary novelty’ coexist synchronically in different disciplines or research applications. The very ambiguity and open-ended nature of such concepts proves useful when applied in diverse contexts, promoting integration, and making productive contributions to shared epistemic goals (Kampourakis and Stern, 2018; Brigandt, 2020; Neto, 2020; Haueis, 2021; Watkins, 2021). The many notions of the term ‘living fossil’ across different entities and disciplines can be seen in the varied criteria that confer an entity’s living fossil status. The concept’s role in exploring integrated multidisciplinary questions becomes apparent and reveals expectations carried by biologists.
The term ‘living fossil’ canonically appears in Charles Darwin’s On the Origin of Species (Darwin, 1859) in reference to Ornithorhynchus (platypus), Lepidosiren (lungfish), and ganoid fishes (e.g., paddlefishes, gars, and sturgeons). Its proliferation through time since 1860 has been astonishing, applied to ever more organisms, taxonomic groups, and non-taxonomic entities, with more and varied justifications for their living fossil status. In short, the concept has grown, becoming a site of pluralist inquiry into a multitude of living forms, parts, and processes (Lidgard and Love, 2018, 2021; Brigandt, 2020). Yet in the last few decades, questions and challenges have harassed the status of even archetypal living fossils (Avise et al., 1994; Nagalingum et al., 2011; Casane and Laurenti, 2013; Mathers et al., 2013; Cavin and Guinot, 2014; Naville et al., 2015; Vaux et al., 2019). Despite these challenges, the living fossil concept continues to be elevated as a hub of inquiries (Lidgard and Love, 2018; Turner, 2019; Zhao et al., 2019; Johnson, 2020; Vargas et al., 2020; Cavin and Alvarez, 2022).
In this paper we explore a new perspective of the living fossil concept—living fossils as a research agenda. Our approach follows from Lidgard and Love (2018), who wrote, “Instead of viewing the concept’s task as categorizing living fossils, we show how its primary role is to mark out what is in need of explanation, accounting for the persistence of both molecular and morphological traits. Rethinking different conceptions of living fossils as specific hypotheses reveals novel avenues for research that integrate phylogenetics, ecological and evolutionary modeling, and evo-devo to produce a more unified theoretical outlook.” In the sense that we use it here, a research agenda consists in a whole set of interrelated, often interdisciplinary, questions that together motivate ongoing explanatory efforts (Love, 2008; Brigandt, 2020; Neto, 2020). We are not aiming to evaluate the merits or weaknesses of particular criticisms of the use of living fossils, nor the strengths and weaknesses of any particular definiton of what a living fossil should be. Indeed, no single definition is sufficient to describe all the ways that the term ‘living fossil’ is applied, all the contexts in which different criteria play a role, and all the entities that are (or can be) relevant, all the conflicting interpretations that arise from prioritizing one suite of parts (e.g., morphology, genes or other biomolecules) versus another in evaluating a claim of living fossil status.
Here we attempt the first comprehensive analysis of the usage of the term ‘living fossil’ from Darwin’s introduction through to its continuing applications in modern biological investigations. We first place the diversity of named living fossil entities into phylogenetic frameworks. This allows us to examine important patterns of change over the past 160 years that shape current understanding—in the diversity of living fossil entities among different taxonomic ranks and of non-taxonomic entities such as biomolecules; in the diversity of entities among the taxonomic groups; among the principal criteria used by scientists to confer living fossil status; and in the use of the term in different biological subject areas of publications. Lastly, we investigate the structure of relationships among groups of living fossil entities and their scored criteria.
The trends that emerge are telling. As biology revised questions towards incorporating phylogenetic, biogeographic, ecological, physiological, molecular, and genetic tools and frameworks, the ways in which living fossils were interesting to scientists changed. What can be designated as a ‘living fossil’ has expanded as well, adding viroids, gene segments, proteins, ecological communities and more to the menagerie. The living fossil concept as a research agenda entails a collection of entities—they are united under the concept in a space of interdisciplinary problems having to do with evolutionary stasis, change and extinction.
Beginning with Ginkgo, several examples reveal how the contours of that space relate to varied living fossil attributes. Darwin had put forward that lower and simpler forms well-adapted to their habitats might sometimes survive unchanged given the long-term persistence of those habitats (Bowler, 2003). Interestingly, in his published work Darwin never termed Ginkgo (as synonym Salisburia) a ‘living fossil.’ Yet he was aware of its place among the most ancient seed plants and as the last survivor of a group that was once much more abundant and diverse (Crane et al., 2013). In his late correspondence he considered it analogous with Thomas Huxley’s “persistent types” (Darwin, C. to G. de Saporta, 1877). Ginkgo and its close fossil relatives played key roles in problems of gymnosperm taxonomy and later on, evolution and phylogeny of land plants. Not only Ginkgo’s ancient pedigree but also different parts of the taxonomic whole (leaves, reproductive structures and fertilization, plastid and nuclear genes) continue to inform links between non-flowering, non-vascular plants and higher seed plants. By the mid-nineteenth century, the taxonomically isolated genus represented “perhaps the oldest type of Gymnospermous structure extant” (Oliver, 1862, 87). There was then little consensus about competing classifications of groups now considered to be gymnosperms (Buggs, 2021). Though Hooker and Binder (1855) suggested Salisburia likely belonged to the conifers, its morphological characters also implied affinities with other groups, both living and fossil. The discovery of swimming sperm cells in Ginkgo (Ikeno and Hirase, 1897), linking it with cycads and separating them from all other gymnosperms, disrupted seed plant taxonomy. By the early twentieth century, Ginkgo was often seen as phylogenetically transitional between lower and higher gymnosperms (Jeffrey, 1917). With recent advances in phylogenetics and genomics, Ginkgo has helped bridge knowledge gaps between ferns and angiosperms (Liu et al., 2021). Despite some conflicting nuclear and plastome gene data and results among coalescent-based, species tree and supermatrix analyses, studies have begun to identify more robust evolutionary and functional patterns among plant genomes (Leebens-Mack et al., 2019; Yang et al., 2022). More living fossil attributes of Ginkgo are important to problems in biogeography (Berry, 1924; Lidgard and Love, 2018), paleoecology and habitat tracking (Zhao et al., 2016; Hohmann et al., 2018), adaptation to climate shifts (Huang et al., 2015; Zhao et al., 2019), and the global carbon cycle (Beerling and Royer, 2002; Steinthorsdottir et al., 2022). Another attribute, the association of persistent morphological stasis with defensive mechanisms against predators and pathogens (Wieland, 1924; Guan et al., 2016), has contributed to recent explosive growth in the study of Ginkgo’s protective secondary metabolites and their genetic foundations (Jacobs and Browner, 2000; Singh et al., 2019; Li et al., 2022). Additional living fossils and their attributes have been prominent for problems entailing rates of evolution and conflicting morphological and genetic signals, for instance in tadpole shrimps (Luchetti et al., 2021), fishes (Clarke et al., 2016, Giles et al., 2017), and Sphenodon, the tuatara (Subramanian et al., 2009; Simões et al., 2022). Lastly, we point to examples in which living fossils have contributed to the early understanding of embryology (Hopwood, 2005) and modern evo-devo (Braasch et al., 2015; Bayramov et al., 2018; Watkins, 2021). Taken together, the history of living fossil entities such as Ginkgo suggests a rich epistemic space of interdisciplinary problems in which change, stasis, and extinction can be centered through integrated study of entities that each offer what Watkins (2021) calls a “productive combination” of both living and fossil taxa for comparison.
The term ‘living fossil’ and the scientific concept living fossil ought not to be conflated (Brigandt, 2010; Neto, 2020). Still, there is illuminating overlap in their uses. The most persistent use of the term ‘living fossils’ is not as a concept that refers narrowly to the same empirical phenomenon. Rather, it has historically served as an umbrella construct (Hirsch and Levin, 1999) for a menagerie of entities that do not meet specific expectations within evolutionary theory: namely, change and extinction. These expectations apply to biomolecular entities as well as taxonomic ones. For instance, a remarkable amount of gene loss (roughly comparable to disappearance of fossil taxa) is inferred across the animal kingdom (Guijarro-Clarke et al., 2020). The term ‘living fossil’ is used to be evocative—hailing something as from another time—or to acknowledge that members of the living fossil menagerie can provide scientists with meaningful inferences about evolutionary rates, constraints, patterns of diversification, foci of natural selection, and survivorship. How the term has been applied over time, and to what, tells a story of changing methods and research programs in the biological sciences. The continued use of a concept does not itself demonstrate a continuation of thought, method, or specific philosophy within a science, but may instead reveal a stubborn problem or underlying assumption that keeps the term and its multifaceted deployments in use. Living fossils serve in this contrarian way to frustrate our expectation that little or nothing exists between the alternatives of omnipresent evolutionary change and inevitable disappearance or extinction.
Materials and methods
Biology has assembled a vast menagerie of living fossils since Darwin first published On the Origin of Species (Darwin, 1859). That menagerie now entails many sorts of entities at different levels of the biological hierarchy, as well as diverse qualities or criteria granting living fossil status. We use an extensive compilation of publications from 1860 through 2020 to explore how a living fossil concept is employed across biology as part of a research agenda (Lidgard and Love, 2018, 2021). We examine the phylogenetic distribution and rank of taxonomic entities now and over historical time. Non-taxonomic entities such as biomolecules are treated concurrently. We score entities and their publications for a variety of attributes to interpret diverse contexts and applications encompassed by the living fossil concept as a research agenda. The full data matrix comprising 719 publications, 1,402 total living fossil entities, 853 unique entity names (excluding duplicates in different publications) and attribute scores is available in Supplementary material.
Searches for relevant publications were conducted using online bibliographies including Google Scholar,1 HathiTrust Digital Library,2 Proquest British Periodicals,3 and Gallica Digital Library,4 in addition to citations found within individual publications. Included publications meet two strict conditions. First, we retain only publications in journals, bulletins, edited volumes (as chapters) or whole books that include the key phrases living fossil, living fossils, or their non-English cognates (e.g., fossiles vivants, lebende Fossilien). Second, retained publications include a definition, justification, or explanatory indication of the status of an entity (or entities) as a living fossil. While terms such as relic, persistent type, survivor, arrested evolution and “formes panchronique” are often associated with living fossils, we do not include publications using these terms unless one of our key phrases is also present. Our compilation represents a very conservative sample relative to a far larger number of entities and publications that either reference or suggest a living fossil concept but do not meet these conditions.
Each entity is a separate entry in the compilation, including its name as originally given, its current name, publication, author(s), date, and attribute scores. Taxonomic entities at different ranks are separate entries: species, genus, family, or higher taxon. Original names are updated to current names reflecting systematic revisions using online sources including Catalogue of Life,5 Global Biodiversity Information Facility,6 Interim Register of Marine and Non-Marine Genera,7 and World Register of Marine Species.8 Each taxonomic entity is assigned to a high-level clade or subgroup to better understand the phylogenetic propagation of designated living fossils across the kingdoms of life. Because many traditional phyla and their interrelationships are undergoing rapid revision due to new genomic data, we illustrate the diversity and phylogenetic disparity of taxonomic entities with phylograms slightly modified from current hypotheses: for Archaea and Bacteria (Coleman et al., 2021; Martinez-Gutierrez and Aylward, 2021); for eukaryotes not including green plants and animals (Keeling and Burki, 2019; Strassert et al., 2019; Burki et al., 2020); for green plants (Ran et al., 2018; Leebens-Mack et al., 2019; Stull et al., 2021; Liu et al., 2022; Zhang et al., 2022); and for animals (Laumer et al., 2019; Li et al., 2021; Redmond and McLysaght, 2021). All of these authors acknowledge areas of phylogenetic uncertainty or conflict, and our phylograms should be viewed accordingly.
In order to evaluate changing patterns of criteria for living fossil status, we score entities as described in each publication in one or more categories, generalized from Lidgard and Love (2018, 2021):
• long duration - prolonged geological duration relative to similar entities;
• general ancestral similarity - overall or unspecific similarity to a posited ancestral fossil, group or other entity;
• character ancestral similarity - implicitly or explicitly comparative phylogenetic inference of specific characters as primitive, basal, plesiomorphic, or inference of specific character similarity to a posited ancestral fossil, group or other entity;
• diversity relic - very low taxonomic richness today compared to the past;
• geographic relic - very reduced geographic range today compared to the past;
• fossil known first - known in the fossil record before being discovered alive;
• genealogical inference - implicitly or explicitly comparative phylogenetic inference of genealogical divergence that may include inference of a basal divergence relative to other characters, entities or groups, an ‘intermediate’ position relative to other characters, entities or groups that diverged in the distant past, characters shared among distantly related lineages or groups, or similar kinds of inferences;
• slow evolutionary rate - inferred or measured rate of evolutionary change lower than rates among similar entities;
• other criteria.
To explore changing patterns of disciplinary focus, we score each publication according to a single primary subject area: cell biology, physiology, morphology/structure, conservation biology, ecology, evolution, biogeography, genetics/molecular biology, developmental biology, natural history, or other subject area. Two more attributes for each entity score whether or not a publication explicitly contains a morphological phylogeny or a molecular phylogeny. Finally, for each publication, we score whether or not categorizing an entity (or entities) as a living fossil is a principal aim. We accept that categorical scoring such as this is hardly perfect; a degree of subjectivity is unavoidable.
Different perspectives of the growth of a living fossil menagerie are shown in plots of cumulative numbers of taxonomic and non-taxonomic entities, of taxonomic entities within kingdoms, of subject areas of publications, and of living fossil criteria from 1860–2020. External factors likely do exert a degree of influence on our data and results. Overall numbers of entities have certainly increased since 1860 with accompanying changes in taxonomic methods and technologies, and new taxa in particular continue to be described at varying rates (Edie et al., 2017). Overall numbers of publications in biological disciplines and the diversification of those disciplines began to increase dramatically in the late twentieth century. Similarly, coverage of publications in several of the online bibliographies is more complete toward the present day. Our temporal analyses of living fossil entities, scored criteria, and attributes are therefore intended to document general patterns rather than exact numbers.
We use nonmetric multidimensional scaling (NMS) to investigate the structure of relationships among groups of entities and their scored criteria in PC-ORD v.7 (McCune and Grace, 2002; Peck, 2016). NMS is well suited to exploratory analyses of data scored as binary variables—here, individual criteria and attributes such as group membership—that are discontinuous and nonnormal. NMS eliminates an assumption of linear relationships among variables. In our application, NMS iteratively optimizes a model that uses a matrix of Sørensen (Bray-Curtis) dissimilarity coefficients from the original entity-criteria data. It then calculates a new ordination exposing the relationship between rank-order distances measured in entity space and distances in criteria space on a reduced number of axes. Each axis reflects the redundant pattern of entity-criteria positions that are represented on that axis. We first plot vectors showing the direction and relative magnitude of the most significant criteria correlated with each axis. Further relationships are then interpreted visually by overplotting each entity group (Animalia, green plants, eukaryotes excluding animals and plants, prokaryotes, and biomolecules) on this ordination configuration. This permits comparison of each group with the positions of significant criteria in the ordination space. These relationships are inferential but not necessarily causal.
Results
The term ‘living fossils’ appears only twice in the first edition of Darwin’s Origin of Species (Darwin, 1859) and only three taxonomic entities are mentioned in direct association with that term. Ornithorhynchus and Lepidosiren are both genera. Ganoid fishes are an unnatural group, but were then widely recognized as a higher taxon, one of four principal Orders in Agassiz’s (1833–1844) great work on fossil fishes. Living fossil status is almost always granted contextually, by comparison with other entities of a similar sort. Before considering changing contexts, we need to detail how today living fossils are distributed widely among the kingdoms of life.
We found 48 living fossil taxa designated among Archaea and Bacteria (Figure 1). The two Archaea taxa in the TACK supergroup were described only recently from hydrothermal environments. Among Bacteria, a physiological assemblage of taxa in Terrabacteria and Gracilicutes is associated with posited “ancient,” largely anaerobic energy-converting metabolic pathways (Martin, 2020; Schoelmerich and Müller, 2020). Another significant Terrabacteria grouping includes particular cyanobacteria whose gross cellular morphology is remarkably similar between Precambrian microfossils and extant genera and species (Schopf, 1994).
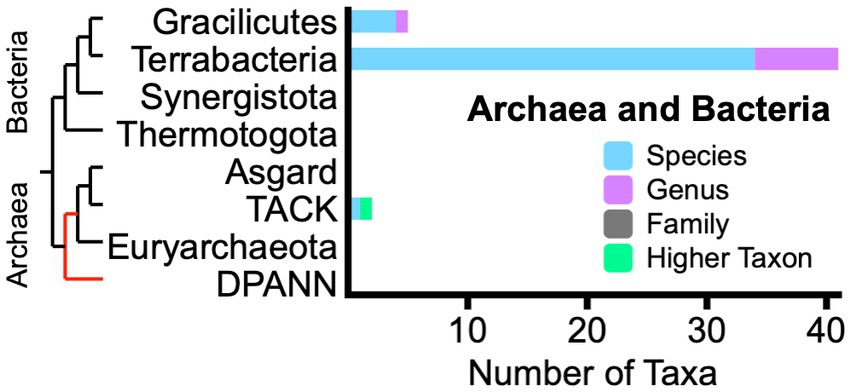
Figure 1. Phylogram with bar graph showing the distribution of living fossil taxa across Archaea and Bacteria. Bar colors indicate taxonomic ranks of 48 unique taxon names. Red section of phylogram indicates substantial uncertainty of relationship. Acronyms: DPANN supergroup (originally Diapherotrites, Parvarchaeota, Aenigmarchaeota, Nanoarchaeota, and Nanohaloarchaea); TACK supergroup (Thaumarchaeota, Aigarchaeota, Crenarchaeota, and Korarchaeota).
Among eukaryotes, excluding green plants in supergroup Archaeplastida and animals in supergroup Amorphea (Figure 2), most living fossil taxa are unicellular organisms. One large set of taxa in the TSAR supergroup stems from the discovery of reproductive and resting spores of living marine dinoflagellates with morphologies nearly identical to Mesozoic and Cenozoic fossils (Wall and Dale, 1966). Foraminiferans and radiolarians comprise smaller groups of TSAR taxa, also recognized by comparing morphological characters of living and fossil forms. Fungi are represented by only three taxa in supergroup Amorphea.
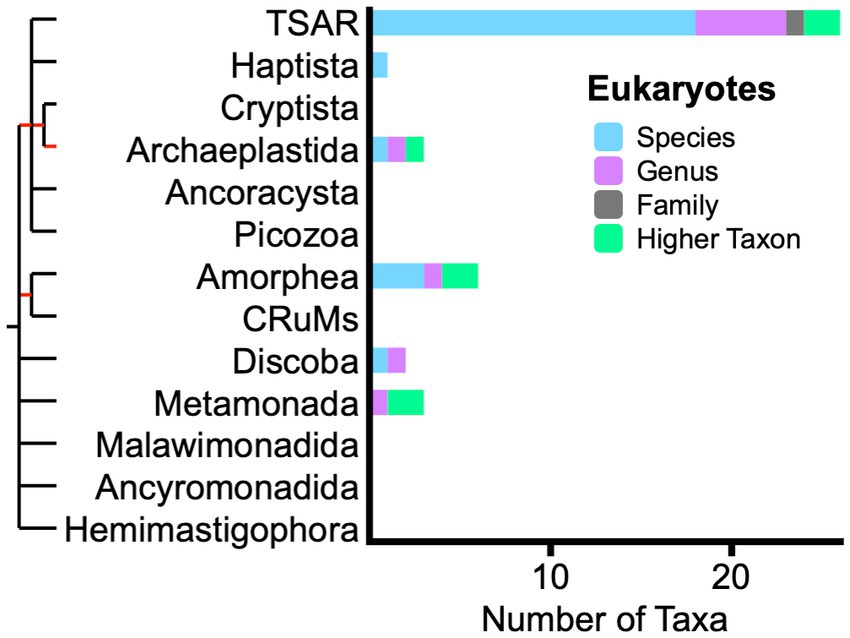
Figure 2. Phylogram with bar graph showing the distribution of living fossil taxa across Eukaryotes, excluding green plants and animals. Bar colors indicate taxonomic ranks of 41 unique taxon names. Red sections of phylogram indicate substantial uncertainty of relationship. Acronyms: TSAR supergroup (telonemids, stramenopiles, alveolates, and Rhizaria); CRuMs supergroup (collodictyonids, Rigifilida, and Mantamona).
Among green plants (Figure 3), 40% of living fossil plant taxa occur in eudicots. This suggests that living fossil attribution is contextual. Eudicots are not a phylogenetically basal plant clade. Rather, they are among the most derived and among the latest to appear in the fossil record. By one recent estimate (Christenhusz and Byng, 2016), eudicots today make up about 79% of 374,000 accepted plant species. Note that this is an indirect comparison (taxa at several ranks versus species alone). Aside from a set of Nothofagus species (van Steenis, 1953), eudicot taxa are scattered among disparate genera and species. Ferns, used here in the broad sense, have 26 taxa; Cupressophytes (non-Pinacea conifers) have 16 taxa; and phylogenetically basal Chlorophytes have 16 taxa, mostly dasycladacean algae. The 159 unique taxa in the figure exclude duplicates of several genus and species names appearing in two or more references, especially canonical living fossils like Ginkgo biloba, Metasequoia glyptostroboides, and others.
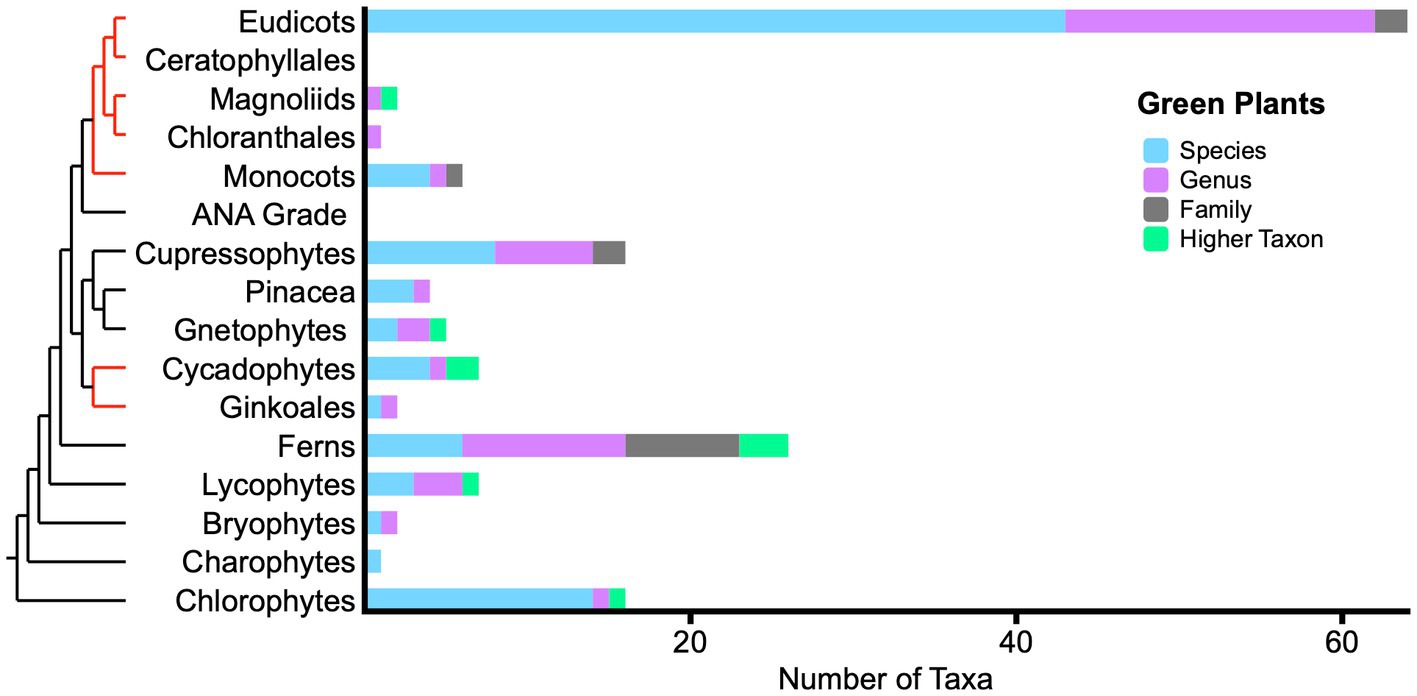
Figure 3. Phylogram with bar graph showing the distribution of living fossil taxa across green plants. Bar colors indicate taxonomic ranks of 159 unique taxon names. Red sections of phylogram indicate substantial uncertainty of relationship. Acronym: ANA grade (Amborellales, Nymphaeales, and Austrobaileyales).
Among animals (Figure 4), the large number of 245 living fossil chordate taxa is most striking, again because two prominent subgroups therein are phylogenetically derived and comparatively late-occurring in the fossil record. Mammalia, with 114 taxa, greatly outnumber all other subgroups, and 18 taxa occur in Aves. Moreover, the results are not strictly correlated with living diversity, the species richness of subgroups. Living fossil Actinopterygia (ray-finned fishes) have 41 taxa; Chondrichthyes have 16; and Crocodylia have 16. Compare these figures proportionally with recent estimates of living species richness among vertebrates alone (Wilkinson et al., 2021): 6,485 mammals, 10,945 birds, 33,913 “finfishes,” and 11,242 reptiles. Living fossil Arthropoda contain 148 taxa, yet terrestrial arthropods alone are estimated to contain seven million species (Stork, 2018). Among living fossil subgroups, nearly all 66 Hexapoda are terrestrial insects. Crustacea include 59 taxa, scattered primarily among marine genera and species. Chelicerata contain 19 taxa, including horseshoe crabs, scorpions, mites, and spiders. Citations of canonical living fossils such as coelacanths, lungfish, platypus, tadpole shrimps and others are duplicated repeatedly in our compilation, but are reduced here in the set of unique taxon names. Similarly, Darwin’s ganoid fishes are prominent with frequent duplication among living fossil taxa as bichirs, bowfin, paddlefishes, gars and sturgeons.
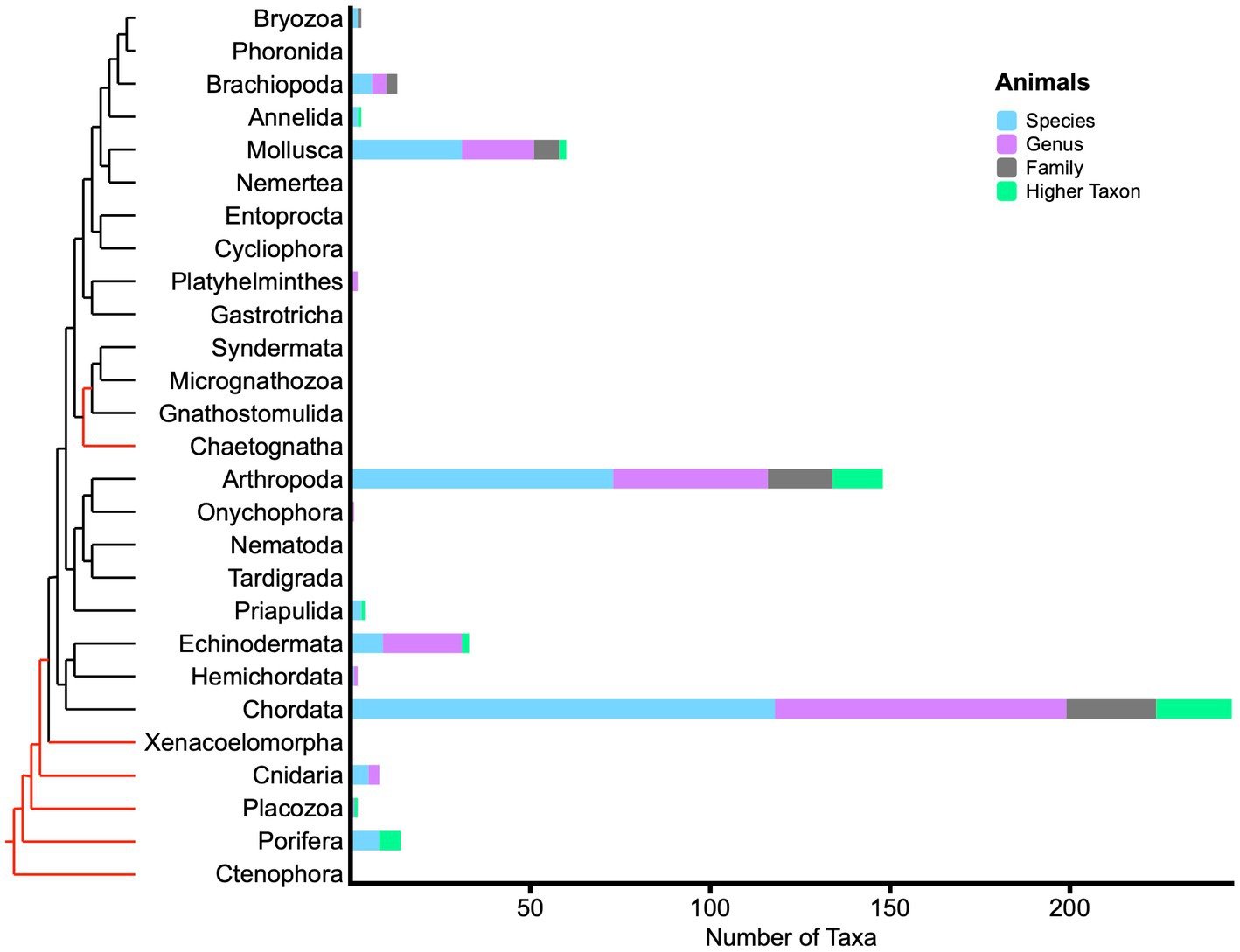
Figure 4. Phylogram with bar graph showing the distribution of living fossil taxa across animals. Bar colors indicate taxonomic ranks of 538 unique taxon names. Red sections of phylogram indicate significant uncertainty of relationship. Several “rogue” phyla that showed instability in a key phylogenomic analysis are not shown (Laumer et al., 2019). In our compilation, none of these latter phyla contain designated living fossils.
Turning next to the growth of the living fossil menagerie, the mix of different sorts of entities in a biological hierarchy has changed significantly over the past 160 years (Figure 5). Genera predominate until the mid-twentieth Century until overtaken by species, whose rate of addition then increases through the present. Taxa at family or higher taxon levels remain comparatively unimportant through the mid-twentieth Century, and are added at modest rates thereafter. Biomolecules as living fossil entities show a stunning increase from about 1990 through the present, paralleling technological advances in molecular biology and genetics. Among 52 unique biomolecule names, most are proteins, RNAs, viroids and short DNA elements, many of which are implicated as enzymes involved in “ancient” metabolic pathways. A small late-appearing group of 12 ‘other’ biological entities includes organelles, vestigial structures, and even a mitochondrial genome (Small, 2013) and a somatic cell line (Wang et al., 2019) as ‘living fossils.’
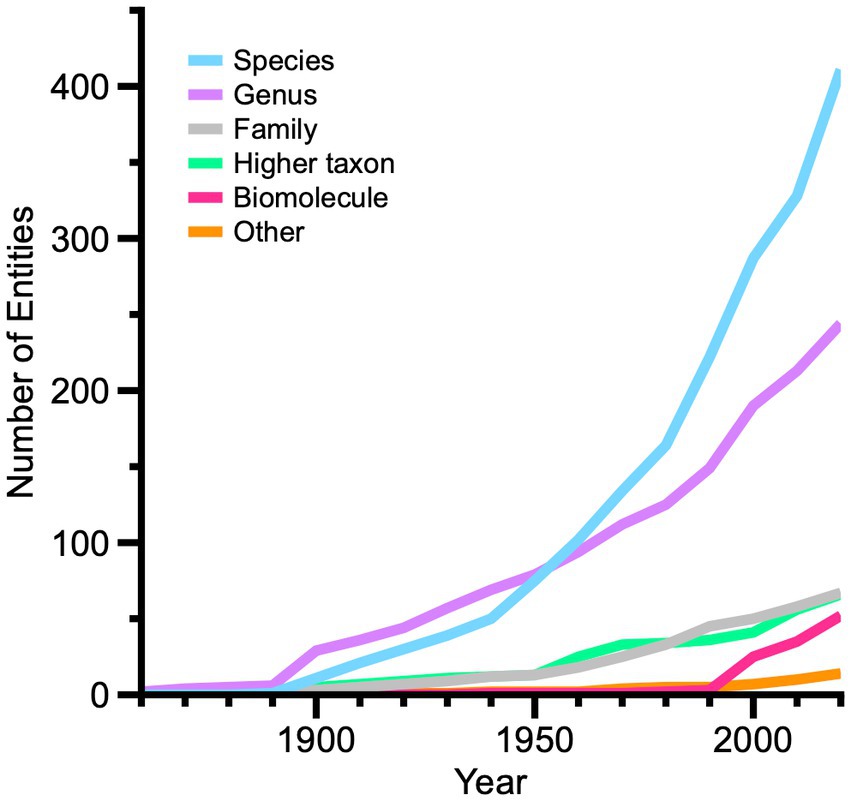
Figure 5. Line graph showing relative changes among 853 unique living fossil entities (taxonomic and non-taxonomic) at different levels in the biological hierarchy from 1860 to 2020. Entity names are summed by decade, based upon earliest occurrences in our compilation.
Recognition of living fossil taxa among the kingdoms of life varied significantly over the past 160 years (Figure 6). Initially and through the mid-twentieth Century, animals were designated most frequently. Mammalia, fishes with ganoid scales, insects and crustaceans were the most diverse subgroups. Green plant taxa were considerably less numerous, dominated strongly by eudicots with scattered designations of ferns, Cupressophytes, Cycadophytes and others. Unicellular taxa were hardly nominated at all during that interval. The rate of animal taxa additions increased thereafter, especially from 1980 onward. Living fossils in prokaryotes and eukaryotes (excluding plants and animals) were only designated significantly from the late twentieth Century to the present.
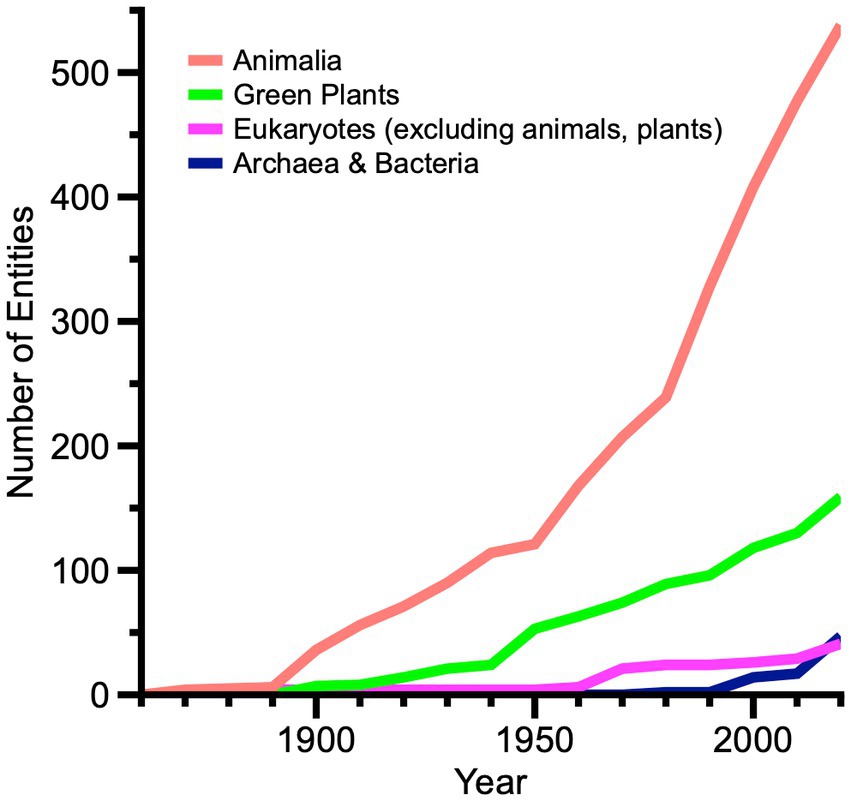
Figure 6. Line graph showing relative changes among 787 unique living fossil taxa among kingdoms of life from 1860 to 2020. Eukaryotes in this graph exclude green plants and animals. Taxonomic entity names are summed by decade, based upon earliest occurrences in our compilation.
The disciplinary focus of biological publications citing living fossil entities has expanded alongside the growth of the menagerie, especially from near the end of the twentieth Century to the present (Figure 7; note different scales in 7A and 7B). From 1860 through the mid-twentieth century, publications citing living fossils largely focused on systematics/taxonomy, natural history, and paleontology. Natural history publications became far less prominent after that time. Between 1951 and 1990, systematics/taxonomy and paleontology continued as leading subject areas, but evolution, morphology/structure and ecology became significant among publications. The interval from 1991 through 2020 saw dramatic upsurges in even more subject areas. While systematics/taxonomy publications continued to lead among subject areas, genetics/molecular biology showed the highest rate of increase and moved into second place. Following in order of prominence were evolution, physiology, paleontology, ecology, conservation biology, and biogeography. The category ‘other’ included modest numbers of publications in cell biology and developmental biology. Importantly, many authors of publications in newer subject areas including genetics/molecular biology, physiology, ecology, conservation biology and biogeography were asking questions about particular characteristics, deliberately choosing a living fossil entity (or entities) as a study system.
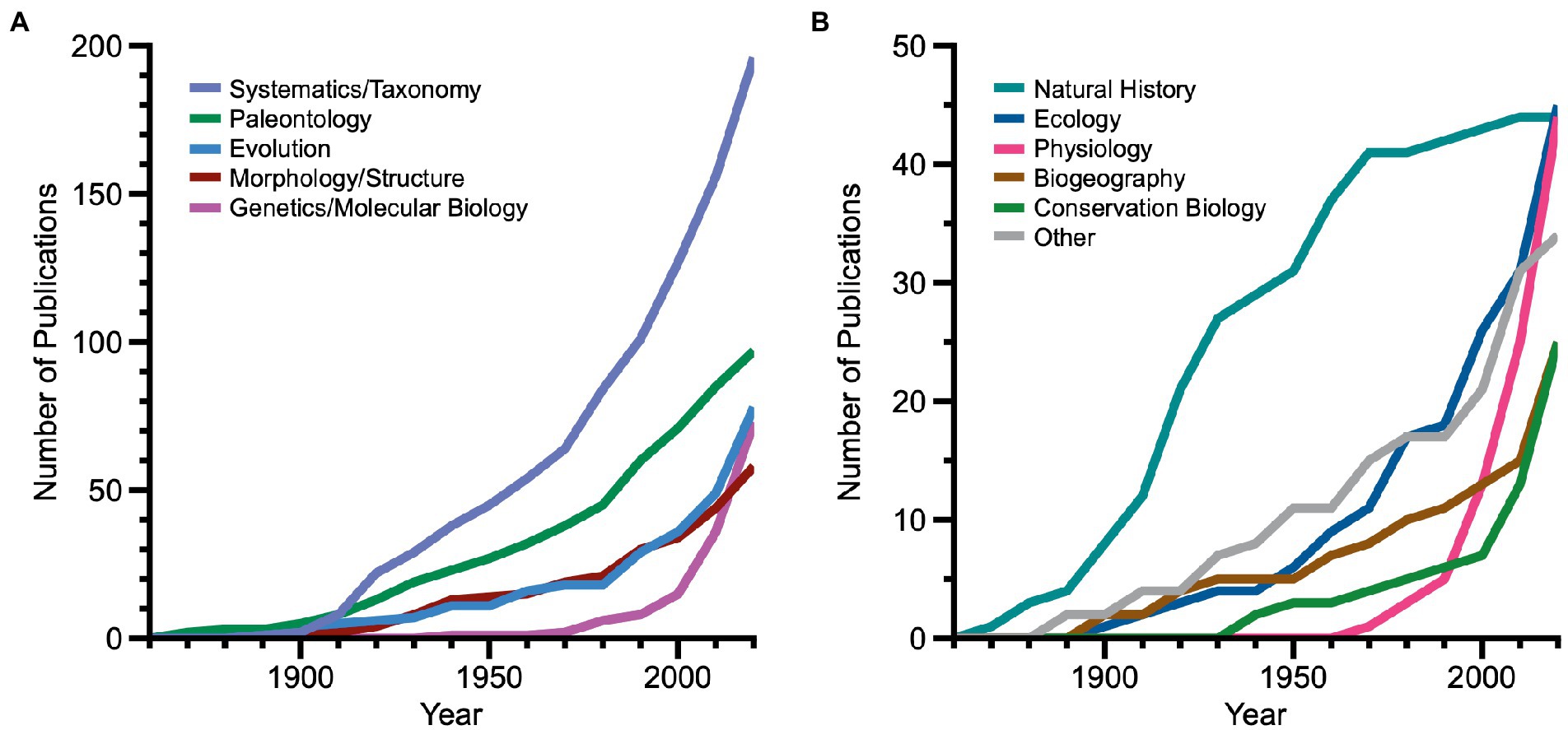
Figure 7. Line graphs showing relative changes in the primary biological subject area for each of 719 publications that designate living fossil entities from 1860 to 2020. Note that more than one entity may be noted in a given publication. (A) Five primary subject areas that each exceed 50 publications. (B) Six primary subject areas with fewer than 50 publications each. Note the different scales for numbers of publications in the two panels.
How have criteria or indications of living fossil status changed during the growth of the menagerie (Figure 8)? Among 273 criteria occurrences from 1860 to 1950, general ancestral similarity is applied most frequently for living fossils at 35%, followed by long duration at 29%, diversity relic at 22%, character ancestral similarity at 18%, geographic relic at 16%, and genealogical inference at 15%. Other criteria are trivial in comparison. Among 406 criteria occurrences from 1951 to 1990, general ancestral similarity remains the most common criterion at 39%, but is then followed by character ancestral similarity at 31%, long duration at 31%, genealogical inference at 23%, geographic relic at 19%, and diversity relic at 13%. The remaining criteria combined total 12%. The interval from 1991 through 2020 shows the most dramatic changes in the assortment of 723 criteria occurrences. Character ancestral similarity dominates at 33%, followed by long duration at 28%, genealogical inference at 23%, general ancestral similarity at 19%, diversity relic at 16%, geographic relic at 15%, slow evolutionary rate at 7%, fossil known first at 6%, and other criteria at 3%.
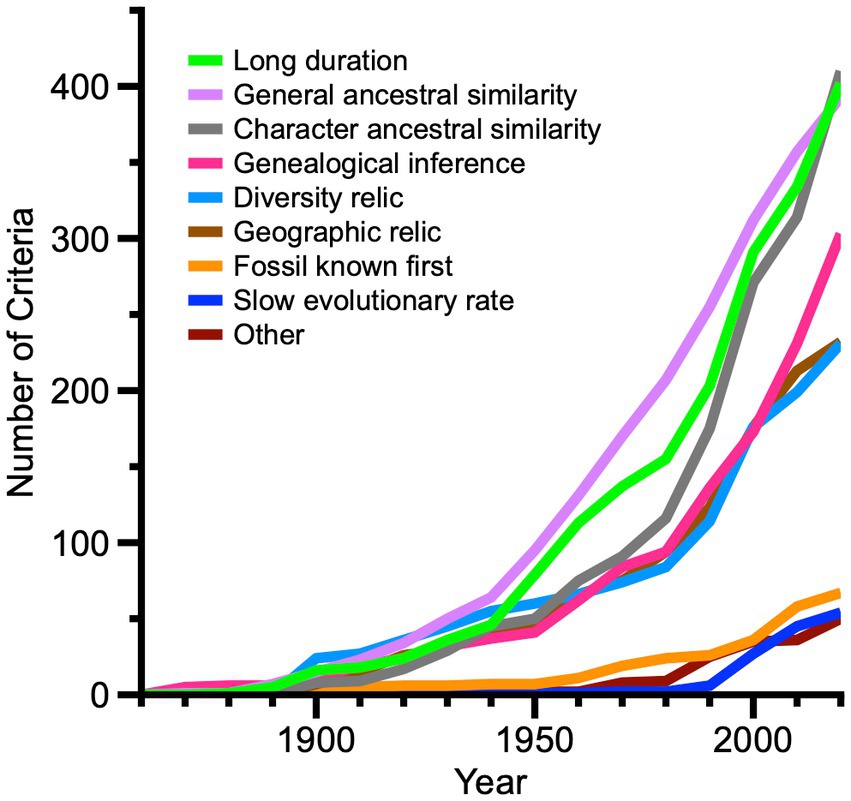
Figure 8. Line graph showing relative changes in criteria for assigning living fossil status among 853 unique living fossil entity names from 1860 to 2020. Note that many authors have used more than one criterion or indication for a given taxonomic or non-taxonomic entity. A given entity may be designated in several publications; after the earliest publication, unique additional criteria for that entity are recorded in relevant later years, but subsequent duplicate criteria are ignored.
How do groups of entities differ among criteria or indications of living fossil status that have been applied to them? Our NMS procedure begins with the full complement of 1,402 entities, including those recurring in different publications, and binary scores assigned for each of eight living fossil criteria based on authors’ original descriptions. We excluded ‘other criteria’ from the analysis; it would not contribute to interpretability, and all entities having this criterion were also scored for one or more additional criteria. Preliminary runs compared solutions from one to six dimensions, evaluating stress levels based on 50 runs apiece. We selected a two-dimensional solution for a run of 200 iterations, with a repetition to assess stability. The final NMS solution is shown in Figure 9 with a stress of 12.67. The proportion of variance represented by Axis 1 is 36%, with Axis 2 contributing an additional 30%. Vectors emanating from the centroid of the ordination space show the direction and relative influence of individual criteria that are correlated most strongly with the two axes (excluding the criteria long duration, fossil known first and slow evolutionary rate that are insignificant on these axes).
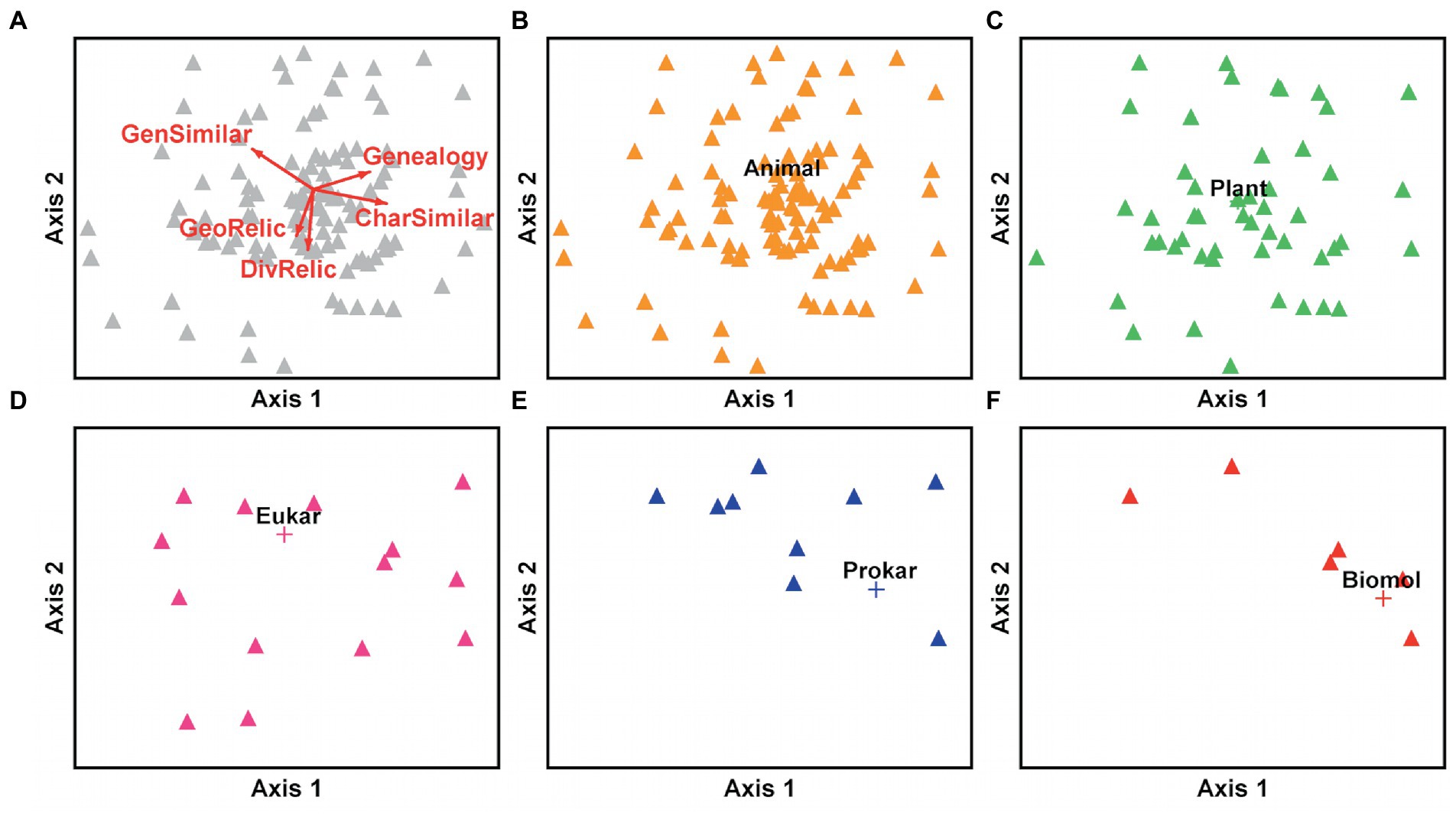
Figure 9. NMS ordination space derived from 1,402 original entities and 8 living fossil criteria, excluding the criterion ‘other.’ (A) Locations of all entities (grey) shown projected on NMS axes 1 and 2. Note that each point may represent one or many entity occurrences. Red vectors indicate significant influence and direction of the most important individual criteria on the two axes: GenSimilar = general ancestral similarity; CharSimilar = character ancestral similarity; Genealogy = genealogical inference; DivRelic = diversity relic; GeoRelic = geographic relic. The subsequent panels show the locations of groups of entities in the same ordination space: (B) Animal = Animalia; (C) Plant = green plants; (D) Eukar = eukaryotes not including animals and plants; (E) Prokar = prokaryotes, Archaea and Bacteria; (F) Biomol = Biomolecules. Group names are shown directly above distance-weighted centroids (+) for all occurrences of entities in each group (including multiple occurrences that sometimes coincide in a single point on these axes).
The criteria genealogical inference and character ancestral similarity strongly influence Axis 1 in a positive direction, in opposition to general ancestral similarity in a negative direction (Figure 9A). Axis 2 is strongly influenced by the criteria diversity relic and geographic relic in a negative direction, and less strongly by general ancestral similarity in a positive direction. These patterns are key to interpreting how groups of entities differ among living fossil criteria that have been applied to them. For instance, if a particular attribute such as membership in a given entity group is heavily present on the positive end of Axis 1, and a particular criterion is strongly and positively correlated with Axis 1, we may reasonably infer a substantial relationship. In subsequent panels, groups of entities are shown separately with distance-weighted centroids indicated as crosses (+) calculated from all of the occurrences in a group, including recurrences obscured under a single point in the ordination space.
One clear result is that no single living fossil criterion dominates among animals (Figure 9B) or green plants (Figure 9C). Authors have in fact applied a variety of criteria, sometimes even within the same publication. A similar disparity of criteria is indicated for eukaryotes, excluding animals and green plants (Figure 9D). Closer inspection of the original data suggests two eukaryote subsets. First, a number of dinoflagellates, foraminifera and diatoms with fossil records reveal criteria combinations of general ancestral similarity, long duration, and geographic or diversity relic. Second, a diverse group of unicellular algae, flagellates, slime molds and others that lack any substantive fossil record reveal combinations of character similarity, genealogical inference, and in a few instances an inference of long duration despite lacking empirical fossil evidence. Prokaryotes (Figure 9E) and biomolecules (Figure 9F) reveal a strikingly different pattern, most characterized by ancestral character similarity and lacking the criteria diversity relic or geographic relic. Some cyanobacteria do have additional designations of general ancestral similarity and long duration, reflecting the striking morphological similarities of living and Precambrian forms illustrated by Schopf (1994). Biomolecules, lacking a fossil record, are also characterized as living fossils via genealogical inference.
We hypothesized that methodological changes in systematics and technological advances in genetics and genomics over time could have an influential bearing on the increase in diversity of living fossil entities, the application of certain criteria, and critiques of previously recognized living fossil entities. Figure 10 shows the explosive increase in numbers of publications involving living fossils that explicitly include morphological or molecular phylogenetic analyses, beginning in the 1980s and 1990s and continuing through the present. Examining the original data, animals dominate the morphological phylogenies, and many have combinations of criteria including character or general ancestral similarity, diversity or geographic relic, or long duration. Systematics/taxonomy is overwhelmingly the most common subject area of these publications, followed by evolution and paleontology. For molecular phylogenies, animals again dominate, but a sizeable number of plants occur, as do smaller numbers of eukaryotes and biomolecules. Molecular phylogenies appear in a wider variety of publication subject areas: first systematics/taxonomy, followed by evolution, molecular biology, and small numbers across all subject areas except natural history.
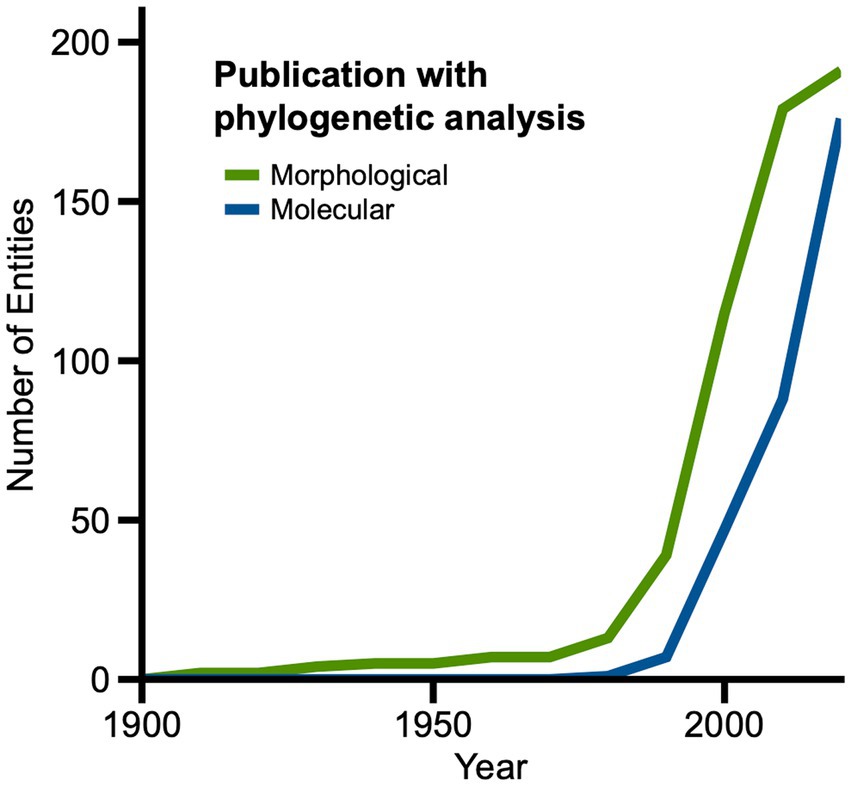
Figure 10. Line graph showing changes in numbers of living fossil entities from 1860 to 2020 designated in publications that incorporate morphological or molecular phylogenetic analyses.
Finally, we looked at changes in the frequency of publications in which one of the explicit aims is categorizing an entity (or entities) as a living fossil (Figure 11). These publications did not begin to become significant until the latter half of the twentieth century with the appearance of several popular science publications on living fossils (Burton, 1956; Silverberg, 1966; Thenius, 1973, 2000) and an important research compendium (Eldredge and Stanley, 1984). Animals and plants (to a much lesser extent) were the focal taxonomic groups of these volumes. Morphological phylogenies were featured in several chapters of the volume edited by Eldredge and Stanley, and more than one chapter challenged previously assigned living fossil status for particular taxa. After 1990, phylogenetic inferences and an increasing number of morphological and molecular phylogenies were used in publications aiming to categorize living fossils. While animals and plants dominate these later studies, biomolecules, prokaryotes and eukaryotes are also present. Combinations of living fossil criteria become more evenly distributed among long duration, general ancestral similarity, diversity relic, geographic relic, character similarity, and genealogic inference, respectively, with lesser but now significant numbers for slow evolutionary rate and fossil known first. Interestingly, the past decade also witnesses a number of papers aiming to revise or challenge prior methods for categorization of certain canonical living fossils, frequently by asserting the priority of one or a few living fossil criteria over others (e.g., Nagalingum et al., 2011; Vanschoenwinkel et al., 2012; Casane and Laurenti, 2013; Mathers et al., 2013; Naville et al., 2015; Vaux et al., 2019).
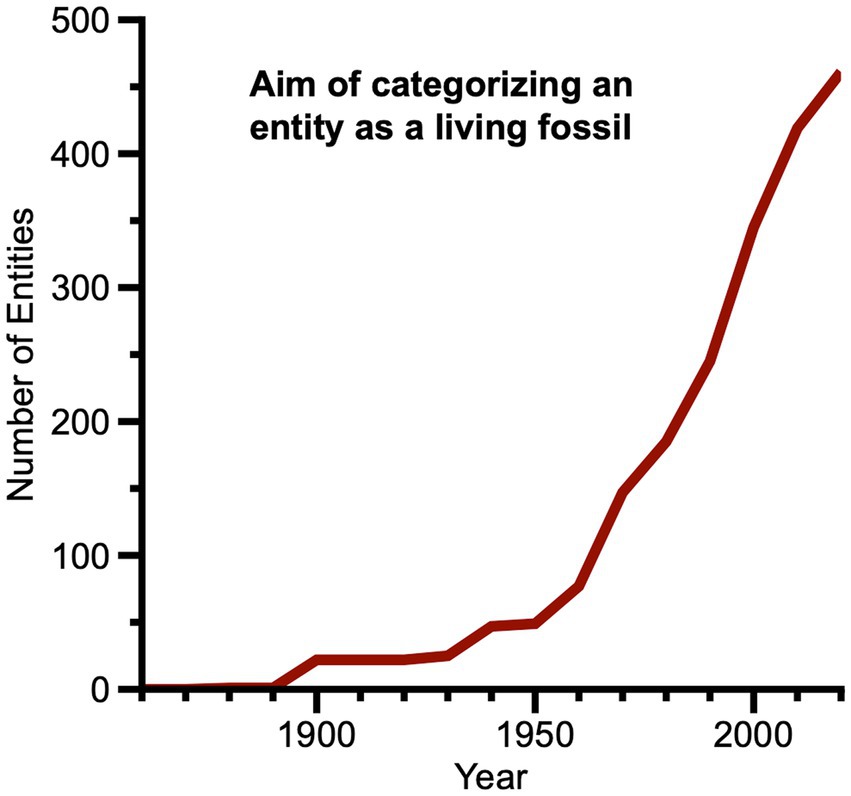
Figure 11. Line graph showing changes from 1860 to 2020 in numbers of entities in publications where at least one of whose aims appeared to be categorizing an entity as a living fossil (or not).
Discussion
We have shown that there are many ways in which the living fossil concept has expanded since 1860. The broad concept and each of our surveyed criteria persisted even as biological theories, technologies, and research programs all diversified and changed, a testament to the continued scientific utility of the concept. In fact, this expansion seems to be not only unavoidable, but also desirable. We believe this concept is a case not unlike that of the gene concept, whose pluralistic use is “context-sensitive” and “promotes scientific practice” (Brigandt, 2010, p 20). The role of a capacious living fossil concept as a research agenda (Lidgard and Love, 2018, 2021) embraces the view that open-ended, to some extent ambiguous scientific concepts can facilitate integration across disciplines, theories and interrelated questions, can be contextualized to serve particular explanatory tasks, and thus can be both productive and forward-looking (Brigandt, 2020; Neto, 2020; Haueis, 2021; Sterner, 2022). The trends in our data suggest this expansion will continue.
The nature of the living fossil concept exposes two central conceits of evolutionary biology: change and extinction. Combining diverse criteria under an umbrella construct that is dynamic rather than static and is geared toward epistemic goals (Brigandt, 2012, 2020) provides a framework to interrogate those conceits. In our discussion we look at the historical trends evidenced by our data and changes that might have facilitated some of those developments. Then, we consider the challenges and utility of that expansion, and where it may go in the future. Finally, we reflect on what the expanded use of the living fossil concept suggests about integrative theorizing in evolutionary biology.
Historical Trends and Expansion. The living fossil concept is not an unfortunate artefact of the nineteenth century that has somehow plodded its way into the present. Rather, its expansions and shifts have followed the course of drastic conceptual and technological changes brought on by the Modern Synthesis and its extensions into evolutionary developmental biology and elsewhere (Laland et al., 2015), phylogenetic systematics (Suárez-Díaz and Anaya-Muñoz, 2008; Rieppel, 2016; Sterner and Lidgard, 2018), the paleobiological revolution (Sepkoski and Ruse, 2015), and genomics (McGuire et al., 2020), among other notable developments in evolutionary disciplines. Prominent examples are the comparatively late twentieth century living fossil assignments for significant numbers of prokaryotes and eukaryotes apart from animals and plants. In the nineteenth century, layered calcareous fossil forms presumed to be biological (e.g., Cryptozoon) were known in strata below the oldest undisputed animals, but it was only in 1954 that definitive living counterparts, stromatolites, were discovered in Shark Bay, Australia (Logan, 1961). Stromatolites, which are organosedimentary deposits whose fossil record exceeds three billion years, are sometimes termed ‘living fossils.’ Complex biofilm communities dominated by a diverse range of Bacteria and Archaea trap and bind detrital sediment and precipitate carbonate minerals, their growth regulating the layered structure (Vasconcelos et al., 2006). The identities of prokaryotes in these communities were first determined using light and electron microscopy, and by the 1990’s analogous cell forms of living and Precambrian taxa were being recognized (Schopf, 1994). Stromatolites were perceived for about a century as biological ‘wholes’ before technological and actualistic advances enabled their prokaryote ‘parts’ to be studied as plausible living fossils in their own right. Broader recognition of living fossil taxa among Archaea, Bacteria, and unicellular eukaryotes lacking much of a fossil record came later with groundbreaking advances in molecular biology, genetics, genomics, and advanced phylogenetic algorithms. The rRNA-based molecular analyses of Carl Woese and others in the late twentieth century broke apart the morphological and biochemical classificatory prokaryote-eukaryote dichotomy that had prevailed for much of that century, replacing it with a now-dominant foundational phylogenetic view based on genetic evidence (Woese, 1998; Sapp, 2005). Subsequent advances opened up the space for inferences of evolutionary relationships and relative age dating among newly recognized groups (and individual taxa) within Archaea, Bacteria and eukaryotes (Rosselló-Mora, 2005; Williams and Embly, 2015; Burki et al., 2020; Coleman et al., 2021; and many others) that had simply been unavailable to previous generations of researchers. Certain of these changes are not without controversy, as challenges such as culture-independent genome sequencing have put traditional phenotypic taxonomic and nomenclatural practices in turmoil (Adl et al., 2019; Hugenholtz et al., 2021). Elsewhere, progress in microbiology, understanding paleoeenvironmental conditions in the Archaean, and a hypothesized RNA world has led researchers to infer “ancient” metabolic pathways and evolutionary relationships in designation of certain ribosomes and viroids as living fossils (Flores et al., 2014; Diener, 2016; Lupas and Alva, 2017).
Scientific use of the living fossil concept has not been at odds with these kinds of changes. Indeed, as the diversity of living fossil entities has increased steadily, the mean number of criteria per entity has risen from 1.2 to 1 in the interval of 1861–1880 to 2.3 to 1 by 2020, with only minor fluctuations. That change in ratio indicates that as the number of entities increase over the intervals of time in our study, criteria assigned by scientists escalate more rapidly. Understanding this growing conceptual diversity is an important task to weigh the effectiveness of a broad living fossil concept. While we will not fully delve into the historical context of the trends our data reveals, it is inescapable to consider the successive contribution of changes in technology, disciplines, and taxonomic methods since 1860 that have shaped the broad purview of the living fossil concept today. Those historical trends are perhaps even more important for revealing why and how ‘living fossil’ has retained its status as an expansive, but operationalizable, term for almost two centuries.
No prior study has looked explicitly at the diversity of living fossil entities, their phylogenetic distribution, taxonomic ranks, and non-taxonomic entities. Doing so reveals several key trends. First, genetics, genomics, and phylogenetics have revolutionized the relationships among groups, and associated technological developments in disciplines such as molecular biology have provided more tools to investigate understudied groups, such as Archaea, Bacteria, unicellular eukaryotes and many lower invertebrates and land plants (Laumer et al., 2019; Leebens-Mack et al., 2019; Burki et al., 2020; Coleman et al., 2021; Tahon et al., 2021). Those same technological developments, including high throughput sequencing, GenBank, phylogenetic algorithms, proteomics, and biochemical pathways research, might have been expected to refine those entities that were considered living fossils. Certainly some studies have referred to certain DNA sequences to push against previous living fossil designations on the basis of inferred rapid rates of molecular evolution or comparatively recent diversification (Graczyk, 2005; Hay et al., 2008; Nagalingum et al., 2011; Vanschoenwinkel et al., 2012; Casane and Laurenti, 2013; Mathers et al., 2013; Wood et al., 2020). Such studies signify the living fossil concept and its menagerie as compelling sites for exploring part-whole relations. Biomolecular and phenotypic characters, when viewed in isolation, may embolden some scientists to champion disagreeing inferences about an entity’s living fossil status. Yet when these characters are instead considered together as complementary evidence within a living fossil research agenda (Lidgard and Love, 2018, 2021), they motivate a much richer integrative understanding and contribute to shared epistemic goals (Brigandt, 2010, 2020). Technology has not restricted the living fossil menagerie. More accurately, it has expanded the toolkit to investigate questions related to living fossil entities as well as added new entities into the menagerie. Studies have likely been motivated to pick certain entities in part based on their living fossil status, treating them as model systems.
Challenges and Future Possibilities. The growing diversity of living fossil entities and criteria might be seen to present a challenge to continuing use of the concept. As a comparison, the gene concept has shifted over time in its use along a narrative from ‘classical’ to ‘molecular,’ even given the nuanced overlap in use demonstrated by historical and philosophical scholarship on the concept (Beurton et al., 2000; Waters, 2014; Brigandt, 2020). Despite the trends presented in our data, there is no easy periodization or well-ordered categorization of the living fossil concept akin to the shift from ‘classical’ to ‘molecular’ epithets of differing meanings of the ‘gene.’ Our data do not warrant this, and in fact it might be a hinderance to the concept’s historical and contemporary usage to cleave it by use along chronological joints that are “fuzzy” at best. Historically and today, there are different uses of the term in operation for any given entity, sometimes even in the same study, that occur within biological disciplines and other sciences. From Darwin’s conceptualization of living fossils, to punctuated equilibrium and protracted evolutionary stasis, to highly conserved genes, proteins and various complex molecules, the living fossil concept is legitimately applied across research questions and domains. As a research agenda, it integrates questions and epistemic goals spurred by seemingly disparate phenomena studied by different disciplines (as evidenced by the compilation in this Research Project; Love, 2008; Lidgard and Love, 2021; Watkins, 2021).
This plurality informs the critics’ sense that the living fossil concept is endlessly capacious, posing several challenges to scientists. While the concept has produced a diverse menagerie of model-like entities through which researchers address myriad related questions, it is not entirely clear how the concept should handle entities at different taxonomic ranks and also non-taxonomic entities. We suggest that as a research agenda, the concept is already well suited towards grappling with multiple scales, as part-whole problems are central to living fossil designations: what is selected as a living fossil differs across entities and criteria. As entities continue to diversify at both greater and smaller scales, the comparative nature of living fossil designations will demand deeper consideration for what exactly is being used to infer a living fossil status: a whole organism or taxon, or parts such as genes or particular morphological characters as proxies for the whole? Inferences about the origin of specific entities from proteins to certain Archaea and Bacteria, based on their ubiquity, functional necessity, or simplicity, have elevated certain contemporary entities to a living fossil-proxy status due to their inferred antiquity and basal phylogenetic positions in the tree of life. Discerning what counts as a living fossil, or what is being conserved in the case of newly added members of the menagerie such as genes—the reading frame, the ‘functional’ components, or the entire sequence—is a continuation of these part-whole problems and scalar decisions. They do not threaten the living fossil concept’s utility, but rather provide new avenues to explore those integrative questions already present in evolutionary biology.
Many of the challenges facing the living fossil concept are encountered in other domains of biological research. For example, as technological improvements allow data on an ever-greater number of entities to fall under the concept’s agenda, our ability to analyze that data will be tested. One area undergoing rapid change is at the intersection of development and evolution (DiFrisco and Wagner, 2022; DiFrisco et al., 2022), where the identity of characters and the meaning of homology pose challenges to the ways we view characters in analyses that compare evolutionary patterns from different perspectives of genes and phenotypes. In another area, genomic data is coming faster than our current algorithmic ability to analyze it. Developments that redraw the lines of phylogenetic relations, for example lateral gene transfer (Blais and Archibald, 2021; Cote-L’Heureux et al., 2022), also raise further questions for how the concept relies on assumptions of descent and lineage. Still, several scholars have already shown that it is just these kinds of scientific problems, those which sit between disciplines and scales, that the living fossil concept is ripe to address (Brigandt, 2020; Lidgard and Love, 2021; Watkins, 2021). Ginkgo, discussed above, presents one case in which the entity’s historic survival, distribution, and evolutionary relationships make it an excellent candidate for pursuing questions of plant evolution between living and fossil taxa. In such cases, the entity’s living fossil attributes are the explanandum—a particular evolutionary development to be explained—but they also offer a remarkably persistent depth of data for other studies, given how long they have existed. Watkins (2021) argues that, similar to homology, living fossils also furnish a “productive combination” in that the entities are both fossilized and living; they enable developmental biology to integrate with paleontology, and the living fossil entities can offer evidence for inferences about the relationship between morphological, phenotypic, and genetic similarity or stasis and broader lineage trends such as persistence and evolutionary rates. As the menagerie expands, these productive combinations may well multiply.
Historical trends and perceptions of living fossils in academic and societal spaces render the living fossil concept ambiguous; no single criterion serves for all purposes and subsidiary questions. In this case, the uncertainty around operationalizing the concept is collective, rather than individual (Solomon and McMullen, 1991; McMahan and Evans, 2018). It is likely that individual scientists themselves are not uncertain about their intended meaning of the term ‘living fossil.’ But among scientists there are several perspectives about what constitutes a living fossil concept, and those perspectives may differ. What options, then, do scientists have for their use of a pluralist living fossil concept? From our perspective, retaining the umbrella living fossil concept for future pluralistic growth of the menagerie and integrative questions is preferable. However, a range of alternative fates exist, for example: jettison the term, keep the term but prioritize qualifiers for different uses, or retain the term with an emphasis on restricting its use to meet the questions within the research program’s problem spaces (see also Turner and Han, 2023). To lose the term entirely would jeopardize the utility of a research agenda or umbrella construct and the integration and interdisciplinarity that comes with it. Fruitful arguments have been made against outright removal of the concept (Turner, 2019; Lidgard and Love, 2021; Watkins, 2021). Offering qualifiers to the term has been proposed, as in the case of an endangered living fossil concept combining conservation and phylogenetic approaches (Vargas et al., 2020). Keeping the term with restrictions towards its use has been proposed as well, from substituting the concept “stabilomorph” for some previously recognized living fossils based on criteria of relative morphological stability (Kin and Blażejowski, 2014), to a quantitative “Evolutionary Performance Index” derived from time-calibrated phylogenetic trees (Bennett et al., 2018), to a phylogenetic living fossil concept based on a smaller cluster of traditional criteria (Turner, 2019; Turner and Han, 2023). We think there is virtue in the ambiguity consequent upon a living fossil concept that does not prescribe its menagerie a priori, but rather allows it to build itself through the questions and observations that the concept itself raises (Lidgard and Love, 2021). While we think it is premature to restrict living fossils as an umbrella concept, as its criteria and therefore integrated problem spaces continue to develop in relation to its diversifying menagerie, future restrictions to aid framing the research program’s questions might be warranted. The pluralistic value that comes from ambiguity in scientific concepts and terms has been shown to be largely positive for interdisciplinary problems, and is reason enough then to keep the term as an umbrella concept, despite the centrifugal pull of its polysemy (Leonelli, 2016; Haueis, 2021; Sterner, 2022).
An Integrative Research Program: Change and Extinction. The living fossil concept has clearly expanded in how it has been operationalized, what has counted as a living fossil, and the reasons why. It has persisted as a research agenda whose utility stems from its integrative questions and its entities as sites for interrogating those questions. What, then, has been retained throughout these changes, that might suggest some continuity in the uses of the concept? Or rather, to what problem areas does the concept offer promise? At its core are two seemingly unchanging critical features of evolutionary theory that demand such an integrative approach: change and extinction. These conceits lie at the center of most uses—public and academic—of living fossils. At their most extreme they suggest a defiance of expected change, or colloquially, an absence of evolution. For a scientist, however, they point towards processes such as stabilizing selection, developmental constraints, conservation of genes or metabolic pathways, and like phenomena (Lidgard and Hopkins, 2015) that warrant attention.
A cursory glance at the use of ‘living fossil’ and ancillary terms in non-academic or non-biological domains further reveals what makes living fossils interesting within scientific practice. Persistent public use of the term ‘living fossils’ reinforces scientific interest in these entities. The term’s historical deployment in non-biological arenas supports the idea that at its colloquial base, it evokes an aberration from the entrenched expectations of evolution. Historically, ‘living fossil’ or related rhetorical terms were deployed to mark something as out of time, or outdated. Within nineteenth-century examinations of prehistory, a comparative method developed, which drew on living peoples as analogues for prehistoric humans (Burrow, 1966; Meek, 1976; Bowler, 1989; Manias, 2015). This method, seen in the influential ethnological work of John Lubbock in Britain and Gabriel de Mortillet in France, informed social evolutionists’ placement of human development along a linear model of progress, in which Europeans represented a pinnacle, and modern so-called ‘savages’ were analogous relics of prehistoric humans (Bowler, 1989; Manias, 2015). In this way, the world was painted as containing pockets of the past—living fossils that had persisted to the present. Sometimes, these pockets spurred concern that along with their disappearance, the knowledge they could impart about the past would be lost as well. In nineteenth and early twentieth-century anthropological discourse, disappearing human populations were frequently described in terms similar to living fossil entities. Anthropologists Baldwin Spencer and F.J. Gillen wrote in The Arunta: A Study of Stone Age People that “Australia is the present home and refuge of creatures, often crude and quaint, that have elsewhere passed away and given place to higher forms. This applies equally to the aboriginal as to the platypus and kangaroo. Just as the platypus, laying its eggs and feebly suckling its young, reveals a mammal in the making, so does the Aboriginal show us, at least in broad outline, what early man must have been like…” (Spencer and Gillen, 1927, vii; for more on this framing in anthropology, see Brantlinger, 2003). The use of terms aligned with ‘living fossils’ in such discourse was almost always pejorative at best, often harmful in its reinforcements of eighteenth and nineteenth-century ideals of racial hierarchies based on supposed measures of societal progress, and contributing to conceptions associated with ‘scientific racism.’
It should be no surprise that Australia, a British colony, was so often featured in discussions of living fossil human populations, as well as relic plants and animals. India, too, was cited in these ways and was seen as critical to understanding human origins (Simpson, 2018). Colonialism was a driving force in both a salvage mindset to record data on places and people before they may be lost, as well as a comparative social framework. In 1839 the British Association for the Advancement of Science drew up a list of questions to be sent to “those who may travel or reside in parts of the globe inhabited by the threatened races” (Darwin et al., 1841). The survey was designed to collect wide-ranging information on races deemed primitive. Colonialism was both the opportunity and the competitive driving force for such an enterprise: “Britain, in her extensive colonial possessions and commerce, and in the number and intelligence of her naval officers, possesses unrivalled facilities for the elucidation of the whole subject; and it would be a stain on her character, as well as a loss to humanity, were she to allow herself to be left behind by other nations in this inquiry” (Darwin et al., 1841).
The given reasons for a group persisting as a living fossil could vary, but usually existed alongside the sense of geographic discovery embedded in such colonial exploration. In many cases, they referred to a state of isolation from the civilized world. Robert Watson Frazer, a scholar and civil servant in British India wrote in 1897 that, “During the taking of the Census of 1872 it was ascertained that one-twelfth of the population of India, nearly twenty millions of human beings, consisted of these living fossils of primeval times. There they remain, a strange study to the historian and anthropologist” (Frazer, 1896). He reasoned that they could persist in such a state as they remained undisturbed in the dense forests of the remote mountain ranges to which they had been driven earlier in their history. Even into the mid-to late-twentieth century, genetic data would furnish studies that sought to examine whether living populations could stand in for ancestral ones, and explored the role of purportedly historically-isolated populations as glimpses into humankind’s ancestral history (Cavalli-Sforza, Carmelli, 1979; see Sommer, 2015 for further discussion of the study). To Darwin, European progress was a likely culprit for preventing progressive development in other populations; as he wrote to Chalres Lyell, “man is ‘improving off the face of the earth’ even races nearly his equals” (Darwin, C. to C. Lyell, 1860). This claim would be echoed by others into the twentieth century, pointing to the stifling effect of subjugation by European imperialism (Sollas, 1911 is a notable example; for further discussion see Manias, 2015). Whether geographic isolation or inflicted stagnation through imperial encounters, the reasons supposed for the presence of so-called primitive human races maintained from the past underscored the role of colonial expansion, collection impulse, and subjugation within the comparative method of ethnologists and naturalists.
Use of the term ‘living fossil’ as a rhetorical device, and use of ancillary terms such as relics and persistent types, have historically conveyed an aberration from an expected descent with modification or extinction. The expectation for extinction in particular was reinforced by the depiction of anthropological living fossils as existing on borrowed time, their demise inevitable (Brantlinger, 2003). The indigenous Australians evoked such extinction narratives (McGregor, 1993; Brantlinger, 2003; Kuklick, 2006). This mentality persisted into the twentieth century, when human blood samples collected via an apparatus of British colonial and metropolitan networks from remote regions were transported and stored to preserve biological information from indigenous communities seen as teetering on the brink (Bangham, 2014; Sommer, 2015; Radin, 2017). Even today, linguists have identified a trend in language preservation, in which eulogizing relics or “living fossil languages” presupposes their inevitable extinction and wards off efforts to protect such languages (Ingebretson, 2022). In the natural world as well, these expectations for extinction and change may explain some of the staying power of living fossil in the public eye. The entities labelled living fossils capture public imagination with their “primitive” forms, and their evocative defiance of the expectation that over time the image of life on earth has drastically changed through diversification and extinction (Ward, 1992).
It is important to recognize the history of the term living fossil to understand when and why it has reached too far. In its current applications to the natural world, however, the fact that these living fossil entities have captured scientific interest and public fascination by seemingly defying both evolutionary expectations is not an obstacle for evolutionary biology research. It is a reminder that the scientific enterprise benefits from systems and research programs that can expand and integrate as much as their underlying theories suggest is the nature of their phenomena of study (Kitcher, 1999; Brigandt, 2012). Unifying the living fossil concept under one criterion, or jettisoning it altogether, presents a problem in that the phenomena of study are not fully unified. One need only consider the range of criteria, differing levels of entities in the biological hierarchy, and the menagerie we have documented to understand that the diversity within the concept is too naturalized in practice and in nature to reduce into a single criterion. Fracturing the concept also disregards the common core that underlies both rhetorical and scientific interest in living fossils: the expectation for evolutionary change and extinction. The integrative nature of living fossil research is rational as it is reflective of the integrative, but not conflated, nature of the phenomenon.
Data availability statement
The original contributions presented in the study are included in the article/Supplementary material, further inquiries can be directed to the corresponding author/s.
Author contributions
SL was primarily responsible for the conception of the idea and the analyses. SL and EK contributed equally to compiling the data, scoring data attributes, and writing. All authors contributed to the article and approved the submitted version.
Funding
This work was supported by the Negaunee Integrative Research Center at Field Museum provided funds for open access publication.
Acknowledgments
S. Clute, M. Juhn, and C. Scheutte provided crucial assistance during initial surveys of relevant publications. Comments from M. Dresow improved the manuscript. Discussions with A. C. Love helped to shape our ideas throughout this project. We are grateful to all.
Conflict of interest
The authors declare that the research was conducted in the absence of any commercial or financial relationships that could be construed as a potential conflict of interest.
Publisher’s note
All claims expressed in this article are solely those of the authors and do not necessarily represent those of their affiliated organizations, or those of the publisher, the editors and the reviewers. Any product that may be evaluated in this article, or claim that may be made by its manufacturer, is not guaranteed or endorsed by the publisher.
Supplementary material
The Supplementary material for this article can be found online at: https://www.frontiersin.org/articles/10.3389/fevo.2023.1112764/full#supplementary-material
Footnotes
1. ^https://scholar.google.com
2. ^https://www.hathitrust.org/
3. ^https://proquest.libguides.com/britishperiodicals
4. ^https://www.bnf.fr/en/gallica-bnf-digital-library
References
Abrams, M. (2012). Measured, modeled, and causal conceptions of fitness. Front. Genet. 3, 1–12. doi: 10.3389/fgene.2012.00196
Adl, S. M., Bass, D., Lane, C. E., Lukeš, J., Schoch, C. L., Smirnov, A., et al. (2019). Revisions to the classification, nomenclature, and diversity of eukaryotes. J. Eukaryot. Microbiol. 66, 4–119. doi: 10.1111/jeu.12691
Agassiz, L. (1833-1844). Recherches sur les poissons fossiles. Neuchatel: Petitpierre. Available at: https://www.biodiversitylibrary.org/item/23069 (Accessed March 13, 2023).
Avise, J. C., Nelson, W. S., and Sugita, H. (1994). A speciational history of" living fossils": molecular evolutionary patterns in horseshoe crabs. Evolution 48, 1986–2001. doi: 10.2307/2410522
Bangham, J. (2014). Blood groups and human groups: collecting and calibrating genetic data after world war two. Stud. Hist. Phil. Biol. Biomed. Sci. 47, 74–86. doi: 10.1016/j.shpsc.2014.05.008
Bayramov, A. V., Ermakova, G. V., Kucheryavyy, A. V., and Zaraisky, A. G. (2018). Lampreys, “living fossils,” in research on early development and regeneration in vertebrates. Russ. J. Dev. Biol. 49, 327–338. doi: 10.1134/S1062360418080015
Beerling, D., and Royer, D. L. (2002). Fossil plants as indicators of the Phanerozoic global carbon cycle. Annu. Rev. Earth Planet. Sci. 30, 527–556. doi: 10.1146/annurev.earth.30.091201.141413
Bennett, D. J., Sutton, M. D., and Turvey, S. T. (2018). Quantifying the living fossil concept. Palaeontol. Electron. 21. doi: 10.26879/750
Berry, E. W. (1924). Age and area as viewed by the paleontologist. Am. J. Bot. 11, 547–557. doi: 10.1002/j.1537-2197.1924.tb05799.x
Beurton, P. J., Falk, R., and Rheinberger, H.-J. (2000). The concept of the gene in development and evolution: Historical and epistemological perspectives. Cambridge: Cambridge University Press.
Blais, C., and Archibald, J. M. (2021). The past, present and future of the tree of life. Curr. Biol. 31, R314–R321. doi: 10.1016/j.cub.2021.02.052
Bowler, P. J. (2003). Evolution: The history of an idea. 3rd ed. Berkeley, CA: University of California Press.
Bowler, P. (1989). The invention of Progress: The Victorians and the past. Cambridge, MA: Blackwell.
Braasch, I., Peterson, S. M., Desvignes, T., McCluskey, B. M., Batzel, P., and Postlethwait, J. H. (2015). A new model army: emerging fish models to study the genomics of vertebrate Evo-devo. J. Exp. Zool. B Mol. Dev. Evol. 324, 316–341. doi: 10.1002/jez.b.22589
Brantlinger, P. (2003). Dark vanishings: Discourse on the extinction of primitive races, 1800–1930. Ithaca: Cornell University Press.
Brigandt, I. (2010). The epistemic goal of a concept: accounting for the rationality of semantic change and variation. Synthese 177, 19–40. doi: 10.1007/s11229-009-9623-8
Brigandt, I. (2012). “The dynamics of scientific concepts: the relevance of epistemic aims and values,” in Scientific concepts and investigative practice. eds. U. Feest and F. Steinle (Berlin: De Gruyter), 75–103.
Brigandt, I. (2020). “How are biology concepts used and transformed?” in Philosophy of science for biologists. eds. K. Kampourakis and T. Uller (Cambridge: Cambridge University Press), 79–101.
Buggs, R. J. A. (2021)., et al.The origin of Darwin’s “abominable mystery.” Am. J. Bot. 108, 22–36. doi: 10.1002/ajb2.1592
Burki, F., Roger, A. J., Brown, M. W., and Simpson, A. G. B. (2020). The new tree of eukaryotes. Trends Ecol. Evol. 35, 43–55. doi: 10.1016/j.tree.2019.08.008
Burrow, J. (1966). Evolution and society: A study in Victorian social theory. Cambridge: Cambridge University Press.
Casane, D., and Laurenti, P. (2013). Why coelacanths are not ‘living fossils.’. BioEssays 35, 332–338. doi: 10.1002/bies.201200145
Cavalli-Sforza, L. L., and Carmelli, D. (1979). “The Ashkenazi gene pool: Interpretations,” in The genetic diseases among Ashkenazi Jews. eds. R. M. Goodman and A. G. Motulsky (New York: Raven Press), 93–104.
Cavin, L., and Alvarez, N. (2022). Why coelacanths are almost “living fossils”? Front. Ecol. Evol. 10. doi: 10.3389/fevo.2022.896111
Cavin, L., and Guinot, G. (2014). Coelacanths as “almost living fossils.”. Front. Ecol. Evol. 2, 1–5. doi: 10.3389/fevo.2014.00049
Cheung, T. (2006). From the organism of a body to the body of an organism: occurrence and meaning of the word “organism” from the seventeenth to the nineteenth centuries. Br. J. Hist. Sci. 39, 319–339. doi: 10.1017/S0007087406007953
Christenhusz, M. J., and Byng, J. W. (2016). The number of known plants species in the world and its annual increase. Phytotaxa 261, 201–217. doi: 10.11646/phytotaxa.261.3.1
Clarke, J. T., Lloyd, G. T., and Friedman, M. (2016). Little evidence for enhanced phenotypic evolution in early teleosts relative to their living fossil sister group. Proc. Natl. Acad. Sci. 113, 11531–11536. doi: 10.1073/pnas.1607237113
Coleman, G. A., Davín, A. A., Mahendrarajah, T. A., Szánthó, L. L., Spang, A., Hugenholtz, P., et al. (2021). A rooted phylogeny resolves early bacterial evolution. Science 372:eabe0511. doi: 10.1126/science.abe0511
Cote-L’Heureux, A., Maurer-Alcalá, X. X., and Katz, L. A. (2022). Old genes in new places: a taxon-rich analysis of interdomain lateral gene transfer events. PLoS Genet. 18:e1010239. doi: 10.1371/journal.pgen.1010239
Crane, P. R., Crane, P., and von Knorring, P. (2013). Ginkgo: the tree that time forgot. New Haven: Yale University Press.
Darwin, C. (1859). On the origin of species by means of natural selection, or the preservation of Favoured races in the struggle for life. 1st ed. London: John Murray, doi: 10.5962/bhl.title.82303
Darwin, C., to C. Lyell. (1860). Darwin correspondence project “letter no. 2925,” accessed on 5 February 2023, https://www.darwinproject.ac.uk/letter/?docId=letters/DCP-LETT-2925.xml.
Darwin, C., to de Saporta, G. (1877). Darwin correspondence project “letter no. 11287,” accessed on 12 November 2022, https://www.darwinproject.ac.uk/letter/?docId=letters/DCP-LETT-11287.xml.
Darwin, C., Prichard, J. C., Hodgkin, T., Yates, J., Gray, J. E., Taylor, R., et al. (1841). Queries respecting the human race, to be addressed to travellers and others. Drawn up by a Committee of the British Association for the advancement of science, appointed in 1839. Report of the British Association for the Advancement of Science 10, 447–458.
Diener, T. O. (2016). Viroids: “living fossils” of primordial RNAs? Biol. Direct 11:15. doi: 10.1186/s13062-016-0116-7
DiFrisco, J., and Wagner, G. P. (2022). Body plan identity: a mechanistic model. Evol. Biol. 49, 123–141. doi: 10.1007/s11692-022-09567-z
DiFrisco, J., Wagner, G. P., and Love, A. C. (2022). Reframing research on evolutionary novelty and co-option: character identity mechanisms versus deep homology. Semin. Cell Dev. Biol. doi: 10.1016/j.semcdb.2022.03.030
Edie, S. M., Smits, P. D., and Jablonski, D. (2017). Probabilistic models of species discovery and biodiversity comparisons. Proc. Natl. Acad. Sci. 114, 3666–3671. doi: 10.1073/pnas.1616355114
Eldredge, N., and Stanley, S. M. eds. (1984). Living Fossils. New York: Springer, doi: 10.1007/978-1-4613-8271-3
Flores, R., Gago-Zachert, S., Serra, P., Sanjuán, R., and Elena, S. F. (2014). Viroids: survivors from the RNA world? Annu. Rev. Microbiol. 68, 395–414. doi: 10.1146/annurev-micro-091313-103416
Giles, S., Xu, G.-H., Near, T. J., and Friedman, M. (2017). Early members of ‘living fossil’ lineage imply later origin of modern ray-finned fishes. Nature 549, 265–268. doi: 10.1038/nature23654
Graczyk, T. K. (2005). Is giardia a living fossil? Trends Parasitol. 21, 104–107. doi: 10.1016/j.pt.2005.01.002
Guan, R., Zhao, Y., Zhang, H., Fan, G., Liu, X., Zhou, W., et al. (2016). Draft genome of the living fossil Ginkgo biloba. GigaScience 5:49. doi: 10.1186/s13742-016-0154-1
Guijarro-Clarke, C., Holland, P. W. H., and Paps, J. (2020). Widespread patterns of gene loss in the evolution of the animal kingdom. Nat Ecol Evol 4, 519–523. doi: 10.1038/s41559-020-1129-2
Haueis, P. (2021). A generalized patchwork approach to scientific concepts. Br. J. Philos. Sci. doi: 10.1086/716179
Hay, J. M., Subramanian, S., Millar, C. D., Mohandesan, E., and Lambert, D. M. (2008). Rapid molecular evolution in a living fossil. Trends Genet. 24, 106–109. doi: 10.1016/j.tig.2007.12.002
Hirsch, P. M., and Levin, D. Z. (1999). Umbrella advocates versus validity police: a life-cycle model. Organ. Sci. 10, 199–212. doi: 10.1287/orsc.10.2.199
Hohmann, N., Wolf, E. M., Rigault, P., Zhou, W., Kiefer, M., Zhao, Y., et al. (2018). Ginkgo biloba’s footprint of dynamic Pleistocene history dates back only 390,000 years ago. BMC Genomics 19:299. doi: 10.1186/s12864-018-4673-2
Hooker, J. D., and Binney, E. W. (1855). VI. On the structure of certain limestone nodules enclosed in seams of bituminous coal, with a description of some trigonocarpons contained in them. Philos. Trans. R. Soc. Lond. 145, 149–156. doi: 10.1098/rstl.1855.0006
Hopwood, N. (2005). Visual standards and disciplinary change: normal plates, tables and stages in embryology. Hist. Sci. 43, 239–303. doi: 10.1177/007327530504300302
Huang, Y., Jacques, F. M., Su, T., Ferguson, D. K., Tang, H., Chen, W., et al. (2015). Distribution of Cenozoic plant relicts in China explained by drought in dry season. Sci. Rep. 5:14212. doi: 10.1038/srep14212
Hugenholtz, P., Chuvochina, M., Oren, A., Parks, D. H., and Soo, R. M. (2021). Prokaryotic taxonomy and nomenclature in the age of big sequence data. ISME J. 15, 1879–1892. doi: 10.1038/s41396-021-00941-x
Ikeno, S., and Hirase, S. (1897). Spermatozoids in gymnosperms. Annals of Botany 11, 344–345. doi: 10.1093/oxfordjournals.aob.a088657
Ingebretson, B. (2022). “Living fossils”: the politics of language preservation in Huangshan, China. Journal of Linguistic Anthropology 32, 116–138. doi: 10.1111/jola.12332
Jacobs, B. P., and Browner, W. S. (2000). Ginkgo biloba: a living fossil. Am. J. Med. 108, 341–342. doi: 10.1016/S0002-9343(00)00290-4
Johnson, R. N. (2020). Tuatara genome reveals diverse insights into a remarkable reptile. Nature 584, 351–352. doi: 10.1038/d41586-020-02063-4
Kampourakis, K., and Stern, F. (2018). Reconsidering the meaning of concepts in biology: why distinctions are so important. BioEssays 40:1800148. doi: 10.1002/bies.201800148
Keeling, P. J., and Burki, F. (2019). Progress towards the tree of eukaryotes. Curr. Biol. 29, R808–R817. doi: 10.1016/j.cub.2019.07.031
Kin, A., and Blażejowski, B. (2014). The horseshoe crab of the genus limulus: living fossil or stabilomorph? PLoS One 9:e108036. doi: 10.1371/journal.pone.0108036
Kitcher, P. (1999). Unification as a regulative ideal. Perspect. Sci. 7, 337–348. doi: 10.1162/posc.1999.7.3.337
Kuklick, H. (2006). Humanity in the chrysalis stage: indigenous Australisans in the anthropological imagination, 1899-1926. Br. J. Hist. Sci. 39, 535–568. doi: 10.1017/S0007087406008405
Laland, K. N., Uller, T., Feldman, M. W., Sterelny, K., Müller, G. B., Moczek, A., et al. (2015). The extended evolutionary synthesis: its structure, assumptions and predictions. Proc. R. Soc. B Biol. Sci. 282:20151019. doi: 10.1098/rspb.2015.1019
Latour, B. (2000). “On the partial existence of existing and nonexisting objects,” in Biographies of Scientific Objects. ed. L. Daston (Chicago, IL: University of Chicago Press), 247–269.
Laumer, C. E., Fernández, R., Lemer, S., Combosch, D., Kocot, K. M., Riesgo, A., et al. (2019). Revisiting metazoan phylogeny with genomic sampling of all phyla. Proc. R. Soc. B Biol. Sci. 286:20190831. doi: 10.1098/rspb.2019.0831
Leebens-Mack, J. H., Barker, M. S., Carpenter, E. J., Deyholos, M. K., Gitzendanner, M. A., Graham, S. W., et al. (2019). One thousand plant transcriptomes and the phylogenomics of green plants. Nature 574, 679–685. doi: 10.1038/s41586-019-1693-2
Leonelli, S. (2016). Data-centric biology: A philosophical study. Chicago: University of Chicago Press.
Li, Y., Shen, X.-X., Evans, B., Dunn, C. W., and Rokas, A. (2021). Rooting the animal tree of life. Mol. Biol. Evol. 38, 4322–4333. doi: 10.1093/molbev/msab170
Li, Y., Zhu, X., Wang, K., Zhu, L., Murray, M., and Zhou, F. (2022). The potential of Ginkgo biloba in the treatment of human diseases and the relationship to Nrf2–mediated antioxidant protection. J. Pharm. Pharmacol. 74, 1689–1699. doi: 10.1093/jpp/rgac036
Lidgard, S., and Hopkins, M. (2015). “Stasis” in Oxford bibliographies in evolutionary biology, vol. 1–31 doi: 10.1093/obo/9780199941728-0067
Lidgard, S., and Love, A. C. (2018). Rethinking living fossils. Bioscience 68, 760–770. doi: 10.1093/biosci/biy084
Lidgard, S., and Love, A. C. (2021). The living fossil concept: reply to Turner. Biol. Philos. 36:13. doi: 10.1007/s10539-021-09789-z
Liu, Y., Wang, S., Li, L., Yang, T., Dong, S., Wei, T., et al. (2022). The Cycas genome and the early evolution of seed plants. Nat. Plants 8, 389–401. doi: 10.1038/s41477-022-01129-7
Liu, H., Wang, X., Wang, G., Cui, P., Wu, S., Ai, C., et al. (2021). The nearly complete genome of Ginkgo biloba illuminates gymnosperm evolution. Nat. Plants 7, 748–756. doi: 10.1038/s41477-021-00933-x
Logan, B. W. (1961). Cryptozoon and associate stromatolites from the recent, Shark Bay, Western Australia. J. Geol. 69, 517–533. doi: 10.1086/626769
Love, A. C. (2008). Explaining evolutionary innovations and novelties: criteria of explanatory adequacy and epistemological prerequisites. Philos. Sci. 75, 874–886. doi: 10.1086/594531
Love, A. C. ed. (2015). Conceptual change in biology: Scientific and philosophical perspectives on evolution and development. Dordrecht: Springer Netherlands doi: 10.1007/978-94-017-9412-1
Luchetti, A., Forni, G., Martelossi, J., Savojardo, C., Martelli, P. L., Casadio, R., et al. (2021). Comparative genomics of tadpole shrimps (Crustacea, Branchiopoda, Notostraca): dynamic genome evolution against the backdrop of morphological stasis. Genomics 113, 4163–4172. doi: 10.1016/j.ygeno.2021.11.001
Lupas, A. N., and Alva, V. (2017). Ribosomal proteins as documents of the transition from unstructured (poly) peptides to folded proteins. J. Struct. Biol. 198, 74–81. doi: 10.1016/j.jsb.2017.04.007
Manias, C. (2015). The problematic construction of ‘Palaeolithic man’: the old stone age and the difficulties of the comparative method, 1859-1914. Studies in the History and Philosophy of Science Part C: Studies in the History and Philosophy of Biological and Biomedical Sciences 51, 32–43. doi: 10.1016/j.shpsc.2015.01.014
Martin, W. F. (2020). Older than genes: the acetyl CoA pathway and origins. Front. Microbiol. 11:817. doi: 10.3389/fmicb.2020.00817
Martinez-Gutierrez, C. A., and Aylward, F. O. (2021). Phylogenetic signal, congruence, and uncertainty across bacteria and archaea. Mol. Biol. Evol. 38, 5514–5527. doi: 10.1093/molbev/msab254
Mathers, T. C., Hammond, R. L., Jenner, R. A., Hänfling, B., and Gómez, A. (2013). Multiple global radiations in tadpole shrimps challenge the concept of 'living fossils'. PeerJ 1:e62. doi: 10.7717/peerj.62
McCune, B., and Grace, J. (2002). Analysis of ecological communities. Gleneden Beach, Or: Mjm Software Design.
McGregor, R. (1993). The doomed race: a scientific axiom of the late nineteenth century. Australian Journal of politics & History 39, 14–22. doi: 10.1111/j.1467-8497.1993.tb00047.x
McGuire, A. L., Gabriel, S., Tishkoff, S. A., Wonkam, A., Chakravarti, A., Furlong, E. E. M., et al. (2020). The road ahead in genetics and genomics. Nat. Rev. Genet. 21, 581–596. doi: 10.1038/s41576-020-0272-6
McMahan, P., and Evans, J. (2018). Ambiguity and engagement. Am. J. Sociol. 124, 860–912. doi: 10.1086/701298
Nagalingum, N. S., Marshall, C. R., Quental, T. B., Rai, H. S., Little, D. P., and Mathews, S. (2011). Recent synchronous radiation of a living fossil. Science 334, 796–799. doi: 10.2307/41351691
Naville, M., Chalopin, D., Casane, D., Laurenti, P., and Volff, J.-N. (2015). The coelacanth: can a “living fossil” have active transposable elements in its genome? Mob. Genet. Elem. 5, 55–59. doi: 10.1080/2159256X.2015.1052184
Neto, C. (2020). When imprecision is a good thing, or how imprecise concepts facilitate integration in biology. Biol. Philos. 35:58. doi: 10.1007/s10539-020-09774-y
Peck, J. E. (2016). Multivariate analysis for ecologists: Step-by-step. Gleneden Beach, OR: MjM Software Design.
Radin, J. (2017). Life on ice: A history of new uses for cold blood. Chicago: University of Chicago Press, doi: 10.7208/chicago/9780226448244.001.0001
Ran, J.-H., Shen, T.-T., Wang, M.-M., and Wang, X.-Q. (2018). Phylogenomics resolves the deep phylogeny of seed plants and indicates partial convergent or homoplastic evolution between Gnetales and angiosperms. Proc. R. Soc. B Biol. Sci. 285:20181012. doi: 10.1098/rspb.2018.1012
Redmond, A. K., and McLysaght, A. (2021). Evidence for sponges as sister to all other animals from partitioned phylogenomics with mixture models and recoding. Nat. Commun. 12:1783. doi: 10.1038/s41467-021-22074-7
Rieppel, O. (2016). Phylogenetic systematics: Haeckel to Hennig. Boca Raton: CRC Press doi: 10.1201/b21805
Rosselló-Mora, R. (2005). Updating prokaryotic taxonomy. J. Bacteriol. 187, 6255–6257. doi: 10.1128/JB.187.18.6255-6257.2005
Sapp, J. (2005). The prokaryote-eukaryote dichotomy: meanings and mythology. Microbiol. Mol. Biol. Rev. 69, 292–305. doi: 10.1128/MMBR.69.2.292-305.2005
Schoelmerich, M. C., and Müller, V. (2020). Energy-converting hydrogenases: the link between H2 metabolism and energy conservation. Cell. Mol. Life Sci. 77, 1461–1481. doi: 10.1007/s00018-019-03329-5
Schopf, J. W. (1994). Disparate rates, differing fates: tempo and mode of evolution changed from the Precambrian to the Phanerozoic. Proc. Natl. Acad. Sci. U. S. A. 91, 6735–6742. doi: 10.1073/pnas.91.15.6735
Sepkoski, D., and Ruse, M. eds. (2015). The Paleobiological revolution: Essays on the growth of modern paleontology. Chicago: University of Chicago Press.
Simões, T. R., Kinney-Broderick, G., and Pierce, S. E. (2022). An exceptionally preserved Sphenodon-like sphenodontian reveals deep time conservation of the tuatara skeleton and ontogeny. Commun Biol 5, 195–119. doi: 10.1038/s42003-022-03144-y
Simpson, T. (2018). “Historicizing humans in colonial India” in Historicizing humans: Deep time, evolution, and race in nineteenth-century British sciences. ed. E. Sera-Shriar (Pittsburgh: University of Pittsburgh Press), 113–137.
Singh, S. K., Srivastav, S., Castellani, R. J., Plascencia-Villa, G., and Perry, G. (2019). Neuroprotective and antioxidant effect of Ginkgo biloba extract against ad and other neurological disorders. Neurotherapeutics 16, 666–674. doi: 10.1007/s13311-019-00767-8
Small, I. (2013). Mitochondrial genomes as living 'fossils'. BMC Biol. 11:30. doi: 10.1186/1741-7007-11-30
Solomon, M., and McMullen, W. J. (1991). Places in the heart: the rhetorical force of an open text. West. J. Speech Commun. 55, 339–353. doi: 10.1080/10570319109374392
Sommer, M. (2015). Population-genetic trees, maps, and narratives of the great human diasporas. Hist. Hum. Sci. 28, 108–145. doi: 10.1177/0952695115573032
Spencer, S. B., and Gillen, F. J. (1927). The Arunta: A study of a stone age people. London: Macmillan.
Steinthorsdottir, M., Jardine, P. E., Lomax, B. H., and Sallstedt, T. (2022). Key traits of living fossil Ginkgo biloba are highly variable but not influenced by climate – implications for palaeo-pCO2 reconstructions and climate sensitivity. Glob. Planet. Chang. 211:103786. doi: 10.1016/j.gloplacha.2022.103786
Sterner, B. (2022). Explaining ambiguity in scientific language. Synthese 200:354. doi: 10.1007/s11229-022-03792-x
Sterner, B., and Lidgard, S. (2018). Moving past the systematics wars. J. Hist. Biol. 51, 31–67. doi: 10.1007/s10739-017-9471-1
Stork, N. E. (2018). How many species of insects and other terrestrial arthropods are there on Earth? Annu. Rev. Entomol. 63, 31–45. doi: 10.1146/annurev-ento-020117-043348
Strassert, J. F. H., Jamy, M., Mylnikov, A. P., Tikhonenkov, D. V., and Burki, F. (2019). New phylogenomic analysis of the enigmatic phylum Telonemia further resolves the eukaryote tree of life. Mol. Biol. Evol. 36, 757–765. doi: 10.1093/molbev/msz012
Stull, G. W., Qu, X.-J., Parins-Fukuchi, C., Yang, Y.-Y., Yang, J.-B., Yang, Z.-Y., et al. (2021). Gene duplications and phylogenomic conflict underlie major pulses of phenotypic evolution in gymnosperms. Nat. Plants 7, 1015–1025. doi: 10.1038/s41477-021-00964-4
Suárez-Díaz, E., and Anaya-Muñoz, V. H. (2008). History, objectivity, and the construction of molecular phylogenies. Studies in History and Philosophy of Science Part C: Studies in History and Philosophy of Biological and Biomedical Sciences 39, 451–468. doi: 10.1016/j.shpsc.2008.09.002
Subramanian, S., Hay, J. M., Mohandesan, E., Millar, C. D., and Lambert, D. M. (2009). Molecular and morphological evolution in tuatara are decoupled. Trends Genet. 25, 16–18. doi: 10.1016/j.tig.2008.11.001
Tahon, G., Geesink, P., and Ettema, T. J. G. (2021). Expanding archaeal diversity and phylogeny: past, present, and future. Annu. Rev. Microbiol. 75, 359–381. doi: 10.1146/annurev-micro-040921-050212
Thenius, E. (1973). Fossils and the life of the past. New York: Springer, doi: 10.1007/978-1-4615-7010-3
Thenius, E. (2000). Lebende Fossilien: Oldtimer der Tier- und Pflanzenwelt Zeugen der Vorzeit. Munchen: Pfeil.
Turner, D. D. (2019). In defense of living fossils. Biol. Philos. 34:23. doi: 10.1007/s10539-019-9678-y
Turner, D., and Han, J. (2023). Living fossils and conservation values. Front. Earth Sci. 11. doi: 10.3389/feart.2023.1086066
van Steenis, G. G. J. (1953). Results of the Archbold expeditions Papuan Nothofagus. Journal of the Arnold Arboretum 34, 301–374. doi: 10.5962/bhl.part.27154
Vanschoenwinkel, B., Pinceel, T., Vanhove, M. P. M., Denis, C., Jocque, M., Timms, B. V., et al. (2012). Toward a global phylogeny of the “living fossil” crustacean order of the Notostraca. PLoS One 7:e34998. doi: 10.1371/journal.pone.0034998
Vargas, P., Jiménez-Mejías, P., and Fernández-Mazuecos, M. (2020). ‘Endangered living fossils’ (ELFs): long-term survivors through periods of dramatic climate change. Environ. Exp. Bot. 170:103892. doi: 10.1016/j.envexpbot.2019.103892
Vasconcelos, C., Warthmann, R., McKenzie, J. A., Visscher, P. T., Bittermann, A. G., and van Lith, Y. (2006). Lithifying microbial mats in Lagoa Vermelha, Brazil: modern Precambrian relics? Sediment. Geol. 185, 175–183. doi: 10.1016/j.sedgeo.2005.12.022
Vaux, F., Morgan-Richards, M., Daly, E. E., and Trewick, S. A. (2019). Tuatara and a new morphometric dataset for Rhynchocephalia: comments on Herrera-Flores, et al. Palaeontology 62, 321–334. doi: 10.1111/pala.12402
Wall, D., and Dale, B. (1966). “Living fossils” in Western Atlantic plankton. Nature 211, 1025–1026. doi: 10.1038/2111025a0
Wang, X., Zhou, B.-W., Yang, M. A., Yin, T.-T., Chen, F.-L., Ommeh, S. C., et al. (2019). Canine transmissible venereal tumor genome reveals ancient introgression from coyotes to pre-contact dogs in North America. Cell Res. 29, 592–595. doi: 10.1038/s41422-019-0183-2
Ward, P. D. (1992). On Methuselah’s trail: Living fossils and the great extinctions. New York: W.H. Freeman.
Waters, C. K. (2014). “Shifting attention from theory to practice in philosophy of biology,” in New directions in the philosophy of science The Philosophy of Science in a European Perspective., eds. M. C. Galavotti, D. Dieks, W. J. Gonzalez, S. Hartmann, T. Uebel, and M. Weber (Cham: Springer International Publishing), 121–139. doi: 10.1007/978-3-319-04382-1_9
Watkins, A. (2021). The epistemic value of the living fossils concept. Philos. Sci. 88, 1221–1233. doi: 10.1086/714875
Wieland, G. R. (1924). “Fossil plants as evidence for resistance to environment,” in Organic adaptation to environment. ed. G. E. Nichols (New Haven: Yale University Press), 149–185.
Wilkinson, B. H., Ivany, L. C., and Drummond, C. N. (2021). Estimating vertebrate biodiversity using the tempo of taxonomy – a view from Hubbert’s peak. Biol. J. Linn. Soc. 134, 402–422. doi: 10.1093/biolinnean/blab080
Williams, T. A., and Embley, T. M. (2015). Changing ideas about eukaryotic origins. Philosophical Transactions of the Royal Society B: Biological Sciences 370:20140318. doi: 10.1098/rstb.2014.0318
Woese, C. R. (1998). Default taxonomy: Ernst Mayr’s view of the microbial world. Proc. Natl. Acad. Sci. U. S. A. 95, 11043–11046. doi: 10.1073/pnas.95.19.11043
Wolfe, C. T. (2014). The organism as ontological go-between: hybridity, boundaries and degrees of reality in its conceptual history. Studies in History and Philosophy of Science Part C: Studies in History and Philosophy of Biological and Biomedical Sciences 48, 151–161. doi: 10.1016/j.shpsc.2014.06.006
Wood, D., Besnard, G., Beerling, D. J., Osborne, C. P., and Christin, P.-A. (2020). Phylogenomics indicates the “living fossil” Isoetes diversified in the Cenozoic. PLoS One 15:e0227525. doi: 10.1371/journal.pone.0227525
Yang, T., Sahu, S. K., Yang, L., Liu, Y., Mu, W., Liu, X., et al. (2022). Comparative analyses of 3,654 plastid genomes unravel insights into evolutionary dynamics and phylogenetic discordance of green plants. Front. Plant Sci. 13. Available at: https://www.frontiersin.org/article/10.3389/fpls.2022.808156.
Zhang, Z., Ma, X., Liu, Y., Yang, L., Shi, X., Wang, H., et al. (2022). Origin and evolution of green plants in the light of key evolutionary events. J. Integr. Plant Biol. 64, 516–535. doi: 10.1111/jipb.13224
Zhao, Y.-P., Fan, G., Yin, P.-P., Sun, S., Li, N., Hong, X., et al. (2019). Resequencing 545 ginkgo genomes across the world reveals the evolutionary history of the living fossil. Nat. Commun. 10:4201. doi: 10.1038/s41467-019-12133-5
Keywords: character evolution, concepts, living fossil, research agenda, scientific racism
Citation: Lidgard S and Kitchen E (2023) Revealing the rise of a living fossil menagerie. Front. Ecol. Evol. 11:1112764. doi: 10.3389/fevo.2023.1112764
Edited by:
Peter David Roopnarine, California Academy of Sciences, United StatesReviewed by:
Carl Simpson, University of Colorado Boulder, United StatesNoel Heim, Tufts University, United States
Copyright © 2023 Lidgard and Kitchen. This is an open-access article distributed under the terms of the Creative Commons Attribution License (CC BY). The use, distribution or reproduction in other forums is permitted, provided the original author(s) and the copyright owner(s) are credited and that the original publication in this journal is cited, in accordance with accepted academic practice. No use, distribution or reproduction is permitted which does not comply with these terms.
*Correspondence: Scott Lidgard, slidgard@fieldmuseum.org