- 1InBIO Laboratório Associado, CIBIO, Centro de Investigação em Biodiversidade e Recursos Genéticos, Universidade do Porto, Vairão, Portugal
- 2BIOPOLIS Program in Genomics, Biodiversity and Land Planning, CIBIO, Vairão, Portugal
- 3ARC Centre of Excellence for Coral Reef Studies, James Cook University, Townsville, QLD, Australia
- 4College of Science and Engineering, James Cook University, Townsville, QLD, Australia
- 5MARE—Marine and Environmental Sciences Centre/ARNET—Aquatic Research Network, Institute for Research and Advanced Training (IIFA), Universidade de Évora, Évora, Portugal
In marine interspecific cleaning mutualisms, small fish known as “cleaners” inspect the surface, gills and sometimes the mouth of “client” reef fish, eating ectoparasites, mucus, scales and dead or infected tissue. These cleaner fish species share similar vivid coloration that makes them recognizable by clients. To gain insight on additional communication roles of cleaner fish color, we tested if differences in color among cleaner wrasse Labroides dimidiatus individuals captured from the wild are explained by sex, body size or parasite loads. We found that males were larger, heavier and tended to have more saturated blue tails than females. We also found blue color saturation to be positively correlated with size, and that this size-dependence explains the trend for the sexes to differ in color. Parasite loads did not predict individual differences in color, but fish in our sample were not heavily parasitized. Other color traits (including yellow color saturation and black color brightness) did not differ between the sexes and were associated with morphological differences. Size-dependence of blue color may thus suggest that it is a condition-dependent signal that could be used in various types of inter- and intraspecific social interactions.
1. Introduction
The Indo-Pacific cleaner wrasse, Labroides dimidiatus, is one of the most studied cleaner fish species and has been a model system for work on the evolution of among-species cooperation (Soares, 2017). This cleaner has a lateral black stripe on a conspicuously vivid blue background, together with some yellow in the front, resembling the coloration of several other cleaner species in the same family (Arnal et al., 2006). These color patterns are used by client fishes to identify cleaners (Stummer et al., 2004; Cheney et al., 2009), and their blue color within the pattern attracts more client fish to cleaning stations (Cheney et al., 2009). Moreover, at least in juvenile L. dimidiatus, their color pattern appears to deter predation (Cheney, 2013). Therefore, the vivid colors of cleaner fish are an interspecific communication signal of cleaning for prospective clients (Vaughan et al., 2017).
In non-cleaner species, vivid colors often signal among-individual differences in some aspects of quality. For example, colorful signals are often sexually dichromatic and used in mate choice and may indicate aspects of individual health or condition such as parasite infection level (Johnstone, 1996; Maan et al., 2008; Megía-Palma et al., 2016). In aposematic species, differences in color can also indicate differences in toxicity among individuals (reviewed in Summers et al., 2015). Similarly to sexual and aposematic color signals, among-individual differences in the vivid colors of cleaner fish might also provide cues to aspects of individual quality that are useful for either conspecifics or to interspecific clients. For example, clients use this blue color to distinguish cleaner fish from non-cleaning species (Cheney et al., 2009), and recent laboratory experiments suggested that blue color saturation in L. dimidiatus is condition-dependent, thus providing cues on the quality of individuals or the quality of the cleaning service they provide (Trigo et al., 2020). These cues could inform clients decisions when evaluating individual cleaners, which in nature would allow choosing a better cleaning service. But natural variation in color within a wild population has never been studied for any cleaner fish species. Therefore, there is a lack of insight from the wild on which individual traits color differences may signal.
In order to gain further understanding on the communication role of cleaner fish color variation, specifically their blue color saturation, here we asked if among-individual differences in coloration are explained by sex, body size or degree of parasitism in L. dimidiatus. This cleaner species is protogynous hermaphrodite, first breeding as females and some eventually changing sex to become male harem owners (Robertson, 1972, 1974). As in other members of the genus Labroides, males are on average larger than females (Robertson, 1972) but, although detailed studies of sex differences in color are lacking, the species is described as sexually monochromatic, that is, females can display the same color patterns as males (Pandian, 2010). Since sex and size are correlated in this protogynous hermaphrodite species, we measured color in males and females, tested for sex differences in color, and whether those differences could be a consequence of, or independent from, body size. Additionally, these cleaner fish also naturally carry ecto and endoparasites (Narvaez et al., 2021), which they obtain from their environment or are transmitted from their clients (e.g., Jones et al., 2004; Narvaez et al., 2022). Because parasite load can be a major challenge to the condition of cleaner fish we also asked if this is reflected in their color signal variation.
2. Methods
2.1. Cleaner collection and measurements
All work carried was in accordance with the James Cook University Animal Ethics guidelines (JCU Animal Ethics approvals A2558). We collected cleaner fish Labroides dimidiatus (n = 30) on the Great Barrier Reef (GBR) at Lizard Island (14° 40′S, 154° 24′E) during November 2018 by SCUBA divers with barrier- and hand-nets at different sites around the island (for a detailed description of locations see Narvaez et al., 2021). Larval settlement of L. dimidiatus at these reefs mostly occurs in November and December (larvae settle about 3 weeks after hatching, Brothers et al., 1983). This indicates that cleaner spawning occurs between October and December (Waldie et al., 2011; Grutter, 2012), when we conducted our work, making it easier to distinguish the sexes. Fish were transferred to individual hermetic plastic bag filled with water to keep them alive and minimize the loss of ectoparasites. At the Research Station (Lizard Island Research Station), we euthanized each fish using an ice slurry. We then measured size (total length, in centimeters), mass (weighted wet, in grams), and photographed (see below) each fish, within the following 3 min, to minimize color loss due to death. We calculated size-corrected mass using residuals from a regression of mass on size for all the individuals, as this is a robust method to obtain indices of body condition (Schulte-Hostedde et al., 2005). Male and female data fitted well the same regression line (Supplementary Figure S1), and therefore we computed the index of body condition together for both sexes. The final sample size was 21 females and 8 males (one individual could not be sexed and was excluded from analyses). Cleaner sex was determined by visually analyzing the gonads. Because our field collection occurred within the spawning season, fish were easily sex based on gonad morphology as female gonads were larger and often contained eggs.
2.2. Determination of cleaner parasite load
To determine the parasite community of the cleaner fish, fish were examined fresh for ecto- and endo-parasites (see Narvaez et al., 2021 for detailed methods). For ecto-parasites, the external surface, fins, gills and oral cavity were visually inspected under a low-power dissection microscope, and a skin scrape was done. Gills were removed and placed on a microscope slide for further analysis of smaller parasites (e.g., protozoan parasites). Fish were then placed into a Petri dish with physiological saline during 10 min to remove any potential external parasites. After all the external evaluation procedures, the fish were dissected for examination of endoparasites. All organs were superficially inspected under a low-power dissection microscope. Smears and squashes of body muscle and internal organs (gall bladder/bile, brain, kidney, spleen, and liver tissue) were produced and inspected for the presence of protozoan and myxozoan infection. Ectoparasite load included isopod crustaceans in the family Gnathiidae and parasitic species in the subclass Copepoda, and endoparasite load included trematode worms in the digestive tract.
We calculated the prevalence (number of fish infected/number of fish examined) and the abundance of parasites (mean ± standard error), for all the individuals.
2.3. Cleaner skin coloration
We measured fish skin colors based on digital photographs taken in same light conditions (two artificial white led lights placed 25 cm above the fish and on each side of the fish). Photographs were taken with a Nikon COOLPIX W300 camera, with a fish placed laterally in a petri dish above a squared sheet of paper (Supplementary Figure S2). We used the software GIMP (Gimp 2.8.20) to quantified the mean RGB (red, green, blue) values in four circles with diameter of 2.5 mm, located at: (a) the dark blue patch at the base of the tail; (b) the blue/yellow patch midway in the fish body (i.e., above the end of the pelvic fin and at the center of the blue/yellow stripe); (c) the yellow patch, measured at the center of the stripe above the beginning of the pelvic fin; and (d) the black stripe, measured at the center of the stripe above the beginning of the anal fin (Supplementary Figure S2). We then converted RGB values into HSB (hue, saturation, and brightness) with the R function rgb2hsv which converts the red, green, and blue values of an RGB image to hue, saturation, and value (HSV) values of an HSV image. Each cleaner was photographed twice, and we averaged the HSB color measurements so as to obtain a single color hue, saturation and brightness per fish per body part. Measurements from with the two photographs on the same individual had high repeatability on tail blue saturation (R = 0.81) and mid-body hue (R = 1.0), and medium repeatability for yellow saturation (R = 0.48) and black brightness (R = 0.33). Therefore, we perform analyses for all the color variables, but results for yellow saturation and black brightness should be interpreted with caution.
These measurements were used to quantify different aspects of the fish coloration. For the blue and yellow patches (measurements in patches a and c, respectively), we used the measurement of color saturation, since this relates to the conspicuousness of color, and color saturation is related to individual quality in several animal species (Weiss, 2006; Simons et al., 2012; Trigo and Mota, 2015). For the black stripe (measurements in patch d) we measured brightness as a means to quantify the purity of black color (as indicated by low brightness). And we used the hue measured midway the blue/yellow stripe (patch b) as an index for the extent of blue: the lower (i.e., the bluer) the hue in the middle of this stripe, the larger is the extent of blue coloration. We thus obtained four color variables: (a) tail blue saturation; (b) mid-body hue, to evaluate individual differences in the extent of blue coloration; (c) yellow saturation, and (d) black brightness.
We standardized all measurements by reference to the background yellow and brown colors in the same squared paper below each fish. For this, we selected a square of the squared paper immediately below the beginning of the anal fin, and measured the RGB values of paper yellow (“py”) using a 2.5 mm circle as before, and of paper brown (“pb”) using a circle small enough to be inside the brown line. We used these measurements to standardize each color measurement following Hill et al. (2009). We first calculated, separately for each fish measurement (“sample”) (sample − py)/(pb − py), which rescales the sample measurement (either H, S or B) to a scale from 0 to 1 where 0 and 1 correspond to the “pb” and “py” values in that same photograph. We then multiplied these rescaled measurements (either H, S, or B) by the mean difference between “py” and “pb” values across the photographs of all fish, and added the mean value of “py” across the photographs of all fish, in order to approximate the original scale of either H, S or B values.
2.4. Statistics
Mass (in grams), size (in cm), size-corrected mass, blue and yellow saturation and mid-body hue followed approximately normal distributions. Black brightness was log-transformed (log10 x + 1) in order to approximate a normal distribution. Ecto and endo-parasite loads were strongly non-normal and, therefore, we analyzed them using non-parametric tests. We verified that there was homogeneity of variance between sexes, for all color variables.
To test for sex differences in body measurements and color variables we ran independent sample t-tests. For sex differences in parasite loads we instead ran non-parametric Mann–Whitney tests. To test for associations between color and morphological traits (size and size-corrected mass) or parasite loads we first used simple Pearson correlations, or non-parametric Spearman’s correlations in the case of parasite loads. Then, to test for these associations with color while controlling for covariates, we ran general linear models (GLM) with a color trait as dependent variable, sex as fixed factor, and size, size-corrected mass and parasite loads as covariates. In these GLM’s we chose to included size and not weight because these two variables are highly correlated (Pearson correlation = 0.96, p < 0.001). We checked that residuals in these GLMs approached normality. Analyses were performed in IBM SPSS Statistics.
3. Results
3.1. Sex differences
Ectoparasite prevalence was 33% for females (i.e., seven females) and 50% for males (i.e., four males). For endoparasites, prevalence in females and males were 29 and 38%, respectively. The complete description of the parasites in these individuals (irrespective of sex) can be found in Narvaez et al. (2021) (Supplementary Table S1).
Males were on average heavier and longer than females (Independent sample t-test, weight: t = 3.06, p = 0.005; size: t = 3.63, p = 0.001; Figures 1A,B), and size-corrected mass did not differ between the sexes (Independent sample t-test, t = −0.81, p = 0.43; Figure 1C). Males show a non-significant tendency to have higher tail blue saturation values than females (Independent sample t-test, t = 1.97, p = 0.06; Figure 1D). Males and females did not differ significantly in ectoparasite (Mann–Whitney test, U = 76.0, p = 0.65; Figure 1E) or endoparasite loads (Mann–Whitney test, U = 77.0, p = 0.68; Figure 1F), nor in the remaining color variables (Independent sample t-test, yellow skin saturation: t = 1.20, p = 0.24, mid-body hue: t = 0.84, p = 0.41, black brightness: t = 0.78, p = 0.44).
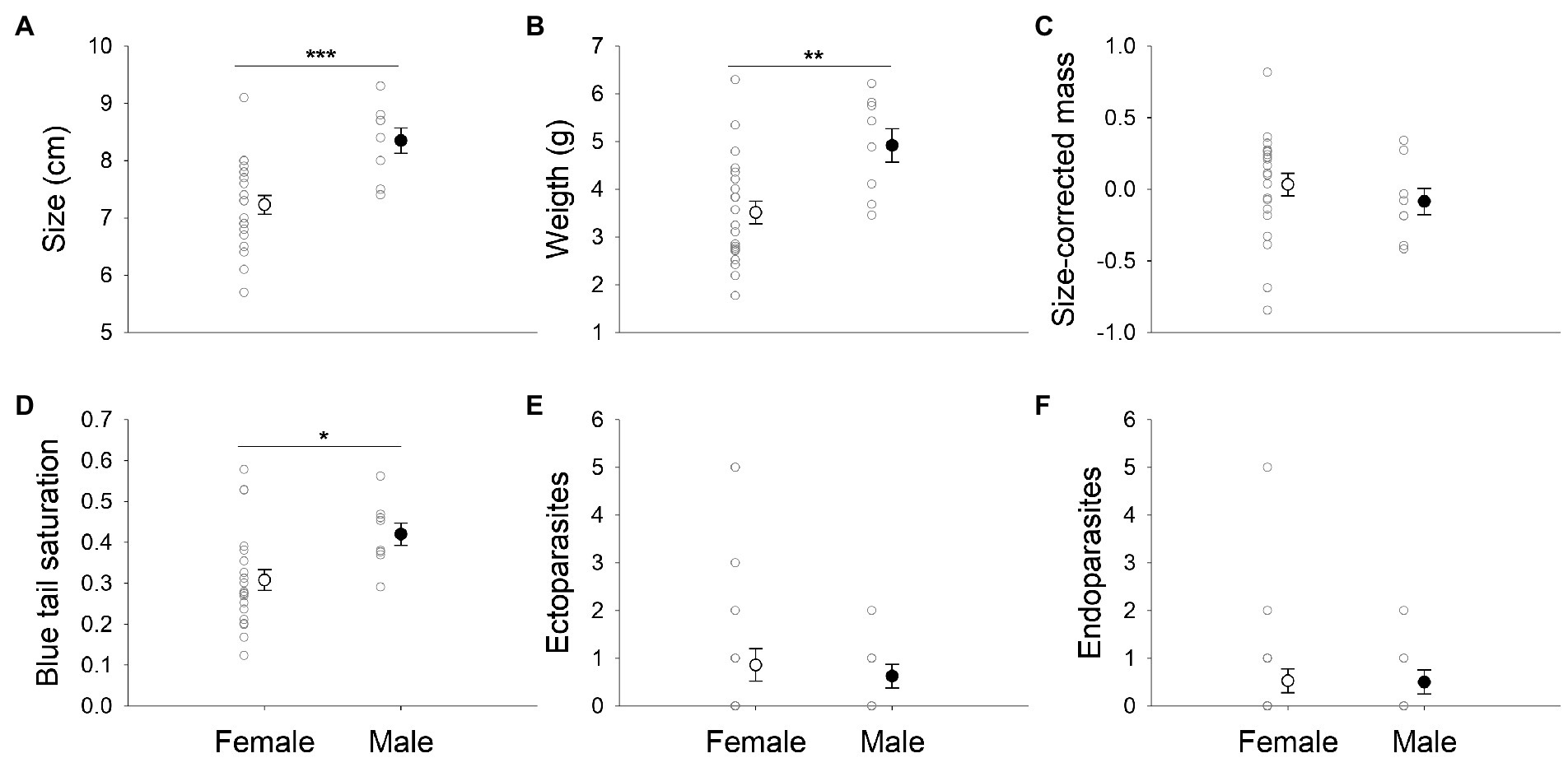
Figure 1. Differences between sexes in morphometric and coloration variables: (A) size (in centimeters); (B) weight (in grams); (C) size-corrected mass; (D) blue tail saturation; (E) ectoparasites; and (F) endoparasites. Represented values are means and standard errors; males are in filled bars and females in open bars, n = 29. *p< 0.05; ** p< 0.005; *** p< 0.001.
3.2. Color, body measures, and parasites
Tail blue saturation was positively correlated with size (Pearson correlation, rp = 0.50, p = 0.006, N = 29; Figure 2A), but not with size-corrected mass (Pearson correlation, rp = −0.14, p = 0.48, N = 29; Figure 2B), ectoparasite (Spearman’s correlation, rs = 0.22, p = 0.24, N = 29; Figure 2C) or endoparasite loads (Spearman’s correlation, rs = −0.07, p = 0.71, N = 29; Figure 2D). All remaining color variables did not have significantly correlations with any of the body or parasite variables (Pearson correlation, all |r| < 0.32, all p > 0.09, N = 29; Supplementary Table S2).
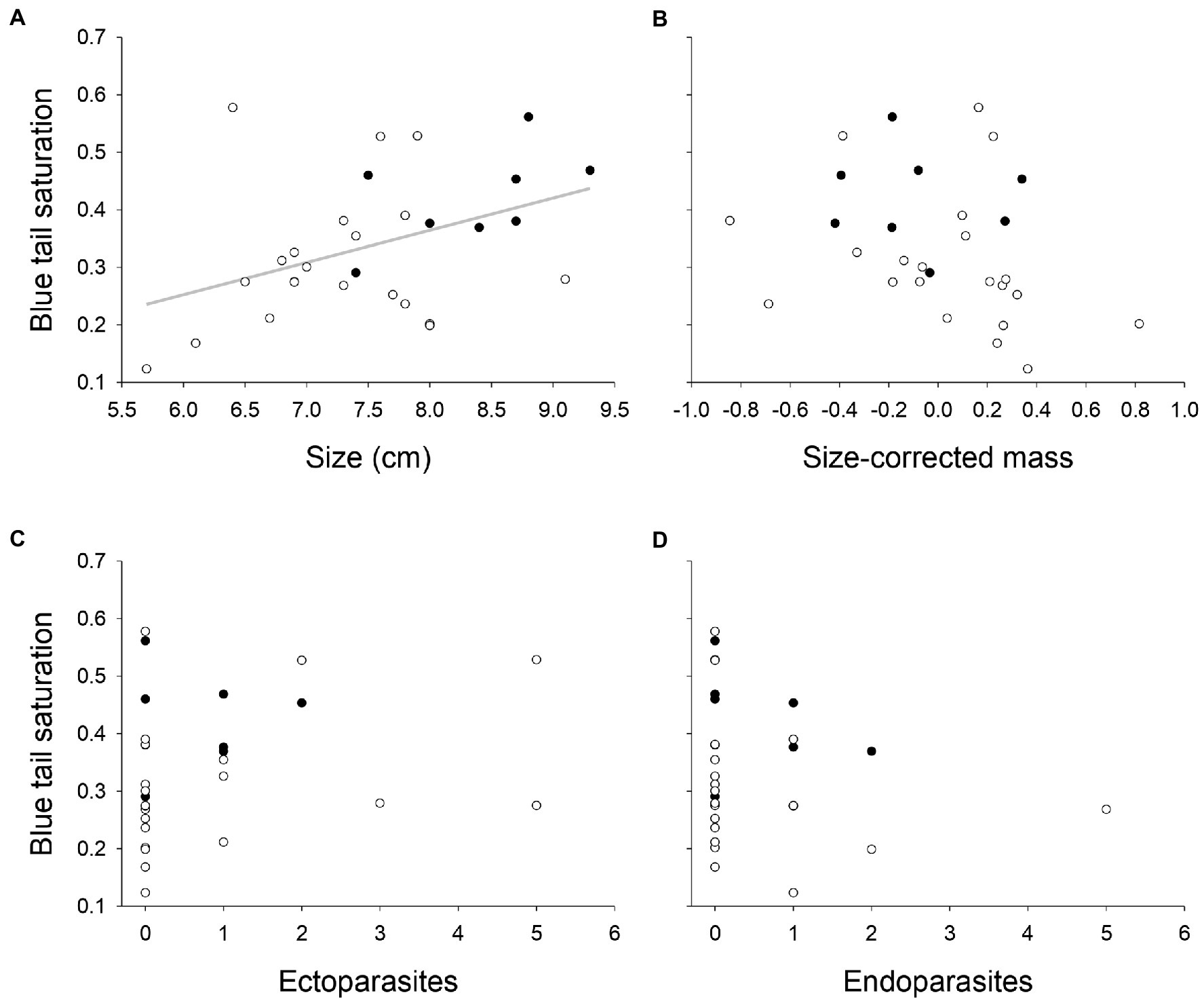
Figure 2. Tail blue saturation in relation to: (A) size (in centimeters); (B) size-corrected mass; (C) ectoparasites; and (D) endoparasites, in males (closed circles) and females (open circles), with regression line for males (solid line) and females (dashed line), n = 29.
When controlling for covariates, however, the association between size and tail blue saturation was slightly weaker (βst = 0.39) and no longer statistically significant with our sample size (General linear model, F1,29 = 2.95, p = 0.10), and a sex difference in blue color saturation was no longer suggested (General linear model, βst = −0.15, F1,29 = 0.34, p = 0.57; Table 1). Other body color variables were not predicted by any of the predictors (General linear model, all |βst| < 0.27, all p > 0.36; Supplementary Table S3).
Because differences in size were associated both with sex and blue color saturation, we repeated the General linear model for blue saturation, now also including the interaction terms between sex and the other predictors. These interaction terms were not significant (interaction sex * size: F1,29 = 1.84, p = 0.19; other results in Supplementary Table S4), indicating that the relation between size and color did not differ between males and females (Figure 2A).
4. Discussion
We found that male cleaner wrasses (L. dimidiatus) are heavier, longer and had a non-significant tendency to show more saturated blue color than females. Endo- and ectoparasite loads did not predict blue color saturation. The saturation of blue color was positively correlated with size but, when using a model with multiple predictors (sex, size, size-corrected mass, parasite loads) to control for the effects of covariates, the trend for sex differences in color was no longer detected, and the association of color with size was also slightly weakened. Therefore, sex differences in color may appear in the cleaner wrasse as a consequence of sex differences in size and an association between size and blue color saturation. The remaining color traits studied here did not differ between the sexes nor were associated with morphology or parasite loads. These latter results should be interpreted with caution, because repeatability was not strong for some of those other color measurements (namely for yellow saturation and brightness of black).
Finding an association between blue color saturation and body size or mass suggests that the conspicuous blue color of L. dimidiatus could signal for physical condition. This agrees with previous experimental results, showing that an oxidative stress treatment was able to reduce blue color saturation and the quality of the cleaning service in these species (Trigo et al., 2020). Such a condition-dependent signal could be used both in intra- and inter-specific contexts. In an inter-specific context, this condition-dependent signal could be used by potential clients when choosing cleaners. In fact, clients are known to exert choice between alternative individual cleaners and cleaning stations (Bshary and Schäffer, 2002). Furthermore, cleaners also advertise their cleaning stations to clients with “dances” (Potts, 1973) in which they swim in an oscillating manner (Youngbluth, 1967; Losey, 1971), with their conspicuous black stripe and strikingly prominent blue tail during these dances. Stummer et al. (2004) used models of L. dimidiatus to show that, in models of normal body size, a larger black stripe increased the number of clients approaching, but not their interest at close distance. They concluded that the black stripe attracts clients at distance, but at close range, the client interest may be based on other cues such as behavioral (e.g., cheating behavior) or sensorial (e.g., chemical cues). Another example of choice exerted by clients, occurs when these eavesdrop on cleaners’ interaction with other clients in order to choose whether to wait and visit that cleaner or search for another (Pinto et al., 2011). Clients benefit from choosing among individual cleaners, because cleaning service can differ significantly: cleaners may attend to clients rapidly or make them wait, they may or may not provide tactile stimulation, and they can also cheat by feeding on healthy body tissue instead of ectoparasites (Bshary, 2002; Bshary and Côté, 2008). Whether or not clients evaluate among-individual differences in blue color saturation to help with their choice remains to be studied. If the color of the blue tail indicates aspects of quality, then clients may use this information when making their choices.
In an intra-specific context, condition-dependent color may perhaps help to assess dominant status among cleaners, which is important for their social behavior. For instance, dominant cleaner males are generally older individuals that live and clean in pairs, choosing for this the largest female of their harem, but patrolling all other females regularly (Robertson, 1972). When the male disappears, usually the largest (and more dominant) female of that harem, or from a nearby territory, changes sex and takes over the harem (Sakai et al., 2001). We can assume that cleaner wrasses compete to increase social status and hence to gain access to better territories. In the case of males also to gain access to female harems, and in the case females also to clean with the dominant male as a pair (since pairs usually inspect larger clients; Bshary et al., 2008) and eventually to gain priority in transitioning sex to a harem-holding male. Since dominance increases with size (Sakai et al., 2001), and having found that blue color saturation covaries with size, we hypothesize that cleaner wrasses may use the blue color of conspecifics to help evaluate social dominance during these various types of social interactions. This too, however, remains to be studied.
The absence of sex or size differences regarding other colors (yellow or black) is not surprising considering the importance of blue guild coloration to distinguish cleaner fish species from non-cleaners (Cheney et al., 2009). While cleaners are more likely to have a combination of blue and yellow colors and there is evolutionary correlation between displaying black stripes and cleaning behavior (Arnal et al., 2006), blue color is still the source of highest chromatic contrast against most microhabitat backgrounds, as evaluated by the visual systems of most client species (Lettieri et al., 2009). Finally, we expected higher parasitism levels affected coloration, since some studies in other fish species have reported negative associations between parasite infection and aspects of individual quality (e.g., size of sand bowers in Copadichromis spp., Taylor et al., 1998, or territory size and red coloration in a diverse lineage of Lake Victoria cichlids, Pundamilia nyererei, Maan et al., 2008). However, we did not find associations between parasite infestation and cleaners, which could be due to parasite loads in the wild being low to affect coloration, while cleaners not being exempted from parasite infection (Narvaez et al., 2021, 2022), or to these parasites not being detrimental.
In this study we found that, unlike commonly assumed, the cleaner wrasse L. dimidiatus may not be entirely sexually monochromatic (Pandian, 2010) but show size-dependency in both their sex and their blue color. Since the cleaner wrasse is able to change sex bidirectionally, depending on sex-ratio and social-environmental factors, perhaps the association between blue color saturation and size makes the blue color a useful signal of sex, or even a sexually-selected trait. Simultaneously, since blue color saturation indicates size, it can be a more general signal of individual quality or condition, useful also for interspecific (cleaning) interactions with clients. Such a condition-dependent signal of quality might be used in various types of inter- and intraspecific interactions, including choice of cleaning stations, competition for resources or individual dominance within harems, thus suggesting several hypotheses for future research.
Data availability statement
The original contributions presented in the study are included in the article/Supplementary material, further inquiries can be directed to the corresponding author.
Author contributions
PN performed the material preparation and data collection. ST performed the analysis and wrote the first draft of the manuscript. All authors commented on previous versions of the manuscript, read and approved the final manuscript, and contributed to the study conception and design.
Funding
PN has been funded by the James Cook University Postgraduate Research Scholarship (JCUPRS). ST, GC, and MCS were supported by Portuguese National Funds through the Fundação para a Ciência e Tecnologia (grant numbers PTDC/BIA-ECO/32210/2017, PTDC/BIA-COM/2644/2020, DL57/2016/CP1440/CT0011, DL57/2013/CP1440/CT0019), and FEDER funds under COMPETE 2020 (CEEC Individual–2021.01458.CEECIND/CP1668/CT0003). Work co-funded by the project NORTE-01-0246-FEDER-000063, supported by Norte Portugal Regional Operational Programme (NORTE2020), under the PORTUGAL 2020 Partnership Agreement, through the European Regional Development Fund (ERDF).
Acknowledgments
We thank Alexia Dubuc and Veronica Radice for their great assistances on the field, and Lizard Island Research Station Staff. We thank the reviewers for their suggestions, which improved the content of this article.
Conflict of interest
The authors declare that the research was conducted in the absence of any commercial or financial relationships that could be construed as a potential conflict of interest.
Publisher’s note
All claims expressed in this article are solely those of the authors and do not necessarily represent those of their affiliated organizations, or those of the publisher, the editors and the reviewers. Any product that may be evaluated in this article, or claim that may be made by its manufacturer, is not guaranteed or endorsed by the publisher.
Supplementary material
The Supplementary material for this article can be found online at: https://www.frontiersin.org/articles/10.3389/fevo.2023.1110079/full#supplementary-material
References
Arnal, C., Verneau, O., and Desdevises, Y. (2006). Phylogenetic relationships and evolution of cleaning behaviour in the family Labridae: Importance of body colour pattern. J. Evol. Biol. 19, 755–763. doi: 10.1111/j.1420-9101.2005.01059.x
Brothers, E. B., Williams, D. M., and Sale, P. F. (1983). Length of larval life in twelve families of fishes at “One Tree Lagoon”, Great Barrier Reef, Australia. Mar. Biol. 76, 319–324. doi: 10.1007/BF00393035
Bshary, R. (2002). Biting cleaner fish use altruism to deceive image-scoring client reef fish. Proc. R. Soc. B Biol. Sci. 269, 2087–2093. doi: 10.1098/rspb.2002.2084
Bshary, R., and Côté, I. M. (2008). “New perspectives on marine cleaning mutualism” in Fish behaviour. eds. C. Magnhagen, V. A. Braithwaite, E. Forsgrenm, and B. G. Kapoor (New Hampshire, NH: Science Publishers), 563–592.
Bshary, R., Grutter, A. S., Willener, A. S., and Leimar, O. (2008). Cooperative pairs of cleaner fish provide their clients with better service quality than singletons. Nature 445, 964–966.
Bshary, R., and Schäffer, D. (2002). Choosy reef fish select cleaner fish that provide high-quality service. Anim. Behav. 63, 557–564. doi: 10.1006/anbe.2001.1923
Cheney, K. L. (2013). Cleaner fish coloration decreases predation risk in aggressive fangblenny mimics. Behav. Ecol. 24, 1161–1165. doi: 10.1093/beheco/art043
Cheney, K. L., Grutter, A. S., Blomberg, S. P., and Marshall, N. J. (2009). Blue and yellow signal cleaning behavior in coral reef fishes. Curr. Biol. 19, 1283–1287. doi: 10.1016/j.cub.2009.06.028
Grutter, A. S. (2012). Enhanced colonization success and competition associated with conspecifics in cleaner fish Labroides dimidiatus juveniles. Coral Reefs 31, 1169–1176. doi: 10.1007/s00338-012-0942-8
Hill, G. E., Hood, W. R., and Huggins, K. (2009). A multifactorial test of the effects of carotenoid access, food intake and parasite load on the production of ornamental feathers and bill coloration in American goldfinches. J. Exp. Biol. 212, 1225–1233. doi: 10.1242/jeb.026963
Johnstone, R. A. (1996). Multiple displays in animal communication: ‘backup signals’ and ‘multiple messages’. Philos. Trans. R. Soc. B 351, 329–338. doi: 10.1098/rstb.1996.0026
Jones, C. M., Grutter, A. S., and Cribb, T. H. (2004). Cleaner fish become hosts: A novel form of parasite transmission. Coral Reefs 23:521. doi: 10.1007/s00338-004-0411-0
Lettieri, L., Cheney, K. L., Mazel, C. H., Boothe, D., Marshall, N. J., and Streelman, J. T. (2009). Cleaner gobies evolve advertising stripes of higher contrast. J. Exp. Biol. 212, 2194–2203. doi: 10.1242/jeb.025478
Losey, G. S. (1971). Communication between fishes in cleaning symbiosis. Baltimore, MD: University Park Press.
Maan, M. E., Rooijen, A. M. C. V., Alphen, J. J. M. V., and Seehausen, O. (2008). Parasite-mediated sexual selection and species divergence in Lake Victoria cichlid fish. Biol. J. Linn. Soc. 94, 53–60. doi: 10.1111/j.1095-8312.2008.00989.x
Megía-Palma, R., Martínez, J., and Merino, S. (2016). A structural colour ornament correlates positively with parasite load and body condition in an insular lizard species. Sci. Nat. 103:52. doi: 10.1007/s00114-016-1378-8
Narvaez, P., Morais, R. A., Vaughan, D. B., Grutter, A. S., and Hutson, K. S. (2022). Cleaner fish are potential super-spreaders. J. Exp. Biol. 225:jeb244469. doi: 10.1242/jeb.244469
Narvaez, P., Yong, R. Q. Y., Grutter, A. S., and Hutson, K. S. (2021). Are cleaner fish clean? Mar. Biol. 168:59. doi: 10.1007/s00227-021-03858-3
Pinto, A., Oates, J., Grutter, A., and Bshary, R. (2011). Cleaner wrasses Labroides dimidiatus are more cooperative in the presence of an audience. Curr. Biol. 21, 1140–1144. doi: 10.1016/j.cub.2011.05.021
Potts, G. W. (1973). The ethology of Labroides dimidiatus (cuv. & val.) (Labridae, Pisces) on Aldabra. Anim. Behav. 21, 250–291. doi: 10.1016/S0003-3472(73)80068-5
Robertson, D. R. (1972). Social control of sex reversal in a coral-reef fish. Science 177, 1007–1009. doi: 10.1126/science.177.4053.1007
Robertson, D. R. (1974). A study of the ethology and reproductive biology of the Labrid fish, Labroides dimidiatus at Heron Island, Great Barrier Reef. Brisbane, QLD: University of Queensland.
Sakai, Y., Kohda, M., and Kuwamura, T. (2001). Effect of changing harem on timing of sex change in female cleaner fish Labroides dimidiatus. Anim. Behav. 62, 251–257. doi: 10.1006/anbe.2001.1761
Schulte-Hostedde, A. I., Zinner, B., Millar, J. S., and Hickling, G. J. (2005). Restitution of mass-size residuals: Validating body condition indices. Ecology 86, 155–163. doi: 10.1890/04-0232
Simons, M. J. P., Cohen, A. A., and Verhulst, S. (2012). What does carotenoid-dependent coloration tell? Plasma carotenoid level signals immunocompetence and oxidative stress state in birds-a meta-analysis. PLoS One 7:e43088. doi: 10.1371/journal.pone.0043088
Soares, M. C. (2017). The neurobiology of mutualistic behavior: The cleanerfish swims into the spotlight. Front. Behav. Neurosci. 11:191. doi: 10.3389/fnbeh.2017.00191
Stummer, L. E., Weller, J. A., Johnson, M. L., and Côté, I. M. (2004). Size and stripes: How fish clients recognize cleaners. Anim. Behav. 68, 145–150. doi: 10.1016/j.anbehav.2003.10.018
Summers, K., Speed, M. P., Blount, J. D., and Stuckert, A. M. M. (2015). Are aposematic signals honest? A review. J. Evol. Biol. 28, 1583–1599. doi: 10.1111/jeb.12676
Taylor, M. I., Turner, G. F., Robinson, R. L., and Stauffer, J. R. J. R. (1998). Sexual selection, parasites and bower height skew in a bower-building cichlid fish. Anim. Behav. 56, 379–384. doi: 10.1006/anbe.1998.0795
Trigo, S., Gomes, A. C. R., Cardoso, S. C., Teixeira, M., Cardoso, G. C., and Soares, M. C. (2020). Cleaner blues: Condition-dependent colour and cleaner fish service quality. Behav. Process. 181:104246. doi: 10.1016/j.beproc.2020.104246
Trigo, S., and Mota, P. G. (2015). What is the value of a yellow patch? Assessing the signalling role of yellow colouration in the European serin. Behav. Ecol. Sociobiol. 69, 481–490. doi: 10.1007/s00265-014-1860-2
Vaughan, D. B., Grutter, A. S., Costello, M. J., and Hutson, K. S. (2017). Cleaner fishes and shrimp diversity and a re-evaluation of cleaning symbioses. Fish Fish. 18, 698–716. doi: 10.1111/faf.12198
Waldie, P. A., Blomberg, S. P., Cheney, K. L., Goldizen, A. W., and Grutter, A. S. (2011). Long-term effects of the cleaner fish Labroides dimidiatus on coral reef fish communities. PLoS One 6:e21201. doi: 10.1371/journal.pone.0021201
Weiss, S. L. (2006). Female-specific color is a signal of quality in the striped plateau lizard (Sceloporus virgatus). Behav. Ecol. 17, 726–732. doi: 10.1093/beheco/arl001
Keywords: cleaner fish, mutualism, coloration, communication, parasites
Citation: Trigo S, Narvaez P, Cardoso GC and Soares MC (2023) Not so monochromatic: Size-dependency of both sex and color in the cleaner wrasse Labroides dimidiatus. Front. Ecol. Evol. 11:1110079. doi: 10.3389/fevo.2023.1110079
Edited by:
Cristiano Bertolucci, University of Ferrara, ItalyCopyright © 2023 Trigo, Narvaez, Cardoso and Soares. This is an open-access article distributed under the terms of the Creative Commons Attribution License (CC BY). The use, distribution or reproduction in other forums is permitted, provided the original author(s) and the copyright owner(s) are credited and that the original publication in this journal is cited, in accordance with accepted academic practice. No use, distribution or reproduction is permitted which does not comply with these terms.
*Correspondence: Sandra Trigo, strigo@cibio.up.pt