- 1Evolutionary Biology, Institute for Biology, Freie Universität Berlin, Berlin, Germany
- 2Research Group Insect Gut Microbiology and Symbiosis, Max Planck Institute for Terrestrial Microbiology, Marburg, Germany
- 3Faculty of Tropical AgriSciences, Czech University of Life Sciences, Prague, Czechia
- 4Laboratory of Experimental and Comparative Ethology, UR 4443, University Sorbonne Paris Nord, Villetaneuse, France
- 5Research Group Chemistry of Social Insects, Institute of Organic Chemistry and Biochemistry, Czech Academy of Sciences, Prague, Czechia
Lower termites harbor diverse consortia of symbiotic gut flagellates. Despite numerous evidence for co-cladogenesis, the evolutionary history of these associations remains unclear. Here, we present Retractinymphidae fam. nov., a monogeneric lineage of Trichonymphida from Serritermitidae. Although Retractinympha glossotermitis gen. nov. sp. nov. morphologically resembles members of the genus Pseudotrichonympha, phylogenetic analysis identified it as sister group of the Teranymphidae. We compared morphology and ultrastructure of R. glossotermitis to that of Pseudotrichonympha and other Teranymphidae, including the so-far undescribed Pseudotrichonympha solitaria sp. nov. from Termitogeton planus (Rhinotermitidae). Like all Teranymphidae, R. glossotermitis is a large, elongated flagellate with a bilaterally symmetric rostrum, an anterior, flagella-free operculum, and an internal rostral tube. However, it is readily distinguished by the length of its rostral flagella, which never exceeds that of the postrostral flagella, and its retractable anterior end. Inclusion of the hitherto unstudied Stylotermes halumicus (Stylotermitidae) in our survey of trichonymphid flagellates in Neoisoptera confirmed that the combined presence of Heliconympha and Retractinympha and absence of Pseudotrichonympha is unique to Serritermitidae. The close phylogenetic relatedness of Heliconympha in Serritermitidae to the spirotrichosomid flagellates in Stolotermitidae provides strong support for their acquisition by horizontal transmission.
Introduction
Symbiotic flagellates play an essential role in the digestion of lignocellulose in the hindguts of lower termites and their phylogenetic sister group, wood-feeding cockroaches of the genus Cryptocercus (Cryptocercidae; Hongoh, 2011; Brune, 2014). Each host family harbors a unique assemblage of flagellate symbionts that is specific for the respective host and typically similar in composition among members of the same termite family [see reviews by Inoue et al. (2000), Kitade (2004), and Ohkuma and Brune (2011)]. This led to the hypothesis that flagellates were present already in the common ancestor of Xylophagoidea [i.e., termites (Isoptera) and Cryptocercidae; Engel, 2011] and have been passed on from parent to offspring (Lo and Eggleton, 2011). This vertical transmission is driven by proctodeal trophallaxis, a behavioral trait that is a synapomorphy of Xylophagoidea (Nalepa, 1991, 2015) and has favored co-cladogenesis between different flagellate lineages and their termite hosts (e.g., Noda et al., 2007, 2018; Ikeda-Ohtsubo and Brune, 2009; Ohkuma et al., 2009; Jasso-Selles et al., 2017; Radek et al., 2018). For termite classification and taxonomic details, see Engel et al. (2009), Krishna et al. (2013), and Wang et al. (2022); their gut flagellates have been covered by Čepička et al. (2016) and Hampl (2016).
While Cryptocercidae and the basal termite families (Mastotermitidae, Teletisoptera, and Kalotermitidae) harbor numerous (typically 10–20) flagellate species from the phyla Parabasalia and Preaxostyla (order Oxymonadida), the diversity of the flagellate communities in the crown families of termites (Neoisoptera) is substantially reduced (e.g., Yamin, 1979; Kitade and Matsumoto, 1993; Inoue et al., 2000; Brugerolle and Bordereau, 2004; Kitade et al., 2012). Termitidae have lost all flagellates, and most Rhinotermitidae have retained only a few lineages of parabasalids (Kitade and Matsumoto, 1998; Jasso-Selles et al., 2017), with the genus Reticulitermes forming a notable exception (see below). A study of the flagellate genus Pseudotrichonympha, a large cellulolytic member of the Teranymphidae (order Trichonymphida), documented co-cladogenesis with Rhinotermitidae, without any obvious host switches (Noda et al., 2007). However, many members of the genus Pseudotrichonympha have been characterized only on a morphological basis, and only few representatives with SSU rRNA gene sequences have been formally described (Noda et al., 2007; Saldarriaga et al., 2011; Jasso-Selles et al., 2017).
The evolutionary history of Trichonymphida in Neoisoptera has been obscured by unresolved relationships among particular host lineages and a lack of information on their flagellate microbiota. However, comparative analyses of mitochondrial genome sequences have provided increasingly robust host phylogenies that have improved our understanding of termite evolution and diversification (Chouvenc et al., 2021). Rhinotermitidae have been shown to be paraphyletic to both Serritermitidae and Termitidae (Bourguignon et al., 2015; Bucek et al., 2019), with Stylotermitidae in a basal position (Wu et al., 2018).
It is well documented that the flagellate assemblages in the genus Reticulitermes (Rhinotermitidae) differ fundamentally from those of other rhinotermitids but resemble those of the genus Hodotermopsis (Hodotermopsidae; Yamin, 1979; Kitade and Matsumoto, 1998). This scenario has been explained by an ancestral horizontal transfer of flagellates (also referred to as “transfaunation”) from a hodotermopsid to a rhinotermitid host (Kitade, 2004; Lo and Eggleton, 2011) – a widely accepted hypothesis that is backed by the close relatedness of the corresponding taxa in molecular phylogenies (Ikeda-Ohtsubo and Brune, 2009; Ohkuma et al., 2009; James et al., 2013; Gile et al., 2018, 2021).
Likewise, also the flagellate communities of Serritermitidae differ fundamentally from those of their rhinotermitid relatives (Radek et al., 2018). Based on morphological and phylogenetic evidence, it has been proposed that the flagellates of the genus Heliconympha, which are exclusively present in Serritermitidae, were acquired by horizontal transfer, presumably from a stolotermitid host (Radek et al., 2018). Since the first molecular data on Spirotrichosomidae from Stolotermes (Izawa et al., 2017) became available only after the study on Heliconympha had been submitted, a test of this hypothesis is still lacking. Also, a description of the second, Pseudotrichonympha-like lineage of flagellates from Serritermitidae, which show a superficial resemblance to members of the genus Pseudotrichonympha but are only distantly related to Teranymphidae (Radek et al., 2018), is still pending. Moreover, there is absolutely no information on the composition of the flagellate assemblages in the Stylotermitidae, the most basal of the extant neoisopteran families (Wu et al., 2018; Barden and Engel, 2020).
Here, we characterize the Pseudotrichonympha-like flagellate from Glossotermes oculatus and Serritermes serrifer (Serritermitidae) and propose a new genus and family for this lineage. Moreover, we describe a new Pseudotrichonympha species from the termite genus Termitogeton, a basal lineage of Rhinotermitidae, and investigate the diversity of flagellates in Stylotermes halumicus and their relationship to the flagellates of other Neoisoptera.
Materials and methods
Termites
Glossotermes oculatus was collected in French Guiana in 2013 and live specimens were processed as described (Radek et al., 2018). Termitogeton planus was collected in West Papua, Indonesia, in 2011 (Dolejšová et al., 2014); specimens were preserved in 96% ethanol. Species identification was verified by sequencing the mitochondrial cytochrome c oxidase subunit II (COII) genes (Inward et al., 2007); the GenBank accession numbers are KY750729 and MN528021. Stylotermes halumicus was collected in China in 2015 (collection ID CHI15-156); specimens were preserved in RNAlater (Invitrogen). Their COII gene sequence was identical to that encoded in the mitochondrial genome previously reported for the same material (KY449049; Wu et al., 2018).
Light microscopy
The hindgut paunch of worker termites was ruptured with fine-tipped forceps, and the content was released in a drop of 0.6% NaCl (for the direct observation of living flagellates) or fixed in a drop of 2.5% glutaraldehyde in 50 mM phosphate buffer (pH 7.0). For the visualization of nucleus, flagella, basal bodies, axostyle, and dictyosomes (parabasal bodies), fixed cells were stained with protargol (silver proteinate) according to procedure A of Foissner (2014). Nuclei were visualized also by fluorescence microscopy after immersing the samples in a solution of 2 ng/ml 4,6-diamidino-2-phenylindole (DAPI; Serva, Heidelberg, Germany) for 10 min.
The slide mounts were observed with an Axiophot light microscope (Zeiss) equipped with differential interference contrast and epifluorescence illumination. Images were recorded with a MicroLive digital camera (Linkenheld, Oppenau, Germany).1 All measurements were taken from protargol-stained slides.
Electron microscopy
For scanning electron microscopy (SEM), hindgut content was fixed in 2.5% glutaraldehyde. Samples were postfixed in 1% OsO4, critical point-dried, sputtered with gold, and inspected with an environmental scanning electron microscope (FEI Quanta 200). For details, see Radek et al. (2018).
For transmission electron microscopy (TEM), the same fixation procedure was used, but ruthenium red was added to both fixation solutions to enhance the contrast of the glycocalyx. Samples were embedded in Spurr’s resin. Ultrathin sections were stained with saturated uranyl acetate and lead citrate and inspected with a Philips EM 208 electron microscope. For details, see Radek et al. (2018).
SSU rRNA gene sequencing and phylogenetic analysis
Termite hindguts were homogenized, DNA was extracted, and SSU rRNA genes were amplified, cloned, and sequenced following the procedure described by Radek et al. (2019), with the following exceptions: For the sample of Stylotermes halumicus, we used the Parabasalia-specific primer pair Para19-36f (5′-CTG CCA AGG AAG YAY AC-3′) and Fla1484-1501r (5′-GTT ACG ACT TCT CCT TCC-3′) at an association temperature (Ta) of 52°C. For the sample of Termitogeton planus, which was strongly degraded, we amplified the SSU rRNA gene of Pseudotrichonympha sp. by nested PCR, using the flagellate-specific primer pair EUK19f (5′-AYY TGG TTG ATY CTG CCA-3′) and EUK1772r (5′-CBG CAG GTT CAC CTA C-3′; Ohkuma et al., 1998) at a Ta of 50°C for the first PCR, and the Pseudotrichonympha-specific primer pair PsTrn41f (5′-GGT CAT AGA TTA AGC CAT GC-3′) and Fla1484r (5′-CTT GTT ACG ACT TCT CCT TCC-3′, Radek et al., 2019) at a Ta of 59°C for the second PCR. Amplified DNA was purified and sequenced directly with the same primers. To confirm the absence of other parabasalids, we also used the Parabasalia-specific primer pair Para936f (5′-GAA TTG ACG GAA GGG CAC A-3′) and Para1201r (5′-GCA TCT RAA GGR CAT CAC G-3′) at a Ta of 57°C for the second PCR. The sequences were deposited at GenBank under accession numbers MT936308–26 and MN523346.
New sequences and parabasalid sequences from public databases that were not yet included in the Silva SSURef database (Quast et al., 2013; version 106)2 were imported using the ARB software package (Ludwig et al., 2004; version 7.0) and aligned with the reference sequences using the Silva Incremental Aligner (SINA version 1.2.11; Pruesse et al., 2012). The alignment was manually refined considering the secondary structure of the rRNA, and ambiguously aligned positions were removed. The final dataset consisted of 1,502 sites, of which 595 were invariant and 752 were parsimony-informative sites.
Phylogenetic trees were reconstructed by maximum-likelihood analysis with IQ-TREE 1.6.12 (Nguyen et al., 2015) using the best-fit evolutionary model (GTR + F + I + G4) suggested by ModelFinder (Kalyaanamoorthy et al., 2017) under the Bayesian information criterion. Tree topology was tested with PhyML v3.0 (Guindon et al., 2010) and by Bayesian inference analysis (MrBayes, Ronquist et al., 2012; 4 chains, 1,000,000 generations, burn-in 0.25). Node support was assessed with the Shimodaira-Hasegawa approximate likelihood ratio test (SH-aLRT, Guindon et al., 2010) and by ultrafast bootstrap analysis (UFBoot, 1,000 replicates, Hoang et al., 2018).
Results
Morphology of the Pseudotrichonympha-like flagellate from Glossotermes oculatus
The largest flagellate in G. oculatus with its straight bands of flagella is easily distinguished from the medium-sized cells of Heliconympha glossotermitis with their spiraled bands of flagella and from the small Hexamastix-like cells with their bundle of six flagella (Radek et al., 2018). It resembles members of the genus Pseudotrichonympha in its fusiform shape, the presence of a rostrum at the anterior cell pole, and an almost complete flagellation of the cell surface (Figure 1A). Protargol-stained cells measured 107–260 (mean 163) μm in length and 60–92 (mean 70) μm in width (n = 40; Figures 1B,C). The pointed rostrum is capped by a hemispherical operculum (diameter ca. 8.5 μm) and surrounded by ca. 60 to 75 flagella (Figures 1A,D,F–H). The border of the operculum is circular (Figures 1G,H). In fixed preparations, and also during live observations, the anterior cell pole was frequently retracted, creating a cup-like invagination of varying depth that completely engulfs the rostrum like a high collar (Figures 1B,C). In such cases, we added the estimated length of the retracted anterior pole to the total cell length to achieve consistent length measurements. The posterior cell pole is moderately pointed in extended cells (Figures 1A,B) and more rounded in strongly contracted cells (Figure 1C).
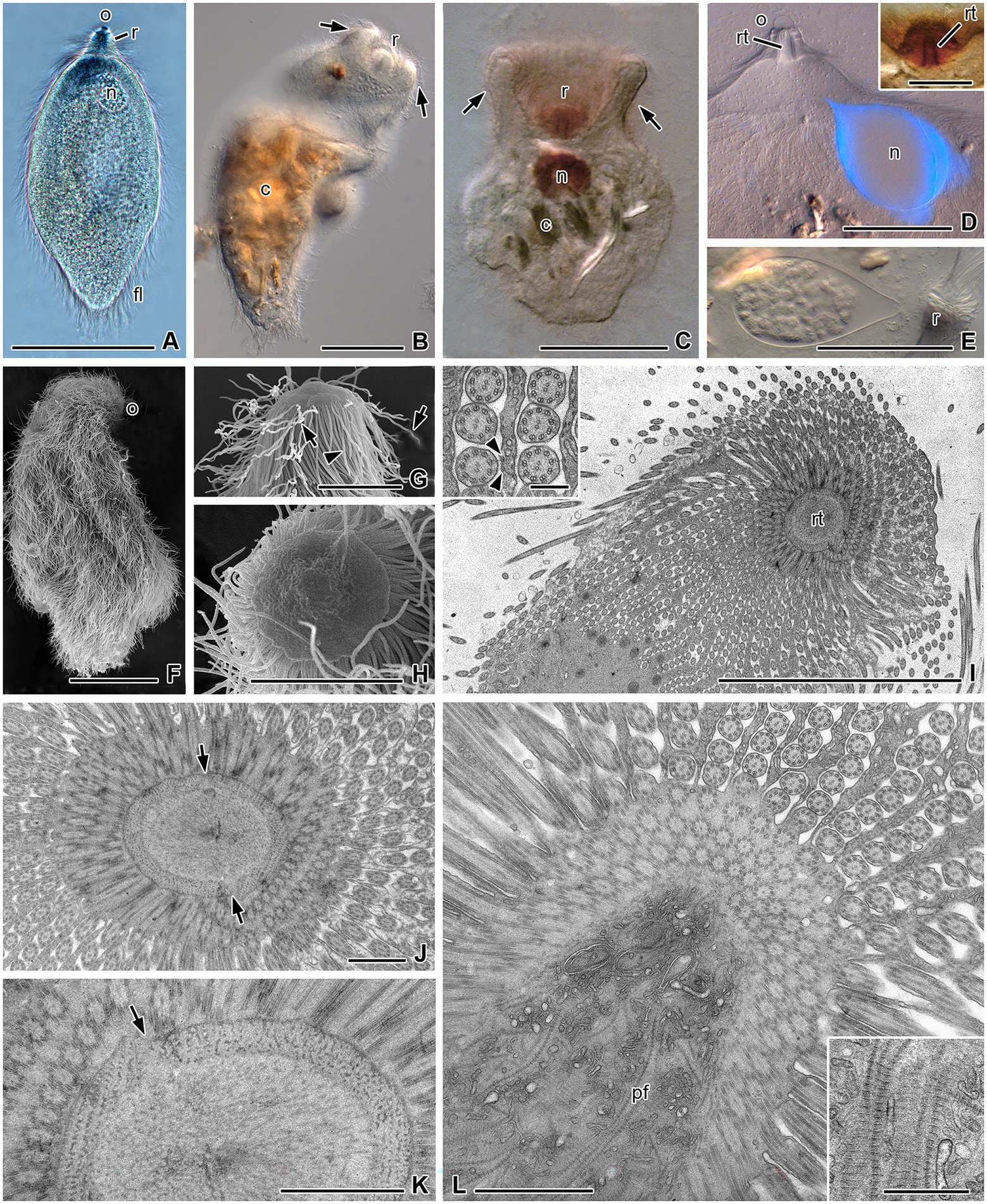
Figure 1. Morphology of Retractinympha glossotermitis and ultrastructural details of its anterior cell pole. (A) Lemon-shaped living cell with a cap-like operculum (o) at the tip of the rostrum (r), a granular nucleus (n), and complete cover with flagella (fl). (B,C) Cells with rostrum retracted in a bowl-like indentation and lignocellulose particles (c). (D) Anterior half of a cell with DAPI-stained, pear-shaped nucleus, hemispherical operculum, and rostral tube (rt). Inset: rostrum with rostral tube. (E) Pear-shaped nucleus with condensed chromosomes and anterior hyaline region. (F) A cell completely covered with flagella, except at the operculum. (G) Dome-shaped operculum, lateral view; helical spirochetes (arrows) are attached between the short flagella (arrowhead). (H) Circular operculum, top view. (I) Oblique section through anterior cell pole with rostral tube and longitudinal rows of flagella flanked by thin cytoplasmic ridges. Inset: Flagella attached to ridges by electron-dense cell contacts (arrowheads). (J,K) Cross-sections through anterior part of rostrum; rostral tube bordered by a dense layer of parabasal filaments and loosely arranged filaments inside tube; arrows indicate disjuncture of rostral tube wall. (L) Oblique section through posterior part of rostrum; interior filled with cross-striated parabasal filaments and vesicles. Inset: Parabasal filaments in higher magnification. (A–E) Light microscopy. (A) Bright field, (B–E) differential interference contrast, (D) DAPI staining, and (inset D) protargol staining. (F–H) Scanning electron microscopy. (I–L) Transmission electron microscopy. Scale bars (A–F) 50 μm, (G–I) 10 μm, (inset I) 0.2 μm, (J–L) 1 μm.
Numerous flagella cover the entire cell surface except the operculum (Figure 1A–D,F–H). They are arranged in longitudinal rows. The rostral flagella adjacent to the operculum (series-1 flagella) are short (5.4–8.7 μm, mean 7.3 μm; n = 5; Figure 1G), while the flagella at the base of the rostrum (series-2 flagella) have the same length as the postrostral flagella (22–28 μm, mean 25 μm; n = 12). Rostral and postrostral flagella move independently of each other. Prokaryotic cells with a helical morphology are attached between the rostral flagella (Figure 1G). Protargol-stained cells show a rostral tube with darkly contrasted borders (Figures 1C,D inset), which measures 12–15.5 μm in length (mean 14.3 μm) and 4.6–6.8 μm in outer diameter (mean 5 μm, n = 10).
The single, drop-shaped nucleus is located in the anterior part of the post-rostral region, typically in a lateral position (Figure 1A,C–E). Its length is 26.5–66.4 μm (mean 45.6 μm) and the width is 14.5–42.1 μm (mean 25.6 μm; n = 9). In expanded cells, the tip of the nucleus points toward the rostrum (Figures 1D,E), but in contracted cells, it is turned sideways (Figure 1C). The obtuse end of the nucleus contains the condensed chromosomes, whereas the pointed end contains hyaline nucleoplasm (Figure 1E). DAPI, a DNA-specific fluorescent dye, strongly stained only the periphery of the nucleus but not the chromatin at the center (Figure 1D). The cytoplasm contains numerous ingested wood fragments that obscured other cell organelles (Figures 1B,C). Axostyles and parabasal bodies were not visible by light microscopy.
Ultrastructure of the Pseudotrichonympha-like flagellate from Glossotermes oculatus
Ultra-thin sections revealed more details of the Pseudotrichonympha-like cells (Figures 1I–L, 2). Oblique transverse sections of the anterior cell pole show the compact and regular inner structure of the rostrum, and the longitudinal rows of rostral and post-rostral flagella (Figures 1I–K). The outer cytoplasmic layer of the rostrum contains about 35 to 45 longitudinal rows of basal bodies that surround the rostral tube. Rostral basal bodies measure roughly 1 μm in length (950 nm; n = 6). The cross-sectioned rostral tube is bounded by a dense ring of regularly spaced parabasal filaments and contains loosely arranged filaments in its center (Figures 1I–K). The ring of parabasal filaments seems to consist of two symmetric plates that face each other (Figures 1J,K). The rows of post-rostral flagella arise from long longitudinal grooves that are separated by ectoplasmic ridges of about 140 nm (n = 10) thickness (Figure 1 inset I, L). The flagella are attached to the ridges by electron-dense material supporting the membranes (Figure 1I inset, Figures 2A,C). At their very proximal end, the flagella sit singly in little pits. Here, electron-dense structures arising at the peripheral side of the microtubular duplets pass the gap between the membrane of the flagellum and the membrane of the pit and end in electron-dense bodies underneath the pit membrane (Figure 2A, arrows). The number of these contact bridges is variable. Apart from the electron dense material of the contact sites, the pits are surrounded by a layer of electron lucent cytoplasm. The basal bodies of the post-rostral flagella are about half as long (540 nm; n = 7) as those of the rostral flagella (Figures 2C,D). An electron-dense plate indicates the transition point from basal bodies to flagella (Figure 2D). In rare cases, a connected cross-striated parabasal filament was observed at the basis of the post-rostral basal bodies (Figure 2D).
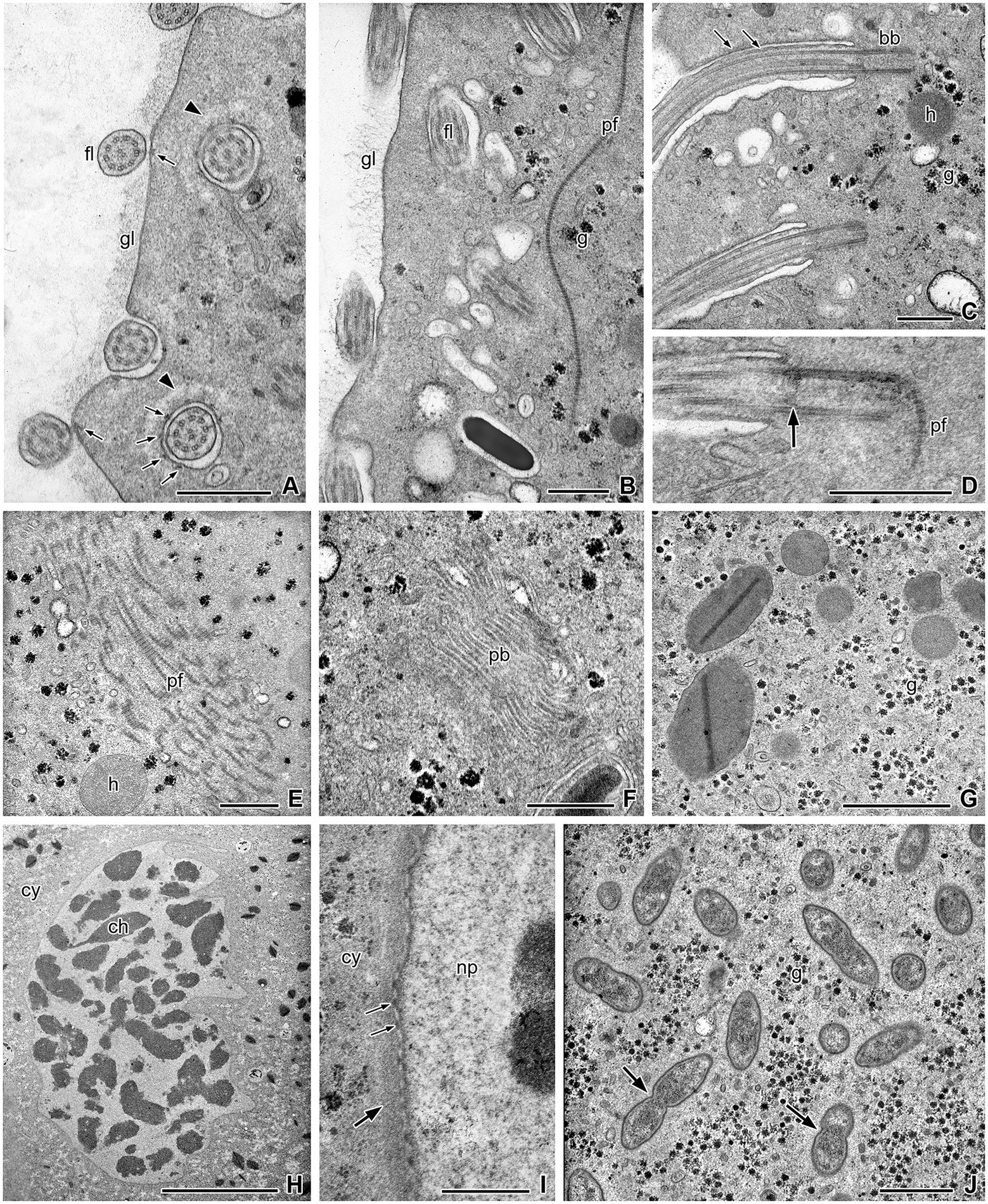
Figure 2. Ultrastructural details of the cell body of Retractinympha glossotermitis. (A) Body surface covered by thick layer of glycocalyx (gl); cross-sectioned flagella (fl) with contact sites to plasma membrane (thin arrows), and basal part of flagella in narrow depressions surrounded by bright zones of cytoplasm (arrowheads). (B) A cross-striated parabasal filament (pf) close to the flagellar region; glycogen (g). (C) Longitudinal sections of flagella and basal bodies (bb), arrows point to attachment sites; hydrogenosome (h). (D) Longitudinal section of basal body with transition plate to flagellum (arrow) and associated parabasal filament. (E) Strand of parabasal filaments. (F) Parabasal body (pb). (G) Small rounded hydrogenosomes and larger hydrogenosomes with interior stained plate. (H) Nucleus with condensed chromatin (ch); cytoplasm (cy). (I) Nuclear envelope with surrounding layer of dense cytoplasm (thick arrow) and nuclear pores (thin arrows); nucleoplasm (np). (J) Endobiotic bacteria (arrows) in direct contact to the cytoplasm. Transmission electron microscopy. Scale bars (A–G,I) 0.5 μm, (H) 10 μm, (J) 1 μm.
The parabasal filaments that arise in the rostral tube continue as a bundle of cross-striated bands with numerous intervening vesicular structures into the foremost part of the post-rostral region (Figure 1L and inset). Another bundle of filaments was observed deeper in the cell body (Figure 2E). Thin single parabasal filaments run parallel to the plasma membrane close to the basal bodies (Figure 2E). Parabasal bodies (dictyosomes) are about 1.2 μm in diameter and possess about 14 to 17 layers of cisterns and peripheral vesicles (n = 4). They occur close to the cell surface but are not regularly associated with the rows of basal bodies (Figure 2F). Connections between parabasal filaments and the cisterns of the dictyosomes were not observed.
The cell surface is covered by a conspicuous glycocalyx (thickness ca. 12 nm; n = 10) that contains fibrils and granules and is reduced in the regions where flagella adhere to the surface (Figures 2A,B). The cytoplasm of the cells consists of an outer, finely granular ectoplasm, in which the flagella are anchored, and an endoplasm that contains the nucleus, numerous hydrogenosomes, glycogen granules, vesicles, endobacteria, and food particles (Figures 2A–J). The nucleus has an elongated but irregular form with folds and contains electron-dense chromatin aggregations in an electron-light nucleoplasm (Figure 2H). The nuclear envelope possesses numerous pores and is surrounded by an electron-dense cytoplasmic layer of about 100 nm thickness (Figure 2I). Hydrogenosomes appear in two different morphological variations. They are either small and rounded, with a homogenous interior, or larger and elongated, with a densely stained interior plate (Figure 2G). Many, but not all cells contain numerous endobacteria that are distributed in the cytoplasm (Figure 2J). The bacteria have tapered ends and are not enclosed in vacuoles; some are in the process of cell division.
Morphology of the Pseudotrichonympha sp. from Termitogeton planus
The hindgut of T. planus harbors only a single morphotype of flagellates. Like other members of the genus Pseudotrichonympha, the cells are long and slender with tapered ends (Figures 3A,B,E,H). They measure 140–235 μm in length (mean 194 μm) and at their greatest diameter 14–30 μm in width (mean 23 μm; n = 20). Contractions of the flexible cells may cause temporary thickening of the body, and swimming cells are often flat and twisted into a wide spiral (Figure 3B). The entire cell surface is covered with flagella except for the dome-shaped operculum and (in some cells), the very posterior end. In the light microscope, two zones with different lengths of flagella are easily observed. A ring of long flagella (mean length 28 μm, n = 10) is at the base of the rostrum (Figures 3A,C,E,G,H). These flagella, which move independently of the postrostral flagella and can flap far to the anterior end (Figure 3C), have been defined as series-2 flagella (De Mello, 1927). The postrostral flagella (series-3 flagella) are oriented backward and are comparably short, measuring only about 13 μm (n = 10). They arise in parallel rows of basal bodies that run from the anterior to the posterior cell pole and are often somewhat oblique to the longitudinal cell axis (Figures 3E,F). In the higher resolution of a scanning electron microscope, a third series of tiny flagella (series-1 flagella) can be observed at the foremost part of the rostrum (Figure 3I). They are partly hidden by a ring of slender, flattened lappets of about 4 μm length arising from the operculum. In top view, the circular operculum shows a central smooth part that tends to collapse in the SEM samples (Figure 3H inset). The smooth center is surrounded by a ring of bulging membrane folds, whose interspaces prolong to the flattened lappets (Figures 3H,I inset). Phase-contrast light microscopy occasionally revealed a structure composed of lappets plus series-1 flagella (Figure 3D).
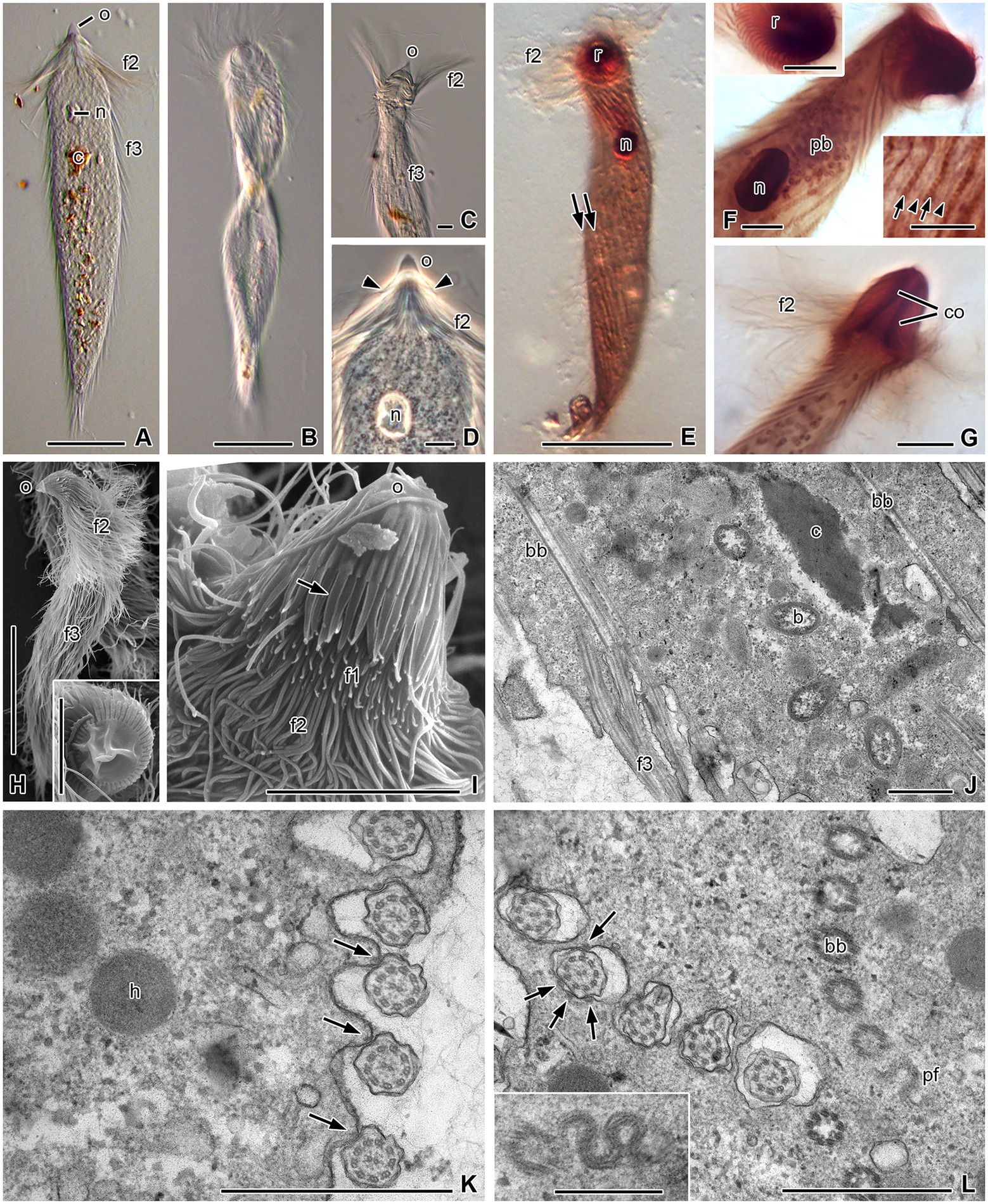
Figure 3. Morphology and ultrastructural details of Pseudotrichonympha solitaria. (A) Typical elongated cell with anterior operculum (o), a series of long flagella at the base of the rostrum (f2), and shorter postrostral flagella (f3). Nucleus (n) in anterior third of cell, ingested lignocellulose particles (c) in cytoplasm. (B) Twisted cell in motion. (C) Long rostral flagella struck forward. (D) Anterior third of body; extensions of operculum (arrowheads) are shorter than f3. (E–G) Protargol-staining contrasts nucleus, rows of basal bodies (arrows), parabasal filaments (arrowheads), parabasal bodies (pb), and columella (co) inside rostrum (r). (H) A total cell with pointed operculum and flagella series f2 and f3. Inset: Operculum in top view. (I) Rostrum in side view; apical operculum with numerous slender, leaf-like lappets posteriorly (arrow), which partially cover short series-1 flagella (f1). (J) Longitudinal section through cell periphery; long basal bodies (bb) of f3, cytoplasmic bacteria (b), and ingested lignocellulose particles. (K) Cell periphery in cross-section; flagella attached to cell surface, with electron-dense structures (arrows) supporting attachment sites; hydrogenosomes (h). (L) Basal part of flagella in pouches of the cell surface, with several contact sites to plasma membrane (arrows); inset: sinus-like parabasal filament (pf) under obliquely cross-sectioned rows of basal bodies. (A–G) Light microscopy: (A–C) differential interference contrast, (D) phase contrast, (E–G) protargol staining. (H,I) Scanning electron microscopy. (J–L) Transmission electron microscopy. Scale bars (A,B,E,H) 50 μm, (C,D,F,G, insets F,H,I) 10 μm, (J–L) 1 μm, (inset L) 0.5 μm.
The rostral region is 21 μm (18–25 μm) long and 15.5 μm (12.5–19.5 μm) wide (n = 10). Protargol-staining reveals a rostral tube of about 17 μm length (n = 10; Figure 3G), and the rows of basal bodies in the periphery of the rostrum are more numerous than in the adjacent cell body (Figure 3F, upper inset). Parabasal filaments appear as thin lines running parallel to the postrostral rows of basal bodies (Figure 3F, lower inset). The nucleus generally lies in the anterior third of the body, about 50 μm (n = 20) behind the anterior cell pole (Figures 3D,E), and rarely in the middle or even posterior region. It has an oval shape, measuring 9.3–13.7 μm (mean 11.8 μm) in length and 5.3–9.4 μm (mean 7.2 μm) in width (n = 20). The endoplasm of the cells contains wood fragments (Figures 3A,J). In protargol-stained specimens, dictyosomes are visible as dark spots or rings (Figure 3F). Their position is not related to the paths of the basal bodies. There are numerous dictyosomes in the cytoplasm anterior to the nucleus (Figure 3F).
Ultrastructure of the Pseudotrichonympha sp. from Termitogeton planus
The ultrastructure of the specimens from T. planus was almost identical to the detailed descriptions of Grimstone and Gibbons (1966) and Hollande and Carruette-Valentin (1971) for other Pseudotrichonympha species. In the following, we comment only on structural details that are either noteworthy or not mentioned in these studies. The postrostral flagella arise from long (ca. 3 μm) basal bodies. Their proximal part is embedded in short pouches (Figure 3J), in which they are attached to the plasma membrane at several contact sites (Figure 3L). The contact sites are supported by electron-dense material located under both plasma and flagellar membranes. Also outside the pouches, the proximal portion of the flagella remains attached to the cell body at one contact site (Figure 3K). In oblique cross-sections of basal body rows, a part of a sinus-like parabasal filament appears when the level of the section is directly underneath a basal body (Figure 3L and inset). The cytoplasm of the cells regularly contains endobiotic bacteria that are not enclosed in vacuoles, food vacuoles with wood particles, and globular hydrogenosomes (Figures 3J,K).
Phylogenetic analysis of Trichonymphida
Our phylogenetic analyses of all SSU rRNA gene sequences of Trichonymphida available to date (Figure 4) confirmed that the Pseudotrichonympha-like flagellate from G. oculatus and the corresponding phylotypes from Serritermes serrifer form a tight and highly supported clade (Cluster I in Radek et al., 2018; Retractinymphidae in Figure 4). Its deep-branching sister position to the Teranymphidae, however, was only weakly supported, and became inconsistent when rapidly evolving positions (up to 124 sites below the 50% identity threshold) were removed from the alignment (details not shown).
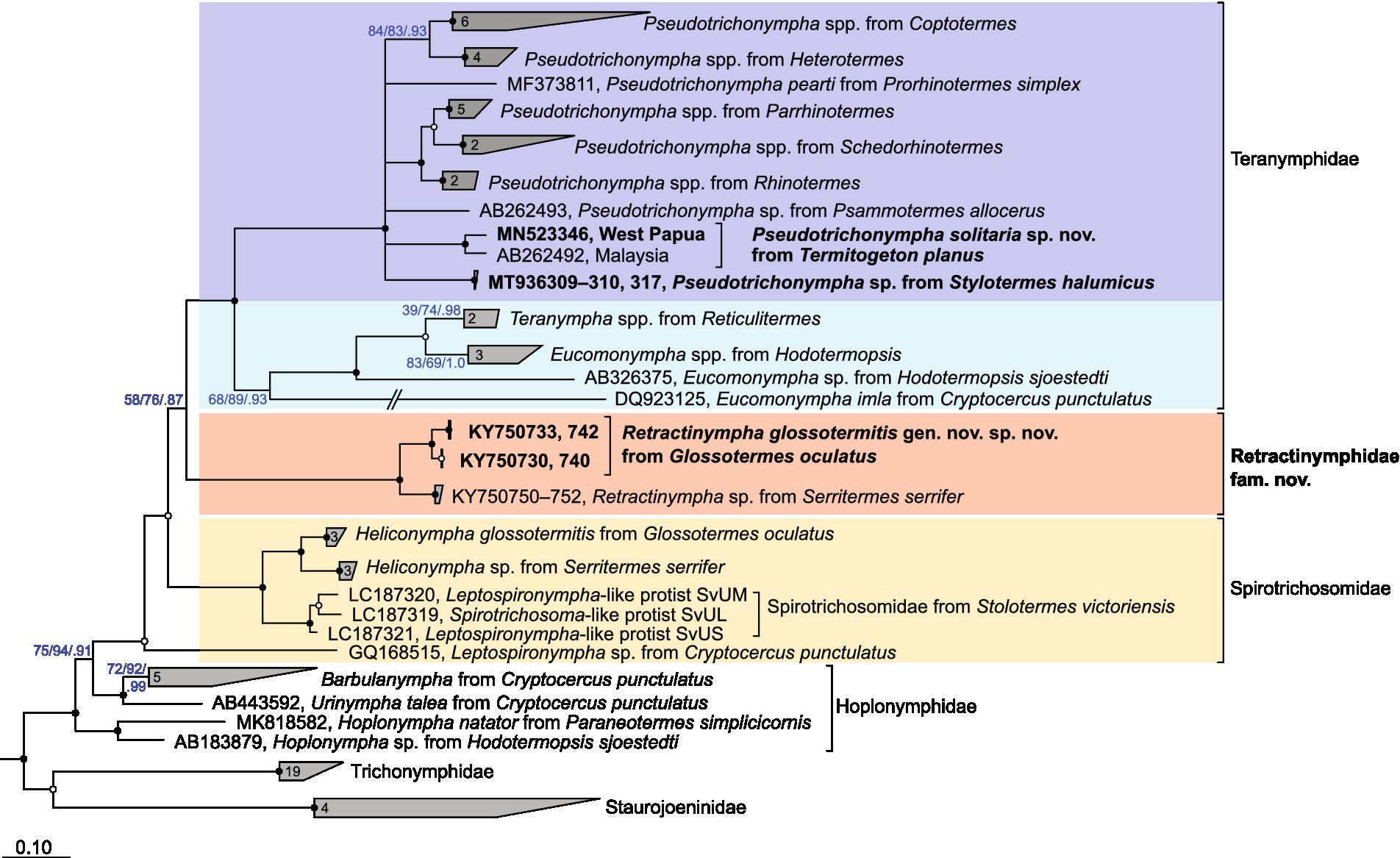
Figure 4. Phylogenetic analysis of the SSU rRNA genes of Trichonymphida. The position of Retractinympha glossotermitis and the Pseudotrichonympha spp. obtained in this study are shown in bold. The maximum-likelihood tree is a consensus tree from 1,000 bootstrap trees reconstructed with IQ-TREE, using Lophomonas striata and members of Trichomonadea as outgroup. Tree topology was tested with PhyML and Bayesian analysis (Ba); conflicting nodes are shown as multifurcation. Bullets indicate high node support in all analyses: SH-aLRT/ultrafast bootstrap/Ba posterior probabilities ≥96/99/1.00 (•), ≥ 80/95/0.98 (•); in other cases, individual values are shown. Collapsed clades are labeled with the number of sequences included. For more details, including accession numbers of all sequences, see Supplementary Figure S1.
The internal topology of the Teranymphidae clade confirms the paraphyly of the genus Eucomonympha (Carpenter and Keeling, 2007; Ohkuma et al., 2009) and the previously reported sister position of the genus Pseudotrichonympha to the Eucomonympha/Teranympha clade (Ohkuma et al., 2005). The internal topology of the Pseudotrichonympha clade confirms the cospeciation of Pseudotrichonympha spp. with their rhinotermitid hosts documented already by Noda et al. (2007). The cospeciation hypothesis agrees with the sister position of the Pseudotrichonympha phylotypes from the two closely related phylotypes of Termitogeton planus (Noda et al., 2007; this study). As in previous SSU rRNA-based studies, the exact relationships between the symbionts of Termitogeton, Psammotermes, and Prorhinotermes spp. remain unresolved (Noda et al., 2007; del Campo et al., 2017). The same applies also to the position of the Pseudotrichonympha phylotype from Stylotermes halumicus.
The Leptospironympha-like flagellates from Serritermitidae (Cluster II in Radek et al., 2018), which had been classified in the genus Heliconympha (Radek et al., 2018), form a well-supported sister group to the spirotrichosomid flagellates from Stolotermes victoriensis (Izawa et al., 2017), whose sequences had not yet been analyzed in this context. The results of the present analysis (Figure 4) fully agree with the morphology and ultrastructure of Heliconympha glossotermitis and the classification of the genus Heliconympha in the family Spirotrichosomidae (Radek et al., 2018). Notably, the single Leptospironympha sequence from Cryptocercus occupies a moderately supported position basal to Spirotrichosomidae from termites. The paraphyletic status of the family Hoplonymphidae agrees with previous results (e.g., Carpenter et al., 2010; Mee et al., 2019).
Flagellate phylotypes from Stylotermes halumicus and Termitogeton planus
For lack of fresh material, we could not obtain any morphological data for the flagellates of Stylotermes halumicus. Amplification of the SSU rRNA genes with flagellate-specific primers yielded a clone library (19 clones) that consisted exclusively of homologs from parabasalids and comprised three phylotypes (>99.5% sequence similarity). One of the phylotypes (11 clones) fell into the radiation of Pseudotrichonympha spp. from Rhinotermitidae (Figure 4). The two other phylotypes (5 and 3 clones, respectively) were highly similar (< 3.5% sequence divergence) and most closely related to a flagellate from Heterotermes tenuis (Rhinotermitidae) that was recently assigned to the genus Cthulhu (De Martini et al., 2021). They form a well-supported clade (<10% sequence divergence) with Cthulhu macrofasciculumque from Prorhinotermes simplex (Rhinotermitidae; James et al., 2013) and unclassified Hexamastix-like flagellates from Glossotermes and Serritermes spp. (Serritermitidae; Radek et al., 2018) in the Honigbergiellida (Figure 5). No PCR products were obtained with Oxymonadida-specific primers (Radek et al., 2019).
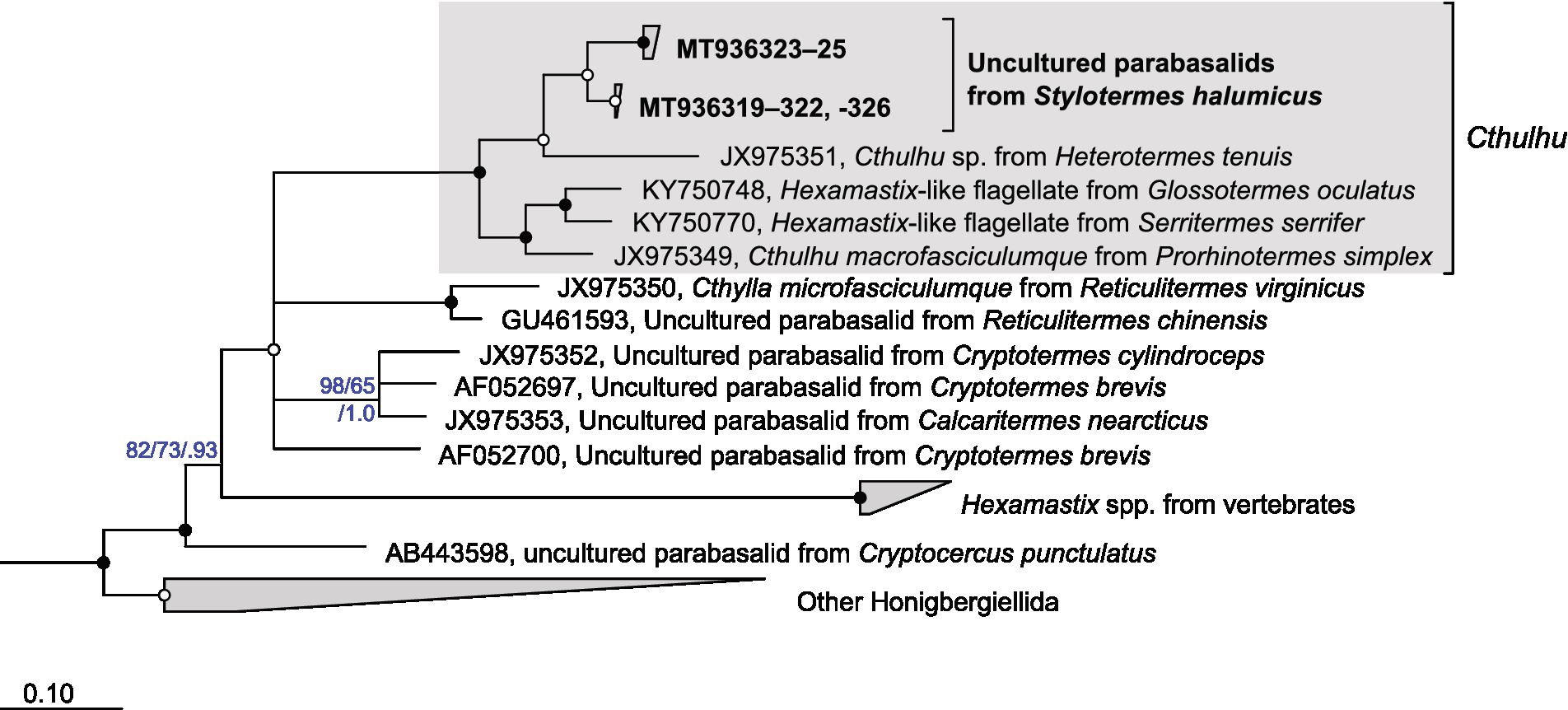
Figure 5. Phylogenetic analysis of the SSU rRNA genes of Honigbergiellida. The position of the uncultured parabasalids from Stylotermes halumicus is shown in bold. The maximum-likelihood tree was reconstructed with IQ-TREE using members of Trichomonadida as outgroup. Tree topology was tested with PhyML and Bayesian analysis (Ba); conflicting nodes are shown as multifurcation. Bullets indicate high node support in all analyses: SH-aLRT/ultrafast bootstrap/posterior probabilities ≥96/99/1.00 (•), ≥ 80/95/0.98 (•); in other cases, individual values are shown. Collapsed clades are labeled with the number of sequences included.
The COII gene sequence of Termitogeton planus was identical to that reported for a specimen previously collected in almost the same location in West Papua (KP026298; Bourguignon et al., 2015) but differed significantly from those of specimens collected in other countries, including those from Malaysia (Supplementary Figure S1), where the type of Termitogeton planus was collected (Bourguignon and Roisin, 2011). This agrees with the notion that specimens from West Papua may represent a separate species (Parmentier and Roisin, 2003). The DNA in the ethanol-fixed samples was strongly degraded, but the SSU rRNA genes were successfully amplified using nested PCR. Direct sequencing yielded a clean sequence read along its entire length, which agrees with the observation that this termite harbors only a single morphotype of gut flagellates (see above). It was most similar (3.5% sequence divergence) to the sequence previously obtained from a suspension of Pseudotrichonympha sp. (AB262492) from specimens of Termitogeton planus collected in Malaysia (AF262598; Noda et al., 2007; Figure 4). A Parabasalia-specific internal primer set yielded the same phylotype, which is consistent with the absence of other gut flagellates.
Discussion
Coevolutionary history of Trichonymphida and Neoisoptera
The exclusive presence of flagellates of the order Trichonymphida in termites and Cryptocercidae suggests that the common ancestor of these groups was already colonized by ancestral lineages of these flagellates (Carpenter et al., 2009; Ohkuma et al., 2009). The basal position of Cryptocercus symbionts in several families of Trichonymphida (Trichonymphidae, Hoplonymphidae, Spirotrichosomidae, and Teranymphidae) strongly suggests that the common ancestor of Cryptocercidae and termites already harbored multiple lineages of Trichonymphida that had diversified before the split of the two lineages and were subsequently lost multiple times during termite evolution. Although the relationships between flagellates and their respective hosts are not always fully resolved, the results of the present study provide new insights into the coevolutionary history of Trichonymphida and their neoisopteran host families (Stylotermitidae, Serritermitidae, and Rhinotermitidae).
The identification of a Pseudotrichonympha phylotype in Stylotermes extends the presence of this flagellate genus to Stylotermitidae, the most basal family of Neoisoptera. Although not all positions were fully resolved, it is likely that an ancestral member of the genus Pseudotrichonympha was present already before the radiation of Neoisoptera and subsequently cospeciated with its host (Noda et al., 2007; Figure 6). The Pseudotrichonympha lineage was lost at least three times, once in a common ancestor of the genus Reticulitermes, once in a common ancestor of Serritermitidae, and once in a common ancestor of Termitidae. Each of these losses was accompanied by the loss of some or – in the case of Termitidae – all other gut flagellates.
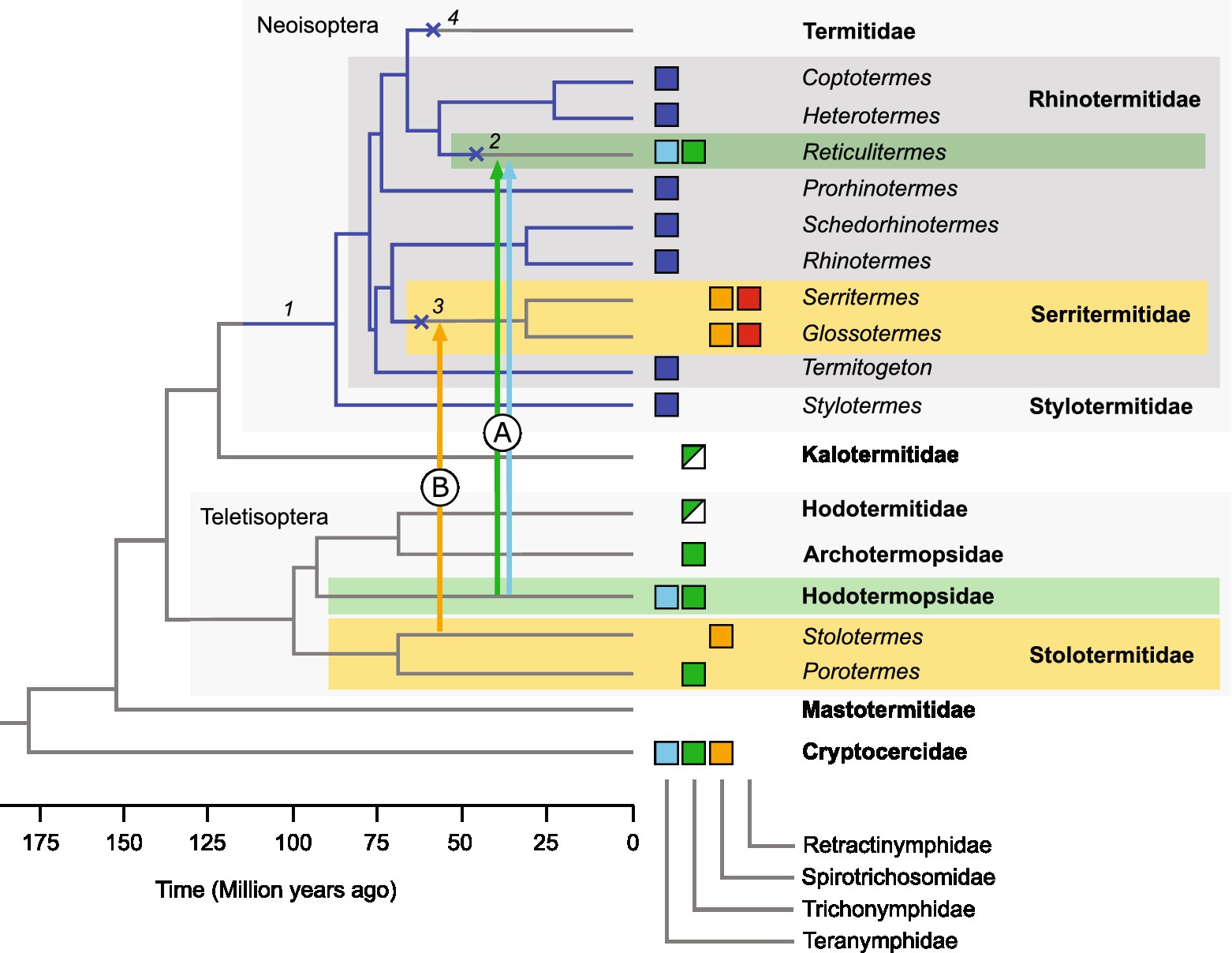
Figure 6. Evolutionary history of Trichonymphida in Neoisoptera. Boxes indicate the occurrence of a particular flagellate family in a termite genus (same colors as in Figure 4). We propose that a member of the genus Pseudotrichonympha was present already in the common ancestor of Neoisoptera (1) and subsequently cospeciated with its host (blue edges). The lineage was lost (×) several times during host evolution (2–4). Arrows indicate horizontal transfer events that led to the acquisition of Trichonymphidae and Teranymphidae in Reticulitermes (A) and of Spirotrichosomidae in Serritermitidae (B). The origin of Retractinymphidae is unclear. The host phylogeny (schematic) is based on Bucek et al. (2019) and Wang et al. (2022).
The loss of Pseudotrichonympha in Reticulitermes coincides with the appearance of several flagellate lineages that are not represented in other Neoisoptera but were apparently acquired by horizontal flagellate transfer from other, more basal termite families (Teletisoptera; Figure 6). The transfer of Trichonympha and Teranympha (Trichonymphida), Spirotrichonympha (Spirotrichonymphida), and several members of Pyrsonymphidae (Oxymonadida) from Hodotermopsidae to an ancestral member of Reticulitermes, which had been proposed already by Kitade (2004), is strongly supported by the close phylogenetic relatedness of the respective species (James et al., 2013; Gile et al., 2018, 2021; Radek et al., 2019; Figure 4). A similar scenario has been employed to explain the unique presence of Heliconympha in Serritermitidae (Radek et al., 2018). Here, the horizontal transfer of flagellates from an ancestral Stolotermes species (Figure 6) is substantiated by the close phylogenetic relatedness of the Spirotrichosomidae from Stolotermes victoriensis to members of the genus Heliconympha (Figure 4).
The origin of Retractinympha (see below), the second trichonymphid in Serritermitidae, however, remains unclear. Members of this genus represent a novel family-level lineage that has no representatives in any other termites investigated, and their presence in Serritermitidae could be explained by different scenarios. One would involve the presence of a separate lineage of trichonymphids in the neoisopteran ancestor that was vertically inherited but subsequently lost in all lineages but Serritermitidae. An alternative explanation would be the horizontal acquisition from a host outside of the Neoisoptera. A plausible opportunity would be the same transfer event that led to the acquisition of Heliconympha from Stolotermitidae. Since data on the molecular diversity of the flagellate communities in Stolotermitidae are available only for a single host species (Izawa et al., 2017), it is possible that relatives of Retractinympha will be discovered once other species of this termite family have been studied.
The genus Retractinympha (Retractinymphidae fam. nov.)
The superficial resemblance of the Pseudotrichonympha-like flagellates from G. oculatus and Serritermes serrifer to members of the genus Pseudotrichonympha stands in stark contrast to the results of the phylogenetic analysis, which identifies them as a deep-branching and only weakly supported sister group of Teranymphidae, with Spirotrichosomidae in a basal position (Radek et al., 2018; Figure 4). Based on phylogenetic evidence and the morphological differences discussed below, we propose to classify these flagellates in the new genus Retractinympha, with Retractinympha glossotermitis from G. oculatus as type species.
Retractinympha glossotermitis shows the general traits of Trichonymphida: (i) a bilaterally symmetric rostrum covered with numerous flagella, except at the operculum, (ii) numerous parabasal filaments that originate from two (or four) parabasal plates and may form a rostral tube, and (iii) the absence of a protruding axostyle (Čepička et al., 2010, 2016). Their fusiform shape and an almost complete flagellation with two series of rostral flagella, is typical also for members of the genus Pseudotrichonympha. However, there are several traits that allow the genus Retractinympha to be distinguished from Teranymphidae also on a morphological basis and to justify its classification in a new family, Retractinymphidae.
The traits distinguishing Retractinymphidae from Teranymphidae are the length ratio of rostral to postrostral flagella, the presence of an axostyle, the shape of the nucleus, and the apparent absence of parabasal bodies. The rostral flagella of Teranymphidae are always longer than the postrostral flagella, both sets are of the same length in Retractinymphidae. The scattered axostyle fibers of Teranymphidae are absent from Retractinymphidae. While the nucleus of Teranymphidae is always rounded or oval, the drop-shaped nucleus of R. glossotermitis with its sharp end oriented toward the anterior cell pole is unique among members of the order Trichonymphida. While Teranymphidae possess numerous small, rounded parabasal bodies that are not in a specific relation to the nucleus, such structures are hardly visible in protargol-stained preparations of R. glossotermitis.
In addition to the family-specific traits, there are other traits that distinguish the genus Retractinympha from individual genera of Teranymphidae (Teranympha, Eucomonympha, and Pseudotrichonympha). While the parabasal filaments of Pseudotrichonympha appear as broad structures that run parallel to the basal body rows (Grimstone and Gibbons, 1966; Hollande and Carruette-Valentin, 1971), the parabasal filaments of Retractinympha are very fine fibers and rarely associated with the basal bodies. The rostral flagella are of uniform length in Eucomonympha and Teranympha but unequal (series 1 and 2) in Retractinympha. While the postrostral flagella of Teranympha are organized in multiple transverse rows that are separated by cytoplasmic bands (Koidzumi, 1921), Retractinympha shows the arrangement in tight longitudinal rows typically found also in other Trichonymphida. In addition, members of the genus Teranympha possess long axostylar bundles (Cleveland, 1938; Hollande and Carruette-Valentin, 1971; Carpenter and Keeling, 2007), whereas Retractinympha has no obvious axostyle.
The family Spirotrichosomidae
So far, members of Spirotrichosomidae (Hollande and Carruette-Valentin, 1971) had been detected exclusively in stolotermitids and in the genus Cryptocercus, which adds further support to their ancestral transfer from a stolotermitid to a serritermitid host (see above). The sister position of the genus Heliconympha to the spirotrichosomid flagellates of Stolotermes victoriensis (Figure 4) agrees with the morphological features shared by members of this family (Radek et al., 2018). Therefore, we propose to include the genus Heliconympha in the Spirotrichosomidae, which requires to emend the family description (see below).
Cryptocercus punctulatus harbors four species of spirotrichosomids (Leptospironympha eupora, Leptospironympha rudis, Leptospironympha wachula, and Macrosporonympha xylopletha, all described by Cleveland et al., 1934), but only a single rRNA gene sequence for an unspecified member of the genus Leptospironympha has been obtained (Carpenter et al., 2010). The four spirotrichosomids in Stolotermes victoriensis are Spirotrichosoma capitata Sutherland 1933, Leptospironympha (Spirotrichosoma) obtusa (Sutherland 1933), Leptospironympha minor Cleveland and Day 1958, and Leptospironympha numida Cleveland and Day 1958. However, the three phylotypes of spirotrichosomids obtained from Stolotermes victoriensis (Izawa et al., 2017), which include both a large Spirotrichosoma-like species (designated SvUL; presumably representing the type species, S. capitata) and two smaller Leptospironympha-like species (SvUM and SvUS), form a tight cluster (<3% sequence dissimilarity), suggesting that they belong to the same genus. This would agree with the original description of L. obtusa as Spirotrichosoma obtusa by Sutherland (1933). Notably, the transfer of S. obtusa to the genus Leptospironympha Cleveland et al. 1934, which had been created to accommodate the three species of Leptospironympha from C. punctulatus (Cleveland et al., 1934), was based entirely on Sutherland’s description. Cleveland et al. (1934) had actually cautioned that their own observations, albeit made from termites preserved in alcohol and hence not very dependable, did not indicate so close a relationship.
Only very few representatives of Spirotrichosomidae have been sequenced to date, but phylogenetic analyses indicate that the family is paraphyletic (Radek et al., 2018; this study). This possibility was raised already by Carpenter et al. (2010) based on the morphological features of Leptospironympha spp. described in Cryptocercus and Stolotermes. If future studies of the morphologically diverse species of spirotrichosomids confirm that the Leptospironympha spp. from Cryptocercus (comprising the type species, L. eupora) are monophyletic and sister to all spirotrichosomids from Stolotermes, the genus Leptospironympha, which had been included in Spirotrichosomidae by Hollande and Carruette-Valentin (1971), should be elevated to family level, and the Leptospironympha species from Stolotermes should be reclassified.
While the flagellar bands of Retractinymphidae and Teranymphidae are organized in straight or slightly slanted bands of flagella with single rows of basal bodies, the more basal Spirotrichosomidae possess spiral bands of flagella that contain many short rows of basal bodies (Čepička et al., 2016; Radek et al., 2018). Based on their ancestral position, it is likely that the spiral organization of Spirotrichosomidae was lost in a common ancestor of Retractinymphidae and Teranymphidae. A loss of the post-rostral spirals and a retention of the longitudinal secondary flagellar bands during the transition from Spirotrichosomidae to Eucomonympha had been suggested already by Carpenter et al. (2010). Alternatively, it is possible that the spiral organization has evolved twice independently in the spirotrichosomids of Cryptocercidae and Stolotermitidae.
The genus Pseudotrichonympha (Teranymphidae)
The genus Pseudotrichonympha comprises 23 described species and subspecies, and numerous representatives that remain to be described (Supplementary Figure S2; Supplementary Table S1). Quite a few species reportedly occur in several hosts but considering that all termites investigated to date harbor a unique phylotype (Noda et al., 2007; Saldarriaga et al., 2011; del Campo et al., 2017; Supplementary Figure S1), it is reasonable to assume that these reports will not hold up to scrutiny if different termite genera are concerned. Even in closely related hosts, the corresponding flagellates are divergent, as illustrated by the case of Termitogeton planus.
The presence of a flagellate of the genus Pseudotrichonympha in the genus Termitogeton (T. umbilicatus) was first reported by Grassi (1919). Almost a century later, the assignment was confirmed in a molecular study of Termitogeton planus (Noda et al., 2007), which identified the only gut flagellate of this termite as a distinct species in the radiation of the genus Pseudotrichonympha. Our characterization of Pseudotrichonympha solitaria did not reveal any structures that are unique to this species. Rather, it is the specific combination of features like variation in body size and form, lengths of the three series of flagella, lengths of apical cap, rostral tube and campanula, and size and position of the nucleus that allows the members of this genus to be distinguished (Das, 1976).
Pseudotrichonympha solitaria possesses all morphological traits shared by other species in the genus (see Brugerolle and Lee, 2000). The cells are large and slender, and except for the dome-shaped operculum, almost completely covered with longitudinal or slightly oblique rows of flagella. Also, other traits that are considered as genus-specific by some authors but not mentioned in most descriptions are present in P. solitaria. They include the instability of the thin-walled operculum during fixation or the stainable threads running along its sides (De Mello, 1954a,b). The latter probably correspond to the ring of slender lappets observed in the SEM images of P. solitaria but were absent from the few SEM images of other Pseudotrichonympha species (Saldarriaga et al., 2011).
Other fine structures, such as the sinus-like parabasal filaments underneath the postrostral rows of basal bodies and the lack of connections (“bandelettes cinétodesmales”) between neighboring basal bodies match the detailed descriptions of other Pseudotrichonympha species (Grimstone and Gibbons, 1966; Hollande and Carruette-Valentin, 1971). The flexibility and torsion of the cell body observed in P. solitaria has been described for other species of the genus [e.g., Pseudotrichonympha bachmani (Calkins, 1936), Pseudotrichonympha cardiformis (Karandikar and Vittal, 1954)]. The ability to deeply retract the anterior cell pole, which is reflected in species epithet of Pseudotrichonympha introflexibilis (Dogiel, 1922), is present also in Pseudotrichonympha leei (del Campo et al., 2017) and in members of the eponymous genus Retractinympha. Such anterior retractions are considered to be elicited by abnormal conditions (Calkins, 1936), but although most pronounced in stained smears and older life preparations, they are common in fresh preparations of R. glossotermitis. The contractility itself may be a feature of the intracellular architecture. De Mello (1954b) considered so-called “myonemes,” which he observed in stained specimens and described as single threads or dichotomously branched structures of whip-like bundles, to be responsible for the mobility of Pseudotrichonympha. However, we did not observe any myoneme-like structures in R. glossotermitis or P. solitaria.
Protologues
ZooBank number of publication: https://zoobank.org/urn:lsid:zoobank.org:pub:7EDA86B0-2C88-4D11-8391-602389366675.
Description of Retractinymphidae fam. nov. Radek and Brune
Taxonomy
Excavata, Parabasalia, Trichonymphea, Trichonymphida.
Etymology
N.L. fem. n. Retractinympha, a genus of flagellates. N.L. fem. n. Retractinymphidae, the family of Retractinympha.
Description
Cells completely covered by longitudinal rows of flagella. Rostral flagella not longer than postrostral flagella. First series of rostral flagella shorter than second series. Drop-shaped nucleus. Reduced axostyle. Parabasal bodies hardly visible.
Type genus
Retractinympha gen. nov.
ZooBank number:
https://zoobank.org/urn:lsid:zoobank.org:act:BD5481B7-9DA6-4B1A-A2A5-10CF3AE140A0.
Description of Retractinympha gen. nov. Radek and Brune
Taxonomy
Excavata, Parabasalia, Trichonymphea, Trichonymphida, Retractinymphidae.
Etymology
L. adj. retractus, perf. pass. part. of retrahere, to pull back, withdraw; L. fem. n. nympha, from Gr. nýmphē a beautiful maiden, nymph, common element of the genus names of hypermastigid flagellates in termite guts; N.L. fem. n. Retractinympha, a termite gut flagellate with a retractable rostrum.
Description
Large elongated cells with retractable rostrum. The genus is presently monospecific.
Type species
Retractinympha glossotermitis gen. nov. sp. nov.
ZooBank number:
https://zoobank.org/urn:lsid:zoobank.org:act:1231DFF9-D6D9-4ABA-A53A-94A78BD7650D.
Description of Retractinympha glossotermitis sp. nov. Radek and Brune
Taxonomy
Excavata, Parabasalia, Trichonymphea, Trichonymphida, Retractinymphidae, Retractinympha.
Etymology
N.L. gen. n. glossotermitis, referring to Glossotermes, the genus of termites colonized by this flagellate species.
Description
Spindle-shaped body measuring 107–260 (mean 163) μm in length and 60–92 (mean 70) μm in width. Short rostral flagella in series 1. Rostral flagella of series 2 and postrostral flagella have the same length of about 22–28 μm (mean 25 μm). The rostral tube measures 12–15.5 μm in length. Drop-shaped nucleus (mean 45.6 × 25.6 μm) located marginally in the upper part of the post-rostral body region; pointed end oriented toward the rostrum. Dictyosomes are neither associated with rows of basal bodies nor with parabasal filaments. Parabasal filaments are thin and rather straight. Axostyle not found.
Type host
The hindgut of Glossotermes oculatus Emerson 1950 (Serritermitidae).
Type host locality
The termites were collected near the Petit-Saut Dam south of Sinnamary (5.0662° N 53.0460° W) and in the Nouragues Natural Reserve (4.0717° N 52.7325° W) in French Guiana.
Hapantotype
Protargol-stained microscopy slide deposited at the Biology Centre of the Upper Austrian Museum, J.-W.-Klein-Strasse 73, 4040 Linz, Austria under type number 2019/66.
Gene sequences
SSU rRNA gene sequence accession numbers KY750730, KY750733.
ZooBank number:
https://zoobank.org/urn:lsid:zoobank.org:act:C170AEB2-19FE-405C-8615-851EADDAD8AF.
Description of Pseudotrichonympha solitaria sp. nov. Radek and Brune
Taxonomy
Excavata, Parabasalia, Trichonymphea, Trichonymphida, Teranymphidae, Pseudotrichonympha.
Etymology
L. fem. adj. solitaria, lonely, solitary; the only flagellate species in its termite host.
Description
Long, slender, completely flagellated cells (140–235 × 14–30 μm) with three series of flagella. Flagella at the tip of the rostrum (series 1) are very short and partially covered by leaf-like lappets of the operculum. Flagella at the base of the rostrum (series 2) are much longer (ca. 28 μm). Postrostral flagella (series 3) are ca. 13 μm long. Rostral tube of about 17 μm length. Oval nucleus (9.3–13.7 × 5.3–9.4 μm; mean 11.8 × 7.2 μm) in anterior third of body.
Type host
The hindgut of Termitogeton planus (Haviland 1898) (Rhinotermitidae), COII gene accession number MN528021.
Type host locality
West Papua, Indonesia, 30 km southeast of Nabire (3°29.213408′ S, 135°42.089227′ E).
Syntype
Protargol-stained microscopy slide deposited at the Biology Centre of the Upper Austrian Museum, J.-W.-Klein-Strasse 73, 4040 Linz, Austria under type number 2019/63.
Gene sequences
SSU rRNA gene accession numbers MN523346 (symbiont of type host; this study) and AB262492 (symbiont of T. planus from Malaysia; Noda et al., 2007).
ZooBank number:
http://zoobank.org/urn:lsid:zoobank.org:act:3567DB03-6C24-476A-AB52-F2FAEE010091.
Emended description of Spirotrichosomidae Hollande and Carruette-Valentin 1971
The description of the family is the same in the original description (Hollande and Carruette-Valentin, 1971), with the following addition:
Included genera
Apospironympha Cleveland and Day 1958; Bispironympha Bobyleva 1969; Colospironympha Cleveland and Day 1958; Heliconympha Radek et al. 2018; Leptospironympha Cleveland et al. 1934; Macrospironympha Cleveland et al. 1934; and Spirotrichosoma Sutherland 1933.
Data availability statement
The datasets presented in this study can be found in online repositories. The names of the repository/repositories and accession number(s) can be found in the article/Supplementary material.
Author contributions
RR and AB conceived the study and wrote the manuscript. JŠ, DS-D, and RH collected termites. RR and DÖ performed the structural analyses. KP and AB performed the molecular work and the phylogenetic analyses. All authors have read and approved the manuscript.
Funding
The work in the lab of RR was funded by the Freie Universität Berlin. The work in the lab of AB was funded by the Max Planck Society. JŠ was supported by the Faculty of Tropical AgriSciences, CZU (IGA project No. 20223112). RH received funding from the Institute of Organic Chemistry and Biochemistry, CAS (RVO: 61388963).
Acknowledgments
We are grateful to Jürgen F. H. Strassert, Berlin, for providing Figure 1A. We thank Florian Conrad, Berlin, for contributions during a student project and Regina Kollmann, Berlin, for technical assistance with electron microscopy.
Conflict of interest
The authors declare that the research was conducted in the absence of any commercial or financial relationships that could be construed as a potential conflict of interest.
Publisher’s note
All claims expressed in this article are solely those of the authors and do not necessarily represent those of their affiliated organizations, or those of the publisher, the editors and the reviewers. Any product that may be evaluated in this article, or claim that may be made by its manufacturer, is not guaranteed or endorsed by the publisher.
Supplementary material
The Supplementary material for this article can be found online at: https://www.frontiersin.org/articles/10.3389/fevo.2023.1111484/full#supplementary-material
Footnotes
References
Barden, P., and Engel, M. S. (2020). “Fossil social insects” in Encyclopedia of Social Insects. ed. C. Starr (Cham, Switzerland: Springer International Publishing AG), 1–21. doi: 10.1007/978-3-319-90306-4_45-1
Bourguignon, T., Lo, N., Cameron, S. L., Šobotník, J., Hayashi, Y., Shigenobu, S., et al. (2015). The evolutionary history of termites as inferred from 66 mitochondrial genomes. Mol. Biol. Evol. 32, 406–421. doi: 10.1093/molbev/msu308
Bourguignon, T., and Roisin, Y. (2011). Revision of the termite family Rhinotermitidae (Isoptera) in New Guinea. ZooKeys 148, 55–103. doi: 10.3897/zookeys.148.1826
Brugerolle, G., and Bordereau, C. (2004). The flagellates of the termite Hodotermopsis sjoestedti with special reference to Hoplonympha, Holomastigotes and Trichomonoides trypanoides n. comb. Europ. J. Protistol. 40, 163–174. doi: 10.1016/j.ejop.2004.05.001
Brugerolle, G., and Lee, J. J. (2000). “Phylum Parabasalida,” in The Illustrated Guide to the Protozoa. eds. J. J. Lee, G. F. Leedale, and P. Bradbury (Lawrence, Society of Protozoologists: Allen Press Inc.), 1196–1250.
Brune, A. (2014). Symbiotic digestion of lignocellulose in termite guts. Nat. Rev. Microbiol. 12, 168–180. doi: 10.1038/nrmicro3182
Bucek, A., Šobotník, J., He, S., Shi, M., McMahon, D. P., Holmes, E. C., et al. (2019). Evolution of termite symbiosis informed by transcriptome-based phylogenies. Curr. Biol. 29, 3728–3734.e4. doi: 10.1016/j.cub.2019.08.076
Calkins, G. N. (1936). Some polymastigote and hypermastigote flagellates from Puerto Rican termites. Puerto Rico J. Publ. Health Trop. Med. 12, 169–187.
Carpenter, K. J., Chow, L., and Keeling, P. J. (2009). Morphology, phylogeny, and diversity of Trichonympha (Parabasalia: Hypermastigida) of the wood-feeding cockroach Cryptocercus punctulatus. J. Eukaryot. Microbiol. 56, 305–313. doi: 10.1111/j.1550-7408.2009.00406.x
Carpenter, K. J., Horak, A., and Keeling, P. J. (2010). Phylogenetic position and morphology of Spirotrichosomidae (Parabasalia): new evidence from Leptospironympha of Cryptocercus punctulatus. Protist 161, 122–132. doi: 10.1016/j.protis.2009.06.003
Carpenter, K. J., and Keeling, P. J. (2007). Morphology and phylogenetic position of Eucomonympha imla (Parabasalia: Hypermastigida). J. Eukaryot. Microbiol. 54, 325–332. doi: 10.1111/j.1550-7408.2007.00263.x
Čepička, I., Dolan, M. F., and Gile, G. H. (2016). “Parabasalia” in Handbook of the Protists. eds. J. M. Archibald, A. G. B. Simpson, and C. H. Slamovits (Cham, Switzerland: Springer International Publishing AG), 1–44. doi: 10.1007/978-3-319-32669-6_9-1
Čepička, I., Hampl, V., and Kulda, J. (2010). Critical taxonomic revision of parabasalids with description of one new genus and three new species. Protist 161, 400–433. doi: 10.1016/j.protis.2009.11.005
Chouvenc, T., Šobotník, J., Engel, M. S., and Bourguignon, T. (2021). Termite evolution: mutualistic associations, key innovations, and the rise of Termitidae. Cell. Mol. Life Sci. 78, 2749–2769. doi: 10.1007/s00018-020-03728-z
Cleveland, L. R., and Day, M. (1958). Spirotrichonymphidae of Stolotermes. Arch. Protistenkd. 103, 1–53.
Cleveland, L. R., Hall, S. R., Sanders, E. P., and Collier, J. (1934). The wood-feeding roach Cryptocercus, its protozoa, and the symbiosis between protozoa and roach. Mem. Am. Acad. Arts Sci. 17, 3–342. doi: 10.2307/25058198
Das, A. K. (1976). Studies on some hypermastigids (protozoa) from the termites of West Bengal, India. Acta Protozool. 15, 101–124.
De Martini, F., Coots, N. L., Jasso-Selles, D. E., Shevat, J., Ravenscraft, A., Stiblík, P., et al. (2021). Biogeography and independent diversification in the protist symbiont community of Heterotermes tenuis. Front. Ecol. Evol. 9:640625. doi: 10.3389/fevo.2021.640625
De Mello, F. (1927). Revision de trichonymphids du Leucotermes indicola Wasm. Arch. Esc. Med. Cirurg. Nova Goa. Ser. A 1, 1–28.
De Mello, I. F. (1954a). Contribution à l’étude des microparasites des termites brésiliens. Flagellés du contenu intestinal d’Heterotermes tenuis. Mem. Inst. Oswaldo Cruz 52, 17–51. doi: 10.1590/S0074-02761954000100003
De Mello, I. F. (1954b). Pseudotrichonympha sertaneja n. sp. (Protozoa, Mastigophora), from the intestine of a new termite (Rugitermes sp.) collected in Brazil. Parasitology 44, 24–29. doi: 10.1017/S0031182000018734
Del Campo, J., James, E. R., Hirakawa, Y., Fiorito, R., Kolisko, M., Irwin, N. A. T., et al. (2017). Pseudotrichonympha leei, Pseudotrichonympha lifesoni, and Pseudotrichonympha pearti, new species of parabasalian flagellates and the description of a rotating subcellular structure. Sci. Rep. 7:16349. doi: 10.1038/s41598-017-16259-8
Dogiel, V. (1922). Researches on the parasitic protozoa from the termites. III. Trichonymphidae. Ruskii Arkhiv Protistologii 1, 172–234.
Dolejšová, K., Krasulová, J., Kutalová, K., and Hanus, R. (2014). Chemical alarm in the termite Termitogeton planus (Rhinotermitidae). J. Chem. Ecol. 40, 1269–1276. doi: 10.1007/s10886-014-0515-0
Engel, M. S. (2011). Family-group names for termites (Isoptera), redux. ZooKeys 148, 171–184. doi: 10.3897/zookeys.148.1682
Engel, M. S., Grimaldi, D. A., and Krishna, K. (2009). Termites (Isoptera): their phylogeny, classification, and rise to ecological dominance. Am. Mus. Novit. 2009, 1–27. doi: 10.1206/651.1
Foissner, W. (2014). An update of ‘basic light and scanning electron microscopic methods for taxonomic studies of ciliated protozoa’. Int. J. Syst. Evol. Microbiol. 64, 271–292. doi: 10.1099/ijs.0.057893-0
Gile, G. H., James, E. R., Tai, V., Harper, J. T., Merrell, T. L., Boscaro, V., et al. (2018). New species of Spirotrichonympha from Reticulitermes and the relationships among genera in Spirotrichonymphea (Parabasalia). J. Euk. Microbiol. 65, 159–169. doi: 10.1111/jeu.12447
Gile, G. H., Taerum, S. J., Jasso-Selles, D. E., Sillam-Dussès, D., Ohkuma, M., Kitade, O., et al. (2021). Molecular phylogenetic position of Microjoenia (Parabasalia: Spirotrichonymphea) from Reticulitermes and Hodotermopsis termite hosts. Protist 172:125836. doi: 10.1016/j.protis.2021.125836
Grimstone, A. V., and Gibbons, I. R. (1966). The fine structure of the centriolar apparatus and associated structures in the complex flagellates Trichonympha and Pseudotrichonympha. Phil. Trans. R. Soc. Lon. Ser. B. Biol. Sci. 250, 215–242.
Guindon, S., Dufayard, J. F., Lefort, V., Anisimova, M., Hordijk, W., and Gascuel, O. (2010). New algorithms and methods to estimate maximum-likelihood phylogenies: assessing the perfomance of PhyML 3.0. System. Biol. 59, 307–321. doi: 10.1093/sysbio/syq010
Hampl, V. (2016). “Preaxostyla” in Handbook of the Protists. eds. J. M. Archibald, A. G. B. Simpson, and C. H. Slamovits (Cham, Switzerland: Springer International Publishing AG), 1–44. doi: 10.1007/978-3-319-32669-6_8-1
Hoang, D. T., Chernomor, O., von Haeseler, A., Minh, B. Q., and Vinh, L. S. (2018). UFBoot2: improving the ultrafast bootstrap approximation. Mol. Biol. Evol. 35, 518–522. doi: 10.1093/molbev/msx281
Hollande, A., and Carruette-Valentin, J. (1971). Les atractophores, l’induction du fuseau et la division cellulaire chez les hypermastigines: Étude infrastructurale et révision systématique des trichonymphines et des spirotrichonymphines. Protistologica 7, 5–100.
Hongoh, Y. (2011). Toward the functional analysis of uncultivable, symbiotic microorganisms in the termite gut. Cell. Mol. Life Sci. 68, 1311–1325. doi: 10.1007/s00018-011-0648-z
Ikeda-Ohtsubo, W., and Brune, A. (2009). Cospeciation of termite gut flagellates and their bacterial endosymbionts: Trichonympha species and ‘Candidatus Endomicrobium trichonymphae’. Mol. Ecol. 18, 332–342. doi: 10.1111/j.1365-294X.2008.04029.x
Inoue, T., Kitade, O., Yoshimura, T., and Yamaoka, I. (2000). “Symbiotic associations with protists,” in Termites: Evolution, sociality, symbioses, ecology. eds. T. Abe, D. E. Bignell, and M. Higashi (Dordrecht: Springer), 275–288. doi: 10.1007/978-94-017-3223-9_13
Inward, D. J. G., Vogler, A. P., and Eggleton, P. (2007). A comprehensive phylogenetic analysis of termites (Isoptera) illuminates key aspects of their evolutionary biology. Mol. Phylogenet. Evol. 44, 953–967. doi: 10.1016/j.ympev.2007.05.014
Izawa, K., Kuwahara, H., Sugaya, K., Lo, N., Ohkuma, M., and Hongoh, Y. (2017). Discovery of ectosymbiotic Endomicrobium lineages associated with protists in the gut of stolotermitid termites. Environ. Microbiol. Rep. 9, 411–418. doi: 10.1111/1758-2229.12549
James, E. R., Tai, V., Scheffrahn, R. H., and Keeling, P. J. (2013). Trichonympha burlesquei n. sp. from Reticulitermes virginicus and evidence against a cosmopolitan distribution of Trichonympha agilis in many termite hosts. Int. Syst. Evol. Microbiol. 63, 3873–3876. doi: 10.1099/ijs.0.054874-0
Jasso-Selles, D. E., De Martini, F., Freeman, K. D., Garcia, M. D., Merrell, T. L., Scheffrahn, R. H., et al. (2017). The parabasalid symbiont community of Heterotermes aureus: molecular and morphological characterization of four new species and reestablishment of the genus Cononympha. Europ. J. Protistol. 61, 48–63. doi: 10.1016/j.ejop.2017.09.001
Kalyaanamoorthy, S., Minh, B., Wong, T., von Haeseler, A., and Jermiin, L. (2017). ModelFinder: fast model selection for accurate phylogenetic estimates. Nat. Methods 14, 587–589. doi: 10.1038/nmeth.4285
Karandikar, K. R., and Vittal, M. (1954). Flagellates in the termites from Dharwar. J. Univ. Bombay 23, 1–24.
Kitade, O., and Matsumoto, T. (1993). Symbiotic protistan faunae of Reticulitermes (Isoptera: Rhinotermitidae) in the Japan archipelago. Sociobiology 23, 135–153.
Kitade, O. (2004). Comparison of symbiotic flagellate faunae between termites and a wood-feeding cockroach of the genus Cryptocercus. Microbes Environ. 19, 215–220. doi: 10.1264/jsme2.19.215
Kitade, O., Hayashi, Y., Takatsuto, K., and Matsumoto, T. (2012). Variation and diversity of symbiotic protist composition in the damp-wood termite Hodotermopsis sjoestedti. Jpn. J. Protozool. 45, 29–36. doi: 10.18980/jjprotozool.45.1-2_29
Kitade, O., and Matsumoto, T. (1998). Characteristics of the symbiotic flagellate composition within the termite family Rhinotermitidae (Isoptera). Symbiosis 25, 271–278.
Koidzumi, M. (1921). Studies on the intestinal protozoa found in the termites of Japan. Parasitology 13, 235–309. doi: 10.1017/S0031182000012506
Krishna, K., Grimaldi, D. A., Krishna, V., and Engel, M. S. (2013). Treatise on the Isoptera of the world: Introduction. Bull. Am. Mus. Nat. Hist. 377, 1–200. doi: 10.1206/377.1
Lo, N., and Eggleton, P. (2011). “Termite phylogenetics and co-cladogenesis with symbionts,” in Biology of Termites: A Modern Synthesis. eds. D. E. Bignell, Y. Roisin, and N. Lo (Dordrecht: Springer), 27–50. doi: 10.1007/978-90-481-3977-4_2
Ludwig, W., Strunk, O., Westram, R., Richter, L., Meier, H., Yadhukumar,, et al. (2004). ARB: a software environment for sequence data. Nucleic Acids Res. 32, 1363–1371. doi: 10.1093/nar/gkh293
Mee, E. D., Gaylor, M. G., Jasso-Selles, D. E., Mizumoto, N., and Gile, G. H. (2019). Molecular phylogenetic position of Hoplonympha natator (Trichonymphea, Parabasalia): horizontal symbiont transfer or differential loss? J. Eukaryot. Microbiol. 67, 268–272. doi: 10.1111/jeu.12765
Nalepa, C. A. (1991). Ancestral transfer of symbionts between cockroaches and termites: an unlikely scenario. Proc. R. Soc. B Biol. Sci. 246, 185–189. doi: 10.1098/rspb.1991.0143
Nalepa, C. A. (2015). Origin of termite eusociality: trophallaxis integrates the social, nutritional, and microbial environments. Ecolog. Entomol. 40, 323–335. doi: 10.1111/een.12197
Nguyen, L.-T., Schmidt, H. A., von Haeseler, A., and Minh, B. Q. (2015). IQ-TREE: a fast and effective stochastic algorithm for estimating maximum likelihood phylogenies. Mol. Biol. Evol. 32, 268–274. doi: 10.1093/molbev/msu300
Noda, S., Kitade, O., Inoue, T., Kawai, M., Kanuka, M., Hiroshima, K., et al. (2007). Cospeciation in the triplex symbiosis of termite gut protists (Pseudotrichonympha spp.), their hosts, and their bacterial endosymbionts. Mol. Ecol. 16, 1257–1266. doi: 10.1111/j.1365-294X.2006.03219.x
Noda, S., Shimizu, D., Yuki, M., Kitade, O., and Ohkuma, M. (2018). Host-symbiont cospeciation of termite-gut cellulolytic protists of the genera Teranympha and Eucomonympha and their Treponema endosymbionts. Microbes Environ. 33, 26–33. doi: 10.1264/jsme2.ME17096
Ohkuma, M., and Brune, A. (2011). “Diversity, structure, and evolution of the termite gut microbial community,” in Biology of Termites: A Modern Synthesis. eds. D. E. Bignell, Y. Roisin, and N. Lo (Dordrecht: Springer), 413–438. doi: 10.1007/978-90-481-3977-4_15
Ohkuma, M., Iida, T., Ohtoko, K., Yuzawa, H., Noda, S., Viscogliosi, E., et al. (2005). Molecular phylogeny of parabasalids inferred from small subunit rRNA sequences, with emphasis on the Hypermastigea. Mol. Phyl. Evol. 35, 646–655. doi: 10.1016/j.ympev.2005.02.013
Ohkuma, M., Noda, S., Hongoh, Y., Nalepa, C. A., and Inoue, T. (2009). Inheritance and diversification of symbiotic trichonymphid flagellates from a common ancestor of termites and the cockroach Cryptocercus. Proc. R. Soc. B 276, 239–245. doi: 10.1098/rspb.2008.1094
Ohkuma, M., Ohtoko, K., Grunau, C., Moriya, S., and Kudo, T. (1998). Phylogenetic identification of the symbiotic hypermastigote Trichonympha agilis in the hindgut of the termite Reticulitermes speratus based on small-subunit rRNA sequence. J. Euk. Microbiol. 45, 439–444. doi: 10.1111/j.1550-7408.1998.tb05096.x
Parmentier, D., and Roisin, Y. (2003). Caste morphology and development in Termitogeton nr. planus (Insecta, Isoptera, Rhinotermitidae). J. Morphol. 255, 69–79. doi: 10.1002/jmor.10047
Pruesse, E., Peplies, J., and Glöckner, F. O. (2012). SINA: accurate high-throughput multiple sequence alignment of ribosomal RNA genes. Bioinformatics 28, 1823–1829. doi: 10.1093/bioinformatics/bts252
Quast, C., Pruesse, E., Yilmaz, P., Gerken, J., Schweer, T., Yarza, P., et al. (2013). The SILVA ribosomal RNA gene database project: improved data processing and web-based tools. Nucleic Acids Res. 41, D590–D596. doi: 10.1093/nar/gks1219
Radek, R., Meuser, K., Altinay, S., Lo, N., and Brune, A. (2019). Novel lineages of oxymonad flagellates from the termite Porotermes adamsoni (Stolotermitidae): the genera Oxynympha and Termitimonas. Protist 170:125683. doi: 10.1016/j.protis.2019.125683
Radek, R., Meuser, K., Strassert, J. F. H., Arslan, O., Teßmer, A., Šobotník, J., et al. (2018). Exclusive gut flagellates of Serritermitidae suggest a major transfaunation event in lower termites: description of Heliconympha glossotermitis gen. nov. spec. nov. J. Eukaryot. Microbiol. 65, 77–92. doi: 10.1111/jeu.12441
Ronquist, F., Teslenko, M., van der Mark, P., Ayres, L., Darling, A., Höhna, S., et al. (2012). MrBayes 3.2: Efficient Bayesian phylogenetic inference and model choice across a large model space. System. Biol. 61, 539–542. doi: 10.1093/sysbio/sys029
Saldarriaga, J. F., Gile, G. H., James, E. R., Horak, A., Scheffrahn, R. H., and Keeling, P. (2011). Morphology and molecular phylogeny of Pseudotrichonympha hertwigi and Pseudotrichonympha paulistana (Trichonymphea, Parabasalia) from neotropical rhinotermitids. J. Eukaryot. Microbiol. 58, 487–496. doi: 10.1111/j.1550-7408.2011.00575.x
Wang, M., Hellemans, S., Šobotník, J., Arora, J., Buček, A., Sillam-Dussès, D., et al. (2022). Phylogeny, biogeography and classification of Teletisoptera (Blattaria: Isoptera). Syst. Entomol. 47, 581–590. doi: 10.1111/syen.12548
Wu, L. W., Bourguignon, T., Šobotník, J., Wen, P., Liang, W. R., and Li, H. F. (2018). Phylogenetic position of the enigmatic termite family Stylotermitidae (Insecta: Blattodea). Invertebr. Syst. 32, 1111–1117. doi: 10.1071/IS17093
Yamin, M. A. (1979). Flagellates of the orders Trichomonadida Kirby, Oxymonadida Grassé, and Hypermastigida Grassi and Foà reported from lower termites (Isoptera families Mastotermitidae, Kalotermitidae, Hodotermitidae, Termopsidae, Rhinotermitidae, and Serritermitidae) and from the wood-feeding roach Cryptocercus (Dictyoptera: Cryptocercidae). Sociobiology 4, 1–119.
Keywords: coevolution, diversity, Neoisoptera, Parabasalia, phylogeny, Pseudotrichonympha, transfaunation, ultrastructure
Citation: Radek R, Platt K, Öztas D, Šobotník J, Sillam-Dussès D, Hanus R and Brune A (2023) New insights into the coevolutionary history of termites and their gut flagellates: Description of Retractinympha glossotermitis gen. nov. sp. nov. (Retractinymphidae fam. nov.). Front. Ecol. Evol. 11:1111484. doi: 10.3389/fevo.2023.1111484
Edited by:
Daniel Aguilera-Olivares, Universidad de Concepción, ChileReviewed by:
Gillian H. Gile, Arizona State University, United StatesPeter Vdacny, Comenius University, Slovakia
Copyright © 2023 Radek, Platt, Öztas, Šobotník, Sillam-Dussès, Hanus and Brune. This is an open-access article distributed under the terms of the Creative Commons Attribution License (CC BY). The use, distribution or reproduction in other forums is permitted, provided the original author(s) and the copyright owner(s) are credited and that the original publication in this journal is cited, in accordance with accepted academic practice. No use, distribution or reproduction is permitted which does not comply with these terms.
*Correspondence: Renate Radek, ✉ renate.radek@fu-berlin.de; Andreas Brune, ✉ brune@mpi-marburg.mpg.de
†ORCID: Renate Radek, https://orcid.org/0000-0001-7605-7546
Katja Platt, https://orcid.org/0000-0001-5093-6155
Jan Šobotník, https://orcid.org/0000-0002-8581-637X
David Sillam-Dussès, https://orcid.org/0000-0001-5027-8703
Robert Hanus, https://orcid.org/0000-0002-7054-1975
Andreas Brune, https://orcid.org/0000-0002-2667-4391